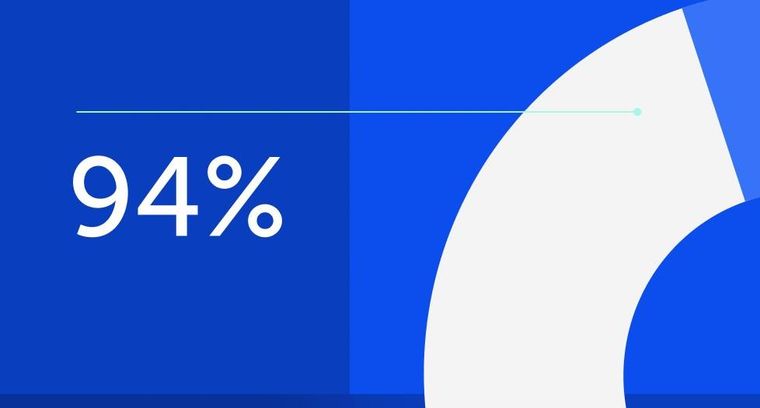
94% of researchers rate our articles as excellent or good
Learn more about the work of our research integrity team to safeguard the quality of each article we publish.
Find out more
ORIGINAL RESEARCH article
Front. Cell. Infect. Microbiol., 26 July 2023
Sec. Clinical Microbiology
Volume 13 - 2023 | https://doi.org/10.3389/fcimb.2023.1157944
Background: In patients with invasive fungal infection (IFI), the steady-state serum trough concentration (Cmin) of voriconazole (VCZ) is highly variable and can lead to treatment failure (Cmin < 0.5 mg/L) and toxicity (Cmin ≥ 5.0 mg/L). However, It remains challenging to determine the ideal maintenance dose to achieve the desired Cmin level quickly.
Aims: This randomized, prospective observational single-center study aimed to identify factors affecting VCZ-Cmin and maintenance dose and create an algorithmic model to predict the necessary maintenance dose. MeThe study enrolled 306 adult IFI patients, split into two groups: non-gene-directed (A) (where CYP2C19 phenotype is not involved in determining VCZ dose) and gene-directed (B) (where CYP2C19 phenotype is involved in determining VCZ dose).
Results: Results indicated that CYP2C19 genetic polymorphisms might significantly impact VCZ loading and maintenance dose selection. CYP2C19 phenotype, C-reaction protein (CRP), and average daily dose/body weight were significant influencers on VCZ-Cmin, while CYP2C19 phenotype, CRP, and body weight significantly impacted VCZ maintenance dose. A feasible predictive formula for VCZ stable maintenance dose was derived from the regression equation as a maintenance dose (mg) =282.774-0.735×age (year)+2.946×body weight(Kg)-19.402×CYP2C19 phenotype (UM/RM/NM:0, IM:1, PM:2)-0.316×CRP (mg/L) (p < 0.001).
Discussion: DiThis formula may serve as a valuable supplement to the Clinical Pharmacogenetics Implementation Consortium (CPIC®) guideline for CYP2C19 and VCZ therapy, especially for IFI patients with highly variable inflammatory cytokines during VCZ therapy.
Despite medical advances, fungal infections are a significant morbidity and mortality cause. Invasive fungal infection (IFI) in critically ill patients continues to present a hurdle and significant challenge for the clinician (Bassetti et al., 2017; Yan et al., 2018; Resendiz-Sharpe et al., 2019). Voriconazole (VCZ), a preferred first-line broad-spectrum triazole antifungal agent, is widely used to treat IFI (Limper et al., 2011; Lortholary et al., 2012; Ullmann et al., 2012), including pathogens of Aspergillus and Candida species, as well as other mold infections (Tissot et al., 2017; Lestrade et al., 2019). Compared with fluconazole, VCZ provides an improved therapeutic option for treating life-threatening fungal infections (Peman et al., 2006).
However, individualized dose adjustment is critical to achieving optimal serum VCZ concentration. VCZ has non-linear pharmacokinetics due to its saturable metabolism. The proportion of exposure dose increase is much more significant than the dose increase for VCZ. Therefore, VCZ serum trough concentrations (VCZ-Cmin) are highly variable in clinical practice, with variability observed among patients and the same patients over time (Schulz et al., 2019). These variability factors are complex and include age, body weight, obesity, baseline status of patients, liver functions, polymorphisms of drug-metabolizing enzymes, drug interactions, and inflammatory factors such as C-reaction protein (CRP), Interleukin-6 (IL-6) and IL-1β (Koselke et al., 2012; Dolton et al., 2014; Valle-T-Figueras et al., 2021; Aiuchi et al., 2022; Li et al., 2022). Many studies and our previous studies have suggested that inflammatory factors [CRP, IL-6, IL-1, and procalcitonina (PCT)] can significantly affect the pharmacokinetics of VCZ, resulting in an increase in VCZ-Cmin in patients (Shah and Smith, 2015; Zeng et al., 2020; Darakjian et al., 2021). However, subtherapeutic and supratherapeutic concentrations of VCZ are associated with increased mortality in patients with IFI and a high frequency of adverse events such as hepatotoxicity, neurotoxicity, and visual disorders, respectively (Farkas et al., 2016). Therapeutic drug monitoring (TDM) can be widely used to assess the efficacy and safety of VCZ (Richards et al., 2017; Takesue et al., 2022). To decrease the risk of therapeutic failure and drug-related toxicity, the Chinese Pharmacological Society has recommended a reasonable VCZ-Cmin range of 0.5-5.0 mg/L for efficacy and safety in the Chinese population (Jin et al., 2016; Chen et al., 2018; Mafuru et al., 2019). Current research mainly focuses on finding the factors that influence the variation of VCZ-Cmin. The highly variable VCZ-Cmin caused by the non-linear pharmacokinetics poses a significant challenge to optimal dosing. It is worth considering how to personalize the VCZ maintenance dose to achieve an effective Cmin.
The high interindividual pharmacokinetic variability of VCZ is mainly influenced by the liver through cytochrome CYP2C19 and, to a lesser extent, by CYP2C9 and CYP3A4 (Xu et al., 2018; Kim et al., 2019). VCZ-Cmin in CYP2C19 intermediate and poor metabolizers is found to be 1.64 and 2.61 times higher compared to normal metabolizers (Miao et al., 2019). The Clinical Pharmacogenetics Implementation Consortium (CPIC®) guideline summarizes evidence from the literature supporting this association. It provides therapeutic recommendations for using VCZ for treatment based on the CYP2C19 genotype, published in Clinical Pharmacology and Therapeutics in 2017 (Moriyama et al., 2017). However, the evidence is still insufficient regarding the relationship between CYP2C19 genetic status and loading dose for VCZ. Formulating a multivariate regression of these related-Cmin factors into the stable maintenance dose formula is still a challenge.
