- 1Department of Clinical Laboratory, Institute of Translational Medicine, Renmin Hospital of Wuhan University, Wuhan, China
- 2State Key Laboratory of Virology, College of Life Sciences, Wuhan University, Wuhan, China
- 3Department of Pharmacy, Renmin Hospital of Wuhan University, Wuhan, China
In the context of the global COVID-19 pandemic, the phenomenon that the elderly have higher morbidity and mortality is of great concern. Existing evidence suggests that senescence and viral infection interact with each other. Viral infection can lead to the aggravation of senescence through multiple pathways, while virus-induced senescence combined with existing senescence in the elderly aggravates the severity of viral infections and promotes excessive age-related inflammation and multiple organ damage or dysfunction, ultimately resulting in higher mortality. The underlying mechanisms may involve mitochondrial dysfunction, abnormal activation of the cGAS-STING pathway and NLRP3 inflammasome, the role of pre-activated macrophages and over-recruited immune cells, and accumulation of immune cells with trained immunity. Thus, senescence-targeted drugs were shown to have positive effects on the treatment of viral infectious diseases in the elderly, which has received great attention and extensive research. Therefore, this review focused on the relationship between senescence and viral infection, as well as the significance of senotherapeutics for the treatment of viral infectious diseases.
1 Introduction
Although the increasing aging population worldwide indicates that the average life expectancy of humans has lengthened, a simultaneous increase in age-related chronic diseases has also been observed. Simultaneously, there has been unprecedented interest in aging-related research, especially during the global COVID-19 pandemic, in which the elderly were found to suffer from higher morbidity and mortality compared to other age groups (Grasselli et al., 2020; Onder et al., 2020).
Age is considered a critical risk for the severity of COVID-19 disease (Zhou et al., 2020; Wang et al., 2020a). Data from China showed that the case fatality rate (CFR) of COVID-19 increased with age, and the CFR for patients aged 40 years or younger was ≤0.4%, but rose to 8.0% in patients aged 70 to 79 years, and 14.8% in patients aged ≥80 years (The Novel Coronavirus Pneumonia Emergency Response Epidemiology Team, 2020). Similarly, data from Italy revealed that CFR was also ≤0.4% for patients aged ≤40 years and, rose to 12.8% and 20.2% in patients aged 70 - 79 years and ≥80 years, respectively (Onder et al., 2020). In addition, a retrospective study on 5256 COVID-19 patients in the United States found that old age, male sex and impaired physical or cognitive function were independent risk factors for 30-day mortality (Panagiotou et al., 2021). Overall, current epidemiological evidence suggests that elderly COVID-19 patients (age ≥80 years) have a significantly higher risk of death than younger patients (Ackermann et al., 2020; Akbar and Gilroy, 2020). Moreover, higher mortality rates have also been reported in the elderly with influenza virus and respiratory syncytial virus infections (Thompson et al., 2003).
Thus, there are several unanswered questions between viral infections and senescence, such as: why do older people have higher morbidity and mortality from viral infections or how do viral infections and senescence interact and influence each other? To answer these questions, this review focuses on the relationship between senescence and viral infections and discusses the significance of senescence-targeted drugs for the treatment of viral infectious diseases, so as to provide insights for better understanding the role of senescence in disease development.
2 Characteristics of senescence
A basic feature of aged organisms is the accumulation of senescent cells. Senescence is a permanent status of cell cycle arrest in normal proliferating cells, described back in the 1960s when Hayflick and Moorhead found that the proliferation ability of cultured human diploid cells was limited and that cells stopped proliferating after serial passage in vitro (Hayflick and Moorhead, 1961; Hayflick, 1965; Lynch et al., 2021). Since then, biologists have gained a more comprehensive understanding on the characteristics associated with senescence.
2.1 Inducing factors of senescence
Cellular senescence is caused by repeated cell divisions and cellular stressors (Kelley et al., 2020). Replicative senescence results from repeated cellular divisions and has been confirmed to be related to the gradual shortening of telomeres during cell division (Shay, 2016; Lynch et al., 2021). Stress-induced senescence arises from cellular stressors, such as oncogene activation, DNA damage, reactive oxygen species (ROS), mitochondrial dysfunction and epigenetic stress (Hernandez-Segura et al., 2018), which may result from irradiation, chemotherapeutic drugs, pathogen infections, long-term exposure to pollutants (cigarette smoke) and certain aging syndromes such as progeria (Nyunoya et al., 2006; Nyunoya et al., 2009; Wheaton et al., 2017; Kelley et al., 2020; Di Micco et al., 2021) (Figure 1). Therefore, cells from both young and aged hosts may exhibit senescent properties (Kelley et al., 2020).
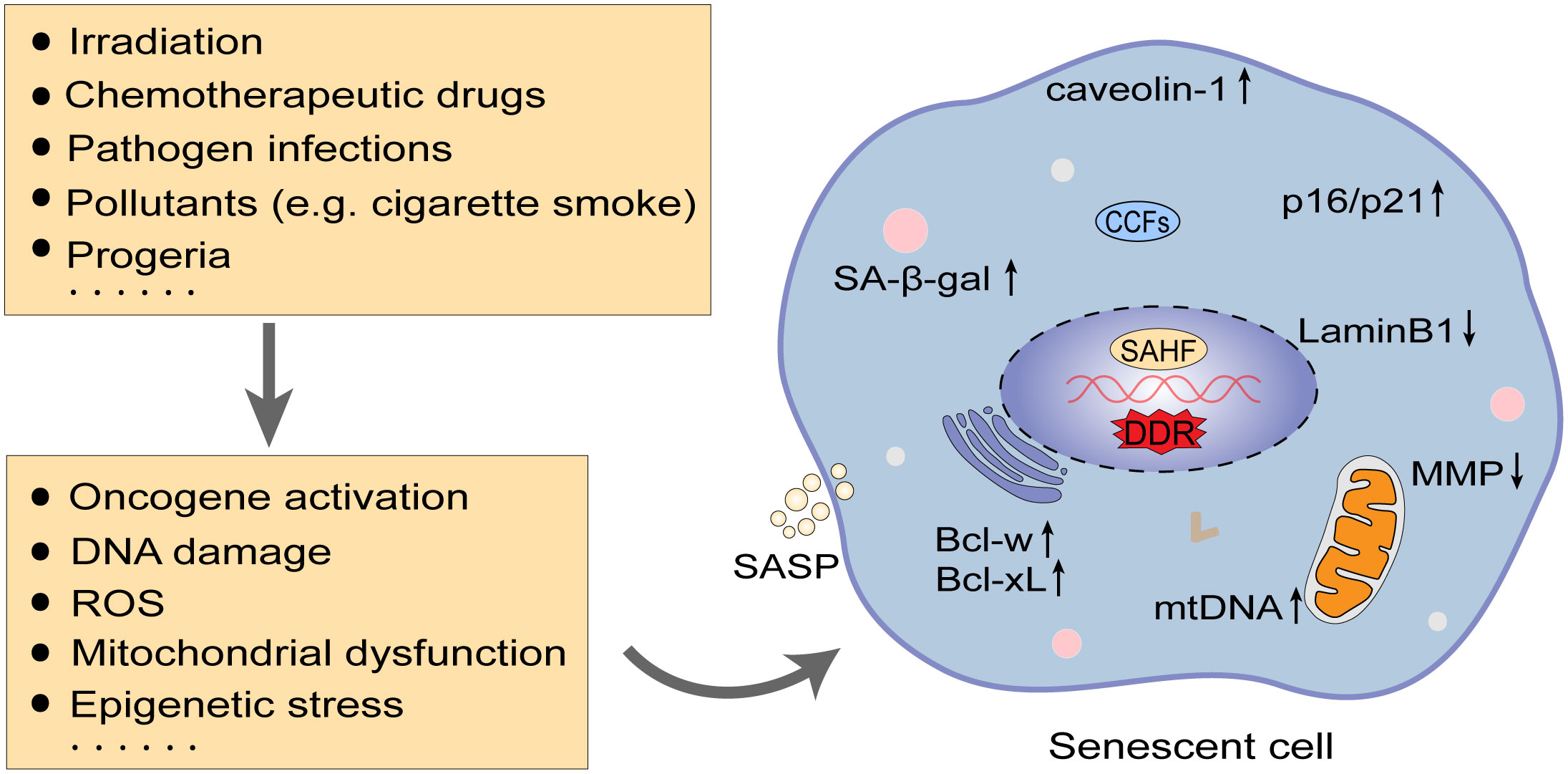
Figure 1 Inducing factors and characteristics of senescence. In addition to replicative senescence induced by telomere shortening, numerous factors such as irradiation, chemotherapeutic drugs, and pathogenic infections can evoke senescence by impacting oncogene activation, DNA damage, mitochondrial dysfunction, etc. DNA damage response (DDR) is an essential mechanism in triggering senescence. Cellular senescence features altered cell morphology and structure, arrest of the cell cycle (upregulation of p16/p21), and enhanced resistance to apoptosis (upregulation of Bcl-xL and Bcl-w), along with detectable nuclear senescence-associated heterochromatin foci (SAHFs), increased senescence-associated β-galactosidase (SA-β-gal) activity, and secretion of senescence-associated secretory phenotype (SASP). ROS, reactive oxygen species; MMP, membrane potential; CCFs, cytoplasmic chromatin fragments; mtDNA, mitochondrial DNA.
Activation of DNA damage response (DDR) signaling cascades initiated by nucleus DNA double-strand breaks (DSBs) is considered to be a common factor to induce cellular senescence (Di Micco et al., 2021). Specifically, there are two kinases upstream of DDR, known as ataxia telangiectasia mutated (ATM) and ATM- and Rad3-Related (ATR) protein kinases, they are respectively activated by the MRE11-RAD50-NBS1 (MRN) complex at DSBs and the TopBP1 or ETAA1 at replication protein A coated ssDNA (RPA-ssDNA). Activated ATR and ATM can further phosphorylate the downstream kinases CHK1 and CHK2, respectively, which in turn activate the p53 pathway, leading to cell cycle arrest (Blackford and Jackson, 2017; Di Micco et al., 2021). Importantly, factors that can cause DNA damage, such as telomere shortening, oncogene activation and ROS, would ultimately be involved in activating the DDR pathway(van Deursen, 2014). If DNA damage from various causes persists, prolonged DDR signaling and proliferation arrest can invoke the onset of cellular senescence (Fumagalli et al., 2014). In addition, studies have reported that IFN-β secreted by senescent cells can stimulate DDR through ROS and generate senescence-like cell cycle arrest in human fibroblasts, which can trigger positive feedback activation of DDR and further amplify the senescence phenotype (Yu et al., 2015).
2.2 Hallmarks of senescence
Senescent cells have various characteristics (Hernandez-Segura et al., 2018) (Figure 1): (1) Morphologically, senescent cells are abnormally enlarged and flattened, with a disproportionate increase in the cytoplasm and nuclei (Bent et al., 2016; Druelle et al., 2016; Cormenier et al., 2018; Di Micco et al., 2021); changes in the composition of the plasma membrane, such as caveolin-1 protein upregulation (Dasari et al., 2006; Chrétien et al., 2008; Althubiti et al., 2014); increased lysosome content and some proteins (Cho and Hwang, 2012); accumulated mitochondria and decreased membrane potential (MMP) (Passos et al., 2007; Korolchuk et al., 2017; Tai et al., 2017); nuclear membrane structural protein loss, such as the downregulation of LaminB1 protein and presence of nuclear senescence-associated heterochromatin foci (SAHFs) with detectable dense 4’,6-diamidino-2-phenylindole (DAPI)-positive nuclear structural features (Di Micco et al., 2011; Sadaie et al., 2013); (2) Elevated senescence-associated β-galactosidase (SA-β-gal) activity: SA-β-gal is a lysosomal-derived enzyme that is regarded as a surrogate marker for increased lysosomal content in senescent cells and is one of the most common markers of senescence (Dimri et al., 1995; Kurz et al., 2000; Lee et al., 2006); (3) Accumulation of cyclin-dependent kinase inhibitors (CDKis): Senescence-related cell cycle arrest is primarily driven by CDKis encoded at CDKN2A (p16INK4a or p16), CDKN2B (p15INK4b or p15) and CDKN1A (p21CIP1 or p21) loci. p21 and p16 maintain the tumor suppressor protein pRb in an inactive hypophosphorylated state, thereby preventing the transcription factor E2F from transcribing genes that promote cell cycle progression, and both are often used as unique senescence hallmarks to identify senescent cells in tissues and cultured cells (Narita et al., 2003; Baker et al., 2011; Di Micco et al., 2021); (4) Senescence-associated secretory phenotype (SASP): SASP consists of various cytokines, chemokines and some enzymes involved in extracellular matrix remodeling, mainly including IL-1α/β, IL-6, IL-8, TNF-α, TGF-β, monocyte chemotactic protein 1 (MCP1, also known as CCL-2) and matrix metalloproteinases (MMPs). SASP is thought to be the main mechanism by which senescent cells exert their pleiotropic biological functions and can also induce paracrine senescence (Freund et al., 2010; Acosta et al., 2013; Tchkonia et al., 2013; Gorgoulis et al., 2019); (5) Enhanced apoptosis resistance: Senescent cells stimulate a wide range of pro-survival factors, such as BCL-2 family members, particularly Bcl-xL and Bcl-w, which can be resistant to apoptosis and favor the survival of senescent cells (Childs et al., 2014; Yosef et al., 2016).
Defects associated with aging of the immune system are another feature of aging, termed “immunosenescence” (Kelley et al., 2020). It is characterized by decreased proliferation of hematopoietic stem cells, dysfunction of innate immunity, degeneration of the thymus and reduced numbers of naïve T and B cells, as well as accumulation of memory T and B cells, and decline in T and B cell functions (Kelley et al., 2020; Xu et al., 2020). Immunosenescence is associated with increased susceptibility to various diseases, such as infections, cancer, cardiovascular diseases, hypertension, diabetes, neurological dysfunction, and autoimmune diseases (Xu et al., 2020).
3 Virus-induced senescence
Virus infections can prematurely stimulate cellular senescence, known as virus-induced senescence (VIS). Studies have shown that some viruses, such as the human immunodeficiency virus (HIV), measles virus (MV), respiratory syncytial virus (RSV) and influenza virus, can induce cell fusion and form multinucleated cells upon infecting the organism as a mechanism for expanding its spread in the infected organisms (Chen and Olson, 2005; Duelli and Lazebnik, 2007; Sapir et al., 2008; Delpeut et al., 2012). MV infection has been proven to induce p53 and p16-pRb pathway-dependent cellular senescence via cell fusion (Chuprin et al., 2013). Epstein–Barr virus (EBV), Kaposi sarcoma herpesvirus (KSHV) and human RSV infections can trigger DNA damage-mediated cellular senescence through replicative stress or induction of mitochondrial ROS (Koopal et al., 2007; Martínez et al., 2016; Hafez and Luftig, 2017). Some viral proteins, such as NS1 of influenza A virus (IAV) (Yan et al., 2017), HBx of hepatitis B virus (HBV) (Idrissi et al., 2016) and IE2 of human cytomegalovirus (CMV) (Noris et al., 2002), were found to induce senescence by increasing the inducible NO synthase (iNOS) expression and NO release and regulating the p21 and p16 pathways, respectively. HIV Tat and Nef proteins can provoke bone marrow mesenchymal stem cells senescence through either enhanced inflammation or reduced autophagy (Beaupere et al., 2015), and HIV Tat can also trigger microglia senescence upon miR-505-SIRT3 axis-mediated mitochondrial oxidative stress (Thangaraj et al., 2021) (Table 1).
