- 1Department of Medical Mycology, Shanghai Skin Disease Hospital, Tongji University School of Medicine, Shanghai, China
- 2College of Plant Protection, Henan Agricultural University, Zhengzhou, China
Previous studies have shown that natural polyacetylene alcohols, such as falcarindiol (FADOH), have good antifungal effects on plant fungi. While its effect on fungi that infect humans remains to be explored. In our study, checkerboard microdilution, drop-plate assay, and time-growth method were employed to analyze the interactions between FADOH and itraconazole (ITC) in vitro against dermatophytes, including 12 Trichophyton rubrum (T. rubrum), 12 Trichophyton mentagrophytes (T. mentagrophytes), and 6 Microsporum canis (M. canis). The results showed that the combination of FADOH and ITC exhibited synergistic and additive activity against 86.7% of all tested dermatophytes. FADOH had an excellent synergistic effect on ITC against T. rubrum and T. mentagrophytes; the synergistic rates were 66.7% and 58.3%, respectively. On the contrary, FADOH combined with ITC showed poor synergistic inhibitory activity (16.7%) against M. canis. Moreover, the additive rates of these two drugs against T. rubrum, T. mentagrophytes, and M. canis were 25%, 41.7%, and 33.3%, respectively. No antagonistic interactions were observed. The drop-plate assay and time-growth curves confirmed that the combination of FADOH and ITC had a potent synergistic antifungal effect. The in vitro synergistic effect of FADOH and ITC against dermatophytes is reported here for the first time. Our findings suggest the potential use of FADOH as an effective antifungal drug in the combined therapy of dermatophytoses caused especially by T. rubrum and T. mentagrophytes.
Introduction
Dermatophyte infections are often superficial and involve the epidermis, skin and keratinized structures like hairs and nails (Akay et al., 2019). On rare occasions, dermatophytes can cause invasive infections involving hair follicles, subcutaneous tissues, and internal organs such as lymph nodes, bones, and the brain (Wang et al., 2021; Silva et al., 2022). Invasive dermatophytoses can be classified as Majocchi’s granuloma, deep dermatophytosis, pseudomycetoma, and disseminated dermatophytosis (Rouzaud et al., 2015; Wang et al., 2021; Silva et al., 2022). A systematic review of invasive dermatophyte infections showed that Trichophyton rubrum (T. rubrum) was the most prevalent pathogen responsible for invasive dermatophytoses, followed by Trichophyton mentagrophytes (T. mentagrophytes), and Microsporum canis (M. canis) (Wang et al., 2021). Deep dermatophytosis is a rare and sometimes life-threatening fungal infection. This condition mainly occurs in immunosuppressed individuals or patients with mutations in genes, including Caspase Recruitment Domain-containing protein 9 (CARD9) and signal transducer and activator of transcription 3 (STAT3) (Wang et al., 2021). Therefore, treating chronic superficial tinea in these immunosuppressed patients and patients with genetic mutations is critical to prevent the development of invasive dermatophytoses.
Although dermatophytoses are generally considered easy to treat, dermatophyte resistance to existing antifungal drugs (e.g., terbinafine, itraconazole, fluconazole, amphotericin B, and posaconazole) is increasing, and infections are more likely to recur(Khurana et al., 2019; Wang et al., 2021). More importantly, these drugs are usually considered to cause some side effects, such as liver function damage. To overcome the limitations of current treatment strategies, it is necessary to explore combination therapies through in vitro experiments.
Natural polyacetylene alcohols can be found in many sources, including natural food products and traditional medicinal materials. Falcarindiol (FADOH, Figure 1), a kind of natural polyacetylene alcohol, is a polyacetylenic oxylipin found in vegetables of the carrot family (Apiaceae) and natural Chinese herbal medicines, such as notopterygium incisum, angelica keiskei and angelica dahurica(Yang et al., 2014; Yao and Li, 2015; Andersen et al., 2020; Zhao et al., 2021). It has been found that FADOH has anticancer, antifungal, antibacterial, and anti-inflammatory effects and can also be used as a dietary supplement for neuroprotection (Dawid et al., 2015; Jeon et al., 2020). There are more and more reports on the antifungal activity against plant fungi using polyacetylene alcohols, but there is little research on the antifungal activity against dermatophytes. Previous studies have found that the mechanism of polyacetylene alcohols against plant fungi and tumors is related to lipid metabolism (Nickel et al., 2015; Andersen et al., 2020). We hypothesize that the mechanism of FADOH against dermatophytes is also related to lipid metabolism. This work mainly explores whether the combination of FADOH and ITC exhibits synergistic inhibitory activity in vitro against dermatophytes.
Materials and methods
Tested strains and culture conditions
Our previous study determined the antifungal activity of FADOH against Candida spp., dermatophytes, Aspergillus and dematiaceous fungi. We found that FADOH had strong antifungal activity against dermatophytes, so we chose dermatophytes for subsequent experiments. In this study, clinical isolates were tested, including 11 T. rubrum, 11 T. mentagrophytes, and 6 M. canis. As reference strains were used T. rubrum ATCC4438 and T. mentagrophytes ATCC4439. All strains were typically preserved and subcultured on potato dextrose agar (PDA). Species were all identified by phenotype analysis and DNA sequencing. For molecular identification of common dermatophytes, extracted DNAs were amplified by the universal fungal primers ITS1 (5’-TCCGTA GGTGAACCTGCG G-3’) and ITS4 (5’-TCCTCCGCT TATTGATATGC-3’), and digested with Mval enzymes. Final Molecular identification was performed by sequencing and BLAST (http://www.ncbi.nlm.nih.gov/BLAST/) from the National Center for Biotechnology Information (NCBI) database.
Antifungal agents
All agents (FADOH and ITC) were purchased from Selleck Biotech Co., Ltd. The stock solutions of tested compounds were filter sterilized and prepared following the broth microdilution method M38-Ed3 as detailed by the Clinical and Laboratory Standards Institute (CLSI) (PA, 2017). The stock solutions of FADOH and ITC were dissolved in dimethyl sulfoxide to 20mg/ml and 200μg/ml, respectively. All stock solutions were stored at -20°C.
