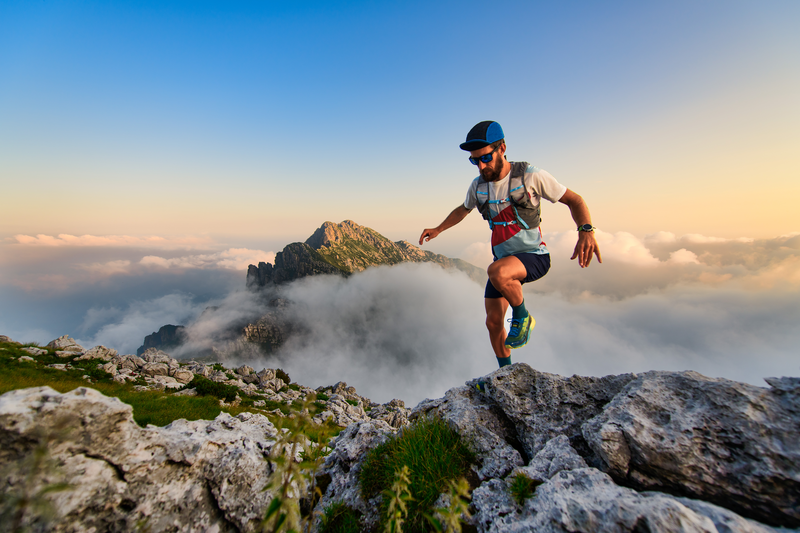
94% of researchers rate our articles as excellent or good
Learn more about the work of our research integrity team to safeguard the quality of each article we publish.
Find out more
ORIGINAL RESEARCH article
Front. Cell. Infect. Microbiol. , 15 February 2023
Sec. Clinical Microbiology
Volume 13 - 2023 | https://doi.org/10.3389/fcimb.2023.1136211
Neisseria meningitidis causes invasive meningococcal disease (IMD), which is associated with significant mortality and long-term consequences, especially among young children. The incidence of IMD in Lithuania was among the highest in European Union/European Economic Area countries during the past two decades; however, the characterization of meningococcal isolates by molecular typing methods has not yet been performed. In this study, we characterized invasive meningococcal isolates (n=294) recovered in Lithuania from 2009 to 2019 by multilocus sequence typing (MLST) and typing of antigens FetA and PorA. The more recent (2017-2019) serogroup B isolates (n=60) were genotyped by analyzing vaccine-related antigens to evaluate their coverage by four-component (4CMenB) and two-component (MenB-Fhbp) vaccines using the genetic Meningococcal Antigen Typing System (gMATS) and Meningococcal Deduced Vaccine Antigen Reactivity (MenDeVAR) Index methods, respectively. The vast majority (90.5%) of isolates belonged to serogroup B. MLST revealed a predominance of clonal complex 32 (74.02%). Serogroup B strain P1.19,15: F4-28: ST-34 (cc32) accounted for 64.1% of IMD isolates. The overall level of strain coverage by the 4MenB vaccine was 94.8% (CI 85.9-98.2%). Most serogroup B isolates (87.9%) were covered by a single vaccine antigen, most commonly Fhbp peptide variant 1 (84.5% of isolates). The Fhbp peptides included in the MenB-Fhbp vaccine were not detected among the analyzed invasive isolates; however, the identified predominant variant 1 was considered cross-reactive. In total, 88.1% (CI 77.5-94.1) of isolates were predicted to be covered by the MenB-Fhbp vaccine. In conclusion, both serogroup B vaccines demonstrate potential to protect against IMD in Lithuania.
The Gram-negative bacterium Neisseria meningitidis is a commensal of the nasopharynx in up to 35% of healthy individuals at any given time (Coureuil et al., 2012; Parikh et al., 2020). It is not fully understood why meningococci in some colonized individuals may pass the mucosal barrier and enter the bloodstream, resulting in septicemia (Coureuil et al., 2012; Schoen et al., 2014). Their further proliferation in the bloodstream may progress to cardiovascular failure and dissemination to other organs. N. meningitidis crosses the blood-brain barrier in about 60% of bacteremia cases causing meningitis (Miller et al., 2013; Nadel and Ninis, 2018). Thus, rapidly evolving invasive meningococcal disease (IMD) is a life-threatening condition that causes significant morbidity and mortality, especially in children and young adults (Nadel and Ninis, 2018).
N. meningitidis may be either unencapsulated or encapsulated, the latter which is divided into 12 serogroups based on different capsular polysaccharide structures (Centers for Disease Control and Prevention, 2016; Whittaker et al., 2017). Only six meningococcal serogroups (A, B, C, W, Y, and X) cause the majority of IMD cases worldwide; however, serogroup distribution and the incidence of IMD vary by continent and region (Bai et al., 2019; Parish et al., 2020). To perform active surveillance of IMD outbreaks and sporadic cases, molecular techniques that precisely characterize invasive and carried meningococci have been developed. Multilocus sequence typing (MLST) allows determination of the sequence type (ST) and clonal complex (cc) of meningococcal isolates (Maiden et al., 1998; Jolley et al., 2007). Typing of several surface proteins (PorA, PorB, and FetA) is also recommended (Jolley et al., 2007). Recently, the Genetic Meningococcal Typing System (gMATS) (Muzzi et al., 2019) and Meningococcal Deduced Vaccine Antigen Reactivity (MenDeVAR) Index methods (Rodrigues et al., 2020) based on sequence analysis of vaccine antigen variants in meningococcal isolates open the possibility of predicting serogroup B (MenB) strain coverage by available meningococcal B vaccines.
