- 1Department of Veterinary Medicine, College of Coastal Agricultural Sciences, Guangdong Ocean University, Zhanjiang, China
- 2College of Traditional Chinese Medicine, Zhanjiang University of Science and Technology, Zhanjiang, China
- 3Maoming Branch, Guangdong Laboratory for Lingnan Modern Agriculture, Maoming, China
Introduction: The widespread use of antibiotics in animal agriculture has increased the resistance of Escherichia coli, and pathogenic E. coli often harbor complex virulence factors. Antimicrobial resistance in pathogenic bacteria can cause public health problems. Correlation analyses of the resistance, virulence, and serotype data from the pathogenic bacteria found on farms and in the surrounding environment can thus provide extremely valuable data to help improve public health management.
Methods: In this investigation, we have assessed the drug resistance and virulence genes as well as the molecular typing characteristics of 30 E. coli strains isolated from duck farms in the Zhanjiang area of China. Polymerase chain reaction was used to detect the drug resistance and virulence genes as well as serotypes, and whole-genome sequencing was used to analyze the multilocus sequence typing.
Results: The detection rates for the oqxA resistance gene and fimC virulence gene were highest (93.3%, respectively). There were no correlations between the drug resistance and virulence gene numbers in the same strain. The epidemic serotype was O81 (5/24), ST3856 was an epidemic sequence type, and strains I-9 and III-6 carried 11 virulence genes. The E. coli strains from the duck farms in the Zhanjiang area were thus found to have a broad drug resistance spectrum, various virulence genes, complex serotypes, and certain pathogenicity and genetic relationship.
Discussion: Monitoring the spread of pathogenic bacteria and the provision of guidance regarding the use of antibiotics in the livestock and poultry industries will be required in the future in the Zhanjiang area.
Introduction
Escherichia coli is a widespread bacteria that can become pathogenic under certain conditions, which can create a threat to human and animal health. Consequently, in recent years, this has become an important research topic in the field of epidemiology (Srivani et al., 2017). Since the discovery of antibiotics, their long-term overuse has resulted in the gradual development of drug resistance in some bacteria. After drug-resistant bacteria are excreted in livestock and poultry faeces, the drug-resistant genes carried by the bacteria can be transmitted to other bacteria through mobile elements such as plasmids, transposons, and integrons, resulting in their spread and diffusion (Choi et al., 2021). The pathogenicity of E. coli is related to its virulence genes, including those encoding adhesins, pathogenicity islands, and outer membrane proteins. When invading the body, numerous virulence genes from E. coli interact with each other, and this enables them to escape and destroy the host’s defense mechanism, resulting in host inflammatory response (Ugwu et al., 2020). The O antigen is an important E. coli structure, which is located in the side chain of the cell wall lipopolysaccharide. Currently, the O antigen serotype classification is the standard for epidemiological judgment (Liu et al., 2019). The pathogenicity of E. coli is not completely consistent with its serotype, and the dominant serotype of E. coli is related to regional, temporal, and source differences. E. coli can be divided into groups A, B1, B2, and D according to its phylogenetic group classification, where B2 and D are highly pathogenic, B1 is less pathogenic, and A is non-pathogenic (Coura et al., 2015). Multilocus sequence typing (MLST) was first used in the typing of Neisseria meningitidis and has been widely used in the molecular typing of other bacteria in recent years. MLST first detects the sequence numbers of 7–10 housekeeping genes, then compares the sequence type (ST) of the bacteria, and uses this ST data to calculate the genetic and evolutionary relationships among strains (Ramadan et al., 2020). Due to scientific and technological progress, MLST is now based on whole genome sequencing (WGS), which greatly improves the accuracy of the method and means that it is becoming increasingly popular in research (Galarce et al., 2021).
Drug-resistant genes have become new environmental pollutants, and they can spread both horizontally and vertically, leading to dramatic increases in the number of drug-resistant bacteria. Antibiotics discharged from the body are scattered in soil, water, or sediment; antibiotic-resistant bacteria and genes are increasing continuously in farms and surrounding environment. In recent years, there have been many reports on drug-resistant genes in E. coli of animal origin; however, there are few reports on drug-resistant genes in E. coli of environmental origin. In this study, 30 strains of E. coli were isolated and identified from soil and sediment samples collected from duck farms in the Zhanjiang area of China. The 30 strains were selected based on their drug-resistant genes, virulence genes, serotypes, phylogenetic group, and MLST analysis to understand the prevalence of E. coli and its drug resistance and virulence in this area and to provide a theoretical basis for the prevention and control of colibacillosis.
Materials and methods
Sample sources and strains
Samples were collected from three duck farms (farms I, II, and III) in Zhanjiang, China. The soil samples were collected from the surface 1–3 cm soil samples of the pond bank of the farm, and the sediment samples were collected from the surface 1–5 cm of the pond underwater sediment. Five soil samples and 5 sediment samples were collected at each farm. One E. coli strain was isolated from each soil and sediment sample, and 30 E. coli strains were isolated overall. Standard E. coli (ATCC 25922) was provided by a basic veterinary laboratory of Guangdong Ocean University as an internal control for Clinical and Laboratory Standards Institute (CLSI) procedures. The five strains of soil E. coli isolated from each duck farm were numbered as 1–5, whereas the five strains of sediment E. coli were numbered 6–10. For example, “I-1” represents the first E. coli strain isolated from the soil of farm I.
Isolation, culture, and purification of E. coli
The samples were diluted to 106 CFU/ml using 0.1% peptone water, coated on MacConkey medium, and cultured in an incubator at 37 °C for 18h. The distribution and morphological characteristics of the colonies on the MacConkey medium were observed, a single pink typical colony was selected for line purification on eosin Meilan medium, and a single black metal-luster typical colony was selected for secondary purification on eosin Meilan medium. A suspected E. coli single colony was then selected and inoculated in a 2-ml tube containing nutritional broth [Beijing Land Bridge Technology Co. LTD, CM106, 2021] and cultured in a 37 °C incubator for 12h; finally, 30% glycerol was added and the sample was stored at −20 °C until further analysis (Sarba et al., 2019).
