- 1Dietary Supplements and Probiotic Research Center, Alborz University of Medical Sciences, Karaj, Iran
- 2Student Research Committee, Arak University of Medical Sciences, Arak, Iran
- 3Student Research Committee, Alborz University of Medical Sciences, Karaj, Iran
- 4Evidence-based Phytotherapy And Complementary Medicine Research Center, Alborz University of Medical Sciences, Karaj, Iran
Objective: Various studies have indicated the application of Coenzyme Q10 and probiotic bacteria such as Ligilactobacillus salivarius (L. salivarius) and Lactiplantibacillus plantarum (L. plantarum) in combating periodontal disease. Considering the positive effect of these two on oral health, and the destructive effect of S. mutans, in this study, we investigate the outcomes of the administration of probiotics and Q10 on infected HEp-2 cell viability and S. mutans adhesion in different settings.
Methods: A 3-week-old human epidermoid laryngeal (HEp-2) cell line was cultured and exposed to two different probiotics and 3 different doses of Q10 doses. Samples were contaminated by S. mutans immediately (therapeutic setting) and after 3 hours (preventive setting). Eventually, the viability of HEp-2 cells was investigated by MTT. Also, the number of adhered S. mutans was explored by direct and indirect adhesion assays.
Results: L. plantarum and L. salivarius protect epithelial cells against S. mutans in both therapeutic and preventive settings, albeit not fully. In contrast, Q10 completely preserves the viability of infected Her HEp-2 cells at all concentrations. The effects of the coexistence of Q10 and probiotics were not quite equal, among which L. salivarius and 5 μg of Q10 form the best results. The microscopic adherence assay of S. mutans revealed that samples containing Q10 had significantly lower adhesion of probiotics and S. mutans to HEp-2 cells. Similarly, plates containing L. salivarius with 5μg or L. plantarum with 1μg Q10 or sole presence of L. salivarius had the lowest S. mutans adherence among others. Also, L. salivarius with 5μg Q10 had one of the highest probiotic adherences.
Conclusion: In conclusion, co-administration of Q10 and probiotics especially in presence of L. salivarius with 5μg Q10 could have remarkable effects on HEp-2 cell viability, S. mutans, and probiotic adherence. Nevertheless, our study, for the first time, showed that Q10 might have an anti-bacterial activity by suppressing the adhesion of tested bacteria to HEp-2 cells. This hypothesis, if correct, suggests that due to their different mechanisms, co-prescription of Q10 and probiotics may lead to better clinical responses, especially in the mentiond dose.
1 Background
The study of human microbiota is rapidly progressing; until now, probiotics have been widely used in intestinal disease treatment. However, recent studies have shown that the function of these cells in the oral cavity can also be helpful (Thaiss et al., 2016; Young et al., 2019). Different hypotheses have been proposed regarding the pathophysiology of these bacteria in preventing dental infections. One of the leading hypotheses states that these bacteria prevent the spread of infection by creating a physical and biochemical barrier against pathobionts-host attachment (Honda and Littman, 2016). Also, these bacteria strengthen the host’s immune system by creating a balance between inflammatory systems in the absence or presence of infection (Kilian, 2018). For example, studies conducted on oral streptococci have shown evidence of immune-modulatory function and reducing pro-inflammatory responses (Kilian, 2018; Silva et al., 2019; Young et al., 2019).
Considering probiotics’ benefits and limited side effects, they have been widely available to the public, especially as probiotic-enriched food, mouthwashes, toothpaste, and anti-bacterial gums (Ranadheera et al., 2010; Jose et al., 2013). Studies have shown that these methods prevent tooth decay by reducing bacterial density in the oral cavity and preventing respiratory infections, colds, and allergies (Caglar et al., 2005; Winkler et al., 2005).
Among hundreds of known probiotics, the impact of Ligilactobacillus salivarius (L. salivarius) and Lactiplantibacillus plantarum (L. plantarum) on oral health is highly discussed (Kucia et al., 2020; Nordström et al., 2021). These two are known as oral cavity normal microbiota, and studies have shown that the presence of these two probiotics could regulate the oral microbiota, especially S. mutans, and prevents periodontal disease. For example, San Miguel et al. showed that L. salivarius protects host tissues from damage caused by other immunostimulatory cells and products by reducing the innate immune response and the NF-kappaB function in epithelial cells (Cosseau et al., 2008). Also, the effect of L. plantarum on S. mutans gene expression suppresses glucan-related genes and eventually prevents biofilm formation (Lim et al., 2020).
