- 1College of Plant Protection, Shenyang Agricultural University, Shenyang, China
- 2State Key Laboratory for Biology of Plant Diseases and Insect Pests, Institute of Plant Protection, Chinese Academy of Agricultural Sciences, Beijing, China
- 3Institute of Vegetable, Tibet Academy of Agriculture and Animal Husbandry Sciences, Lhasa, China
Introduction: The bacterial endosymbiont, Wolbachia spp. induce thelytokous parthenogenesis in certain parasitoid wasps, such as the egg parasitoid wasps Trichogramma spp. To complete the cycle of vertical transmission, Wolbachia displays efficient transovarial transmission by targeting the reproductive tissues and often exhibits strong tissue-specific tropism in their host.
Method: The present study aimed to describe the basic Wolbachia distribution patterns that occur during the development of Wolbachia-infected, thelytokous Trichogramma dendrolimi, and T. pretiosum. We used fluorescence in situ hybridization (FISH) to investigate Wolbachia signal dynamics during early embryogenesis (from 30 to 120 min). Wolbachia titers and distributions from the embryo to adult stages of Trichogramma after early embryogenesis were detected by absolute quantitative polymerase chain reaction (AQ-PCR) and FISH. The symmetry ratios (SR) of the Wolbachia signals were calculated using the SR odds ratios in the anterior and posterior parts of the host. The SR was determined to describe Wolbachia tropism during early embryogenesis and various developmental stages of Trichogramma.
Results: Wolbachia was concentrated in the posterior part of the embryo during early embryogenesis and the various developmental stages of both T. dendrolimi and T. pretiosum. Wolbachia density increased with the number of nuclei and the initial mitotic division frequency during early embryogenesis. The total Wolbachia titer increased with postembryogenesis development in both T. dendrolimi and T. pretiosum. However, the Wolbachia densities relative to body size were significantly lower at the adult and pupal stages than they were at the embryonic stage.
Discussion: The present work revealed that posterior Wolbachia concentration during early host embryogenesis determined Wolbachia localization in adult wasps. By this mechanism, Wolbachia exhibits efficient vertical transmission across generations by depositing only female Wolbachia-infected offspring. The results of this study describe the dynamics of Wolbachia during the development of their Trichogramma host. The findings of this investigation helped clarify Wolbachia tropism in Trichogramma wasps.
Introduction
The bacterial endosymbiont, Wolbachia spp. resides in the cells of more than 40% of all terrestrial arthropod species (LePage et al., 2017; Salje, 2021). To ensure their transovarial transmission from host mothers to offspring, Wolbachia must invade host reproductive tissues and often cause reproductive alterations in their hosts such as cytoplasmic incompatibility, parthenogenesis, male killing, and feminization (Kaur et al., 2021). Wolbachia can induce thelytokous parthenogenesis in certain parasitoid wasps, such as Trichogramma spp. (Huigens et al., 2000; Zhou et al., 2022a; Zhou et al., 2023). Thelytokous parthenogenesis, also known as female-producing parthenogenesis, is defined as the production of female offspring without genetic contribution from paternal males (Ma and Schwander, 2017). In host wasps, Wolbachia can convert male-destined haploid eggs into homozygous diploid female eggs by inducing gamete duplication during the initial mitotic division (Wu et al., 2020; Zhou et al., 2022b).
The penetrance of parthenogenesis in Trichogramma wasps relies on the parthenogenesis-inducing (PI) Wolbachia titers in their germ line (Zhou et al., 2022b). Wolbachia invade host eggs, and complete vertical transmission cycles by targeting the germ line during host development, and exhibit strong tissue-specific tropism (Pietri et al., 2016). This tropism is determined by three different strategies (Landmann et al., 2012). First, the concentration of Wolbachia in the germ line is determined by their posterior localization in the early embryo, which is influenced by both the symmetric and asymmetric cell division segregation patterns during oogenesis and early embryogenesis (Albertson et al., 2009; Landmann et al., 2010). Second, this tropism is associated with cell-to-cell transmission (Serbus et al., 2008; Newton et al., 2015; Pietri et al., 2016). A previous study examined the penetration of Wolbachia into host cells and their subsequent migration from somatic tissues to the germ line, elucidating this tropism (Guo et al., 2019). Third, Wolbachia activates immune responses and modifies their titers in different tissues (Masson et al., 2016). As a rule, the host germ line originates from the posterior pole of the egg and the early embryo (Pietri et al., 2016). The fundamental distribution pattern of Wolbachia in developing host adult tissues may be determined by their asymmetrical distribution in the early embryo. Several studies have proposed that the tissue-specific distributions of Wolbachia are influenced by the localization pattern of these endosymbionts in the host embryo.
The distributions of Wolbachia during embryogenesis have been documented in numerous host species, revealing different patterns. Previous studies have reported that Wolbachia may exhibit an anterior concentration (Wright & Barr, 1981; Boyle et al., 1993; Guo et al., 2019), a posterior concentration (Zchori-Fein et al., 1998; Strunov et al., 2022), or a broad distribution (Breeuwer & Werren, 1990; Pintureau et al., 2000; Strunov et al., 2022) in different Wolbachia-host systems. Despite documentation of Wolbachia distribution in parasitoid embryos of Nasonia and Trichogramma wasps, different results have been reported even among closely related parasitoid species. For instance, the Wolbachia wVitA strain localizes to the posterior end at low density in N. vitripennis embryos, while in N. giraulti embryos, it localizes to the posterior end at high density and then spreads toward the anterior end (Chafee et al., 2011; Funkhouser-Jones et al., 2018). Wolbachia has been reported in the posterior poles of T. cordubensis embryos, whereas the posterior concentration of Wolbachia is not found in T. dendrolimi (Pintureau et al., 2000). However, the study on Trichogramma embryos was conducted at only one-time point. Little is known about how the dynamic distribution of Wolbachia during early embryogenesis shapes the germ-line-specific distribution of Wolbachia in adult Trichogramma. Further comprehensive studies are required to elucidate this process.
The egg parasitoid wasps Trichogramma dendrolimi Matsumura and T. pretiosum Riley have been widely used in agriculture and forestry for the biological control of various lepidopteran pests (Lindsey et al., 2018; Zhou et al., 2019). The sex determination mechanism of Trichogramma wasps is haplodiploid, where females arise from a diploid fertilized egg and males develop from unfertilized haploid eggs. Wolbachia infection often induces thelytokous parthenogenesis in Trichogramma wasps by triggering gamete duplication in early embryos. At least 15 Trichogramma species have been documented to exhibit thelytokous parthenogenesis when infected with PI-Wolbachia (Ma and Schwander, 2017; Wu et al., 2020; Zhou et al., 2022b). Compared to uninfected bisexual parasitoid wasps, Wolbachia-infected thelytokous wasps produce nearly 100% female offspring, which are effective in controlling insect pests (Zhou et al., 2020). Our previous study revealed that the potency of parthenogenesis in Wolbachia-infected wasps is related to the Wolbachia titers in the germ line. This study aimed to elucidate the dynamics of Wolbachia during early embryogenesis in the host and establish how these dynamics shape tissue-specific distributions of Wolbachia, enabling efficient vertical transmission in Trichogramma wasps. Fluorescence in situ hybridization (FISH) was used to detect dynamic Wolbachia signals during early embryogenesis (30–120 min) in T. dendrolimi and T. pretiosum. Absolute quantitative polymerase chain reaction (AQ-PCR) and FISH were employed to clarify the loading and distribution dynamics of Wolbachia from the embryonic to adult stages of T. dendrolimi and T. pretiosum. The results of the present study provided a detailed description of Wolbachia dynamics during early embryogenesis and throughout different developmental stages of the host. These findings contribute to our understanding of how Wolbachia tropism enhances its vertical transmission across generations of Trichogramma wasps.
Materials and methods
Insects
Wolbachia-infected thelytokous isofemale Trichogramma dendrolimi and T. pretiosum lines were reared on eggs of the rice moth Corcyra cephalonica [Stainton] (Lepidoptera: Pyralidae) at 25°C ± 1°C and 75% ± 5% RH and under a 16-h/8-h light/dark cycle. The C. cephalonica host was reared on a semi-artificial diet.