Therefore, the study aimed to (і) Determine the guiding significance of the CYP2C19 genetic polymorphisms for the initial loading dose of VCZ in ill patients; (ii) Determine factors affecting VCZ-Cmin and maintenance dose; (iii) Establish the prediction model formula for a stable maintenance dose of VCZ.
A prospective cohort study was conducted at Zhengzhou Central Hospital Affiliated to Zhengzhou University from August 2018 to August 2021. Hospitalized patients who met the following inclusion criteria were eligible for the study: (і) age ≥ 18 years old; (ii) diagnosed with invasive fungal infection according to the criteria established by De Pauw et al. (De Pauw et al., 2008); (iii) received VCZ therapy for ≥ 14 days; and (iv) had accurate, complete, and available efficacy and safety data. The exclusion criteria were: (і) patients allergic to VCZ or with poor compliance; (ii) use of other antifungal drugs during VCZ treatment; (iii) not eligible for blood sampling monitored by blood concentration; (iv) patients with severe liver function impairment (alanine aminotransferase (ALT) and aspartate aminotransferase (AST) before VCZ treatment are more significant than 3 times the standard upper limit, total bilirubin (TBIL) is greater than 2 times the standard upper limit); (v) pregnant or lactating women; and (vi) those who had participated in other clinical trials in the past three months. A total of 306 patients were screened for the study (Figure 1). This study was conducted following the Declaration of Helsinki, and it was approved by the Investigational Review Board at Zhengzhou Central Hospital Affiliated to Zhengzhou University (No:201973). The clinical trials were registered in the clinical trial registry at https://clinicaltrials.gov/ (NCT04004078). All patients or their legal representatives provided written informed consent.
Figure 1 Study flow diagram. VCZ, voriconazole; Cmin, serum trough concentration. UM, Ultrarapid metabolizer; RM, rapid metabolizer; NM, normal metabolizer; IM, intermediate metabolizer; PM, poor metabolizer.
The patients were randomly assigned to either the non-gene-directed group (Group A, n=158), where CYP2C19 phenotype was not considered in determining VCZ dose, or the gene-directed group (Group B, n=148), where CYP2C19 phenotype was involved in determining VCZ dose. Group A received VCZ doses according to the drug instructions. After VCZ treatment, the CYP2C19 genotype of group A was determined. The patients were categorized into ultrarapid metabolizer (UM)/rapid metabolizer (RM)/normal metabolizer (NM) group (C1), intermediate metabolizer (IM) group (D1), and poor metabolizer (PM) group (E1), prepare for the construction of mathematical models in the derivation group. Before VCZ administration, Group B patients were categorized into UM/RM/NM group (C2), IM group (D2), and PM group (E2) based on their CYP2C19 genotype. All the recruited patients were treated with VCZ for a duration of 14 to 93 days, and the dosage of the administration is outlined below. Group A received VCZ intravenously or orally 2 times at a loading dose of 450, 400, 350, or 300 mg at 12-hour intervals, followed by a maintenance dose of 350, 300, 250, or 200 mg at 12-hour intervals. For group B patients with RM, NM, and IM, the dosage of VCZ was the same as that of group A. For patients with PM, VCZ was intravenously or orally administered 2 times at a loading dose of 300 mg at 12-hour intervals, followed by a maintenance dose of 200 or 150 mg at 12-hour intervals. For the patients with UM, VCZ was administered at a dose that was 50% higher than the standard dose.
CYP2C19 genotype was determined from peripheral blood, which was extracted and stored in an EDTA anticoagulant tube. Real-time fluorescence quantitative PCR (ThermoFisher Applied Biosystems 7500 fast PCR) was performed using a Human CYP2C19 gene detection kit (PCR-fluorescence probe method, Wuhan YZY Medical Science and Technology Co., Ltd, China) following the manufacturer’s instructions. Genomic DNA was isolated from whole blood using QIAamp DNA blood kits (Qiagen, Hilden, Germany). According to nomenclature by CPIC®, the CYP2C19 genotype was classified as ultrarapid metabolizer (*17/*17), rapid metabolizer (*1/*17), normal metabolizer (*1/*1), intermediate metabolizer (*1/*2, *1/*3, *2/*17, *3/*17), or poor metabolizer (*2/*2, *2/*3, *3/*3).
The blood samples collected from the enrolled patients were centrifuged at 3500 r/min for 10 minutes. VCZ-Cmin levels were measured at steady-state, 30 minutes before VCZ administration, using high-performance liquid chromatography with acetonitrile-water (53:47) as the mobile phase, a flow rate of 1.0 mL/min, and a wavelength of 256 nm. The Cmin levels were monitored at least once for each patient. The Translational Medicine Center implemented TDM. The linearity range was 0.0635 ~ 21.16 mg/L (R2 = 0.9999), and the limit of quantitation and the detection limit were 0.8 ng and 0.3 ng, respectively. The intra-day and inter-day precisions were 1.37% and 1.86%, respectively. The average extraction recovery of VCZ was 98.03%, and the average method recovery was 99.52%, with an RSD of 0.83%.
CRP, PCT, and IL-6 were detected using validated sandwich ELISA kits according to the manufacturer’s instructions at the Zhengzhou Clinical Laboratory Center. The interval for drawing blood between inflammatory factors and Cmin is not more than 24 hours.
The hospital medical records of all patients included in the study were screened, reviewed, and analyzed by trained reviewers using a hospital information system. Clinical data were collected, including demographics, comorbidities, concomitant use of proton pump inhibitors (PPIs) and glucocorticoid, clinical information regarding VCZ dosing, duration of VCZ therapy, patients’ symptoms, body temperature, and chest X-Rays. Laboratory data included white blood cell counts, CRP, PCT, IL-6, gamma-glutamyl transpeptidase (GGT), AST, ALT, alkaline phosphatase (ALP), TBIL, albumin, blood urea nitrogen (BUN), serum creatinine (Scr), and Creatinine clearance.
Patients who received VCZ for suspected invasive fungal infection (IFI) were classified according to the Invasive Fungal Infection Group criteria of the European Organization for Research and Treatment of Cancer and Mycoses Study Group of the National Institute of Allergy and Infectious Diseases. Efficacy assessment was performed by a team of three physicians led by the chief physician. The final clinical response to VCZ was evaluated based on the patients’ clinical symptoms, laboratory data, the Galactomannan experiment, 1,3-β-D-glucan experiment, bacteriological findings, and computed tomography. The response was classified as complete, partial, or treatment failure, with a complete response being defined as the resolution of signs and symptoms of the infectious process, including chest X-ray, and a partial response is defined as at least a 50% improvement in pulmonary infiltrates and signs and symptoms of the infection. Treatment failure was defined as the withdrawal of the treatment due to poor response, toxicity, or death associated with the infection (Ruiz et al., 2019).
