- Department of Pathogen Biology, School of Medicine, Nantong University, Nantong, Jiangsu, China
Toxoplasma gondii (T. gondii), a ubiquitous and obligatory intracellular protozoa, not only alters peripheral immune status, but crosses the blood-brain barrier to trigger brain parenchymal injury and central neuroinflammation to establish latent cerebral infection in humans and other vertebrates. Recent findings underscore the strong correlation between alterations in the peripheral and central immune environment and mood disorders. Th17 and Th1 cells are important pro-inflammatory cells that can drive the pathology of mood disorders by promoting neuroinflammation. As opposed to Th17 and Th1, regulatory T cells have inhibitory inflammatory and neuroprotective functions that can ameliorate mood disorders. T. gondii induces neuroinflammation, which can be mediated by CD4+ T cells (such as Tregs, Th17, Th1, and Th2). Though the pathophysiology and treatment of mood disorder have been currently studied, emerging evidence points to unique role of CD4+ T cells in mood disorder, especially those caused by T. gondii infection. In this review, we explore some recent studies that extend our understanding of the relationship between mood disorders and T. gondii.
Introduction
Mood disorders are a group of global common mental diseases, recognized by the World Health Organization as one of the major causes of disability all over the world, seriously affecting health and life expectancy, and often imposing a significant economic burden on society (Moussavi et al., 2007; McCarron et al., 2016), which mainly includes major depression disorder (MDD) and bipolar disorder (BD) (Thase and Denko, 2008; Whiteford et al., 2013). MDD has a lifetime prevalence of 2%-15% and BD has a lifetime prevalence of about 2%, both of which contribute to increased mortality (Grande et al., 2016; Park and Zarate, 2019). Patients with mood disorders suffer from cognitive decline, anxiety and suicidal thoughts with varying degrees of behavioral changes (Rakofsky and Rapaport, 2018). Little is known about the pathophysiology of mood disorders, and relevant mechanistic hypotheses are mainly derived from neuroinflammation, genetics, neurotransmitters, neurotrophic factors, and stress axis. However, there is growing evidence to support the involvement of immunoinflammatory pathways in the mechanisms of mood disorders (Sayana et al., 2017). Mood disorders are closely related to the immune system, and changes in the peripheral and central immune environment have been shown to be involved in the pathophysiological process of mood disorders (Dantzer et al., 2008). Patients with mood disorders have been observed to have inflammatory signals and immune cells transmitted from the periphery to the brain, as well as activated microglia (Miller and Raison, 2016). Studies have found the increased levels of CD4+ T cells in peripheral blood of MDD patients or groups with anxiety and depression symptoms (Beurel et al., 2020). The main subtypes of CD4+ T cells are regulatory T cells (Tregs), T-helper 1 (Th1), T-helper 2 (Th2), and T-helper 17 (Th17). Several experiments have shown that in the peripheral blood of patients with mood disorders, Tregs are reduced (Bauer and Teixeira, 2021), Th17 cells and their marker cytokine interleukin (IL)-17 are increased (Chen et al., 2011; Davami et al., 2016), accompanied by an increase in Th1 cells, Th1/Th2 ratio and Th1/Th2 cytokine levels with pathological imbalance (Huang and Lee, 2007; Beurel et al., 2020). A new study suggests that metabolic disturbance of CD4+ T cells is a key factor in chronic stress anxiety, a high-risk symptom of MDD (Jacobson and Newman, 2017; Fan et al., 2019).
Toxoplasma gondii (T. gondii), an obligate intracellular parasite from the phylum Apicomplexa with a complex, multistage life cycle, infects approximately one-third of the global population (White et al., 2014; Lourido, 2019). Felines are the definitive hosts of T. gondii, and humans possibly acquire infection by eating undercooked meat containing cysts, food or water contaminated with oocysts, and mother-to-child transmission (Halonen and Weiss, 2013). Depending on the host immune function, toxoplasmosis can manifest as asymptomatic infection, encephalitis, chorioretinitis, multi-organ involvement, or genotypic infection (Galli et al., 2019). T. gondii is capable of being present in warm-blooded animal tissues, especially muscle and brain, in the form of cysts, but it can only complete its life cycle by reproducing sexually in the feline body. So to complete its life cycle progression, this parasite evolved to influence host behavior, such as changing from an aversion to cat odor to a preference and increased mobility, making its ingestion by cats more likely. Indeed, T. gondii is neurotropic and may be associated with structural abnormalities of the brain, capable of forming cysts in the brain parenchyma and remaining in the host brain for life, infecting microglia, astrocytes, neurons and stimulating the production of multiple cytokines that affect host behavior and cognitive function (Coryell et al., 2020; Erickson et al., 2021; Gale et al., 2021).
In recent years, there has been an increasing interest in parasites affecting human behavior and mental disorders. The main parasites affecting central nervous system (CNS) include T. gondii, Trypanosoma brucei, Entamoeba histolytica, Plasmodium falciparum, and Schistosoma japonicum (Gondim et al., 2019). These parasites are all capable of establishing infections in the brain and are associated with cognitive and behavioral changes and psychiatric disorders, and although the causal mechanisms between them have not been elucidated, the association is plausible (Table 1). In particular, T. gondii is the most common neurotropic protozoan in humans, and an increasing number of epidemiological studies have highlighted the strong association of T. gondii infection with mood disorders and its involvement in their pathogenesis (Suvisaari et al., 2017; Galli et al., 2019; Yalin Sapmaz et al., 2019; Lin et al., 2020). For instance, T. gondii IgG antibody titers were also positively correlated with anxiety and depression in pregnant women (Groer et al., 2011; Nourollahpour Shiadeh et al., 2016). The prevalence of T. gondii infection detected by immunohistochemistry is around 8.0% (7/87) in the prefrontal cortex and amygdala in suicide victims, and T. gondii infection was highly associated with a history of MDD in suicides (Alvarado-Esquivel et al., 2021).
However, some studies have not found a link between T. gondii and mood disorders (Galli et al., 2019; Gao et al., 2019; Nayeri Chegeni et al., 2019). The contradictory findings may be due to differences in sample size, as samples for such studies are not easy to obtain, geographical differences between samples, life span of infection, differences in T. gondii genotypes around the world, factors such as life circumstances, economic status, life stress, and MDD or BD typing can alter the association between T. gondii infection and mood disorders. Moreover, differences in the type and dose of T. gondii strains used to induce behavioral changes during the construction of animal models, as well as host species and sex, can lead to inconsistent study results (Worth et al., 2014). Although the causal relationship between T. gondii infection and mood disorders is not yet clear, research on them is full of implications, and indeed, studies on the relationship between T. gondii and MDD or BD have been increasing in the last decade.
This article focuses on the positive association between T. gondii and mood disorders and analyzes the possible mechanisms of this association, with the immune system serving as a possible bridge between the two T. gondii infection alters the peripheral and central immune status, with an increase in peripheral CD4+ T cells and their recruitment into the brain parenchyma, accompanied by the development and transfer of inflammation (Ploix et al., 2011; Severance et al., 2016), or directly into the CNS to promote microglia activation (Courret et al., 2006; Matta et al., 2021) (Figure 1). T. gondii is involved in the pathological development of neuropsychiatric disorders by communicating with the CNS through the immune system as a bridge. Interestingly, this mechanism is similar to the microbial “gut-brain axis”, in that gut microbes have been shown to communicate with the brain, including through the immune system, in neuropsychiatric disorders such as anxiety, schizophrenia, Parkinson’s disease and Alzheimer’s disease (AD) (Cryan et al., 2019).
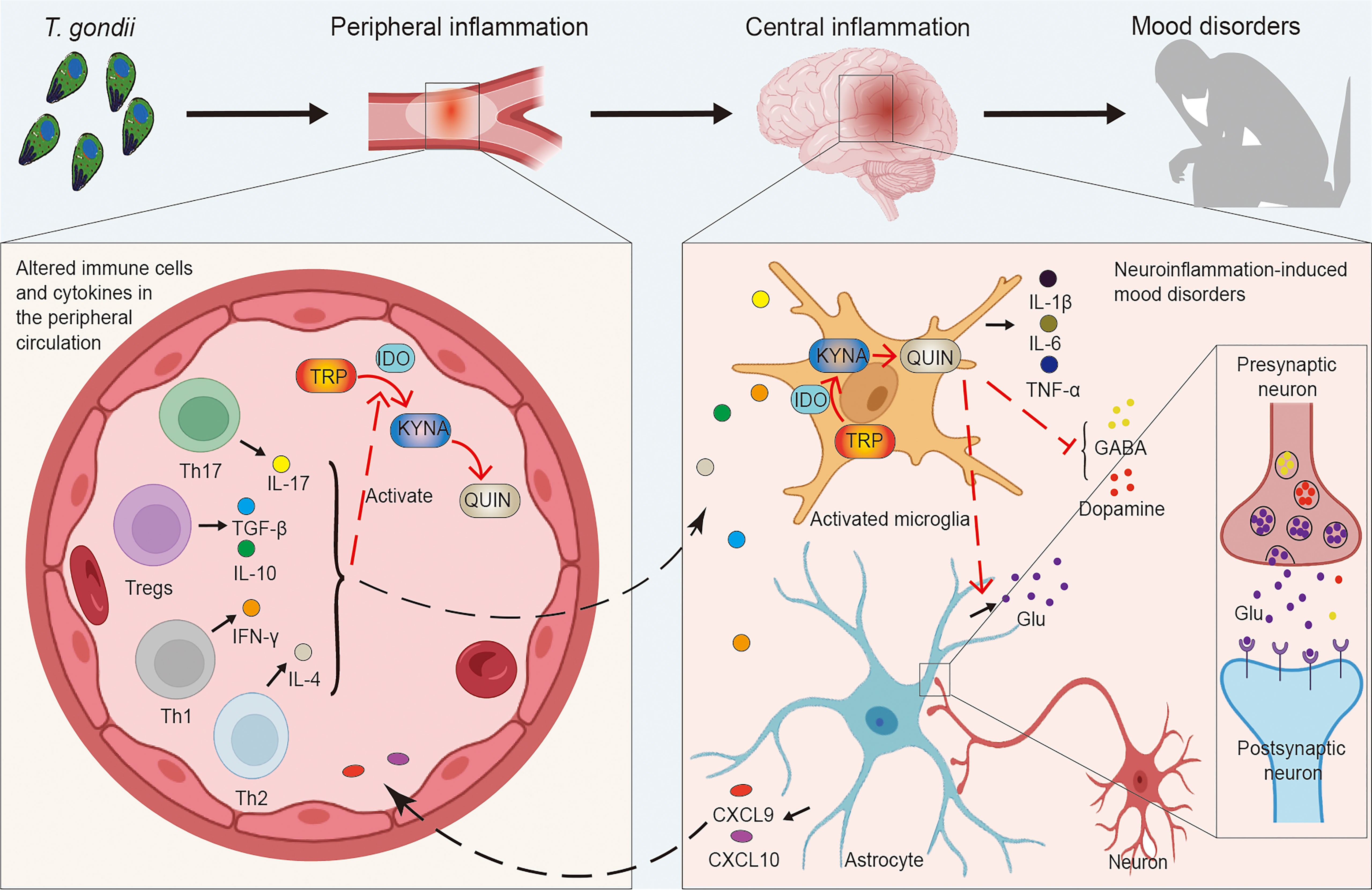
Figure 1 Links between Toxoplasma gondii-induced peripheral and central inflammation and mood disorders. T. gondii infection induces an imbalance in a series of immune cells (e.g. Tregs, Th1, Th2, Th17, etc.) as well as cytokines (e.g. IL-4, IL-10, IL-17, IFN-γ, TGF-β, etc.), causing peripheral inflammation. Inflammatory cytokines activate IDO to promote the decomposition of TRP into KYNA and QUIN, while peripheral inflammation can influence the CNS, which induces central neuroinflammation and affects neurons as well as neural circuits. Microglia are activated to release inflammatory factors (e.g. IL-1β, IL-6, TNF-α, etc.) and promote the TRP pathway. Simultaneously, QUIN activates increased Glu release from astrocytes, leading to the increased levels of intra- and extra-synaptic Glu, while the production and release of neurotransmitter dopamine and GABA are inhibited as well. This complex series of responses induces the development of mood disorders. CNS, central nervous system; GABA, gamma-aminobutyric acid; Glu, glutamate; IDO, indoleamine 2, 3-dioxygenase; IFN, interferon; IL, interleukin; KYNA, kynurenine; QUIN, quinolinic acid; TGF, transforming growth factor; Th, T-helper cells; TNF, tumor necrosis factor; Tregs, regulatory T cells; TRP, tryptophan; T. gondii, Toxoplasma gondii.
T. gondii life cycle and strain types
The life cycle of T. gondii has three main distinct developmental stages: oocysts containing sporozoites, tachyzoites, and tissue cysts containing bradyzoites. Oocysts are the product of sexual reproduction of T. gondii, which occurs only in the epithelial cells of the intestine of the definitive host (felines). In stark contrast to oocysts, tachyzoites and bradyzoites are the other two asexual reproductive stages in the cycle of T. gondii. When intermediate hosts (warm-blooded animals) ingest sporulated oocysts in feline feces, the sporozoites in the oocysts are released in the small intestine of the intermediate hosts and infect the epithelial cells, at which point they transform into tachyzoites, which then form parasitic vesicles and enter a rapid division phase. The tachyzoites are the most destructive pathogenic stage in the life cycle of T. gondii, dividing and attacking all tissues of the host until they are controlled by the host’s immune system. The tachyzoites then divide into bradyzoites, which divide slowly and remain dormant, mainly in the brain, bones and heart and form tissue cysts to evade the host’s immune response, thus causing persistent infection (Blader et al., 2015; Elsheikha et al., 2021). Humans acquires infection mainly via ingesting food or water, which is contaminated with oocysts or tissue cysts of T. gondii. Benefit from the development of the T. gondii-Cre system, which is capable of identifying host-parasite interactions and imaging infected cells, it was found that T. gondii invades parenchymal cells such as astrocytes and neurons after CNS invasion, but it prefers to infect neurons and form cysts in neuronal synapses (Cabral et al., 2016).
