- 1Department of Respiratory and Critical Care Medicine, The Second Affiliated Hospital of Nanjing Medical University, Nanjing, Jiangsu, China
- 2Department of Infectious Diseases, The Second Affiliated Hospital of Nanjing Medical University, Nanjing, Jiangsu, China
- 3Department of Respiratory and Critical Care Medicine, The Affiliated Jiangning Hospital of Nanjing Medical University, Nanjing, Jiangsu, China
Background: Clinical values of metagenomic next-generation sequencing (mNGS) in patients with severe pneumonia remain controversial. Therefore, we conduct this meta-analysis to evaluate the diagnostic performance of mNGS for pathogen detection and its role in the prognosis of severe pneumonia.
Methods: We systematically searched the literature published in PubMed, Embase, Cochrane Library, Web of Science, Clinical Trials.gov, CNKI, Wanfang Data, and CBM from the inception to the 28th September 2022. Relevant trials comparing mNGS with conventional methods applied to patients with severe pneumonia were included. The primary outcomes of this study were the pathogen-positive rate, the 28-day mortality, and the 90-day mortality; secondary outcomes included the duration of mechanical ventilation, the length of hospital stay, and the length of stay in the ICU.
Results: Totally, 24 publications with 3220 patients met the inclusion criteria and were enrolled in this study. Compared with conventional methods (45.78%, 705/1540), mNGS (80.48%, 1233/1532) significantly increased the positive rate of pathogen detection [OR = 6.81, 95% CI (4.59, 10.11, P < 0.001]. The pooled 28-day and 90-day mortality in mNGS group were 15.08% (38/252) and 22.36% (36/161), respectively, which were significantly lower than those in conventional methods group 33.05% (117/354) [OR = 0.35, 95% CI (0.23, 0.55), P < 0.001, I2 = 0%] and 43.43%(109/251) [OR = 0.34, 95% CI (0.21, 0.54), P < 0.001]. Meanwhile, adjusted treatment based on the results of mNGS shortened the length of hospital stay [MD = -2.76, 95% CI (− 3.56, − 1.96), P < 0.001] and the length of stay in ICU [MD = -4.11, 95% CI (− 5.35, − 2.87), P < 0.001].
Conclusion: The pathogen detection positive rate of mNGS was much higher than that of conventional methods. Adjusted treatment based on mNGS results can reduce the 28-day and 90-day mortality of patients with severe pneumonia, and shorten the length of hospital and ICU stay. Therefore, mNGS advised to be applied to severe pneumonia patients as early as possible in addition to conventional methods to improve the prognosis and reduce the length of hospital stay.
Introduction
Severe pneumonia is a common critical illness with an increasing morbidity and mortality, patients with this disease often require admission to intensive care unit (ICU) (Kalil et al., 2016; Metlay et al., 2019; Zhou et al., 2021). Despite significant advances in its etiological investigation and antimicrobial therapy, severe pneumonia remains the leading cause of death among infectious diseases worldwide (De Pascale et al., 2011). There is no denying that early, rapid, and accurate pathogenic diagnosis is crucial in guiding promt antibiotic treatment, thus improving prognosis and reducing fatality (Cillóniz et al., 2021).
Traditionally, clinicians select antibiotics empirically and then adjust treatment based on the results of conventional methods. However, microbiological culture-based tests do not meet clinical needs due to their time-consuming nature, low sensitivity, lack of diagnostic tests for rare pathogens and vulnerability to external influences (Torres et al., 2021). Inspiringly, metagenomic next-generation sequencing (mNGS), a new pathogen detection technique with high efficiency, broad pathogen spectrum and increased sensitivity, has been widely used in clinic gradually (Gu et al., 2019). mNGS theoretically performs unbiased and detailed high-throughput sequencing of the total DNA or RNA content of almost all known pathogens, including bacteria, fungi, viruses, mycobacterium tuberculosis, parasites and atypical pathogens, and then compares the obtained sequence information with databases (Chiu and Miller, 2019). Several case reports and clinical trials have demonstrated the great value of mNGS in the pathogenic diagnosis of severe and complex cases, including rare pathogens, mixed infections (Wang et al., 2019) and infectious diseases presenting with atypical symptoms, such as Chlamydia psittaci (Li et al., 2021), Chlamydia abortus (Zhu et al., 2022), leptospirosis presenting as severe alveolar hemorrhage (Chen M. et al., 2021) and so on. Several studies (Huang et al., 2020; Chen S. et al., 2022; Wu et al., 2022) have revealed that the sensitivity and specificity of mNGS detection are markedly superior than that of conventional methods.
However, the results of studies on the diagnostic and prognositic values of mNGS on severe pneumonia are controversial (Xie et al., 2019; Zhang et al., 2020; Xi et al., 2022). In addition, to our knowledge, there is no relevant systematic review and meta-analysis to provide a higher level of evidence on the clinical values of mNGS results on severe pneumonia. Therefore, we performed this meta-analysis to evaluate and compare mNGS and conventional methods on the diagnostic performance and prognostic impact of severe pneumonia.
Methods
We strictly followed the standards of the Preferred Reporting Items for Systematic Reviews and Meta-Analyses (PRISMA) (http://www.prisma-statement.org/) in reporting the findings of this review. The protocol for this study was registered in PROSPERO (CRD42022325564).
Search strategy and data sources
PubMed, Embase, Cochrane Library, Web of Science, Clinical Trials.gov, CNKI, Wanfang DATA and CBM were searched from inception to 28th September 2022. Search terms included the following: (“Next generation sequencing” OR “Metagenomic next generation sequencing” OR “NGS” OR “mNGS”) AND (“severe pneumonia” OR “serious pneumonia” OR “severe respiratory infection” OR “severe lung infection” OR “severe community-acquired pneumonia” OR “severe hospital-acquired pneumonia”). Researchers manually scanned the references of all retrieved articles and other relevant publications for additional articles. The exhaustive search strategy is reported in Table S1.
Eligibility criteria and study selection
Two authors (Lv M and Zhu C) screened the relevant literature independently and then checked the title and abstract of each retrieved article to decide which required further assessment. The full text of potentially eligible records was retrieved, reviewed and eligible studies were included. When there were disagreements, Lv M, Zhu C and Du X discussed thoroughly to reach an agreement. Severe pneumonia was defined in patients with either one major criterion or at least three minor criteria of the Infectious Diseases Society of America (IDSA)/American Thoracic Society (ATS) criteria (Metlay et al., 2019).