Antifungal susceptibility test
The minimum inhibitory concentrations (MICs) of FADOH and ITC in case of tested dermatophyte strains were determined by the broth microdilution method based on the CLSI M38-Ed3 (PA, 2017). The final concentrations of FADOH and ITC from column 2 to column 11 in 96-well plates were 200–0.39μg/ml and 0.5–0.001μg/ml, respectively (100μl). RPMI1640 medium without drug was added to columns 1 and 12 as blank control and growth control, respectively. All strains were subcultured on PDA at 28°C for five days. Conidia were suspended in sterile saline solution (0.85%), and the suspension was twofold more concentrated than the density needed for testing (1×103 to 3×103 CFU/ml). 100µl serially diluted drugs and 100 µl cell suspensions were added to 96-well plates and then incubated at 35°C for four days. The growth inhibition was measured by optical density (OD) at 600 nm (OD600) using a microplate reader (Tecan SUNRISE). The MIC was defined as the concentration of tested compound that inhibited growth and proliferation of dermatophyte cells in 80% comparing to growth control (background OD subtracted). All assays were performed in triplicate and repeated on another day.
Checkerboard method
For the broth microdilution checkerboard assays, each drug was serially diluted 2-fold in RPMI 1640 medium. The final FADOH and ITC concentrations ranged from 50 to 0.78µg/ml and 0.5 to 0.001µg/ml, respectively. A 50µl aliquot from solutions with the appropriate concentration of ITC and FADOH was added to the wells in the 2nd to 11th columns and the B to H lines, respectively. Row A and column 1 contained the ITC and FADOH alone, respectively. The well at the intersection of row A and column 1 was the drug-free one that performed in 200µl RPMI1640 as blank control. The 12th column served as the growth control. 100µl cell suspensions to a final concentration of 1–3 × 103 CFU/ml were added to each well mentioned above. The plates were incubated at 35°C for four days. Drug interactions were identified based on the fractional inhibitory concentration index (FICI) (Saracino et al., 2022). A synergistic effect is observed when FICI value ≤ 0.5; an additive effect when 0.5 < FICI value ≤ 1; an indifferent effect when 1 < FICI value ≤ 4; and an antagonistic effect when FICI value > 4. The above tests were performed in triplicate and repeated on another day.
Drop-plate assay
One strain for which the synergistic effect between FADOH and ITC was selected as a representative strain to perform the drop-plate assay. The YPD solid medium plate was divided into sectors, where each sector contained different concentrations of FADOH (12.5–0.78μg/ml) and ITC (0.125–0.001μg/ml) tested. Standardized inoculum of tested dermatophyte strain, the final concentration of 2× 103 CFU/ml, was spotted on individual sectors. The plate was incubated in a 35°C incubator for four days to observe the growth.
Time-growth curves
To investigate the dynamic inhibitory activity of FADOH and ITC against dermatophytes, OD values of different drug-treated groups were measured, and time-growth curves were graphed. The time-growth curves were determined by a microplate reader in microtiter plates according to the practical steps of A. B. Alio et al. (Alio et al., 2005). Three strains for which the synergistic effect between FADOH and ITC were chosen as the representative strains, including one T. rubrum, one T. mentagrophytes, and one M.canis. Conidia were diluted to the final concentration of 2× 103 CFU/ml with a culture medium (RPMI 1640) and treated with FADOH (0.78μg/ml), ITC (0.016gμg/ml) or exposed to FADOH + ITC (0.78μg/ml + 0.016gμg/ml). The positive control contained 100μl RPMI 1640 medium plus 100μl of the respective isolate. The negative control had 200μl RPMI 1640 medium as the optical density blank. The plates were inoculated at 35°C, and then the OD values were measured at 600 nm after 8h, 16h, 24h, 48h, 72h, 96h, 120h, and 168h of incubating. Each assay was carried out in triplicate.
Statistical analyses
All experiments were performed three times, and the mean values were recorded as the final result. The SPSS (Statistical Package for the Social Sciences, Chicago, IL) 21 software package was used for the analyses. One-way analysis of variance (ANOVA) was utilized to examine if there were statistically significant differences between the means of different independent groups. P < 0.05 indicates that the difference was considered statistically significant.
Results
In vitro antifungal activities of individual FADOH and ITC used solely against dermatophytes
The MIC values of FADOH against T. rubrum, T. mentagrophytes, and M.canis tested strains were comprised in the range of 1.56–50μg/ml, 1.56–100μg/ml, and 1.56–100μg/ml, respectively. The MIC values of ITC against T. rubrum, T. mentagrophytes, and M.canis were 0.008–0.5μg/ml, 0.002–0.25μg/ml, and 0.002–0.5μg/ml, respectively. All the determined MIC values are summarized in Table 1.
