- 1The Second Clinical College, Guizhou University of Traditional Chinese Medicine, Guiyang, Guizhou, China
- 2Clinical Medical Laboratory of the Second Affiliated Hospital, Guizhou University of Traditional Chinese Medicine, Guiyang, Guizhou, China
- 3Clinical Laboratory, Hangzhou Women’s Hospital, Hangzhou, Zhejiang, China
- 4Department of Quality Control, Guizhou Provincial Center for Clinical Laboratory, Guiyang, Guizhou, China
- 5Department of Clinical Laboratory, Zhejiang Hospital, Hangzhou, Zhejiang, China
- 6Department of Infectious Disease, Guizhou Provincial People’s Hospital, Guiyang, Guizhou, China
Sexually transmitted chlamydia and gonorrhea infections caused by the bacteria Chlamydia trachomatis and Neisseria gonorrhoeae remain a major public health concern worldwide, particularly in less developed nations. It is crucial to use a point of care (POC) diagnostic method that is quick, specific, sensitive, and user-friendly to treat and control these infections effectively. Here, a novel molecular diagnostic assay, combining multiplex loop-mediated isothermal amplification (mLAMP) with a visual gold nanoparticles-based lateral flow biosensor (AuNPs-LFB) was devised and used for highly specific, sensitive, rapid, visual, and easy identification of C. trachomatis and N. gonorrhoeae. Two unique independent primer pairs were successful designed against the ompA and orf1 genes of C. trachomatis and N. gonorrhoeae, respectively. The optimal mLAMP-AuNPs-LFB reaction conditions were determined to be 67°C for 35 min. The detection procedure, involving crude genomic DNA extraction (~5 min), LAMP amplification (35 min), and visual results interpretation (<2 min), can be completed within 45 min. Our assay has a detection limit of 50 copies per test, and we did not observe any cross-reactivity with any other bacteria in our testing. Hence, our mLAMP-AuNPs-LFB assay can potentially be used for POC testing to detect C. trachomatis and N. gonorrhoeae in clinical settings, particularly in underdeveloped regions.
Introduction
Sexually transmitted infections (STIs) are a major global public health concern and a cause of serious genital and reproductive morbidity and mortality, especially in underdeveloped regions (Kojima and Klausner, 2021;Tsevat et al., 2017). Chlamydia trachomatis and Neisseria gonorrhoeae are the two most common pathogens responsible for STIs, with around 130 and 87 million new cases annually worldwide, respectively, according to the World Health Organization (WHO) (World Health Organization, 2016; Rowley et al., 2019). Both infections are mainly asymptomatic, but if left untreated, they can lead to many severe complications (Foschi et al., 2020). They are strongly associated with orchitis, prostatitis, epididymitis, and urethritis in males (Wilkinson et al., 2021). Infections may result in pelvic inflammatory illness, salpingitis, ectopic pregnancy, and infertility in females (Uprety and Cardenas, 2019;Herzog et al., 2012). Fetal infection, premature delivery, stillbirth, low birth weight, and miscarriage can arise during pregnancy with infected mothers (Gaydos et al., 2019; Niles et al., 2021). Furthermore, C. trachomatis and N. gonorrhoeae are associated with a higher risk of human immunodeficiency virus infections (Tsevat et al., 2017; Goulart et al., 2020; Wilkinson et al., 2021). Therefore, developing point-of-care (POC) diagnostic instruments that are fast, sensitive, specific, and easily understandable is crucial to treat and contain these infections.
Loop-mediated isothermal amplification (LAMP) is an intriguing alternative to the polymerase chain reaction (PCR) and PCR-related technologies for POC nucleic acid testing (Augustine et al., 2020; Chaouch, 2021). Strong target gene amplification is achieved by performing nucleic acid isothermal amplification at a fixed temperature (58°C–69°C) for 30–60 min using Bacillus stearothermophilus (Bst) DNA polymerase with strand-displacement activity (Notomi et al., 2000; Shang et al., 2020). Two outside primers (F3 and B3), two interior primers (FIP and BIP), and two loop primers (LF and LB) are designed to span eight different target fragment areas to amplify target gene sequences (Varona and Anderson, 2021). The LAMP method has previously been used for the immediate, specific, sensitive, and affordable detection of pathogens such as the severe acute respiratory syndrome coronavirus 2, Zika virus, and human papillomavirus (Silva et al., 2020; Janikova et al., 2021; Vo and Story, 2021). Meanwhile, the LAMP has already been used to identify C. trachomatis and N. gonorrhoeae. Choopara et al. and Somboonna et al. combined LAMP with hydroxynaphthol blue for visual detection of C. trachomatis by the naked eye (Choopara et al., 2017; Somboonna and Choopara, 2019). Liu et al. combined LAMP with fluorescence dye for visual identification of N. gonorrhoeae by the naked eye (Liu et al., 2017). However, the results of each assay were ambiguous when the LAMP product concentration was low, and is inadequate for simultaneously detecting two target genes in a single test. Eboigbodin et al. combined LAMP with fluorescence reader for simultaneously detection of C. trachomatis and N. gonorrhoeae (Eboigbodin, 2019). However, this assay requires expensive equipments.
In order to overcome these shortcomings, the paper- and gold nanoparticle (AuNPs)-based lateral flow biosensor (LFB) platform is designed and widely recognized as an ideal POC diagnostic testing method due to its high reliability, selectivity, accuracy, minimal maintenance, prolonged stability, and limit of detection (LoD) (Quesada-González and Merkoçi, 2015;Kim et al., 2019). Recent advances in using AuNPs as biosensors have enabled AuNPs-LFBs to identify various biomarkers, including proteins, nucleic acids, and even entire cells (Koczula and Gallotta, 2016; Wang et al., 2021). Based on these features, AuNPs-LFBs are already widely used in biomedicine, agriculture, food safety, and environmental sciences (Aldewachi et al., 2017).In this study, multiplex LAMP (mLAMP) was combined with an AuNPs-based LFB (mLAMP-AuNPs-LFB) to rapidly, simply, and visually identify C. trachomatis and N. gonorrhoeae by targeting their ompA and orf1 genes, respectively (Edwards et al., 2014; Somboonna et al., 2018). Both genes showed no homology to other microbial genomes in BLAST searches of the GenBank database. The mLAMP-AuNPs-LFB assay principle and workflow are shown in Figures 1, 2, respectively. Its feasibility was confirmed using clinical genital secretion samples from patients. This assay can be completed within 45 min without any expensive facilities. Therefore, the mLAMP-AuNPs-LFB assay appears promising for POC testing to identify C. trachomatis and N. gonorrhoeae, particularly in situations with limited diagnostic tools.
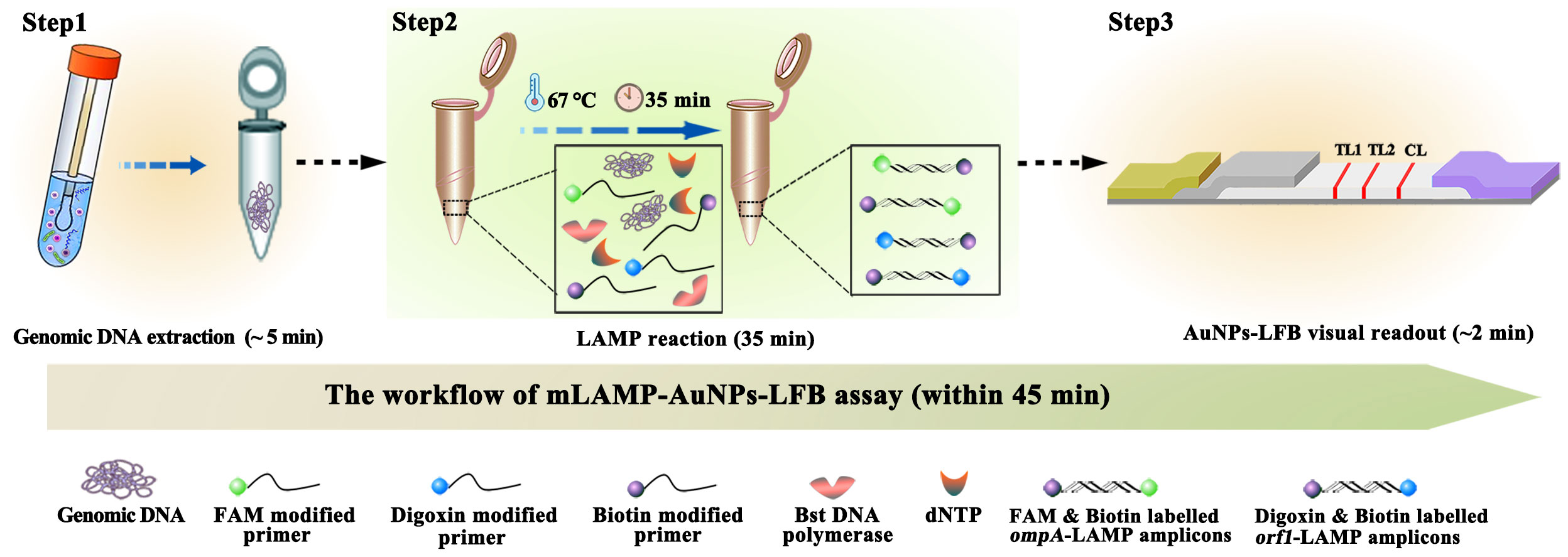
Figure 1 mLAMP-AuNPs-LFB assay workflow The mLAMP-AuNPs-LFB assay’s workflow includes genomic DNA extraction (5 min), mLAMP reaction (35 min), and AuNPs-LFB visual readout (<2 min) and can be completed within 45 min.