Wolbachia detection in Trichogramma by fluorescence in situ hybridization
FISH was conducted to detect Wolbachia signals in the early embryo (30, 50, 60, 70, 90, and 120 min) and at various developmental stages (24 h (late embryo), 48 h (larva), 72 h (larva), 120 h (pre-pupa), 168 h (pupa), and 240 h (adult)) of T. dendrolimi and T. pretiosum according to previously described procedures (Zhou et al., 2022a; Zhou et al., 2023). An egg card containing ~300 host eggs was introduced into a Durham’s tube (30 mm in length × 6 mm in diameter) and could be parasitized by ~500 T. dendrolimi or T. pretiosum females within 5 min. The parasitized eggs were then individually excised from the egg card at various times and dissected under a stereomicroscope (SV6; Carl Zeiss AG, Oberkochen, Germany) within 5 min. Trichogramma sample size uniformity was established and maintained by fixing the samples in Carnoy’s solution (1:3 (v/v) glacial acetic acid: methanol) for 48 h, rinsing them thrice in 50% (v/v) ethanol for 300 s each time, and treating them with destainer (6% (v/v) ethanol dissolved in perhydrol) for 48 h. The Trichogramma samples were then subjected twice to phosphate-buffered saline (PBS) for 300 s each time and transferred to a hybridization oven (HybriLinker HL-2000, UVP, USA) at 45 °C for 45 min. Wolbachia signals were indicated by two 5′-rhodamine-labeled Wolbachia probes (F: 5′-AATCCGGCCGAACCGACCC-3′ and R: 5′-CTTCTGTGAGTACCGTCATTATC-3′) targeting Wolbachia 16S rRNA. The Wolbachia distributions in the Trichogramma samples were photographed under a confocal laser scanning microscope (CLSM; Olympus FV3000, Monolith, Tokyo, Japan). The Wolbachia densities in the Trichogramma samples were quantified using the area of each Wolbachia signal weighted by the area of each Trichogramma sample via log transformation and with ImageJ software Version 1.53t (National Institutes of Health (NIH), Bethesda, MD, USA). To delineate asymmetric Wolbachia distributions in the Trichogramma samples, the early embryos were divided into anterior and posterior parts using the intermediate vertical boundaries of the lines from the anterior to posterior poles of the embryos. The symmetric ratios (SR) of the Wolbachia signals were calculated based on the odd ratios of the Wolbachia densities in the anterior and posterior parts of the embryos. The rates of the initial embryonic divisions were estimated based on the number of nuclei and the mitotic frequencies at various developmental times.
Wolbachia titer quantification by AQ-PCR
Trichogramma progeny were dissected and isolated from their host eggs to evaluate the Wolbachia titers in T. dendrolimi and T. pretiosum at 48 h (larva), 72 h (larva), 120 h (pre-pupa), 168 h (pupa), and 240 h (adult) after parasitization. As Trichogramma embryos are very small, those that developed at ≤ 24 h were not subjected to AQ-PCR. In total, 50 progeny served as a sample replicate. The Chelex-100 method was used to extract total DNA from the Trichogramma samples. The Wolbachia titers in the Trichogramma samples were measured by AQ-PCR and a specific primer pair (F: 5′-ATGATGTAGCCCCAGAAAT-3′; R: 5′-CACCAAAAGTGTTGTAAAGAA-3′) that was designed according to the Wolbachia wsp sequences (GenBank Accession No. MG914000) (Zhou et al., 2019). The AQ-PCR method used here was previously described by Zhou et al. (2019).
Data analysis
A structural equation model (SEM) was used to enumerate the nuclei and determine the mitotic frequency directly or indirectly influenced by the developmental stage, Wolbachia density, and SR (Grace, 2007). The SEM was simplified according to the lowest Akaike information criterion (AIC) value. The model was not rejected when the χ2 test p > 0.05, the standardized root mean square residual (SRMR) < 0.1, and the comparative fit index (CFI) > 0.90. The standardized coefficient was used to estimate the correlation between variable pairs by weighting the units and the changes in the different variables (Abrams, 2007; Grace, 2007). A one-way analysis of variance (ANOVA) was used to analyze the influences of the developmental stage on the Wolbachia density and the SR. Tukey’s test was applied for post hoc comparisons. All analyses were conducted in R software version 4.2.0 (R Development Core Team, 2021).
Results
Wolbachia dynamics at the early Trichogramma embryo stage
In the initial divisions of both the T. dendrolimi and T. pretiosum embryos, we detected more Wolbachia signals at the posterior than the anterior part. The SR values for the T. dendrolimi embryos were 0.070 ± 0.013 (30 min), 0.13 ± 0.038 (50 min), 0.12 ± 0.027 (60 min), 0.30 ± 0.047 (70 min), 0.17 ± 0.026 (90 min), and 0.31 ± 0.030 (120 min). The SR values for the T. pretiosum embryos were 0.16 ± 0.021 (30 min), 0.16 ± 0.022 (50 min), 0.13 ± 0.025 (60 min), 0.12 ± 0.031 (70 min), 0.16 ± 0.028 (90 min), and 0.19 ± 0.035 (120 min) (Figures 1, 2).
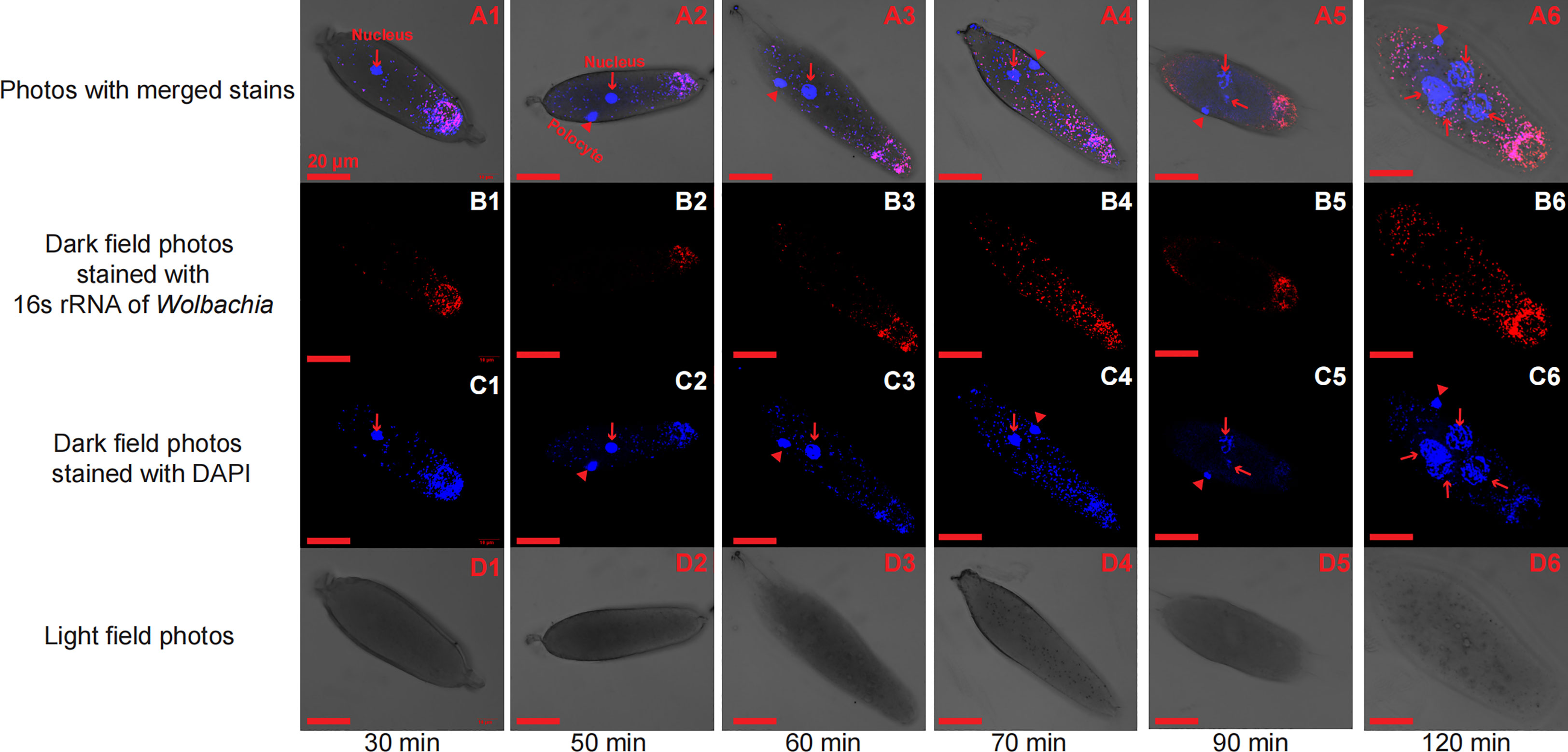
Figure 1 Early T. dendrolimi embryos stained with Wolbachia 16S rRNA (red) and DAPI (blue) probes at 30, 50, 60, 70, 90, and 120 min. A1, A2, A3, A4, A5, and A6 are light field photos stained with 16S rRNA of Wolbachia and DAPI signals. B1, B2, B3, B4, B5, and B6 are dark field photos stained with 16S rRNA of Wolbachia. C1, C2, C3, C4, C5, and C6 are dark field photos stained with DAPI signals. D1, D2, D3, D4, D5, and D6 are light field photos.