Polymerase chain reaction identification of E. coli
According to the instructions of the bacterial DNA extraction kit [Tiagen Biochemical Technology (Beijing) Co., Ltd, DP302, 2021], the genomic DNA of each strain was extracted as a template, with reference to the relevant literature (Hu et al., 2011). E. coli alkaline phosphatase gene (phoA) is the household gene and present in all E. coli, which can be used for specificity identification. The preserved strains were identified by polymerase chain reaction (PCR) using the phoA gene. Primer information was shown in Table 1. The PCR reaction system was 15 μl [DNA template 1 μl, actual master mix [TaKaRa Biomedical Technology (Beijing) Co., Ltd, RR030Q] 13 μl, and upstream and downstream primers (25 μmol/liter) 0.5 μl each]. PCR reaction conditions were as follows: pre-denaturation at 94 °C for 7 min, denaturation at 94 °C for 30 s, annealing at 55 °C for 30 s, and extension at 72 °C for 30 s, and this cycle was repeated 30 times followed by a final extension at 72 °C for 5 min (Niu et al., 2020). After the reaction, a 5 μl amplification product was used for 1.5% agarose gel electrophoresis, and a 622 bp gene sequence length was identified as E. coli.
Detection of drug-resistant phenotype and genes in E. coli
Kirby-Bauer method was used to test the antimicrobial susceptibility of E. coli isolated from three farms. These experiments were carried out in accordance with guidelines recommended by the Clinical and Laboratory Standards Institute (Clinical and Laboratory Standards Institute (CLSI), 2021). The phenotypic analysis was verified using a standard strain (ATCC 25922). PCR was used to detect related drug-resistant genes. The 16 resistance genes were β-lactam (blaCIT, blaTEM), carbapenems (blaDHA), amidols (floR, fexB), aminoglycosides (optrA, aphA1, aac (Ugwu et al., 2020)-II), sulfonamides (sul2), tetracycline (tetM, tetC), macrocyclic lipids (ermB), quinolones (oqxA, qnrS), and colistin (mcr1, mcr2). The E. coli DNA was extracted using a kit [Tiagen Biochemical Technology (Beijing) Co., Ltd, DP302, 2021], and the annealing temperature, reaction system, and reaction conditions (annealing temperature according to primers) for the 16 pairs of drug-resistan genes were referring to the corresponding literature. Primer information was shown in Table 1.
Identification of phylogenetic group
The phylogenetic group genes of the 30 strains of E. coli were detected using triple PCR, and the phylogenetic groups were determined based on the existence or deletion of chuA, yjaA, and tspE4.C2 genes. Primer information was shown in Table 1.
Study of serotype, virulence gene, and multi-site sequence typing
Virulence factors of E.coli include fimil (papA and papC), adherin (fimC and tsh), iron uptake system (iutA and iucC), pathogenicity island (irp2, irpN, fyuA, and ler), serum resistance protein (ompT and iss), capsule polysaccharide (kpsMII and traT), toxin (astA, vat, and cvaC), hemolysin (hlyB), outer membrane protein (eaeA), invasitin (ibeB), and other related genes.
According to the sample delivery requirements, E. coli were cultured overnight in nutritional broth, then washed several times. The whole genome of E. coli was sequenced using Illumina NovaSeq PE150 at the Beijing Novogene Bioinformatics Technology Co., Ltd. All good quality paired reads were assembled using the SOAPdenovo (http://soap.genomics.org.cn/soapdenovo.html). The default value for the k-mer was 63, and contigs with > 500 bp were retained. The assembly results were integrated using CISAV20140304 (https://bio.tools/cisa). The serotype, virulence genes, and MLST results were counted using the Centre for Genomic Epidemiology website (http://genomicepidemiology.org/). The ST results were used for cluster analysis using goeBURST of PHYLOVIZ-2.0, and the spliced gene sequences were analyzed using MEGA 7.0 software for making of phylogenetic tree.
Results
Bacteria isolation, culture, and identification
The isolated strain showed a single pink colony on MacConkey medium and purplish-black with a metallic luster on the eosin Meilan medium. The 622 bp target bands of the isolated strains were detected by gel electrophoresis of the PCR products, and all 30 isolated strains were confirmed to be E. coli.
Drug resistance detection
The results of resistance of E. coli isolates to 23 antibacterial agents were shown in Table 2. The resistance rates of Penicillin, Amoxicillin, Ampicillin, Tetracycline, Sulfamethoxa, Chloramphenicol, Flufenicol, and Rifampicin were higher than 70%. The drug-resistant genes in the 30 identified E. coli strains were detected using PCR. The dominant drug-resistant genes identified in the samples from farm I included optrA, oqxA, and qnrS; the dominant drug-resistant gene from farm II was oqxA; and the dominant drug-resistant gene from farm III was floR (Table 3). Overall, 93.3% of the strains carried the drug-resistant gene oqxA, which was the highest total detection rate, and this was followed by blaCIT, blaTEM, floR, optrA, sul2, and qnrS, with detection rates of > 60%. mcr2 was not detected in any of the isolate.
Carrying status of virulence genes
The whole genome database for the 30 strains of E. coli was succesfully sequenced and found to contain 20 different virulence genes (Figure 1). Overall, the 30 strains of E. coli showed higher carrying rates for three virulence genes, fimC (93.3%), tsh (66.7%), and ompT (53.3%); the carrying rates of cvaC, ler, and ibeB were the lowest (13.3%), and the carrying rates of the remaining 14 virulence genes were 16.7%–33.3%. The scatter plot showed no correlation between the number of drug-resistant genes and virulence genes carried by the same strain (Figure 2).