The severity of disease caused by T. gondii infection also varies greatly, and this is highly dependent on the type of strain of T. gondii as well as the host. It is generally accepted that there are three main clonal lineages of T. gondii, types I, II and III. This typology is based on a large number of studies of T. gondii genotypes in Europe and North America, but a different lineage from these three types has since been identified in Brazil. Genetic analysis of T. gondii in Asia also identified ToxoDB#9 (Chinese 1), which is distributed in many provinces of China, and is considered to be an important clonal lineage of T. gondii alongside types I-III, which are also common in Asia (Pena et al., 2008; Chaichan et al., 2017). Different strains of T. gondii are not equally virulent, with Type I being very virulent and 100% lethal (LD100) in a single injection of T. gondii in the house mouse (Mus musculus -M.m.- domesticus), Type II being a low virulence strain (LD50>103) and Type III being even less virulent (LD50>105) (Fukumoto et al., 2020). This virulence of different strains is also reflected in humans, for example, human ocular toxoplasmosis is not associated with types II and III, but is associated with type I (Grigg et al., 2001). Intriguingly, the infectivity of T. gondii is species-dependent. T. gondii strains endemic in a given region have extremely low virulence against native mice. For example, types II and III endemic in Europe and North America do not kill the major European and North American mouse subspecies M. m. - domesticus, whereas type I endemic in North and East Asia is extremely virulent against M. m. - domesticus but less virulent against the major local mouse subspecies M. m. castaneus and M. m. musculus. Some animals living in the absence of felines or in higher habitats away from oocysts (e.g. wallabies and new world monkeys) are highly susceptible to T. gondii, whereas humans and some farm animals (e.g. cattle, sheep, pigs) are more resistant to T. gondii (Mukhopadhyay et al., 2020).
Hosts such as humans and mice can sense T. gondii infection through pattern recognition receptors such as toll-like receptors (TLRs). After T. gondii has invaded the organism and is sensed, three major signaling pathways are activated: (i) nuclear factor κB (NF-κB), (ii) mitogen-activated protein kinases (MAPKs), and (iii) IFN regulatory factors (IRFs). Activation of these pathways induces the production of IL-12, tumor necrosis factor (TNF)-α and interferon (IFN) and the recruitment of immune cells such as monocytes, neutrophils and dendritic cells (DC) to the site of infection. IL-12 stimulates the production of IFN-γ by natural killer (NK) cells and T cells (CD4+ and CD8+), which are important mediators of parasite eradication (Dupont et al., 2012; Mukhopadhyay et al., 2020). CD4+ and CD8+ T cells are also activated during the course of infection. It has been reported that IFN-γ-mediated macrophage activation and CD8+ T cell-mediated cytotoxicity act in the acute and chronic phases of T. gondii infection, respectively, and that CD8+ T cells can induce the elimination of T. gondii cysts through perforin-mediated cytotoxicity against chronic infection with T. gondii. The CD4+ T cell population has an important helper function in CD8+ T cells, via increasing numbers and enhancing function of CD8+ T cells. T. gondii infection induces a strong CD4+ T cell response, which is the main source of IFN-γ during infection (Suzuki et al., 2010; Khan et al., 2019). IFN-γ strongly stimulates cellular autonomic immune responses in response to infection, induces cellular expression of GTPase, carbon monoxide synthase and indoleamine 2, 3-dioxygenase (IDO) expression, inhibits T. gondii growth and mediates direct killing (Sasai et al., 2018).
T. gondii marches to the brain
T. gondii can metastasize to a variety of tissues, including the eyes, heart, liver, lungs, lymph nodes and muscles. More importantly, it can enter the brain, invade the CNS and cause encephalitis, establishing a persistent chronic infection in nerve and other brain cells (Elsheikha et al., 2021). After T. gondii infects the host, it can invade CD11b+ monocytes, which then carry T. gondii and spread to distant tissues along with the blood circulation. T. gondii can rely on this “Trojan horse” pathway to invade and colonize the brain parenchyma through the blood-brain barrier (BBB) (Courret et al., 2006). Endothelial cells (ECs) can also act as a bridge for T. gondii to invade the CNS. Free T. gondii in the peripheral circulation and released T. gondii from positive monocytes can invade cerebral vascular ECs, replicate in ECs, and then dissolve ECs to damage the BBB, and finally enter the CNS (Konradt et al., 2016). In parallel, T. gondii can also use the paracellular pathway to enter the brain parenchyma directly through the endothelial cell space of BBB. In conclusion, there are three possible ways that T. gondii invades the CNS: CD11b+ monocyte-dependent “Trojan horse” mechanism; BBB endothelial cell lysis; BBB paracellular pathway (Matta et al., 2021) (Figure 2). Further analysis of the entry of T. gondii into the brain parenchyma revealed that in humans and mice, initial infection with T. gondii in the brain parenchyma was mild or non-existent (Ross et al., 2022). The minimal number of T. gondii in the brain parenchyma compared to initial infections in other organ parenchyma (spleen, liver, lung) facilitates the avoidance of a strong immune response to remove T. gondii, allowing for progressive development and persistent infection in the CNS. In the late stages of infection the brain endothelium becomes infiltrated with leukocytes and there is a marked inflammatory response which promotes the passage of T. gondii through the BBB. In addition, T. gondii can facilitate crossing the BBB through protein tyrosine kinase 2 (PTK2), which regulates cell adhesion signaling, and integrin-dependent enhancement of host cell migration. Of course, the complex mechanisms of how T. gondii enters the CNS have not been extensively studied, but this process must be clarified if the problem of cerebral toxoplasmosis is to be solved.
After reaching the CNS, T. gondii forms cysts mainly in neurons and establishes persistent or latent infection. Significantly, recent studies have found that IFN-γ stimulates neurons and upregulates IFN-γ-responsive related genes and proteins [e.g., GTPase system (IRG)] in vivo and in vitro, killing intracellular T. gondii in neurons via IRG and mediating T. gondii resistance in neurons. The data suggest that IFN-γ reduces neuronal infection in both mouse and human neurons, but this clearance efficiency is strain type dependent, for example, RH strains are IRG resistant and cannot be cleared (Chandrasekaran et al., 2022). This important IFN-γ-dependent mechanism of T. gondii clearance may be the focus of research on cerebral toxoplasmosis. T. gondii can also severely infect microglia, and activated microglia play a central role in neuroinflammation (Gonzalez and Pacheco, 2014; Matta et al., 2021). Microglia are the primary cells of immune defense after pathogens have passed through the BBB, and activated microglia can produce pro-inflammatory cytokines (like IL-6, IL-1, and IFN-γ) (Forrester et al., 2018). In a mouse model of T. gondii infection, 30% of microglia were found to be invaded (da Silva and Langoni, 2009). Brain-resident cells (most likely microglia) can produce IFN-γ and thereby can inhibit T. gondii replication in the brain, while activating astrocyte cells to produce chemokines CXC ligand (CXCL) 9 and CXCL10 to recruit immune cells (such as CD4+ T cells) from the peripheral blood to the CNS (Schluter and Barragan, 2019; Suzuki, 2020). Pharmacological studies have found that arctigenin (AG) and ginsenoside Rh2 (GRh2) can ameliorate host or host offspring MDD caused by T. gondii infection, mainly because AG and GRh2 can inhibit microglia activation and neuroinflammation via the TLR4/NF-κB, TNF receptor 1 (TNFR1)/NF-κB signaling pathways and high mobility group box 1 (HMGB1)/TLR4/NF-κB signaling pathway, respectively (Cheng et al., 2020; Xu et al., 2022).
T. gondii may contribute to the onset and development of psychiatric symptoms by altering brain structure and function, such as cytokine-induced neuroinflammation; abnormalities in brain parenchyma and alterations in neuroplasticity; and imbalances in neurotransmitters such as dopamine and glutamate (Galli et al., 2019). Data from experiments in mice suggest that T. gondii infection can promote inflammation in the brain. In chronically infected mice, distinct areas of inflammation appear in the soft meninges, hippocampus and perihippocampal vessels, and hippocampal abnormalities are associated with many human neurological disorders, including AD, depression and schizophrenia. Both the degree of T. gondii cysts and the level of inflammatory factors have been shown to be highly correlated with behavioral changes in mice (Hermes et al., 2008; Boillat et al., 2020). There is now a consensus that there is an association between T. gondii infection and Psychiatric Disorders. Individuals with chronic T. gondii infection are more likely to develop psychiatric disorders such as bipolar disorder, MDD, anxiety, and schizophrenia (Nolan, 2019; Tyebji et al., 2019). We have described above the mechanisms by which T. gondii enters the brain and the immune changes induced in the brain, which set the stage for a specific mechanism of association between T. gondii infection and psychiatric disorders. The immune activation and formation of an inflammatory environment in the brain caused by T. gondii infection is an important basis for the pathology of behavioral changes and mood disorders, and further avenues of research include whether the negative effects of T. gondii infection on the peripheral and central immune systems make individuals with T. gondii infection more susceptible to mood disorders. This may drive the development of drugs to prevent infection and alleviate and ameliorate psychiatric disorders brought on by T. gondii.
Inflammation and mood disorders
T. gondii invasion of organisms not only induces innate and adaptive immune responses, but also alters the immune state in the brain, where large numbers of immune cells and inflammatory factors accumulate in the CNS to form a pro-inflammatory state, and this inflammatory state in the brain is associated with the pathogenesis of mood disorders. T. gondii infection has been found to cause immune dysregulation, activating peripheral and central inflammatory responses, and induce an imbalance of pro-inflammatory factors (such as IL-6, IFN-γ) and anti-inflammatory factors (such as IL-10) (Del Grande et al., 2017). The CNS inflammatory response can induce the symptoms of mood disorders and participate in the occurrence and development of mood disorders (Martynowicz et al., 2019; Boillat et al., 2020). CD4+ T cells are critical for resistance to T. gondii infection, and toxoplasmosis often leads to alterations in the number and function of CD4+ T cells (Dupont et al., 2012). Studies have demonstrated that T. gondii infection inhibits Tregs proliferation, resulting in a decrease in Tregs levels (Gao et al., 2021). However, T. gondii triggers a strong Th1 cell response to defend against pathogen infection (Sana et al., 2022), while Th2 cells can suppress the Th1 cell response, so the Th1/Th2 balance plays a vital role during T. gondii infection (Xu et al., 2021a). Interestingly, IL-27 inhibits pathological Th17 cell responses during CNS infection by T. gondii, preventing excessive inflammatory responses (Stumhofer et al., 2006). In conclusion, T. gondii infection leads to chronic CNS infection and neuroinflammation (Klein et al., 2017), and even before T. gondii enters the CNS, the peripheral immune system can be activated to produce large numbers of pro-inflammatory cells, leading to neuroinflammation (Tyebji et al., 2019), including altered numbers of CD4+ T cells and secretion of cytokine IFN-γ (Aliberti, 2005; Yarovinsky, 2014).
Studies have shown that in patients with MDD and BD, the level of pro-inflammatory factors in peripheral blood will increase, IFN-γ, IL-6, IL-1, and TNF-α involved (Sayana et al., 2017; Fries et al., 2019). Also in a count of 606 MDD patients, an increase in peripheral blood C-reactive protein (CRP) was found, with approximately 47% of patients having high CRP levels (≥ 3.0 mg/L) and roughly 29% of patients having higher CRP levels (≥ 5.0 mg/L) (Rethorst et al., 2014). Interestingly, the use of inflammatory cytokines can induce depression-like behavior in experimental animals and humans, such as long-term acceptance of IFN-α. Up to 30%-50% of treated patients have MDD symptoms (Haroon et al., 2012). Chronic T. gondii infection can lead to the development of neuroinflammation, which is also thought to be responsible for behavioral abnormalities (Hermes et al., 2008; Boillat et al., 2020). There is growing evidence that mood disorders and inflammation are intertwined and reinforce each other. Mood disorders tend to facilitate inflammatory responses and exhibit characteristics of inflammation, which in turn promotes mood disorders and other neuropsychiatric disorders (Bauer and Teixeira, 2019).
So how does inflammation affect mood disorders? Cytokines, although relatively large protein molecules (15-25 KD), have been shown to enter the brain and drive CNS inflammation. It can directly or indirectly affect CNS function through BBB penetration, automatic transport of saturated transport molecules, activation of cerebrovascular intimal cells, and binding to receptors on peripheral afferent nerve fibers (Miller et al., 2009). Once cytokines enter the brain, they affect neurotransmitters and neural circuits. Cytokines activate IDO and alter tryptophan (TRP) (a major precursor of serotonin) metabolism, thereby reducing serotonin production and increasing kynurenine acid (KYNA) levels to induce MDD. On the other hand, KYNA can be converted to quinolinic acid (QUIN), which induces astrocyte glutamate (GLU) release and inhibits reuptake, resulting in increased intra- and extra-synaptic GLU levels. Higher GLU levels are associated with increased depressive symptoms. In addition, cytokines have been shown to reduce the release of dopamine, which can lead to decreased motivation and anhedonia, the core symptoms of MDD (Miller and Raison, 2016). The effects of cytokines on neural circuits (basal ganglia, anterior cingulate cortex), neurogenesis, and neuroendocrine functions (such as the hypothalamic-pituitary-adrenergic axis) are systematically described, all of which are highly related to the occurrence and development of mood disorders (Haroon et al., 2012).
Like many infections, the body’s immune cells and cytokines and their induced effector mechanisms control T. gondii infection, yet severe infection-induced hyperinflammation can induce the pathology of CNS. There is growing evidence that inflammation can affect the brain, and that the production of inflammatory responses can lead to depressive symptoms and increase the risk of psychiatric disorders such as BD, MDD, and anxiety disorders (Miller, 2020). As a foreign pathogen, T. gondii can induce inflammation in the peripheral and CNS (McGovern et al., 2021; Saraav et al., 2021), which may speculate that the inflammatory response is a hub between T. gondii and mood disorders, and some inflammatory factors may be the protagonists in this link mechanism. Therefore, it is highly likely that T. gondii is involved in the pathogenesis of mood disorders by indirectly affecting peripheral and central immune status and inducing CNS inflammation and this immunological hypothesis may support the pathogenesis of some neuropsychiatric disorders or behavioral abnormalities in which T. gondii is involved. We next focus on CD4+ T cell numbers and function during T. gondii infection and mood disorders.
Tregs and mood disorders
Tregs are a cell population essential for immune regulation, which modulates pathogen-induced innate and adaptive immune responses. And after T. gondii invades the organism, it will cause an immune response, and the number and function of Tregs will also alter (Hall et al., 2012). Tregs were significantly reduced in number and function collapsed in a model of T. gondii oral infection (Oldenhove et al., 2009). When T. gondii infected pregnant humans or mice, it was also accompanied by a decrease in the number of Tregs (Rezende-Oliveira et al., 2020). Significantly, the immunosuppressive effect of Tregs impedes host immune defenses and increases pathogen susceptibility, so a reduction in Tregs numbers and functional collapse may rescue the organism from the pathological hazards caused by infection. In this section we describe the immunosuppressive and regulatory inflammatory functions and neuroprotective effects of Tregs and show that Tregs are often present in altered numbers in patients with mood disorders. We hypothesize that Tregs exert their regulatory and protective functions to alleviate the pathological symptoms of T. gondii infection when it causes neuroinflammation and brain parenchymal damage.