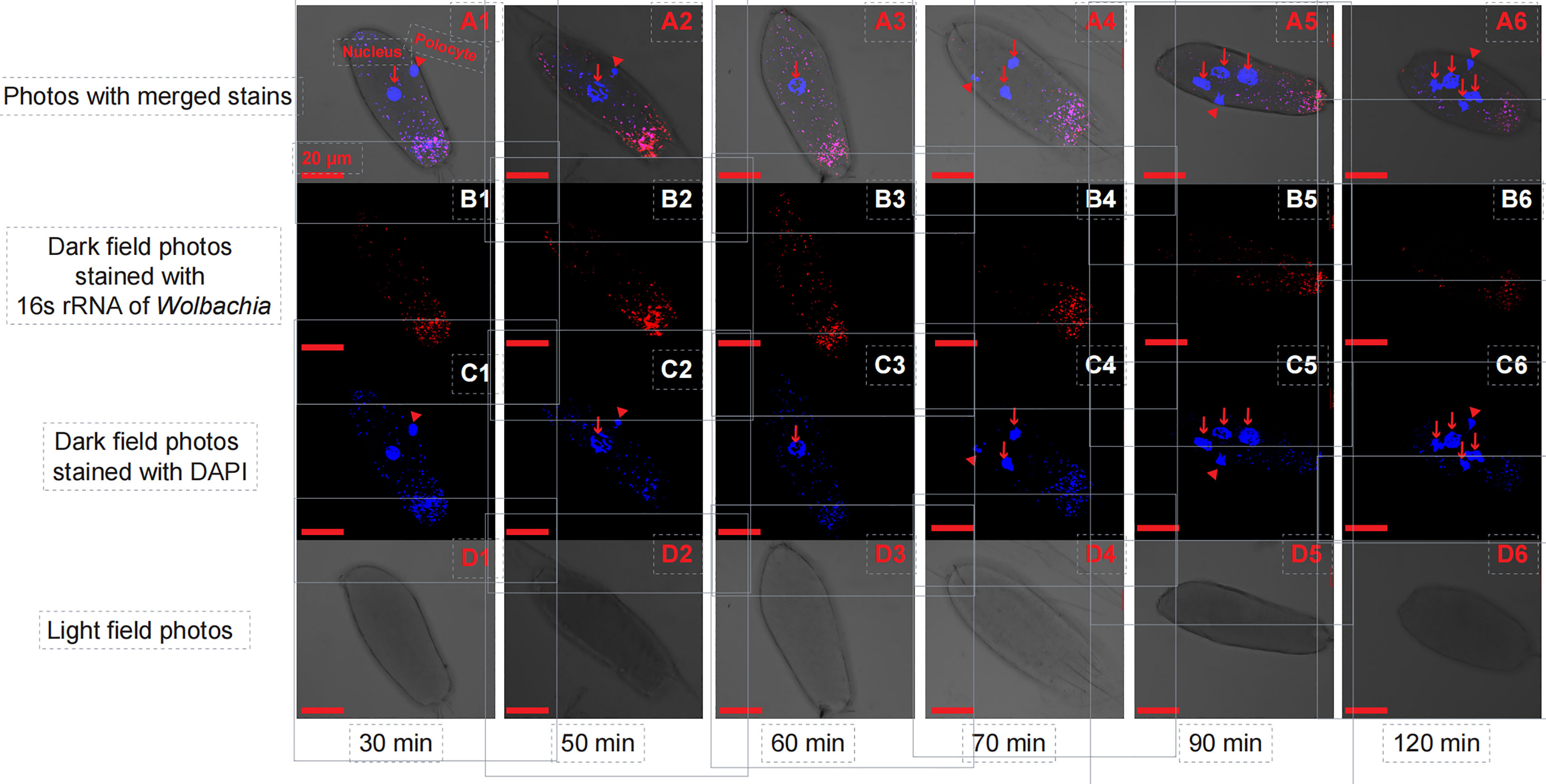
Figure 2 Early T. pretiosum embryos stained with Wolbachia 16S rRNA (red) and DAPI (blue) probes at 30, 50, 60, 70, 90, and 120 min. A1, A2, A3, A4, A5, and A6 are light field photos stained with 16S rRNA of Wolbachia and DAPI signals. B1, B2, B3, B4, B5, and B6 are dark field photos stained with 16S rRNA of Wolbachia. C1, C2, C3, C4, C5, and C6 are dark field photos stained with DAPI signals. D1, D2, D3, D4, D5, and D6 are light field photos.
The SEM for the T. dendrolimi embryos was not rejected after one path was excluded (χ2 = 0.28, df = 1, p = 0.60, CFI = 1.00, SRMR = 0.007). The Wolbachia density, SR, number of nuclei, and mitotic frequency increased with the stage of early embryonic development. The number of nuclei also increased with Wolbachia density, and the latter was positively correlated with the SR. The number of nuclei was positively correlated with the mitotic frequency (Figures 3–5).
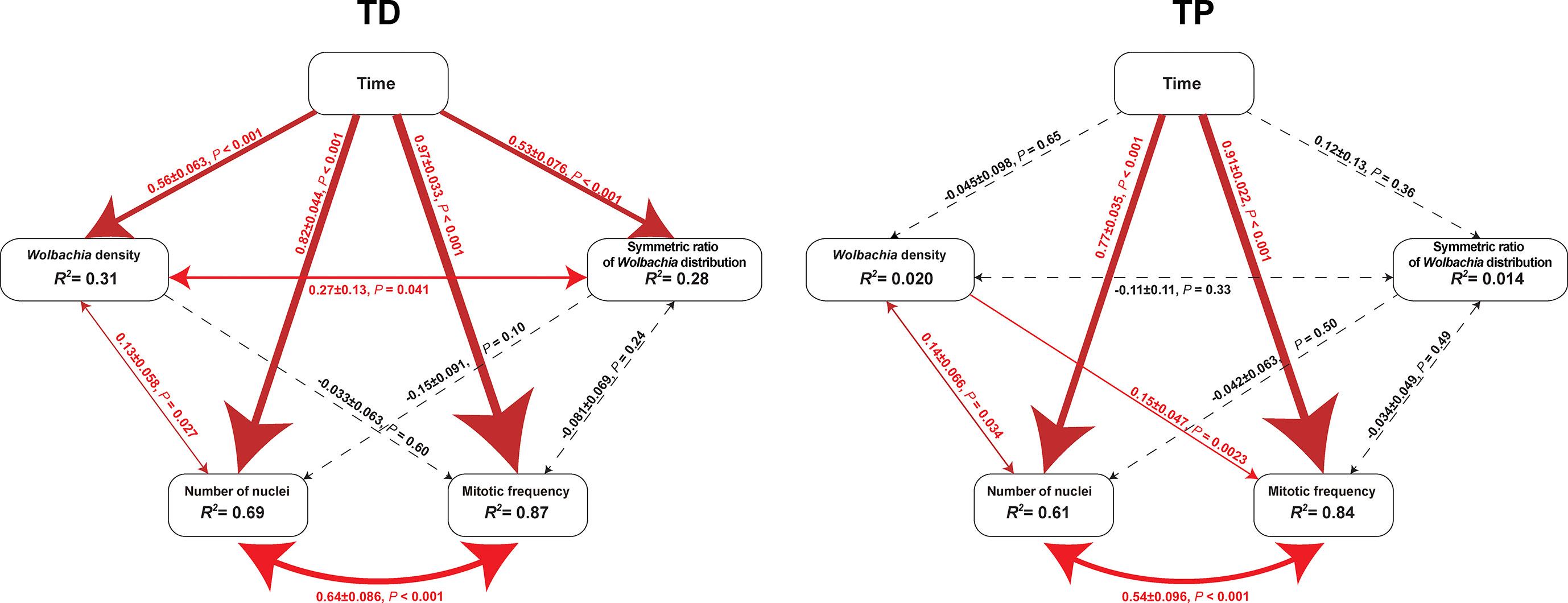
Figure 3 Diagrams of SEM paths of early embryogenesis in T. dendrolimi (TD) and T. pretiosum (TP). Red solid arrows indicate a significant correlation between the two variables. Black dashed arrows indicate a nonsignificant correlation between the two variables. The values alongside the lines indicate standardized coefficients and p-values for variable pairs.
