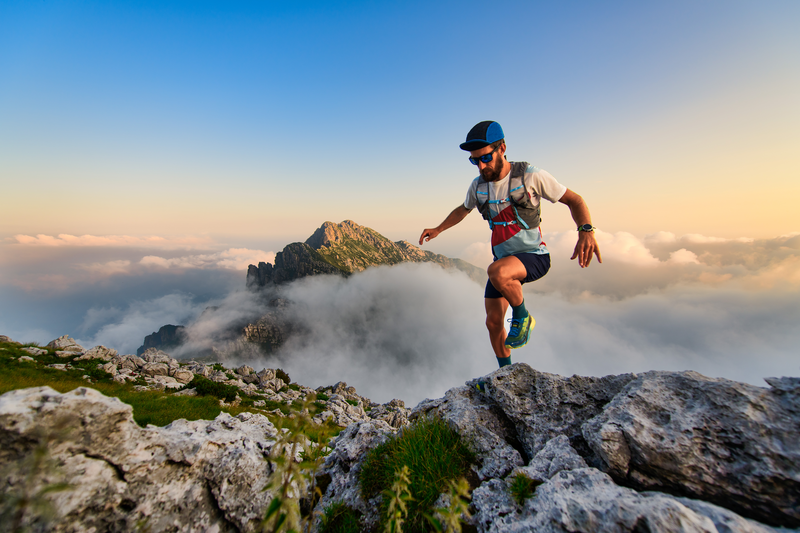
94% of researchers rate our articles as excellent or good
Learn more about the work of our research integrity team to safeguard the quality of each article we publish.
Find out more
REVIEW article
Front. Cell. Infect. Microbiol. , 06 June 2023
Sec. Microbiome in Health and Disease
Volume 13 - 2023 | https://doi.org/10.3389/fcimb.2023.1169500
This article is part of the Research Topic Reviews in Microbiome in Health & Disease View all 13 articles
Kidney transplantation is an effective method to improve the condition of patients with end-stage renal disease. The gut microbiota significantly affects the immune system and can be used as an influencing factor to change the prognoses of patients who have undergone kidney transplantation. Recipients after kidney transplantation showed a lower abundance of Firmicutes and Faecalibacterium prausnitzii and a higher proportion of Bacteroidetes and Proteobacteria. After using prebiotics, synbiotics, and fecal microbiota transplantation to regulate the microbial community, the prognoses of patients who underwent kidney transplantation evidently improved. We aimed to determine the relationship between gut microbiota and various postoperative complications inpatients who have undergone kidney transplantation in recent years and to explore how gut microecology affects post-transplant complications. An in-depth understanding of the specific functions of gut microbiota and identification of the actual pathogenic flora during complications in patients undergoing kidney transplantation can help physicians develop strategies to restore the normal intestinal microbiome of transplant patients to maximize their survival and improve their quality of life.
Kidney transplantation is among the most effective treatments for end-stage kidney diseases compared with conventional therapies. Kidney transplantation was first introduced in the 1950s (Meena et al., 2021), and since then, it has drastically improved the survival rates of patients with renal complications (Hariharan et al., 2000). Transplantation can cause various medical complications ranging from mild symptoms to life-threatening conditions (Adams, 2006). Herrington et al. (2019) revealed that cardiovascular disease (31% of deaths), infection (31%), and cancer (7%) were the leading causes of death following graft function in the first year after transplantation (Herrington et al., 2019). In the first year after kidney transplantation, the leading causes of death include cancer (29%), cardiovascular disease (23%), and infection (12%) (Hariharan et al., 2021). Furthermore, the high incidence of complications such as diarrhea, infection, and graft rejection after transplantation is a persistent challenge (Chong and Alegre, 2012; Mori et al., 2014). The administration of immunosuppressant drugs after transplantation, including tacrolimus and mycophenolate mofetil, is a primary strategy for preventing graft rejection (Tonshoff, 2020). It increases the likelihood of survival but concurrently results in a greater risk of complications after transplantation. Therefore, the development of new and feasible therapies that can attenuate the severity of complications after transplantation is necessary.
Contrary to expectations, substantial evidence has revealed that the adverse reactions observed after kidney transplantation are closely related to the gut microbiota of patients. The human body contains approximately 100 trillion microbial cells, including bacterial, archaeal, viral, and eukaryotic microbial communities, which directly inhabit the interface of the human body that is exposed to or connected to the external environment (Dominguez-Bello et al., 2019; Eng and Borenstein, 2019). Microorganisms play major roles in human health and can directly or indirectly affect human physiology in various ways (Dominguez-Bello et al., 2019). They can guide immune cells to rapidly and effectively respond to pathogenic invasion, simultaneously promote the completion of various metabolic functions, and affect most physiological functions via these basic functions (Gomaa, 2020).
Recently, it has been reported that gut microbiota dysregulation adversely affects the prognoses of patients undergoing kidney transplantation (Xu et al., 2017). Increasing evidence has indicated the presence of a bidirectional relationship between gut microbes and nephropathy (Anders et al., 2013; Rukavina et al., 2020). Gut microbes aid in rebuilding the host–microbiome symbiosis, which can help alleviate kidney disease to a certain extent (Al Khodor and Shatat, 2017). An increasing number of scholars and research teams are investigating the relationship between gut microbiota and complications after kidney transplantation, refining the research on this topic (Figure 1). However, current research and reviews have focused only on specific types of complications. In this article, we discuss the evidence regarding gut microbiota from a more comprehensive perspective to evaluate the relationship between gut microbiota and various kidney-related complications.
Figure 1 Clustering correlation between kidney transplantation and gut microbiota in published literature. (A) Analytical, diagnostic, and therapeutic techniques and equipment categories. (B) Phenomena and processes categories. (C) Diseases category. (D) Organisms category.
The gut microbiome is an important but “invisible organ” in humans (Khanna and Tosh, 2014). Although this “organ” is not composed of actual organs, it is distributed throughout the body, with the intestine being the primary point of distribution (Gomaa, 2020). The total length of the gut is 175 m, leading to differences in the geographical distribution of gut microbiota in humans. The most abundant bacteria in the human gut are Firmicutes, Escherichia, and Bacteroidetes, which account for 95% of the total normal flora in healthy individuals; the remaining 5% include Clostridium, Actinobacteria, and other microbiota (Eckburg et al., 2005). Gut microbiota are among the most important components of the body and are related to various physiological conditions.
Gut microbiota, widely known as the mainstay of the digestive system in the body, were once considered to constitute an invisible organ with a metabolic capacity exceeding that of the liver (Nallu et al., 2017). In glycolipid metabolism, gut microbiota produce metabolites of short-chain fatty acids (colonic fiber fermentation), including acetate, propionate, and butyrate (Cummings et al., 1987), which positively contribute to the health and functioning of various systems. Along with mediating metabolic functions, the gut and microbiota, which collectively function as endogenous organs, play various physiological roles in the host, such as roles in liver damage, cardiovascular disease, sleep regulation, and circadian rhythms (Matenchuk et al., 2020), forming the microbe–gut–brain axis (Quigley, 2017), which maintains intestinal mucosal immunity (Shi et al., 2017). This suggests that gut microbiota dysbiosis is not only associated with digestive system diseases but also with other systemic diseases, such as obesity (Abenavoli et al., 2019), diabetes (Wu et al., 2020), and cardiovascular diseases (Witkowski et al., 2020). Winichakoon et al. (2022) comprehensively summarized and discussed major findings from in vivo and clinical data pertaining to the effects of gut microbiota on kidney transplantation (Winichakoon et al., 2022).