The occurrence of VIS was assessed in a basic research study (Lee et al., 2021), which found that human diploid fibroblast models exposed to high-titer retrovirus exhibited typical characteristics of senescence and the activated cyclic GMP-AMP synthase-stimulator of interferon genes (cGAS-STING) pathway after the fifth day of infection. Consistently, VIS was detectable in human lung carcinoma cells and non-malignant epithelial cells upon infection with lentivirus, adeno-associated virus (AAV), vesicular stomatitis virus (VSV) and the low-pathogenic human alphacoronavirus NL63 (HCoV-NL63). In parallel, canonical cellular senescence phenotype were found in SARS-CoV-2-infected human primary nasal epithelial cells (HNEpc), alveolar epithelial cells (AEC), normal human bronchus epithelial (NHBE) cells and macrophages, and COVID-19 patients also displayed marked signs of senescence in their nasopharyngeal and lung tissue specimens and elevated serum levels of SASP factors, suggesting that SASP-mediated effects are pivotal factors in secondary paracrine senescence, lung disease, hyperinflammation, tissue damage, and coagulation disorders of patients infected with SARS-CoV-2 (Wiley et al., 2019; Ackermann et al., 2020; Lee et al., 2021; Evangelou et al., 2022; Schmitt et al., 2022). Likewise, a recent study (Lv et al., 2022) also indicated that aged mice deficient in telomerase RNA (Tere-/-) were extremely sensitive to IAV, SARS-CoV-2 and other respiratory virus infections. Tere-/- mice showed typical features of cellular senescence and aberrant activation of the cGAS-STING pathway and NOD-like receptor family pyrin domain containing 3 (NLRP3) inflammasome mediated by leaked mitochondrial DNA (mtDNA), which could contribute to an excessive inflammatory response, particularly following viral exposure, thereby were more likely to develop severe viral pneumonia in non-fatal respiratory virus infections and abnormally increased mortality for Tere-/- mice (Ackermann et al., 2020; Akbar and Gilroy, 2020; Decout et al., 2021; Sanchez-Vazquez et al., 2021; Xian et al., 2021; Lv et al., 2022). Further, latent herpes simplex virus 1 (HSV-1) infection in the key brainstem regions of female mice induces senescence by activating the p53/p16 pathway and NLRP3, resulting in neuroinflammation and neurodegeneration (Sivasubramanian et al., 2022).
HIV infection can also induce a senescent phenotype with the same characteristics as normal senescence (Appay et al., 2007). Owing to antiretroviral therapy (ART), the life expectancy of HIV-infected persons (PLWH) has increased (Wandeler et al., 2016). However, there is still persistent immune activation and inflammation in PLWH even though the virus is effectively suppressed, thus contributing to premature aging (Blanco et al., 2021; Chauvin and Sauce, 2022).
Acquired immune deficiency syndrome (AIDS) patients are often co-infected with the herpes virus (CMV, EBV and HSV), HBV and hepatitis C virus (HCV), of which CMV is the most common chronic infection (Blanco et al., 2021). Chronic CMV infection is highly prevalent in the HIV-negative general elderly population and nearly universal in the HIV-positive elderly population, enabling T-cell clonal expansion and leading to immunosenescence and chronic low-grade inflammation (Khan et al., 2002; Koch et al., 2007; Leng and Margolick, 2020), whereas CMV and HIV co-infection can cause further adverse effects (Leng and Margolick, 2020). Interestingly, AIDS patients have reduced levels of Kupffer cells and CD4+ T cells in the presence of HIV and HCV co-infection, which can lead to a decrease in the clearance of microbial products and an increase in the levels of soluble CD14 (sCD14), lipopolysaccharide (LPS), peptidoglycan, and ribosomal DNA in the blood via microbial translocation (Ancuta et al., 2008; Sandler and Douek, 2012; Blanco et al., 2021). These microbial products can bind to pattern recognition receptors (PRRs) and trigger signaling cascades that favor chronic immune activation and inflammation (Sandler and Douek, 2012; Vázquez-Castellanos et al., 2015; Dillon et al., 2016). At the same time, a reduction in the number of CD4+ T cells during HIV infection may promote the replication of HCV, resulting in CD8+ T cells being continuously activated. This reciprocates the cycle of viral replication and immune activation, showing signs of activation, exhaustion, and immunosenescence (Appay and Kelleher, 2016; Hoffmann et al., 2016; Blanco et al., 2021).
PLWH has similar features as natural immunosenescence. For instance, PLWH is associated with decreased numbers and impaired proliferative capacity of circulating CD34+ hematopoietic progenitor cells (HPCs), thymic degeneration, reduced initial T cells and an accumulation of memory T cells. They also have reduced CD56++NK cells and CD14++CD16-classical monocytes while increased CD14++ CD16+ intermediate and CD14+ CD16++ non-classical monocytes (Hakim et al., 2005; Seidler et al., 2010; Sauce et al., 2011; Hearps et al., 2012; Naranbhai et al., 2013; Massanella et al., 2015; Chauvin and Sauce, 2022). These factors can increase the risk of various age-related diseases in PLWH, such as cardiovascular diseases, renal failure, liver diseases, osteoporosis, cancer and cognitive dysfunctions (Deeks et al., 2013; Gallant et al., 2017).
4 Consequences of senescence
With an increase in age, organisms tend to turn into a pro-inflammatory state characterized by low levels of circulating pro-inflammatory factors and perpetuate chronic inflammation in the elderly population (Franceschi et al., 2000; Xu et al., 2020). However, in the event of viral infections, virus-induced senescence combined with an existing senescence in aged or vulnerable hosts may trigger a more intense inflammatory cascade response, leading to more severe symptoms and multi-organ damage or even dysfunction in the elderly infected population, and thus a higher mortality rate (Figure 2).
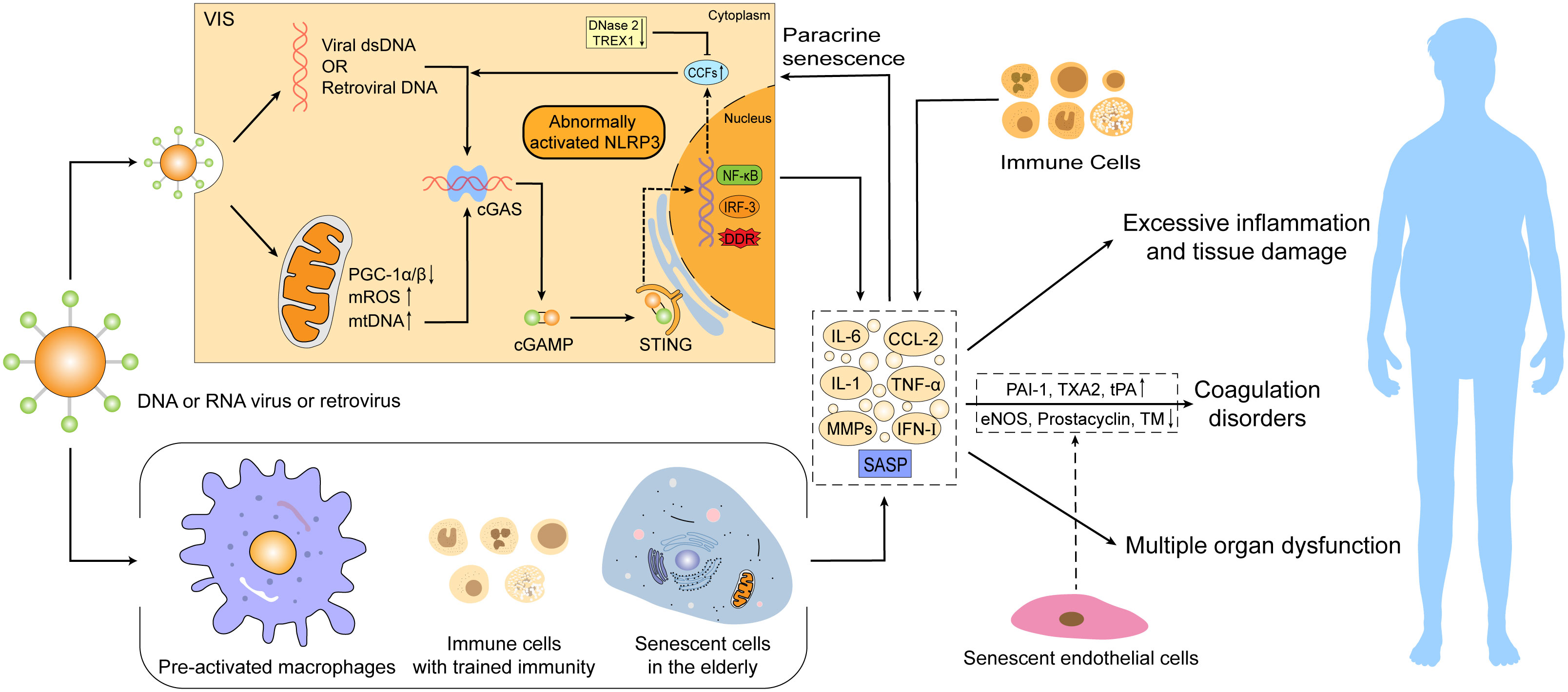
Figure 2 Virus-induced senescence (VIS) combined with an existing senescence in the elderly intensifies the severity of viral infections. Senescence can promote the development of viral infectious diseases via abnormal activation of the mtDNA/CCFs-cGAS-STING pathway and NLRP3 inflammasome, pre-activated macrophages, over-recruited immune cells, and accumulation of innate immune cells with “trained immunity” characteristics. These alterations can trigger excessive SASP production and secondary paracrine senescence, resulting in hyperinflammation, tissue damage, coagulation disorders, and even multiple organ dysfunction, thus leading to a higher mortality risk. SASP, senescence-associated secretory phenotype; cGAMP, cyclic GMP–AMP; CCFs, cytoplasmic chromatin fragments; DDR, DNA damage response; eNOS, endothelial NO synthase; PAI-1, plasminogen activator inhibitor-1; TXA2, thromboxane A2; TM, thrombomodulin; ROS, reactive oxygen species.
4.1 Excessive inflammation and tissue damage associated with aging
As aging occurs, cumulative senescent cells accelerate chronic inflammation through senescence-associated secretory phenotype (SASP), whereby SASP demonstrates a double-edged sword role (Hernandez-Segura et al., 2017). On the one hand, short-term SASP secretion promotes tissue repair and wound healing (Jun and Lau, 2010; Demaria et al., 2014) and enhances immune surveillance to inhibit tumor progression and pathological fibrosis (Xue et al., 2007; Krizhanovsky et al., 2008; Kang et al., 2011; Sagiv et al., 2016), while on the other hand, prolonged SASP secretion contributes to the development of age-related chronic inflammatory diseases by triggering over-recruitment of immune cells (Muñoz-Espín and Serrano, 2014; Childs et al., 2015; He and Sharpless, 2017). Excessive SASP can also recruit immature myeloid cells to favor tumorigenesis and tumor progression in a paracrine manner and affect tissue regeneration by limiting the proliferative potential of stem and progenitor cells (Yoshimoto et al., 2013; Di Mitri et al., 2014; Eggert et al., 2016; Gonzalez-Meljem et al., 2017; Di Micco et al., 2021). The mechanisms of excessive inflammation and tissue damage caused by aging may involve the abnormal activation of the mtDNA/CCFs-cGAS-STING pathway and NLRP3 inflammasome, the role of pre-activated macrophages and over-recruited immune cells and the accumulation of innate immune cells with trained immunity (Figure 2).
4.1.1 Abnormal activation of the mtDNA/CCFs-cGAS-STING pathway and NLRP3 inflammasome
Aging-related mitochondrial dysfunction is identified as a potential mechanism leading to increased inflammation. Mitochondria are extremely important organelles involved in a wide range of cellular activities, such as oxidative phosphorylation, ATP synthesis, apoptosis, autophagy and immune responses (Nunnari and Suomalainen, 2012; Mills et al., 2017; Lv et al., 2022). Thus, complete mitochondrial structure and function are essential for maintaining cellular homeostasis and physiological function (Nunnari and Suomalainen, 2012). However, the adaptability and integrity of mitochondria gradually deteriorate with age, thereby provoking a decline in oxidative phosphorylation efficiency, decrease in MMP, impairment in ATP generation, increase in ROS production and altered autophagic activity, ultimately prompting the leakage of mtDNA from dysfunctional mitochondria (López-Otín et al., 2013; Korolchuk et al., 2017; Hopfner and Hornung, 2020; Lv et al., 2022). Importantly, mtDNA can be recognized by the cGAS-STING system to trigger immune and inflammatory responses (Hopfner and Hornung, 2020; Lv et al., 2022). Specifically, cGAS can directly bind to DNA released into the cytoplasm and subsequently synthesize cyclic GMP–AMP (cGAMP) from GTP and ATP. STING is bound and activated by cGAMP, which activates the NF-κB and IRF3 pathways, thus inducing the production of type I interferon and pro-inflammatory cytokines such as IL-1 and IL-6 (Hopfner and Hornung, 2020; Yang et al., 2021).
In addition to mitochondrial-derived mtDNA, there is evidence that age-related reduction of the LaminB1 protein compromises nuclear envelope integrity and causes the accumulation of cytoplasmic chromatin fragments (CCFs), which can also activate the cGAS-STING pathway and intensify the production of pro-inflammatory factors (Ivanov et al., 2013; Shah et al., 2013; Dou et al., 2017; Glück et al., 2017). Likewise, increased DNA in the cytoplasm caused by telomere dysfunction can be detected by cGAS (Chen et al., 2017; Nassour et al., 2019). The cumulation of nuclear DNA in the cytoplasm is associated with the downregulation of DNases involved in cytoplasmic DNA degradation in senescent cells, such as DNase 2 and TREX1 (Takahashi et al., 2018). Moreover, senescence-related impairment of autophagy, which delays the clearance of activated STING and other cellular debris, can also lead to further accumulation of cytoplasmic DNA and amplify the cGAS-STING pathway and inflammation (Paul et al., 2021).