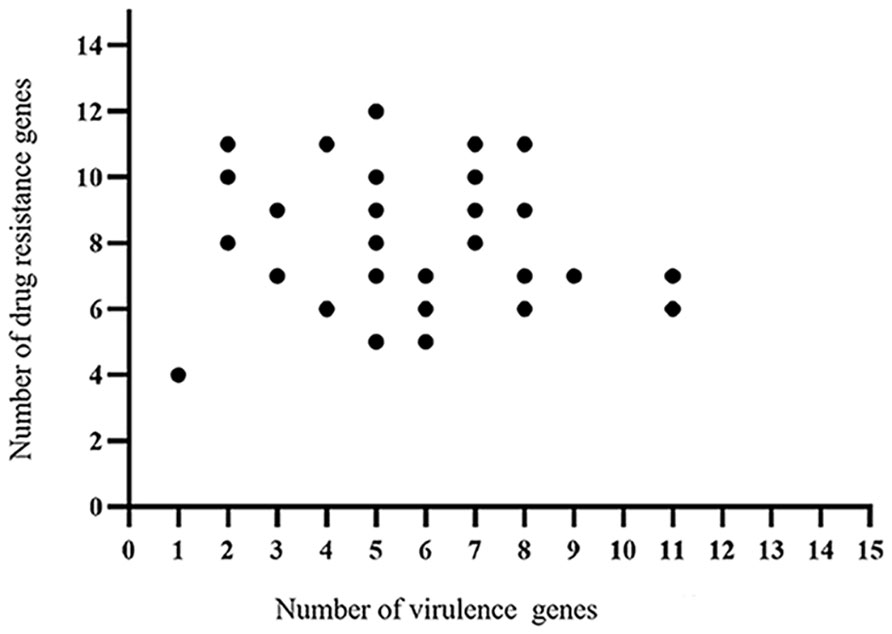
Figure 2 Correlation analysis between the virulence genes and drug-resistant genes identified in the 30 strains E. coli.
O antigen serotype of E. coli
Serotype analysis showed that 24 strains of E.coli were classified, covering 15 serotypes, and no cross-serotypes were detected. There were significant differences in the serotypes among the three duck farms, and only I-9 and III-6 shared a common serotype O9. The most prevalent serotype was O81 (5/24), and this was followed by O174 (2/24), O9 (2/24), O51 (2/24), O98 (2/24), and O86 (2/24) (Table 4).
Phylogenetic group identification
The phylogenetic groups of the 30 E. coli strains were studied using triple PCR. The isolates from farms I and III were mainly from group D, and the isolates from farm II were mainly from group B2 (Table 5). Overall, among the 30 isolates, more strains were identified as part of the highly pathogenic groups D (46.7%) and B2 (26.7%), and fewer strains belonged to the non-pathogenic group A (20%) and low pathogenic group B1 (6.7%). Analysis of the correlation between drug-resistant genes and the phylogenetic groups of E. coli revealed the types of drug-resistant genes carried by groups B2 and D were more abundant than those carried by groups A and B1 (Figure 3). Group B2 isolates mainly carried blaTEM and oqxA but did not carry mcr2, whereas group D isolates mainly carried oqxA, qnrS, blaTEM, and sul2 but did not carry aac (Ugwu et al., 2020)-II, and mcr2. E. coli carrying the resistance genes blaTEM and blaCIT were mainly distributed in groups B2 and D.
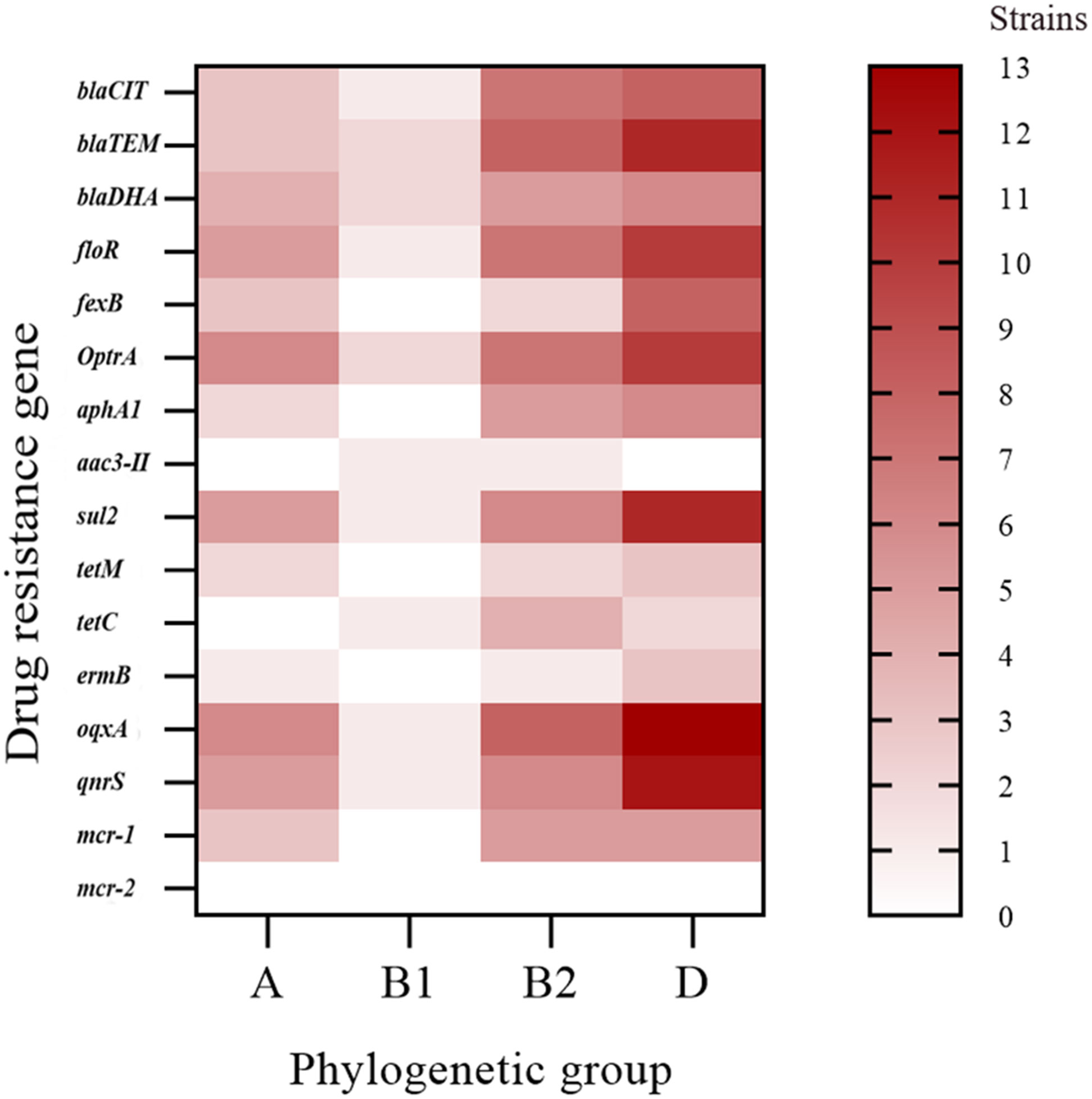
Figure 3 Distribution heat map showing the drug-resistant genes in the different phylogenetic groups.