Kidney transplantation has been the gold standard renal replacement therapy for end-stage renal disease (ESRD) because it leads to superior survival and quality of life among patients compared with other replacement therapies (Wolfe et al., 1999). This has been consistently reported since the first successful kidney transplantation between identical twins in the 1950s (Force and Andreu, 2005). Transplantation and dialysis are the primary treatments for ESRD and these two treatments can significantly prolong a patient’s lifespan and improve their quality of life. According to statistics, patients aged 20–39 years survived for 8 years on dialysis and 25 years after transplantation (Augustine, 2018). In the United States, the 5-year survival rates of patients who underwent primary kidney transplantations from deceased and living donors were 72% and 85% (Hariharan et al., 2021), respectively, which were slightly different from those in Australia (81% and 90%), Europe (79% and 87%), and Canada (81% and 91%) (Wang et al., 2016). Compared to dialysis, kidney transplantation not only increases life expectancy but also improves the quality of life of several patients with ESRD. It also reduces the costs incurred due to other chronic treatments.
As noted earlier, after kidney transplantation, patients suffer from complications, such as cardiovascular disease (31% of deaths), infections (31% of deaths), and cancer (7% of deaths) (Hariharan et al., 2021). To reduce complications, patients undergoing kidney transplantation are chronically administered immunosuppressive agents to suppress the immune response after allogeneic kidney implantation. This inhibitory effect and the body’s autoimmune response to constantly reject foreign bodies are key determinants of transplantation (Ranganathan et al., 2006). Although these complications are not life-threatening, they greatly reduce the patients’ quality of life and affect their prognostic statuses. These complications differ from acute rejection, viral infection, cancer, and other uncontrollable risk factors and are closely related to the body’s immunity. A delicate balance exists between the two, and if this balance is disrupted because of other factors, patients undergoing kidney transplantation experience postoperative complications including infection and transplant rejection. Studies have revealed that the homeostasis of the gut microbiota significantly influences the immune system and can be used to alter the prognoses of patients undergoing kidney transplantation (Gioco et al., 2020).
The effects of dysbiosis that activate human immune responses, allograft rejection, and the pharmacokinetics of immunosuppressive drugs remain largely unknown (Anders et al., 2013) and may result in certain complications after transplantation such as the risk of infection (urinary infections and infectious diarrhea), adverse immune phenomena (autoimmune hemolytic anemia), transplant rejection, and increased mortality. Human and mouse studies have revealed that the abundance of the gut microbiota is significantly inversely associated with transplant prognosis, the abundance of microbial species, the number of donors and recipients, the estimated glomerular filtration rate within 6 months of transplantation, and species-differentiated microbiota are associated with an increased frequency of infection-related complications after transplantation. Thus, the presence of certain species in the gut before kidney transplantation is significantly associated with subsequent rejection. Therefore, the microbiota can affect the quality of life of patients after kidney transplantation (Ranganathan et al., 2006). Winichakoon et al. (2022) reported that the microbiota of an individual could alter the immune response of organ transplantation hosts via specific signaling pathways, such as the Myd88 and TLR9 pathways (Winichakoon et al., 2022).
Kim et al. (2020) reported that differences in the donor–recipient microbial community before transplantation can affect the function of early allografts, and this relationship can affect the incidence of infection within 6 months of transplantation. In genetically unrelated donor transplantations, unrelated individuals exhibited a more pronounced correlation between graft function and microbial similarity (Kim et al., 2020). Kim et al. (2020) suggested that the effects of unknown environmental and traditional genetic factors on the microbial community should be considered during post-transplantation management. Therefore, future studies investigating the relationship between gut microbiota and organ transplantation should consider sex, age, and ethnicity. Therefore, sex, age, and race should be considered when investigating the association between gut microbiota and organ transplantation.
Interestingly, we also found that various types of specific bacterial species were responsible for dysbiosis in patients undergoing kidney transplantation. Xiao et al. (2018) revealed that in densely populated areas in a multidimensional space composed of the same community (Xiao et al., 2018), the type and abundance of the human gut microbiota were similar. Arumugam et al. (2011) named this gut microbial composition “enterotype” (Arumugam et al., 2011; Costea et al., 2018) and found that its abundance was related to the age, sex, cultural background, and geographic location of individuals. In a study cohort, Costea et al. (2018) categorized enterotypes into three types and found that Bacteroidetes were dominant in Enterotype 1, Prevotella in Enterotype 2, and Ruminococcus in Enterotype 3. These intestinal types directly affect intestinal homeostasis and are closely associated with various acute and chronic diseases. In summary, based on this theory, personalized treatment should be administered in different populations; that is, different treatment methods should be selected for patients with different intestinal types.
Notably, a negative relationship exists between gut microbiota and the kidney. After drugs modulate the gut microbiota, kidney disease progression significantly slows down. When probiotics such as Bacillus pasteuri and Lactobacillus sporogenes were used to treat a rat model of chronic kidney disease (CKD), blood urea nitrogen (BUN) levels significantly reduced (Ranganathan et al., 2005), and the use of urease-positive Pasteurella reduced intestinal BUN levels. The concentration of urea, which also improves urinary protein excretion, reduces the glomerular sclerosis index (Ranganathan et al., 2006; Yoshifuji et al., 2016). Based on the findings of clinical studies summarized in Table 1, gut microbiota profiles differ considerably based on the pre- and post-transplantation status, as observed in 16S ribosomal ribonucleic acid polymerase chain reaction experiments.
Table 1 Clinical studies on the profiles of gut microbiota in patients after kidney transplantation.
As early as 2009, Jang et al. (2009) used mice to confirm the important role of the microecology of gut microbiota in kidney disease. They revealed that germ-free mice bred via technical means could be used to monitor in vivo abnormalities under the same exposure. A large population of killer T cells and extremely low concentrations of interleukin (IL)-4 were observed. However, after the gut microbiota were reintroduced to establish a stable gut microbiome, the conditions of the mice showed significant improvement (Figure 2). Accumulating evidence suggests that after gut microbiota dysbiosis affects the intestinal mucosa and causes intestinal inflammation, it eventually causes systemic inflammation by activating the nuclear factor (NF)-κB pathway (Anders et al., 2013). These findings provide a basis for the existence of the gut–immune axis in vivo. In view of the findings of these studies, a method to rebuild the intestinal microbiota and stabilize intestinal microecology can be developed, which can be innovatively used for the prevention and treatment of kidney diseases.
When gut microbiota are disturbed, inflammatory reactions can be activated via the NF-KB pathway (Anders et al., 2013), the degree of cresol and IL-4 (Jang et al., 2009)in intestinal mucosa can be reduced, and the degree of urea nitrogen can be increased (Ranganathan et al., 2005), which will lead to kidney damage.
The presence of multiple factors in patients before, during, and/or after kidney transplantation can result in changes in the type and quantity of the microbiota, thereby destabilizing it (Jang et al., 2009; Yoshifuji et al., 2016). Some scientists have revealed that the gut microbiota in patients undergoing kidney transplantation who have undergone fecal microbiota transplantation (FMT) are highly similar to the type composition of the donor’s gut microbiota and will tend to gradually normalize (Ianiro et al., 2020). Wang et al. (2021a) revealed that in kidney transplantation recipients (RTRs), the dominant flora (Prevotella_9, relative abundance 35.9%) (Wang et al., 2021a) was Bacteroides (relative abundance 60.6%) after 2 months of FMT administration.