It was previously reported (Lv et al., 2022) that Tere-/- aged mice were more sensitive to respiratory viral infections such as IAV and SARS-CoV-2, exhibiting excessive inflammatory responses, typical senescence features and increased mortality, which further abnormally activated the cGAS-STING pathway and NLRP3 inflammasome by a process that is mainly mediated by leaked mtDNA. Compared with normal controls, the mitochondria in Tere-/- macrophages showed a swollen shape, irregular rarefied cristae and compromised ATP generation, as well as increased mROS stress. Consistently, there was an elevated amount of cytoplasmic mtDNA in Terc−/− macrophages upon IAV infection, while less mtDNA was retained in mitochondria. Of note, the above phenotypes were more visible following viral infection. However, targeted inhibition of mtDNA release via VBIT-4 significantly weakened the abnormal activation of the cGAS-STING pathway in Tere-/- macrophages relative to controls (Lv et al., 2022), suggesting the importance of aging-related mitochondrial dysfunction in response to viral infection in triggering exaggerated inflammatory responses and causing severe organ damage. Further effects of viral infection on mitochondrial function through the induction of more VIS may play a vital role in the higher levels of mtDNA liberation, leading to stronger inflammatory responses.
An increasing number of research showed that aberrant activation of the aging-associated cGAS-STING pathway and NLRP3 inflammasome underlies the increased lethality of SARS-CoV-2 infection in the elderly, and activation of the NLRP3 inflammasome may be mediated via the cGAS-STING pathway (Lara et al., 2020; Wang et al., 2020b; Lv et al., 2022). Specifically, telomere dysfunction in the elderly stimulates p53-mediated cellular responses and inhibits major regulators of mitochondrial function such as PGC-1α and PGC-1β, conducing to impaired mitochondrial function, enhanced oxidative stress and mtDNA accumulation. Higher levels of mtDNA can generate sustained activation of the cGAS-STING pathway and NLRP3 inflammasome, as well as elevated levels of pro-inflammatory factors. When viral infection occurs, VIS further boosts these pathways and facilitates more production of pro-inflammatory factors, inflicting greater damage to the organism (Kang et al., 2018; Lara et al., 2020; Lv et al., 2022). In addition to SARS-CoV-2 and IAV, when exposed to a series of RNA or DNA viruses or viral products such as human rhinovirus, dengue virus, adenovirus, HCV, MV, RSV, HIV and HSV, organisms demonstrate antiviral effects by activating inflammasomes, such as NLRP3 and AIM2 (Shrivastava et al., 2016), and PRRs, such as Toll-like receptors and RIG-I-like receptors, which are important for recognizing viruses in addition to cGAS (Kawai and Akira, 2008; Wilkins and Gale, 2010). Thereinto, the toll-like receptor-3 (TLR-3) proved to exacerbate SASP secretion of human senescent cells upon SARS-CoV-2 infection (Tripathi et al., 2021). The Toll-like receptor 2 (TLR2) and its partner TLR10 were shown to be key mediators of senescence in vitro and in murine models during oncogene-induced senescence (OIS). TLR2 can promote cell cycle arrest by regulating tumor suppressors p53-p21, p16 and p15 and modulate the SASP production by inducing acute-phase serum amyloids A1 and A2 (Hari et al., 2019). However, little is known about whether antiviral responses induced by other PRRs or inflammasomes are linked to aging or aging-related excessive inflammation and tissue damage.
Notably, RNA viruses such as IAV and SARS-CoV-2 are thought to be recognized by RNA receptors such as Toll-like receptors and RIG-I rather than cGAS, a DNA receptor, upon infections (Liu et al., 2016). In this regard, as previously described, for non-DNA virus infection, the infectious agent may indirectly trigger cGAS-STING activation by directly or indirectly inducing mitochondrial stress to leak mtDNA (Hanada et al., 2020; Hopfner and Hornung, 2020). Such a situation applies to the dengue virus and HSV. Dengue virus is a single positive-stranded RNA virus whose infection generates an endogenous source of cytoplasmic DNA through the release of mtDNA, which drives cGAS to produce cGAMP, with the latter subsequently binding and activating STING, which in return activate the NF-κB and IRF3 pathways and trigger an innate immune antiviral response (Ha et al., 2011; Aguirre et al., 2017; Sun et al., 2017). Although HSV is a DNA virus, its infection can also stimulate the liberation of mtDNA and activate the cGAS-STING pathway (West et al., 2015). Additionally, intracellular accumulation of retrotransposable elements can be reactivated during aging in somatic tissues to drive cGAS-dependent type I interferon responses and contribute to the maintenance of age-related inflammation (De Cecco et al., 2019). For example, HIV, a retrovirus, can trigger cGAS-STING reaction with its reverse-transcribed HIV DNA, and inhibitors of HIV reverse transcriptase can block the induction of interferon response by this virus (Gao et al., 2013). The binding of cGAS to HIV DNA is assisted by the host factor NONO, a multifunctional protein that binds nucleic acids and HIV capsid proteins in the nucleus. NONO is thought to directly recognize HIV DNA by nuclear-localized cGAS (Lahaye et al., 2018). In addition to NONO, host proteins such as PQBP1 (Yoh et al., 2015), ZCCHC3 (Lian et al., 2018) and G3BP1 (Liu et al., 2019) can also contribute to cGAS sensing of reverse-transcribed DNA.
4.1.2 Pre-activated macrophages and over-recruitment of immune cells
It was found that compared to resting macrophages in the lungs of young mice, resident pulmonary macrophages from old mice were in an activated state and more likely to be activated in response to infections. Moreover, these aged lung macrophages harbored higher basal levels of circulating pro-inflammatory cytokines, such as IL-1β, IL-6 and TNF-α (Canan et al., 2014). Saskia L Smits et al. previously reported that SARS-CoV-infected aged macaques developed more severe pathology and higher lethality with a stronger host response than young adult animals, even though viral replication levels were similar and the mRNA levels of IFN-β were negatively correlated with gross pathology. However, treatment with type I interferon significantly diminished the expression of pro-inflammatory genes and attenuated the pathological response in old macaques (Smits et al., 2010). This could be explained by the role of pre-activated macrophages and higher basal levels of pro-inflammatory factors in aged individuals, and also to some extent by the fact that fatal viral infections in the elderly are often associated with exuberant inflammatory cell infiltration and delayed interferon production (Arunachalam et al., 2020).
In addition, previous studies showed that aging could increase mortality from influenza virus infection (Thompson et al., 2003). Senescent alveolar epithelial cells recruit excessive neutrophils (PMNs) in old mice by secreting higher levels of chemokines CXCL1 and CXCL2 upon influenza virus infection (Kulkarni et al., 2019). More importantly, activated PMNs can also generate more pro-inflammatory factors, further recruiting immune cells and leading to more severe inflammatory responses and tissue damage relative to young mice (Peiró et al., 2018; Kulkarni et al., 2019). However, the depletion of PMNs following viral infection can substantially improve the survival of aged mice without altering viral clearance (Kulkarni et al., 2019).
Collectively, the pre-existing inflammatory state and the over-recruitment of immune cells in response to viral infections in older individuals can precipitate increased inflammatory responses to external pathogens, resulting in a massive release of inflammatory mediators and potentially causing widespread tissue damage in common and non-fatal infections for the elderly.
4.1.3 Role of immune cells with trained immunity
Immune memory is traditionally regarded as an exclusive hallmark of adaptive immunity. However, activation of the innate immune system can also lead to an enhanced response to secondary infections, termed “trained immunity”, which is actually a form of innate immune memory (Netea et al., 2020a). Maojun You et al. revealed the establishment of trained immunity in COVID-19 convalescent individuals via the single-cell epigenomic landscape of peripheral immune cells, showing that trained and activated states of CD14+ and CD16+ monocytes were dominantly enriched in individuals recovering from COVID-19 (You et al., 2021). These observations indicate that innate immune cells can form a non-specific but stable immune memory after initial infection, although it may be transient compared to classical T and B cells (Netea et al., 2020a; You et al., 2021). Furthermore, this epigenomic regulation of the innate immune memory response may not be specific to SARS-CoV-2 but also be elicited following other infections such as SARS-CoV-1, MERS, HIV, or vaccination, which is deemed to be a fundamental characteristic of host defense of multicellular organisms, including mammals (Netea et al., 2020a; You et al., 2021; Sviridov et al., 2022).
Evidence accumulated in recent years suggests that trained immunity caused by epigenetic and metabolic reprogramming is a double-edged sword. Although it enables a rapid and efficient host immune response to reinfected pathogens, it can also induce chronic inflammatory diseases (Netea et al., 2020a; Netea et al., 2020b; You et al., 2021). In the elderly, the accumulation of immune cells with trained immunity in the body may promote excessive inflammation and cause more severe tissue damage in the event of reinfection. Thus, appropriate targeting of immune cells with trained immunity in elderly individuals might be beneficial to relieve inflammation (You et al., 2021; Lv et al., 2022).
4.2 Aging-related multi-organ dysfunction
The effects of viral infections on the organism often involve multiple systems and organs. For example, SARS-CoV-2, a respiratory virus, in addition to causing lung infection, the virus can also replicate in cells of the intestine, liver and kidney, thus causing a variety of clinical symptoms other than the respiratory tract, such as gastrointestinal disorders, liver and kidney dysfunction (Chu et al., 2020) and even multi-organ failure (Chen et al., 2020). However, there is growing evidence supporting the role of aging in multi-organ dysfunction caused by viral infections (Figure 2).
Coagulation abnormalities and thrombosis can occur in the late stages in patients with viral infections and are often associated with poor prognosis (Nehme et al., 2020). Several factors, however, including aging, have been shown to be risk factors for vascular dysfunction (Nehme et al., 2020). On the one hand, senescent cells can secrete large amounts of SASP pro-inflammatory mediators that may trigger endothelial injury and favor thrombosis. On the other hand, together with inflammatory factors, senescent endothelial cells can shift the balance between pro- and anticoagulant pathways towards an elevated risk for thrombosis via the upregulation of factors that induce platelet aggregation such as plasminogen activator inhibitor-1 (PAI-1), thromboxane A2 (TXA2) and von Willebrand factor (vWF), while downregulating factors that inhibit platelet aggregation such as endothelial NO synthase (eNOS), prostacyclin and thrombomodulin (Bochenek et al., 2016; Wiley et al., 2019; Nehme et al., 2020). Therefore, the elderly may develop more severe coagulation disorders if infected by SARS-CoV, MERS-CoV, H1N1, HIV or other viruses (Obi et al., 2019; Ackermann et al., 2020; de Magalhães et al., 2020; Giannis et al., 2020). It was found that compared with young controls, aged hamsters exhibited prolongation of PT, intravascular clotting and acute kidney damage upon SARS-CoV-2 infection (Ohno et al., 2021). These changes are often present in patients with COVID-19 and strongly associated with disease severity and higher mortality (Helms et al., 2020; Porfidia et al., 2020; Loo et al., 2021). In addition, H1N1 acute respiratory distress syndrome (ARDS) patients possessed a 23.3-fold higher risk for pulmonary embolism and a 17.9-fold increased risk for venous thromboembolism (Obi et al., 2019). Simultaneously, in HIV-infected patients, endothelial dysfunction caused by HIV replication may also lead to a hypercoagulable state (Kuller et al., 2008; Armah et al., 2012), while aging-related inflammation and cellular changes may further contribute to coagulation dysfunction (de Magalhães et al., 2020).
Additionally, due to abnormal immune responses and excessive inflammation associated with aging, the elderly are more prone to complications such as liver and kidney dysfunction, myocardial injury, and neurological symptoms in the event of viral infections such as SARS-CoV-2. In particular, the massive secretion of SASP may generate fibrosis or cause injuries in organs other than the lungs, such as the liver, kidney and cardiovascular system (Cai et al., 2020; George et al., 2020; Napoli et al., 2020; Sharma et al., 2020; D'Agnillo et al., 2021). A decline in the blood-brain barrier function with aging may also cause infection of the central nervous system, leading to neurological symptoms (Yamazaki et al., 2016; Mao et al., 2020; Propson et al., 2021).
4.3 Dual role of aging antiviral response
Presently, it is believed that aging may cause dual effects during antiviral infection. Primarily, SASP cytokines and chemokines from senescent cells and accordingly recruited innate immune cells such as PMNs may predispose virus-induced senescence to become a part of the antiviral immune response (Baz-Martínez et al., 2016). This antiviral mechanism may enable the secretion of SASP factors by virus-induced senescent cells to restrict virus replication in neighboring cells and avoid its spread (Kelley et al., 2020). Moreover, the human papillomavirus (HPV), HBV, EBV and KSHV have evolved various mechanisms that can specifically combat cellular senescence (Yang et al., 2000; Oishi et al., 2007; Leidal et al., 2012; Zhi et al., 2015; Estêvão et al., 2019), indirectly suggesting that senescence may lead to antiviral defense in certain circumstances (Kelley et al., 2020).
Conversely, it was documented that senescence is conducive to the pathophysiology of viral infections and may promote viral replication and mutagenesis (Kelley et al., 2020; Evangelou et al., 2022). For instance, RSV infection can alter human airway epithelial differentiation and trigger the senescence of lung epithelial cells both in vivo and in vitro by generating ROS and causing DNA damage, thereby contributing to airway tissue remodeling and the severity and long-term consequences of RSV infections (Persson et al., 2014; Martínez et al., 2016). The influenza and varicella-zoster viruses can replicate more efficiently in senescent human bronchial epithelial cells and senescent human dermal fibroblasts, respectively, compared with non-senescent cells (Kim et al., 2016). The possible reasons for this phenomenon are the downregulation of type I interferon induction upon senescence and the defective mitochondrial dynamics of senescent cells, which consequently inhibit interferon expression and early interferon responses, thus favoring viral replication (Kim et al., 2016; Kelley et al., 2020). However, Baz-Martínez M et al. found that primary or chemotherapy-induced senescence reduces VSV replication (Baz-Martínez et al., 2016), suggesting that senescence plays a different role in response to diverse viral infections under distinguishing conditions. Remarkably, recent studies revealed that infected senescent cells might be a source of apolipoprotein B mRNA-editing (APOBEC) enzyme-mediated SARS-CoV-2 mutations (Evangelou et al., 2022).
Taken together, the effects of senescence on the body’s antiviral immune response are multifaceted (Figure 3). In this regard, it has been hypothesized from the perspective of acute respiratory viral infections that aging may play different roles in viral infections depending on host resilience (Kelley et al., 2020). In young hosts, VIS may enhance antiviral immunity by recruiting PMNs and other immune cells via SASP, thereby promoting viral clearance and tissue repair. However, in old or vulnerable hosts, VIS coupled with an existing senescence condition may lead to excessive immune responses with high levels of SASP cytokines and chemokines, resulting in secondary senescence and over-recruitment of immune cells, eliciting severe tissue damage and multi-organ failure (Kelley et al., 2020; Camell et al., 2021).
5 Significance of senotherapeutics in viral infectious diseases
In the presence of viral infections, VIS plus naturally occurring senescence are pivotal in precipitating excessive inflammatory responses, severe organ damage, and higher mortality in the elderly. Hence, senotherapeutics seem to be of great importance in alleviating clinical symptoms and organ damage and influencing disease regression in elderly individuals with viral infections.