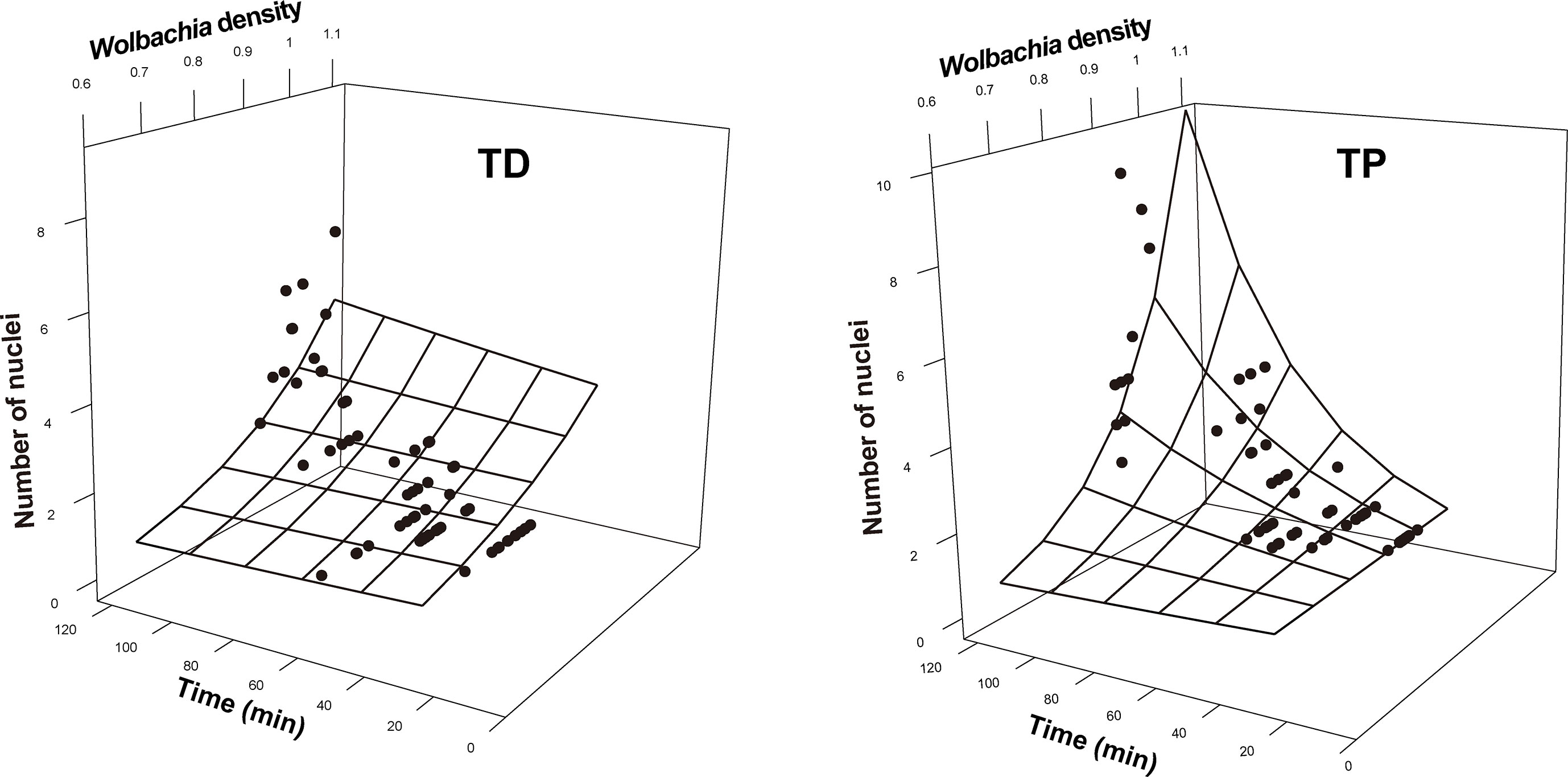
Figure 4 Number of nuclei as a function of developmental time and Wolbachia density in the early embryonic stages of T. dendrolimi (TD) and T. pretiosum (TP). The surface was estimated by log-linear models.
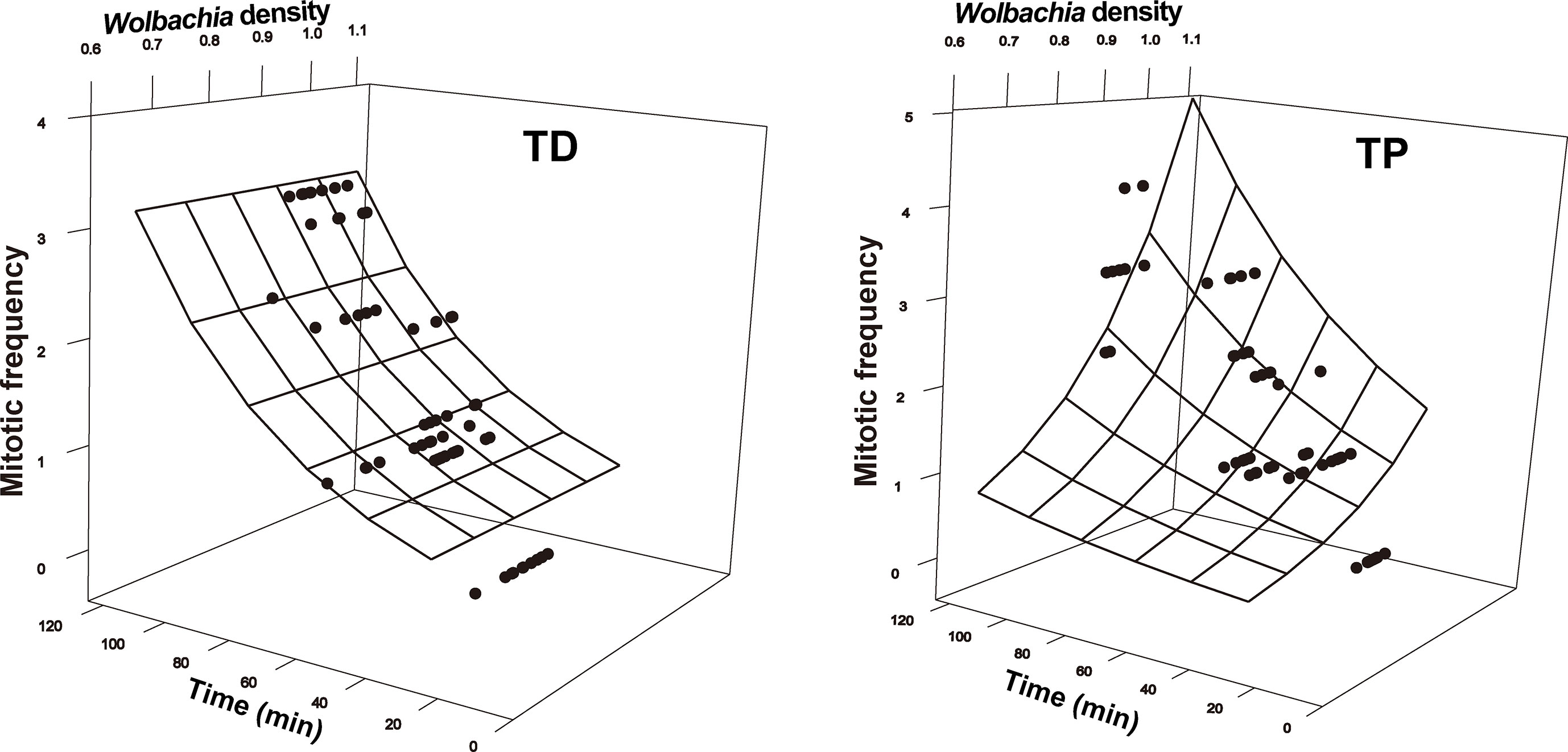
Figure 5 Mitotic frequency as a function of developmental time and Wolbachia density in the early embryonic stages of T. dendrolimi (TD) and T. pretiosum (TP). The surface was estimated by log-linear models.
The SEM for the T. pretiosum embryos was not rejected after two paths were excluded (χ2 = 1.35, df = 2, p = 0.50, CFI = 1.00, SRMR < 0.001). Early embryonic developmental time had no apparent effect on the Wolbachia density or the SR. The number of nuclei and the mitotic frequency increased with early embryonic development. Both the number of nuclei and the mitotic frequency increased with the Wolbachia density. The number of nuclei was positively correlated with the mitotic frequency. The correlation between the Wolbachia density and the SR was not significant (Figures 3–5).
In both T. dendrolimi and T. pretiosum embryos, we observed a higher number of Wolbachia signals at the posterior part compared to the anterior part. The number of nuclei and the mitotic frequency increased with the developmental time of the early embryo, irrespective of Trichogramma species. There was a positive correlation between Wolbachia density and the number of nuclei in both T. dendrolimi and T. pretiosum embryos. Additionally, the Wolbachia density, SR, and mitotic frequency increased with the developmental time of T. dendrolimi embryos, whereas this was not observed in T. pretiosum embryos.
Wolbachia dynamics at different Trichogramma developmental stages
The average SR values for the T. dendrolimi (0.19–0.89) and the T. pretiosum (0.46–0.84) offspring were < 1 at all stages (Figures 6, 7). The average SR value for the T. dendrolimi offspring was highest at 168 h (0.89 ± 0.19) and significantly higher than those at 24 h (0.31 ± 0.042; z = 2.14, p = 0.032), 48 h (0.36 ± 0.091; z = 2.12, p = 0.033), 72 h (0.34 ± 0.079; z = 2.15, p = 0.031), and 120 h (0.19 ± 0.043; z = 3.18, p = 0.0015), but not significantly higher than that at 240 h (0.73 ± 0.15; z = 2.17, p = 0.86). For the T. dendrolimi offspring, the SR at 24, 48, 72, and 120 h did not significantly differ. For the T. pretiosum offspring, the average SR value was highest at 120 h (0.84 ± 0.16) and significantly higher than that at 24 h (0.46 ± 0.065; z = 2.45, p = 0.014). However, the SR at 48 h (0.58 ± 0.082), 72 h (0.67 ± 0.088), 120 h, 168 h (0.52 ± 0.043), and 240 h (0.48 ± 0.031) did not significantly differ (Figure 7). For the T. dendrolimi offspring, the average Wolbachia density was highest at 24 h (0.86 ± 0.016) and significantly higher than those at 48 h (0.69 ± 0.013; z = 3.82, p < 0.001), 72 h (0.73 ± 0.015; z = 2.62, p = 0.0087), 120 h (0.72 ± 0.012; z = 2.90, p = 0.0037), 168 h (0.61 ± 0.026; z = 4.65, p < 0.001), and 240 h (0.56 ± 0.016; z = 5.15, p < 0.001). The average Wolbachia density of the T. dendrolimi offspring was lowest at 240 h, significantly lower than those at 24 h, 48 h (z = 2.03, p = 0.042), 72 h (z = 2.50, p = 0.012), and 120 h (z = 2.28, p = 0.023), and not significantly lower than that at 168 h (z = 0.43, p = 0.66). The average Wolbachia density of the T. pretiosum offspring was highest at 24 h (0.86 ± 0.0062) and significantly higher than those at 48 h (0.77 ± 0.015; z = 3.62, p < 0.001), 72 h (0.79 ± 0.0076; z = 2.88, p = 0.0039), 120 h (0.76 ± 0.012; z = 3.93, p < 0.001), 168 h (0.77 ± 0.0035; z = 3.18, p = 0.0015), and 240 h (0.73 ± 0.020; z = 4.46, p < 0.001) (Figure 8).
