- Department of General Practice, The Fourth Affiliated Hospital of Nanjing Medical University, Nanjing, China
The liver is a vital metabolism and detoxification organ of human body, which is involved in the biotransformation and metabolism of the organism. Hepatitis - cirrhosis - liver cancer are significant and common part of liver diseases. The pathogenesis of liver diseases is generally as followed: inflammation and other pathogenic factors cause persistent damage to the liver, leading to the activation of hepatic stellate cells (HSCs) and excessive deposition of extracellular matrix. Patients with chronic hepatitis have a high risk of developing into liver fibrosis, cirrhosis, and even life-threatening liver cancer, which poses a great threat to public health.As the first organ to come into contact with blood from the gut, the liver is profoundly affected by the intestinal flora and its metabolites, with leaky gut and flora imbalance being the triggers of the liver’s pathological response. So far, no one has reviewed the role of intestinal flora in this process from the perspective of the progression of hepatitis-cirrhosis-liver cancer and this article reviews the evidence supporting the effect of intestinal flora in the progression of liver disease.
1 Introduction
The causes of hepatitis are diverse: alcohol, virus, drug-related, etc. According to the World Health Organization, at least 296 million people worldwide have chronic hepatitis B virus (HBV) infection, and the number of deaths caused by cirrhosis and hepatocellular carcinoma due to HBV infection is approximately 820,000 (Sung et al., 2021). The persistent infection of hepatitis B virus is a worldwide public health problem, so this paper focuses on hepatitis B. Chronic hepatitis B (CHB) renders sustaining liver damage, resulting in cirrhosis and even liver cancer as the disease progresses, but not everyone progresses to liver cancer and there are individual differences, suggesting that there are a number of genetic and environmental influences in addition to the disease itself. The intestinal flora has recently been recognised as a major environmental factor influencing the pathogenesis of several human diseases, including cirrhosis and liver cancer (Tilg et al., 2016; Yang et al., 2020).
The intestinal flora is a complex and dynamic community composed of bacteria, fungi, protozoa, vibrios and viruses. Current research mainly focuses on bacteria among these microbiota.There are nine main phyla of intestinal bacteria, of which Firmicutes and Bacteroidetes are the dominant ones. Under normal circumstances, intestinal flora maintains a certain balance, but when the intestinal barrier function changes and the intestinal environment changes,some conditional pathogenic bacteria such as Escherichia coli and Proteus multiply in the intestine, resulting in intestinal flora imbalance and causing body diseases.The liver and intestine communicate bilaterally through the portal vein, bile duct and systemic circulation. The interaction between gut microbiota and gut-liver forms the gut-liver axis. Based on the gut-liver axis, intestinal flora regulates pro-inflammatory changes in the liver and intestine, thereby affecting the development of hepatitis, liver fibrosis, cirrhosis and hepatocellular carcinogenesis (Woodhouse et al., 2018).
A large number of clinical and experimental evidence supports the role of intestinal flora in various liver diseases. However, its role in the development and progression of hepatitis-cirrhosis-hepatocellular carcinogenesis has not been outlined.This article focuses on a review of the laboratory evidence supporting this link.
2 Gut–liver axis and the intestinal barrier
The gut-liver axis is a bidirectional communication pathway composed of intestinal flora, hepatic portal system and biliary system, which is the physiological basis for the interaction between intestinal flora and liver (Yang et al., 2020). The liver is the first organ exposed to blood from the intestine, which accounts for about 70% of the liver blood supply. Under physiological conditions, the liver transmits nutrients to the intestine. The intestinal mucosal barrier ensures the intestinal absorption of nutrients while limiting the absorption of pathogenic bacteria and microbiota-derived molecules ((lipopolysaccharide (LPS), bacterial DNA, flagellin, peptidoglycan, etc.))into the portal circulation and liver. The intestinal mucosal barrier is an anatomically functional structure consisting of (i) a mechanical barrier: intact intestinal mucosal epithelial cells and tight intercellular junctions, (ii) an immune barrier: intestinal-associated lymphoid tissue including: intraepithelial lymphocytes, lymphoid follicles, Peyer’s cells and mesenteric lymph nodes, and (iii) a secretory barrier: mucus secreted by intestinal mucosal cup cells, immunoglobulin (Ig) A and antimicrobial products. These three components make up the intestinal mucosal barrier (Sanduzzi Zamparelli et al., 2017; Guo et al., 2022; Li et al., 2022). However, in a state of liver injury, the intestinal mucosal barrier function is disrupted and the intestinal flora and its metabolites can reach the liver as antigenic signals, inducing inflammatory responses and immune regulation, while the liver influences the number and composition of the intestinal flora by regulating the secretion of bile acids and the signalling pathways mediated by them (Wei et al., 2013; Iannacone and Guidotti, 2022). Intestine flora promotes the progression of liver diseases by regulating proinflammatory/immune signaling pathways (Albhaisi et al., 2020) (Figure 1).
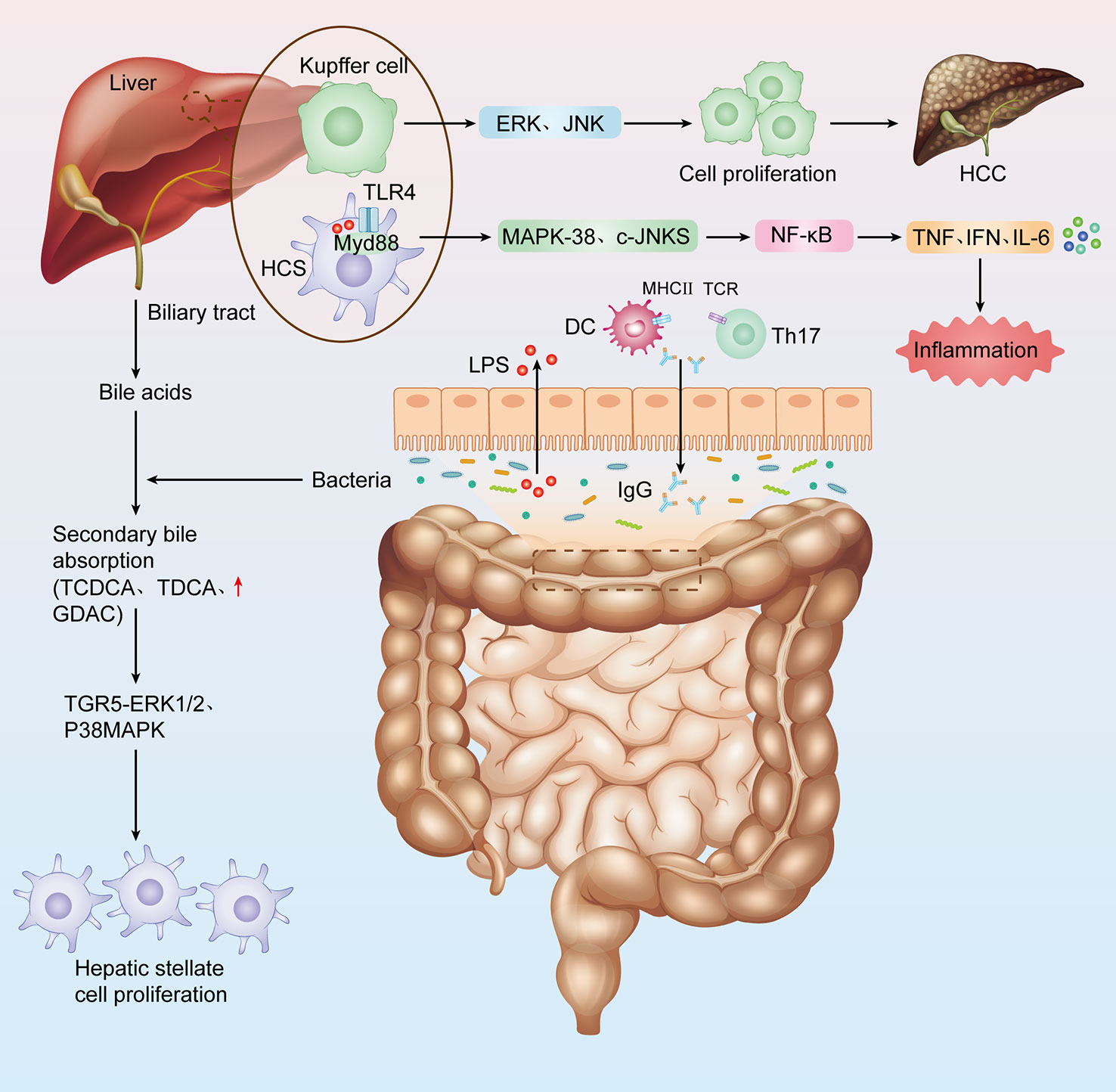
Figure 1 The link between intestinal flora and hepatitis-cirrhosis- Hepatocellular carcinoma. Impaired liver function, decreased bile secretion and albumin synthesis, and portal hypertension all cause decreased intestinal mucosal barrier function, intestinal flora imbalance, increased bacterial load, bacterial translocation, and bacteria and their metabolites aggravate liver injury.