Multilocus sequence typing
Overall, 10 STs were identified from farm I, eight from farm II, and six from farm III (Table 6). ST2179 was the most widely distributed ST and was identified on farms I and III, whereas the rest of the ST were only identified at single duck farms. Newly discovered STs (N1–N5) were found on each farm.
The 30 E. coli strains can be divided into 23 STs, which were ST1629, N1, ST12233, ST6803, ST7013, N2, ST6707, ST3871, ST2179, ST10591, ST6709, ST48, N3, ST3107, N4, ST701, ST226, ST6790, ST1286, ST7201, ST155, ST3856, and N5. Among these, ST3856 (n = 5) was the dominant sequence type, accounting for 16.7%, followed by ST2179 (n = 2), ST48 (n = 2), and ST6790 (n = 2), each accounting for 6.7%, while the remaining 19 STs accounted for 3.3% (n = 1).
According to the combination of STs, virulence genes and phylogenetic groups, strains I-9 and III-6 both belonged to ST2179, serotype O9, which carried 11 virulence genes, and they were classified into phylogenetic group D. Strains III-4, III-5, III-8, III-9, and III-10 all belonged to ST3856, and their serotype was O81, but their virulence gene types and phylogenetic groups were quite different. Strain III-8 carried only one virulence gene and was the least virulent among the 30 strains of E. coli. Different phylogenetic groups were associated with the number of virulence gene types.
Genetic evolution analysis
Cluster analysis of the ST results was performed using the goeBURST program in PHYLOVIZ-2.0 (Figure 4). Each circle represented an ST, the circle size represented the number of strains, and groups 1 and 2 represented two clone groups, respectively. Cluster analysis showed that the 30 isolates were divided into two clonal complexes (CCs) and 19 independent types, the CC1 included ST48 (farm II) and N3 (farm II), and the CC2 included ST7013 (farm I) and ST6790 (farm II).
The phylogenetic tree of the STs from the 30 E. coli strains was constructed using the neighbor-joining (NJ) method in MEGA7.0. The 30 E. coli isolates were divided into six branches: The same duck strains were relatively concentrated in the same branch, and the different duck strains were located in the different branch (Figure 5).
Discussion
Analysis of the drug resistance results
Drug-resistant genes have become new environmental pollutants, leading to dramatic increases in the number of drug-resistant bacteria. In recent years, there are few reports on drug-resistant genes in E. coli of environmental origin. In this study, the resistance rate of E. coli isolates to several kinds of antibiotics was higher. The third-generation cephalosporin is a broad-spectrum antibiotic, which has bacteriostatic effect on enterobacterium. The resistance rate of E. coli isolated from farm II to Cefotaxime and Ceftriazone was up to more than 50%, which was similar to the study of Guo et al. (2023). This may be related to the frequent use of such drugs in clinic. Excessive or unreasonable use of antibiotics leads to serious drug resistance. Rifampicin is effective against most gram-positive and negative bacteria. E. coli isolated from three farms had a 100% resistance rate, which was similar to the results of Zhang; E. coli isolated had a 97% resistance rate from duck farms in Sichuan province of China (Zhang et al., 2021). In the study on Campylobacter isolated from duck farms in China, the rate of resistance to Chloramphenicol was 42.7% (Han et al., 2019), which was similar to the results of this study, whereas Zendehbad et al. reported that the rate of resistance was 5.3% (Zendehbad et al., 2015), and Jamali reported that the rate of resistance was 4.4% (Jamali et al., 2015). The reason for this phenomenon may be different countries and regions in the treatment of animal diseases or in the addition of antibiotics in feed type and dosage standards.
Thirthy E. coli strains were assessed, and 93.3% were found to carry the drug-resistant gene oqxA, which was the highest detection rate of all resistance genes assessed. The oqxA resistance gene may thus be the dominant drug-resistant gene in duck farms in the Zhanjiang area of China. oqxA is an efflux pump that mediates bacterial resistance to quinolones via plasmids. It can coexist with other quinolone genes and also spread horizontally among different bacteria (Yamane et al., 2007). Extended spectrum β-lactamases (ESBLs) are the main mechanism of resistance to β -lactamase, and TEM is one of the main genotypes of ESBLs. Mijalli et al (Al-Mijalli, 2016). found that the blaTEM carrying rate was 20.5% in 75 urine E. coli strains isolated from urology clinics in Saudi Arabia, and Lalzampuia et al. (2013) found that the blaTEM carrying rate was 2.2% in eight ESBL strains from pigs in northeast India. In this study, 76.7% of the E. coli isolates carried blaTEM, which was relatively high, indicating that the epidemic distribution of ESBL-producing strains varied with different regions and sources. In addition, the SHV, CTX-M, and OXA genes have also become a focus of research in relation to ESBL-producing E. coli. The present study also explored the presence of other ESBL-producing genes.
Analysis of virulence genes in E. coli
The 30 E. coli strains used in this study were found to contain 20 virulence genes, and duck farms I, II, and III carried 18, 20, and 19 virulence genes, respectively. Overall, the 30 E. coli strains mainly carried fimC (93.3%), tsh (66.7%), and ompT (53.3%) virulence genes. fimC is an adhesion factor virulence gene, tsh is an E. coli serine protease autotransporter, and ompT can cause host diseases by processing or degrading a variety of host proteins (Goudarztalejerdi et al., 2020). This data thus indicated that if the E. coli from the duck farms in the Zhanjiang area enters the animal body, it could cause disease, mainly through the action of adhesins, transport systems, and enzymes. Adhesion is the basis of bacterial pathogenicity, and fimC is an important component of type I fimbriae. Goudarztalejerdi et al. reported that 87% of fimC was detected in 100 avian pathogenic E. coli strains and 95% of fimC was detected in 100 avian faecal E. coli strains in Iran (Goudarztalejerdi et al., 2020). In this investigation, the carrying rate of fimC was 93.3%, which is similar to the results reported above. Furthermore, there was no correlation found between the number of drug-resistant genes and virulence genes carried by the same strain.