A plethora of evidence supports the idea that gut microbiota dysbiosis plays a vital role in the outcomes of organ transplantation, especially kidney transplantation. Modification of the gut microbiota in response to routine therapeutic approaches, such as the administration of immunosuppressive drugs and prophylactic antibiotics post transplantation (Kim and Song, 2020), may adversely affect graft outcomes, thus leading to graft rejection, infection, fibrosis, and alterations in drug metabolism. As summarized in Table 2, prebiotics, synbiotics, and FMT can transform a state of gut dysbiosis into colonization by healthy gut microbiota.
According to Guida et al. (2017), synbiotics can effectively lower plasma p-cresol levels in patients undergoing kidney transplantation. P-cresol generally accumulates when its production by the dysbiotic gut microbiome increases (Guida et al., 2017). In a study, 27 recipients of kidney transplantation with gastrointestinal symptoms received a prebiotic powder suspension with breakfast for 7 weeks (Chan et al., 2022). In this randomized placebo-controlled trial, prebiotics significantly suppressed gastrointestinal symptoms. In another study by Lin, three RTRs showed no further CDI recurrence or diarrheal symptoms during the follow-up period after receiving FMT treatment via colonoscopy (Lin et al., 2018) (Figure 3).
Figure 3 Schematic view of the link between gut microbiota and kidney transplantation complications. The red apples represent an upward trend in the abundance of gut microbiota. The green apples represent a downward trend in the abundance of gut microbiota.
Furthermore, clinical studies have revealed that probiotic supplementation effectively improves kidney function. Ranganathan et al. (2010) and other researchers administered probiotic strains such as Lactobacillus acidophilus, Lactobacillus acidophilus, and Streptococcus thermophilus to 46 patients with CKD III or IV and found that their renal function and quality of life significantly improved after probiotic intake for 6 months (Ranganathan et al., 2010). Grosen et al. (2019) reported a case wherein a patient suffered from urinary tract infections (UTIs) caused by broad-spectrum lactamase-producing (ESBL+) Klebsiella pneumoniae but showed no symptoms of UTI after FMT treatment (Grosen et al., 2019). In 2021, Wang et al. (2021a) presented a case report where carbapenem-resistant Klebsiella pneumoniae (CRKP) infection was treated using FMT in China (Wang et al., 2021a). After FMT, not only did the CRKP test of urine and anal swab cultures yield negative results but a greater relative abundance of Phascolarctobacterium and Lachnoclostridiumwas noted along with the depletion of Klebsiella.
Kim reported that gut microbiota profiles considerably vary between recipients and donors (Kim et al., 2020). Recipients exhibited lower levels of Prevotellaceae and Prevotella, whereas the opposite was observed in donors. Lee et al. (2015) revealed that in patients after kidney transplantation, a lower diversity of Firmicuteswas observed (Lee et al., 2015). Lee et al. (2019a) also reported lower relative abundances of Faecalibacterium prausnitzii, Holdemanella biformis, Eubacterium dolichum, Coprococcus comes, Subdoligranulum variabile, and Eubacterium rectale (Lee et al., 2019a). Swarte et al. (2020) reported increased proportions of Firmicutes and Proteobacteria in 139 kidney transplant recipients and 105 healthy controls (Swarte et al., 2020). Fricke et al. (2014) reported that increased Actinobacteria and Firmicutes abundance is associated with an increased risk of graft dysfunction, as identified by an increase in chromium concentration after kidney transplantation (Fricke et al., 2014). Zaza et al. (2017) revealed increased abundances of Actinobacteria, Firmicutes, and Proteobacteria in patients who were administered different immunosuppressive drug regimens after kidney transplantation (Zaza et al., 2017). Contrary to expectations, Guirong et al. (2018) reported a decrease in Firmicutes abundance instead of an increase in recipients of kidney transplants (Guirong et al., 2018). Chan et al. (2021) classified Firmicutes into two types and reported a significantly greater abundance of Firmicutes A and a relatively lower abundance of Firmicutes G (Chan et al., 2021). This may explain the contradictory results reported by different studies.
Diarrhea after transplantation is a common complication observed after solid organ transplantation (Zhang et al., 2021), and a large registry analysis identified that diarrhea after transplantation negatively affects graft and patient survival (Aulagnon et al., 2014). Diarrhea is generally considered to exert a mild impact on patients’ quality of life, but severe episodes have a significant impact on drug metabolism and the prognoses and survival of patients (Zhang et al., 2021), such as a drastic decline in the functionality of the transplanted kidney or a significant increase in patient mortality (Ekberg et al., 2007). According to Medicare claims registered with the United Network for Organ Sharing, diarrhea occurs in 20%–50% of solid organ transplant recipients in the first year after transplantation (Angarone and Snydman, 2019), with an incidence of diarrhea after kidney transplantation of 11.5% and the cumulative incidences of diarrhea at 2 and 3 years of 17.5% and 22.6% (Bunnapradist et al., 2008), respectively.
Along with certain dietary elements, antibiotic use, the induction of transplant-specific lymphatic failure (Li et al., 2013), and immune rejection also contribute to an imbalance in the gut microbiota. Some studies have revealed that only a few patients who harbored gastrointestinal pathogens during transplantation developed diarrhea after transplantation, and only a few patients exhibited the same pathogenic flora colonizing the gastrointestinal tract before and after transplantation (Westblade et al., 2019). Diarrhea can be induced by various factors. Bunnapradist et al. (2006) showed that tacrolimus and mycophenolate mofetil increase the risk of noninfectious diarrhea, graft loss, and patient death (Bunnapradist et al., 2006). Therefore, pathogens that cause diarrhea in the gastrointestinal tract are more likely to colonize and cause chronic infections in the body, forging a relationship with the progression of diarrhea after transplantation. Study findings have further indicated that gut microbiota can be isolated from diarrheal stool samples, with reduced microbial diversity and decreased abundance of commensal bacterial species. Most cases of diarrhea after transplantation are different from common infectious diarrhea, which is related to intestine-related dysbiosis. Patients undergoing kidney transplantation show a greater abundance of Proteobacteria than healthy individuals (Arslan et al., 2007). After kidney transplantation, patients are more susceptible to opportunistic pathogens than immunocompromised healthy individuals (Aulagnon et al., 2014).
Interestingly, Westblade et al. (2019) revealed that in the first 3 months after transplantation, 47 (33%) of 142 kidney transplant recipients developed diarrhea after transplantation, of which 24 recipients exhibited extensive microbial colonization (Westblade et al., 2019). Lee et al. (2014) reported differences in the diversity of the gut microbiota between healthy individuals and kidney transplant recipients. The latter exhibited an increase in Enterococcus, Escherichia, and Lachnoclostridiumand a decrease in Eubacterium, Anaerostipes, Coprococcus, Romboutsia, Ruminococcus, Dorea, Faecalibacterium, Fusicatenibacter, Oscillibacter, Ruminiclostridium, Blautia, Bifidobacterium, and Bacteroides. Lee et al. (2014) also reported that an increase in Faecalibacterium abundance was positively correlated with future tacrolimus dosing at 1 month (Lee et al., 2014). Swarte et al. (2020) reported an increasing trend in the abundance of Proteobacteria and a lower abundance of Faecalibacterium prausnitzii, Gemmiger formicilis, Eubacterium rectale, Coprococcus catus, Coprococcus comes, and Roseburia (Swarte et al., 2020). This study also reported the depletion of butyrate-producing bacteria caused by antibiotics and immunosuppressive agents and this was considered to be associated with diarrhea after kidney transplant. Wei et al. (2019) reported increased abundance of Lachnospiraceae, Ruminococcaceae, and Veillonellaceae, and decreased abundance of Proteobacteria, Enterobacteriaceae, and Alcaligenaceae in a 32-year-old kidney transplant recipient. These findings reveal that butyrate-producing bacteria are lost in RTRs (Flint et al., 2015). Diarrhea after transplantation was not associated with common infectious diarrheal pathogens but with dysbiosis.