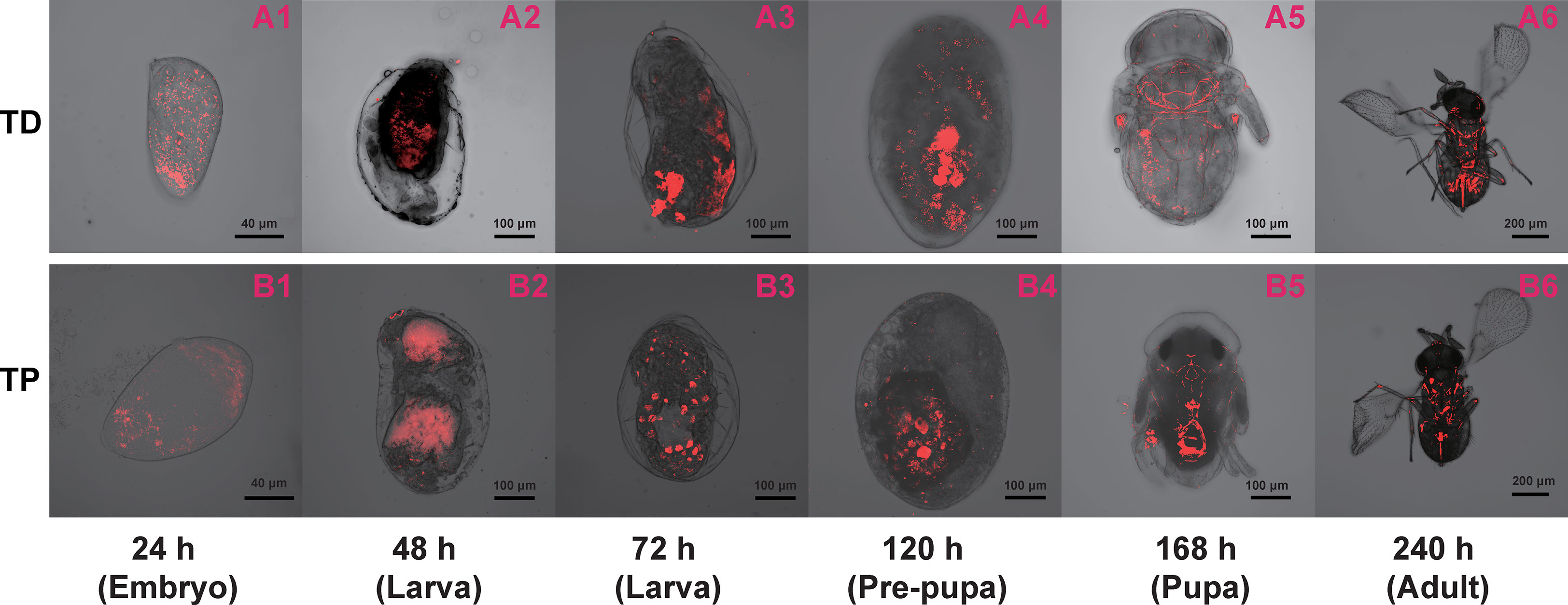
Figure 6 Immature T. dendrolimi (TD) and T. pretiosum (TP) offspring stained with Wolbachia 16S rRNA probes. A1, A2, A3, A4, A5, and A6 are T. dendrolimi offspring at 24, 48, 72, 120, 168, and 240 h, respectively. B1, B2, B3, B4, B5, and B6 are T. pretiosum offspring at 24, 48, 72, 120, 168, and 240 h, respectively.
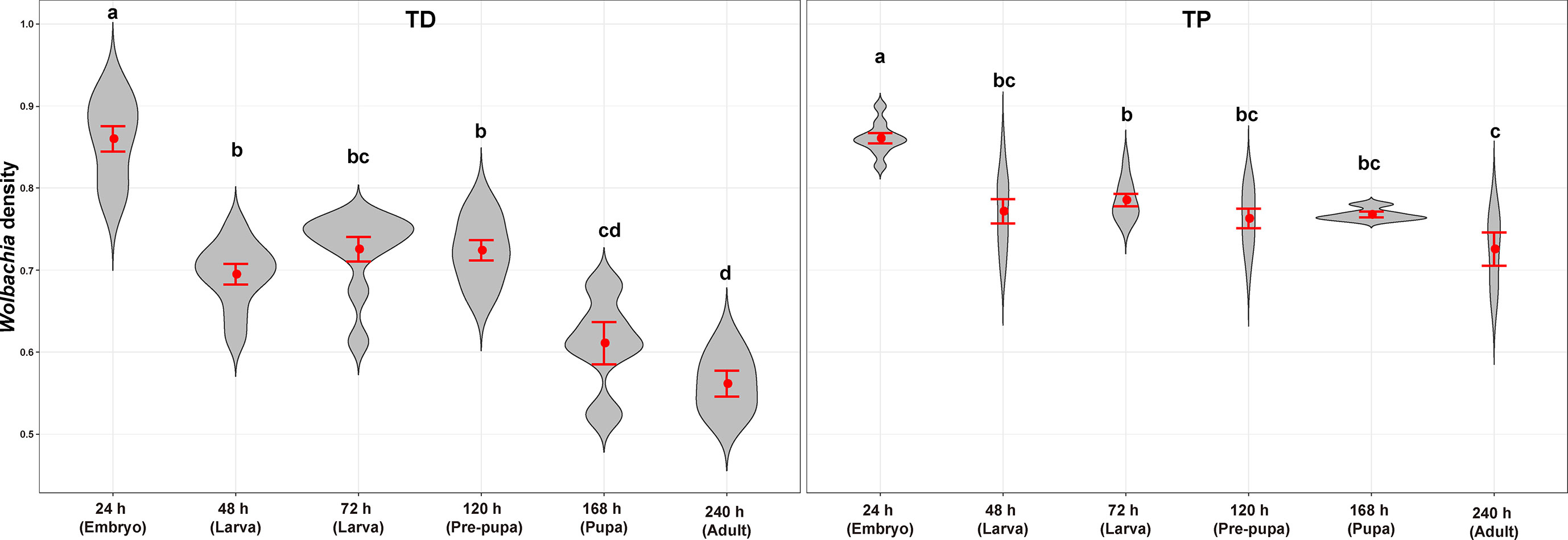
Figure 7 Symmetric ratios of the Wolbachia distribution of T. dendrolimi (TD) and T. pretiosum (TP) at different developmental stages. Red points indicate means. Error bars indicate 95% confidential intervals (CI). The red violin area indicates data distribution.
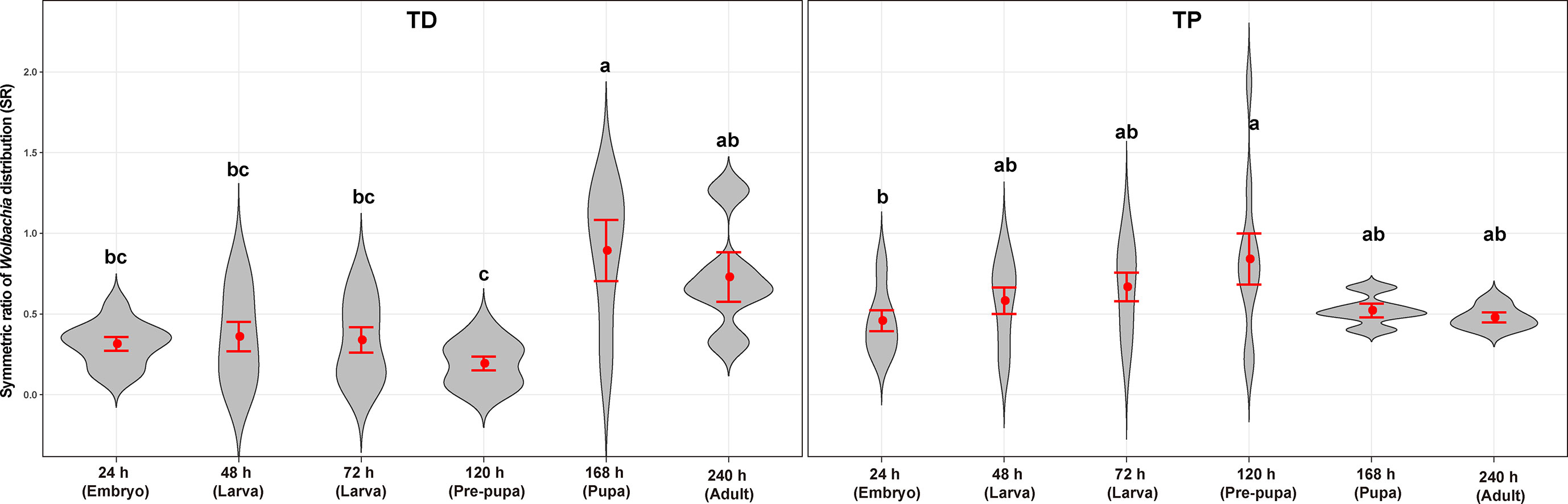
Figure 8 Wolbachia density of T. dendrolimi (TD) and T. pretiosum (TP) at different developmental stages. Red points indicate means. Error bars indicate 95% CI. The red violin area indicates data distribution.