Tregs, accounting for approximately 5%-10% of the total CD4+ T cells (Gao et al., 2021), are crucial to maintain self-tolerance and immune homeostasis. Tregs are known as suppressor T cells, which can regulate the functions of other immune cells like DC, macrophages, CD4+ T cells, and B cells (Hong et al., 2013; Mohr et al., 2018). Researchers generally divide Tregs into two subtypes, natural regulatory T cells (nTregs) and induced regulatory T cells (iTregs), which act synergistically to enhance immune tolerance in peripheral blood (Curotto de Lafaille and Lafaille, 2009; Yang et al., 2020). Tregs utilize multiple immunomodulatory mechanisms to limit inflammatory responses and enhance immunosuppressive functions, including contact-dependent mechanisms, secretion of immunomodulatory factors like transforming growth factor (TGF)-β and IL-10, and perturbation of target cell metabolism (Georgiev et al., 2019). In pregnant women with seropositive anti-T. gondii antibodies, Tregs expressing T-lymphocyte antigen 4 (CTLA-4) increased, while T. gondii infection can also affect the expression of TGF-β and IL-10 (Rezende-Oliveira et al., 2020). Tregs down-regulate CD80 and CD86 on antigen-presenting cells (APCs) via CTLA-4, thereby hindering the activation of other T cells by APCs. Additionally, CTLA-4 stimulates IDO expression in DC subsets and then induces the catabolism of TRP to metabolites such as KYNA, which can exert immunosuppressive effects through cytotoxicity (Mellor and Munn, 2004; Miyara and Sakaguchi, 2007). Additionally, the immunomodulatory role of TGF-β and IL-10 is the central in T. gondii-induced immunopathogenesis (Butler et al., 2013). Adoptive transfer of Tregs extracted from normal pregnant mice into T. gondii-infected pregnant mice was found to improve adverse pregnancy outcomes, which may be related to the upregulation of TGF-β and IL-10 expression by Tregs (Liu et al., 2014). TGF-β has neuroprotective effects and affects memory formation and synaptic plasticity (Qiu et al., 2021), and when T. gondii infection induces neuroinflammation, TGF-β suppresses inflammation and reduces neuronal damage (Cekanaviciute et al., 2014). Tregs are involved in the immunopathology of T. gondii through surface factors (CTLA-4) and cytokines (TGF-β and IL-10), and similarly, Tregs may regulate T. gondii-induced mood disorders through this immune mechanism.
T. gondii infection can induce intense brain inflammation (Batista et al., 2020), and the development of mood disorders has been associated with CNS inflammation. Tregs may indirectly affect mood disorders by modulating immune-inflammatory responses. Adolescents at high risk for mood disorders had fewer Tregs than normal healthy individuals, and were often accompanied by symptoms of high inflammation (Snijders et al., 2016). Combining several studies, it has also been found that MDD patients tended to have increased Th1/Th2 and Th17/Tregs ratios when the number of Tregs in peripheral blood decreased, indicating that the decreased number of Tregs may be related to the immune imbalance in MDD patients (Li et al., 2010; Chen et al., 2011). And by investigating the alterations of cytokines and Tregs during antidepressant treatment in 16 MDD patients, it was found that the number of Tregs increased while the production of anti-inflammatory factors decreased during treatment (Himmerich et al., 2010). In animal studies, increased levels of peripheral blood Tregs were also found in mice treated with the antidepressant Shen-Qi-Jie-Yu-Fang (SJF) or fluoxetine for postpartum depression (Li et al., 2016). When Tregs-deficient and wildtype mice were simultaneously exposed to stress, the former were more prone to anxiety- and depression-like behaviors, accompanied with increased levels of pro-inflammatory factors (Kim et al., 2012). Xu et al.(Xu et al., 2021b) infected female mice with soluble tachyzoite antigens of T. gondii to induce maternal immune activation, and offspring mice developed anxiety-like behaviors. Then, adoptively transferring the pathogen-activated maternal Tregs into the offspring mice, could improve abnormal behavior symptoms of offspring mice.
After T. gondii invades the CNS, it can lead to neuronal apoptosis and cause severe damage to the brain parenchyma (An et al., 2018). Many areas of the brain are involved in regulating emotions, including areas such as the frontal cortex, hippocampus, striatum, and amygdala, and they form neural circuits associated with mood disorders (Nestler et al., 2002), while patients with mood disorders have pathological features such as the reduced survival of neuroblasts and immature neurons, damaged neural circuits, and decreased levels of neurotrophic factors (Eyre and Baune, 2012). In Tregs-deficient mouse models, Tregs was found to stimulate oligodendrocyte differentiation and myelin regeneration in the CNS (Dombrowski et al., 2017). During ischemic brain injury, Tregs will accumulate in large numbers in the mouse brain and promote neurological recovery and inhibit neurotoxic astrocyte proliferation by producing amphiregulin (Ito et al., 2019). Furthermore, in a mouse model in which mice were infected with human immunodeficiency virus-1 (HIV-1), Tregs were found to suppress inflammatory cytokines and reactive oxygen species (ROS) production, improve neurotoxicity and increase neuronal survival, while upregulating the expressions of brain-derived neurotrophic factor (BDNF) and glial cell-derived neurotrophic factor (GDNF) (Liu et al., 2009). Tregs can also exert neuroprotective effects by producing TGF-β and IL-10 (Kipnis et al., 2004; Reynolds et al., 2007), which can activate microglia and increase neuronal survival in the hippocampus (Kipnis et al., 2004), and TGF-β can also increase the potency of neurotrophic factors (Rook et al., 2011). Concurrently, in a survey of women with prenatal depression, it was found that TGF-β could regulate MDD during pregnancy, optimize the development of the amygdala in offspring, and reduce the incidence of MDD in offspring (Qiu et al., 2021).
We mentioned above that Tregs can regulate immune homeostasis and suppress inflammation, and have neuroprotective effects that may improve mood disorders. However, T. gondii infection often leads to a decrease in Tregs levels, which may exacerbate the symptoms of T. gondii -induced mood disorders, suggesting that Tregs and the cytokines TGF-β and IL-10 are important modulators. However, there are no trials that have examined T. gondii infection, Tregs and mood disorders together, which is an important direction that could be of great help in both the treatment of T. gondii infection and the regulation of mood disorders.
Th17 cells and mood disorders
Th17 cells, a subtype of CD4+ T cells (Harrington et al., 2005), are a unique pro-inflammatory lineage of Th cells that were originally identified and named for the production of the pro-inflammatory factor IL-17 (Miossec and Kolls, 2012; Yang et al., 2014). IL-17 is the major functional cytokine with pro-inflammatory effects expressed by Th17, and only Th17 cells among helper T cells enable to produce IL-17 (Gu et al., 2013; Ghosh et al., 2022). Th17 cells produce a series of inflammatory cytokines like IL-22, IL-21, and IL-26 as well (Yang et al., 2014). Th17 cells play dual functions in the process of T. gondii infection. In the early stage of infection, Th17 can resist the invasion and damage of T. gondii, while in the later stage, Th17 can trigger immune pathological changes in the body, resulting in severe inflammatory response (Ma et al., 2020). Clinical studies have found that Th17 cells and the proinflammatory factor IL-17 are increased in toxoplasmosis (Dutra et al., 2013; Silva et al., 2014), and IL-17 will further promote the inflammatory response and expand tissue damage after T. gondii infection (Guiton et al., 2010). IL-17 also plays a central role in the ocular pathology of T. gondii, and it can act as a hallmark inflammatory factor in ocular toxoplasmosis (Greigert et al., 2020). This suggests that Th17 cells are involved in the immune inflammatory response to T. gondii and play an essential role in it.
Th17 cells can promote inflammation, attract macrophages and neutrophils to the site of pathology, and release a series of inflammatory products like IL-17, IL-22, IL-21, and cysteine–cysteine motif chemokine ligand 20 (CCL-20) to help amplify the inflammatory response (Dong, 2008). Th17 cells have been shown to be highly pro-inflammatory and can induce severe autoimmunity (Bettelli et al., 2007). Therefore, since Th17 cells were first identified in 2005, many inflammatory diseases and autoimmune diseases have been found to be related to Th17 cells like multiple sclerosis (MS), psoriasis, systemic lupus erythematosus (SLE), etc. (Tesmer et al., 2008). Notably, in a cohort study of patients suffered from SLE and RA based on a large population, up to 50% of patients with autoimmune diseases exhibited depressive-like symptoms (Pryce and Fontana, 2017). As an essential activator of autoimmunity, is Th17 cells associated with the pathological progression of MDD? We were inspired by a study of 40 MDD patients and 30 healthy control subjects, which measured serum antinuclear antibody (ANA) levels, the number of Th17 cells and Tregs in peripheral blood, serum IL-17 concentration, and RORγt expression levels. The results showed that compared with the control group, Th17 cells in peripheral blood from MDD patients were increased, accompanied by the decreased number of Tregs and increased production of IL-17 and RORγt. The positive rate of ANA as an autoimmune indicator in the MDD patients (around 27.50%) was also higher than that in the healthy individuals (around 5.00%), which indicated that Th17 cells were most likely involved in the pathological mechanism of MDD (Chen et al., 2011).
Th17 cells are vital regulators in the pathological development of mood disorders by affecting neuroinflammation (Slyepchenko et al., 2016; Ghosh et al., 2022). It has been shown that Th17 cells can invade the CNS and induce neuroinflammation, thereby mediating the development of schizophrenia (Debnath and Berk, 2014). Th17 cells can also invade the brain parenchyma, release inflammatory cytokines (IL-17 and IL-22) or induce neuroinflammation and neuronal apoptosis through the Fas/FasL pathway to participate in the etiopathogenesis of AD (Zhang et al., 2013a). In the experimental autoimmune encephalomyelitis (EAE) model, Th17 cells were also found to be involved in nerve damage in MS neuroinflammation (Siffrin et al., 2010). And in a mouse model of learned-helplessness, Beurel et al. (Beurel et al., 2018) detected abundant pro-inflammatory Th17 cells in the prefrontal cortex (PFC) and hippocampus, two brain sites whose functions are associated with MDD. Peripheral Th17 cells may cross the BBB into the brain parenchyma under the action of several cytokines, chemokines, and adhesion factors (Sonar and Lal, 2017), such as cysteine–cysteine motif chemokine ligand 20 (CCL20) and chemokine receptor 6 (CCR6), which can induce and assist this process (Zhang et al., 2013b). Th17 cells can activate microglia in the CNS (Murphy et al., 2010; Slyepchenko et al., 2016). Microglia have been found to express IL-17 receptors, and IL-17 can stimulate microglia to produce inflammatory cytokines (e.g., IL-6) by acting on the IL-17 receptor in microglia (Kawanokuchi et al., 2008). This suggests that Th17 cells may induce neuroinflammation by activating microglia. Activated microglia can release pro-inflammatory cytokines to expand neuroinflammation, produce ROS, eicosanoids mediate neurotoxicity, and thus affect neuroplasticity, all of which have been involved in the etiopathogenesis of MDD (McNally et al., 2008). Th17 cells can promote the production of ROS and act on endothelial cells to induce BBB injury, so as to make peripheral immune cells and cytokines enter the brain parenchyma and promote brain inflammation, or IL-17 can directly play a neurotoxic role and lead to brain injury (Huppert et al., 2010; Waisman et al., 2015).
In truth, although many studies have shown a correlation between Th17 cells and mood disorders, the roles and mechanisms of Th17 cells in mood disorders have not been studied extensively, possibly owing to the comparatively late discovery of Th17 cells. Above we summarized that Th17 cells are involved in immune responses during T. gondii infection as pro-inflammatory cells and that Th17 cells and IL-17 can promote inflammation in the brain parenchyma. We have reason to believe that Th17 cells are involved in the pathogenesis of mood disorders and that this pathogenesis may be highly relevant to T. gondii-induced mood disorders. Therefore, we can next use Th17 cells as an important research target. In addition, the mechanism by which Th17 cells enter the CNS and affect the neuroinflammation of mood disorders remains unclear, and more research is needed to verify. However, there is no doubt that the mechanism of Th17 cells affecting mood disorder via affecting neuroinflammation has broad research prospects.
Th1, Th2 and mood disorders
Th1 cells and Th2 cells are two important Th cell subtypes (Berger, 2000). Th1 cells are associated with cell-mediated inflammation and delayed-type hypersensitivity reactions, mainly secreting cytokines such as IL-2, IFN-γ and TNF. While Th2 is mainly associated with allergic reactions (like asthma) and specific diseases (like MS), it mainly produces IL-10, IL-4 and IL-5 (Raphael et al., 2015). In the immune response, Th1 cells mainly exhibit pro-inflammatory properties, while Th2 cells mainly have anti-inflammatory properties, and the balance of these two cells has a positive effect on maintaining the immune balance of the human body. Mood disorders are associated with immune balance, and some studies have demonstrated Th1 and Th2 cell imbalances in MDD and BD patients (Myint et al., 2005; Brambilla et al., 2014). Significantly, T. gondii infection also involves alterations in Th1 and Th2 cells and cytokines (Xu et al., 2021a). In T. gondii infection, the body produces a strong Th1 cell response to resist pathogen invasion (Jankovic et al., 2010; Cortez et al., 2014), while Th1 cells also express IL-27 and IFN-γ to influence the development and differentiation of Tregs (Jankovic et al., 2007; Hall et al., 2012).