Although investigating probiotics and their preventive-therapeutic outcomes still needs much research, the results show that using these bacteria alone or simultaneously can effectively treat or prevent oral infections (Chugh et al., 2020). Like probiotics, micronutrition, especially coenzyme Q10 (Q10), is wildly administered as a preventive or therapeutic agent in periodontal disease (Dahiya et al., 2022). Q10 is an intracellular antioxidant and one of the crucial components of the electron transport chain, which plays an essential role in ATP formation (Hargreaves et al., 2020). Studies have shown that increasing its concentration in the oral epithelium suppresses periodontal inflammation (Rasoolzadeh et al., 2022). Similar to probiotic-containing supplements, oral gels containing Q10 are available and prescribed for periodontitis treatment. Due to the human larynx epithelioma cancer (HEp-2) resemblance to human oral epithelial cells, this cell line has been used as a model of infection induction in this study. This study was designed and implemented to investigate the outcomes of the administration of probiotics and Q10 on infected HEp-2 cell viability and S. mutans adhesion in different settings.
2 Materials and methods
2.1 Bacterial strain and media
The Ligilactobacillus salivarius (DSM 20555; Germany) and Lactiplantibacillus plantarum (PTCC 1896; Iran) were cultured in De man Rogosa Sharp (MRS) broth or on MRS agar plates (Merck Co. Germany) at 37°C under anaerobic conditions (in an anaerobic jar, Oxoid Ltd, UK) for 18-20 hours and prepared in a concentration of 109 colony-forming units (CFU)/ml.
S. mutans (PTCC 1683; Iran) was purchased from the Persian Type Culture Collection (PTCC; Iran) and cultivated in mitis -salivarius -bacitracin (MSB) agar (Merck Co. Germany). The turbidity of bacterial suspension was adjusted to McFarland standard no. 1, equivalent to 109 CFU/ml. Nutrient Broth medium (NB) was used as a diluent.
2.2 Cell culture
A 3-week-old human epidermoid laryngeal (HEp-2) cell line (ATCC® CCL-23™) was used. Various studies have widely used these cells as oral epithelial cell models (Mack et al., 1999; Haeri et al., 2012; Khodaii et al., 2017). HEp-2 cells were cultured in a 75 cm2 flask. The culture media was Eagle’s Minimum Essential Medium (EMEM) (ATCC® 30-2003™), fetal calf serum (Lablech 4-101-500) 10%, and Penicillin 10,000 unit/ml & streptomycin 10,000 g/ml (GIBCO 15140-122) 1%. cells were incubated at 37°C in a humidified atmosphere of 5% CO2 and 95% air and the medium was replaced by a new one every two days.
To observe the growth characteristics of HEp-2 cells, suspension of cells containing 2*104 cells/well was applied to 24-well plates. Cell number was counted every day for 6 days after plating. The doubling time of HEp-2 cells without any treatment was 47.6 h.
2.3 Addition of Q10 and probiotics
HEp-2 cells were seeded in 12 sets of 24-well plates at 2*104 cells/cm2 and cultured in the mentioned medium. After 72 h of culture, cells were washed with dulbecco’s phosphate-buffered saline (DPBS) and cultured with an antibiotic-free culture medium. After 12 h cells were washed twice with DPBS and 1 mL of culture medium without antibiotics was added.
Q10 was dissolved in distilled water and filtered. Plates’ first column did not receive any amount of Q10, in the second column, each well received 1μg Q10, and the third and fourth columns received 5μg and 10μg of Q10, respectively. The fifth and sixth columns remain empty. On the other hand, no probiotics were added to the first row but the second and third rows were treated with a 1.5 ml volume of L. salivarius and L. plantarum suspension at a concentration of 1*106 cells/ml (as a total cell count), respectively. The fourth row carries both L. salivarius and L. plantarum. Finally, all plates were incubated for 1 hour (Figure 1).