The Wolbachia titers in the T. dendrolimi and T. pretiosum offspring were evaluated by AQ-PCR. The average Wolbachia titer in the T. dendrolimi offspring was lowest at 48 h (5.05 ± 0.10), significantly lower than those at 120 h (5.90 ± 0.014; z = 3.07, p = 0.0035), 168 h (6.40 ± 0.012; z = 4.64, p < 0.001), and 240 h (6.51 ± 0.018; z = 6.10, p < 0.001), and not significantly lower than that at 72 h (5.55 ± 0.025; z = 1.53, p = 0.13). The Wolbachia titers in the T. dendrolimi offspring at 168 and 240 h were significantly higher than those at 24 and 72 h (z = 3.11, p = 0.0019; z = 4.56, p < 0.001) and 120 h (z = 2.10, p = 0.035; z = 3.03, p = 0.0024). The average Wolbachia titers in the T. pretiosum offspring were lowest at 48 h (5.17 ± 0.099), significantly lower than those at 120 h (5.90 ± 0.010; z = 2.98, p = 0.0029), 168 h (6.04 ± 0.0079; z = 4.67, p < 0.001), and 240 h (6.19 ± 0.019; z = 5.97, p < 0.001), and not significantly lower than that at 72 h (5.59 ± 0.031; z = 1.35, p = 0.18). The average Wolbachia titers were highest in the T. pretiosum offspring at 240 h, significantly higher than those at 48 and 72 h (z = 4.63, p < 0.001) and 120 h (z = 3.00, p = 0.0027), and not significantly lower than that at 168 h (z = 1.40, p = 0.16) (Figure 9).
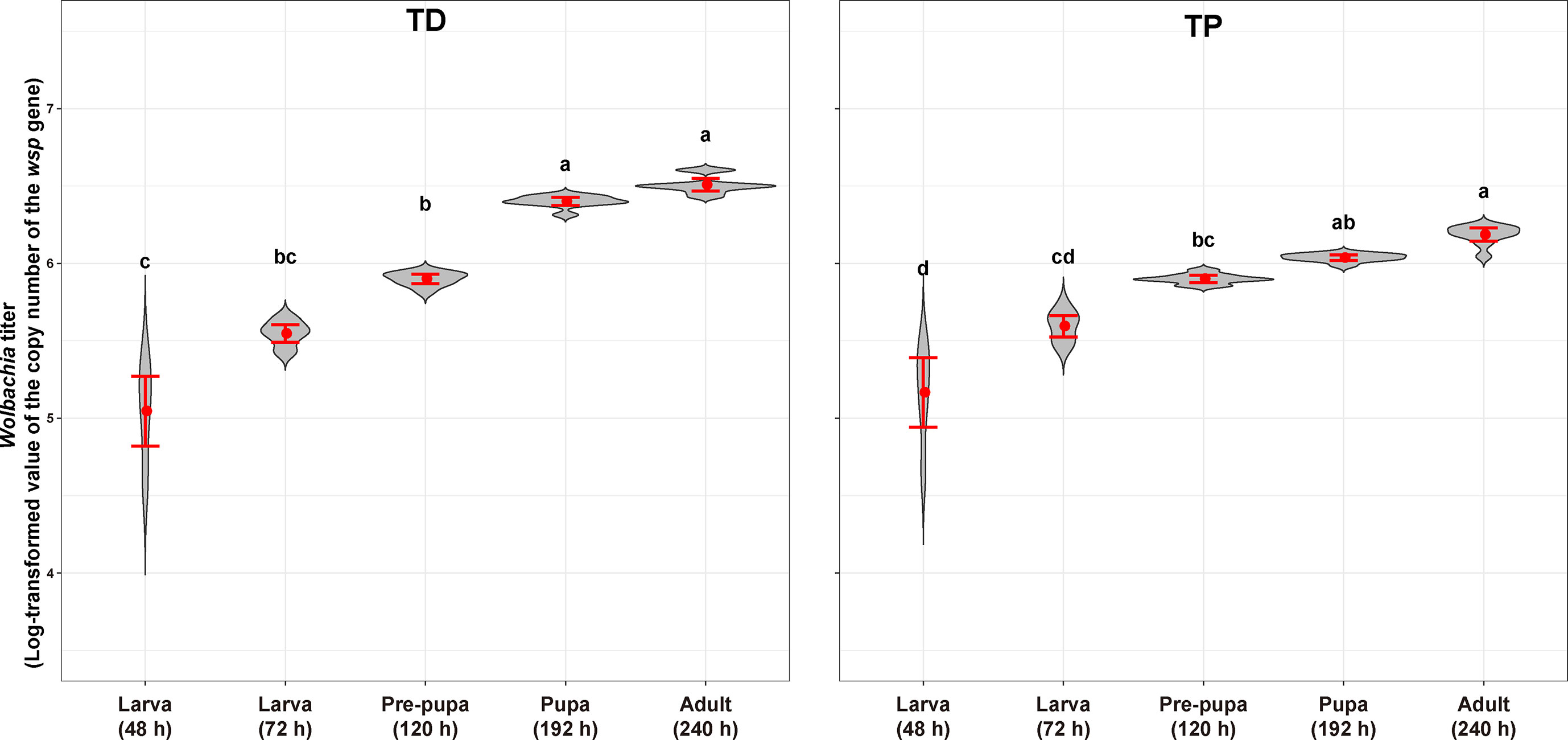
Figure 9 Wolbachia titer of T. dendrolimi (TD) and T. pretiosum (TP) at different developmental stages. Red points indicate means. Error bars indicate 95% CI. The red violin area indicates data distribution.
The Wolbachia density and the SR were significantly influenced by the developmental stage in both T. dendrolimi and T. pretiosum. We observed a higher number of Wolbachia signals in the posterior part of each embryo compared to the anterior part. Regardless of Trichogramma species, Wolbachia density decreased with developmental time, while Wolbachia titers increased with developmental time.
Discussion
Wolbachia presented efficient vertical transmission across host generations, as evidenced by the nearly 100% female offspring deposited by thelytokous Trichogramma females, all of which were infected by Wolbachia. We found that Wolbachia were concentrated in the posterior part of each embryo throughout the early stages of T. dendrolimi and T. pretiosum embryogenesis. Though the SR values varied with the Trichogramma wasp stage, the Wolbachia were nonetheless more concentrated in the posterior than the anterior regions of each embryo. These results provide evidence that the posterior concentration of Wolbachia during embryogenesis dynamically shapes tissue tropism during the postembryonic stages in two Trichogramma species, T. dendrolimi and T. pretiosum.
