- Department of Pathobiology, College of Veterinary Medicine, University of Illinois Urbana-Champaign, Urbana, IL, United States
The intracellular protozoan parasite of the genus Cryptosporidium is among the leading causes of waterborne diarrheal disease outbreaks throughout the world. The parasite is transmitted by ingestion of infective oocysts that are highly stable in the environment and resistant to almost all conventional disinfection methods and water treatments. Control of the parasite infection is exceedingly difficult due to the excretion of large numbers of oocysts in the feces of infected individuals that contaminate the environment and serve as a source of infection for susceptible hosts including humans and animals. Drug development against the parasite is challenging owing to its limited genetic tractability, absence of conventional drug targets, unique intracellular location within the host, and the paucity of robust cell culture platforms for continuous parasite propagation. Despite the high prevalence of the parasite, the only US Food and Drug Administration (FDA)-approved treatment of Cryptosporidium infections is nitazoxanide, which has shown moderate efficacy in immunocompetent patients. More importantly, no effective therapeutic drugs are available for treating severe, potentially life-threatening cryptosporidiosis in immunodeficient patients, young children, and neonatal livestock. Thus, safe, inexpensive, and efficacious drugs are urgently required to reduce the ever-increasing global cryptosporidiosis burden especially in low-resource countries. Several compounds have been tested for both in vitro and in vivo efficacy against the disease. However, to date, only a few experimental compounds have been subjected to clinical trials in natural hosts, and among those none have proven efficacious. This review provides an overview of the past and present anti-Cryptosporidium pharmacotherapy in humans and agricultural animals. Herein, we also highlight the progress made in the field over the last few years and discuss the different strategies employed for discovery and development of effective prospective treatments for cryptosporidiosis.
1. Introduction
1.1. History
The intracellular protozoan parasite Cryptosporidium is one of the most common parasitic pathogens causing enteric disease in humans and in a broad range of animals worldwide (Chalmers, 2014). First recognized and described briefly in 1907 by Ernest Tyzzer in the gastric glands of the common mouse (Tyzzer, 1907), Cryptosporidium was later described in greater detail in 1910, again from histological preparations from the murine gastric mucosa (Tyzzer, 1910). Tyzzer proposed the name Cryptosporidium muris for the parasite (Tyzzer, 1907; Tyzzer, 1910). In 1912, Tyzzer described another species with smaller oocysts than those of C. muris in the small intestine of experimentally infected laboratory mice, which he named Cryptosporidium parvum (Tyzzer, 1912). Although Cryptosporidium was subsequently identified in a wide range of domesticated animals, this genus of parasites only gained importance in the 1970s (after almost 7 decades from its initial discovery), when the parasite was found to be linked to gastrointestinal disease in humans and farm animals (Panciera et al., 1971; Meuten et al., 1974; Meisel et al., 1976; Nime et al., 1976). In the 1980s, cryptosporidiosis gained more widespread recognition after reports of fatal cryptosporidiosis in AIDS patients (Soave et al., 1984), zoonotic cryptosporidiosis in immunocompetent and immunodeficient humans (Current et al., 1983), waterborne human diarrheal outbreaks (D'Antonio et al., 1985; Hayes et al., 1989), and diarrheal disease in children (Sallon et al., 1988) and animals (Tzipori et al., 1980; Moon and Bemrick, 1981; Angus et al., 1982). In 1993, Cryptosporidium caused the largest documented drinking water outbreak in US history, which affected an estimated 403,000 people in Milwaukee, Wisconsin, and resulted in over $96 million in combined healthcare costs and productivity losses (Mac Kenzie et al., 1994; Hoxie et al., 1997; Corso et al., 2003). The enormity of the Milwaukee outbreak sparked concern among the public and attracted generous funds for Cryptosporidium research from governmental agencies all over the world during the next decade. This resulted in further advances in our knowledge about the basic biology of the parasite and the development of reliable molecular detection tools for estimating the global burden of the disease.
1.2. Life cycle
The life cycle of Cryptosporidium is direct and complex (Figure 1), consisting of both asexual multiplication and sexual reproduction phases within a single host that culminate in the production of environmentally resistant oocysts (Current and Garcia, 1991). Following ingestion of sporulated thick-walled oocysts, four infectious sporozoites are released from each oocyst that attach to the apical surface of intestinal epithelial cells, and then actively invade the host cell membrane to form an intracellular but extracytoplasmic parasitophorous vacuole (Current and Reese, 1986). Within the vacuole, sporozoites mature into trophozoites, which undergo three rounds of asexual proliferation, followed by a single generation of sexual stages to generate either thin-walled or thick-walled oocysts, each containing four haploid sporozoites (Current and Reese, 1986; English et al., 2022). Thick-walled oocysts containing two-layered membranes are environmentally resistant and are passed out of the body in feces, where they are immediately infectious for other susceptible hosts. Thin-walled oocysts rupture in the intestinal lumen, releasing naked infectious sporozoites that autoinfect other enteric cells to ensure continued infection of the same host.
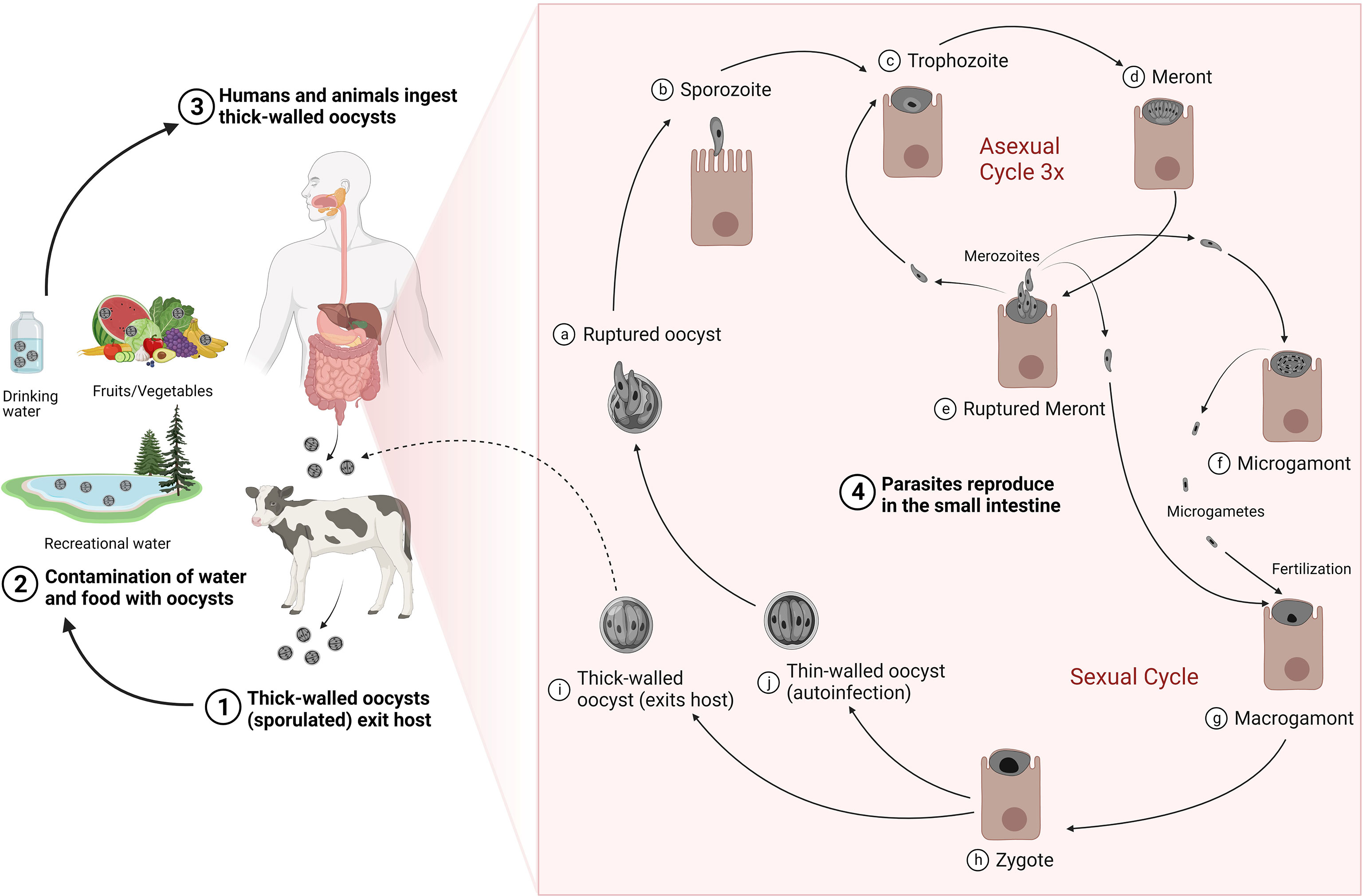
Figure 1 Life cycle and transmission of Cryptosporidium. Thick-walled sporulated oocysts are released in the feces of infected hosts (1) that contaminate food and water sources (2). Transmission occurs mainly by ingestion of contaminated water or food by susceptible hosts (3). Following ingestion, oocyst ruptures (4a) to release four sporozoites (4b). Sporozoites exhibit gliding motility, enter the host epithelial cells and mature into trophozoites (4c), which undergo three rounds of asexual multiplication to produce meronts (4d) that invariably release eight merozoites (4e). Merozoites released from the third round of asexual proliferation give rise to the sexual stages upon reinvasion of host cells: the male microgamonts (4f) and the female macrogamonts (4g). Microgametes released from the microgamont penetrate and fertilize macrogamonts to form diploid zygotes (4h). The zygotes undergo meiosis and sporogony generating either thin-walled (4i) or thick-walled (4j) oocysts, each containing four haploid sporozoites. Thick-walled oocysts are released into the lumen of the intestine and excreted into the environment, where they are instantly infectious. The thin-walled oocysts, in contrast, excyst to cause autoinfection in the same host. Adapted with modification from (CDC, 2019). Created with BioRender.com.
1.3. Transmission
In general, cryptosporidiosis is transmitted through the fecal-oral route (Figure 1) and contact with animals, manure or contaminated food and water is believed to lead to infections in humans (Xiao et al., 2004). Transmission in animals mainly occurs via ingestion of oocysts excreted by infected animals especially neonates in overcrowded or mixed housing facilities. Manure produced by livestock, especially cattle, is an important source of infection to both animals and people and it has been estimated that the global Cryptosporidium load in livestock manure is approximately 3.2 × 1023 oocysts per year (Vermeulen et al., 2017). Oocysts are highly stable in the environment and resistant to almost all conventional disinfection methods and water treatments such as chlorination (Fayer et al., 2000). Indeed, these persistent parasites have been found to be responsible for majority of the global protozoal water outbreaks that occurred from 2004–2010 (Karanis, 2018) and pose the biggest pathogen threat to the water industry (Chalmers, 2012). In the United States, exposure to treated recreational water such as swimming pools and water playgrounds was responsible for nearly 35% of the reported cryptosporidiosis outbreaks resulting in almost 57% cases during 2009–2017 (Gharpure et al., 2019). In addition to treated recreational water, contact with infected cattle (~15%), and contact with infected persons in childcare settings (~13%) were the other predominant causes of these outbreaks (Gharpure et al., 2019). Cryptosporidium is also recognized as an important foodborne pathogen, being responsible for more than 40 documented foodborne outbreaks to date, and more than 8 million cases of foodborne illnesses annually (Zahedi and Ryan, 2020). However, these numbers may be highly under-reported due to the lack of proper surveillance and the difficulties in tracing the source of foodborne disease outbreaks. Food can be contaminated at any point along the food production chain (during processing, distribution, or preparation) by direct contact with infected food handlers or by indirect exposure to water, preparation surfaces, equipment, or utensils contaminated with oocysts. Raw unpasteurized milk, unpasteurized apple cider, and salads are most associated with foodborne outbreaks of cryptosporidiosis (Gharpure et al., 2019; Zahedi and Ryan, 2020).
2. Cryptosporidiosis global disease burden in humans and animals
Cryptosporidium spp. enjoy high parasitic success due to their wide host range, low infective threshold, high excretion of resistant oocysts from infected individuals, and water-borne route of transmission (Innes et al., 2020). Protozoa of this genus are associated with diarrheal disease throughout the world with a higher incidence in developing countries (Shirley et al., 2012). Cryptosporidiosis is a major cause of public health concern in developed countries as well, with reported cases on the rise mainly due to the leading role of Cryptosporidium in causing waterborne outbreaks (Gharpure et al., 2019). Unfortunately, the global burden of cryptosporidiosis is likely to be underestimated, due to the lack of cheap and consistent methods of diagnosis, under-recognized disease in immunocompetent patients, lack of proper surveillance in developed countries, and difficulties observed in measuring the impact of the disease in poor-resource areas. Many infected individuals either do not exhibit symptoms or exhibit mild symptoms due to a self-limiting illness, and such infections often go unrecognized. There is a wide range of disease severity that is affected by the host’s age, nutritional, and immune status, and perhaps by the parasite species and subtype (Shirley et al., 2012). Differences in sensitivity of methods, type of diagnostics used, and study populations have resulted in a large variation in burden estimates for diarrhea from Cryptosporidium infection in humans and animals. However, the recent advances in knowledge and the development of highly sensitive and superior diagnostic and typing tools have improved our understanding of the epidemiology and true burden of the disease.
2.1. Humans
Out of the currently documented 44 species of Cryptosporidium, Cryptosporidium hominis (C. hominis) and Cryptosporidium parvum (C. parvum) are responsible for most human infections (Ryan et al., 2021). While C. hominis is primarily anthroponotic and only infects humans, C. parvum is a zoonotic parasite that can be transmitted between humans and animals. Apart from C. hominis and C. parvum, 21 other Cryptosporidium species and genotypes including C. meleagridis, C. felis, C. canis, C. ubiquitum, C. cuniculus, C. ditrichi, C. erinacei, C. fayeri, C. scrofarum, C. tyzzeri, C. viatorum, C. muris, C. andersoni, C. suis, C. bovis, C. occultus, C. xiaoi, horse genotype, chipmunk genotype I, skunk genotype, and mink genotype have also been reported in humans (Ryan et al., 2021).
Cryptosporidiosis is considered a high-risk and often lethal opportunistic disease for patients with compromised immune systems such as those suffering from HIV/AIDS (O'Connor R et al., 2011) or those receiving organ transplants (Danziger-Isakov, 2014; Bhadauria et al., 2015). The global prevalence of Cryptosporidium in HIV/AIDS patients was 10.09% during the period from 2007 to 2017 (Wang et al., 2018). However, the greatest burden of cryptosporidiosis occurs among young children living in less developed countries. Cryptosporidium prevalence is higher in such areas that lack proper sanitation facilities, mainly drinking water and sewage, which led the World Health Organization (WHO) to include it in the water sanitation and health program (WHO, 2009). Several epidemiological studies conducted in the previous decade have estimated the disease burden of diarrheal pathogens in developing countries. In the Global Enteric Multicenter Study (GEMS) conducted at seven sites in sub-Saharan Africa and South Asia, Cryptosporidium was found to be the main cause of linear growth faltering and the second leading cause of moderate-to-severe diarrhea in infants (0–11 months of age) (Kotloff et al., 2013; Nasrin et al., 2021). Cryptosporidium infection was also associated with a higher risk of mortality in diarrheic children aged 12–23 months who were admitted to hospitals (Kotloff et al., 2013). The 2016 Global Burden of Diseases, Injuries, and Risk Factors study (GBD) identified Cryptosporidium as a leading cause of diarrheal mortality in children younger than 5 years old with an estimated loss of 4.2 million disability-adjusted life years (DALYs) (Troeger et al., 2018). However, this study focused on acute illness alone, and as such, the number increased to 12.9 million DALYs, when long-term-effects of cryptosporidiosis such as growth retardation and cognitive defects were also considered (Khalil et al., 2018). Furthermore, the MAL-ED (Etiology, Risk Factors, and Interactions of Enteric Infections and Malnutrition and the Consequences for Child Health and Development Project) study carried out at eight sites in South America, sub-Saharan Africa, and Asia, found that Cryptosporidium along with four other pathogens exhibited the highest attributable burdens of diarrhea in community clinics in the first year of life (Platts-Mills et al., 2015).
2.2. Cattle
At least four main Cryptosporidium species infect cattle: C. parvum, C. bovis, C. ryanae, and C. andersoni (Lindsay et al., 2000; Santin et al., 2004; Fayer et al., 2005; Fayer et al., 2008), although other species have also been reported in sporadic cases, including C. felis, C. hominis, C. suis, C. canis, C. scrofarum, C. tyzzeri, C. serpentis, and C. occultus (formerly known as the C. suis-like genotype) (Robertson et al., 2014; Santin, 2020). The occurrence of C. parvum, C. bovis, C. ryanae, and C. andersoni in cattle follows an age-related pattern: the zoonotic C. parvum infects mostly pre-weaned calves, C. bovis and C. ryanae are found mostly in post-weaned calves, whereas C. andersoni is the predominant species found in heifers and adults (de Graaf et al., 1999; Santin et al., 2004; Fayer et al., 2006; Santin et al., 2008; Khan et al., 2010).
Cryptosporidiosis is one of the most important global causes of diarrhea in neonatal farm ruminants including calves. Cryptosporidium parasites invade intestinal epithelial cells and cause severe mucosal erosion resulting in villus shortening and fusion, and hypertrophy of crypts at small intestinal sites that lead to impaired digestion and increased intestinal permeability (Tzipori et al., 1983). The resulting diarrhea causes high production losses including mortality, reduced live weight gain, veterinary costs, and the added feeding and rearing costs for affected animals with slowed growth rates (Innes et al., 2020; Santin, 2020; Shaw et al., 2020). Cryptosporidiosis is recognized as endemic in cattle worldwide and the prevalence of bovine cryptosporidiosis varies substantially between countries, age groups, and studies, ranging from 11.7 to 78%, with the highest incidence reported in pre-weaned calves (Santin et al., 2004; Watanabe et al., 2005; Fayer et al., 2006; Maddox-Hyttel et al., 2006; Trotz-Williams et al., 2007; Khan et al., 2010; Amer et al., 2013; Thomson et al., 2017; Hatam-Nahavandi et al., 2019). An 18-month longitudinal study that focused on 2,545 dairy heifer calves from birth to weaning at 104 dairy operations in 13 US states identified at least 1 calf positive for Cryptosporidium at almost all operations (Urie et al., 2018b). Furthermore, the overall prevalence of Cryptosporidium in pre-weaned heifer calves was 43.1% with the disease more prevalent among young calves less than 2 weeks of age (63.3%) compared with calves older than 6 weeks (9.1%) (Urie et al., 2018a).
2.3. Small ruminants
Of the species infecting small ruminants, C. parvum, C. ubiquitum, and C. xiaoi are the most frequently detected species (Fayer and Santin, 2009; Fayer et al., 2010; Santin, 2020). In addition, C. andersoni, C. bovis, C. ryanae, C. hominis, C. fayeri, C. baileyi, and C. suis have been identified sporadically in sheep and goats (Hatam-Nahavandi et al., 2019; Santin, 2020).
Cryptosporidium causes significant morbidity and mortality in neonatal lambs and goat kids (de Graaf et al., 1999; Wright and Coop, 2007). Diarrhea resulting in reduced productivity and growth has been associated with Cryptosporidium infections in lambs and kids (Paraud and Chartier, 2012; Jacobson et al., 2016; Jacobson et al., 2018). Cryptosporidium shedding was also associated with less carcass weight and lowered dressing percentage in both symptomatic and apparently asymptomatic sheep on Australian farms (Jacobson et al., 2016). A wide range of Cryptosporidium prevalence based on microscopy and molecular detection methods has been reported in small ruminants worldwide ranging from 12.5% to 77.4% in lambs (Causape et al., 2002; Ryan et al., 2005; Castro-Hermida et al., 2007; Goma et al., 2007; Santin et al., 2007; Geurden et al., 2008) and from 4.8% to 70.8% in goat kids (Noordeen et al., 2001; Watanabe et al., 2005; Castro-Hermida et al., 2007; Goma et al., 2007; Geurden et al., 2008). As in cattle, Cryptosporidium oocysts are found mostly in feces of very young animals (1−3 weeks of age), with a lower incidence in older animals (de Graaf et al., 1999; Noordeen et al., 2001; Causape et al., 2002; Santín and Trout, 2007).
2.4. Pigs
The most common species and subtypes found in pigs are C. parvum, C. suis, and C. scrofarum formerly known as Cryptosporidium pig genotype II (Zahedi and Ryan, 2020), although C. muris and C. tyzzeri have also been reported occasionally (Robertson et al., 2014). As seen in ruminants, Cryptosporidium species tend to generally follow an age-related pattern: C. suis is more commonly found in piglets whereas starter pigs and fatteners primarily host C. scrofarum (Petersen et al., 2015).
There is a huge disparity in prevalence rates (0.1% to 100%) reported from all over the world (Robertson et al., 2014). Nonetheless, it is obvious that prevalence and intensity of infection is predominant in younger animals than older ones (Maddox-Hyttel et al., 2006; Petersen et al., 2015). While natural or experimental infections with the pig-adapted species, C. suis and C. scrofarum, are usually asymptomatic and cause mild or no illness (Robertson et al., 2014), experimental infection of piglets with either C. parvum or C. hominis results in watery diarrhea, anorexia, mucosal lesions, and increased mortality (Tzipori et al., 1994; Theodos et al., 1998; Lee et al., 2017).
3. Treatment options in humans and animals: The past and current state of affairs
Immunocompromised patients, neonatal animals, and young children especially malnourished ones are the most vulnerable to cryptosporidiosis, and hence, are the ones in most urgent need for effective therapeutics. Although cryptosporidiosis causes a self-limiting diarrheal illness in immunocompetent humans, patients do face a considerable risk for longer-term sequelae, especially in low-income countries. Additionally, Cryptosporidium infections in adult animals can lead to reduced production and result in economic losses to the livestock and food industry. As such, there is an urgent need for the development of safe, inexpensive, and efficacious drugs to reduce the ever-increasing worldwide burden of this disease. However, despite the widespread occurrence of the parasite, current effective treatment and prophylactic options for human and animal Cryptosporidium infections are virtually non-existent.
3.1. Humans
Several drugs with in vitro and in vivo anti-Cryptosporidium activity have been tested against human cases of cryptosporidiosis in uncontrolled/controlled clinical trials, open label/blinded studies, and case reports. These include macrolides, rifamycin derivatives, letrazuril, paromomycin, nitazoxanide, clofazimine, and other pharmacological agents (Table 1). In addition to drugs having direct anti-parasitic activity, other medications that augment the host immunity or ameliorate the symptoms/pathology of cryptosporidiosis have also been tested for the management of the disease (Table 2). However, unfortunately most of these treatments showed limited efficacy and inconsistent results when tested in the most susceptible target population including immunocompromised individuals and young children.