In Lithuania, which has 2.8 million inhabitants, the incidence of IMD cases was among the highest in European Union/European Economic Area countries during 2009-2019, reaching 3 and 2.9 cases/105 in 2013 and 2017, respectively (National Public Health Center under the Ministry of Health, 2021). Serogroup B is responsible for most IMD cases in Lithuania (National Public Health Center under the Ministry of Health, 2021), but invasive N. meningitidis isolates have not been characterized by molecular typing methods. In 2018, the only four-component meningococcal B vaccine (4CMenB) was introduced to Lithuania’s National Immunization program and administered at 3, 5, and 12-15 months of age. The two-component meningococcal B vaccine (MenB-Fhbp) is registered for the vaccination of individuals 10 years and older, but the cost of the vaccine is not reimbursed. Studies on the potential coverage of MenB vaccines in Lithuania would contribute to better monitoring of vaccination impact and planning of future prophylactic strategies.
The aim of this study was to characterize invasive N. meningitidis isolates collected in Lithuania between 2009-2019 by molecular typing and to evaluate the potential 4CMenB and MenB-Fhbp vaccine coverage of isolates from 2017-2019 IMD cases.
All cases of IMD require mandatory reporting in Lithuania. Since 2009, invasive N. meningitidis isolates are requested to be sent to the National Public Health Surveillance Laboratory (NPHSL) (Legal acts of the Republic of Lithuania, Ministry of Health, 2009). The NPHSL received 316 invasive meningococcal isolates between 1 July 2009 and 31 Dec 2019. All meningococci were recovered from the blood or cerebrospinal fluid (CSF) of individuals with IMD. Nine isolates could not be cultured and were excluded from this study. If more than one isolate was received from the same individual, only one isolate was included. A total of 294 N. meningitidis isolates were analyzed. Information on the gender and age of the individual and the region of isolation was obtained from the NPHSL. The study was approved by the Vilnius Regional Biomedical Research Ethics Committee (http://www.eurecnet.org/information/lithuania.html), approval no. 2021/9-1369-846, issued 21-09-2021.
Meningococcal isolates were stored frozen (-80°C) at the NPHSL. For this study, isolates were cultured on Chocolate agar (Oxoid, UK) for 12-14 h at 36°C and 5% CO2. DNA from cultured isolates was extracted using a GeneJET genomic DNA purification kit (Thermo Fisher Scientific, Vilnius, Lithuania) according to the manufacturer’s instructions. About 20 ng of purified chromosomal DNA was used as a template for amplification of all genes. Meningococcal isolates were serogrouped by slide agglutination by the isolate providers. Serogroup verification was performed by singleplex polymerase chain reaction (PCR)-based assay (Fraisier et al., 2009; Zhu et al., 2012). PCR was performed with Phire Green Hot Start II PCR Master Mix (Thermo Fisher Scientific, Vilnius, Lithuania) in 20-µL reaction volume comprising 0.4 µM of each primer (Metabion International AG, Germany). Amplification reactions included initial denaturation at 98°C for 30 s, 30 amplification cycles consisting of denaturation for 5 s at 98°C, annealing for 5 s at 60°C, and extension for 10 s at 72°C. These were followed by a final extension step for 1 min at 72°C. No template controls were included in the PCR assays. Amplification products were separated on 2.5% agarose gel.
MLST, PorA, and FetA typing for isolates received in 2009-2017 were performed according to the procedure described on the PubMLST Neisseria website (http://pubmlst.org/neisseria/; Jolley et al., 2018). The amplification enzymes used were Phire Hot Start II DNA polymerase included in the master mix (Thermo Fisher Scientific) in the case of porA and fetA, and DreamTaq Hot Start DNA polymerase included in the master mix (Thermo Fisher Scientific) for each of the MLST housekeeping genes. The reactions were performed in a 25-µL final volume. PCR conditions for amplification of porA and fetA were, as follows: initial denaturation at 98°C for 30 s, 30 amplification cycles consisting of denaturation for 5 s at 98°C, annealing for 5 s at 60°C, and extension for 15 s at 72°C. A final extension step lasted for 1 min at 72°C. Amplification reaction mixture with DreamTaq Hot Start DNA polymerase was subjected to initial denaturation at 95°C for 3 min, 35 cycles of denaturation at 95°C for 30 s, primer annealing at 57°C (for abcZ, adk, aroE, fumC, and pdhC) or 67°C (for pgm) for 30 s, extension at 72°C for 40 s, followed by a final extension step at 72°C for 7 min. Amplification products were analyzed on 1.5% agarose gel.
PCR products were purified using a DNA Clean & Concentrator kit (Zymo Research, Irvin, CA, US) and subjected to Sanger sequencing (Base Clear B.V., Leiden, The Netherlands). Allele assignment and MLST ST and cc were obtained from the PubMLST Neisseria database. For isolates received in 2017, amplification of vaccine antigens nhba, fhbp, and nadA was performed as previously described (Bambini et al., 2009; Lucidarme et al., 2010). PCR reaction mixture with Phire Hot Start II DNA polymerase included in the master mix was subjected to initial denaturation at 98°C for 30 s, 30 amplification cycles consisting of denaturation for 5 s at 98°C, annealing for 5 s at 60°C (for nhba and nadA) or 63°C (for fhbp), extension for 15 s at 72°C, followed by a final extension step at 72°C for 1 min.
Internally directed primers nadaintF and nadaintR for detecting nadA were used as described by Lucidarme et al. (2009). Briefly, PCR was performed with Phire Hot Start II DNA polymerase included in the master mix using reaction conditions described for amplification of porA and fetA.
For isolates not subjected to whole genome sequencing (WGS), the presence/absence of nadA in the meningococcal genome was confirmed using internally directed primers in case the application of primers targeting the flanking regions did not yield any PCR product (Lucidarme et al., 2009). The PubMLST Neisseria database was used to assign allelic and peptide variants of vaccine antigens.
Isolates received in 2018-2019 (n=25) underwent WGS with support from the European Center for Disease Prevention and Control. WGS was conducted by Eurofins Genomics Europe Sequencing Gmbh (Konstanz, Germany) using the Illumina NovaSeq platform. Assembly was performed using SPAdes 3.11 (Bankevich et al., 2012) with careful mode enabled. Genome contigs were submitted to the PubMLST Neisseria database (see Data Availability Statement). The allelic profile of seven MLST genes and variable regions of porA and fetA were determined by automatic scanning of genome contigs (Jolley et al., 2018). Allelic and peptide variants of vaccine antigens (fhbp, nhba, and nadA) were also defined.