3 Intestinal flora and chronic hepatitis B
Simple HBV infection does not directly cause liver injury,and immune-mediated hepatocellular injury is the main cause of liver injury in chronic viral hepatitis B.The cytotoxic immune response of the host against virus-infected hepatocytes can lead to liver injury, for example, cytotoxic lymphocytes (e.g. natural killer cells) or virus-specific cytotoxic T lymphocytes (CTL) eliminate infected hepatocytes, cytokines secreted by activated lymphocytes and monocytes, and cause hepatocyte destruction while eliminating virus from hepatocytes (Iannacone and Guidotti, 2022). Natural killer cells belong to the innate immune system, but they do not express conventional T-lymphocyte receptors.Instead, they express receptors that recognize the major histocompatibility complex (MHC) that is abnormally expressed by virus-infected cells. These virus-infected cells are destroyed by exocytotic particles(containing apoptosis initiation factors) released by natural killer cells (Bertoletti and Naoumov, 2003; Peter et al., 2015). The CTL is stimulated by a dual signal of the MHC/antigenic peptide/T-lymphocyte receptor complex as the first signal and the combination of CD95 on the target cell and CD95L on the CTL as the second signal, which releases a large number of cytokines, perforins, granuprotease, etc. The perforins released by the CTL can form pores in the hepatocyte membrane, which allow cytokines and granuprotease to enter the cell. CD95/CD95L binding activates a death-inducing signalling complex consisting of many molecules, including Procaspase 8/10, which activates caspase-8 and activates the cascade of caspases,and also induces apoptosis (Wang et al., 2022). In one study, mice treated with vancomycin (Van) and gentamicin (Gen), respectively, were found to have a reduced CD95/CD95L-mediated hepatocyte apoptosis in Gen-treated mice by inhibiting CD95 expression on the surface of hepatocytes. Further using 16sRNA sequencing and correlation analysis, we isolated the five strains with the highest relative abundance from the feces of Gen-treated mice, of which only Bacteroides acidifaciens was protective against liver injury, which may become a new target for the treatment of liver diseases in the future (Kassa et al., 2021; Wang et al., 2022). Recent studies have found that the natural immune response induced by pathogen-associated molecular patterns(PAMPs) generated by dysbiosis of the intestinal flora also plays an important role in the progression of hepatitis B. Pathogen-associated molecular patterns (PAMPs) are a type of pattern recognition receptors (PRRs), and Toll-like receptors (TLRs) are one of the important members of PRRs. PAMPs are recognized by human immune cells by binding to TLRs and induce immune responses(West and Heagy, 2002; Jiao et al., 2020). TLR4 is expressed by a variety of cells in the liver, such as Kupffer cells and hepatic stellate cells (HSC). Dysbiosis of the intestina flora increases endotoxin (LPS) load. LPS binds to LPS binding proteins, and TLR4 recognizes this binding, inducing signaling cascades (e.g., the nuclear factor-κb pathway) and secreting cytokines (e.g., IL1, IL-6, TNF-α), which trigger chronic inflammation. Due to the special anatomical location between the liver and the intestine, which requires frequent exposure to LPS from intestinal sources, and long-term exposure to LPS at low levels,chronic low-level exposure to LPS without signs of inflammation in the healthy liver is a phenomenon known as endotoxin tolerance, on the one hand, because of the relatively low expression of TLR4 and its receptor molecules in the liver, on the other hand, in the case of a healthy organism, the liver negatively regulates Toll-like receptor 4 signaling pathway at different levels (Luedde and Schwabe, 2011). If TLR4 is exposed to increased lipopolysaccharide(LPS) expression, as well as increased TOLL-like receptor 4 sensitivity, disruption of hepatic tolerance may result in an inappropriate immune response, leading to chronic inflammatory liver disease. Other translocated intestinal bacteria can also activate inflammatory responses throughTLRs, and TLR2 is involved in the recognition of the cell wall components peptidoglycan (PGN) and lipophosphate (TA) in Gram-positive bacteria (Akira et al., 2006; Kawai and Akira, 2010). TLR3 recognizes double-stranded RNA (dsRNA) in bacteria,TLR5 recognizes bacterial flagellin and TLR-9 recognizes unmethylated CpG DNA,and TLR7 expressed on conventional dendritic cells (cDC) senses RNA species from bacteria, such as group B Streptococcus,and induces type I interferon (Mancuso et al., 2009; Coban et al., 2010; Kawai and Akira, 2010; Guiducci et al., 2013). The final downstream effect following recognition of the above signals is to induce the production of inflammatory cytokines and type I interferons via NF-κB or IRF7, respectively(Shen et al., 2023). On the other hand, patients with hepatitis have impaired liver function, reduced bile secretion and albumin synthesis, causing edema in the intestinal wall and affecting intestinal blood supply and peristalsis, resulting in intestinal mucosal barrier damage and increased permeability. Impaired intestinal mucosal barrier function can lead to the translocation of bacterial components such as lipopolysaccharide, bacterial DNA, peptidoglycan, extracellular vesicles and their metabolites into the liver, and alteration in the composition and function of intestinal flora (reduced number of beneficial bacteria, reduced colonisation resistance,excessive growth of pathogenic bacteria, etc.), which aggravates liver injury by activating immune cells and inflammatory cells.
In recent years, many scholars have explored the diversity of intestinal flora and the role of its products in hepatitis B (Lu et al., 2011; Zeng et al., 2020; Li et al., 2022). The microbiome of patients with chronic hepatitis B exhibits ecological dysbiosis. Zeng et al. (2020) et al. found in their study that compared with healthy people,the abundance of Firmicutes was lower and that of Bacteroidetes was higher at the phylum level in CHB patients,the potential mechanism may be that Bacteroidetes are dominated by Gram-negative bacteria, which are the main donors for the synthesis of endotoxin LPS,and more endotoxin load promotes the progression of chronic liver disease,while Firmicutes were dominated by Gram-positive bacteria. At the family level, Enterobacteriaceae, a taxon containing a variety of pathogenic bacteria (such as Klebsiella, Escherichia coli, Proteus, Enterobacteriaceae, and other potential pathogenic bacteria), is highly abundant.This may be related to the fact that Enterobacteriaceae are mostly ethanol-producing bacteria, which can regulate autophagy through the IL-17 signaling pathway to induce liver injury (Yuan et al., 2019; Li et al., 2021). Bifidobacteriaceae and Clostridiaceae, two groups composed of many beneficial bacteria, were significantly decreased. Bifidobacteriaceae is one of the most important probiotics, which can benefit human health by reducing plasma and intestinal endotoxin levels, regulating intestinal flora composition, producing antimicrobial factors, improving intestinal barrier function, and regulating local and systemic immunity (Xu et al., 2012; Wan and El-Nezami, 2018). There shows a decrease in Clostridium spp. and Rumenococcus spp. in CHB patients,which resulted in a decrease in the production of short-chain fatty acids (SCFAs).Short-chain fatty acids are a variety of indigestible polysaccharides that are fermented by intestinal bacteria to become absorbable in the intestine, including propionate, butyrate and acetate (Huang et al., 2018; Wang et al., 2018). SCFAs can activate G protein-coupled receptors to trigger intracellular signaling or act as histone deacetylase inhibitors to regulate gene expression (Yu and Zhong, 2022). In addition, SCFAs can also participate in intestinal immunity by regulating the differentiation and activation of Treg cells or proinflammatory TH1 and TH17 cells, plasma cell differentiation and IgA secretion (Zhang et al., 2019). Butyric acid is the most direct source of energy for intestinal mucosal cells and plays an important role in maintaining the homeostasis of the intestinal mucosa (Thibault et al., 2010; Canani et al., 2011; Donohoe et al., 2012). In a study conducted by Pant et al. (2019), it was found that HepG2.2.15 cells (HBV-expressing cells) of CHB patients with a high viral load had higher expression of histone deacetylase (SIRT-1) and lower Ac-p53 levels than CHB patients with a low viral load. Butyrate can increase the expression level of Ac-p53 by inhibiting SIRT-1, and the up-regulated Ac-p53 can inhibit the expression of p-akt and cyclin D1, thus inhibiting cell proliferation, thus we speculate that butyrate may inhibit HBV replication and host cell proliferation through SIRT-1.Interestingly, another study showed that SCFAs (especially butyrate) produced by inulin digestion promote the development of hepatocellular carcinoma (HCC), and inulin induces HCC through early cholestasis, hepatocyte death, and subsequent development of hepatic neutrophilic inflammation (Singh et al., 2018).
