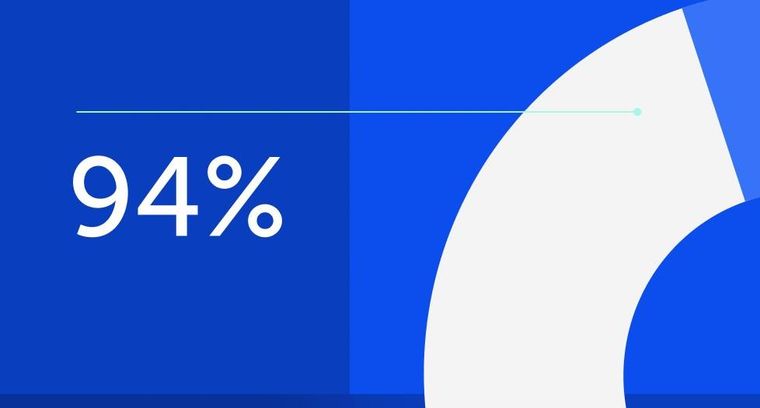
94% of researchers rate our articles as excellent or good
Learn more about the work of our research integrity team to safeguard the quality of each article we publish.
Find out more
REVIEW article
Front. Cell. Infect. Microbiol., 12 May 2023
Sec. Extra-intestinal Microbiome
Volume 13 - 2023 | https://doi.org/10.3389/fcimb.2023.1178399
Obesity is a complex metabolic disease, with cognitive impairment being an essential complication. Gut microbiota differs markedly between individuals with and without obesity. The microbial–gut–brain axis is an important pathway through which metabolic factors, such as obesity, affect the brain. Probiotics have been shown to alleviate symptoms associated with obesity and neurobehavioral disorders. In this review, we evaluated previously published studies on the effectiveness of probiotic interventions in reducing cognitive impairment, depression, and anxiety associated with obesity or a high-fat diet. Most of the probiotics studied have beneficial health effects on obesity-induced cognitive impairment and anxiety. They positively affect immune regulation, the hypothalamic–pituitary–adrenal axis, hippocampal function, intestinal mucosa protection, and glucolipid metabolism regulation. Probiotics can influence changes in the composition of the gut microbiota and the ratio between various flora. However, probiotics should be used with caution, particularly in healthy individuals. Future research should further explore the mechanisms underlying the gut–brain axis, obesity, and cognitive function while overcoming the significant variation in study design and high risk of bias in the current evidence.
Obesity has become a global epidemic, especially in the last few decades, and its prevalence is increasing at an alarming rate. Nearly 2 billion adults worldwide are considered to have overweight, with more than half of them having obesity (Ridaura et al., 2013; Hoffman et al., 2021). The impact of obesity on the quality of life is significant, as evidenced by its wide range of effects on metabolic and cognitive function (Sellbom and Gunstad, 2012; Monda et al., 2017; Agustí et al., 2018). A sizeable human cohort study reported a linear association between obesity and cognitive impairment (Farruggia and Small, 2019), which not only causes brain damage but also accelerates aging of the brain (Mattson and Arumugam, 2018). Thus, individuals with obesity may be at an increased risk of developing neurodegenerative diseases (O'Brien et al., 2017). In addition, differences in dietary habits have also been associated with cognitive performance and age-related cognitive decline (Otaegui-Arrazola et al., 2014; Proctor et al., 2017). For example, multiple studies have reported that people with obesity and animal models with diet-induced obesity show impaired learning and memory abilities (Bocarsly et al., 2015; Cordner and Tamashiro, 2015; Almeida-Suhett et al., 2017; Fazzari et al., 2018).
Moreover, individuals with obesity and animal models with diet-induced obesity are prone to excessive anxiety and depression-like behavior (Le Port et al., 2012; Foster and McVey Neufeld, 2013; de Noronha et al., 2017; Alonso-Caraballo et al., 2019). Obesity is frequently associated with cognitive impairment. Interactions between the gut flora and the microbial–gut–brain axis play an important role in the association observed between obesity and cognitive impairment (Figure 1).
Figure 1 A summary of some mechanisms based on the microbial-gut-brain axis for the harm caused by obesity.
Changes in the intestinal flora are closely linked to obesity. Gut microbiota composition in people with obesity differs from that in people without obesity; as such, people with obesity have gut dysbiosis (Aggarwal et al., 2013; Abenavoli et al., 2019). Dietary factors are the primary cause of obesity. Nutritional changes affect intestinal flora and cause intestinal ecological disorders to some extent. Western or high-fat diets (HFD) can cause ecological intestinal diseases (Turnbaugh et al., 2008; Hildebrandt et al., 2009; Li et al., 2009). The exact mechanisms linking intestinal ecological dysregulation and obesity are not yet fully understood. However, several possible pathways have been identified. Gut dysbiosis promotes weight gain and obesity by inducing an inflammatory response, reducing fat and cholesterol metabolism, and decreasing insulin sensitivity (Baothman et al., 2016; Rogers et al., 2016; Bliss and Whiteside, 2018). Intervention at the microbiological level using probiotics for gut ecological dysbiosis can change the composition of the gut flora (Ji et al., 2012) and thus reduce obesity-related symptoms, such as fat and cholesterol levels as well as weight gain (Ji et al., 2012; Park et al., 2013).
Additionally, gut flora affects the cognitive behavior of organisms via the microbial–gut–brain axis. This influence of the gut flora on the gut–brain relationship results in various mood or cognitive disorders such as anxiety, depression, and disturbances in learning and memory (Noble et al., 2017; Cryan et al., 2019). Previous studies have found that gut flora can influence cognitive behavior by affecting insulin sensitivity and inflammatory pathways; gut flora also affects obesity in the same ways (Pistell et al., 2010). This overlap could partly explain the higher susceptibility of people with obesity to cognitive deficits, anxiety, and depressive behaviors as compared to healthy people. Transplantation of gut microbiota of individuals with obesity into healthy individuals can also cause neurobehavioral changes (Bruce-Keller et al., 2015). This strongly suggests that early dietary modification and intervention centered on the microbial–gut–brain axis are noteworthy non-pharmacological therapies that may benefit both obesity and cognitive behavior.
Several metabolites produced by intestinal flora during dietary metabolism, such as trimethylamine (Vogt et al., 2018), short-chain fatty acids (Qian et al., 2021), and bile acids (MahmoudianDehkordi et al., 2019), are associated with neurodegenerative diseases. Several new therapeutic modalities, including prebiotics and probiotics, may normalize gut microbiota composition, modulate the gut–brain barrier, suppress neuroinflammation, and reduce the risk of developing neurodegenerative diseases (Green et al., 2020). As microbiota can have a wide range of effects on metabolic and cognitive behavior, probiotic interventions at the gut microbiota level are a promising approach to preventing or treating obesity-related cognitive deficits, anxiety, and depression.
The beneficial effects of probiotic interventions in obesity and metabolic diseases have been extensively reviewed (Million et al., 2012; Kobyliak et al., 2016; Azad et al., 2018). At the same time, probiotics can also improve cognitive impairment and some neurobehavioral disorders, such as anxiety, major depression, and Alzheimer’s disease (AD), all closely related to gut ecological disorders (Luna and Foster, 2015; Leblhuber et al., 2021). In previous animal and clinical studies, interventions with gut microbes using a single or a combination of probiotic strains exerted a positive effect on cognitive impairment and neurobehavioral disorders (Ansari et al., 2020). These benefits were demonstrated by an adequate reduction in cognitive impairment, depression, and anxiety in some patients after probiotic treatment, accompanied by improved communication and social skills (Zhu et al., 2021). In addition, probiotic treatment has also shown definite improvements in various disease parameters, such as specific biomarkers for diseases including amyloid beta in AD, gastrointestinal symptoms often seen in patients with major depression, and hypothalamic–pituitary–adrenal (HPA)-related stress responses in patients with anxiety states (Slyepchenko et al., 2014; Pirbaglou et al., 2016; Ng et al., 2019; Kesika et al., 2021).
Despite the growing body of scientific evidence on probiotic interventions in the microbial–gut–brain axis to promote weight loss and prevent cognitive dysfunction, a review of the effectiveness of probiotics in treating obesity-related neurobehavioral symptoms is yet to be published. To fill this gap in the literature, we comprehensively reviewed the effects of probiotic therapy on behavioral outcomes in populations with obesity, HFD-induced cognitive impairment, and anxiety-like and depression-like behaviors in animal studies. The data obtained from this review was then sorted on the basis of the potential mechanisms of probiotics and the microbial–gut–brain axis in the prevention and treatment of obesity-related cognitive decline disorders, as shown in Figure 2.
Figure 2 Summary of mechanisms by which probiotics modulate obesity-related cognitive impairment. Probiotic therapy can maintain intestinal barrier stability, reduce intestinal permeability, inhibit inflammatory responses, regulate intestinal microbiota composition, modulate the gut-liver-brain axis, regulate glucolipid metabolism, promote neurogenesis in the hippocampus, regulate the hypothalamic-pituitary-adrenal axis and modulate the serotonergic system. These mechanisms are essential channels for probiotics to treat obesity-related cognitive impairment.
Obesity in humans is negatively associated with cognitive performance, and individuals suffering from obesity for extended periods of time are associated with a high risk of developing cognitive impairment and dementia (O'Brien et al., 2017). Several clinical studies have explored the relationship between obesity and cognitive impairment (Morys et al., 2021; Ren et al., 2021; Anand et al., 2022; Li et al., 2022; Zhang et al., 2022). A recent cross-sectional analysis found that systemic and visceral obesity were associated with reduced cognitive scores after adjusting for cardiovascular risk factors, education level, and cerebrovascular impairment, and that strategies to prevent or reduce obesity could protect cognitive function in adults (Anand et al., 2022). A cohort study with an 8-year follow-up showed that metabolic disorders caused by obesity are potential mediators of cognitive impairment and dementia (Morys et al., 2021). The gold standard marker for obesity, the body mass index (BMI), has been positively correlated with the degree of cognitive impairment (Zhang et al., 2022). In addition to BMI, waist-to-hip ratio (WHR), fat mass (FM), and abdominal fat (AF) are markers that are strongly associated with cognitive performance in the older population (Zhang et al., 2022). Trajectory studies have shown that, in older adults with high BMI and FM, lowering WHR or AF can prevent the onset of cognitive impairment or cognitive decline (Li et al., 2022). In addition to overweight, underweight also increases the risk of cognitive impairment in older people (Ren et al., 2021). This suggests an inextricable relationship between weight and cognitive ability which is independent of sex (Guo et al., 2021).
Further evidence suggests that prenatal maternal obesity affects the neurodevelopment and cognitive function of the offspring (Liu et al., 2021). In a cross-sectional study of 778 individuals, Liu et al. (2021) found that maternal pre-pregnancy overweight and obesity were strongly associated with poor cognitive performance and social skills in children. Further studies have found that maternal transplantation of gut microbiota from donors with obesity also leads to mental and social behavioral impairment in offspring (Liu et al., 2021). Maternal obesity may impair the placenta by modulating gut microbial composition, and the microbial–gut–brain axis may be the primary mechanism by which maternal obesity leads to cognitive and social dysfunction in offspring (Liu et al., 2021). These findings suggest a strong link between obesity and cognitive impairment, which can cross gender and age and even have a genetic impact on offspring (Liu et al., 2021).
In addition to clinical evidence, the relationship between obesity and cognitive impairment has been confirmed in animal studies. Consistent with clinical data, numerous experimental studies using animal models of HFD-induced obesity have revealed multiple mechanisms of obesity-induced cognitive impairment (Shi et al., 2020). For example, obesity can cause cognitive impairment by damaging the hippocampi (Shi et al., 2020). High-fat diets reduce neurogenesis, synaptic plasticity, and neuronal growth markers in mice (Shi et al., 2020). The induction of these diets also led to altered inflammatory gene expression in the hippocampi of mice (manifested by elevated monocyte chemoattractant protein-1 [MCP-1]) and an increase in microglia while promoting premature aging of the hippocampus (Henn et al., 2022). Obesity can indirectly cause cognitive impairment through altered metabolism and dysbiosis in the intestinal environment. A HFD causes thinning of the colonic mucus layer, reduction in tight junction proteins, and an increase in intestinal permeability in mice, which further causes changes in the gut microbial composition, resulting in endotoxins entering circulation and triggering neuroinflammation (Shi et al., 2020). This suggests that gut microbial composition is influenced by obesity and HFD. This change further induces neuroinflammation through the microbial–gut–brain axis, damaging the hippocampus and leading to cognitive impairment. Therefore, it is crucial to identify the potential mechanisms underlying the role of the microbe–gut–brain axis in obesity-associated mental decline.