The gMATS method was used to predict strain coverage by the 4CMenB vaccine as described by Muzzi et al. (2019). Genes of vaccine antigens were obtained either by PCR and sequencing (isolates recovered in 2017) or WGS (isolates recovered in 2018-2019). Alleles and corresponding peptide identifiers were assigned by the PubMLST Neisseria database. Estimation of antigen-specific predicted coverage by 4CMenB was performed based on criteria described by Muzzi et al. (2019). NadA was not considered as contributing to coverage in gMATS (Muzzi et al., 2019).
The potential strain coverage by the MenB-Fhbp vaccine was assessed using the MenDeVAR Index method according to criteria outlined in Rodrigues et al. (2020).
Data were analyzed using the R statistical computer program, version 4.2.2 (R Core Team, 2017), and Microsoft Excel. Ninety-five percent confidence intervals (CIs) of proportions were calculated using Wilson’s method (Agresti and Coull, 1998) implemented in the R package DescTools, version 0.99.47, and then converted to percentages.
During the study period 2009-2019, 745 cases of sporadic IMD were reported in Lithuania, corresponding to an overall IMD incidence of 2.24 cases/105 inhabitants (National Public Health Center under the Ministry of Health, 2021). The highest incidence was observed in 2013, with 3 cases/105 inhabitants (National Public Health Center under the Ministry of Health, 2021) (Table 1). The largest number of IMD cases was observed in <5-year-olds (47.5%), whereas ≥30-year-olds accounted for 18.1% of cases. The worst disease-affected region was the Vilnius region, accounting for the majority of disease cases across all age groups (National Public Health Center under the Ministry of Health, 2021).
The proportion of cases diagnosed by PCR without culture is unknown. The clinical guidelines (Diagnostic and Treatment Protocols, Ministry of the Republic of Lithuania, 2015) recommend obtaining a sample of blood and/or CSF for culturing. The 294 isolates analyzed in this study were from culture-proven IMDs, which accounted for 39.4% of all reported IMD cases during the study period (Table 1). The highest proportion of isolates (51-53%) from annually reported IMD cases was in 2015-2017, while the lowest was in 2009-2010, which could be related to adapting to the recently introduced requirement to send invasive isolates to NPHSL (Legal acts of the Republic of Lithuania, Ministry of Health, 2009).
The vast majority of cases (90.5%, n=266) were caused by N. meningitidis serogroup B (Table 2). Serogroups C, Y, and W accounted for 7.5% (n=22), 1% (n=3), and 0.68% (n=2) of cases, respectively. Serogroups B and C were detected in all age groups, whereas MenY and MenW caused disease mostly in adults (National Public Health Center under the Ministry of Health, 2021) (Table 3).
All 294 isolates were subjected to MLST analysis. The MLST profile was incomplete for 13 isolates (4.4%) because the PCR product of the gene(s), mainly fumC/pdhC, was not obtained, whereas the subtypes of finetyping antigens PorA and FetA were determined. The 281 isolates with complete MLST profiles were grouped into 73 STs, of which 63 (86.3%) were detected only once. The most common ST was ST-34 (cc32), accounting for 64.8% (n=182, 59.0-70.1%) of all isolates. Thirty-four isolates (12.1%) belonged to 31 STs that were not assigned to any known cc (ccUA). Among 45 designated new STs, 20 STs were grouped into five ccs, of which the most frequent was cc18, whereas 25 STs were not associated with any cc.
The isolates were assigned to nine ccs, the most frequent of which were cc32 (74.02%, n=208), cc41/44 (6.4%, n=18), and cc18 (3.6%, n=10) (Figure 1). The most common combination of PorA VR1/VR2 was P1.19,15, which belonged to cc32 isolates. Antigen finetyping showed that the serogroup B meningococcal strain P1.19,15: F4-28: ST-34 (cc32) was the most prevalent (64.1%, n=180) among IMD isolates.
Figure 1 Distribution of clonal complexes in the panel of 294 isolates by year. ccUA, sequence type not assigned to any cc; ND, MLST profile not available; cc, clonal complex; n, number of isolates.
Generally, the meningococcal isolates in most age groups belonged to three different ccs, although cc32 was dominant (Figure 2). Among 5-14- and 15-24-year-olds, cc32 comprised 96.4% and 88.9% of isolates, respectively. Approximately 50% of isolates among children <1 year of age were attributed to cc32; however, 27.3% (CI 15.1-44.2%) of isolates were not assigned to any known cc. The proportion of ccUA was lower (9.8%) among 1-4-year-olds.
Figure 2 Distribution of clonal complexes according to age. ND, no age information of IMD cases (n=8); ccUA, sequence type not assigned to any cc; cc, clonal complex; n, number of isolates.
The subtyping results of fhbp, nhba, and nadA genes performed for 60 MenB isolates of the IMD cases reported in 2017-2019 are shown in Supplementary Table 1.
For one isolate (ST-15979, cc18), the Fhbp peptide variant was not determined due to difficulties in obtaining the PCR product. Eleven fhbp alleles were found to encode 11 different peptide variants. Fhbp variant 1 (subfamily B), which is included in the 4CMenB vaccine, was found in 47 of 59 isolates (79.7%). The nhba gene could not be amplified for one isolate (ST-16207, ccUA). Four new nhba alleles were identified (1920, 1921, 1922, and 1930), three of which coded for the new Nhba peptide variants (1708, 1709, and 1715). The Nhba peptide variant 2 included in the 4CMenB vaccine was present in one isolate (ST-16176, ccUA), whereas the most prevalent peptide was variant 187 (47 of 59 isolates).