Norovirus has been attributed to approximately 25% of cases with diarrhea after transplantation. Clostridium difficile infection occurs in 2%–7% of kidney transplant recipients (Chan et al., 2022). Evidence from several studies has suggested that C. difficile also causes nosocomial infections that patients contract within the first few months after transplantation (Guida et al., 2017; Lin et al., 2018). Kujawa-Szewieczek et al. (2015) attempted to use Lactobacillus plantarum 299v as a preventive agent against this infection in patients receiving antibiotics and observed that probiotics could efficiently prevent C. difficile infection in RTRs (Kujawa-Szewieczek et al., 2015). Lee et al. (2019b) also reported that some patients continued to experience diarrhea despite repeated monitoring tests for C. difficile before FMT, which yielded negative results. The authors also revealed that the abundance of Ruminococcus significantly decreased in samples collected after the onset of diarrhea symptoms (Lee et al., 2019b) compared to samples collected before diarrhea. A summary of these findings is provided in Table 3.
Table 3 Association between gut microbiota and diarrhea in kidney transplantation recipients: evidence from clinical studies.
Modulation of the gut microbiota profile via FMT or administration of probiotics has positively affected RTRs. Aulagnon et al. (2014) reported the clinical characteristics of a patient with chronic diarrhea after kidney transplantation (Aulagnon et al., 2014). After Masson’s trichrome staining, the intracellular accumulation of calcium oxalate monohydrate was microscopically observed, and the deposition of massive crystals disrupted the tubular structure, thus inducing inflammation and fibrosis in the scarred area. Gu et al. (2018) showed that diarrhea symptoms in patients undergoing kidney transplantation improved after FMT (Gu et al., 2018). Zhang et al. (2021) recently revealed that fecal β-glucuronidase activity could serve as a novel biomarker for gastrointestinal tract-related mycophenolate mofetil toxicity.
UTI is an infection primarily caused by bacteria invading urothelial cells, inducing an immune response in the body to resist infection (Fiorentino et al., 2019). The incidence of kidney transplantation is considerably higher in patients with UTIs than in the general population (Alangaden et al., 2006; Rice and Safdar, 2009; Karuthu and Blumberg, 2012). Asymptomatic bacteriuria constitutes 44% of UTI cases, whereas uncomplicated and complicated UTI account for 32% and 24% of the cases, respectively. Patients undergoing kidney transplantation account for approximately 40%–50% of all infectious complications (Meena et al., 2021), with bacteriuria detectable in approximately 40% of patients within the first year (Magruder et al., 2019). Furthermore, nearly 50% of UTI cases occur within 3 years of transplantation. UTIs not only affect patients’ quality of life but also increase the risk of other complications in transplant recipients, which affect long-term graft survival and rejection (Castaneda et al., 2013; de Castro Rodrigues Ferreira et al., 2017).
Fiorante et al. (2010) reported that asymptomatic bacteriuria alone was associated with a seven-fold increased risk of pyelonephritis in a population of patients undergoing kidney transplantation (Fiorante et al., 2010), and each episode of UTI that occurred after the transplantation reduced graft function to some extent (Fiorentino et al., 2019). In a retrospective study, approximately 1%–3% of patients with UTIs developed bacteremia, and >20,000 kidney transplant recipients developed UTIs 6 months after transplantation (Lee et al., 2013), which significantly increased the risk of death and graft loss. Recent studies support the role of gut microbiota in UTI pathogenesis, with Enterobacteriaceae being the most common cause of UTI after transplantation (Lee et al., 2013). In a study of nontransplantation patients, children with Escherichia coli UTIs exhibited greater gut E. coli abundance than other children. In another experiment including kidney transplant recipients, the identification of urine strains revealed that the E. coli present in urine was genetically similar to the E. coli present in stool samples collected from the same participants, supporting the notion that gut microbiota dysbiosis is the primary source of UTIs (Magruder et al., 2020). Magruder et al. (2020) showed that RTRs with UTIs experienced the same shifts in microbial diversity, with an increased abundance of Firmicutes and Proteobacteria (Magruder et al., 2019; Magruder et al., 2020). A summary of these findings is provided in Table 4.
Table 4 Association between gut microbiota and urinary tract infection in kidney transplantation recipients: evidence from clinical studies.
Interestingly, some patients with UTI after kidney transplantation show improvements in clinical symptoms and urinary sensation without antibiotics. A preliminary speculation may be related to the body’s response to acute infection. This implies that unconventional methods can be used to stabilize the type and abundance of microbiota. Contrary to expectations, Biehl reported that FMT (via frozen capsulized microbiota) improved UTI symptoms in kidney transplant recipients (Biehl et al., 2018).
Gram-negative bacteria are responsible for the occurrence of 70% of UTIs in RTRs, with E. coli accounting for 30%–80% of kidney transplantation-related UTIs (Valera et al., 2006; Pelle et al., 2007). Klebsiella, Pseudomonas aeruginosa, other gram-negative bacteria, and Proteus are common pathogens that cause UTIs. When gut microbiota invade the body to induce UTI or bacteriuria, the bacteria continuously invade the urothelium by producing virulence factors and specific adhesins on the surface of the bacterial membrane to stimulate urothelial cells, which produce pro-inflammatory factors, such as IL-8. These factors promote the migration of neutrophils to infected urothelial cells and facilitate inflammatory immune responses (Fiorentino et al., 2019). Modulation of gut microbiota may play a role in the treatment of UTI in RTRs.
Thirty-nine percent of patients experience at least one acute rejection within 1 year of transplantation (Carron et al., 2019). Acute rejection can lead to graft failure within a short duration (Kidney Disease: Improving Global Outcomes (KDIGO) Transplant Work Group, 2009).It can also lead to a greater three-fold risk of graft glomerular disease and loss of graft function in patients within 1 year of transplantation than in healthy controls (Gloor et al., 2007). Gut microbiota have been associated with the occurrence of acute rejection, and the relative abundances of Lactobacillus, Enterococcus, anaerobic bacteria, and Clostridium termites were found to be higher in patients with acute rejection, whereas those of Clostridium, Bacteroides, Cyanobacteria, and Lachnospirawere lower (Lee et al., 2014). Wang revealed that 29 recipients of kidney transplantation (including 24 acute rejection recipients) reported a risk of graft rejection in response to altered proportions of Firmicutes and Bacteroidetes compared to those of Proteobacteria, Actinobacteria, Lactobacillales, Clostridia, and Faecalibacterium, with the abundances of the former decreasing and that of the latter increasing (Wang et al., 2021b). Carron et al. (2019) reported that the concentrations of circulating sCD14 and intestinal fatty acid-binding protein (iFABP) inversely correlated with a stronger prompt for acute rejection after transplantation in 788 recipients of kidney transplantation (Carron et al., 2019). A summary of these findings is provided in Table 5.
Table 5 Association between gut microbiota and acute rejection in kidney transplantation recipients: evidence from clinical studies.
