- 1Department of Periodontology, The Affiliated Stomatological Hospital of Nanjing Medical University, Nanjing, China
- 2Jiangsu Province Key Laboratory of Oral Diseases, Nanjing, China
- 3Jiangsu Province Engineering Research Center of Stomatological Translational Medicine, Nanjing, China
Introduction: Neutrophil extracellular trap (NET) is a novel defense strategy of neutrophils and found to be induced by Porphyromonas gingivalis (P. gingivalis) lipopolysaccharide (LPS) or high glucose. The aim of this study was to investigate the roles and mechanisms of NET formation in high glucose inflammatory microenvironment.
Methods: NETs induced by 1 μg/ml P. gingivalis LPS and/or 25 mM glucose were visualized using a fluorescence microscopy and the levels of extracellular DNA were determined by a microplate reader. The bactericidal efficiency of NETs was assessed by quantifying the survival P. gingivalis in neutrophils. The levels of NLRP3 and IL-1β in THP-1 derived-macrophages, and the expressions of p-PKC βII, p-MEK1/2, p-ERK1/2, ORAI1 and ORAI2 in neutrophils were detected by Western blot. Moreover, levels of intracellular Ca2+ and reactive oxygen species (ROS) in neutrophils were explored by flow cytometry.
Results: P. gingivalis LPS enhanced the formation of NETs and increased the levels of extracellular DNA in high glucose microenvironment (p < 0.05). Compared with normal glucose inflammatory microenvironment, quantities of extra- and intracellular viable P. gingivalis in neutrophils exposed to NETs induced in high glucose inflammatory one were increased (p < 0.05) and the expressions of NLRP3 and IL-1β were dramatically increased in macrophages co-cultured with NETs from high glucose inflammatory microenvironment (p < 0.05). In addition, levels of ROS, intracellular Ca2+, p-PKC βII, p-MEK1/2, p-ERK1/2, ORAI1 and ORAI2 were increased in neutrophils stimulated with both high glucose and P. gingivalis LPS compared with the single stimulus groups (p < 0.05).
Discussion: In high glucose inflammatory microenvironment, formation of NETs was enhanced via oxidative stress, which failed to reverse the decreased bactericidal capacity in high glucose microenvironment, and instead aggravated the subsequent inflammatory responses.
Introduction
Periodontitis is a chronic inflammatory disease caused by a variety of periodontal pathogens and eventually leads to alveolar bone resorption and tooth loss (Hajishengallis, 2022). Porphyromonas gingivalis (P. gingivalis), a member of the red complex, is one of the most important periodontal pathogens (Chigasaki et al., 2021). Lipopolysaccharide (LPS), a cell wall component of P. gingivalis, triggers immune and inflammatory responses in the host due to its potential toxicity and antigenicity (Xu et al., 2020).
As two major global health threats, diabetes and periodontitis have received a lot of attention in recent years. Growing evidences suggest a bidirectional relationship between these two diseases (Salhi and Reners, 2022). Periodontitis is the sixth most common complication of diabetes. Epidemiological evidences indicate that people with diabetes are at high risk for severe periodontitis (Genco and Borgnakke, 2020), while there is an increased incidence of diabetes in periodontitis patients (Genco et al., 2020). On the one hand, serve periodontal infection impairs insulin sensitivity and worsens glycemic control. On the other hand, diabetes exacerbates oxidative stress and inflammation, which contributes to serious immune damages in periodontal tissues (Polak et al., 2020). However, the exact mechanisms by which the two diseases interact are not fully understood.
As the most abundant circulating leukocytes in peripheral blood, neutrophils play an important role in innate immune responses (Liew and Kubes, 2019). They are recruited to the site of infection, where they kill invading microorganisms by phagocytosis, degranulation, release of reactive oxygen species (ROS) and cytokine production (Burn et al., 2021). In addition, cross-talk between neutrophils and other immune cells, such as macrophages, natural killer, dendritic, B and T lymphocytes, are disclosed by accumulating evidences (Tsai et al., 2021; Herrero-Cervera et al., 2022). Normally, there are increased numbers of neutrophils with declinied biological functions, including impaired phagocytosis, adhesion and chemotaxis, delayed apoptosis and enhanced release of inflammatory mediators in diabetes patients, which leads to excessive inflammatory responses and immune destructions in periodontal tissues (Graves et al., 2020; Dowey et al., 2021; Giovenzana et al., 2022).
In 2004, a novel bactericidal strategy, neutrophil extracellular traps (NETs) were firstly found by Brinkmann (Brinkmann et al., 2004). NETs can be induced by various stimulations, such as PMA, LPS, IL-8 and so on, and are characterized as decondensed extracellular DNA structures decorated with a variety of antibacterial proteins, including histones, neutrophil elastase and myeloperoxidase (MPO) (Wang et al., 2021). Invading pathogens can be entrapped by NETs, and then be killed. However, excessive NET production may lead to tissue damages in the host and are related with a broad spectrum of autoimmune diseases and cancers (Ravindran et al., 2019).
Roles of NETs in the development of periodontitis are gradually uncovered. Abundance of NETs was detected in purulent crevicular exudate and gingival crevicular fluid (GCF) from patients with periodontitis (Magán-Fernández et al., 2020). NETs may be powerful protectors of periodontal tissues, involving in the capturing and killing periodontal pathogens, as well as resolving periodontal inflammation. However, exaggerated NETs may cause epithelial cytotoxicity, damage basal lamina and impair tissue regeneration (Vitkov et al., 2020). Peptidylarginine deiminase 4 (PAD4) and Ca2+ was crucial for P. gingivalis LPS-induced NET formation (Chen et al., 2022). Meanwhile, high glucose promotes the production of NETs, which is closely associated with the development of diabetes and its complications (Njeim et al., 2020). NADPH oxidase-derived ROS was also involved in NET formation in diabetic retinopathy (Wang et al., 2019). Excessive NET formations in diabetic patients may lead to extracellular matrix degradation and delayed wound healing (Sabbatini et al., 2021). However, it still remains obscure whether the effects of diabetes on the development of periodontitis can be achieved by modulating NET formation in high glucose inflammatory microenvironment, and what exact regulatory mechanisms are involved. In the present study, it was hypothesized that exposure to P. gingivalis LPS and high glucose might have synergistic effects on NET formation. The possible involvement of oxidative stress and intracellular Ca2+ in the regulation of NET production in neutrophils stimulated with P. gingivalis LPS combined with high glucose was also explored.
Materials and methods
Reagents
P. gingivalis ATCC 33277 LPS were purchased from InvivoGen (CA, USA). SYTOX Green was acquired from Life Technologies (CA, USA). 2′,7′-Dichlorofluorescein diacetate (DCFH-DA) was obtained from Sigma-Aldrich (MI, USA). Anti-MPO, ORAI1, ORAI2 and NLRP3 antibodies was supplied by Abcam (Cambridge, UK). Antibody against PCK βII was from Huabio (Hangzhou, China) and primary anti-bodies against ERK1/2, p-ERK1/2, MEK, p-MEK and p-PKC βII were gotten from Cell Signaling (MA, USA). Antibodies to IL-1β and β-actin were provided by Affinity (OH, USA).
Cell culture
This study was approved by the Ethical Committee Department, The Affiliated Stomatological Hospital of Nanjing Medical University in accordance with the ethical guidelines of the Declaration of Helsinki and written informed consents were obtained from all recruits.
Neutrophils were isolated from peripheral venous blood collected from systemically and periodontally healthy donors by density gradient centrifugation (Silvestre-Roig et al., 2019). The purity and viability of isolated neutrophils were more than 95%, which were determined by Diff-Quik staining and flow cytometry, respectively. Then, the freshly isolated neutrophils were resuspended in RPMI 1640 medium (Gibco, USA) containing 5.5 mM (normal glucose, NG) or 25 mM (high glucose, HG) glucose for subsequent experiments.
THP−1 cells were obtained from Shanghai Institutes for Biological Sciences, Chinese Academy of Sciences (Shanghai, China) and incubated in RPMI 1640 medium with 100 ng/mL PMA (Sigma, Germany). After a 48 h-differentiation, nonattached cells were aspirated and the remaining adherent macrophages were cultured in fresh RPMI 1640 medium for additional 24 h.
NET detection
Neutrophils (5 x 105 cells/well) were cultured in 12-well plates and divided into four groups. Group 1 and 3 were incubated in RPMI 1640 medium containing 5.5 mM glucose. Group 2 and 4 were incubated in medium containing 25 mM glucose. Both Group 3 and 4 were stimulated with 1 μg/ml P. gingivalis LPS for 3 h.