4 Intestinal flora and chronic hepatitis C and E
Compared with CHB, the intestinal flora of patients with Chronic hepatitis C(CHC) and Chronic hepatitis E(CHE) has been less studied. HCV infect 130-210 million people worldwide, and HCV is another important risk factor for end-stage liver disease such as cirrhosis and HCC (Pradat et al., 2018). HCV infection is easy to become chronic, about 50-80% of patients become chronic infection, which may be related to the high variability of HCV, its pantropism towards extrahepatic cells and the low titer and weak immunogenicity of HCV in the blood. HCV enters the body and first causes viremia, which appears intermittently throughout the course of the disease. HCV RNA can be detected in blood or liver tissue within the first week. Endotoxemia is also common in patients with chronic hepatitis C, and there is a significant positive correlation between the severity of endotoxemia and viral replication as well as laboratory tests (alanine and aspartate aminotransferase levels, and lobular necrosis) (Sozinov, 2002). Dolganiuc et al. (2007). Found that LPS, IFN-γ, and HCV core protein act synergistically to induce and maintain monocyte/macrophage activation, leading to the loss of the body’s tolerance to TLR and ultimately leading to the persistent inflammatory state in HCV patients. LPS levels are closely related to the persistent inflammatory response in patients with viral hepatitis. On the other hand, HCV can induce a large number of dysfunctional CD4+T cells and CD8+T cells. Immune activation in the host as it clears HCV may lead to chronic inflammation. It is the persistent chronic inflammatory state that leads to the development of progressive fibrosis and cirrhosis (Rehermann and Thimme, 2019; Wang et al., 2022). HCV causes liver damage through direct killing, host immunity (CD4+ T cells and CD8+ T cells), autoimmunity, apoptosis and other mechanisms, with consequent reduction in bile salt secretion and protein synthesis, ultimately leading to bacterial overgrowth and changes in the intestinal microenvironment and microbial community composition (Duboc et al., 2013; Ridlon et al., 2014; Aly et al., 2016). HCV can also play a role in the homeostasis of the intestinal flora by regulating the secretion of bile acids. Heidrich et al. (2018) and Inoue et al. (2018)‘s study found that intestinal flora changes in CHC patients were mainly characterized by an increase in Enterobacteriaceae and Bacteroidetes, but a decrease in Firmicutes. Some pathogenic bacteria, such as Enterobacteriaceae, Staphylococcus, and Enterococci, reduced bile acid levels in HCV-infected patients with cirrhosis. Antiviral treatment with ribavirin (RBV) and the immunomodulator pegylated interferon (PEG-IFN) for HCV has no direct effect on intestinal dysbiosis, but the treatment increases bile acid, which is important for regulating intestinal flora. (Ponziani et al., 2018). Aly et al. (2016) and Preveden et al. (2017)‘s studies shows that HCV patients were more enriched in Prevotella spp. and Faecaliberium spp. while the healthy subjects were more enriched in Ruminococcus spp., Bifidobacterium spp. and Clostridium spp. Possible reasons behind this change in microbial composition: (i) Prevotella plays a role in promoting carbohydrate fermentation, and in HCV patients, impaired digestion and absorption may lead to increased carbohydrate concentrations in the colon. In addition, Prevotella also contains enzymes that play an important role in mucin degradation, which may lead to increased intestinal permeability. (ii) Due to the common embryonic origin of hepatocytes and gastric cells, HCV can also invade gastric cells and infect gastric B lymphocytes, leading to the decrease of IgA secretion (Aly et al., 2016). IgA can regulate the composition and abundance of intestinal microbiome, so reduced IgA secretion leads to increased abundance of Prevotella and other intestinal flora structure and function disorders. In addition, reduced IgA secretion increases intestinal permeability, leading to increased bacterial translocation (Mantis et al., 2011; Milosevic et al., 2021). It has also been suggested that Lactobacillus acidophilus and Bifidobacterium can be used as a complementary treatment to effectively reduce LPS in patients with hepatitis C. Lactitol is known as a prebiotic, and Chen et al. (2007) et al. found that oral administration of lactitol not only modulates gut microbiota, but also reduces plasma LPS in HCV patients more effectively than conventional treatment. It can be used as an effective adjuvant method for the treatment of HCV. A study by Pérez-Matute et al. (2019) found that alpha diversity was not recovered after clearance of hepatitis C virus from the body with novel direct antiviral agents (DAAs) in patients with HCV infection but not yet cirrhosis. However, after 3 months of observation, they found partial recovery of inflammation, alpha diversity in patients with less fibrosis and therefore early initiation of DAA therapy may have a role in improving the dysbiosis of the intestinal flora caused by HCV infection.
The pathogenesis of hepatitis E is not well known. Acute viral hepatitis caused by hepatitis E virus infection is an important community health problem, and hepatitis E is a self-limiting disease with no direct pathogenic effect on hepatocytes. As hepatitis E is transmitted by the faecal-oral route, it may have a devastating effect on the intestinal flora (Lemon et al., 2017). Wu et al. (2022)‘s study found that acute hepatitis E(AHE) was more enriched in Proteobacteria, Gammaproteobacteria, and Enterobacteriaceae compared to healthy controls (HCs). The abundance of Gammaproteobacteria can distinguish AHE patients from HCs and play a role in predicting the severity of AHE patients.In a study by Kreuzer et al. (2012), two groups of sows and piglets were fed a conventional diet and a diet supplemented with healthy probiotics such as Enterococcus faecalis NCIMB 10415, and it was found that the probiotics affected the reduction and clearance of intestinal HEV virus in pigs, which may be related to immune factors, but the exact mechanism remains to be elucidated. Kuss et al. (2011) found in a study that enteroviruses use intestinal microbes to enhance replication and transmission and that antibiotic-mediated microbiota depletion is effective in reducing viral infections.In another study, it was found that reducing the number of bacteria in the host animal significantly reduced the number of hatching T. muris eggs, and thus, intestinal microbes could induce the hatching of intestinal nematodes in mice (Hayes et al., 2010). The above study shows that a variety of pathogens use bacteria (microbiota) to proliferate, so can it be speculated that intestine microbes also play an important role in the proliferation of hepatitis viruses? More research is needed to identify and validate the specific mechanisms by which hepatitis viruses utilize intestine microbes.
5 Intestinal flora and liver cirrhosis
There are two main features of liver cirrhosis: liver structural disorder and portal hypertension, which are mutually causal and promote the progression of the disease. On the one hand, portal hypertension, causing structural changes in the intestinal wall, including vascular congestion, oedema, fibromuscular hyperplasia, thickening of the mucosal muscle layer and reduction or loosening of the tight junctions (TJ), and an increase in the intestinal flora and endotoxin load, leads to the activation of PRR on immune cells, especially macrophages, resulting in the activation of quiescent HSC into activated HSC (Hrncir et al., 2021; Kassa et al., 2021). Subsequently, activated HSC proliferate in response to various cytokines, secreting type I collagen fibres and forming liver fibrosis (Kendall et al., 2009). Moreover, TLR4 is expressed in hepatic stellate cells (HSC), where increased LPS load releases large amounts of LPS-TLR4 pathway-dependent extracellular matrix proteins that promote the progression of liver fibrosis (Kassa et al., 2021), On the other hand, by activating TLR-4, it initiates intrahepatocellular signaling cascades, causing activation of nuclear factor NF-κβ, P38 MAPK and JNK, triggering immune inflammation and sustained inflammatory stimulation, resulting in regenerative repair of hepatocytes after repeated damage and promoting the progression of liver cirrhosis (Zheng et al., 2020). Studies have found that reduced gastric acid secretion and altered bile acid secretion in patients with cirrhosis resulting in impaired intestinal motility, reduced antimicrobial activity of defensins, and reduced mucosal IgA level. Bile acids exert antibacterial effects by directly destroying bacterial cell membranes and indirectly acting as signaling molecules by binding and activating farnesol X receptor (FXR), the nuclear receptor for bile acids (Wiest et al., 2014; Ridlon et al., 2015). FXR also regulates the expression of genes that play a crucial role in preventing bacterial overgrowth and maintaining intestinal epithelial integrity (Inagaki et al., 2006). Interestingly, dysbiosis of intestinal flora alters the levels of bile acid metabolites, with most conjugated bile acids,such as TCDCA, TDCA, and GDCA increasing and TDCA or GDCA promoting the proliferation of hepatic stellate cells and the formation of liver fibrosis by activating the TGR5-ERK1/2 and P38 MAPK signaling pathways (Inagaki et al., 2006). In summary, intestinal flora promotes the development and progression of cirrhosis and its complications by disrupting the mucosal barrier or translocation of intestinal flora and its products.
Qin et al. (2014)showed that Bacteroidetes and Firmicutes dominated the intestinal flora in the feces of the healthy control group and the cirrhosis group at the phylum level.Compared with healthy controls, patients with cirrhosis had fewer Bacteroidetes but more Proteobacteria and Fusobacteria.At the genus level,Bacteroides was the dominant species in both groups,with a significant decrease in the cirrhosis group. Among the remaining genus, Veillonella, Streptococcus, Clostridium and Prevotella were enriched in the cirrhosis group, and Eubacterium and Alistipes were enriched in the healthy control group. Among the 20 species with the highest number increase in the cirrhosis group, four were Streptococcus spp. and six were Clostridium spp. suggesting that these two genus may play an important role in cirrhosis. Of the species with the greatest decrease in number in the cirrhosis group, twelve were Bacteroidetes and seven were Firmicutes, especially from the Clostridium. Interestingly, Enterobacteriaceae and Streptococcaceae were enriched in patients with cirrhosis and positively correlated with Child-Pugh scores, and another study also showed similar results. The microbiota of patients with compensated cirrhosis (Child-Pugh A and B) and decompensated cirrhosis (Child-Pugh C) were significantly different,with significantly higher levels of Proteobacteria, particularly Enterobacteriaceae, in patients with decompensated cirrhosis (Liu et al., 2018). This may be due to the fact that bacteria of Enterobacteriaceae (including Escherichia coli, Klebsiella, Proteus and Enterobacter) are all considered to have PAMPs (Chen et al., 2011; Lu et al., 2011; Bajaj et al., 2012a), among which Proteus mirabilis and Escherichia coli can also activate the NLRP3 inflammasome to promote monocytes to release a large amount of IL-1β and aggravate inflammatory damage (Hirota et al., 2011; Seo et al., 2015; Liao et al., 2019). In terms of functional metabolism, compared with the healthy group, the enrichment of ammonia-producing modules in the cirrhosis group indicates that the intestinal flora produces excessive ammonia, and the GABA biosynthesis module is enriched in the patients, and that the GABA neurotransmitter system is involved in the pathogenesis of human hepatic encephalopathy. Bajaj et al. (2012b) also reached a similar conclusion, they found that specific bacterial families such as Alcaligeneceae, Porphyromonadaceae, Enterobacteriaceae were strongly associated with cognition and inflammation in patients with hepatic encephalopathy. All these findings suggest that dysbiosis of the intestinal flora plays an important role in hepatic encephalopathy, a complication of cirrhosis. With the progress of research, many clinical studies have found that dysbiosis of the intestinal flora also plays an important role in other complications of liver cirrhosis, including ascites formation and infection. Escherichia coli and Streptococcus are significantly enriched in patients with co-infections in cirrhosis (Riordan and Williams, 2006; Chen et al., 2011; Kakiyama et al., 2013).