The gastrointestinal tract contains a diverse population of microorganisms that maintain the intestinal barrier and promote compound metabolism. Obesity usually reduces this diversity (Le Chatelier et al., 2013) and affects cognitive function (Leigh and Morris, 2020). Therefore, post-obesity gut microbiome may serve as a reliable therapeutic target. This is reflected in the “microbe–gut–brain axis” between obesity and cognitive impairment, where alterations in the microbiome can activate neural, immune, and hormonal communication systems between the gut and brain, thereby affecting behavior and neurophysiology. However, the interplay between these mechanisms and how they affect the overall metabolic state of individuals remain to be fully understood. Therefore, discussing how the microbiome can target obesity-related cognitive impairment requires examining the unique channels mediating obesity–gut microbe–brain interactions.
The gut microbiota is a complex ecosystem of microbes, archaea, viruses, and their genes. It includes more than 1000 unique strains of bacteria with prokaryotic populations, exceeding the total number of human cells by orders of magnitude (Dinan and Cryan, 2017). Bacteria are vital members of the gut microbiota (Almeida et al., 2019). Macrogenome sequencing of healthy adult feces has identified Firmicutes and Bacteroidaceae as significant groups of intestinal flora (He et al., 2021). Microbial diversity is associated with the host’s health and pathological states. An increased susceptibility to obesity may occur in the presence of insufficient microbial diversity to maintain these functions or in the presence of a metabolically disordered gut microbiota. Recent studies have confirmed that the gut microbiota in individuals with obesity is significantly lower in diversity compared to that of individuals without obesity (Abenavoli et al., 2019) (Le Chatelier et al., 2013).
Although complex genetic, social, and environmental factors contribute to the development of obesity, its core cause is chronic energy imbalance. The intestinal flora can promote energy storage by inhibiting heat production in brown adipose tissue and promoting the expansion of white adipose tissue. In addition, the microbiome can dynamically respond to various internal and external physiological signals such as food intake, energy demand, and stress to maintain metabolic homeostasis. Short-chain fatty acids in the body are products of microbial activity in the gut and can facilitate the digestion of dietary fiber (Wong et al., 2006; Koh et al., 2016). Ding et al (Bäckhed et al., 2004). established an HFD mouse model in a sterile environment and identified a causal relationship between the microbiome and the obesity phenotype, with the gut microbiota influencing energy harvesting and storage in the host diet. Gut microbes assist the host in digesting difficult nutrients. Gut bacteria can degrade plant polysaccharides and complex carbohydrates into short-chain fatty acids such as butyric, propionic, and acetic acids (Turnbaugh et al., 2006). The composition and size of the short-chain-rich acid pool are essential determinants of the host metabolic state (Nehra et al., 2016).
Obesity is a generalized low-grade inflammatory state. A wide range of inflammatory markers and proinflammatory cytokines are strongly associated with the development of obesity (Bahceci et al., 2007; Choi et al., 2013). Although the causal relationship between obesity and inflammation is not fully understood, several lines of evidence suggest that an abnormal gut microbiota is a crucial regulator of immune signaling in the obesity state (Ukena et al., 2007; Telesford et al., 2015; Vallianou et al., 2019). First, lipopolysaccharides (LPS) from the cell walls of pathogenic gram-negative microorganisms in the gut can bind to Toll-like receptors (TLRs) in the mucosa and peripheral tissues, initiating a proinflammatory signaling cascade (Ukena et al., 2007). Bacteroidaceae can also promote differentiation of regulatory T cells and prevent inflammatory responses (Telesford et al., 2015). Second, intestinal flora is crucial for maintaining the integrity of the intestinal epithelium. If this function is compromised, the transit of endotoxins from the intestine into the bloodstream increases (Vallianou et al., 2019). Elevated plasma LPS levels can be detected in patients and mice with obesity, a condition known as metabolic endotoxemia (Creely et al., 2007; Cani et al., 2008; Clemente-Postigo et al., 2019). Metabolic endotoxemia causes a decrease in gram-negative (Bacteroidaceae) and gram-positive bacteria (Ondee et al., 2021; Fan et al., 2022). Animal studies have shown that endotoxemia triggers cognitive impairment via the gut microbiome–brain axis. The application of broad-spectrum antibiotics and beta-glucan can reduce gut microbiota shifts (a decrease in the proportion of Bacteroidaceae) and improve cognitive function (Shi et al., 2020). Mechanistic studies have shown that HFD can alter the composition of the gut microbiota and regulate the TLR4 signaling pathway. This change leads to increased plasma LPS levels and inflammatory response, thereby accelerating obesity (Kim et al., 2012).
Notably, many immune cells reside at the mucosal–luminescent interface of the gastrointestinal tract and exchange immunogenic molecules with the microbiota, thus directing the immune system to recognize potentially harmful pathogens. The microbial–microglia axis is a significant immunoreactive interaction that occurs at the gut and brain levels (Abdel-Haq et al., 2019). It is mediated by cytokines, chemokines, neuropeptides, and neurotransmitters, which can act either through the blood and lymphatic systems or on the vagus nerve and spinal cord afferent projections to the brain. Once activated, the central nervous system (CNS) microglia can release large amounts of cytokines (Pocock and Kettenmann, 2007) and chemokines and recruit monocytes from the periphery via tumor necrosis factor-α (TNF-α)-mediated microglial activation (D'Mello et al., 2009). In mice with HFD-induced obesity, hypothalamic microglia undergo two activations: the first with an inflammatory response and a reduction in inflammatory markers; the second is the gradual replacement of these cells with bone marrow-derived myeloid cells and the return of higher levels of inflammatory markers (Valdearcos et al., 2017). In some cases, inflammatory factors such as TNF-α in the peripheral circulation of individuals with obesity stimulate microglia to produce MCP-1/C-C motif chemokine 2 (CCL2) and brain monocytes (Kerfoot et al., 2006; D'Mello et al., 2009) and activate vagal afferent neurons to induce central inflammation (Valdearcos et al., 2017).
Bile acids (BAs) promote intestinal fat absorption and play an essential role as signaling molecules in the host-gut flora dialogue. The altered gut microbiome in the obesity phenotype is accompanied by altered BAs pool composition and metabolism, which predicts a close relationship between elevated BAs levels and metabolic diseases such as obesity (Ma and Patti, 2014). Studies have shown that HFD feeding leads to an increase in total BAs in the tissues of individuals with obesity, particularly deoxycholic acid (DCA), which is associated with the reorganization of the taxonomic microbiome in favor of the growth of strains capable of handling BAs (Lin et al., 2019). In addition to their powerful antimicrobial properties, BAs may affect the development of intestinal microorganisms and exert selective pressure on bacterial genetics through their antimicrobial activity, often referred to as “intestinal soap” (Wang et al., 2021). Earlier, researchers found that direct BA supplementation caused changes in the composition of the gut microbiome, including an increase in members of the DCA-producing Firmicutes species, a change similar to that induced by an HFD (Islam et al., 2011). Thus, the conversion of BA by the intestinal flora can cause changes in the size of the BA pool. Bile acids can cause changes in the diversity and composition of the intestinal flora, dramatically affecting host physiology.
When considering the role of gut flora in the etiology of obesity, the development of obesity-related complications can be attributed to impaired gut barrier integrity and the subsequent development of metabolic endotoxemia and consequent inflammatory responses in the presence of dysbiosis of the microbiota structure.
The microbial–gut–brain axis is thought to be a key regulator bridging the gap between the endocrine and nervous systems (Cryan et al., 2019). Altered intestinal flora structure and abundance of short-chain fatty acids led to cognitive impairment in preterm rats, which was observed based on significant changes reported in soluble factors associated with the microbial–gut–brain axis (5-hydroxytryptamine [5-HT], gamma-aminobutyric acid [GABA], brain-derived neurotrophic factor [BDNF], interleukin [IL]-6, and TNF-α) (Lu et al., 2022). In addition, the microbiota and brain communicate with each other through chemical signals, such as LPS, neurotransmitters, microbial metabolites (short-chain fatty acids, branched-chain amino acids, peptidoglycans, and indole derivatives), endogenous cannabinoid mediators, and N-acrylamides, to regulate the body’s energy balance (Forte et al., 2020). These molecules move between the gut and brain through anatomical pathways and are released into the blood or act on the vagus nerve, enteric nervous system, and immune system. For example, conventional colony colonization reverses altered myelin formation in germ-free mice at transcriptional and ultrastructural levels (Hoban et al., 2016). In patients with obesity, concomitant with peripheral and systemic inflammation, gut ecological dysregulation and LPS-mediated endotoxemia affect brain inflammation by enhancing the passage of circulating inflammatory factors across the blood–brain barrier (BBB) (Rhea et al., 2017), stimulating microglia via TLR4 (Kabouridis et al., 2015) and inhibiting vagal afferent neurons (Rolig et al., 2017).
This suggests that understanding how the altered immune status is caused by obesity-induced neuroinflammation and cognitive impairment will provide new insights into the development of probiotic interventions. Restoring gut microbiota composition and microbe–gut–brain axis signaling is a new treatment for obesity-related cognitive impairment (Torres-Fuentes et al., 2017).
A constant two-way communication is maintained between the gut and brain. The relationship between the host, microbiota, and microbe–gut–brain axis is critical for influencing microbial populations (Suganya and Koo, 2020). Imbalances in the gut microbiota may affect brain health, and vice versa (Cryan et al., 2020; Jena et al., 2020). An animal study observed the effects of altered gut microbial composition (Higarza et al., 2021). The absence of Lactobacillus, and increased abundance of Enterobacteriaceae, Bacteroidaceae, and Peptostreptococcaceae in rats with non-alcoholic fatty liver disease (NAFLD) exhibited cognitive deficits and reduced spatial working memory (Higarza et al., 2021). Recent clinical studies have observed significantly increased levels of Enterobacteriaceae, TLR4, LPS, and peripheral inflammatory markers in the gut of patients with post-stroke cognitive impairment (Wang et al., 2022). After transplanting fecal colonies from stroke patients into mice, researchers observed that the mice had impaired BBB integrity, microglial hyperactivation, neuronal apoptosis in the CA1 region of the hippocampus, and increased Aβ deposition in the thalamus (Wang et al., 2022). Significant interactions between gut microbiota and alpha-beta deposition have also been observed in patients with AD. This interaction may be mediated by the mitogen-activated protein kinase (MAPK) signaling pathway (Li et al., 2020).
In previous clinical studies, microbiota belonging to the Bacteroidaceae family has been associated with cognitive and neurodegenerative disorders (Carlson et al., 2018; Saji et al., 2019). One study found that infants with high levels of Bacteroidaceae in the gut at one year of age had higher cognitive abilities as compared to those of infants at two years of age (Carlson et al., 2018). In a cross-sectional study, the abundance of Bacteroidaceae was lower in the gut flora of patients with dementia than that in regular patients (Saji et al., 2019). At the species level, the abundance of Bacteroidaceae was lower in patients with cognitive impairment (Cattaneo et al., 2017). Diseases may exhibit specific gut microbial profiles on opposite sides of the spectrum. For example, a randomized controlled trial observed that a particular gut microbiota was correlated with cerebrospinal fluid biomarkers of AD, with the phylum Firmicutes positively associated with tau-p181 (Nagpal et al., 2019).
In addition to the microbiota, there are interactions between the metabolites of microorganisms and the brain. Acetate is a metabolite of gut microbes. Chronic acetate deficiency leads to impaired learning and memory in mice (Zheng et al., 2021). Exogenous acetate supplementation or fecal microbial transplantation restored hippocampal synaptophysin levels in vancomycin-treated type 1 diabetic mice, whereas vagal inhibition or vagotomy attenuated these effects (Zheng et al., 2021).
In summary, there is an inextricable interaction between gut microbiota and the brain. The microbiome can affect cognitive function, anxiety, and depression-like behaviors through community characteristics and metabolite production.