Some scholars have studied how the gut microbiota can cause acute rejection and proposed that if patients with ESRD maintain a stable total lipopolysaccharide (LPS) concentration for a long duration and a state of low LPS activity, the level of inflammatory biomarkers in patients undergoing kidney transplantation can be improved 1 year after transplantation. This could help protect patients who have undergone kidney transplantation from acute rejection (Carron et al., 2019). Authors have also proposed the observation of an intestinal epithelial cell damage marker, iFABP (Piton and Capellier, 2016), to assess the integrity of the intestinal epithelial barrier and the measurement of inflammatory biomarkers (sCD14 and cytokines) concurrently to explore the pathways and mechanisms underlying LPS elimination (Lee et al., 2014) and body-induced acute immune rejection.
The gut microbiota exerts certain regulatory effects on various organ systems, and kidney-related diseases are no exception. An increasing number of individuals are now focusing on the bidirectional relationship between gut microbiota and kidney transplantation complications. Low kidney function can result in intestinal microbiota imbalance, and an imbalance in the microecological environment can further accelerate the progression of complications in kidney transplant recipients. However, prophylactic drugs can be administered to regulate the microbiota of patients undergoing kidney transplantation to reduce the occurrence and progression of subsequent complications. However, this question does not yet have a definitive answer.
The results of the human microbiome project are still being reported, and the roles of treatments with prebiotics and gut microbiota transplantation in human health and disease prevention remain unclear. However, it is conceivable that in the near future, we will use probiotics or other drugs that regulate gut microecology instead of traditional drugs that exert significant adverse effects. A clinical team used probiotics for the postoperative treatment of patients undergoing kidney transplantation and reported a significant decrease in the incidence of diarrhea and intestinal complications. However, the number of test groups was small, and the results are not generally representative. More basic clinical studies are required to explore this topic.
It is necessary to evaluate whether this preventive treatment can accurately control the patient’s condition and reduce the occurrence and progression of complications, and whether early microorganisms in the body interact with drugs including antibiotics and prebiotics. Conversely, Clostridiales has been reported as a potential mechanistic biomarker in different irritable bowel syndrome subtypes and represents a potential therapeutic target. We also identified a potential mechanistic biomarker that could be used as an early monitoring indicator of postoperative complications or disease development in patients undergoing kidney transplantation.
JXY, FFH, JS, ZZ, YMW and JJY drafted the manuscript, JXY, FFH, JS and ZZ wrote the manuscript. All authors read, edited and approved the final manuscript.
This work was supported by grants from the National Natural Science Foundation of China (No. 81570657 and No.81974097), Hubei Provincial Natural Science Foundation of China (No.2018CFB485).
The authors declare that the research was conducted in the absence of any commercial or financial relationships that could be construed as a potential conflict of interest.
All claims expressed in this article are solely those of the authors and do not necessarily represent those of their affiliated organizations, or those of the publisher, the editors and the reviewers. Any product that may be evaluated in this article, or claim that may be made by its manufacturer, is not guaranteed or endorsed by the publisher.
KTRs, kidney transplantation recipients; UTI, urinary tract infection; AR, acute rejection; LPS, lipopolysaccharide; iFABP, intestinal fatty acid-binding protein.
Abenavoli, L., Scarpellini, E., Colica, C., Boccuto, L., Salehi, B., Sharifi-Rad, J., et al. (2019). Gut microbiota and obesity: a role for probiotics. Nutrients 11 (11):2690. doi: 10.3390/nu11112690
Adams, P.-L. (2006). Long-term patient survival: strategies to improve overall health. Am. J. Kidney Dis. 47 (4 Suppl 2), S65–S85. doi: 10.1053/j.ajkd.2005.12.043
Alangaden, G.-J., Thyagarajan, R., Gruber, S.-A., Morawski, K., Garnick, J., El-Amm, J. M., et al. (2006). Infectious complications after kidney transplantation: current epidemiology and associated risk factors. Clin. Transplant. 20 (4), 401–409. doi: 10.1111/j.1399-0012.2006.00519.x
Al Khodor, S., Shatat, I.-F. (2017). Gut microbiome and kidney disease: a bidirectional relationship. Pediatr. Nephrol. 32 (6), 921–931. doi: 10.1007/s00467-016-3392-7
Anders, H.-J., Andersen, K., Stecher, B. (2013). The intestinal microbiota, a leaky gut, and abnormal immunity in kidney disease. Kidney Int. 83 (6), 1010–1016. doi: 10.1038/ki.2012.440
Angarone, M., Snydman, D.-R. (2019). Diagnosis and management of diarrhea in solid-organ transplant recipients: guidelines from the American society of transplantation infectious diseases community of practice. Clin. Transplant. 33 (9), e13550. doi: 10.1111/ctr.13550
Arslan, H., Inci, E.-K., Azap, O.-K., Karakayali, H., Torgay, A., Haberal, M., et al. (2007). Etiologic agents of diarrhea in solid organ recipients. Transpl Infect. Dis. 9 (4), 270–275. doi: 10.1111/j.1399-3062.2007.00237.x
Arumugam, M., Raes, J., Pelletier, E., Le Paslier, D., Yamada, T., Mende, D. R., et al. (2011). Enterotypes of the human gut microbiome. Nature 473 (7346), 174–180. doi: 10.1038/nature09944
Augustine, J. (2018). Kidney transplant: new opportunities and challenges. Cleve Clin. J. Med. 85 (2), 138–144. doi: 10.3949/ccjm.85gr.18001
Aulagnon, F., Scemla, A., DeWolf, S., Legendre, C., Zuber, J. (2014). Diarrhea after kidney transplantation: a new look at a frequent symptom. Transplantation 98 (8), 806–816. doi: 10.1097/TP.0000000000000335
Biehl, L.-M., Cruz, A. R., Farowski, F., Hahn, W., Nowag, A., Wisplinghoff, H., et al. (2018). Fecal microbiota transplantation in a kidney transplant recipient with recurrent urinary tract infection. Infection 46 (6), 871–874. doi: 10.1007/s15010-018-1190-9
Bunnapradist, S., Lentine, K.-L., Burroughs, T.-E., Pinsky, B. W., Hardinger, K. L., Brennan, DC., et al. (2006). Mycophenolate mofetil dose reductions and discontinuations after gastrointestinal complications are associated with renal transplant graft failure. Transplantation 82 (1), 102–107. doi: 10.1097/01.tp.0000225760.09969.1f