To visualize NETs, neutrophils (5 x 105 cells/well) were seeded on poly-L-lysine-coated slides in 12-well plates. After a 3 h-stimulation, cells were fixed with 4% paraformaldehyde, permeabilized with 0.2% Triton X-100, blocked with 2% bovine serum albumin and then incubated with antibody against MPO (1:250) at 4°C overnight. Subsequently, neutrophils were incubated with Alexa Fluor 488–conjugated anti-rabbit IgG (H + L) (Beyotime, China) for 3 h and stained with DAPI (Beyotime, China) for 2 min. Finally, NETs were observed by a fluorescence microscope (Leica, Germany) and percentages of neutrophils forming NETs under 3 random fields of views were counted.
In addition, NET formation was quantified by SYTOX Green staining as described in previously study (Chen et al., 2022). Briefly, 5 μM SYTOX Green were added into the conditioned culture medium. After 30 min, the amounts of extracellular DNA in culture medium were detected by SpectraMax M2e microplate reader (Molecular Devices, Germany) using 504 nm excitation and 523 nm emission filters.
Bacterial killing assay
P. gingivalis ATCC 33277 was kindly provided by Jiangsu Province Key Laboratory of Oral Diseases (Nanjing, China). It was cultured on brain heart infusion (BHI) agar plates containing 5% sheep blood anaerobically for 5-7 days and then enriched in BHI broth for an additional 24-72 h. Neutrophils (5 x 105 cells/well) were cultured in 12-well plates and divided into five groups. Group 1-4 were stimulated as described before. Group 5 (blank control group) was blank medium containing 5.5 mM glucose without neutrophils. All groups were challenged with P. gingivalis at a MOI of 100:1 for 3 h. Cells were lysed with deionized water for 10 min to release intracellular bacteria and cultured on BHI agar plates anaerobically for 7-10 days. The number of viable bacteria relative to blank control group was calculated to evaluate the bactericidal efficiency of NETs in each group.
NET isolation and co-culture with THP-1 cells
Neutrophils (1 x 106 cells/well) were divided into four groups. Group 1 and 2 were stimulated with 1 μg/ml P. gingivalis LPS in RPMI 1640 medium containing 5.5 mM glucose for 3 h. Group 3 and 4 were stimulated with 1 μg/ml P. gingivalis LPS in medium containing 25 mM glucose for 3 h. Then, group 2 and 4 were incubated with 5 μg/ml DNase I (Sigma, USA) for 1 h to digest NETs. NET-containing supernatants were collected and mixed with an equal volume of fresh RPMI 1640 medium. THP−1-derived macrophages were cultured with the prepared conditioned medium for 12 h and then collected for subsequent western blot.
Cytosolic Ca2+ measurement
Fluo-4AM (Beyotime, China) is a Ca2+ fluorescent dye that can penetrate cell membrane. Neutrophils were divided into 4 groups as described in 4.3 and incubated with 3 μM Fluo-4AM for 30 min. Fluorescence intensity of intracellular Ca2+ was measured by flow cytometry (FACSCalibur, BD, USA).
Two potent Ca2+ chelators, EGTA and BAPTA, can chelate free extracellular and intracellular Ca2+, respectively. To confirm the roles of cytosolic Ca2+ in NET formation, neutrophils were pretreated with 1 mM EGTA for 30 min or 5 μM BAPTA for 50 min in dark. Then, the levels of Ca2+ and extracellular DNA were measured as described previously.
Detection of oxidative burst product
To measure the production of ROS, neutrophils(1 x 106 cells/well)were grouped as described in 4.3 and incubated with 10 μΜ DCFH-DA, an oxidation-sensitive fluorescent probe, for 40 min. Then, cells were washed, resuspended in ice-cold PBS and measured immediately by FACSCalibur flow cytometer.
NADP+/NADPH ratio in neutrophils was detected using a NADP+/NADPH Assay Kit (Beyotime, China) based on WST-8 assay. Neutrophils were stimulated with 1 ug/ml P. gingivalis LPS in RPMI 1640 medium containing 5.5 mM or 25 mM glucose and lysed with NADP+/NADPH extraction buffer on ice. After a-12,000 g centrifugation for 10 min, the supernatant was collected and separated into two portions. One portion was heated at 60°C for 30 min to destroy NADP+ (only NADPH left), while the other was left on ice to detect both NADP+ and NADPH. Each of the two parts was individually mixed with the working solution for 10 min and then measured at 450 nm spectrophotometrically.
To further confirm the roles of ROS in NET production, neutrophils were pretreated with 10 μM Diphenylene iodonium (DPI), an inhibitor of NADPH oxidase, or 5.9 nM LY333531, an inhibitor of PKC βII, for 30 min, and then incubated with SYTOX Green dye to explore the levels of extracellular DNA.
Western blotting
Neutrophils and THP-1 derived-macrophages were harvested in an ice-cold RIPA buffer (Beyotime, China) for protein extraction. After a-10% SDS-PAGE and electrotransfer, membranes were blocked with 5% non-fat milk, incubated with primary antibodies against MEK, p-MEK, ERK, p-ERK, PKC βII, p-PKC βII, ORAI1, ORAI2, NLRP3, IL-1β and β-actin at 4°C overnight. After an incubation with goat anti-rabbit or goat anti-mouse secondary antibody for 1 h, the proteins were detected with an ECL chemiluminescence kit (Beyotime, China) and semi−quantitively analysis using Image J software (National Institutes of Health, MD, USA) was then performed. All results were normalized to the levels of β-actin, and phosphorylated proteins were expressed as the relative gray value to non-phosphorylated proteins.
Statistical analyses
All data are presented as mean ± SD. Statistical analysis were performed using one-way ANOVA and differences between groups were compared by LSD test. p value less than 0.05 was regarded as statistically significant.
Results
High glucose promoted the formation of NETs
NET formation was firstly observed by immunofluorescence microscopy. Without P. gingivalis LPS stimulation, neutrophils in NG medium (5.5 mM) were founded to be round-like cells and there was almost no extracellular DNA network formation. Extracellular network-like DNA strands (blue) decorated with MPO (green) were observed in neutrophils stimulated with HG (25 mM) and/or P. gingivalis LPS, indicating the formation of classical NETs (Figure 1A). Moreover, there were more NET-positive cells in HG + P. gingivalis LPS group compared with the rest of the groups (p < 0.05, Figure 1B).
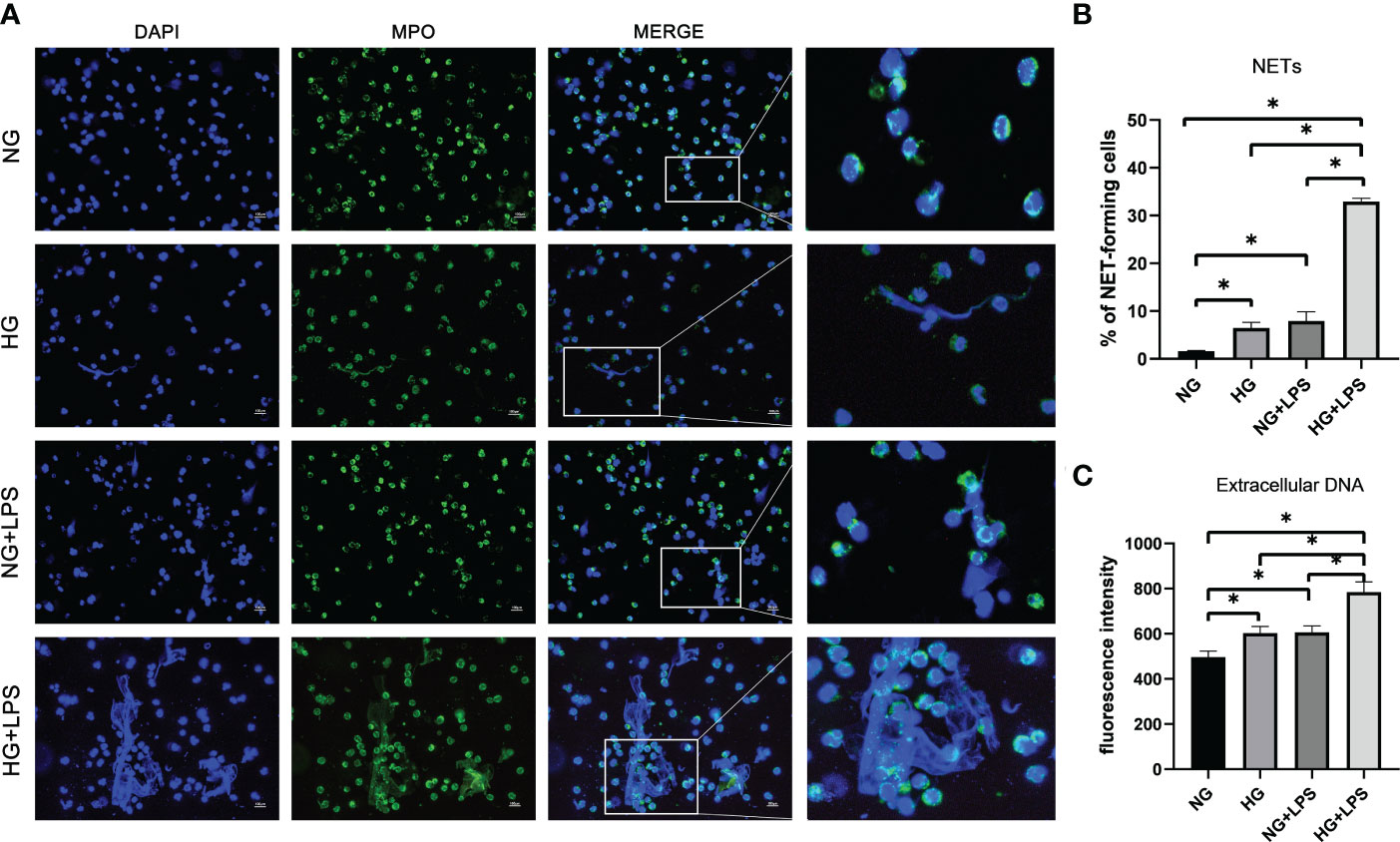
Figure 1 P gingivalis LPS exacerbated NET formations in a high glucose microenvironment. Neutrophils were stimulated with 1 μg/ml P. gingivalis LPS for 3 h in culture medium with 5.5 mM glucose or 25 mM glucose. (A) Cells were stained with an antibody to MPO (green) and DAPI (blue), and then visualized by an immunofluorescence microscopy. One representative result of three independent experiments is shown. Scale bar was 100 μm. (B) Percentage of NET-forming neutrophils to total cells counted was calculated. (C) Quantities of extracellular DNA were determined by Sytox Green assay. Data are presented as mean ± SD (n = 3 donors per group). *p < 0.05.