6 Intestinal flora and hepatocellular carcinoma
The pathogenesis of HCC is diverse and HCC is often characterised by a high level of immune cell infiltration. Therefore, immune status may play a significant role in the progression of HCC. Helper T lymphocytes (Th) 17 are capable of promoting tumour angiogenesis through the production of pro-inflammatory and pro-angiogenic mediators, such as IL17, IL-8 and TNF-a (Kryczek et al., 2009; Su et al., 2010), while the tumour microenvironment also mediates Th17 cell chemotaxis and accumulation at the tumour site (Miyahara et al., 2008; Zou and Restifo, 2010; Ye et al., 2013). IL-17 may enhance the proliferation of hepatocellular carcinoma cells by activating the IL-6/STAT3 pathway (Hu et al., 2017). Th17 levels in the liver of healthy individuals are low and help protect the intestinal mucosa from pathogens, and its differentiation depends on the cellular response to commensal bacteria, and studies have shown that intestinal segmented filamentous bacteria can induce differentiation of Th17 cells by activating intrinsic dendritic cells in the intestinal mucosa (Hu et al., 2017). The flagellin on the surface of Salmonella upregulates the expression of major histocompatibility complex II(MHCII) molecules, CD80 and CD86, by binding to and activating TLR5 on the surface of dendritic cells in the lamina propria of the intestine, and secretes interleukin-23 (IL-23), which promotes the activation of B lymphocytes to secrete immunoglobulins (Cunningham et al., 2004; Sanders et al., 2006; Bates et al., 2009). On the other hand, flagellin as the only ligand of TLR5, stimulates innate immunity. Studies have found a novel bacterial flagellin:CBir1 produced by Clostridium, is recognized by CBir1 T cells and induces TH17 differentiation in the intestine (Liu et al., 2016). In addition, there is an interaction between intestinal flora and programmed death ligand-1 (PD-L1) and cytotoxic T-lymphocyte antigen-4 (CTLA-4). The key role of Bifidobacterium and Bacteroides in blocking the immunostimulatory effects of PD-L1 and CTLA-4, respectively, was identified through the establishment of a mouse tumour model (Sivan et al., 2015; Vétizou et al., 2015). The intestinal flora also affects the immune system through various metabolites that are released into the circulation through the intestinal barrier. For example, SCFAs play important roles in the differentiation and activation of regulatory T cells (Treg cells) or Th17 and 1 cells and immunoglobulin A (IgA) -producing B cells (Zhang et al., 2019; Lee et al., 2020; Yu and Zhong, 2022). Intestinal flora can also signal to immune cells in gut-associated lymphoid tissue and mesenteric lymph nodes by influencing the family of pattern recognition receptors (PRRs) to promote immune responses (Mogensen, 2009; Bermejo-Jambrina et al., 2018). For example, high levels of LPS in the liver activate TOLL-like receptor 4 (TLR4) in Kupffer cells and hepatic stellate cells (HSC), and the LPS-TLR4 pathway is a key initiator of HCC development, acting mainly through: (i) After excessive LPS into the liver, it is recognized by TLR4 on Kupffer cells, and the LPS-TLR4 signal transduction pathway is activated, leading to increased production of inflammatory mediators, promoting liver inflammation and oxidative damage, inhibiting cell apoptosis, and causing DNA damage(Dapito et al., 2012), (ii) Studies have shown that LPS-TLR4-induced activation of ERK and JNK signaling promotes cell proliferation by regulating Bax translocation to mitochondria, which plays a role in promoting the survival and proliferation of hepatocellular carcinoma cells (Wang et al., 2013; Yang et al., 2015), (iii) LPS activates myeloid differentiation protein 88 (MyD88) -dependent LPS-TLR4 signaling pathway by recognizing TLR4 receptor on hepatic endothelial cells (LECs). MyD88 regulates angiogenesis by regulating extracellular proteases, and angiogenesis is a key link in the progression of HCC,allowing cirrhosis to progress to HCC (Jagavelu et al., 2010). LPS can also induce hepatic progenitor cells (HPCs) to differentiate into myofibroblasts. By secreting IL-6 and TNF-α, it further induces the activation of tumor-related signaling pathway Ras and the inactivation of tumor suppressor signaling pathway p53 in HPCs, thereby ultimately promoting the abnormal proliferation and transformation of HPCs and hepatocarcinogenesis (Ren et al., 2019; Gram and Kowalewski, 2022). Gut microbes also control the aggregation of hepatic natural killer T (NKT) cells through the BA-CXCL16-CXCR6 axis, and NKT cells initiate hepatic anti-tumour immune mechanisms through the production of IFN-γ (Zhang et al., 2019). CXCL16 is the only ligand for the chemokine receptor CXCR6, which mediates the accumulation of NKT cells (Garrett, 2019), and primary bile acids increase CXCL16 expression, whereas secondary bile acids show the opposite effect (Ponziani et al., 2018). Whereas intestinal flora mediates the metabolism of bile acids (BA) in the gut, when intestinal flora is dysregulated, hepatic bile acid metabolic homeostasis is disrupted, toxicity levels increase and liver tumour progression is promoted via the BA-CXCL16-CXCR6 axis (Ponziani et al., 2018). In addition, certain bacterial species can be indirect causes of disease by stimulating an inflammatory state, such as inflammation by Enterotoxigenic Bacteroides fragilis (ETBF) induces cancer development through Stat3 and Th17-dependent pathways (Wu et al., 2009; Boleij et al., 2015), while an inflammatory state also increases the production of reactive oxygen species (ROS) which induces DNA damage and promotes tumour progression, e.g. Enterococcus faecalis induces ROS production in colonic epithelial cells to induce DNA damage (Mangerich et al., 2012; Kostic et al., 2013; Sun et al., 2017). Dapito et al. (2012) treated mice with low-dose non-toxic LPS via a subcutaneous osmotic pump for 12 weeks in a mouse model of diethylnitrosamine (DEN) and hepatotoxin carbon tetrachloride (CCl4) induced hepatocellular carcinoma. This low-dose LPS treatment resulted in significant increases in inflammatory gene expression, tumor number, tumor size, and liver weight to body weight ratio, further confirming that our LPS-TLR4 pathway promotes HCC development, including mediating cell proliferation, promoting hepatocyte mitogenic epiregulin expression, and inhibiting apoptosis, suggesting that intestinal flora and TLR4 may be therapeutic targets to prevent liver cancer progression. In another HCC mouse model, the beneficial effects of probiotics on liver immune differentiation and carcinogenesis were confirmed by using a novel probiotic mixture (Prohep). Prohep treatment slowed tumor growth and reduced tumor volume by 40% compared with untreated mice.The levels of Prevotella and Oscilibacter were increased in the fecal microbiota of mice treated with probiotics, indicating that the anti-inflammatory effect of these beneficial bacteria can positively affect hepatocarcinogenesis (Li et al., 2016). We have listed the composition of the intestinal flora in the studies mentioned above on patients with hepatitis, cirrhosis and hepatocellular carcinoma in Table 1.
7 Probiotics in the treatment of hepatic diseases
Probiotics are a group of active microorganisms that have beneficial effects on the host,including: yeasts, Lactobacillus, Bifidobacterium, actinomycetes,etc., and act by modulating the host’s mucosal and systemic immune function or by regulating intestinal inflammation and flora balance.