Analysis of serotype results
Bacterial serotypes were counted by WGS (Zhang et al., 2015). Among the 30 E. coli strains isolated in this study, 24 (80%) were serotyped, and the typing rate was high. There were 15 serotypes with large differences within each duck farm. Only I-9 and III-6 had the same serotype, O9, which proved that the serotypes of strains from different farms in the same area were different, and the same serotype strains probably existed in different farms. O81, O174, O9, O51, O98, and O86 were the epidemic serotypes of the isolates, among which O81 was the most common, and no mixed serotype strains were found. Shimaa et al. found that the dominant serotype of E. coli isolated from falcons and Great Spotted Falcons was O158 (Shimaa and Samy, 2016). Thanh et al. isolated 975 strains of E. coli from chickens, farm wild animals (ants, geckos, flies, and mice), and the environment and found that the dominant serotype was O18 (Nguyen et al., 2021). The dominant serotypes of the E. coli from different sources vary. In addition, some studies have also shown that the E. coli serotype is associated with pathogenicity, and thus serotype could help to indicate the pathogenicity of E. coli.
Analysis of phylogenetic group results
Phylogenetic analysis showed that the 30 isolates belonged mainly to groups D (46.7%) and B2 (26.7%). This was different from previous phylogenetic group results for E. coli strains, which found that they mainly belonging to group A; such as Rehman et al. who isolated E. coli from yaks with diarrhoea in the Qinghai Plateau, China; Rahayuningtyas et al. who isolated 140 strains of E. coli from raw sewage in Kuwait; and Nancy et al. who isolated 116 strains of human symbiotic E. coli, most of which belonged to group A (Rehman et al., 2017; Stoppe et al., 2017; Rahayuningtyas et al., 2020). A certain association between phylogenetic group and virulence factors has been reproted. Group B2 has the strongest virulence, with more than three virulence genes, followed by group D with two to three virulence genes (Hesam et al., 2015). Our study showed that strains I-9 and III-6 carried the most virulence genes and both belonged to group D, which is different from previous reports. According to the correlation analysis between drug-resistant genes and phylogenetic groups of E. coli, the types of drug-resistant genes in groups B2 and D were more abundant than those in groups A and B1, and the numbers of β-lactam resistance genes blaTEM and blaCIT in groups B2 and D were greater than those in groups A and B1. It was speculated that the E. coli in groups B2 and D could easily acquire drug resistance mechanisms and that there may be a positive correlation between blaTEM and blaCIT resistance genes and virulence, which needs to be further explored.
Analysis of multi-point sequence typing results
Multisite sequence typing reflects the evolutionary biology of bacteria. According to the statistics in our study, the ST of E. coli isolated from duck farm III was the least, whereas that from farm I was the most, and all 10 strains had different STs, which indicated that E. coli in farm III had good homology and E. coli in farm I had poor homology. ST2179 E. coli was also detected in farms I and III (I-9 and III-6). Cluster and phylogenetic tree analyses showed that the E. coli had certain genetic relationship in different areas and that the E. coli had spread across different duck farms. The serotype of the two strains of ST2179 E. coli with the most abundant virulence genes was O9. This serotype is common in pig and sheep farms and poses a threat to farmed animals (Chen et al., 2004), indicating that ST2179 E. coli is not only widely distributed but also has strong toxicity and pathogenicity. Factors such as time, location, and strain source affect the distribution of the dominant STs. The 30 E. coli isolates were divided into 23 STs (including five new STs), with ST3856 being the dominant type. Epidemiological survey data have shown that certain STs are associated with specific diseases. For example, some researchers have found that E. coli sequence type ST131 is an epidemic sequence with a high incidence of parenteral pathogenic E. coli infection. ST131 E. coli can cause urinary tract infections, meningitis, blood infections, and septicemia (Sabiha et al., 2017). Sana et al. found that the dominant ST of 119 ESBLs and carbapenase-producing E. coli strains isolated from Islamabad, Pakistan, was ST131 (Ilyas et al., 2021). Seni et al. studied a population of parenterally pathogenic E. coli (ExPEC) in Africa and identified different evolutionary branches of ST131 (Seni et al., 2018). However, the relationship between ST131 strain and specific diseases is not absolute, as ST131 was detected in healthy people in Portugal (Rodrigues et al., 2016), and ST131 was not detected in Avian pathogenic E. coli (APEC) in Spain (Sola-Gines et al., 2015). At present, the relationship between the E. coli ST3856 sequence type and specific diseases has not been determined.
In summary, E. coli from aquaculture farms carried abundant drug-resistant genes, and nine kinds of drug-resistant genes were detected to varying degrees, with the highest detection rate of oqxA gene, indicating that the E. coli from Zhanjiang duck farms was mainly resistant to quinolone antibiotics. Thirty strains of E. coli mainly carried fimC, tsh, and ompT virulence genes, indicating that the strains caused the disease mainly through adhesion, transport system or enzyme action. The strains of E. coli in farm II carried the most abundant virulence genes, and the virulence effect was diverse. There was no correlation between the number of resistance genes and virulence genes. The serotyping rate of 30 strains of E. coli was 80%, and O81 was the dominant serotype. Most of the isolates belonged to groups B2 and D; they carried more drug-resistant gene types than groups A and B1. There were 23 STs in 30 E. coli strains, and ST3856 was an epidemic ST. Phylogenetic group and phylogenetic tree showed that there was a certain genetic relationship between strains from different farms. However, this study also has some limitations such as few sampling points and lack of more comprehensive analysis.
Data availability statement
The original contributions presented in the study are included in the article/supplementary material. Further inquiries can be directed to the corresponding authors.
Ethics statement
The animal study was reviewed and approved by Ethics Committee of Guangdong Ocean University.