The primary outcomes were pathogen detection positive rate, 28-day mortality, and 90-day mortality. Secondary outcomes included duration of mechanical ventilation, length of hospital stay, and length of stay in the ICU. The eligibility criteria were as follows: (1) patients with severe pneumonia; (2) participants above 18 years old; (3) reports comparing the pathogen detection positive rate or prognosis outcomes of mNGS with conventional methods. Articles were excluded based on the following criteria: (1) reviews, letters, case report, or case series with < 10 patients; (2) enrolled patients did not suffer from severe pneumonia; (3) not provide data on pathogen-detection positive rate, mortality or prognostic factors; (4) studies not compare positive rate, mortality or prognostic factors between mNGS group and conventional methods group; (5) low quality or can not obtain relevant data.
Qualitative assessment and data extraction
The quality of each study was independently evaluated by two authors (Lv M and Zhu C) using the Newcastle-Ottawa scale. Two authors (Lv M and Zhu C) independently extracted data with a customized data extraction form and assessed the risk of bias. The data extraction form included the following detailed information: (1) references and publication date; (2) type of research; (3) sample types; (4) mean age; (5) gender; (6) the initial value of the diagnostic performance indicators; (7) 28-day mortality; (8) 90-day mortality; (9) duration of mechanical ventilation; (10) length of hospital stay; and (11) length of stay in ICU.
Meta-analysis and statistical methods
Meta-analysis was used to synthesize the outcome measure estimates. Data analyses were performed by Review Manager 5.4. A funnel plot was applied to check for publication bias, and I2 was applied to estimate the total variation attributed to heterogeneity among studies. Sensitivity analysis was performed by Stata 15. For dichotomous variables, odds ratios (ORs) were used for statistical calculations, whereas mean differences (MDs) were used for continuous variables. For I2 ≥ 50%, the random effect model of the restricted maximum likelihood probability method is used. Otherwise, the fixed effect model of the reverse variance method is used.
Results
Study selection process and data extraction
The search identified 1367 records, among these 434 were removed as duplicates. After screening titles and abstracts, 860 were found to be ineligible. Overall, 73 full-text articles were assessed for eligibility. Ultimately, 24 studies met the inclusion criteria (Wu, 2019; Xie et al., 2019; Zhuo et al., 2019; Chen, 2020; Fan, 2020; Pan, 2020; Pan and Lv, 2020; Ren et al., 2020; Song et al., 2020; Sun et al., 2020; Wu et al., 2020; Zhang et al., 2020; Chen J. et al., 2021; Huang, 2021; Lu et al., 2021; Ma et al., 2021; Xu, 2021; Zhu and Cao, 2021; Chen Y. et al., 2022; Huang, 2022; Liu et al., 2022; Tan et al., 2022; Zhang et al., 2022; Zhou and Yang, 2022). The detailed literature retrieval and screening process is shown in Figure 1.
Characteristics of the included studies
The characteristics of the eligible studies are presented in Table 1. Totally, 24 studies with 3220 patients were enrolled. Nineteen (Wu, 2019; Xie et al., 2019; Chen, 2020; Fan, 2020; Pan, 2020; Pan and Lv, 2020; Ren et al., 2020; Song et al., 2020; Sun et al., 2020; Zhang et al., 2020; Chen J. et al., 2021; Lu et al., 2021; Ma et al., 2021; Xu, 2021; Chen Y et al., 2022; Huang, 2022; Liu et al., 2022; Tan et al., 2022; Zhou and Yang, 2022) studies were retrospective and five (Zhuo et al., 2019; Wu et al., 2020; Huang, 2021; Zhu and Cao, 2021; Zhang et al., 2022) were prospective. Eighteen studies included patients with severe pneumonia and six studies included severe pneumonia patients with other complications, such as immunosuppression (Ma et al., 2021), immunodeficiency (Lu et al., 2021), bloodstream infection (Chen J. et al., 2021), acute respiratory distress syndrome (ARDS) (Zhang et al., 2020), after renal transplantation (Zhuo et al., 2019) and autoimmune diseases (Wu, 2019). The sample types collected mainly included blood, sputum, or bronchoalveolar lavage fluid (BALF). Among them, 23 studies (95.8%), 6 studies (25.0%), and 4 studies (16.7%) reported pathogen detection positive rate, 28-day mortality, and 90-day mortality, respectively. Some studies assessed detection positive rate of bacteria, fungi, virus and other pathogens respectively, which are listed in Table S2. Useable data for the duration of mechanical ventilation, length of hospital stay and length of stay in ICU were provided in 5 studies (20.8%), 6 studies (25.0%), and 4 studies (16.7%). The mean study quality score of the studies was 6.38 (SD=0.88) out of 9 on the Newcastle-Ottawa Scale, representing moderate to high methodological quality. A detailed quality assessment is presented in Table S3.
Meta-analysis: mNGS versus conventional methods
Pathogen detection positive rate
A total of 3072 cases in 23 studies were pooled for assessing pathogen detection positive rate. The random-effect model was applied given the heterogeneity test indicated moderate heterogeneity (I2 > 50%, P < 0.001). We introduced subgroups including BALF and other types of specimens group. The meta-analysis results showed that compared with the conventional methods group (45.78%, 705/1540), mNGS group (80.48%, 1233/1532) significantly increased the pathogen detection positive rate [OR = 6.81, 95% CI (4.59, 10.11), P < 0.001 (Figure 2). Sensitivity analysis (Figure 3) was conducted on the included 23 studies, and excluding any one of the studies had no significant impact on the pooled effect value, which confirmed the stability of the final results of this study. The funnel chart showed absence of obvious publication bias (Figure 4).