Quantification analysis of extracellular DNA was consistent with NET visualization. It was revealed that both HG and P. gingivalis LPS stimulation increased the levels of extracellular DNA compared with those in unstimulated group (p < 0.05, Figure 1C), and the level of extracellular DNA in HG + P. gingivalis LPS group was much higher than those with a single stimulus (p < 0.05, Figure 1C).
Enhanced formation of NETs failed to reverse the decreased bactericidal ability of neutrophils in high glucose microenvironment
Previous studies have revealed the roles of NETs in enhancing the efficacy of neutrophils in killing periodontal pathogens (Chen et al., 2022). In HG culture medium, P. gingivalis LPS induced more NET formation, and then bactericidal activities of neutrophils exposed to these NETs were explored by bacterial viability assays (Figure 2A). The number of viable bacteria represents P. gingivalis not killed by neutrophils. The amount of viable of P. gingivalis was significantly decreased in NG + P. gingivalis LPS group compared with NG group (p < 0.05, Figure 2B). Despite the enhanced formation of NETs, the quantities of viable bacteria in HG group were significantly more than those in NG group (p < 0.05, Figure 2B). Furthermore, a similar increase in the amount of viable bacteria were also found in HG + P. gingivalis LPS group compared with NG + P. gingivalis LPS group (p < 0.05).
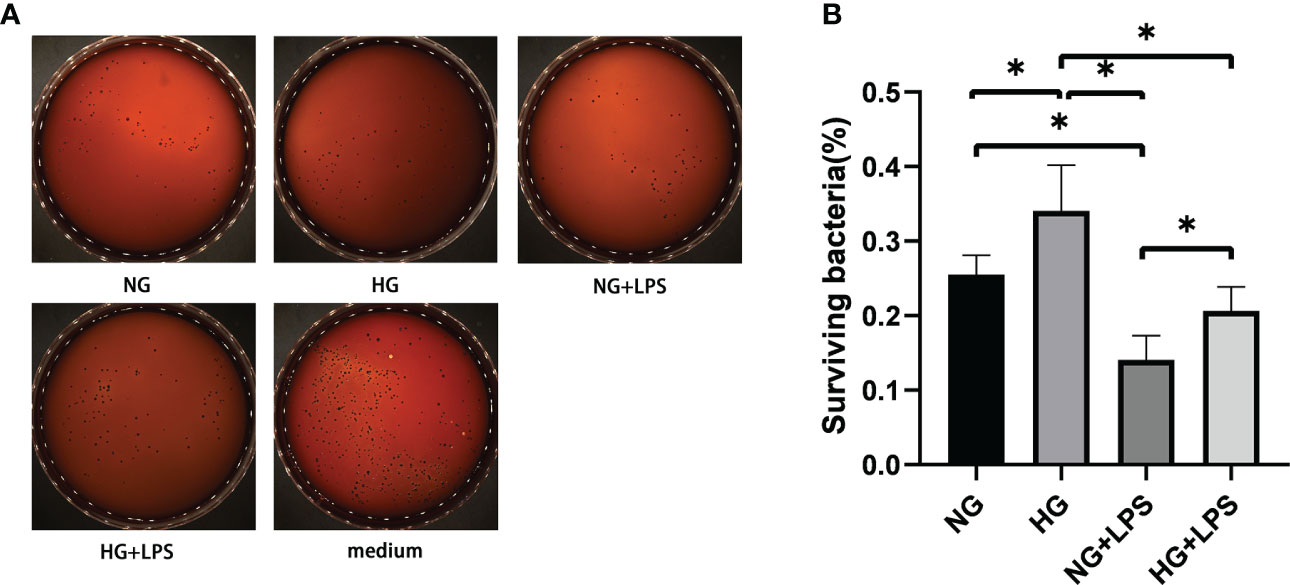
Figure 2 Enhanced formation of NETs was inconsistent with the decreased bactericidal ability of neutrophils in high glucose microenvironment. Neutrophils in culture medium with 5.5 mM or 25 mM glucose were treated with 1 μg/ml P. gingivalis LPS for 3 h. NG (5.5 mM) culture medium without neutrophils served as a negative control. Then, neutrophils in conditioned supernatants containing NETs were stimulated with P. gingivalis at a MOI of 100:1 for another 3 h. Cells were lyzed with ultrapure water and living P. gingivalis on agar plates were counted after 7-10 days. (A) Representative result of three independent experiments is shown. (B) The percentage of surviving bacteria was calculated by plate counting method. Data are presented as mean ± SD (n = 3 donors per group). *p < 0.05.
P. gingivalis LPS and glucose-induced NETs contributed to the up-regulated expressions of Nod-like receptor family, pyrin domain containing 3 and IL-1β in macrophages
Patients with periodontitis combined with diabetes are prone to develop uncontrolled periodontal inflammation, and then it was investigated whether the increased formation of NETs in high glucose inflammatory environment was involved in the subsequent inflammatory responses in macrophages. To determine whether NETs were able to promote the up-regulation of NLRP3 and IL-1β expressions in macrophages, THP−1-derived macrophages were co-cultured with NET-containing supernatants. The expressions of NLRP3 and IL-1β in macrophages stimulated with NETs from P. gingivalis LPS + HG group were significantly increased compared with P. gingivalis LPS + NG group (p < 0.05). Moreover, after treatment with DNase I, there were significant decreases in NLRP3 and IL-1β expression levels in THP−1-derived macrophages stimulated with NETs (p < 0.05, Figure 3), which suggested that NLRP3 activation in macrophages was attributed to the induction by NETs.