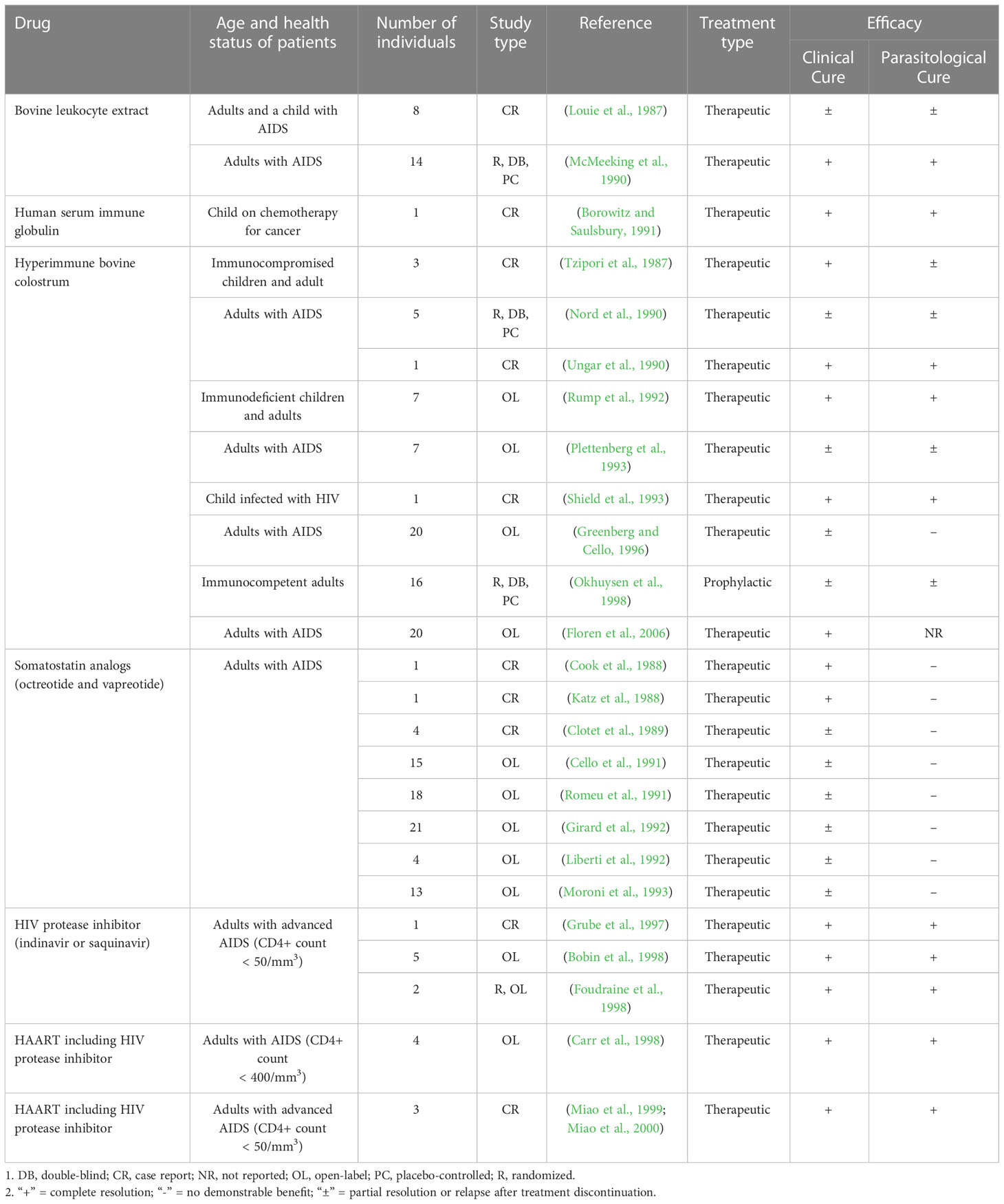
Table 2 Other immunological and supportive treatments tested for efficacy against cryptosporidiosis in human patients.
3.1.1. Nitazoxanide
Thus far, nitazoxanide is the only drug approved by the United States Food and Drug Administration (FDA) for the treatment of cryptosporidiosis in immunocompetent human patients (Checkley et al., 2015). Nitazoxanide is a member of the thiazole class of drugs that was initially developed as a veterinary anthelmintic but was later reported to have broad-spectrum activity against parasites, viruses, and bacteria. This drug acts by inhibiting the pyruvate:ferredoxin/flavodoxin oxidoreductase (PFOR), an enzyme essential for the anaerobic energy metabolism of various microorganisms (Hoffman et al., 2007). However, the exact mechanism of action against Cryptosporidium remains questionable since these parasites encode a unique PFOR with a fused C-terminal cytochrome P450 domain (Rotte et al., 2001). Interestingly, nitazoxanide was shown to inhibit the growth of C. parvum by more than 90% at a concentration of 10 µg/ml (32 µM) in cell culture but was ineffective in the anti-IFN-γ-conditioned SCID mouse model of cryptosporidiosis even at high doses (Theodos et al., 1998). Furthermore, nitazoxanide has also been found to be ineffective in other immunodeficient or immunocompromised animal models of cryptosporidiosis, questioning the true efficacy of the drug (Lee et al., 2017; Jumani et al., 2018).
Various randomized placebo-controlled studies have found nitazoxanide to be helpful in treating cryptosporidiosis in adults and children without HIV resulting in reduced duration of both diarrhea and oocyst shedding (Rossignol et al., 2001; Amadi et al., 2002; Rossignol et al., 2006; Hussien et al., 2013; Abaza et al., 2016). Studies conducted in Egyptian immunocompetent adults and children demonstrated significantly higher clinical and parasitological cure rates compared with the placebo-treated groups (Rossignol et al., 2001; Rossignol et al., 2006). In a randomized controlled trial involving malnourished children in Zambia, nitazoxanide treatment for 3 days yielded a partial but significantly better cure than placebo (Amadi et al., 2002). Recent controlled trials have also reported complete clinical and parasitological recovery in most immunocompetent children with cryptosporidiosis (Hussien et al., 2013; Abaza et al., 2016).
However, a meta-analysis of seven randomized controlled trials involving 169 participants with cryptosporidiosis confirmed the absence of obvious evidence of efficacy of nitazoxanide in HIV-seropositive patients (Abubakar et al., 2007). A course of nitazoxanide does not appear to improve the resolution of diarrhea and parasitological outcome in HIV-infected and immunocompromised patients (Doumbo et al., 1997; Rossignol et al., 1998; Amadi et al., 2002; Zulu et al., 2005; Amadi et al., 2009; Abaza et al., 2016). In the first randomized controlled trial of this drug in adult HIV patients with cryptosporidiosis, better overall parasite clearance rates were seen in the treated group compared with the placebo one, but significant differences were only seen in those with CD4+ T-cell counts above 50/mm3 (Rossignol et al., 1998). Other randomized placebo-controlled trials conducted by Amadi and others in HIV-positive children in Zambia have also documented no beneficial effect of nitazoxanide over placebo in terms of clinical and parasitological cure rates or mortality (Amadi et al., 2002; Amadi et al., 2009). Moreover, only moderate efficacy was achieved in a study of cryptosporidiosis in immunocompromised children even after prolonged nitazoxanide treatment of up to 28 days (Abaza et al., 2016). While prolonged therapy with higher doses of the drug is somewhat effective in treating cryptosporidiosis in patients with compromised immunity, normal prescribed doses and short-term duration of therapy are inadequate for preventing recurrence of disease symptoms after treatment discontinuation (Abraham et al., 2008; Krause et al., 2012; Ali et al., 2014; Bhadauria et al., 2015; Demonchy et al., 2021). Therefore, it is evident that nitazoxanide therapy is clearly futile in treating cryptosporidiosis in advanced AIDS patients and other severely immunocompromised patients.
Lack of efficacy in immunocompromised animal models and humans suggests that a healthy host immune system is essential to the effectiveness of nitazoxanide. Nitazoxanide treatment has been recently shown to result in broad amplification of the host cell innate immune response to viral infections, including an increase in interferon activities (Jasenosky et al., 2019). If an immune defect renders the host incapable of generating an interferon-γ-dependent response, nitazoxanide would be expected to be ineffective in such immunocompromised hosts (Theodos et al., 1998; Jumani et al., 2018), given the importance of these innate responses in controlling Cryptosporidium at its initial stages of infection (McDonald et al., 2013). Similarly, lack of curative effect of nitazoxanide in advanced AIDS patients (with low CD4+ T-cell counts) suffering from chronic cryptosporidiosis can be explained by the fact that adaptive immunity plays a crucial role in clearing the parasites completely from the infected host (Mead, 2014). Thus, the efficacy of nitazoxanide seems to be closely related to both the innate and adaptive immune status of the host.
3.1.2. Paromomycin
Another well studied drug, a poorly absorbed aminoglycoside paromomycin, has been investigated against cryptosporidiosis in three published controlled trials (White et al., 1994; Hewitt et al., 2000; Hussien et al., 2013), but results have been highly divergent and mostly discouraging. Paromomycin, like other aminoglycoside antibiotics, inhibits protein synthesis by binding to the 30S ribosomal subunit and shows broad spectrum activity against bacteria and some protozoa (Lin et al., 2018). Several uncontrolled trials and case studies in AIDS patients suffering from cryptosporidiosis have reported favorable clinical outcomes after paromomycin treatment (Gathe et al., 1990; Clezy et al., 1991; Armitage et al., 1992; Danziger et al., 1993; Fichtenbaum et al., 1993; Wallace et al., 1993; Bissuel et al., 1994; Scaglia et al., 1994; Hashmey et al., 1997). However, the patient responses in most cases were short-lived and continuous maintenance therapy was required to prevent frequent relapses after treatment discontinuation suggesting that complete parasitological cure was not achieved in these cases. Children suffering from cryptosporidiosis have been reported to respond favorably after treatment with paromomycin, although the overall clinical and parasitological response is reduced as compared to nitazoxanide or azithromycin (Trad et al., 2003; Vandenberg et al., 2012; Hussien et al., 2013).
3.1.3. Macrolides
Macrolides are a class of antibiotics that disrupt bacterial protein synthesis by binding to the 50S subunit of the ribosome (Lin et al., 2018). Among the macrolides tested for efficacy against human cryptosporidiosis, azithromycin remains the most studied. In a multi-center, placebo-controlled, double-blind study, preliminary data analysis revealed no significant improvement in clinical symptoms and oocyst numbers in azithromycin-treated AIDS patients with cryptosporidiosis (Soave et al., 1993). Interestingly, however, a statistically significant decrease in cryptosporidial oocyst shedding was reported in patients with appropriate azithromycin serum concentrations (Soave et al., 1993). By contrast, azithromycin was found to have no therapeutic or prophylactic efficacy in the management of cryptosporidial diarrhea in AIDS patients (Blanshard et al., 1997; Holmberg et al., 1998). While short-term azithromycin treatment for cryptosporidiosis was unable to achieve total parasitological clearance and prevent relapses in AIDS patients, long-term and low dose maintenance therapy was associated with noticeable clinical and parasitological benefits (Dionisio et al., 1998; Kadappu et al., 2002). Nevertheless, azithromycin seems to be more effective in treating children with cryptosporidiosis. Prompt clinical improvement and high parasite clearance rates have been reported after azithromycin therapy in both immunocompetent and immunocompromised children (Vargas et al., 1993; Hicks et al., 1996; Allam and Shehab, 2002; Trad et al., 2003).
Clarithromycin has been tested in humans with AIDS for prophylactic effectiveness against cryptosporidiosis. But studies have reported conflicting results with some indicating a highly protective effect against the development of cryptosporidiosis (Jordan, 1996; Holmberg et al., 1998) while others concluding that the drug is not useful in preventing cryptosporidiosis in this patient population (Fichtenbaum et al., 2000). Similarly, spiramycin has also shown inconsistent results in treating cryptosporidiosis in controlled and uncontrolled trials involving infants (Saez-Llorens et al., 1989; Wittenberg et al., 1989) and adult patients (Woolf et al., 1987; Moskovitz et al., 1988) with reports of acute intestinal injury in some patients receiving high doses of the drug (Weikel et al., 1991). Another macrolide, roxithromycin has proven effective in uncontrolled studies of AIDS patients with cryptosporidial enteritis. However, complete parasite clearance was only achieved in half of the treated patients and the results were not compared with a placebo-treated control group (Sprinz et al., 1998; Uip et al., 1998).
3.1.4. Rifamycin derivatives
Rifamycins are a group of drugs that are highly active against mycobacterial infections. Members of this antibiotic class inhibit RNA synthesis by selective binding of the bacterial DNA-dependent RNA polymerase (Hartmann et al., 1967). Several uncontrolled studies have evaluated rifamycin derivatives, namely rifabutin and rifaximin, for prophylactic and therapeutic efficacy respectively, against cryptosporidiosis in HIV-infected humans. Rifabutin has been found to be highly effective in preventing the development of cryptosporidiosis in AIDS patients receiving chemoprophylaxis for Mycobacterium avium complex infection (Holmberg et al., 1998; Fichtenbaum et al., 2000). Similarly, some studies have demonstrated a significant clinical and parasitological benefit of rifaximin, a poorly absorbed rifamycin, in the treatment of cryptosporidiosis in solid organ transplant recipient patients (Burdese et al., 2005) and a small number of HIV-infected adults and children with CD4+ T-cell counts ranging from <50 cells/mm3 to >200 cells/mm3 (Amenta et al., 1999; Gathe et al., 2008). Thus, these results warrant the testing of these drugs in larger randomized controlled clinical trials for confirmation of anti-Cryptosporidium efficacy.
3.1.5. Benzene acetonitrile derivatives
Diclazuril and letrazuril have been both shown to be active against Eimeria species, parasites closely related to Cryptosporidium, although the exact mode of action of these drugs is currently unknown. Yet, diclazuril failed to show any obvious effect in severe cryptosporidiosis in adults with HIV infection (Connolly et al., 1990). In another study, a single patient showed both clinical and parasitological response to diclazuril treatment but the infection was less severe and the patient also received antiretroviral therapy during and after the treatment course (Menichetti et al., 1991). Additionally, letrazuril, the p-fluor analog of diclazuril, shows only partial efficacy against advanced AIDS-related cryptosporidial diarrhea (Harris et al., 1994; Loeb et al., 1995; Blanshard et al., 1997) and treated patients develop temporary drug-related side-effects including abnormal liver function tests and skin rashes that tend to resolve after treatment discontinuation (Murdoch et al., 1993; Harris et al., 1994; Loeb et al., 1995).
3.1.6. Miscellaneous antimicrobials
3.1.6.1. Clofazimine
Clofazimine, an FDA-approved antimycobacterial drug primarily used to treat leprosy, was found to be effective against both C. parvum and C. hominis in vitro and showed promising efficacy against C. parvum in a mouse model (Love et al., 2017). Interestingly, the mechanism of action of clofazimine as an anti-mycobacterial drug is not well understood. A recent randomized controlled clinical trial tested the efficacy of the drug in enrolled patients with advanced HIV infection and Cryptosporidium-associated diarrhea. The findings of the study, however, failed to demonstrate any effectiveness of clofazimine in reducing fecal parasite shedding and stool frequency in immunocompromised patients (Iroh Tam et al., 2021). Moreover, unexpected adverse events were higher in the clofazimine-treated patients as compared to the placebo control group.
3.1.6.2. Miltefosine
Like clofazimine, miltefosine an anti-Leishmania drug, has also shown promising in vitro efficacy against C. parvum as per anecdotal observations but its specific mode of action is not entirely known. This drug, however, showed modest clinical improvement without evidence of oocyst clearance in treated HIV-infected malnourished individuals (Sinkala et al., 2011). Furthermore, adverse events including hepatic dysfunction and renal failure were observed in some patients, leading to premature termination of the phase-1–phase-2 trial.
3.1.6.3. Albendazole
Albendazole is a broad-spectrum anthelmintic drug that is known to bind to β-tubulin and inhibit microtubule assembly in helminth worms (Lacey, 1988). This benzimidazole derivative was shown to have a significant effect on the duration of diarrhea in a randomized, controlled trial in HIV-seropositive patients with persistent diarrhea (Kelly et al., 1996). Later, another study assessed the effect of albendazole on C. parvum and other intracellular protozoa including Isospora and microsporidia in HIV-positive patients and found the drug to exhibit complete C. parvum clearance when used at doses higher than the normal prescribed dose (Zulu et al., 2002). However, the number of HIV patients with cryptosporidiosis in this study was very small as compared to patients infected with other protozoa, and the results cannot be considered credible due to the lack of an untreated control group for comparison. Nevertheless, it does seem that albendazole has some activity against Cryptosporidium at high doses (Fayer and Fetterer, 1995), but, to the best of our knowledge, no other study has evaluated albendazole for anti-Cryptosporidium efficacy in immunocompromised humans.
3.1.7. Other treatments
Although most studies on the treatment of cryptosporidiosis have been carried out by repurposing the use of antibacterial drugs, some studies have tested other treatments with alternate modes of action such as immune cell extracts, immunoglobulins, hyperimmune colostrum, somatostatin analogs, and highly active antiretroviral therapy (HAART) (Table 2).
3.1.7.1. Immunotherapy
Passive immunotherapy by oral administration of immunoglobulins derived from bovine colostrum or human serum was shown to be effective in Cryptosporidium-infected immunosuppressed humans in several open-label uncontrolled studies and case reports (Tzipori et al., 1987; Ungar et al., 1990; Borowitz and Saulsbury, 1991; Rump et al., 1992; Shield et al., 1993; Floren et al., 2006), but results obtained from randomized double-blind controlled studies have been disappointing (Nord et al., 1990; Okhuysen et al., 1998). Similarly, treatment of AIDS-associated cryptosporidial diarrhea by oral administration of cellular extracts prepared from lymphocytes obtained from immunized calves produced mixed results (Louie et al., 1987; McMeeking et al., 1990).
3.1.7.2. Somatostatin analogs
Somatostatin analogs including octreotide and vapreotide have been reported to improve secretory diarrhea by inhibiting the motility and secretions of the gastro-intestinal tract. Patients with AIDS-related chronic diarrhea, especially those without specific pathogens, may benefit from treatment with this class of drugs. However, these agents have mostly been ineffective or partially effective in reducing the fecal output in Cryptosporidium-associated diarrhea in HIV-infected patients and show no parasitological cure (Cook et al., 1988; Katz et al., 1988; Clotet et al., 1989; Cello et al., 1991; Romeu et al., 1991; Girard et al., 1992; Liberti et al., 1992; Moroni et al., 1993).
3.1.7.3. Highly active antiretroviral therapy
Cryptosporidiosis is typically a self-limiting illness in immunocompetent individuals, and therefore, immune reconstitution by restoring CD4+ T-cell levels in particular, is an essential part of the disease management strategy in the immunocompromised (Flanigan et al., 1992). HIV-infected patients with CD4+ T-cell counts below 200/mm3 tend to be susceptible to a higher frequency of cryptosporidial infections highlighting the relationship of these opportunistic pathogens with the immune status of an individual (de Oliveira-Silva et al., 2007; Tuli et al., 2008; Adamu et al., 2013; Nsagha et al., 2016; Cerveja et al., 2017; Amoo et al., 2018). The use of HAART in HIV-infected patients has significantly reduced the global frequency and severity of cryptosporidiosis in this patient population (Le Moing et al., 1998; Call et al., 2000; Conti et al., 2000; Ives et al., 2001; Babiker et al., 2002; Bachur et al., 2008; Missaye et al., 2013). HAART re-establishes CD4+ T-cell counts and inhibits viral replication using a combination of nucleoside reverse transcriptase inhibitors (NRTIs), non-nucleoside reverse transcriptase inhibitors (NNRTIs), and HIV protease inhibitors. However, chronic diarrhea at initiation of HAART in HIV-positive patients has been associated with increased early mortality, emphasizing the need for early anti-retroviral therapy before the onset of diarrhea (Dillingham et al., 2009). Most studies involving HAART for the treatment of cryptosporidiosis have used HIV protease inhibitors either individually or in combination with other antiretrovirals to successfully treat the disease with major clinical and parasitological benefits (Grube et al., 1997; Bobin et al., 1998; Carr et al., 1998; Foudraine et al., 1998; Miao et al., 1999; Miao et al., 2000). Such a therapy may exert its pharmacological effect against AIDS-associated cryptosporidiosis both by restoration of circulating CD4+ T-cell counts and direct inhibition of Cryptosporidium proteases (Mele et al., 2003; Pantenburg et al., 2009). But individuals with other causes of weakened immunity, including primary immunodeficiency, immunosuppressive therapy in organ transplant recipients, and chemotherapy in cancer patients, remain at high risk of severe cryptosporidiosis.
3.1.8. Combination therapy
Several combination therapies involving the use of either nitazoxanide or paromomycin in conjunction with macrolides, rifamycin derivatives, or HAART have shown promising efficacy for cryptosporidiosis in small uncontrolled trials and case studies with both clinical improvement and parasite elimination in a range of affected immunocompromised individuals (Table 3). However, these results need to be replicated in large, controlled trials before any definite conclusions can be drawn regarding the efficacy of such combinations. Huang and colleagues conducted a randomized placebo-controlled trial to investigate the therapeutic effects of acetylated spiramycin and garlicin on Cryptosporidium infection in institutionalized drug users. Although the combination treatment achieved high parasitological cure rates, this study was carried out in asymptomatic Cryptosporidium carriers without ascertaining the HIV/immune status of the enrolled individuals, and therefore has limited clinical significance (Huang et al., 2015).
Certain clinical case reports have documented favorable clinical and parasitological outcomes in HIV-infected and organ transplant recipient patients diagnosed with extra-intestinal and intestinal cryptosporidiosis, after antimicrobial combination therapy with azithromycin and paromomycin (Palmieri et al., 2005; Meamar et al., 2006; Denkinger et al., 2008). In a small open-label, uncontrolled study, patients with AIDS (<100 CD4+ T-cells/mm3) and chronic cryptosporidiosis, showed a marked improvement in stool frequency and a significant decrease in fecal excretion of Cryptosporidium oocysts in response to azithromycin/paromomycin combination therapy (Smith et al., 1998). However, follow-up study after the completion of treatment revealed the persistence of chronic, mild diarrhea in some patients.
Complete resolution of diarrhea as well as elimination of the parasite has been reported in immunosuppressed children and adults suffering from cryptosporidiosis after dual therapy with azithromycin and nitazoxanide (Legrand et al., 2011; Bakliwal et al., 2021; Dupuy et al., 2021). Additionally, triple therapy involving azithromycin, nitazoxanide, and paromomycin or rifaximin led to complete clinical and parasitological cure with no relapse in renal transplant patients (Hong et al., 2007; Tomczak et al., 2022). Another study successfully treated cryptosporidiosis in a pediatric renal transplant patient using a triple therapy consisting of spiramycin, nitazoxanide, and paromomycin (Acikgoz et al., 2012).