The imbalance of Th1 and Th2 may be involved in the pathophysiology of MDD. In the study of immune imbalance in MDD patients, it was found that the ratio of Th1/Th2 in peripheral blood of patients was increased (Li et al., 2010), and the decrease of Th2 cells also appeared in patients with MDD (Becking et al., 2018). One study examined the relationship between Th1 and Th2 cytokines and MDD, and then found that patients suffered from MDD had substantially higher levels of TNF-α, which is secreted primarily by Th1 (Huang and Lee, 2007). Another recent study similarly found elevated TNF-α in patients with MDD (Alvarez-Mon et al., 2021). A 2020 analysis of peripheral blood cytokines in 5166 MDD patients and 5083 controls also demonstrated significantly reduced IL-4 levels in MDD patients (Osimo et al., 2020). IL-12 can promote Th1 cell responses, favoring the differentiation of Th1 cells. One study detected high level of IL-12 in the peripheral blood of MDD patients, but IL-12 levels decreased after treatment (Kim et al., 2002). Changes in IL-12 levels during this process may be positively correlated with Th1 cells. In addition, elevated Th1 cell levels were also found in common models of MDD in mice (learned helplessness and chronic restraint stress) (Beurel et al., 2013). These studies indicated that MDD may be linked with a Th1/Th2 imbalance that favors Th1 cells, which may be linked to the pro-inflammatory properties of Th1 cells. Interestingly, behavioral abnormalities and hostility have been suggested to be associated with high level of Th1 cytokine-mediated inflammation (Janicki-Deverts et al., 2010). During T. gondii infection, IFN-γ can drive a powerful immune response to inhibit parasite proliferation, and microglia can be activated by it to fight T. gondii infection (Lima and Lodoen, 2019; Mukhopadhyay et al., 2020). A review also described the promotion of MDD by the pro-inflammatory factor IFN-γ, which induces IDO1, activates hypothalamic-pituitary-adrenal (HPA) axis as well as microglia, and drives Th1 cell infiltration into the CNS, thus participating in the pathogenesis of MDD (Inserra et al., 2019). Although there is no clear evidence, it can be speculated that Th1 cells are involved in the neuroinflammation of MDD. In parallel, BD patients also have Th1/Th2 imbalance. The Th1 chemokine receptor CCR5 is downregulated in BD, whereas the typical cytokine IL-4 produced by Th2 is upregulated, suggesting an imbalance between Th1 and Th2 cells (Brambilla et al., 2014). Asthma is often comorbid with BD, and one experiment using an integrin β4 (ITGB4)-deficient mouse model to study the association between airway inflammation and BD-like behavior found that the Th2 inflammatory response promotes BD-like behavior in asthmatics (Han et al., 2018).
So far, T. gondii infection has been shown to induce a Th1 response, but little research has been done on the relevance and mechanisms of Th1 and Th2 cells to mood disorders. Most of the above related studies only reflect the levels of Th1 cells and Th2 cells by detecting cytokines, which has limitations. Some cytokines (IFN-γ, TNF-α, IL-4, etc.) are not specifically expressed by Th1 cells or Th2 cells, and may also be due to alterations in some living habits (such as smoking, drinking, etc.) (Brambilla et al., 2014). Therefore, further studies need to be conducted, and cells like Th1 and Th2 cells as well as some cytokines (e.g. IFN-γ) may become targets for further research on T. gondii-induced mood disorders.
Microglia and mood disorders
Mood disorder belongs to a neuropsychiatric disease in which microglia plays a vital role (Beumer et al., 2012), which is evidenced by the fact that activation of microglia has been found in both MDD and anxiety disorders (Frick et al., 2013). And one article exposes that MDD can be caused by altered microglia function and therefore can be considered as a microglial disorder (Yirmiya et al., 2015). As resident macrophages in the CNS (Kettenmann et al., 2011), microglia is mainly activated by damage-associated molecular patterns (DAMPs) and pathogen-associated molecular patterns (PAMPs) (Spittau et al., 2020). Those activated microglia can be divided into two phenotypes: the pro-inflammatory/injury-inducing M1 phenotype (M1-type microglia) and the anti-inflammatory/neuroprotective M2 phenotype (M2-type microglia) (Tschoe et al., 2020). M1-type microglia can induce hippocampal neuronal apoptosis by producing inflammatory cytokines like IL-6 (Monje et al., 2003), IL-1β (Goshen et al., 2008), and TNF-α (Cacci et al., 2005), inhibit neurogenesis, and produce NO and ROS for neurotoxicity, while the intracellular IDO enzyme is activated to promote the production of KYNA and QUIN by the tryptophan pathway as well (Jia et al., 2021). M2-type microglia have anti-inflammatory effects, promote inflammation regression, repair damage, and release nutritional factors (Zhao et al., 2015). Taken together, these functional changes after microglia activation can affect the occurrence and development of MDD.
Within T. gondii infection, T. gondii can enter the brain parenchyma and form brain cysts, causing dopamine dysregulation. Tachyzoites can infect about 30% of microglia and cause CNS inflammation. In addition, after infection with T. gondii, CD4+ T cells and CD8+ T cells secrete inflammatory factors such as TNF-α and IL-1 to stimulate microglia, and interfere with gamma-aminobutyric acid (GABA) and 5-hydroxytryptamine (5-HT) synthesis via the KYNA pathway (Mukhopadhyay et al., 2020; Yin et al., 2022). This may be the physiological basis as well as the contributing factor for T. gondii-induced mood disorders and behavioral abnormalities.
In MDD, microglia are primarily activated by immune cells or inflammatory cytokines in infectious or non-infectious inflammatory responses (Yirmiya et al., 2015). CD4+ T cells can cross the BBB, infiltrate the CNS, directly or indirectly interact with microglia, and participate in the activation of M1 or M2 microglia (Solleiro-Villavicencio and Rivas-Arancibia, 2018; Das and Chinnathambi, 2019). Among them, Th1 and Th17 cells contribute to M1-type cell transformation and promote neuroinflammation, while Tregs and Th2 induce microglia anti-inflammatory M2 phenotype and neuroprotection (Gonzalez and Pacheco, 2014). M1 polarization is dependent on IFN-γ secreted by Th1 cells and IL-17 expressed by Th17 cells. Similarly, IL-2 and IL-10 produced by Th2 cells and TGF-β and IL-10 produced by Tregs can promote M2 polarization of microglia (Chen et al., 2020; Tschoe et al., 2020). Bias of Th1/Th2 or Th17/Tregs can de-affect the development of MDD by affecting microglial M1/M2 polarization, while the role of inflammation and inflammatory factors runs through it, whether it is possible to try to push microglia to the M2 phenotype, improve neuroinflammation and protect nerves, and then achieve the purpose of treating MDD.
During T. gondii infection of the CNS, microglia can respond to T. gondii infection by responding to and releasing pro-inflammatory factors and recruit T cells into the brain to fight the infection by releasing chemokines CXCL9 and CXCL10. Microglia are also specialized APCs in the CNS, and some microglia (~3%) can express MHC class II molecules to help activate CD4+ T cells to maintain immune defenses (Cowan et al., 2022). On the other hand, the inflammatory response due to microglia activation may lead to neurodegeneration and neurotoxicity, and this immune activation is detrimental. Therefore, during T. gondii infection of CNS, an immune balance between control of the pathogen and immune tolerance should be emphasized, and excessive Toxoplasma virulence, immunodeficiency and overly strong immune responses can compromise this balance and lead to CNS pathology or death. Next, we should further investigate the anti-infective function of microglia and their neuroprotective role in psychiatric disorders such as mood disorders.
Summary
A growing body of data support the idea that T. gondii infection promotes mood disorders. T. gondii is a widespread and complex parasite that infects humans universally and establishes lifelong infection, but causes widely varying clinical symptoms. In immunocompetent individuals, lifelong infection with T. gondii does not cause symptoms, but in immunocompromised individuals such as AIDS patients and fetuses, T. gondii infection inducing a variety of toxoplasmosis diseases greatly increases the risk of death. Therefore, the outcome of the infection depends to a large extent on the immune capacity of the host. In addition, different types of strains cause very different consequences. Compared to type II and type III strains, type I strains tend to be rigidly virulent and can lead to easy death of the host, while type II strains can induce a stronger inflammatory response, causing inflammatory diseases such as encephalitis or ileitis. It is worth noting that most of the past studies on T. gondii-induced behavioral changes used different types of T. gondii strains (e.g., RH, HIF, PRU, etc.), and this may lead to different experimental results due to T. gondii strain variability. Type I strains are too virulent and often lethal to the host, while type II and III strains are relatively less virulent, which may make it easier to establish chronic infections in the host, while facilitating our study of behavioral changes due to chronic infections. Therefore, the choice of strains to study psychiatric diseases in the future is a question worth considering. Moreover, the susceptibility of different hosts to T. gondii varies, with different genetic backgrounds and immune mechanisms involved. Most of the experimental animal models we discussed above are primarily of mouse origin, so we should consider the question: can T. gondii-induced behavioral changes in animals really be analogous to humans? Some simple behavioral changes may be possible, and one article gives us empirical evidence to support this analogy (Flegr, 2013). However, when it comes to psychiatric disorders with more complex symptoms and mechanisms, such as MDD, bipolar disorder, and schizophrenia, we should consider whether the complexity of these disorders can be reflected by animal models. But there is no doubt that animal models will help us to understand all aspects of psychiatric disorders, providing us with novel insights in the epidemiology and pathophysiology of T. gondii-induced mood disorders.
In recent years, research on the association between T. gondii and mood disorders has gradually increased, with a growing number of epidemiological studies highlighting the potential role of T. gondii in the pathogenesis of mood disorders, and above we show the correlation between them. However, this correlation may be bidirectional, as individuals with psychiatric disorders may be more susceptible to exposure and increased susceptibility to neurotropic pathogens due to behavioral abnormalities or reduced immune competence. Infection with neurotropic pathogens such as T. gondii can lead to neuronal damage, central inflammation, neurotransmitter and hormonal changes that can cause and exacerbate behavioral changes or neuropsychiatric disorders. Most current models of T. gondii-induced mood disorders suggest that changes in brain parenchyma and altered immune status lead to cognitive and emotional deficits and manifest as mood disorder symptoms such as behavioral abnormalities. However, it is noteworthy that most of the current studies on the role of T. gondii in mood disorders are associative and do not establish a causal relationship, and the exact mechanism of their influence remains unknown. Here we summarize the association between T. gondii, the immune system and mood disorders, and attempt to build a framework for the “immune-brain axis” of T. gondii. We also show evidence that inflammation is an intermediate pathway between T. gondii and mood disorders, and that T. gondii triggers an inflammatory process that leads to structural and functional damage in the CNS, causing changes in mood, social behavior, and cognitive performance. In this review, we explore the ability of T. gondii to cause alterations in CD4+ T cell numbers and function and the link between several important CD4+ T cells and mood disorders, including Tregs, Th1, Th2, and Th17 cells. These cells and secreted inflammatory factors are involved in immune inflammatory responses and can be recruited to the CNS, activate microglia, and participate in processes such as neuroinflammation and neurotoxicity. We therefore propose a reasonable hypothesis that inflammation has a positive effect on mood disorders and that CD4+ T cells may be involved in the pathogenesis of T. gondii -induced mood disorders by regulating and participating in immune inflammatory mechanisms. The involvement of T. gondii in the pathology of mood disorders through immunoinflammatory mechanisms may be an attractive theoretical framework. Of course, this framework currently lacks experimental studies on the causal relationship between T. gondii and mood disorders, so a lot of attempts need to be invested to defeat this series of diseases.
Furthermore, in future studies we should consider whether different strains of T. gondii induce different types and degrees of psychiatric disorders in humans? Are patients with Psychiatric Disorders more susceptible to T. gondii infection due to changes in their immune system or lifestyle habits? What influences the differences in pathogenicity of T. gondii in different intermediate hosts? Can a clear pathological mechanism be established between T. gondii and mood disorders through immune cells and inflammatory factors, and do CD4+ T cells and associated inflammatory factors represent new and effective therapeutic targets for mood disorders, especially those caused by T. gondii infection? In conclusion, more in-depth studies are needed to explore the mechanisms linking T. gondii and mood disorders., 2
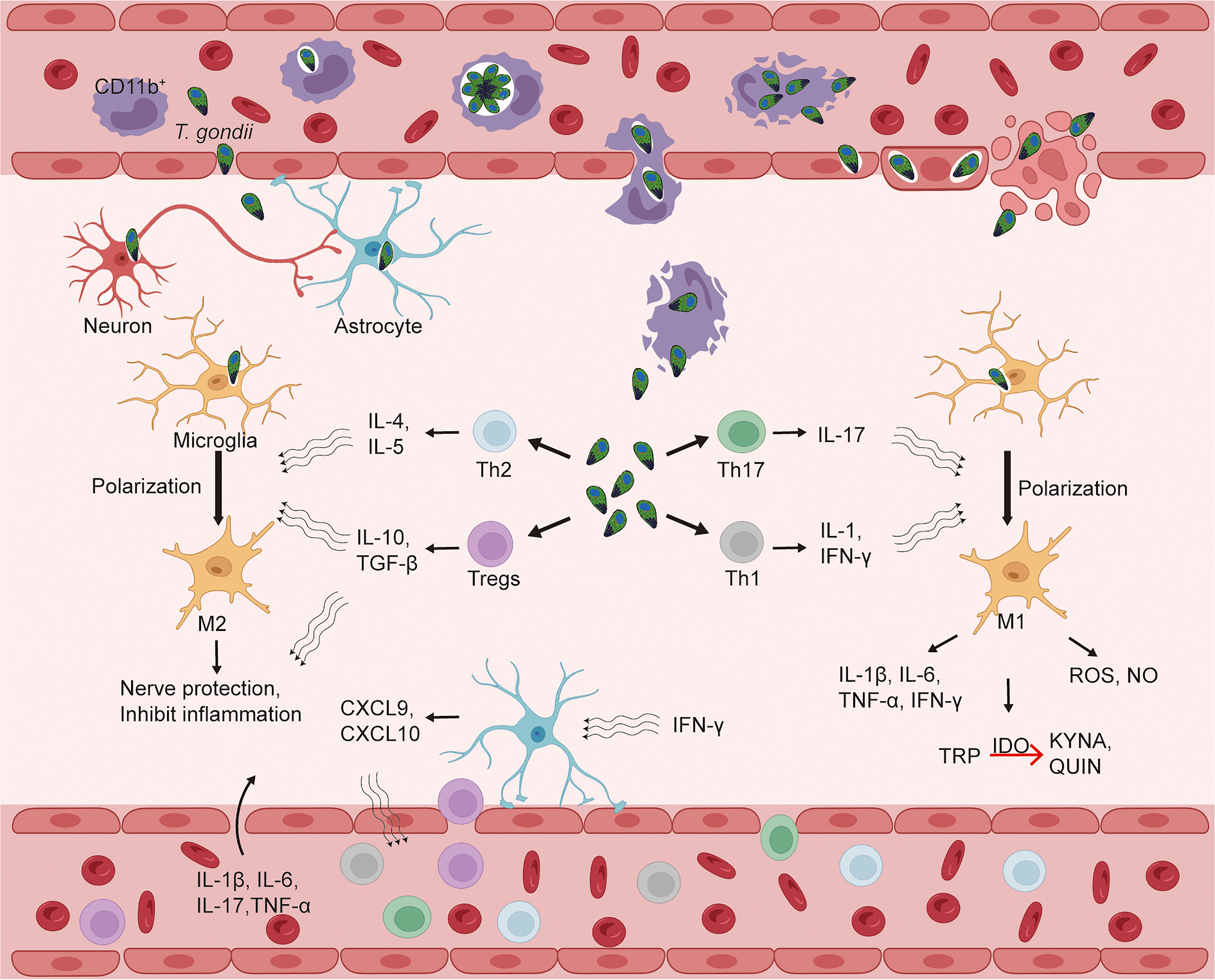
Figure 2 Toxoplasma gondii infects the central nervous system and CD4+ T cells enter the brain parenchyma, causing alterations in the immune status of the brain. The blood-brain barrier (BBB) is an important barrier to protect the central nervous system (CNS) from external aggression. T. gondii can enter the bloodstream after infection and then break through the BBB to the CNS, which may be achieved by three mechanisms: a “Trojan horse” mechanism that relies on CD11b+ monocytes; BBB endothelial cell lysis; and the BBB paracellular pathway. After entering the CNS, T. gondii invades a variety of parenchymal cells, such as microglia, astrocytes, and neurons. T. gondii infection can cause alterations in the levels of CD4+ T cells (Tregs, Th1, Th2, Th17) and some cytokines in peripheral blood, while IFN-γ in CNS can activate astrocytes to produce chemokines (CXCL9, CXCL10) to induce CD4+ T cells to enter CNS via BBB in response to T. gondii infection. In the brain parenchyma, cytokines released by Tregs, Th1, Th2, and Th17 can polarize microglia into M1 or M2 types, which can exert pro-inflammatory/neurotoxic or anti-inflammatory/neuroprotective effects, respectively. CXCL, CXC ligand; IDO, indoleamine 2, 3-dioxygenase; IFN, interferon; IL, interleukin; KYNA, kynurenine; QUIN, quinolinic acid; TGF, transforming growth factor; Th, T-helper cells; Tregs, regulatory T cells; TRP, tryptophan; T. gondii, Toxoplasma gondii.