Interventions targeting gut microbiota are promising therapies for improving cognitive function. The use of probiotic supplements in interventional studies exploring the relationship between microbiome and cognition is a mainstream therapeutic approach. A meta-analysis showed that probiotics may improve awareness in patients with AD and mild cognitive impairment by reducing inflammatory and oxidative biomarkers (Den et al., 2020). In a mouse aging model (Yang et al., 2020), researchers observed that ProBiotic-4 (consisting of Bifidobacterium lactis, Lactobacillus casei, Bifidobacterium bifidum, and Lactobacillus acidophilus) significantly attenuated age-related disruption of the intestinal barrier and BBB and improved cognitive deficits. This may be related to probiotics acting on the microbial–gut–brain axis to inhibit the TLR4- and RIG-I-mediated nuclear factor-κB (NF-κB) signaling pathways and inflammatory responses. Probiotic supplements are currently in full clinical use. A recent randomized multicenter trial showed that B. bifidum BGN4 and Bifidobacterium longum BORI promoted psychological healing and improved cognition in older people (Kim et al., 2021). This study, which was conducted in healthy older adults, provided ample evidence of the system-wide effects of probiotics on the gut-brain. Furthermore, a clinical study, microbiome analysis identified Prevotella ruminicola, Bacteroides thetaiotaomicron, and Bacteroides xylanisolvens as the microbiota associated with mild cognitive impairment (Aljumaah et al., 2022).
In conclusion, there is growing evidence from preclinical models and clinical studies that interventions that affect the composition of the global microbiome and target specific bacterial species can effectively change perceptions.
There is a strong relationship between obesity, gut microbial composition, and cognitive function. Probiotics are a promising solution for dysbiosis of the gut microbiota. The World Health Organization defines probiotics as live strains of microorganisms that confer health benefits to their hosts when administered at appropriate doses. Probiotics can alter the microbial populations in the gut microbiota and regulate the function of the intestinal ecosystem, which has implications for endocrine, immune, and central nervous system health (Pelsser et al., 2017; Nishida et al., 2019). Emerging evidence suggests that probiotics have potential therapeutic effects on significant nervous system disorders such as anxiety, depression, autism, and Parkinson’s disease (Lof et al., 2022). Additionally, probiotic therapy is widely used to treat metabolic diseases, such as obesity (Borgeraas et al., 2018; Pontes et al., 2021). Non-traditional studies using the microbiome and microbial–gut–brain axis as entry points for treating obesity-related cognitive impairment have been dominated by probiotic supplements, with few studies using whole fecal transfer or antibiotic treatment (Green et al., 2020). Currently, most research is conducted on mouse models. However, the journey from the preclinical to clinical stage is rigorous and difficult to cross. Fecal transplants are challenging to achieve in humans and are associated with ethical issues. The lack of targeted antibiotic therapy results in the colonization of resistant bacteria. Overall, research describing the role of the microbial–gut–brain axis in health and disease and the potential therapeutic utility of probiotics for treating obesity-related neurodegenerative and psychiatric disorders is an exciting area.
An effective way to reorganize the intestinal flora is to take live strains of bacteria (probiotics) orally, thereby promoting probiotic integration into the intestinal ecosystem. Probiotics are mostly in the form of capsules containing the required concentrations of live bacterial cells. In modern markets, consumers have access to foods enriched with probiotic flora as functional ingredients designed to improve digestive health. Not all probiotics are created equally, and their benefits to the host are host-specific and influenced by strain concentration and type. Targeted probiotic therapy requires an enhanced understanding of microbiota-host interactions to ensure the desired metabolic outcomes. Therefore, this section discusses the preclinical and clinical evidence surrounding the use of probiotics for the treatment of obesity-related cognitive impairment. The results are presented in Tables 1 and 2.
Table 1 Metabolic alterations and cognitive changes due to obesity in the included existing studies.
Table 2 Metabolic changes and cognitive changes following probiotic treatment in the included available studies.
Current research on the use of probiotics for the treatment of obesity-related cognitive impairment has been conducted primarily using preclinical models. In this section, we review the last three years of preclinical research on probiotics for obesity-related cognitive impairment. Notably, cognitive impairment occurred secondary to obesity in the animal experiments described in this section.
In this part of the study, the animals were administered a single strain of probiotic bacteria. We present the results according to the type of strain used.
Akkermansia muciniphila (AKK) of the phylum Verrucomicrobia is the only representative human intestinal microorganism (Collado et al., 2007). Obesity-related cognitive impairment may be treated by AKK via its influence on weight loss and metabolic improvement. In an HFD-fed AD mouse model, AKK treatment reduced body weight (Yang et al., 2019), lowered serum cholesterol and triglycerides, and promoted brown adipocyte growth (Ou et al., 2020). In the intestine, AKK treatment enhanced the intestinal barrier by improving glucolipid metabolism in AD mice, reducing serum diamine oxidase levels, and promoting colonic mucus cell growth (Lof et al., 2022). In terms of cognitive function, AKK administration delayed the pathological changes in the brain caused by AD in the HFD-fed AD mouse model. It alleviated spatial learning and memory deficits, as evidenced by an increased completion rate of the y-maze test in mice, perhaps through a reduction in cortical Aβ levels in AD mice (Ou et al., 2020). In addition to this, AKK treatment reverses HFD-induced microglial cell proliferation in the hippocampus and specifically rescues cognitive function (Yang et al., 2019). This human intestinal microorganism is often compared with the classic probiotic Lactobacillus rhamnosus GG (LGG). In a high-fat, high-cholesterol diet (HFHC)-induced NAFLD rat model, treatment with both LGG and AKK CIP107961 significantly reduced the abundance of Bacteroidaceae, resulting in notable changes in the microbial composition at the phylum level (Higarza et al., 2021). Compared with LGG, AKK treatment was more helpful in restoring impaired spatial working memory. AKK reversed the decline in HFHC-related cytochrome c oxidase (CCO) activity in most brain regions that were previously affected by the HFHC diet (Higarza et al., 2021). Interestingly, neither LGG nor AKK caused any significant rearrangement of fecal microbiota. The AKK strain not only improves cognitive function but also metabolic function.
Lactobacillus plantarum (LP EMCC-1039) is present in many fermented foods. In a rat model of HFD-induced NAFLD, LP EMCC-1039 improved cognitive function by improving fatty liver function as evidenced by an increase in the number of viable cells, increased contractility and thickness of cone cells, and significant improvements in lipid and liver enzyme indices (Mohammed et al., 2020). Lactobacillus plantarum DR7 (DR7) is a probiotic isolated from fresh milk. DR7 treatment significantly reduces body weight and increases telomere length in aged rats with obesity (Zaydi et al., 2020). In a behavioral assessment of aged HFD rats, DR7 treatment reduced anxiety and enhanced memory (Zaydi et al., 2020). At the same time, DR7 decreases the expression of indoleamine dioxygenase and P53 and increased the expression of TPH1, suggesting that DR7 may play a role in serotonin signaling and oxidative senescence pathways (Zaydi et al., 2020). Lactobacillus plantarum JBC5 (LPJBC5) is extracted from curdled milk. LPJBC5 treatment improves learning and memory in worms while improving intestinal integrity by upregulating the expression of the intestinal tight junction protein ZOO-1 (Kumar et al., 2022). Similar to DR7, LPJBC5 treatment upregulated the expression of serotonin signaling-related genes (ser-1, mod-1, and tph-1) (Kumar et al., 2022).
Lactobacillus paracasei D3-5 strain (D3-5) is a dead probiotic strain isolated from the intestinal tracts of infants. The D3-5 strain improves fatty liver, regulates lipid metabolism, and improves cognitive function in mice with obesity-related cognitive impairment (Wang et al., 2020). Lipoteichoic acid (LTA) is a D3-5 cell wall component that increases mucin production (Мokrozub et al., 2015). This component suppresses inflammation and improves leaky gut by regulating the TLR2/p38/MAPK/NF-κB pathway and promoting the expression of Muc2, a major isoform of mucin abundantly expressed in the intestine (Мokrozub et al., 2015). The D3-5 strain can improve cognitive function by modulating LTA levels (Wang et al., 2020). To compare the therapeutic effects of live and dead probiotics, mice were administered Lactobacillus sakei OK67, tyndallized OK67 (tOK67), and heat-stable L. sakei PK16 (Jang et al., 2019). All three probiotics reduced weight and improve lipid metabolism (Jang et al., 2019). In terms of cognitive function, all three probiotics acted on the NF-κB/AMPK pathway, inhibited colonic inflammation, adjusted the ratio of Proteobacteria to Bacteroidaceae, reduced anxiety-like behavior in mice, and promoted the expression of SIRT-1 in the liver and BDNF in the hippocampus (Jang et al., 2019). Overall, the anti-obesity and anti-anxiety effects of live OK67 are more potent than those of tOK67 (Jang et al., 2019).
Bacillus pseudocatenulatum CECT 7765 (CECT 7765) is a probiotic isolated from healthy infants. In a mouse model of obesity with depression-like behavior, CECT 7765 treatment reduced adiposity, partially restored reduced adrenaline concentrations and 5-HT levels in the hypothalamus, and improved obesity-induced pleasure-deprived depressive behavior (reduced sucrose intake) (Agusti et al., 2018). This may be achieved by lowering the fecal corticosterone levels (Agusti et al., 2018). Additionally, CECT 7765 treatment reduces obesity-induced increases in trim intestinal TLR2 protein levels; Bifidobacterium does not have this effect (Agusti et al., 2018).
Lactobacillus helveticus is a probiotic isolated from dairy products. Lactobacillus helveticus treatment reduces anxiety-like behavior in mice with obesity (Ohland et al., 2013). Interestingly, no alterations in short-chain fatty acids were observed in mice receiving L. helveticus, suggesting that this probiotic did not alter carbohydrate metabolism (Ohland et al., 2013). Under certain dietary conditions, L. helveticus induces innate immunity (Ohland et al., 2013). This suggests that the diet of the host and the presence or absence of active inflammation may significantly alter the ability of probiotics to regulate the physiological functions of the host.
In this part of the study, animals were administered probiotic drugs made from a mixture of probiotic strains in a specific ratio of two or more probiotic strains simultaneously.
The Lab4b consortium combines four strains of lactic acid bacteria and bifidobacteria- Bifidobacterium animalis subsp. lactis and Bifidobacterium bifidum, Lactobacillus salivarius and Lactobacillus paracasei (Michael et al., 2019). In a mouse model of HFD-induced AD, Lab4b supplementation reduced body weight by decreasing very-low-density and low-density lipoprotein (VLDL/LDL) levels and reducing neuronal loss in the CA1 region of the hippocampus, as evidenced by increased miRNA levels of complexin-2 (CPLX2), glutamate receptor ionotropic AMPA type subunit 1 (GRIA1), neuronal migration factor, and BDNF (Webberley et al., 2022). Immunologically, Lab4b did not affect immune cell composition in the brain, but increased the transcription rate of key inflammatory markers, as evidenced by decreased messenger RNA (mRNA) expression levels of IL18, NLRP1a, and caspase-1 and increased expression levels of NLRC4 in brain tissue extracts from mice (Webberley et al., 2022). Additionally, supplementation with Lab4b improved the lipid profile and regulated the expression of lipogenic genes in the liver (Webberley et al., 2022). This suggests that Lab4b can slow cognitive decline and AD pathological progression during metabolic challenges, while also providing metabolic improvements which include limiting weight gain and lowering blood lipids (Webberley et al., 2022).
Lactobacillus brevis DPC6108 and DSM32386 are short lactic acid bacteria that produce GABA. These two GABA-producing strains were administered to mice with HFD-induced metabolic dysfunction (Patterson et al., 2019). One study observed that probiotic treatment improved glucose metabolism and insulin sensitivity in mice, while improving depression-like behavior, basal corticosterone levels, and cognitive function (Patterson et al., 2019). This may be related to insulin metabolism and tubular GABA content.