Moreover, quite a few small uncontrolled studies suggest that a combination of antimicrobials and HAART (especially with protease inhibitors) dramatically accelerates the clinical response in AIDS patients suffering from cryptosporidiosis (Maggi et al., 2000; Schmidt et al., 2001; Moling et al., 2005). But data from randomized controlled trials is required to support these results given the self-limiting nature of the disease. Nevertheless, increasing evidence has demonstrated that combination therapy achieves better clinical and microbiological resolution rates than monotherapy for the treatment of cryptosporidiosis in immunocompromised patients (Maggi et al., 2000; Bhadauria et al., 2015; Lanternier et al., 2017; Bakliwal et al., 2021; Tomczak et al., 2022).
3.2. Animals
Numerous antimicrobial compounds have been screened and evaluated for efficacy against naturally acquired and experimentally induced cryptosporidiosis in animals (Table 4), albeit with limited success. Most of the tested drugs exhibit only partial prophylactic and therapeutic efficacy in reducing oocyst excretion and disease severity in affected animals. Thus far, no effective currently licensed therapeutics are available in the United States for Cryptosporidium infections in animals (Santin, 2020; Zahedi and Ryan, 2020). Alternatively, several supportive and immunological therapies have also been tested for the management of cryptosporidiosis in livestock (Table 5), but none have shown promise in changing the course of the disease.
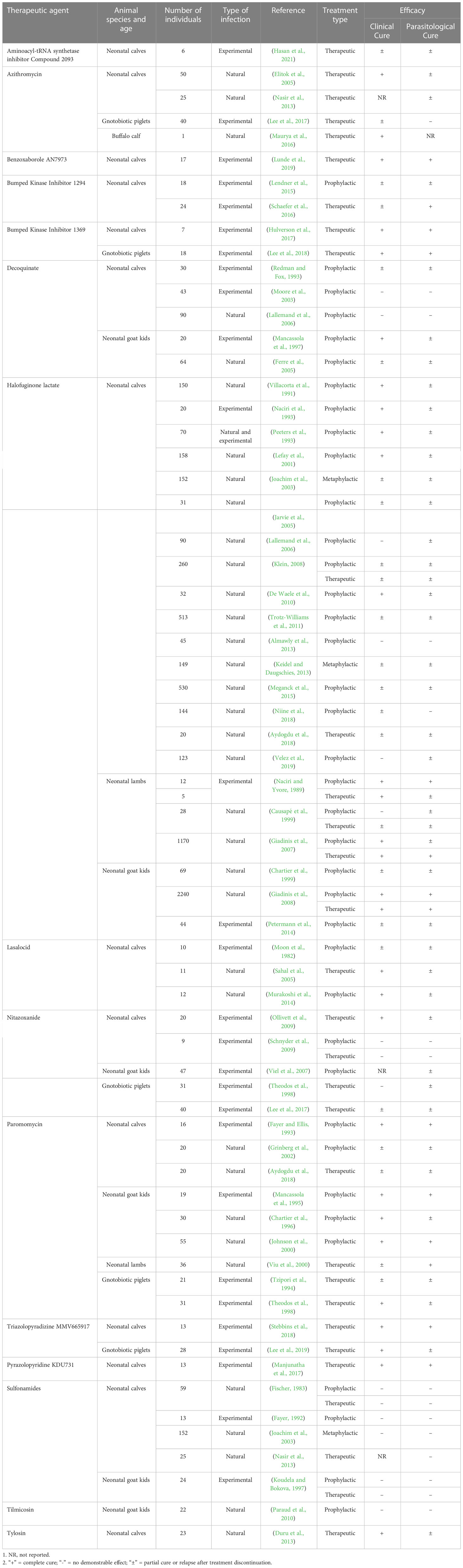
Table 4 Anti-cryptosporidial efficacies of various antimicrobial and novel treatments in farm animals and natural host animal models.
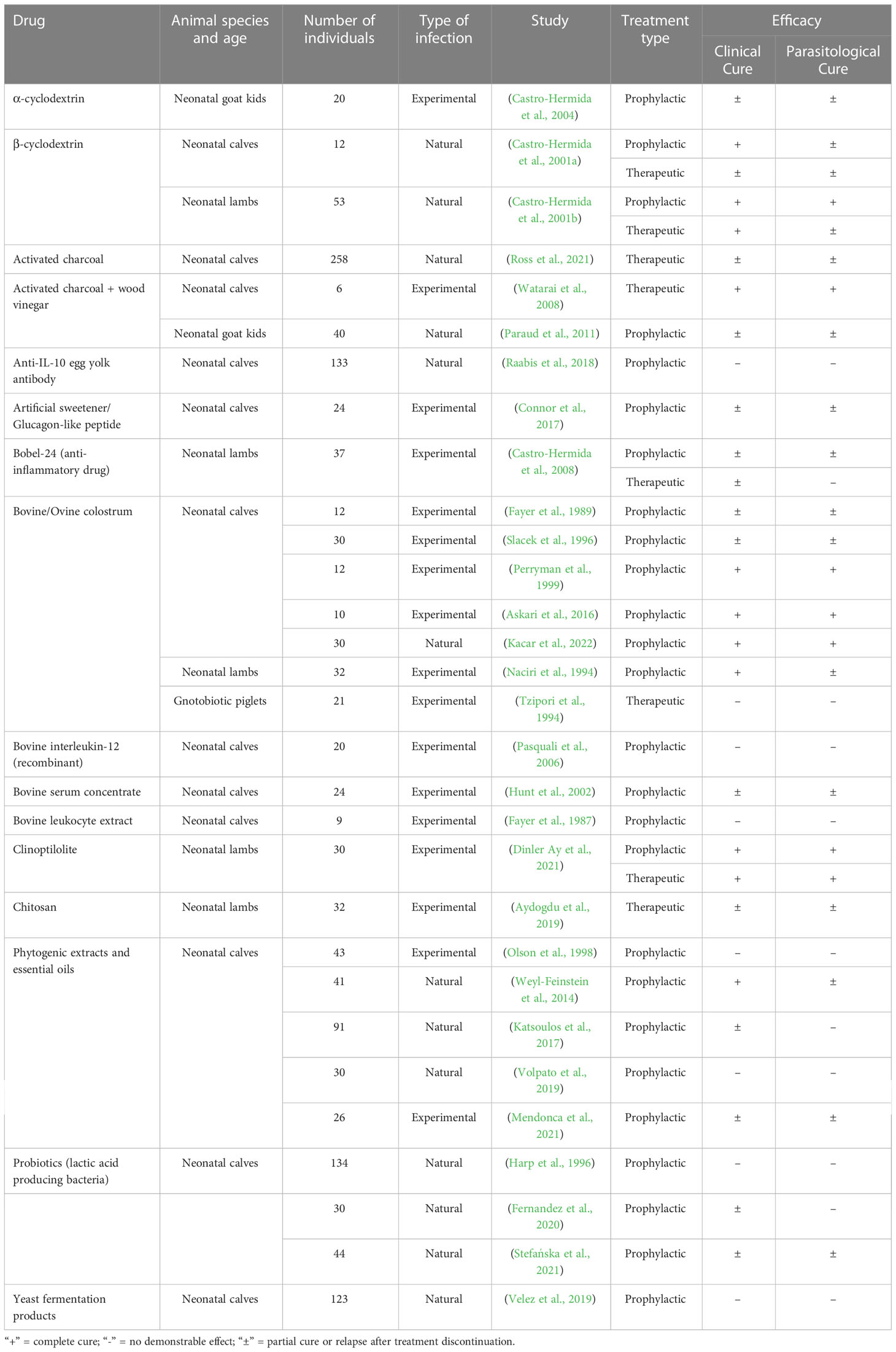
Table 5 Alternate treatments tested for efficacy against cryptosporidiosis in farm animals and natural host animal models.
3.2.1. Anticoccidials
3.2.1.1. Halofuginone lactate
Halofuginone lactate, a prolyl-tRNA synthetase inhibitor, is a synthetic quinazolinone coccidiostat primarily used in veterinary medicine for the prevention and treatment of Eimeria infections in avian species. This medication is licensed for veterinary use in cattle against cryptosporidiosis in several European countries as well as Canada, although it is not labeled for use in the United States. Halofuginone lactate has a narrow safety index and is contraindicated in dehydrated animals suffering from diarrhea: clinical signs typical of cryptosporidiosis in neonatal animals. Hence, this drug is not suitable for therapeutic purposes and is generally used as a prophylactic to prevent cryptosporidial diarrhea in newborn farm animals. Recently, Brainard and others conducted a systematic review of literature and used meta-analysis to evaluate key outcomes such as oocyst shedding, diarrhea, mortality, and weight gain for the treatment of calf cryptosporidiosis with halofuginone lactate. The authors concluded that prophylactic halofuginone treatment was associated with significantly lower incidence of oocyst shedding, diarrhea burden, and mortality especially when the treatment was started early in life (Brainard et al., 2021). Furthermore, Giadinis et al. conducted two extensive field trials in Greece and found the drug to be effective in preventing and treating cryptosporidiosis, and reducing deaths associated with the disease in neonatal lambs and goat kids (Giadinis et al., 2007; Giadinis et al., 2008).
A number of early reports suggested that halofuginone showed effectiveness in protecting young ruminants from severe cryptosporidiosis, but relapses occurred after treatment discontinuation in calves (Villacorta et al., 1991; Naciri et al., 1993; Peeters et al., 1993; Lefay et al., 2001), lambs (Naciri and Yvore, 1989; Causapé et al., 1999), and goat kids (Chartier et al., 1999), questioning the effectiveness of the preventative treatment. Moreover, although halofuginone lactate treatment reduces oocyst shedding in infected animals, it fails to provide complete protection and cure, implying that treatment along with good animal husbandry practices including individual housing, proper hygiene measures, and suitable disinfection are required to prevent environmental contamination and disease transmission among animals on farms (Joachim et al., 2003; Jarvie et al., 2005; Klein, 2008; De Waele et al., 2010; Trotz-Williams et al., 2011; Keidel and Daugschies, 2013). Likewise, a few studies also showed some efficacy in reducing excretion of Cryptosporidium oocysts in treated animals as compared to untreated controls, but no significant effect on the prevalence of diarrhea or body weight gain was noted (Causapé et al., 1999; Lallemand et al., 2006; Trotz-Williams et al., 2011; Almawly et al., 2013). Interestingly, preventive treatment with halofuginone lactate was also found to be associated with reduced weight gain in calves (Niine et al., 2018; Velez et al., 2019). Thus, the preventive and therapeutic effectiveness of halofuginone lactate in animals remains controversial.
3.2.1.2. Decoquinate
Decoquinate is a quinolone coccidiostat most used for controlling coccidiosis in ruminants and poultry. This drug inhibits the mitochondrial respiration by blocking electron transport in Eimeria parasites (Wang, 1976). Decoquinate produces limited-to-no clinical and parasitological response when used preventatively before the development of signs and symptoms of cryptosporidiosis in experimentally or naturally infected calves (Redman and Fox, 1993; Moore et al., 2003; Lallemand et al., 2006). However, it significantly reduces oocyst shedding and severity of cryptosporidiosis in neonatal kids, but without complete eradication of infection (Mancassola et al., 1997; Ferre et al., 2005).
3.2.1.3. Lasalocid
Lasalocid is an ionophore antibiotic and a coccidiostat that is commonly used as a feed additive for promoting growth and preventing coccidiosis in ruminants. This drug has been used as a prophylactic or therapeutic to treat Cryptosporidium infections in calves. Based on anecdotal reports, short-term dosing (3-4 days) of lasalocid (6-15 mg/kg/day) was effective in treating severe cryptosporidiosis in calves (Gobel, 1987a; Gobel, 1987b; Sahal et al., 2005). However, mortality and serious side effects resulting from lasalocid toxicosis have been described in animals when long-term therapy or a dose higher than the label dose was used as a preventative for cryptosporidiosis (Moon et al., 1982; Benson et al., 1998). More recently however, Murakoshi and others demonstrated a highly beneficial effect of lasalocid, without any side effects, when used at a lower dose (3 mg/kg/day) to prevent calf cryptosporidiosis. But the treatment was not found to be protective after the 7-day dosing period (Murakoshi et al., 2014).
3.2.1.4. Sulfonamides
Sulfonamides are broadly active antimicrobial agents that inhibit dihydropteroate synthase, an enzyme involved in folate synthesis (Henry, 1943). They have been widely used in veterinary medicine to prevent coccidiosis and treat bacterial infections in animals and poultry. However, prophylactic or therapeutic treatment of natural or experimental cryptosporidiosis with a variety of sulfonamides and potentiated sulfonamides including sulfadimidine, sulfadimethoxine, and cotrimoxazole (trimethoprim in combination with sulfamethoxazole) has failed miserably in calves (Moon et al., 1982; Fischer, 1983; Fayer, 1992; Joachim et al., 2003; Nasir et al., 2013) and goat kids (Naciri et al., 1984; Koudela and Bokova, 1997).
3.2.2. Paromomycin
In addition to humans, paromomycin has also been extensively tested for anti-Cryptosporidium efficacy in various food animals. However, as has been the case in humans, results have been varied and the treatment failed to achieve complete parasitological cure in most studies. Prophylactic administration of paromomycin was found to decrease the duration and severity of diarrhea as well as the duration and intensity of oocyst shedding in calves experimentally infected with C. parvum (Fayer and Ellis, 1993). Similar positive results were reported in a controlled-blind field trial of natural infection in calves, but the treated group started shedding oocysts and developed diarrhea after the treatment withdrawal (Grinberg et al., 2002). However, paromomycin does seem to be more effective in small ruminants. Treatment has been shown to reduce both cryptosporidial oocyst output and severity of clinical signs, when used prophylactically in neonatal goat kids (Mancassola et al., 1995; Chartier et al., 1996; Johnson et al., 2000) and therapeutically in neonatal lambs (Viu et al., 2000). This agent was also proven to be therapeutically effective against moderate cryptosporidiosis but ineffective against severe cryptosporidiosis in infected gnotobiotic piglets (Tzipori et al., 1994; Theodos et al., 1998). However, paromomycin, like many aminoglycosides, is potentially nephrotoxic and detrimental effects on growth have been observed after treatment in young animals (Viu et al., 2000). In addition, the drug is expensive and therefore, its use in agricultural animals is impractical.
3.2.3. Nitazoxanide
Nitazoxanide, the only licensed treatment available in humans, has also been tested for efficacy in animal cryptosporidiosis, although reports on treatment outcomes have been conflicting. While Ollivett and others found this medication to significantly reduce the duration of oocyst shedding and clinical severity in experimentally infected calves as compared to the placebo treated group (Ollivett et al., 2009), another controlled study found no prophylactic or therapeutic effect of nitazoxanide on clinical appearance or oocyst excretion in calves infected with C. parvum (Schnyder et al., 2009). Furthermore, while nitazoxanide reduced oocyst shedding in experimentally challenged newborn goat kids, no reduction in mortality rates or improvement in weight gains were recorded in the treated groups compared with the control group (Viel et al., 2007). Importantly, the authors of this study suggested that the mortalities seen in kid neonates in the nitazoxanide treated groups were caused by severe drug toxicity (Viel et al., 2007). In the gnotobiotic piglet diarrhea model, nitazoxanide demonstrated only partial efficacy at high doses in reducing C. parvum oocyst shedding, induced drug-related diarrhea, and was not as effective as paromomycin (Theodos et al., 1998). In another study performed in the same animal model but infected with C. hominis, nitazoxanide reduced diarrhea and oocyst shedding in only the initial phase of treatment and had no clinical or parasitological effect at the later stages of the disease (Lee et al., 2017).
3.2.4. Macrolides
Macrolides have been evaluated as anti-Cryptosporidium agents in a range of animals. Azithromycin significantly suppressed Cryptosporidium oocyst shedding and resulted in significant clinical improvement and weight gain in naturally infected dairy calves when used as a therapeutic at high doses, but high costs of treatment are a concern (Elitok et al., 2005). Similar reports of azithromycin efficacy against C. parvum infection in calves (Nasir et al., 2013) and a buffalo calf (Maurya et al., 2016) have also been published. Treatment of gnotobiotic neonatal piglets infected with C. hominis alleviated clinical disease only for the first few days and azithromycin treated piglets exhibited no reduction of oocyst excretion compared with untreated animals (Lee et al., 2017). In combination with nitazoxanide, azithromycin led to significant clinical improvement in infected piglets but did not eliminate oocyst excretion after producing a transient initial reduction in oocyst shedding in treated animals (Lee et al., 2017).
Experience with other macrolides has also been mixed. While tilmicosin failed to prevent severe cryptosporidiosis in newborn kids raised on a commercial dairy goat farm (Paraud et al., 2010), tylosin was found to be therapeutically effective in reducing fecal oocyst excretion and clinical signs of disease in naturally infected calves (Duru et al., 2013).
3.2.5. Other treatments
3.2.5.1. Immunotherapy
Passive immunotherapy using bovine colostrum or bovine serum concentrate containing specific antibodies to Cryptosporidium provided only partial protection against cryptosporidiosis by reducing duration of diarrhea and oocyst shedding in experimentally infected calves (Fayer et al., 1989; Slacek et al., 1996; Hunt et al., 2002). However, much better protection from Cryptosporidium infection was noted in some studies after prophylactic oral administration of bovine/ovine colostrum comprising of anti-cryptosporidial antibodies in infected calves and lambs (Naciri et al., 1994; Perryman et al., 1999; Askari et al., 2016; Kacar et al., 2022). Moreover, therapeutic administration of hyperimmune colostrum-immunoglobulin in experimentally infected gnotobiotic piglets reduced oocyst shedding but had little-to-no effect on diarrhea and intestinal mucosal damage caused by the parasite (Tzipori et al., 1994). Similarly, preventive treatment of experimentally induced calf cryptosporidiosis by recombinant bovine interleukin-12 (rBoIL-12) or lymphocyte extracts from immunized calves failed to provide prophylaxis (Fayer et al., 1987; Pasquali et al., 2006).
3.2.5.2. Adsorbents
Oral intestinal adsorbents have been used worldwide as a remedy to treat diarrhea of various causes. A product consisting of activated charcoal and wood vinegar was found to be highly effective in treating experimental C. parvum infection in calves (Watarai et al., 2008) and provided partial protection to newborn kids against natural infection (Paraud et al., 2011). More recently, activated charcoal also showed a partial curative effect on neonatal calf diarrhea caused mainly by C. parvum at a commercial calf-raising farm (Ross et al., 2021). Similarly, another adsorbent clinoptilolite also demonstrated a good prophylactic and therapeutic effect against C. parvum in experimentally infected lambs (Dinler Ay et al., 2021). These adsorbents seem to be effective against cryptosporidial infections probably because of their potential to adsorb and thereby trap parasites and prevent host cell invasion. Although this adsorption principle has been demonstrated in an in vitro adsorption test (Watarai et al., 2008), the same needs to be confirmed in further in vivo studies.
3.2.5.3. Polysaccharides
Cyclodextrins are cyclic oligosaccharides that are commonly used as drug excipients to enhance the solubility, safety, stability, and bioavailability of drugs. After showing some unexpected activity against C. parvum experimental infection in mice, β-cyclodextrin has been tested for both prophylactic as well as therapeutic efficacy against cryptosporidiosis in young ruminants with results showing that the preventive effect is greater than the curative one. While β-cyclodextrin showed partial efficacy in reducing diarrhea and oocyst shedding in naturally infected calves (Castro-Hermida et al., 2001a), this drug reduced mortality and produced an even better clinical and parasitological response in infected lambs under field conditions (Castro-Hermida et al., 2001b). Another drug of this class, α-cyclodextrin was tested for prophylactic effectiveness in experimentally infected neonatal kids and showed some reduction in the intensity of infection and oocyst shedding, but almost half treated kids died probably due to drug-related side effects (Castro-Hermida et al., 2004).
Chitosan, a natural linear polysaccharide has also been investigated for efficacy in C. parvum infected lambs. Therapeutic treatment after the onset of disease improved clinical signs and fecal consistency, and reduced oocyst excretion, but did not eliminate cryptosporidiosis completely in treated lambs (Aydogdu et al., 2019).
Researchers have suggested various modes of action of polysaccharides such as cyclodextrins and chitosan in controlling viral, bacterial, and parasitic infections that involve use of their antimicrobial properties, osmotic properties, and cholesterol-sequestering ability, among others (Aydogdu et al., 2019; Braga, 2019). It is likely that, in the case of Cryptosporidium, these polysaccharides might form a protective film over the intestinal surface due to their adhesive properties, which may act as a physical barrier and prevent cell invasion by parasites. However, to date, the exact mechanism of action of these pharmaceutical agents against Cryptosporidium remains unknown.
3.2.5.4. Natural plant-based products
Various natural products like phytogenic extracts, essential oils, and phytobiotics have been used to treat animal cryptosporidiosis, but with unconvincing results. A randomized controlled study evaluated allicin, a sulfur-containing component of garlic, in experimentally infected neonatal calves and found it to have no effect on the duration of diarrhea or weight gain in treated calves (Olson et al., 1998). Another study conducted in Israel showed that a concentrated pomegranate extract feed supplement partially reduced clinical signs and fecal oocyst counts in natural calf cryptosporidiosis (Weyl-Feinstein et al., 2014). Similarly, experimentally infected calves receiving plant-based isoquinoline alkaloids as feed additive suffered from less intense diarrhea for a shorter period but shed similar number of oocysts daily compared with the control group (Mendonca et al., 2021). Furthermore, administration of essential oils or essential oil-based phytogenic products to newborn calves also failed to produce any preventive effect on parasite shedding in infected calves (Katsoulos et al., 2017; Volpato et al., 2019).
3.2.5.5. Probiotics
A few animal studies suggest some potential for the use of probiotics for prophylactic treatment of cryptosporidiosis, though bacterial mechanisms involved in protection against Cryptosporidium infection are not known. Daily oral administration of lactic acid producing bacteria for 10 consecutive days to C. parvum infected dairy calves had limited effect on clinical signs and no effect on parasite abundance (Harp et al., 1996; Fernandez et al., 2020), although a partial reduction in the severity of diarrhea, prevalence of cryptosporidial infection, and oocyst excretion was noted when probiotics combined with phytobiotics were dispensed to calves under field conditions (Stefańska et al., 2021). Likewise, feeding of yeast fermentation products had no clinical and parasitological benefits in bovine cryptosporidiosis (Velez et al., 2019).
3.2.5.6. Miscellaneous treatments
Apart from drugs that have a direct anti-parasitic effect, other medications that have no known anti-Cryptosporidium activity but act by improving the symptoms of cryptosporidiosis have also been tested in animals. Such drugs might show some reduction of parasite load in young animals probably by relieving the symptoms of disease and allowing natural host immunity to develop and act against the infection. One such anti-inflammatory drug, Bobel-24, was unable to completely prevent or treat experimentally induced C. parvum infection in neonatal lambs but showed some prophylactic efficacy in reducing the duration and intensity of oocyst shedding and the presence of diarrhea (Castro-Hermida et al., 2008). Also, preventive administration of anti-IL-10 egg yolk antibodies for 11 days had no effect on the prevalence of Cryptosporidium infection in calves reared under field conditions (Raabis et al., 2018). In another study, administration of glucagon-like peptide 2 or artificial sweetener therapy before a low-level experimental C. parvum exposure reduced severity of diarrhea, fecal oocyst excretion, and intestinal pathology in neonatal calves (Connor et al., 2017).
