- Department of Rehabilitation Medicine, The Affiliated Suzhou Hospital of Nanjing Medical University, Suzhou Municipal Hospital, Gusu School, Nanjing Medical University, Suzhou, Jiangsu, China
As the most common type of stroke, ischemic stroke, also known as cerebral infarction (CI), with its high mortality and disability rate, has placed a huge burden on social economy and public health. Treatment methods for CI mainly include thrombectomy, thrombolysis, drug therapy, and so on. However, these treatments have certain timeliness and different side effects. In recent years, the gut-brain axis has become a hot topic, and its role in nervous system diseases has been confirmed by increasing evidences. The intestinal microbiota, as an important part of the gut-brain axis, has a non-negligible impact on the progression of CI through mechanisms such as inflammatory response and damage-associated molecular patterns, and changes in the composition of intestinal microbiota can also serve as the basis for predicting CI. At the same time, the diagnosis of CI requires more high-throughput techniques, and the analysis method of metabolomics just fits this demand. This paper reviewed the changes of intestinal microbiota in patients within CI and the effects of the intestinal microbiota on the course of CI, and summarized the therapeutic methods of the intervention with the intestinal microbiota. Furthermore, metabolic changes of CI patients were also discussed to reveal the molecular characteristics of CI and to elucidate the potential pathologic pathway of its interference.
1. Introduction
Cerebral infarction (CI) is caused by vascular occlusion or arterial stenosis, which is clinically defined as brain tissue injury caused by insufficient blood supply in specific areas, resulting in permanent neuronal damage or even death (Benjamin et al., 2018). CI-induced brain injury is the result of a series of complex neuropathophysiological and neuropathological events, including excitotoxicity, oxidative stress, neuroinflammation, apoptosis, amyloid production, and so on (Kowalski and Mulak, 2019; Pluta et al., 2020; Radenovic et al., 2020). It has been proved that up to 90% of CI cases are related to behavioral factors, including malnutrition, insufficient exercise, smoking and alcohol abuse, and metabolic factors such as diabetes, obesity, hyperlipidemia and hypertension (Li et al., 2019). Identifying potential risk factors and potential pathogenesis that influence the prognosis of CI is of great importance to improve the management and treatment of CI.
The gut-brain axis is a two-way communication system between the brain and the intestine, which regulates intestinal homeostasis and the central nervous system through the neural network, neuroendocrine, immune and inflammatory pathways (Luan et al., 2019; Shaik et al., 2020). Several studies have shown that the gut-brain axis plays an important role in various neurological diseases such as Parkinson’s disease (PD), Alzheimer’s disease (AD), and cerebrovascular diseases (Caputi and Giron, 2018; Megur et al., 2020). Gut microbiota and microbial derived metabolites play a key role in brain function by regulating the gut-brain axis signaling pathway. Recently, it has been shown that intestinal flora imbalance can cause CI and affect the prognosis of CI through inflammatory response and translocation of microorganisms and metabolites caused by intestinal leakage (Chidambaram et al., 2022; Wang et al., 2022a). By exploring the relationship between intestinal microbiota and the pathogenesis and prognosis of CI, feasible strategies will be developed for the prevention and treatment of CI.
The clinical diagnosis of CI is based on neurovascular imaging data or computed tomography, magnetic resonance imaging, ultrasound and angiography (Birenbaum et al., 2011). Unfortunately, incorrect imaging can lead to errors or missed diagnosis, causing delays in receiving adequate treatment and an increased risk of recurrence of CI (Mendelson and Prabhakaran, 2021). Therefore, new biomarker-based tests are still needed to rapidly and accurately diagnose and differentiate CI. Metabolite disturbance is considered to be a key event leading to CI (Floegel et al., 2018). As one of the important components of system biology, metabolomics, together with genomics, transcriptomics, and proteomics, will explain the whole process of life from microscopic DNA molecules to the secretion of small molecule metabolites (Wishart, 2019). Metabolomics can detect the changes of small molecule metabolites after organisms are disturbed, and find their types, quantities and their changing rules (Newgard, 2017). The emergence of this new analytical technique will provide a way to identify key metabolic biomarkers with potential diagnostic and prognostic value in CI.
In this review, the changes of gut microbiota in CI and the mechanism of how they influence the occurrence and development of CI were reviewed, and the literature on human metabolomics in CI, especially amino acid and lipid, was also summarized, aiming to provide new ideas for the potential mechanism and treatment of CI.
2. Cerebral infarction
As the second leading cause of death and a major cause of disability worldwide, stroke poses a significant threat to public health (Feigin et al., 2014; Feigin et al., 2017). CI, also known as ischemic stroke (IS), refers to the ischemic necrosis or softening of localized brain tissue caused by a blockage of the blood supply to the brain. CI is the most common clinical type of stroke, accounting for approximately 70%~80%. The mortality rate of CI is about 10%~15%, and the disability rate is high and can be recurrent (Katan and Luft, 2018). According to a 2019 study, there were 13.7 million new CI cases worldwide in 2016, 40% of which were in China. China accounts for 30% of the 5.5 million deaths from CI (Wu et al., 2019).
CI can cause different degrees and types of brain damage, including brain tissue lesions and structural damage, as well as neuronal death and defects (DeLong et al., 2022; Walter, 2022). Patients with disorders including AD, motor abnormalities, low intelligence quotient scores, and numerous cognitive deficiencies have been reported to have clinical symptoms of these sorts of impairments (de Montferrand et al., 2019; Waziry et al., 2020). The recanalization of obstructed arteries and restoration of cerebral blood flow are the ultimate therapeutic goals in the treatment of CI in effort to minimize neuronal damage (Prabhakaran et al., 2015). Nowadays, the most effective treatment for acute CI (ACI) is thrombectomy and thrombolysis (Jolugbo and Ariëns, 2021; DeLong et al., 2022). Although thrombectomy and thrombolysis are effective, not all patients benefit from them without significant side effects and they have high time requirements to take effect (Varona, 2010). Therefore, the diagnosis and treatment of CI require more effective schemes, and more evidence has shown that the role of the gut-brain axis in the diagnosis and treatment of CI needs further exploration.
3. Gut microbiome and cerebral infarction
As one of the largest interfaces between the host, the environment, and human antigens, the human gastrointestinal tract contains three types of microorganisms: bacteria, archaea, and eukaryotes. The gut microbiota participates in the operation of the human digestive system, endocrine system, circulatory system, neuroimmune system, and other processes, and plays a vital role in human health and disease processes (Fung et al., 2017; Scheithauer et al., 2020; Wu et al., 2020; Qi et al., 2021; Wu et al., 2022a). Under normal physiological conditions, the gut microbiota maintains a relatively stable state to ensure the health of the body (Wang et al., 2022b). However, changes in the internal and external environment of the body will trigger the imbalance of intestinal microbial flora, which may affect the metabolic and immune response of the host, which can further lead to gastrointestinal dysfunction and various extra-gastrointestinal diseases (de Vos et al., 2022).