For one isolate (ST-16207, ccUA), the PCR product for PorA and Nhba was not obtained. The 4CMenB vaccine VR2:4 variant was found in two isolates attributed to cc41/44. For 29 isolates received in 2017, the PCR product of nadA was not obtained with previously described primers (Bambini et al., 2009; Lucidarme et al., 2010); therefore, the internally directed primers nadaintF and nadaintR (Lucidarme et al., 2009) were used to confirm the presence/absence of nadA elsewhere in the genome (Supplementary Table 1). The obtained PCR product of ~1,000 bp of nadA-positive isolates was verified by sequencing; however, the peptide variant was not determined. The NadA coding gene was detected in 48 of 60 isolates (80.0%), of which the majority were assigned to ST-34 (cc32). For 2018-2019 isolates for which WGS was performed, all nadA-positive isolates carried peptide variant 1 (Supplementary Table 1).
Vaccine coverage using gMATS (Muzzi et al., 2019) was estimated for 58 MenB isolates (2017-2019), as PCR of vaccine antigens was not obtained for two isolates (id 13530 and id 26292, Supplementary Table 1). gMATS predicted 53 (91.4%, CI 81.4-96.3%) covered isolates, 1 (1.7%) non-covered isolate, and 4 (6.9%) unpredictable isolates. Considering one-half of the unpredictable isolates as potentially covered, an overall level of coverage was 94.8% (55 of 58, CI 85.9-98.2%).
The predicted coverage of strains assigned to cc32 was 97.9% (47 of 48, CI 89.1-99.6%), and the antigen contributing to coverage was Fhbp peptide variant 1 (Table 4). Among the ‘unpredictable’ strains category, two ccUA isolates possessed alleles for Nhba peptide 42 and 53 and an allele for Fhbp peptide 13, one cc41/44 isolate possessed an allele for Nhbp peptide 1709, and one cc32 isolate possessed an allele for Fhbp peptide 180 (Table 4). Most MenB isolates (87.9%) were covered by a single antigen, namely Fhbp (84.5%) and Nhba (3.4%, peptides 2 and 20). One isolate was predicted to be covered by a combination of two antigens (Fhbp+PorA_VR2) and one isolate by three antigens (Fhbp+Nhba+PorA_VR2) (Table 4).
A new tool, MenDeVAR Index (Rodrigues et al., 2020), was used to predict coverage of 59 MenB isolates by the MenB-Fhbp vaccine, as fhbp PCR product was not obtained for one isolate (ST-15979, cc18). All 59 isolates contained the fhbp gene, among which two isolates possessed an A/B hybrid variant (allele 231, peptide 207). The Fhbp peptides 45 and 55 included in the MenB-Fhbp vaccine were not detected among Lithuanian (2017-2019) isolates. The most frequent peptide 1 associated with cc32 was considered cross-reactive (‘amber’ MenDeVAR Index) (Table 5). There were insufficient data (‘grey’ Index) for the assessment of seven isolates associated with ccUA, cc18, and cc41/44. In total, 52 isolates from the analyzed 59 isolates (88.1%, CI 77.5-94.1) were considered potentially covered by the MenB-Fhbp vaccine (Table 5).
Table 5 Predicted coverage of MenB isolates by the MenB-Fhbp vaccine according to the MenDeVAR Index method.
Estimation of predicted 4CMenB and MenB-Fhbp coverage was performed using the gMATS and MenDeVAR Index methods, respectively. Although the MenDeVAR Index also estimates reactivity of 4CMenB, in contrast to gMATS, this method includes NadA peptide as a predictor (Rodrigues et al., 2020). We were not able to detect the nadA allele using targeted PCR for some isolates (Supplementary Table 1); therefore, 4CMenB coverage was not assessed using the MenDeVAR Index. Although the methods use slightly different criteria for defining cross-reactive antigens (Muzzi et al., 2019; Rodrigues et al., 2020), 49 (84.5%, CI 73.1-91.6%) isolates were potentially covered by both vaccines (comprising ‘amber’ cross-reactive antigens for evaluation by the MenDeVAR Index). Seven isolates showed diverging vaccine coverage predictions by the Fhbp antigen, which mostly contributed to the potential protection of 4CMenB (Table 6).
During the study period (2009-2019), Lithuania, along with more populated and distant countries such as the United Kingdom and Ireland, ranked high in number of IMD cases per 105 population among European Union/European Economic Area countries (Ladhani et al., 2012; Bennet et al., 2019; European Centre of Disease Prevention and Control, 2022). The incidence of IMD was significantly lower in neighboring Lithuania countries such as Poland, Latvia, and Estonia accounting for 0.36, 0.6, and 0.3 cases per 105 population, respectively (European Centre of Disease Prevention and Control, 2022). In 2018-2019, the incidence of IMD decreased in Lithuania (Table 1), but we assume that it is more related to the temporal patterns associated with the disease (Mosavi-Jarrahi et al., 2009) than to the effect of vaccination. 4CMenB vaccination started in the middle of 2018.
Although the clinical practice of IMD management is based on culture proven-diagnosis (Diagnostic and Treatment Protocols, Ministry of the Republic of Lithuania, 2015), invasive meningococcal isolates stored at the NPHSL account for approximately 40% of all reported disease cases from 2009 to 2019, which is much less than in Poland, the Netherlands, and Finland (Bodini et al., 2020; Freudenburg-de Graaf et al., 2020; Waśko et al., 2020). Several studies report low recovery (35-43%) of N. meningitidis from sterile sites of the human body (Bronska et al., 2006; Heinsbroek et al., 2013; Lee et al., 2018). It needs to be clarified whether the missed isolates are due to difficulties in recovering N. meningitidis from the samples, neglect of mandatory sending of invasive isolates to the NPHSL, or the increasing number of PCR-confirmed IMD cases.
During the period of 2009-2019, serogroup B of invasive N. meningitidis was the most common serogroup (90.5% of isolates), whereas MenC accounted for 7.5% of isolates. A small number of serogroup Y and W isolates were from cases diagnosed after 2015. In Lithuania, MenY and MenW cases were found only in adults, consistent with findings from Canada (Tsang et al., 2020) and the Netherlands (Freudenburg-de Graaf et al., 2020).