4. New potential treatments for humans and animals
So far, no satisfactory prophylactics or therapeutics are available for the prevention or treatment of severe cryptosporidiosis in humans and animals. The limited progress made in this field can be directly attributed to the limited genetic tractability of Cryptosporidium, lack of conventional apicomplexan targets, as well as the unique intracellular but extracytoplasmic location within the host cells. Furthermore, the lack of reliable cell culture platforms and limited availability of technical tools to study the parasite in biological systems, lead to an inadequate knowledge about the host-parasite interactions (Checkley et al., 2015; Innes et al., 2020). Nevertheless, breakthrough genetic modification of the parasite that has been made recently has advanced Cryptosporidium research, although the approach is complicated compared to methods developed for other apicomplexan parasites, as it requires the passage of the transgenic parasites in laboratory animals (Vinayak et al., 2015). Importantly, some significant progress has been made in generating genetically modified C. parvum strains in vitro, using mouse-derived intestinal organoid cultures grown in a modified air-liquid-interface system, that enables the completion of the life cycle and produces viable oocysts that are infectious in cell culture and immunocompromised mice (Wilke et al., 2019; Wilke et al., 2020). Indeed, recent advances in genetic manipulation and culture of Cryptosporidium have resulted in substantial progress in anti-Cryptosporidium drug discovery in recent years, and several compounds of preclinical, lead, and late lead status have emerged from target-based and phenotypic screens and are currently in development (Love and Choy, 2021).
C. parvum infects both humans and cattle as natural hosts, with the human disease closely resembling the one found in neonatal calves (Santín and Trout, 2007). Thus, the use of the neonatal calf infection model is highly recommended for assessment of efficacy of candidate compounds before advancement to human clinical trials and should ensure the safety and efficacy of promising compounds in both humans and livestock. Recently some compounds have shown promising efficacy in treating cryptosporidiosis in natural animal host models including the neonatal calf model without any major safety issues. These include bumped kinase inhibitors (BKIs), pyrazolopyridine-based KDU731, triazolopyradizine MMV665917, benzoxaborole AN7973, and compound 2093 (Table 4).
BKIs inhibit the Cryptosporidium parvum calcium-dependent protein kinase 1 (CpCDPK1), an enzyme that is essential for host cell invasion and does not have any mammalian analogs (Van Voorhis et al., 2021). Recent studies have assessed novel BKIs as a possible cure for cryptosporidiosis. In one study, Lendner et al. evaluated a bumped kinase inhibitor BKI-1294 for efficacy against C. parvum in experimentally infected neonatal calves and concluded that BKI-1294 reduced oocyst shedding but had no effect on diarrhea and dehydration in treated calves (Lendner et al., 2015). In another study, Schaefer and others demonstrated that BKI-1294 significantly improved clinical appearance, diarrhea, and parasitological outcomes but failed to eliminate diarrhea and other clinical symptoms of bovine cryptosporidiosis (Schaefer et al., 2016). Nonetheless, another CpCDPK1 inhibitor, BKI-1369, has emerged as an encouraging lead compound for anti-Cryptosporidium therapy in animals (Van Voorhis et al., 2021). This compound has shown promising efficacy against cryptosporidiosis in both the C. parvum infected neonatal calf, and the C. hominis infected gnotobiotic piglet models (Hulverson et al., 2017; Lee et al., 2018). Unfortunately, these BKIs possess potent human Ether-à-go-go-Related Gene (hERG) inhibitory activity, which is associated with a potentially fatal disorder called long QT syndrome and cardiotoxicity in humans, effectively removing them from the anti-cryptosporidial drug development pipeline for humans (Van Voorhis et al., 2021). Nevertheless, BKI-1369 displayed both efficacy and safety in the neonatal calf model with a 30-fold reduction in total oocyst excretion and, therefore, calls for additional development as an anti-Cryptosporidium therapeutic for cattle (Hulverson et al., 2017).
The pyrazolopyridine derivative KDU731 is another promising anti-cryptosporidial drug candidate that inhibits the enzymatic activity of Cryptosporidium lipid kinase PI(4)K (phosphatidylinositol-4-OH-kinase) and is active against both C. parvum and C. hominis. Oral treatment with KDU731 resulted in significant reduction in oocyst shedding, duration of severe diarrhea, and dehydration without any adverse drug-related effects in neonatal calves experimentally infected with C. parvum (Manjunatha et al., 2017). Intriguingly, KDU731 displayed limited systemic exposure in pharmacokinetic analysis of the drug in C. parvum-infected calves, suggesting that systemic exposure may not be important for therapeutic efficacy.
Recently, a piperazine derivative MMV665917 with an unknown molecular mechanism of action (MMOA), was identified within the open access “Malaria Box” collection of antimalarial compounds and found to have potent in vitro activity against both C. parvum and C. hominis in addition to excellent in vivo anti-Cryptosporidium efficacy in mouse models of acute (IFN-γ KO) and chronic (NSG) cryptosporidiosis (Jumani et al., 2018). This compound was later tested in the neonatal calf model of cryptosporidiosis by Stebbins and colleagues and treatment resulted in rapid resolution and reduced duration of diarrhea, as well as a 94% reduction in total fecal excretion of cryptosporidial oocysts in treated calves compared with the control group (Stebbins et al., 2018). In another study conducted in the gnotobiotic piglet model, MMV665917 was shown to significantly reduce fecal C. hominis oocyst shedding, intestinal lesions, parasite colonization, and severity of diarrhea, compared with untreated control piglets (Lee et al., 2019). Unfortunately, like BKIs, this promising compound shows partial hERG inhibition and is potentially cardiotoxic in humans. However, similarities between the modes of action of BKIs and MMV665917 cannot be drawn based on this finding since hERG inhibition is generally an off-target effect and several compounds with diverse structures and modes of action are known to promiscuously block this channel (Witchel, 2011). Hence, studies to determine the MMOA of MMV665917 are needed to aid further lead optimization efforts to reduce the affinity for hERG binding.
Another compound that has been discovered by phenotypic screening of an antimalarial compound library for Cryptosporidium growth inhibitors is the 6-carboxamide benzoxaborole AN7973 (Lunde et al., 2019). Like MMV665917, AN7973 is active against both C. parvum and C. hominis in cell culture and shows promising efficacy in both the acute and chronic murine models of cryptosporidiosis but does not have a validated target in Cryptosporidium. In the calf clinical model of cryptosporidiosis, AN7973 demonstrated exceptional efficacy in reducing the total parasite fecal excretion by >90% with complete elimination of diarrhea and significant reduction in dehydration in treated calves. Furthermore, the compound possesses favorable safety, stability, and pharmacokinetic characteristics and does not inhibit hERG, a major liability for development of other potential anti-cryptosporidial therapeutics including BKIs and MMV665917 for the human disease (Lunde et al., 2019).
Aminoacyl-tRNA synthetase inhibitors have emerged as promising therapeutic candidates for targeting protein synthesis in Cryptosporidium for the development of anti-cryptosporidial drugs (Jain et al., 2017; Baragana et al., 2019; Buckner et al., 2019; Vinayak et al., 2020). Amongst these compounds, only the potent Cryptosporidium parvum methionyl-tRNA synthetase (CpMetRS) inhibitor, compound 2093, has been tested in the neonatal calf efficacy model of cryptosporidiosis so far (Hasan et al., 2021). In dairy calves experimentally infected with C. parvum, compound 2093 initially reduced total oocyst shedding, diarrhea, and dehydration during the first 4 days of infection but most treated calves relapsed later with a severe progressive disease indicating the likely emergence of drug resistance. Sequencing analysis of parasite DNA extracted from feces of relapsed animals revealed the presence of two mutant parasite strains with different single amino acid substitutions in the CpMetRS genomic locus that potentially conferred MetRS inhibitor resistance. Further genome editing, structural modeling, and enzymatic studies confirmed the spontaneous emergence of drug resistant Cryptosporidium parasites, an alarming finding that demands immediate attention (Hasan et al., 2021).
In addition to the above discussed compounds, several other promising compounds have been unveiled in the last few years and found to be effective in both in vitro and mouse models of cryptosporidial infection. These include but are not limited to benzoxaboroles (Swale et al., 2019; Bellini et al., 2020), 5-aminopyrazole-4-carboxamide-based BKIs (Huang et al., 2019), C. parvum prolyl-tRNA synthetase (CpPRS) inhibitors (Jain et al., 2017), C. parvum lysyl-tRNA synthetase (CpKRS) inhibitors (Baragana et al., 2019), C. parvum phenylalanyl-tRNA synthetase (CpPheRS) inhibitors (Vinayak et al., 2020), piperazine derivatives (Oboh et al., 2021), and glycolytic enzyme inhibitors (Li et al., 2019; Khan et al., 2022b). However, demonstration of efficacy and safety of these compounds in the neonatal calf and gnotobiotic piglet infection models is essential before further advancement to the next stages of development. Nevertheless, availability of multiple potential anti-cryptosporidial compounds is advantageous as a diverse pool of candidate compounds would be needed to account for the high attrition rate that is typical of drug development programs.
5. Vaccine development
Since efficacious anti-cryptosporidial drug options are currently lacking, vaccines could be a relevant option for the control of this disease. However, there are currently no vaccines available to prevent cryptosporidiosis. In any case, humans and animals with healthy immune systems suffer from a mild self-limiting illness and improve without treatment. Therefore, it is unclear whether vaccination is justified in these patient groups. However, vaccination could be particularly useful in preventing cryptosporidiosis in neonatal animals, immunocompromised individuals, and malnourished children living in underdeveloped countries. An effective vaccine should provide rapid long-lasting immunity in vaccinated individuals and minimize disease in livestock with a reduction in shedding of oocysts in feces thereby preventing the spread of the disease. A degree of cross-protective immunity against multiple species and subtypes, albeit less possible, will also be beneficial. The most viable strategy would be to vaccinate cattle, as they are the most significant contributors to contaminated manure globally (Vermeulen et al., 2017). However, it might be difficult to generate protective immunity in neonatal calves rapidly enough through active vaccination (Thomson et al., 2017). Therefore, passive immunization by transfer of anti-Cryptosporidium antibodies from immunized dams to calves through colostrum is a feasible alternate approach to protect them during the early days of life (Innes et al., 2020). Several immunogenic Cryptosporidium antigens, such as gp15, Cp15, and Cp23 that are involved in attachment or penetration of host cells, are being explored as vaccine candidates especially in the form of a multivalent vaccine, incorporating multiple antigens or antigenic epitopes (Mead, 2014; Innes et al., 2020). However, a major obstacle to the development of vaccines is our current limited understanding of the protective immune response against Cryptosporidium infection (Checkley et al., 2015).
6. Concluding remarks
The target patient population for anti-Cryptosporidium drug development is mainly comprised of young children, neonatal calves, and immunocompromised patients. These groups frequently suffer from co-morbidities due to their underdeveloped immunity or immunodeficiency and thus, there is an increased likelihood of such patients receiving other treatments. A highly safe pharmacological profile with a minimal risk of drug-drug interactions is, therefore, a key selection criterion for anti-Cryptosporidium drug candidates. Establishment of in vitro safety profiles of candidate compounds early in the drug development process is also crucial as it can help researchers filter out compounds with potential toxicity issues before they enter the costlier late stages of drug development. In addition to safety-related pharmacological properties, assessment of the absorption, distribution, metabolism, and excretion (ADME) properties of a lead compound is also critical to its initial selection and clinical success. Failure of translation of excellent in vitro efficacy into in vivo clinical potency may be caused by insufficient drug concentrations at the target site. Because cryptosporidiosis is primarily an enteric disease, optimal local gastrointestinal concentrations, in addition to systemic concentrations, might be essential for in vivo anti-Cryptosporidium efficacy of the compounds (Hulverson et al., 2017; Manjunatha et al., 2017; Stebbins et al., 2018; Lunde et al., 2019).
Modern drug-discovery projects utilize either a phenotype-based or target-based screening approach to identify lead candidate compounds for further development. Cryptosporidium drug discovery programs in the recent past have mostly used phenotypic screening methods to successfully discover or repurpose compounds with in vitro and in vivo activity against the parasite (Love et al., 2017; Jumani et al., 2018; Lunde et al., 2019; Li et al., 2020; Khan et al., 2022a). However, such an approach invariably results in the identification of candidate compounds that are difficult to optimize as their MMOA and structure-activity relationships (SAR) are generally unknown. This inflexibility typically leads to higher failure rates once a roadblock is reached in the drug development process. As such, molecular target identification and validation by various genetic and molecular “target deconvolution” methodologies are essential for hits identified from phenotype-based screens (Swinney and Anthony, 2011).
Another approach to anti-cryptosporidial drug discovery is to target biochemical pathways that are unique to the parasite and at the same time, essential for its survival, infection, or multiplication within the host. This strategy has also been used for anti-Cryptosporidium drug discovery, albeit less commonly than the phenotypic one. In the last few years, several enzymes have been identified as potential drug targets, including calcium-dependent protein kinases (Van Voorhis et al., 2021), aminoacyl-tRNA synthetases (Jain et al., 2017; Baragana et al., 2019; Buckner et al., 2019; Vinayak et al., 2020), lipid kinase PI(4)K (Manjunatha et al., 2017), and glycolytic enzymes (Witola et al., 2017; Eltahan et al., 2018; Zhang et al., 2018; Eltahan et al., 2019; Li et al., 2019; Velez et al., 2021; Khan et al., 2022b), among others. The advantage with this approach is that discovery of drug candidates with known MMOA and clearer SAR will create better opportunities for structure-based drug optimization. Indeed, while phenotypic screening has historically had more success in identifying first-in-class drugs, target-based screening has produced more best-in-class drugs (Swinney and Anthony, 2011). However, both approaches need to go hand in hand to identify safe and efficacious anti-Cryptosporidium lead compounds for further development.
Finally, the field will need to leverage the advantages of combination therapy for animal and human cryptosporidiosis to 1) increase the efficacy of treatment, 2) reduce the chances of host toxicity, and 3) prevent the inevitable rise of drug resistance in the future. As pointed out earlier in this review (Table 3), several drug combinations tested in the past have yielded superior results than monotherapy for treating Cryptosporidium infections in humans, though the number of studies evaluating drug combinations in animals is too small to draw a similar conclusion. Besides, given the success of combination therapies for other related apicomplexan diseases such as malaria, babesiosis, and toxoplasmosis, and the alarming acquisition of spontaneous drug resistant mutations during anti-cryptosporidial therapy by parasites in a recent study, more focus should be put on testing drug combinations against cryptosporidiosis in at-risk individuals.
Author contributions
SK: conceptualization, literature review, and manuscript writing (original draft and revision). WW: conceptualization, supervision, manuscript writing (proof-reading, corrections, editing, and revision). All authors contributed to the article and approved the submitted version.
Funding
The work to compile this review and for open access publications was funded by University of Illinois Urbana-Champaign indirect cost recovery funds to WW.
Conflict of interest
The authors declare that the research was conducted in the absence of any commercial or financial relationships that could be construed as a potential conflict of interest.
Publisher’s note
All claims expressed in this article are solely those of the authors and do not necessarily represent those of their affiliated organizations, or those of the publisher, the editors and the reviewers. Any product that may be evaluated in this article, or claim that may be made by its manufacturer, is not guaranteed or endorsed by the publisher.
References
Abaza, B. E., Hamza, R. S., Farag, T. I., Abdel-Hamid, M. A., Moustafa, R. A. (2016). Assessing the efficacy of nitazoxanide in treatment of cryptosporidiosis using pcr examination. J. Egypt Soc. Parasitol. 46 (3), 683–692.
Abraham, D. R., Rabie, H., Cotton, M. F. (2008). Nitazoxanide for severe cryptosporidial diarrhea in human immunodeficiency virus infected children. Pediatr. Infect. Dis. J. 27 (11), 1040–1041. doi: 10.1097/inf.0b013e318186257b
Abubakar, I., Aliyu, S. H., Arumugam, C., Hunter, P. R., Usman, N. K. (2007). Prevention and treatment of cryptosporidiosis in immunocompromised patients. Cochrane Database Syst. Rev. 1), CD004932. doi: 10.1002/14651858.CD004932.pub2
Acikgoz, Y., Ozkaya, O., Bek, K., Genc, G., Sensoy, S. G., Hokelek, M. (2012). Cryptosporidiosis: a rare and severe infection in a pediatric renal transplant recipient. Pediatr. Transplant. 16 (4), E115–E119. doi: 10.1111/j.1399-3046.2011.01473.x
Adamu, H., Wegayehu, T., Petros, B. (2013). High prevalence of diarrhoegenic intestinal parasite infections among non-ART HIV patients in fitche hospital, Ethiopia. PloS One 8 (8), e72634. doi: 10.1371/journal.pone.0072634
Ali, S., Mumar, S., Kalam, K., Raja, K., Baqi, S. (2014). Prevalence, clinical presentation and treatment outcome of cryptosporidiosis in immunocompetent adult patients presenting with acute diarrhoea. J. Pak Med. Assoc. 64 (6), 613–618.
Allam, A. F., Shehab, A. Y. (2002). Efficacy of azithromycin, praziquantel and mirazid in treatment of cryptosporidiosis in school children. J. Egypt Soc. Parasitol. 32 (3), 969–978.
Almawly, J., Prattley, D., French, N. P., Lopez-Villalobos, N., Hedgespeth, B., Grinberg, A. (2013). Utility of halofuginone lactate for the prevention of natural cryptosporidiosis of calves, in the presence of co-infection with rotavirus and Salmonella typhimurium. Vet. Parasitol. 197 (1-2), 59–67. doi: 10.1016/j.vetpar.2013.04.029
Amadi, B., Mwiya, M., Musuku, J., Watuka, A., Sianongo, S., Ayoub, A., et al. (2002). Effect of nitazoxanide on morbidity and mortality in Zambian children with cryptosporidiosis: a randomised controlled trial. Lancet 360 (9343), 1375–1380. doi: 10.1016/S0140-6736(02)11401-2
Amadi, B., Mwiya, M., Sianongo, S., Payne, L., Watuka, A., Katubulushi, M., et al. (2009). High dose prolonged treatment with nitazoxanide is not effective for cryptosporidiosis in HIV positive Zambian children: a randomised controlled trial. BMC Infect. Dis. 9 (1), 195. doi: 10.1186/1471-2334-9-195
Amenta, M., Dalle Nogare, E. R., Colomba, C., Prestileo, T. S., Di Lorenzo, F., Fundaro, S., et al. (1999). Intestinal protozoa in HIV-infected patients: effect of rifaximin in Cryptosporidium parvum and Blastocystis hominis infections. J. Chemother. 11 (5), 391–395. doi: 10.1179/joc.1999.11.5.391
Amer, S., Zidan, S., Adamu, H., Ye, J., Roellig, D., Xiao, L., et al. (2013). Prevalence and characterization of cryptosporidium spp. in dairy cattle in Nile river delta provinces, Egypt. Exp. Parasitol. 135 (3), 518–523. doi: 10.1016/j.exppara.2013.09.002
Amoo, J. K., Akindele, A. A., Amoo, A. O. J., Efunshile, A. M., Ojurongbe, T. A., Fayemiwo, S. A., et al. (2018). Prevalence of enteric parasitic infections among people living with HIV in abeokuta, Nigeria. Pan Afr Med. J. 30, 66. doi: 10.11604/pamj.2018.30.66.13160
Angus, K. W., Tzipori, S., Gray, E. W. (1982). Intestinal lesions in specific-pathogen-free lambs associated with a cryptosporidium from calves with diarrhea. Vet. Pathol. 19 (1), 67–78. doi: 10.1177/030098588201900110
Armitage, K., Flanigan, T., Carey, J., Frank, I., MacGregor, R. R., Ross, P., et al. (1992). Treatment of cryptosporidiosis with paromomycin. a report of five cases. Arch. Intern. Med. 152 (12), 2497–2499. doi: 10.1001/archinte.1992.00400240111018
Askari, N., Shayan, P., Mokhber-Dezfouli, M. R., Ebrahimzadeh, E., Lotfollahzadeh, S., Rostami, A., et al. (2016). Evaluation of recombinant P23 protein as a vaccine for passive immunization of newborn calves against Cryptosporidium parvum. Parasite Immunol. 38 (5), 282–289. doi: 10.1111/pim.12317
Aydogdu, U., Coskun, A., Atas, A. D., Basbug, O., Agaoglu, Z. T. (2019). The determination of treatment effect of chitosan oligosaccharide in lambs with experimentally cryptosporidiosis. Small Rumin Res. 180, 27–34. doi: 10.1016/j.smallrumres.2019.09.021
Aydogdu, U., Isik, N., Ekici, O. D., Yildiz, R., Sen, I., Coskun, A. (2018). Comparison of the effectiveness of halofuginone lactate and paromomycin in the treatment of calves naturally infected with Cryptosporidium parvum. Acta Scientiae Veterinariae 46 (1), 9. doi: 10.22456/1679-9216.81809
Babiker, A., Darbyshire, J., Pezzotti, P., Porter, K., Rezza, G., Walker, S. A., et al. (2002). Changes over calendar time in the risk of specific first AIDS-defining events following HIV seroconversion, adjusting for competing risks. Int. J. Epidemiol. 31 (5), 951–958. doi: 10.1093/ije/31.5.951
Bachur, T. P., Vale, J. M., Coelho, I. C., Queiroz, T. R., Chaves Cde, S. (2008). Enteric parasitic infections in HIV/AIDS patients before and after the highly active antiretroviral therapy. Braz. J. Infect. Dis. 12 (2), 115–122. doi: 10.1590/s1413-86702008000200004
Bakliwal, A., Nath, U. K., Mohanty, A., Gupta, P. (2021). Life-threatening Cryptosporidium diarrhea in a child on induction chemotherapy for acute lymphoblastic leukemia. Cureus 13 (9), e18340. doi: 10.7759/cureus.18340
Baragana, B., Forte, B., Choi, R., Nakazawa Hewitt, S., Bueren-Calabuig, J. A., Pisco, J. P., et al. (2019). Lysyl-tRNA synthetase as a drug target in malaria and cryptosporidiosis. Proc. Natl. Acad. Sci. U.S.A. 116 (14), 7015–7020. doi: 10.1073/pnas.1814685116
Bellini, V., Swale, C., Brenier-Pinchart, M. P., Pezier, T., Georgeault, S., Laurent, F., et al. (2020). Target identification of an antimalarial oxaborole identifies AN13762 as an alternative chemotype for targeting CPSF3 in apicomplexan parasites. iScience 23 (12), 101871. doi: 10.1016/j.isci.2020.101871
Benson, J. E., Ensley, S. M., Carson, T. L., Halbur, P. G., Janke, B. H., Quinn, W. J. (1998). Lasalocid toxicosis in neonatal calves. J. Vet. Diagn. Invest. 10 (2), 210–214. doi: 10.1177/104063879801000224
Bhadauria, D., Goel, A., Kaul, A., Sharma, R. K., Gupta, A., Ruhela, V., et al. (2015). Cryptosporidium infection after renal transplantation in an endemic area. Transpl Infect. Dis. 17 (1), 48–55. doi: 10.1111/tid.12336
Bissuel, F., Cotte, L., Rabodonirina, M., Rougier, P., Piens, M. A., Trepo, C. (1994). Paromomycin: an effective treatment for cryptosporidial diarrhea in patients with AIDS. Clin. Infect. Dis. 18 (3), 447–449. doi: 10.1093/clinids/18.3.447
Blanshard, C., Shanson, D. C., Gazzard, B. G. (1997). Pilot studies of azithromycin, letrazuril and paromomycin in the treatment of cryptosporidiosis. Int. J. STD AIDS 8 (2), 124–129. doi: 10.1258/0956462971919543
Bobin, S., Bouhour, D., Durupt, S., Boibieux, A., Girault, V., Peyramond, D. (1998). Importance of antiproteases in the treatment of microsporidia and/or cryptosporidia infections in HIV-seropositive patients. Pathol. Biol. (Paris) 46 (6), 418–419.