The distribution of Wolbachia in early embryos has been investigated in Nasonia and Trichogramma wasps, both parasitic wasps. As mentioned in the introduction, different patterns of Wolbachia distribution have been observed in these studies. Though Wolbachia exhibit posterior concentration in both T. dendrolimi and T. pretiosum embryos. The Wolbachia density, SR, and mitotic frequency increased with the developmental time of T. dendrolimi embryos, whereas this was not observed in T. pretiosum embryos. Similar to our findings, the Wolbachia wVitA strain in N. giraulti embryos localizes to the posterior end at a high density and then spreads toward the anterior end. Conversely, in N. vitripennis embryos, the Wolbachia wVitA strain localizes to the posterior end at a low density (Chafee et al., 2011; Funkhouser-Jones et al., 2018). In Trichogramma wasps, the posterior concentration of Wolbachia was also reported in T. cordubensis embryos, but not in T. dendrolimi (Pintureau et al., 2000). It is noteworthy that previous studies involving Nasonia or Trichogramma wasps have only described Wolbachia distribution at a single time point during embryogenesis, with one or several replicates. The process of Wolbachia tropism in postembryonic stages has not been described in parasitic wasps. The concentration of Wolbachia at the posterior pole during early embryogenesis is crucial for ensuring efficient vertical transmission. Notably, the distribution of embryonic Wolbachia varies widely among host arthropod species, including parasitoid species. The differences in Wolbachia localization patterns among insect host systems can be attributed to two factors. First, different Wolbachia strains may exhibit distribution patterns. For example, the tissue-specific distributions of Wolbachia were determined by its asymmetric localization and segregation during the initial mitotic divisions in early Drosophila embryos (Albertson et al., 2009). The Wolbachia wPau and wWil strains concentrated in the primordial germ cells of D. paulistorum and D. willistoni embryos, shaping the concentration of Wolbachia in the germ line. However, the broad distribution of Wolbachia wMel and wWil strains in D. melanogaster and D. simulans embryos results in systemic infections in different tissues of these species (Strunov et al., 2022). Second, the localization patterns of Wolbachia may vary among the embryos of different host species. For example, Wolbachia were concentrated in the anterior part of the embryos of the mosquito Aedes polynesiensis (Wright and Barr, 1981; Boyle et al., 1993) and the small brown planthopper Laodelphax striatellus (Guo et al., 2019), whereas a posterior Wolbachia concentration has been reported in embryos of the aphelinid Aphytis (Zchori-Fein et al., 1998). As mentioned earlier, the Wolbachia wVitA strain localizes to the posterior end at low density in the N. vitripennis embryo, but is concentrated in the posterior end at high density and then spread toward the anterior end in the N. giraulti wasp embryo (Chafee et al., 2011; Funkhouser-Jones et al., 2018). The foregoing results suggest that the Wolbachia localization patterns in the host embryo vary with the host background and Wolbachia strain. Phylogenetic studies have shown that 75% of Wolbachia strains from Trichogramma species belong to the Sib group clade in supergroup B (Almeida and Stouthamer, 2018). Our previous study confirmed that the Wolbachia strains from T. dendrolimi and T. pretiosum belong to the Sib group. Therefore, the present results suggest that the posterior concentration of Wolbachia during embryogenesis may be common in Trichogramma wasps infected by Wolbachia strains in the Sib group.
We found that Wolbachia density increased with the number of nuclei and the frequency of the initial mitotic divisions during early embryogenesis. According to the AQ-PCR, the total Wolbachia titer increased with T. dendrolimi and T. pretiosum offspring development. Relative to body size, however, Wolbachia density was significantly lower at the adult and pupal stages than in the embryo of T. dendrolimi and T. pretiosum. Similarly, the Wolbachia titers were high in the early embryos of Drosophila sturtevanti, D. paulistorum, and D. willistoni but low in late embryogenesis. For D. septentriosaltans and D. simulans, however, the Wolbachia titers were high during embryogenesis but relatively lower at the later developmental stages (Strunov et al., 2022). The observed reduction in Wolbachia density at the later stages of Trichogramma development may be explained by two factors. First, Wolbachia density may decrease later in host development because of the dilution effect of multiple cell divisions (Strunov et al., 2022). Second, Wolbachia activates host immune responses that could modify the distribution of the endosymbionts during the later developmental stages of the host. For example, the restricted Wolbachia infection pattern observed in the somatic tissues of Drosophila spp. is determined by selective autophagy involving interactions between Wolbachia and the endoplasmic reticulum (ER). ER stress could activate cell defense and immune responses (Celli & Tsolis, 2015; Strunov et al., 2022). Our study describes the dynamic pattern of Wolbachia distribution throughout the immature stages of Trichogramma hosts and serves as a fundamental reference for investigating the mechanisms underlying Wolbachia density and distribution dynamics. Thelytokous Trichogramma populations have the advantage of easier colonization in fields without the need for mating with males and potentially exhibit higher reproductive rates than their uninfected counterparts since all offspring are female (Zhou et al., 2019). The PI strength in Trichogramma wasps is strongly influenced by Wolbachia density in the germ line and eggs. Previous studies showed that weak PI strength is often associated with biotic (e.g., continuous oviposition) or abiotic (e.g., high temperature) factors that can affect the transmission rate of Wolbachia (Zhou et al., 2022a; Guo et al., 2023). The unstable PI strength of Wolbachia-infected Trichogramma can impact the quality of Trichogramma offspring and their efficacy in pest control. Our findings have practical implications for the future application of Wolbachia-infected Trichogramma in biological control programs.
Though Wolbachia were concentrated at the posterior part of the embryo throughout early embryogenesis, the SR values were significantly higher at the pupal stage of T. dendrolimi and the prepupal stage of T. pretiosum than at the embryonic stage. We detected Wolbachia signals in the anterior part of the embryo, the 48- and 72-h larvae, and the pre-pupae of T. dendrolimi and T. pretiosum. However, no Wolbachia signals were detected in the heads of pupal or adult T. dendrolimi and T. pretiosum. Our previous study revealed that Wolbachia infection caused memory loss, increased the incidence of superparasitism, and attenuated the host discrimination mechanism in T. dendrolimi (Zhou et al., 2022a). Though the Wolbachia titers in the heads of Trichogramma adults were low, the endosymbiont nonetheless caused behavioral changes in the wasps. The results of the present work suggest that Wolbachia colonization in the nervous tissues of immature Trichogramma progeny may impair central nervous system development and function in this insect host. However, the precise mechanisms by which Wolbachia infection induces behavioral changes in Trichogramma wasps remain to be elucidated.
The present study showed that posterior Wolbachia concentration during early Trichogramma embryogenesis dynamically shapes Wolbachia localization in this host wasp. In this way, Wolbachia can undergo efficient vertical transmission across generations and ensures that thelytokous Trichogramma females deposit only female Wolbachia-infected offspring. The present work clarified the localization pattern of Wolbachia during the embryogenesis and various developmental stages of Trichogramma spp. These findings may help understand the interactions between Wolbachia and other host insects and provide a fundamental reference for the application of Wolbachia-infected thelytokous Trichogramma.
Data availability statement
The original contributions presented in the study are included in the article. Further inquiries can be directed to the corresponding authors.
Author contributions
This study was designed by J-CZ, W-NC, HD, and L-SZ. Q-JD, DS, S-FN, and YW carried out the experiments. J-CZ, Q-JD, and DS also help to analyze the phenotype as well as the data. J-CZ, W-NC, and H-HZ also participated in the data analysis. J-CZ wrote the paper. All authors contributed to the article and approved the submitted version.
Funding
This research was funded by the Projects of the National Natural Science Foundation of China (32172476, 32102275, 31972339), the Agricultural Science and Technology Innovation Program (CAAS-ZDRW202203, CAAS-ZDRW202108), Central Funds Guiding the Local Science and Technology Development (XZ202301YD0042C), and Fundamental Research Funds for the Universities of Liaoning Province (LJKZ0646).
Acknowledgments
We would like to thank EditChecks (https://editchecks.com.cn/) for providing linguistic assistance during the preparation of this manuscript.
Conflict of interest
The authors declare that the research was conducted in the absence of any commercial or financial relationships that could be construed as a potential conflict of interest.
Publisher’s note
All claims expressed in this article are solely those of the authors and do not necessarily represent those of their affiliated organizations, or those of the publisher, the editors and the reviewers. Any product that may be evaluated in this article, or claim that may be made by its manufacturer, is not guaranteed or endorsed by the publisher.
