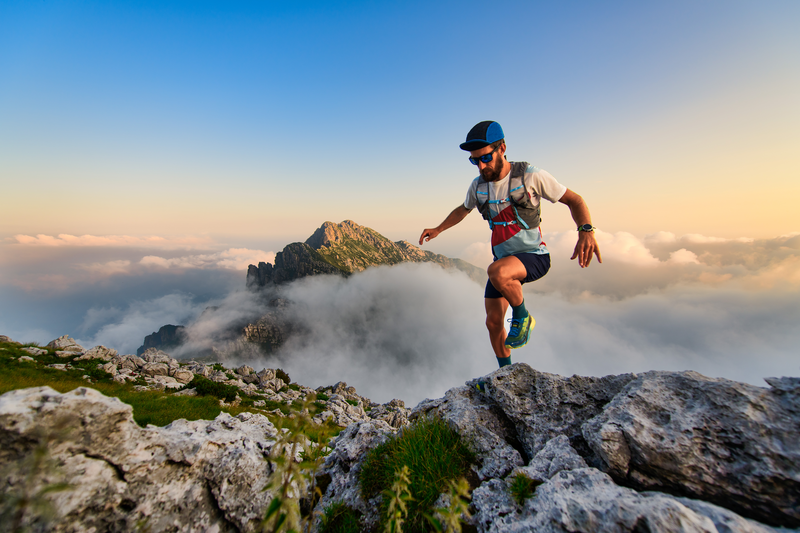
94% of researchers rate our articles as excellent or good
Learn more about the work of our research integrity team to safeguard the quality of each article we publish.
Find out more
BRIEF RESEARCH REPORT article
Front. Cell. Infect. Microbiol. , 15 February 2023
Sec. Parasite and Host
Volume 13 - 2023 | https://doi.org/10.3389/fcimb.2023.1108348
This article is part of the Research Topic Biology and Pathogenicity of Non-falciparum Malaria - Vol II View all 4 articles
Plasmodium vivax is a public health problem and the most common type of malaria outside sub-Saharan Africa. The capacity of cytoadhesion, rosetting, and liver latent phase development could impact treatment and disease control. Although the ability to P. vivax gametocyte develop rosetting is known, it is not yet clear which role it plays during the infection and transmission process to the mosquito. Here, we used ex vivo approaches for evaluate the rosetting P. vivax gametocytes capacity and we have investigated the effect of this adhesive phenotype on the infection process in the vector Anopheles aquasalis mosquito. Rosette assays were performed in 107 isolates, and we have observed an elevated frequency of cytoadhesive phenomena (77,6%). The isolates with more than 10% of rosettes have presented a higher infection rate in Anopheles aquasalis (p=0.0252). Moreover, we found a positive correlation between the frequency of parasites in rosetting with the infection rate (p=0.0017) and intensity (p=0.0387) in the mosquito. The disruption of P. vivax rosette formation through mechanical rupture assay confirmed the previously findings, since the paired comparison showed that isolates with disrupted rosettes have a lower infection rate (p<0.0001) and intensity (p=0.0003) compared to the control group (no disruption). Herein we have demonstrated for the first time a potential effect of the rosette phenomenon on the infection process in the mosquito vector An. aquasalis, favoring its capacity and intensity of infection, thus allowing the perpetuation of the parasite cycle life.
Despite efforts to control malaria, this disease remains a public health problem. In 2021 were estimated more than 247 million cases and 619,000 deaths worldwide (WHO, 2022). Although malaria in humans is caused by six species (P. falciparum, P. vivax, P. ovale, P. malariae, P. knowlesi and P. cynomolgi), two are responsible for the most significant impact of this disease in the world. P. falciparum is responsible for the highest-burden of infections and deaths globally, followed by infections of P. vivax, the most geographically widespread malaria species (Battle et al., 2019; Antonelli et al., 2020).
In Plasmodium spp. infection, red blood cells (RBCs) could cointain parasite´s asexual mature forms or gametocytes which may be sequestered in the microvasculature of vital organs and can present the vascular endothelium attaching ability (cytoadherence) or aggregation of uninfected red blood cells (rosetting) (Chakravorty et al., 2008; Carvalho et al., 2010; Heussler et al., 2017; Salazar Alvarez et al., 2021). The rosette phenomenon has been observed in P. falciparum, P. vivax, and P. ovale infections (Wahlgren et al., 1994; Udomsanpetch et al., 1995; Angus et al., 1996) and are currently known to be formed by trophozoites, schizonts and gametocytes (Chotivanich et al., 1998). In P. falciparum infection, rosetting is associated with severe malaria (Ho et al., 1991; Rowe et al., 1995). However, this phenomenon is more frequently observed for P. vivax than P. falciparum infections (Chotivanich et al., 1998) and for the late has been commonly observed in non-severe malaria (Lee et al., 2014), although its specific role in its pathophysiology is unknown.