Borowitz, S. M., Saulsbury, F. T. (1991). Treatment of chronic cryptosporidial infection with orally administered human serum immune globulin. J. Pediatr. 119 (4), 593–595. doi: 10.1016/s0022-3476(05)82412-6
Braga, S. S. (2019). Cyclodextrins: Emerging medicines of the new millennium. Biomolecules 9 (12), 801. doi: 10.3390/biom9120801
Brainard, J., Hammer, C. C., Hunter, P. R., Katzer, F., Hurle, G., Tyler, K. (2021). Efficacy of halofuginone products to prevent or treat cryptosporidiosis in bovine calves: a systematic review and meta-analyses. Parasitology 148 (4), 408–419. doi: 10.1017/S0031182020002267
Buckner, F. S., Ranade, R. M., Gillespie, J. R., Shibata, S., Hulverson, M. A., Zhang, Z., et al. (2019). Optimization of methionyl tRNA-synthetase inhibitors for treatment of Cryptosporidium infection. Antimicrob. Agents Chemother. 63 (4), e02061–e02018. doi: 10.1128/AAC.02061-18
Burdese, M., Veglio, V., Consiglio, V., Soragna, G., Mezza, E., Bergamo, D., et al. (2005). A dance teacher with kidney-pancreas transplant and diarrhoea: what is the cause? Nephrol. Dial Transplant. 20 (8), 1759–1761. doi: 10.1093/ndt/gfh881
Call, S. A., Heudebert, G., Saag, M., Wilcox, C. M. (2000). The changing etiology of chronic diarrhea in HIV-infected patients with CD4 cell counts less than 200 cells/mm3. Am. J. Gastroenterol. 95 (11), 3142–3146. doi: 10.1111/j.1572-0241.2000.03285.x
Carr, A., Marriott, D., Field, A., Vasak, E., Cooper, D. A. (1998). Treatment of HIV-1-associated microsporidiosis and cryptosporidiosis with combination antiretroviral therapy. Lancet 351 (9098), 256–261. doi: 10.1016/S0140-6736(97)07529-6
Castro-Hermida, J. A., Garcia-Presedo, I., Gonzalez-Warleta, M., Mezo, M., Fenoy, S., Rueda, C., et al. (2008). Activity of an anti-inflammatory drug against cryptosporidiosis in neonatal lambs. Vet. Parasitol. 155 (3-4), 308–313. doi: 10.1016/j.vetpar.2008.05.012
Castro-Hermida, J. A., Gonzalez-Losada, Y., Freire-Santos, F., Mezo-Menendez, M., Ares-Mazas, E. (2001a). Evaluation of beta-cyclodextrin against natural infections of cryptosporidiosis in calves. Vet. Parasitol. 101 (2), 85–89. doi: 10.1016/s0304-4017(01)00505-2
Castro-Hermida, J. A., Gonzalez-Warleta, M., Mezo, M. (2007). Natural infection by Cryptosporidium parvum and Giardia duodenalis in sheep and goats in Galicia (NW Spain). Small Ruminant Res. 72 (2-3), 96–100. doi: 10.1016/j.smallrumres.2006.08.008
Castro-Hermida, J. A., Pors, I., Otero-Espinar, F., Luzardo-Alvarez, A., Ares-Mazas, E., Chartier, C. (2004). Efficacy of alpha-cyclodextrin against experimental cryptosporidiosis in neonatal goats. Vet. Parasitol. 120 (1-2), 35–41. doi: 10.1016/j.vetpar.2003.12.012
Castro-Hermida, J. A., Quilez-Cinca, J., Lopez-Bernad, F., Sanchez-Acedo, C., Freire-Santos, F., Ares-Mazas, E. (2001b). Treatment with beta-cyclodextrin of natural Cryptosporidium parvum infections in lambs under field conditions. Int. J. Parasitol. 31 (10), 1134–1137. doi: 10.1016/s0020-7519(01)00220-x
Causape, A. C., Quilez, J., Sanchez-Acedo, C., del Cacho, E., Lopez-Bernad, F. (2002). Prevalence and analysis of potential risk factors for Cryptosporidium parvum infection in lambs in zaragoza (northeastern Spain). Vet. Parasitol. 104 (4), 287–298. doi: 10.1016/s0304-4017(01)00639-2
Causapé, A. C., Sanchez-Acedo, C., Quilez, J., Del Cacho, E., Viu, M. (1999). Efficacy of halofuginone lactate against natural Cryptosporidium parvum infections in lambs. Res. Rev. Parasitol. 59, 41–46.
CDC (2019)DPDx cryptosporidiosis. In: Centers for disease control and prevention. Available at: https://www.cdc.gov/dpdx/cryptosporidiosis/index.html (Accessed December 30 2022).
Cello, J. P., Grendell, J. H., Basuk, P., Simon, D., Weiss, L., Wittner, M., et al. (1991). Effect of octreotide on refractory AIDS-associated diarrhea. a prospective, multicenter clinical trial. Ann. Intern. Med. 115 (9), 705–710. doi: 10.7326/0003-4819-115-9-705
Cerveja, B. Z., Tucuzo, R. M., Madureira, A. C., Nhacupe, N., Langa, I. A., Buene, T., et al. (2017). Prevalence of intestinal parasites among HIV infected and HIV uninfected patients treated at the 1 degrees de maio health centre in Maputo, Mozambique. EC Microbiol. 9 (6), 231–240.
Chalmers, R. M. (2012). Waterborne outbreaks of cryptosporidiosis. Ann. Ist Super Sanita. (San Diego, CA, USA: Academic Press, Elsevier) 48 (4), 429–446. doi: 10.4415/ANN_12_04_10
Chalmers, R. M. (2014). “Cryptosporidium,” in Microbiology of waterborne diseases. Eds. Percival, S. L., Yates, M. V., Williams, D. W., Chalmers, R. M., Gray, N. F. (Elsevier Ltd), 287–326.
Chartier, C., Mallereau, M. P., Lenfant, D. (1999). Halofuginone lactate in the control of cryptosporidiosis in neonate kids. Rev. Medecine Veterinaire 150 (4), 341–346.
Chartier, C., Mallereau, M. P., Naciri, M. (1996). Prophylaxis using paromomycin of natural cryptosporidial infection in neonatal kids. Prev. Veterinary Med. 25 (3-4), 357–361. doi: 10.1016/0167-5877(95)00511-0
Checkley, W., White, A. C., Jr., Jaganath, D., Arrowood, M. J., Chalmers, R. M., Chen, X. M., et al. (2015). A review of the global burden, novel diagnostics, therapeutics, and vaccine targets for cryptosporidium. Lancet Infect. Dis. 15 (1), 85–94. doi: 10.1016/S1473-3099(14)70772-8
Clezy, K., Gold, J., Blaze, J., Jones, P. (1991). Paromomycin for the treatment of cryptosporidial diarrhoea in AIDS patients. AIDS 5 (9), 1146–1147.
Clotet, B., Sirera, G., Cofan, F., Monterola, J. M., Tortosa, F., Fox, M. (1989). Efficacy of the somatostatin analogue (SMS-201-995), sandostatin, for cryptosporidial diarrhoea in patients with AIDS. AIDS 3 (12), 857–858. doi: 10.1097/00002030-198912000-00016
Connolly, G. M., Youle, M., Gazzard, B. G. (1990). Diclazuril in the treatment of severe cryptosporidial diarrhoea in AIDS patients. AIDS 4 (7), 700–701. doi: 10.1097/00002030-199007000-00020
Connor, E. E., Wall, E. H., Bravo, D. M., Evock-Clover, C. M., Elsasser, T. H., Baldwin, R. L. T., et al. (2017). Reducing gut effects from Cryptosporidium parvum infection in dairy calves through prophylactic glucagon-like peptide 2 therapy or feeding of an artificial sweetener. J. Dairy Sci. 100 (4), 3004–3018. doi: 10.3168/jds.2016-11861
Conti, S., Masocco, M., Pezzotti, P., Toccaceli, V., Vichi, M., Boros, S., et al. (2000). Differential impact of combined antiretroviral therapy on the survival of italian patients with specific AIDS-defining illnesses. J. Acquir. Immune Defic. Syndr. 25 (5), 451–458. doi: 10.1097/00042560-200012150-00011
Cook, D. J., Kelton, J. G., Stanisz, A. M., Collins, S. M. (1988). Somatostatin treatment for cryptosporidial diarrhea in a patient with the acquired immunodeficiency syndrome (AIDS). Ann. Intern. Med. 108 (5), 708–709. doi: 10.7326/0003-4819-108-5-708
Corso, P. S., Kramer, M. H., Blair, K. A., Addiss, D. G., Davis, J. P., Haddix, A. C. (2003). Cost of illness in the 1993 waterborne Cryptosporidium outbreak, Milwaukee, Wisconsin. Emerg. Infect. Dis. 9 (4), 426–431. doi: 10.3201/eid0904.020417
Current, W. L., Garcia, L. S. (1991). Cryptosporidiosis. Clin. Microbiol. Rev. 4 (3), 325–358. doi: 10.1128/CMR.4.3.325
Current, W. L., Reese, N. C. (1986). A comparison of endogenous development of three isolates of Cryptosporidium in suckling mice. J. Protozool 33 (1), 98–108. doi: 10.1111/j.1550-7408.1986.tb05567.x
Current, W. L., Reese, N. C., Ernst, J. V., Bailey, W. S., Heyman, M. B., Weinstein, W. M. (1983). Human cryptosporidiosis in immunocompetent and immunodeficient persons. studies of an outbreak and experimental transmission. N Engl. J. Med. 308 (21), 1252–1257. doi: 10.1056/NEJM198305263082102
D'Antonio, R. G., Winn, R. E., Taylor, J. P., Gustafson, T. L., Current, W. L., Rhodes, M. M., et al. (1985). A waterborne outbreak of cryptosporidiosis in normal hosts. Ann. Intern. Med. 103(6 (6 ( Pt 1), 886–888. doi: 10.7326/0003-4819-103-6-886
Danziger-Isakov, L. (2014). Gastrointestinal infections after transplantation. Curr. Opin. Gastroenterol. 30 (1), 40–46. doi: 10.1097/MOG.0000000000000016
Danziger, L. H., Kanyok, T. P., Novak, R. M. (1993). Treatment of cryptosporidial diarrhea in an AIDS patient with paromomycin. Ann. Pharmacother. 27 (12), 1460–1462. doi: 10.1177/106002809302701209
de Graaf, D. C., Vanopdenbosch, E., Ortega-Mora, L. M., Abbassi, H., Peeters, J. E. (1999). A review of the importance of cryptosporidiosis in farm animals. Int. J. Parasitol. 29 (8), 1269–1287. doi: 10.1016/s0020-7519(99)00076-4
Demonchy, J., Cordier, C., Frealle, E., Demarquette, H., Herbaux, C., Escure, G., et al. (2021). Case report: Two cases of cryptosporidiosis in heavily pretreated patients with myeloma. Clin. Lymphoma Myeloma Leuk 21 (6), e545–e547. doi: 10.1016/j.clml.2021.01.019
Denkinger, C. M., Harigopal, P., Ruiz, P., Dowdy, L. M. (2008). Cryptosporidium parvum-associated sclerosing cholangitis in a liver transplant patient. Transpl Infect. Dis. 10 (2), 133–136. doi: 10.1111/j.1399-3062.2007.00245.x
de Oliveira-Silva, M. B., de Oliveira, L. R., Resende, J. C., Peghini, B. C., Ramirez, L. E., Lages-Silva, E., et al. (2007). Seasonal profile and level of CD4+ lymphocytes in the occurrence of cryptosporidiosis and cystoisosporidiosis in HIV/AIDS patients in the triangulo mineiro region, Brazil. Rev. Soc. Bras. Med. Trop. 40 (5), 512–515. doi: 10.1590/s0037-86822007000500004
De Waele, V., Speybroeck, N., Berkvens, D., Mulcahy, G., Murphy, T. M. (2010). Control of cryptosporidiosis in neonatal calves: use of halofuginone lactate in two different calf rearing systems. Prev. Vet. Med. 96 (3-4), 143–151. doi: 10.1016/j.prevetmed.2010.06.017
Dillingham, R. A., Pinkerton, R., Leger, P., Severe, P., Guerrant, R. L., Pape, J. W., et al. (2009). High early mortality in patients with chronic acquired immunodeficiency syndrome diarrhea initiating antiretroviral therapy in Haiti: a case-control study. Am. J. Trop. Med. Hyg 80 (6), 1060–1064. doi: 10.4269/ajtmh.2009.80.1060
Dinler Ay, C., Voyvoda, H., Ulutas, P. A., Karagenc, T., Ulutas, B. (2021). Prophylactic and therapeutic efficacy of clinoptilolite against Cryptosporidium parvum in experimentally challenged neonatal lambs. Vet. Parasitol. 299, 109574. doi: 10.1016/j.vetpar.2021.109574
Dionisio, D., Orsi, A., Sterrantino, G., Meli, M., Di Lollo, S., Ibba Manneschi, L., et al. (1998). Chronic cryptosporidiosis in patients with AIDS: stable remission and possible eradication after long-term, low dose azithromycin. J. Clin. Pathol. 51 (2), 138–142. doi: 10.1136/jcp.51.2.138
Doumbo, O., Rossignol, J. F., Pichard, E., Traore, H. A., Dembele, T. M., Diakite, M., et al. (1997). Nitazoxanide in the treatment of cryptosporidial diarrhea and other intestinal parasitic infections associated with acquired immunodeficiency syndrome in tropical Africa. Am. J. Trop. Med. Hyg 56 (6), 637–639. doi: 10.4269/ajtmh.1997.56.637
Dupuy, F., Valot, S., Dalle, F., Sterin, A., L'Ollivier, C. (2021). Disseminated Cryptosporidium infection in an infant with CD40L deficiency. IDCases 24, e01115. doi: 10.1016/j.idcr.2021.e01115
Duru, S. Y., Öcal, N., Yağcİ, B. B., Gazyağcİ, S., Duru, Ö., Yİldİz, K. (2013). The therapeutic efficacy of tylosin in calf cryptosporidiosis Vol. 19 (Kars: Kafkas Üniversitesi Veteriner Fakültesi Dergisi), A175–A180.
Elitok, B., Elitok, O. M., Pulat, H. (2005). Efficacy of azithromycin dihydrate in treatment of cryptosporidiosis in naturally infected dairy calves. J. Vet. Intern. Med. 19 (4), 590–593. doi: 10.1892/0891-6640(2005)19[590:eoadit]2.0.co;2
Eltahan, R., Guo, F., Zhang, H., Xiang, L., Zhu, G. (2018). Discovery of ebselen as an inhibitor of Cryptosporidium parvum glucose-6-phosphate isomerase (CpGPI) by high-throughput screening of existing drugs. Int. J. Parasitol. Drugs Drug Resist. 8 (1), 43–49. doi: 10.1016/j.ijpddr.2018.01.003
Eltahan, R., Guo, F., Zhang, H., Zhu, G. (2019). The action of the hexokinase inhibitor 2-deoxy-d-glucose on Cryptosporidium parvum and the discovery of activities against the parasite hexokinase from marketed drugs. J. Eukaryot Microbiol. 66 (3), 460–468. doi: 10.1111/jeu.12690
English, E. D., Guerin, A., Tandel, J., Striepen, B. (2022). Live imaging of the Cryptosporidium parvum life cycle reveals direct development of male and female gametes from type I meronts. PloS Biol. 20 (4), e3001604. doi: 10.1371/journal.pbio.3001604
Fayer, R. (1992). Activity of sulfadimethoxine against cryptosporidiosis in dairy calves. J. Parasitol. 78 (3), 534–537. doi: 10.2307/3283662
Fayer, R., Andrews, C., Ungar, B. L., Blagburn, B. (1989). Efficacy of hyperimmune bovine colostrum for prophylaxis of cryptosporidiosis in neonatal calves. J. Parasitol. 75 (3), 393–397. doi: 10.2307/3282595
Fayer, R., Ellis, W. (1993). Paromomycin is effective as prophylaxis for cryptosporidiosis in dairy calves. J. Parasitol. 79 (5), 771–774. doi: 10.2307/3283619
Fayer, R., Fetterer, R. (1995). Activity of benzimidazoles against cryptosporidiosis in neonatal BALB/c mice. J. Parasitol. 81 (5), 794–795. doi: 10.2307/3283980
Fayer, R., Klesius, P. H., Andrews, C. (1987). Efficacy of bovine transfer factor to protect neonatal calves against experimentally induced clinical cryptosporidiosis. J. Parasitol. 73 (5), 1061–1062. doi: 10.2307/3282539
Fayer, R., Morgan, U., Upton, S. J. (2000). Epidemiology of Cryptosporidium: transmission, detection and identification. Int. J. Parasitol. 30 (12-13), 1305–1322. doi: 10.1016/s0020-7519(00)00135-1
Fayer, R., Santin, M. (2009). Cryptosporidium xiaoi n. sp. (Apicomplexa: Cryptosporidiidae) in sheep (Ovis aries). Vet. Parasitol. 164 (2-4), 192–200. doi: 10.1016/j.vetpar.2009.05.011
Fayer, R., Santin, M., Macarisin, D. (2010). Cryptosporidium ubiquitum n. sp. in animals and humans. Vet. Parasitol. 172 (1-2), 23–32. doi: 10.1016/j.vetpar.2010.04.028
Fayer, R., Santin, M., Trout, J. M. (2008). Cryptosporidium ryanae n. sp. (Apicomplexa: Cryptosporidiidae) in cattle (Bos taurus). Vet. Parasitol. 156 (3-4), 191–198. doi: 10.1016/j.vetpar.2008.05.024
Fayer, R., Santin, M., Trout, J. M., Greiner, E. (2006). Prevalence of species and genotypes of Cryptosporidium found in 1-2-year-old dairy cattle in the eastern united states. Vet. Parasitol. 135 (2), 105–112. doi: 10.1016/j.vetpar.2005.08.003
Fayer, R., Santin, M., Xiao, L. (2005). Cryptosporidium bovis n. sp. (Apicomplexa: Cryptosporidiidae) in cattle (Bos taurus). J. Parasitol. 91 (3), 624–629. doi: 10.1645/GE-3435
Fernandez, S., Fraga, M., Castells, M., Colina, R., Zunino, P. (2020). Effect of the administration of Lactobacillus spp. strains on neonatal diarrhoea, immune parameters and pathogen abundance in pre-weaned calves. Benef Microbes 11 (5), 477–488. doi: 10.3920/BM2019.0167
Ferre, I., Benito-Pena, A., Garcia, U., Osoro, K., Ortega-Mora, L. M. (2005). Effect of different decoquinate treatments on cryptosporidiosis in naturally infected cashmere goat kids. Vet. Rec 157 (9), 261–262. doi: 10.1136/vr.157.9.261
Fichtenbaum, C. J., Ritchie, D. J., Powderly, W. G. (1993). Use of paromomycin for treatment of cryptosporidiosis in patients with AIDS. Clin. Infect. Dis. 16 (2), 298–300. doi: 10.1093/clind/16.2.298
Fichtenbaum, C. J., Zackin, R., Feinberg, J., Benson, C., Griffiths, J. K., Team, A.C.T.G.N.W.C.S (2000). Rifabutin but not clarithromycin prevents cryptosporidiosis in persons with advanced HIV infection. AIDS 14 (18), 2889–2893. doi: 10.1097/00002030-200012220-00010
Fischer, O. (1983). Attempted therapy and prophylaxis of cryptosporidiosis in calves by administration of sulphadimidine. Acta Veterinaria Brno 52 (3-4), 183–190. doi: 10.2754/avb198352030183
Flanigan, T. P., Ramratnam, B., Graeber, C., Hellinger, J., Smith, D., Wheeler, D., et al. (1996). Prospective trial of paromomycin for cryptosporidiosis in AIDS. Am. J. Med. 100 (3), 370–372. doi: 10.1016/S0002-9343(97)89499-5
Flanigan, T., Whalen, C., Turner, J., Soave, R., Toerner, J., Havlir, D., et al. (1992). Cryptosporidium infection and CD4 counts. Ann. Intern. Med. 116 (10), 840–842. doi: 10.7326/0003-4819-116-10-840
Floren, C. H., Chinenye, S., Elfstrand, L., Hagman, C., Ihse, I. (2006). ColoPlus, a new product based on bovine colostrum, alleviates HIV-associated diarrhoea. Scand. J. Gastroenterol. 41 (6), 682–686. doi: 10.1080/00365520500380817
Foudraine, N. A., Weverling, G. J., van Gool, T., Roos, M. T., de Wolf, F., Koopmans, P. P., et al. (1998). Improvement of chronic diarrhoea in patients with advanced HIV-1 infection during potent antiretroviral therapy. AIDS 12 (1), 35–41. doi: 10.1097/00002030-199801000-00005
Gathe, J. C., Jr., Mayberry, C., Clemmons, J., Nemecek, J. (2008). Resolution of severe cryptosporidial diarrhea with rifaximin in patients with AIDS. J. Acquir. Immune Defic. Syndr. 48 (3), 363–364. doi: 10.1097/QAI.0b013e31817beb78
Gathe, J., Piot, D., Hawkins, K., Bernal, A., Clemmons, J., Stool, E. (1990). “Treatment of gastrointestinal cryptosporidiosis with paromomycin (abstract 2121),” in International Conference on AIDS(San Francisco, CA).