CEREBIOME® (containing Lactobacillus helveticus R0052 and B. longum R0175) is a specific formulation of a probiotic blend. CEREBIOME® treatment significantly improved the anxiety behavior observed in animals with obesity (Myles et al., 2020). Interestingly, the study observed that the particular benefits of CEREBIOME® treatment varied by gender (Myles et al., 2020). In female mice, CEREBIOME® treatment increased leptin levels compared to the placebo and was not accompanied by weight change. In males, leptin levels did not change following CEREBIOME® treatment. This suggests that using sex as a factor in the study design may be unnecessary in future probiotic-related studies. VSL#3 (Consists of three strains of bifidobacteria [B. longum DSM 24736, B. infantis DSM 24737, and B. breve DSM 24732], four strains of Lactobacillus [L. acidophilus DSM 24735, L. paracasei DSM 24733, L. bulgaricus DSM 24734, and L. plantarum DSM 24730], and one strain of Streptococcus salivarius subsp. thermophilus DSM 24731) is a probiotic blend. VSL#3 treatment increased the levels of specific taxa in the gut of cafeteria diet-fed rats, such as Streptococcus, Lactobacillus, and Butyrivibrio (Beilharz et al., 2018). However, the administration of VSL#3 did not affect metabolic outcomes, including weight and fat levels (Beilharz et al., 2018). This suggests that probiotics are beneficial for gut ecological disorders in which memory deficits are evident, but may be detrimental in healthy subjects (Beilharz et al., 2018). These results support the development of bespoke probiotics that may be more effective in reorganizing the gut flora in a healthy direction (Beilharz et al., 2018).
In addition to previously named probiotic mixes, some studies have used unnamed probiotic mixes. Abildgaard et al. (2017) used a mixture of probiotics (consisting of eight strains: B. bifidum W23, B. lactis W52, L. acidophilus W37, L. brevis W63, L. casei W56, L. salivarius W24, and Lc. lactis W19, Lc. Lactis W58) and observed reduced hippocampal transcript levels of HPA axis-related regulators (CRH-R1, CRH-R2, and MR) in HFD-fed mice, whereas HFD alone increased these levels. Metabolomic analysis revealed that the probiotic mix increased the number of a potential neuroprotective agent (indole-3-propionic acid) (Abildgaard et al., 2017). Zeltser et al. (2020) used a mixture of probiotics (consisting of Pediococcus acidilactici and pentosaceus, Lactobacillus plantarum, and Bacillus amyloliquefaciens) to treat NAFLD in Iberian pigs. Studies have shown that feeding probiotics to pigs may impair its cognitive function and increase the levels of essential myelin proteins. It is possible that the translocation of probiotics to the intestinal mucosa caused systemic damage in young pigs (Zeltser et al., 2020).
In existing preclinical models, probiotic interventions affect the metabolism and cognition of experimental animals. These probiotics need to be further studied in humans to determine their safety and whether they will benefit target patients.
Although probiotic-rich fermented foods and dairy products have been introduced into human life, clinical studies on probiotic interventions for patients with obesity-related cognitive impairment are relatively limited. There is a bidirectional relationship between obesity and neuropsychiatric states (Foroozan et al., 2021), which constitutes a subtype of the disease with a unique pathophysiological mechanism called “metabolic-mood-syndrome” (MMS) (Vogelzangs et al., 2011; de Melo et al., 2017). In a clinical study that included 74 patients with MMS, patients were randomized to consume Probio-Tec® BG-VCap-6.5 (containing L. rhamnosus [LGG®] and Bifidobacterium animalis subsp. lactis BB-12®) (Mahboobi et al., 2022). The combination of probiotics and magnesium reduced CRP levels and suppressed inflammation after nine weeks of treatment. However, the probiotics did not exert any effect on mood, cognition, or gut integrity in individuals with obesity and depression (Mahboobi et al., 2022).
The transition from preclinical to clinical trials is the end of probiotic therapy, and more clinical trials should be conducted to verify the safety and therapeutic efficacy of probiotics.
Nearly three years of preclinical and clinical evidence have confirmed that the microbial–gut–brain axis bridges the regulation of obesity-related cognitive impairment by probiotics. In addition to focusing on the therapeutic effects of probiotics, it is essential to understand the specific mechanisms by which probiotics intervene in the microbial–intestinal–brain axis.
An essential feature of obesity is alteration of the intestinal flora (Turnbaugh et al., 2006) and destruction of the intestinal barrier (Cani et al., 2008; Cani et al., 2009). There is clear evidence that intestinal flora affects systemic metabolism by influencing energy balance (Cani et al., 2012), intestinal permeability (Cani et al., 2009; Muccioli et al., 2010), metabolic endotoxemia (Cani et al., 2007), and metabolic inflammation associated with obesity and related diseases (Turnbaugh et al., 2006). Disturbances in the intestinal environment can induce cognitive impairment via bidirectional gut-brain communication, many of which is mediated by metabolites or immune factors produced by intestinal microbes (Doifode et al., 2021). In the animal experiments mentioned earlier, obesity-related cognitive impairment was often accompanied by increased intestinal permeability and inflammation (Jang et al., 2019). Therefore, the gut is a critical node in the microbe–gut–brain axis. Many probiotics use the gut as an entry point to improve metabolic and cognitive functions in animals with obesity by maintaining gut integrity, inhibiting inflammatory factors, and modulating the microbe–gut–brain axis (Мokrozub et al., 2015; Jang et al., 2019; Ou et al., 2020).
In the intestine, there is a physical barrier between the lumen, lamina propria, and mucosa-associated lymphoid tissue, which is comprised of epithelial cells. Therefore, disruption of the intestinal epithelial barrier caused by obesity can lead to the entry of intestinal secretions and LPS into the bloodstream, further inducing an inflammatory response (Kumar et al., 2018; Xu et al., 2018). Diamine oxidase (DAO) is a highly reactive intracellular enzyme that reflects the integrity and extent of damage to the intestinal mechanical barrier and is closely associated with intestinal mucosal cells. The AKK strain normalizes the composition of intestinal flora, which is associated with an improved metabolic profile. AKK supplementation improves cognitive function by lowering serum DAO levels, protecting colonic mucus cells, reducing intestinal permeability, and inhibiting LPS entry into circulation (Ou et al., 2020). Supplementation with D3-5 can improve cognitive function by increasing LTA levels in the gut to regulate the TLR2/p38/MAPK/NF-kB pathway, promote Muc2 expression and inhibit inflammatory factors (Мokrozub et al., 2015). Supplementation with LPJBC5 upregulates the expression of the tight junction protein ZOO-1, which is closely associated with inflammatory factors and improves cognitive function (Kumar et al., 2022). Therefore, by regulating the “microbial–gut–brain axis,” probiotics can reverse HFD-induced metabolic disorders and promote the secretion of intestinal mucus protein-related molecules, thereby reducing intestinal permeability, inhibiting intestinal inflammation, and ultimately improving cognitive function.
The most crucial trigger for the development of obesity-related cognitive impairment is metabolic disturbances caused by obesity. Probiotics can improve the metabolic status and reduce body weight by producing a variety of metabolites, such as short-chain fatty acids (SCFAs), which affect the microbe–gut–brain axis and improve cognitive function. Increased levels of Bacteroides and Bilophila in the gut were observed in a swine model of HFHC-induced NAFLD, leading to increased synthesis of microbial-derived bile acids and hydrogen sulfide, impaired gut barrier function, and cognitive impairment (Zeltser et al., 2020). This suggests that the gut–liver–brain axis may play an important role in obesity-related cognitive impairment (Zeltser et al., 2020). Supplementation with LP EMCC-1039 may improve NAFLD and cognitive impairment by affecting the intestine–liver–brain axis and hippocampal TLR4/BDNF signaling pathway (Mohammed et al., 2020). The intestinal flora metabolite, GABA, regulates the metabolic pattern of the host. Supplementation with GABA-producing Lactobacillus increases endogenous GABA concentration in the small intestine. Supplementation with L. brevis DPC6108 or DSM32386 improves HFD-induced glucose intolerance and impairs insulin sensitivity, depression-like behavior, basal corticosterone levels, and cognitive function in mice (Patterson et al., 2019). This may be related to probiotic interventions that increase luminal GABA content in the small intestine (Patterson et al., 2019).
Thus, probiotics can influence the microbial–gut–brain axis by modulating the metabolites of the intestinal flora and regulating glucolipid metabolism, thus exerting an effect on improving cognitive function.
The brain is one of the critical nodes of the microbe–gut–brain axis and the leading site for cognitive function formation. An imbalance in the F/B ratio of the gut flora can lead to abnormal host metabolism, disruption of serotonin signaling pathways, and further impairment of cognitive function (Liang et al., 2020; Ni et al., 2021). Probiotics can influence the microbial–gut–brain axis to improve cognitive function by modulating the structure of the intestinal flora and promoting the secretion of hormones and neurotransmitters.
The hippocampal region of the brain is responsible for the learning and memory capacities of living organisms. In animals with obesity-related cognitive impairment, neuronal damage can be observed in the hippocampal region, with reduced synapses and hippocampal plasticity accompanied by overactivation of microglia (Chunchai et al., 2018; Yang et al., 2019). Supplementation with L. paracasei HII01 increases the density of dendritic spines in the hippocampus. It inhibits hippocampal apoptosis and mitochondrial brain dysfunction, thereby increasing hippocampal plasticity and improving cognitive function in rats with obesity (Chunchai et al., 2018). Supplementation with L. paracasei HII01 results in inhibition of microglial overactivation through signaling pathways involved in neuroglial cell communication (Chunchai et al., 2018). Similarly, AKK supplementation promotes the formation of CA1 pyramidal neurons in the hippocampal region of newborn mice and improves synaptic plasticity in the hippocampal region, thereby improving cognitive function in mice with obesity (Yang et al., 2019).
Previous studies have shown an interaction between gut flora and the HPA axis (Desbonnet et al., 2008). Probiotic treatment significantly alters hippocampal transcript levels of CRH-R1 and CRH-R2 associated with HPA axis feedback (Abildgaard et al., 2017). Increased CRH-R1 signaling is significantly associated with stress and depression (Dale et al., 2015). Corticosterone is the primary hormone produced by the adrenal cortex in response to hypothalamic-pituitary stimulation. Under pressure, corticosterone concentrations were higher in the feces of mice with obesity than in those of normal mice. Supplementation with CECT 7765 reduces the fecal corticosterone concentration in mice and improves the stress response caused by obesity (Agusti et al., 2018). Similarly, LGG supplementation may reduce anxiety-related behavior by lowering serum corticosterone levels (Foroozan et al., 2021).
Serotonin signaling pathways are essential for emotional and cognitive processes (Dale et al., 2016). Ninety percent of 5-HT in the body is distributed in the intestinal canal and basal plane, with the remainder being widely distributed in the brain tissue, digestive system, and peripheral blood. It is known that 5-HT levels are high in the cerebral cortex and synapses and may act as a bridge between the gut and brain. Supplementation with CECT 7765 ameliorates obesity-induced stress by reducing 5-HT levels in the hippocampus and intestines of anxious mice (Agusti et al., 2018). Supplementation with DR7 reduces anxiety and enhances memory capacity by decreasing indoleamine dioxygenase and P53 expression and increasing TPH1 expression (Zaydi et al., 2020). DR7 may play a role in the serotonin and oxidative senescence pathways (Zaydi et al., 2020).
Thus, probiotics can influence the microbial–gut–brain axis by modulating the HPA axis and serotonin signaling pathways, promoting the secretion of hormones and neurotransmitters, and exerting an effect on improving cognitive function.
The critical roles of the gut microbiota and the microbe–gut–brain axis in obesity-related cognitive impairment have been extensively studied and validated. Through combination, we know that the inflammatory response, abnormalities in the HPA axis, and altered intestinal permeability in people with obesity are all essential mechanisms that contribute to cognitive impairment, and that the gut microbiota is intimately involved in these mechanisms. The microbial–gut–brain axis links the gut microbiota in close crosstalk with organ tissues. Probiotic therapy targets the gut microbiota and the microbe–gut–brain axis and has shown significant improvement in symptoms associated with obesity and neurobehavioral disorders.
To summarize the current animal experiments and clinical trials involving probiotics, it is known that (i) probiotics are effective in reducing body weight, lowering blood lipids, improving metabolism, and improving neurocognitive function; (ii) probiotics can target changes in the composition of the gut microbiota and the ratio between flora, depending on the type of probiotic. Still, it is worth noting that the regulated use of probiotics does not cause significant rearrangement of the gut microbiota; (iii) Several mechanisms of action of probiotic intervention in metabolic and neuropsychiatric disorders have been progressively identified, including gut microbial alterations, intestinal inflammation, and intestinal permeability, neurons in the hippocampus, neuroinflammation, the HPA axis, the serotonergic system, and GABA, but none have been fully elucidated; (iv) Some probiotics (VSL#3) may be harmful to healthy people, even though these probiotics can be very therapeutic when consumed in disease states.