In recent years, the concept of brain-gut axis has been proposed, pointing out that the brain-gut axis is a two-way neural pathway connecting the brain, central nervous system (CNS), enteric nervous system, and autonomic nervous system (Luan et al., 2019). After the brain integrates the relevant external information and the body, it can act directly on the smooth muscle cells of the gastrointestinal tract, or transmit regulatory information to the neural plexus of the gastrointestinal tract along the autonomic nerve and neuroendocrine system, so as to perform the operation of the gut-brain axis (Agirman et al., 2021; Mayer et al., 2022). Intestinal microbial flora imbalance has been implicated to the onset and progression of several neurological diseases, including PD, AD, multiple sclerosis, depression, and so on, according to an increasing number of studies (Sun and Shen, 2018; Simpson et al., 2021; Chen et al., 2022; Xie et al., 2023). More research is also pointing to the pivotal function gut microbiota plays in CI.
3.1. Changes of gut microbiota in cerebral infarction
CI is closely related to changes in the composition of the intestinal microbiota (Table 1). Analysis of the proportion of various components of the intestinal microbiota in patients is helpful in identifying possible symptoms of CI as early as possible. Yamashiro et al. analyzed the fecal gut microbiota composition of CI patients and control subjects. The results showed that CI was closely associated with an increase in Atopobium cluster and Lactobacillus ruminis, and a decrease in the Lactobacillus sakei subgroup (Yamashiro et al., 2017). A prospective observational study of short-chain fatty acid (SCFA) profiles in patients with ACI in China showed that SCFAs-producing bacteria (Roseburia, Bacteroides, Lachnospiraceae, Faecalibacterium, Blautia, and Anaerostipes) were less abundant in ACI patients while Lactobacillaceae, Akkermansia, Enterobacteriaceae, and Porphyromonadaceae were overgrown, which reflected the dysregulation of the intestinal microbiota in ACI patients (Tan et al., 2021). However, another study showed the opposite. The analysis of the gut microbiota of ACI patients and healthy controls by Li et al. showed an increase in SCFAs producing bacteria in patients, including Odoribacter, Akkermansia, Ruminococcaceae_UCG_005, and Victivallis (Li et al., 2019).
Atherosclerosis is a possible cause of CI. The gut microbiota analysis of patients with atherosclerotic plaque who had undergone carotid endovascular resection for mild CI, transient ischemic attack, or transient amaurosis leaurosis showed that Collinsella enriched in symptomatic atherosclerotic plaque, while Eubacterium and Roseburia decreased. Patients’ metagenome is rich in genes associated with peptidoglycan biosynthesis, suggesting that intestinal metagenomic increased peptidoglycan production may promote symptomatic atherosclerosis by primes the innate immune system and enhances neutrophilic function (Karlsson et al., 2012). Although studies have demonstrated a relationship between clot histology and CI, few cases involving Candida have been reported. Clot analysis of a patient with ACI showed a notable presence of Candida albicans (Walker et al., 2019).
3.2. Possible mechanisms by which gut microbiota alters cerebral infarction
The inflammatory response to sterile tissue injury is a key pathophysiology of organ-specific injury, including CI (Chamorro et al., 2012). Previous studies have shown that pro-inflammatory Th1, Th17, and γδ T cells are associated with increased inflammatory damage and poor prognosis, while Treg cells suppress neuroinflammatory responses to brain injury (Gelderblom et al., 2012; Liesz et al., 2013). The evidence suggested that the gut microbiota is a key regulator of T cell homeostasis and is closely related to the maturation of the immune system and the coexistence of the maintenance host and microbes. Benakis et al. showed that intestinal dysbiosis affects Treg and IL-17 γδT cells and found that intestinal lymphocyte migration to the ischemic brain may be associated with increased infarct volume, while antibiotic-induced changes in the intestinal microbiota significantly reduced ischemic brain injury in mice (Benakis et al., 2016). Singh et al. observed dysbiosis of the gut microbiota by pressure-mediated intestinal palsy after CI, which in turn was causally associated with changes in T cell homeostasis, induction of the pro-inflammatory response, and worsening of stroke outcomes. Fecal microbiota transplantation (FMT) will contribute to improved stroke outcomes (Singh et al., 2016).
In addition, gut-derived damage-associated molecular patterns (DAMPs) and cytokine storms may affect the outcome of CI by regulating CNS antigen specific immune response. Tascilar et al. found that some intestinal bacteria in a mouse model of occlusion of the middle cerebral artery were translocated from the gut to the blood prior to the onset of the symptoms of CI. In addition, intestinal bacteria were transferred from the gut to the testes and other organs after CI in mice, and systemic inflammatory reactions occurred in some models later, causing post-stroke infection. However, the exact mechanism involved in this process remains unclear and needs further study (Tascilar et al., 2010). The gut microbiota of young mice with CI was altered, and in aged mice, the ratio of the two main bacterial phylums Firmicutes to Bacteroides phylum (F:B) increased approximately 9-fold compared to young mice, indicating dysbiosis. After altering the microbiota of young mice by fecal transplant gavage to increase their F:B ratio by about 6 times, cytokine levels were found to be significantly increased (Spychala et al., 2018). As a result, intestinal dysbiosis can create a vicious pro-inflammatory cycle that reduces the prognosis after stroke.
3.3. Therapeutic potentials of gut microbiota in cerebral infarction
Regulation of the gut microbiota by antibiotics is a proven strategy, which can remove or prevent bacterial colonization in the human body, greatly affect the composition of the gut microbiota and reduce its biodiversity (Angelucci et al., 2019). The feasibility of alleviating CI by regulating intestinal microbial imbalance through antibiotics is being verified by various studies. Benakis et al. treated male mice with either a combination of antibiotics or a single antibiotic, respectively, and found that mice treated with a combination of antibiotics showed a significant reduction in infarct volume during the acute phase of stroke, while a single antibiotic treatment with ampicillin or vancomycin also reduced infarct volume and improved motor sensory function within 3 days after stroke (Benakis et al., 2020). Chen et al. applied antibiotics to CI rats, which decreased the α diversity of the intestinal microbiome, infarcted volume, and significantly increased acetic acid and valeric acid levels in ischemic rats (Chen et al., 2019). However, to date, there is no clear evidence to support prophylactic antibiotic therapy in the first hours after ACI to control ecological dissonance (Ulm et al., 2017). Two large randomized controlled phase III clinical trials did not show an improvement in results after ACI with prophylactic administration, and according to the results, antibiotics are not recommended for the prevention of CI (Kalra et al., 2015; Westendorp et al., 2015).