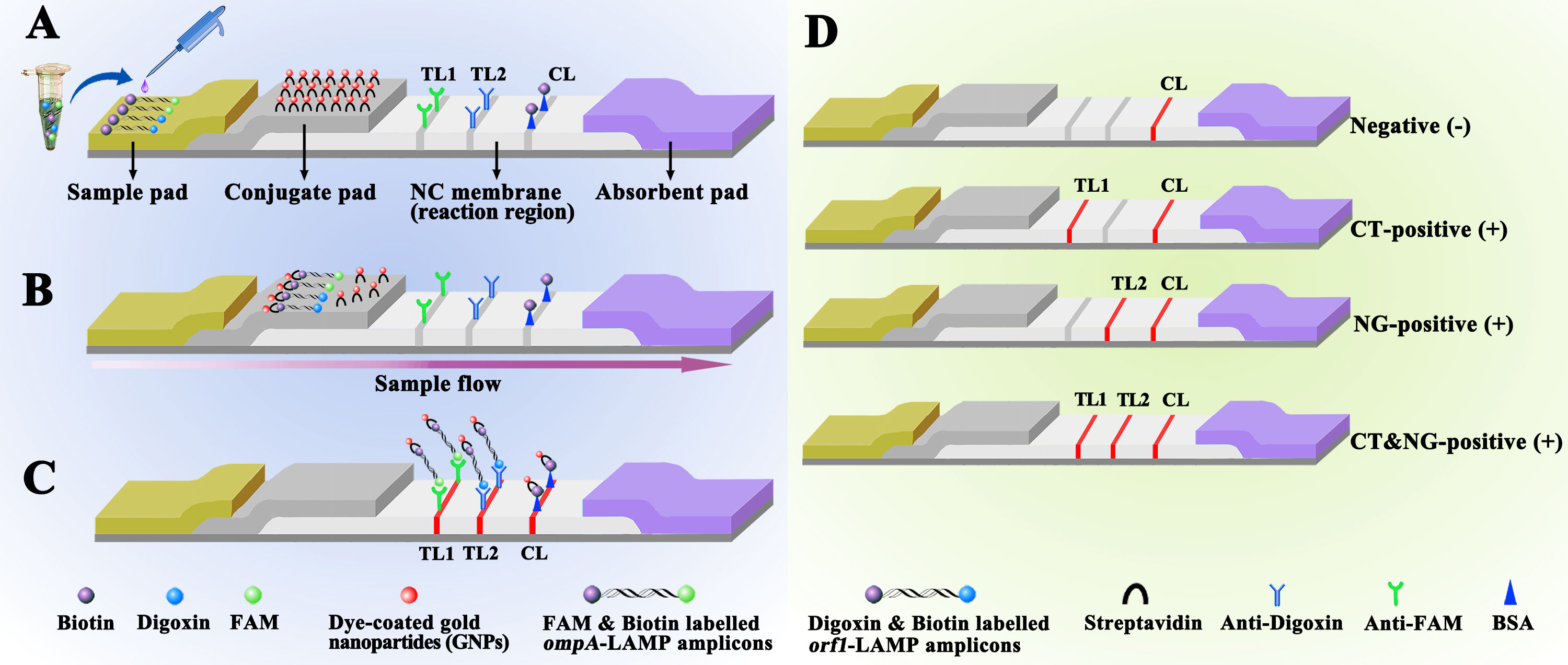
Figure 2 Schematic diagram showing AuNPs-LFB principles for visual mLAMP amplification product interpretation (A) mLAMP amplification products (0.5 μL) and running buffer (100 μL) were simultaneously added to the sample pad. (B) The running buffer containing the mLAMP products moves forward onto the conjugate pad and nitrocellulose membrane by capillary action. SA-AuNPss are hydrated, rapidly released, and combined with C. trachomatis or N. gonorrhoeae LAMP products at the conjugate pad. (C) FAM/biotin-labeled C. trachomatis LAMP products are captured by anti-FAM at TL1, Dig/biotin-labeled N. gonorrhoeae LAMP products are captured by anti-Dig at TL2, and SA-AuNPs are captured by biotin-BSA at the CL. (D) Interpretation of the mLAMP-AuNPs-LFB assay. C. trachomatis positive results are indicated by CL and TL1 bands on the AuNPs-LFB, N. gonorrhoeae positive results are indicated by CL and TL2 bands on the AuNPs-LFB, and C. trachomatis and N. gonorrhoeae positive results are indicated by TL1, TL2, and CL bands on the AuNPs-LFB. Negative results are indicated when only the CL band appears on the AuNPs-LFB. Key: CL, control line; TL1, test line one; TL2, test line two; CT, C. trachomatis; NG, N. gonorrhoeae; NC, nitrocellulose membrane.
Materials and methods
Reagents
We obtained AuNPs-based LFB materials, including biotinylated bovine serum albumin (biotin-BSA; 4 mg/mL), a rabbit anti-fluorescein antibody (anti-FAM; 0.2 mg/mL), and a sheep anti-digoxigenin antibody (anti-Dig; 0.25 mg/mL) from Abcam Co., Ltd. (Shanghai, China), and crimson red dye streptavidin-coated AuNPs (SA-AuNPs; 40 ± 5 nm, 10 mg/mL, 100 mM borate [pH 8.5] with 0.1% BSA, 0.05% Tween 20, and 10 mM ethylenediaminetetraacetic acid) from Bangs Laboratories Inc. (Fishers, IN, USA). Four LFB components (sample, conjugate, absorption pads, and nitrocellulose membranes) were manufactured and laminated on plastic adhesive backing by HuiDeXin Biotech. Co., Ltd. (Tianjin, China) according to our design scheme (Figure 2). Nucleic acid releasers were obtained from BaiAoLaiBo Technique Ltd. (Beijing, China). Universal isothermal amplification kits and the colorimetric indicator, malachite green (MG), were obtained from HuiDeXin Biotech. Co., Ltd. (Tianjin, China). Commercial PCR diagnostic kits for C. trachomatis and N. gonorrhoeae were obtained from DaAn Gene Co., Ltd. (Guangzhou, China).
Preparing target DNA and clinical samples
Full-length ompA sequences from 14 C. trachomatis serological variants (A-K and L1-L3; GenBank accession numbers JX548318.1, JX559518.1, JX559519.1, KP164991.1, JX559522.1, JX564244.1, JX564245.1, JX564246.1, JX564247.1, JX648604.1, JX564248.1, JX569832.1, KP120855.1, and JX569834.1) and the N. gonorrhoeae orf1 gene (M84113.1) were synthetically produced and cloned into the pUC57 vector. The basal concentration of all plasmids was 1×108 copies. The synthesized plasmids were used as positive controls.
Participants at the Hangzhou Women’s Hospital provided 146 vaginal discharge samples for examination between August 2021 and March 2022 that were believed to include STIs caused by C. trachomatis and/or N. gonorrhoeae. Nucleic acid-releasing agents were used to extract crude genomic DNA according to the manufacturer’s guidelines. Briefly, a sample was incubated with 100 μl of a nucleic acid releasing agent for 5 min. The resulting supernatant was used as a template for LAMP amplification. The quantities of genomic DNA were calculated using the 260/280 nm absorbance ratio using a Nano-Drop ND 2000 instrument (Thermo Fisher Scientific; Waltham, MA, USA). Other microorganisms investigated in this study are listed in Table 1.
AuNPs-based biosensor construction
Figure 2 shows that the AuNPs-LFB, with dimensions of 60 mm × 4 mm, is divided into four sections: the sample pad, the conjugate pad, the detection region (nitrocellulose membrane), and the absorption pad. SA-AuNPs containing crimson red dye are deposited on top of the conjugate pad. Rabbit anti-FAM (0.2 mg/mL), sheep anti-Dig (0.25 mg/mL), and biotin-BSA (4 mg/mL) are immobilized onto the nitrocellulose membrane of test 1 (TL1) and 2 (TL2) and control (CL) lines, respectively, which were separated by 5 mm. Finally, the four separate parts are bonded together on a plastic card with an adhesive backing. The AuNPs-LFB was kept dry and at room temperature until used. The workflow of AuNPs-LFB assay as following, LAMP products and running buffer were added to the AuNPs-LFB sample pad concurrently. A capillary action carries the LAMP product-containing flowing buffer along the biosensor, rehydrating the SA-AuNPs immobilized in crimson red dye. Biotin-BSA was used to capture SA-AuNPs at the CL. Anti-FAM was used to capture FAM/biotin-labeled C. trachomatis-LAMP products at TL1. Anti-Dig was used to capture Dig/biotin-labeled N. gonorrhoeae-LAMP amplicons at the TL2. The purpose of control line (CL) is to monitor whether the AuNPs-LFB test is valid. The CL band must be shown in each test. When the CL brand is not appeared, indicating the test is invalid.
LAMP primer design
Two LAMP primer sets were created based on the ompA and orf1 target genes to detect C. trachomatis and N. gonorrhoeae, respectively. The ompA genes from 14 different C. trachomatis serological variations (serovars A, B, C, D, E, F, G, H, I, J, K, L1, L2, and L3) were aligned using the MEGA-X software (https://www.megasoftware.net/) (Supplementary Figure S1). Conserved sequences were used for C. trachomatis LAMP primer design with the Primer Explorer v.5 (http://primerexplorer.jp/e/) and Primer Premier v.5.0 software. Primer pair specificity was confirmed using the BLAST analysis tool. Table 2 lists the primer sequences and alterations, and Supplementary Figure S1 shows the primer locations. All primers were produced and purified via high-performance liquid chromatography by The TsingKe Biotechnology Company (Beijing, China).