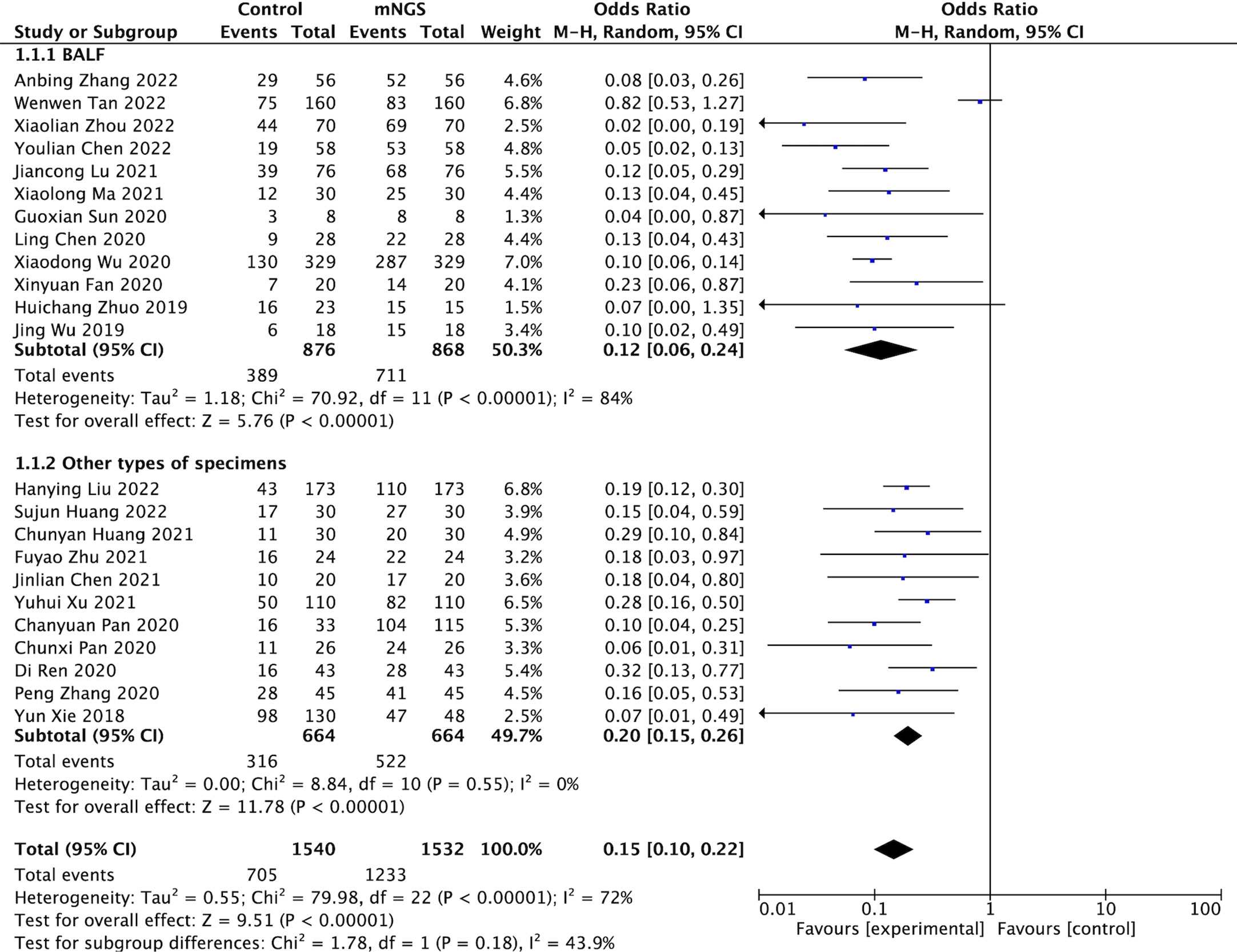
Figure 2 Comparison of pathogen detection positive rate between mNGS and conventional methods group.
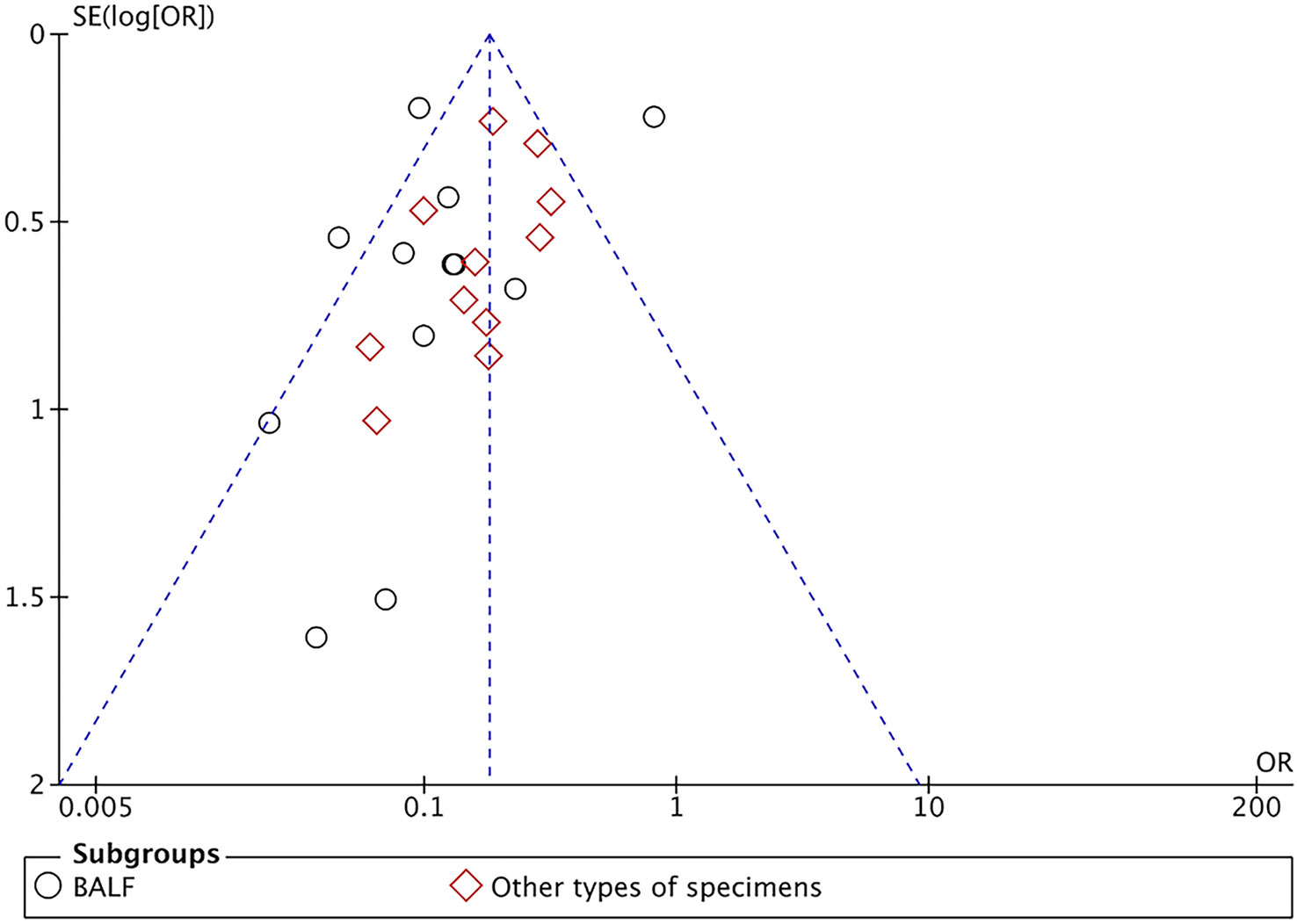
Figure 4 Funnel plot of pathogen detection positive rate between mNGS and conventional methods group.