Even though the capacity of rosetting development in P. vivax infection has been described more than two decades ago, little information is found about this cytoadhesive phenomenon (Udomsanpetch et al., 1995). It has been shown that the receptors traditionally involved in the rosetting process in P. falciparum infections, such as the ABO and CD35 antigens, do not participate in the formation of rosettes in P. vivax-infected erythrocytes (Rowe et al., 1997; Barragan et al., 2000; Rowe et al., 2007; Vigan-Womas et al., 2012). Rosette formation in P. vivax seems related to the parasite and the glycophorin C (CD236R) receptor in erythrocytes (Lee et al., 2014). Furthermore, it has been shown that in P. vivax infections, rosette stability is not affected by shear stress in vitro, suggesting that rosetting is not affected by hemodynamic forces of in vivo circulation (Zhang et al., 2016). Recently, it has been shown that rosetting is possibly associated with immune evasion strategy (Albrecht et al., 2020). Lee et al. (2020) observed that when P. vivax or P. falciparum infected erythrocytes are exposed to leukocytes, the rosetting rate increased by insulin growth factor binding protein 7 (IGFBP7), leading to phagocytosis reduction (Lee et al., 2020). Furthermore, it has also been observed that P. falciparum rosetting can protect schizont from artemisinin treatment (Lee et al., 2021). Thus, it is clear that this cytoadhesive phenotype is an essential adaptation for the parasite survival during the life cycle in the human host; however, it is unclear what is the relationship of rosettes in gametogenesis, even in falciparum malaria, and if this phenomenon has any implication during the parasite transmission process from the human to the mosquito vector.
Experimental studies with Leishmania spp. showed that agglomeration between the parasites and host erythrocytes causes a limitation of the parasite exposure to the digestive enzymes of the sand fly’s midgut, leading to the parasite protection in early development stages within the insect vector (Pimenta et al., 1997). It has recently been shown that lower red blood cell concentration decreases P. vivax establishment of infection ability in An. aquasalis, indicating that the high RBC concentrations protect P. vivax from killing (Baia-da-Silva et al., 2018). These facts, associated with the ability of P. vivax (Pv-pRBC) infected erythrocytes to form rosettes, lead to the hypothesis of the possible role of rosettes in P. vivax gametocytes transmission, helping the parasite survival in the mosquito early stages of development. Here, we evaluate through ex vivo approaches the ability of gametocytes from non-severe P. vivax isolates to rosetting. In addition, we assessed whether this cytoadhesive phenomenon could contribute to the An. aquasalis mosquito infection.
Blood samples were collected at the Fundação de Medicina Tropical Dr. Heitor Vieira Dourado (FMT-HVD), located in the city of Manaus, Amazonas state, Brazil. 107 adults’ patients (aged >18 years) with P. vivax mono-infection, microscopically diagnosed with parasitemia higher than or equal 1,000 parasites/µL and no current antimalarial treatment were included in the study. Before the treatment, a sample of 9 mL of peripheral blood was withdrawn using heparin-coated vacutainer tubes. Patients were treated following the Brazilian Health Ministry malaria treatment guidelines (Ministério da Saúde do Brasil, 2021).
An. aquasalis were reared in a well-established colony in the Medical Entomology Laboratory at FMT-HVD. Colonies were kept at 24–26 °C and relative humidity of 70–80% on a 12:12 light-dark cycle. Larvae were hatched at room temperature in water containing salt at a final concentration of 2g/L, and a fish food TetraMin® (Madison, Wisconsin, USA) was provided daily. Larvae were allowed to pupate and become adults in an enclosed mesh-covered cage with water provided and fed ad libitum with a 10% sucrose solution until two days before being given the infective blood meals (Baia-da-Silva et al., 2018).
An aliquot of total blood was reserved to direct membrane feeding assay (DMFA) (control group). The percentage of P. vivax gametocytes-were determined by counting the blood smears stained with Giemsa. The blood was immediately processed to obtain enriched P. vivax - parasitized red blood cells (Pv-pRBC). Blood was centrifuged, separating plasma from RBCs. Plasma was stored at 4°C for rosette assay. The RBC were washed twice with RPMI-1640 medium (Sigma-Aldrich®, USA) and filtered in a cellulose column (Sigma-Aldrich®, USA) to remove leukocytes and platelets as previously described (Russell et al., 2011). A Percoll (GE Healthcare, USA) cushion (45%) was performed to separate the mature asexual parasites and gametocytes from the younger asexual forms and non-infected erythrocytes as previously described (Vera et al., 2015). The total number of gametocytes (gPv-pRBC) after the purification process was determined by counting the percentage of gametocytes in thin blood smears from the Percoll interface and determining the quantity of cells per ml using a Neubauer Chamber.