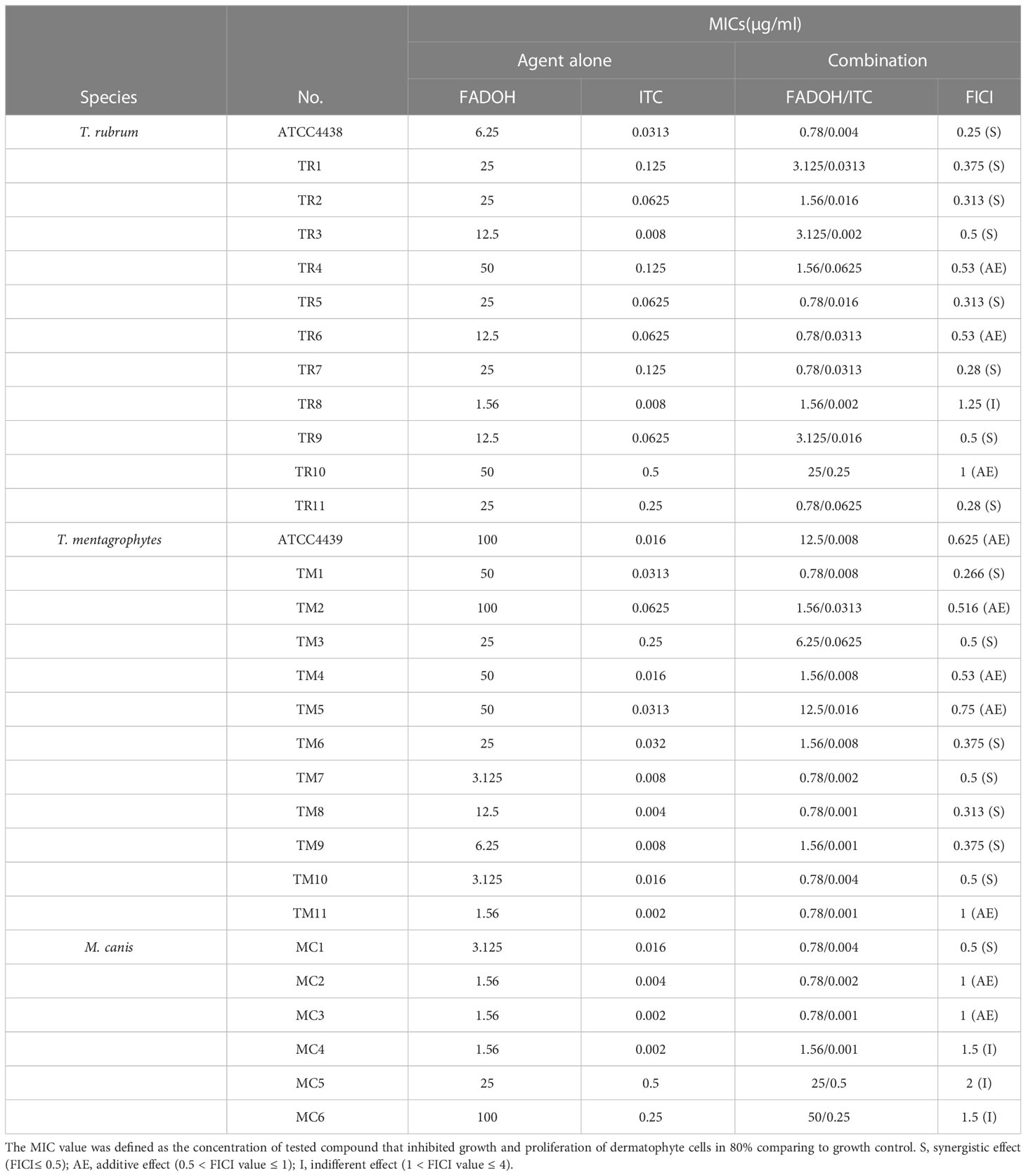
Table 1 Assessment of the type of interactions between FADOH and ITC based on susceptibility tests performed for tested dermatophyte strains.
Checkerboard assay to determine type of interactions between tested antifungals
The synergistic effect between FADOH and ITC is shown in Table 1 based on the checkerboard assay to assess the susceptibility of different dermatophyte strains. Combining FADOH and ITC reduced the MIC values for these two drugs to 0.78–50μg/ml and 0.001–0.5μg/ml, respectively, exhibiting synergistic and additive activity against 53.3% and 33.3% of all tested dermatophytes, respectively. Among 12 T. rubrum strains, synergistic effect, additive effect, and indifferent effect could be observed between tested compounds in eight strains (66.7%), three strains (25%), and one strain (8.3%), respectively. In 12 T. mentagrophytes strains, synergistic effect and additive effect could be observed between tested compounds in seven strains (58.3%) and five strains (41.7%), respectively. As for 6 M. canis strains, synergistic effect, additive effect, and indifferent effect could be observed between tested compounds in one strain (16.7%), two strains (33.3%), and three strains (50%), respectively.
Taking the T. rubrum TR1 as a representative strain, the MIC results of the checkerboard assay are shown in Figure 2. This figure showed that the MIC values for FADOH and ITC used solely were equal to 25μg/ml and 0.125μg/ml (yellow box), respectively. The combination of FADOH and ITC decreased the MIC values to 3.125μg/ml and 0.0313μg/ml (red box), respectively, showing synergistic activity (FICI=0.375). It could be observed that the combination of FADOH at higher concentrations with ITC, used even at the lowest tested concentrations, strongly inhibited cell growth and proliferation (blue box).