Hepatotoxicity was defined as ALT or AST more than three times the upper limit of the institution’s normal reference ranges (ALT 0–40 U/L, AST 0–35 U/L), or TBil more than two times the upper limit of the institution’s normal reference ranges (TBIL 5.1–22 umol/L). For patients with abnormal values at baseline, hepatotoxicity was defined as ALT or AST more than three times or TBil more than two times the baseline value. The relationship between hepatotoxicity and VCZ was evaluated.
Statistical analyses and randomization were performed using the Statistical Package for the Social Sciences software (ver.19.0; SPSS Inc.). Data were presented as the number of categorical variables or as mean ± standard deviation (mean ± SD) or median values (minimum-maximum) and interquartile range (IQR) for continuous variables. Chi-squared or Fisher’s exact test compared groups for categorical variables. Comparisons were conducted using the Student’s t-test or the Mann-Whitney U-test for continuous variables, one-way ANOVA analysis of variance, or the Kruskal-Wallis test. Pearson’s correlation analysis was used to analyze the relationship between inflammatory markers and Cmin. Multiple stepwise regression analyses were used to analyze significant influencing factors affecting VCZ-Cmin and the VCZ maintenance dose. Multiple linear regression analyses established a VCZ stable maintenance dose prediction model. All tests were two-tailed, and a p-value of less than 0.05 was considered statistically significant.
The demographic and clinical characteristics of the included patients are presented in Table 1. The age of the studied patients ranged from 18 to 98 years. There were no significant differences in all indices between groups A and B, as well as between any two of all subgroups (group C1, C2, D1, D2, E1, and E2) (p > 0.05).
A patient can undergo multiple Cmin monitoring; 412 VCZ-Cmin measurements were available from the included patient cohort. Of these, 306 initial VCZ-Cmin measurements were available from each patient. Of the 412 concentrations, 370, 318, and 190 matched available CRP, PCT, and IL-6 determination samples. The VCZ-Cmin was 3.97 ± 2.27 and 4.30 ± 2.36 mg/L in groups B and A, respectively (p = 0.203) (Figure 2A). The VCZ-Cmin in group E1 was significantly higher than that in other groups (groups A, B, C1, C2, D1, D2, and E2, all p < 0.05), respectively (Figures 2A, B). That is to say, patients with different CYP2C19 genotypes were given the same dose of VCZ, while patients with the PM genotype can achieve higher VCZ-Cmin. This study found that VCZ-Cmin for 0.63% and 31.01% of the patients in group A were subtherapeutic concentration (< 0.5 mg/L) and supratherapeutic concentration (≥ 5.0 mg/L), respectively. Notably, the proportion of therapeutic concentration (0.5-5.0 mg/L) was significantly higher in group E2 compared to group A (93.75% vs. 68.35%, p = 0.041) and group E1 (93.75% vs. 50.00%, p = 0.008) (Figures 2C, D).
Figure 2 (A) The difference of VCZ-Cmin between groups (A, B). (B) The difference of VCZ-Cmin among subgroups (group C1, C2, D1, D2, E1, and E2). (C) The percentage of patients obtaining Cmin levels of < 0.5 mg/L, 0.5 mg/L ≤ Cmin < 5.0 mg/L, and Cmin > 5.0mg/L between groups (A, B). (D) The percentage of patients achieving Cmin level of < 0.5 mg/L, 0.5 mg/L ≤ Cmin < 5.0 mg/L and Cmin > 5.0 mg/L among subgroups (group C1, C2, D1, D2, E1, and E2). Data were expressed as mean ± SD and were analyzed using Student’s t-test. *p<0.05; **p<0.01.VCZ-Cmin, voriconazole serum trough concentration; UM, Ultrarapid metabolizer; RM, rapid metabolizer; NM, normal metabolizer; IM, intermediate metabolizer; PM, poor metabolizer.
Twenty-eight out of 306 patients had complete indicators for VCZ-Cmin, CRP, PCT, and IL-6 measured simultaneously during their hospital stay. There was no dose adjustment for VCZ after each VCZ-Cmin measurement for these 28 patients. Fourteen patients were measured twice, ten were measured three times, and four were measured four times. The changes in VCZ-Cmin, CRP, PCT, and IL-6 were consistent in 22 patients who were measured multiple times (Supplementary Figures 1A-K, O-U, Y-AB).
CRP, PCT, and IL-6 levels were significantly correlated with VCZ-Cmin (r = 0.428, p < 0.001; r = 0.423, p < 0.001; r = 0.463, p < 0.001), respectively (Figures 3A-C).
Figure 3 (A–C) The linear correlation between VCZ-Cmin (mg/L) and CRP (mg/L), PCT (ng/mL), and IL-6 (pg/mL), respectively. (A) VCZ-Cmin vs. CRP; (B) VCZ-Cmin vs. PCT; (C) VCZ-Cmin vs. IL-6. (D–F) Comparison of CRP, PCT, and IL-6 among Cmin < 0.5 mg/L, 0.5 mg/L ≤ Cmin < 5.0 mg/L, and Cmin ≥ 5.0 mg/L. (D) CRP; (E) PCT; (F) IL-6. Data were expressed as the median values (range) and interquartile range (IQR) and were analyzed by Mann-Whitney U-test and Kruskal-Wallis test. ***p<0.001. VCZ-Cmin, voriconazole serum trough concentration; CRP, C-reactive protein; PCT, procalcitonin; IL-6, interleukin-6.
There were significant differences in CRP, PCT, and IL-6 levels among patients with VCZ-Cmin < 0.5 mg/L, 0.5 mg/L ≤ VCZ-Cmin<5.0 mg/L, and VCZ-Cmin ≥ 5.0 mg/L, respectively (28.09 [2.68-37.30] vs. 38.04 [0.80-336.70] vs. 65.33 mg/L [2.94-453.00], p < 0.001; 0.12 [0.02-0.13] vs. 0.19 [0.02-22.84] vs. 0.86 ng/mL [0.02-43.00], p < 0.001; 13.60 [6.80-14.90] vs. 18.90 [1.50-423.10] vs. 49.60 pg/mL [1.60-1362.00], p < 0.001) (Figures 3D-F). The median concentrations of CRP, PCT, and IL-6 increased as the VCZ-Cmin increased. VCZ-Cmin ≥ 5.0 mg/L was predicted by CRP, PCT and IL-6, respectively. The cutoff values of CRP, PCT, and IL-6 were 48.82 mg/L, 0.995 ng/mL, and 28.50 pg/mL.