Overall, the current literature provides overwhelming evidence of the benefits of probiotics as supplements and their therapeutic potential for obesity-related cognitive impairment. Probiotic treatment modulates body weight and metabolic parameters (insulin sensitivity, inflammatory response, and energy metabolism) and can improve cognitive behavior. However, the gross generalization of these results should be interpreted with caution, as some studies have shown that some probiotics may be ineffective or may even exert a paradoxical effect. In addition, lack of consistency in study sample sizes, probiotic dosing parameters, treatment duration, and dosing methods hindered the possibility of conducting a comparative analysis between studies.
In the face of these existential problems, we believe that (i) more research should be done to explore the current knowledge on microbiota-host interactions and how probiotics can be used to regulate this dynamic relationship; (ii) Standardized clinical protocols for applying probiotic preparations should be developed, and research at the human level should be increased; (iii) Designing of probiotic functional foods that are highly viable, stress-resistant, and suitable for transport to improve microbial viability, stable colonization, and long-term dietary adherence must be carried out in the near future. Much work remains to ensure that probiotic therapies meet the medical application safety, purity, and efficacy standards. By further understanding the mechanisms of probiotic benefits and harm to host health, there is an opportunity to develop safe and targeted therapies that maximize the potential of probiotics in combating metabolic pathologies and neurological diseases.
YC conceived and drafted the article. PL, XZ, and JY performed the literature search. QC critically revised the work. YC had primary responsibility for the final content. All authors read and approved the final manuscript. All authors contributed to the article.
This study was supported by the construction and regional promotion and application of the intelligent management innovation system of TCM for metabolic diseases based on internet of things technology (CKY2021088).
We would like to thank Editage (www.editage.com) for English language editing.
The authors declare that the research was conducted in the absence of any commercial or financial relationships that could be construed as a potential conflict of interest.
All claims expressed in this article are solely those of the authors and do not necessarily represent those of their affiliated organizations, or those of the publisher, the editors and the reviewers. Any product that may be evaluated in this article, or claim that may be made by its manufacturer, is not guaranteed or endorsed by the publisher.
Abdel-Haq, R., Schlachetzki, J. C. M., Glass, C. K., Mazmanian, S. K. (2019). Microbiome-microglia connections via the gut-brain axis. J. Exp. Med. 216 (1), 41–59. doi: 10.1084/jem.20180794
Abenavoli, L., Scarpellini, E., Colica, C., Boccuto, L., Salehi, B., Sharifi-Rad, J., et al. (2019). Gut microbiota and obesity: a role for probiotics. Nutrients 11 (11), 2690. doi: 10.3390/nu11112690
Abildgaard, A., Elfving, B., Hokland, M., Wegener, G., Lund, S. (2017). Probiotic treatment reduces depressive-like behaviour in rats independently of diet. Psychoneuroendocrinology 79, 40–48. doi: 10.1016/j.psyneuen.2017.02.014
Aggarwal, J., Swami, G., Kumar, M. (2013). Probiotics and their effects on metabolic diseases: an update. J. Clin. Diagn. Res. 7 (1), 173–177. doi: 10.7860/JCDR/2012/5004.2701
Agustí, A., García-Pardo, M. P., López-Almela, I., Campillo, I., Maes, M., Romaní-Pérez, M., et al. (2018). Interplay between the gut-brain axis, obesity and cognitive function. Front. Neurosci. 12, 155. doi: 10.3389/fnins.2018.00155
Agusti, A., Moya-Pérez, A., Campillo, I., Montserrat-de la Paz, S., Cerrudo, V., Perez-Villalba, A., et al. (2018). Bifidobacterium pseudocatenulatum CECT 7765 ameliorates neuroendocrine alterations associated with an exaggerated stress response and anhedonia in obese mice. Mol. Neurobiol. 55 (6), 5337–5352. doi: 10.1007/s12035-017-0768-z
Aljumaah, M. R., Bhatia, U., Roach, J., Gunstad, J., Azcarate Peril, M. A. (2022). The gut microbiome, mild cognitive impairment, and probiotics: a randomized clinical trial in middle-aged and older adults. Clin. Nutr. 41 (11), 2565–2576. doi: 10.1016/j.clnu.2022.09.012
Almeida, A., Mitchell, A. L., Boland, M., Forster, S. C., Gloor, G. B., Tarkowska, A., et al. (2019). A new genomic blueprint of the human gut microbiota. Nature 568 (7753), 499–504. doi: 10.1038/s41586-019-0965-1
Almeida-Suhett, C. P., Graham, A., Chen, Y., Deuster, P. (2017). Behavioral changes in male mice fed a high-fat diet are associated with IL-1β expression in specific brain regions. Physiol. Behav. 169, 130–140. doi: 10.1016/j.physbeh.2016.11.016
Alonso-Caraballo, Y., Hodgson, K. J., Morgan, S. A., Ferrario, C. R., Vollbrecht, P. J. (2019). Enhanced anxiety-like behavior emerges with weight gain in male and female obesity-susceptible rats. Behav. Brain Res. 360, 81–93. doi: 10.1016/j.bbr.2018.12.002
Anand, S. S., Friedrich, M. G., Lee, D. S., Awadalla, P., Després, J. P., Desai, D., et al. (2022). Evaluation of adiposity and cognitive function in adults. JAMA Netw. Open 5 (2), e2146324. doi: 10.1001/jamanetworkopen.2021.46324
Ansari, F., Pourjafar, H., Tabrizi, A., Homayouni, A. (2020). The effects of probiotics and prebiotics on mental disorders: a review on depression, anxiety, Alzheimer, and autism spectrum disorders. Curr. Pharm. Biotechnol. 21 (7), 555–565. doi: 10.2174/1389201021666200107113812
Azad, M. A. K., Sarker, M., Li, T., Yin, J. (2018). Probiotic species in the modulation of gut microbiota: an overview. BioMed. Res. Int. 2018, 9478630. doi: 10.1155/2018/9478630
Bäckhed, F., Ding, H., Wang, T., Hooper, L. V., Koh, G. Y., Nagy, A., et al. (2004). The gut microbiota as an environmental factor that regulates fat storage. Proc. Natl. Acad. Sci. U. S. A. 101 (44), 15718–15723. doi: 10.1073/pnas.0407076101
Bahceci, M., Gokalp, D., Bahceci, S., Tuzcu, A., Atmaca, S., Arikan, S. (2007). The correlation between adiposity and adiponectin, tumor necrosis factor alpha, interleukin-6 and high sensitivity c-reactive protein levels. is adipocyte size associated with inflammation in adults? J. Endocrinol. Invest. 30 (3), 210–214. doi: 10.1007/BF03347427
Baothman, O. A., Zamzami, M. A., Taher, I., Abubaker, J., Abu-Farha, M. (2016). The role of gut microbiota in the development of obesity and diabetes. Lipids Health Dis. 15, 108. doi: 10.1186/s12944-016-0278-4
Beilharz, J. E., Kaakoush, N. O., Maniam, J., Morris, M. J. (2018). Cafeteria diet and probiotic therapy: cross talk among memory, neuroplasticity, serotonin receptors and gut microbiota in the rat. Mol. Psychiatry 23 (2), 351–361. doi: 10.1038/mp.2017.38
Bliss, E. S., Whiteside, E. (2018). The gut-brain axis, the human gut microbiota and their integration in the development of obesity. Front. Physiol. 9, 900. doi: 10.3389/fphys.2018.00900
Bocarsly, M. E., Fasolino, M., Kane, G. A., LaMarca, E. A., Kirschen, G. W., Karatsoreos, I. N., et al. (2015). Obesity diminishes synaptic markers, alters microglial morphology, and impairs cognitive function. Proc. Natl. Acad. Sci. U S A. 112 (51), 15731–15736. doi: 10.1073/pnas.1511593112
Borgeraas, H., Johnson, L. K., Skattebu, J., Hertel, J. K., Hjelmesaeth, J. (2018). Effects of probiotics on body weight, body mass index, fat mass and fat percentage in subjects with overweight or obesity: a systematic review and meta-analysis of randomized controlled trials. Obes. Rev. 19 (2), 219–232. doi: 10.1111/obr.12626
Bruce-Keller, A. J., Salbaum, J. M., Luo, M., Blanchard, E., Taylor, C. M., Welsh, D. A., et al. (2015). Obese-type gut microbiota induce neurobehavioral changes in the absence of obesity. Biol. Psychiatry 77 (7), 607–615. doi: 10.1016/j.biopsych.2014.07.012
Cani, P. D., Amar, J., Iglesias, M. A., Poggi, M., Knauf, C., Bastelica, D., et al. (2007). Metabolic endotoxemia initiates obesity and insulin resistance. Diabetes 56 (7), 1761–1772. doi: 10.2337/db06-1491
Cani, P. D., Bibiloni, R., Knauf, C., Waget, A., Neyrinck, A. M., Delzenne, N. M., et al. (2008). Changes in gut microbiota control metabolic endotoxemia-induced inflammation in high-fat diet-induced obesity and diabetes in mice. Diabetes. 57 (6), 1470–1481. doi: 10.2337/db07-1403
Cani, P. D., Osto, M., Geurts, L., Everard, A. (2012). Involvement of gut microbiota in the development of low-grade inflammation and type 2 diabetes associated with obesity. Gut Microbes 3 (4), 279–288. doi: 10.4161/gmic.19625
Cani, P. D., Possemiers, S., Van de Wiele, T., Guiot, Y., Everard, A., Rottier, O., et al. (2009). Changes in gut microbiota control inflammation in obese mice through a mechanism involving GLP-2-driven improvement of gut permeability. Gut 58 (8), 1091–1103. doi: 10.1136/gut.2008.165886
Carlson, A. L., Xia, K., Azcarate-Peril, M. A., Goldman, B. D., Ahn, M., Styner, M. A., et al. (2018). Infant gut microbiome associated with cognitive development. Biol. Psychiatry 83 (2), 148–159. doi: 10.1016/j.biopsych.2017.06.021
Cattaneo, A., Cattane, N., Galluzzi, S., Provasi, S., Lopizzo, N., Festari, C., et al. (2017). Association of brain amyloidosis with pro-inflammatory gut bacterial taxa and peripheral inflammation markers in cognitively impaired elderly. Neurobiol. Aging. 49, 60–68. doi: 10.1016/j.neurobiolaging.2016.08.019
Choi, J., Joseph, L., Pilote, L. (2013). Obesity and c-reactive protein in various populations: a systematic review and meta-analysis. Obes. Rev. 14 (3), 232–244. doi: 10.1111/obr.12003
Chunchai, T., Thunapong, W., Yasom, S., Wanchai, K., Eaimworawuthikul, S., Metzler, G., et al. (2018). Decreased microglial activation through gut-brain axis by prebiotics, probiotics, or synbiotics effectively restored cognitive function in obese-insulin resistant rats. J. Neuroinflammation. 15 (1), 11. doi: 10.1186/s12974-018-1055-2
Clemente-Postigo, M., Oliva-Olivera, W., Coin-Aragüez, L., Ramos-Molina, B., Giraldez-Perez, R. M., Lhamyani, S., et al. (2019). Metabolic endotoxemia promotes adipose dysfunction and inflammation in human obesity. Am. J. Physiol. Endocrinol. Metab. 316 (2), E319–EE32. doi: 10.1152/ajpendo.00277.2018