LAMP reactions and detection
The single LAMP reactions for C. trachomatis or N. gonorrhoeae were performed in 25 μL reaction volumes. Each reaction contained 1 μL of each standard plasmid template (5 μL of the clinical sample template); 0.8 μM of F3, B3, LF, and LB; 1.6 μM of FIP and BIP; 1 μL of Bst 2.0 DNA polymerase (8 U); 12.5 μL of 2× reaction buffer (2 M betaine, 16 mM magnesium sulfate, 40 mM potassium chloride, 20 mM ammonium sulfate, 40 mM Tris-hydrochloride [pH 8.8], and 0.2% Tween-20); 1 μL of the colorimetric indicator (MG); supplemented with double-distilled water (DW) to 25 μL.
The one-step mLAMP reaction was performed in a 25 μl reaction volume containing 1 μL of each standard plasmid template (5 μL of the clinical sample template); 0.3 μM of ompA-F3, ompA-B3, ompA-LF, and ompA-LB; 0.6 μM of ompA-FIP and ompA-BIP; 0.5 μM of orf1-F3, orf1-B3, orf1-LF, and orf1-LB; 1 μM each orf1-FIP, and orf1-BIP; 12.5 μL of 2×reaction buffer; 1 μL of Bst 2.0 DNA polymerase (8 U); 1 μL of the colorimetric indicator (MG); supplemented with DW to 25 μl in DW water. The reaction was performed in a heat-block or water bath at a constant temperature (conditions were optimized as outlined below).
Real-time turbidity LA-500 (Lumiprobe; Tokyo, Japan), visual detection reagents (MG), and the AuNPs-LFB were used to track the LAMP amplification products. A turbidity value of >0.1 indicated a successful outcome. Reaction mixtures that turned bright green indicated a successful MG visual detection; a colorless reaction indicated a failed detection. CL and TL1 simultaneously appearing on the AuNPs-LFB indicated a positive C. trachomatis-LAMP result. CL and TL2 simultaneously appearing on the AuNPs-LFB indicated a positive N. gonorrhoeae-LAMP result. CL only appearing on the AuNPs-LFB indicated a negative result.
Assay condition optimization
The optimal mLAMP-AuNPs-LFB reaction temperature was determined by incubating the C. trachomatis-LAMP and N. gonorrhoeae-LAMP reactions at 62°C to 69°C (in 1°C increments) and assessing their amplification results using real-time turbidity. Then, the incubation time was varied from 15 to 45 min (in 10 min increments) at the optimal reaction temperature with the amplification results detected by AuNPs-LFB. Each assay was performed in triplicate.
mLAMP-AuNPs-LFB assay sensitivity
Two conventional plasmids, the ompA and orf1 plasmids, were produced and then serially diluted by a factor of ten to provide copy numbers ranging from 5.0×104 to 5.0×10-1. LAMP reactions were performed under optimum conditions, and the data were examined using MG and AuNPs-LFB.
mLAMP-AuNPs-LFB assay specificity
Synthetic nucleic acid sequences and bacterial, viral, and fungal nucleic acid templates were detected at ≥1.0×104 copies to assess the mLAMP-AuNPs-LFB assay’s specificity (Table 1). All assays were performed at least thrice, with DW serving as the negative control.
Feasibility of the mLAMP-AuNPs-LFB for clinical samples
Genital secretion samples were obtained from 146 women at the Hangzhou Women’s Hospital who were believed to have STIs caused by C. trachomatis and/or N. gonorrhoeae. Genomic DNA templates were obtained rapidly using the Nucleic Acid Releasers Kit (BaiAoLaiBo Technique Ltd.) according to the manufacturer’s guidelines. We compared the mLAMP-AuNPs-LFB assay to commercially available real-time TaqMan PCR Kits for C. trachomatis and N. gonorrhoeae (DaAn Gene Co., Ltd.; Guangzhou, China) on an Applied Biosystems™ 7500 Real-Time PCR System (Life Technologies; Singapore). The basis for determining the copy number of the samples according to the corresponding standard curve. C. trachomatis and N. gonorrhoeae concentrations of >500 copies were considered positive based on the manufacturer’s recommendations. The Human Ethics Committee of Hangzhou Women’s Hospital approved the lawful collection and analysis of these genomic DNA templates (approval no. [2021]-K[2]-8). The identification investigations were performed at biosafety level 2 based on the WHO Biosafety Manual (3rd Edition). The mLAMP-AuNPs-LFB data were compared with quantitative real-time PCR (qPCR) data.
Results
mLAMP-AuNPs-LFB assay system overview
A representative schematic and workflow of the mLAMP-AuNPs-LFB assay are shown in Figures 1, 2, respectively. Briefly, genomic DNA templates were rapidly extracted using nucleic acid releasers (Figure 1, step 1). The ompA-FIP* and ompA-LF* primers were 5’-labeled with fluorescein (FAM) and biotin, and orf1-FIP* and orf1-LF* with digoxigenin (Dig) and biotin. The ompA-LAMP products were labeled with FAM and biotin and the orf1-LAMP products with Dig and biotin after a 35 min reaction at 67°C (Figure 1, step 2). Finally, the mLAMP products were visually monitored with an AuNPs-LFB within 2 min (Figure 1, step 3).
LAMP products (0.5 µL) and running buffer (100 µL; 100 mM phosphate-buffered saline with 1% Tween 20 [pH 7.4]) were added to the AuNPs-LFB sample pad concurrently (Figure 2A). A capillary action carries the LAMP product-containing flowing buffer along the biosensor, rehydrating the SA-AuNPs immobilized in crimson red dye (Figure 2B). Biotin-BSA was used to capture SA-AuNPs at the CL. Anti-FAM was used to capture FAM/biotin-labeled ompA-LAMP products at TL1. Anti-Dig was used to capture Dig/biotin-labeled orf1-LAMP amplicons at the TL2. The result is negative when only biotin-BSA captures the SA-AuNPs at the CL (Figure 2C). The interpretation of the mLAMP-AuNPs-LFB assay is outlined in Figure 2D.
mLAMP-AuNPs-LFB assay confirmation
The ompA-, orf1-, or mLAMP-amplification mixes were incubated at a fixed temperature (65°C) for 1 h to confirm the reaction system with the two LAMP primer sets. Then, a visual detection reagent (MG) and the AuNPs-LFB were used to assess the ompA/orf1 and mLAMP amplification results, respectively. DW was used as the negative control. While positive ompA-, orf1-, and mLAMP reactions changed from colorlessness to bright green, the negative control remained colorless (Figure 3A). The co-appearance of crimson-red TL1 and CL bands with AuNPs-LFB detection indicated a positive ompA-LAMP result. The co-appearance of crimson-red TL2 and CL bands indicated a positive orf1-LAMP result. A positive mLAMP result was indicated by the simultaneous appearance of TL1, TL2, and CL bands in the AuNPs-LFB. In contrast, the negative control showed only a crimson red CL band (Figure 3B). In conclusion, our findings supported using both LAMP primer sets in the mLAMP reaction.
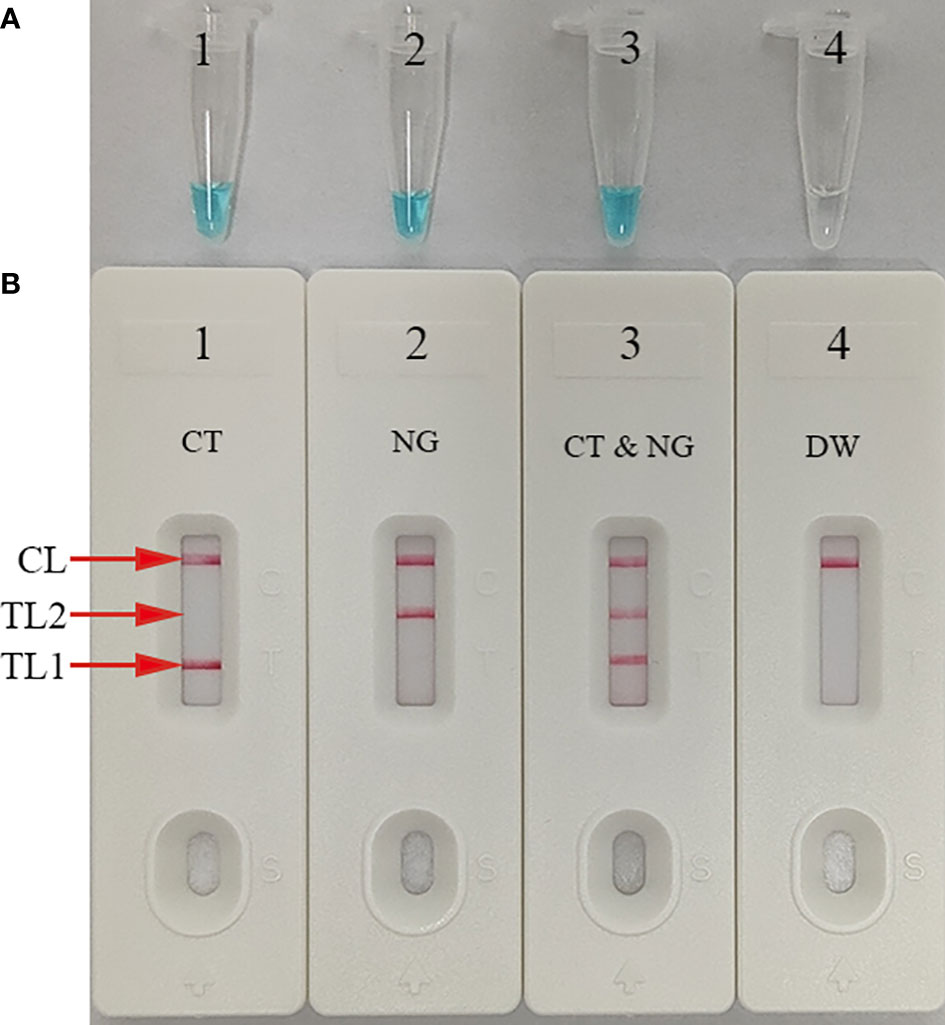
Figure 3 mLAMP product confirmation and verification The mLAMP products were simultaneously identified using (A) malachite green and (B) the AuNPs-LFB. Tube/AuNPs-LFB 1 shows a positive C. trachomatis result. Tube/AuNPs-LFB 2 shows a positive N. gonorrhoeae result. Tube/AuNPs-LFB 3 shows a positive C. trachomatis and N. gonorrhoeae result. Tube/AuNPs-LFB 4 shows negative control (DW). Key: CL, control line; TL1, test line one; TL2, test line two; CT, C. trachomatis; NG, N. gonorrhoeae.