Actually, for viral infectious diseases in aged people, there are currently two main areas of research in senotherapeutics (Table 2). The first one is the targeted removal of senescent cells, termed “senolytics”, mainly by propping up apoptosis of senescent cells, such as quercetin and fisetin (natural flavonoids), navitoclax (an inhibitor of BCL-2 pro-survival family) and dasatinib (a tyrosine kinase inhibitor). The second one is the inhibition of diverse components of SASP or inflammatory pathways involved in SASP synthesis, known as “senomorphics”, such as targeted inhibition of the cGAS-STING and NF-κB pathways or IL-1 and IL-6 cytokines. Intriguingly, available evidence implies a positive effect on mitigating aging-related diseases by eliminating senescent cells or inhibiting SASP secretion, and various clinical studies on senotherapeutics applied to viral infectious diseases are ongoing or have been completed (Table 3).
5.1 Senolytics
The most widely studied therapeutic strategy for the targeted elimination of senescent cells by senolytics is the combination therapy of dasatinib (D) with quercetin (Q). “D+Q” treatment was shown to reduce the cellular stress of aged mice, lessen vascular sclerosis, strengthen vasodilatory functions and impel lung function in mice with pulmonary fibrosis, thus improving the health status and lifespan of elderly mice (Roos et al., 2016; Lehmann et al., 2017; Xu et al., 2018). Likewise, preliminary clinical trials have demonstrated that “D+Q” therapy significantly improved the physical function of elderly patients with pulmonary fibrosis, reduced the burden of senescent cells in diabetic patients with chronic kidney disease, decreased the levels of major circulating SASP factors, and slowed disease progression (Hickson et al., 2019; Justice et al., 2019).
In terms of viral infectious diseases, Camell et al. discovered that human endothelial senescent cells initiated excessive inflammation upon exposure to SARS-CoV-2, which was accompanied by enhanced SASP expression and a pronounced increase in cellular senescence, inflammation and mortality among aged mice with similar β-coronavirus infection (Camell et al., 2021). However, the use of fisetin or the “D+Q” therapy with senescence-targeted ability selectively combated senescent cells, substantially reduced the signs of senescence and the levels of inflammatory markers and decreased viral infections-related mortality (Camell et al., 2021). In a recent study (Lee et al., 2021), SARS-CoV-2 virus infection and the subsequent VIS were determined to be driving factors in modulating COVID-19-related cytokine storm and tissue damage. However, the targeted removal of senescent cells with senescence-targeted drugs such as navitoclax, D+Q and fisetin lead to a significant reduction in senescent cells and a marked attenuation of senescence-related traits, both in vitro and in the respiratory epithelium of hamster models. Further, COVID-19-related lung diseases, inflammation, tissue damage and coagulation disorders significantly subsided (Lee et al., 2021). Additionally, in senescent macrophages exposed to IAV or SARS-CoV-2 (Lv et al., 2022), fisetin prominently suppressed the aberrant activation of the cGAS-STING pathway, NLRP3 inflammasome and the resultant excessive inflammatory responses in senescent macrophages via the induction of apoptosis and reduction of dysfunctional mitochondrial load. In the Tere-/- aged mice model, fisetin was also found to dampen pathogenic inflammation mediated by the cGAS-STING pathway and NLRP3 inflammasome by lowering senescent cells-related burden and improving mitochondrial integrity, thus providing a considerable improvement in the survival rate of Tere-/- mice infected with IAV. Comparable results were obtained upon validation using the “D+Q” combination treatment (Lv et al., 2022).
In prospective randomized controlled clinical trials among patients with COVID-19, quercetin appeared to be effective in decreasing viral load and relieving clinical symptoms during the early application of viral infection in combination with standardized care, which offered protection against serious complications and conferred a high safety profile (Di Pierro et al., 2021a; Di Pierro et al., 2021b). Correspondingly, a systematic review of quercetin revealed that quercetin and its derivatives were associated with significantly reduced mean viral load and generation of pro-inflammatory cytokines, chemokines, reactive oxygen species, mucus and airway resistance in animals infected with respiratory viruses such as influenza virus and human rhinovirus. These observations were associated with a significant reduction in infected animal fatality and were considered a potential strategy for treating lower respiratory tract viral diseases (Brito et al., 2021).
5.2 Senomorphics
The mammalian target of rapamycin (mTOR) pathway has been demonstrated to facilitate SASP production in recent years by regulating the translation of mRNA subsets, including those encoding IL-1α (Herranz et al., 2015; Laberge et al., 2015). Rapamycin can target the mTOR pathway to inhibit the activity of the mTORC1 complex, which is known to regulate mRNA translation, leading to reduced mRNA levels of cytokines such as IL-6 and IL-10 and selective inhibition of the translation of IL-1α. This creates a drop in SASP production and reduces the risk of age-related cognitive decline and cardiac or hepatic dysfunction, eventually extending the lifespan of mice and improving immune functions in the elderly (Majumder et al., 2012; Wilkinson et al., 2012; Flynn et al., 2013; Mannick et al., 2014; Laberge et al., 2015). More importantly, the application of rapamycin can potentiate antiviral activity in the event of SARS-CoV-2, MERS-CoV, H1N1 and other viral infections, which can be conducive to attenuating the severity of diseases (Wang et al., 2014; Kindrachuk et al., 2015; Husain and Byrareddy, 2020). Currently, ongoing clinical trials are assessing the safety and efficacy of rapamycin in the treatment or prevention of COVID-19 (Geier and Perl, 2021).
In addition, metformin, a biguanide that combats age-related diseases to extend health span, is the first drug for age-targeted effects in a large clinical trial (Kulkarni et al., 2020). Available evidence supports that metformin can dampen aging-related features directly or indirectly through multiple pathways, such as improving nutrient perception, inhibiting the NF-κB pathway and pro-inflammatory factors production, enhancing cellular autophagy and intercellular communication, regulating mitochondrial function, modulating gut microbiota, delaying stem cell aging, curtailing telomere attrition, and attenuating cellular senescence (Kulkarni et al., 2020). It was reported that metformin could extend the lifespan of mice by inhibiting the production of pro-inflammatory factors and diminishing DNA damage (Martin-Montalvo et al., 2013; Moiseeva et al., 2013; Valencia et al., 2017). It could also decrease age-related chronic inflammation and the risk of cardiovascular diseases, cancer, neurodegenerative diseases and cognitive dysfunction, ultimately exerting a constructive effect on improving the overall health status and prolonging the lifespan of aged persons (Barzilai et al., 2016; Campbell et al., 2017; Markowicz-Piasecka et al., 2017; Campbell et al., 2018; Tizazu et al., 2019).
Data from China revealed that the metformin treatment was associated with lower mortality in hospital patients with COVID-19 (Li et al., 2020; Luo et al., 2020) and fewer COVID-19-related heart failure and inflammation compared with other anti-diabetic agents (Cheng et al., 2020). Similarly, metformin could also cause an almost 11-fold reduction in the odds ratio of death among COVID-19 African-American patients with T2DM (Crouse et al., 2020). A recent study confirmed that metformin inhibited mtDNA synthesis and cytoplasmic Ox-mtDNA production in macrophages, thereby suppressing NLRP3 inflammasome activation, IL-1β generation and IL-6 secretion and relieving lung inflammation in human ACE2 transgenic mice infected with SARS-CoV-2 (Xian et al., 2021). Such protective effects were independent of glycemic control and correlated with the anti-inflammatory properties of metformin (Valencia et al., 2017; Marcucci et al., 2020). Nevertheless, it should also be noted that metformin use is linked to a high incidence of acidosis, especially in severe COVID-19 cases (Cheng et al., 2020), and careful consideration should be made on tackling the complications during clinical administration.
Anakinra, an IL-1 receptor antagonist, was shown to cause a rapid decrease in inflammatory and febrile symptoms, lower oxygen requirements, increase the duration of non-invasive mechanical ventilation and improve various clinical conditions when administered early in COVID-19 patients (Cauchois et al., 2020). In parallel, another cohort study also concluded that therapy with Anakinra was associated with fewer needs for invasive mechanical ventilation, lowered the mortality of patients with severe COVID-19, and, importantly, did not cause serious side effects (Huet et al., 2020). Moreover, a multicenter cohort study enrolling 3924 COVID-19 patients suggested that treatment with a monoclonal antibody of the IL-6 receptor (Tocilizumab) during the first 2 days of patient admission to the ICU could significantly reduce the risk of in-hospital mortality (Gupta et al., 2021). A randomized controlled clinical trial from the United Kingdom also demonstrated that Tocilizumab lowered the probability of invasive mechanical ventilation needs and 28-day mortality in patients with COVID-19 (Group, 2021). Further, a Bruton tyrosine kinase (BTK) inhibitor was also found to reduce BTK-dependent activation of NF-κB and NLRP3 inflammasome, which suppressed pro-inflammatory factors production and COVID-19 cytokine storm, thereby improving the prognosis of COVID-19 patients (Roschewski et al., 2020).
Altogether, these findings not only further illustrate the critical role of SASP components in the development of viral infectious diseases such as COVID-19 but also demonstrate the high interest in targeting SASP components or the inflammatory pathways involved in their synthesis for the treatment of aging-related viral infectious diseases.
6 Conclusion and outlook
Senescence and viral infections interact in a reciprocal relationship. In general, viral infections can induce senescence and increase the susceptibility and severity of viral infections via multiple mechanisms, such as immunodeficiency, mitochondrial dysfunction, SASP secretion, pre-activated macrophages, over-recruitment of immune cells, and accumulation of innate immune cells with trained immunity. In the elderly, virus-induced senescence, in addition to their pre-existing senescent condition, is believed to aggravate the underlying disease outcomes, but could be counteracted by senotherapeutics, which was shown to mitigate the severity of viral infections.
Undeniably, well-controlled senescence onset may positively enhance antiviral immunity, yet excessive inflammatory responses by accumulated senescent cells are critical factors underlying the development of multiple aging-related diseases. However, the relationship between viral infections and senescence should be further clarified, because it remains undetermined whether the effects are fully compatible between virus-induced senescence and naturally occurred senescence on the antiviral responses of hosts, the exact mechanisms of virus-induced senescence are not fully clear, the optimal doses of anti-senescence therapeutic drugs remain investigational, and the specific adverse events are not yet fully known. Thus, further research and clinical trials are needed to prolong a healthy lifespan of the elderly.
Author contributions
ZL, MT, and CZ conceptualized and designed this study. ZL, MT, and GW wrote the original draft and prepared the diagrams. XC, J’eM, and SL researched data and collected the references. XC and BS reviewed and edited the manuscript. CZ, XX, KW, and FL critically revised the manuscript. All authors contributed to the article and approved the submitted version.
Funding
This work was supported by the Fundamental Research Funds for the Central Universities (No.2042022kf1215), the Special Funds for Innovation in Scientific Research Program of Zhongshan under Grant 2020AG024, Chinese Foundation for Hepatitis Prevention and Control-Tian Qing Liver Disease Research Fund Subject (TGQB20210109), the Open Funds of Key Laboratory of Diagnosis and Treatment of Digestive System Tumors of Zhejiang Province (KFJJ-202005, KFJJ-201907), the Open Research Program of the State Key Laboratory of Virology of China (2021KF002, 2021KF006) and the Key Research and Development Project of Hubei Province (2022BCA009).
Conflict of interest
The authors declare that the research was conducted in the absence of any commercial or financial relationships that could be construed as a potential conflict of interest.
Publisher’s note
All claims expressed in this article are solely those of the authors and do not necessarily represent those of their affiliated organizations, or those of the publisher, the editors and the reviewers. Any product that may be evaluated in this article, or claim that may be made by its manufacturer, is not guaranteed or endorsed by the publisher.