Author contributions
Conception or Design of the Work: JC; Drafting the Article: QW, YZ, NC, and JC; All authors reviewed the manuscript. All authors contributed to the article and approved the submitted version.
Funding
This work was supported by National Natural Science Foundation of China (81401683); by Science and Technology Innovation and Demonstration Promotion Project of Natural Science Foundation of Nantong City (MS22020001); by Scientific Research Project of Medical School of Nantong University (TDYX2021005); and by Undergraduate Innovation and Entrepreneurship Training Program of Jiangsu Province (202210304124Y).
Conflict of interest
The authors declare that the research was conducted in the absence of any commercial or financial relationships that could be construed as a potential conflict of interest.
Publisher’s note
All claims expressed in this article are solely those of the authors and do not necessarily represent those of their affiliated organizations, or those of the publisher, the editors and the reviewers. Any product that may be evaluated in this article, or claim that may be made by its manufacturer, is not guaranteed or endorsed by the publisher.
References
Adebiyi, O. E., Omobowale, T. O., Abatan, M. O. (2021). Neurocognitive domains and neuropathological changes in experimental infection with Trypanosoma brucei brucei in Wistar rats. Heliyon 7, e08260. doi: 10.1016/j.heliyon.2021.e08260
Akide Ndunge, O. B., Kilian, N., Salman, M. M. (2022). Cerebral malaria and neuronal implications of plasmodium falciparum infection: From mechanisms to advanced models. Adv. Sci. (Weinh). 9, e2202944. doi: 10.1002/advs.202202944
Aliberti, J. (2005). Host persistence: Exploitation of anti-inflammatory pathways by toxoplasma gondii. Nat. Rev. Immunol. 5, 162–170. doi: 10.1038/nri1547
Alvarado-Esquivel, C., Mendoza-Larios, L. A., Garcia-Dolores, F., Sanchez-Anguiano, L. F., Antuna-Salcido, E. I., Hernandez-Tinoco, J., et al. (2021). Association between toxoplasma gondii infection in brain and a history of depression in suicide decedents: A cross-sectional study. Pathogens. 10, 1313. doi: 10.3390/pathogens10101313
Alvarez-Mon, M. A., Gomez-Lahoz, A. M., Orozco, A., Lahera, G., Diaz, D., Ortega, M. A., et al. (2021). Expansion of CD4 T lymphocytes expressing interleukin 17 and tumor necrosis factor in patients with major depressive disorder. J. Pers. Med. 11, 220. doi: 10.3390/jpm11030220
An, R., Tang, Y., Chen, L., Cai, H., Lai, D. H., Liu, K., et al. (2018). Encephalitis is mediated by ROP18 of toxoplasma gondii, a severe pathogen in AIDS patients. Proc. Natl. Acad. Sci. U S A. 115, E5344–E5352. doi: 10.1073/pnas.1801118115
Batista, S. J., Still, K. M., Johanson, D., Thompson, J. A., O'brien, C. A., Lukens, J. R., et al. (2020). Gasdermin-d-dependent IL-1alpha release from microglia promotes protective immunity during chronic toxoplasma gondii infection. Nat. Commun. 11, 3687. doi: 10.1038/s41467-020-17491-z
Bauer, M. E., Teixeira, A. L. (2019). Inflammation in psychiatric disorders: What comes first? Ann. N Y Acad. Sci. 1437, 57–67. doi: 10.1111/nyas.13712
Bauer, M. E., Teixeira, A. L. (2021). Neuroinflammation in mood disorders: Role of regulatory immune cells. Neuroimmunomodulation. 28, 99–107. doi: 10.1159/000515594
Becking, K., Haarman, B. C. M., Grosse, L., Nolen, W. A., Claes, S., Arolt, V., et al. (2018). The circulating levels of CD4+ t helper cells are higher in bipolar disorder as compared to major depressive disorder. J. Neuroimmunol. 319, 28–36. doi: 10.1016/j.jneuroim.2018.03.004
Berger, A. (2000). Th1 and Th2 responses: What are they? Bmj. 321, 424. doi: 10.1136/bmj.321.7258.424
Berger, J. R. (2022). Amebic infections of the central nervous system. J Neurovirol. 28, 467–472. doi: 10.1007/s13365-022-01096-x
Bettelli, E., Oukka, M., Kuchroo, V. K. (2007). T(H)-17 cells in the circle of immunity and autoimmunity. Nat. Immunol. 8, 345–350. doi: 10.1038/ni0407-345
Beumer, W., Gibney, S. M., Drexhage, R. C., Pont-Lezica, L., Doorduin, J., Klein, H. C., et al. (2012). The immune theory of psychiatric diseases: A key role for activated microglia and circulating monocytes. J. Leukoc. Biol. 92, 959–975. doi: 10.1189/jlb.0212100
Beurel, E., Harrington, L. E., Jope, R. S. (2013). Inflammatory T helper 17 cells promote depression-like behavior in mice. Biol. Psychiatry 73, 622–630. doi: 10.1016/j.biopsych.2012.09.021
Beurel, E., Lowell, J. A., Jope, R. S. (2018). Distinct characteristics of hippocampal pathogenic TH17 cells in a mouse model of depression. Brain Behav. Immun. 73, 180–191. doi: 10.1016/j.bbi.2018.04.012
Beurel, E., Toups, M., Nemeroff, C. B. (2020). The bidirectional relationship of depression and inflammation: Double trouble. Neuron. 107, 234–256. doi: 10.1016/j.neuron.2020.06.002
Blader, I. J., Coleman, B. I., Chen, C. T., Gubbels, M. J. (2015). Lytic cycle of toxoplasma gondii: 15 years later. Annu. Rev. Microbiol. 69, 463–485. doi: 10.1146/annurev-micro-091014-104100
Boillat, M., Hammoudi, P. M., Dogga, S. K., Pages, S., Goubran, M., Rodriguez, I., et al. (2020). Neuroinflammation-associated aspecific manipulation of mouse predator fear by toxoplasma gondii. Cell Rep. 30, 320–334 e326. doi: 10.1016/j.celrep.2019.12.019
Brambilla, P., Bellani, M., Isola, M., Bergami, A., Marinelli, V., Dusi, N., et al. (2014). Increased M1/decreased M2 signature and signs of Th1/Th2 shift in chronic patients with bipolar disorder, but not in those with schizophrenia. Transl. Psychiatry 4, e406. doi: 10.1038/tp.2014.46
Butler, N. S., Harris, T. H., Blader, I. J. (2013). Regulation of immunopathogenesis during plasmodium and toxoplasma infections: More parallels than distinctions? Trends Parasitol. 29, 593–602. doi: 10.1016/j.pt.2013.10.002
Cabral, C. M., Tuladhar, S., Dietrich, H. K., Nguyen, E., Macdonald, W. R., Trivedi, T., et al. (2016). Neurons are the primary target cell for the brain-tropic intracellular parasite toxoplasma gondii. PloS Pathog. 12, e1005447. doi: 10.1371/journal.ppat.1005447
Cacci, E., Claasen, J. H., Kokaia, Z. (2005). Microglia-derived tumor necrosis factor-alpha exaggerates death of newborn hippocampal progenitor cells in vitro. J. Neurosci. Res. 80, 789–797. doi: 10.1002/jnr.20531
Cekanaviciute, E., Dietrich, H. K., Axtell, R. C., Williams, A. M., Egusquiza, R., Wai, K. M., et al. (2014). Astrocytic TGF-beta signaling limits inflammation and reduces neuronal damage during central nervous system toxoplasma infection. J. Immunol. 193, 139–149. doi: 10.4049/jimmunol.1303284
Chaichan, P., Mercier, A., Galal, L., Mahittikorn, A., Ariey, F., Morand, S., et al. (2017). Geographical distribution of toxoplasma gondii genotypes in Asia: A link with neighboring continents. Infect. Genet. Evol. 53, 227–238. doi: 10.1016/j.meegid.2017.06.002
Chandrasekaran, S., Kochanowsky, J. A., Merritt, E. F., Lagas, J. S., Swannigan, A., Koshy, A. A. (2022). IFN-gamma stimulated murine and human neurons mount anti-parasitic defenses against the intracellular parasite toxoplasma gondii. Nat. Commun. 13, 4605. doi: 10.1038/s41467-022-32225-z
Chen, Y., Jiang, T., Chen, P., Ouyang, J., Xu, G., Zeng, Z., et al. (2011). Emerging tendency towards autoimmune process in major depressive patients: A novel insight from Th17 cells. Psychiatry Res. 188, 224–230. doi: 10.1016/j.psychres.2010.10.029
Chen, J., Liu, X., Zhong, Y. (2020). Interleukin-17A: The key cytokine in neurodegenerative diseases. Front. Aging Neurosci. 12. doi: 10.3389/fnagi.2020.566922
Cheng, J. H., Xu, X., Li, Y. B., Zhao, X. D., Aosai, F., Shi, S. Y., et al. (2020). Arctigenin ameliorates depression-like behaviors in toxoplasma gondii-infected intermediate hosts via the TLR4/NF-kappaB and TNFR1/NF-kappaB signaling pathways. Int. Immunopharmacol. 82, 106302. doi: 10.1016/j.intimp.2020.106302
Clausen, M. R., Meyer, C. N., Krantz, T., Moser, C., Gomme, G., Kayser, L., et al. (1996). Trichinella infection and clinical disease. Qjm 89, 631–636. doi: 10.1093/qjmed/89.8.631
Ciampi De Andrade, D., Rodrigues, C. L., Abraham, R., Castro, L. H., Livramento, J. A., Machado, L. R., et al. (2010). Cognitive impairment and dementia in neurocysticercosis: A cross-sectional controlled study. Neurology 74, 1288–1295. doi: 10.1212/WNL.0b013e3181d9eda6
Cortez, V. S., Cervantes-Barragan, L., Song, C., Gilfillan, S., Mcdonald, K. G., Tussiwand, R., et al. (2014). CRTAM controls residency of gut CD4+CD8+ T cells in the steady state and maintenance of gut CD4+ Th17 during parasitic infection. J. Exp. Med. 211, 623–633. doi: 10.1084/jem.20130904
Coryell, W., Wilcox, H., Evans, S. J., Pandey, G. N., Jones-Brando, L., Dickerson, F., et al. (2020). Latent infection, inflammatory markers and suicide attempt history in depressive disorders. J. Affect. Disord. 270, 97–101. doi: 10.1016/j.jad.2020.03.057
Cossu, G., Preti, A., Gyppaz, D., Gureje, O., Carta, M.G.. (2022). Association between toxoplasmosis and bipolar disorder: A systematic review and meta-analysis. J Psychiatr Res. 153, 284–291. doi: 10.1016/j.jpsychires.2022.07.013
Courret, N., Darche, S., Sonigo, P., Milon, G., Buzoni-Gatel, D., Tardieux, I. (2006). CD11c- and CD11b-expressing mouse leukocytes transport single toxoplasma gondii tachyzoites to the brain. Blood. 107, 309–316. doi: 10.1182/blood-2005-02-0666
Cowan, M. N., Sethi, I., Harris, T. H. (2022). Microglia in CNS infections: Insights from toxoplasma gondii and other pathogens. Trends Parasitol. 38, 217–229. doi: 10.1016/j.pt.2021.12.004
Cryan, J. F., O'riordan, K. J., Cowan, C. S. M., Sandhu, K. V., Bastiaanssen, T. F. S., Boehme, M., et al. (2019). The microbiota-Gut-Brain axis. Physiol. Rev. 99, 1877–2013. doi: 10.1152/physrev.00018.2018
Curotto de Lafaille, M. A., Lafaille, J. J. (2009). Natural and adaptive foxp3+ regulatory T cells: More of the same or a division of labor? Immunity. 30, 626–635. doi: 10.1016/j.immuni.2009.05.002
Dantzer, R., O'connor, J. C., Freund, G. G., Johnson, R. W., Kelley, K. W. (2008). From inflammation to sickness and depression: When the immune system subjugates the brain. Nat. Rev. Neurosci. 9, 46–56. doi: 10.1038/nrn2297
Darsaud, A., Bourdon, L., Chevrier, C., Keita, M., Bouteille, B., Queyroy, A., et al (2003). Clinical follow-up in the rat experimental model of African trypanosomiasis. Exp Biol Med (Maywood) 228, 1355–1362. doi: 10.1177/153537020322801114
Das, R., Chinnathambi, S. (2019). Microglial priming of antigen presentation and adaptive stimulation in alzheimer's disease. Cell Mol. Life Sci. 76, 3681–3694. doi: 10.1007/s00018-019-03132-2
da Silva, R. C., Langoni, H. (2009). Toxoplasma gondii: Host-parasite interaction and behavior manipulation. Parasitol. Res. 105, 893–898. doi: 10.1007/s00436-009-1526-6
Davami, M. H., Baharlou, R., Ahmadi Vasmehjani, A., Ghanizadeh, A., Keshtkar, M., Dezhkam, I., et al. (2016). Elevated IL-17 and TGF-beta serum levels: A positive correlation between T-helper 17 cell-related pro-inflammatory responses with major depressive disorder. Basic Clin. Neurosci. 7, 137–142. doi: 10.15412/J.BCN.03070207
Debnath, M., Berk, M. (2014). Th17 pathway-mediated immunopathogenesis of schizophrenia: Mechanisms and implications. Schizophr. Bull. 40, 1412–1421. doi: 10.1093/schbul/sbu049
Del Brutto, O. H., Rajshekhar, V., White, A. C., Jr., Tsang, V. C., Nash, T. E., Takayanagui, O. M., et al. (2001). Proposed diagnostic criteria for neurocysticercosis. Neurology 57, 177–183. doi: 10.1212/wnl.57.2.177