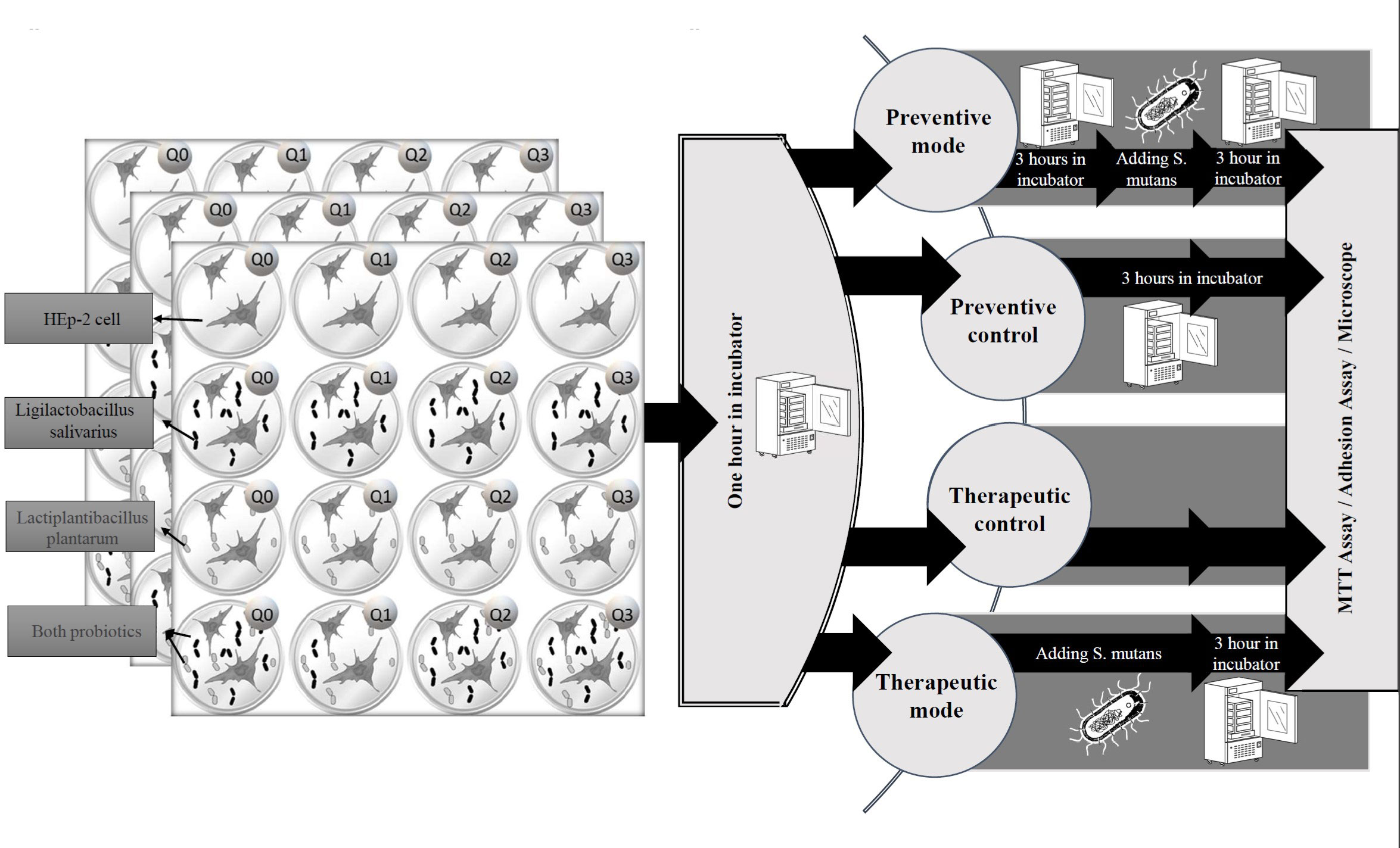
Figure 1 The experimental protocol. The study was conducted on treatment and preventive settings. In treatment mode, HEp-2 cells were infected by S. mutans immediately after administration of probiotics or Q10, and in preventive mode, the probiotics or Q10 were added three hours before infection.
2.4 Study protocol
To investigate the immediate and delayed effect of S. mutans on each combination, this experiment was established three times in four settings, containing: therapeutic mode, preventive mode, and their control. For therapeutic control adhesion, microscopic, and MTT assays were performed an hour after adding probiotics or Q10, but for preventive control, plates were incubated at the mentioned condition for 3 hours before any investigation. Each assay was carried out in duplicate and on two different occasions.
