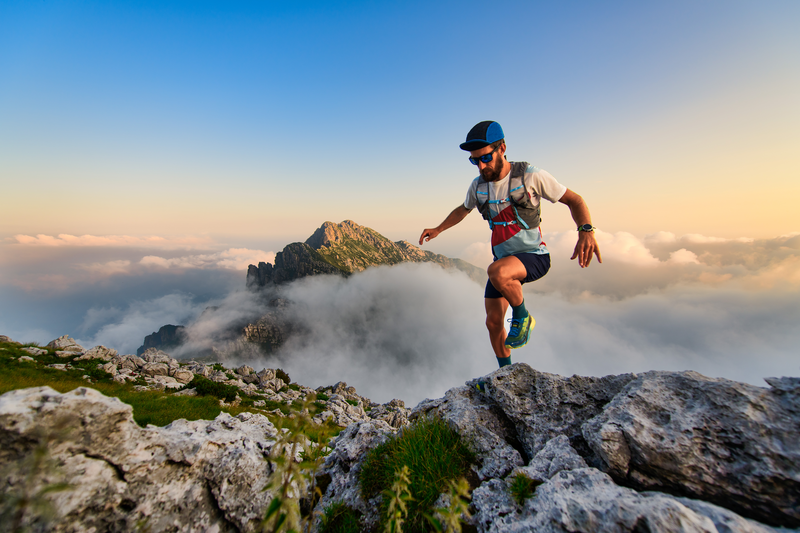
94% of researchers rate our articles as excellent or good
Learn more about the work of our research integrity team to safeguard the quality of each article we publish.
Find out more
BRIEF RESEARCH REPORT article
Front. Cell. Infect. Microbiol. , 05 April 2023
Sec. Parasite and Host
Volume 13 - 2023 | https://doi.org/10.3389/fcimb.2023.1066407
This article is part of the Research Topic Strategies in the drug discovery and development for leishmaniasis: immunomodulators, natural products, synthetic compounds, and drug repositioning View all 13 articles
Current treatment for visceral leishmaniasis is based on drugs such as pentavalent antimony and amphotericin B. However, this treatment remains mostly ineffective and expensive, resulting in several side effects and generating resistance. Apigenin, a flavonoid present in fruits and vegetables, has demonstrated several biological functions. In the present study, we observed a concentration-dependent inhibition of the L. infantum promastigote in the presence of apigenin, exhibiting an IC50 value of 29.9 µM. Its effect was also evaluated in L. infantum-infected murine peritoneal macrophages, presenting an C50 value against intracellular amastigotes of 2.3 µM and a selectivity index of 34.3. In a murine model of visceral leishmaniasis, the in vivo effect of apigenin was measured using short-term and long-term treatment schemes. Treatment with apigenin demonstrated 99.7% and 94% reductions in the liver parasite load in the short-term and long-term treatment schemes, respectively. Furthermore, no alterations in serological and hematological parameters were observed. Taken together, these results suggest that apigenin is a potential candidate for visceral leishmaniasis chemotherapy by oral administration.
Leishmaniasis is a neglected tropical disease that is endemic in 98 countries and present in 200 territories, and 12 million people are affected worldwide. Visceral leishmaniasis is a form of this disease with a 95% fatality rate in untreated cases, and it is estimated that 30,000 new cases occur annually (Ruiz-Postigo et al., 2021). This disease has a high prevalence in Brazil, where 96% of cases are reported (Pan American Health Organization, 2018).
In endemic areas of visceral leishmaniasis, a rise in Leishmania–HIV coinfection has been observed. Visceral leishmaniasis accelerates HIV replication and progression, and HIV can influence the disease’s epidemiology, clinical manifestations, and course. Additionally, Leishmania–HIV coinfected patients are more difficult to treat, and they do not respond well to standard treatments, facing more frequent and more severe side effects and higher risks of disease recurrence and death (Alvar et al., 2008; van Griensven and Diro, 2012; DNDi, 2022).