MLST analysis revealed the dominance of cc32 (74.02%), which has been found globally and is widespread in the Netherlands (32%) (Freudenburg-de Graaf et al., 2020), Czech Republic (29%) (Krizova and Honskus, 2019), France (27.5%) (Hong et al., 2021), Poland (20.1%) (Waśko et al., 2020), Germany (21.6%), Norway (17.1%), and Italy (14.8%) (Vogel et al., 2013). In the past, the hypervirulent cc32 caused outbreaks in Norway, Cuba, France, the Netherlands, and the United Kingdom (Harrison et al., 2009; Racloz and Liuz, 2010). There are no data on invasive meningococcal isolates in neighboring and demographically similar countries Latvia and Estonia (https://pubmlst.org/neisseria/, accessed December 2022).
Clonal complex 41/44 was the second most dominant cc (6.4%) with strains associated with serogroups B and C, which confirms their propensity to rapid evolutionary changes in serotypes and genotypes (Whelan et al., 2015). Isolates of cc41/44 were representative of 14 different STs, with ST-2871 being the most common, although it is found in very few countries (https://pubmlst.org/neisseria/, accessed December 2022). We detected only three isolates that belong to the hyperinvasive cc11 potentially associated with high morbidity and mortality (Lucidarme et al., 2015).
Among the cc32 isolates, ST-34 was the most frequently detected ST among the invasive meningococci in Lithuania. Finetyping of PorA and FetA variable regions showed that the strain B: P1.19,15: F4-28: ST-34 (cc32) accounted for 64.1% of all analyzed meningococcal isolates. The distribution of this strain remained almost stable over the study period. According to PubMLST Neisseria data, this invasive strain was recovered in a small number of countries, including England (n=17 isolates, 2010-2020), Poland (n=3, 2012), France (n=2), and Spain (n=2). Thus, the hypervirulent strain B: P1.19,15: F4-28: ST-34 (cc32) has not spread to other countries but instead is well-established in Lithuania. This strain was found among all age groups, most commonly among 5-14- and 15-24-year-olds. The characteristic feature of the prolonged cc32 MenB epidemics in Cuba and Norway (1980s) and in Oregon in the United States (1990-2000s) was the predominance of a single bacterial strain (>85%) rather than a mixture of heterologous strains (Diermayer et al., 1999; Harrison et al., 2009). Keeping in mind that isolates from approximately 50% of IMD cases in Lithuania were not included in the analysis, the prevalence of the strain B: P1.19,15: F4-28: ST-34 (cc32) may be even higher than detected.
Given that vaccination against MenB is widely accessible in Lithuania, genetic methods were used to assess the potential coverage of the most recent MenB isolates by the available vaccines. The current study shows that the predicted strain coverage by 4CMenB using gMATS was 91.4% (CI 85.9-98.2%). Only a few isolates possessed 4CMenB-covered Nhba or PorA variants (Table 4, Supplementary Table 1). In Lithuanian isolates, the Nhba non-covered variant 187 was linked with cc32, whereas in Polish isolates, peptides 3 and 20 were mostly detected in cc32 (Waśko et al., 2020). A high proportion of isolates (84.5%) was predicted to be covered by a single 4CMenB vaccine antigen, Fhbp, most often by its variant 1. This peptide variant is associated with cc32 (Freudenburg-de Graaf et al., 2020; Waśko et al., 2020); therefore, it is expected that the predominant ST-34 (cc32) is also potentially covered by the two-component MenB-Fhbp vaccine due to its cross-reactivity (i.e., ‘amber’ MenDeVAR Index).
Some Lithuanian MenB isolates (9.0%) were not assigned to any clonal complex, whereas isolates of ccUA accounted for 22% in Norway, 19.4% in Germany, 9.3% in Italy (Vogel et al., 2013), 20.2% in Poland (Waśko et al., 2020), and 6.1% in Greece (Tzanakaki et al., 2021). The predicted coverage data of isolates collected from 2017 to 2019 showed that the antigen peptide variants present in ccUA strains often fall into the grey zone characterized by ‘insufficient data’ or ‘unpredictable’ categories according to the gMATS and/or MenDeVAR Index methods (Mulhall et al., 2018; Moreno et al., 2020). The Lithuanian isolates of ccUA were unevenly distributed across age groups, with the highest proportion found among children < 1-year-old (Figure 2). Children of this age are potential targets for vaccination; therefore, attention should be paid to obtaining and analyzing more isolates of IMD cases from this age group. Participation in the Global Meningococcal Initiative in Eastern Europe (Bai et al., 2019) and other surveillance projects would open new possibilities for cooperation with other study groups to apply MATS and meningococcal antigen surface expression (MEASURE), assays for coverage prediction of novel STs along with genomic data to update the gMATS and MenDeVAR Index databases.
One limitation of this study is that all individuals eligible for molecular typing of meningococcal isolates accounted for less than one-half of all reported IMD cases. We encourage clinicians to take blood and CSF samples for culturing if possible before initiating antibiotic therapy. Second, the number of MenB isolates of IMD cases subjected to vaccine-predicted coverage estimation was relatively small and did not allow evaluation of age-specific distributions. Third, the absence of genomic characterization of nadA for some MenB isolates did not permit use of the MenDeVAR Index method to predict their coverage by the 4CMenB vaccine.
In conclusion, the study provides the first comprehensive genetic characterization of invasive meningococcal isolates collected in Lithuania from 2009 to 2019. The vast majority of isolates belonged to serogroup B, and the most prevalent cc was cc32. The strain B: P1.19,15: F4-28: ST-34 (cc32) accounted for more than one-half of all collected invasive isolates over the study period. This strain is rare in neighboring and distant European countries. Application of well-recognized genetic tools revealed that 84.5% (CI 73.1-91.6%) of isolates collected in 2017-2019 were covered by both MenB vaccines. Assessment of strain coverage by MenB vaccines using genomic analysis of vaccine-related antigens is convenient and valuable, as not all countries may perform MATS assays. The continuation of active IMD surveillance in Lithuania is needed to monitor changes in circulating meningococcal strains that would allow evidence-based implementation of a national vaccination strategy.