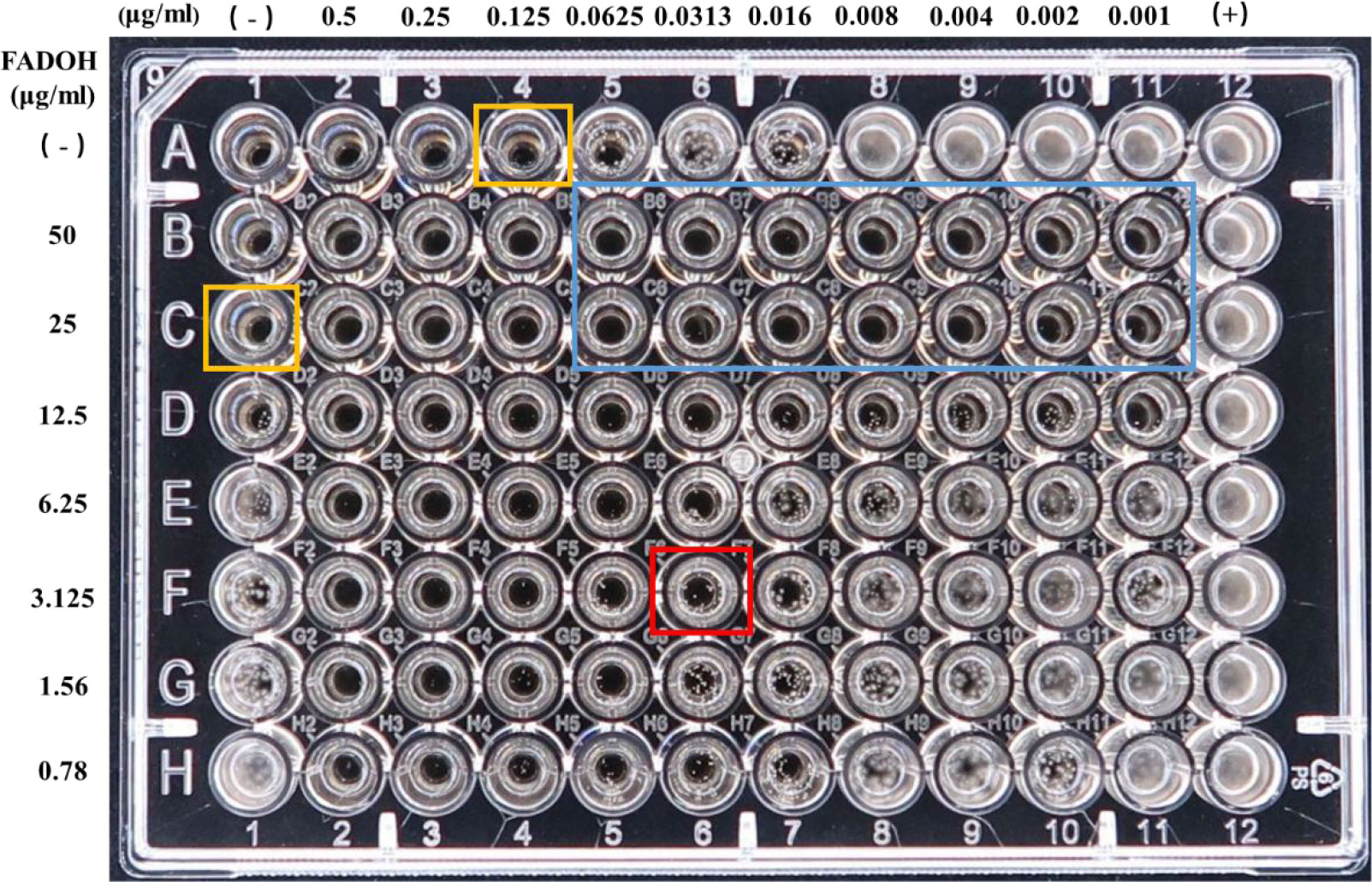
Figure 2 The MIC values determined for FADOH and ITC used solely and in different combinations in the checkerboard assay for T. rubrum TR1.
Drop-plate assay to visualize synergistic effect between FADOH and ITC
To show the synergistic antifungal activity of FADOH and ITC more clearly, a representative strain, T. mentagrophytes TM10, was selected for the drop-plate assay. The result showed after combining with FADOH, the decreased growth of fungi indicated that the antifungal activity of ITC was significantly enhanced (Figure 3). Especially when combined with 12.5 μg/ml and 6.25μg/ml of FADOH, even the low concentration of ITC still had a strong inhibitory effect on the strain.
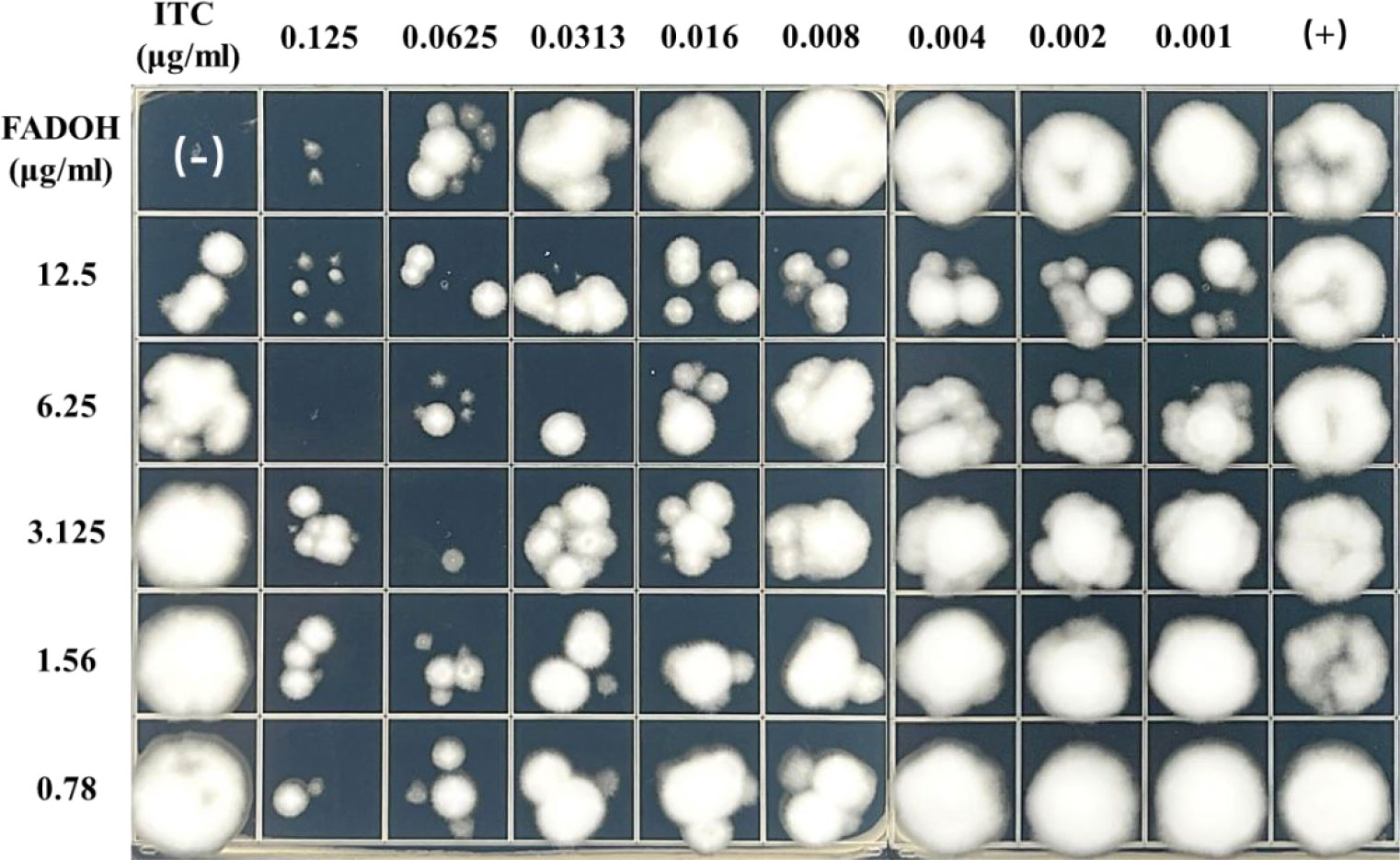
Figure 3 The antifungal activity of FADOH and ITC used solely and in combination toward T. mentagrophytes TM10 was assessed based on the dope-plate assay.