In addition, twelve studies with a total of 2066 cases assessing detection positive rate of bacteria, fungi, viruses, and other pathogens, respectively. We introduced subgroups including bacteria, fungi, viruses, and other pathogens. In bacteria subgrop, their pooled result indicated that there was no difference in the mNGS group (55.97%, 553/988) and conventional methods group (41.93%, 452/1078) (OR = 1.73, 95% CI (0.73, 4.08), P = 0.21 and I2 = 94%). The meta-analysis results showed that mNGS (17.75%, 163/973, 22.57%, 223/988 and 23.49%, 128/545) effectively increased the detection positive rate of fungi, virus, and other pathogens compared with the conventional methods group (8.44%, 89/1055) (OR = 2.04, 95% CI (1.13, 3.67), P = 0.02 and I2 = 68%), (4.64%, 50/1078) (OR = 4.68, 95% CI (1.43, 15.39), P = 0.01 and I2 = 82%) and (5.79%, 32/553) (OR = 2.59, 95% CI (1.68, 3.98), P=0.001 and I2 = 72%) (Figure S1). The funnel chart indicated publication bias that are presented in Figure S2.
28-day mortality
A total of six studies investigated the 28-day mortality. 252 cases were included in mNGS group, with a pooled mortality of 15.08% (38/252), significantly lower than that in the conventional methods group (33.05%, 117/354). The synthesis of these results derived from comparison with the conventional methods group indicated that mNGS can significantly promote patient survival, with an OR = 0.35, 95% CI (0.23, 0.55), P < 0.001, and I2 = 0% (Figure 5A). The funnel chart indicated no obvious publication bias (Figure S3).
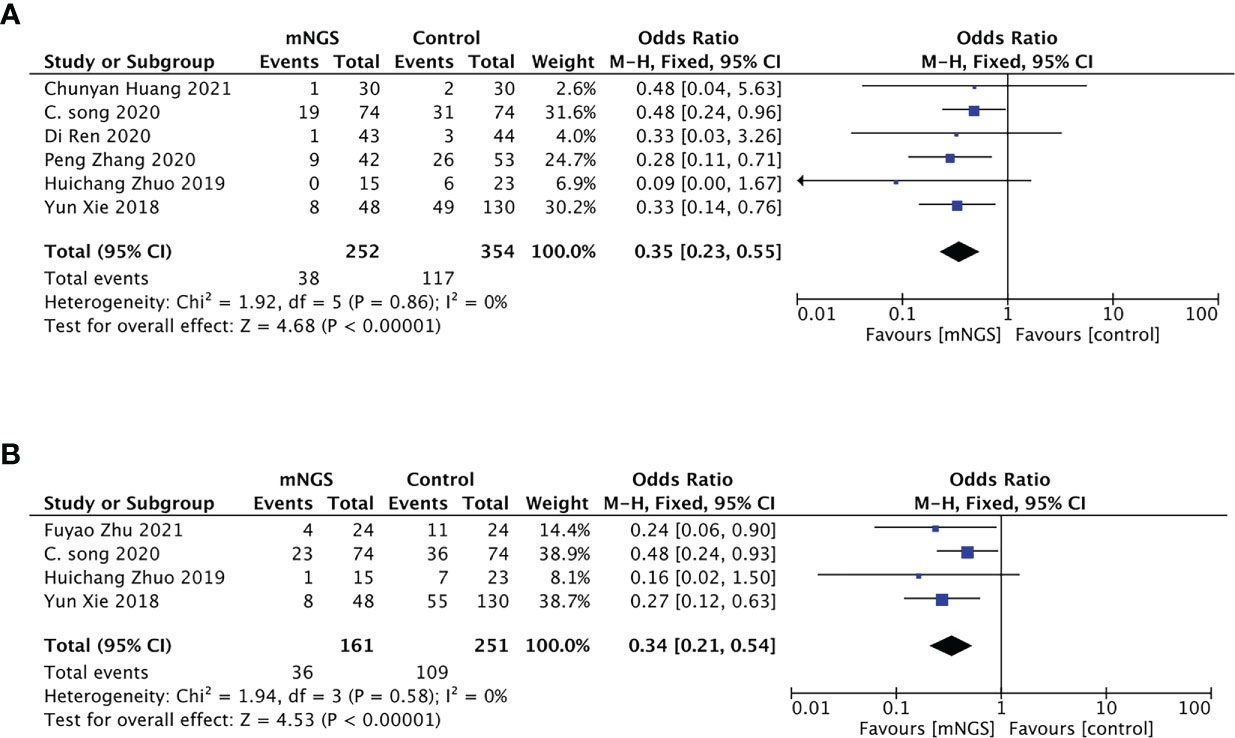
Figure 5 Primary outcome of the meta-analyses of mNGS compared with conventional methods group: (A), 28-day mortality; (B), 90-day mortality.
90-day mortality
Four studies with 161 cases in mNGS group and 251 cases in the conventional methods group reported 90-day mortality. Adjusting treatment based on the pathogen detection results, the pooled 90-day mortality of the mNGS group was significantly lower than that in the conventional methods group (22.36%,36/161 vs 43.43%,109/251) (Figure 5B). There was no obvious publication bias (Figure S4).
Duration of mechanical ventilation
In total, 5 studies including 285 cases in the mNGS group and 296 cases in the conventional methods group investigated the duration of mechanical ventilation. Their pooled result using random model indicated that there was no difference in the mNGS group and the conventional methods group (MD = − 1.82, 95% CI (− 4.39, 0.74), P = 0.16, I2 = 57%) (Figure 6A). The funnel chart (Figure S5) showed no obvious publication bias.