ompA- and orf1-LAMP assay temperature optimization
Optimizing the incubation temperature for LAMP reactions is essential. Therefore, we used a standard plasmid copy number (1.0×104) to evaluate temperatures from 62°C to 69°C in 1°C increments. The LAMP amplification results were tracked in real-time using turbidity measurements. The results indicated that robust ompA-LAMP amplification occurred at 66°C to 69°C and orf1-LAMP amplification at 67°C to 69°C (Figure 4). Therefore, 67°C was the most suitable amplification temperature for our assay.
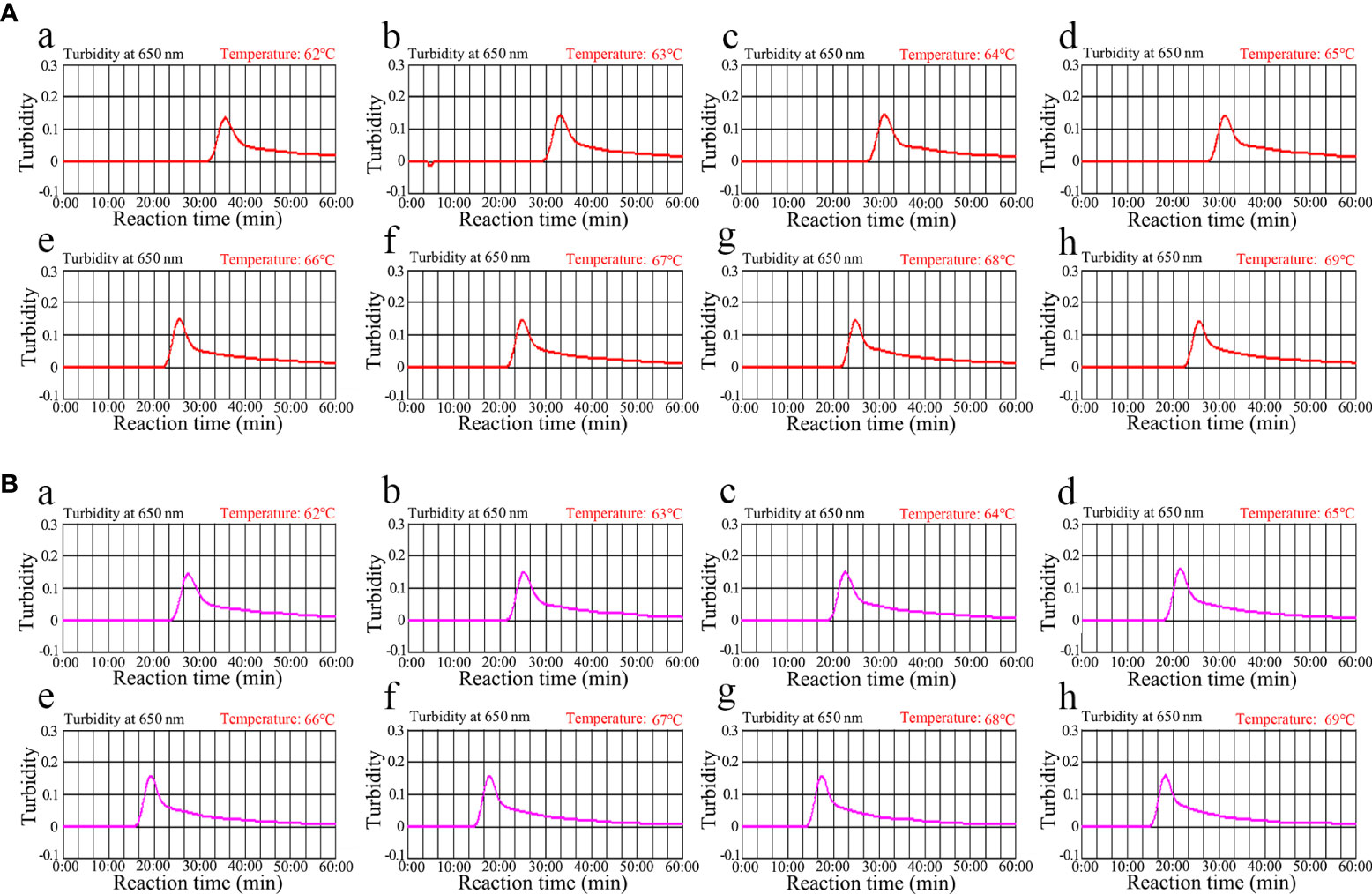
Figure 4 Temperature optimization for the (C) trachomatis and N. gonorrhoeae LAMP amplification LAMP amplifications for (A) C. trachomatis and (B) N. gonorrhoeae were monitored using real-time turbidity, and their corresponding amplicon curves are shown as graphs. A turbidity >0.1 indicated a positive result. Eight kinetic graphs were obtained at different temperatures (62°C–69°C in 1°C increments) with 1×104 target gene copies. Graphs e (66°C) to h (69°C) in (A) showed robust amplification. Graphs from f (67°C) to h (69°C) in (B) showed robust amplification.
mLAMP-AuNPs-LFB assay sensitivity
Serial dilutions of nucleic acid templates (ompA- and orf1-plasmid constructs) with 5.0×104 to 5.0×10-1 copies were used to determine the LoD of our assay. mLAMP reactions were performed as described above, and the results were visualized using MG and the AuNPs-LFB. The sensitivity of our assay was 50 copies per test for both the ompA (Figures 5A, B) and orf1 (Figures 5C, D) plasmids. The two target genes were simultaneously detected and identified in a single reaction (Figures 5E, F). The mLAMP results using the AuNPs-LFB were consistent with the visual detection reagent (MG; Figure 5). However, it should be noted that the visual detection method (MG) cannot discriminate target genes in a multiplex assay. The sensitivity of mLAMP-AuNPs-LFB was consistent with single ompA and orf1 mLAMP-AuNPs-LFB assays (Figure 5).
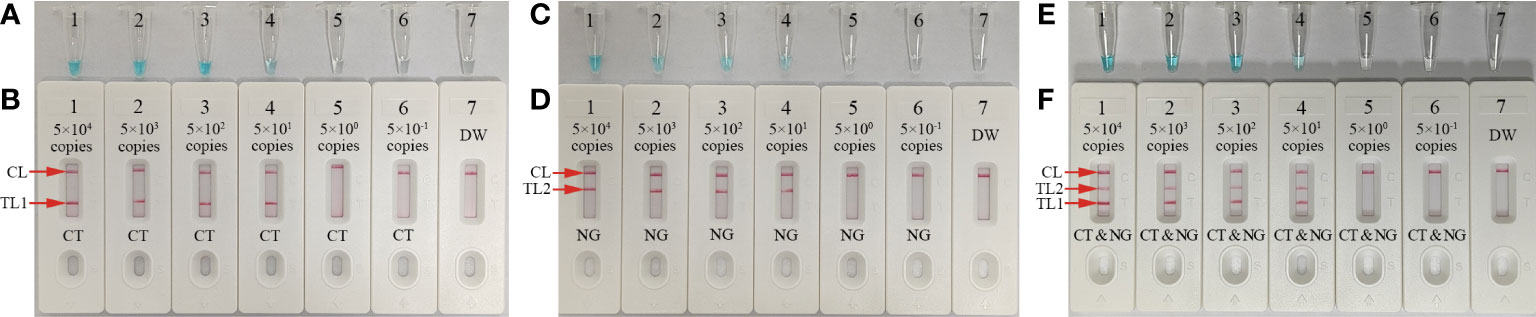
Figure 5 Sensitivity analysis of the mLAMP-AuNPs-LFB assay with serial nucleic acid template dilutions Serial dilutions (5.0×104, 5.0×103, 5.0×102, 5.0×101, 5.0×100, and 5.0×10-1 copies) of C. trachomatis ompA and N. gonorrhoeae orf1 plasmids were used as templates, and DW was used as the negative control. Results were simultaneously analyzed by visual reagent malachite green and the AuNPs-LFB. (A, B): A sensitivity analysis of the C. trachomatis LAMP assay indicated its LoD was 50 copies per reaction. (C, D): A sensitivity analysis of the N. gonorrhoeae LAMP assay indicated its LoD was 50 copies per reaction. (E, F): A sensitivity analysis of the mLAMP assay for ompA and orf1 indicated its LoD was 50 copies of the nucleic acid template per reaction. Key: CL, control line; TL1, test line one; TL2, test line two; CT, C. trachomatis; NG, N. gonorrhoeae; MG, malachite green.
mLAMP-AuNPs-LFB assay reaction time optimization
Four assay reaction times (15, 25, 35, and 45 min) were evaluated at 67°C to determine the optimal reaction time for the LAMP amplification stage of the protocol. The AuNPs-LFB was used to determine the outcomes. A 35 min LAMP reaction was found to be optimal at the genomic DNA template LoD (50 copies; Figure 6). Therefore, our assay’s entire detection process can be completed within 45 min: rapid genomic DNA preparation (5 min), mLAMP amplification (35 min), and visual outcome (<2 min).