Current treatment for visceral leishmaniasis is based on drugs such as pentavalent antimonials, which have been used as first-line agents for many years, and amphotericin B deoxycholate and lipid formulation amphotericin B (Uliana et al., 2017). These drugs are administered intramuscularly, leading to long-established administration, high cost, and high toxicity. Additionally, pentavalent antimonials are becoming increasingly ineffective due to resistance, and liposomal amphotericin B is a high-cost formulation, making this drug prohibitive in several endemic regions (Uliana et al., 2017; Zulfiqar et al., 2017). Miltefosine has emerged as an alternative treatment for visceral leishmaniasis; however, it is expensive and teratogenic, and its use is not licensed worldwide (Rijal et al., 2013). Therefore, it is necessary to search for new alternatives for the treatment of visceral leishmaniasis. In this context, several natural products have been demonstrated to have antileishmanial activities (Gervazoni et al., 2020).
We had previously demonstrated that apigenin, a flavonoid present in common fruits and vegetables, such as parsley, lemons and berries, has antiparasitic activity in vitroin vitro against Leishmania amazonensis-infected macrophages and can be used as an oral treatment in an experimental model of cutaneous leishmaniasis caused by L. amazonensis (Fonseca-Silva et al., 2016; Emiliano and Almeida-Amaral, 2018). In the present study, we investigated the in vitro activity of apigenin against promastigote and intracellular amastigotes of Leishmania infantum, the etiological agent of visceral leishmaniasis in the New World, and its in vivo activity in an experimental model of visceral leishmaniasis caused by L. infantum.
Apigenin (molecular formula: C15H10O5; molecular weight: 270.24 g/mol; purity ≥95%; lot WE445301/1) and other reagents were obtained from Merck KGaA. Apigenin was diluted in dimethyl sulfoxide (DMSO) such that the solvent concentration did not exceed 0.2% in the final solution and added to the culture medium. Endotoxin-free sterile disposables were used in all experiments. The chemical structure of apigenin is presented in Supplementary Figure 1.
This study was performed in strict accordance with the recommendations of the Guide for the Care and Use of Laboratory Animals of the Brazilian National Council of Animal Experimentation (CONCEA). The protocol was approved by the Committee on the Ethics of Animal Experiments of the Instituto Oswaldo Cruz (CEUA-IOC, License Number: L-11/2017 A2).
In this study, we used a strain of L. infantum (MHOM/MA/67/ITMAP263). L. infantum promastigotes were cultivated at 26°C in Schneider’s Drosophila medium (pH 6.9) supplemented with 20% fetal bovine serum (v/v), 100 μg/mL streptomycin, and 100 U/mL penicillin. Parasite maintenance was promoted by culture passage every 3 days. Female BALB/c mice (8-10 weeks; provided by the Instituto Ciencias e Tecnologia em Biomodelos, ICTB/FIOCRUZ) were used in this study. All animals were bred and maintained at the Instituto Oswaldo Cruz according to the Guide for the Care and Use of Laboratory Animals of the Brazilian National Council of Animal Experimentation (CONCEA).
L. infantum promastigotes (1.0 x 106 cell/mL) were incubated in the absence (DMSO 0.2%) or presence of different concentrations of apigenin (12 µM, 24 µM, 36 µM, 48 µM, 60 µM, 72 µM, 80 µM and 96 µM) for 72 h. Cellular proliferation was determined by the Alamar Blue assay. Fluorescence was monitored at excitation and emission wavelengths of 560 and 590 nm, respectively, using a spectrofluorometer. The 50% inhibitory concentration (IC50) was determined by logarithmic regression analysis using GraphPad Prism 7 (GraphPad Software, La Jolla, CA, USA). The experiments were performed in triplicate.