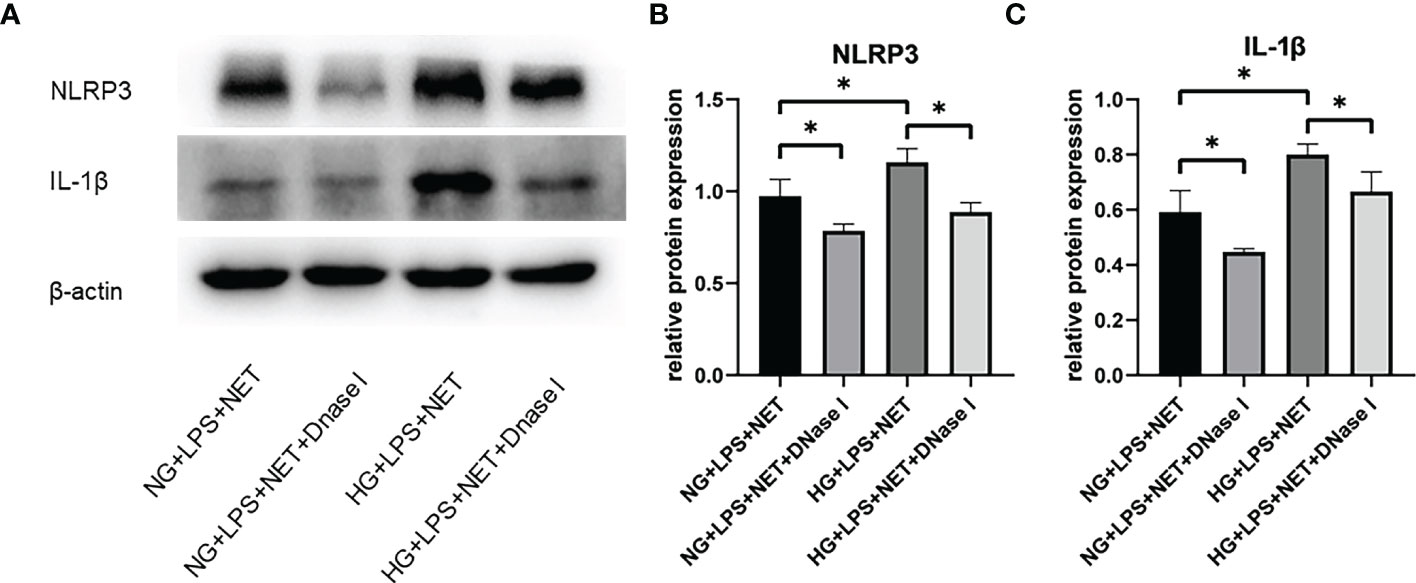
Figure 3 NETs induced in high glucose inflammatory microenvironment contributed to NLRP3 inflammasome activation and IL-1β release in macrophage. Neutrophils in 5.5 mM or 25 mM glucose culture medium were stimulated with 1 μg/ml P. gingivalis LPS and then incubated with 5 μg/ml DNase I for 1 h. THP-1-derived macrophages were incubated with supernatants containing NETs in the absence or presence of DNase I for additional 12 h. Expression levels of NLRP3 and IL-1β were detected by Western blot. (A) One representative result of three independent experiments is shown. (B) Relative levels of NLRP3/β-actin (B) and IL-1β/β-actin (C) were determined by gray value analysis. Data are presented as mean ± SD (n = 3 donors per group). *p < 0.05.
Store-operated Ca2+ entry -mediated Ca2+ influx was involved in NET formation in high glucose inflammatory microenvironment
A common feature of defense responses against invading pathogens in neutrophils is the regulation by Ca2+ signaling. SOCE process has been found to initiate Ca2+ influx that regulates many biological functions of neutrophils, including the formation of NETs. Stronger fluorescence was observed in HG/P. gingivalis LPS group compared with NG group (p < 0.05, Figure 4A), indicating the increased intracellular Ca2+ levels in the first two groups. Moreover, the level of intracellular Ca2+ was remarkably increased in HG + P. gingivalis LPS group compared with the rest of the groups (p < 0.05, Figure 4A).
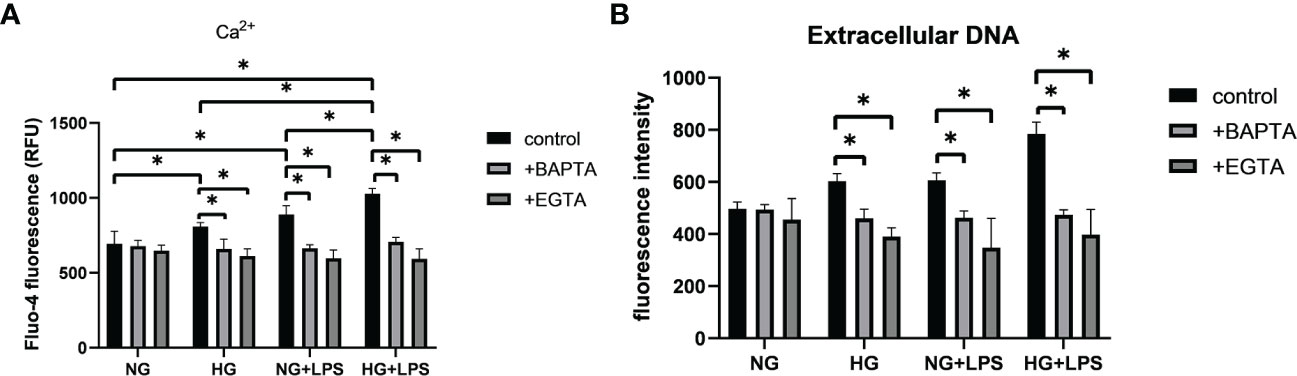
Figure 4 Intracellular Ca2+ contributed to the formation of NETs in high glucose inflammatory microenvironment. Neutrophils were pre-incubated with or without Ca2+ inhibitors (EGTA or BAPTA), and then stimulated with 1 μg/ml P. gingivalis LPS in culture medium with 5.5 mM or 25 mM glucose for 30 min to detect (A) intracellular Ca2+ levels and 3 h for (B) extracellular DNA quantification. Data are presented as mean ± SD (n = 3 donors per group). *p < 0.05.
To further confirm the effect of Ca2+ on NET formation, EGTA and BAPTA were used to chelate free extracellular and intracellular Ca2+, respectively. As illustrated in Figure 4A, intracellular Ca2+ levels were significantly decreased in groups pre-treated with BAPTA or EGTA compared with those without pretreatment (p < 0.05). Moreover, the levels of extracellular DNA in neutrophils pretreated with BAPTA or EGTA were significantly reduced compared with those without Ca2+ chelators pretreatment (p < 0.05, Figure 4B).
ORAI1 and ORAI2 are the essential components of plasma-membrane calcium-release activated calcium (CRAC) channels in neutrophils, which were controlled by SOCE process. Expressions of ORAI1 and ORAI2 were significantly increased in HG group and P. gingivalis LPS group compared with NG group (p < 0.05, Figures 5A–C). In addition, protein expression levels of ORAI1 and ORAI2 in HG + P. gingivalis LPS group were much higher than both HG group and P. gingivalis LPS group (p < 0.05, Figures 5A–C).
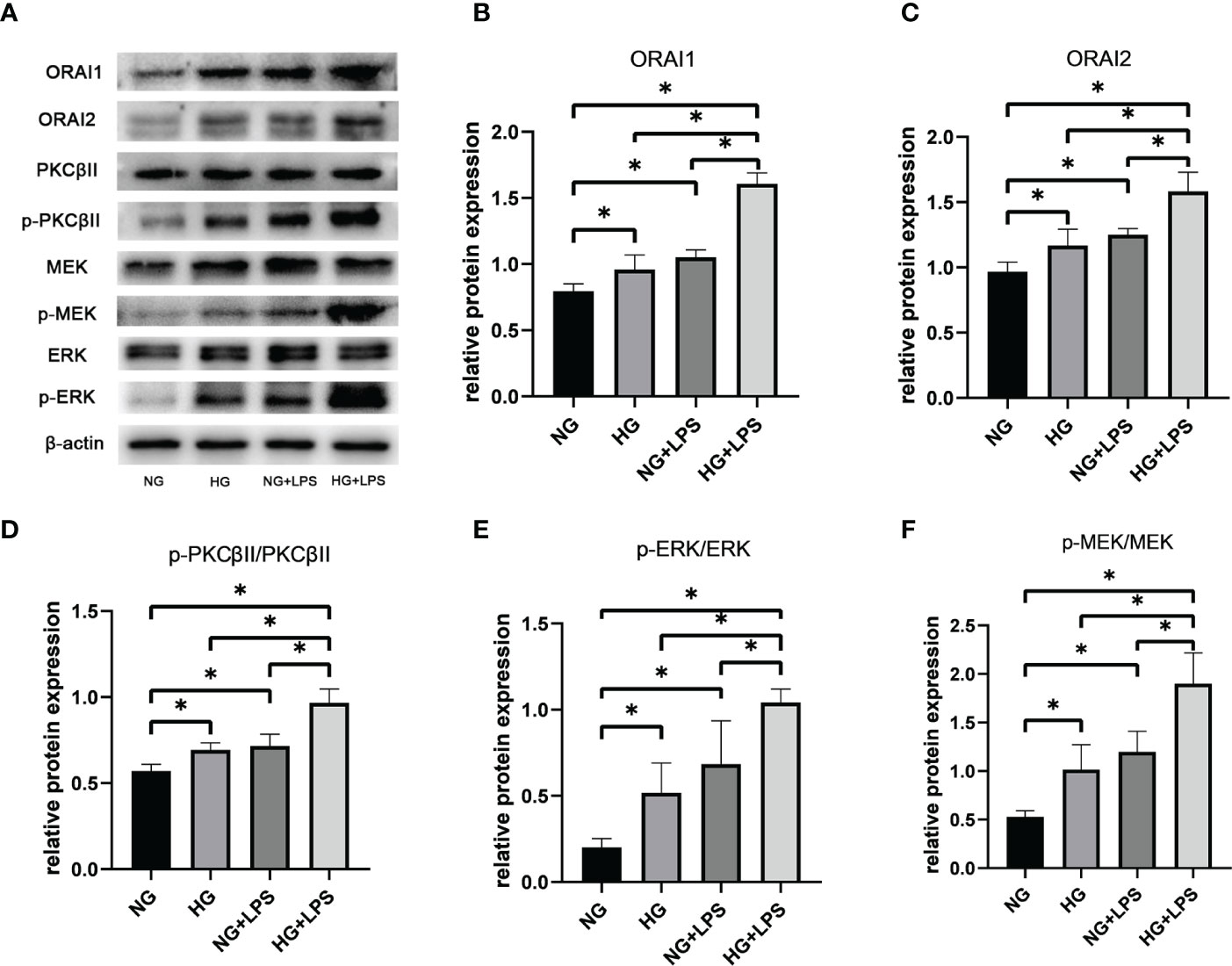
Figure 5 Roles of ORAI1/2, PKC βII, MEK1/2 and ERK1/2 in NET formation induced by P. gingivalis LPS in high glucose medium. Neutrophils were stimulated as described in Figure 1 After 30 min, expression levels of ORAI1, ORAI2, PKC βII, p-PKC βII, MEK1/2, p-MEK1/2, ERK1/2 and p-ERK1/2 were detected by Western blot. (A) One representative result of three independent experiments is shown. Relative levels of (B) ORAI1/β-actin, (C) ORAI2/β-actin, (D) p-PKC βII/PKC βII, (E) p-MEK1/2/MEK1/2 and (F) p-ERK1/2/ERK1/2 were determined by gray value analysis. Data are presented as mean ± SD (n = 3 donors per group). *p < 0.05.