In preventive mode, three plates (each containing 16 full wells) were placed in the incubator for 3 hours at 37°C and then contaminated by live S. mutans and for therapeutic mode, an immediate infection with this bacteria was performed.
2.5 S mutans infection protocol
An overnight culture of S. mutans was centrifuged, and the growth medium was removed. The bacterial pellet was washed with DPBS and resuspended in the culture medium without antibiotics and anti-mycotic (D -MEM without P/S) to give about 106 CFU/ml. Then one milliliter of mentioned medium with 106 CFU S. mutans, with a multiplicity of infection (MOI) of 30 bacteria: 1 cell, were incubated with the pre-formed monolayer of HEp -2 cells for 3 hours at optimal conditions. The suspension with unattached S. mutans cells was removed, then the monolayers with adhered bacteria were washed three times with DPBS to remove unattached bacteria.
2.6 HEp-2 cell viability
HEp-2 cell Viability was measured by both microscopic and MTT assay. To conduct MTT colorimetric assay, a commercially available MTT assay kit (Sigma-Aldrich, UK) using a modified version of the Nga N method was used (Nga et al., 2020). MTT assay reagents were prepared as 5 mg/ml stock solutions in DPBS, sterilized by Millipore filtration, and stored in the dark. At appropriate time points, MTT stock solution (10% of total volume) was added to each well. After 3 hours of incubation at 37°C and 5% CO2, the reagent was aspirated and 200 µL of MTT solvent (Sigma-Aldrich, UK) was added to dissolve the formazan crystals. The solution was uniformly agitated on a shaker for 15 min to ensure proper dissolution. Spectrophotometer read the optical densities of formazan solutions at 570 nm on an ELISA plate reader (Tecan Sunrise, Tecan Austria) and background absorbance values were measured at 650 nm. Recorded absorbance values were assumed to be proportional to the number of viable cells in each sample well. Also, HEp-2 cell viability was investigated by counting alive HEp-2 cells in every plate under the microscope.
2.7 Indirect adhesion assay
The adhesion assay was also measured with both direct and indirect methods. In the indirect method, HEp-2 cell monolayers were washed three times with DPBS. HEp-2 cells with attached bacteria were solubilized by incubation with EDTA-Trypsin at 37° C. The number of adhered bacteria was determined by plating out of diluted bacterial suspension on blood agar or MRS agar plates depending on the bacterial strain. The developed colonies indicated adhered bacteria to the cell line (Ouwehand et al., 1999).
2.8 Direct adhesion assay
Also, the adherence assay was conducted using direct microscopy. After washing HEp-2 cell monolayers with DPBS, The remaining bacteria attached to the monolayer were stained with 1% [w/v] in water Crystal violet for 5 min and quantified using an inverted light microscope (Nikon Diaphot, x 400 magnification), according to Negri et al. (2010). The number of S. mutans and probiotics, adhering to 100 epithelial cells were counted microscopically at a magnification of A400 in 5 fields.
2.9 Statistical analysis
All the data is reported in the form of mean ± SD. The differences between other groups are measured by ANOVA and later with the Duncan test, and a p-value under 0.05 is considered significant.
3 Results
3.1 HEp-2 cell viability
3.1.1 Effect of interventions and infection on normal HEp-2 cells
Data showed that regardless of setting, the simultaneous growth of HEp-2 cells and defined interventions did not significantly differ from the growth of HEp-2 cells alone (Figure 2). Also, as demonstrated in Figure 2, there is a significant decrease in the HEp-2 survival rate after infection by S. mutans (p-value: 0.0001).