In parallel to the DMFA (next section) gPv-pRBC were adjusted to 10% parasitemia and 5% hematocrit in a rosetting medium (McCoy5A medium supplemented with 20% native autologous plasma); after that, 50 µL of this suspension was plated in a 96 well plate (u- bottom), followed by incubation for 40 minutes at 37°C. Then, they were stained with 50µL of acridine orange (50 µg/mL) and incubated for 10 minutes at 37°C. Subsequently, one drop of the stained suspension was pipetted onto a glass slide and immediately covered with a glass cover slip. Finally, the rosette formation was examined by direct light and fluorescence microscopy (Nikon Eclipse 50i, filter 96311 B-2E/C) (Udomsanpetch et al., 1995). The proportion of gPv-pRBC in rosettes was measured by counting 100 gPv-pRBC in each experiment in duplicate. A rosette was determined by the adhesion of two or more uninfected erythrocytes to a gPv-pRBC (Supplementary Figure S1). Giant erythrocyte rosettes surrounding a P. vivax infected cell were also counted (Marin-Menendez et al., 2013). The isolates were classified into rosette (rosette formation rate higher than or equal to 10%) and non-rosette-forming (rosette forming rate less than 10%).
After the incubation to evaluate the rosette formation, the cell suspension from 4 wells of the 96 well plates was collected in a 1.5 mL microtube per group (200 µL in total). One microtube were maintained in static condition at room temperature (intact rosettes) and the other was exposed to constant vortex agitation for 5 min after completely disrupting rosettes using a needle (disrupted rosettes). Subsequently, membrane feeding assay was performed with these samples (intact and disrupted rosettes) following the parameters described in P. vivax DMFA.
The DMFA was conducted according Fabbri et al., 2021 with few modifications. Three different sets of DMFA experiments were performed. The first one was performed in order to verify if the procedure of parasite purification interfere with An. aquasalis infection, and therefore DMFA were performed comparing two groups: total blood and gPv-pRBC. For this first set of experiments, 500 µL of the total blood were separated and maintained at 37 °C until be used in the DMFA. The remaining blood was processed for gametocyte purification as described above. The pellet of cells obtained was suspended in non-infected O-type blood and AB serum to a hematocrit of 40%. Both suspensions were given to fed mosquitos in the DMFA.
The second set of experiments was performed using 47 isolates enriched to gPv-pRBC to verify the impact of P. vivax rosette at Anopheles infection. For this, part of the purified sample was used to set the rosetting assay as described above and around 1x105 gPv-pRBC were suspended in non-infect O-type blood and autologous plasma to a hematocrit of 40% and given to fed mosquitoes immediately.
The last set of experiments was performed to evaluate the rosette effect in Anopheles infection in the same isolate and for this purpose a mechanical rupture of rosettes were performed. Briefly, after the identification of positive samples for the rosette phenomenon (rosette formation rate higher than or equal to 10%), these were divided into two aliquots for mosquito feeding. One aliquot was kept at room temperature in static conditions (intact rosettes), and the other was subjected to mechanical rupture (disrupted rosettes). After that, the samples were centrifuged and suspended in non-infect O-type blood and autologous plasma to a hematocrit of 40% and given to fed mosquitoes immediately.
The blood suspension in different conditions was offered to groups of 120-150 An. aquasalis females between 90-120 minutes through membrane feeders at 37 °C. After the blood-feeding experiments, only fully engorged mosquitoes were transferred to rearing containers; they were maintained in the insectary at 26 °C, 70–80% relative humidity, and fed daily on a 10% sugar solution. Seven days post-infection, the surviving mosquitoes from each experimental group were dissected. The midguts were stained with 2% commercial Mercurochrome (Merbromin, Sigma- Aldrich, USA), placed under a cover glass, and examined for the presence of oocysts in an optical microscope. The infection rate (percentage of infected mosquito midguts) and the infection intensity (mean number of oocysts per midgut) on each mosquito were recorded and compared among the experimental groups.
Shapiro-Wilk test was evaluated to verify the normality of the distribution. A non-parametric Kruskal-Wallis test or Wilcoxon test were used for infection intensity analysis and for the infection rate, Mann Whitney parametric distribution. Correlations were analyzed using Pearson test. Statistical significance was defined as p < 0.05. All statistical analyses were conducted using GraphPad Prism version 8 software (GraphPad Software, Inc., San Diego, CA). The statistical tests applied are indicated in the figure’s caption, and (n) means the number of samples analyzed.