This study, 306 patients were divided into groups based on PPIs (esomeprazole, omeprazole, pantoprazole, rabeprazole) and a control group. The demographic and clinical characteristics of the included patients are displayed in Table 2. There were no significant differences in gender, age, body weight, albumin, ALT, AST, ALP, GGT, BUN, Scr, and CRP among the five groups or between any two of the five groups.
Each VCZ-Cmin was adjusted according to body weight and daily dose to calculate dose-normalized VCZ-Cmin. It showed no significant difference in dose-normalized VCZ-Cmin among the five groups (p = 0.584) (Figure 4A). The calculation for dose-normalized VCZ-Cmin was as follows: Dose-normalized VCZ-Cmin=[VCZ-Cmin (mg/L)×body weight (Kg)]/average daily dose (mg). There were no significant differences in the proportion of 0.5 mg/L ≤ Cmin<5.0 mg/L and Cmin ≥ 5.0 mg/L among the five groups (p = 0.701; p = 0.775), respectively (Figure 4B).
Figure 4 (A) Comparison of dose-normalized VCZ-Cmin among control, esomeprazole, omeprazole, rabeprazole and pantoprazole groups. (B) The percentage of patients obtaining Cmin < 0.5 mg/L, 0.5 mg/L ≤ Cmin <5.0 mg/L, and Cmin ≥ 5.0 mg/L among different groups. Data were expressed as mean ± SD and were analyzed by Student’s t-test. ***p<0.001. Dose-normalized VCZ-Cmin=[VCZ-Cmin (mg/L)×body weight (Kg)]/average daily dose (mg); VCZ-Cmin,voriconazole serum trough concentration.
As shown in Table 3, the clinical response was evaluated in 306 patients receiving VCZ for the treatment. There was no significant difference in total favorable response (complete plus partial) between groups B and A (p = 0.575) or among subgroups (group C1, C2, D1, D2, E1, and E2) (p = 0.735).
Out of 306 patients, 40 had hepatotoxicity. We assessed the effects of different levels of VCZ-Cmin (< 0.5, 0.5-5.0, ≥ 0.5 mg/L) on the incidence of hepatotoxicity. Hepatotoxicity differed significantly among the different levels of VCZ-Cmin(< 0.5, 0.5-5.0, ≥ 0.5 mg/L) (χ2 = 19.253, p < 0.0001). The incidence of hepatotoxicity was higher in the supratherapeutic range compared to the therapeutic and subtherapeutic ranges (p < 0.001), respectively (Figure 5A). There was no significant difference in the incidence of hepatotoxicity between groups A and B (p = 0.211) (Figure 5B). No significant differences in hepatotoxicity were found among subgroups (group C1, C2, D1, D2, E1, and E2, p = 0.309). However, the incidence of hepatotoxicity was lower in group E2 compared to group E1 (p = 0.031), respectively (Figure 5C).
Figure 5 The frequency of hepatotoxicity among groups. (A) The frequency of hepatotoxicity among different VCZ-Cmin (< 0.5, 0.5-5.0, ≥ 0.5 mg/L); (B) The frequency of hepatotoxicity between group A and group (B, C) The frequency of hepatotoxicity among subgroups (UM/RM/NM (C1), UM/RM/NM (C2), IM (D1), IM (D2), PM (E1), and PM (E2)). Data were described as their number for categorical variables and were analyzed by a Chi-squared test or Fisher’s exact test. *p<0.05; ****p<0.0001. VCZ-Cmin, voriconazole serum trough concentration; UM, Ultrarapid metabolizer; RM, rapid metabolizer; NM, normal metabolizer; IM, intermediate metabolizer; PM, poor metabolizer.
Factors affecting VCZ-Cmin were evaluated in 302 patients using a Chi-squared test. The results showed that age, CYP2C19 phenotype (PM), CRP, PCT, IL-6, and average daily dose/body weight were risk factors for VCZ-Cmin ≥ 5.0 mg/L (p < 0.05) (Table 4). The CRP, PCT, IL-6, and average daily dose/body weight values were stratified according to the cutoff values predicted by the ROC curve. A multiple linear stepwise regression analysis was performed by using VCZ-Cmin (Y) as the dependent variable, and sex (x1), age (x2), body weight (x3), VCZ route of administration (x4), CYP2C19 phenotype (x5), the average daily dose (x6), combined PPIs (x7), combined methylprednisolone (x8), CRP (x9) and average daily dose/body weight (x10) as independent variables. The regression equation is Y=1.616 + 0.015x9 + 0.169x10 + 0.379x5 (p < 0.001). It showed that CRP, average daily dose/body weight, and CYP2C19 phenotype were significant factors influencing VCZ-Cmin, respectively (p < 0.05) (Table 5).
A multiple linear stepwise regression analysis was performed using VCZ stable maintenance doses (Y) as a dependent variable and sex (x1), age (x2), VCZ route of administration (x3), body weight (x4), CYP2C19 phenotype (x5), combined PPIs (x6), combined glucocorticoid (x7), CRP (x8) as independent variables. The regression equation is Y=360.126-30.325x5 -0.215x8 + 0.888x4 (p < 0.001). The results showed that the CYP2C19 phenotype, CRP, and body weight were significant factors influencing VCZ maintenance dose, respectively (p ≤ 0.05) (Table 6). Although age also affected the stable maintenance dose of VCZ, it was not significant (p = 0.242).
According to the admission order, the 302 patients were divided into the derivation and validation groups. There were no significant differences in the general clinical data (sex, age, body weight, albumin, co-administered PPIs, co-administered hormones, CYP2C19 phenotype, underlying disease, etc.) between the derivation and validation groups (p > 0.05).
In the derivation group, the stable maintenance dose was used as the independent variable (Y), and age (x1), body weight (x2), CYP2C19 phenotype (x3), and CRP (x4) were the dependent variables. The predictive model is Y=282.774-0.735x1 + 2.946x2-19.402x3-0.316x4, p < 0.001. To make the precise dose predicted by the model more convenient for clinicians and nurses, the predicted doses of 175~224, 225~274, 275~324, 325~374, 375~424, 425~474, 475~524, 525~574, and 575~624 mg were adjusted to 200, 250, 300, 350, 400, 450, 500, 550, and 600 mg, respectively.