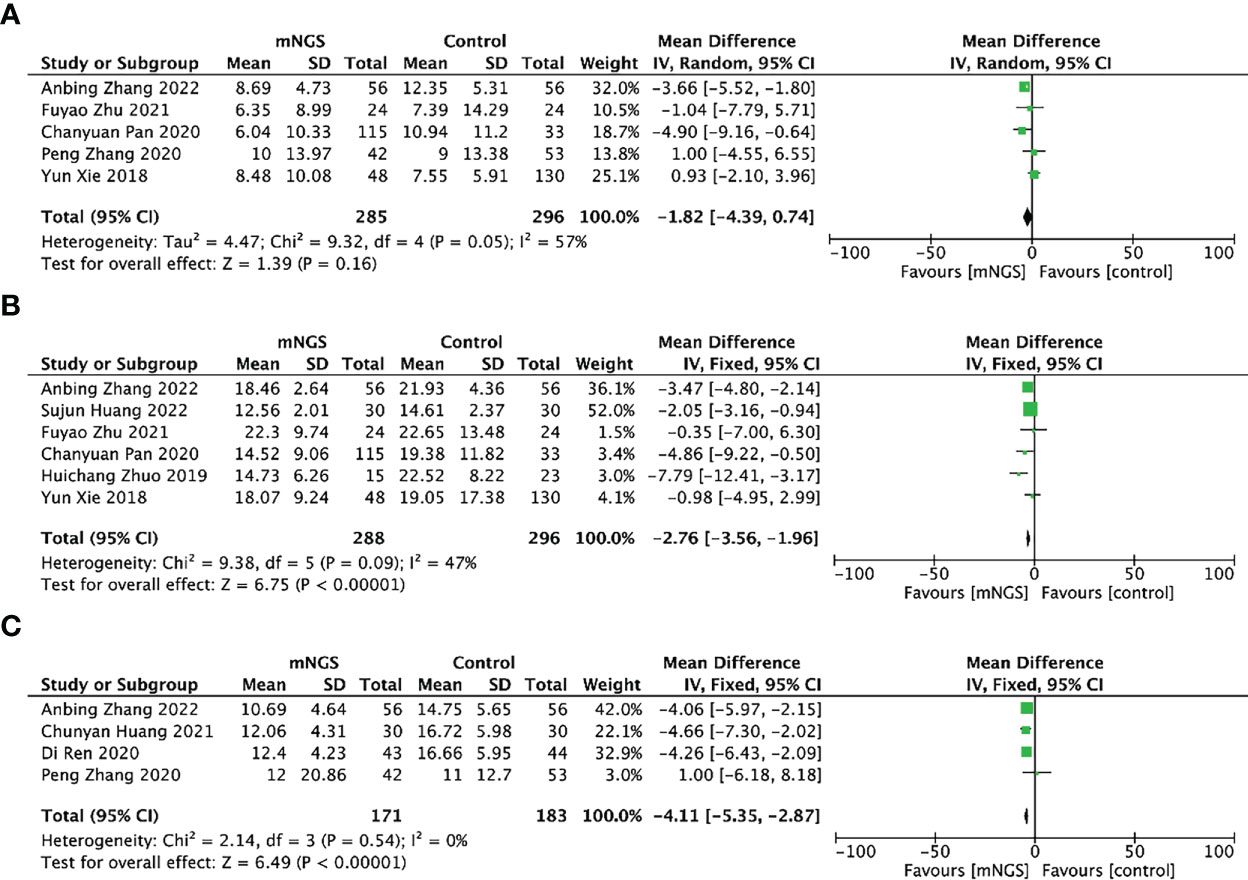
Figure 6 Secondary outcomes of the meta-analyses of mNGS compared with conventional methods group: (A), duration of mechanical ventilation; (B), length of hospital stay; (C), length of stay in ICU.
Length of hospital stay
Six studies including 288 cases in the mNGS group and 296 cases in the conventional methods group reported the length of hospital stay. The mean length of hospital stay of the mNGS group was 16.33, shorter than that of the conventional methods group(19.74). The synthesized results proved that adjusting therapy according to mNGS results could reduce the length of hospital stay when compared with the conventional methods group, MD = -2.76, 95% CI [− 3.56, − 1.96], P < 0.00001, I2 = 47% (Figure 6B). The funnel chart (Figure S6) showed absence of obvious publication bias.
Length of stay in ICU
Four studies including 171 cases in mNGS group and 183 cases in conventional methods group provided data on the length of stay in ICU. The mean length of stay in ICU of mNGS group and conventional methods group were 11.68 and 14.69. Patients who received mNGS detection had shorter length of stay in ICU than those receiving conventional methods detection [MD = -4.11, 95% CI (− 5.35, − 2.87), P < 0.001, I2 = 0%] (Figure 6C). The funnel chart (Figure S7) indicated no obvious publication bias.
Antibiotic regiments changes based on NGS results
We carefully examined the studies included. The effects of mNGS results on antibiotic use were noted in four papers. Among them, Zhang et al. (Zhang et al., 2022) reported that early identification of pathogens through mNGS and timely adjustment of treatment regimens significantly reduced the frequency and duration of antimicrobial drug adjustment, which provides a new direction for antimicrobial drug management. Xu (Xu, 2021) and Chen (Chen, 2020) reported that adjusting antibiotic regimens based on mNGS detection results improved patient outcomes. Pan (Pan, 2020) reported that adjusted treatment based on mNGS results reduced length of hospital stay and duration of mechanical ventilation, and effectively reduced patient mortality.
Discussion
Severe pneumonia is one of the most critical diseases with leading mortality among infectious diseases in ICU (Nair and Niederman, 2021). In the clinic, conventional methods sometimes fail to find the pathogen in patients with severe pneumonia. A reliable study (Chalmers et al., 2011) shows that the fatality rate is as high as 50% in patients who receive only empiric therapy without reliable evidence of pathogenic microorganisms. Prompt targeted antimicrobial therapy treatment is an effective means to improve the survival rate of patients with severe pneumonia.
The detection of DNA or RNA by mNGS provides rapid, efficient, and accurate access to the entire pathogen genome information within the whole test sample, which has been used widely for pathogen diagnosis in clinic in recent years (Miller et al., 2019). However, to our knowledge, there is no relevant systematic review and meta-analysis to provide more reliable evidence on the clinical values of mNGS on severe pneumonia. This study is performed to evaluate the diagnostic value and prognostic impact of mNGS compared with conventional methods in patients with severe pneumonia.
In order to accurately assess the pathogenic diagnostic value of mNGS in severe pneumonia and its impact on prognosis, we comprehensively collected the studies published on the application of mNGS to the etiological diagnostic of severe pneumonia compared with conventional methods, and finally included 23 studies for meta-analysis. The pathogen detection positive rate of the mNGS group was 80.48% (1233/1532), much higher than that of the conventional methods group (45.78%, 705/1540), which indicated that mNGS has a higher diagnostic value than conventional methods and can be used as an effective method for rapid etiological diagnosis of severe pneumonia. Bacteria can be detected by both mNGS and traditional detection methods. mNGS is superior for detecting fungi, viruses, and rare pathogens, which can be used as an adjunct or complementary tool to conventional microbial testing methods to provide an etiologic basis for the accurate anti-infection treatment of severe pneumonia. It is well known that the traditional microbial detection method is susceptible to the influence of normal bacteria in the body, especially after using antibiotics. Patients with severe pneumonia are often initially treated with an anti-microbial therapy, which reduces the positive rate of detection by conventional methods. mNGS can improve the pathogen detection positive rate and is less affected by external influences (Gu et al., 2019). Moreover, some reports have confirmed that mNGS achieve a faster diagnosis of pathogens and can detect unknown pathogens and even drug-resistance genes (Crofts et al., 2017). Another clinical application of mNGS is to identify microbial colonization or infection by monitoring the immune response of patients, ultimately achieving rational application of antibiotics, suppressing bacterial resistance, and reducing the economic and social burden of infectious diseases (Besser et al., 2018).