More and more studies have found that probiotics play a unique role in the treatment of hepatitis-cirrhosis-hepatocellular carcinoma: (i)Probiotics can inhibit the translocation of endotoxin and improve endotoxemia.Through in vivo and in vitro experiments, Dai et al. (2012). found that VSL#3 probiotics increased the expression of tight junction proteins by activating p38 and ERK signaling pathways, protecting the intestinal epithelial barrier, and preventing bacterial products such as endotoxin from entering the portal circulation from the intestine. In another study of patients with HBV and HCV infection, plasma endotoxin levels were decreased by increasing the number of Bifidobacterium and Lactobacillus (Chen et al., 2007). (ii)Probiotics can reduce the toxicity of carcinogens such as aflatoxin B1 (AFB1) and zearalenone (ZEA) and reduce the risk of liver cancer.According to Huang’s research: Bacillus subtilis can degrade AFB1 and ZEA, and a higher degradation rate was observed when Bacillus subtilis, Lactobacillus casein and Candida utilis were mixed in a certain proportion (Huang et al., 2018). (iii) Probiotics can also reduce liver damage by regulating bile acids(BA).In vivo studies showed that Lactobacillus rhamnosus GG (LGG) can be used as a complementary treatment to increase intestinal FGF-15 expression and subsequently reduce hepatic cholesterol 7α-hydroxylase and BA synthesis in mice, and enhance urinary and faecal excretion of BA, preventing liver injury and liver fibrosis induced by excessive BA in mice. In vitro studies also showed that LGG inhibited the inhibitory effect of T-βMCA on the expression of FXR and FGF-19 in Caco-2 cells (Foley et al., 2021). (iv) Probiotic preparations reduce the concentration of ammonia in the arteries, improve hepatic encephalopathy (HE). Application of a probiotic preparation (Clostridium butyricum combined with Bifidobacterium infantis) to treat patients with hepatitis B-related cirrhosis combined with mild hepatic encephalopathy showed that in the probiotic treatment group, Clostridium group I and Bifidobacterium were significantly enriched, while Enterococci and Enterobacteriaceae were decreased. Indicating that probiotics promoted the growth of beneficial flora and decreased the growth of harmful flora, significant reduction in intravenous ammonia was also observed in the probiotic treatment group. In addition, the parameters of intestinal permeability (LPS and D-lactate) and Mucosal damage index (DAO) was significantly improved after probiotic treatment, which may account for the improved cognition and decreased ammonia levels (Xia et al., 2018). (v)Probiotics have beneficial effects on liver immune differentiation and carcinogenesis. Li et al. (2016), in their study establishing a mouse model of HCC, found that feeding Prohep (a novel probiotic mixture) significantly slowed tumour growth and reduced tumour size and weight by 40% compared to the control group. From a mechanistic point of view, the downregulation of IL-17 and the dramatic decrease in the level of its main producer Th17 cells play a key role in tumor suppression after probiotic feeding. Cell staining showed that the decrease in Th17 cells in the tumors of the probiotic treatment group was mainly due to the decreased frequency of migratory Th17 cells from the intestine and peripheral blood. On the other hand, this study also found that probiotics could shift the intestine microbiota toward certain beneficial flora, such as Prevotella and Oscillibacter, which could produce anti-inflammatory cytokines that inhibit Th-17 cells, reduce Th17 polarization, and promote the differentiation of anti-inflammatory Treg/Tr1 cells in the intestine. (vi) In addition, it was found that probiotics enhanced mucosal defense against pathogenic virions (Sanduzzi Zamparelli et al., 2017). Rigo-Adrover et al. (2018) established the suckling rat double- Rotavirus infection mode and found that the probiotic intervention group improved the clinical symptoms after the first infection and significantly reduced the shedding of virus. But all interventions showed a higher viral load after a second infection than the Rotavirus group, enhancing the early immune response to cope with future reinfection. Study has demonstrated that vitamin A plays an anti-norovirus role by regulating specific microbiota(Lactobacillaceae families). In an in vitro model of RAW264.7 cells, Lee et al. identified and demonstrated the antiviral causal effect of Lactobacillus. In a mouse model, the antiviral immune response to murine norovirus was found to be mediated by upregulation of IFN-β (Lee and Ko, 2016). In conclusion, probiotics play a positive effect in the treatment of liver inflammation and cirrhosis, and can effectively prevent the occurrence of liver cancer. It can be used as the key research direction for subsequent clinical work, with extremely broad research prospects.
8 Conclusions
Recent studies have shown that intestinal flora plays an important role in inducing and promoting the process of hepatitis-cirrhosis-hepatocellular carcinoma. Regulation of intestinal flora appears to be a promising therapeutic approach, but intestinal flora is a dynamic microenvironment that is affected by many factors and is difficult to be precisely regulated. More large-scale and in-depth studies are needed to fill the knowledge gap of the role of intestinal flora in liver diseases and its specific mechanism, and to translate basic theory into practical clinical application.
Author contributions
XY was responsible for writing the outline and final review of the manuscript, and SL was responsible for writing and summarizing the entire manuscript and making the charts. All authors contributed to the article and approved the submitted version.
Conflict of interest
The authors declare that the research was conducted in the absence of any commercial or financial relationships that could be construed as a potential conflict of interest.
Publisher’s note
All claims expressed in this article are solely those of the authors and do not necessarily represent those of their affiliated organizations, or those of the publisher, the editors and the reviewers. Any product that may be evaluated in this article, or claim that may be made by its manufacturer, is not guaranteed or endorsed by the publisher.
References
Akira, S., Uematsu, S., Takeuchi, O. (2006). Pathogen recognition and innate immunity. Cell 124 (4), 783–801. doi: 10.1016/j.cell.2006.02.015
Albhaisi, S. A. M., Bajaj, J. S., Sanyal, A. J. (2020). Role of gut microbiota in liver disease. Am. J. Physiol. Gastrointest Liver Physiol. 318 (1), G84–g98. doi: 10.1152/ajpgi.00118.2019
Aly, A. M., Adel, A., El-Gendy, A. O., Essam, T. M., Aziz, R. K. (2016). Gut microbiome alterations in patients with stage 4 hepatitis c. Gut Pathog. 8 (1), 42. doi: 10.1186/s13099-016-0124-2
Bajaj, J. S., Hylemon, P. B., Ridlon, J. M., Heuman, D. M., Daita, K., White, M. B., et al. (2012a). Colonic mucosal microbiome differs from stool microbiome in cirrhosis and hepatic encephalopathy and is linked to cognition and inflammation. Am. J. Physiol. Gastrointest Liver Physiol. 303 (6), G675–G685. doi: 10.1152/ajpgi.00152.2012
Bajaj, J. S., Ridlon, J. M., Hylemon, P. B., Thacker, L. R., Heuman, D. M., Smith, S., et al. (2012b). Linkage of gut microbiome with cognition in hepatic encephalopathy. Am. J. Physiol. Gastrointest Liver Physiol. 302 (1), G168–G175. doi: 10.1152/ajpgi.00190.2011
Bates, J. T., Uematsu, S., Akira, S., Mizel, S. B. (2009). Direct stimulation of tlr5+/+ CD11c+ cells is necessary for the adjuvant activity of flagellin. J. Immunol. 182 (12), 7539–7547. doi: 10.4049/jimmunol.0804225
Bermejo-Jambrina, M., Eder, J., Helgers, L. C., Hertoghs, N., Nijmeijer, B. M., Stunnenberg, M., et al. (2018). C-type lectin receptors in antiviral immunity and viral escape. Front. Immunol. 9, 590. doi: 10.3389/fimmu.2018.00590
Bertoletti, A., Naoumov, N. V. (2003). Translation of immunological knowledge into better treatments of chronic hepatitis b. J. Hepatol. 39 (1), 115–124. doi: 10.1016/S0168-8278(03)00126-0