Author contributions
SL: conceived and designed the experiments. CL: methodology and writing of the original draft. CL and YDa: formal analysis and investigation. CL, ST, and TZ: data curation. JP, YDi, and YM: study conceptualization. JP, YDi, and YM: supervised the study. YDi and YM: writing, review, and editing. All authors contributed to the manuscript and approved the submitted version.
Funding
This work was supported by the Natural Science Foundation of Guangdong Province, China (2023A1515012181), the Zhanjiang Science and Technology Bureau 2021 Provincial Science and Technology Special Funds (“Big Special + Task List”) Competitive Allocation Project (2021A05231), and the Start-Up Research Project of Maoming Laboratory, Guangdong Laboratory for Lingnan Modern Agriculture (2021TDQD002).
Acknowledgments
We would like to thank the Funding for supporting the successful completion of this study and the editors for their revision suggestions.
Conflict of interest
The authors declare that the research was conducted in the absence of any commercial or financial relationships that could be construed as a potential conflict of interest.
Publisher’s note
All claims expressed in this article are solely those of the authors and do not necessarily represent those of their affiliated organizations, or those of the publisher, the editors and the reviewers. Any product that may be evaluated in this article, or claim that may be made by its manufacturer, is not guaranteed or endorsed by the publisher.
References
Al-Mijalli, S. H. S. (2016). Extended-spectrum β-lactamase enzymes (ESBLs) produced by Escherichia coli urinary pathogens at Riyadh, Saudi Arabia. Int. J. Antimicrob. Ag. 2, 1–9. doi: 10.4172/2472-1212.1000125
Alneama, R. T., Al-Massody, A. J., Mahmud, B. M., Ghasemianc, A. (2021). The existence and expression of aminoglycoside resistance genes among multidrug-resistant Escherichia coli isolates in intensive care unit centers. Gene Rep. 25, 101315. doi: 10.1016/j.genrep.2021.101315
Chen, X., Gao, S., Jiao, X. N., Liu, X. F. (2004). Prevalence of serogroups and virulence factors of Escherichia coli strains isolated from pigs with postweaning diarrhoea in eastern China. Vet. Microbiol. 103 (1), 13–20. doi: 10.1016/j.vetmic.2004.06.014
Choi, J. H., Moon, D. C., Mechesso, A. F., Kang, H. Y., Kim, S. J., Song, H. J., et al. (2021). Antimicrobial resistance profiles and macrolide resistance mechanisms of Campylobacter coli isolated from pigs and chickens. Microorganisms. 9, 1077. doi: 10.3390/microorganisms9051077
Clermont, O., Bonacorsi, S., Bingen, E. (2000). Rapid and simple determination of the Escherichia coli phylogenetic group. Appl. Environ. Microb. 66, 4555–4558. doi: 10.1128/AEM.66.10.4555-4558.2000
Clinical and Laboratory Standards Institute (CLSI) (2021). Performance standards for antimicrobial susceptibility testing. Ed. Wayne, T. (PA: Clinical and Laboratory Standards Institute,CLSI document M100-S31) https://clsi.org/media/c40o0gu1/m100ed31_correction_notice_web_20210409.pdf.
Cloeckaert, A., Baucheron, S., Flaujac, G., Schwarz, S., Kehrenberg, C., Martel, J. L., et al. (2000). Plasmid-mediated florfenicol resistance encoded by the floR gene in Escherichia coli isolated from cattle. Antimicrob. Agents Ch. 44, 2858–2860. doi: 10.1128/AAC.44.10.2858-2860.2000
Coura, F. M., Diniz, S. D. A., Silva, M. X., Mussi, J. M. S., Barbosa, S. M., Lage, A. P., et al. (2015). Phylogenetic group determination of Escherichia coli isolated from animals samples. Sci. World J. 258424. doi: 10.1155/2015/258424
Galarce, N., Sanchez, F., Escobar, B., Lapierre, L., Cornejo, J., Alegria-Moran, R., et al. (2021). Genomic epidemiology of shiga toxin-producing Escherichia coli isolated from the livestock-Food-Human interface in south America. Animals. 11, 1845. doi: 10.3390/ani11071845
Goudarztalejerdi, A., Mohammadzadeh, A., Najafi, S. V., Nargesi, F., Joudari, S. (2020). Serogrouping, phylotyping, and virulence genotyping of commensal and avian pathogenic Escherichia coli isolated from broilers in hamedan, Iran. Comp. Immunol. Microb. 73, 101558. doi: 10.1016/j.cimid.2020.101558
Guo, L. L., Xiao, T. A., Wu, L. Q., Li, Y., Duan, X. X., Liu, W. H., et al. (2023). Comprehensive profiling of serotypes, antimicrobial resistance and virulence of salmonella isolates from food animals in China, 2015-2021. Front. Microbiol. 14. doi: 10.3389/fmicb.2023.1133241
Han, X. F., Guan, X. A., Zeng, H., Li, J. K., Huang, X. L., Wen, Y. P., et al. (2019). Prevalence, antimicrobial resistance profiles and virulence-associated genes of thermophilic campylobacter spp. isolated from ducks in a Chinese slaughterhouse. Food Control. 104, 157–166. doi: 10.1016/j.foodcont.2019.04.038
Hardiati, A., Safika, S., Wibawan, I. W. T., Indrawati, A., Pasaribu, F. H. (2021). Isolation and detection of antibiotics resistance genes of Escherichia coli from broiler farms in sukabumi, Indonesia. J. Adv. Vet. Anim. Res. 1, 84–90. doi: 10.5455/javar.2021.h489
Hesam, A., Reza, G., Mohammad, R. A., Mohammad, H. S. (2015). Determination of phylogenetic groups and antibiotic resistance pattern of enterotoxigenic Escherishia coli isolates from diarrheoic cases in bam city by PCR. Iranian J. Med. Microbiol. 9, 6–13.
Hosseini, S. S., Eslami, G., Zandi, H., Vakili, M. (2016). Frequency of oqxA and oqxB plasmid-mediated quinolone resistance genes in Escherichia coli isolated from urine of inpatients with urinary tract infections in yazd city, Iran. J. Isfahan Med. School. 34, 1211–1217.