Time-growth curves
Time-growth curves were fitted to further understand the dynamic suppressive action of FADOH on ITC against dermatophytes. We took T. rubrum TR5, T. mentagrophytes TM6, and M.canis MC1 as the representative strains. The results showed that the combination of FADOH and ITC against all three strains demonstrated a potent synergistic antifungal effect at 96h, which was still very pronounced from 96h to 168h (Figure 4).
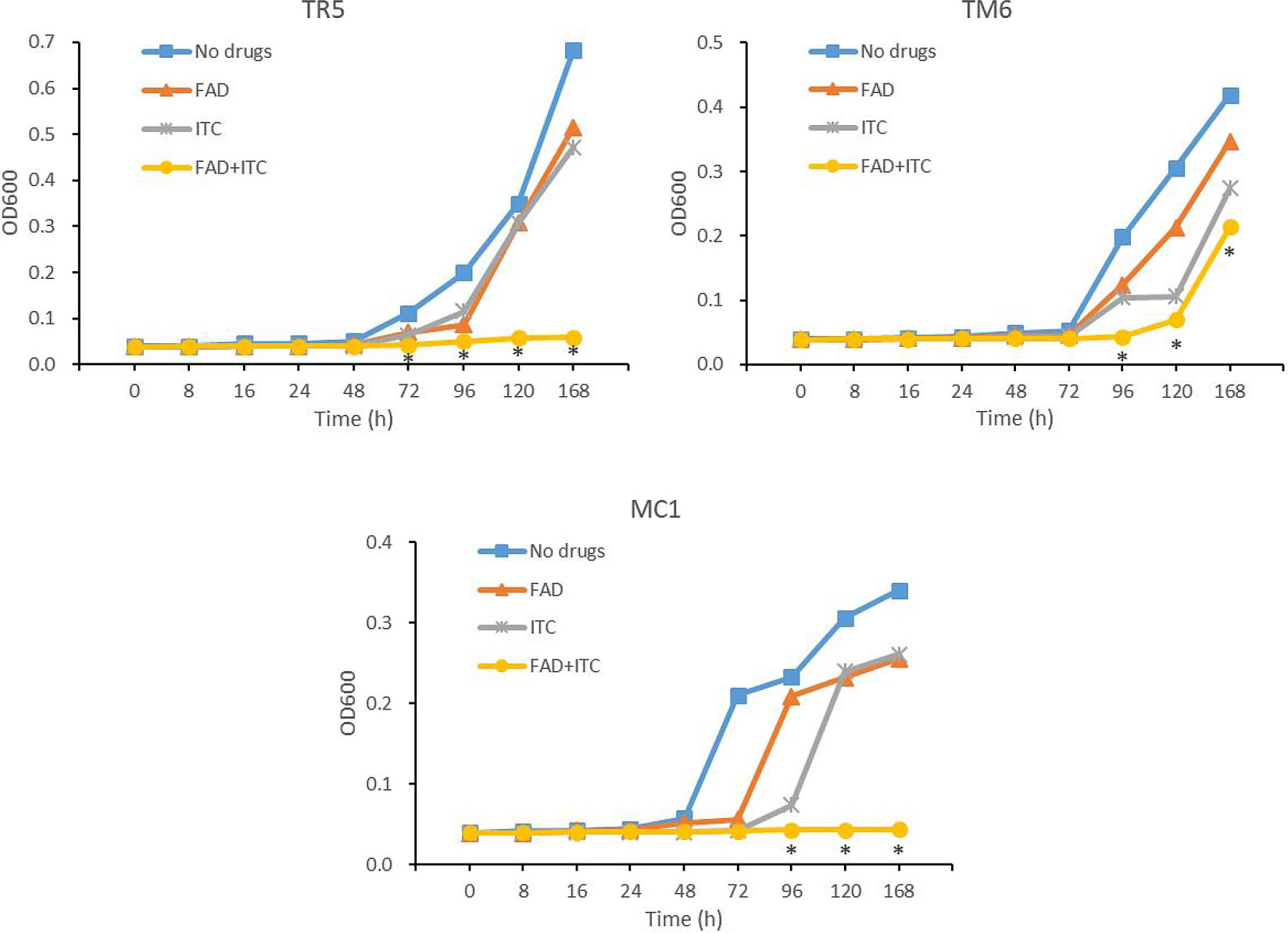
Figure 4 Growth curves of T. rubrum TR5, T. mentagrophytes TM6, and M.canis MC1 after the treatment of FADOH, ITC, and the combination of FADOH and ITC, which was measured by optical density (OD at 600 nm). *P < 0.05, the combination group showed a statistically significant difference from the control group, FADOH group, and ITC group.