The data presented in the study are available in the publicly accessible PubMLST Neisseria repository (https://pubmlst.org/neisseria). The names of accession numbers can be found below: 110094-110096, 110181, 110183, 110185-110188, 110190-110194, 110196-110204, 110206, and 110207.
The studies involving human participants were reviewed and approved by the Vilnius Regional Biomedical Research Ethics Committee (http://www.eurecnet.org/information/lithuania.html), approval no. 2021/9-1369-846, issued 21-09-2021. Written informed consent for participation of children was not provided by the participants’ legal guardians/next of kin because: this is a retrospective study that included all meningococcal isolates from invasive meningococcal disease received in the National Public Health Surveillance Laboratory from 2009 to 2019.
AZ and MP contributed to the conception and design of the study. ES, RP, AP, DS, IK-K, and MP performed the experiments. VG performed the statistical analysis. MP, AZ, and II wrote the manuscript. MP and AP submitted data to the PubMLST Neisseria database. All authors contributed to the manuscript revision and approved the submitted version.
This study received funding from the European Regional Development Fund (project No 01.2.2-LMT-K-718-03-0036) under a grant agreement with the Research Council of Lithuania (LMTLT).
We thank Dr. Keith Jolley from Oxford University for his assistance and infinite patience in receiving submissions to the PubMLST Neisseria database. This publication made use of the PubMLST Neisseria website (http://pubmlst.org/neisseria) hosted by the University of Oxford (Jolley et al., 2018). The development of this website was funded by the Welcome Trust. We are also grateful to our colleagues from NPHSL who provided isolates and epidemiological data used in this study.
The authors declare that the research was conducted in the absence of any commercial or financial relationships that could be construed as a potential conflict of interest.
All claims expressed in this article are solely those of the authors and do not necessarily represent those of their affiliated organizations, or those of the publisher, the editors and the reviewers. Any product that may be evaluated in this article, or claim that may be made by its manufacturer, is not guaranteed or endorsed by the publisher.
The Supplementary Material for this article can be found online at: https://www.frontiersin.org/articles/10.3389/fcimb.2023.1136211/full#supplementary-material
Agresti, A., Coull, B. A. (1998). Approximate is better than “exact” for interval estimation of binomial proportions. Am. Statistician. 52, 119–126. doi: 10.1080/00031305.1998.10480550
Bai, X., Borrow, R., Bukovski, S., Caugant, D. A., Culic, D., Delic, S., et al. (2019). Prevention and control of meningococcal disease: Updates from the global meningococcal initiative in Eastern Europe. J. Infect. 79, 528–541. doi: 10.1016/j.jinf.2019.10.018
Bambini, S., Muzzi, A., Olcen, P., Rappuoli, R., Pizza, M., Comanducci, M. (2009). Distribution and genetic variability of three vaccine components in a panel of strains representative of the diversity of serogroup b meningococcus. Vaccine 27, 2794–2803. doi: 10.1016/j.vaccine.2009.02.098
Bankevich, A., Nurk, S., Antipov, D., Gurevich, A. A., Dvorkin, M., Kulikov, A. S., et al. (2012). SPAdes: A new genome assembly algorithm and its applications to single-cell sequencing. J. Comput. Biol. 19, 455–477. doi: 10.1089/cmb.2012.0021
Bennet, D., O’Lorcain, P., Morgan, S., Cotter, S., Cafferkey, M., Cunney, R. (2019). Epidemiology of two decades of invasive meningococcal disease in the republic of Ireland: an analysis of national surveillance data on laboratory-confirmed cases from 1996 to 2016. Epidemiol. Infect. 147, e142. doi: 10.1017/S0950268819000396
Bodini, M., Brozzi, A., Giuliani, M., Nohynek, H., Vainio, A., Kuusi, M., et al. (2020). Genomic characterization of invasive meningococcal serogroup b isolates and estimation of 4CMenB vaccine coverage in Finland. mSphere 5, e00376–e00320. doi: 10.1128/mSphere.00376-20
Bronska, E., Kalmusova, J., Dzupova, O., Maresova, V., Kriz, P., Benes, J. (2006). Dynamics of PCR-based diagnosis in patients with invasive meningococcal disease. Clin. Microbiol. Infect. 12, 137–141. doi: 10.1111/j.1469-0691.2005.01327.x
Centers for Disease Control and Prevention (2016) Meningoccocal disease, laboratory methods for the diagnosis of meningitis (USA: CDC Atlanta, GA) (Accessed November 2022).
Coureuil, M., Join-Lambert, O., Lécuyer, H., Bourdoulous, S., Marullo, S., Nassif, X. (2012). Mechanism of meningeal invasion by neisseria meningitidis. Virulence 3, 164–172. doi: 10.4161/viru.18639
Diagnostic and Treatment Protocols, Ministry of the Republic of Lithuania (2015) Protocols for the field of children’s diseases (Vilnius, Lithuania) (Accessed January 2023).
Diermayer, M., Hedberg, K., Hoesly, F., Fischer, M., Perkins, B., Reeves, M., et al. (1999). Epidemic serogroup b meningococcal disease in Oregon: The evolving epidemiology of the ET-5 strain. JAMA 281, 1493–1497. doi: 10.1001/jama.281.16.1493
European Centre of Disease Prevention and Control (2022) Surveillance atlas for meningococcal disease (Stockholm: ECDC) (Accessed November 2022).