Hu, Q. H., Tu, J., Han, X. G., Zhu, Y. Y., Ding, C., Yu, S. Q. (2011). Development of multiplex PCR assay for rapid detection of Riemerella anatipestifer, Escherichia coli, and Salmonella enterica simultaneously from ducks. J. Microbiol. Meth. 87, 64–69. doi: 10.1016/j.mimet.2011.07.007
Ilyas, S., Rasool, M. H., Arshed, M. J., Qamar, M. U., Aslam, B., Almatroudi, A., et al. (2021). The Escherichia coli sequence type 131 harboring extended-spectrum beta-lactamases and carbapenemases genes from poultry birds. Infect. Drug Resist. 14, 805–813. doi: 10.2147/IDR.S296219
Jamali, H., Ghaderpour, A., Radmehr, B., Wei, K. S. C., Chai, L. C., Ismail, S. (2015). Prevalence and antimicrobial resistance of campylobacter species isolates in ducks and geese. Food Control. 50, 328–330. doi: 10.1016/j.foodcont.2014.09.016
Jiang, H., Cheng, H., Liang, Y., Yu, S. T., Yu, T., Fang, J. H., et al. (2019). Diverse mobile genetic elements and conjugal transferability of sulfonamide resistance genes (sul1, sul2, and sul3) in Escherichia coli isolates from penaeus vannamei and pork from Large markets in zhejiang, China. Front. Microbiol. 10. doi: 10.3389/fmicb.2020.01793
Lalzampuia, H., Dutta, T. K., Warjri, I., Chandra, R. (2013). PCR-based detection of extended-spectrum β-lactamases (bla CTX-M-1 and bla TEM ) in Escherichia coli, Salmonella spp. and Klebsiella pneumoniae isolated from pigs in north Eastern India (Mizoram). Indian J. Microbiol. 53, 291–296. doi: 10.1007/s12088-013-0378-z
Liu, B., Furevi, A., Perepelov, A. V., Guo, X., Cao, H. C., Wang, Q., et al. (2019). Structure and genetics of Escherichia coli O antigens. FEMS Microbiol. Rev. 44, 655–683. doi: 10.1093/femsre/fuz028
Liu, Y. Y., Wang, Y., Walsh, T. R., Yi, L. X., Zhang, R., Spencer, J., et al. (2016). Emergence of plasmid-mediated colistin resistance mechanism MCR-1 in animals and human beings in China: a microbiological and molecular biological study. Infect. Dis-Nor. 16, 161–168. doi: 10.1016/S1473-3099(15)00424-7
Monstein, H. J., Ostholm-Balkhed, A., Nilsson, M. V., Nilsson, M., Dornbusch, K., Nilsson, L. E. (2007). Multiplex PCR amplification assay for the detection of blaSHV, blaTEM and blaCTX-m genes in Enterobacteriaceae. APMIS. 115, 1400–1408. doi: 10.1111/j.16000463.2007.00722.x
Nguyen, L. T., Thuan, N. K., Tam, N. T., Huyen, T. C. T., Khanh, N. P., Bich, T. N., et al. (2021). Prevalence and genetic relationship of predominant Escherichia coli serotypes isolated from poultry, wild animals, and environment in the Mekong delta, Vietnam. Veterinary Med. Int. 6504648. doi: 10.1155/2021/6504648
Niu, J. L., Peng, J. J., Ming, Y. Y., Ma, Q. C., Liu, W. C., Ma, Y. (2020). Identification of drug resistance genes and drug resistance analysis of Salmonella in the duck farm environment of zhanjiang, China. Environ. Sci. pollut. Res. Int. 27, 24999–25008. doi: 10.1007/s11356-020-09007-5
Rahayuningtyas, I., Indrawati, A., Wibawan, I. W. T., Palupi, M. F., Istiyaningsih, I. (2020). Phylogenetic group determination and plasmid virulence gene profiles of colistin-resistant Escherichia coli originated from the broiler meat supply chain in bogor, Indonesia. Vet. world. 13, 1807–1814. doi: 10.14202/vetworld.2020.1807-1814
Ramadan, H., Jackson, C. R., Frye, J. G., Hiott, L. M., Samir, M., Awad, A., et al. (2020). Antimicrobial resistance, genetic diversity and multilocus sequence typing of Escherichia coli from humans, retail chicken and ground beef in Egypt. Pathogens. 9, 357. doi: 10.3390/pathogens9050357
Rehman, M. U., Zhang, H., Iqbal, M. K., Mehmood, K., Huang, S. C., Nabi, F., et al. (2017). Antibiotic resistance, serogroups, virulence genes, and phylogenetic groups of escherichia coli isolated from yaks with diarrhea in qinghai plateau, China. Gut Pathog. 9, 24. doi: 10.1186/s13099-017-0174-0
Rodrigues, C., Machado, E., Fernandes, S., Peixe, L., Novais, A. (2016). An update on faecal carriage of ESBL-producing Enterobacteriaceae by Portuguese healthy humans: detection of the H30 subclone of B2-ST131 Escherichia coli producing CTX-M-27. J. Antimicrob. Chemoth. 71, 1120–1122. doi: 10.1093/jac/dkv443
Sabiha, S., Amit, R., Sumeet, K. T., Arif, H., Nishant, N., Narender, K., et al. (2017). Comparative genomic analysis of globally dominant ST131 clone with other epidemiologically successful extraintestinal path ogenic Escherichia coli (ExPEC) lineages. ASM. 8, e01596–e01517. doi: 10.1128/mBio.01596-17
Saenz, Y., Brinas, L., Dominguez, E., Ruiz, J., Zarazaga, M., Vila, J., et al. (2004). Mechanisms of resistance in multiple-antibiotic-resistant Escherichia coli strains of human, animal, and food origins. Antimicrob. Agents Ch. 10, 3996–4001. doi: 10.1128/AAC.48.10.3996-4001.2004
Sarba, E. J., Kelbesa, K. A., Bayu, M. D., Gebremedhin, E. Z., Borena, B. M., Teshale, A. (2019). Identification and antimicrobial susceptibility profile of Escherichia coli isolated from backyard chicken in and around ambo, central Ethiopia. BMC Vet. Res. 15, 85. doi: 10.1186/s12917-019-1830-z