Peritoneal macrophages were collected from BALB/c mice in RPMI 1640 medium and plated at 2.0 x 106 macrophages/mL (0.4 mL/well) onto Lab-Tek eight-chamber slides for 1 h at 37°C in an atmosphere of 5% CO2. Stationary-phase L. infantum promastigotes were washed with PBS, counted in a Neubauer chamber, and added to macrophages at a multiplicity of infection (MOI) of 5:1 for 5 h at 37°C in an atmosphere of 5% CO2. Then, 2% heat-inactivated horse serum (v/v) was added for 18 hours (Inacio et al., 2019). After this, successive washes with RPMI 1640 medium were performed to remove free parasites. Then, L. infantum-infected macrophages were incubated in the absence (DMSO 0.2%) or presence of different concentrations of apigenin (3 µM, 6 µM, 12 µM and 24 µM) for 72 hours and stained using Instant Prov (Newprov, Curitiba/Brazil). The percentage of infected macrophages was determined by light microscopy, and at least 200 cells on each coverslip were randomly counted in duplicate. The results are expressed as the infection index (% infected macrophages x number of amastigotes/total number of macrophages). The IC50 was determined by logarithmic regression analysis using GraphPad Prism 7. The selectivity index (SI) was obtained as murine peritoneal macrophage CC50/intracellular amastigote IC50. The macrophage CC50 for apigenin is 78.7 µM (Fonseca-Silva et al., 2016).
BALB/c mice (five animals per group) were maintained under specific pathogen-free conditions. The animals were infected via the peritoneum with 100 µL of stationary-phase L. infantum promastigotes at a concentration of 1.0 x 108 cells/ml as described (Cunha-Junior et al., 2016). At 7 days post-infection, the animals were separated into three different groups (control group, apigenin-treated group, and positive control group). The positive control group was treated using meglumine antimoniate. At the end of the treatment, mice were euthanized, and the livers were removed, weighed, and macerated in Schneider’s medium with 20% FBS for analysis of the parasite load by limiting dilution assay (LDA). The number of viable parasites in each liver was estimated from the highest dilution at which promastigotes could be grown after 7 days of incubation at 26°C (Inacio et al., 2019).
In the short-term therapeutic treatment scheme, the infected mice, divided into three groups, were treated with vehicle (DMSO; 0.2% v/v), which was incorporated in an oral suspension and administered orally by gavage twice per day (control group); apigenin (1 mg/kg diluted in DMSO 0.2% v/v), which was incorporated in an oral suspension and administered orally by gavage twice per day, reaching a maximum daily dose of 2 mg/kg/day (apigenin-treated group); and meglumine antimoniate, which was administered intramuscularly once per day at a dose of 200 mg Sb5+/kg/day (positive control group). These treatments started 7 days post-infection and lasted 5 days. At the end of the experiment (Day 14), the animals were euthanized, and the liver was obtained for analysis of the parasite load by limiting dilution assay (LDA) as described above.
In the long-term therapeutic treatment scheme, L. infantum-infected mice were divided into three groups and treated with vehicle (DMSO 0.2% v/v), which was incorporated in an oral suspension and administered orally by gavage twice per day (control group); apigenin (1 mg/kg diluted in DMSO 0.2% v/v), which was incorporated in an oral suspension and administered orally twice per day by gavage, reaching a maximum daily dose of 2 mg/kg/day (apigenin-treated group); and meglumine antimoniate, which was administered intramuscularly once per day at a dose of 100 mg Sb5+/kg/day (positive control group). The animals were treated for 5 consecutive days beginning 7 days post-infection. At 18 days after treatment (Day 30), mice were euthanized, and the livers were obtained for analysis of the parasite load by limiting dilution assay (LDA) as described above.
Before euthanasia, BALB/c mice were anesthetized with a solution of ketamine (200 mg/kg) and xylazine (16 mg/kg) administered intraperitoneally. Blood was collected (1 mL) via cardiac puncture and distributed in EDTA-containing microtubes for hematological analysis or centrifuged to obtain serum. Both serum (toxicology markers) and total blood (hematological parameters) from the infected BALB/c mice treated as described above were measured by Technological Platforms Network -FIOCRUZ, Platforms of Clinical Analysis in Laboratory Animals - RPT12C.