The P. vivax gametocytes’ capacity to develop rosetting was assessed in 107 isolates obtained from patients with non-severe vivax malaria blood. The rosetting assay was developed with native autologous plasma, and 77.5% (83/107) of the tested samples presented rosette formation capacity (Figure 1A). The percentage of rosettes in gPv-pRBC in the samples evaluated presented a mean of 12.97% ± 13.61, ranging from 0 to 50% (Figure 1B). Additionally, we observed that the average of gPv-pRBC in rosette formation was higher in isolates from patients who had contracted malaria previously than in the primo infection ones (14.08 and 10.59% respectively); however, it was not statistically significant (Student’s t-test, p = 0.1091, n = 48) (Figure 1C). Moreover, no correlation between the number of previous episodes and rosettes rate was observed (Spearman’s test, p = 0.749; Pearson test) (Figure 1D). Finally, correlation analysis with the hematocrit, parasitemia and gametocytemia levels of the patients, showed no correlation with the rosetting capacity of the isolates analyzed in the present study. (Supplementary Figures S2A–C).
Figure 1 Formation of rosettes and previous episodes of malaria. (A) Samples tested with rosette formation capacity (n= 107). (B) Percentage of gPv-pRBC with capacity of rosettes forming for each isolate (n=107). (C) gPv-pRBC rate forming rosettes in primoinfection and in multiple infections by P. vivax. (D) Correlation between the rate of gPv-pRBC in rosette formation and the number of previous episodes of malaria. Data are shown as the mean ± standard deviation. Rosetting rate among isolates was compared by unpaired-t test; p=0.1091; n=48.
Initially, we evaluated whether the purification process of gPv-pRBC could affect mosquito infectivity to verify if this process does not interfere with the parasites’ ability to infect the mosquitoes. No changes were observed in the An. aquasalis infection rate (p = 0.0986; Student’s t-test) and in the infection intensity (p = 0.5075; Mann Whitney test) (Supplementary Figure S3). Subsequently, among the 107 samples assessed for rosetting ability, 47 were used to evaluate the role of rosetting in Anopheles aquasalis infection by DMFA. Twenty-nine isolates of gPv-pRBC (61.7%) were rosette producers and showed 10 to 50% of rosette formation rates. In these isolates, infection rate was 49.25%, while the non-rosette forming isolates (n=18) had a 33.98% (p = 0.0114; Student’s t-test) (Figure 2A). Moreover, a positive correlation between the rosette and infection rate was found (p = 0.001; Pearson test) (Figure 2B). However, no difference between rosette forming and non-rosette forming group in infection intensity was observed (Figure 2C) but a positive correlation between the rosette rate and intensity of infection for these isolates was evidenced (p = 0.0387; Pearson test) ((Figure 2D).
Figure 2 Infection of Anopheles aquasalis with gPvRBC and rosette formation rates (DMFA (2)). (A) Infection rate of An. aquasalis with isolates of gPv-pRBC not rosette-forming (<10%; n=18) or rosette formers (>10%; n=29) (B) Correlation between the rate of gPv-pRBC in rosette formation and the infection rate in An. aquasalis. (C) Infection intensity of An. aquasalis with gPv-pRBC rosette formers (≥10%; n =29) and non-rosetters formers (<10%; n=18). (D) Correlation between the rate of gPv-pRBC in rosetting formation and the intensity of infection in An. aquasalis. The data are shown as the mean ± standard deviation from a total of 47 biological replicates (isolates). Isolation rate and intensity of infection were compared by t-test and Mann Whitney test, respectively. The correlational analyses were performed using the Spearman test.
Once rosetting seems to be correlated with infection rate and intensity, 23 rosette rupture assays were performed. The mechanical rosette rupture process was effective, showing a decrease in rosette rate, which ranged from 0 to 5% after the procedure; and it was significantly different compared to the same untreated sample (p < 0.0001, Wilcoxon paired test) (Figure 3A). Therefore, the investigation of the ability to infect mosquitoes were performed by DMFA comparing the two groups: intact and disrupted rosettes. It was observed that all isolates assessed were able to infect An. aquasalis, presenting a mean infection rate of 37.6%, ranging from 7.14 to 72.2%, and a mean infection intensity of 28.67 ± 33.26, ranging from 0.30 to 32.42. The paired comparison between groups showed that the infection rate was higher in the intact (37.95 ± 19.20) than in the ruptured (26.23 ± 17.73) rosette groups (p < 0.0001; Wilcoxon test) (Figure 3B). Moreover, the infection intensity was also higher in the intact rosettes (4.49 ± 7.83) than in the rupture group (2.93 ± 4.91) (p= 0.0003; Wilcoxon paired test) (Figure 3C).