Del Grande, C., Galli, L., Schiavi, E., Dell'osso, L., Bruschi, F. (2017). Is toxoplasma gondii a trigger of bipolar disorder? Pathogens. 6, 3. doi: 10.3390/pathogens6010003
De Miranda, A. S., Lacerda-Queiroz, N., De Carvalho Vilela, M., Rodrigues, D. H., Rachid, M. A., Quevedo, J., et al. (2011). Anxiety-like behavior and proinflammatory cytokine levels in the brain of C57BL/6 mice infected with plasmodium berghei (strain ANKA). Neurosci. Lett. 491, 202–206. doi: 10.1016/j.neulet.2011.01.038
Dombrowski, Y., O'hagan, T., Dittmer, M., Penalva, R., Mayoral, S. R., Bankhead, P., et al. (2017). Regulatory T cells promote myelin regeneration in the central nervous system. Nat. Neurosci. 20, 674–680. doi: 10.1038/nn.4528
Dong, C. (2008). TH17 cells in development: An updated view of their molecular identity and genetic programming. Nat. Rev. Immunol. 8, 337–348. doi: 10.1038/nri2295
Dupont, C. D., Christian, D. A., Hunter, C. A. (2012). Immune response and immunopathology during toxoplasmosis. Semin. Immunopathol. 34, 793–813. doi: 10.1007/s00281-012-0339-3
Dutra, M. S., Bela, S. R., Peixoto-Rangel, A. L., Fakiola, M., Cruz, A. G., Gazzinelli, A., et al. (2013). Association of a NOD2 gene polymorphism and T-helper 17 cells with presumed ocular toxoplasmosis. J. Infect. Dis. 207, 152–163. doi: 10.1093/infdis/jis640
Elsheikha, H. M., Marra, C. M., Zhu, X. Q. (2021). Epidemiology, pathophysiology, diagnosis, and management of cerebral toxoplasmosis. Clin. Microbiol. Rev. 34, e00115–19. doi: 10.1128/CMR.00115-19
Erickson, L. D., Brown, B. L., Gale, S. D., Hedges, D. W. (2021). Association between toxoplasma gondii seropositivity and serointensity and brain volume in adults: A cross-sectional study. PloS One 16, e0245994. doi: 10.1371/journal.pone.0245994
Eyre, H., Baune, B. T. (2012). Neuroplastic changes in depression: A role for the immune system. Psychoneuroendocrinology. 37, 1397–1416. doi: 10.1016/j.psyneuen.2012.03.019
Fan, K. Q., Li, Y. Y., Wang, H. L., Mao, X. T., Guo, J. X., Wang, F., et al. (2019). Stress-induced metabolic disorder in peripheral CD4(+) T cells leads to anxiety-like behavior. Cell. 179, 864–879 e819. doi: 10.1016/j.cell.2019.10.001
Flegr, J. (2013). Influence of latent toxoplasma infection on human personality, physiology and morphology: pros and cons of the toxoplasma-human model in studying the manipulation hypothesis. J. Exp. Biol. 216, 127–133. doi: 10.1242/jeb.073635
Forrester, J. V., Mcmenamin, P. G., Dando, S. J. (2018). CNS infection and immune privilege. Nat. Rev. Neurosci. 19, 655–671. doi: 10.1038/s41583-018-0070-8
Frick, L. R., Williams, K., Pittenger, C. (2013). Microglial dysregulation in psychiatric disease. Clin. Dev. Immunol. 2013, 608654. doi: 10.1155/2013/608654
Fries, G. R., Walss-Bass, C., Bauer, M. E., Teixeira, A. L. (2019). Revisiting inflammation in bipolar disorder. Pharmacol. Biochem. Behav. 177, 12–19. doi: 10.1016/j.pbb.2018.12.006
Fukumoto, J., Yamano, A., Matsuzaki, M., Kyan, H., Masatani, T., Matsuo, T., et al. (2020). Molecular and biological analysis revealed genetic diversity and high virulence strain of toxoplasma gondii in Japan. PloS One 15, e0227749. doi: 10.1371/journal.pone.0227749
Gale, S. D., Erickson, L. D., Brown, B. L., Hedges, D. W. (2021). Examining the relationship between toxoplasma gondii and seropositivity and serointensity and depression in adults from the united kingdom and the united states: A cross-sectional study. Pathogens. 10, 1101. doi: 10.3390/pathogens10091101
Galli, L., Del Grande, C., Rindi, L., Mangia, C., Mangano, V., Schiavi, E., et al. (2019). Lack of circulating toxoplasma gondii DNA in seropositive patients with bipolar or schizophrenia spectrum disorders. Psychiatry Res. 273, 706–711. doi: 10.1016/j.psychres.2019.01.104
Garcia-Martinez, C. E., Scatularo, C. E., Farina, J. M., Saldarriaga, C., Perez, G. E., Wyss, F., et al. (2022). Cysticercosis & heart: A systematic review. Curr. Probl Cardiol. 101195. doi: 10.1016/j.cpcardiol.2022.101195
Gao, J. M., He, Z. H., Xie, Y. T., Hide, G., Lai, D. H., Lun, Z. R. (2019). The association between toxoplasma gondii infection and postpartum blues. J. Affect. Disord. 250, 404–409. doi: 10.1016/j.jad.2019.03.013
Gao, X., Zhong, Y., Liu, Y., Ding, R., Chen, J. (2021). The role and function of regulatory T cells in toxoplasma gondii-induced adverse pregnancy outcomes. J. Immunol. Res. 2021, 8782672. doi: 10.1155/2021/8782672
Garcia, H. H., Gonzalez, A. E., Gilman, R. H. (2020). Taenia solium cysticercosis and its impact in neurological disease. Clin. Microbiol. Rev. 33. doi: 10.1128/cmr.00085-19
Georgiev, P., Charbonnier, L. M., Chatila, T. A. (2019). Regulatory T cells: The many faces of Foxp3. J. Clin. Immunol. 39, 623–640. doi: 10.1007/s10875-019-00684-7
Ghosh, R., Mitra, P., Kumar, P., Goyal, T., Sharma, P. (2022). T Helper cells in depression: Central role of Th17 cells. Crit. Rev. Clin. Lab. Sci. 59, 19–39. doi: 10.1080/10408363.2021.1965535
Gondim, B. L. C., Da Silva Catarino, J., De Sousa, M. A. D., De Oliveira Silva, M., Lemes, M. R., De Carvalho-Costa, T. M., et al. (2019). Nanoparticle-mediated drug delivery: Blood-brain barrier as the main obstacle to treating infectious diseases in CNS. Curr. Pharm. Des. 25, 3983–3996. doi: 10.2174/1381612825666191014171354
Gonzalez, H., Pacheco, R. (2014). T-Cell-mediated regulation of neuroinflammation involved in neurodegenerative diseases. J. Neuroinflammation. 11, 201. doi: 10.1186/s12974-014-0201-8
Goshen, I., Kreisel, T., Ben-Menachem-Zidon, O., Licht, T., Weidenfeld, J., Ben-Hur, T., et al. (2008). Brain interleukin-1 mediates chronic stress-induced depression in mice via adrenocortical activation and hippocampal neurogenesis suppression. Mol. Psychiatry 13, 717–728. doi: 10.1038/sj.mp.4002055
Grande, I., Berk, M., Birmaher, B., Vieta, E. (2016). Bipolar disorder. Lancet 387, 1561–1572. doi: 10.1016/s0140-6736(15)00241-x
Greigert, V., Bittich-Fahmi, F., Pfaff, A. W. (2020). Pathophysiology of ocular toxoplasmosis: Facts and open questions. PloS Negl. Trop. Dis. 14, e0008905. doi: 10.1371/journal.pntd.0008905
Grigg, M. E., Ganatra, J., Boothroyd, J. C., Margolis, T. P. (2001). Unusual abundance of atypical strains associated with human ocular toxoplasmosis. J. Infect. Dis. 184, 633–639. doi: 10.1086/322800
Groer, M. W., Yolken, R. H., Xiao, J. C., Beckstead, J. W., Fuchs, D., Mohapatra, S. S., et al. (2011). Prenatal depression and anxiety in toxoplasma gondii-positive women. Am. J. Obstet Gynecol. 204, 433.e431–e437. doi: 10.1016/j.ajog.2011.01.004
Gu, C., Wu, L., Li, X. (2013). IL-17 family: Cytokines, receptors and signaling. Cytokine. 64, 477–485. doi: 10.1016/j.cyto.2013.07.022
Guiton, R., Vasseur, V., Charron, S., Arias, M. T., Van Langendonck, N., Buzoni-Gatel, D., et al. (2010). Interleukin 17 receptor signaling is deleterious during toxoplasma gondii infection in susceptible BL6 mice. J. Infect. Dis. 202, 427–435. doi: 10.1086/653738
Hall, A. O., Beiting, D. P., Tato, C., John, B., Oldenhove, G., Lombana, C. G., et al. (2012). The cytokines interleukin 27 and interferon-gamma promote distinct treg cell populations required to limit infection-induced pathology. Immunity. 37, 511–523. doi: 10.1016/j.immuni.2012.06.014
Halonen, S. K., Weiss, L. M. (2013). Toxoplasmosis. Handb. Clin. Neurol. 114, 125–145. doi: 10.1016/B978-0-444-53490-3.00008-X
Han, L., Wang, L., Tang, S., Yuan, L., Wu, S., Du, X., et al. (2018). ITGB4 deficiency in bronchial epithelial cells directs airway inflammation and bipolar disorder-related behavior. J. Neuroinflammation. 15, 246. doi: 10.1186/s12974-018-1283-5
Haroon, E., Raison, C. L., Miller, A. H. (2012). Psychoneuroimmunology meets neuropsychopharmacology: Translational implications of the impact of inflammation on behavior. Neuropsychopharmacology. 37, 137–162. doi: 10.1038/npp.2011.205
Harrington, L. E., Hatton, R. D., Mangan, P. R., Turner, H., Murphy, T. L., Murphy, K. M., et al. (2005). Interleukin 17-producing CD4+ effector T cells develop via a lineage distinct from the T helper type 1 and 2 lineages. Nat. Immunol. 6, 1123–1132. doi: 10.1038/ni1254
Hermes, G., Ajioka, J. W., Kelly, K. A., Mui, E., Roberts, F., Kasza, K., et al. (2008). Neurological and behavioral abnormalities, ventricular dilatation, altered cellular functions, inflammation, and neuronal injury in brains of mice due to common, persistent, parasitic infection. J. Neuroinflammation. 5, 48. doi: 10.1186/1742-2094-5-48
Himmerich, H., Milenovic, S., Fulda, S., Plumakers, B., Sheldrick, A. J., Michel, T. M., et al. (2010). Regulatory T cells increased while IL-1beta decreased during antidepressant therapy. J. Psychiatr. Res. 44, 1052–1057. doi: 10.1016/j.jpsychires.2010.03.005
Hong, M., Zheng, J., Ding, Z. Y., Chen, J. H., Yu, L., Niu, Y., et al. (2013). Imbalance between Th17 and treg cells may play an important role in the development of chronic unpredictable mild stress-induced depression in mice. Neuroimmunomodulation. 20, 39–50. doi: 10.1159/000343100
Huang, T. L., Lee, C. T. (2007). T-Helper 1/T-helper 2 cytokine imbalance and clinical phenotypes of acute-phase major depression. Psychiatry Clin. Neurosci. 61, 415–420. doi: 10.1111/j.1440-1819.2007.01686.x
Huppert, J., Closhen, D., Croxford, A., White, R., Kulig, P., Pietrowski, E., et al. (2010). Cellular mechanisms of IL-17-induced blood-brain barrier disruption. FASEB J. 24, 1023–1034. doi: 10.1096/fj.09-141978
Inserra, A., Mastronardi, C. A., Rogers, G., Licinio, J., Wong, M. L. (2019). Neuroimmunomodulation in major depressive disorder: Focus on caspase 1, inducible nitric oxide synthase, and interferon-gamma. Mol. Neurobiol. 56, 4288–4305. doi: 10.1007/s12035-018-1359-3
Ito, M., Komai, K., Mise-Omata, S., Iizuka-Koga, M., Noguchi, Y., Kondo, T., et al. (2019). Brain regulatory T cells suppress astrogliosis and potentiate neurological recovery. Nature. 565, 246–250. doi: 10.1038/s41586-018-0824-5
Jacobson, N. C., Newman, M. G. (2017). Anxiety and depression as bidirectional risk factors for one another: A meta-analysis of longitudinal studies. Psychol. Bull. 143, 1155–1200. doi: 10.1037/bul0000111
Janicki-Deverts, D., Cohen, S., Doyle, W. J. (2010). Cynical hostility and stimulated Th1 and Th2 cytokine production. Brain Behav. Immun. 24, 58–63. doi: 10.1016/j.bbi.2009.07.009
Jankovic, D., Kugler, D. G., Sher, A. (2010). IL-10 production by CD4+ effector T cells: A mechanism for self-regulation. Mucosal Immunol. 3, 239–246. doi: 10.1038/mi.2010.8
Jankovic, D., Kullberg, M. C., Feng, C. G., Goldszmid, R. S., Collazo, C. M., Wilson, M., et al. (2007). Conventional T-bet(+)Foxp3(-) Th1 cells are the major source of host-protective regulatory IL-10 during intracellular protozoan infection. J. Exp. Med. 204, 273–283. doi: 10.1084/jem.20062175
Jia, T. W., Zhou, X. N., Wang, X. H., Utzinger, J., Steinmann, P., Wu, X. H. (2007). Assessment of the age-specific disability weight of chronic schistosomiasis japonica. Bull. World Health Organ. 85, 458–465. doi: 10.2471/blt.06.033035
Jia, T. W., Utzinger, J., Deng, Y., Yang, K., Li, Y. Y., Zhu, J. H., et al. (2011). Quantifying quality of life and disability of patients with advanced schistosomiasis japonica. PloS Negl. Trop. Dis. 5, e966. doi: 10.1371/journal.pntd.0000966