Increased phosphorylations of PKC βII, MEK1/2 and ERK1/2 in NET formations in high glucose inflammatory microenvironment
Conventional activation of PKC is Ca2+-dependent and activated PKC can be involved in a wide range of cellular activities, including NET formation, via MEK1/2 and ERK1/2. Phosphorylation of PKC βII, MEK and ERK1/2 were upregulated in HG group and P. gingivalis LPS group compared with the group without any stimulation (p < 0.05, Figures 5A, D–F). Moreover, expressions of p-PKC βII, p-MEK and p-ERK1/2 in HG + P. gingivalis LPS group were remarkably increased compared with those in HG group and P. gingivalis LPS group (p < 0.05, Figures 5A, D–F).
High glucose enhanced P. gingivalis LPS-induced NET formation via a NADPH oxidase-dependent pathway
PKC βII, MEK1/2 and ERK1/2 are involved in NET formation by activating NADPH oxidase (Hakkim et al., 2011). In the above process, ROS was vital by promoting the collapse of nuclear membrane. Thus, a cell permeable fluorescein probe, DCFH-DA, was used to detect the production of ROS. DPI and LY333531 were employed to inhibit NADPH oxidase and PKC βII, respectively. First, HG and P. gingivalis LPS stimulations resulted in a greater level of ROS in neutrophils (p < 0.05, Figure 6A). The level of ROS in HG + P. gingivalis LPS group was remarkably increased compared with the rest of the groups (p < 0.05, Figure 6A). Further analysis indicated that the up-regulated levels of ROS and extracellular DNA were significantly suppressed by DPI or LY333531-preincubation (p < 0.05, Figures 6A, B).
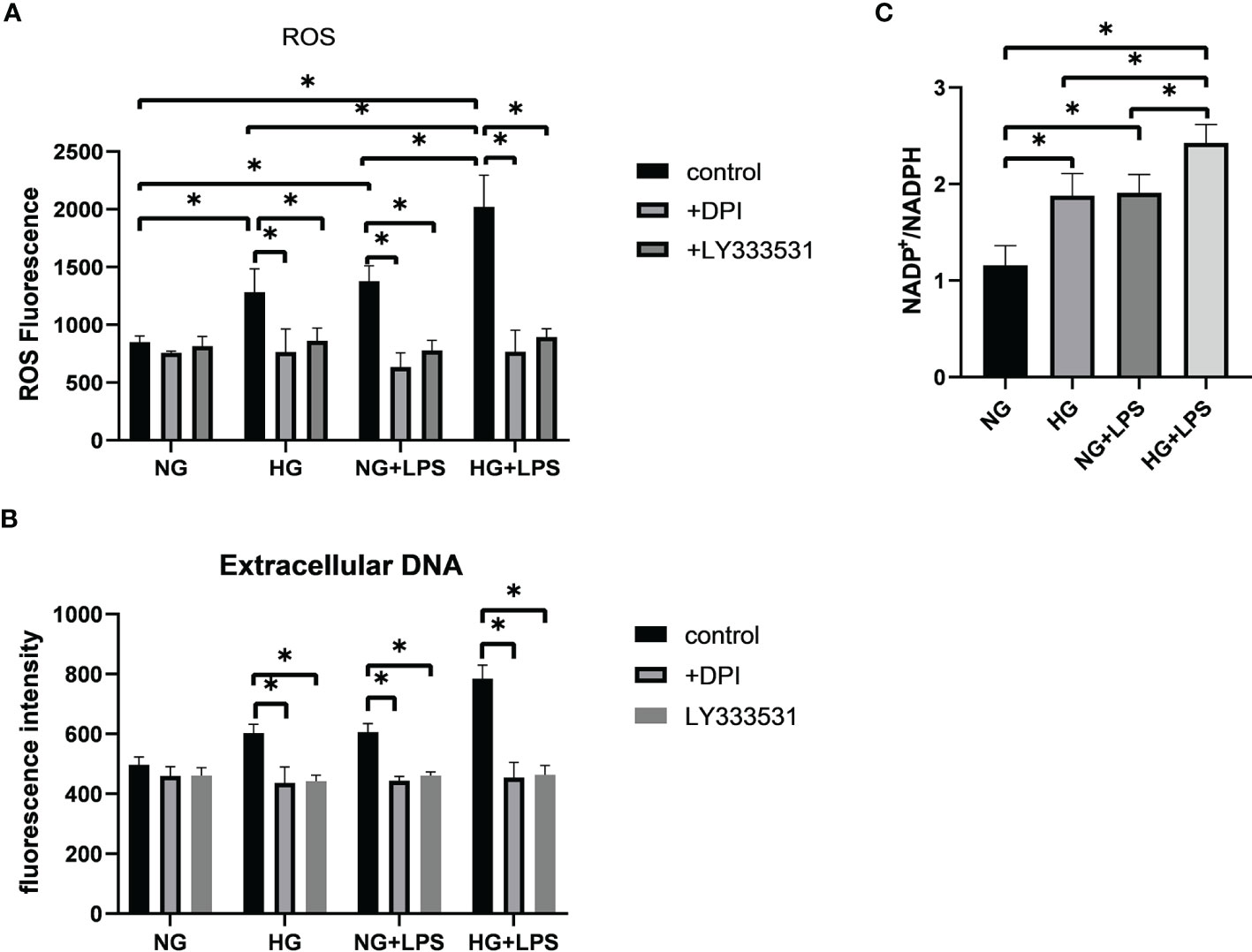
Figure 6 Oxidative stress contributed to the formation of NETs in high glucose inflammatory microenvironment. Neutrophils were pre-treated with 10 μM DPI or 5.9 nM LY333531 for 30 min, and then stimulated with 1 μg/ml P. gingivalis LPS in medium containing 5.5 mM or 25 mM glucose. (A) ROS production in neutrophils was measured by flow cytometry and (B) levels of extracellular DNA were quantified by a microplate reader. (C) NADP+/NADPH ratio were measured by a microplate reader. Data are presented as mean ± SD (n = 3 donors per group). *p < 0.05.
NADPH oxidase is identified as an important enzyme for respiratory burst in neutrophils. Thus, NADP+/NADPH ratio was detected to disclose the activity of NADPH oxidase. The ratio of NADP+/NADPH was increased in HG group and P. gingivalis LPS group compared with NG group (p < 0.05, Figure 6C). Moreover, this ratio was also remarkably increased in HG + P. gingivalis LPS group compared with HG group and P. gingivalis LPS group (p < 0.05, Figure 6C).
Discussion
In recent years, the influences of the inflammatory microenvironment on NET formation are receiving more and more attentions. Even though the backbone of NETs is similar, there are many differences in the composition and function of NETs depending on distinct stimuli and disorder-specific microenvironment (Mitsios et al., 2016). In our previous study, P. gingivalis LPS, one of the classical virulence factors, was demonstrated to trigger the formation of NETs, which was critical in maintaining homeostasis in periodontal tissues (Chen et al., 2022). It has been shown that hyperglycaemic environments in vitro and in vivo induce the release of NETs (Shafqat et al., 2022). However, NET formation in microenvironment with high glucose and intense inflammation had not been clearly investigated. In this study, enhanced formation of NETs due to high glucose microenvironment combined with P. gingivalis LPS-induced inflammation was disclosed for the first time. However, the increased amounts of NETs failed to enhance the bactericidal capacity of neutrophils, but instead amplified inflammatory responses in macrophages.