There was no significant difference between the predicted VCZ maintenance dose and the actual maintenance dose according to the predictive model and the actual maintenance dose (376.10 ± 57.87 vs. 374.00 ± 47.93 mg, p = 0.686). The difference rate (20%~50% and ≥ 50%) between the predicted dose and actual stable dose was 12.00% (12/100) and 1.00% (1/100), respectively.
The data of 13 patients with different rates (≥ 20%) were compared and analyzed. It was shown that the Cmin of 6 patients (5.34 mg/L ≤ Cmin ≤ 10.17 mg/L) was ≥ 5.0 mg/L, and 7 patients (1.35 mg/L ≤ Cmin ≤ 4.17 mg/L) were in the range of 0.5-5.0 mg/L. The essential daily maintenance was achieved when Cmin was in the 0.5-5.0 mg/L range by adjusting the dosage of VCZ. Subsequently, the maintenance dosage predicted by the model was used for the 13 patients, and VCZ-Cmin was measured 5 days later. It was shown that the VCZ-Cmin of 11 patients was in the range of 0.5-5.0 mg/L, except for two patients (5.06 mg/L and 5.39 mg/L). This means that if 100 patients received maintenance doses according to the predictive model, the VCZ-Cmin for 98% of all patients would be in the 0.5-5.0 mg/L range. Because the target VCZ-Cmin is a range, even if there is a deviation of more than 20% between the predicted stable maintenance dose and the maintenance dose initially adjusted to the target Cmin, the VCZ-Cmin will ultimately be in the 0.5-5.0 mg/L range by using the dose of VCZ according to the predictive model.
In many studies, most CYP2C19 mutations involved three common alleles in the Chinese Han population, namely CYP2C19 *2,*3, and *17 (Chow et al., 2019). The current study was conducted on the Henan Chinese Han patient population, and the allele frequencies for CYP2C19 *2,*3, and *17 were found to be 30.8%, 3.8%, and 1.3%, respectively. A Chi-squared test revealed that the distribution of each genotype was consistent with the Hardy-Weinberg law. These allele frequencies were similar to those reported by Botton MR et al. in Asians (28%, 7%, and 2%) and by Zuo LJ et al. in Chinese populations (50.0%, 6.3%, and 2.1%) (Zuo et al., 2012; Botton et al., 2021). It is well known that genetic polymorphism of CYP2C19 can cause phenotypic variability (Chen et al., 2022), contributing to the high variability of VCZ exposure, affecting drug efficacy, and leading to ADRs.
Due to the high bioavailability of oral dosage form (96%) according to VCZ’s label, the intravenous and oral routes of administration are interchangeable when clinically indicated. In the study, 306 patients were treated with different routes of administration for VCZ (Intravenous, oral and sequential therapy). The results showed no noticeable difference in different routes of administration between groups A and B (p > 0.05). Additionally, there was no significant difference in VCZ-Cmin among the three routes of administration (p > 0.05). Our findings highlight the role that CYP2C19 phenotype may have in managing IFI patients with low-dose VCZ in PMs. Furthermore, no relationship between VCZ efficacy and CYP2C19 status was found, consistent with Wang T et al.’s study (Wang et al., 2014). The study also found that CYP2C19 gene-directed administration has an excellent guiding effect on the use of VCZ and reduces the occurrence of hepatotoxicity, especially for patients with PM phenotype. CYP2C19 phenotype is a risk factor affecting VCZ-Cmin and plays a critical role in the initial loading dose of VCZ.
Although CRP and IL-6 are known to be involved in the high fluctuation of VCZ-Cmin (Encalada Ventura et al., 2016; Bolcato et al., 2021), little is known about the influence of PCT on it. We hypothesize that increased inflammatory factors will increase the risk of high VCZ-Cmin and may increase VCZ-related toxicity during treatment. This is the first study to show that inflammation, as reflected by CRP, PCT, and IL-6, can independently influence VCZ-Cmin, completely masking the effects of other potential risk factors. In our study, by measuring CRP, PCT, and IL-6 levels at the different therapeutic ranges, we can determine their potential contribution to the upregulation of VCZ-Cmin. CRP, PCT, and IL-6 in the supratherapeutic range are significantly higher than in other treatment ranges. Our study found that inflammation, as assessed by CRP level, is a risk factor affecting VCZ-Cmin by Stepwise regression analysis. This finding is consistent with Van Wanrooy MJ et al. (Van Wanrooy et al., 2014). In our study, for every 1mg/L increase in the CRP concentration, the VCZ-Cmin was 0.015 mg/L higher (VCZ-Cmin (mg/L)=1.616 + 0.379×CYP2C19 phenotype+0.015×CRP (mg/L)+0.169×average daily dose/body weight (mg/Kg)) (p < 0.001). To further clarify this finding, we analyzed the data changes of VCZ-Cmin accompanied by inflammatory factors measured at different times in the same patient. The results showed that VCZ-Cmin changes more significantly along with the inflammatory factors in the same direction.
These findings indicate that high inflammation, reflected by CRP, PCT, and IL-6, can affect the pharmacokinetics of VCZ, resulting in an increase in Cmin in patients. This is probably related to inflammation-induced phenoconversion (Shah and Smith, 2015), where elevated amounts of inflammatory cytokines can cause down-regulation of cytochrome P450 isoenzymes at the level of gene transcription, resulting in a decrease in corresponding mRNA, protein, and enzyme activities. Inflammation stimulates the release of cytokines, which can modulate the liver’s transcription factor activities. As a result, the metabolism of VCZ metabolized by CYP2C19 decreases, causing a greater risk of overdose, and hence the VCZ-Cmin increases (Shah and Smith, 2015; Darakjian et al., 2021). We also find that PCT influences VCZ-Cmin. During inflammation, PCT production is increased by stimulating IL-1, IL-6, and TNF-α, which can down-regulate expression enzymes and change the patient’s metabolic phenotype. Our study indicates that VCZ-Cmin is more likely to exceed 5.0 mg/L when PCT is ≥ 0.995 ng/mL (p < 0.001), suggesting that these patients may be more likely to suffer from VCZ toxicity. These findings are consistent with those of Zeng G et al. (Zeng et al., 2020).
Our study found that a multiple linear stepwise regression did not identify PPIs or glucocorticoids as significant covariates for VCZ exposure. These covariates may be due to multiple factors, such as high inflammation and poor metabolism genotype, that could mask the results of drug interactions. Previous studies have shown that VCZ exposure increases with the type of PPI used (Blanco Dorado et al., 2020), but the influence of glucocorticoids on VCZ exposure remains controversial (Li et al., 2017; Li et al., 2018).