FMT is defined as the transfer of healthy gut bacteria via donor stool to a patient, aiming to obtain therapeutic benefits by directly altering or normalizing the gut microbiota of recipients (Khoruts and Sadowsky, 2016). Recent studies have confirmed FMT as a possible strategy to regulated intestinal ecological disorders in patients with neuropsychiatric disorders and patients affected by CI (Evrensel and Ceylan, 2016). Wang et al. performed FMT in a CI mouse model to verify the influence of different sexes microbiomes on the prognosis of CI. The results showed increased survival, reduced infarct size, improved performance in behavioral tests, increased release of beneficial metabolites, and reduced levels of inflammation in mice receiving the female gut microbiome. In contrast, mice that received the male microbiome were less effective in preventing brain damage and restoring neural function (Wang et al., 2022c). Chen et al. showed that FMT intervention significantly changed the intestinal microbiome composition of CI, reduced pathogenic bacteria and increased beneficial bacteria, and thus reduced neurological function damage, eliminated cerebral edema and reduced infarct volume. Further studies have shown that regulating SCFA levels such as isobutyric acid, butyric acid, and isovalerate may be the mechanism by which FMT alleviates CI (Chen et al., 2019).
The benefits of probiotics on host health have been extensively studied and clearly defined (Hill et al., 2014). Several studies have confirmed the efficacy and mechanism of probiotics in preventing CI by regulating the composition of the gut microbiota, improving intestinal barrier function, and regulating local and systemic immunity. Rodent models have verified the roles of Lactobacillus, Clostridium butyricum, and Bacillus licheniformis in CI (Fang et al., 2022). Through the regulation of TLR-4/NF-kappa B signaling, Lactobacillus reduced the extent of cerebral infarction, lowered oxidative stress, and blocked the death of brain cells, which improved neurobehavioral scores (Wanchao et al., 2018). After pretreatment with C. butyricum, butyrate content in the brain increases significantly, regulating CNS function and alleviating CI (Sun et al., 2016). “Synbiotics” is a combination of probiotics and matrices (Swanson et al., 2020). As opposed to inulin alone or SCFA-producing bacteria alone, Lee et al. discovered a synergistic impact of inulin and SCFA-producing bacteria, which improved neurodeficiency scores and behavioral outcomes in mice following stroke (Lee et al., 2020).
4. Metabolomics and cerebral infarction
Metabolomics is one of the important components of system biology, which is the science of studying the type, quantity and change law of metabolites after an organism is perturbed (Johnson et al., 2016; Wu et al., 2022b). Metabolomics can reflect a series of biological events that occur in a pathophysiological process by revealing the trajectory of the overall metabolism under the influence of internal and external factors (Tian et al., 2022; Xiang et al., 2022). Studying CI through metabolomics can reveal its molecular signature and elucidate potential pathological pathways in which diseases are disturbed (Table 2).
4.1. Amino acid metabolism and cerebral infarction
At the cellular level, excitotoxicity is a key cellular mechanism for cerebral ischemic injury (Rothman and Olney, 1986). This is triggered by metabolic homeostasis failure and secreted metabolites including glutamate, glycine, D-serine, and polyamines. Significant glutamate accumulation and down-regulation of glutamine ratios have been shown in ACI mouse models and in patients with cardiovascular diseases, including CI (Kimberly et al., 2013; Zheng et al., 2016).
Branched chain amino acids (BCAAs) are components of the glutamate/glutamine cycle between astrocytes and neurons and are essential for signaling in excitatory neurons (Salcedo et al., 2021). Metabolomic analysis of plasma in patients with mild and moderate ACI has found that reduced concentrations of BCAAs, including leucine, isoleucine, and valine, are associated with stroke severity and a worse prognosis (Kimberly et al., 2013). Wang et al. obtained the serum metabolic profile of stroke patients using the untargeted metabonomic method, and found that there were metabolic disorders in patients with ACI. Compared to HCs, the levels of 4-hydroxyproline, L-glutamine, L-arginine, and L-proline in patients with ACI were lower, revealing an increased risk of cerebrovascular diseases (Wang et al., 2020). Hu et al. directed injection liquid crystal mass spectrometry analysis of dried blood spots in patients with CI showed a significant increase in the ratio of tyrosine, citrulline, and proline, which also implied a decrease in neuronal autophagy, apoptosis, and platelet dysfunction (Hu et al., 2016).
4.2. Lipid metabolism and cerebral infarction
As a subset of metabolomics, lipidomics shows functions similar to metabolomics (Lam et al., 2017). In recent years, the analysis of lipids has been greatly improved by matrix-assisted laser mass spectrometry and other techniques (Adibhatla et al., 2006). Several studies have shown that lipids play an important role in the occurrence and development of diseases. Lipids cross the blood-brain barrier more easily than proteins and enter brain cells, and the high abundance of polyunsaturated fatty acids in brain lipids makes the brain more susceptible to oxidative stress than most tissues and organs (Hamilton et al., 2007).
Animal models and human studies related to CI have shown that abnormal lipid metabolism is closely related to the prediction and prognosis of CI, including phospholipids, sphingolipids (SLs), and glycerides (Ye et al., 2022). Sheth et al. measured and evaluated the changes in plasma SL concentration in the mouse model of ACI, and the results showed that SLs were highly enriched in the brain, among which the most obvious changes were ceramide and sphingomyelin (SM), and the concentration of multiple SLs in the brain and plasma differed by more than 1000 times. The feasibility of this targeted lipid analysis was verified in patients with ACI (Sheth et al., 2015). Human serum metabolomics based on nuclear magnetic resonance was performed on blood phosphorus in patients with CI, and it was found that serum phosphatidylcholine, phosphoethanolamine and SM levels were lower in CI patients compared with healthy individuals (Grandizoli et al., 2014). Therefore, phosphorus-containing compounds in human serum can be considered important biomarkers in CI research.
4.3. Other metabolites and cerebral infraction
Oxidative stress in cerebral ischemia is caused by the excessive production of oxygen derivatives and metabolic dysfunction. Stroke causes heterogeneous changes in tissue oxygenation and produces lactic acid as the end product, thus making the cytoplasmic environment acidic (Liu et al., 2004). Excess protons convert oxygen into hydrogen peroxide and reactive hydroxyl radicals (Shin et al., 2020). Jung et al. found that the levels of lactic acid, pyruvate, glycolic acid and formate in plasma and urine of patients with cerebral ischemia increased, while the levels of glutamine and methanol decreased, which reflected the oxidative stress state of cerebral ischemia (Jung et al., 2011).