Discussion
Immunosuppressed patients have a higher incidence of superficial dermatophytosis and are more prone to develop devastating invasive dermatophyte infection (Marconi et al., 2010). However, antifungal drugs for treating severe invasive fungal infections are still limited (Revie et al., 2018). Moreover, the frequent and preventive use of antifungal drugs has led to the development of antifungal drug resistance (Revie et al., 2018). The four main classes of antifungal drugs are polyenes, azoles, allylamines and echinocandins. Azole antifungal agents, such as fluconazole and ITC, have been widely used in treating dermatophytosis. Unlike allylamines (e.g., terbinafine), azoles could potentiate drug resistance development in dermatophytes (Ghannoum, 2016). Combination therapy might effectively treat severe refractory fungal infections (Xu et al., 2019). It has been found that the combination of new antifungal drugs and traditional antifungal drugs has certain synergistic or at least additive activity against many fungi in vitro (Vazquez, 2007). Natural Chinese herbal medicine contains complex constituents with good safety and a broad antifungal spectrum, including Candida, Cryptococcus neoformans and all kinds of plant fungi (Tian et al., 2010; Zhang et al., 2020; Yang et al., 2022). In addition to the main components, there are a lot of unknown trace components with novel structures and strong biological activity, which are considered as one of the important therapeutic substances and a huge source of innovative drugs of traditional Chinese medicine (Xia et al., 2022). Chinese herbal medicine can be used as a resource for the detection of new antifungal targets and has become an essential research focus and focal point in recent years.
The antifungal activity of polyacetylene alcohols isolated from apiaceous vegetables and Chinese herbal medicine has recently attracted attention. Yoon et al. found that polyacetylalcohol ciryneol A had good antifungal activity against plant fungi, such as Magnaporthe oryzae, Colletotrichum coccodes, and Pythium ultimum, with IC50 values below 50μg/ml (Kim et al., 2011). Lecomte M et al.found that FADOH had a good inhibitory effect on the fungal leaf blight pathogen Alternaria alternata (IC50 ranging from 34 to 102 μM) (Lecomte et al., 2012). Liu et al. found that C18 polyacetylenes from panax stipuleanatus exhibited high potencies against eight pathogenic fungal species tested, such as Colletotrichum gloeosporioiles, Fusarium graminearum, and Fusarium pseudograminearum, with half-maximum effective concentrations ranging from 8 to 425μg/ml (Liu et al., 2020). However, no antifungal effects of polyacetylene alcohols against dermatophytes have been reported so far.
Our study showed that FADOH had a specific antifungal effect against dermatophytes, with MICs ranging from 1.56 to 100μg/ml. FADOH combined with ITC had good synergistic and additive activity against most dermatophytes (86.7%), particularly T.rubrum and T. mentagrophytes. Interestingly, FADOH and ITC had synergistic or additive effects against all T. mentagrophytes. In addition, FADOH could reduce the MIC values of ITC against most dermatophytes (93.3%) to 2-8 times. Furthermore, drop-plate assay verified the synergistic activity of FADOH and ITC visually. We selected one strain per species for the time-growth curve test to verify the dynamic synergistic effect of FADOH and ITC on dermatophytes. The time-growth curves showed that the combination of FADOH and ITC against all three strains demonstrated a potent synergistic antifungal effect at 96h, which was still very pronounced from 96h to 168h. The combination of the two drugs against T. rubrum TM5 showed an obvious synergistic antifungal effect at 72 hours. Unlike T. rubrum TR5 and M. canis MC1, T. mentagrophytes TM6 started to grow similarly like in the control after 120h. As for whether the combination of FADOH and ITC against T. rubrum shortens the onset time of antifungal activity and whether the synergistic inhibitory effect on T. mentagrophytes is not as strong as the other two dermatophytes, we need to conduct time-growth curve tests on more strains in the future for further verification.
Although there is no report on the anti-dermatophyte mechanism of natural polyacetylene alcohols, some reports have been made on its anti-plant fungus and antitumor mechanism. HR Jin et al. found that FADOH exerted its antitumor activity by inducing endoplasmic reticulum (ER) stress and apoptosis (Jin et al., 2012). Proteomics also found that polyacetylene and other compounds were mainly involved in the metabolism of lipids and fatty acids, interfering with the activities of lipid-modifying enzymes and various membrane-related proteins such as endoplasmic reticulum (Nickel et al., 2015). CB Andersen et al. found that FADOH could activate the expression of sterol transporter, causing the redistribution of lipids and increased formation of lipid droplets in cells, which led to endoplasmic reticulum stress and cell death (Andersen et al., 2020). Fungi and human cells are eukaryotes, and sterols are the membrane lipids of most eukaryotes (Chen et al., 2007). The change of sterol in the cell membrane will destroy the integrity and fluidity of the cell membrane and affect the expression of membrane protein, thus affecting the growth and metabolism of cells (Jorda and Puig, 2020). Sterols in fungi are called ergosterol, a vital component of the fungal plasma membrane (Shapourzadeh et al., 2016). Significant structural differences in fatty acid between fungi and mammalian cells exist, making it a promising antifungal target (Bitencourt et al., 2013). The fungal cytochrome P450 enzyme sterol 14α-demethylase is a key enzyme in the ergosterol biosynthesis pathway and is the target of azoles (Rosam et al., 2020). Therefore, we speculate that the synergistic mechanism of polyacetylene alcohols and azoles against dermatophytes may mainly affect the metabolism of ergosterol and lipid, induce endoplasmic reticulum stress, and thus lead to fungal cell apoptosis.
Although further work is needed to explore the underlying mechanism for the observed synergistic activity, our findings demonstrate the potential activity of FADOH as an antifungal adjuvant to enhance the antifungal activity of azoles against dermatophytes.
Conclusions
In summary, FADOH combined with ITC has synergistic and additive antifungal activity against most dermatophytes, indicating that natural polyacetylene alcohols may be a more reliable and safe combination therapy for treating dermatophytoses and invasive dermatophyte infection.
Data availability statement
The original contributions presented in the study are included in the article/supplementary material. Further inquiries can be directed to the corresponding author.