Figure 3 Integrity to gPv-pRBC rosetting correlates with An. aquasalis infectivity (DMFA (3)). (A) Effect to treatment to disrupted integrity to gPv-pRBC with capacity rosetting (n=23) (B) Infection rate of An. aquasalis with isolates of gPv-pRBC Rosette-forming vs. Disrupted Rosettes (n=23) (C) Infection intensity of An. aquasalis with isolates of gPv-pRBC Rosette-forming vs. Disrupted Rosettes (n=23). Data are shown as the mean ± standard deviation. The data are shown as the mean ± standard deviation from a total of 23 isolates. Isolation rate and intensity of infection before and after processing were compared by paired t-test and Wilcoxon test, respectively. The correlational analyses were performed using the Spearman test.
Rosetting is a cytoadhesive Plasmodium spp. phenotype that frequently occurs in P. falciparum, P. vivax, and P. ovale infections (David et al., 1988; Angus et al., 1996; Chotivanich et al., 1998). P. vivax present ability of rosettes formation in asexual and sexual parasites (Lee et al., 2014). In this study, we observed the high ability of P. vivax gametocytes to form rosettes in samples from non-severe vivax malaria, corroborating previous studies (Lee et al., 2014; Albrecht et al., 2020). Noteworthy, we have revealed the impact of rosette formation in mosquito infectivity.
Previous studies on the rosette formation phenomenon have been able to shed light on some factors involved in this phenotype at a molecular level, however the biological role of rosettes formation is not clear yet (Rowe et al., 1997; Chen et al., 1998; Barragan et al., 2000; Marti et al., 2014; Goel et al., 2015). According to some studies, the process of rosette is involved in parasite immune evasion strategy (Moll et al., 2015; Albrecht et al., 2020; Lee et al., 2020).
Although this phenomenon gives a biological advantage to the parasite, until then its role in the mosquito vector was not investigated. Herein, we demonstrated that rosette formation seems to be involved in an increase in the infectivity of the parasite to the mosquito since isolates that possess gPv-pRBC in rosette formation presented a higher rate and intensity infection in An. aquasalis compared to those who didn´t develop this phenotype. Recently, Zhang et al., 2016 showed that rosette formation in P. vivax infections is highly stable and not destroyed even under high pressure, leading us to hypothesize that rosettes are maintained during the mosquito blood-feeding. Furthermore, we found a positive correlation between the gPv-pRBC in rosettes rate and the infection intensity and rate, which directly impacts parasite transmission. Similarly, studies in Leishmania spp. (Pimenta et al., 1997) and P. chabaudi (Trager et al., 2005) have shown the relationship between blood cell density and parasite survival favors the early part of the infection on mosquito vector midgut.
Baia-da-Silva et al. (2018) have demonstrated that hematocrit affects P. vivax parasite in the Anopheles aquasalis midgut with lower hematocrit resulting in a lower infection in mosquitoes. The authors suggested that the observed may be due to the proximity of digestive enzymes, since the food bolus is small in the mosquitoes fed with low hematocrit. Similarly, our findings may be due to the protection of digestive enzymes by the non-infected erythrocytes around the infected one in the rosetting isolates.
Finally, we have move away the possibility of the rosettes effect in the increase in P. vivax infectivity in An. aquasalis observed be attributed to the genetic variation presented by the distinct phenotype isolates (rosette formers or non-formers) since we have demonstrated the same effect in a paired analysis using the same isolate exposed or not to mechanical rosetting rupture.
In conclusion, rosette phenomenon could protect the parasite from recognition by the immune system and the rosette formation seems to impact the transmission capacity of P. vivax by An. Aquasalis significantly. However, more studies should be carried out to comprehend the role of this phenomenon in parasite survival and transmission to the mosquito.
The original contributions presented in the study are included in the article/Supplementary Material. Further inquiries can be directed to the corresponding authors.
The studies involving human participants were reviewed and approved by the ethical board of Fundação de Medicina Tropical Dr. Heitor Vieira Dourado (CAAE: 54532416.0.0000.0005, approval number 1.591.958). The patients/participants provided their written informed consent to participate in this study.
LCSA, VCB, SCPL and OVL performed P. vivax gametocytes purification, rosetting assay and sample organization. LCSA, OVL, CF, VCB were responsible for experimental infection and sample organization. LCSA, WMM and SCPL helped with statistical analysis. LCSA, LA, CF, DCBS, MVGL, PFPP, FTMC, WMM and SCPL helped draft the manuscript and manuscript revision. LCSA, LA, MVGL, FTMC, PFPP, WMM and SCPL participated in study design, coordination, and writing the finalversion of the manuscript. All authors read and approved the final manuscript.