In a high glucose microenvironment, neutrophils undergo oxidative stress and release a lot of pro-inflammatory cytokines, such as IL-6 and TNF-α, which are strong inducers of NET formation (Dowey et al., 2021; Johnson et al., 2022). Previous studies confirmed that circulating NET components were increased in serum of diabetic patients (Uribe-Querol and Rosales, 2022). Another research also demonstrated that NET formations were exacerbated in high glucose conditions both in vitro and in vivo, suggesting that high glucose might have an initiating effect on NETs production (Zhu et al., 2021). Additional stimulation, such as lonomycin, further induces a large quantities of NETs in high glucose condition in vitro, suggesting that high glucose might have an priming effect on NET formation (Wong et al., 2015). Paradoxically, study of Joshi showed that high glucose induced NET formation, but suppressed further NET production induced by subsequent LPS stimulation (Joshi et al., 2020). The first possible reason for the above-mentioned discrepancy might be the difference in the method of neutrophil isolation. The second possible explanation might be due to the difference in incubation time. The former employed pre-incubation in high glucose culture medium for 1 h, while Joshi selected a 24 h-incubation continuously to mimic the high glucose microenvironment in vivo. However, neutrophils are short-lived cells (12-24 h) and should be best stimulated within 2-4 h following collection (Oh et al., 2008; Burn et al., 2021). Pre-incubation for 24 h in vitro may have some negative effects on the activity and biological functions of neutrophils. Moreover, the last possible explanation might be the differences in blood glucose levels and complications in diabetic patients whose peripheral venous blood was collected, which may affect the homogeneity of the host. Thus, after neutrophil isolation, stimulation with high glucose and P. gingivalis LPS, but without pre-incubation, were employed in this study to avoid any possible effects on neutrophil activities as Menegazzo’s research (Menegazzo et al., 2015).
As the first line of host defense in periodontal tissues, a large number of neutrophils are found in gingival crevice and release NETs, which can trap and kill invading bacteria, fungi and parasites. Jayaprakash’s previous study and ours demonstrated that the bactericidal efficiency of neutrophils against P. gingivalis was enhanced by phagocytosis and NETs (Jayaprakash et al., 2015; Chen et al., 2022). However, both bactericidal activity and phagocytic capacity of neutrophils are reduced due to diabetes (Battaglia et al., 2019; Kumar and Dikshit, 2019). Despite the increased formation of NETs in high glucose inflammatory microenvironment, far more P. gingivalis survived than those in normal glucose inflammatory group, suggesting that the increased NETs induced by P. gingivalis LPS and high glucose did not reverse the impaired bactericidal ability of neutrophils in high glucose microenvironment. Therefore, it might be extremely difficult to control bacterial infection in periodontitis patients combined with diabetes. It should be pointed out that only total bactericidal capacity of neutrophils was assessed in this study, rather than the bactericidal capacity of NETs and there are some other bactericidal strategies, such as phagocytosis, degranulation, respiratory burst and some unknown bactericidal strategies. It is very difficult to evaluate the bactericidal activities of single NETs, excluding the effects of other bactericidal functions.
Macrophages are innate immune cells that are extensively involved in the production of cytokines, as well as the destruction and repair of periodontal tissues. In addition, macrophages recruit and interact with neutrophils in response to invading pathogens, which is necessary for periodontal homeostasis. During the interaction between macrophages and neutrophils, NETs may act as a bridge through inflammasome. Inflammasome is a multi-protein complex activated by various physiological or pathogenic stimuli, such as microorganisms, their virulence factors and danger-associated molecular patterns (DAMPs) (Bullon et al., 2018; Gora et al., 2021). Composition of NLRP3 inflammasome requires NLRP3, apoptosis-associated spec-like protein containing a CARD (ASC), and pro-caspase-1. Active caspase-1 cleaves pro-IL-1β into mature and biologically active IL-1β, which is a potent proinflammatory mediator (Moretti and Blander, 2021). PMA-induced NETs have been shown to upregulate NLRP3 and IL-1β levels via a NF-κB signaling pathway in the tumour microenvironment in vitro (Wang et al., 2022). In this present study, it was confirmed that NETs further up-regulated NLRP3 and IL-1β expression in a high glucose inflammatory condition. Thus, in addition to impairing bacteria‐killing ability of neutrophils and leading to the spread of infection, excess production of NETs in a high glucose inflammatory microenvironment might further amplify the inflammatory responses and contribute to a persistent immune damage. Therefore, controlling the excessive formation of NETs in periodontitis patients with diabetes may provide a new insight into the management of persistent infections.
Ca2+ signals are widely involved in the defense responses of neutrophils against pathogenic microorganisms (Hann et al., 2020). Kenny and Gupta’s studies demonstrated a close link between Ca2+ influx and NET induction (Gupta et al., 2014; Kenny et al., 2017). This present study firstly revealed the involvement of Ca2+ influx in the formation of NETs in a high glucose inflammatory microenvironment. Ca2+ signals are initiated by the SOCE process, which controls the opening of CRAC channels depending on the content of Ca2+ stores (Emrich et al., 2022). CRAC channels are composed of ORAI1, ORAI2 and ORAI3, among which ORAI1 and ORAI2 are the major components in neutrophils. Therefore, their deletions impair multiple bactericidal abilities of neutrophils in S. aureus skin infection and the formation of NETs against parasite Neospora caninum (Zhang et al., 2019; Grimes et al., 2020). In this study, increased protein expressions of ORAI1 and ORAI2 contributed to the enhanced formation of NETs, but not enhanced bactericidal ability, which implied that the components, microstructure and function of NETs remain to be further investigated, as well as whether other bactericidal mechanisms of neutrophils play an important role in high glucose inflammatory microenvironment.
Protein kinase C (PKC) isozymes are members of a Ser/Thr kinase family, which is consists of conventional, novel and atypical isoforms (Kikkawa, 2019). Activation of a conventional PKC isoform, PKC β, is firstly initiated by its phosphorylation, and then binding Ca2+ to the C2 domain to recruit itself to the plasma membrane (Newton, 2018). In the development of atherosclerosis, PKC/Raf/MEK/ERK signaling pathway is involved in endothelial cell proliferation triggered by native LDL (N-LDL) (Pintus et al., 2003). PKC β also takes part in the activation of NADPH oxidase and is the main PKC isoforms involved in NET formation (Gray et al., 2013; Damascena et al., 2022). Recent studies indicated that elevated phosphorylation of PKC βII contributed to the enhanced formation of NETs in diabetic patients (Das et al., 2018; Menegazzo et al., 2018). This present study also found that in high glucose inflammatory microenvironment, phosphorylation of PKC βII was enhanced and PKC βII inhibitor, LY333531, further suppressed ROS production and NET formations, confirming the roles of PKC βII in oxidative stress and NET production.
Oxidative stress plays a crucial role in the pathogenesis of both diabetes and periodontitis (Sczepanik et al., 2020; Yaribeygi et al., 2020). ROS is one of the classical signals of NET formation (Vorobjeva and Chernyak, 2020). The major enzyme responsible for ROS production in neutrophils is NADPH oxidase, which is activated by PKCs or MEK/ERK, and then leads to a rapid release of superoxide accompanied by oxidation of NADPH to NADP+ (Rabani et al., 2020). Fan’s study demonstrated that NADPH levels were decreased in high glucose-stimulated rat retinal capillary endothelial cells, which resulted in an obvious increase in the NADP+/NADPH ratios (Fan et al., 2017). The activation of NADPH oxidase has been demonstrated to participate in NET production, which is triggered by high glucose in patients with diabetic retinopathy (Wang et al., 2019). PKC and Raf-MEK-ERK pathways locate upstream of NADPH oxidase and extensively involved in NET formation (Hakkim et al., 2011). Effects of high glucose and inflammation on ROS production are cumulative, which might be related with the intracellular NADPH oxidase activation and phosphorylation of PKC βII and MEK/ERK. It has been shown that PKC isoforms can phosphorylate the MEK/ERK pathway (Damascena et al., 2022). However, given that there are several isozymes in PKC family and different isozymes do not work in exactly the same way, the relationship between PKC βII and MEK/ERK still needs to be further investigated.
In summary, P. gingivalis LPS might contribute to oxidative stress in high glucose microenvironment through a Ca2+-PKC-MEK-ERK-NADPH oxidase-ROS pathway, thereby promoting NET formation. Furthermore, enhanced formation of NETs might not reverse the impaired bactericidal activities of neutrophils caused by high glucose, instead lead to a serious inflammatory response in macrophages, which would be a challenge for the control of infection in periodontitis patients with diabetes. Further studies are still needed to explore the roles of high glucose inflammatory microenvironment in NET production in vivo, as well as the exact mechanisms involved.
Data availability statement
The original contributions presented in the study are included in the article/Supplementary Material. Further inquiries can be directed to the corresponding author.
Ethics statement
The studies involving human participants were reviewed and approved by the Ethical Committee Department, The Affiliated Stomatological Hospital of Nanjing Medical University. The patients/participants provided their written informed consent to participate in this study.