The data were analyzed using the Mann−Whitney test or one-way analysis of variance (ANOVA) followed by Tukey’s post-test in GraphPad Prism 7 (GraphPad Software). The results were considered significant at p ≤ 0.05. The data are expressed as the means ± standard errors.
To evaluate the in vitro effect of apigenin, promastigote forms and intracellular amastigote forms of L. infantum were used. Promastigotes were incubated in the absence or presence of different concentrations of apigenin (12-96 µM) for 72 h (Figure 1). Apigenin inhibited the cellular proliferation of L. infantum in a concentration-dependent manner, reaching 94.6% inhibition at the highest concentration (96 µM) with an IC50 value of 29.9 µM.
Figure 1 Effect of apigenin on L. infantum promastigotes. L. infantum promastigotes were incubated in Schneider’s Drosophila medium in the absence or presence of increasing concentrations of apigenin (12-96 µM) for 72 h. Cellular proliferation was evaluated using the Alamar Blue assay. The values are presented as the mean ± standard error of three different experiments. * indicates a significant difference relative to the control (p < 0.001).
To determine the effect of apigenin on L. infantum intracellular amastigotes, peritoneal BALB/c mouse macrophages were infected with promastigotes of L. infantum for 5 h and then incubated with increasing concentrations of apigenin (3-24 μM) for 72 h. Apigenin was able to reduce the infection index in a concentration-dependent manner (Figure 2). The IC50 was 2.3 µM, reaching 88% inhibition at the highest concentration (24 µM). The reported CC50 value of apigenin is 78.7 µM (Fonseca-Silva et al., 2016), and the selectivity index (SI) was calculated to be 34.3.
Figure 2 Effect of apigenin on L. infantum-infected macrophages. L. infantum-infected macrophages were incubated in the absence or presence of increasing concentrations of apigenin (3-24 µM) for 72 h. In control samples (absence of apigenin), a similar volume of vehicle (0.2% DMSO) was added to the cells. The infection index was determined using light microscopy to count at least 200 macrophages in each duplicated coverslip. The values presented refer to the mean ± standard error of three different experiments. * indicates a significant difference relative to the control (p < 0.05).
According to the observed in vitro effect of apigenin, the efficacy of this molecule in vivo was evaluated using a murine model of visceral leishmaniasis using two therapeutic schemes. The first was short-term, where L. infantum-infected BALB/c mice were treated for 5 days beginning at 7 days post-infection and euthanized at 2 days post-treatment (Day 14). In the long-term therapeutic scheme, L. infantum-infected BALB/c mice were also treated for 5 days beginning at 7 days post-infection, but euthanization occurred at 18 days post-treatment (Day 30) (Le Fichoux et al., 1998).
As shown in Figure 3, during the short-term treatment, apigenin orally administered to L. infantum-infected BALB/c mice reduced the liver-parasite load, reaching 99.7% inhibition. However, we did not observe any significant differences between mice treated with apigenin and those treated with meglumine antimoniate.
Figure 3 Short-term therapeutic efficacy of apigenin against L. infantum-infected BALB/c mice. Parasitic load of L. infantum-infected BALB/c mice (5 animals per group) untreated or treated with apigenin or meglumine antimoniate. Liver parasite load was estimated by a limiting dilution assay (LDA). These data represent one independent experiment with five mice per group (n = 5). * indicates a significant difference relative to the control group (p < 0.01).
Furthermore, serological toxicology parameters, such as total levels of albumin, creatinine kinase, urea, alkaline phosphatase, alanine aminotransferase (ALT), aspartate aminotransferase (AST), cholesterol, iron, calcium, sodium, and potassium (Supplementary Table 1), and several hematological parameters (Supplementary Table 2) were evaluated, and no significant alterations were observed, suggesting a lack of toxicity.