In individuals with cerebral ischemia, oxidative stress and blood-brain barrier damage can also aggravate the inflammatory response (Sidorov et al., 2019). Glial cells, neutrophils, monocytes, and lymphocytes are seen in higher concentrations with the activation of inflammation, along with pro-inflammatory cytokines and metabolites that are connected to inflammation (Shin et al., 2020). The increase of C4/C2 could indicate the deficiency of short-chain acyl-coA dehydrogenase. This is closely related to the inactivation of peroxisome proliferator-activated receptor alpha, which may reflect platelet dysfunction. The increase of alanine level is believed to be related to the increase of carnosin content in blood samples of cerebral ischemia patients, and is closely related to neuronal autophagy (Hu et al., 2016).
5. Conclusions
The physiological functions of brain and intestine are closely related. Intestinal flora can interact with the brain through various mechanisms. The imbalance of intestinal flora will promote the occurrence of CI and is closely related to the prognosis, while CI may aggravate the ecological imbalance of intestinal flora. Intestinal microorganisms significantly affect CI through neuroinflammation and other pathways (Figure 1). There is evidence that the cohabitation of the maintenance host and microorganisms, as well as the gut microbiota, are directly associated to immune system development and the regulation of T cell homeostasis. Additionally, gut microbiota may influence how CI turns out by gut-derived DAMPs and cytokine storms. Nowadays, CI treatment by altering the intestinal microbiota, including FMT, antibiotics and probiotics, still has some limitations, and more research is needed. Future research may focus on the mechanisms of microbe-host interactions, the use of high-throughput sequencing of gut microbial genomes, and the development of drugs based on these data. Therefore, as the omics closest to the phenotype, metabolomics emerged at the historic moment and has become an important breakthrough in scientific research. Excitotoxicity, which is brought on by the loss of metabolic homeostasis and specific types of amino acids, is a crucial biological mechanism for cerebral ischemia damage at the cellular level. Lipids are able to infiltrate brain cells and bridge the blood-brain barrier more readily than proteins. Additionally, the brain is more vulnerable to oxidative stress than most other tissues and organs due to the high concentration of polyunsaturated fatty acids in brain lipids. The analysis of amino acids, lipid and other metabolites in CI patients is helpful to discover new biomarkers of CI, new pathophysiological mechanisms and innovative therapeutic methods. To translate these studies into clinical applications, complete prospective and longitudinal studies are essential.
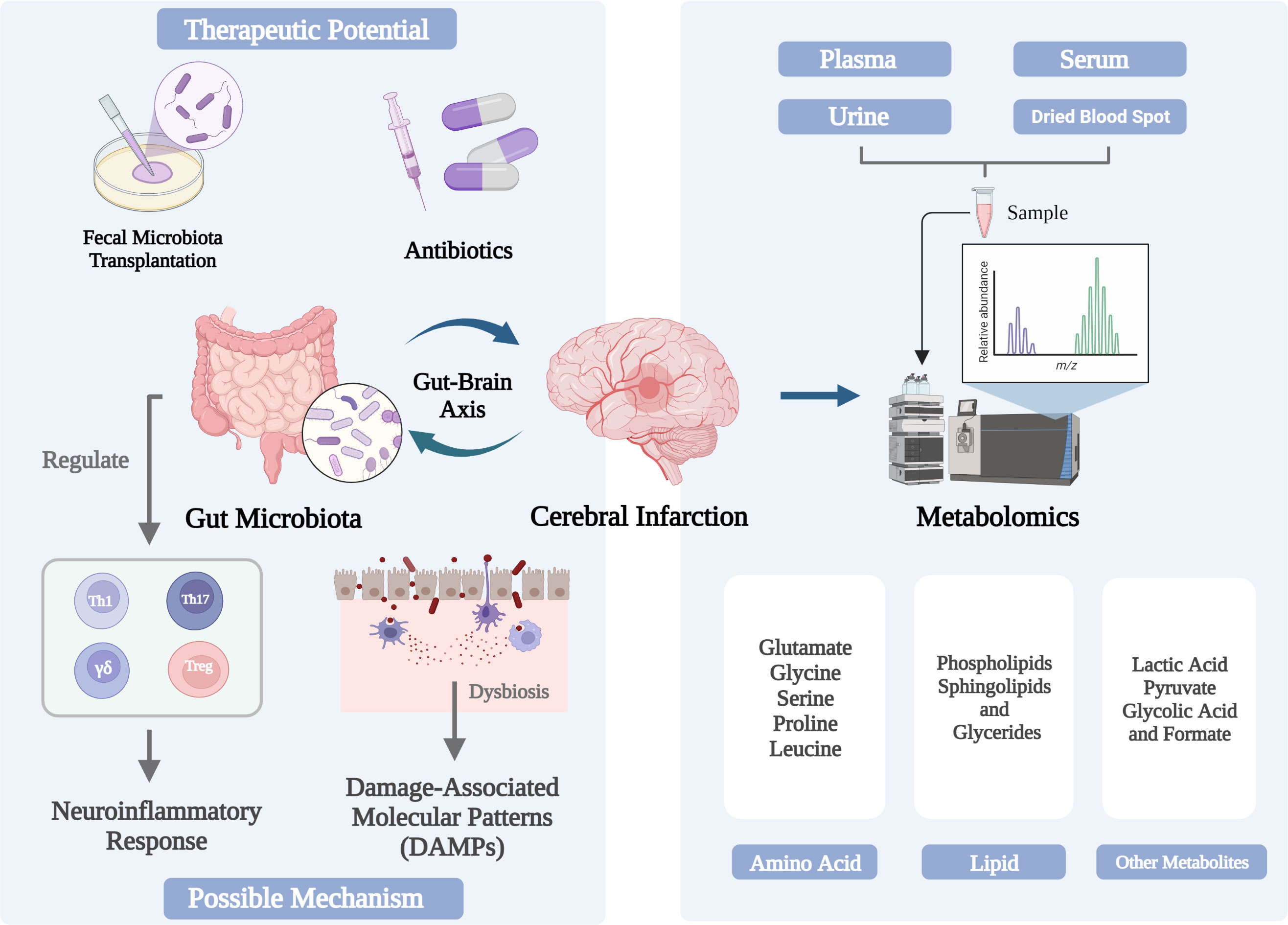
Figure 1 Changes and in the gut microbiome and metabolome associated with cerebral infarction and their triggering mechanisms.