Jia, X., Gao, Z., Hu, H. (2021). Microglia in depression: Current perspectives. Sci. China Life Sci. 64, 911–925. doi: 10.1007/s11427-020-1815-6
Kawanokuchi, J., Shimizu, K., Nitta, A., Yamada, K., Mizuno, T., Takeuchi, H., et al. (2008). Production and functions of IL-17 in microglia. J. Neuroimmunol. 194, 54–61. doi: 10.1016/j.jneuroim.2007.11.006
Kettenmann, H., Hanisch, U. K., Noda, M., Verkhratsky, A. (2011). Physiology of microglia. Physiol. Rev. 91, 461–553. doi: 10.1152/physrev.00011.2010
Khan, I. A., Hwang, S., Moretto, M. (2019). Toxoplasma gondii: CD8 T cells cry for CD4 help. Front. Cell Infect. Microbiol. 9. doi: 10.3389/fcimb.2019.00136
Kim, S. J., Lee, H., Lee, G., Oh, S. J., Shin, M. K., Shim, I., et al. (2012). CD4+CD25+ regulatory T cell depletion modulates anxiety and depression-like behaviors in mice. PloS One 7, e42054. doi: 10.1371/journal.pone.0042054
Kim, Y. K., Suh, I. B., Kim, H., Han, C. S., Lim, C. S., Choi, S. H., et al. (2002). The plasma levels of interleukin-12 in schizophrenia, major depression, and bipolar mania: Effects of psychotropic drugs. Mol. Psychiatry 7, 1107–1114. doi: 10.1038/sj.mp.4001084
Kipnis, J., Avidan, H., Caspi, R. R., Schwartz, M. (2004). Dual effect of CD4+CD25+ regulatory T cells in neurodegeneration: A dialogue with microglia. Proc. Natl. Acad. Sci. U S A. 101 (Suppl 2), 14663–14669. doi: 10.1073/pnas.0404842101
Klein, R. S., Garber, C., Howard, N. (2017). Infectious immunity in the central nervous system and brain function. Nat. Immunol. 18, 132–141. doi: 10.1038/ni.3656
Konradt, C., Ueno, N., Christian, D. A., Delong, J. H., Pritchard, G. H., Herz, J., et al. (2016). Endothelial cells are a replicative niche for entry of toxoplasma gondii to the central nervous system. Nat. Microbiol. 1, 16001. doi: 10.1038/nmicrobiol.2016.1
Li, Y., Xiao, B., Qiu, W., Yang, L., Hu, B., Tian, X., et al. (2010). Altered expression of CD4(+)CD25(+) regulatory T cells and its 5-HT(1a) receptor in patients with major depression disorder. J. Affect. Disord. 124, 68–75. doi: 10.1016/j.jad.2009.10.018
Li, J., Zhao, R., Li, X., Sun, W., Qu, M., Tang, Q., et al. (2016). Shen-Qi-Jie-Yu-Fang exerts effects on a rat model of postpartum depression by regulating inflammatory cytokines and CD4(+)CD25(+) regulatory T cells. Neuropsychiatr. Dis. Treat. 12, 883–896. doi: 10.2147/NDT.S98131
Lima, T. S., Lodoen, M. B. (2019). Mechanisms of human innate immune evasion by toxoplasma gondii. Front. Cell. Infection Microbiol. 9. doi: 10.3389/fcimb.2019.00103
Lin, H. A., Chien, W. C., Huang, K. Y., Chung, C. H., Chen, L. C., Lin, H. C., et al. (2020). Infection with toxoplasma gondii increases the risk of psychiatric disorders in Taiwan: A nationwide population-based cohort study. Parasitology. 147, 1577–1586. doi: 10.1017/S0031182020001183
Liu, J., Gong, N., Huang, X., Reynolds, A. D., Mosley, R. L., Gendelman, H. E. (2009). Neuromodulatory activities of CD4+CD25+ regulatory T cells in a murine model of HIV-1-associated neurodegeneration. J. Immunol. 182, 3855–3865. doi: 10.4049/jimmunol.0803330
Liu, Y., Zhao, M., Xu, X., Liu, X., Zhang, H., Jiang, Y., et al. (2014). Adoptive transfer of treg cells counters adverse effects of toxoplasma gondii infection on pregnancy. J. Infect. Dis. 210, 1435–1443. doi: 10.1093/infdis/jiu265
Ma, Z., Yan, K., Jiang, R., Guan, J., Yang, L., Huang, Y., et al. (2020). A novel wx2 gene of toxoplasma gondii inhibits the parasitic invasion and proliferation in vitro and attenuates virulence in vivo via immune response modulation. Front. Microbiol. 11. doi: 10.3389/fmicb.2020.00399
Martynowicz, J., Augusto, L., Wek, R. C., Boehm, S. L., 2nd, Sullivan, W. J., Jr (2019). Guanabenz reverses a key behavioral change caused by latent toxoplasmosis in mice by reducing neuroinflammation. mBio. 10, e00381–19. doi: 10.1128/mBio.00381-19
Matta, S. K., Rinkenberger, N., Dunay, I. R., Sibley, L. D. (2021). Toxoplasma gondii infection and its implications within the central nervous system. Nat. Rev. Microbiol. 19, 467–480. doi: 10.1038/s41579-021-00518-7
McCarron, R. M., Vanderlip, E. R., Rado, J. (2016). Depression. Ann. Intern. Med. 165, ITC49–ITC64. doi: 10.7326/AITC201610040
McGovern, K. E., Nance, J. P., David, C. N., Harrison, R. E. S., Noor, S., Worth, D., et al. (2021). SPARC Coordinates extracellular matrix remodeling and efficient recruitment to and migration of antigen-specific T cells in the brain following infection. Sci. Rep. 11, 4549. doi: 10.1038/s41598-021-83952-0
McNally, L., Bhagwagar, Z., Hannestad, J. (2008). Inflammation, glutamate, and glia in depression: A literature review. CNS Spectr. 13, 501–510. doi: 10.1017/s1092852900016734
Mellor, A. L., Munn, D. H. (2004). IDO expression by dendritic cells: Tolerance and tryptophan catabolism. Nat. Rev. Immunol. 4, 762–774. doi: 10.1038/nri1457
Miller, A. H. (2020). Beyond depression: The expanding role of inflammation in psychiatric disorders. World Psychiatry 19, 108–109. doi: 10.1002/wps.20723
Miller, A. H., Maletic, V., Raison, C. L. (2009). Inflammation and its discontents: The role of cytokines in the pathophysiology of major depression. Biol. Psychiatry 65, 732–741. doi: 10.1016/j.biopsych.2008.11.029
Miller, A. H., Raison, C. L. (2016). The role of inflammation in depression: From evolutionary imperative to modern treatment target. Nat. Rev. Immunol. 16, 22–34. doi: 10.1038/nri.2015.5
Miossec, P., Kolls, J. K. (2012). Targeting IL-17 and TH17 cells in chronic inflammation. Nat. Rev. Drug Discovery 11, 763–776. doi: 10.1038/nrd3794
Miyara, M., Sakaguchi, S. (2007). Natural regulatory T cells: Mechanisms of suppression. Trends Mol. Med. 13, 108–116. doi: 10.1016/j.molmed.2007.01.003
Mohr, A., Malhotra, R., Mayer, G., Gorochov, G., Miyara, M. (2018). Human FOXP3(+) T regulatory cell heterogeneity. Clin. Transl. Immunol. 7, e1005. doi: 10.1002/cti2.1005
Monje, M. L., Toda, H., Palmer, T. D. (2003). Inflammatory blockade restores adult hippocampal neurogenesis. Science. 302, 1760–1765. doi: 10.1126/science.1088417
Moussavi, S., Chatterji, S., Verdes, E., Tandon, A., Patel, V., Ustun, B. (2007). Depression, chronic diseases, and decrements in health: Results from the world health surveys. Lancet 370, 851–858. doi: 10.1016/s0140-6736(07)61415-9
Moxon, C. A., Gibbins, M. P., Mcguinness, D., Milner, D. A., Jr., Marti, M. (2020). New insights into malaria pathogenesis. Annu. Rev. Pathol. 15, 315–343. doi: 10.1146/annurev-pathmechdis-012419-032640
Mukhopadhyay, D., Arranz-Solís, D., Saeij, J. P. J. (2020). Influence of the host and parasite strain on the immune response during toxoplasma infection. Front. Cell. Infection Microbiol. 10. doi: 10.3389/fcimb.2020.580425
Murphy, A. C., Lalor, S. J., Lynch, M. A., Mills, K. H. (2010). Infiltration of Th1 and Th17 cells and activation of microglia in the CNS during the course of experimental autoimmune encephalomyelitis. Brain Behav. Immun. 24, 641–651. doi: 10.1016/j.bbi.2010.01.014
Myint, A. M., Leonard, B. E., Steinbusch, H. W., Kim, Y. K. (2005). Th1, Th2, and Th3 cytokine alterations in major depression. J. Affect. Disord. 88, 167–173. doi: 10.1016/j.jad.2005.07.008
Nayeri Chegeni, T., Sharif, M., Sarvi, S., Moosazadeh, M., Montazeri, M., Aghayan, S. A., et al. (2019). Is there any association between toxoplasma gondii infection and depression? a systematic review and meta-analysis. PloS One 14, e0218524. doi: 10.1371/journal.pone.0218524
Nestler, E. J., Barrot, M., Dileone, R. J., Eisch, A. J., Gold, S. J., Monteggia, L. M. (2002). Neurobiology of depression. Neuron. 34, 13–25. doi: 10.1016/s0896-6273(02)00653-0
Newton, C. R. (2005). Interaction between plasmodium falciparum and human immunodeficiency virus type 1 on the central nervous system of african children. J. Neurovirol. 11 Suppl 3, 45–51. doi: 10.1080/13550280500511881
Nolan, Y. M. (2019). Toxoplasma gondii: An unwelcome visitor that damages social and neuronal connections. Brain Behav. Immun. 80, 4–5. doi: 10.1016/j.bbi.2019.05.046
Nourollahpour Shiadeh, M., Rostami, A., Pearce, B. D., Gholipourmalekabadi, M., Newport, D. J., Danesh, M., et al. (2016). The correlation between toxoplasma gondii infection and prenatal depression in pregnant women. Eur. J. Clin. Microbiol. Infect. Dis. 35, 1829–1835. doi: 10.1007/s10096-016-2734-5
Oldenhove, G., Bouladoux, N., Wohlfert, E. A., Hall, J. A., Chou, D., Dos Santos, L., et al. (2009). Decrease of Foxp3+ treg cell number and acquisition of effector cell phenotype during lethal infection. Immunity. 31, 772–786. doi: 10.1016/j.immuni.2009.10.001
Ong, T. Y. Y., Khan, N. A., Siddiqui, R. (2017). Brain-eating amoebae: Predilection sites in the brain and disease outcome. J. Clin. Microbiol. 55, 1989–1997. doi: 10.1128/jcm.02300-16
Osimo, E. F., Pillinger, T., Rodriguez, I. M., Khandaker, G. M., Pariante, C. M., Howes, O. D. (2020). Inflammatory markers in depression: A meta-analysis of mean differences and variability in 5,166 patients and 5,083 controls. Brain Behav. Immun. 87, 901–909. doi: 10.1016/j.bbi.2020.02.010
Park, L. T., Zarate, C. A., Jr. (2019). Depression in the primary care setting. N Engl. J. Med. 380, 559–568. doi: 10.1056/NEJMcp1712493
Pena, H. F., Gennari, S. M., Dubey, J. P., Su, C. (2008). Population structure and mouse-virulence of toxoplasma gondii in Brazil. Int. J. Parasitol. 38, 561–569. doi: 10.1016/j.ijpara.2007.09.004
Ploix, C. C., Noor, S., Crane, J., Masek, K., Carter, W., Lo, D. D., et al. (2011). CNS-derived CCL21 is both sufficient to drive homeostatic CD4+ T cell proliferation and necessary for efficient CD4+ T cell migration into the CNS parenchyma following toxoplasma gondii infection. Brain Behav. Immun. 25, 883–896. doi: 10.1016/j.bbi.2010.09.014
Postolache, T. T., Wadhawan, A., Rujescu, D., Hoisington, A. J., Dagdag, A., Baca-Garcia, E., et al. (2021). Toxoplasma gondii, suicidal behavior, and intermediate phenotypes for suicidal behavior. Front. Psychiatry 12. doi: 10.3389/fpsyt.2021.665682
Pryce, C. R., Fontana, A. (2017). Depression in autoimmune diseases. Curr. Top. Behav. Neurosci. 31, 139–154. doi: 10.1007/7854_2016_7
Qiu, A., Zhang, H., Wang, C., Chong, Y. S., Shek, L. P., Gluckman, P. D., et al. (2021). Canonical TGF-beta signaling regulates the relationship between prenatal maternal depression and amygdala development in early life. Transl. Psychiatry 11, 170. doi: 10.1038/s41398-021-01292-z
Rakofsky, J., Rapaport, M. (2018). Mood disorders. Continuum 24, 804–827. doi: 10.1212/con.0000000000000604
Raphael, I., Nalawade, S., Eagar, T. N., Forsthuber, T. G. (2015). T Cell subsets and their signature cytokines in autoimmune and inflammatory diseases. Cytokine. 74, 5–17. doi: 10.1016/j.cyto.2014.09.011
Rethorst, C. D., Bernstein, I., Trivedi, M. H. (2014). Inflammation, obesity, and metabolic syndrome in depression: Analysis of the 2009-2010 national health and nutrition examination survey (NHANES). J. Clin. Psychiatry 75, e1428–e1432. doi: 10.4088/JCP.14m09009
Reynolds, A. D., Banerjee, R., Liu, J., Gendelman, H. E., Mosley, R. L. (2007). Neuroprotective activities of CD4+CD25+ regulatory T cells in an animal model of parkinson's disease. J. Leukoc. Biol. 82, 1083–1094. doi: 10.1189/jlb.0507296
Rezende-Oliveira, K., Gomez-Hernandez, C., Da Silva, M. V., Helmo, F. R., Rodrigues, V. (2020). Analysis of regulatory T cells and CTLA-4 expression in pregnant women according to seropositivity to toxoplasma gondii. Parasitology. 147, 810–815. doi: 10.1017/S0031182020000475