Meanwhile, compared with the conventional methods group, adjusting the treatment based on the mNGS results significantly decreased 28-day and 90-day mortality and shortened the length of hospital and ICU stay of patients with severe pneumonia. Patients with serious conditions have a short window of time for clinicians to save their lives and precise treatment is crucial to their prognosis. mNGS was faster, taking an average of two days, whereas conventional methods required at least three to five days (Xie et al., 2019). In addition, the higher pathogen-positive rate of mNGS detection gives clinicians the opportunity to select accurate anti-microbial drugs as early as possible, greatly increasing the proportion of target treatments. mNGS lead to more rapid required, accurate diagnosis than conventional methods in severe pneumonia. As a result, mNGS was associated with a better clinical prognosis of severe pneumonia.
mNGS facilitates accurate diagnosis and treatment. Still, there are many challenges, such as the lack of common standards for outcome analysis and common guidelines for report interpretation. The quality and stability of mNGS analysis results may not be stable to some extent, which is closely related to the operator’s skill and the detection efficiency of different laboratories may not be compared. A key challenge inherent to mNGS is that microbial nucleic acids from most patients’ samples are dominated by human host backgrounds (Hasan et al., 2016). Alternative methods exist for the depletion of human background DNA during the preanalytical phase, one approach is to selectively lyse human white blood cells using saponin or other chemical reagents (Marotz et al., 2018), and a different approach is to target low-molecular-weight cell-free DNA or RNA and remove high-molecular-weight genetic content that is often associated with human genomic material (Bukowska-Ośko et al., 2016). Another potential disadvantage of mNGS is the contamination of the sample. We need strictly adhere to quality control procedures for reagents and workflows to maintain as sterile and nucleic acid-free a testing environment as possible. The use of negative controls, reagent evaluation, and regular brushing is needed to ensure that laboratory and sample cross-contamination does not produce false positive results (Schlaberg et al., 2017). In addition, mNGS detection is highly sensitive and requires clinicians with rich experience to comprehensively consider the patient’s condition to make a judgment.
The included studies have limitations, such as small sample sizes, single-centered and retrospective analysis (Pan, 2020; Sun et al., 2020; Zhang et al., 2022), and confounding bias that could not be completely excluded. Besides, all 24 studies included were from China, making the inclusion of a homogeneous population and thus limiting the extensibility of the results. This limits the external generalizability of the results to some degree. Finally, we could not conduct a SORC curve analysis to assess the diagnostic efficacy of mNGS and conventional methods for severe pneumonia due to the scarcity of studies reporting sensitivity and specificity.
Conclusion
The pathogen detection positive rate of mNGS was much higher than that of conventional methods, thereby indicating that mNGS has an extremely good diagnostic performance for severe pneumonia. Besides, adjusting treatment based on mNGS results can reduce the 28-day and 90-day mortality of patients with severe pneumonia, and shorten the length of hospital and ICU stay. Therefore, we suggest that patients with severe pneumonia should be tested for mNGS in addition to traditional culture as early as possible to improve the prognosis and reduce the length of hospital stay.
Author contributions
We describe contributions to the paper as follows: conceptualization - ML, XD and GF. methodology - ML and XD. validation - ML and JY. formal analysis - ML and XD. data curation – ML, ChaZ and JY. writing original draft – ML. writing, review and editing - all listed. visualization - ML, XD and GF. supervision - XD and GF. All authors contributed to the article and approved the submitted version.
Funding
This work was supported by the National Natural Science Foundation of China (82070017, 82100014), Natural Science Foundation of Jiangsu Province (BK20210981), and Basic Science Foundation of Jiangsu Universities (21KJB320002).
Conflict of interest
The authors declare that the research was conducted in the absence of any commercial or financial relationships that could be construed as a potential conflict of interest.
Publisher’s note
All claims expressed in this article are solely those of the authors and do not necessarily represent those of their affiliated organizations, or those of the publisher, the editors and the reviewers. Any product that may be evaluated in this article, or claim that may be made by its manufacturer, is not guaranteed or endorsed by the publisher.
Supplementary material
The Supplementary Material for this article can be found online at: https://www.frontiersin.org/articles/10.3389/fcimb.2023.1106859/full#supplementary-material
References
Besser, J., Carleton, H. A., Gerner-Smidt, P., Lindsey, R. L., Trees, E. (2018). Next-generation sequencing technologies and their application to the study and control of bacterial infections. Clin. Microbiol. Infect. 24, 335–341. doi: 10.1016/j.cmi.2017.10.013
Bukowska-Ośko, I., Perlejewski, K., Nakamura, S., Motooka, D., Stokowy, T., Kosińska, J., et al. (2016). Sensitivity of next-generation sequencing metagenomic analysis for detection of RNA and DNA viruses in cerebrospinal fluid: the confounding effect of background contamination. Adv. Exp. Med. Biol 944, 53–62. doi: 10.1007/5584_2016_42
Chalmers, J. D., Taylor, J. K., Mandal, P., Choudhury, G., Singanayagam, A., Akram, A. R., et al. (2011). Validation of the infectious diseases society of America/American thoratic society minor criteria for intensive care unit admission in community-acquired pneumonia patients without major criteria or contraindications to intensive care unit care. Clin. Infect. Dis. 53, 503–511. doi: 10.1093/cid/cir463
Chen, L. (2020). Application value of next - generation sequencing in pulmonary infectious diseases (Nanhua University). MD. doi: 10.27234/d.cnki.gnhuu.2020.000208
Chen, S., Kang, Y., Li, D., Li, Z. (2022). Diagnostic performance of metagenomic next-generation sequencing for the detection of pathogens in bronchoalveolar lavage fluid in patients with pulmonary infections: systematic review and meta-analysis. Int. J. Infect. Dis. 122, 867–873. doi: 10.1016/j.ijid.2022.07.054
Chen, Y., Liu, X., Chen, H., Hong, C., Zhang, H. (2022). Clinical value of bronchoalveolar lavage fluid metagenomic next-generation sequencing in patients with severe pneumonia. Modern Med Health 38 (1), 31–34. doi: 10.3969/j.issn.1009-5519.2022.01.007
Chen, M., Lu, W., Wu, S., Wang, S., Lu, T., Peng, C. (2021). Metagenomic next-generation sequencing in the diagnosis of leptospirosis presenting as severe diffuse alveolar hemorrhage: a case report and literature review. BMC Infect. Dis. 21, 1230. 10/gpqrcw. doi: 10.1186/s12879-021-06923-w
Chen, J., Zhao, Y., Shang, Y., Lin, Z., Xu, G., Bai, B., et al. (2021). The clinical significance of simultaneous detection of pathogens from bronchoalveolar lavage fluid and blood samples by metagenomic next-generation sequencing in patients with severe pneumonia. J. Med. Microbiol. 70 (1), 001259. doi: 10.1099/jmm.0.001259
Chiu, C. Y., Miller, S. A. (2019). Clinical metagenomics. Nat. Rev. Genet. 20, 341–355. 10/gfxjff. doi: 10.1038/s41576-019-0113-7
Cillóniz, C., Torres, A., Niederman, M. S. (2021). Management of pneumonia in critically ill patients. BMJ 375, e065871. doi: 10.1136/bmj-2021-065871
Crofts, T. S., Gasparrini, A. J., Dantas, G. (2017). Next-generation approaches to understand and combat the antibiotic resistome. Nat. Rev. Microbiol. 15, 422–434. 10/f9z9ps. doi: 10.1038/nrmicro.2017.28
De Pascale, G., Bello, G., Antonelli, M. (2011). Steroids in severe pneumonia: a literature review. Minerva Anestesiol 77, 902–910.