Author contributions
WQ, MW, and SQ had the idea for the article; CX and YG performed the literature search and data analysis; SQ drafted and critically revised the work. All authors contributed to the article and approved the submitted version.
Conflict of interest
The authors declare that the research was conducted in the absence of any commercial or financial relationships that could be construed as a potential conflict of interest.
Publisher’s note
All claims expressed in this article are solely those of the authors and do not necessarily represent those of their affiliated organizations, or those of the publisher, the editors and the reviewers. Any product that may be evaluated in this article, or claim that may be made by its manufacturer, is not guaranteed or endorsed by the publisher.
Abbreviations
CI, Cerebral infarction; ACI, Acute cerebral infarction; FMT, Fecal microbiota transplantation; PD, Parkinson’s disease; AD, Alzheimer’s disease; CNS, Central nervous system; SCFA, Short-chain fatty acid; BCAAs, Branched chain amino acids; SM, Sphingomyelin; SLs, Sphingolipids.
References
Adibhatla, R. M., Hatcher, J. F., Dempsey, R. J. (2006). Lipids and lipidomics in brain injury and diseases. AAPS J. 8 (2), E314–E321. doi: 10.1007/bf02854902
Agirman, G., Yu, K. B., Hsiao, E. Y. (2021). Signaling inflammation across the gut-brain axis. Science 374 (6571), 1087–1092. doi: 10.1126/science.abi6087
Angelucci, F., Cechova, K., Amlerova, J., Hort, J. (2019). Antibiotics, gut microbiota, and alzheimer's disease. J. Neuroinflamm. 16 (1), 108. doi: 10.1186/s12974-019-1494-4
Benakis, C., Brea, D., Caballero, S., Faraco, G., Moore, J., Murphy, M., et al. (2016). Commensal microbiota affects ischemic stroke outcome by regulating intestinal γδ T cells. Nat. Med. 22 (5), 516–523. doi: 10.1038/nm.4068
Benakis, C., Poon, C., Lane, D., Brea, D., Sita, G., Moore, J., et al. (2020). Distinct commensal bacterial signature in the gut is associated with acute and long-term protection from ischemic stroke. Stroke 51 (6), 1844–1854. doi: 10.1161/strokeaha.120.029262
Benjamin, E. J., Virani, S. S., Callaway, C. W., Chamberlain, A. M., Chang, A. R., Cheng, S., et al. (2018). Heart disease and stroke statistics-2018 update: A report from the American heart association. Circulation 137 (12), e67–e492. doi: 10.1161/cir.0000000000000558
Birenbaum, D., Bancroft, L. W., Felsberg, G. J. (2011). Imaging in acute stroke. Western J. Emergency Med. 12 (1), 67.
Caputi, V., Giron, M. C. (2018). Microbiome-Gut-Brain axis and toll-like receptors in parkinson's disease. Int. J. Mol. Sci. 19 (6), 1689. doi: 10.3390/ijms19061689
Chamorro, Á., Meisel, A., Planas, A. M., Urra, X., van de Beek, D., Veltkamp, R. (2012). The immunology of acute stroke. Nat. Rev. Neurol. 8 (7), 401–410. doi: 10.1038/nrneurol.2012.98
Chen, C., Liao, J., Xia, Y., Liu, X., Jones, R., Haran, J., et al. (2022). Gut microbiota regulate alzheimer's disease pathologies and cognitive disorders via PUFA-associated neuroinflammation. Gut 71 (11), 2233–2252. doi: 10.1136/gutjnl-2021-326269
Chen, R., Xu, Y., Wu, P., Zhou, H., Lasanajak, Y., Fang, Y., et al. (2019). Transplantation of fecal microbiota rich in short chain fatty acids and butyric acid treat cerebral ischemic stroke by regulating gut microbiota. Pharmacol. Res. 148, 104403. doi: 10.1016/j.phrs.2019.104403
Chidambaram, S. B., Rathipriya, A. G., Mahalakshmi, A. M., Sharma, S., Hediyal, T. A., Ray, B., et al. (2022). The influence of gut dysbiosis in the pathogenesis and management of ischemic stroke. Cells 11 (7), 1239. doi: 10.3390/cells11071239
DeLong, J. H., Ohashi, S. N., O'Connor, K. C., Sansing, L. H. (2022). Inflammatory responses after ischemic stroke. Semin. Immunopathol. 44 (5), 625–648. doi: 10.1007/s00281-022-00943-7
de Montferrand, C., Vassel-Hitier, J., Yvon-Chaou, E., Câmara-Costa, H., Dellatolas, G., Chevignard, M. (2019). Language and cognitive outcomes after childhood stroke: Theoretical implications for hemispheric specialization. Cortex 120, 509–523. doi: 10.1016/j.cortex.2019.07.020
de Vos, W. M., Tilg, H., Van Hul, M., Cani, P. D. (2022). Gut microbiome and health: mechanistic insights. Gut 71 (5), 1020–1032. doi: 10.1136/gutjnl-2021-326789
Evrensel, A., Ceylan, M. E. (2016). Fecal microbiota transplantation and its usage in neuropsychiatric disorders. Clin. Psychopharmacol. Neurosci. 14 (3), 231–237. doi: 10.9758/cpn.2016.14.3.231
Fang, Z., Chen, M., Qian, J., Wang, C., Zhang, J. (2022). The bridge between ischemic stroke and gut microbes: Short-chain fatty acids. Cell Mol. Neurobiol. doi: 10.1007/s10571-022-01209-4
Feigin, V. L., Abajobir, A. A., Abate, K. H., Abd-Allah, F., Abdulle, A. M., Abera, S. F., et al. (2017). Global, regional, and national burden of neurological disorders during 1990–2015: a systematic analysis for the global burden of disease study 2015. Lancet Neurol. 16 (11), 877–897. doi: 10.1016/S1474-4422(17)30299-5
Feigin, V. L., Forouzanfar, M. H., Krishnamurthi, R., Mensah, G. A., Connor, M., Bennett, D. A., et al. (2014). Global and regional burden of stroke during 1990–2010: findings from the global burden of disease study 2010. Lancet 383 (9913), 245–255. doi: 10.1016/s0140-6736(13)61953-4