Collado, M. C., Derrien, M., Isolauri, E., de Vos, W. M., Salminen, S. (2007). Intestinal integrity and akkermansia muciniphila, a mucin-degrading member of the intestinal microbiota present in infants, adults, and the elderly. Appl. Environ. Microbiol. 73 (23), 7767–7770. doi: 10.1128/AEM.01477-07
Cordner, Z. A., Tamashiro, K. L. K. (2015). Effects of high-fat diet exposure on learning & memory. Physiol. Behav. 152 (Pt B), 363–371. doi: 10.1016/j.physbeh.2015.06.008
Creely, S. J., McTernan, P. G., Kusminski, C. M., Fisher, fM, Da Silva, N. F., Khanolkar, M., et al. (2007). Lipopolysaccharide activates an innate immune system response in human adipose tissue in obesity and type 2 diabetes. Am. J. Physiol. Endocrinol. Metab. 292 (3), E740–E7E7. doi: 10.1152/ajpendo.00302.2006
Cryan, J. F., O'Riordan, K. J., Cowan, C. S. M., Sandhu, K. V., Bastiaanssen, T. F. S., Boehme, M., et al. (2019). The microbiota-Gut-Brain axis. Physiol. Rev. 99 (4), 1877–2013. doi: 10.1152/physrev.00018.2018
Cryan, J. F., O'Riordan, K. J., Sandhu, K., Peterson, V., Dinan, T. G. (2020). The gut microbiome in neurological disorders. Lancet Neurol. 19 (2), 179–194. doi: 10.1016/S1474-4422(19)30356-4
D'Mello, C., Le, T., Swain, M. G. (2009). Cerebral microglia recruit monocytes into the brain in response to tumor necrosis factoralpha signaling during peripheral organ inflammation. J. Neurosci. 29 (7), 2089–2102. doi: 10.1523/JNEUROSCI.3567-08.2009
Dale, E., Bang-Andersen, B., Sánchez, C. (2015). Emerging mechanisms and treatments for depression beyond SSRIs and SNRIs. Biochem. Pharmacol. 95 (2), 81–97. doi: 10.1016/j.bcp.2015.03.011
Dale, E., Pehrson, A. L., Jeyarajah, T., Li, Y., Leiser, S. C., Smagin, G., et al. (2016). Effects of serotonin in the hippocampus: how SSRIs and multimodal antidepressants might regulate pyramidal cell function. CNS Spectr. 21 (2), 143–161. doi: 10.1017/S1092852915000425
de Melo, L. G. P., Nunes, S. O. V., Anderson, G., Vargas, H. O., Barbosa, D. S., Galecki, P., et al. (2017). Shared metabolic and immune-inflammatory, oxidative and nitrosative stress pathways in the metabolic syndrome and mood disorders. Prog. Neuropsychopharmacol. Biol. Psychiatry 78, 34–50. doi: 10.1016/j.pnpbp.2017.04.027
Den, H., Dong, X., Chen, M., Zou, Z. (2020). Efficacy of probiotics on cognition, and biomarkers of inflammation and oxidative stress in adults with alzheimer's disease or mild cognitive impairment - a meta-analysis of randomized controlled trials. Aging (Albany NY). 12 (4), 4010–4039. doi: 10.18632/aging.102810
de Noronha, S. R., Campos, G. V., Abreu, A. R., de Souza, A. A., Chianca, D. A., de Menezes, R. C. (2017). High fat diet induced-obesity facilitates anxiety-like behaviors due to GABAergic impairment within the dorsomedial hypothalamus in rats. Behav. Brain Res. 316, 38–46. doi: 10.1016/j.bbr.2016.08.042
Desbonnet, L., Garrett, L., Clarke, G., Bienenstock, J., Dinan, T. G. (2008). The probiotic bifidobacteria infantis: an assessment of potential antidepressant properties in the rat. J. Psychiatr. Res. 43 (2), 164–174. doi: 10.1016/j.jpsychires.2008.03.009
Dinan, T. G., Cryan, J. F. (2017). Gut-brain axis in 2016: brain-gut-microbiota axis - mood, metabolism and behaviour. Nat. Rev. Gastroenterol. Hepatol. 14 (2), 69–70. doi: 10.1038/nrgastro.2016.200
Doifode, T., Giridharan, V. V., Generoso, J. S., Bhatti, G., Collodel, A., Schulz, P. E., et al. (2021). The impact of the microbiota-gut-brain axis on alzheimer's disease pathophysiology. Pharmacol. Res. 164, 105314. doi: 10.1016/j.phrs.2020.105314
Fan, Z., Zhang, X., Shang, Y., Zou, M., Zhou, M., E, Q., et al. (2022). Intestinal flora changes induced by a high-fat diet promote activation of primordial follicles through macrophage infiltration and inflammatory factor secretion in mouse ovaries. Int. J. Mol. Sci. 23 (9), 4797. doi: 10.3390/ijms23094797
Farruggia, M. C., Small, D. M. (2019). Effects of adiposity and metabolic dysfunction on cognition: a review. Physiol. Behav. 208, 112578. doi: 10.1016/j.physbeh.2019.112578
Fazzari, G., Zizza, M., Di Vito, A., Alò, R., Mele, M., Bruno, R., et al. (2018). Reduced learning and memory performances in high-fat treated hamsters related to brain neurotensin receptor1 expression variations. Behav. Brain Res. 347, 227–233. doi: 10.1016/j.bbr.2018.03.015
Foroozan, P., Koushkie Jahromi, M., Nemati, J., Sepehri, H., Safari, M. A., Brand, S. (2021). Probiotic supplementation and high-intensity interval training modify anxiety-like behaviors and corticosterone in high-fat diet-induced obesity mice. Nutrients 13 (6), 1762. doi: 10.3390/nu13061762
Forte, N., Fernández-Rilo, A. C., Palomba, L., Di Marzo, V., Cristino, L. (2020). Obesity affects the microbiota-Gut-Brain axis and the regulation thereof by endocannabinoids and related mediators. Int. J. Mol. Sci. 21 (5), 1554. doi: 10.3390/ijms21051554
Foster, J. A., McVey Neufeld, K.-A. (2013). Gut-brain axis: how the microbiome influences anxiety and depression. Trends Neurosci. 36 (5), 305–312. doi: 10.1016/j.tins.2013.01.005
Green, M., Arora, K., Prakash, S. (2020). Microbial medicine: prebiotic and probiotic functional foods to target obesity and metabolic syndrome. Int. J. Mol. Sci. 21 (8), 2890. doi: 10.3390/ijms21082890
Guo, D., Zhang, X., Zhan, C., Lin, Q., Liu, J., Yang, Q., et al. (2021). Sex differences in the association between obesity and cognitive impairment in a low-income elderly population in rural China: a population-based cross-sectional study. Front. Neurol. 12, 669174. doi: 10.3389/fneur.2021.669174
He, T., Cheng, X., Xing, C. (2021). The gut microbial diversity of colon cancer patients and the clinical significance. Bioengineered. 12 (1), 7046–7060. doi: 10.1080/21655979.2021.1972077
Henn, R. E., Elzinga, S. E., Glass, E., Parent, R., Guo, K., Allouch, A. A., et al. (2022). Obesity-induced neuroinflammation and cognitive impairment in young adult versus middle-aged mice. Immun. Ageing. 19 (1), 67. doi: 10.1186/s12979-022-00323-7
Higarza, S. G., Arboleya, S., Arias, J. L., Gueimonde, M., Arias, N. (2021). Akkermansia muciniphila and environmental enrichment reverse cognitive impairment associated with high-fat high-cholesterol consumption in rats. Gut Microbes 13 (1), 1–20. doi: 10.1080/19490976.2021.1880240
Hildebrandt, M. A., Hoffmann, C., Sherrill-Mix, S. A., Keilbaugh, S. A., Hamady, M., Chen, Y.-Y., et al. (2009). High-fat diet determines the composition of the murine gut microbiome independently of obesity. Gastroenterology 137 (5), 1716–24.e1-2. doi: 10.1053/j.gastro.2009.08.042
Hoban, A. E., Stilling, R. M., Ryan, F. J., Shanahan, F., Dinan, T. G., Claesson, M. J., et al. (2016). Regulation of prefrontal cortex myelination by the microbiota. Transl. Psychiatry 6 (4), e774. doi: 10.1038/tp.2016.42
Hoffman, D. J., Powell, T. L., Barrett, E. S., Hardy, D. B. (2021). Developmental origins of metabolic diseases. Physiol. Rev. 101 (3), 739–795. doi: 10.1152/physrev.00002.2020
Islam, K. B. M. S., Fukiya, S., Hagio, M., Fujii, N., Ishizuka, S., Ooka, T., et al. (2011). Bile acid is a host factor that regulates the composition of the cecal microbiota in rats. Gastroenterology 141 (5), 1773–1781. doi: 10.1053/j.gastro.2011.07.046
Jang, H.-M., Han, S.-K., Kim, J.-K., Oh, S.-J., Jang, H.-B., Kim, D.-H. (2019). Lactobacillus sakei alleviates high-Fat-Diet-Induced obesity and anxiety in mice by inducing AMPK activation and SIRT1 expression and inhibiting gut microbiota-mediated NF-κB activation. Mol. Nutr. Food Res. 63 (6), e1800978. doi: 10.1002/mnfr.201800978
Jena, A., Montoya, C. A., Mullaney, J. A., Dilger, R. N., Young, W., McNabb, W. C., et al. (2020). Gut-brain axis in the early postnatal years of life: a developmental perspective. Front. Integr. Neurosci. 14, 44. doi: 10.3389/fnint.2020.00044
Ji, Y. S., Kim, H. N., Park, H. J., Lee, J. E., Yeo, S. Y., Yang, J. S., et al. (2012). Modulation of the murine microbiome with a concomitant anti-obesity effect by lactobacillus rhamnosus GG and lactobacillus sakei NR28. Benef Microbes 3 (1), 13–22. doi: 10.3920/BM2011.0046
Kabouridis, P. S., Lasrado, R., McCallum, S., Chng, S. H., Snippert, H. J., Clevers, H., et al. (2015). Microbiota controls the homeostasis of glial cells in the gut lamina propria. Neuron 85 (2), 289–295. doi: 10.1016/j.neuron.2014.12.037
Kerfoot, S. M., D'Mello, C., Nguyen, H., Ajuebor, M. N., Kubes, P., Le, T., et al. (2006). TNF-alpha-secreting monocytes are recruited into the brain of cholestatic mice. Hepatology 43 (1), 154–162. doi: 10.1002/hep.21003
Kesika, P., Suganthy, N., Sivamaruthi, B. S., Chaiyasut, C. (2021). Role of gut-brain axis, gut microbial composition, and probiotic intervention in alzheimer's disease. Life Sci. 264, 118627. doi: 10.1016/j.lfs.2020.118627
Kim, C.-S., Cha, L., Sim, M., Jung, S., Chun, W. Y., Baik, H. W., et al. (2021). Probiotic supplementation improves cognitive function and mood with changes in gut microbiota in community-dwelling older adults: a randomized, double-blind, placebo-controlled, multicenter trial. J. Gerontol A Biol. Sci. Med. Sci. 76 (1), 32–40. doi: 10.1093/gerona/glaa090
Kim, K.-A., Gu, W., Lee, I.-A., Joh, E.-H., Kim, D.-H. (2012). High fat diet-induced gut microbiota exacerbates inflammation and obesity in mice via the TLR4 signaling pathway. PloS One 7 (10), e47713. doi: 10.1371/journal.pone.0047713
Kobyliak, N., Conte, C., Cammarota, G., Haley, A. P., Styriak, I., Gaspar, L., et al. (2016). Probiotics in prevention and treatment of obesity: a critical view. Nutr. Metab. (Lond). 13, 14. doi: 10.1186/s12986-016-0067-0
Koh, A., De Vadder, F., Kovatcheva-Datchary, P., Bäckhed, F. (2016). From dietary fiber to host physiology: short-chain fatty acids as key bacterial metabolites. Cell 165 (6), 1332–1345. doi: 10.1016/j.cell.2016.05.041
Kumar, A., Chatterjee, I., Anbazhagan, A. N., Jayawardena, D., Priyamvada, S., Alrefai, W. A., et al. (2018). Cryptosporidium parvum disrupts intestinal epithelial barrier function via altering expression of key tight junction and adherens junction proteins. Cell Microbiol. 20 (6), e12830. doi: 10.1111/cmi.12830
Kumar, A., Joishy, T., Das, S., Kalita, M. C., Mukherjee, A. K., Khan, M. R. (2022). A potential probiotic lactobacillus plantarum JBC5 improves longevity and healthy aging by modulating antioxidative, innate immunity and serotonin-signaling pathways in caenorhabditis elegans. Antioxidants (Basel) 11 (2), 268. doi: 10.3390/antiox11020268