Although CPIC® guidelines for VCZ treatment specify therapy and drug alternatives for VCZ based on CYP2C19, other factors affecting VCZ-Cmin have not been considered. In order to achieve the steady-state target VCZ-Cmin quickly, the present study was performed to develop a mathematical model of the VCZ maintenance dose for IFIs adult patients with normal liver function based on multiple risk factors, including the CYP2C19 phenotype, CRP, body weight, and age. This model aims to provide an individualized treatment strategy for VCZ. During periods of high variation in inflammatory factors, the VCZ maintenance dose can be adjusted to reach the target Cmin at any time using the mathematical model. Our study found that satisfactory clinical efficacy can also be achieved when the standard or increased dosing is administered for patients with UM and RM based on multiple factors. The mathematical model developed in this study may be a valuable supplement to CPIC® guidelines for VCZ treatment. We included CRP instead of PCT and IL-6 in the prediction model because some patients lack data on PCT and IL-6. CRP concentrations are widely used as markers for inflammation in daily practice.
In addition to the significant covariates that affect the VCZ maintenance dose mentioned above, several other covariates must be considered. Liver dysfunction affects the clearance of VCZ, and patients with abnormal liver function often experience increased VCZ exposure due to slowed clearance when using conventional doses. The established model is based on patients with normal liver function without considering the effect of liver injury on the dose. Therefore, for patients with abnormal liver function, the dose should be appropriately reduced based on the recommended dose of the model during clinical treatment with VCZ. For individual patients whose VCZ maintenance dose is less than 200 mg/d or more than 600 mg/d, a significant deviation from the predicted dose appears in the established prediction model. There may be some other influencing factors that still need to be discovered.
Our study provides evidence of the variability of inflammatory factors affecting VCZ-Cmin. Consequently, physicians should be aware of this phenomenon to avoid the toxicity caused by increased VCZ concentration due to high inflammation. Based on these significant factors, we have also established a predictive model of the VCZ stable maintenance dose. Proper stable maintenance administration of the predictive model can help patients quickly reach the target Cmin. This model may be a valuable supplement to CPIC® guidelines for VCZ treatment, and awareness of it may improve the benefit/risk ratio of the drug.
The original contributions presented in the study are included in the article/Supplementary Materials, further inquiries can be directed to the corresponding authors.
The studies involving human participants were reviewed and approved by The Investigational Review Board at Zhengzhou Central Hospital Affiliated to Zhengzhou University. The patients/participants provided their written informed consent to participate in this study. Written informed consent was obtained from the individual(s) for the publication of any potentially identifiable images or data included in this article.
LZ, LC, and QX designed the research, analyzed and interpreted the data, performed the statistical analysis, drafted the manuscript, and incorporated feedback from all authors. ML and HL coordinated and managed study data acquisition. LZ, ZS, and HL examined all the specimens and collected clinical data. ZG, YG, HZ, and FQ collected clinical specimens, supervised data acquisition, and analyzed and interpreted data. WC co-conceptualized the study, designed the research, and revised the manuscript. All authors contributed to the article and approved the submitted version.
This work was supported by the Health and Family Planning Commission of Henan Province (LHGJ20210762, LHGJ20191056), the Science and Technology Project of Henan Province (222102310224, 192102310096), Henan Educational Medicine Research Project (Wjlx2021410), and Co-construction Project of Henan Medical Science and Technology (SB201903032).
The authors acknowledge the Translational Medicine Center, the Department of Respiratory Medicine, and the Department of Hematology at Zhengzhou Central Hospital for their assistance with TDM, blood samples, and clinical data collection.
The authors declare that the research was conducted in the absence of any commercial or financial relationships that could be construed as a potential conflict of interest.
All claims expressed in this article are solely those of the authors and do not necessarily represent those of their affiliated organizations, or those of the publisher, the editors and the reviewers. Any product that may be evaluated in this article, or claim that may be made by its manufacturer, is not guaranteed or endorsed by the publisher.
The Supplementary Material for this article can be found online at: https://www.frontiersin.org/articles/10.3389/fcimb.2023.1157944/full#supplementary-material
Supplementary Figure 1 | (A-AB) The values of VCZ-Cmin (mg/L), CRP (mg/L), PCT (ng/mL) and IL-6 (pg/mL) of each patient at different time points. A–AB represent different patients, respectively. The first, second, third, and fourth time points are 3-5 days, 7-10 days, 11-14 days, and 16-20 days after VCZ administration. VCZ-Cmin, voriconazole serum trough concentration; CRP, C-reactive protein; PCT, procalcitonin; IL-6, interleukin-6.
Aiuchi, N., Nakagawa, J., Sakuraba, H., Takahata, T., Kamata, K., Saito, N., et al. (2022). Impact of polymorphisms of pharmacokinetics-related genes and the inflammatory response on the metabolism of voriconazole. Pharmacol.Res. Perspect. 10 (2), e00935. doi: 10.1002/prp2.935
Bassetti, M., Garnacho-Montero, J., Calandra, T., Kullberg, B., Dimopoulos, G., Azoulay, E., et al. (2017). Intensive care medicine research agenda on invasive fungal infection in critically ill patients. Intensive Care Med. 43, 1225–1238. doi: 10.1007/s00134-017-4731-2