Bunnapradist, S., Neri, L., Wong, W., Lentine, K. L., Burroughs, T. E., Pinsky, B. W., et al. (2008). Incidence and risk factors for diarrhea following kidney transplantation and association with graft loss and mortality. Am. J. Kidney Dis. 51 (3), 478–486. doi: 10.1053/j.ajkd.2007.11.013
Carron, C., Pais de Barros, J. P., Gaiffe, E., Deckert, V., Adda-Rezig, H., Roubiou, C., et al. (2019). End-stage renal disease-associated gut bacterial translocation: evolution and impact on chronic inflammation and acute rejection after renal transplantation. Front. Immunol. 10, 1630. doi: 10.3389/fimmu.2019.01630
Castaneda, D.-A., Leon, K., Martin, R., López, L., Pérez, H., Lozano, E., et al. (2013). Urinary tract infection and kidney transplantation: a review of diagnosis, causes, and current clinical approach. Transplant. Proc. 45 (4), 1590–1592. doi: 10.1016/j.transproceed.2013.01.014
Chan, S., Hawley, C. M., Pascoe, E. M., Cao, C., Campbell, S. B., Campbell, K. L., et al. (2022). Prebiotic supplementation in kidney transplant recipients for preventing infections and gastrointestinal upset a randomized controlled feasibility study. J. Renal Nutr. 22 (S1051-2276), 33–34. doi: 10.1053/j.jrn.2022.02.006
Chan, S., Morrison, M., Hawley, C.-M., Campbell, S. B., Francis, R. S., Isbel, N. M., et al. (2021). Characteristics of the gastrointestinal microbiota in paired live kidney donors and recipients. Nephrology 26 (5), 471–478. doi: 10.1111/nep.13853
Chong, A.-S., Alegre, M.-L. (2012). The impact of infection and tissue damage in solid-organ transplantation. Nat. Rev. Immunol. 12 (6), 459–471. doi: 10.1038/nri3215
Costea, P.-I., Hildebrand, F., Arumugam, M., Bäckhed, F., Blaser, M. J., Bushman, F. D., et al. (2018). Enterotypes in the landscape of gut microbial community composition. Nat. Microbiol. 3 (1), 8–16. doi: 10.1038/s41564-017-0072-8
Cummings, J.-H., Pomare, E.-W., Branch, W.-J., Naylor, C. P., Macfarlane, G. T. (1987). Short chain fatty acids in human large intestine, portal, hepatic and venous blood. Gut 28 (10), 1221–1227. doi: 10.1136/gut.28.10.1221
de Castro Rodrigues Ferreira, F., Cristelli, M.-P., Paula, M.-I., Proença, H., Felipe Rosso, C., Tedesco-Silva, H., et al. (2017). Infectious complications as the leading cause of death after kidney transplantation: analysis of more than 10,000 transplants from a single center. J. Nephrol. 30 (4), 601–606. doi: 10.1007/s40620-017-0379-9
Dominguez-Bello, M.-G., Godoy-Vitorino, F., Knight, R., Blaser, M. J. (2019). Role of the microbiome in human development. Gut 68 (6), 1108–1114. doi: 10.1136/gutjnl-2018-317503
Eckburg, P.-B., Bik, E.-M., Bernstein, C.-N., Purdom, E., Dethlefsen, L., Sargent, M., et al. (2005). Diversity of the human intestinal microbial flora. Science 308 (5728), 1635–1638. doi: 10.1126/science.1110591
Ekberg, H., Kyllönen, L., Madsen, S., Grave, G., Solbu, D., Holdaas, H., et al. (2007). Clinicians underestimate gastrointestinal symptoms and overestimate quality of life in renal transplant recipients: a multinational survey of nephrologists. Transplantation 84 (8), 1052–1054. doi: 10.1097/01.tp.0000284983.89207.1a
Eng, A., Borenstein, E. (2019). Microbial community design: methods, applications, and opportunities. Curr. Opin. Biotechnol. 58, 117–128. doi: 10.1016/j.copbio.2019.03.002
Fiorante, S., Lopez-Medrano, F., Lizasoain, M., Lalueza, A., Juan, R. S., Andrés, A., et al. (2010). Systematic screening and treatment of asymptomatic bacteriuria in renal transplant recipients. Kidney Int. 78 (8), 774–781. doi: 10.1038/ki.2010.286
Fiorentino, M., Pesce, F., Schena, A., Simone, S., Castellano, G., Gesualdo, L., et al. (2019). Updates on urinary tract infections in kidney transplantation. J. Nephrol. 32 (5), 751–761. doi: 10.1007/s40620-019-00585-3
Flint, H.-J., Duncan, S.-H., Scott, K.-P., Louis, P. (2015). Links between diet, gut microbiota composition and gut metabolism. Proc. Nutr. Soc. 74 (1), 13–22. doi: 10.1017/S0029665114001463
Force, E., Andreu, L. (2005). Kidney transplantation. EDTNA ERCA J. 31 (4), 172–175. doi: 10.1111/j.1755-6686.2005.tb00423.x
Fricke, W.-F., Maddox, C., Song, Y., Bromberg, J. S.. (2014). Human microbiota characterization in the course of renal transplantation. Am. J. Transplant. 14 (2), 416–427. doi: 10.1111/ajt.12588
Gioco, R., Corona, D., Ekser, B., Puzzo, L., Inserra, G., Pinto, F., et al. (2020). Gastrointestinal complications after kidney transplantation. World J. Gastroenterol. 26 (38), 5797–5811. doi: 10.3748/wjg.v26.i38.5797
Gloor, J.-M., Sethi, S., Stegall, M.-D., Park, W. D., Moore, S. B., DeGoey, S., et al. (2007). Transplant glomerulopathy: subclinical incidence and association with alloantibody. Am. J. Transplant. 7 (9), 2124–2132. doi: 10.1111/j.1600-6143.2007.01895.x
Gomaa, E.-Z. (2020). Human gut microbiota/microbiome in health and diseases: a review. Antonie Van Leeuwenhoek 113 (12), 2019–2040. doi: 10.1007/s10482-020-01474-7
Grosen, A.-K., Povlsen, J.-V., Lemming, L.-E., Jørgensen, S. M. D., Dahlerup Frederik, J., Hvas, C. L, et al. (2019). Faecal microbiota transplantation eradicated extended-spectrum beta-Lactamase-Producing Klebsiella pneumoniae from a renal transplant recipient with recurrent urinary tract infections. Case Rep. Nephrol. Dialysis 9 (2), 102–107. doi: 10.1159/000502336
Gu, B., Bo, G.-Z., Ke, C. (2018). Exploration of fecal microbiota transplantation in the treatment of refractory diarrhea after renal transplantation. Transplant. Proc. 50 (5), 1326–1331. doi: 10.1016/j.transproceed.2018.03.013
Guida, B., Cataldi, M., Memoli, A., Trio, R., di Maro, M., Grumetto, L., et al. (2017). Effect of a short-course treatment with synbiotics on plasma p-cresol concentration in kidney transplant recipients. J. Am. Coll. Nutr. 36 (7), 586–591. doi: 10.1080/07315724.2017.1334602
Guirong, Y. E., Zhou, M., Lixin, Y. U., Junsheng, Y. E., Lin, Y., Lisha, S., et al. (2018). Gut microbiota in renal transplant recipients, patients with chronic kidney disease and healthy subjects. J. South. Med. Univ. 38 (12), 1401–1408. doi: 10.12122/j.issn.1673-4254.2018.12.01
Hariharan, S., Israni, A.-K., Danovitch, G. (2021). Long-term survival after kidney transplantation. N Engl. J. Med. 385 (8), 729–743. doi: 10.1056/NEJMra2014530
Hariharan, S., Johnson, C.-P., Bresnahan, B.-A., Taranto, S. E., McIntosh, M. J., Stablein, D., et al. (2000). Improved graft survival after renal transplantation in the united states, 1988 to 1996. N Engl. J. Med. 342 (9), 605–612. doi: 10.1056/NEJM200003023420901