Geurden, T., Thomas, P., Casaert, S., Vercruysse, J., Claerebout, E. (2008). Prevalence and molecular characterisation of Cryptosporidium and Giardia in lambs and goat kids in Belgium. Vet. Parasitol. 155 (1-2), 142–145. doi: 10.1016/j.vetpar.2008.05.002
Gharpure, R., Perez, A., Miller, A. D., Wikswo, M. E., Silver, R., Hlavsa, M. C. (2019). Cryptosporidiosis outbreaks - united state -2017. MMWR Morb Mortal Wkly Rep. 68 (25), 568–572. doi: 10.15585/mmwr.mm6825a3
Giadinis, N. D., Papadopoulos, E., Lafi, S. Q., Panousis, N. K., Papazahariadou, M., Karatzias, H. (2008). Efficacy of halofuginone lactate for the treatment and prevention of cryptosporidiosis in goat kids: An extensive field trial. Small Ruminant Res. 76 (3), 195–200. doi: 10.1016/j.smallrumres.2008.01.007
Giadinis, N. D., Papadopoulos, E., Panousis, N., Papazahariadou, M., Lafi, S. Q., Karatzias, H. (2007). Effect of halofuginone lactate on treatment and prevention of lamb cryptosporidiosis: an extensive field trial. J. Vet. Pharmacol. Ther. 30 (6), 578–582. doi: 10.1111/j.1365-2885.2007.00900.x
Girard, P. M., Goldschmidt, E., Vittecoq, D., Massip, P., Gastiaburu, J., Meyohas, M. C., et al. (1992). Vapreotide, a somatostatin analogue, in cryptosporidiosis and other AIDS-related diarrhoeal diseases. AIDS 6 (7), 715–718. doi: 10.1097/00002030-199207000-00015
Gobel, E. (1987a). Diagnosis and treatment of acute cryptosporidiosis in the calf. Tieraerztliche Umschau. 42, 863–869.
Gobel, E. (1987b). Possibilities of therapy of cryptosporidiosis in calves in problematic farms. zentralblatt für bakteriologie, mikrobiologie und hygiene. Ser. A: Med. Microbiology Infect. Diseases Virology Parasitol. 265 (3-4), 490–490.
Goma, F. Y., Geurden, T., Siwila, J., Phiri, I. G. K., Gabriel, S., Claerebout, E., et al. (2007). The prevalence and molecular characterisation of cryptosporidium spp. in small ruminants in Zambia. Small Ruminant Res. 72 (1), 77–80. doi: 10.1016/j.smallrumres.2006.08.010
Greenberg, P. D., Cello, J. P. (1996). Treatment of severe diarrhea caused by Cryptosporidium parvum with oral bovine immunoglobulin concentrate in patients with AIDS. J. Acquir. Immune Defic. Syndr. Hum. Retrovirol 13 (4), 348–354. doi: 10.1097/00042560-199612010-00008
Grinberg, A., Markovics, A., Galindez, J., Lopez-Villalobos, N., Kosak, A., Tranquillo, V. M. (2002). Controlling the onset of natural cryptosporidiosis in calves with paromomycin sulphate. Vet. Rec 151 (20), 606–608. doi: 10.1136/vr.151.20.606
Grube, H., Ramratnam, B., Ley, C., Flanigan, T. P. (1997). Resolution of AIDS associated cryptosporidiosis after treatment with indinavir. Am. J. Gastroenterol. 92 (4), 726.
Harp, J. A., Jardon, P., Atwill, E. R., Zylstra, M., Checel, S., Goff, J. P., et al. (1996). Field testing of prophylactic measures against Cryptosporidium parvum infection in calves in a California dairy herd. Am. J. Vet. Res. 57 (11), 1586–1588.
Harris, M., Deutsch, G., MacLean, J. D., Tsoukas, C. M. (1994). A phase I study of letrazuril in AIDS-related cryptosporidiosis. AIDS 8 (8), 1109–1113. doi: 10.1097/00002030-199408000-00011
Hartmann, G., Honikel, K. O., Knusel, F., Nuesch, J. (1967). The specific inhibition of the DNA-directed RNA synthesis by rifamycin. Biochim. Biophys. Acta 145 (3), 843–844. doi: 10.1016/0005-2787(67)90147-5
Hasan, M. M., Stebbins, E. E., Choy, R. K. M., Gillespie, J. R., de Hostos, E. L., Miller, P., et al. (2021). Spontaneous selection of Cryptosporidium drug resistance in a calf model of infection. Antimicrob. Agents Chemother. 65 (6), e00023–21. doi: 10.1128/AAC.00023-21
Hashmey, R., Smith, N. H., Cron, S., Graviss, E. A., Chappell, C. L., White, A. C., Jr. (1997). Cryptosporidiosis in Houston, texas. a report of 95 cases. Med. (Baltimore) 76 (2), 118–139. doi: 10.1097/00005792-199703000-00004
Hatam-Nahavandi, K., Ahmadpour, E., Carmena, D., Spotin, A., Bangoura, B., Xiao, L. (2019). Cryptosporidium infections in terrestrial ungulates with focus on livestock: a systematic review and meta-analysis. Parasit Vectors 12 (1), 453. doi: 10.1186/s13071-019-3704-4
Hayes, E. B., Matte, T. D., O'Brien, T. R., McKinley, T. W., Logsdon, G. S., Rose, J. B., et al. (1989). Large Community outbreak of cryptosporidiosis due to contamination of a filtered public water supply. N Engl. J. Med. 320 (21), 1372–1376. doi: 10.1056/NEJM198905253202103
Henry, R. J. (1943). The mode of action of sulfonamides. Bacteriol Rev. 7 (4), 175–262. doi: 10.1128/br.7.4.175-262.1943
Hewitt, R. G., Yiannoutsos, C. T., Higgs, E. S., Carey, J. T., Geiseler, P. J., Soave, R., et al. (2000). Paromomycin: no more effective than placebo for treatment of cryptosporidiosis in patients with advanced human immunodeficiency virus infection. AIDS clinical trial group. Clin. Infect. Dis. 31 (4), 1084–1092. doi: 10.1086/318155
Hicks, P., Zwiener, R. J., Squires, J., Savell, V. (1996). Azithromycin therapy for Cryptosporidium parvum infection in four children infected with human immunodeficiency virus. J. Pediatr. 129 (2), 297–300. doi: 10.1016/s0022-3476(96)70258-5
Hoffman, P. S., Sisson, G., Croxen, M. A., Welch, K., Harman, W. D., Cremades, N., et al. (2007). Antiparasitic drug nitazoxanide inhibits the pyruvate oxidoreductases of Helicobacter pylori, selected anaerobic bacteria and parasites, and Campylobacter jejuni. Antimicrob. Agents Chemother. 51 (3), 868–876. doi: 10.1128/AAC.01159-06
Holmberg, S. D., Moorman, A. C., Von Bargen, J. C., Palella, F. J., Loveless, M. O., Ward, D. J., et al. (1998). Possible effectiveness of clarithromycin and rifabutin for cryptosporidiosis chemoprophylaxis in HIV disease. HIV outpatient study (HOPS) investigators. JAMA 279 (5), 384–386. doi: 10.1001/jama.279.5.384
Hong, D. K., Wong, C. J., Gutierrez, K. (2007). Severe cryptosporidiosis in a seven-year-old renal transplant recipient: case report and review of the literature. Pediatr. Transplant. 11 (1), 94–100. doi: 10.1111/j.1399-3046.2006.00593.x
Hoxie, N. J., Davis, J. P., Vergeront, J. M., Nashold, R. D., Blair, K. A. (1997). Cryptosporidiosis-associated mortality following a massive waterborne outbreak in Milwaukee, Wisconsin. Am. J. Public Health 87 (12), 2032–2035. doi: 10.2105/ajph.87.12.2032
Huang, W., Hulverson, M. A., Choi, R., Arnold, S. L. M., Zhang, Z., McCloskey, M. C., et al. (2019). Development of 5-Aminopyrazole-4-carboxamide-based bumped-kinase inhibitors for cryptosporidiosis therapy. J. Med. Chem. 62 (6), 3135–3146. doi: 10.1021/acs.jmedchem.9b00069
Huang, M. Z., Li, J., Guan, L., Li, D. Q., Nie, X. M., Gui, R., et al. (2015). Therapeutic effects of acetylspiramycin and garlicin on cryptosporidiosis among drug users. Int. J. Parasitol. Drugs Drug Resist. 5 (3), 185–190. doi: 10.1016/j.ijpddr.2015.09.002
Hulverson, M. A., Choi, R., Arnold, S. L. M., Schaefer, D. A., Hemphill, A., McCloskey, M. C., et al. (2017). Advances in bumped kinase inhibitors for human and animal therapy for cryptosporidiosis. Int. J. Parasitol. 47 (12), 753–763. doi: 10.1016/j.ijpara.2017.08.006
Hunt, E., Fu, Q., Armstrong, M. U., Rennix, D. K., Webster, D. W., Galanko, J. A., et al. (2002). Oral bovine serum concentrate improves cryptosporidial enteritis in calves. Pediatr. Res. 51 (3), 370–376. doi: 10.1203/00006450-200203000-00017
Hussien, S. M., Abdella, O. H., Abu-Hashim, A. H., Aboshiesha, G. A., Taha, M. A., El-Shemy, A. S., et al. (2013). Comparative study between the effect of nitazoxanide and paromomycine in treatment of cryptosporidiosis in hospitalized children. J. Egypt Soc. Parasitol. 43 (2), 463–470.
Innes, E. A., Chalmers, R. M., Wells, B., Pawlowic, M. C. (2020). A one health approach to tackle cryptosporidiosis. Trends Parasitol. 36 (3), 290–303. doi: 10.1016/j.pt.2019.12.016
Iroh Tam, P., Arnold, S. L. M., Barrett, L. K., Chen, C. R., Conrad, T. M., Douglas, E., et al. (2021). Clofazimine for treatment of cryptosporidiosis in human immunodeficiency virus infected adults: An experimental medicine, randomized, double-blind, placebo-controlled phase 2a trial. Clin. Infect. Dis. 73 (2), 183–191. doi: 10.1093/cid/ciaa421
Ives, N. J., Gazzard, B. G., Easterbrook, P. J. (2001). The changing pattern of AIDS-defining illnesses with the introduction of highly active antiretroviral therapy (HAART) in a London clinic. J. Infect. 42 (2), 134–139. doi: 10.1053/jinf.2001.0810
Jacobson, C., Al-Habsi, K., Ryan, U., Williams, A., Anderson, F., Yang, R., et al. (2018). Cryptosporidium infection is associated with reduced growth and diarrhoea in goats beyond weaning. Vet. Parasitol. 260, 30–37. doi: 10.1016/j.vetpar.2018.07.005
Jacobson, C., Williams, A., Yang, R., Ryan, U., Carmichael, I., Campbell, A. J., et al. (2016). Greater intensity and frequency of Cryptosporidium and Giardia oocyst shedding beyond the neonatal period is associated with reductions in growth, carcase weight and dressing efficiency in sheep. Vet. Parasitol. 228, 42–51. doi: 10.1016/j.vetpar.2016.08.003
Jain, V., Yogavel, M., Kikuchi, H., Oshima, Y., Hariguchi, N., Matsumoto, M., et al. (2017). Targeting prolyl-tRNA synthetase to accelerate drug discovery against malaria, leishmaniasis, toxoplasmosis, cryptosporidiosis, and coccidiosis. Structure 25 (10), 1495–1505 e1496. doi: 10.1016/j.str.2017.07.015
Jarvie, B. D., Trotz-Williams, L. A., McKnight, D. R., Leslie, K. E., Wallace, M. M., Todd, C. G., et al. (2005). Effect of halofuginone lactate on the occurrence of Cryptosporidium parvum and growth of neonatal dairy calves. J. Dairy Sci. 88 (5), 1801–1806. doi: 10.3168/jds.S0022-0302(05)72854-X
Jasenosky, L. D., Cadena, C., Mire, C. E., Borisevich, V., Haridas, V., Ranjbar, S., et al. (2019). The FDA-approved oral drug nitazoxanide amplifies host antiviral responses and inhibits Ebola virus. iScience 19, 1279–1290. doi: 10.1016/j.isci.2019.07.003
Joachim, A., Krull, T., Schwarzkopf, J., Daugschies, A. (2003). Prevalence and control of bovine cryptosporidiosis in German dairy herds. Vet. Parasitol. 112 (4), 277–288. doi: 10.1016/s0304-4017(03)00006-2
Johnson, E. H., Windsor, J. J., Muirhead, D. E., King, G. J., Al-Busaidy, R. (2000). Confirmation of the prophylactic value of paromomycin in a natural outbreak of caprine cryptosporidiosis. Vet. Res. Commun. 24 (1), 63–67. doi: 10.1023/a:1006381522986
Jordan, W. C. (1996). Clarithromycin prophylaxis against Cryptosporidium enteritis in patients with AIDS. J. Natl. Med. Assoc. 88 (7), 425–427.
Jumani, R. S., Bessoff, K., Love, M. S., Miller, P., Stebbins, E. E., Teixeira, J. E., et al. (2018). A novel piperazine-based drug lead for cryptosporidiosis from the medicines for malaria venture open-access malaria box. Antimicrob. Agents Chemother. 62 (4), e01505–e01517. doi: 10.1128/AAC.01505-17
Kacar, Y., Baykal, A. T., Aydin, L., Batmaz, H. (2022). Evaluation of the efficacy of cow colostrum in the treatment and its effect on serum proteomes in calves with cryptosporidiosis. Vet. Immunol. Immunopathol. 248, 110429. doi: 10.1016/j.vetimm.2022.110429
Kadappu, K., Nagaraja, M., Rao, P., Shastry, B. (2002). Azithromycin as treatment for cryptosporidiosis in human immunodeficiency virus disease. J. Postgraduate Med. 48 (3), 179–181.
Karanis, P. (2018). “Cryptosporidium: Waterborne and foodborne transmission and worldwide outbreaks,” in Recent advances in environmental science from the Euro-Mediterranean and surrounding regions (Cham: Cham: Springer), 41–44.
Katsoulos, P. D., Karatzia, M. A., Dovas, C. I., Filioussis, G., Papadopoulos, E., Kiossis, E., et al. (2017). Evaluation of the in-field efficacy of oregano essential oil administration on the control of neonatal diarrhea syndrome in calves. Res. Vet. Sci. 115, 478–483. doi: 10.1016/j.rvsc.2017.07.029
Katz, M. D., Erstad, B. L., Rose, C. (1988). Treatment of severe cryptosporidium-related diarrhea with octreotide in a patient with AIDS. Drug Intell. Clin. Pharm. 22 (2), 134–136. doi: 10.1177/106002808802200206
Keidel, J., Daugschies, A. (2013). Integration of halofuginone lactate treatment and disinfection with p-chloro-m-cresol to control natural cryptosporidiosis in calves. Vet. Parasitol. 196 (3-4), 321–326. doi: 10.1016/j.vetpar.2013.03.003
Kelly, P., Lungu, F., Keane, E., Baggaley, R., Kazembe, F., Pobee, J., et al. (1996). Albendazole chemotherapy for treatment of diarrhoea in patients with AIDS in Zambia: a randomised double blind controlled trial. BMJ 312 (7040), 1187–1191. doi: 10.1136/bmj.312.7040.1187
Khalil, I. A., Troeger, C., Rao, P. C., Blacker, B. F., Brown, A., Brewer, T. G., et al. (2018). Morbidity, mortality, and long-term consequences associated with diarrhoea from Cryptosporidium infection in children younger than 5 years: a meta-analyses study. Lancet Global Health 6 (7), E758–E768. doi: 10.1016/S2214-109x(18)30283-3
Khan, S. M., Debnath, C., Pramanik, A. K., Xiao, L., Nozaki, T., Ganguly, S. (2010). Molecular characterization and assessment of zoonotic transmission of Cryptosporidium from dairy cattle in West Bengal, India. Vet. Parasitol. 171 (1-2), 41–47. doi: 10.1016/j.vetpar.2010.03.008
Khan, S. M., Garcia Hernandez, A., Allaie, I. M., Grooms, G. M., Li, K., Witola, W. H., et al. (2022a). Activity of (1-benzyl-4-triazolyl)-indole-2-carboxamides against Toxoplasma gondii and Cryptosporidium parvum. Int. J. Parasitol. Drugs Drug Resist. 19, 6–20. doi: 10.1016/j.ijpddr.2022.04.001
Khan, S. M., Zhang, X., Witola, W. H. (2022b). Cryptosporidium parvum pyruvate kinase inhibitors with in vivo anti-cryptosporidial efficacy. Front. Microbiol. 12. doi: 10.3389/fmicb.2021.800293
Klein, P. (2008). Preventive and therapeutic efficacy of halofuginone-lactate against Cryptosporidium parvum in spontaneously infected calves: a centralised, randomised, double-blind, placebo-controlled study. Vet. J. 177 (3), 429–431. doi: 10.1016/j.tvjl.2007.05.007
Kotloff, K. L., Nataro, J. P., Blackwelder, W. C., Nasrin, D., Farag, T. H., Panchalingam, S., et al. (2013). Burden and aetiology of diarrhoeal disease in infants and young children in developing countries (the global enteric multicenter study, GEMS): a prospective, case-control study. Lancet 382 (9888), 209–222. doi: 10.1016/S0140-6736(13)60844-2
Koudela, B., Bokova, A. (1997). The effect of cotrimoxazole on experimental Cryptosporidium parvum infection in kids. Vet. Res. 28 (4), 405–412.