Floegel, A., Kühn, T., Sookthai, D., Johnson, T., Prehn, C., Rolle-Kampczyk, U., et al. (2018). Serum metabolites and risk of myocardial infarction and ischemic stroke: a targeted metabolomic approach in two German prospective cohorts. Eur. J. Epidemiol. 33 (1), 55–66. doi: 10.1007/s10654-017-0333-0
Fung, T. C., Olson, C. A., Hsiao, E. Y. (2017). Interactions between the microbiota, immune and nervous systems in health and disease. Nat. Neurosci. 20 (2), 145–155. doi: 10.1038/nn.4476
Gelderblom, M., Weymar, A., Bernreuther, C., Velden, J., Arunachalam, P., Steinbach, K., et al. (2012). Neutralization of the IL-17 axis diminishes neutrophil invasion and protects from ischemic stroke. Blood 120 (18), 3793–3802. doi: 10.1182/blood-2012-02-412726
Grandizoli, C. W., Lange, M. C., Novak, F., Campos, F. R., Barison, A. (2014). Ischemic stroke progress evaluation by 31P NMR-based metabonomic of human serum. J. Braz. Chem. Soc. 25, 1143–1149. doi: 10.5935/0103-5053.20140084
Hamilton, J. A., Hillard, C. J., Spector, A. A., Watkins, P. A. (2007). Brain uptake and utilization of fatty acids, lipids and lipoproteins: application to neurological disorders. J. Mol. Neurosci. 33 (1), 2–11. doi: 10.1007/s12031-007-0060-1
Hill, C., Guarner, F., Reid, G., Gibson, G. R., Merenstein, D. J., Pot, B., et al. (2014). Expert consensus document. the international scientific association for probiotics and prebiotics consensus statement on the scope and appropriate use of the term probiotic. Nat. Rev. Gastroenterol. Hepatol. 11 (8), 506–514. doi: 10.1038/nrgastro.2014.66
Hu, Z., Zhu, Z., Cao, Y., Wang, L., Sun, X., Dong, J., et al. (2016). Rapid and sensitive differentiating ischemic and hemorrhagic strokes by dried blood spot based direct injection mass spectrometry metabolomics analysis. J. Clin. Lab. Anal. 30 (6), 823–830. doi: 10.1002/jcla.21943
Johnson, C. H., Ivanisevic, J., Siuzdak, G. (2016). Metabolomics: beyond biomarkers and towards mechanisms. Nat. Rev. Mol. Cell Biol. 17 (7), 451–459. doi: 10.1038/nrm.2016.25
Jolugbo, P., Ariëns, R. A. S. (2021). Thrombus composition and efficacy of thrombolysis and thrombectomy in acute ischemic stroke. Stroke 52 (3), 1131–1142. doi: 10.1161/strokeaha.120.032810
Jung, J. Y., Lee, H.-S., Kang, D.-G., Kim, N. S., Cha, M. H., Bang, O.-S., et al. (2011). 1H-NMR-Based metabolomics study of cerebral infarction. Stroke 42 (5), 1282–1288. doi: 10.1161/STROKEAHA.110.598789
Kalra, L., Irshad, S., Hodsoll, J., Simpson, M., Gulliford, M., Smithard, D., et al. (2015). Prophylactic antibiotics after acute stroke for reducing pneumonia in patients with dysphagia (STROKE-INF): a prospective, cluster-randomised, open-label, masked endpoint, controlled clinical trial. Lancet 386 (10006), 1835–1844. doi: 10.1016/s0140-6736(15)00126-9
Karlsson, F. H., Fåk, F., Nookaew, I., Tremaroli, V., Fagerberg, B., Petranovic, D., et al. (2012). Symptomatic atherosclerosis is associated with an altered gut metagenome. Nat. Commun. 3, 1245. doi: 10.1038/ncomms2266
Katan, M., Luft, A. (2018). Global burden of stroke. Semin. Neurol. 38 (02), 208–211. doi: 10.1055/s-0038-1649503
Khoruts, A., Sadowsky, M. J. (2016). Understanding the mechanisms of faecal microbiota transplantation. Nat. Rev. Gastroenterol. Hepatol. 13 (9), 508–516. doi: 10.1038/nrgastro.2016.98
Kimberly, W. T., Wang, Y., Pham, L., Furie, K. L., Gerszten, R. E. (2013). Metabolite profiling identifies a branched chain amino acid signature in acute cardioembolic stroke. Stroke 44 (5), 1389–1395. doi: 10.1161/strokeaha.111.000397
Kowalski, K., Mulak, A. (2019). Brain-Gut-Microbiota axis in alzheimer's disease. J. Neurogastroenterol Motil. 25 (1), 48–60. doi: 10.5056/jnm18087
Lam, S. M., Tian, H., Shui, G. (2017). Lipidomics, en route to accurate quantitation. Biochim. Biophys. Acta Mol. Cell Biol. Lipids 1862 (8), 752–761. doi: 10.1016/j.bbalip.2017.02.008
Lee, J., d'Aigle, J., Atadja, L., Quaicoe, V., Honarpisheh, P., Ganesh, B. P., et al. (2020). Gut microbiota-derived short-chain fatty acids promote poststroke recovery in aged mice. Circ. Res. 127 (4), 453–465. doi: 10.1161/circresaha.119.316448
Liesz, A., Zhou, W., Na, S. Y., Hämmerling, G. J., Garbi, N., Karcher, S., et al. (2013). Boosting regulatory T cells limits neuroinflammation in permanent cortical stroke. J. Neurosci. 33 (44), 17350–17362. doi: 10.1523/jneurosci.4901-12.2013
Liu, S., Shi, H., Liu, W., Furuichi, T., Timmins, G. S., Liu, K. J. (2004). Interstitial pO2 in ischemic penumbra and core are differentially affected following transient focal cerebral ischemia in rats. J. Cereb Blood Flow Metab. 24 (3), 343–349. doi: 10.1097/01.Wcb.0000110047.43905.01
Li, N., Wang, X., Sun, C., Wu, X., Lu, M., Si, Y., et al. (2019). Change of intestinal microbiota in cerebral ischemic stroke patients. BMC Microbiol. 19 (1), 191. doi: 10.1186/s12866-019-1552-1
Luan, H., Wang, X., Cai, Z. (2019). Mass spectrometry-based metabolomics: Targeting the crosstalk between gut microbiota and brain in neurodegenerative disorders. Mass Spectrom Rev. 38 (1), 22–33. doi: 10.1002/mas.21553