Boleij, A., Hechenbleikner, E. M., Goodwin, A. C., Badani, R., Stein, E. M., Lazarev, M. G., et al. (2015). The bacteroides fragilis toxin gene is prevalent in the colon mucosa of colorectal cancer patients. Clin. Infect. Dis. 60 (2), 208–215. doi: 10.1093/cid/ciu787
Canani, R. B., Costanzo, M. D., Leone, L., Pedata, M., Meli, R., Calignano, A. (2011). Potential beneficial effects of butyrate in intestinal and extraintestinal diseases. World J. Gastroenterol. 17 (12), 1519–1528. doi: 10.3748/wjg.v17.i12.1519
Chen, C., Li, L., Wu, Z., Chen, H., Fu, S. (2007). Effects of lactitol on intestinal microflora and plasma endotoxin in patients with chronic viral hepatitis. J. Infect. 54 (1), 98–102. doi: 10.1016/j.jinf.2005.11.013
Chen, Y., Yang, F., Lu, H., Wang, B., Chen, Y., Lei, D., et al. (2011). Characterization of fecal microbial communities in patients with liver cirrhosis. Hepatology 54 (2), 562–572. doi: 10.1002/hep.24423
Coban, C., Igari, Y., Yagi, M., Reimer, T., Koyama, S., Aoshi, T., et al. (2010). Immunogenicity of whole-parasite vaccines against plasmodium falciparum involves malarial hemozoin and host TLR9. Cell Host Microbe 7 (1), 50–61. doi: 10.1016/j.chom.2009.12.003
Cunningham, A. F., Khan, M., Ball, J., Toellner, K. M., Serre, K., Mohr, E., et al. (2004). Responses to the soluble flagellar protein FliC are Th2, while those to FliC on salmonella are Th1. Eur. J. Immunol. 34 (11), 2986–2995. doi: 10.1002/eji.200425403
Dai, C., Zhao, D. H., Jiang, M. (2012). VSL3 probiotics regulate the intestinal epithelial barrier in vivo and in vitro via the p38 and ERK signaling pathways. Int. J. Mol. Med. 29 (2), 202–208. doi: 10.3892/ijmm.2011.839
Dapito, D. H., Mencin, A., Gwak, G. Y., Pradere, J. P., Jang, M. K., Mederacke, I., et al. (2012). Promotion of hepatocellular carcinoma by the intestinal microbiota and TLR4. Cancer Cell 21 (4), 504–516. doi: 10.1016/j.ccr.2012.02.007
Dolganiuc, A., Norkina, O., Kodys, K., Catalano, D., Bakis, G., Marshall, C., et al. (2007). Viral and host factors induce macrophage activation and loss of toll-like receptor tolerance in chronic HCV infection. Gastroenterology 133 (5), 1627–1636. doi: 10.1053/j.gastro.2007.08.003
Donohoe, D. R., Collins, L. B., Wali, A., Bigler, R., Sun, W., Bultman, S. J. (2012). The warburg effect dictates the mechanism of butyrate-mediated histone acetylation and cell proliferation. Mol. Cell 48 (4), 612–626. doi: 10.1016/j.molcel.2012.08.033
Duboc, H., Rajca, S., Rainteau, D., Benarous, D., Maubert, M. A., Quervain, E., et al. (2013). Connecting dysbiosis, bile-acid dysmetabolism and gut inflammation in inflammatory bowel diseases. Gut 62 (4), 531–539. doi: 10.1136/gutjnl-2012-302578
Foley, M. H., O'Flaherty, S., Allen, G., Rivera, A. J., Stewart, A. K., Barrangou, R., et al. (2021). Lactobacillus bile salt hydrolase substrate specificity governs bacterial fitness and host colonization. Proc. Natl. Acad. Sci. U.S.A. 118 (6), e2017709118. doi: 10.1073/pnas.2017709118
Garrett, W. S. (2019). The gut microbiota and colon cancer. Science 364 (6446), 1133–1135. doi: 10.1126/science.aaw2367
Gram, A., Kowalewski, M. P. (2022). Molecular mechanisms of lipopolysaccharide (LPS) induced inflammation in an immortalized ovine luteal endothelial cell line (OLENDO). Vet. Sci. 9 (3), 99. doi: 10.3390/vetsci9030099
Guiducci, C., Gong, M., Cepika, A. M., Xu, Z., Tripodo, C., Bennett, L., et al. (2013). RNA Recognition by human TLR8 can lead to autoimmune inflammation. J. Exp. Med. 210 (13), 2903–2919. doi: 10.1084/jem.20131044
Guo, X., Okpara, E. S., Hu, W., Yan, C., Wang, Y., Liang, Q., et al. (2022). Interactive relationships between intestinal flora and bile acids. Int. J. Mol. Sci. 23 (15), 8343. doi: 10.3390/ijms23158343
Hayes, K. S., Bancroft, A. J., Goldrick, M., Portsmouth, C., Roberts, I. S., Grencis, R. K. (2010). Exploitation of the intestinal microflora by the parasitic nematode trichuris muris. Science 328 (5984), 1391–1394. doi: 10.1126/science.1187703
Heidrich, B., Vital, M., Plumeier, I., Döscher, N., Kahl, S., Kirschner, J., et al. (2018). Intestinal microbiota in patients with chronic hepatitis c with and without cirrhosis compared with healthy controls. Liver Int. 38 (1), 50–58. doi: 10.1111/liv.13485
Hirota, S. A., Ng, J., Lueng, A., Khajah, M., Parhar, K., Li, Y., et al. (2011). NLRP3 inflammasome plays a key role in the regulation of intestinal homeostasis. Inflammation Bowel Dis. 17 (6), 1359–1372. doi: 10.1002/ibd.21478
Hrncir, T., Hrncirova, L., Kverka, M., Hromadka, R., Machova, V., Trckova, E., et al. (2021). Gut microbiota and NAFLD: Pathogenetic mechanisms, microbiota signatures, and therapeutic interventions. Microorganisms 9 (5), 957. doi: 10.3390/microorganisms9050957
Hu, Z., Luo, D., Wang, D., Ma, L., Zhao, Y., Li, L. (2017). IL-17 activates the IL-6/STAT3 signal pathway in the proliferation of hepatitis b virus-related hepatocellular carcinoma. Cell Physiol. Biochem. 43 (6), 2379–2390. doi: 10.1159/000484390
Huang, W., Chang, J., Wang, P., Liu, C., Yin, Q., Zhu, Q., et al. (2018). Effect of the combined compound probiotics with mycotoxin-degradation enzyme on detoxifying aflatoxin B(1) and zearalenone. J. Toxicol. Sci. 43 (6), 377–385. doi: 10.2131/jts.43.377
Huang, Y., Li, S. C., Hu, J., Ruan, H. B., Guo, H. M., Zhang, H. H., et al. (2018). Gut microbiota profiling in han Chinese with type 1 diabetes. Diabetes Res. Clin. Pract. 141, 256–263. doi: 10.1016/j.diabres.2018.04.032
Iannacone, M., Guidotti, L. G. (2022). Immunobiology and pathogenesis of hepatitis b virus infection. Nat. Rev. Immunol. 22 (1), 19–32. doi: 10.1038/s41577-021-00549-4
Inagaki, T., Moschetta, A., Lee, Y. K., Peng, L., Zhao, G., Downes, M., et al. (2006). Regulation of antibacterial defense in the small intestine by the nuclear bile acid receptor. Proc. Natl. Acad. Sci. U.S.A. 103 (10), 3920–3925. doi: 10.1073/pnas.0509592103
Inoue, T., Nakayama, J., Moriya, K., Kawaratani, H., Momoda, R., Ito, K., et al. (2018). Gut dysbiosis associated with hepatitis c virus infection. Clin. Infect. Dis. 67 (6), 869–877. doi: 10.1093/cid/ciy205
Jagavelu, K., Routray, C., Shergill, U., O'Hara, S. P., Faubion, W., Shah, V. H. (2010). Endothelial cell toll-like receptor 4 regulates fibrosis-associated angiogenesis in the liver. Hepatology 52 (2), 590–601. doi: 10.1002/hep.23739
Jiao, Y., Wu, L., Huntington, N. D., Zhang, X. (2020). Crosstalk between gut microbiota and innate immunity and its implication in autoimmune diseases. Front. Immunol. 11, 282. doi: 10.3389/fimmu.2020.00282
Kakiyama, G., Pandak, W. M., Gillevet, P. M., Hylemon, P. B., Heuman, D. M., Daita, K., et al. (2013). Modulation of the fecal bile acid profile by gut microbiota in cirrhosis. J. Hepatol. 58 (5), 949–955. doi: 10.1016/j.jhep.2013.01.003
Kassa, Y., Million, Y., Gedefie, A., Moges, F. (2021). Alteration of gut microbiota and its impact on immune response in patients with chronic HBV infection: A review. Infect. Drug Resist. 14, 2571–2578. doi: 10.2147/IDR.S305901
Kawai, T., Akira, S. (2010). The role of pattern-recognition receptors in innate immunity: Update on toll-like receptors. Nat. Immunol. 11 (5), 373–384. doi: 10.1038/ni.1863
Kendall, T. J., Hennedige, S., Aucott, R. L., Hartland, S. N., Vernon, M. A., Benyon, R. C., et al. (2009). p75 neurotrophin receptor signaling regulates hepatic myofibroblast proliferation and apoptosis in recovery from rodent liver fibrosis. Hepatology 49 (3), 901–910. doi: 10.1002/hep.22701
Kostic, A. D., Chun, E., Robertson, L., Glickman, J. N., Gallini, C. A., Michaud, M., et al. (2013). Fusobacterium nucleatum potentiates intestinal tumorigenesis and modulates the tumor-immune microenvironment. Cell Host Microbe 14 (2), 207–215. doi: 10.1016/j.chom.2013.07.007
Kreuzer, S., Machnowska, P., Aßmus, J., Sieber, M., Pieper, R., Schmidt, M. F., et al. (2012). Feeding of the probiotic bacterium enterococcus faecium NCIMB 10415 differentially affects shedding of enteric viruses in pigs. Vet. Res. 43 (1), 58. doi: 10.1186/1297-9716-43-58
Kryczek, I., Banerjee, M., Cheng, P., Vatan, L., Szeliga, W., Wei, S., et al. (2009). Phenotype, distribution, generation, and functional and clinical relevance of Th17 cells in the human tumor environments. Blood 114 (6), 1141–1149. doi: 10.1182/blood-2009-03-208249