Fraisier, C., Stor, R., Tenebray, B., Sanson, Y., Nicolas, P. (2009). Use of a new single multiplex PCR-based assay for direct simultaneous characterization of six neisseria meningitidis serogroups. J. Clin. Microbiol. 47, 2662–2666. doi: 10.1128/JCM.02415-08
Freudenburg-de Graaf, W., Knol, M. J., van der Ende, A. (2020). Predicted coverage by 4CMenB vaccine against invasive meningococcal disease cases in the Netherlands. Vaccine 38, 7850–7857. doi: 10.1016/j.vaccine.2020.10.008
Harrison, L. H., Trotter, C. L., Ramsay, M. E. (2009). Global epidemiology of meningococcal disease. Vaccine 27 Suppl 2, B51–B63. doi: 10.1016/j.vaccine.2009.04.063
Heinsbroek, E., Ladhani, S., Gray, S., Guiver, M., Kaczmarski, E., Borrow, R., et al. (2013). Added value of PCR-testing for confirmation of invasive meningococcal disease in England. J. Infect. 67, 385–390. doi: 10.1016/j.jinf.2013.06.007
Hong, E., Terrade, A., Muzzi, A., De Paola, R., Boccadifuoco, G., La Gaetana, R., et al. (2021). Evolution of strain coverage by the multicomponent meningococcal serogroup b vaccine (4CMenB) in France. Hum. Vaccin. Immunother. 17, 5614–5622. doi: 10.1080/21645515.2021.2004055
Jolley, K. A., Bray, J. E., Maiden, M. C. J. (2018). Open-access bacterial population genomics: BIGSdb software, the PubMLST.org website and their applications. Wellcome. Open Res. 3, 124. doi: 10.12688/wellcomeopenres.14826.1
Jolley, K. A., Brehony, C., Maiden, M. C. J. (2007). Molecular typing of meningococci: recommendations for target choice and nomenclature. FEMS Microbiol. Rev. 31, 89–96. doi: 10.1111/j.1574-6976.2006.00057.x
Krizova, P., Honskus, M. (2019). Genomic surveillance of invasive meningococcal disease in the Czech republic 2015-2017. PloS One 14, e0219477. doi: 10.1371/journal.pone.0219477
Ladhani, S. N., Flood, J. S., Ramsay, M. E., Campbell, H., Gray, S. J., Kaczmarski, E. B., et al. (2012). Invasive meningococcal disease in England and Wales: implications for the introduction of new vaccines. Vaccine 30, 3710–3716. doi: 10.1016/j.vaccine.2012.03.011
Lee, H., Seo, Y., Kim, K. H., Lee, K., Choe, K. W. (2018). Prevalence and serogroup changes of neisseria meningitidis in south Korea 2010–2016. Sci. Rep. 8, 5292. doi: 10.1038/s41598-018-23365-8
Legal acts of the Republic of Lithuania, Ministry of Health (2009) Regarding the approval of the description of the identification and typing procedure of pathogenic bacteria identified in the laboratories of personal and public health care institutions, the act no. V-385, 21-05-2009 (Vilnius, Lithuania) (Accessed January 2022).
Lucidarme, J., Comanducci, M., Findlow, J., Gray, S. J., Kaczmarski, E. B., Guiver, M., et al. (2009). Characterization of fHbp, nhba (gna2132), nadA, porA, sequence type (ST), and genomic presence of IS1301 in group b meningococcal ST269 clonal complex isolates from England and Wales. J. Clin. Microbiol. 47, 3577–3585. doi: 10.1128/JCM.00936-09
Lucidarme, J., Comanducci, M., Findlow, J., Gray, S. J., Kaczmarski, E. B., Guiver, M., et al. (2010). Characterization of fHbp, nhba (gna2132), nadA, porA, and sequence type in group b meningococcal case isolates collected in England and Wales during January 2008 and potential coverage of an investigational group b meningococcal vaccine. Clin. Vaccine Immunol. 17, 919–929. doi: 10.1128/CVI.00027-10
Lucidarme, J., Hill, D. M. C., Bratcher, H. B., Gray, S. J., du Plessis, M., Tsang, R. S. W., et al. (2015). Genomic resolution of an aggressive, widespread, diverse and expanding meningococcal serogroup b, c and W lineage. J. Infect. 71, 544–552. doi: 10.1016/j.jinf.2015.07.007
Maiden, M. C., Bygraves, J. A., Feil, E., Morelli, G., Russell, J. E., Urwin, R., et al. (1998). Multilocus sequence typing: a portable approach to the identification of clones within populations of pathogenic microorganisms. Proc. Natl. Acad. Sci. U. S. A. 95, 3140–3145. doi: 10.1073/pnas.95.6.3140
Miller, F., Lécuyer, H., Join-Lambert, O., Bourdoulous, S., Marullo, S., Nassif, X., et al. (2013). Neisseria meningitidis colonization of the brain endothelium and cerebrospinal fluid invasion. Cell Microbiol. 15, 512–519. doi: 10.1111/cmi.12082
Moreno, J., Alarcon, Z., Parra, E., Duarte, C., Sanabria, O., Prada, D., et al. (2020). Molecular characterization of neisseria meningitidis isolates recovered from patients with invasive meningococcal disease in Colombia from 2013 to 2016. PloS One 15, e0234475. doi: 10.1371/journal.pone.0234475
Mosavi-Jarrahi, A., Esteghamati, A., Asgari, F., Heidarnia, M., Mousavi-Jarrahi, Y., Goya, M. (2009). Temporal analysis of the incidence of meningitis in the Tehran metropolitan area 1999-2005. Popul. Health Metr. 7, 19. doi: 10.1186/1478-7954-7-19
Mulhall, R. M., Bennet, D., Cunney, R., Borrow, R., Lucidarme, J., Findlow, J., et al. (2018). Potential coverage of the 4CMenB vaccine against invasive serogroup b neisseria meningitidis ysolated from 2009 to 2013 in the republic of Ireland. mSphere 3, e00196–e00118. doi: 10.1128/mSphere.00196-18
Muzzi, A., Brozzi, A., Serino, L., Bodini, M., Abad, R., Caugant, D., et al. (2019). Genetic meningococcal antigen typing system (gMATS): A genotyping tool that predicts 4CMenB strain coverage worldwide. Vaccine 37, 991–1000. doi: 10.1016/j.vaccine.2018.12.061
Nadel, S., Ninis, N. (2018). Invasive meningococcal disease in the vaccine era. Front. Pediatr. 6. doi: 10.3389/fped.2018.00321
National Public Health Center under the Ministry of Health (2021) Incidence of infection diseases in Lithuania – statistical report (Vilnius, Lithuania: NPHC) (Accessed September 2022).