Mayer, E. A., Nance, K., Chen, S. (2022). The gut-brain axis. Annu. Rev. Med. 73, 439–453. doi: 10.1146/annurev-med-042320-014032
Megur, A., Baltriukienė, D., Bukelskienė, V., Burokas, A. (2020). The microbiota-Gut-Brain axis and alzheimer's disease: Neuroinflammation is to blame? Nutrients 13 (1), 37. doi: 10.3390/nu13010037
Mendelson, S. J., Prabhakaran, S. (2021). Diagnosis and management of transient ischemic attack and acute ischemic stroke: A review. Jama 325 (11), 1088–1098. doi: 10.1001/jama.2020.26867
Newgard, C. B. (2017). Metabolomics and metabolic diseases: Where do we stand? Cell Metab. 25 (1), 43–56. doi: 10.1016/j.cmet.2016.09.018
Pluta, R., Ułamek-Kozioł, M., Januszewski, S., Czuczwar, S. J. (2020). Shared genomic and proteomic contribution of amyloid and tau protein characteristic of alzheimer's disease to brain ischemia. Int. J. Mol. Sci. 21 (9), 3186. doi: 10.3390/ijms21093186
Prabhakaran, S., Ruff, I., Bernstein, R. A. (2015). Acute stroke intervention: a systematic review. Jama 313 (14), 1451–1462. doi: 10.1001/jama.2015.3058
Qi, X., Yun, C., Pang, Y., Qiao, J. (2021). The impact of the gut microbiota on the reproductive and metabolic endocrine system. Gut Microbes 13 (1), 1–21. doi: 10.1080/19490976.2021.1894070
Radenovic, L., Nenadic, M., Ułamek-Kozioł, M., Januszewski, S., Czuczwar, S. J., Andjus, P. R., et al. (2020). Heterogeneity in brain distribution of activated microglia and astrocytes in a rat ischemic model of alzheimer's disease after 2 years of survival. Aging (Albany NY) 12 (12), 12251–12267. doi: 10.18632/aging.103411
Rothman, S. M., Olney, J. W. (1986). Glutamate and the pathophysiology of hypoxic–ischemic brain damage. Ann. Neurol. 19 (2), 105–111. doi: 10.1002/ana.410190202
Salcedo, C., Andersen, J. V., Vinten, K. T., Pinborg, L. H., Waagepetersen, H. S., Freude, K. K., et al. (2021). Functional metabolic mapping reveals highly active branched-chain amino acid metabolism in human astrocytes, which is impaired in iPSC-derived astrocytes in alzheimer's disease. Front. Aging Neurosci. 13. doi: 10.3389/fnagi.2021.736580
Scheithauer, T. P. M., Rampanelli, E., Nieuwdorp, M., Vallance, B. A., Verchere, C. B., van Raalte, D. H., et al. (2020). Gut microbiota as a trigger for metabolic inflammation in obesity and type 2 diabetes. Front. Immunol. 11. doi: 10.3389/fimmu.2020.571731
Shaik, L., Kashyap, R., Thotamgari, S. R., Singh, R., Khanna, S. (2020). Gut-brain axis and its neuro-psychiatric effects: A narrative review. Cureus 12 (10), e11131–e11131. doi: 10.7759/cureus.11131
Sheth, S. A., Iavarone, A. T., Liebeskind, D. S., Won, S. J., Swanson, R. A. (2015). Targeted lipid profiling discovers plasma biomarkers of acute brain injury. PloS One 10 (6), e0129735. doi: 10.1371/journal.pone.0129735
Shin, T. H., Lee, D. Y., Basith, S., Manavalan, B., Paik, M. J., Rybinnik, I., et al. (2020). Metabolome changes in cerebral ischemia. Cells 9 (7), 1630. doi: 10.3390/cells9071630
Sidorov, E., Sanghera, D. K., Vanamala, J. K. P. (2019). Biomarker for ischemic stroke using metabolome: A clinician perspective. J. Stroke 21 (1), 31–41. doi: 10.5853/jos.2018.03454
Simpson, C. A., Diaz-Arteche, C., Eliby, D., Schwartz, O. S., Simmons, J. G., Cowan, C. S. M. (2021). The gut microbiota in anxiety and depression - a systematic review. Clin. Psychol. Rev. 83, 101943. doi: 10.1016/j.cpr.2020.101943
Singh, V., Roth, S., Llovera, G., Sadler, R., Garzetti, D., Stecher, B., et al. (2016). Microbiota dysbiosis controls the neuroinflammatory response after stroke. J. Neurosci. 36 (28), 7428–7440. doi: 10.1523/jneurosci.1114-16.2016
Spychala, M. S., Venna, V. R., Jandzinski, M., Doran, S. J., Durgan, D. J., Ganesh, B. P., et al. (2018). Age-related changes in the gut microbiota influence systemic inflammation and stroke outcome. Ann. Neurol. 84 (1), 23–36. doi: 10.1002/ana.25250
Sun, J., Ling, Z., Wang, F., Chen, W., Li, H., Jin, J., et al. (2016). Clostridium butyricum pretreatment attenuates cerebral ischemia/reperfusion injury in mice via anti-oxidation and anti-apoptosis. Neurosci. Lett. 613, 30–35. doi: 10.1016/j.neulet.2015.12.047
Sun, M. F., Shen, Y. Q. (2018). Dysbiosis of gut microbiota and microbial metabolites in parkinson's disease. Ageing Res. Rev. 45, 53–61. doi: 10.1016/j.arr.2018.04.004
Swanson, K. S., Gibson, G. R., Hutkins, R., Reimer, R. A., Reid, G., Verbeke, K., et al. (2020). The international scientific association for probiotics and prebiotics (ISAPP) consensus statement on the definition and scope of synbiotics. Nat. Rev. Gastroenterol. Hepatol. 17 (11), 687–701. doi: 10.1038/s41575-020-0344-2
Tan, C., Wu, Q., Wang, H., Gao, X., Xu, R., Cui, Z., et al. (2021). Dysbiosis of gut microbiota and short-chain fatty acids in acute ischemic stroke and the subsequent risk for poor functional outcomes. JPEN J. Parenter Enteral Nutr. 45 (3), 518–529. doi: 10.1002/jpen.1861
Tascilar, N., Irkorucu, O., Tascilar, O., Comert, F., Eroglu, O., Bahadir, B., et al. (2010). Bacterial translocation in experimental stroke: what happens to the gut barrier? Bratisl Lek Listy 111 (4), 194–199.