In the long-term therapeutic scheme (Figure 4), oral administration of apigenin to L. infantum-infected BALB/c mice resulted in a 94% reduction in the liver parasite load. Furthermore, differences between the infected mice treated with apigenin and meglumine antimoniate were observed in terms of parasite load, and meglumine antimoniate reduced the liver parasite load by only 55%.
Figure 4 Long-term therapeutic efficacy of apigenin against L. infantum-infected BALB/c mice. Parasitic load of L. infantum-infected BALB/c mice (five animals per group) untreated or treated with apigenin or meglumine antimoniate. The liver parasite load was estimated by a limiting dilution assay (LDA). These data represent one independent experiment with five mice per group (n = 5). * indicates a significant difference relative to the control group (p < 0.01). # indicates a significant difference relative to the meglumine antimoniate group (positive control group) (p < 0.01).
Current visceral leishmaniasis chemotherapy has presented many problems, such as several collateral effects, increased ineffectiveness due to resistance, and high cost making these drugs unaffordable in several endemic regions. Therefore, more research is necessary to improve this drug arsenal, giving priority to compounds with greater efficacy, reduced toxicity, and better affordability. Natural products have become an interesting alternative, and flavonoids have been studied as promising compounds for leishmaniasis treatment (Fonseca-Silva et al., 2011; Inacio et al., 2012; Fonseca-Silva et al., 2013; Inacio et al., 2013; Ndjonka et al., 2013; Inacio et al., 2014; Fonseca-Silva et al., 2015; Fonseca-Silva et al., 2016; Emiliano and Almeida-Amaral, 2018; Gervazoni et al., 2018; Inacio et al., 2019; Gervazoni et al., 2020). Apigenin has demonstrated a key role in the treatment of some health issues, such as diabetes, amnesia and Alzheimer’s disease, depression, insomnia, and cancer (Salehi et al., 2019). Moreover, the activity of apigenin has been described against L. donovani, T. brucei rhodesiense, T. cruzi, Encephalitozoon intestinalis and Cryptosporidium parvum (Mead and McNair, 2006; Tasdemir et al., 2006).
Cellular proliferation of the promastigote forms of L. infantum in the presence of apigenin was inhibited in a concentration-dependent manner, presenting an IC50 of 29.9 µM. A similar effect of apigenin was observed in the promastigote forms of L. amazonensis, which showed an IC50 value of 23.7 µM (Fonseca-Silva et al., 2015).
Some conditions for the eligibility of a new drug for the treatment of visceral leishmaniasis have been described. The new drug should have an IC50 less than 10 μM against intracellular amastigotes; be, at a minimum, tenfold more active against intracellular amastigotes than against mammalian cells (SI ≥ 10); be active in vivo; and cause a reduction in liver parasite load more than 70% in a relevant small animal model after at most 5 doses at 50 mg/kg, preferably when administered by the oral route once or twice per day (Pink et al., 2005; Katsuno et al., 2015).
In the present study, we demonstrated that apigenin was active against intracellular amastigotes, with an IC50 of 2.3 µM and a selectivity index of 34.3. We also observed that the treatment of BALB/c mice infected with L. infantum with apigenin (1 mg/kg twice a day, reaching a maximum daily dose of 2 mg/kg/day, orally administered) was capable of reducing the liver parasite load by greater than 70% in both therapeutic schemes (short-term and long-term). It is important to point out that the treatment of apigenin did not compromise the overall health of the infected mice, satisfying all the criteria described above. A similar effect was observed with (-)-epigallocatechin 3-O-gallate, an abundant flavonoid constituent of green tea, that had an IC50 value of 2.6 µM for intracellular amastigotes and demonstrated a reduction of the liver parasite load, reaching 98.7% inhibition at 50 mg/kg/day (Inacio et al., 2019).
Taken together, the results indicate apigenin as a new compound for the treatment of visceral leishmaniasis and support further studies to determine the ideal therapeutic and optimal drug dose regimen.
The original contributions presented in the study are included in the article/Supplementary Material. Further inquiries can be directed to the corresponding author.