Krause, I., Amir, J., Cleper, R., Dagan, A., Behor, J., Samra, Z., et al. (2012). Cryptosporidiosis in children following solid organ transplantation. Pediatr. Infect. Dis. J. 31 (11), 1135–1138. doi: 10.1097/INF.0b013e31826780f7
Lacey, E. (1988). The role of the cytoskeletal protein, tubulin, in the mode of action and mechanism of drug resistance to benzimidazoles. Int. J. Parasitol. 18 (7), 885–936. doi: 10.1016/0020-7519(88)90175-0
Lallemand, M., Villeneuve, A., Belda, J., Dubreuil, P. (2006). Field study of the efficacy of halofuginone and decoquinate in the treatment of cryptosporidiosis in veal calves. Vet. Rec 159 (20), 672–676. doi: 10.1136/vr.159.20.672
Lanternier, F., Amazzough, K., Favennec, L., Mamzer-Bruneel, M. F., Abdoul, H., Tourret, J., et al. (2017). Cryptosporidium spp. infection in solid organ transplantation: The nationwide "TRANSCRYPTO" study. Transplantation 101 (4), 826–830. doi: 10.1097/TP.0000000000001503
Lee, S., Ginese, M., Beamer, G., Danz, H. R., Girouard, D. J., Chapman-Bonofiglio, S. P., et al. (2018). Therapeutic efficacy of bumped kinase inhibitor 1369 in a pig model of acute diarrhea caused by Cryptosporidium hominis. Antimicrob. Agents Chemother. 62 (7), e00147–e00118. doi: 10.1128/AAC.00147-18
Lee, S., Ginese, M., Girouard, D., Beamer, G., Huston, C. D., Osbourn, D., et al. (2019). Piperazine-derivative MMV665917: An effective drug in the diarrheic piglet model of Cryptosporidium hominis. J. Infect. Dis. 220 (2), 285–293. doi: 10.1093/infdis/jiz105
Lee, S., Harwood, M., Girouard, D., Meyers, M. J., Campbell, M. A., Beamer, G., et al. (2017). The therapeutic efficacy of azithromycin and nitazoxanide in the acute pig model of Cryptosporidium hominis. PloS One 12 (10), e0185906. doi: 10.1371/journal.pone.0185906
Lefay, D., Naciri, M., Poirier, P., Chermette, R. (2001). Efficacy of halofuginone lactate in the prevention of cryptosporidiosis in suckling calves. Vet. Rec 148 (4), 108–112. doi: 10.1136/vr.148.4.108
Legrand, F., Grenouillet, F., Larosa, F., Dalle, F., Saas, P., Millon, L., et al. (2011). Diagnosis and treatment of digestive cryptosporidiosis in allogeneic haematopoietic stem cell transplant recipients: a prospective single centre study. Bone Marrow Transplant. 46 (6), 858–862. doi: 10.1038/bmt.2010.200
Le Moing, V., Bissuel, F., Costagliola, D., Eid, Z., Chapuis, F., Molina, J.-M., et al. (1998). Decreased prevalence of intestinal cryptosporidiosis in HIV-infected patients concomitant to the widespread use of protease inhibitors. AIDS 12 (11), 1395–1397. doi: 10.1097/00002030-199811000-00026
Lendner, M., Bottcher, D., Delling, C., Ojo, K. K., Van Voorhis, W. C., Daugschies, A. (2015). A novel CDPK1 inhibitor–a potential treatment for cryptosporidiosis in calves? Parasitol. Res. 114 (1), 335–336. doi: 10.1007/s00436-014-4228-7
Liberti, A., Bisogno, A., Izzo, E. (1992). Octreotide treatment in secretory and cyrptosporidial diarrhea in patients with acquired immunodeficiency syndrome (AIDS): clinical evaluation. J. Chemother. 4 (5), 303–305. doi: 10.1080/1120009x.1992.11739182
Li, K., Grooms, G. M., Khan, S. M., Hernandez, A. G., Witola, W. H., Stec, J. (2020). Novel acyl carbamates and acyl / diacyl ureas show in vitro efficacy against Toxoplasma gondii and Cryptosporidium parvum. Int. J. Parasitol. Drugs Drug Resist. 14, 80–90. doi: 10.1016/j.ijpddr.2020.08.006
Li, K., Nader, S. M., Zhang, X., Ray, B. C., Kim, C. Y., Das, A., et al. (2019). Novel lactate dehydrogenase inhibitors with in vivo efficacy against Cryptosporidium parvum. PloS Pathog. 15 (7), e1007953. doi: 10.1371/journal.ppat.1007953
Lindsay, D. S., Upton, S. J., Owens, D. S., Morgan, U. M., Mead, J. R., Blagburn, B. L. (2000). Cryptosporidium andersoni n. sp. (Apicomplexa: Cryptosporiidae) from cattle, Bos taurus. J. Eukaryot Microbiol. 47 (1), 91–95. doi: 10.1111/j.1550-7408.2000.tb00016.x
Lin, J., Zhou, D., Steitz, T. A., Polikanov, Y. S., Gagnon, M. G. (2018). Ribosome-targeting antibiotics: Modes of action, mechanisms of resistance, and implications for drug design. Annu. Rev. Biochem. 87 (1), 451–478. doi: 10.1146/annurev-biochem-062917-011942
Loeb, M., Walach, C., Phillips, J., Fong, I., Salit, I., Rachlis, A., et al. (1995). Treatment with letrazuril of refractory cryptosporidial diarrhea complicating AIDS. J. Acquir. Immune Defic. Syndr. Hum. Retrovirol 10 (1), 48–53. doi: 10.1097/00042560-199509000-00007
Louie, E., Borkowsky, W., Klesius, P. H., Haynes, T. B., Gordon, S., Bonk, S., et al. (1987). Treatment of cryptosporidiosis with oral bovine transfer factor. Clin. Immunol. Immunopathol. 44 (3), 329–334. doi: 10.1016/0090-1229(87)90077-8
Love, M. S., Beasley, F. C., Jumani, R. S., Wright, T. M., Chatterjee, A. K., Huston, C. D., et al. (2017). A high-throughput phenotypic screen identifies clofazimine as a potential treatment for cryptosporidiosis. PloS Negl. Trop. Dis. 11 (2), e0005373. doi: 10.1371/journal.pntd.0005373
Love, M. S., Choy, R. K. M. (2021). Emerging treatment options for cryptosporidiosis. Curr. Opin. Infect. Dis. 34 (5), 455–462. doi: 10.1097/QCO.0000000000000761
Lunde, C. S., Stebbins, E. E., Jumani, R. S., Hasan, M. M., Miller, P., Barlow, J., et al. (2019). Identification of a potent benzoxaborole drug candidate for treating cryptosporidiosis. Nat. Commun. 10 (1), 2816. doi: 10.1038/s41467-019-10687-y
Mac Kenzie, W. R., Hoxie, N. J., Proctor, M. E., Gradus, M. S., Blair, K. A., Peterson, D. E., et al. (1994). A massive outbreak in Milwaukee of cryptosporidium infection transmitted through the public water supply. N Engl. J. Med. 331 (3), 161–167. doi: 10.1056/NEJM199407213310304
Maddox-Hyttel, C., Langkjaer, R. B., Enemark, H. L., Vigre, H. (2006). Cryptosporidium and Giardia in different age groups of Danish cattle and pigs–occurrence and management associated risk factors. Vet. Parasitol. 141 (1-2), 48–59. doi: 10.1016/j.vetpar.2006.04.032
Maggi, P., Larocca, A. M., Quarto, M., Serio, G., Brandonisio, O., Angarano, G., et al. (2000). Effect of antiretroviral therapy on cryptosporidiosis and microsporidiosis in patients infected with human immunodeficiency virus type 1. Eur. J. Clin. Microbiol. Infect. Dis. 19 (3), 213–217. doi: 10.1007/s100960050461
Mancassola, R., Reperant, J. M., Naciri, M., Chartier, C. (1995). Chemoprophylaxis of Cryptosporidium parvum infection with paromomycin in kids and immunological study. Antimicrob. Agents Chemother. 39 (1), 75–78. doi: 10.1128/AAC.39.1.75
Mancassola, R., Richard, A., Naciri, M. (1997). Evaluation of decoquinate to treat experimental cryptosporidiosis in kids. Vet. Parasitol. 69 (1-2), 31–37. doi: 10.1016/s0304-4017(96)01094-1
Manjunatha, U. H., Vinayak, S., Zambriski, J. A., Chao, A. T., Sy, T., Noble, C. G., et al. (2017). A Cryptosporidium PI(4)K inhibitor is a drug candidate for cryptosporidiosis. Nature 546 (7658), 376–380. doi: 10.1038/nature22337
Maurya, P. S., Sahu, S., Sudhakar, N. R., Jaiswal, V., Prashant, D. G., Rawat, S., et al. (2016). Cryptosporidiosis in a buffalo calf at meerut, uttar pradesh and its successful therapeutic management. J. Parasit Dis. 40 (4), 1583–1585. doi: 10.1007/s12639-015-0734-5
McDonald, V., Korbel, D. S., Barakat, F. M., Choudhry, N., Petry, F. (2013). Innate immune responses against Cryptosporidium parvum infection. Parasite Immunol. 35 (2), 55–64. doi: 10.1111/pim.12020
McMeeking, A., Borkowsky, W., Klesius, P. H., Bonk, S., Holzman, R. S., Lawrence, H. S. (1990). A controlled trial of bovine dialyzable leukocyte extract for cryptosporidiosis in patients with AIDS. J. Infect. Dis. 161 (1), 108–112. doi: 10.1093/infdis/161.1.108
Mead, J. R. (2014). Prospects for immunotherapy and vaccines against Cryptosporidium. Hum. Vaccin Immunother. 10 (6), 1505–1513. doi: 10.4161/hv.28485
Meamar, A. R., Rezaian, M., Rezaie, S., Mohraz, M., Kia, E. B., Houpt, E. R., et al. (2006). Cryptosporidium parvum bovine genotype oocysts in the respiratory samples of an AIDS patient: efficacy of treatment with a combination of azithromycin and paromomycin. Parasitol. Res. 98 (6), 593–595. doi: 10.1007/s00436-005-0097-4
Meganck, V., Hoflack, G., Piepers, S., Opsomer, G. (2015). Evaluation of a protocol to reduce the incidence of neonatal calf diarrhoea on dairy herds. Prev. Vet. Med. 118 (1), 64–70. doi: 10.1016/j.prevetmed.2014.11.007
Meisel, J. L., Perera, D. R., Meligro, C., Rubin, C. E. (1976). Overwhelming watery diarrhea associated with a cryptosporidium in an immunosuppressed patient. Gastroenterology 70 (6), 1156–1160. doi: 10.1016/S0016-5085(76)80331-9
Mele, R., Morales, M. A. G., Tosini, F., Pozio, E. (2003). Indinavir reduces Cryptosporidium parvum infection in both in vitro and in vivo models. Int. J. Parasitol. 33 (7), 757–764. doi: 10.1016/S0020-7519(03)00093-6
Mendonca, F. L. M., Carvalho, J. G., Silva, R. J., Ferreira, L. C. A., Cerqueira, D. M., Rogge, H. I., et al. (2021). Use of a natural herbal-based feed additive containing isoquinoline alkaloids in newborn calves with cryptosporidiosis. Vet. Parasitol. 300, 109615. doi: 10.1016/j.vetpar.2021.109615
Menichetti, F., Moretti, M. V., Marroni, M., Papili, R., Di Candilo, F. (1991). Diclazuril for cryptosporidiosis in AIDS. Am. J. Med. 90 (2), 271–272. doi: 10.1016/0002-9343(91)80174-K
Meuten, D. J., Van Kruiningen, H. J., Lein, D. H. (1974). Cryptosporidiosis in a calf. J. Am. Vet. Med. Assoc. 165 (10), 914–917.
Miao, Y. M., Awad-El-Kariem, F. M., Franzen, C., Ellis, D. S., Muller, A., Counihan, H. M., et al. (2000). Eradication of cryptosporidia and microsporidia following successful antiretroviral therapy. J. Acquir. Immune Defic. Syndr. 25 (2), 124–129. doi: 10.1097/00042560-200010010-00006
Miao, Y. M., Awad-El-Kariem, F. M., Gibbons, C. L., Gazzard, B. G. (1999). Cryptosporidiosis: eradication or suppression with combination antiretroviral therapy? AIDS 13 (6), 734–735. doi: 10.1097/00002030-199904160-00019
Missaye, A., Dagnew, M., Alemu, A., Alemu, A. (2013). Prevalence of intestinal parasites and associated risk factors among HIV/AIDS patients with pre-ART and on-ART attending dessie hospital ART clinic, northeast Ethiopia. AIDS Res. Ther. 10 (1), 7. doi: 10.1186/1742-6405-10-7
Moling, O., Avi, A., Rimenti, G., Mian, P. (2005). Glutamine supplementation for patients with severe cryptosporidiosis. Clin. Infect. Dis. 40 (5), 773–774. doi: 10.1086/427948
Moon, H. W., Bemrick, W. J. (1981). Fecal transmission of calf cryptosporidia between calves and pigs. Vet. Pathol. 18 (2), 248–255. doi: 10.1177/030098588101800213
Moon, H. W., Woode, G. N., Ahrens, F. A. (1982). Attempted chemoprophylaxis of cryptosporidiosis in calves. Vet. Rec 110 (8), 181. doi: 10.1136/vr.110.8.181-a
Moore, D. A., Atwill, E. R., Kirk, J. H., Brahmbhatt, D., Herrera Alonso, L., Hou, L., et al. (2003). Prophylactic use of decoquinate for infections with Cryptosporidium parvum in experimentally challenged neonatal calves. J. Am. Vet. Med. Assoc. 223 (6), 839–845. doi: 10.2460/javma.2003.223.839
Moroni, M., Esposito, R., Cernuschi, M., Franzetti, F., Carosi, G. P., Fiori, G. P. (1993). Treatment of AIDS-related refractory diarrhoea octreotide. Digestion 54 (Suppl. 1), 30–32. doi: 10.1159/000201073
Moskovitz, B. L., Stanton, T. L., Kusmierek, J. J. (1988). Spiramycin therapy for cryptosporidial diarrhoea in immunocompromised patients. J. Antimicrob. Chemother. 22 Suppl B (Supplement_B), 189–191. doi: 10.1093/jac/22.supplement_b.189
Murakoshi, F., Takeuchi, M., Inomata, A., Horimoto, T., Ito, M., Suzuki, Y., et al. (2014). Administration of lasalocid-NA is preventive against cryptosporidiosis of newborn calves. Vet. Rec 175 (14), 353. doi: 10.1136/vr.102508
Murdoch, D. A., Bloss, D. E., Glover, S. C. (1993). Successful treatment of cryptosporidiosis in an AIDS patient with letrazuril. AIDS 7 (9), 1279–1280. doi: 10.1097/00002030-199309000-00026
Naciri, M., Mancassola, R., Reperant, J. M., Canivez, O., Quinque, B., Yvore, P. (1994). Treatment of experimental ovine cryptosporidiosis with ovine or bovine hyperimmune colostrum. Veterinary Parasitol. 53 (3-4), 173–190. doi: 10.1016/0304-4017(94)90181-3
Naciri, M., Mancassola, R., Yvore, P., Peeters, J. E. (1993). The effect of halofuginone lactate on experimental Cryptosporidium parvum infections in calves. Vet. Parasitol. 45 (3-4), 199–207. doi: 10.1016/0304-4017(93)90075-x
Naciri, M., Yvore, P. (1989). Efficiency of halofuginone lactate in the treatment of experimental cryptosporidiosis in lambs. Recueil Medecine Veterinaire 165 (10), 823–826.
Naciri, M., Yvoré, P., Levieux, D. (1984). Cryptosporidiose expérimentale du chevreau. influence de la prise du colostrum. essais de traitements. Les maladies la chèvre. INRA Publications Les colloques l’INRA 28), 465–471.
Nasir, A., Avais, M., Khan, M. S., Khan, J. A., Hameed, S., Reichel, M. P. (2013). Treating Cryptosporidium parvum infection in calves. J. Parasitol. 99 (4), 715–717. doi: 10.1645/12-42.1
Nasrin, D., Blackwelder, W. C., Sommerfelt, H., Wu, Y., Farag, T. H., Panchalingam, S., et al. (2021). Pathogens associated with linear growth faltering in children with diarrhea and impact of antibiotic treatment: The global enteric multicenter study. J. Infect. Dis. 224 (12 Suppl 2), S848–S855. doi: 10.1093/infdis/jiab434
Niine, T., Dorbek-Kolin, E., Lassen, B., Orro, T. (2018). Cryptosporidium outbreak in calves on a large dairy farm: Effect of treatment and the association with the inflammatory response and short-term weight gain. Res. Vet. Sci. 117, 200–208. doi: 10.1016/j.rvsc.2017.12.015
Nime, F. A., Burek, J. D., Page, D. L., Holscher, M. A., Yardley, J. H. (1976). Acute enterocolitis in a human being infected with the protozoan Cryptosporidium. Gastroenterology 70 (4), 592–598. doi: 10.1016/s0016-5085(76)80503-3
Noordeen, F., Faizal, A. C., Rajapakse, R. P., Horadagoda, N. U., Arulkanthan, A. (2001). Excretion of Cryptosporidium oocysts by goats in relation to age and season in the dry zone of Sri Lanka. Vet. Parasitol. 99 (1), 79–85. doi: 10.1016/s0304-4017(01)00449-6
Nord, J., Ma, P., DiJohn, D., Tzipori, S., Tacket, C. O. (1990). Treatment with bovine hyperimmune colostrum of cryptosporidial diarrhea in AIDS patients. AIDS 4 (6), 581–584. doi: 10.1097/00002030-199006000-00015
Nsagha, D. S., Njunda, A. L., Assob, N. J. C., Ayima, C. W., Tanue, E. A., Kibu, O. D., et al. (2016). Intestinal parasitic infections in relation to CD4(+) T cell counts and diarrhea in HIV/AIDS patients with or without antiretroviral therapy in Cameroon. BMC Infect. Dis. 16, 9. doi: 10.1186/s12879-016-1337-1
O'Connor R, M., Shaffie, R., Kang, G., Ward, H. D. (2011). Cryptosporidiosis in patients with HIV/AIDS. AIDS 25 (5), 549–560. doi: 10.1097/QAD.0b013e3283437e88
Oboh, E., Schubert, T. J., Teixeira, J. E., Stebbins, E. E., Miller, P., Philo, E., et al. (2021). Optimization of the urea linker of triazolopyridazine MMV665917 results in a new anticryptosporidial lead with improved potency and predicted hERG safety margin. J. Med. Chem. 64 (15), 11729–11745. doi: 10.1021/acs.jmedchem.1c01136
Okhuysen, P. C., Chappell, C. L., Crabb, J., Valdez, L. M., Douglass, E. T., DuPont, H. L. (1998). Prophylactic effect of bovine anti-Cryptosporidium hyperimmune colostrum immunoglobulin in healthy volunteers challenged with Cryptosporidium parvum. Clin. Infect. Dis. 26 (6), 1324–1329. doi: 10.1086/516374
Ollivett, T. L., Nydam, D. V., Bowman, D. D., Zambriski, J. A., Bellosa, M. L., Linden, T. C., et al. (2009). Effect of nitazoxanide on cryptosporidiosis in experimentally infected neonatal dairy calves. J. Dairy Sci. 92 (4), 1643–1648. doi: 10.3168/jds.2008-1474
Olson, E. J., Epperson, W. B., Zeman, D. H., Fayer, R., Hildreth, M. B. (1998). Effects of an allicin-based product on cryptosporidiosis in neonatal calves. J. Am. Vet. Med. Assoc. 212 (7), 987–990.
Palmieri, F., Cicalini, S., Froio, N., Rizzi, E. B., Goletti, D., Festa, A., et al. (2005). Pulmonary cryptosporidiosis in an AIDS patient: successful treatment with paromomycin plus azithromycin. Int. J. STD AIDS 16 (7), 515–517. doi: 10.1258/0956462054308332
Panciera, R. J., Garner, F. M., Thomassen, R. W. (1971). Cryptosporidial infection in a calf. Veterinary Pathol. 8 (5), 479–47+. doi: 10.1177/0300985871008005-00610
Pantenburg, B., Cabada, M. M., White, A. C., Jr. (2009). Treatment of cryptosporidiosis. Expert Rev. Anti Infect. Ther. 7 (4), 385–391. doi: 10.1586/eri.09.24
Paraud, C., Chartier, C. (2012). Cryptosporidiosis in small ruminants. Small Rumin Res. 103 (1), 93–97. doi: 10.1016/j.smallrumres.2011.10.023
Paraud, C., Pors, I., Chartier, C. (2010). Evaluation of oral tilmicosin efficacy against severe cryptosporidiosis in neonatal kids under field conditions. Vet. Parasitol. 170 (1-2), 149–152. doi: 10.1016/j.vetpar.2010.01.024
Paraud, C., Pors, I., Journal, J. P., Besnier, P., Reisdorffer, L., Chartier, C. (2011). Control of cryptosporidiosis in neonatal goat kids: efficacy of a product containing activated charcoal and wood vinegar liquid (Obionekk) in field conditions. Vet. Parasitol. 180 (3-4), 354–357. doi: 10.1016/j.vetpar.2011.03.022
Pasquali, P., Fayer, R., Zarlenga, D., Canals, A., Marez, T., Gomez Munoz, M. T., et al. (2006). Recombinant bovine interleukin-12 stimulates a gut immune response but does not provide resistance to Cryptosporidium parvum infection in neonatal calves. Vet. Parasitol. 135 (3-4), 259–268. doi: 10.1016/j.vetpar.2005.05.062
Peeters, J. E., Villacorta, I., Naciri, M., Vanopdenbosch, E. (1993). Specific serum and local antibody responses against Cryptosporidium parvum during medication of calves with halofuginone lactate. Infect. Immun. 61 (10), 4440–4445. doi: 10.1128/iai.61.10.4440-4445.1993
Perryman, L. E., Kapil, S. J., Jones, M. L., Hunt, E. L. (1999). Protection of calves against cryptosporidiosis with immune bovine colostrum induced by a Cryptosporidium parvum recombinant protein. Vaccine 17 (17), 2142–2149. doi: 10.1016/s0264-410x(98)00477-0
Petermann, J., Paraud, C., Pors, I., Chartier, C. (2014). Efficacy of halofuginone lactate against experimental cryptosporidiosis in goat neonates. Vet. Parasitol. 202 (3-4), 326–329. doi: 10.1016/j.vetpar.2014.02.027
Petersen, H. H., Jianmin, W., Katakam, K. K., Mejer, H., Thamsborg, S. M., Dalsgaard, A., et al. (2015). Cryptosporidium and Giardia in Danish organic pig farms: Seasonal and age-related variation in prevalence, infection intensity and species/genotypes. Vet. Parasitol. 214 (1-2), 29–39. doi: 10.1016/j.vetpar.2015.09.020
Platts-Mills, J. A., Babji, S., Bodhidatta, L., Gratz, J., Haque, R., Havt, A., et al. (2015). Pathogen-specific burdens of community diarrhoea in developing countries: a multisite birth cohort study (MAL-ED). Lancet Glob Health 3 (9), e564–e575. doi: 10.1016/S2214-109X(15)00151-5
Plettenberg, A., Stoehr, A., Stellbrink, H. J., Albrecht, H., Meigel, W. (1993). A preparation from bovine colostrum in the treatment of HIV-positive patients with chronic diarrhea. Clin. Investig. 71 (1), 42–45. doi: 10.1007/BF00210962
Raabis, S. M., Ollivett, T. L., Cook, M. E., Sand, J. M., McGuirk, S. M. (2018). Health benefits of orally administered anti-IL-10 antibody in milk-fed dairy calves. J. Dairy Sci. 101 (8), 7375–7382. doi: 10.3168/jds.2017-14270
Redman, D. R., Fox, J. E. (1993). “The effect of varying levels of DECCOX® on experimental cryptosporidia infections in Holstein bull calves,” in American Association of Bovine Practitioners Proceedings of the Annual Conference. (Albuquerque, New Mexico: Frontier Printers, Inc.) 157–159.
Robertson, L. J., Björkman, C., Axén, C., Fayer, R. (2014). “Cryptosporidiosis in farmed animals,” in Cryptosporidium: parasite and disease (Vienna: Springer), 149–235.
Romeu, J., Miro, J. M., Sirera, G., Mallolas, J., Arnal, J., Valls, M. E., et al. (1991). Efficacy of octreotide in the management of chronic diarrhoea in AIDS. AIDS 5 (12), 1495–1499. doi: 10.1097/00002030-199112000-00012
Rossignol, J. F. (2006). Nitazoxanide in the treatment of acquired immune deficiency syndrome-related cryptosporidiosis: results of the united states compassionate use program in 365 patients. Aliment Pharmacol. Ther. 24 (5), 887–894. doi: 10.1111/j.1365-2036.2006.03033.x
Rossignol, J. F., Ayoub, A., Ayers, M. S. (2001). Treatment of diarrhea caused by Cryptosporidium parvum: a prospective randomized, double-blind, placebo-controlled study of nitazoxanide. J. Infect. Dis. 184 (1), 103–106. doi: 10.1086/321008
Rossignol, J. F., Hidalgo, H., Feregrino, M., Higuera, F., Gomez, W. H., Romero, J. L., et al. (1998). A double-'blind' placebo-controlled study of nitazoxanide in the treatment of cryptosporidial diarrhoea in AIDS patients in Mexico. Trans. R Soc. Trop. Med. Hyg 92 (6), 663–666. doi: 10.1016/s0035-9203(98)90804-5
Rossignol, J. F., Kabil, S. M., el-Gohary, Y., Younis, A. M. (2006). Effect of nitazoxanide in diarrhea and enteritis caused by cryptosporidium species. Clin. Gastroenterol. Hepatol. 4 (3), 320–324. doi: 10.1016/j.cgh.2005.12.020
Ross, J., Schatz, C., Beaugrand, K., Zuidhof, S., Ralston, B., Allan, N., et al. (2021). Evaluation of activated charcoal as an alternative to antimicrobials for the treatment of neonatal calf diarrhea. Vet. Med. (Auckl) 12, 359–369. doi: 10.2147/VMRR.S337698
Rotte, C., Stejskal, F., Zhu, G., Keithly, J. S., Martin, W. (2001). Pyruvate : NADP+ oxidoreductase from the mitochondrion of Euglena gracilis and from the apicomplexan Cryptosporidium parvum: a biochemical relic linking pyruvate metabolism in mitochondriate and amitochondriate protists. Mol. Biol. Evol. 18 (5), 710–720. doi: 10.1093/oxfordjournals.molbev.a003853
Rump, J. A., Arndt, R., Arnold, A., Bendick, C., Dichtelmuller, H., Franke, M., et al. (1992). Treatment of diarrhoea in human immunodeficiency virus-infected patients with immunoglobulins from bovine colostrum. Clin. Investig. 70 (7), 588–594. doi: 10.1007/BF00184800
Ryan, U. M., Bath, C., Robertson, I., Read, C., Elliot, A., McInnes, L., et al. (2005). Sheep may not be an important zoonotic reservoir for Cryptosporidium and Giardia parasites. Appl. Environ. Microbiol. 71 (9), 4992–4997. doi: 10.1128/AEM.71.9.4992-4997.2005
Ryan, U., Zahedi, A., Feng, Y., Xiao, L. (2021). An update on zoonotic Cryptosporidium species and genotypes in humans. Anim. (Basel) 11 (11), 3307. doi: 10.3390/ani11113307
Saez-Llorens, X., Odio, C. M., Umana, M. A., Morales, M. V. (1989). Spiramycin vs. placebo for treatment of acute diarrhea caused by Cryptosporidium. Pediatr. Infect. Dis. J. 8 (3), 136–140.
Sahal, M., Karaer, Z., Yasa Duru, S., Cizmeci, S., Tanyel, B. (2005). Cryptosporidiosis in newborn calves in Ankara region: clinical, haematological findings and treatment with lasalocid-NA. Dtsch Tierarztl Wochenschr 112 (6), 203–208, 210.