Seni, J., Peirano, G., Okon, K. O., Jibrin, Y. B., Mohammed, A., Mshana, S. E., et al. (2018). The population structure of clinical extra-intestinal Escherichia coli in a teaching hospital from Nigeria. Diagn. Microbiol. Infect. Dis. 92, 46–49. doi: 10.1016/j.diagmicrobio.2018.04.001
Shimaa, M. A., Samy, A. K. (2016). Molecular studies on E.coli serotypes in zoo birds. Alexandria J. Veterinary Sci. 51, 7. doi: 10.5455/ajvs.230647
Sola-Gines, M., Cameron-Veas, K., Badiola, I., Dolz, R., Majo, N., Dahbi, G., et al. (2015). Diversity of multi-drug resistant avian pathogenic Escherichia coli (APEC) causing outbreaks of colibacillosis in broilers during 2012 in Spain. PloS One 10, 143191. doi: 10.1371/journal.pone.0143191
Srivani, M., Narasimha, Y. R., Subramanyam, K. V., Ramakoti, M. R., Srinivasa, T. R. (2017). Prevalence and antimicrobial resistance pattern of shiga toxigenic Escherichia coli in diarrheic buffalo calves. Veterinary World. 10, 774–778. doi: 10.14202/vetworld.2017.774-778
Stoppe, N. D., Silva, J. S., Carlos, C., Sato, M. I. Z., Saraiva, A. M., Ottoboni, L. M., et al. (2017). Worldwide phylogenetic group patterns of Escherichia coli from commensal human and wastewater treatment plant isolates. Front. Microbiol. 8. doi: 10.3389/fmicb.2017.02512
Sun, C. T., Wang, Y. C., Ma, S. Z., Zhang, S., Liu, D. J., Wang, Y., et al. (2021). Surveillance of antimicrobial resistance in Escherichia coli and Enterococci from food products at retail in Beijing, China. Food Control. 119, 107483. doi: 10.1016/j.foodcont.2020.107483
Ugwu, I. C., Lee-Ching, L., Ugwu, C., Chah, K., Okoye, J. O. A. (2020). Virulence-associated genes of avian pathogenic e. coli isolated from chickens with colibacillosis in enugu state, Nigeria. Int. J. Infect. Dis. 101, 541. doi: 10.1016/j.ijid.2020.09.1404
Wu, J. J., Ko, W. C., Tsai, S. H., Yan, J. J. (2007). Prevalence of plasmid-mediated quinolone resistance determinants QnrA, QnrB, and QnrS among clinical isolates of Enterobacter cloacae in a Taiwanese hospital. Antimicrob. Agents Ch. 51, 1223–1227. doi: 10.1128/AAC.01195-06
Xavier, B. B., Lammens, C., Ruhal, R., Kumar-Singh, S., Butaye, P., Goossens, H., et al. (2016). Identification of a novel plasmid-mediated colistin-resistance gene, mcr-2, in Escherichia coli, Belgium, June 2016. Eurosurveillance. 21, 30280. doi: 10.2807/1560-7917.ES.2016.21.27.30280
Yamane, K., Wachino, J. I., Suzuki, S., Kimura, K., Shibata, N., Kato, H., et al. (2007). New plasmid-mediated fluoroquinolone efflux pump, QepA, found in an Escherichia coli clinical isolate. Antimicrob. Agents Ch. 51, 3354–3360. doi: 10.1128/AAC.00339-07
Ying, Y. Y., Wu, F., Wu, C. Y., Jiang, Y., Yin, M., Zhou, W. X., et al. (2019). Florfenicol resistance in enterobacteriaceae and whole-genome sequence analysis of florfenicol-resistant Leclercia adecarboxylata strain R25. Int. J. Genomics 9828504. doi: 10.1155/2019/9828504
Zendehbad, B., Khayatzadeh, J., Alipour, A. (2015). Prevalence, seasonality and antibiotic susceptibility of campylobacter spp. isolates of retail broiler meat in Iran. Food Control. 53, 41–45. doi: 10.1016/j.foodcont.2015.01.008
Zhang, S. Q., Chen, S. L., Abbas, M., Wang, M. S., Jia, R. Y., Chen, S., et al. (2021). High incidence of multi-drug resistance and heterogeneity of mobile genetic elements in escherichia coli isolates from diseased ducks in sichuan province of China. Ecotox Environ. Safe. 222, 112475. doi: 10.1016/j.ecoenv.2021.112475
Zhang, T., Wang, C. G., Lv, J. C., Wang, R. S., Zhong, X. H. (2012). Survey on tetracycline resistance and antibiotic-resistant genotype of avian Escherichia coli in north China. Poultry Sci. 91, 2774–2777. doi: 10.3382/ps.2013-92-2-0581
Keywords: Escherichia coli, drug resistance gene, virulence gene, molecular typing, duck farm
Citation: Luo S, Liao C, Peng J, Tao S, Zhang T, Dai Y, Ding Y and Ma Y (2023) Resistance and virulence gene analysis and molecular typing of Escherichia coli from duck farms in Zhanjiang, China. Front. Cell. Infect. Microbiol. 13:1202013. doi: 10.3389/fcimb.2023.1202013
Received: 12 April 2023; Accepted: 22 May 2023;
Published: 15 June 2023.
Edited by:
Juan Xicohtencatl-Cortes, Hospital Infantil de México Federico Gómez, MexicoReviewed by:
Aamir Ali, National Institute for Biotechnology and Genetic Engineering, PakistanSara A. OchoaHospital Infantil de México Federico Gómez, Mexico
Copyright © 2023 Luo, Liao, Peng, Tao, Zhang, Dai, Ding and Ma. This is an open-access article distributed under the terms of the Creative Commons Attribution License (CC BY). The use, distribution or reproduction in other forums is permitted, provided the original author(s) and the copyright owner(s) are credited and that the original publication in this journal is cited, in accordance with accepted academic practice. No use, distribution or reproduction is permitted which does not comply with these terms.
*Correspondence: Yuexia Ding, ZGluZ3l1ZXhpYTIwMDZAMTYzLmNvbQ==; Yi Ma, bWF5aTc2MUAxNjMuY29t
†These authors have contributed equally to this work