References
Ackermann, M., Verleden, S. E., Kuehnel, M., Haverich, A., Welte, T., Laenger, F., et al. (2020). Pulmonary vascular endothelialitis, thrombosis, and angiogenesis in covid-19. N Engl. J. Med. 383 (2), 120–128. doi: 10.1056/NEJMoa2015432
Acosta, J. C., Banito, A., Wuestefeld, T., Georgilis, A., Janich, P., Morton, J. P., et al. (2013). A complex secretory program orchestrated by the inflammasome controls paracrine senescence. Nat. Cell Biol. 15 (8), 978–990. doi: 10.1038/ncb2784
Aguirre, S., Luthra, P., Sanchez-Aparicio, M. T., Maestre, A. M., Patel, J., Lamothe, F., et al. (2017). Dengue virus NS2B protein targets cGAS for degradation and prevents mitochondrial DNA sensing during infection. Nat. Microbiol. 2, 17037. doi: 10.1038/nmicrobiol.2017.37
Akbar, A. N., Gilroy, D. W. (2020). Aging immunity may exacerbate COVID-19. Science 369 (6501), 256–257. doi: 10.1126/science.abb0762
Althubiti, M., Lezina, L., Carrera, S., Jukes-Jones, R., Giblett, S. M., Antonov, A., et al. (2014). Characterization of novel markers of senescence and their prognostic potential in cancer. Cell Death Dis. 5 (11), e1528. doi: 10.1038/cddis.2014.489
Ancuta, P., Kamat, A., Kunstman, K. J., Kim, E. Y., Autissier, P., Wurcel, A., et al. (2008). Microbial translocation is associated with increased monocyte activation and dementia in AIDS patients. PloS One 3 (6), e2516. doi: 10.1371/journal.pone.0002516
Appay, V., Almeida, J. R., Sauce, D., Autran, B., Papagno, L. (2007). Accelerated immune senescence and HIV-1 infection. Exp. Gerontol 42 (5), 432–437. doi: 10.1016/j.exger.2006.12.003
Appay, V., Kelleher, A. D. (2016). Immune activation and immune aging in HIV infection. Curr. Opin. HIV AIDS 11 (2), 242–249. doi: 10.1097/coh.0000000000000240
Armah, K. A., McGinnis, K., Baker, J., Gibert, C., Butt, A. A., Bryant, K. J., et al. (2012). HIV Status, burden of comorbid disease, and biomarkers of inflammation, altered coagulation, and monocyte activation. Clin. Infect. Dis. 55 (1), 126–136. doi: 10.1093/cid/cis406
Arunachalam, P. S., Wimmers, F., Mok, C. K. P., Perera, R., Scott, M., Hagan, T., et al. (2020). Systems biological assessment of immunity to mild versus severe COVID-19 infection in humans. Science 369 (6508), 1210–1220. doi: 10.1126/science.abc6261
Baker, D. J., Wijshake, T., Tchkonia, T., LeBrasseur, N. K., Childs, B. G., van de Sluis, B., et al. (2011). Clearance of p16Ink4a-positive senescent cells delays ageing-associated disorders. Nature 479 (7372), 232–236. doi: 10.1038/nature10600
Barzilai, N., Crandall, J. P., Kritchevsky, S. B., Espeland, M. A. (2016). Metformin as a tool to target aging. Cell Metab. 23 (6), 1060–1065. doi: 10.1016/j.cmet.2016.05.011
Baz-Martínez, M., Da Silva-Álvarez, S., Rodríguez, E., Guerra, J., El Motiam, A., Vidal, A., et al. (2016). Cell senescence is an antiviral defense mechanism. Sci. Rep. 6, 37007. doi: 10.1038/srep37007
Beaupere, C., Garcia, M., Larghero, J., Fève, B., Capeau, J., Lagathu, C. (2015). The HIV proteins tat and nef promote human bone marrow mesenchymal stem cell senescence and alter osteoblastic differentiation. Aging Cell 14 (4), 534–546. doi: 10.1111/acel.12308
Bent, E. H., Gilbert, L. A., Hemann, M. T. (2016). A senescence secretory switch mediated by PI3K/AKT/mTOR activation controls chemoprotective endothelial secretory responses. Genes Dev. 30 (16), 1811–1821. doi: 10.1101/gad.284851.116
Blackford, A. N., Jackson, S. P. (2017). ATM, ATR, and DNA-PK: The trinity at the heart of the DNA damage response. Mol. Cell 66 (6), 801–817. doi: 10.1016/j.molcel.2017.05.015
Blanco, J. R., Negredo, E., Bernal, E., Blanco, J. (2021). Impact of HIV infection on aging and immune status. Expert Rev. Anti Infect. Ther. 19 (6), 719–731. doi: 10.1080/14787210.2021.1848546
Bochenek, M. L., Schütz, E., Schäfer, K. (2016). Endothelial cell senescence and thrombosis: Ageing clots. Thromb. Res. 147, 36–45. doi: 10.1016/j.thromres.2016.09.019
Brito, J. C. M., Lima, W. G., Cordeiro, L. P. B., da Cruz Nizer, W. S. (2021). Effectiveness of supplementation with quercetin-type flavonols for treatment of viral lower respiratory tract infections: Systematic review and meta-analysis of preclinical studies. Phytother. Res. 35 (9), 4930–4942. doi: 10.1002/ptr.7122
Cai, Q., Huang, D., Yu, H., Zhu, Z., Xia, Z., Su, Y., et al. (2020). COVID-19: Abnormal liver function tests. J. Hepatol. 73 (3), 566–574. doi: 10.1016/j.jhep.2020.04.006
Camell, C. D., Yousefzadeh, M. J., Zhu, Y., Prata, L., Huggins, M. A., Pierson, M., et al. (2021). Senolytics reduce coronavirus-related mortality in old mice. Science 373 (6552). doi: 10.1126/science.abe4832
Campbell, J. M., Bellman, S. M., Stephenson, M. D., Lisy, K. (2017). Metformin reduces all-cause mortality and diseases of ageing independent of its effect on diabetes control: A systematic review and meta-analysis. Ageing Res. Rev. 40, 31–44. doi: 10.1016/j.arr.2017.08.003
Campbell, J. M., Stephenson, M. D., de Courten, B., Chapman, I., Bellman, S. M., Aromataris, E. (2018). Metformin use associated with reduced risk of dementia in patients with diabetes: A systematic review and meta-analysis. J. Alzheimers Dis. 65 (4), 1225–1236. doi: 10.3233/jad-180263
Canan, C. H., Gokhale, N. S., Carruthers, B., Lafuse, W. P., Schlesinger, L. S., Torrelles, J. B., et al. (2014). Characterization of lung inflammation and its impact on macrophage function in aging. J. Leukoc. Biol. 96 (3), 473–480. doi: 10.1189/jlb.4A0214-093RR
Cauchois, R., Koubi, M., Delarbre, D., Manet, C., Carvelli, J., Blasco, V. B., et al. (2020). Early IL-1 receptor blockade in severe inflammatory respiratory failure complicating COVID-19. Proc. Natl. Acad. Sci. U.S.A. 117 (32), 18951–18953. doi: 10.1073/pnas.2009017117
Chang, J., Wang, Y., Shao, L., Laberge, R. M., Demaria, M., Campisi, J., et al. (2016). Clearance of senescent cells by ABT263 rejuvenates aged hematopoietic stem cells in mice. Nat. Med. 22 (1), 78–83. doi: 10.1038/nm.4010
Chauvin, M., Sauce, D. (2022). Mechanisms of immune aging in HIV. Clin. Sci. (Lond) 136 (1), 61–80. doi: 10.1042/cs20210344
Chen, E. H., Olson, E. N. (2005). Unveiling the mechanisms of cell-cell fusion. Science 308 (5720), 369–373. doi: 10.1126/science.1104799
Chen, Y. A., Shen, Y. L., Hsia, H. Y., Tiang, Y. P., Sung, T. L., Chen, L. Y. (2017). Extrachromosomal telomere repeat DNA is linked to ALT development via cGAS-STING DNA sensing pathway. Nat. Struct. Mol. Biol. 24 (12), 1124–1131. doi: 10.1038/nsmb.3498
Chen, N., Zhou, M., Dong, X., Qu, J., Gong, F., Han, Y., et al. (2020). Epidemiological and clinical characteristics of 99 cases of 2019 novel coronavirus pneumonia in wuhan, China: a descriptive study. Lancet 395 (10223), 507–513. doi: 10.1016/s0140-6736(20)30211-7
Cheng, X., Liu, Y. M., Li, H., Zhang, X., Lei, F., Qin, J. J., et al. (2020). Metformin is associated with higher incidence of acidosis, but not mortality, in individuals with COVID-19 and pre-existing type 2 diabetes. Cell Metab. 32 (4), 537–547.e533. doi: 10.1016/j.cmet.2020.08.013
Childs, B. G., Baker, D. J., Kirkland, J. L., Campisi, J., van Deursen, J. M. (2014). Senescence and apoptosis: Dueling or complementary cell fates? EMBO Rep. 15 (11), 1139–1153. doi: 10.15252/embr.201439245
Childs, B. G., Durik, M., Baker, D. J., van Deursen, J. M. (2015). Cellular senescence in aging and age-related disease: From mechanisms to therapy. Nat. Med. 21 (12), 1424–1435. doi: 10.1038/nm.4000
Cho, S., Hwang, E. S. (2012). Status of mTOR activity may phenotypically differentiate senescence and quiescence. Mol. Cells 33 (6), 597–604. doi: 10.1007/s10059-012-0042-1
Chrétien, A., Piront, N., Delaive, E., Demazy, C., Ninane, N., Toussaint, O. (2008). Increased abundance of cytoplasmic and nuclear caveolin 1 in human diploid fibroblasts in H(2)O(2)-induced premature senescence and interplay with p38alpha(MAPK). FEBS Lett. 582 (12), 1685–1692. doi: 10.1016/j.febslet.2008.04.026
Chu, H., Chan, J. F., Yuen, T. T., Shuai, H., Yuan, S., Wang, Y., et al. (2020). Comparative tropism, replication kinetics, and cell damage profiling of SARS-CoV-2 and SARS-CoV with implications for clinical manifestations, transmissibility, and laboratory studies of COVID-19: An observational study. Lancet Microbe 1 (1), e14–e23. doi: 10.1016/s2666-5247(20)30004-5
Chuprin, A., Gal, H., Biron-Shental, T., Biran, A., Amiel, A., Rozenblatt, S., et al. (2013). Cell fusion induced by ERVWE1 or measles virus causes cellular senescence. Genes Dev. 27 (21), 2356–2366. doi: 10.1101/gad.227512.113
Cormenier, J., Martin, N., Deslé, J., Salazar-Cardozo, C., Pourtier, A., Abbadie, C., et al. (2018). The ATF6α arm of the unfolded protein response mediates replicative senescence in human fibroblasts through a COX2/prostaglandin E(2) intracrine pathway. Mech. Ageing Dev. 170, 82–91. doi: 10.1016/j.mad.2017.08.003
Crouse, A. B., Grimes, T., Li, P., Might, M., Ovalle, F., Shalev, A. (2020). Metformin use is associated with reduced mortality in a diverse population with COVID-19 and diabetes. Front. Endocrinol. (Lausanne) 11. doi: 10.3389/fendo.2020.600439
D'Agnillo, F., Walters, K. A., Xiao, Y., Sheng, Z. M., Scherler, K., Park, J., et al. (2021). Lung epithelial and endothelial damage, loss of tissue repair, inhibition of fibrinolysis, and cellular senescence in fatal COVID-19. Sci. Transl. Med. 13 (620), eabj7790. doi: 10.1126/scitranslmed.abj7790
Dasari, A., Bartholomew, J. N., Volonte, D., Galbiati, F. (2006). Oxidative stress induces premature senescence by stimulating caveolin-1 gene transcription through p38 mitogen-activated protein kinase/Sp1-mediated activation of two GC-rich promoter elements. Cancer Res. 66 (22), 10805–10814. doi: 10.1158/0008-5472.Can-06-1236
De Cecco, M., Ito, T., Petrashen, A. P., Elias, A. E., Skvir, N. J., Criscione, S. W., et al. (2019). L1 drives IFN in senescent cells and promotes age-associated inflammation. Nature 566 (7742), 73–78. doi: 10.1038/s41586-018-0784-9
Decout, A., Katz, J. D., Venkatraman, S., Ablasser, A. (2021). The cGAS-STING pathway as a therapeutic target in inflammatory diseases. Nat. Rev. Immunol. 21 (9), 548–569. doi: 10.1038/s41577-021-00524-z
Deeks, S. G., Lewin, S. R., Havlir, D. V. (2013). The end of AIDS: HIV infection as a chronic disease. Lancet 382 (9903), 1525–1533. doi: 10.1016/s0140-6736(13)61809-7
Delpeut, S., Noyce, R. S., Siu, R. W., Richardson, C. D. (2012). Host factors and measles virus replication. Curr. Opin. Virol. 2 (6), 773–783. doi: 10.1016/j.coviro.2012.10.008
de Magalhães, M. C., Sánchez-Arcila, J. C., Lyra, A. C. B., Long, L. F. B., Vasconcellos de Souza, I., Ferry, F. R. A., et al. (2020). Hemostasis in elderly patients with human immunodeficiency virus (HIV) infection-cross-sectional study. PloS One 15 (2), e0227763. doi: 10.1371/journal.pone.0227763
Demaria, M., Ohtani, N., Youssef, S. A., Rodier, F., Toussaint, W., Mitchell, J. R., et al. (2014). An essential role for senescent cells in optimal wound healing through secretion of PDGF-AA. Dev. Cell 31 (6), 722–733. doi: 10.1016/j.devcel.2014.11.012
Dillon, S. M., Frank, D. N., Wilson, C. C. (2016). The gut microbiome and HIV-1 pathogenesis: A two-way street. AIDS 30 (18), 2737–2751. doi: 10.1097/qad.0000000000001289
Di Micco, R., Krizhanovsky, V., Baker, D., d'Adda di Fagagna, F. (2021). Cellular senescence in ageing: From mechanisms to therapeutic opportunities. Nat. Rev. Mol. Cell Biol. 22 (2), 75–95. doi: 10.1038/s41580-020-00314-w
Di Micco, R., Sulli, G., Dobreva, M., Liontos, M., Botrugno, O. A., Gargiulo, G., et al. (2011). Interplay between oncogene-induced DNA damage response and heterochromatin in senescence and cancer. Nat. Cell Biol. 13 (3), 292–302. doi: 10.1038/ncb2170
Di Mitri, D., Toso, A., Chen, J. J., Sarti, M., Pinton, S., Jost, T. R., et al. (2014). Tumour-infiltrating gr-1+ myeloid cells antagonize senescence in cancer. Nature 515 (7525), 134–137. doi: 10.1038/nature13638
Dimri, G. P., Lee, X., Basile, G., Acosta, M., Scott, G., Roskelley, C., et al. (1995). A biomarker that identifies senescent human cells in culture and in aging skin in vivo. Proc. Natl. Acad. Sci. U.S.A. 92 (20), 9363–9367. doi: 10.1073/pnas.92.20.9363
Di Pierro, F., Derosa, G., Maffioli, P., Bertuccioli, A., Togni, S., Riva, A., et al. (2021a). Possible therapeutic effects of adjuvant quercetin supplementation against early-stage COVID-19 infection: A prospective, randomized, controlled, and open-label study. Int. J. Gen. Med. 14, 2359–2366. doi: 10.2147/ijgm.S318720
Di Pierro, F., Iqtadar, S., Khan, A., Ullah Mumtaz, S., Masud Chaudhry, M., Bertuccioli, A., et al. (2021b). Potential clinical benefits of quercetin in the early stage of COVID-19: Results of a second, pilot, randomized, controlled and open-label clinical trial. Int. J. Gen. Med. 14, 2807–2816. doi: 10.2147/ijgm.S318949