Robert-Gangneux, F., Darde, M. L. (2012). Epidemiology of and diagnostic strategies for toxoplasmosis. Clin. Microbiol. Rev. 25, 264–296. doi: 10.1128/CMR.05013-11
Rook, G. A., Lowry, C. A., Raison, C. L. (2011). Lymphocytes in neuroprotection, cognition and emotion: Is intolerance really the answer? Brain Behav. Immun. 25, 591–601. doi: 10.1016/j.bbi.2010.12.005
Rosca, E. C., Tudor, R., Cornea, A., Simu, M. (2021). Central nervous system involvement in trichinellosis: A systematic review. Diagnostics (Basel). 11. doi: 10.3390/diagnostics11060945
Ross, A. G., Mcmanus, D. P., Farrar, J., Hunstman, R. J., Gray, D. J., Li, Y. S. (2012). Neuroschistosomiasis. J. Neurol. 259, 22–32. doi: 10.1007/s00415-011-6133-7
Ross, E. C., Olivera, G. C., Barragan, A. (2022). Early passage of toxoplasma gondii across the blood-brain barrier. Trends Parasitol. 38, 450–461. doi: 10.1016/j.pt.2022.02.003
Sana, M., Rashid, M., Rashid, I., Akbar, H., Gomez-Marin, J. E., Dimier-Poisson, I. (2022). Immune response against toxoplasmosis-some recent updates RH: Toxoplasma gondii immune response. Int. J. Immunopathol. Pharmacol. 36, 3946320221078436. doi: 10.1177/03946320221078436
Saraav, I., Cervantes-Barragan, L., Olias, P., Fu, Y., Wang, Q., Wang, L., et al. (2021). Chronic toxoplasma gondii infection enhances susceptibility to colitis. Proc. Natl. Acad. Sci. U S A. 118, e2106730118. doi: 10.1073/pnas.2106730118
Saric, J., Li, J. V., Swann, J. R., Utzinger, J., Calvert, G., Nicholson, J. K., et al. (2010). Integrated cytokine and metabolic analysis of pathological responses to parasite exposure in rodents. J. Proteome Res. 9, 2255–2264. doi: 10.1021/pr901019z
Sasai, M., Pradipta, A., Yamamoto, M. (2018). Host immune responses to toxoplasma gondii. Int. Immunol. 30, 113–119. doi: 10.1093/intimm/dxy004
Sayana, P., Colpo, G. D., Simoes, L. R., Giridharan, V. V., Teixeira, A. L., Quevedo, J., et al. (2017). A systematic review of evidence for the role of inflammatory biomarkers in bipolar patients. J. Psychiatr. Res. 92, 160–182. doi: 10.1016/j.jpsychires.2017.03.018
Schluter, D., Barragan, A. (2019). Advances and challenges in understanding cerebral toxoplasmosis. Front. Immunol. 10. doi: 10.3389/fimmu.2019.00242
Severance, E. G., Xiao, J., Jones-Brando, L., Sabunciyan, S., Li, Y., Pletnikov, M., et al. (2016). Toxoplasma gondii-a gastrointestinal pathogen associated with human brain diseases. Int. Rev. Neurobiol. 131, 143–163. doi: 10.1016/bs.irn.2016.08.008
Siffrin, V., Radbruch, H., Glumm, R., Niesner, R., Paterka, M., Herz, J., et al. (2010). In vivo imaging of partially reversible th17 cell-induced neuronal dysfunction in the course of encephalomyelitis. Immunity. 33, 424–436. doi: 10.1016/j.immuni.2010.08.018
Silva, J. L., Rezende-Oliveira, K., Da Silva, M. V., Gomez-Hernandez, C., Peghini, B. C., Silva, N. M., et al. (2014). IL-17-expressing CD4(+) and CD8(+) T lymphocytes in human toxoplasmosis. Mediators Inflamm. 2014, 573825. doi: 10.1155/2014/573825
Slyepchenko, A., Maes, M., Kohler, C. A., Anderson, G., Quevedo, J., Alves, G. S., et al. (2016). T Helper 17 cells may drive neuroprogression in major depressive disorder: Proposal of an integrative model. Neurosci. Biobehav. Rev. 64, 83–100. doi: 10.1016/j.neubiorev.2016.02.002
Snijders, G., Schiweck, C., Mesman, E., Grosse, L., De Wit, H., Nolen, W. A., et al. (2016). A dynamic course of T cell defects in individuals at risk for mood disorders. Brain Behav. Immun. 58, 11–17. doi: 10.1016/j.bbi.2016.05.007
Solleiro-Villavicencio, H., Rivas-Arancibia, S. (2018). Effect of chronic oxidative stress on neuroinflammatory response mediated by CD4(+)T cells in neurodegenerative diseases. Front. Cell Neurosci. 12. doi: 10.3389/fncel.2018.00114
Sonar, S. A., Lal, G. (2017). Differentiation and transmigration of CD4 T cells in neuroinflammation and autoimmunity. Front. Immunol. 8. doi: 10.3389/fimmu.2017.01695
Spittau, B., Dokalis, N., Prinz, M. (2020). The role of TGFbeta signaling in microglia maturation and activation. Trends Immunol. 41, 836–848. doi: 10.1016/j.it.2020.07.003
Stich, A., Abel, P. M., Krishna, S. (2002). Human african trypanosomiasis. Bmj 325, 203–206. doi: 10.1136/bmj.325.7357.203
Stumhofer, J. S., Laurence, A., Wilson, E. H., Huang, E., Tato, C. M., Johnson, L. M., et al. (2006). Interleukin 27 negatively regulates the development of interleukin 17-producing T helper cells during chronic inflammation of the central nervous system. Nat. Immunol. 7, 937–945. doi: 10.1038/ni1376
Suvisaari, J., Torniainen-Holm, M., Lindgren, M., Harkanen, T., Yolken, R. H. (2017). Toxoplasma gondii infection and common mental disorders in the Finnish general population. J. Affect. Disord. 223, 20–25. doi: 10.1016/j.jad.2017.07.020
Suzuki, Y. (2020). The immune system utilizes two distinct effector mechanisms of T cells depending on two different life cycle stages of a single pathogen, toxoplasma gondii, to control its cerebral infection. Parasitol. Int. 76, 102030. doi: 10.1016/j.parint.2019.102030
Suzuki, Y., Wang, X., Jortner, B. S., Payne, L., Ni, Y., Michie, S. A., et al. (2010). Removal of toxoplasma gondii cysts from the brain by perforin-mediated activity of CD8+ T cells. Am. J. Pathol. 176, 1607–1613. doi: 10.2353/ajpath.2010.090825
Tan, Z., Lei, Z., Zhang, Z., Zhang, H., Shu, K., Hu, F., et al. (2019). Identification and characterization of microglia/macrophages in the granuloma microenvironment of encephalic schistosomiasis japonicum. BMC Infect. Dis. 19, 1088. doi: 10.1186/s12879-019-4725-5
Taratuto, A. L., Venturiello, S. M. (1997). Trichinosis. Brain Pathol. 7, 663–672. doi: 10.1111/j.1750-3639.1997.tb01081.x
Tesmer, L. A., Lundy, S. K., Sarkar, S., Fox, D. A. (2008). Th17 cells in human disease. Immunol. Rev. 223, 87–113. doi: 10.1111/j.1600-065X.2008.00628.x
Thase, M. E., Denko, T. (2008). Pharmacotherapy of mood disorders. Annu. Rev. Clin. Psychol. 4, 53–91. doi: 10.1146/annurev.clinpsy.2.022305.095301
Torrey, E. F., Yolken, R. H. (2003). Toxoplasma gondii and schizophrenia. Emerg. Infect. Dis. 9, 1375–1380. doi: 10.3201/eid0911.030143
Trivedi, S., Chakravarty, A. (2022). Neurological complications of malaria. Curr. Neurol. Neurosci. Rep. 22, 499–513. doi: 10.1007/s11910-022-01214-6
Tschoe, C., Bushnell, C. D., Duncan, P. W., Alexander-Miller, M. A., Wolfe, S. Q. (2020). Neuroinflammation after intracerebral hemorrhage and potential therapeutic targets. J. Stroke. 22, 29–46. doi: 10.5853/jos.2019.02236
Tyebji, S., Seizova, S., Hannan, A. J., Tonkin, C. J. (2019). Toxoplasmosis: A pathway to neuropsychiatric disorders. Neurosci. Biobehav. Rev. 96, 72–92. doi: 10.1016/j.neubiorev.2018.11.012
Van Hal, S. J., Stark, D. J., Fotedar, R., Marriott, D., Ellis, J. T., Harkness, J. L. (2007). Amoebiasis: Current status in australia. Med. J. Aust. 186, 412–416. doi: 10.5694/j.1326-5377.2007.tb00975.x
Waisman, A., Hauptmann, J., Regen, T. (2015). The role of IL-17 in CNS diseases. Acta Neuropathol. 129, 625–637. doi: 10.1007/s00401-015-1402-7
White, M. W., Radke, J. R., Radke, J. B. (2014). Toxoplasma development - turn the switch on or off? Cell Microbiol. 16, 466–472. doi: 10.1111/cmi.12267
Whiteford, H. A., Degenhardt, L., Rehm, J., Baxter, A. J., Ferrari, A. J., Erskine, H. E., et al. (2013). Global burden of disease attributable to mental and substance use disorders: Findings from the global burden of disease study 2010. Lancet 382, 1575–1586. doi: 10.1016/s0140-6736(13)61611-6
Worth, A. R., Andrew Thompson, R. C., Lymbery, A. J. (2014). Reevaluating the evidence for toxoplasma gondii-induced behavioural changes in rodents. Adv. Parasitol. 85, 109–142. doi: 10.1016/B978-0-12-800182-0.00003-9
Xiao, J. (2020). Toxoplasma-induced behavioral changes: An aspecific consequence of neuroinflammation. Trends Parasitol. 36, 317–318. doi: 10.1016/j.pt.2020.01.005
Xu, X., Lu, Y. N., Cheng, J. H., Lan, H. W., Lu, J. M., Jin, G. N., et al. (2022). Ginsenoside Rh2 reduces depression in offspring of mice with maternal toxoplasma infection during pregnancy by inhibiting microglial activation via the HMGB1/TLR4/NF-kappaB signaling pathway. J. Ginseng Res. 46, 62–70. doi: 10.1016/j.jgr.2021.04.003
Xu, Y., Wu, J., Yuan, X., Liu, W., Pan, J., Xu, B. (2021a). MicroRNA-155 contributes to host immunity against toxoplasma gondii. Parasite. 28, 83. doi: 10.1051/parasite/2021082
Xu, Z., Zhang, X., Chang, H., Kong, Y., Ni, Y., Liu, R., et al. (2021b). Rescue of maternal immune activation-induced behavioral abnormalities in adult mouse offspring by pathogen-activated maternal treg cells. Nat. Neurosci. 24, 818–830. doi: 10.1038/s41593-021-00837-1
Yalin Sapmaz, S., Sen, S., Ozkan, Y., Kandemir, H. (2019). Relationship between toxoplasma gondii seropositivity and depression in children and adolescents. Psychiatry Res. 278, 263–267. doi: 10.1016/j.psychres.2019.06.031
Yang, J., Sundrud, M. S., Skepner, J., Yamagata, T. (2014). Targeting Th17 cells in autoimmune diseases. Trends Pharmacol. Sci. 35, 493–500. doi: 10.1016/j.tips.2014.07.006
Yang, J., Wei, P., Barbi, J., Huang, Q., Yang, E., Bai, Y., et al. (2020). The deubiquitinase USP44 promotes treg function during inflammation by preventing FOXP3 degradation. EMBO Rep. 21, e50308. doi: 10.15252/embr.202050308
Yarovinsky, F. (2014). Innate immunity to toxoplasma gondii infection. Nat. Rev. Immunol. 14, 109–121. doi: 10.1038/nri3598
Yin, K., Xu, C., Zhao, G., Xie, H. (2022). Epigenetic manipulation of psychiatric behavioral disorders induced by toxoplasma gondii. Front. Cell. Infection Microbiol. 12. doi: 10.3389/fcimb.2022.803502
Yirmiya, R., Rimmerman, N., Reshef, R. (2015). Depression as a microglial disease. Trends Neurosci. 38, 637–658. doi: 10.1016/j.tins.2015.08.001
Zhang, J., Ke, K. F., Liu, Z., Qiu, Y. H., Peng, Y. P. (2013a). Th17 cell-mediated neuroinflammation is involved in neurodegeneration of abeta1-42-induced alzheimer's disease model rats. PloS One 8, e75786. doi: 10.1371/journal.pone.0075786
Zhang, X., Wu, C., Song, J., Gotte, M., Sorokin, L. (2013b). Syndecan-1, a cell surface proteoglycan, negatively regulates initial leukocyte recruitment to the brain across the choroid plexus in murine experimental autoimmune encephalomyelitis. J. Immunol. 191, 4551–4561. doi: 10.4049/jimmunol.1300931
Keywords: mood disorders, Toxoplasma gondii, CD4+ T cell, regulatory T cells, Th17
Citation: Wang Q, Zhong Y, Chen N and Chen J (2023) From the immune system to mood disorders especially induced by Toxoplasma gondii: CD4+ T cell as a bridge. Front. Cell. Infect. Microbiol. 13:1078984. doi: 10.3389/fcimb.2023.1078984
Received: 24 October 2022; Accepted: 23 March 2023;
Published: 03 April 2023.
Edited by:
Debanjan Mukhopadhyay, Presidency University, IndiaReviewed by:
David Arranz-Solís, Complutense University of Madrid, SpainArpita Konar, Presidency University, India
Copyright © 2023 Wang, Zhong, Chen and Chen. This is an open-access article distributed under the terms of the Creative Commons Attribution License (CC BY). The use, distribution or reproduction in other forums is permitted, provided the original author(s) and the copyright owner(s) are credited and that the original publication in this journal is cited, in accordance with accepted academic practice. No use, distribution or reproduction is permitted which does not comply with these terms.
*Correspondence: Jinling Chen, chenchennt@ntu.edu.cn