Leblhuber, F., Ehrlich, D., Steiner, K., Geisler, S., Fuchs, D., Lanser, L., et al. (2021). The immunopathogenesis of alzheimer's disease is related to the composition of gut microbiota. Nutrients 13 (2), 361. doi: 10.3390/nu13020361
Le Chatelier, E., Nielsen, T., Qin, J., Prifti, E., Hildebrand, F., Falony, G., et al. (2013). Richness of human gut microbiome correlates with metabolic markers. Nature 500 (7464), 541–546. doi: 10.1038/nature12506
Leigh, S.-J., Morris, M. J. (2020). Diet, inflammation and the gut microbiome: mechanisms for obesity-associated cognitive impairment. Biochim. Biophys. Acta Mol. Basis Dis. 1866 (6), 165767. doi: 10.1016/j.bbadis.2020.165767
Le Port, A., Gueguen, A., Kesse-Guyot, E., Melchior, M., Lemogne, C., Nabi, H., et al. (2012). Association between dietary patterns and depressive symptoms over time: a 10-year follow-up study of the GAZEL cohort. PloS One 7 (12), e51593. doi: 10.1371/journal.pone.0051593
Li, W., Dowd, S. E., Scurlock, B., Acosta-Martinez, V., Lyte, M. (2009). Memory and learning behavior in mice is temporally associated with diet-induced alterations in gut bacteria. Physiol. Behav. 96 (4-5), 557–567. doi: 10.1016/j.physbeh.2008.12.004
Li, T.-C., Li, C.-I., Liu, C.-S., Lin, C.-H., Yang, S.-Y., Lin, C.-C. (2022). Obesity marker trajectories and cognitive impairment in older adults: a 10-year follow-up in taichung community health study for elders. BMC Psychiatry 22 (1), 748. doi: 10.1186/s12888-022-04420-1
Li, Z., Zhu, H., Guo, Y., Du, X., Qin, C. (2020). Gut microbiota regulate cognitive deficits and amyloid deposition in a model of alzheimer's disease. J. Neurochem. 155 (4), 448–461. doi: 10.1111/jnc.15031
Liang, Y., Liu, D., Zhan, J., Luo, M., Han, J., Wang, P., et al. (2020). New insight into the mechanism of POP-induced obesity: evidence from DDE-altered microbiota. Chemosphere 244, 125123. doi: 10.1016/j.chemosphere.2019.125123
Lin, H., An, Y., Tang, H., Wang, Y. (2019). Alterations of bile acids and gut microbiota in obesity induced by high fat diet in rat model. J. Agric. Food Chem. 67 (13), 3624–3632. doi: 10.1021/acs.jafc.9b00249
Liu, X., Li, X., Xia, B., Jin, X., Zou, Q., Zeng, Z., et al. (2021). High-fiber diet mitigates maternal obesity-induced cognitive and social dysfunction in the offspring via gut-brain axis. Cell Metab. 33 (5), 923–938.e6. doi: 10.1016/j.cmet.2021.02.002
Lof, J., Smits, K., Melotte, V., Kuil, L. E. (2022). The health effect of probiotics on high-fat diet-induced cognitive impairment, depression and anxiety: a cross-species systematic review. Neurosci. Biobehav. Rev. 136, 104634. doi: 10.1016/j.neubiorev.2022.104634
Lu, X., Xue, Z., Qian, Y., Wei, S., Qiao, Y., Zhang, W., et al. (2022). Changes in intestinal microflora and its metabolites underlie the cognitive impairment in preterm rats. Front. Cell Infect. Microbiol. 12, 945851. doi: 10.3389/fcimb.2022.945851
Luna, R. A., Foster, J. A. (2015). Gut brain axis: diet microbiota interactions and implications for modulation of anxiety and depression. Curr. Opin. Biotechnol. 32, 35–41. doi: 10.1016/j.copbio.2014.10.007
Ma, H., Patti, M. E. (2014). Bile acids, obesity, and the metabolic syndrome. Best Pract. Res. Clin. Gastroenterol. 28 (4), 573–583. doi: 10.1016/j.bpg.2014.07.004
Mahboobi, S., Ghasvarian, M., Ghaem, H., Alipour, H., Alipour, S., Eftekhari, M. H. (2022). Effects of probiotic and magnesium co-supplementation on mood, cognition, intestinal barrier function and inflammation in individuals with obesity and depressed mood: a randomized, double-blind placebo-controlled clinical trial. Front. Nutr. 9, 1018357. doi: 10.3389/fnut.2022.1018357
MahmoudianDehkordi, S., Arnold, M., Nho, K., Ahmad, S., Jia, W., Xie, G., et al. (2019). Altered bile acid profile associates with cognitive impairment in alzheimer's disease-an emerging role for gut microbiome. Alzheimers Dement. 15 (1), 76–92. doi: 10.1016/j.jalz.2018.07.217
Mattson, M. P., Arumugam, T. V. (2018). Hallmarks of brain aging: adaptive and pathological modification by metabolic states. Cell Metab. 27 (6), 1176–1199. doi: 10.1016/j.cmet.2018.05.011
Michael, D. R., Davies, T. S., Loxley, K. E., Allen, M. D., Good, M. A., Hughes, T. R., et al. (2019). In vitro neuroprotective activities of two distinct probiotic consortia. Benef. Microbes 10 (4), 437–447. doi: 10.3920/BM2018.0105
Million, M., Angelakis, E., Paul, M., Armougom, F., Leibovici, L., Raoult, D. (2012). Comparative meta-analysis of the effect of lactobacillus species on weight gain in humans and animals. Microb. Pathog. 53 (2), 100–108. doi: 10.1016/j.micpath.2012.05.007
Mohammed, S. K., Magdy, Y. M., El-Waseef, D. A., Nabih, E. S., Hamouda, M. A., El-Kharashi, O. A. (2020). Modulation of hippocampal TLR4/BDNF signal pathway using probiotics is a step closer towards treating cognitive impairment in NASH model. Physiol. Behav. 214, 112762. doi: 10.1016/j.physbeh.2019.112762
Мokrozub, V. V., Lazarenko, L. M., Sichel, L. M., Babenko, L. P., Lytvyn, P. M., Demchenko, O. M., et al. (2015). The role of beneficial bacteria wall elasticity in regulating innate immune response. EPMA J. 6 (1), 13. doi: 10.1186/s13167-015-0035-1
Monda, V., La Marra, M., Perrella, R., Caviglia, G., Iavarone, A., Chieffi, S., et al. (2017). Obesity and brain illness: from cognitive and psychological evidences to obesity paradox. Diabetes Metab. Syndr. Obes. 10, 473–479. doi: 10.2147/DMSO.S148392
Morys, F., Dadar, M., Dagher, A. (2021). Association between midlife obesity and its metabolic consequences, cerebrovascular disease, and cognitive decline. J. Clin. Endocrinol. Metab. 106 (10), e4260–e4e74. doi: 10.1210/clinem/dgab135
Muccioli, G. G., Naslain, D., Bäckhed, F., Reigstad, C. S., Lambert, D. M., Delzenne, N. M., et al. (2010). The endocannabinoid system links gut microbiota to adipogenesis. Mol. Syst. Biol. 6, 392. doi: 10.1038/msb.2010.46
Myles, E. M., O'Leary, M. E., Smith, R., MacPherson, C. W., Oprea, A., Melanson, E. H., et al. (2020). Supplementation with combined lactobacillus helveticus R0052 and bifidobacterium longum R0175 across development reveals sex differences in physiological and behavioural effects of Western diet in long-Evans rats. Microorganisms 8 (10), 1527. doi: 10.3390/microorganisms8101527
Nagpal, R., Neth, B. J., Wang, S., Craft, S., Yadav, H. (2019). Modified Mediterranean-ketogenic diet modulates gut microbiome and short-chain fatty acids in association with alzheimer's disease markers in subjects with mild cognitive impairment. EBioMedicine 47, 529–542. doi: 10.1016/j.ebiom.2019.08.032
Nehra, V., Allen, J. M., Mailing, L. J., Kashyap, P. C., Woods, J. A. (2016). Gut microbiota: modulation of host physiology in obesity. Physiol. (Bethesda). 31 (5), 327–335. doi: 10.1152/physiol.00005.2016
Ng, Q. X., Loke, W., Venkatanarayanan, N., Lim, D. Y., Soh, A. Y. S., Yeo, W. S. (2019). A systematic review of the role of prebiotics and probiotics in autism spectrum disorders. Medicina (Kaunas) 55 (5), 129. doi: 10.3390/medicina55050129
Ni, Y., Hu, L., Yang, S., Ni, L., Ma, L., Zhao, Y., et al. (2021). Bisphenol a impairs cognitive function and 5-HT metabolism in adult male mice by modulating the microbiota-gut-brain axis. Chemosphere 282, 130952. doi: 10.1016/j.chemosphere.2021.130952
Nishida, K., Sawada, D., Kuwano, Y., Tanaka, H., Rokutan, K. (2019). Health benefits of lactobacillus gasseri CP2305 tablets in young adults exposed to chronic stress: a randomized, double-blind, placebo-controlled study. Nutrients 11 (8), 1859. doi: 10.3390/nu11081859
Noble, E. E., Hsu, T. M., Kanoski, S. E. (2017). Gut to brain dysbiosis: mechanisms linking Western diet consumption, the microbiome, and cognitive impairment. Front. Behav. Neurosci. 11, 9. doi: 10.3389/fnbeh.2017.00009
O'Brien, P. D., Hinder, L. M., Callaghan, B. C., Feldman, E. L. (2017). Neurological consequences of obesity. Lancet Neurol. 16 (6), 465–477. doi: 10.1016/S1474-4422(17)30084-4
Ohland, C. L., Kish, L., Bell, H., Thiesen, A., Hotte, N., Pankiv, E., et al. (2013). Effects of lactobacillus helveticus on murine behavior are dependent on diet and genotype and correlate with alterations in the gut microbiome. Psychoneuroendocrinology 38 (9), 1738–1747. doi: 10.1016/j.psyneuen.2013.02.008
Ondee, T., Pongpirul, K., Visitchanakun, P., Saisorn, W., Kanacharoen, S., Wongsaroj, L., et al. (2021). Lactobacillus acidophilus LA5 improves saturated fat-induced obesity mouse model through the enhanced intestinal akkermansia muciniphila. Sci. Rep. 11 (1), 6367. doi: 10.1038/s41598-021-85449-2
Otaegui-Arrazola, A., Amiano, P., Elbusto, A., Urdaneta, E., Martínez-Lage, P. (2014). Diet, cognition, and alzheimer's disease: food for thought. Eur. J. Nutr. 53 (1), 1–23. doi: 10.1007/s00394-013-0561-3
Ou, Z., Deng, L., Lu, Z., Wu, F., Liu, W., Huang, D., et al. (2020). Protective effects of akkermansia muciniphila on cognitive deficits and amyloid pathology in a mouse model of alzheimer's disease. Nutr. Diabetes 10 (1), 12. doi: 10.1038/s41387-020-0115-8
Park, D.-Y., Ahn, Y.-T., Park, S.-H., Huh, C.-S., Yoo, S.-R., Yu, R., et al. (2013). Supplementation of lactobacillus curvatus HY7601 and lactobacillus plantarum KY1032 in diet-induced obese mice is associated with gut microbial changes and reduction in obesity. PloS One 8 (3), e59470. doi: 10.1371/journal.pone.0059470
Patterson, E., Ryan, P. M., Wiley, N., Carafa, I., Sherwin, E., Moloney, G., et al. (2019). Gamma-aminobutyric acid-producing lactobacilli positively affect metabolism and depressive-like behaviour in a mouse model of metabolic syndrome. Sci. Rep. 9 (1), 16323. doi: 10.1038/s41598-019-51781-x
Pelsser, L. M., Frankena, K., Toorman, J., Rodrigues Pereira, R. (2017). Diet and ADHD, reviewing the evidence: a systematic review of meta-analyses of double-blind placebo-controlled trials evaluating the efficacy of diet interventions on the behavior of children with ADHD. PloS One 12 (1), e0169277. doi: 10.1371/journal.pone.0169277
Pirbaglou, M., Katz, J., de Souza, R. J., Stearns, J. C., Motamed, M., Ritvo, P. (2016). Probiotic supplementation can positively affect anxiety and depressive symptoms: a systematic review of randomized controlled trials. Nutr. Res. 36 (9), 889–898. doi: 10.1016/j.nutres.2016.06.009
Pistell, P. J., Morrison, C. D., Gupta, S., Knight, A. G., Keller, J. N., Ingram, D. K., et al. (2010). Cognitive impairment following high fat diet consumption is associated with brain inflammation. J. Neuroimmunol 219 (1-2), 25–32. doi: 10.1016/j.jneuroim.2009.11.010