Dou, Z., Ghosh, K., Vizioli, M. G., Zhu, J., Sen, P., Wangensteen, K. J., et al. (2017). Cytoplasmic chromatin triggers inflammation in senescence and cancer. Nature 550 (7676), 402–406. doi: 10.1038/nature24050
Druelle, C., Drullion, C., Deslé, J., Martin, N., Saas, L., Cormenier, J., et al. (2016). ATF6α regulates morphological changes associated with senescence in human fibroblasts. Oncotarget 7 (42), 67699–67715. doi: 10.18632/oncotarget.11505
Duelli, D., Lazebnik, Y. (2007). Cell-to-cell fusion as a link between viruses and cancer. Nat. Rev. Cancer 7 (12), 968–976. doi: 10.1038/nrc2272
Eggert, T., Wolter, K., Ji, J., Ma, C., Yevsa, T., Klotz, S., et al. (2016). Distinct functions of senescence-associated immune responses in liver tumor surveillance and tumor progression. Cancer Cell 30 (4), 533–547. doi: 10.1016/j.ccell.2016.09.003
Estêvão, D., Costa, N. R., Gil da Costa, R. M., Medeiros, R. (2019). Hallmarks of HPV carcinogenesis: The role of E6, E7 and E5 oncoproteins in cellular malignancy. Biochim. Biophys. Acta Gene Regul. Mech. 1862 (2), 153–162. doi: 10.1016/j.bbagrm.2019.01.001
Evangelou, K., Veroutis, D., Paschalaki, K., Foukas, P. G., Lagopati, N., Dimitriou, M., et al. (2022). Pulmonary infection by SARS-CoV-2 induces senescence accompanied by an inflammatory phenotype in severe COVID-19: Possible implications for viral mutagenesis. Eur. Respir. J. 60 (2). doi: 10.1183/13993003.02951-2021
Flynn, J. M., O'Leary, M. N., Zambataro, C. A., Academia, E. C., Presley, M. P., Garrett, B. J., et al. (2013). Late-life rapamycin treatment reverses age-related heart dysfunction. Aging Cell 12 (5), 851–862. doi: 10.1111/acel.12109
Franceschi, C., Bonafè, M., Valensin, S., Olivieri, F., De Luca, M., Ottaviani, E., et al. (2000). Inflamm-aging. an evolutionary perspective on immunosenescence. Ann. N Y Acad. Sci. 908, 244–254. doi: 10.1111/j.1749-6632.2000.tb06651.x
Freund, A., Orjalo, A. V., Desprez, P. Y., Campisi, J. (2010). Inflammatory networks during cellular senescence: Causes and consequences. Trends Mol. Med. 16 (5), 238–246. doi: 10.1016/j.molmed.2010.03.003
Fumagalli, M., Rossiello, F., Mondello, C., d'Adda di Fagagna, F. (2014). Stable cellular senescence is associated with persistent DDR activation. PloS One 9 (10), e110969. doi: 10.1371/journal.pone.0110969
Gallant, J., Hsue, P. Y., Shreay, S., Meyer, N. (2017). Comorbidities among US patients with prevalent HIV infection-a trend analysis. J. Infect. Dis. 216 (12), 1525–1533. doi: 10.1093/infdis/jix518
Gao, D., Wu, J., Wu, Y. T., Du, F., Aroh, C., Yan, N., et al. (2013). Cyclic GMP-AMP synthase is an innate immune sensor of HIV and other retroviruses. Science 341 (6148), 903–906. doi: 10.1126/science.1240933
Geier, C., Perl, A. (2021). Therapeutic mTOR blockade in systemic autoimmunity: Implications for antiviral immunity and extension of lifespan. Autoimmun Rev. 20 (12), 102984. doi: 10.1016/j.autrev.2021.102984
George, P. M., Wells, A. U., Jenkins, R. G. (2020). Pulmonary fibrosis and COVID-19: the potential role for antifibrotic therapy. Lancet Respir. Med. 8 (8), 807–815. doi: 10.1016/s2213-2600(20)30225-3
Giannis, D., Ziogas, I. A., Gianni, P. (2020). Coagulation disorders in coronavirus infected patients: COVID-19, SARS-CoV-1, MERS-CoV and lessons from the past. J. Clin. Virol. 127, 104362. doi: 10.1016/j.jcv.2020.104362
Glück, S., Guey, B., Gulen, M. F., Wolter, K., Kang, T. W., Schmacke, N. A., et al. (2017). Innate immune sensing of cytosolic chromatin fragments through cGAS promotes senescence. Nat. Cell Biol. 19 (9), 1061–1070. doi: 10.1038/ncb3586
Gonzalez-Meljem, J. M., Haston, S., Carreno, G., Apps, J. R., Pozzi, S., Stache, C., et al. (2017). Stem cell senescence drives age-attenuated induction of pituitary tumours in mouse models of paediatric craniopharyngioma. Nat. Commun. 8 (1), 1819. doi: 10.1038/s41467-017-01992-5
Gorgoulis, V., Adams, P. D., Alimonti, A., Bennett, D. C., Bischof, O., Bishop, C., et al. (2019). Cellular senescence: Defining a path forward. Cell 179 (4), 813–827. doi: 10.1016/j.cell.2019.10.005
Grasselli, G., Zangrillo, A., Zanella, A., Antonelli, M., Cabrini, L., Castelli, A., et al. (2020). Baseline characteristics and outcomes of 1591 patients infected with SARS-CoV-2 admitted to ICUs of the Lombardy region, Italy. JAMA 323 (16), 1574–1581. doi: 10.1001/jama.2020.5394
Group, R. C. (2021). Tocilizumab in patients admitted to hospital with COVID-19 (RECOVERY): A randomised, controlled, open-label, platform trial. Lancet 397 (10285), 1637–1645. doi: 10.1016/s0140-6736(21)00676-0
Gupta, S., Wang, W., Hayek, S. S., Chan, L., Mathews, K. S., Melamed, M. L., et al. (2021). Association between early treatment with tocilizumab and mortality among critically ill patients with COVID-19. JAMA Intern. Med. 181 (1), 41–51. doi: 10.1001/jamainternmed.2020.6252
Ha, T. T., Huy, N. T., Murao, L. A., Lan, N. T., Thuy, T. T., Tuan, H. M., et al. (2011). Elevated levels of cell-free circulating DNA in patients with acute dengue virus infection. PloS One 6 (10), e25969. doi: 10.1371/journal.pone.0025969
Hafez, A. Y., Luftig, M. A. (2017). Characterization of the EBV-induced persistent DNA damage response. Viruses 9 (12). doi: 10.3390/v9120366
Hakim, F. T., Memon, S. A., Cepeda, R., Jones, E. C., Chow, C. K., Kasten-Sportes, C., et al. (2005). Age-dependent incidence, time course, and consequences of thymic renewal in adults. J. Clin. Invest. 115 (4), 930–939. doi: 10.1172/jci22492
Hanada, Y., Ishihara, N., Wang, L., Otera, H., Ishihara, T., Koshiba, T., et al. (2020). MAVS is energized by mff which senses mitochondrial metabolism via AMPK for acute antiviral immunity. Nat. Commun. 11 (1), 5711. doi: 10.1038/s41467-020-19287-7
Hari, P., Millar, F. R., Tarrats, N., Birch, J., Quintanilla, A., Rink, C. J., et al. (2019). The innate immune sensor toll-like receptor 2 controls the senescence-associated secretory phenotype. Sci. Adv. 5 (6), eaaw0254. doi: 10.1126/sciadv.aaw0254
Hayflick, L. (1965). The limited in vitro lifetime of human diploid cell strains. Exp. Cell Res. 37, 614–636. doi: 10.1016/0014-4827(65)90211-9
Hayflick, L., Moorhead, P. S. (1961). The serial cultivation of human diploid cell strains. Exp. Cell Res. 25, 585–621. doi: 10.1016/0014-4827(61)90192-6
He, S., Sharpless, N. E. (2017). Senescence in health and disease. Cell 169 (6), 1000–1011. doi: 10.1016/j.cell.2017.05.015
Hearps, A. C., Martin, G. E., Angelovich, T. A., Cheng, W. J., Maisa, A., Landay, A. L., et al. (2012). Aging is associated with chronic innate immune activation and dysregulation of monocyte phenotype and function. Aging Cell 11 (5), 867–875. doi: 10.1111/j.1474-9726.2012.00851.x
Helms, J., Tacquard, C., Severac, F., Leonard-Lorant, I., Ohana, M., Delabranche, X., et al. (2020). High risk of thrombosis in patients with severe SARS-CoV-2 infection: A multicenter prospective cohort study. Intensive Care Med. 46 (6), 1089–1098. doi: 10.1007/s00134-020-06062-x
Hernandez-Segura, A., de Jong, T. V., Melov, S., Guryev, V., Campisi, J., Demaria, M. (2017). Unmasking transcriptional heterogeneity in senescent cells. Curr. Biol. 27 (17), 2652–2660.e2654. doi: 10.1016/j.cub.2017.07.033
Hernandez-Segura, A., Nehme, J., Demaria, M. (2018). Hallmarks of cellular senescence. Trends Cell Biol. 28 (6), 436–453. doi: 10.1016/j.tcb.2018.02.001
Herranz, N., Gallage, S., Mellone, M., Wuestefeld, T., Klotz, S., Hanley, C. J., et al. (2015). mTOR regulates MAPKAPK2 translation to control the senescence-associated secretory phenotype. Nat. Cell Biol. 17 (9), 1205–1217. doi: 10.1038/ncb3225
Hickson, L. J., Langhi Prata, L. G. P., Bobart, S. A., Evans, T. K., Giorgadze, N., Hashmi, S. K., et al. (2019). Senolytics decrease senescent cells in humans: Preliminary report from a clinical trial of dasatinib plus quercetin in individuals with diabetic kidney disease. EBioMedicine 47, 446–456. doi: 10.1016/j.ebiom.2019.08.069
Hoffmann, M., Pantazis, N., Martin, G. E., Hickling, S., Hurst, J., Meyerowitz, J., et al. (2016). Exhaustion of activated CD8 T cells predicts disease progression in primary HIV-1 infection. PloS Pathog. 12 (7), e1005661. doi: 10.1371/journal.ppat.1005661
Hopfner, K. P., Hornung, V. (2020). Molecular mechanisms and cellular functions of cGAS-STING signalling. Nat. Rev. Mol. Cell Biol. 21 (9), 501–521. doi: 10.1038/s41580-020-0244-x
Huet, T., Beaussier, H., Voisin, O., Jouveshomme, S., Dauriat, G., Lazareth, I., et al. (2020). Anakinra for severe forms of COVID-19: A cohort study. Lancet Rheumatol 2 (7), e393–e400. doi: 10.1016/s2665-9913(20)30164-8
Husain, A., Byrareddy, S. N. (2020). Rapamycin as a potential repurpose drug candidate for the treatment of COVID-19. Chem. Biol. Interact. 331, 109282. doi: 10.1016/j.cbi.2020.109282
Idrissi, M. E., Hachem, H., Koering, C., Merle, P., Thénoz, M., Mortreux, F., et al. (2016). HBx triggers either cellular senescence or cell proliferation depending on cellular phenotype. J. Viral Hepat 23 (2), 130–138. doi: 10.1111/jvh.12450
Ivanov, A., Pawlikowski, J., Manoharan, I., van Tuyn, J., Nelson, D. M., Rai, T. S., et al. (2013). Lysosome-mediated processing of chromatin in senescence. J. Cell Biol. 202 (1), 129–143. doi: 10.1083/jcb.201212110
Jun, J. I., Lau, L. F. (2010). The matricellular protein CCN1 induces fibroblast senescence and restricts fibrosis in cutaneous wound healing. Nat. Cell Biol. 12 (7), 676–685. doi: 10.1038/ncb2070
Justice, J. N., Nambiar, A. M., Tchkonia, T., LeBrasseur, N. K., Pascual, R., Hashmi, S. K., et al. (2019). Senolytics in idiopathic pulmonary fibrosis: Results from a first-in-human, open-label, pilot study. EBioMedicine 40, 554–563. doi: 10.1016/j.ebiom.2018.12.052
Kang, T. W., Yevsa, T., Woller, N., Hoenicke, L., Wuestefeld, T., Dauch, D., et al. (2011). Senescence surveillance of pre-malignant hepatocytes limits liver cancer development. Nature 479 (7374), 547–551. doi: 10.1038/nature10599
Kang, Y., Zhang, H., Zhao, Y., Wang, Y., Wang, W., He, Y., et al. (2018). Telomere dysfunction disturbs macrophage mitochondrial metabolism and the NLRP3 inflammasome through the PGC-1α/TNFAIP3 axis. Cell Rep. 22 (13), 3493–3506. doi: 10.1016/j.celrep.2018.02.071
Kawai, T., Akira, S. (2008). Toll-like receptor and RIG-i-like receptor signaling. Ann. N Y Acad. Sci. 1143, 1–20. doi: 10.1196/annals.1443.020
Kelley, W. J., Zemans, R. L., Goldstein, D. R. (2020). Cellular senescence: Friend or foe to respiratory viral infections? Eur. Respir. J. 56 (6). doi: 10.1183/13993003.02708-2020
Khan, N., Shariff, N., Cobbold, M., Bruton, R., Ainsworth, J. A., Sinclair, A. J., et al. (2002). Cytomegalovirus seropositivity drives the CD8 T cell repertoire toward greater clonality in healthy elderly individuals. J. Immunol. 169 (4), 1984–1992. doi: 10.4049/jimmunol.169.4.1984
Kim, J. A., Seong, R. K., Shin, O. S. (2016). Enhanced viral replication by cellular replicative senescence. Immune Netw. 16 (5), 286–295. doi: 10.4110/in.2016.16.5.286
Kindrachuk, J., Ork, B., Hart, B. J., Mazur, S., Holbrook, M. R., Frieman, M. B., et al. (2015). Antiviral potential of ERK/MAPK and PI3K/AKT/mTOR signaling modulation for middle East respiratory syndrome coronavirus infection as identified by temporal kinome analysis. Antimicrob. Agents Chemother. 59 (2), 1088–1099. doi: 10.1128/aac.03659-14
Koch, S., Larbi, A., Ozcelik, D., Solana, R., Gouttefangeas, C., Attig, S., et al. (2007). Cytomegalovirus infection: A driving force in human T cell immunosenescence. Ann. N Y Acad. Sci. 1114, 23–35. doi: 10.1196/annals.1396.043
Koopal, S., Furuhjelm, J. H., Järviluoma, A., Jäämaa, S., Pyakurel, P., Pussinen, C., et al. (2007). Viral oncogene-induced DNA damage response is activated in kaposi sarcoma tumorigenesis. PloS Pathog. 3 (9), 1348–1360. doi: 10.1371/journal.ppat.0030140
Korolchuk, V. I., Miwa, S., Carroll, B., von Zglinicki, T. (2017). Mitochondria in cell senescence: Is mitophagy the weakest link? EBioMedicine 21, 7–13. doi: 10.1016/j.ebiom.2017.03.020
Krizhanovsky, V., Yon, M., Dickins, R. A., Hearn, S., Simon, J., Miething, C., et al. (2008). Senescence of activated stellate cells limits liver fibrosis. Cell 134 (4), 657–667. doi: 10.1016/j.cell.2008.06.049
Kulkarni, A. S., Gubbi, S., Barzilai, N. (2020). Benefits of metformin in attenuating the hallmarks of aging. Cell Metab. 32 (1), 15–30. doi: 10.1016/j.cmet.2020.04.001
Kulkarni, U., Zemans, R. L., Smith, C. A., Wood, S. C., Deng, J. C., Goldstein, D. R. (2019). Excessive neutrophil levels in the lung underlie the age-associated increase in influenza mortality. Mucosal Immunol. 12 (2), 545–554. doi: 10.1038/s41385-018-0115-3