Kuss, S. K., Best, G. T., Etheredge, C. A., Pruijssers, A. J., Frierson, J. M., Hooper, L. V., et al. (2011). Intestinal microbiota promote enteric virus replication and systemic pathogenesis. Science 334 (6053), 249–252. doi: 10.1126/science.1211057
Lee, J., d'Aigle, J., Atadja, L., Quaicoe, V., Honarpisheh, P., Ganesh, B. P., et al. (2020). Gut microbiota-derived short-chain fatty acids promote poststroke recovery in aged mice. Circ. Res. 127 (4), 453–465. doi: 10.1161/CIRCRESAHA.119.316448
Lee, H., Ko, G. (2016). Antiviral effect of vitamin a on norovirus infection via modulation of the gut microbiome. Sci. Rep. 6, 25835. doi: 10.1038/srep25835
Lemon, S. M., Ott, J. J., Van Damme, P., Shouval, D. (2017). Type a viral hepatitis: A summary and update on the molecular virology, epidemiology, pathogenesis and prevention. J. Hepatol. 5. doi: 10.1016/j.jhep.2017.08.034
Li, S., Han, W., He, Q., Zhang, W., Zhang, Y. (2022). Relationship between intestinal microflora and hepatocellular cancer based on gut-liver axis theory. Contrast Media Mol. Imaging 2022, 6533628. doi: 10.1155/2022/6533628
Li, Y. N., Kang, N. L., Jiang, J. J., Zhu, Y. Y., Liu, Y. R., Zeng, D. W., et al. (2022). Gut microbiota of hepatitis b virus-infected patients in the immune-tolerant and immune-active phases and their implications in metabolite changes. World J. Gastroenterol. 28 (35), 5188–5202. doi: 10.3748/wjg.v28.i35.5188
Li, N. N., Li, W., Feng, J. X., Zhang, W. W., Zhang, R., Du, S. H., et al. (2021). High alcohol-producing klebsiella pneumoniae causes fatty liver disease through 2,3-butanediol fermentation pathway in vivo. Gut Microbes 13 (1), 1979883. doi: 10.1080/19490976.2021.1979883
Li, J., Sung, C. Y., Lee, N., Ni, Y., Pihlajamäki, J., Panagiotou, G., et al. (2016). Probiotics modulated gut microbiota suppresses hepatocellular carcinoma growth in mice. Proc. Natl. Acad. Sci. U.S.A. 113 (9), E1306–E1315. doi: 10.1073/pnas.1518189113
Liao, L., Schneider, K. M., Galvez, E. J. C., Frissen, M., Marschall, H. U., Su, H., et al. (2019). Intestinal dysbiosis augments liver disease progression via NLRP3 in a murine model of primary sclerosing cholangitis. Gut 68 (8), 1477–1492. doi: 10.1136/gutjnl-2018-316670
Liu, H., Chen, F., Wu, W., Cao, A. T., Xue, X., Yao, S., et al. (2016). TLR5 mediates CD172α(+) intestinal lamina propria dendritic cell induction of Th17 cells. Sci. Rep. 6, 22040. doi: 10.1038/srep22040
Liu, Y., Li, J., Jin, Y., Zhao, L., Zhao, F., Feng, J., et al. (2018). Splenectomy leads to amelioration of altered gut microbiota and metabolome in liver cirrhosis patients. Front. Microbiol. 9, 963. doi: 10.3389/fmicb.2018.00963
Lu, H., Wu, Z., Xu, W., Yang, J., Chen, Y., Li, L. (2011). Intestinal microbiota was assessed in cirrhotic patients with hepatitis b virus infection. intestinal microbiota of HBV cirrhotic patients. Microb. Ecol. 61 (3), 693–703. doi: 10.1007/s00248-010-9801-8
Luedde, T., Schwabe, R. F. (2011). NF-κB in the liver–linking injury, fibrosis and hepatocellular carcinoma. Nat. Rev. Gastroenterol. Hepatol. 8 (2), 108–118. doi: 10.1038/nrgastro.2010.213
Mancuso, G., Gambuzza, M., Midiri, A., Biondo, C., Papasergi, S., Akira, S., et al. (2009). Bacterial recognition by TLR7 in the lysosomes of conventional dendritic cells. Nat. Immunol. 10 (6), 587–594. doi: 10.1038/ni.1733
Mangerich, A., Knutson, C. G., Parry, N. M., Muthupalani, S., Ye, W., Prestwich, E., et al. (2012). Infection-induced colitis in mice causes dynamic and tissue-specific changes in stress response and DNA damage leading to colon cancer. Proc. Natl. Acad. Sci. U.S.A. 109 (27), E1820–E1829. doi: 10.1073/pnas.1207829109
Mantis, N. J., Rol, N., Corthésy, B. (2011). Secretory IgA's complex roles in immunity and mucosal homeostasis in the gut. Mucosal Immunol. 4 (6), 603–611. doi: 10.1038/mi.2011.41
Milosevic, I., Russo, E., Vujovic, A., Barac, A., Stevanovic, O., Gitto, S., et al. (2021). Microbiota and viral hepatitis: State of the art of a complex matter. World J. Gastroenterol. 27 (33), 5488–5501. doi: 10.3748/wjg.v27.i33.5488
Miyahara, Y., Odunsi, K., Chen, W., Peng, G., Matsuzaki, J., Wang, R. F. (2008). Generation and regulation of human CD4+ IL-17-producing T cells in ovarian cancer. Proc. Natl. Acad. Sci. U.S.A. 105 (40), 15505–15510. doi: 10.1073/pnas.0710686105
Mogensen, T. H. (2009). Pathogen recognition and inflammatory signaling in innate immune defenses. Clin. Microbiol. Rev. 22 (2), 240–273. doi: 10.1128/CMR.00046-08
Pant, K., Mishra, A. K., Pradhan, S. M., Nayak, B., Das, P., Shalimar, D., et al. (2019). Butyrate inhibits HBV replication and HBV-induced hepatoma cell proliferation via modulating SIRT-1/Ac-p53 regulatory axis. Mol. Carcinog 58 (4), 524–532. doi: 10.1002/mc.22946
Pérez-Matute, P., Íñiguez, M., Villanueva-Millán, M. J., Recio-Fernández, E., Vázquez, A. M., Sánchez, S. C., et al. (2019). Short-term effects of direct-acting antiviral agents on inflammation and gut microbiota in hepatitis c-infected patients. Eur. J. Intern. Med. 67, 47–58. doi: 10.1016/j.ejim.2019.06.005
Peter, M. E., Hadji, A., Murmann, A. E., Brockway, S., Putzbach, W., Pattanayak, A., et al. (2015). The role of CD95 and CD95 ligand in cancer. Cell Death Differ 22 (4), 549–559. doi: 10.1038/cdd.2015.3
Ponziani, F. R., Putignani, L., Paroni Sterbini, F., Petito, V., Picca, A., Del Chierico, F., et al. (2018). Influence of hepatitis c virus eradication with direct-acting antivirals on the gut microbiota in patients with cirrhosis. Aliment Pharmacol. Ther. 48 (11-12), 1301–1311. doi: 10.1111/apt.15004
Pradat, P., Virlogeux, V., Trépo, E. (2018). Epidemiology and elimination of HCV-related liver disease. Viruses 10 (10), 545. doi: 10.3390/v10100545
Preveden, T., Scarpellini, E., Milić, N., Luzza, F., Abenavoli, L. (2017). Gut microbiota changes and chronic hepatitis c virus infection. Expert Rev. Gastroenterol. Hepatol. 11 (9), 813–819. doi: 10.1080/17474124.2017.1343663
Qin, N., Yang, F., Li, A., Prifti, E., Chen, Y., Shao, L., et al. (2014). Alterations of the human gut microbiome in liver cirrhosis. Nature 513 (7516), 59–64. doi: 10.1038/nature13568
Rehermann, B., Thimme, R. (2019). Insights from antiviral therapy into immune responses to hepatitis b and c virus infection. Gastroenterology 156 (2), 369–383. doi: 10.1053/j.gastro.2018.08.061
Ren, Z., Li, A., Jiang, J., Zhou, L., Yu, Z., Lu, H., et al. (2019). Gut microbiome analysis as a tool towards targeted non-invasive biomarkers for early hepatocellular carcinoma. Gut 68 (6), 1014–1023. doi: 10.1136/gutjnl-2017-315084
Ridlon, J. M., Kang, D. J., Hylemon, P. B., Bajaj, J. S. (2014). Bile acids and the gut microbiome. Curr. Opin. Gastroenterol. 30 (3), 332–338. doi: 10.1097/MOG.0000000000000057
Ridlon, J. M., Kang, D. J., Hylemon, P. B., Bajaj, J. S. (2015). Gut microbiota, cirrhosis, and alcohol regulate bile acid metabolism in the gut. Dig Dis. 33 (3), 338–345. doi: 10.1159/000371678
Rigo-Adrover, M. D. M., van Limpt, K., Knipping, K., Garssen, J., Knol, J., Costabile, A., et al. (2018). Preventive effect of a synbiotic combination of galacto- and fructooligosaccharides mixture with bifidobacterium breve m-16V in a model of multiple rotavirus infections. Front. Immunol. 9, 1318. doi: 10.3389/fimmu.2018.01318
Riordan, S. M., Williams, R. (2006). The intestinal flora and bacterial infection in cirrhosis. J. Hepatol. 45 (5), 744–757. doi: 10.1016/j.jhep.2006.08.001
Sanders, C. J., Yu, Y., Moore, D. A., 3rd, Williams, I. R., Gewirtz, A. T. (2006). Humoral immune response to flagellin requires T cells and activation of innate immunity. J. Immunol. 177 (5), 2810–2818. doi: 10.4049/jimmunol.177.5.2810
Sanduzzi Zamparelli, M., Rocco, A., Compare, D., Nardone, G. (2017). The gut microbiota: A new potential driving force in liver cirrhosis and hepatocellular carcinoma. United Eur. Gastroenterol. J. 5 (7), 944–953. doi: 10.1177/2050640617705576
Seo, S. U., Kamada, N., Muñoz-Planillo, R., Kim, Y. G., Kim, D., Koizumi, Y., et al. (2015). Distinct commensals induce interleukin-1β via NLRP3 inflammasome in inflammatory monocytes to promote intestinal inflammation in response to injury. Immunity 42 (4), 744–755. doi: 10.1016/j.immuni.2015.03.004