Fundação de Amparo à Pesquisa do Estado de São Paulo (FAPESP) (Grant 2017/18611-7), Conselho Nacional de Desenvolvimento Científico e Tecnológico (CNPq) (Grant 301795/2013-4), Fundação de Amparo à Pesquisa do Estado do Amazonas (FAPEAM) (POSGRAD) e Coordenação de Aperfeiçoamento de Pessoal de Nível Superior (CAPES) (PDPG AMAZONIA LEGAL). VB, LCSA and OV received scholarships from one of the following Brazilian agencies: FAPEAM, CAPES and FAPESP. DB is a national visiting researcher II with financial support from FAPEAM. FC, ML, PP, and WM are CNPq fellows. The funders had no role in study design, data collection and analysis, decision to publish or preparation of the manuscript.
We also thank the hospital staff for collaboration and the study subjects for their participation.
The authors declare that the research was conducted in the absence of any commercial or financial relationships that could be construed as a potential conflict of interest.
All claims expressed in this article are solely those of the authors and do not necessarily represent those of their affiliated organizations, or those of the publisher, the editors and the reviewers. Any product that may be evaluated in this article, or claim that may be made by its manufacturer, is not guaranteed or endorsed by the publisher.
The Supplementary Material for this article can be found online at: https://www.frontiersin.org/articles/10.3389/fcimb.2023.1108348/full#supplementary-material
Albrecht, L., Lopes, S. C. P., da Silva, A. B. I. E., Barbosa, V., Almeida, R. P., Siqueira, A. M., et al. (2020). Rosettes integrity protects plasmodium vivax of being phagocytized. Sci. Rep. 10, 16706. doi: 10.1038/s41598-020-73713-w
Angus, B. J., Thanikkul, K., Silamut, K., White, N. J., Udomsangpetch, R. (1996). Short report: Rosette formation in plasmodium ovale infection. Am. J. Trop. Med. Hyg. 55, 560–561. doi: 10.4269/ajtmh.1996.55.560
Antonelli, L. R., Junqueira, C., Vinetz, J. M., Golenbock, D. T., Ferreira, M. U., Gazzinelli, R. T. (2020). The immunology of plasmodium vivax malaria. Immunol. Rev. 293, 163–189. doi: 10.1111/imr.12816
Baia-da-Silva, D. C., Alvarez, L. C. S., Lizcano, O. V., Costa, F. T. M., Lopes, S. C. P., Orfanó, A. S., et al. (2018). The role of the peritrophic matrix and red blood cell concentration in plasmodium vivax infection of anopheles aquasalis. Parasitol. Vectors 11, 148. doi: 10.1186/s13071-018-2752-5
Barragan, A., Kremsner, P. G., Wahlgren, M., Carlson, J. (2000). Blood group a antigen is a coreceptor in plasmodium falciparum rosetting. Infect. Immun. 68, 2971–2975. doi: 10.1128/IAI.68.5.2971-2975.2000
Battle, K. E., Lucas, T. C. D., Nguyen, M., Howes, R. E., Nandi, A. K., Twohig, K. A., et al. (2019). Mapping the global endemicity and clinical burden of plasmodium vivax 2000-17: a spatial and temporal modelling study. Lancet (London England) 394, 332–343. doi: 10.1016/S0140-6736(19)31096-7
Carvalho, B. O., Lopes, S. C., Nogueira, P. A., Orlandi, P. P., Bargieri, D. Y., Blanco, Y. C., et al. (2010). On the cytoadhesion of plasmodium vivax-infected erythrocytes. J. Infect. Dis. 202, 638–647. doi: 10.1086/654815
Chakravorty, S. J., Hughes, K. R., Craig, A. G. (2008). Host response to cytoadherence in plasmodium falciparum. Biochem. Soc Trans. 36, 221–228. doi: 10.1042/BST0360221