Herrington, C.-S., Poulsom, R., Coates, P.-J. (2019). Recent advances in pathology: the 2019 annual review issue of the journal of pathology. J. Pathol. 247 (5), 535–538. doi: 10.1002/path.5255
Ianiro, G., Rossi, E., Thomas, A.-M., Schinzari, G., Masucci, L., Quaranta, G., et al. (2020). Faecal microbiota transplantation for the treatment of diarrhoea induced by tyrosine-kinase inhibitors in patients with metastatic renal cell carcinoma. Nat. Commun. 11 (1). doi: 10.1038/s41467-020-18127-y
Jang, H.-R., Gandolfo, M.-T., Ko, G.-J., Satpute, S., Racusen, L., Rabb, H., et al. (2009). Early exposure to germs modifies kidney damage and inflammation after experimental ischemia-reperfusion injury. Am. J. Physiol. Renal Physiol. 297 (5), F1457–F1465. doi: 10.1152/ajprenal.90769.2008
Karuthu, S., Blumberg, E.-A. (2012). Common infections in kidney transplant recipients. Clin. J. Am. Soc. Nephrol. 7 (12), 2058–2070. doi: 10.2215/CJN.04410512
Khanna, S., Tosh, P.-K. (2014). A clinician’s primer on the role of the microbiome in human health and disease. Mayo Clin. Proc. 89 (1), 107–114. doi: 10.1016/j.mayocp.2013.10.011
Kidney Disease: Improving Global Outcomes (KDIGO) Transplant Work Group(2009). KDIGO clinical practice guideline for the care of kidney transplant recipients. Am. J. Transplant. 9 (Suppl 3), S1–S155. doi: 10.1111/j.1600-6143.2009.02834.x
Kim, J.-E., Kim, H.-E., Cho, H., Park, J. I., Kwak, M.-J., Kim, B.-Y., et al. (2020). Effect of the similarity of gut microbiota composition between donor and recipient on graft function after living donor kidney transplantation. Sci. Rep. 10 (1), 18881. doi: 10.1038/s41598-020-76072-8
Kim, S.-M., Song, I.-H. (2020). The clinical impact of gut microbiota in chronic kidney disease. Korean J. Internal Med. 35 (6), 1305–1316. doi: 10.3904/kjim.2020.411
Kujawa-Szewieczek, A., Adamczak, M., Kwiecien, K., Dudzicz, S., Gazda, M., Więcek, A., et al. (2015). The effect of lactobacillus plantarum 299v on the incidence of clostridium difficile infection in high risk patients treated with antibiotics. Nutrients 7 (12), 10179–10188. doi: 10.3390/nu7125526
Lecronier, M., Tashk, P., Tamzali, Y., Tenaillon, O., Denamur, E., Barrou, B., et al. (2020). Gut microbiota composition alterations are associated with the onset of diabetes in kidney transplant recipients. PloS One 15 (1), e227373. doi: 10.1371/journal.pone.0227373
Lee, J.-R., Bang, H., Dadhania, D., Hartono, C., Aull, M. J., Satlin, M., et al. (2013). Independent risk factors for urinary tract infection and for subsequent bacteremia or acute cellular rejection: a single-center report of 1166 kidney allograft recipients. Transplantation 96 (8), 732–738. doi: 10.1097/TP.0b013e3182a04997
Lee, J.-R., Huang, J., Magruder, M., Zhang, L. T., Gong, C., Sholi, A. D. N., et al. (2019a). Butyrate-producing gut bacteria and viral infections in kidney transplant recipients: a pilot study. Transplant. Infect. Dis. 21 (6):e13180. doi: 10.1111/tid.13180
Lee, J.-R., Magruder, M., Zhang, L., Westblade, L. F., Satlin, M. J., Robertson, A., et al. (2019b). Gut microbiota dysbiosis and diarrhea in kidney transplant recipients. Am. J. Transplant. 19 (2), 488–500. doi: 10.1111/ajt.14974
Lee, J.-R., Muthukumar, T., Dadhania, D., Toussaint, N. C., Ling, L., Pamer, E., et al. (2014). Gut microbial community structure and complications after kidney transplantation: a pilot study. Transplantation 98 (7), 697–705. doi: 10.1097/TP.0000000000000370
Lee, J.-R., Muthukumar, T., Dadhania, D., Taur, Y., Jenq, R. R., Toussaint, N. C., et al. (2015). Gut microbiota and tacrolimus dosing in kidney transplantation. PloS One 10 (3), e122399. doi: 10.1371/journal.pone.0122399
Li, Q.-R., Wang, C.-Y., Tang, C., He, Q., Li, N., Li, J. S., et al. (2013). Reciprocal interaction between intestinal microbiota and mucosal lymphocyte in cynomolgus monkeys after alemtuzumab treatment. Am. J. Transplant. 13 (4), 899–910. doi: 10.1111/ajt.12148
Lin, S.-C., Alonso, C.-D., Moss, A.-C. (2018). Fecal microbiota transplantation for recurrentClostridium difficile infection in patients with solid organ transplants: an institutional experience and review of the literature. Transplant. Infect. Dis. 20 (6), e12967. doi: 10.1111/tid.12967
Magruder, M., Edusei, E., Zhang, L., Albakry, S., Satlin, M. J., Westblade, L. F., et al. (2020). Gut commensal microbiota and decreased risk for enterobacteriaceae bacteriuria and urinary tract infection. Gut Microbes 12 (1), 1805281. doi: 10.1080/19490976.2020.1805281
Magruder, M., Sholi, A.-N., Gong, C., Zhang, L., Edusei, E., Huang, J., et al. (2019). Gut uropathogen abundance is a risk factor for development of bacteriuria and urinary tract infection. Nat. Commun. 10 (1):5521. doi: 10.1038/s41467-019-13467-w
Matenchuk, B.-A., Mandhane, P.-J., Kozyrskyj, A.-L. (2020). Sleep, circadian rhythm, and gut microbiota. Sleep Med. Rev. 53, 101340. doi: 10.1016/j.smrv.2020.101340
Meena, P., Bhargava, V., Rana, D.-S., Bhalla, A. K. (2021). Urinary tract infection in renal transplant recipient: a clinical comprehensive review. Saudi J. Kidney Dis. Transpl 32 (2), 307–317. doi: 10.4103/1319-2442.335441
Mori, D.-N., Kreisel, D., Fullerton, J.-N., Gilroy, D. W., Goldstein, D. R. (2014). Inflammatory triggers of acute rejection of organ allografts. Immunol. Rev. 258 (1), 132–144. doi: 10.1111/imr.12146
Nallu, A., Sharma, S., Ramezani, A., Muralidharan, J., Raj, D. (2017). Gut microbiome in chronic kidney disease: challenges and opportunities. Transl. Res. 179, 24–37. doi: 10.1016/j.trsl.2016.04.007
Pelle, G., Vimont, S., Levy, P.-P., Hertig, A., Ouali, N., Chassin, C., et al. (2007). Acute pyelonephritis represents a risk factor impairing long-term kidney graft function. Am. J. Transplant. 7 (4), 899–907. doi: 10.1111/j.1600-6143.2006.01700.x
Piton, G., Capellier, G. (2016). Biomarkers of gut barrier failure in the ICU. Curr. Opin. Crit. Care 22 (2), 152–160. doi: 10.1097/MCC.0000000000000283
Quigley, E. M. M. (2017). Microbiota-Brain-Gut axis and neurodegenerative diseases. Curr. Neurol. Neurosci. Rep. 17 (12), 94. doi: 10.1007/s11910-017-0802-6
Ranganathan, N., Patel, B., Ranganathan, P., Marczely, J., Dheer, R., Chordia, T., et al. (2005). Probiotic amelioration of azotemia in 5/6th nephrectomized sprague-dawley rats. ScientificWorldJournal 5, 652–660. doi: 10.1100/tsw.2005.86
Ranganathan, N., Patel, B.-G., Ranganathan, P., Marczely, J., Dheer, R. (2006). In vitro and in vivo assessment of intraintestinal bacteriotherapy in chronic kidney disease. ASAIO J. 52 (1), 70–79. doi: 10.1097/01.mat.0000191345.45735.00