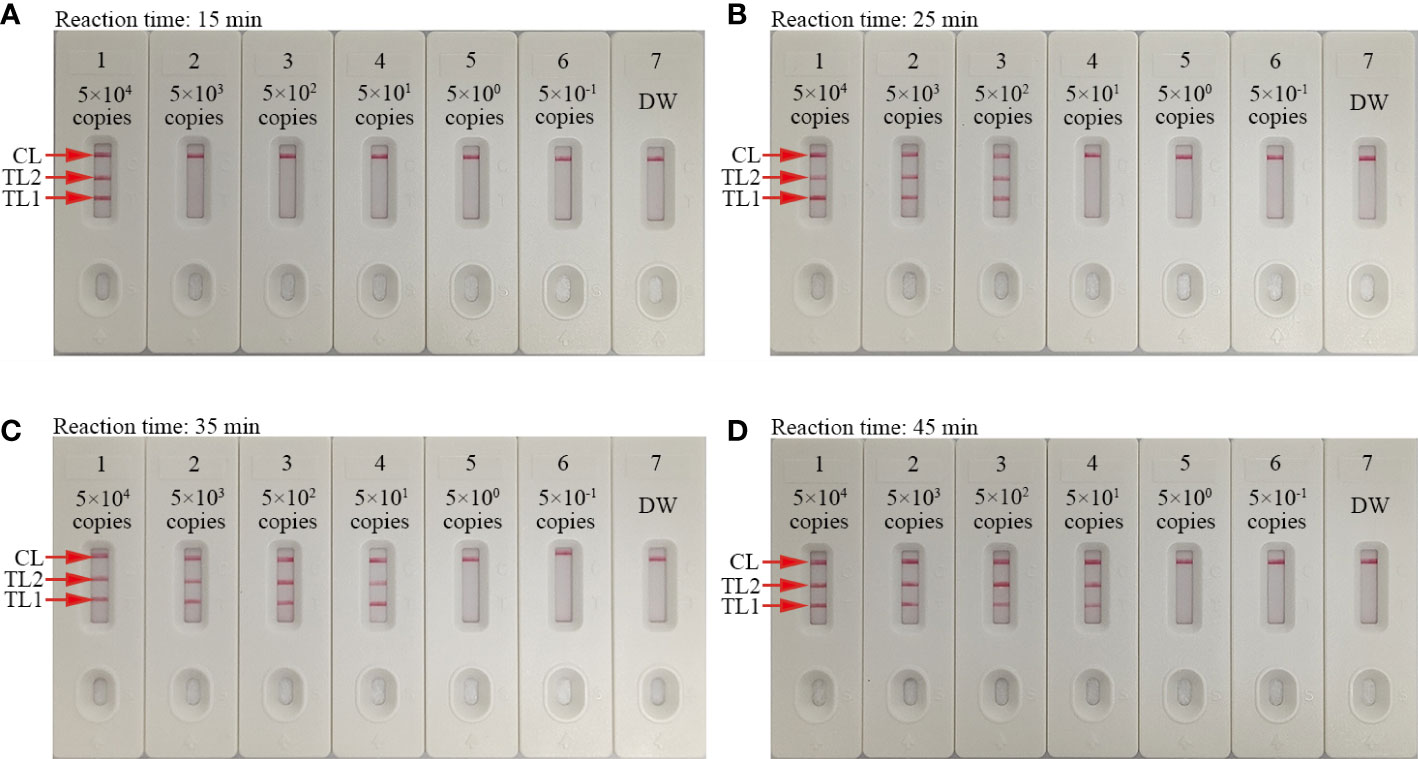
Figure 6 Amplification time optimization for the mLAMP-AuNPs-LFB assay Four LAMP reaction times were evaluated at 67°C: (A) 15 min, (B) 25 min, (C) 35 min, and (D) 45 min. AuNPs-LFBs 1–7 represent nucleic acid template levels 5.0×104, 5.0×103, 5.0×102, 5.0×101, 5.0×100, and 5.0×10-1 copies and negative control (DW), respectively. Results were analyzed using AuNPs-LFBs. The optimal LoD occurred with a 35 min reaction time (C). Key: CL, control line; TL1, test line one; TL2, test line two.
mLAMP-AuNPs-LFB assay specificity
Our assay’s specificity was evaluated using C. trachomatis ompA plasmids (serovars A, B, C, D, E, F, G, H, I, G, K, L1, L2, and L3), an N. gonorrhoeae orf1 plasmid, positive C. trachomatis and N. gonorrhoeae clinical samples (confirmed by qPCR), and 15 other pathogens (Table 1). Both C. trachomatis and N. gonorrhoeae strains’ genomic DNA extractions were successful. Positive C. trachomatis and N. gonorrhoeae results were indicated by the presence of three red bands (TL1, TL2, and CL) on the AuNPs-LFB. C. trachomatis isolates caused TL1 and CL bands on the AuNPs-LFB, indicating success. N. gonorrhoeae strains caused TL2 and CL bands on the AuNPs-LFB, indicating success. All other pathogens and control groups examined returned negative results (Figure 7). Therefore, our assay could distinguish between C. trachomatis and N. gonorrhoeae strains.
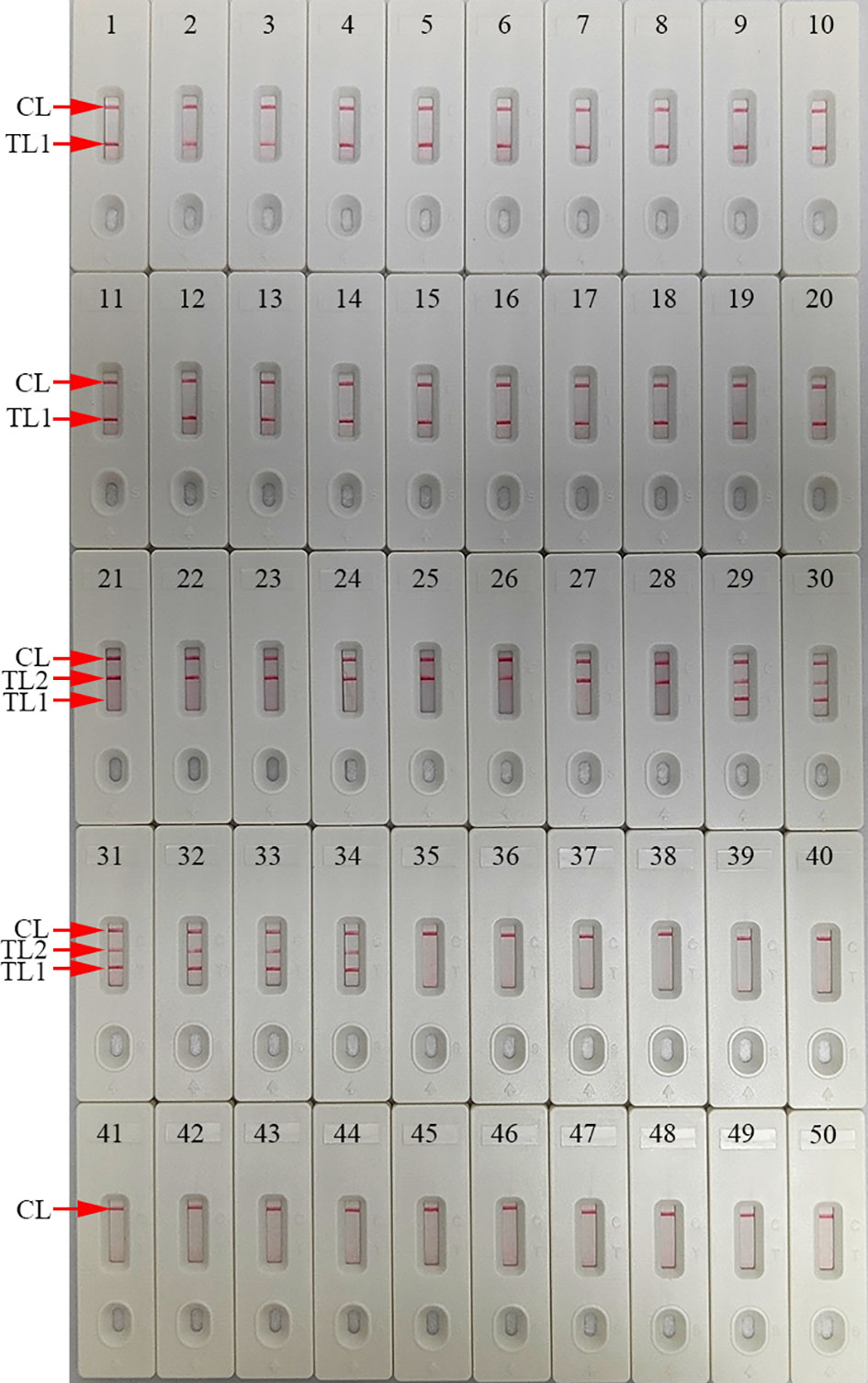
Figure 7 mLAMP-AuNPs-LFB assay specificity with different strains Assay specificity was evaluated using different nucleic acid templates. Amplification products were tested using AuNPs-LFBs: 1–14, C. trachomatis serovars A, B, C, D, E, F, G, H, I, J, K, L1, L2, and L3 ompA plasmids; 15–20, C. trachomatis clinical samples; 21, N. gonorrhoeae orf1 plasmids; 22, N. gonorrhoeae reference strain ATCC 49926; 23–28, N. gonorrhoeae clinical samples; 29–34, C. trachomatis and N. gonorrhoeae clinical samples; 35, Neisseria meningitides; 36, Ureaplasma urealyticum; 37, Escherichia coli; 38, Staphylococcus aureus; 39, human papillomavirus; 40, Mycoplasma pneumonia; 41, Haemophilus influenza; 42, Streptococcus pyogenes; 43, human enterovirus EV71; 44, Coxsackie virus CAV16; 45, Klebsiella pneumoniae; 46, Pseudomonas aeruginosa;47, Candida glabrata; 48, Cryptococcus neoformans; 49, Listeria monocytogenes; 50, negative control (DW). Key: CL, control line; TL, test line.