The animal study was reviewed and approved by L-11/2017 A2.
Conceptualization, YE and EA-A; Methodology, YE and EA-A; Validation, YE and EA-A; Formal analysis, YE; Investigation, YE and EA-A; Data curation, YE and EA-A; Writing—original draft preparation, YE and EA-A; Writing—review and editing, EA-A; Supervision, EA-A. All authors contributed to the article and approved the submitted version.
This work was supported by Fundação Carlos Chagas Filho de Amparo a Pesquisa do Estado do Rio de Janeiro (FAPERJ), Conselho Nacional de Desenvolvimento Cientı́fico e Tecnológico (CNPq), Instituto Oswaldo Cruz (IOC), Coordenação de Aperfeiçoamento de Pessoal da Nivel Superior (CAPES) and Fundação Oswaldo Cruz (FIOCRUZ). YSSE received a scholarship from CAPES, and EEA-A is the recipient of a research scholarship from Conselho Nacional de Desenvolvimento Cientı́fico e Tecnológico (CNPq). The funders had no role in the study design, data collection, analysis, decision to publish, or preparation of the manuscript.
We are grateful for the support given by Instituto Oswaldo Cruz (IOC), Conselho Nacional de Desenvolvimento Científico e Tecnológico (CNPq), Fundação Carlos Chagas Filho de Amparo a Pesquisa do Estado do Rio de Janeiro (FAPERJ) and Fundação Oswaldo Cruz (Fiocruz).
The authors declare that the research was conducted in the absence of any commercial or financial relationships that could be construed as a potential conflict of interest.
The reviewers DR and VEV declared a shared affiliation with the authors to the handling editor at the time of review.
All claims expressed in this article are solely those of the authors and do not necessarily represent those of their affiliated organizations, or those of the publisher, the editors and the reviewers. Any product that may be evaluated in this article, or claim that may be made by its manufacturer, is not guaranteed or endorsed by the publisher.
The Supplementary Material for this article can be found online at: https://www.frontiersin.org/articles/10.3389/fcimb.2023.1066407/full#supplementary-material
Alvar, J., Aparicio, P., Aseffa, A., Den Boer, M., Canavate, C., Dedet, J. P., et al. (2008). The relationship between leishmaniasis and AIDS: The second 10 years. Clin. Microbiol. Rev. 21 (2), 334–359. doi: 10.1128/CMR.00061-07
Cunha-Junior, E. F., Martins, T. M., Canto-Cavalheiro, M. M., Marques, P. R., Portari, E. A., Coelho, M. G., et al. (2016). Preclinical studies evaluating subacute toxicity and therapeutic efficacy of LQB-118 in experimental visceral leishmaniasis. Antimicrob. Agents Chemother. 60 (6), 3794–3801. doi: 10.1128/AAC.01787-15
DNDi (2022). “DNDi 2021 annual report - unlocking the promise of medical innovation for all,” in Anual report. (Geneva, Switzerland: Drugs for Neglected Diseases initiative)
Emiliano, Y. S. S., Almeida-Amaral, E. E. (2018). Efficacy of apigenin and miltefosine combination therapy against experimental cutaneous leishmaniasis. J. Nat. Prod. 81 (8), 1910–1913. doi: 10.1021/acs.jnatprod.8b00356
Fonseca-Silva, F., Canto-Cavalheiro, M. M., Menna-Barreto, R. F., Almeida-Amaral, E. E. (2015). Effect of apigenin on leishmania amazonensis is associated with reactive oxygen species production followed by mitochondrial dysfunction. J. Nat. Prod. 78 (4), 880–884. doi: 10.1021/acs.jnatprod.5b00011