Pocock, J. M., Kettenmann, H. (2007). Neurotransmitter receptors on microglia. Trends Neurosci. 30 (10), 527–535. doi: 10.1016/j.tins.2007.07.007
Pontes, K., Guedes, M. R., Cunha, M., Mattos, S., Barreto Silva, M. I., Neves, M. F., et al. (2021). Effects of probiotics on body adiposity and cardiovascular risk markers in individuals with overweight and obesity: a systematic review and meta-analysis of randomized controlled trials. Clin. Nutr. 40 (8), 4915–4931. doi: 10.1016/j.clnu.2021.06.023
Proctor, C., Thiennimitr, P., Chattipakorn, N., Chattipakorn, S. C. (2017). Diet, gut microbiota and cognition. Metab. Brain Dis. 32 (1), 1–17. doi: 10.1007/s11011-016-9917-8
Qian, X.-H., Song, X.-X., Liu, X.-L., S-d, C., Tang, H.-D. (2021). Inflammatory pathways in alzheimer's disease mediated by gut microbiota. Ageing Res. Rev. 68, 101317. doi: 10.1016/j.arr.2021.101317
Ren, Z., Li, Y., Li, X., Shi, H., Zhao, H., He, M., et al. (2021). Associations of body mass index, waist circumference and waist-to-height ratio with cognitive impairment among Chinese older adults: based on the CLHLS. J. Affect. Disord. 295, 463–470. doi: 10.1016/j.jad.2021.08.093
Rhea, E. M., Salameh, T. S., Logsdon, A. F., Hanson, A. J., Erickson, M. A., Banks, W. A. (2017). Blood-brain barriers in obesity. AAPS J. 19 (4), 921–930. doi: 10.1208/s12248-017-0079-3
Ridaura, V. K., Faith, J. J., Rey, F. E., Cheng, J., Duncan, A. E., Kau, A. L., et al. (2013). Gut microbiota from twins discordant for obesity modulate metabolism in mice. Science 341 (6150), 1241214. doi: 10.1126/science.1241214
Rogers, G. B., Keating, D. J., Young, R. L., Wong, M. L., Licinio, J., Wesselingh, S. (2016). From gut dysbiosis to altered brain function and mental illness: mechanisms and pathways. Mol. Psychiatry 21 (6), 738–748. doi: 10.1038/mp.2016.50
Rolig, A. S., Mittge, E. K., Ganz, J., Troll, J. V., Melancon, E., Wiles, T. J., et al. (2017). The enteric nervous system promotes intestinal health by constraining microbiota composition. PloS Biol. 15 (2), e2000689. doi: 10.1371/journal.pbio.2000689
Saji, N., Niida, S., Murotani, K., Hisada, T., Tsuduki, T., Sugimoto, T., et al. (2019). Analysis of the relationship between the gut microbiome and dementia: a cross-sectional study conducted in Japan. Sci. Rep. 9 (1), 1008. doi: 10.1038/s41598-018-38218-7
Sellbom, K. S., Gunstad, J. (2012). Cognitive function and decline in obesity. J. Alzheimers Dis. 30 Suppl 2, S89–S95. doi: 10.3233/JAD-2011-111073
Shi, H., Yu, Y., Lin, D., Zheng, P., Zhang, P., Hu, M., et al. (2020). β-glucan attenuates cognitive impairment via the gut-brain axis in diet-induced obese mice. Microbiome. 8 (1), 143. doi: 10.1186/s40168-020-00920-y
Slyepchenko, A., Carvalho, A. F., Cha, D. S., Kasper, S., McIntyre, R. S. (2014). Gut emotions - mechanisms of action of probiotics as novel therapeutic targets for depression and anxiety disorders. CNS Neurol. Disord. Drug Targets. 13 (10), 1770–1786. doi: 10.2174/1871527313666141130205242
Suganya, K., Koo, B.-S. (2020). Gut-brain axis: role of gut microbiota on neurological disorders and how Probiotics/Prebiotics beneficially modulate microbial and immune pathways to improve brain functions. Int. J. Mol. Sci. 21 (20), 7551. doi: 10.3390/ijms21207551
Telesford, K. M., Yan, W., Ochoa-Reparaz, J., Pant, A., Kircher, C., Christy, M. A., et al. (2015). A commensal symbiotic factor derived from bacteroides fragilis promotes human CD39(+)Foxp3(+) T cells and treg function. Gut Microbes 6 (4), 234–242. doi: 10.1080/19490976.2015.1056973
Torres-Fuentes, C., Schellekens, H., Dinan, T. G., Cryan, J. F. (2017). The microbiota-gut-brain axis in obesity. Lancet Gastroenterol. Hepatol. 2 (10), 747–756. doi: 10.1016/S2468-1253(17)30147-4
Turnbaugh, P. J., Bäckhed, F., Fulton, L., Gordon, J. I. (2008). Diet-induced obesity is linked to marked but reversible alterations in the mouse distal gut microbiome. Cell Host Microbe 3 (4), 213–223. doi: 10.1016/j.chom.2008.02.015
Turnbaugh, P. J., Ley, R. E., Mahowald, M. A., Magrini, V., Mardis, E. R., Gordon, J. I. (2006). An obesity-associated gut microbiome with increased capacity for energy harvest. Nature 444 (7122), 1027–1031. doi: 10.1038/nature05414
Ukena, S. N., Singh, A., Dringenberg, U., Engelhardt, R., Seidler, U., Hansen, W., et al. (2007). Probiotic escherichia coli nissle 1917 inhibits leaky gut by enhancing mucosal integrity. PloS One 2 (12), e1308. doi: 10.1371/journal.pone.0001308
Valdearcos, M., Douglass, J. D., Robblee, M. M., Dorfman, M. D., Stifler, D. R., Bennett, M. L., et al. (2017). Microglial inflammatory signaling orchestrates the hypothalamic immune response to dietary excess and mediates obesity susceptibility. Cell Metab. 26 (1), 185-197.e3. doi: 10.1016/j.cmet.2017.05.015
Vallianou, N., Stratigou, T., Christodoulatos, G. S., Dalamaga, M. (2019). Understanding the role of the gut microbiome and microbial metabolites in obesity and obesity-associated metabolic disorders: current evidence and perspectives. Curr. Obes. Rep. 8 (3), 317–332. doi: 10.1007/s13679-019-00352-2
Vogelzangs, N., Beekman, A. T. F., Boelhouwer, I. G., Bandinelli, S., Milaneschi, Y., Ferrucci, L., et al. (2011). Metabolic depression: a chronic depressive subtype? findings from the InCHIANTI study of older persons. J. Clin. Psychiatry 72 (5), 598–604. doi: 10.4088/JCP.10m06559
Vogt, N. M., Romano, K. A., Darst, B. F., Engelman, C. D., Johnson, S. C., Carlsson, C. M., et al. (2018). The gut microbiota-derived metabolite trimethylamine n-oxide is elevated in alzheimer's disease. Alzheimers Res. Ther. 10 (1), 124. doi: 10.1186/s13195-018-0451-2
Wang, S., Ahmadi, S., Nagpal, R., Jain, S., Mishra, S. P., Kavanagh, K., et al. (2020). Lipoteichoic acid from the cell wall of a heat killed lactobacillus paracasei D3-5 ameliorates aging-related leaky gut, inflammation and improves physical and cognitive functions: from c. elegans to mice. Geroscience 42 (1), 333–352. doi: 10.1007/s11357-019-00137-4
Wang, D., Doestzada, M., Chen, L., Andreu-Sánchez, S., van den Munckhof, I. C. L., Augustijn, H. E., et al. (2021). Characterization of gut microbial structural variations as determinants of human bile acid metabolism. Cell Host Microbe 29 (12), 1802–1814.e5. doi: 10.1016/j.chom.2021.11.003
Wang, H., Zhang, M., Li, J., Liang, J., Yang, M., Xia, G., et al. (2022). Gut microbiota is causally associated with poststroke cognitive impairment through lipopolysaccharide and butyrate. J. Neuroinflammation 19 (1), 76. doi: 10.1186/s12974-022-02435-9
Webberley, T. S., Masetti, G., Bevan, R. J., Kerry-Smith, J., Jack, A. A., Michael, D. R., et al. (2022). The impact of probiotic supplementation on cognitive, pathological and metabolic markers in a transgenic mouse model of alzheimer's disease. Front. Neurosci. 16, 843105. doi: 10.3389/fnins.2022.843105
Wong, J. M. W., de Souza, R., Kendall, C. W. C., Emam, A., Jenkins, D. J. A. (2006). Colonic health: fermentation and short chain fatty acids. J. Clin. Gastroenterol. 40 (3), 235–243. doi: 10.1097/00004836-200603000-00015
Xu, P., Becker, H., Elizalde, M., Masclee, A., Jonkers, D. (2018). Intestinal organoid culture model is a valuable system to study epithelial barrier function in IBD. Gut 67 (10), 1905–1906. doi: 10.1136/gutjnl-2017-315685
Yang, X., Yu, D., Xue, L., Li, H., Du, J. (2020). Probiotics modulate the microbiota-gut-brain axis and improve memory deficits in aged SAMP8 mice. Acta Pharm. Sin. B. 10 (3), 475–487. doi: 10.1016/j.apsb.2019.07.001
Yang, Y., Zhong, Z., Wang, B., Xia, X., Yao, W., Huang, L., et al. (2019). Early-life high-fat diet-induced obesity programs hippocampal development and cognitive functions via regulation of gut commensal akkermansia muciniphila. Neuropsychopharmacology 44 (12), 2054–2064. doi: 10.1038/s41386-019-0437-1
Zaydi, A. I., Lew, L. C., Hor, Y. Y., Jaafar, M. H., Chuah, L. O., Yap, K. P., et al. (2020). Lactobacillus plantarum DR7 improved brain health in aging rats via the serotonin, inflammatory and apoptosis pathways. Benef Microbes 11 (8), 753–766. doi: 10.3920/BM2019.0200
Zeltser, N., Meyer, I., Hernandez, G. V., Trahan, M. J., Fanter, R. K., Abo-Ismail, M., et al. (2020). Neurodegeneration in juvenile Iberian pigs with diet-induced nonalcoholic fatty liver disease. Am. J. Physiol. Endocrinol. Metab. 319 (3), E592–E606. doi: 10.1152/ajpendo.00120.2020
Zhang, Y., Huang, B., Yang, W., Zhong, S., Lai, S., Zhao, H., et al. (2022). Correlations between endocrine hormones and cognitive function in patients with obesity: a cross-sectional study. Obes. Surg. 32 (7), 2299–2308. doi: 10.1007/s11695-022-06076-y
Zheng, H., Xu, P., Jiang, Q., Xu, Q., Zheng, Y., Yan, J., et al. (2021). Correction to: depletion of acetate-producing bacteria from the gut microbiota facilitates cognitive impairment through the gut-brain neural mechanism in diabetic mice. Microbiome 9 (1), 164. doi: 10.1186/s40168-021-01127-5
Keywords: probiotics, obesity, gut-brain axis, cognitive function, microbiota
Citation: Cai Y, Liu P, Zhou X, Yuan J and Chen Q (2023) Probiotics therapy show significant improvement in obesity and neurobehavioral disorders symptoms. Front. Cell. Infect. Microbiol. 13:1178399. doi: 10.3389/fcimb.2023.1178399
Received: 04 March 2023; Accepted: 24 April 2023;
Published: 12 May 2023.
Edited by:
Zhengwei Huang, Shanghai Jiao Tong University, ChinaReviewed by:
Xiaoshuang Dai, Beijing Genomics Institute (BGI), ChinaCopyright © 2023 Cai, Liu, Zhou, Yuan and Chen. This is an open-access article distributed under the terms of the Creative Commons Attribution License (CC BY). The use, distribution or reproduction in other forums is permitted, provided the original author(s) and the copyright owner(s) are credited and that the original publication in this journal is cited, in accordance with accepted academic practice. No use, distribution or reproduction is permitted which does not comply with these terms.
*Correspondence: Qiu Chen, Y2hlbnFpdTEwMDVAY2R1dGNtLmVkdS5jbg==
Disclaimer: All claims expressed in this article are solely those of the authors and do not necessarily represent those of their affiliated organizations, or those of the publisher, the editors and the reviewers. Any product that may be evaluated in this article or claim that may be made by its manufacturer is not guaranteed or endorsed by the publisher.
Research integrity at Frontiers
Learn more about the work of our research integrity team to safeguard the quality of each article we publish.