Blanco Dorado, S., Maroñas Amigo, O., Latorre-Pellicer, A., Rodriguez Jato, M. T., Lopez-Vizcaino, A., Gomez Marquez, A., et al. (2020). A multicentre prospective study evaluating the impact of proton-pump inhibitors omeprazole and pantoprazole on voriconazole plasma concentrations. Br. J. Clin. Pharmacol. 86 (8), 1661–1666. doi: 10.1111/bcp.14267
Bolcato, L., Khouri, C., Veringa, A., Alffenaar, J. W. C., Yamada, T., Naito, T., et al. (2021). Combined impact of inflammation and pharmacogenomic variants on voriconazole trough concentrations: A meta-analysis of individual data. J. Clin. Med. 10(10), 2089. doi: 10.3390/jcm10102089
Botton, M. R., Whirl-Carrillo, M., Del Tredici, A. L., Sangkuhl, K., Cavallari, L. H., Agundez, J. A. G., et al. (2021). PharmVar geneFocus: CYP2C19. Clin. Pharmacol. Ther. 109 (2), 352–366. doi: 10.1002/cpt.1973
Chen, X., Xiao, Y., Li, H., Huang, Z., Gao, J., Zhang, X, et al. (2022). Therapeutic drug monitoring and CYP2C19 genotyping guide the application of voriconazole in children. Transl. Pediatr. 11 (8), 1311–1322. doi: 10.21037/tp-22-156
Chen, K., Zhang, X. L., Ke, X. Y., Du, G., Yang, K. H., Zhai, S. D. (2018). Individualized medication of voriconazole: A practice guideline of the division of therapeutic drug monitoring, Chinese pharmacological society. Ther. Drug Monit. 40 (6), 663–674. doi: 10.1097/FTD.0000000000000561
Chow, E., Poon, E. W., Fok, B. S., Chan, J. C., Tomlinson, B. (2019). CYP2C19*2 polymorphism is associated with impaired oral clearance of gliclazide in healthy Chinese. Pharmgenomics. Pers. Med. 12, 397–401. doi: 10.2147/PGPM.S226200
Darakjian, L., Deodhar, M., Turgeon, J., Michaud, V. (2021). Chronic inflammatory status observed in patients with type 2 diabetes induces modulation of cytochrome P450 expression and activity. Int. J. .Mol .Sci. 22 (9), 4967. doi: 10.3390/ijms22094967
De Pauw, B., Walsh, T. J., Donnelly, J. P., Stevens, D. A., Edwards, J. E., Calandra, T., et al. (2008). Revised definitions of invasive fungal disease from the European Organization for Research and Treatment of Cancer/Invasive Fungal Infections Cooperative Group and the National Institute of Allergy and Infectious Diseases Mycoses Study Group (EORTC/MSG) Consensus Group. Clin. Infect. Dis. 46 (12), 1813–1821. doi: 10.1086/588660
Dolton, M. J., Mikus, G., Weiss, J., Ray, J. E., Mclachlan, A. J. (2014). Understanding variability with voriconazole using a population pharmacokinetic approach: implications for optimal dosing. J. Antimicrob. Chemother. 69 (6), 1633–1641. doi: 10.1093/jac/dku031
Encalada Ventura, M. A., Van Wanrooy, M. J., Span, L. F., Rodgers, M. G., Van den Heuvel, E. R., Uges, D. R., et al. (2016). Longitudinal analysis of the effect of inflammation on voriconazole trough concentrations. Antimicrob. Agents. Chemother. 60 (5), 2727–2731. doi: 10.1128/AAC.02830-15
Farkas, A., Daroczi, G., Villasurda, P., Dolton, M., Nakagaki, M., Roberts, J. A. (2016). Comparative evaluation of the predictive performances of three different structural population pharmacokinetic models to predict future voriconazole concentrations. Antimicrob. Agents. Chemother. 60 (11), 6806–6812. doi: 10.1128/AAC.00970-16
Jin, H., Wang, T. S., Falcione, B. ,. A., Olsen, K. M., Chen, K., Tang, H., et al. (2016). Trough concentration of voriconazole and its relationship with efficacy and safety: a systematic review and meta-analysis. J. .Amtimicrob. Chemother. 71 (7), 1772–1785. doi: 10.1093/jac/dkw045
Kim, Y., Rhee, S. J., Park, W. B., Yu, K. S., Jang, I. J., Lee, S. (2019). A personalized CYP2C19 phenotype-guided dosing regimen of voriconazole using a population pharmacokinetic analysis. J. Clin. .Med. 8 (2), 227. doi: 10.3390/jcm8020227
Koselke, E., Kraft, S., Smith, J., Nagel, J. (2012). Evaluation of the effect of obesity on voriconazole serum concentrations. J. Antimicrob. Chemother. 67 (12), 2957–2962. doi: 10.1093/jac/dks312
Lestrade, P. P. A., Meis, J. F., Melchers, W. J. G., Verweij, P. E. (2019). Triazole resistance in Aspergillus fumigatus: recent insights and challenges for patient management. Clin. Microbiol. Infect. 25 (7), 799–806. doi: 10.1016/j.cmi.2018.11.027
Li, X., Lai, F., Jiang, Z., Li, M., Chen, Z., Cheng, J., et al. (2022). Effects of inflammation on voriconazole levels: A systematic review. Brit. J. Clin. Pharmaco. 88 (12), 5166–5182. doi: 10.1111/bcp.15495
Li, Z. W., Peng, F. H., Yan, M., Liang, W., Liu, X. L., Wu, Y. Q., et al. (2017). Impact of CYP2C19 genotype and liver function on voriconazole pharmacokinetics in renal transplant recipients. Ther. Drug Monit. 39 (4), 422–428. doi: 10.1097/FTD.0000000000000425
Li, M. X., Zhu, L. Q., Chen, L., Li, N., Qi, F. (2018). Assessment of drug-drug interactions between voriconazole and glucocorticoids. J. Chemother. 30 (5), 296–303. doi: 10.1080/1120009X.2018.1506693
Limper, A. H., Knox, K. S., Sarosi, G. A., Ampel, N. M., Bennett, J. E., Catanzaro, A., et al. (2011). An official American Thoracic Society statement: treatment of fungal infections in adult pulmonary and critical care patients. Am. J.Respir.Crit.Care.Med. 183 (1), 96–128. doi: 10.1164/rccm.2008-740ST
Lortholary, O., Petrikkos, G., Akova, M., Arendrup, M. C., ArikanAkdagli, S., Bassetti, M., et al. (2012). ESCMID guideline for the diagnosis and management of Candida diseases 2012: patients with HIV infection or AIDS. Clin Microbiol Infect. 18 (supply 7), 68–77. doi: 10.1111/1469-0691.12042
Mafuru, M., Wu, S. L., He, S. J., Lu, X. A., Huang, J. G., Jiang, H. L. (2019). The influence of proinflammatory cytokines on voriconazole trough concentration in patients with different forms. J. .Clin. Pharmacol. 59 (10), 1340–1350. doi: 10.1002/jcph.1422
Miao, Q., Tang, J. T., Van Gelder, T., Li, Y. M., Bai, Y. J., Zou, Y. G., et al. (2019). Correlation of CYP2C19 genotype with plasma voriconazole exposure in South-western Chinese Han patients with invasive fungal infections. Medicine(Baltimore) 98 (3), e14137. doi: 10.1097/MD.0000000000014137
Moriyama, B., Obeng, A. O., Barbarino, J., Penzak, S. R., Henning, S. A., Scott, S. A., et al. (2017). Clinical pharmacogenetics implementation consortium (CPIC®) guidelines for CYP2C19 and voriconazole therapy. Clin. Pharmacol. Ther. 102 (1), 45–51. doi: 10.1002/cpt.583