Author contributions
Conceptualization, YS and YT. Methodology, YT, YX, LF and JS. Validation, YT and YX. Data curation, YT and YX. Writing—original draft preparation, YT. Writing—review and editing, YS. Project administration, YS. Funding acquisition, YS. All authors contributed to the article and approved the submitted version.
Funding
This research was funded by National Natural Science Foundation of China, grant number 81771075 and a project funded by the Priority Academic Program Development of Jiangsu Higher Education Institutions, grant number 2018-87.
Acknowledgments
The authors would like to acknowledge the technical expertise and support of M.F.Yang.
Conflict of interest
The authors declare that the research was conducted in the absence of any commercial or financial relationships that could be construed as a potential conflict of interest.
Publisher’s note
All claims expressed in this article are solely those of the authors and do not necessarily represent those of their affiliated organizations, or those of the publisher, the editors and the reviewers. Any product that may be evaluated in this article, or claim that may be made by its manufacturer, is not guaranteed or endorsed by the publisher.
References
Battaglia, M., Petrelli, A., Vecchio, F. (2019). Neutrophils and type 1 diabetes: current knowledge and suggested future directions. Curr. Opin. Endocrinol. Diabetes Obes. 26, 201–206. doi: 10.1097/MED.0000000000000485
Brinkmann, V., Reichard, U., Goosmann, C., Fauler, B., Uhlemann, Y., Weiss, D. S., et al. (2004). Neutrophil extracellular traps kill bacteria. Science 303, 1532–1535. doi: 10.1126/science.1092385
Bullon, P., Pavillard, L. E., de la Torre-Torres, R. (2018). Inflammasome and oral diseases. Exp. Suppl. 108, 153–176. doi: 10.1007/978-3-319-89390-7_7
Burn, G. L., Foti, A., Marsman, G., Patel, D. F., Zychlinsky, A. (2021). The neutrophil. Immunity 54, 1377–1391. doi: 10.1016/j.immuni.2021.06.006
Chen, J.-L., Tong, Y., Zhu, Q., Gao, L.-Q., Sun, Y. (2022). Neutrophil extracellular traps induced by porphyromonas gingivalis lipopolysaccharide modulate inflammatory responses via a Ca2+-dependent pathway. Arch. Oral. Biol. 141, 105467. doi: 10.1016/j.archoralbio.2022.105467
Chigasaki, O., Aoyama, N., Sasaki, Y., Takeuchi, Y., Mizutani, K., Ikeda, Y., et al. (2021). Porphyromonas gingivalis, the most influential pathogen in red-complex bacteria: A cross-sectional study on the relationship between bacterial count and clinical periodontal status in Japan. J. Periodontol 92, 1719–1729. doi: 10.1002/JPER.21-0011
Damascena, H. L., Silveira, W. A. A., Castro, M. S., Fontes, W. (2022). Neutrophil activated by the famous and potent PMA (Phorbol myristate acetate). Cells 11, 2889. doi: 10.3390/cells11182889
Das, S. K., Yuan, Y.-F., Li, M.-Q. (2018). Specific PKC βII inhibitor: one stone two birds in the treatment of diabetic foot ulcers. Biosci. Rep. 38, BSR20171459. doi: 10.1042/BSR20171459
Dowey, R., Iqbal, A., Heller, S. R., Sabroe, I., Prince, I. R. (2021). A bittersweet response to infection in diabetes; targeting neutrophils to modify inflammation and improve host immunity. Front. Immunol. 12. doi: 10.3389/fimmu.2021.678771
Emrich, S. M., Yoast, R. E., Trebak, M. (2022). Physiological functions of CRAC channels. Annu. Rev. Physiol. 84, 355–379. doi: 10.1146/annurev-physiol-052521-013426
Fan, C.-L., Qiao, Y., Tang, M.-K. (2017). Notoginsenoside R1 attenuates high glucose-induced endothelial damage in rat retinal capillary endothelial cells by modulating the intracellular redox state. Drug Des. Devel Ther. 11, 3343–54. doi: 10.2147/DDDT.S149700
Genco, R. J., Borgnakke, W. S. (2020). Diabetes as a potential risk for periodontitis: association studies. Periodontol 2000 83, 40–45. doi: 10.1111/prd.12270
Genco, R. J., Graziani, F., Hasturk, H. (2020). Effects of periodontal disease on glycemic control, complications, and incidence of diabetes mellitus. Periodontol 2000 83, 59–65. doi: 10.1111/prd.12271
Giovenzana, A., Carnovale, D., Phillips, B., Petrelli, A., Giannoukakis, N. (2022). Neutrophils and their role in the aetiopathogenesis of type 1 and type 2 diabetes. Diabetes Metab. Res. Rev. 38, e3483. doi: 10.1002/dmrr.3483
Gora, I. M., Ciechanowska, A., Ladyzynski, P. (2021). NLRP3 inflammasome at the interface of inflammation, endothelial dysfunction, and type 2 diabetes. Cells 10, 314. doi: 10.3390/cells10020314
Graves, D. T., Ding, Z., Yang, Y. (2020). The impact of diabetes on periodontal diseases. Periodontol 2000 82, 214–224. doi: 10.1111/prd.12318
Gray, R. D., Lucas, C. D., MacKellar, A., Li, F., Hiersemenzel, K., Haslett, C., et al. (2013). Activation of conventional protein kinase c (PKC) is critical in the generation of human neutrophil extracellular traps. J. Inflammation (Lond) 10, 12. doi: 10.1186/1476-9255-10-12
Grimes, D., Johnson, R., Pashos, M., Cummings, C., Kang, C., Sampedro, G. R., et al. (2020). ORAI1 and ORAI2 modulate murine neutrophil calcium signaling, cellular activation, and host defense. Proc. Natl. Acad. Sci. U.S.A. 117, 24403–24414. doi: 10.1073/pnas.2008032117
Gupta, A. K., Giaglis, S., Hasler, P., Hahn, S. (2014). Efficient neutrophil extracellular trap induction requires mobilization of both intracellular and extracellular calcium pools and is modulated by cyclosporine a. PLoS One 9, e97088. doi: 10.1371/journal.pone.0097088
Hajishengallis, G. (2022). Interconnection of periodontal disease and comorbidities: Evidence, mechanisms, and implications. Periodontol 2000 89, 9–18. doi: 10.1111/prd.12430
Hakkim, A., Fuchs, T. A., Martinez, N. E., Hess, S., Prinz, H., Zychlinsky, A., et al. (2011). Activation of the raf-MEK-ERK pathway is required for neutrophil extracellular trap formation. Nat. Chem. Biol. 7, 75–77. doi: 10.1038/nchembio.496
Hann, J., Bueb, J.-L., Tolle, F., Bréchard, S. (2020). Calcium signaling and regulation of neutrophil functions: Still a long way to go. J. Leukoc. Biol. 107, 285–297. doi: 10.1002/JLB.3RU0719-241R
Herrero-Cervera, A., Soehnlein, O., Kenne, E. (2022). Neutrophils in chronic inflammatory diseases. Cell Mol. Immunol. 19, 177–191. doi: 10.1038/s41423-021-00832-3
Jayaprakash, K., Demirel, I., Khalaf, H., Bengtsson, T. (2015). The role of phagocytosis, oxidative burst and neutrophil extracellular traps in the interaction between neutrophils and the periodontal pathogen porphyromonas gingivalis. Mol. Oral. Microbiol. 30, 361–375. doi: 10.1111/omi.12099
Johnson, J., Jaggers, R. M., Gopalkrishna, S., Dahdah, A., Murphy, A. J., Hanssen, N. M. J., et al. (2022). Oxidative stress in neutrophils: implications for diabetic cardiovascular complications. Antioxid Redox Signal 36, 652–666. doi: 10.1089/ars.2021.0116
Joshi, M. B., Ahamed, R., Hegde, M., Nair, A. S., Ramachandra, L., Satyamoorthy, K. (2020). Glucose induces metabolic reprogramming in neutrophils during type 2 diabetes to form constitutive extracellular traps and decreased responsiveness to lipopolysaccharides. Biochim. Biophys. Acta Mol. Basis Dis. 1866, 165940. doi: 10.1016/j.bbadis.2020.165940
Kenny, E. F., Herzig, A., Krüger, R., Muth, A., Mondal, S., Thompson, P. R., et al. (2017). Diverse stimuli engage different neutrophil extracellular trap pathways. Elife 6, e24437. doi: 10.7554/eLife.24437
Kikkawa, U. (2019). The story of PKC: A discovery marked by unexpected twists and turns. IUBMB Life 71, 697–705. doi: 10.1002/iub.1963