Fonseca-Silva, F., Inacio, J. D., Canto-Cavalheiro, M. M., Almeida-Amaral, E. E. (2011). Reactive oxygen species production and mitochondrial dysfunction contribute to quercetin induced death in leishmania amazonensis. PloS One 6 (2), e14666. doi: 10.1371/journal.pone.0014666
Fonseca-Silva, F., Inacio, J. D., Canto-Cavalheiro, M. M., Almeida-Amaral, E. E. (2013). Reactive oxygen species production by quercetin causes the death of leishmania amazonensis intracellular amastigotes. J. Nat. Prod. 76 (8), 1505–1508. doi: 10.1021/np400193m
Fonseca-Silva, F., Inacio, J. D., Canto-Cavalheiro, M. M., Menna-Barreto, R. F., Almeida-Amaral, E. E. (2016). Oral efficacy of apigenin against cutaneous leishmaniasis: Involvement of reactive oxygen species and autophagy as a mechanism of action. PloS Negl. Trop. Dis. 10 (2), e0004442. doi: 10.1371/journal.pntd.0004442
Gervazoni, L. F. O., Barcellos, G. B., Ferreira-Paes, T., Almeida-Amaral, E. E. (2020). Use of natural products in leishmaniasis chemotherapy: An overview. Front. Chem. 8 (1031). doi: 10.3389/fchem.2020.579891
Gervazoni, L. F. O., Goncalves-Ozorio, G., Almeida-Amaral, E. E. (2018). 2’-hydroxyflavanone activity in vitro and in vivo against wild-type and antimony-resistant leishmania amazonensis. PloS Negl. Trop. Dis. 12 (12), e0006930. doi: 10.1371/journal.pntd.0006930
Inacio, J. D., Canto-Cavalheiro, M. M., Almeida-Amaral, E. E. (2013). In vitro and in vivo effects of (-)-epigallocatechin 3-o-gallate on leishmania amazonensis. J. Nat. Prod. 76 (10), 1993–1996. doi: 10.1021/np400624d
Inacio, J. D., Canto-Cavalheiro, M. M., Menna-Barreto, R. F., Almeida-Amaral, E. E. (2012). Mitochondrial damage contribute to epigallocatechin-3-gallate induced death in leishmania amazonensis. Exp. Parasitol. 132 (2), 151–155. doi: 10.1016/j.exppara.2012.06.008
Inacio, J. D. F., Fonseca, M. S., Almeida-Amaral, E. E. (2019). (-)-Epigallocatechin 3-O-Gallate as a new approach for the treatment of visceral leishmaniasis. J. Nat. Prod. 82 (9), 2664–2667. doi: 10.1021/acs.jnatprod.9b00632
Inacio, J. D., Gervazoni, L., Canto-Cavalheiro, M. M., Almeida-Amaral, E. E. (2014). The effect of (-)-epigallocatechin 3-O–gallate in vitro and in vivo in leishmania braziliensis: Involvement of reactive oxygen species as a mechanism of action. PloS Negl. Trop. Dis. 8 (8), e3093. doi: 10.1371/journal.pntd.0003093
Katsuno, K., Burrows, J. N., Duncan, K., van Huijsduijnen, R. H., Kaneko, T., Kita, K., et al. (2015). Hit and lead criteria in drug discovery for infectious diseases of the developing world. Nat. Rev. Drug Discov. 14, 751. doi: 10.1038/nrd4683
Le Fichoux, Y., Rousseau, D., Ferrua, B., Ruette, S., Lelievre, A., Grousson, D., et al. (1998). Short- and long-term efficacy of hexadecylphosphocholine against established leishmania infantum infection in BALB/c mice. Antimicrob. Agents Chemother. 42 (3), 654–658. doi: 10.1128/AAC.42.3.654
Mead, J., McNair, N. (2006). Antiparasitic activity of flavonoids and isoflavones against cryptosporidium parvum and encephalitozoon intestinalis. FEMS Microbiol. Lett. 259 (1), 153–157. doi: 10.1111/j.1574-6968.2006.00263.x
Ndjonka, D., Rapado, L. N., Silber, A. M., Liebau, E., Wrenger, C. (2013). Natural products as a source for treating neglected parasitic diseases. Int. J. Mol. Sci. 14 (2), 3395–3439. doi: 10.3390/ijms14023395
Pan American Health Organization (2018). Leishmaniasis: Epidemiological report in the americas (Washington: Pan American Health Organization).