References
Abrams, P. A. (2007). Defining and measuring the impact of dynamic traits on interspecific interactions. Ecology 88, 2555–2562. doi: 10.1890/06-1381.1
Albertson, R., Casper-Lindley, C., Cao, J., Tram, U., Sullivan, W. (2009). Symmetric and asymmetric mitotic segregation patterns influence Wolbachia distribution in host somatic tissue. J. Cell Sci. 122, 4570–4583. doi: 10.1242/jcs.054981
Almeida, R. P., Stouthamer, R. (2018). Phylogeny of the Trichogramma endosymbiont Wolbachia, an alpha-proteobacteria (Rickettsiae). Braz. J. Biol. 78, 421–428. doi: 10.1590/1519-6984.166671
Boyle, L., O'Neill, S. L., Robertson, H. M., Karr, T. L. (1993). Interspecific and intraspecific horizontal transfer of Wolbachia in Drosophila. Science 260, 1796–1799. doi: 10.1126/science.8511587
Breeuwer, J. A., Werren, J. H. (1990). Microorganisms associated with chromosome destruction and reproductive isolation between two insect species. Nature 346, 558–560. doi: 10.1038/346558a0
Celli, J., Tsolis, R. M. (2015). Bacteria, the endoplasmic reticulum and the unfolded protein response: friends or foes? Nat. Rev. Microbiol. 13, 71–82. doi: 10.1038/nrmicro3393
Chafee, M. E., Zecher, C. N., Gourley, M. L., Schmidt, V. T., Chen, J. H., Bordenstein, S. R., et al. (2011). Decoupling of host-symbiont-phage coadaptations following transfer between insect species. Genetics 187, 203. doi: 10.1534/genetics.110.120675
Funkhouser-Jones, L. J., Van Opstal, E. J., Sharma, A., Bordenstein, S. R. (2018). The maternal effect gene wds controls Wolbachia titer in Nasonia. Curr. Biol. 28, 1692–1702. doi: 10.1016/j.cub.2018.04.010
Grace, J. B. (2007). Structural equation modeling for observational studies. J. Wildlife Manage. 72, 14–22. doi: 10.2193/2007-307
Guo, Y., Gong, J. T., Mo, P. W., Huang, H. J., Hong, X. Y. (2019). Wolbachia localization during Laodelphax striatellus embryogenesis. J. Insect Physiol. 116, 125–133. doi: 10.1016/j.jinsphys.2019.05.006
Guo, W., Zhang, M., Lin, L., Zeng, C., Zhang, Y., He, X. (2023). Bacterial community survey of Wolbachia-infected parthenogenetic parasitoid Trichogramma pretiosum (Hymenoptera: trichogrammatidae) treated with antibiotics and high temperature. Int. J. Mol. Sci. 24, 8448. doi: 10.3390/ijms24098448
Huigens, M. E., Luck, R. F., Klaassen, R. H., Maas, M. F., Timmermans, M. J., Stouthamer, R. (2000). Infectious parthenogenesis. Nature 405, 178–179. doi: 10.1038/35012066
Kaur, R., Shropshire, J. D., Cross, K. L., Leigh, B., Mansueto, A. J., Stewart, V. (2021). Living in the endosymbiotic world of Wolbachia: a centennial review. Cell Host Microbe 29, 879–893. doi: 10.1016/j.chom.2021.03.006
Landmann, F., Bain, O., Martin, C., Uni, S., Taylor, M. J., Sullivan, W. (2012). Both asymmetric mitotic segregation and cell-to-cell invasion are required for stable germline transmission of Wolbachia in filarial nematodes. Biol. Open 1, 536–547. doi: 10.1242/bio.2012737
Landmann, F., Foster, J. M., Slatko, B., Sullivan, W. (2010). Asymmetric Wolbachia segregation during early Brugia malayi embryogenesis determines its distribution in adult host tissues. PloS Negl. Trop. Dis. 4, e758. doi: 10.1371/journal.pntd.0000758
LePage, D. P., Metcalf, J. A., Bordenstein, S. R., On, J., Perlmutter, J. I., Shropshire, J. D., et al. (2017). Prophage WO genes recapitulate and enhance Wolbachia-induced cytoplasmic incompatibility. Nature 543, 243–247. doi: 10.1038/nature21391
Lindsey, A. R. I., Kelkar, Y. D., Wu, X., Sun, D., Martinson, E. O., Yan, Z., et al. (2018). Comparative genomics of the miniature wasp and pest control agent Trichogramma pretiosum. BMC Biol. 16, 54. doi: 10.1186/s12915-018-0520-9
Ma, W. J., Schwander, T. (2017). Patterns and mechanisms in instances of endosymbiont-induced parthenogenesis. J. Evol. Biol. 30 (5), 868–888. doi: 10.1111/jeb.13069
Masson, F., Zaidman-Remy, A., Heddi, A. (2016). Antimicrobial peptides and cell processes tracking endosymbiont dynamics. Philos. Trans. R. Soc B. Biol. Sci. 371, 20150298. doi: 10.1098/rstb.2015.0298
Newton, I. L., Savytskyy, O., Sheehan, K. B. (2015). Wolbachia utilize host actin for efficient maternal transmission in Drosophila melanogaster. PloS Pathog. 11, e1004798. doi: 10.1371/journal.ppat.1004798
Pietri, J. E., DeBruhl, H., Sullivan, W. (2016). The rich somatic life of Wolbachia. Microbiologyopen 5, 923–936. doi: 10.1002/mbo3.390
Pintureau, B., Grenier, S., Boléat, B., Lassablière, F., Heddi, A., Khatchadourian, C. (2000). Dynamics of Wolbachia populations in transfected lines of Trichogramma. J. Invertebr. Pathol. 76, 20–25. doi: 10.1006/jipa.2000.4953
R Development Core Team (2021). R: a language and environment for statistical computing (Vienna, Austria: R Foundation for Statistical Computing). Available at: https://www.R-project.org/.
Salje, J. (2021). Cells within cells: rickettsiales and the obligate intracellular bacterial lifestyle. Nat. Rev. Microbiol. 19, 375–390. doi: 10.1038/s41579-020-00507-2
Serbus, L. R., Casper-Lindley, C., Landmann, F., Sullivan, W. (2008). The genetics and cell biology of Wolbachia-host interactions. Annu. Rev. Genet. 42, 683–707. doi: 10.1146/annurev.genet.41.110306.130354
Strunov, A., Schmidt, K., Kapun, M., Miller, W. J. (2022). Restriction of Wolbachia bacteria in early embryogenesis of Neotropical Drosophila species via endoplasmic reticulum-mediated autophagy. mBio 13, e0386321. doi: 10.1128/mbio.03863-21
Wright, J. D., Barr, A. R. (1981). Wolbachia and the normal and incompatible eggs of Aedes polynesiensis (Diptera: culicidae). J. Invertebr. Pathol. 38, 409–418. doi: 10.1016/0022-2011(81)90109-9
Wu, X., Lindsey, A. R. I., Chatterjee, P., Werren, J. H., Stouthamer, R., Yi, S. V. (2020). Distinct epigenomic and transcriptomic modifications associated with Wolbachia-mediated asexuality. PloS Pathog. 16, e1008397. doi: 10.1371/journal.ppat.1008397
Zchori-Fein, E., Roush, R. T., Rosen, D. (1998). Distribution of parthenogenesis-inducing symbionts in ovaries and eggs of Aphytis (Hymenoptera: aphelinidae). Curr. Microbiol. 36, 1–8. doi: 10.1007/s002849900270
Zhou, J. C., Li, Y. Y., Liu, Q. Q., Ning, S. F., Che, W. N., Dong, H. (2019). Effects of temperature and superparasitism on quality and characteristics of thelytokous Wolbachia-infected Trichogramma dendrolimi matsumura (Hymenoptera: trichogrammatidae) during mass rearing. Sci. Rep. 9, 18114. doi: 10.1038/s41598-019-54719-5
Zhou, J. C., Liu, Q. Q., Wang, Q. R., Ning, S. F., Che, W. N., Dong, H. (2020). Optimal clutch size for quality control of bisexual and Wolbachia-infected thelytokous lines of Trichogramma dendrolimi matsumura (Hymenoptera: trichogrammatidae) mass reared on eggs of a substitutive host, Antheraea pernyi guerin-meneville (Lepidoptera: saturniidae). Pest Manage. Sci. 76, 2635–2644. doi: 10.1002/ps.5805
Zhou, J. C., Shang, D., Liu, S. M., Zhang, C., Huo, L. X., Zhang, L. S., et al. (2023). Wolbachia-infected Trichogramma dendrolimi is outcompeted by its uninfected counterpart in superparasitism but does not have developmental delay. Pest Manage. Sci. 79, 1005–1017. doi: 10.1002/ps.7269
Zhou, J. C., Shang, D., Qian, Q., Zhang, C., Zhang, L. S., Dong, H. (2022a). Penetrance during Wolbachia-mediated parthenogenesis of Trichogramma wasps is reduced by continuous oviposition, associated with exhaustion of Wolbachia titers in ovary and offspring eggs. Pest Manage. Sci. 78, 3080–3089. doi: 10.1002/ps.6934
Keywords: endosymbiont, thelytokous parthenogenesis, vertical transmission, biological control, Trichogramma dendrolimi, Trichogramma pretiosum, egg parasitoid
Citation: Zhou J-C, Dong Q-J, Shang D, Ning S-F, Zhang H-H, Wang Y, Che W-N, Dong H and Zhang L-S (2023) Posterior concentration of Wolbachia during the early embryogenesis of the host dynamically shapes the tissue tropism of Wolbachia in host Trichogramma wasps. Front. Cell. Infect. Microbiol. 13:1198428. doi: 10.3389/fcimb.2023.1198428
Received: 01 April 2023; Accepted: 06 June 2023;
Published: 22 June 2023.
Edited by:
Iliano V. Coutinho-Abreu, University of California, San Diego, United StatesReviewed by:
Fabio Gomes, Federal University of Rio de Janeiro, BrazilGuan-Hong Wang, Chinese Academy of Sciences (CAS), China
Copyright © 2023 Zhou, Dong, Shang, Ning, Zhang, Wang, Che, Dong and Zhang. This is an open-access article distributed under the terms of the Creative Commons Attribution License (CC BY). The use, distribution or reproduction in other forums is permitted, provided the original author(s) and the copyright owner(s) are credited and that the original publication in this journal is cited, in accordance with accepted academic practice. No use, distribution or reproduction is permitted which does not comply with these terms.
*Correspondence: Wu-Nan Che, Y2hld3VuYW5Ac3lhdS5lZHUuY24=; Hui Dong, YmlvY29udHJvbEAxNjMuY29t; Li-Sheng Zhang, emhhbmdsZWVzaGVuZ0AxNjMuY29t
†These authors have contributed equally to this work and share first authorship