Evaluation of mLAMP-AuNPs-LFB assay with clinical samples
We further evaluated the clinical feasibility of our mLAMP-AuNPs-LFB assay for accurately identifying C. trachomatis and N. gonorrhoeae with 146 suspected C. trachomatis and/or N. gonorrhoeae genital secretion samples examined using qPCR and our assay. Forty-eight of the 146 samples were qPCR-confirmed (>500 copies) as C. trachomatis-positive, 29 as N. gonorrhoeae-positive, and six as C. trachomatis and N. gonorrhoeae co-infections. All C. trachomatis and N. gonorrhoeae positive samples were confirmed by our mLAMP-AuNPs-LFB assay. In addition, three C. trachomatis-negative and one N. gonorrhoeae-negative samples (genomic DNA concentrations of 100–500 copies) were positive with our mLAMP-AuNPs-LFB assay (Table 3 and Supplementary Table S1). Our assay’s positive results with these four discordant samples were confirmed by PCR amplification and DNA sequencing (Supplementary Table S1). Therefore, these data indicate that our assay can function as an advanced clinical diagnostic tool for C. trachomatis and N. gonorrhoeae.
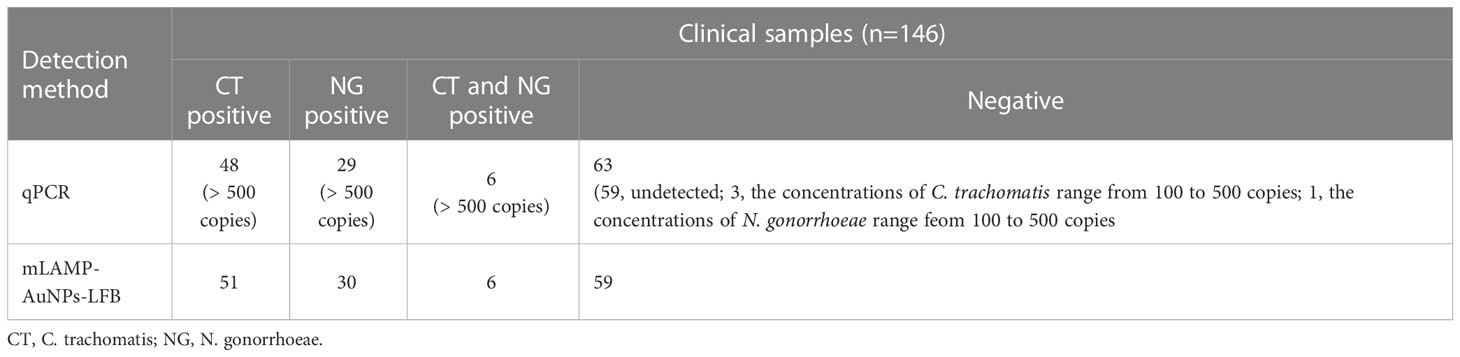
Table 3 Comparing C. trachomatis and N. gonorrhoeae levels in clinical samples using our mLAMP-AuNPs-LFB assay and qPCR method.
Discussion
This study successfully developed and tested a novel mLAMP-AuNPs-LFB POC testing platform to identify C. trachomatis and N. gonorrhoeae. It uses mLAMP for ompA and orf1 amplification, followed by a visual AuNPs-LFB readout. It was used to test genital secretion samples from individuals with suspected STIs caused by C. trachomatis and/or N. gonorrhoeae, and its results were compared to a commercial qPCR assay.
Various laboratory diagnostic approaches have recently been used to test chlamydia and gonorrhea and have changed considerably (Uprety and Cardenas, 2019;Adamson et al., 2020; Adamson and Klausner, 2022). Cultivation was considered the initial gold standard for C. trachomatis and N. gonorrhoeae identification (Centers for Disease Control and Prevention, 2014). However, both pathogens are fastidious and require stringent transport and culture conditions (Centers for Disease Control and Prevention, 2014). Furthermore, culture methods for C. trachomatis and N. gonorrhoeae isolation are difficult to standardize, are labor-intensive, have long turnaround times (48–72 h), require experienced analysts, and have relatively low sensitivity (Eboigbodin, 2019; Zhai et al., 2021). Serologic tests are not recommended because they lack precision for identifying active infections (Centers for Disease Control and Prevention, 2014). Nucleic acid amplification tests (NAATs) are currently recommended for diagnosing C. trachomatis and N. gonorrhoeae infections due to their high specificity and sensitivity and ease of sample transport and storage (Adamson et al., 2020; Inoue et al., 2021). Inoue et al. used multiplex PCR to simultaneously detect N. gonorrhoeae and C. trachomatis and identified 100 copies within 30 min (Inoue et al., 2021). While NAATs have improved STI diagnostic ability, they require expensive thermal cyclers and specific laboratory infrastructure, limiting accessibility in low- and middle-income countries. Our mLAMP-AuNPs-LFB POC assay only needs simple facilities and basic equipment, such as a water bath, heating block, or even a thermos cup, that can maintain reactions at 67°C for 30 min to perform the LAMP amplification step. Then, the outcomes can be visually interpreted using the AuNPs-LFB. The Bst DNA polymerase used in the LAMP reaction has fewer inhibitors than the Taq DNA polymerase used in traditional PCR. Therefore, crude genomic DNA is sufficient for LAMP amplification (Somboonna et al., 2018). Consequently, our mLAMP-AuNPs-LFB assay, which comprises crude genomic DNA extraction (~5 min), LAMP amplification (35 min), and visual result interpretation (<2 min), can be completed within 45 min.
Isothermal amplification technologies, such as recombinase polymerase (RPA) and cross-priming (CPA) amplification, have also been used to identify C. trachomatis and N. gonorrhoeae. Zhai et al. used multiplex RPA to detect C. trachomatis and N. gonorrhoeae and identified 200 copies per test within 25 min (Zhai et al., 2021). Yu et al. used multiplex CPA and detected 45 and 65 copies per test for C. trachomatis and N. gonorrhoeae, respectively, within 1.5 h (Yu et al., 2016).
This study used LAMP to pre-amplify the target genes, rapidly and robustly amplifying DNA amplicons at a constant temperature and providing 100-fold greater detection capability than traditional PCR (Notomi et al., 2000; Avendano and Patarroyo, 2020). Extremely specific amplicons are generated in the LAMP amplification system using the Bst DNA polymerase with four or six primer sets that span 6 or 8 unique sections of the target sequence (Notomi et al., 2000). The primer set includes forward and reverse outer (F3 and B3) and inner (FIP and BIP) primers; the four primers are usually sufficient for amplification. The reaction’s efficiency and specificity can be improved by incorporating two extra loop primers (LF and LB) into the LAMP reaction (McGinnis et al., 2021). We have previously used LAMP to detect C. trachomatis and N. gonorrhoeae, respectively, with an LoD as low as 50 copies per test (Chen et al., 2021; Chen et al., 2022). However, it was inadequate for simultaneously detecting these two important pathogens in a single test.
This study used the mLAMP method to simultaneously pre-amplify two target genes for C. trachomatis and N. gonorrhoeae. LAMP primer sets specific to the C. trachomatis ompA gene and N. gonorrhoeae orf1 gene were successfully designed. C. trachomatis LAMP primers were designed to target eight areas of the ompA gene based on 14 serological C. trachomatis variations (serovars A-K and L1-L3). The N. gonorrhoeae LAMP primers were designed to target eight areas within the orf1 gene.
The specificity of the mLAMP-AuNPs-LFB assay was confirmed with several C. trachomatis serological variants (A-K and L1-L3), N. gonorrhoeae strains, and 15 other pathogens. Our assay showed 100% specificity to both C. trachomatis’ ompA gene and N. gonorrhoeae’s orf1 gene (Table 1 and Figure 7). Furthermore, its LoD was as low as 50 copies (Figure 6). We also examined 146 genital secretion samples for C. trachomatis and N. gonorrhoeae using qPCR and our mLAMP-AuNPs-LFB assay to assess its clinical practicality. The data indicated that our assay had high specificity and sensitivity in detecting genital secretion samples (Tables 3, S1). Nevertheless, a second trial should examine clinical samples with significantly lower copy numbers using our assay to better reflect clinical applications.