Fan, X. (2020). Application value of high-throughput sequencing technology in pulmonary infectious diseases (MD. Zhejiang University). doi: 10.27461/d.cnki.gzjdx.2020.002789
Gu, W., Miller, S., Chiu, C. Y. (2019). Clinical metagenomic next-generation sequencing for pathogen detection. Annu. Rev. Pathol. 14, 319–338. 10/ggdk5v. doi: 10.1146/annurev-pathmechdis-012418-012751
Hasan, M. R., Rawat, A., Tang, P., Jithesh, P. V., Thomas, E., Tan, R., et al. (2016). Depletion of human DNA in spiked clinical specimens for improvement of sensitivity of pathogen detection by next-generation sequencing. J. Clin. Microbiol. 54, 919–927. doi: 10.1128/JCM.03050-15
Huang, C. (2021). Value of high-throughput sequencing NGS technology in the examination of severe pulmonary infection. Dietary Health 25, 267. doi: 2095-8439(2021)25-0267-01
Huang, S. (2022). Effect of clinical pharmacists participating in anti-infective therapy of severe pneumonia under metagenomics sequencing. J. Modern Diagnosis Treat. 33 (07), 1063–1065. doi: 1001-8174(2022)07-1063-02
Huang, J., Jiang, E., Yang, D., Wei, J., Zhao, M., Feng, J., et al. (2020). Metagenomic next-generation sequencing versus traditional pathogen detection in the diagnosis of peripheral pulmonary infectious lesions. Infect. Drug Resist. 13, 567–576. 10/gh3skv. doi: 10.2147/IDR.S235182
Kalil, A. C., Metersky, M. L., Klompas, M., Muscedere, J., Sweeney, D. A., Palmer, L. B., et al. (2016). Executive summary: management of adults with hospital-acquired and ventilator-associated pneumonia: 2016 clinical practice guidelines by the infectious diseases society of America and the American thoracic society. Clin. Infect. Dis. 63, 575–582. doi: 10.1093/cid/ciw504
Li, N., Li, S., Tan, W., Wang, H., Xu, H., Wang, D. (2021). Metagenomic next-generation sequencing in the family outbreak of psittacosis: the first reported family outbreak of psittacosis in China under COVID-19. Emerg. Microbes Infect. 10, 1418–1428. 10/gmsjh3. doi: 10.1080/22221751.2021.1948358
Liu, H., Zhang, Y., Chen, G., Sun, S., Wang, J., Chen, F., et al. (2022). Diagnostic significance of metagenomic next-generation sequencing for community-acquired pneumonia in southern China. Front. Med. (Lausanne) 9, 807174. 10/gpspgm doi: 10.3389/fmed.2022.807174
Lu, J., Xie, Y., Ye, K., Lin, J. (2021). Clinical significance of mNGS in the diagnosis of pathogens in patients with severe immunodeficiency pneumonia. J. Pulmonary Disease 14 (3), 360–362. doi: 10.3877/cma.j.issn.1674-6902.2021.03.026
Ma, X., Gao, F., Feng, S. (2021). Application of next generation gene sequencing in the diagnosis and treatment of severe pneumonia complicated with immunosuppression. Modern Pract. Med. 33 (12), 1564–1566. doi: 10.3969/j.issn.1671-0800.2021.12.010
Marotz, C. A., Sanders, J. G., Zuniga, C., Zaramela, L. S., Knight, R., Zengler, K. (2018). Improving saliva shotgun metagenomics by chemical host DNA depletion. Microbiome 6, 42. doi: 10.1186/s40168-018-0426-3
Metlay, J. P., Waterer, G. W., Long, A. C., Anzueto, A., Brozek, J., Crothers, K., et al. (2019). Diagnosis and treatment of adults with community-acquired pneumonia. An official clinical practice guideline of the American thoracic society and infectious diseases society of America. Am. J. Respir. Crit. Care Med. 200, e45–e67. doi: 10.1164/rccm.201908-1581ST
Miller, S., Naccache, S. N., Samayoa, E., Messacar, K., Arevalo, S., Federman, S., et al. (2019). Laboratory validation of a clinical metagenomic sequencing assay for pathogen detection in cerebrospinal fluid. Genome Res. 29, 831–842. doi: 10.1101/gr.238170.118
Nair, G. B., Niederman, M. S. (2021). Updates on community acquired pneumonia management in the ICU. Pharmacol. Ther. 217, 107663. 10/gmzz9g. doi: 10.1016/j.pharmthera.2020.107663
Pan, C. (2020) Clinical diagnosis and treatment value of next generation sequencing in severe pneumonia. doi: 10.27137/d.cnki.ghusu.2020.000833.