Peman, J., Salavert, M., Canton, E., Jarque, I., Roma, E., Zaragoza, R., et al. (2006). Voriconazole in the management of nosocomial invasive fungal Infections. Ther. Clin. Risk. Manage. 2 (2), 129–158. doi: 10.2147/tcrm.2006.2.2.129
Resendiz-Sharpe, A., Mercier, T., Lestrade, P. P. A., van der Beek, M. T., Von dem Borne, P. A., Cornelissen, J. J., et al. (2019). Prevalence of voriconazole-resistant invasive aspergillosis and its impact on mortality in haematology patients. J. Antinicrob. Chemother. 74, 2759–2766. doi: 10.1093/jac/dkz258
Richards, P. G., Dang, K. M., Kauffman, C. A., Stalker, K. L., Sduekun, D., Kerr, L., et al. (2017). Therapeutic drug monitoring and use of an adjusted body Weight strategy for high-dose voriconazole therapy. J. Antimicrob. Chemother. 72 (4), 1178–1183. doi: 10.1093/jac/dkw550
Ruiz, J., Gordon, M., Villarreal, E., Peruccioni, M., Marques, M. R., Poveda-Andres, J. L., et al. (2019). Impact of voriconazole plasma concentrations on treatment response in critically ill patients. J. Clin. Pharm. Ther. 44 (4), 572–578. doi: 10.1111/jcpt.12817
Schulz, J., Kluwe, F., Mikus, G., Michelet, R., Kloft, C. (2019). Novel insights into the complex pharmacokinetics of voriconazole: a review of its metabolism. Drug Metab. Rev. 51 (3), 247–265. doi: 10.1080/03602532.2019.1632888
Shah, R. R., Smith, R. L. (2015). Inflammation-induced phenoconversion of polymorphic drug metabolizing enzymes: Hypothesis with implications for personalized medicine. Drug Metab. Dispos. 43 (3), 400–410. doi: 10.1124/dmd.114.061093
Takesue, Y., Hanai, Y., Oda, K., Hamada, Y., Ueda, T., Mayumi, T., et al. (2022). Clinical practice guideline for the therapeutic drug monitoring of voriconazole in Non-Asian and Asian adult patients: consensus review by the Japanese society of chemotherapy and the Japanese society of therapeutic drug monitoring. Clin. Ther. 44 (12), 1604–1623. doi: 10.1016/j.clinthera.2022.10.005
Tissot, F., Agrawal, S., Pagano, L., Petrikkos, G., Groll, A. H., Skiada, A., et al. (2017). ECIL-6 guidelines for the treatment of invasive candidiasis, aspergillosis and mucormycosis in leukemia and hematopoietic stemcell transplant patients. Haematologica 102 (3), 433–444. doi: 10.3324/haematol.2016.152900
Ullmann, A. J., Akova, M., Herbrecht, R., Viscoli, C., Arendrup, M. C., Arikan-Akadgli, S., et al. (2012). ESCMID guideline for the diagnosis and management of Candida diseases 2012: adults with haematological malignancies and after haematopoietic stem cell transplantation (HCT). Clin.Microbiol.Infect. 18, 53–67. doi: 10.1111/1469-0691.12041
Valle-T-Figueras, J. M., Renedo Miro, B., Benitez Carabante, M. I., Diaz-de-Heredia, C., Vima Bofarull, J., Mendoza-Palomar, N., et al. (2021). Voriconazole use in children: therapeutic drug monitoring and control of inflammation as key points for optimal treatment. J. Fungi (Basel) 7 (6), 456. doi: 10.3390/jof7060456
Van Wanrooy, M. J., Span, L. F., Rodgers, M. G., Van den Heuvel, E. R., Uges, D. R., van der Werf, T. S., et al. (2014). Inflammation is associated with voriconazole troughConcentrations. Antimicrob. Agents.Chemother. 58 (12), 7098–7101. doi: 10.1128/AAC.03820-14
Wang, T., Zhu, H., Sun, J., Cheng, X., Xie, J., Dong, H., et al. (2014). Efficacy and safety of voriconazole and CYP2C19 polymorphism for optimised dosage regimens in patients with invasive fungal infections. Int. J. Antimicrob. Agents. 44 (5), 436–442. doi: 10.1016/j.ijantimicag.2014.07.013
Xu, R. A., Gu, E. M., Liu, T. H., Ou-Yang, Q. G., Hu, G. X., Cai, J. P. (2018). The effects of cytochrome P450 2C19 polymorphism on the metabolism of voriconazole in vitro. Infect. Drug Resist. 11, 2129–2135. doi: 10.2147/IDR.S179078
Yan, C. G., Xu, J., Liang, C. Y., Wei, Q., Wu, Y. K., Xiong, W., et al. (2018). Radiation dose reduction by using CT with iterative model reconstruction in patients with pulmonary invasive fungal infection. Radiolog 288, 285–292. doi: 10.1148/radiol.2018172107
Zeng, G., Wang, L., Shi, L., Li, H., Zhu, M., Luo, J., et al. (2020). Variability of voriconazole concentrations in patients with hematopoietic stem cell transplantation and hematological malignancies: influence of loading dose, procalcitonin, and pregnane X receptor polymorphisms. Eur. J. Clin. Pharmocol. 76 (4), 515–523. doi: 10.1007/s00228-020-02831-1
Keywords: voriconazole, CYP2C19, prediction model, proinflammatory cytokines, security
Citation: Zhou L, Li M, Li H, Guo Z, Gao Y, Zhang H, Qin F, Sang Z, Xing Q, Cheng L and Cao W (2023) Establishment of a mathematical prediction model for voriconazole stable maintenance dose: a prospective study. Front. Cell. Infect. Microbiol. 13:1157944. doi: 10.3389/fcimb.2023.1157944
Received: 09 February 2023; Accepted: 11 July 2023;
Published: 26 July 2023.
Edited by:
Gaoqian Feng, Nanjing Medical University, ChinaReviewed by:
Jessica K. Roberts, Cognigen, United StatesCopyright © 2023 Zhou, Li, Li, Guo, Gao, Zhang, Qin, Sang, Xing, Cheng and Cao. This is an open-access article distributed under the terms of the Creative Commons Attribution License (CC BY). The use, distribution or reproduction in other forums is permitted, provided the original author(s) and the copyright owner(s) are credited and that the original publication in this journal is cited, in accordance with accepted academic practice. No use, distribution or reproduction is permitted which does not comply with these terms.
*Correspondence: Wei Cao, Y2Fvd2VpeXVAaG90bWFpbC5jb20=; Long Cheng, ZHIuY2hAMTM5LmNvbQ==
Disclaimer: All claims expressed in this article are solely those of the authors and do not necessarily represent those of their affiliated organizations, or those of the publisher, the editors and the reviewers. Any product that may be evaluated in this article or claim that may be made by its manufacturer is not guaranteed or endorsed by the publisher.
Research integrity at Frontiers
Learn more about the work of our research integrity team to safeguard the quality of each article we publish.