An AuNPs-LFB was used in our assay for visual and rapid readout of mLAMP results. This paper-based platform is extremely applicable to POC testing due to its easy operation, sensitivity, specificity, low cost, and visual interpretation (Cui and Zhou, 2020;Huang et al., 2019). The biosensor had four parts: the sample, conjugate, adsorbent pads, and the nitrocellulose membrane. The cellulose sample pad was an excellent medium for delivering the examined mLAMP products to the biosensor elements. The conjugate pad contained crimson red dyed SA-AuNPs. Nitrocellulose membranes immobilized target reactive molecules, such as anti-FAM, anti-Dig, and BSA-biotin, at the TL1, TL2, and CL positions, respectively. All three antigens (CL, TL1, and TL2) appeared simultaneously on the AuNPs-LFB for C. trachomatis- and N. gonorrhoeae-positive samples. CL and TL1 appeared simultaneously on the AuNPs-LFB for C. trachomatis-positive samples. CL and TL2 appeared simultaneously on the AuNPs-LFB for N. gonorrhoeae-positive samples. Only CL appeared on the AuNPs-LFB for C. trachomatis- and N. gonorrhoeae-negative samples. The adsorbent pad acts as a bibulous paper to direct the LAMP product flow from the sample pad to the reaction zone. It is located at the tip of the AuNPs-LFB (nitrocellulose membrane). A turbidimeter and visual reagent (MG) were also used to analyze mLAMP results. However, each requires specific devices or reagents and is inadequate for simultaneously detecting two target genes in a single test. Our AuNPs-LFB assay was also cheaper (~US$2.0/test). Therefore, the total cost for each test, including genomic DNA extraction (~US$0.50), LAMP reactions (~US$3.00), and AuNPs-LFB readout (~US$2.00), was approximately US$5.50.
Nevertheless, our AuNPs-LFB assay has some weaknesses. First, the result obtained with the naked eye is qualitative but not quantitative. Quantitative measurements with the mLAMP-AuNPs-LFB assay require further study. Second, the mLAMP reaction tube must be opened to be read by the AuNPs-LFB, increasing the risk of carry-over contamination. However, spraying 70% ethanol and 10%–15% sodium hypochlorite solution soon after completing each AuNPs-LFB assay is an effective way to avoid nucleic acid contamination. In our laboratory, cross-contamination appeared to have been effectively controlled since we encountered no false-positive results. In order to better fit the clinical application, it is necessary to improve the mLAMP-AuNPs-LFB assay system, and design a device for avoiding the opening tube procedure to reduce aerosol pollution.
Conclusions
This study combined mLAMP isothermal amplification with a visual AuNPs-LFB interpretation to create a novel mLAMP-AuNPs-LFB assay for the highly specific, sensitive, and rapid identification of C. trachomatis and N. gonorrhoeae in clinical settings. Our assay had a 50-copy LoD and showed no cross-reactivity with other pathogens. The entire test process can be completed within 45 min and does not require sophisticated equipment. Therefore, it meets the WHO-recommended assured POC testing requirements: low cost, sensitive, specific, user friendly, robust, equipment free, and attainable.
Data availability statement
The original contributions presented in the study are included in the article/Supplementary Material. Further inquiries can be directed to the corresponding authors.
Ethics statement
This study was approved by the Human Ethics Committee of Hangzhou Women’s Hospital (Approval No. [2021]-K (2)-8).
Author contributions
XC, SD and XL were involved in study conceptualization, supervision, and project administration. QZ, WY and YS performed experiments and data curation. XC and QZ involved in study funding acquisition and methodology. XC, QZ, WY, YS and SD collected clinical samples. QZ, WY and YS were involved in validation studies and visualization. XC was involved in writing–original draft. SD and XL were involved in writing–review and editing. All authors contributed to the article and approved the submitted version.
Funding
This work was supported by Zhejiang Provincial Natural Science Foundation of China (Grant No. LTGY23H190004), the Program of Scientific and Technological Project in Guizhou Province (Grant No. Qian Ke He [2020]4Y184), the Program of Science and Technology of Guizhou Provincial Health Commission (gzwjkj2022-1-497), and the Scientific and Technological in Guiyang City (Grant No. Zhu Ke He [2020]-10-5).
Acknowledgments
We thank the medical personnel at Hangzhou Women’s Hospital, 2nd GZUTCM, and GZCCL for their cooperation in this study. We also thank the patients who provided samples.
Conflict of interest
The authors declare that the research was conducted in the absence of any commercial or financial relationships that could be construed as a potential conflict of interest.
Publisher’s note
All claims expressed in this article are solely those of the authors and do not necessarily represent those of their affiliated organizations, or those of the publisher, the editors and the reviewers. Any product that may be evaluated in this article, or claim that may be made by its manufacturer, is not guaranteed or endorsed by the publisher.
Supplementary material
The Supplementary Material for this article can be found online at: https://www.frontiersin.org/articles/10.3389/fcimb.2023.1067554/full#supplementary-material
Supplementary Figure 1 | Nucleotide sequences and location of the C. trachomatis-ompA and N. gonorrhoeae-orf1 genes used to designed the mLAMP primers.
Supplementary Table 1 | Comparison of mLAMP-AuNPs-LFB and qPCR methods for assessment of C. trachomatis and N. gonorrhoeae in clinical samples.
References
Adamson, P. C., Klausner, J. D. (2022). Diagnostic tests for detecting Chlamydia trachomatis and Neisseria gonorrhoeae in rectal and pharyngeal specimens. J. Clin. Microbiol. 60 (4), e21121. doi: 10.1128/JCM.00211-21
Adamson, P. C., Pandori, M. W., Doernberg, S. B., Komarow, L., Sund, Z., Tran, T., et al. (2020). Analytical evaluation of the abbott tealtime CT/NG assay for detection of Chlamydia trachomatis and Neisseria gonorrhoeae in rectal and pharyngeal swabs. J. Mol. Diagn. 22 (6), 811–816. doi: 10.1016/j.jmoldx.2020.03.004
Aldewachi, H., Chalati, T., Woodroofe, M. N., Bricklebank, N., Sharrack, B., Gardiner, P. (2017). Gold nanoparticle-based colorimetric biosensors. Nanoscale. 10 (1), 18–33. doi: 10.1039/c7nr06367a
Augustine, R., Hasan, A., Das, S., Ahmed, R., Mori, Y., Notomi, T., et al. (2020). Loop-mediated isothermal amplification (LAMP): a rapid, sensitive, specific, and cost-effective point-of-care test for coronaviruses in the context of COVID-19 pandemic. Biology. 9 (8), 182. doi: 10.3390/biology9080182
Avendano, C., Patarroyo, M. A. (2020). Loop-mediated isothermal amplification as point-of-care diagnosis for neglected parasitic infections. Int. J. Mol. Sci. 21 (21), 7981. doi: 10.3390/ijms21217981
Centers for Disease Control and Prevention (2014). Recommendations for the laboratory-based detection of Chlamydia trachomatis and Neisseria gonorrhoeae–2014. MMWR Recomm Rep. 63, 1–19.
Chaouch, M. (2021). Loop-mediated isothermal amplification (LAMP): an effective molecular point-of-care technique for the rapid diagnosis of coronavirus SARS-CoV-2. Rev. Med. Virol. 31 (6), e2215. doi: 10.1002/rmv.2215
Chen, X., Zhou, Q., Tan, Y., Wang, R., Wu, X., Liu, J., et al. (2022). Nanoparticle-based lateral flow biosensor integrated with loop-mediated isothermal amplification for rapid and visual identification of Chlamydia trachomatis for point-of-care use. Front. Microbiol. 13. doi: 10.3389/fmicb.2022.914620
Chen, X., Zhou, Q., Wu, X., Wang, S., Liu, R., Dong, S., et al. (2021). Visual and rapid diagnosis of Neisseria gonorrhoeae using loop-mediated isothermal amplification combined with a polymer nanoparticle-based biosensor in clinical application. Front. Mol. Biosci. 8. doi: 10.3389/fmolb.2021.702134
Choopara, I., Arunrut, N., Kiatpathomchai, W., Dean, D., Somboonna, N. (2017). Rapid and visual Chlamydia trachomatis detection using loop-mediated isothermal amplification and hydroxynaphthol blue. Lett. Appl. Microbiol. 64 (1), 51–56. doi: 10.1111/lam.12675
Cui, F., Zhou, H. S. (2020). Diagnostic methods and potential portable biosensors for coronavirus disease 2019. Biosens Bioelectron. 165, 112349. doi: 10.1016/j.bios.2020.112349
Eboigbodin, K. E. (2019). Application of loop-mediated isothermal amplification assay for the detection of Chlamydia trachomatis and Neisseria gonorrhoeae. Methods Mol. Biol. 2042, 19–25. doi: 10.1007/978-1-4939-9694-0_3
Edwards, T., Burke, P. A., Smalley, H. B., Gillies, L., Hobbs, G. (2014). Loop-mediated isothermal amplification test for detection of Neisseria gonorrhoeae in urine samples and tolerance of the assay to the presence of urea. J. Clin. Microbiol. 52 (6), 2163–2165. doi: 10.1128/JCM.00314-14
Foschi, C., Zagarrigo, M., Belletti, M., Marangoni, A., Re, M. C., Gaspari, V. (2020). Genital and extra-genital Chlamydia trachomatis and Neisseria gonorrhoeae infections in young women attending a sexually transmitted infections (STI) clinic. New Microbiol. 43 (3), 115–120.