Pink, R., Hudson, A., Mouries, M. A., Bendig, M. (2005). Opportunities and challenges in antiparasitic drug discovery. Nat. Rev. Drug Discov. 4 (9), 727–740. doi: 10.1038/nrd1824
Rijal, S., Ostyn, B., Uranw, S., Rai, K., Bhattarai, N. R., Dorlo, T. P., et al. (2013). Increasing failure of miltefosine in the treatment of kala-azar in Nepal and the potential role of parasite drug resistance, reinfection, or noncompliance. Clin. Infect. Dis. 56 (11), 1530–1538. doi: 10.1093/cid/cit102
Ruiz-Postigo, J. A., Jain, S., Mikhailov, A., Maia-Elkhoury, A. N., Valadas, S., Warusavithana, S., et al. (2021). “Global leishmaniasis surveillance: 2019–2020, a baseline for the 2030 roadmap,” in Weekly epidemiological record (Geneva, Switzerland: WHO). C.o.N.T. Diseases.
Salehi, B., Venditti, A., Sharifi-Rad, M., Kregiel, D., Sharifi-Rad, J., Durazzo, A., et al. (2019). The therapeutic potential of apigenin. Int. J. Mol. Sci. 20 (6), 1–26. doi: 10.3390/ijms20061305
Tasdemir, D., Kaiser, M., Brun, R., Yardley, V., Schmidt, T. J., Tosun, F., et al. (2006). Antitrypanosomal and antileishmanial activities of flavonoids and their analogues: In vitro, in vivo, structure-activity relationship, and quantitative structure-activity relationship studies. Antimicrob. Agents Chemother. 50 (4), 1352–1364. doi: 10.1128/AAC.50.4.1352-1364.2006
Uliana, S. R., Trinconi, C. T., Coelho, A. C. (2017). Chemotherapy of leishmaniasis: Present challenges. Parasitology 145, 1–17. doi: 10.1017/S0031182016002523
van Griensven, J., Diro, E. (2012). Visceral leishmaniasis. Infect. Dis. Clin. North Am. 26 (2), 309–322. doi: 10.1016/j.idc.2012.03.005
Keywords: natural products, oral treatment, flavonoid, short-term, long-term, in vivo, in vitro, leishmaniasis chemotherapy
Citation: Emiliano YSS and Almeida-Amaral EE (2023) Apigenin is a promising molecule for treatment of visceral leishmaniasis. Front. Cell. Infect. Microbiol. 13:1066407. doi: 10.3389/fcimb.2023.1066407
Received: 10 October 2022; Accepted: 07 March 2023;
Published: 05 April 2023.
Edited by:
Taís Fontoura de Almeida, Federal University of Rio de Janeiro, BrazilReviewed by:
Daniela Melo Resende, Oswaldo Cruz Foundation (Fiocruz), BrazilCopyright © 2023 Emiliano and Almeida-Amaral. This is an open-access article distributed under the terms of the Creative Commons Attribution License (CC BY). The use, distribution or reproduction in other forums is permitted, provided the original author(s) and the copyright owner(s) are credited and that the original publication in this journal is cited, in accordance with accepted academic practice. No use, distribution or reproduction is permitted which does not comply with these terms.
*Correspondence: Elmo E. Almeida-Amaral, ZWxtb0Bpb2MuZmlvY3J1ei5icg==
Disclaimer: All claims expressed in this article are solely those of the authors and do not necessarily represent those of their affiliated organizations, or those of the publisher, the editors and the reviewers. Any product that may be evaluated in this article or claim that may be made by its manufacturer is not guaranteed or endorsed by the publisher.
Research integrity at Frontiers
Learn more about the work of our research integrity team to safeguard the quality of each article we publish.