Kumar, S., Dikshit, M. (2019). Metabolic insight of neutrophils in health and disease. Front. Immunol. 10, 2099. doi: 10.3389/fimmu.2019.02099
Liew, P. X., Kubes, P. (2019). The neutrophil’s role during health and disease. Physiol. Rev. 99, 1223–1248. doi: 10.1152/physrev.00012.2018
Magán-Fernández, A., Rasheed Al-Bakri, S. M., O’Valle, F., Benavides-Reyes, C., Abadía-Molina, F., Mesa, F. (2020). Neutrophil extracellular traps in periodontitis. Cells 9, E1494. doi: 10.3390/cells9061494
Menegazzo, L., Ciciliot, S., Poncina, N., Mazzucato, M., Persano, M., Bonora, B., et al. (2015). NETosis is induced by high glucose and associated with type 2 diabetes. Acta Diabetol. 52, 497–503. doi: 10.1007/s00592-014-0676-x
Menegazzo, L., Scattolini, V., Cappellari, R., Bonora, B. M., Albiero, M., Bortolozzi, M., et al. (2018). The antidiabetic drug metformin blunts NETosis in vitro and reduces circulating NETosis biomarkers in vivo. Acta Diabetol. 55, 593–601. doi: 10.1007/s00592-018-1129-8
Mitsios, A., Arampatzioglou, A., Arelaki, S., Mitroulis, I., Ritis, K. (2016). NETopathies? unraveling the dark side of old diseases through neutrophils. Front. Immunol. 7. doi: 10.3389/fimmu.2016.00678
Moretti, J., Blander, J. M. (2021). Increasing complexity of NLRP3 inflammasome regulation. J. Leukoc. Biol. 109, 561–571. doi: 10.1002/JLB.3MR0520-104RR
Newton, A. C. (2018). Protein kinase c: perfectly balanced. Crit. Rev. Biochem. Mol. Biol. 53, 208–230. doi: 10.1080/10409238.2018.1442408
Njeim, R., Azar, W. S., Fares, A. H., Azar, S. T., Kfoury Kassouf, H., Eid, A. A. (2020). NETosis contributes to the pathogenesis of diabetes and its complications. J. Mol. Endocrinol. 65, R65–R76. doi: 10.1530/JME-20-0128
Oh, H., Siano, B., Diamond, S. (2008). Neutrophil isolation protocol. J. Vis. Exp. 745. doi: 10.3791/745
Pintus, G., Tadolini, B., Posadino, A. M., Sanna, B., Debidda, M., Carru, C., et al. (2003). PKC/Raf/MEK/ERK signaling pathway modulates native-LDL-induced E2F-1 gene expression and endothelial cell proliferation. Cardiovasc. Res. 59, 934–944. doi: 10.1016/s0008-6363(03)00526-1
Polak, D., Sanui, T., Nishimura, F., Shapira, L. (2020). Diabetes as a risk factor for periodontal disease-plausible mechanisms. Periodontol 2000 83, 46–58. doi: 10.1111/prd.12298
Rabani, R., Cossette, C., Graham, F., Powell, W. S. (2020). Protein kinase c activates NAD kinase in human neutrophils. Free Radic. Biol. Med. 161, 50–59. doi: 10.1016/j.freeradbiomed.2020.09.022
Ravindran, M., Khan, M. A., Palaniyar, N. (2019). Neutrophil extracellular trap formation: physiology, pathology, and pharmacology. Biomolecules 9, E365. doi: 10.3390/biom9080365
Sabbatini, M., Magnelli, V., Renò, F. (2021). NETosis in wound healing: When enough is enough. Cells 10, 494. doi: 10.3390/cells10030494
Salhi, L., Reners, M. (2022). Update on the bidirectional link between diabetes and periodontitis. Adv. Exp. Med. Biol. 1373, 231–240. doi: 10.1007/978-3-030-96881-6_12
Sczepanik, F. S. C., Grossi, M. L., Casati, M., Goldberg, M., Glogauer, M., Fine, N., et al. (2020). Periodontitis is an inflammatory disease of oxidative stress: We should treat it that way. Periodontol 2000 84, 45–68. doi: 10.1111/prd.12342
Shafqat, A., Abdul Rab, S., Ammar, O., Al Salameh, S., Alkhudairi, A., Kashir, J., et al. (2022). Emerging role of neutrophil extracellular traps in the complications of diabetes mellitus. Front. Med. (Lausanne) 9. doi: 10.3389/fmed.2022.995993
Silvestre-Roig, C., Fridlender, Z. G., Glogauer, M., Scapini, P. (2019). Neutrophil diversity in health and disease. Trends Immunol. 40, 565–583. doi: 10.1016/j.it.2019.04.012
Tsai, C.-Y., Hsieh, S.-C., Liu, C.-W., Lu, C.-S., Wu, C.-H., Liao, H.-T., et al. (2021). Cross-talk among polymorphonuclear neutrophils, immune, and non-immune cells via released cytokines, granule proteins, microvesicles, and neutrophil extracellular trap formation: A novel concept of biology and pathobiology for neutrophils. Int. J. Mol. Sci. 22, 3119. doi: 10.3390/ijms22063119
Uribe-Querol, E., Rosales, C. (2022). Neutrophils actively contribute to obesity-associated inflammation and pathological complications. Cells 11, 1883. doi: 10.3390/cells11121883
Vitkov, L., Minnich, B., Knopf, J., Schauer, C., Hannig, M., Herrmann, M. (2020). NETs are double-edged swords with the potential to aggravate or resolve periodontal inflammation. Cells 9, 2614. doi: 10.3390/cells9122614
Vorobjeva, N. V., Chernyak, B. V. (2020). NETosis: molecular mechanisms, role in physiology and pathology. Biochem. (Mosc) 85, 1178–1190. doi: 10.1134/S0006297920100065
Wang, Y., Liu, F., Chen, L., Fang, C., Li, S., Yuan, S., et al. (2022). Neutrophil extracellular traps (NETs) promote non-small cell lung cancer metastasis by suppressing lncRNA MIR503HG to activate the NF-κB/NLRP3 inflammasome pathway. Front. Immunol. 13, 867516. doi: 10.1038/nm.3887
Wang, J., Zhou, Y., Ren, B., Zou, L., He, B., Li, M. (2021). The role of neutrophil extracellular traps in periodontitis. Front. Cell Infect. Microbiol. 11. doi: 10.3389/fcimb.2021.639144
Wang, L., Zhou, X., Yin, Y., Mai, Y., Wang, D., Zhang, X. (2019). Hyperglycemia induces neutrophil extracellular traps formation through an NADPH oxidase-dependent pathway in diabetic retinopathy. Front. Immunol. 9, 3076. doi: 10.3389/fimmu.2018.03076
Wong, S. L., Demers, M., Martinod, K., Gallant, M., Wang, Y., Goldfine, A. B., et al (2015). Diabetes primes neutrophils to undergo NETosis, which impairs wound healing. Nat Med. 21, 815–19. doi: 10.1038/nm.3887
Xu, W., Zhou, W., Wang, H., Liang, S. (2020). Roles of porphyromonas gingivalis and its virulence factors in periodontitis. Adv. Protein Chem. Struct. Biol. 120, 45–84. doi: 10.1016/bs.apcsb.2019.12.001
Yaribeygi, H., Sathyapalan, T., Atkin, S. L., Sahebkar, A. (2020). Molecular mechanisms linking oxidative stress and diabetes mellitus. Oxid. Med. Cell Longev 2020, 8609213. doi: 10.1155/2020/8609213
Zhang, B., Guo, H., Yang, W., Li, M., Zou, Y., Loor, J. J., et al. (2019). Effects of ORAI calcium release-activated calcium modulator 1 (ORAI1) on neutrophil activity in dairy cows with subclinical hypocalcemia1. J. Anim. Sci. 97, 3326–3336. doi: 10.1093/jas/skz209
Keywords: Porphyromonas gingivalis, lipopolysaccharide, neutrophil extracellular traps, reactive oxygen species, inflammatory responses
Citation: Tong Y, Xin Y, Fu L, Shi J and Sun Y (2023) Excessive neutrophil extracellular trap formation induced by Porphyromonas gingivalis lipopolysaccharide exacerbates inflammatory responses in high glucose microenvironment. Front. Cell. Infect. Microbiol. 13:1108228. doi: 10.3389/fcimb.2023.1108228
Received: 25 November 2022; Accepted: 10 January 2023;
Published: 20 January 2023.
Edited by:
Hasan Zaki, University of Texas Southwestern Medical Center, United StatesReviewed by:
Santiago Partida Sanchez, Nationwide Children’s Hospital, United StatesXiang Wang, Nanjing University, China
Copyright © 2023 Tong, Xin, Fu, Shi and Sun. This is an open-access article distributed under the terms of the Creative Commons Attribution License (CC BY). The use, distribution or reproduction in other forums is permitted, provided the original author(s) and the copyright owner(s) are credited and that the original publication in this journal is cited, in accordance with accepted academic practice. No use, distribution or reproduction is permitted which does not comply with these terms.
*Correspondence: Ying Sun, ZWJvbGFzdW5AbmptdS5lZHUuY24=