Gaydos, C. A., Ako, M. C., Lewis, M., Hsieh, Y. H., Rothman, R. E., Dugas, A. F. (2019). Use of a rapid diagnostic for Chlamydia trachomatis and Neisseria gonorrhoeae for women in the emergency department can improve clinical management: report of a randomized clinical trial. Ann. Emerg. Med. 74 (1), 36–44. doi: 10.1016/j.annemergmed.2018.09.012
Goulart, A., Farnezi, H., Franca, J., Santos, A. D., Ramos, M. G., Penna, M. (2020). HIV, HPV and Chlamydia trachomatis: impacts on male fertility. JBRA Assist. Reprod. 24 (4), 492–497. doi: 10.5935/1518-0557.20200020
Herzog, S. A., Heijne, J. C., Althaus, C. L., Low, N. (2012). Describing the progression from Chlamydia trachomatis and Neisseria gonorrhoeae to pelvic inflammatory disease: systematic review of mathematical modeling studies. Sex Transm. Dis. 39 (8), 628–637. doi: 10.1097/OLQ.0b013e31825159ff
Huang, Y., Xu, T., Wang, W., Wen, Y., Li, K., Qian, L., et al. (2019). Lateral flow biosensors based on the use of micro- and nanomaterials: a review on recent developments. Mikrochim Acta 187 (1), 70. doi: 10.1007/s00604-019-3822-x
Inoue, T., Chihara, Y., Kiba, K., Hirao, S., Tanaka, M., Yoneda, T., et al. (2021). Rapid multiplex PCR assay for simultaneous detection of Neisseria gonorrhoeae and Chlamydia trachomatis in genitourinary samples: a 30-minute assay. J. Microbiol. Methods 180, 106103. doi: 10.1016/j.mimet.2020.106103
Janikova, M., Hodosy, J., Boor, P., Klempa, B., Celec, P. (2021). Loop-mediated isothermal amplification for the detection of SARS-CoV-2 in saliva. Microb. Biotechnol. 14 (1), 307–316. doi: 10.1111/1751-7915.13737
Kim, H., Chung, D. R., Kang, M. (2019). A new point-of-care test for the diagnosis of infectious diseases based on multiplex lateral flow immunoassays. Analyst. 144 (8), 2460–2466. doi: 10.1039/c8an02295j
Koczula, K. M., Gallotta, A. (2016). Lateral flow assays. Essays Biochem. 60 (1), 111–120. doi: 10.1042/EBC20150012
Kojima, N., Klausner, J. D. (2021). Patients may accurately self-collect pharyngeal and rectal specimens for Neisseria gonorrhoeae and Chlamydia trachomatis infection: but is there benefit? Clin. Infect. Dis. 73 (9), e3181–e3182. doi: 10.1093/cid/ciaa1272
Liu, M. L., Xia, Y., Wu, X. Z., Huang, J. Q., Guo, X. G. (2017). Loop-mediated isothermal amplification of Neisseria gonorrhoeae porA pseudogene: a rapid and reliable method to detect gonorrhea. AMB Express. 7, 48. doi: 10.1186/s13568-017-0349-6
McGinnis, E., Chan, G., Hudoba, M., Markin, T., Yakimec, J., Roland, K. (2021). Malaria screening using front-line loop-mediated isothermal amplification. Am. J. Clin. Pathol. 155 (5), 690–697. doi: 10.1093/ajcp/aqaa173
Niles, J. K., Kaufman, H. W., Peterman, T. A., Tao, G., Gift, T. L., Alagia, D. P. (2021). Chlamydia trachomatis and Neisseria gonorrhoeae in pregnancy: trends in united states 2010 to 2018. Sex Transm. Dis. 48 (12), 932–938. doi: 10.1097/OLQ.0000000000001504
Notomi, T., Okayama, H., Masubuchi, H., Yonekawa, T., Watanabe, K., Amino, N., et al. (2000). Loop-mediated isothermal amplification of DNA. Nucleic Acids Res. 28 (12), E63. doi: 10.1093/nar/28.12.e63
Quesada-González, D., Merkoçi, A. (2015). Nanoparticle-based lateral flow biosensors. Biosens Bioelectron. 73, 47–63. doi: 10.1016/j.bios.2015.05.050
Rowley, J., Vander, H. S., Korenromp, E., Low, N., Unemo, M., Abu-Raddad, L. J., et al. (2019). Chlamydia, gonorrhoea, trichomoniasis and syphilis: global prevalence and incidence estimates 2016. Bull. World Health Organ. 97 (8), 548–562. doi: 10.2471/BLT.18.228486
Shang, Y., Sun, J., Ye, Y., Zhang, J., Zhang, Y., Sun, X. (2020). Loop-mediated isothermal amplification-based microfluidic chip for pathogen detection. Crit. Rev. Food Sci. Nutr. 60 (2), 201–224. doi: 10.1080/10408398.2018.1518897
Silva, S. J. R. D., Pardee, K., Pena, L. (2020). Loop-mediated isothermal amplification (LAMP) for the diagnosis of zika virus: a review. Viruses. 12 (1), 19. doi: 10.3390/v12010019
Somboonna, N., Choopara, I. (2019). Point-of-care Chlamydia trachomatis detection using loop-mediated isothermal amplification and hydroxynaphthol blue. Methods Mol. Biol. 2042, 11–17. doi: 10.1007/978-1-4939-9694-0_2
Somboonna, N., Choopara, I., Arunrut, N., Sukhonpan, K., Sayasathid, J., Dean, D., et al. (2018). Rapid and sensitive detection of Chlamydia trachomatis sexually transmitted infections in resource-constrained settings in Thailand at the point-of-care. PloS Negl. Trop. Dis. 12 (12), e6900. doi: 10.1371/journal.pntd.0006900
Tsevat, D. G., Wiesenfeld, H. C., Parks, C., Peipert, J. F. (2017). Sexually transmitted diseases and infertility. Am. J. Obstet. Gynecol. 216 (1), 1–9. doi: 10.1016/j.ajog.2016.08.008
Uprety, P., Cardenas, A. M. (2019). Extragenital screening is essential for comprehensive detection of Chlamydia trachomatis and Neisseria gonorrhoeae in the pediatric population. J. Clin. Microbiol. 57 (6), e00335-19. doi: 10.1128/JCM.00335-19
Varona, M., Anderson, J. L. (2021). Advances in mutation detection using loop-mediated isothermal amplification. ACS Omega. 6 (5), 3463–3469. doi: 10.1021/acsomega.0c06093
Vo, D. T., Story, M. D. (2021). Facile and direct detection of human papillomavirus (HPV) DNA in cells using loop-mediated isothermal amplification (LAMP). Mol. Cell Probes. 59, 101760. doi: 10.1016/j.mcp.2021.101760
Wang, T., Chen, L., Chikkanna, A., Chen, S., Brusius, I., Sbuh, N., et al. (2021). Development of nucleic acid aptamer-based lateral flow assays: a robust platform for cost-effective point-of-care diagnosis. Theranostics. 11 (11), 5174–5196. doi: 10.7150/thno.56471
Wilkinson, L. A., Carter, M. T., Wattengel, B. A., Lesse, A. J., Sellick, J. A., Mergenhagen, K. A. (2021). Societal factors contributing to infections caused by Chlamydia trachomatis and Neisseria gonorrhoeae in a veteran population. Int. J. STD AIDS. 32 (9), 845–851. doi: 10.1177/0956462421999276
World Health Organization (2016). WHO guidelines for the treatment of chlamydia trachomatis (Geneva: World Health Organization).
Yu, B., An, Y., Xu, G., Shan, H. (2016). Detection of Chlamydia trachomatis and Neisseria gonorrhoeae based on cross-priming amplification. Lett. Appl. Microbiol. 62 (5), 399–403. doi: 10.1111/lam.12560
Keywords: Chlamydia trachomatis, Neisseria gonorrhoeae, loop-mediated isothermal amplification, gold nanoparticle-based lateral flow biosensor, point-of-care testing
Citation: Chen X, Zhou Q, Yuan W, Shi Y, Dong S and Luo X (2023) Visual and rapid identification of Chlamydia trachomatis and Neisseria gonorrhoeae using multiplex loop-mediated isothermal amplification and a gold nanoparticle-based lateral flow biosensor. Front. Cell. Infect. Microbiol. 13:1067554. doi: 10.3389/fcimb.2023.1067554
Received: 12 October 2022; Accepted: 15 February 2023;
Published: 28 February 2023.
Edited by:
Hongchao Gou, Guangdong Academy of Agricultural Sciences, ChinaReviewed by:
Yan Han, Chinese Academy of Medical Sciences and Peking Union Medical College, ChinaQijie Lin, South China Agricultural University, China
Copyright © 2023 Chen, Zhou, Yuan, Shi, Dong and Luo. This is an open-access article distributed under the terms of the Creative Commons Attribution License (CC BY). The use, distribution or reproduction in other forums is permitted, provided the original author(s) and the copyright owner(s) are credited and that the original publication in this journal is cited, in accordance with accepted academic practice. No use, distribution or reproduction is permitted which does not comply with these terms.
*Correspondence: Xinhua Luo, bHVveGgwOUAxNjMuY29t; Shilei Dong, ZHNsMTY2QDEyNi5jb20=; Xu Chen, eHVjaGVuMTIyMEAxMjYuY29t
†These authors have contributed equally to this work