Pan, C., Lv, L. (2020). Application value of metagenome-based next generation sequencing technology in rapid pathogen detection in patients with severe pneumonia. Chin. J. New Clin. Med. 13, 370–373. doi: 10.3969/j.issn.1674-3806.2020.04.12
Ren, D., Li, Y., Zeng, J., Tan, T. (2020). Application effect of high-throughput sequencing in patients with severe pulmonary infection. China Med. Rev. 17 (7), 94–97. doi: 1673-7210(2020)03(a)-0094-04
Schlaberg, R., Chiu, C. Y., Miller, S., Procop, G. W., Weinstock, G., Professional Practice Committeeand Committee on Laboratory Practices of the American Society for Microbiology, et al. (2017). Validation of metagenomic next-generation sequencing tests for universal pathogen detection. Arch. Pathol. Lab. Med. 141, 776–786. doi: 10.5858/arpa.2016-0539-RA
Song, C., Fan, Y., Lin, F., Pan, P., Li, Y. (2020). Next-generation sequencing guides the treatment of severe pneumonia with initial empiric therapy failure. Am. J. Respir. Crit. Care Med. 201, A2941. doi: 10.1164/ajrccm-conference.2020.201.1_MeetingAbstracts.A2941
Sun, G., Liu, W., Zheng, Q., Yuan, W., Hou, H. (2020). Application of next generation sequencing of pulmonary alveolar lavage fluid in patients with severe pneumonia with unknown pathogenic bacteria. J Chin. Primary Med. 27 (24), 2951–2955. doi: 10.3760/cma.j.issn.1008-6706.2020.24.003
Tan, W., Li, J., Pan, C., Tang, C., Liu, H. (2022). Analysis of the positive status of next generation sequencing and influencing factors in elderly patients with severe pneumonia bacterial infection. J. Hunan Normal University(Medical Science) 19, 23–27. doi: 10.3969/j.issn.1673-016X.2022.02.007
Torres, A., Cilloniz, C., Niederman, M. S., Menéndez, R., Chalmers, J. D., Wunderink, R. G., et al. (2021). Pneumonia. Nat. Rev. Dis. Primers 7, 25. 10/gjqxsw. doi: 10.1038/s41572-021-00259-0
Wang, J., Han, Y., Feng, J. (2019). Metagenomic next-generation sequencing for mixed pulmonary infection diagnosis. BMC Pulm Med. 19, 252. 10/ghcwnb. doi: 10.1186/s12890-019-1022-4
Wu, J. (2019). Application of macrogene next-generation sequencing in the diagnosis of autoimmune diseases complicated with severe pneumonia. MD. Zhejiang Univ, 1-55. doi: 10.27461/d.cnki.gzjdx.2019.001558
Wu, X., Li, Y., Zhang, M., Li, M., Zhang, R., Lu, X., et al. (2020). Etiology of severe community-acquired pneumonia in adults based on metagenomic next-generation sequencing: A prospective multicenter study. Infect. Dis. Ther. 9, 1003–1015. 10/gpsp7g. doi: 10.1007/s40121-020-00353-y
Wu, D., Wang, W., Xun, Q., Wang, H., Liu, J., Zhong, Z., et al. (2022). Metagenomic next-generation sequencing indicates more precise pathogens in patients with pulmonary infection: a retrospective study. Front. Cell Infect. Microbiol. 12. doi: 10.3389/fcimb.2022.977591
Xi, Y., Zhou, J., Lin, Z., Liang, W., Yang, C., Liu, D., et al. (2022). Patients with infectious diseases undergoing mechanical ventilation in the intensive care unit have better prognosis after receiving metagenomic next-generation sequencing assay. Int. J. Infect. Dis. 122, 959–969. doi: 10.1016/j.ijid.2022.07.062
Xie, Y., Du, J., Jin, W., Teng, X., Cheng, R., Huang, P., et al. (2019). Next generation sequencing for diagnosis of severe pneumonia: China 2010-2018. J. Infect. 78, 158–169. 10/gnc4k5. doi: 10.1016/j.jinf.2018.09.004
Xu, Y. (2021). The value of metagenomic next generation sequencing in the etiological detection of severe pneumonia. MD. Zhejiang University, 1–60. doi: 10.27162/d.cnki.gjlin.2021.006486
Zhang, P., Chen, Y., Li, S., Li, C., Zhang, S., Zheng, W., et al. (2020). Metagenomic next-generation sequencing for the clinical diagnosis and prognosis of acute respiratory distress syndrome caused by severe pneumonia: a retrospective study. PeerJ 8, e9623. doi: 10.7717/peerj.9623
Zhang, A., Yuan, X., Xia, X., Lv, Y., Zhang, D., Liang, J. (2022). Application of macrogene next-generation sequencing technology in pathogen detection and treatment planning of severe pneumonia. Shandong Pharm 62 (3), 16–19. doi: 10.3969/j.issn.1002-266X.2022.03.004
Zhou, H., Larkin, P. M. K., Zhao, D., Ma, Q., Yao, Y., Wu, X., et al. (2021). Clinical impact of metagenomic next-generation sequencing of bronchoalveolar lavage in the diagnosis and management of pneumonia: a multicenter prospective observational study. J. Mol. Diagn. 23, 1259–1268. 10/gppfsx. doi: 10.1016/j.jmoldx.2021.06.007
Zhou, X., Yang, J. (2022). Metagenomic next-generation sequencing in the detection of alveolar lavage fluid in severe pneumonia. J. Geomatics Wuhan Univ. (Medical Edition), 1–6. doi: 10.14188/j.1671-8852.2021.0912
Zhu, F., Cao, Z. (2021). Application value of metagenomic next generation gene sequencing of alveolar lavage fluid in etiological diagnosis of severe pneumonia. Contemp. Med. Literature 4, 5. doi: 10.3969/j.issn.2095-7629.2021.10.048
Zhu, C., Lv, M., Huang, J., Zhang, C., Xie, L., Gao, T., et al. (2022). Bloodstream infection and pneumonia caused by chlamydia abortus infection in China: A case report. BMC Infect. Dis. 22, 181. 10/gpqrc3. doi: 10.1186/s12879-022-07158-z
Keywords: metagenomic next-generation sequencing, severe pneumonia, diagnosis, prognosis, conventional methods
Citation: Lv M, Zhu C, Zhu C, Yao J, Xie L, Zhang C, Huang J, Du X and Feng G (2023) Clinical values of metagenomic next-generation sequencing in patients with severe pneumonia: a systematic review and meta-analysis. Front. Cell. Infect. Microbiol. 13:1106859. doi: 10.3389/fcimb.2023.1106859
Received: 24 November 2022; Accepted: 22 March 2023;
Published: 06 April 2023.
Edited by:
Michael Marceau, Université Lille Nord de France, FranceReviewed by:
İlhami Çelik, University of Health Sciences, TürkiyeYuanlin Song, Fudan University, China
Copyright © 2023 Lv, Zhu, Zhu, Yao, Xie, Zhang, Huang, Du and Feng. This is an open-access article distributed under the terms of the Creative Commons Attribution License (CC BY). The use, distribution or reproduction in other forums is permitted, provided the original author(s) and the copyright owner(s) are credited and that the original publication in this journal is cited, in accordance with accepted academic practice. No use, distribution or reproduction is permitted which does not comply with these terms.
*Correspondence: Xingran Du, eGluZ3JhbmR1QG5qbXUuZWR1LmNu; Ganzhu Feng, Zmd6NjI2OTFAMTYzLmNvbQ==