Author contributions
SK carried out the in vitro antifungal experiment and wrote the manuscript. ZG, SL, and HY collected and analyzed the experimental data. LA, JT, QC, and RN designed and interpreted the experiment data. LY revised the manuscript critically for important content. All authors contributed to the article and approved the submitted version.
Funding
This work was supported by the National Natural Science Foundation of China (Grant No. 82173429 to LY), the Science and Technology Commission of Shanghai Municipality (Grant No. 21Y11904900 to LY) and the Clinical Research and Cultivation Project of Shanghai Skin Disease Hospital (Grant No. lcfy2022-05 to SK).
Conflict of interest
The authors declare that the research was conducted in the absence of any commercial or financial relationships that could be construed as a potential conflict of interest.
Publisher’s note
All claims expressed in this article are solely those of the authors and do not necessarily represent those of their affiliated organizations, or those of the publisher, the editors and the reviewers. Any product that may be evaluated in this article, or claim that may be made by its manufacturer, is not guaranteed or endorsed by the publisher.
References
Akay, B. N., Demirdag, H. G., Evren, E., Karahan, Z. C., Okcu Heper, A., Kundakci, N. (2019). Deep fungal infection caused by trichophyton rubrum after heart transplantation: a case report with dermoscopy. Australas. J. Dermatol. 60 (3), e252–e254. doi: 10.1111/ajd.12993
Alio, A. B., Mendoza, M., Zambrano, E. A., Diaz, E., Cavallera, E. (2005). Dermatophytes growth curve and in vitro susceptibility test: a broth micro-titration method. Med. Mycol 43 (4), 319–325. doi: 10.1080/13693780500092947
Andersen, C. B., Runge Walther, A., Pipo-Olle, E., Notabi, M. K., Juul, S., Eriksen, M. H., et al. (2020). Falcarindiol purified from carrots leads to elevated levels of lipid droplets and upregulation of peroxisome proliferator-activated receptor-gamma gene expression in cellular models. Front. Pharmacol. 11. doi: 10.3389/fphar.2020.565524
Bitencourt, T. A., Komoto, T. T., Massaroto, B. G., Miranda, C. E., Beleboni, R. O., Marins, M., et al. (2013). Trans-chalcone and quercetin down-regulate fatty acid synthase gene expression and reduce ergosterol content in the human pathogenic dermatophyte trichophyton rubrum. BMC Complement Altern. Med. 13, 229. doi: 10.1186/1472-6882-13-229
Chen, L. L., Wang, G. Z., Zhang, H. Y. (2007). Sterol biosynthesis and prokaryotes-to-Eukaryotes evolution. Biochem. Biophys. Res. Commun. 363 (4), 885–888. doi: 10.1016/j.bbrc.2007.09.093
Dawid, C., Dunemann, F., Schwab, W., Nothnagel, T., Hofmann, T. (2015). Bioactive C(1)(7)-polyacetylenes in carrots (Daucus carota l.): current knowledge and future perspectives. J. Agric. Food Chem. 63 (42), 9211–9222. doi: 10.1021/acs.jafc.5b04357
Ghannoum, M. (2016). Azole resistance in dermatophytes: prevalence and mechanism of action. J. Am. Podiatr Med. Assoc. 106 (1), 79–86. doi: 10.7547/14-109
Jeon, J. E., Kim, J. G., Fischer, C. R., Mehta, N., Dufour-Schroif, C., Wemmer, K., et al. (2020). A pathogen-responsive gene cluster for highly modified fatty acids in tomato. Cell 180 (1), 176–187.e119. doi: 10.1016/j.cell.2019.11.037
Jin, H. R., Zhao, J., Zhang, Z., Liao, Y., Wang, C. Z., Huang, W. H., et al. (2012). The antitumor natural compound falcarindiol promotes cancer cell death by inducing endoplasmic reticulum stress. Cell Death Dis. 3 (8), e376. doi: 10.1038/cddis.2012.122
Jorda, T., Puig, S. (2020). Regulation of ergosterol biosynthesis in saccharomyces cerevisiae. Genes (Basel) 11 (7), 795. doi: 10.3390/genes11070795
Khurana, A., Sardana, K., Chowdhary, A. (2019). Antifungal resistance in dermatophytes: recent trends and therapeutic implications. Fungal Genet. Biol. 132, 103255. doi: 10.1016/j.fgb.2019.103255
Kim, J.-C., Yoon, M.-Y., Choi, G. J., Choi, Y. H., Jang, K. S., Cha, B. (2011). Antifungal activity of polyacetylenes isolated from cirsium japonicum roots against various phytopathogenic fungi. Ind. Crops Products 34 (1), 882–887. doi: 10.1016/j.indcrop.2011.02.013
Lecomte, M., Beruyer, R., Hamama, L. (2012). Inhibitory effects of the carrot metabolites 6-methoxymellein and falcarindiol on development of the fungal leaf blight pathogen Alternaria dauci. Physiol. Mol. Plant Pathol. 80 (5), 58–67. doi: 10.1016/j.pmpp.2012.10.002
Liu, J., Lu, S., Feng, J., Li, C., Wang, W., Pei, Y., et al. (2020). Enantioselective synthesis and antifungal activity of C18 polyacetylenes. J. Agric. Food Chem. 68 (7), 2116–2123. doi: 10.1021/acs.jafc.9b07967
Marconi, V. C., Kradin, R., Marty, F. M., Hospenthal, D. R., Kotton, C. N. (2010). Disseminated dermatophytosis in a patient with hereditary hemochromatosis and hepatic cirrhosis: case report and review of the literature. Med. Mycol 48 (3), 518–527. doi: 10.3109/13693780903213512
Nickel, S., Serwa, R. A., Kaschani, F., Ninck, S., Zweerink, S., Tate, E. W., et al. (2015). Chemoproteomic evaluation of the polyacetylene callyspongynic acid. Chemistry 21 (30), 10721–10728. doi: 10.1002/chem.201500934