Tian, H., Ni, Z., Lam, S. M., Jiang, W., Li, F., Du, J., et al. (2022). Precise metabolomics reveals a diversity of aging-associated metabolic features. Small Methods 6 (7), e2200130. doi: 10.1002/smtd.202200130
Ulm, L., Hoffmann, S., Nabavi, D., Hermans, M., Mackert, B. M., Hamilton, F., et al. (2017). The randomized controlled STRAWINSKI trial: Procalcitonin-guided antibiotic therapy after stroke. Front. Neurol. 8. doi: 10.3389/fneur.2017.00153
Varona, J. F. (2010). Long-term prognosis of ischemic stroke in young adults. Stroke Res. Treat 2011, 879817. doi: 10.4061/2011/879817
Walker, M., Levitt, M. R., Gibbons, E. F., Horne, D. J., Corcorran, M. A. (2019). Clot analysis in acute ischemic stroke. Stroke 50 (4), e106–e109. doi: 10.1161/strokeaha.118.023700
Wanchao, S., Chen, M., Zhiguo, S., Futang, X., Mengmeng, S. (2018). Protective effect and mechanism of lactobacillus on cerebral ischemia reperfusion injury in rats. Braz. J. Med. Biol. Res. 51 (7), e7172. doi: 10.1590/1414-431x20187172
Wang, J., Zhang, P., Chen, S., Duan, H., Xie, L. (2022b). Microbiota and gut health: Promising prospects for clinical trials from bench to bedside. Advanced Gut Microbiome Res. 2022. doi: 10.1155/2022/2290052
Wang, J., Zhang, H., He, J., Xiong, X. (2022a). The role of the gut microbiota in the development of ischemic stroke. Front. Immunol. 13. doi: 10.3389/fimmu.2022.845243
Wang, X., Zhang, L., Sun, W., Pei, L. L., Tian, M., Liang, J., et al. (2020). Changes of metabolites in acute ischemic stroke and its subtypes. Front. Neurosci. 14. doi: 10.3389/fnins.2020.580929
Wang, J., Zhong, Y., Zhu, H., Mahgoub, O. K., Jian, Z., Gu, L., et al. (2022c). Different gender-derived gut microbiota influence stroke outcomes by mitigating inflammation. J. Neuroinflamm. 19 (1), 245. doi: 10.1186/s12974-022-02606-8
Waziry, R., Chibnik, L. B., Bos, D., Ikram, M. K., Hofman, A. (2020). Risk of hemorrhagic and ischemic stroke in patients with Alzheimer disease: A synthesis of the literature. Neurology 94 (6), 265–272. doi: 10.1212/wnl.0000000000008924
Westendorp, W. F., Vermeij, J. D., Zock, E., Hooijenga, I. J., Kruyt, N. D., Bosboom, H. J., et al. (2015). The preventive antibiotics in stroke study (PASS): a pragmatic randomised open-label masked endpoint clinical trial. Lancet 385 (9977), 1519–1526. doi: 10.1016/s0140-6736(14)62456-9
Wishart, D. S. (2019). Metabolomics for investigating physiological and pathophysiological processes. Physiol. Rev. 99 (4), 1819–1875. doi: 10.1152/physrev.00035.2018
Wu, J., Bortolanza, M., Zhai, G., Shang, A., Ling, Z., Jiang, B., et al. (2022a). Gut microbiota dysbiosis associated with plasma levels of interferon-γ and viral load in patients with acute hepatitis e infection. J. Med. Virol. 94 (2), 692–702. doi: 10.1002/jmv.27356
Wu, J., Huang, F., Ling, Z., Liu, S., Liu, J., Fan, J., et al. (2020). Altered faecal microbiota on the expression of Th cells responses in the exacerbation of patients with hepatitis e infection. J. Viral Hepatitis 27 (11), 1243–1252. doi: 10.1111/jvh.13344
Wu, S., Wu, B., Liu, M., Chen, Z., Wang, W., Anderson, C. S., et al. (2019). Stroke in China: advances and challenges in epidemiology, prevention, and management. Lancet Neurol. 18 (4), 394–405. doi: 10.1016/S1474-4422(18)30500-3
Wu, J., Xu, Y., Cui, Y., Bortolanza, M., Wang, M., Jiang, B., et al. (2022b). Dynamic changes of serum metabolites associated with infection and severity of patients with acute hepatitis e infection. J. Med. Virol. 94 (6), 2714–2726. doi: 10.1002/jmv.27669
Xiang, Z., Li, J., Lu, D., Wei, X., Xu, X. (2022). Advances in multi-omics research on viral hepatitis. Front. Microbiol. 3365. doi: 10.3389/fmicb.2022.987324
Xie, H., Zhang, J., Gu, Q., Yu, Q., Xia, L., Yao, S., et al. (2023). Cohort profile: A prospective study of gut microbiota in patients with acute ischemic stroke. Advanced Gut Microbiome Res. 2023. doi: 10.1155/2023/3944457
Yamashiro, K., Tanaka, R., Urabe, T., Ueno, Y., Yamashiro, Y., Nomoto, K., et al. (2017). Gut dysbiosis is associated with metabolism and systemic inflammation in patients with ischemic stroke. PloS One 12 (2), e0171521. doi: 10.1371/journal.pone.0171521
Ye, X., Zhu, B., Chen, Y., Wang, Y., Wang, D., Zhao, Z., et al. (2022). Integrated metabolomics and lipidomics approach for the study of metabolic network and early diagnosis in cerebral infarction. J. Proteome Res. 21 (11), 2635–2646. doi: 10.1021/acs.jproteome.2c00348
Zheng, Y., Hu, F. B., Ruiz-Canela, M., Clish, C. B., Dennis, C., Salas-Salvado, J., et al. (2016). Metabolites of glutamate metabolism are associated with incident cardiovascular events in the PREDIMED PREvención con DIeta MEDiterránea (PREDIMED) trial. J. Am. Heart Assoc. 5 (9), e003755. doi: 10.1161/jaha.116.003755
Keywords: cerebral infarction, gut microbiome, metabolome, gut-brain axis, fecal microbiota transplantation (FMT)
Citation: Qian W, Wu M, Qian T, Xie C, Gao Y and Qian S (2023) The roles and mechanisms of gut microbiome and metabolome in patients with cerebral infarction. Front. Cell. Infect. Microbiol. 13:1112148. doi: 10.3389/fcimb.2023.1112148
Received: 30 November 2022; Accepted: 12 January 2023;
Published: 25 January 2023.
Edited by:
Zongxin Ling, Zhejiang University, ChinaReviewed by:
Gang Chen, Tongji University School of Medicine, ChinaJiong Yu, Zhejiang University, China
Ming Li, Dalian Medical University, China
Copyright © 2023 Qian, Wu, Qian, Xie, Gao and Qian. This is an open-access article distributed under the terms of the Creative Commons Attribution License (CC BY). The use, distribution or reproduction in other forums is permitted, provided the original author(s) and the copyright owner(s) are credited and that the original publication in this journal is cited, in accordance with accepted academic practice. No use, distribution or reproduction is permitted which does not comply with these terms.
*Correspondence: Surong Qian, cWlhbnN1cm9uZ0BzaW5hLmNvbQ==
†These authors have contributed equally to this work