Shen, Y., Wu, S. D., Chen, Y., Li, X. Y., Zhu, Q., Nakayama, K., et al. (2023). Alterations in gut microbiome and metabolomics in chronic hepatitis b infection-associated liver disease and their impact on peripheral immune response. Gut Microbes 15 (1), 2155018. doi: 10.1080/19490976.2022.2155018
Singh, V., Yeoh, B. S., Chassaing, B., Xiao, X., Saha, P., Aguilera Olvera, R., et al. (2018). Dysregulated microbial fermentation of soluble fiber induces cholestatic liver cancer. Cell 175 (3), 679–694.e622. doi: 10.1016/j.cell.2018.09.004
Sivan, A., Corrales, L., Hubert, N., Williams, J. B., Aquino-Michaels, K., Earley, Z. M., et al. (2015). Commensal bifidobacterium promotes antitumor immunity and facilitates anti-PD-L1 efficacy. Science 350 (6264), 1084–1089. doi: 10.1126/science.aac4255
Sozinov, A. S. (2002). Systemic endotoxemia during chronic viral hepatitis. Bull. Exp. Biol. Med. 133 (2), 153–155. doi: 10.1023/A:1015546821875
Su, X., Ye, J., Hsueh, E. C., Zhang, Y., Hoft, D. F., Peng, G. (2010). Tumor microenvironments direct the recruitment and expansion of human Th17 cells. J. Immunol. 184 (3), 1630–1641. doi: 10.4049/jimmunol.0902813
Sun, M., Wu, W., Liu, Z., Cong, Y. (2017). Microbiota metabolite short chain fatty acids, GPCR, and inflammatory bowel diseases. J. Gastroenterol. 52 (1), 1–8. doi: 10.1007/s00535-016-1242-9
Sung, H., Ferlay, J., Siegel, R. L., Laversanne, M., Soerjomataram, I., Jemal, A., et al. (2021). Global cancer statistics 2020: GLOBOCAN estimates of incidence and mortality worldwide for 36 cancers in 185 countries. CA Cancer J. Clin. 71 (3), 209–249. doi: 10.3322/caac.21660
Thibault, R., Blachier, F., Darcy-Vrillon, B., de Coppet, P., Bourreille, A., Segain, J. P. (2010). Butyrate utilization by the colonic mucosa in inflammatory bowel diseases: A transport deficiency. Inflammation Bowel Dis. 16 (4), 684–695. doi: 10.1002/ibd.21108
Tilg, H., Cani, P. D., Mayer, E. A. (2016). Gut microbiome and liver diseases. Gut 65 (12), 2035–2044. doi: 10.1136/gutjnl-2016-312729
Vétizou, M., Pitt, J. M., Daillère, R., Lepage, P., Waldschmitt, N., Flament, C., et al. (2015). Anticancer immunotherapy by CTLA-4 blockade relies on the gut microbiota. Science 350 (6264), 1079–1084. doi: 10.1126/science.aad1329
Wan, M. L. Y., El-Nezami, H. (2018). Targeting gut microbiota in hepatocellular carcinoma: Probiotics as a novel therapy. Hepatobiliary Surg. Nutr. 7 (1), 11–20. doi: 10.21037/hbsn.2017.12.07
Wang, L., Cao, Z. M., Zhang, L. L., Li, J. M., Lv, W. L. (2022). The role of gut microbiota in some liver diseases: From an immunological perspective. Front. Immunol. 13, 923599. doi: 10.3389/fimmu.2022.923599
Wang, Y., Gao, X., Ghozlane, A., Hu, H., Li, X., Xiao, Y., et al. (2018). Characteristics of faecal microbiota in paediatric crohn's disease and their dynamic changes during infliximab therapy. J. Crohns Colitis 12 (3), 337–346. doi: 10.1093/ecco-jcc/jjx153
Wang, H., Wang, Q., Yang, C., Guo, M., Cui, X., Jing, Z., et al. (2022). Bacteroides acidifaciens in the gut plays a protective role against CD95-mediated liver injury. Gut Microbes 14 (1), 2027853. doi: 10.1080/19490976.2022.2027853
Wang, L., Zhu, R., Huang, Z., Li, H., Zhu, H. (2013). Lipopolysaccharide-induced toll-like receptor 4 signaling in cancer cells promotes cell survival and proliferation in hepatocellular carcinoma. Dig Dis. Sci. 58 (8), 2223–2236. doi: 10.1007/s10620-013-2745-3
Wei, X., Yan, X., Zou, D., Yang, Z., Wang, X., Liu, W., et al. (2013). Abnormal fecal microbiota community and functions in patients with hepatitis b liver cirrhosis as revealed by a metagenomic approach. BMC Gastroenterol. 13, 175. doi: 10.1186/1471-230X-13-175
West, M. A., Heagy, W. (2002). Endotoxin tolerance: A review. Crit. Care Med. 30 (1 Suppl), S64–S73. doi: 10.1097/00003246-200201001-00009
Wiest, R., Lawson, M., Geuking, M. (2014). Pathological bacterial translocation in liver cirrhosis. J. Hepatol. 60 (1), 197–209. doi: 10.1016/j.jhep.2013.07.044
Woodhouse, C. A., Patel, V. C., Singanayagam, A., Shawcross, D. L. (2018). Review article: The gut microbiome as a therapeutic target in the pathogenesis and treatment of chronic liver disease. Aliment Pharmacol. Ther. 47 (2), 192–202. doi: 10.1111/apt.14397
Wu, J., Bortolanza, M., Zhai, G., Shang, A., Ling, Z., Jiang, B., et al. (2022). Gut microbiota dysbiosis associated with plasma levels of interferon-γ and viral load in patients with acute hepatitis e infection. J. Med. Virol. 94 (2), 692–702. doi: 10.1002/jmv.27356
Wu, S., Rhee, K. J., Albesiano, E., Rabizadeh, S., Wu, X., Yen, H. R., et al. (2009). A human colonic commensal promotes colon tumorigenesis via activation of T helper type 17 T cell responses. Nat. Med. 15 (9), 1016–1022. doi: 10.1038/nm.2015
Xia, X., Chen, J., Xia, J., Wang, B., Liu, H., Yang, L., et al. (2018). Role of probiotics in the treatment of minimal hepatic encephalopathy in patients with HBV-induced liver cirrhosis. J. Int. Med. Res. 46 (9), 3596–3604. doi: 10.1177/0300060518776064
Xu, M., Wang, B., Fu, Y., Chen, Y., Yang, F., Lu, H., et al. (2012). Changes of fecal bifidobacterium species in adult patients with hepatitis b virus-induced chronic liver disease. Microb. Ecol. 63 (2), 304–313. doi: 10.1007/s00248-011-9925-5
Yang, J., Li, M., Zheng, Q. C. (2015). Emerging role of toll-like receptor 4 in hepatocellular carcinoma. J. Hepatocell Carcinoma 2, 11–17. doi: 10.2147/JHC.S44515
Yang, X., Lu, D., Zhuo, J., Lin, Z., Yang, M., Xu, X. (2020). The gut-liver axis in immune remodeling: New insight into liver diseases. Int. J. Biol. Sci. 16 (13), 2357–2366. doi: 10.7150/ijbs.46405
Yang, X. A., Lv, F., Wang, R., Chang, Y., Zhao, Y., Cui, X., et al. (2020). Potential role of intestinal microflora in disease progression among patients with different stages of hepatitis b. Gut Pathog. 12, 50. doi: 10.1186/s13099-020-00391-4
Ye, J., Livergood, R. S., Peng, G. (2013). The role and regulation of human Th17 cells in tumor immunity. Am. J. Pathol. 182 (1), 10–20. doi: 10.1016/j.ajpath.2012.08.041
Yu, Y., Zhong, W. (2022). Interaction of microbiome and immunity in tumorigenesis and clinical treatment. BioMed. Pharmacother. 156, 113894. doi: 10.1016/j.biopha.2022.113894
Yuan, J., Chen, C., Cui, J., Lu, J., Yan, C., Wei, X., et al. (2019). Fatty liver disease caused by high-Alcohol-Producing klebsiella pneumoniae. Cell Metab. 30 (4), 675–688.e677. doi: 10.1016/j.cmet.2019.08.018
Zeng, Y., Chen, S., Fu, Y., Wu, W., Chen, T., Chen, J., et al. (2020). Gut microbiota dysbiosis in patients with hepatitis b virus-induced chronic liver disease covering chronic hepatitis, liver cirrhosis and hepatocellular carcinoma. J. Viral Hepat 27 (2), 143–155. doi: 10.1111/jvh.13216
Zhang, Z., Tang, H., Chen, P., Xie, H., Tao, Y. (2019). Demystifying the manipulation of host immunity, metabolism, and extraintestinal tumors by the gut microbiome. Signal Transduct Target Ther. 4, 41. doi: 10.1038/s41392-019-0074-5
Zheng, R., Wang, G., Pang, Z., Ran, N., Gu, Y., Guan, X., et al. (2020). Liver cirrhosis contributes to the disorder of gut microbiota in patients with hepatocellular carcinoma. Cancer Med. 9 (12), 4232–4250. doi: 10.1002/cam4.3045
Keywords: intestinal flora, bacterial translocation, dysbiosis, hepatitis, cirrhosis, liver cancer
Citation: Liu S and Yang X (2023) Intestinal flora plays a role in the progression of hepatitis-cirrhosis-liver cancer. Front. Cell. Infect. Microbiol. 13:1140126. doi: 10.3389/fcimb.2023.1140126
Received: 08 January 2023; Accepted: 23 February 2023;
Published: 09 March 2023.
Edited by:
Julio Plaza-Diaz, Children’s Hospital of Eastern Ontario (CHEO), CanadaReviewed by:
Ibrahim M. Sayed, Assiut University, EgyptStefania Tocci, University of Massachusetts Lowell, United States
Copyright © 2023 Liu and Yang. This is an open-access article distributed under the terms of the Creative Commons Attribution License (CC BY). The use, distribution or reproduction in other forums is permitted, provided the original author(s) and the copyright owner(s) are credited and that the original publication in this journal is cited, in accordance with accepted academic practice. No use, distribution or reproduction is permitted which does not comply with these terms.
*Correspondence: Xilan Yang, eGlsYW55YW5nQG5qbXUuZWR1LmNu