PA, W. (2017). Reference method for broth dilution antifungal susceptibility testing of filamentous fungi. 3rd Ed (Clsi Standard M38 Clinical and Laboratory Standards Institute (CLSI). Available at: https://clsi.org/standards/products/microbiology/documents/m38/
Revie, N. M., Iyer, K. R., Robbins, N., Cowen, L. E. (2018). Antifungal drug resistance: evolution, mechanisms and impact. Curr. Opin. Microbiol. 45, 70–76. doi: 10.1016/j.mib.2018.02.005
Rosam, K., Monk, B. C., Lackner, M. (2020). Sterol 14alpha-demethylase ligand-binding pocket-mediated acquired and intrinsic azole resistance in fungal pathogens. J. Fungi (Basel) 7 (1), 1. doi: 10.3390/jof7010001
Rouzaud, C., Hay, R., Chosidow, O., Dupin, N., Puel, A., Lortholary, O., et al. (2015). Severe dermatophytosis and acquired or innate immunodeficiency: a review. J. Fungi (Basel) 2 (1), 4. doi: 10.3390/jof2010004
Saracino, I. M., Foschi, C., Pavoni, M., Spigarelli, R., Valerii, M. C., Spisni, E. (2022). Antifungal activity of natural compounds vs. candida spp.: a mixture of cinnamaldehyde and eugenol shows promising in vitro results. Antibiotics (Basel) 11 (1), 73. doi: 10.3390/antibiotics11010073
Shapourzadeh, A., Rahimi-Verki, N., Atyabi, S. M., Shams-Ghahfarokhi, M., Jahanshiri, Z., Irani, S., et al. (2016). Inhibitory effects of cold atmospheric plasma on the growth, ergosterol biosynthesis, and keratinase activity in trichophyton rubrum. Arch. Biochem. Biophys. 608, 27–33. doi: 10.1016/j.abb.2016.07.012
Silva, L., Sousa, J., Toscano, C., Viana, I. (2022). Deep dermatophytosis caused by trichophyton rubrum in immunocompromised patients. Bras. Dermatol. 97 (2), 223–227. doi: 10.1016/j.abd.2021.05.014
Tian, J., Shen, Y., Yang, X., Liang, S., Shan, L., Li, H., et al. (2010). Antifungal cyclic peptides from psammosilene tunicoides. J. Nat. Prod 73 (12), 1987–1992. doi: 10.1021/np100363a
Vazquez, J. A. (2007). Combination antifungal therapy: the new frontier. Future Microbiol. 2 (2), 115–139. doi: 10.2217/17460913.2.2.115
Wang, R., Huang, C., Zhang, Y., Li, R. (2021). Invasive dermatophyte infection: a systematic review. Mycoses 64 (4), 340–348. doi: 10.1111/myc.13212
Xia, X. F., Xia, G. Y., Wu, Y. Z., Xia, H., Wang, L. Y., Shang, H. C., et al. (2022). Trace therapeutic substances of traditional Chinese medicine: great resources of innovative drugs derived from traditional Chinese medicine. Zhongguo Zhong Yao Za Zhi 47 (7), 1705–1729. doi: 10.19540/j.cnki.cjcmm.20220210.201
Xu, J., Liu, R., Sun, F., An, L., Shang, Z., Kong, L., et al. (2019). Eucalyptal d enhances the antifungal effect of fluconazole on fluconazole-resistant candida albicans by competitively inhibiting efflux pump. Front. Cell Infect. Microbiol. 9. doi: 10.3389/fcimb.2019.00211
Yang, C. J., Li, H. X., Wang, J. R., Zhang, Z. J., Wu, T. L., Liu, Y. Q., et al. (2022). Design, synthesis and biological evaluation of novel evodiamine and rutaecarpine derivatives against phytopathogenic fungi. Eur. J. Med. Chem. 227, 113937. doi: 10.1016/j.ejmech.2021.113937
Yang, Z. M., Zhao, J., Lao, K. M., Chen, X. J., Leong, F., Wang, C. Z., et al. (2014). Determination of six polyynes in oplopanax horridus and oplopanax elatus using polyethylene glycol modified reversed migration microemulsion electrokinetic chromatography. Electrophoresis 35 (20), 2959–2964. doi: 10.1002/elps.201400159
Yao, Y. Z., Li, S. H. (2015). Chemical constituents from angelica keiskei. Zhong Yao Cai 38 (8), 1656–1660.
Zhang, Z., Bai, J., Zeng, Y., Cai, M., Yao, Y., Wu, H., et al. (2020). Pharmacology, toxicity and pharmacokinetics of acetylshikonin: a review. Pharm. Biol. 58 (1), 950–958. doi: 10.1080/13880209.2020.1818793
Keywords: falcarindiol, itraconazole, dermatophytes, synergistic, additive, polyacetylene alcohols
Citation: Kan S, Tan J, Cai Q, An L, Gao Z, Yang H, Liu S, Na R and Yang L (2023) Synergistic activity of the combination of falcarindiol and itraconazole in vitro against dermatophytes. Front. Cell. Infect. Microbiol. 13:1128000. doi: 10.3389/fcimb.2023.1128000
Received: 20 December 2022; Accepted: 24 April 2023;
Published: 03 May 2023.
Edited by:
Yi Sun, Jingzhou Hospital Affiliated to Yangtze University, ChinaReviewed by:
Mariusz Dyląg, University of Wroclaw, PolandQiaoyun Lu, Hubei University of Arts and Science, China
Qunling Zhang, Fudan University, China
Copyright © 2023 Kan, Tan, Cai, An, Gao, Yang, Liu, Na and Yang. This is an open-access article distributed under the terms of the Creative Commons Attribution License (CC BY). The use, distribution or reproduction in other forums is permitted, provided the original author(s) and the copyright owner(s) are credited and that the original publication in this journal is cited, in accordance with accepted academic practice. No use, distribution or reproduction is permitted which does not comply with these terms.
*Correspondence: Lianjuan Yang, lianjuanyang@163.com