Chen, Q., Barragan, A., Fernandez, V., Sundström, A., Schlichtherle, M., Sahlén, A., et al. (1998). Identification of plasmodium falciparum erythrocyte membrane protein 1 (PfEMP1) as the rosetting ligand of the malaria parasite p. falciparum. J. Exp. Med. 187, 15–23. doi: 10.1084/jem.187.1.15
Chotivanich, K. T., Pukrittayakamee, S., Simpson, J. A., White, N. J., Udomsangpetch, R. (1998). Characteristics of plasmodium vivax-infected erythrocyte rosettes. Am. J. Trop. Med. Hyg. 59, 73–76. doi: 10.4269/ajtmh.1998.59.73
David, P. H., Handunnetti, S. M., Leech, J. H., Gamage, P., Mendis, K. N. (1988). Rosetting: a new cytoadherence property of malaria-infected erythrocytes. Am. J. Trop. Med. Hyg. 38, 289–297. doi: 10.4269/ajtmh.1988.38.289
Fabbri, C., Trindade, A. O., Andrade, F. S., Souza, M. F., Ríos-Velásquez, C. M., de Lacerda, M. V. G., et al. (2021). Transmission-blocking compound candidates against plasmodium vivax using p. berghei as an initial screening. Mem. Inst. Oswaldo Cruz 116, e200513. doi: 10.1590/0074-02760200513
Goel, S., Palmkvist, M., Moll, K., Joannin, N., Lara, P., Akhouri, R. R., et al. (2015). RIFINs are adhesins implicated in severe plasmodium falciparum malaria. Nat. Med. 21, 314–317. doi: 10.1038/nm.3812
Heussler, V. T., Baro, B., Deroost, K., Raiol, T., Brito, M., Almeida, A. C., et al. (2017). Plasmodium vivax gametocytes in the bone marrow of an acute malaria patient and changes in the erythroid miRNA profile. PloS Negl. Trop. Dis. 11, e0005365. doi: 10.1371/journal.pntd.0005365
Ho, M., Davis, T. M., Silamut, K., Bunnag, D., White, N. J. (1991). Rosette formation of plasmodium falciparum-infected erythrocytes from patients with acute malaria. Infect. Immun. 59, 2135–2139. doi: 10.1128/iai.59.6.2135-2139.1991
Lee, W. C., Malleret, B., Lau, Y. L., Mauduit, M., Fong, M. Y., Cho, J. S., et al. (2014). Glycophorin c (CD236R) mediates vivax malaria parasite rosetting to normocytes. Blood 123, e100–e109. doi: 10.1182/blood-2013-12-541698
Lee, W.-C., Russell, B., Lee, B., Chu, C. S., Phyo, A. P., Sriprawat, K., et al. (2021). Plasmodium falciparum rosetting protects schizonts against artemisinin. EBioMedicine 73, 103680. doi: 10.1016/j.ebiom.2021.103680
Lee, W.-C., Russell, B., Sobota, R. M., Ghaffar, K., Howland, S. W., Wong, Z. X., et al. (2020). Plasmodium-infected erythrocytes induce secretion of IGFBP7 to form type II rosettes and escape phagocytosis. Elife 9, e51546. doi: 10.7554/eLife.51546
Marin-Menendez, A., Bardaji, A., Martinez-Espinosa, F. E., Botto-Menezes, C., Lacerda, M. V., Ortiz, J., et al. (2013). Rosetting in plasmodium vivax: a cytoadhesion phenotype associated with anaemia. PloS Negl. Trop. Dis. 7, e2155. doi: 10.1371/journal.pntd.0002155
Marti, M., Niang, M., Bei, A. K., Madnani, K. G., Pelly, S., Dankwa, S., et al. (2014). STEVOR is a plasmodium falciparum erythrocyte binding protein that mediates merozoite invasion and rosetting. Cell Host Microbe 16, 81–93. doi: 10.1016/j.chom.2014.06.004
Ministério da Saúde do Brasil (2021) Guia de tratamento da malária no brasil. 2o edição. Available at: https://www.gov.br/saude/pt-br/centrais-de-conteudo/publicacoes/publicacoes-svs/malaria/guia_tratamento_malaria_2nov21_isbn_site.pdf/view.