Sallon, S., Deckelbaum, R. J., Schmid, I. I., Harlap, S., Baras, M., Spira, D. T. (1988). Cryptosporidium, malnutrition, and chronic diarrhea in children. Am. J. Dis. Child 142 (3), 312–315. doi: 10.1001/archpedi.1988.02150030086027
Santin, M. (2020). Cryptosporidium and Giardia in ruminants. Vet. Clin. North Am. Food Anim. Pract. 36 (1), 223–238. doi: 10.1016/j.cvfa.2019.11.005
Santín, M., Trout, J. M. (2007). “Livestock,” in Cryptosporidium and cryptosporidiosis. Eds. Fayer, R., Xiao, L. (Boca Raton, FL: CRC Press), 451–484.
Santin, M., Trout, J. M., Fayer, R. (2007). Prevalence and molecular characterization of Cryptosporidium and Giardia species and genotypes in sheep in Maryland. Vet. Parasitol. 146 (1-2), 17–24. doi: 10.1016/j.vetpar.2007.01.010
Santin, M., Trout, J. M., Fayer, R. (2008). A longitudinal study of cryptosporidiosis in dairy cattle from birth to 2 years of age. Vet. Parasitol. 155 (1-2), 15–23. doi: 10.1016/j.vetpar.2008.04.018
Santin, M., Trout, J. M., Xiao, L., Zhou, L., Greiner, E., Fayer, R. (2004). Prevalence and age-related variation of Cryptosporidium species and genotypes in dairy calves. Vet. Parasitol. 122 (2), 103–117. doi: 10.1016/j.vetpar.2004.03.020
Scaglia, M., Atzori, C., Marchetti, G., Orso, M., Maserati, R., Orani, A., et al. (1994). Effectiveness of aminosidine (paromomycin) sulfate in chronic Cryptosporidium diarrhea in AIDS patients: an open, uncontrolled, prospective clinical trial. J. Infect. Dis. 170 (5), 1349–1350. doi: 10.1093/infdis/170.5.1349
Schaefer, D. A., Betzer, D. P., Smith, K. D., Millman, Z. G., Michalski, H. C., Menchaca, S. E., et al. (2016). Novel bumped kinase inhibitors are safe and effective therapeutics in the calf clinical model for cryptosporidiosis. J. Infect. Dis. 214 (12), 1856–1864. doi: 10.1093/infdis/jiw488
Schmidt, W., Wahnschaffe, U., Schafer, M., Zippel, T., Arvand, M., Meyerhans, A., et al. (2001). Rapid increase of mucosal CD4 T cells followed by clearance of intestinal cryptosporidiosis in an AIDS patient receiving highly active antiretroviral therapy. Gastroenterology 120 (4), 984–987. doi: 10.1053/gast.2001.22557
Schnyder, M., Kohler, L., Hemphill, A., Deplazes, P. (2009). Prophylactic and therapeutic efficacy of nitazoxanide against Cryptosporidium parvum in experimentally challenged neonatal calves. Vet. Parasitol. 160 (1-2), 149–154. doi: 10.1016/j.vetpar.2008.10.094
Shaw, H. J., Innes, E. A., Morrison, L. J., Katzer, F., Wells, B. (2020). Long-term production effects of clinical cryptosporidiosis in neonatal calves. Int. J. Parasitol. 50 (5), 371–376. doi: 10.1016/j.ijpara.2020.03.002
Shield, J., Melville, C., Novelli, V., Anderson, G., Scheimberg, I., Gibb, D., et al. (1993). Bovine colostrum immunoglobulin concentrate for cryptosporidiosis in AIDS. Arch. Dis. Child 69 (4), 451–453. doi: 10.1136/adc.69.4.451
Shirley, D. A., Moonah, S. N., Kotloff, K. L. (2012). Burden of disease from cryptosporidiosis. Curr. Opin. Infect. Dis. 25 (5), 555–563. doi: 10.1097/QCO.0b013e328357e569
Sinkala, E., Katubulushi, M., Sianongo, S., Obwaller, A., Kelly, P. (2011). In a trial of the use of miltefosine to treat HIV-related cryptosporidiosis in Zambian adults, extreme metabolic disturbances contribute to high mortality. Ann. Trop. Med. Parasitol. 105 (2), 129–134. doi: 10.1179/136485911X12899838683160
Slacek, B., Ankenbauer-Perkins, K. L., Tunnicliffe, A. (1996). Evaluation of colostrum and whey-derived gamma globulins for prevention of cryptosporidiosis in artificially infected neonatal calves. N Z Vet. J. 44 (4), 158–160. doi: 10.1080/00480169.1996.35962
Smith, N. H., Cron, S., Valdez, L. M., Chappell, C. L., White, A. C., Jr. (1998). Combination drug therapy for cryptosporidiosis in AIDS. J. Infect. Dis. 178 (3), 900–903. doi: 10.1086/515352
Soave, R., Danner, R. L., Honig, C. L., Ma, P., Hart, C. C., Nash, T., et al. (1984). Cryptosporidiosis in homosexual men. Ann. Intern. Med. 100 (4), 504–511. doi: 10.7326/0003-4819-100-4-504
Soave, R., Havlir, D., Lancaster, D., Joseph, P., Leedom, J., Clough, W., et al. (1993). “Azithromycin therapy of AIDS-related cryptosporidial diarrhea: A multi-center, placebo-controlled, double-blind study (abstract 405),” in 33rd Interscience Conference on Antimicrobial Agents and Chemotherapy(New Orleans, LA).
Sprinz, E., Mallman, R., Barcellos, S., Silbert, S., Schestatsky, G., Bem David, D. (1998). AIDS-related cryptosporidial diarrhoea: an open study with roxithromycin. J. Antimicrob. Chemother. 41 Suppl B (suppl 2), 85–91. doi: 10.1093/jac/41.suppl_2.85
Stebbins, E., Jumani, R. S., Klopfer, C., Barlow, J., Miller, P., Campbell, M. A., et al. (2018). Clinical and microbiologic efficacy of the piperazine-based drug lead MMV665917 in the dairy calf cryptosporidiosis model. PloS Negl. Trop. Dis. 12 (1), e0006183. doi: 10.1371/journal.pntd.0006183
Stefańska, B., Sroka, J., Katzer, F., Goliński, P., Nowak, W. (2021). The effect of probiotics, phytobiotics and their combination as feed additives in the diet of dairy calves on performance, rumen fermentation and blood metabolites during the preweaning period. Anim. Feed Sci. Technol. 272, 114738. doi: 10.1016/j.anifeedsci.2020.114738
Swale, C., Bougdour, A., Gnahoui-David, A., Tottey, J., Georgeault, S., Laurent, F., et al. (2019). Metal-captured inhibition of pre-mRNA processing activity by CPSF3 controls Cryptosporidium infection. Sci. Transl. Med. 11 (517), eaax7161. doi: 10.1126/scitranslmed.aax7161
Swinney, D. C., Anthony, J. (2011). How were new medicines discovered? Nat. Rev. Drug Discovery 10 (7), 507–519. doi: 10.1038/nrd3480
Theodos, C. M., Griffiths, J. K., D'Onfro, J., Fairfield, A., Tzipori, S. (1998). Efficacy of nitazoxanide against Cryptosporidium parvum in cell culture and in animal models. Antimicrob. Agents Chemother. 42 (8), 1959–1965. doi: 10.1128/AAC.42.8.1959
Thomson, S., Hamilton, C. A., Hope, J. C., Katzer, F., Mabbott, N. A., Morrison, L. J., et al. (2017). Bovine cryptosporidiosis: impact, host-parasite interaction and control strategies. Vet. Res. 48 (1), 42. doi: 10.1186/s13567-017-0447-0
Tomczak, E., McDougal, A. N., White, A. C., Jr. (2022). Resolution of cryptosporidiosis in transplant recipients: Review of the literature and presentation of a renal transplant patient treated with nitazoxanide, azithromycin, and rifaximin. Open Forum Infect. Dis. 9 (1), ofab610. doi: 10.1093/ofid/ofab610
Trad, O., Jumaa, P., Uduman, S., Nawaz, A. (2003). Eradication of Cryptosporidium in four children with acute lymphoblastic leukemia. J. Trop. Pediatr. 49 (2), 128–130. doi: 10.1093/tropej/49.2.128
Troeger, C., Blacker, B. F., Khalil, I. A., Rao, P. C., Cao, S. J., Zimsen, S. R. M., et al. (2018). Estimates of the global, regional, and national morbidity, mortality, and aetiologies of diarrhoea in 195 countries: a systematic analysis for the global burden of disease study 2016. Lancet Infect. Dis. 18 (11), 1211–1228. doi: 10.1016/S1473-3099(18)30362-1
Trotz-Williams, L. A., Jarvie, B. D., Peregrine, A. S., Duffield, T. F., Leslie, K. E. (2011). Efficacy of halofuginone lactate in the prevention of cryptosporidiosis in dairy calves. Vet. Rec 168 (19), 509. doi: 10.1136/vr.d1492
Trotz-Williams, L. A., Wayne Martin, S., Leslie, K. E., Duffield, T., Nydam, D. V., Peregrine, A. S. (2007). Calf-level risk factors for neonatal diarrhea and shedding of Cryptosporidium parvum in Ontario dairy calves. Prev. Vet. Med. 82 (1-2), 12–28. doi: 10.1016/j.prevetmed.2007.05.003
Tuli, L., Gulati, A. K., Sundar, S., Mohapatra, T. M. (2008). Correlation between CD4 counts of HIV patients and enteric protozoan in different seasons - an experience of a tertiary care hospital in varanasi (India). BMC Gastroenterol. 8, 36. doi: 10.1186/1471-230X-8-36
Tyzzer, E. E. (1907). A sporozoan found in the peptic glands of the common mouse. Exp. Biol. Med. 5 (1), 12–13. doi: 10.3181/00379727-5-5
Tyzzer, E. E. (1910). An extracellular coccidium, Cryptosporidium muris (Gen. et sp. nov.), of the gastric glands of the common mouse. J. Med. Res. 23 487-510 (3), 483.
Tyzzer, E. E. (1912). Cryptosporidium parvum (sp. nov.), a coccidium found in the small intestine of the common mouse. Arch. Protistenkd 26, 394–412.
Tzipori, S., Campbell, I., Sherwood, D., Snodgrass, D. R., Whitelaw, A. (1980). An outbreak of calf diarrhoea attributed to cryptosporidial infection. Vet. Rec 107 (25-26), 579–580.
Tzipori, S., Rand, W., Griffiths, J., Widmer, G., Crabb, J. (1994). Evaluation of an animal model system for cryptosporidiosis: therapeutic efficacy of paromomycin and hyperimmune bovine colostrum-immunoglobulin. Clin. Diagn. Lab. Immunol. 1 (4), 450–463. doi: 10.1128/cdli.1.4.450-463.1994
Tzipori, S., Roberton, D., Cooper, D. A., White, L. (1987). Chronic cryptosporidial diarrhoea and hyperimmune cow colostrum. Lancet 2 (8554), 344–345. doi: 10.1016/s0140-6736(87)90944-5
Tzipori, S., Smith, M., Halpin, C., Angus, K. W., Sherwood, D., Campbell, I. (1983). Experimental cryptosporidiosis in calves: clinical manifestations and pathological findings. Vet. Rec 112 (6), 116–120. doi: 10.1136/vr.112.6.116
Uip, D. E., Lima, A. L., Amato, V. S., Boulos, M., Neto, V. A., Bem David, D. (1998). Roxithromycin treatment for diarrhoea caused by Cryptosporidium spp. in patients with AIDS. J. Antimicrob. Chemother. 41 Suppl B (suppl 2), 93–97. doi: 10.1093/jac/41.suppl_2.93
Ungar, B. L., Ward, D. J., Fayer, R., Quinn, C. A. (1990). Cessation of Cryptosporidium-associated diarrhea in an acquired immunodeficiency syndrome patient after treatment with hyperimmune bovine colostrum. Gastroenterology 98 (2), 486–489. doi: 10.1016/0016-5085(90)90842-o
Urie, N. J., Lombard, J. E., Shivley, C. B., Adams, A. E., Kopral, C. A., Santin, M. (2018a). Preweaned heifer management on US dairy operations: Part III. factors associated with Cryptosporidium and Giardia in preweaned dairy heifer calves. J. Dairy Sci. 101 (10), 9199–9213. doi: 10.3168/jds.2017-14060
Urie, N. J., Lombard, J. E., Shivley, C. B., Kopral, C. A., Adams, A. E., Earleywine, T. J., et al. (2018b). Preweaned heifer management on US dairy operations: Part i. descriptive characteristics of preweaned heifer raising practices. J. Dairy Sci. 101 (10), 9168–9184. doi: 10.3168/jds.2017-14010
Vandenberg, O., Robberecht, F., Dauby, N., Moens, C., Talabani, H., Dupont, E., et al. (2012). Management of a Cryptosporidium hominis outbreak in a day-care center. Pediatr. Infect. Dis. J. 31 (1), 10–15. doi: 10.1097/INF.0b013e318235ab64
Van Voorhis, W. C., Hulverson, M. A., Choi, R., Huang, W., Arnold, S. L. M., Schaefer, D. A., et al. (2021). One health therapeutics: Target-based drug development for cryptosporidiosis and other apicomplexa diseases. Vet. Parasitol. 289, 109336. doi: 10.1016/j.vetpar.2020.109336
Vargas, S. L., Shenep, J. L., Flynn, P. M., Pui, C. H., Santana, V. M., Hughes, W. T. (1993). Azithromycin for treatment of severe Cryptosporidium diarrhea in two children with cancer. J. Pediatr. 123 (1), 154–156. doi: 10.1016/s0022-3476(05)81562-8
Velez, J., Lange, M. K., Zieger, P., Yoon, I., Failing, K., Bauer, C. (2019). Long-term use of yeast fermentation products in comparison to halofuginone for the control of cryptosporidiosis in neonatal calves. Vet. Parasitol. 269, 57–64. doi: 10.1016/j.vetpar.2019.04.008
Velez, J., Velasquez, Z., Silva, L. M. R., Gartner, U., Failing, K., Daugschies, A., et al. (2021). Metabolic signatures of Cryptosporidium parvum-infected HCT-8 cells and impact of selected metabolic inhibitors on C. parvum infection under physioxia and hyperoxia. Biol. (Basel) 10 (1), 1–24. doi: 10.3390/biology10010060
Vermeulen, L. C., Benders, J., Medema, G., Hofstra, N. (2017). Global Cryptosporidium loads from livestock manure. Environ. Sci. Technol. 51 (15), 8663–8671. doi: 10.1021/acs.est.7b00452
Viel, H., Rocques, H., Martin, J., Chartier, C. (2007). Efficacy of nitazoxanide against experimental cryptosporidiosis in goat neonates. Parasitol. Res. 102 (1), 163–166. doi: 10.1007/s00436-007-0744-z
Villacorta, I., Peeters, J. E., Vanopdenbosch, E., Ares-Mazas, E., Theys, H. (1991). Efficacy of halofuginone lactate against Cryptosporidium parvum in calves. Antimicrob. Agents Chemother. 35 (2), 283–287. doi: 10.1128/AAC.35.2.283
Vinayak, S., Jumani, R. S., Miller, P., Hasan, M. M., McLeod, B. I., Tandel, J., et al. (2020). Bicyclic azetidines kill the diarrheal pathogen Cryptosporidium in mice by inhibiting parasite phenylalanyl-tRNA synthetase. Sci. Transl. Med. 12 (563), eaba8412–eaba8412. doi: 10.1126/scitranslmed.aba8412
Vinayak, S., Pawlowic, M. C., Sateriale, A., Brooks, C. F., Studstill, C. J., Bar-Peled, Y., et al. (2015). Genetic modification of the diarrhoeal pathogen Cryptosporidium parvum. Nature 523 (7561), 477–480. doi: 10.1038/nature14651
Viu, M., Quilez, J., Sanchez-Acedo, C., del Cacho, E., Lopez-Bernad, F. (2000). Field trial on the therapeutic efficacy of paromomycin on natural Cryptosporidium parvum infections in lambs. Vet. Parasitol. 90 (3), 163–170. doi: 10.1016/s0304-4017(00)00241-7
Volpato, A., Crecencio, R. B., Tomasi, T., Galli, G. M., Griss, L. G., Da Silva, A. D., et al. (2019). Phytogenic as feed additive for suckling dairy calves' has a beneficial effect on animal health and performance. Anais Da Academia Bras. Cienc. 91 (4), e20180747. doi: 10.1590/0001-3765201920180747
Wallace, M. R., Nguyen, M. T., Newton, J. A., Jr. (1993). Use of paromomycin for the treatment of cryptosporidiosis in patients with AIDS. Clin. Infect. Dis. 17 (6), 1070–1071. doi: 10.1093/clinids/17.6.1070
Wang, C. C. (1976). Inhibition of the respiration of Eimeria tenella by quinolone coccidiostats. Biochem. Pharmacol. 25 (3), 343–349. doi: 10.1016/0006-2952(76)90225-2
Wang, R. J., Li, J. Q., Chen, Y. C., Zhang, L. X., Xiao, L. H. (2018). Widespread occurrence of Cryptosporidium infections in patients with HIV/AIDS: Epidemiology, clinical feature, diagnosis, and therapy. Acta Trop. 187, 257–263. doi: 10.1016/j.actatropica.2018.08.018
Watanabe, Y., Yang, C. H., Ooi, H. K. (2005). Cryptosporidium infection in livestock and first identification of Cryptosporidium parvum genotype in cattle feces in Taiwan. Parasitol. Res. 97 (3), 238–241. doi: 10.1007/s00436-005-1428-1
Watarai, S., Tana, Koiwa, M. (2008). Feeding activated charcoal from bark containing wood vinegar liquid (Nekka-rich) is effective as treatment for cryptosporidiosis in calves. J. Dairy Sci. 91 (4), 1458–1463. doi: 10.3168/jds.2007-0406
Weikel, C., Lazenby, A., Belitsos, P., McDewitt, M., Fleming, H. E., Jr., Barbacci, M. (1991). Intestinal injury associated with spiramycin therapy of Cryptosporidium infection in AIDS. J. Protozool 38 (6), 147S.
Weyl-Feinstein, S., Markovics, A., Eitam, H., Orlov, A., Yishay, M., Agmon, R., et al. (2014). Short communication: effect of pomegranate-residue supplement on Cryptosporidium parvum oocyst shedding in neonatal calves. J. Dairy Sci. 97 (9), 5800–5805. doi: 10.3168/jds.2013-7136
White, A. C., Jr., Chappell, C. L., Hayat, C. S., Kimball, K. T., Flanigan, T. P., Goodgame, R. W. (1994). Paromomycin for cryptosporidiosis in AIDS: a prospective, double-blind trial. J. Infect. Dis. 170 (2), 419–424. doi: 10.1093/infdis/170.2.419
WHO (2009) Risk assessment of cryptosporidium in drinking water. Available at: https://www.who.int/publications/i/item/WHO-HSE-WSH-09.04 (Accessed October 26 2022).
Wilke, G., Funkhouser-Jones, L. J., Wang, Y., Ravindran, S., Wang, Q., Beatty, W. L., et al. (2019). A stem-Cell-Derived platform enables complete Cryptosporidium development In vitro and genetic tractability. Cell Host Microbe 26 (1), 123–134 e128. doi: 10.1016/j.chom.2019.05.007
Wilke, G., Wang, Y., Ravindran, S., Stappenbeck, T., Witola, W. H., Sibley, L. D. (2020). “In vitro culture of cryptosporidium parvum using stem cell-derived intestinal epithelial monolayers,” in Methods in molecular biology (New York: Springer), 351–372.
Witchel, H. J. (2011). Drug-induced hERG block and long QT syndrome. Cardiovasc. Ther. 29 (4), 251–259. doi: 10.1111/j.1755-5922.2010.00154.x
Witola, W. H., Zhang, X., Kim, C. Y. (2017). Targeted gene knockdown validates the essential role of lactate dehydrogenase in Cryptosporidium parvum. Int. J. Parasitol. 47 (13), 867–874. doi: 10.1016/j.ijpara.2017.05.002
Wittenberg, D. F., Miller, N. M., van den Ende, J. (1989). Spiramycin is not effective in treating cryptosporidium diarrhea in infants: results of a double-blind randomized trial. J. Infect. Dis. 159 (1), 131–132. doi: 10.1093/infdis/159.1.131
Woolf, G. M., Townsend, M., Guyatt, G. (1987). Treatment of cryptosporidiosis with spiramycin in AIDS. an "N of 1" trial. J. Clin. Gastroenterol. 9 (6), 632–634. doi: 10.1097/00004836-198712000-00005
Wright, S. E., Coop, R. L. (2007). “Cryptosporidiosis and coccidiosis,” in Diseases of sheep (Ames, IA, USA: Blackwell Publishing), 179–185.
Xiao, L., Fayer, R., Ryan, U., Upton, S. J. (2004). Cryptosporidium taxonomy: recent advances and implications for public health. Clin. Microbiol. Rev. 17 (1), 72–97. doi: 10.1128/CMR.17.1.72-97.2004
Zahedi, A., Ryan, U. (2020). Cryptosporidium - an update with an emphasis on foodborne and waterborne transmission. Res. Vet. Sci. 132, 500–512. doi: 10.1016/j.rvsc.2020.08.002
Zhang, X., Kim, C. Y., Worthen, T., Witola, W. H. (2018). Morpholino-mediated in vivo silencing of Cryptosporidium parvum lactate dehydrogenase decreases oocyst shedding and infectivity. Int. J. Parasitol. 48 (8), 649–656. doi: 10.1016/j.ijpara.2018.01.005
Zulu, I., Kelly, P., Njobvu, L., Sianongo, S., Kaonga, K., McDonald, V., et al. (2005). Nitazoxanide for persistent diarrhoea in Zambian acquired immune deficiency syndrome patients: a randomized-controlled trial. Aliment Pharmacol. Ther. 21 (6), 757–763. doi: 10.1111/j.1365-2036.2005.02394.x
Keywords: Cryptosporidium, cryptosporidiosis, treatment, prevention, drug discovery, protozoa, diarrhea
Citation: Khan SM and Witola WH (2023) Past, current, and potential treatments for cryptosporidiosis in humans and farm animals: A comprehensive review. Front. Cell. Infect. Microbiol. 13:1115522. doi: 10.3389/fcimb.2023.1115522
Received: 04 December 2022; Accepted: 09 January 2023;
Published: 24 January 2023.
Edited by:
Sudhir Kumar, Seattle Children’s Research Institute, United StatesReviewed by:
Franziska Dengler, University of Veterinary Medicine Vienna, AustriaPraveen Kumar, Institute of Medical Sciences, Banaras Hindu University, India
Copyright © 2023 Khan and Witola. This is an open-access article distributed under the terms of the Creative Commons Attribution License (CC BY). The use, distribution or reproduction in other forums is permitted, provided the original author(s) and the copyright owner(s) are credited and that the original publication in this journal is cited, in accordance with accepted academic practice. No use, distribution or reproduction is permitted which does not comply with these terms.
*Correspondence: William H. Witola, d2h3aXQzNUBpbGxpbm9pcy5lZHU=