Kuller, L. H., Tracy, R., Belloso, W., De Wit, S., Drummond, F., Lane, H. C., et al. (2008). Inflammatory and coagulation biomarkers and mortality in patients with HIV infection. PloS Med. 5 (10), e203. doi: 10.1371/journal.pmed.0050203
Kurz, D. J., Decary, S., Hong, Y., Erusalimsky, J. D. (2000). Senescence-associated (beta)-galactosidase reflects an increase in lysosomal mass during replicative ageing of human endothelial cells. J. Cell Sci. 113 (Pt 20), 3613–3622. doi: 10.1242/jcs.113.20.3613
Laberge, R. M., Sun, Y., Orjalo, A. V., Patil, C. K., Freund, A., Zhou, L., et al. (2015). MTOR regulates the pro-tumorigenic senescence-associated secretory phenotype by promoting IL1A translation. Nat. Cell Biol. 17 (8), 1049–1061. doi: 10.1038/ncb3195
Lahaye, X., Gentili, M., Silvin, A., Conrad, C., Picard, L., Jouve, M., et al. (2018). NONO detects the nuclear HIV capsid to promote cGAS-mediated innate immune activation. Cell 175 (2), 488–501.e422. doi: 10.1016/j.cell.2018.08.062
Lara, P. C., Macías-Verde, D., Burgos-Burgos, J. (2020). Age-induced NLRP3 inflammasome over-activation increases lethality of SARS-CoV-2 pneumonia in elderly patients. Aging Dis. 11 (4), 756–762. doi: 10.14336/ad.2020.0601
Lee, B. Y., Han, J. A., Im, J. S., Morrone, A., Johung, K., Goodwin, E. C., et al. (2006). Senescence-associated beta-galactosidase is lysosomal beta-galactosidase. Aging Cell 5 (2), 187–195. doi: 10.1111/j.1474-9726.2006.00199.x
Lee, S., Yu, Y., Trimpert, J., Benthani, F., Mairhofer, M., Richter-Pechanska, P., et al. (2021). Virus-induced senescence is a driver and therapeutic target in COVID-19. Nature 599 (7884), 283–289. doi: 10.1038/s41586-021-03995-1
Lehmann, M., Korfei, M., Mutze, K., Klee, S., Skronska-Wasek, W., Alsafadi, H. N., et al. (2017). Senolytic drugs target alveolar epithelial cell function and attenuate experimental lung fibrosis ex vivo. Eur. Respir. J. 50 (2). doi: 10.1183/13993003.02367-2016
Leidal, A. M., Cyr, D. P., Hill, R. J., Lee, P. W., McCormick, C. (2012). Subversion of autophagy by kaposi's sarcoma-associated herpesvirus impairs oncogene-induced senescence. Cell Host Microbe 11 (2), 167–180. doi: 10.1016/j.chom.2012.01.005
Leng, S. X., Margolick, J. B. (2020). Aging, sex, inflammation, frailty, and CMV and HIV infections. Cell Immunol. 348, 104024. doi: 10.1016/j.cellimm.2019.104024
Li, J., Wei, Q., Li, W. X., McCowen, K. C., Xiong, W., Liu, J., et al. (2020). Metformin use in diabetes prior to hospitalization: Effects on mortality in covid-19. Endocr. Pract. 26 (10), 1166–1172. doi: 10.4158/ep-2020-0466
Lian, H., Wei, J., Zang, R., Ye, W., Yang, Q., Zhang, X. N., et al. (2018). ZCCHC3 is a co-sensor of cGAS for dsDNA recognition in innate immune response. Nat. Commun. 9 (1), 3349. doi: 10.1038/s41467-018-05559-w
Liu, Z. S., Cai, H., Xue, W., Wang, M., Xia, T., Li, W. J., et al. (2019). G3BP1 promotes DNA binding and activation of cGAS. Nat. Immunol. 20 (1), 18–28. doi: 10.1038/s41590-018-0262-4
Liu, J., Qian, C., Cao, X. (2016). Post-translational modification control of innate immunity. Immunity 45 (1), 15–30. doi: 10.1016/j.immuni.2016.06.020
Loo, J., Spittle, D. A., Newnham, M. (2021). COVID-19, immunothrombosis and venous thromboembolism: biological mechanisms. Thorax 76 (4), 412–420. doi: 10.1136/thoraxjnl-2020-216243
López-Otín, C., Blasco, M. A., Partridge, L., Serrano, M., Kroemer, G. (2013). The hallmarks of aging. Cell 153 (6), 1194–1217. doi: 10.1016/j.cell.2013.05.039
Luo, P., Qiu, L., Liu, Y., Liu, X. L., Zheng, J. L., Xue, H. Y., et al. (2020). Metformin treatment was associated with decreased mortality in COVID-19 patients with diabetes in a retrospective analysis. Am. J. Trop. Med. Hyg 103 (1), 69–72. doi: 10.4269/ajtmh.20-0375
Lv, N., Zhao, Y., Liu, X., Ye, L., Liang, Z., Kang, Y., et al. (2022). Dysfunctional telomeres through mitostress-induced cGAS/STING activation to aggravate immune senescence and viral pneumonia. Aging Cell 21 (4), e13594. doi: 10.1111/acel.13594
Lynch, S. M., Guo, G., Gibson, D. S., Bjourson, A. J., Rai, T. S. (2021). Role of senescence and aging in SARS-CoV-2 infection and COVID-19 disease. Cells 10 (12). doi: 10.3390/cells10123367
Majumder, S., Caccamo, A., Medina, D. X., Benavides, A. D., Javors, M. A., Kraig, E., et al. (2012). Lifelong rapamycin administration ameliorates age-dependent cognitive deficits by reducing IL-1β and enhancing NMDA signaling. Aging Cell 11 (2), 326–335. doi: 10.1111/j.1474-9726.2011.00791.x
Mannick, J. B., Del Giudice, G., Lattanzi, M., Valiante, N. M., Praestgaard, J., Huang, B., et al. (2014). mTOR inhibition improves immune function in the elderly. Sci. Transl. Med. 6 (268), 268ra179. doi: 10.1126/scitranslmed.3009892
Mao, L., Jin, H., Wang, M., Hu, Y., Chen, S., He, Q., et al. (2020). Neurologic manifestations of hospitalized patients with coronavirus disease 2019 in wuhan, China. JAMA Neurol. 77 (6), 683–690. doi: 10.1001/jamaneurol.2020.1127
Marcucci, F., Romeo, E., Caserta, C. A., Rumio, C., Lefoulon, F. (2020). Context-dependent pharmacological effects of metformin on the immune system. Trends Pharmacol. Sci. 41 (3), 162–171. doi: 10.1016/j.tips.2020.01.003
Markowicz-Piasecka, M., Sikora, J., Szydłowska, A., Skupień, A., Mikiciuk-Olasik, E., Huttunen, K. M. (2017). Metformin - a future therapy for neurodegenerative diseases : Theme: Drug discovery, development and delivery in alzheimer's disease guest Editor: Davide brambilla. Pharm. Res. 34 (12), 2614–2627. doi: 10.1007/s11095-017-2199-y
Martínez, I., García-Carpizo, V., Guijarro, T., García-Gomez, A., Navarro, D., Aranda, A., et al. (2016). Induction of DNA double-strand breaks and cellular senescence by human respiratory syncytial virus. Virulence 7 (4), 427–442. doi: 10.1080/21505594.2016.1144001
Martin-Montalvo, A., Mercken, E. M., Mitchell, S. J., Palacios, H. H., Mote, P. L., Scheibye-Knudsen, M., et al. (2013). Metformin improves healthspan and lifespan in mice. Nat. Commun. 4, 2192. doi: 10.1038/ncomms3192
Massanella, M., Gómez-Mora, E., Carrillo, J., Curriu, M., Ouchi, D., Puig, J., et al. (2015). Increased ex vivo cell death of central memory CD4 T cells in treated HIV infected individuals with unsatisfactory immune recovery. J. Transl. Med. 13, 230. doi: 10.1186/s12967-015-0601-2
Mills, E. L., Kelly, B., O'Neill, L. A. J. (2017). Mitochondria are the powerhouses of immunity. Nat. Immunol. 18 (5), 488–498. doi: 10.1038/ni.3704
Moiseeva, O., Deschênes-Simard, X., St-Germain, E., Igelmann, S., Huot, G., Cadar, A. E., et al. (2013). Metformin inhibits the senescence-associated secretory phenotype by interfering with IKK/NF-κB activation. Aging Cell 12 (3), 489–498. doi: 10.1111/acel.12075
Muñoz-Espín, D., Serrano, M. (2014). Cellular senescence: from physiology to pathology. Nat. Rev. Mol. Cell Biol. 15 (7), 482–496. doi: 10.1038/nrm3823
Napoli, C., Tritto, I., Benincasa, G., Mansueto, G., Ambrosio, G. (2020). Cardiovascular involvement during COVID-19 and clinical implications in elderly patients. a review. Ann. Med. Surg. (Lond) 57, 236–243. doi: 10.1016/j.amsu.2020.07.054
Naranbhai, V., Altfeld, M., Karim, S. S., Ndung'u, T., Karim, Q. A., Carr, W. H. (2013). Changes in natural killer cell activation and function during primary HIV-1 infection. PloS One 8 (1), e53251. doi: 10.1371/journal.pone.0053251
Narita, M., Nũnez, S., Heard, E., Narita, M., Lin, A. W., Hearn, S. A., et al. (2003). Rb-Mediated heterochromatin formation and silencing of E2F target genes during cellular senescence. Cell 113 (6), 703–716. doi: 10.1016/s0092-8674(03)00401-x
Nassour, J., Radford, R., Correia, A., Fusté, J. M., Schoell, B., Jauch, A., et al. (2019). Autophagic cell death restricts chromosomal instability during replicative crisis. Nature 565 (7741), 659–663. doi: 10.1038/s41586-019-0885-0
Nehme, J., Borghesan, M., Mackedenski, S., Bird, T. G., Demaria, M. (2020). Cellular senescence as a potential mediator of COVID-19 severity in the elderly. Aging Cell 19 (10), e13237. doi: 10.1111/acel.13237
Netea, M. G., Domínguez-Andrés, J., Barreiro, L. B., Chavakis, T., Divangahi, M., Fuchs, E., et al. (2020a). Defining trained immunity and its role in health and disease. Nat. Rev. Immunol. 20 (6), 375–388. doi: 10.1038/s41577-020-0285-6
Netea, M. G., Giamarellos-Bourboulis, E. J., Domínguez-Andrés, J., Curtis, N., van Crevel, R., van de Veerdonk, F. L., et al. (2020b). Trained immunity: a tool for reducing susceptibility to and the severity of SARS-CoV-2 infection. Cell 181 (5), 969–977. doi: 10.1016/j.cell.2020.04.042
Noris, E., Zannetti, C., Demurtas, A., Sinclair, J., De Andrea, M., Gariglio, M., et al. (2002). Cell cycle arrest by human cytomegalovirus 86-kDa IE2 protein resembles premature senescence. J. Virol. 76 (23), 12135–12148. doi: 10.1128/jvi.76.23.12135-12148.2002
Nunnari, J., Suomalainen, A. (2012). Mitochondria: In sickness and in health. Cell 148 (6), 1145–1159. doi: 10.1016/j.cell.2012.02.035
Nyunoya, T., Monick, M. M., Klingelhutz, A. L., Glaser, H., Cagley, J. R., Brown, C. O., et al. (2009). Cigarette smoke induces cellular senescence via werner's syndrome protein down-regulation. Am. J. Respir. Crit. Care Med. 179 (4), 279–287. doi: 10.1164/rccm.200802-320OC
Nyunoya, T., Monick, M. M., Klingelhutz, A., Yarovinsky, T. O., Cagley, J. R., Hunninghake, G. W. (2006). Cigarette smoke induces cellular senescence. Am. J. Respir. Cell Mol. Biol. 35 (6), 681–688. doi: 10.1165/rcmb.2006-0169OC
Obi, A. T., Tignanelli, C. J., Jacobs, B. N., Arya, S., Park, P. K., Wakefield, T. W., et al. (2019). Empirical systemic anticoagulation is associated with decreased venous thromboembolism in critically ill influenza a H1N1 acute respiratory distress syndrome patients. J. Vasc. Surg. Venous Lymphat Disord. 7 (3), 317–324. doi: 10.1016/j.jvsv.2018.08.010
Ohno, M., Sasaki, M., Orba, Y., Sekiya, T., Masum, M. A., Ichii, O., et al. (2021). Abnormal blood coagulation and kidney damage in aged hamsters infected with severe acute respiratory syndrome coronavirus 2. Viruses 13 (11). doi: 10.3390/v13112137
Oishi, N., Shilagardi, K., Nakamoto, Y., Honda, M., Kaneko, S., Murakami, S. (2007). Hepatitis b virus X protein overcomes oncogenic RAS-induced senescence in human immortalized cells. Cancer Sci. 98 (10), 1540–1548. doi: 10.1111/j.1349-7006.2007.00579.x
Onder, G., Rezza, G., Brusaferro, S. (2020). Case-fatality rate and characteristics of patients dying in relation to COVID-19 in Italy. JAMA 323 (18), 1775–1776. doi: 10.1001/jama.2020.4683
Panagiotou, O. A., Kosar, C. M., White, E. M., Bantis, L. E., Yang, X., Santostefano, C. M., et al. (2021). Risk factors associated with all-cause 30-day mortality in nursing home residents with COVID-19. JAMA Intern. Med. 181 (4), 439–448. doi: 10.1001/jamainternmed.2020.7968
Passos, J. F., Saretzki, G., Ahmed, S., Nelson, G., Richter, T., Peters, H., et al. (2007). Mitochondrial dysfunction accounts for the stochastic heterogeneity in telomere-dependent senescence. PloS Biol. 5 (5), e110. doi: 10.1371/journal.pbio.0050110
Paul, B. D., Snyder, S. H., Bohr, V. A. (2021). Signaling by cGAS-STING in neurodegeneration, neuroinflammation, and aging. Trends Neurosci. 44 (2), 83–96. doi: 10.1016/j.tins.2020.10.008
Peiró, T., Patel, D. F., Akthar, S., Gregory, L. G., Pyle, C. J., Harker, J. A., et al. (2018). Neutrophils drive alveolar macrophage IL-1β release during respiratory viral infection. Thorax 73 (6), 546–556. doi: 10.1136/thoraxjnl-2017-210010
Persson, B. D., Jaffe, A. B., Fearns, R., Danahay, H. (2014). Respiratory syncytial virus can infect basal cells and alter human airway epithelial differentiation. PloS One 9 (7), e102368. doi: 10.1371/journal.pone.0102368
Porfidia, A., Valeriani, E., Pola, R., Porreca, E., Rutjes, A. W. S., Di Nisio, M. (2020). Venous thromboembolism in patients with COVID-19: Systematic review and meta-analysis. Thromb. Res. 196, 67–74. doi: 10.1016/j.thromres.2020.08.020
Propson, N. E., Roy, E. R., Litvinchuk, A., Köhl, J., Zheng, H. (2021). Endothelial C3a receptor mediates vascular inflammation and blood-brain barrier permeability during aging. J. Clin. Invest. 131 (1). doi: 10.1172/jci140966
Roos, C. M., Zhang, B., Palmer, A. K., Ogrodnik, M. B., Pirtskhalava, T., Thalji, N. M., et al. (2016). Chronic senolytic treatment alleviates established vasomotor dysfunction in aged or atherosclerotic mice. Aging Cell 15 (5), 973–977. doi: 10.1111/acel.12458
Roschewski, M., Lionakis, M. S., Sharman, J. P., Roswarski, J., Goy, A., Monticelli, M. A., et al. (2020). Inhibition of bruton tyrosine kinase in patients with severe COVID-19. Sci. Immunol. 5 (48). doi: 10.1126/sciimmunol.abd0110
Sadaie, M., Salama, R., Carroll, T., Tomimatsu, K., Chandra, T., Young, A. R., et al. (2013). Redistribution of the lamin B1 genomic binding profile affects rearrangement of heterochromatic domains and SAHF formation during senescence. Genes Dev. 27 (16), 1800–1808. doi: 10.1101/gad.217281.113