Ranganathan, N., Ranganathan, P., Friedman, E.-A., Joseph, A., Delano, B., Goldfarb, D. S., et al. (2010). Pilot study of probiotic dietary supplementation for promoting healthy kidney function in patients with chronic kidney disease. Adv. Ther. 27 (9), 634–647. doi: 10.1007/s12325-010-0059-9
Rice, J.-C., Safdar, N. (2009). Urinary tract infections in solid organ transplant recipients. Am. J. Transplant. 9 Suppl 4, S267–S272. doi: 10.1111/j.1600-6143.2009.02919.x
Rukavina, M.-N., Kouyoumdzian, N.-M., Choi, M.-R. (2020). Gut microbiota and chronic kidney disease: evidences and mechanisms that mediate a new communication in the gastrointestinal-renal axis. Pflugers Arch. 472 (3), 303–320. doi: 10.1007/s00424-020-02352-x
Shi, N., Li, N., Duan, X., Niu, H. (2017). Interaction between the gut microbiome and mucosal immune system. Mil Med. Res. 4, 14. doi: 10.1186/s40779-017-0122-9
Swarte, J.-C., Douwes, R.-M., Hu, S., Vila Vich, A., Eisenga, M. F., van Londen, M., et al. (2020). Characteristics and dysbiosis of the gut microbiome in renal transplant recipients. J. Clin. Med. 9 (2), 386. doi: 10.3390/jcm9020386
Swarte, J.-C., Martinez, I., Sun, Y., Zhang, L. T., Westblade, L. F., Iqbal, F., et al. (2012). Characteristics and dysbiosis of the gut microbiome in renal transplant recipients. Am. J. Transplant. 12 (3), 753–762. doi: 10.1111/j.1600-6143.2011.03860.x
Tonshoff, B. (2020). Immunosuppressants in organ transplantation. Handb. Exp. Pharmacol. 261, 441–469. doi: 10.1007/164_2019_331
Valera, B., Gentil, M.-A., Cabello, V., Fijo, J., Cordero, E., Cisneros, J. M., et al. (2006). Epidemiology of urinary infections in renal transplant recipients. Transplant. Proc. 38 (8), 2414–2415. doi: 10.1016/j.transproceed.2006.08.018
Wang, J., Li, X., Wu, X., Wang, Z., Wu, X., Wang, S., et al. (2021a). Fecal microbiota transplantation as an effective treatment for carbapenem-resistant klebsiella pneumoniae infection in a renal transplant patient. Infect. Drug Resist. 14, 1805–1811. doi: 10.2147/IDR.S308308
Wang, J., Li, X., Wu, X., Wang, Z., Zhang, C., Cao, G., et al. (2021b). Gut microbiota alterations associated with antibody-mediated rejection after kidney transplantation. Appl. Microbiol. Biotechnol. 105 (6), 2473–2484. doi: 10.1007/s00253-020-11069-x
Wang, J.-H., Skeans, M.-A., Israni, A.-K. (2016). Current status of kidney transplant outcomes: dying to survive. Adv. Chronic Kidney Dis. 23 (5), 281–286. doi: 10.1053/j.ackd.2016.07.001
Wei, Y., Li, N., Xing, H., Guo, T., Gong, H., Chen, D., et al. (2019). Effectiveness of fecal microbiota transplantation for severe diarrhea after drug-induced hypersensitivity syndrome. Medicine 98 (52), e18476. doi: 10.1097/MD.0000000000018476
Westblade, L.-F., Satlin, M.-J., Albakry, S., Botticelli, B., Robertson, A., Alston, T., et al. (2019). Gastrointestinal pathogen colonization and the microbiome in asymptomatic kidney transplant recipients. Transpl Infect. Dis. 21 (6), e13167. doi: 10.1111/tid.13167
Winichakoon, P., Chaiwarith, R., Chattipakorn, N., Chattipakorn, S. C. (2022). Impact of gut microbiota on kidney transplantation. Transplant. Rev. (Orlando) 36 (1), 100668. doi: 10.1016/j.trre.2021.100668
Witkowski, M., Weeks, T.-L., Hazen, S.-L. (2020). Gut microbiota and cardiovascular disease. Circ. Res. 127 (4), 553–570. doi: 10.1161/CIRCRESAHA.120.316242
Wolfe, R.-A., Ashby, V.-B., Milford, E.-L., Ojo, A. O., Ettenger, R. E., Agodoa, L. Y., et al. (1999). Comparison of mortality in all patients on dialysis, patients on dialysis awaiting transplantation, and recipients of a first cadaveric transplant. N Engl. J. Med. 341 (23), 1725–1730. doi: 10.1056/NEJM199912023412303
Wu, H., Tremaroli, V., Schmidt, C., Lundqvist, A., Olsson, L. M., Krämer, M., et al. (2020). The gut microbiota in prediabetes and diabetes: a population-based cross-sectional study. Cell Metab. 32 (3), 379–390. doi: 10.1016/j.cmet.2020.06.011
Xiao, J., Peng, Z., Liao, Y., Sun, H., Chen, W., Chen, X., et al. (2018). Organ transplantation and gut microbiota: current reviews and future challenges. Am. J. Transl. Res. 10 (11), 3330–3344.
Xu, K.-Y., Xia, G.-H., Lu, J.-Q., Chen, M.-X., Zhen, X., Wang, S., et al. (2017). Impaired renal function and dysbiosis of gut microbiota contribute to increased trimethylamine-n-oxide in chronic kidney disease patients. Sci. Rep. 7 (1), 1145. doi: 10.1038/s41598-017-01387-y
Yoshifuji, A., Wakino, S., Irie, J., Tajima, T., Hasegawa, K., Kanda, T., et al. (2016). Gut lactobacillus protects against the progression of renal damage by modulating the gut environment in rats. Nephrol. Dial Transplant. 31 (3), 401–412. doi: 10.1093/ndt/gfv353
Zaza, G., Dalla Gassa, A., Felis, G., Granata, S., Torriani, S., Lupo, A., et al. (2017). Impact of maintenance immunosuppressive therapy on the fecal microbiome of renal transplant recipients: comparison between an everolimus- and a standard tacrolimus-based regimen. PloS One 12 (5), e178228. doi: 10.1371/journal.pone.0178228
Keywords: kidney transplantation, gut microbiota, complication, diarrhea, rejection, probiotic
Citation: Ye J, Yao J, He F, Sun J, Zhao Z and Wang Y (2023) Regulation of gut microbiota: a novel pretreatment for complications in patients who have undergone kidney transplantation. Front. Cell. Infect. Microbiol. 13:1169500. doi: 10.3389/fcimb.2023.1169500
Received: 19 February 2023; Accepted: 23 May 2023;
Published: 06 June 2023.
Edited by:
Rosa Sessa, Sapienza University of Rome, ItalyReviewed by:
Wei Liang, Renmin Hospital of Wuhan University, ChinaCopyright © 2023 Ye, Yao, He, Sun, Zhao and Wang. This is an open-access article distributed under the terms of the Creative Commons Attribution License (CC BY). The use, distribution or reproduction in other forums is permitted, provided the original author(s) and the copyright owner(s) are credited and that the original publication in this journal is cited, in accordance with accepted academic practice. No use, distribution or reproduction is permitted which does not comply with these terms.
*Correspondence: Yumei Wang, d2FuZ3l1bWVpNzVAMTYzLmNvbQ==
†These authors have contributed equally to this work
Disclaimer: All claims expressed in this article are solely those of the authors and do not necessarily represent those of their affiliated organizations, or those of the publisher, the editors and the reviewers. Any product that may be evaluated in this article or claim that may be made by its manufacturer is not guaranteed or endorsed by the publisher.
Research integrity at Frontiers
Learn more about the work of our research integrity team to safeguard the quality of each article we publish.