Parikh, S. R., Campbell, H., Bettinger, J. A., Harrison, L. H., Marshall, H. S., Martinon-Torres, F., et al. (2020). The everchanging epidemiology of meningococcal disease worldwide and the potential for prevention through vaccination. J. Infect. 81, 483–498. doi: 10.1016/j.jinf.2020.05.079
Racloz, V. N., Liuz, S. D. J. (2010). The elusive meningococcal meningitis serogroup: a systematic review of serogroup b epidemiology. BMC Infect. Dis. 10, 175. doi: 10.1186/1471-2334-10-175
R Core Team (2017). R: a language and environment for statistical computing (Vienna: R Foundation for Statistical Computing).
Rodrigues, C. M. C., Jolley, K. A., Smith, A., Cameron, J. C., Feavers, I. M., Maiden, M. C. J. (2020). Meningococcal deduced vaccine antigen reactivity (MenDeVAR) index: a rapid and accessible tool that exploits genomic data in public health and clinical microbiology applications. J. Clin. Microbiol. 59, e02161–e02120. doi: 10.1128/JCM.02161-20
Schoen, C., Kischkies, L., Elias, J., Ampattu, B. J. (2014). Metabolism and virulence in Neisseria meningitidis. Front. Cell. Infect. Microbiol. 4. doi: 10.3389/fcimb.2014.00114
Tsang, R. S. W., Law, D. K. S., De Paola, R., Giuliani, M., Stella, M., Zhou, J., et al. (2020). Culture-confirmed invasive meningococcal disease in Canada 2010 to 2014: Characterization of serogroup b Neisseria meningitidis strains and their predicted coverage by the 4CMenB vaccine. mSphere 5, e00883–e00819. doi: 10.1128/mSphere.00883-19
Tzanakaki, G., Xirogianni, A., Tsitsika, A., Clark, S. A., Kesanopoulos, K., Bratcher, H. B., et al. (2021). Estimated strain coverage of serogroup b meningococcal vaccines: A retrospective study for disease and carrier strains in Greece, (2010–2017). Vaccine 39, 1621–1630. doi: 10.1016/j.vaccine.2021.01.073
Vogel, U., Taha, M. K., Vazquez, J. A., Findlow, J., Claus, H., Stefanelli, P., et al. (2013). Predicted strain coverage of a meningococcal multicomponent vaccine (4CMenB) in Europe: A qualitative and quantitative assessment. Lancet Infect. Dis. 13, 416–425. doi: 10.1016/S1473-3099(13)70006-9
Waśko, I., Gołębiewska, A., Kiedrowska, M., Ronkiewicz, P., Wróbel-Pawelczyk, I., Kuch, A., et al. (2020). Genetic variability of polish serogroup b meningococci, (2010–2016) including the 4CMenB vaccine component genes. Vaccine 38, 1943–1952. doi: 10.1016/j.vaccine.2020.01.021
Whelan, J., Bambini, S., Biolchi, A., Brunelli, B., Robert-Du Ry van Beest Holle, R. (2015). Outbreaks of meningococcal b infection and the 4CMenB vaccine: historical and future perspectives. Expert Rev. Vaccines 14, 713–736. doi: 10.1586/14760584.2015.1004317
Whittaker, R., Dias, G. J., Ramliden, M., Ködmön, C., Economopolou, A., Beer, N., et al. (2017). The epidemiology of invasive meningococcal disease in EU/EEA countries 2004-2014. Vaccine 35, 2034–2041. doi: 10.1016/j.vaccine.2017.03.007
Keywords: Neisseria meningitidis, serogroup B, invasive meningococcal disease, multilocus sequence typing, gMATS, MenDeVAR Index, vaccine coverage
Citation: Sereikaitė E, Plepytė R, Petrutienė A, Stravinskienė D, Kučinskaitė-Kodzė I, Gėgžna V, Ivaškevičienė I, Žvirblienė A and Plečkaitytė M (2023) Molecular characterization of invasive Neisseria meningitidis isolates collected in Lithuania (2009-2019) and estimation of serogroup B meningococcal vaccine 4CMenB and MenB-Fhbp coverage. Front. Cell. Infect. Microbiol. 13:1136211. doi: 10.3389/fcimb.2023.1136211
Received: 02 January 2023; Accepted: 06 February 2023;
Published: 15 February 2023.
Edited by:
Michael Marceau, Université Lille Nord de France, FranceReviewed by:
Wieke Freudenburg - De Graaf, Amsterdam University Medical Center, NetherlandsCopyright © 2023 Sereikaitė, Plepytė, Petrutienė, Stravinskienė, Kučinskaitė-Kodzė, Gėgžna, Ivaškevičienė, Žvirblienė and Plečkaitytė. This is an open-access article distributed under the terms of the Creative Commons Attribution License (CC BY). The use, distribution or reproduction in other forums is permitted, provided the original author(s) and the copyright owner(s) are credited and that the original publication in this journal is cited, in accordance with accepted academic practice. No use, distribution or reproduction is permitted which does not comply with these terms.
*Correspondence: Milda Plečkaitytė, bWlsZGEucGxlY2thaXR5dGVAYnRpLnZ1Lmx0
Disclaimer: All claims expressed in this article are solely those of the authors and do not necessarily represent those of their affiliated organizations, or those of the publisher, the editors and the reviewers. Any product that may be evaluated in this article or claim that may be made by its manufacturer is not guaranteed or endorsed by the publisher.
Research integrity at Frontiers
Learn more about the work of our research integrity team to safeguard the quality of each article we publish.