Sagiv, A., Burton, D. G., Moshayev, Z., Vadai, E., Wensveen, F., Ben-Dor, S., et al. (2016). NKG2D ligands mediate immunosurveillance of senescent cells. Aging (Albany NY) 8 (2), 328–344. doi: 10.18632/aging.100897
Sanchez-Vazquez, R., Guío-Carrión, A., Zapatero-Gaviria, A., Martínez, P., Blasco, M. A. (2021). Shorter telomere lengths in patients with severe COVID-19 disease. Aging (Albany NY) 13 (1), 1–15. doi: 10.18632/aging.202463
Sandler, N. G., Douek, D. C. (2012). Microbial translocation in HIV infection: causes, consequences and treatment opportunities. Nat. Rev. Microbiol. 10 (9), 655–666. doi: 10.1038/nrmicro2848
Sapir, A., Avinoam, O., Podbilewicz, B., Chernomordik, L. V. (2008). Viral and developmental cell fusion mechanisms: conservation and divergence. Dev. Cell 14 (1), 11–21. doi: 10.1016/j.devcel.2007.12.008
Sauce, D., Larsen, M., Fastenackels, S., Pauchard, M., Ait-Mohand, H., Schneider, L., et al. (2011). HIV Disease progression despite suppression of viral replication is associated with exhaustion of lymphopoiesis. Blood 117 (19), 5142–5151. doi: 10.1182/blood-2011-01-331306
Schmitt, C. A., Tchkonia, T., Niedernhofer, L. J., Robbins, P. D., Kirkland, J. L., Lee, S. (2022). COVID-19 and cellular senescence. Nat. Rev. Immunol. 1-13. doi: 10.1038/s41577-022-00785-2
Seidler, S., Zimmermann, H. W., Bartneck, M., Trautwein, C., Tacke, F. (2010). Age-dependent alterations of monocyte subsets and monocyte-related chemokine pathways in healthy adults. BMC Immunol. 11, 30. doi: 10.1186/1471-2172-11-30
Shah, P. P., Donahue, G., Otte, G. L., Capell, B. C., Nelson, D. M., Cao, K., et al. (2013). Lamin B1 depletion in senescent cells triggers large-scale changes in gene expression and the chromatin landscape. Genes Dev. 27 (16), 1787–1799. doi: 10.1101/gad.223834.113
Sharma, P., Uppal, N. N., Wanchoo, R., Shah, H. H., Yang, Y., Parikh, R., et al. (2020). COVID-19-Associated kidney injury: A case series of kidney biopsy findings. J. Am. Soc. Nephrol. 31 (9), 1948–1958. doi: 10.1681/asn.2020050699
Shay, J. W. (2016). Role of telomeres and telomerase in aging and cancer. Cancer Discov 6 (6), 584–593. doi: 10.1158/2159-8290.Cd-16-0062
Shrivastava, G., León-Juárez, M., García-Cordero, J., Meza-Sánchez, D. E., Cedillo-Barrón, L. (2016). Inflammasomes and its importance in viral infections. Immunol. Res. 64 (5-6), 1101–1117. doi: 10.1007/s12026-016-8873-z
Sivasubramanian, M. K., Monteiro, R., Harrison, K. S., Plakkot, B., Subramanian, M., Jones, C. (2022). Herpes simplex virus type 1 preferentially enhances neuro-inflammation and senescence in brainstem of female mice. J. Virol. 96 (17), e0108122. doi: 10.1128/jvi.01081-22
Smits, S. L., de Lang, A., van den Brand, J. M., Leijten, L. M., van, I. W. F., Eijkemans, M. J., et al. (2010). Exacerbated innate host response to SARS-CoV in aged non-human primates. PloS Pathog. 6 (2), e1000756. doi: 10.1371/journal.ppat.1000756
Sun, B., Sundström, K. B., Chew, J. J., Bist, P., Gan, E. S., Tan, H. C., et al. (2017). Dengue virus activates cGAS through the release of mitochondrial DNA. Sci. Rep. 7 (1), 3594. doi: 10.1038/s41598-017-03932-1
Sviridov, D., Miller, Y. I., Bukrinsky, M. I. (2022). Trained immunity and HIV infection. Front. Immunol. 13. doi: 10.3389/fimmu.2022.903884
Tai, H., Wang, Z., Gong, H., Han, X., Zhou, J., Wang, X., et al. (2017). Autophagy impairment with lysosomal and mitochondrial dysfunction is an important characteristic of oxidative stress-induced senescence. Autophagy 13 (1), 99–113. doi: 10.1080/15548627.2016.1247143
Takahashi, A., Loo, T. M., Okada, R., Kamachi, F., Watanabe, Y., Wakita, M., et al. (2018). Downregulation of cytoplasmic DNases is implicated in cytoplasmic DNA accumulation and SASP in senescent cells. Nat. Commun. 9 (1), 1249. doi: 10.1038/s41467-018-03555-8
Tchkonia, T., Zhu, Y., van Deursen, J., Campisi, J., Kirkland, J. L. (2013). Cellular senescence and the senescent secretory phenotype: therapeutic opportunities. J. Clin. Invest. 123 (3), 966–972. doi: 10.1172/jci64098
Thangaraj, A., Chivero, E. T., Tripathi, A., Singh, S., Niu, F., Guo, M. L., et al. (2021). HIV TAT-mediated microglial senescence: Role of SIRT3-dependent mitochondrial oxidative stress. Redox Biol. 40, 101843. doi: 10.1016/j.redox.2020.101843
The Novel Coronavirus Pneumonia Emergency Response Epidemiology Team (2020). The epidemiological characteristics of an outbreak of 2019 novel coronavirus diseases (COVID-19) - China 2020. China CDC Wkly 2 (8), 113–122. doi: 10.46234/ccdcw2020.032
Thompson, W. W., Shay, D. K., Weintraub, E., Brammer, L., Cox, N., Anderson, L. J., et al. (2003). Mortality associated with influenza and respiratory syncytial virus in the united states. JAMA 289 (2), 179–186. doi: 10.1001/jama.289.2.179
Tizazu, A. M., Nyunt, M. S. Z., Cexus, O., Suku, K., Mok, E., Xian, C. H., et al. (2019). Metformin monotherapy downregulates diabetes-associated inflammatory status and impacts on mortality. Front. Physiol. 10. doi: 10.3389/fphys.2019.00572
Tripathi, U., Nchioua, R., Prata, L., Zhu, Y., Gerdes, E. O. W., Giorgadze, N., et al. (2021). SARS-CoV-2 causes senescence in human cells and exacerbates the senescence-associated secretory phenotype through TLR-3. Aging (Albany NY) 13 (18), 21838–21854. doi: 10.18632/aging.203560
Valencia, W. M., Palacio, A., Tamariz, L., Florez, H. (2017). Metformin and ageing: improving ageing outcomes beyond glycaemic control. Diabetologia 60 (9), 1630–1638. doi: 10.1007/s00125-017-4349-5
van Deursen, J. M. (2014). The role of senescent cells in ageing. Nature 509 (7501), 439–446. doi: 10.1038/nature13193
Vázquez-Castellanos, J. F., Serrano-Villar, S., Latorre, A., Artacho, A., Ferrús, M. L., Madrid, N., et al. (2015). Altered metabolism of gut microbiota contributes to chronic immune activation in HIV-infected individuals. Mucosal Immunol. 8 (4), 760–772. doi: 10.1038/mi.2014.107
Wandeler, G., Johnson, L. F., Egger, M. (2016). Trends in life expectancy of HIV-positive adults on antiretroviral therapy across the globe: comparisons with general population. Curr. Opin. HIV AIDS 11 (5), 492–500. doi: 10.1097/coh.0000000000000298
Wang, C. H., Chung, F. T., Lin, S. M., Huang, S. Y., Chou, C. L., Lee, K. Y., et al. (2014). Adjuvant treatment with a mammalian target of rapamycin inhibitor, sirolimus, and steroids improves outcomes in patients with severe H1N1 pneumonia and acute respiratory failure. Crit. Care Med. 42 (2), 313–321. doi: 10.1097/CCM.0b013e3182a2727d
Wang, L., He, W., Yu, X., Hu, D., Bao, M., Liu, H., et al. (2020a). Coronavirus disease 2019 in elderly patients: Characteristics and prognostic factors based on 4-week follow-up. J. Infect. 80 (6), 639–645. doi: 10.1016/j.jinf.2020.03.019
Wang, W., Hu, D., Wu, C., Feng, Y., Li, A., Liu, W., et al. (2020b). STING promotes NLRP3 localization in ER and facilitates NLRP3 deubiquitination to activate the inflammasome upon HSV-1 infection. PloS Pathog. 16 (3), e1008335. doi: 10.1371/journal.ppat.1008335
West, A. P., Khoury-Hanold, W., Staron, M., Tal, M. C., Pineda, C. M., Lang, S. M., et al. (2015). Mitochondrial DNA stress primes the antiviral innate immune response. Nature 520 (7548), 553–557. doi: 10.1038/nature14156
Wheaton, K., Campuzano, D., Ma, W., Sheinis, M., Ho, B., Brown, G. W., et al. (2017). Progerin-induced replication stress facilitates premature senescence in Hutchinson-gilford progeria syndrome. Mol. Cell Biol. 37 (14). doi: 10.1128/mcb.00659-16
Wiley, C. D., Liu, S., Limbad, C., Zawadzka, A. M., Beck, J., Demaria, M., et al. (2019). SILAC analysis reveals increased secretion of hemostasis-related factors by senescent cells. Cell Rep. 28 (13), 3329–3337.e3325. doi: 10.1016/j.celrep.2019.08.049
Wilkins, C., Gale, M., Jr (2010). Recognition of viruses by cytoplasmic sensors. Curr. Opin. Immunol. 22 (1), 41–47. doi: 10.1016/j.coi.2009.12.003
Wilkinson, J. E., Burmeister, L., Brooks, S. V., Chan, C. C., Friedline, S., Harrison, D. E., et al. (2012). Rapamycin slows aging in mice. Aging Cell 11 (4), 675–682. doi: 10.1111/j.1474-9726.2012.00832.x
Xian, H., Liu, Y., Rundberg Nilsson, A., Gatchalian, R., Crother, T. R., Tourtellotte, W. G., et al. (2021). Metformin inhibition of mitochondrial ATP and DNA synthesis abrogates NLRP3 inflammasome activation and pulmonary inflammation. Immunity 54 (7), 1463–1477.e1411. doi: 10.1016/j.immuni.2021.05.004
Xu, M., Pirtskhalava, T., Farr, J. N., Weigand, B. M., Palmer, A. K., Weivoda, M. M., et al. (2018). Senolytics improve physical function and increase lifespan in old age. Nat. Med. 24 (8), 1246–1256. doi: 10.1038/s41591-018-0092-9
Xu, W., Wong, G., Hwang, Y. Y., Larbi, A. (2020). The untwining of immunosenescence and aging. Semin. Immunopathol. 42 (5), 559–572. doi: 10.1007/s00281-020-00824-x
Xue, W., Zender, L., Miething, C., Dickins, R. A., Hernando, E., Krizhanovsky, V., et al. (2007). Senescence and tumour clearance is triggered by p53 restoration in murine liver carcinomas. Nature 445 (7128), 656–660. doi: 10.1038/nature05529
Yamazaki, Y., Baker, D. J., Tachibana, M., Liu, C. C., van Deursen, J. M., Brott, T. G., et al. (2016). Vascular cell senescence contributes to blood-brain barrier breakdown. Stroke 47 (4), 1068–1077. doi: 10.1161/strokeaha.115.010835
Yan, Y., Du, Y., Zheng, H., Wang, G., Li, R., Chen, J., et al. (2017). NS1 of H7N9 influenza a virus induces NO-mediated cellular senescence in Neuro2a cells. Cell Physiol. Biochem. 43 (4), 1369–1380. doi: 10.1159/000481848
Yang, B., Dan, X., Hou, Y., Lee, J. H., Wechter, N., Krishnamurthy, S., et al. (2021). NAD(+) supplementation prevents STING-induced senescence in ataxia telangiectasia by improving mitophagy. Aging Cell 20 (4), e13329. doi: 10.1111/acel.13329
Yang, X., He, Z., Xin, B., Cao, L. (2000). LMP1 of Epstein-Barr virus suppresses cellular senescence associated with the inhibition of p16INK4a expression. Oncogene 19 (16), 2002–2013. doi: 10.1038/sj.onc.1203515
Yoh, S. M., Schneider, M., Seifried, J., Soonthornvacharin, S., Akleh, R. E., Olivieri, K. C., et al. (2015). PQBP1 is a proximal sensor of the cGAS-dependent innate response to HIV-1. Cell 161 (6), 1293–1305. doi: 10.1016/j.cell.2015.04.050
Yosef, R., Pilpel, N., Tokarsky-Amiel, R., Biran, A., Ovadya, Y., Cohen, S., et al. (2016). Directed elimination of senescent cells by inhibition of BCL-W and BCL-XL. Nat. Commun. 7, 11190. doi: 10.1038/ncomms11190
Yoshimoto, S., Loo, T. M., Atarashi, K., Kanda, H., Sato, S., Oyadomari, S., et al. (2013). Obesity-induced gut microbial metabolite promotes liver cancer through senescence secretome. Nature 499 (7456), 97–101. doi: 10.1038/nature12347
You, M., Chen, L., Zhang, D., Zhao, P., Chen, Z., Qin, E. Q., et al. (2021). Single-cell epigenomic landscape of peripheral immune cells reveals establishment of trained immunity in individuals convalescing from COVID-19. Nat. Cell Biol. 23 (6), 620–630. doi: 10.1038/s41556-021-00690-1
Yu, Q., Katlinskaya, Y. V., Carbone, C. J., Zhao, B., Katlinski, K. V., Zheng, H., et al. (2015). DNA-Damage-induced type I interferon promotes senescence and inhibits stem cell function. Cell Rep. 11 (5), 785–797. doi: 10.1016/j.celrep.2015.03.069
Zhi, H., Zahoor, M. A., Shudofsky, A. M., Giam, C. Z. (2015). KSHV vCyclin counters the senescence/G1 arrest response triggered by NF-κB hyperactivation. Oncogene 34 (4), 496–505. doi: 10.1038/onc.2013.567
Keywords: senescence, virus, COVID-19, cGAS-STING, NLRP3 inflammasome, senotherapeutics
Citation: Li Z, Tian M, Wang G, Cui X, Ma J, Liu S, Shen B, Liu F, Wu K, Xiao X and Zhu C (2023) Senotherapeutics: An emerging approach to the treatment of viral infectious diseases in the elderly. Front. Cell. Infect. Microbiol. 13:1098712. doi: 10.3389/fcimb.2023.1098712
Received: 15 November 2022; Accepted: 06 March 2023;
Published: 29 March 2023.
Edited by:
You Zhou, Cardiff University, United KingdomReviewed by:
Aftab Alam, University at Buffalo, United StatesLarissa Langhi Prata, Mayo Clinic, United States
Copyright © 2023 Li, Tian, Wang, Cui, Ma, Liu, Shen, Liu, Wu, Xiao and Zhu. This is an open-access article distributed under the terms of the Creative Commons Attribution License (CC BY). The use, distribution or reproduction in other forums is permitted, provided the original author(s) and the copyright owner(s) are credited and that the original publication in this journal is cited, in accordance with accepted academic practice. No use, distribution or reproduction is permitted which does not comply with these terms.
*Correspondence: Chengliang Zhu, emh1Y2hlbmdsaWFuZ0B3aHUuZWR1LmNu; Xuan Xiao, eGlhb3h1YW4xMTExQHdodS5lZHUuY24=
†These authors have contributed equally to this work and share first authorship