Moll, K., Palmkvist, M., Ch’ng, J., Kiwuwa, M. S., Wahlgren, M. (2015). Evasion of immunity to plasmodium falciparum: Rosettes of blood group a impair recognition of PfEMP1. PloS One 10, e0145120. doi: 10.1371/journal.pone.0145120
Pimenta, P. F., Modi, G. B., Pereira, S. T., Shahabuddin, M., Sacks, D. L. (1997). A novel role for the peritrophic matrix in protecting leishmania from the hydrolytic activities of the sand fly midgut. Parasitology 115, 359–369. doi: 10.1017/S0031182097001510
Rowe, J. A., Handel, I. G., Thera, M. A., Deans, A. M., Lyke, K. E., Kone, A., et al. (2007). Blood group O protects against severe plasmodium falciparum malaria through the mechanism of reduced rosetting. Proc. Natl. Acad. Sci. U.S.A. 104, 17471–17476. doi: 10.1073/pnas.0705390104
Rowe, J. A., Moulds, J. M., Newbold, C. I., Miller, L. H. (1997). P. falciparum rosetting mediated by a parasite-variant erythrocyte membrane protein and complement-receptor 1. Nature 388, 292–295. doi: 10.1038/40888
Rowe, A, Obeiro, J, Newbold, CI, Marsh, K (1995). Plasmodium falciparum rosetting is associated with malaria severity in Kenya. Infect Immun. 63 (6), 2323–2326. doi: 10.1128/iai.63.6.2323-2326.1995
Russell, B., Suwanarusk, R., Borlon, C., Costa, F. T., Chu, C. S., Rijken, M. J., et al. (2011). A reliable ex vivo invasion assay of human reticulocytes by plasmodium vivax. Blood 118, e74–e81. doi: 10.1182/blood-2011-04-348748
Salazar Alvarez, L. C., Vera Lizcano, O., da Silva Barros, D. K. A., Baia-da-Silva, D. C., Monteiro, W. M., Pimenta, P. F. P., et al. (2021). Plasmodium vivax gametocytes adherence to bone marrow endothelial cells. Front. Cell. Infect. Microbiol. 11. doi: 10.3389/fcimb.2021.614985
Trager, W., Mackinnon, M. J., Bell, A., Read, A. F. (2005). The effects of mosquito transmission and population bottlenecking on virulence, multiplication rate and rosetting in rodent malaria. Trends Parasitol. 35, 145–153. doi: 10.1016/j.ijpara.2004.11.011
Udomsanpetch, R., Thanikkul, K., Pukrittayakamee, S., White, N. J. (1995). Rosette formation by plasmodium vivax. Trans. R Soc. Trop. Med. Hyg. 89, 635–637. doi: 10.1016/0035-9203(95)90422-0
Vera, O., Brelas de Brito, P., Albrecht, L., Martins-Campos, K. M., Pimenta, P. F., Monteiro, W. M., et al. (2015). Purification methodology for viable and infective plasmodium vivax gametocytes that is compatible with transmission-blocking assays. Antimicrob. Agents Chemother. 59, 6638–6641. doi: 10.1128/aac.01136-15
Vigan-Womas, I., Guillotte, M., Juillerat, A., Hessel, A., Raynal, B., England, P., et al. (2012). Structural basis for the ABO blood-group dependence of plasmodium falciparum rosetting. PloS Pathog. 8, e1002781. doi: 10.1371/journal.ppat.1002781
Wahlgren, M., Fernandez, V., Scholander, C., Carlson, J. (1994). Rosetting. Parasitol. Today 10, 73–79. doi: 10.1016/0169-4758(94)90400-6
WHO (2022). World malaria report 2022 (WHO). Available at: https://www.who.int/teams/global-malaria-programme/reports/world-malaria-report-2022.
Keywords: malaria, gametocyte, rosetting, cytoadhesion, Plasmodium vivax, vector, anopheles
Citation: Salazar Alvarez LC, Carneiro Barbosa V, Vera Lizcano O, Baia da Silva DC, Gonçalves Santana RA, Fabbri C, Paoluci Pimenta PF, Monteiro WM, Albrecht L, Guimarães de Lacerda MV, Trindade Maranhão Costa F and Costa Pinto Lopes S (2023) Rosette formation by Plasmodium vivax gametocytes favors the infection in Anopheles aquasalis. Front. Cell. Infect. Microbiol. 13:1108348. doi: 10.3389/fcimb.2023.1108348
Received: 25 November 2022; Accepted: 23 January 2023;
Published: 15 February 2023.
Edited by:
Rajesh Chandramohanadas, Rajiv Gandhi Centre for Biotechnology, IndiaReviewed by:
Benoit Malleret, National University of Singapore, SingaporeCopyright © 2023 Salazar Alvarez, Carneiro Barbosa, Vera Lizcano, Baia da Silva, Gonçalves Santana, Fabbri, Paoluci Pimenta, Monteiro, Albrecht, Guimarães de Lacerda, Trindade Maranhão Costa and Costa Pinto Lopes. This is an open-access article distributed under the terms of the Creative Commons Attribution License (CC BY). The use, distribution or reproduction in other forums is permitted, provided the original author(s) and the copyright owner(s) are credited and that the original publication in this journal is cited, in accordance with accepted academic practice. No use, distribution or reproduction is permitted which does not comply with these terms.
*Correspondence: Stefanie Costa Pinto Lopes, c3RlZmFuaWVjcGxvcGVzQGdtYWlsLmNvbQ==; Fabio Trindade Maranhão Costa, ZmFiaW90bWM3MkBnbWFpbC5jb20=
†These authors have contributed equally to this work
Disclaimer: All claims expressed in this article are solely those of the authors and do not necessarily represent those of their affiliated organizations, or those of the publisher, the editors and the reviewers. Any product that may be evaluated in this article or claim that may be made by its manufacturer is not guaranteed or endorsed by the publisher.
Research integrity at Frontiers
Learn more about the work of our research integrity team to safeguard the quality of each article we publish.