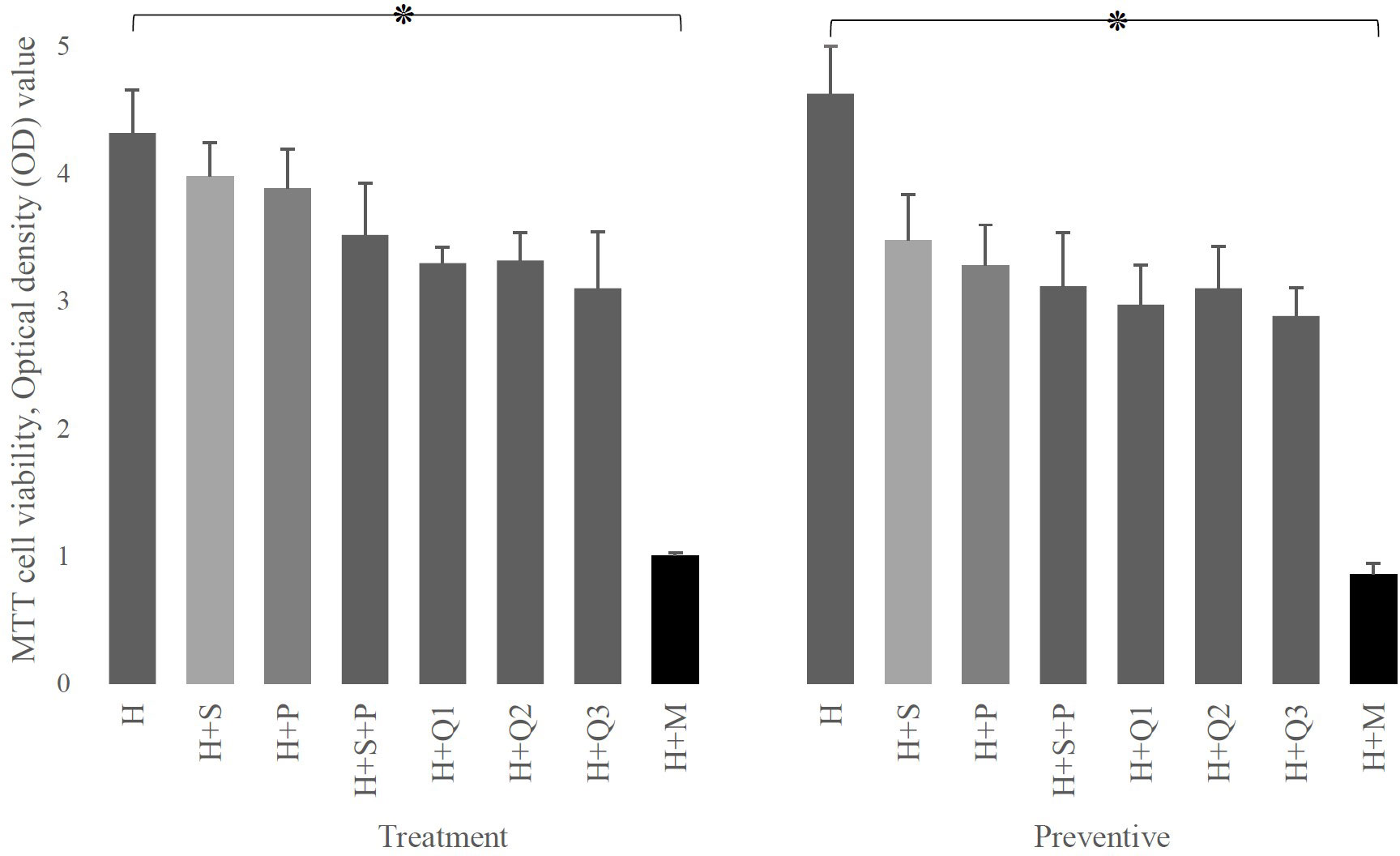
Figure 2 The effect of P (L. plantarum), S (L. salivarius), and M (S. mutans) on the viability of H (HEp-2 cell) in treatment and preventive mode. As shown, the presence of any of the substances used in this study (different doses of Q10 or probiotics) either immediately (therapeutic settings) or after 3 hours (preventive settings) by itself does not have a destructive effect on HEp-2 cells, while the presence of mutans strongly affects the survival of these cells. The values are the mean ± SD of these assays. Asterisk (*) indicates a significant difference (p-value<0.05) in the values when compared to the control.
3.1.2 The sole effect of probiotics or Q10 on infected HEp-2 cells
As shown in Figure 3, even in the presence of probiotics, infection of HEp-2 cells with S. mutans leads to a significant reduction in HEp-2 cell survival rate, except for prevention with L. salivarius (p-value: 0.0652). Albeit this decrease in HEp-2 cell viability was not as much as their absence (p-value less than 0.001).
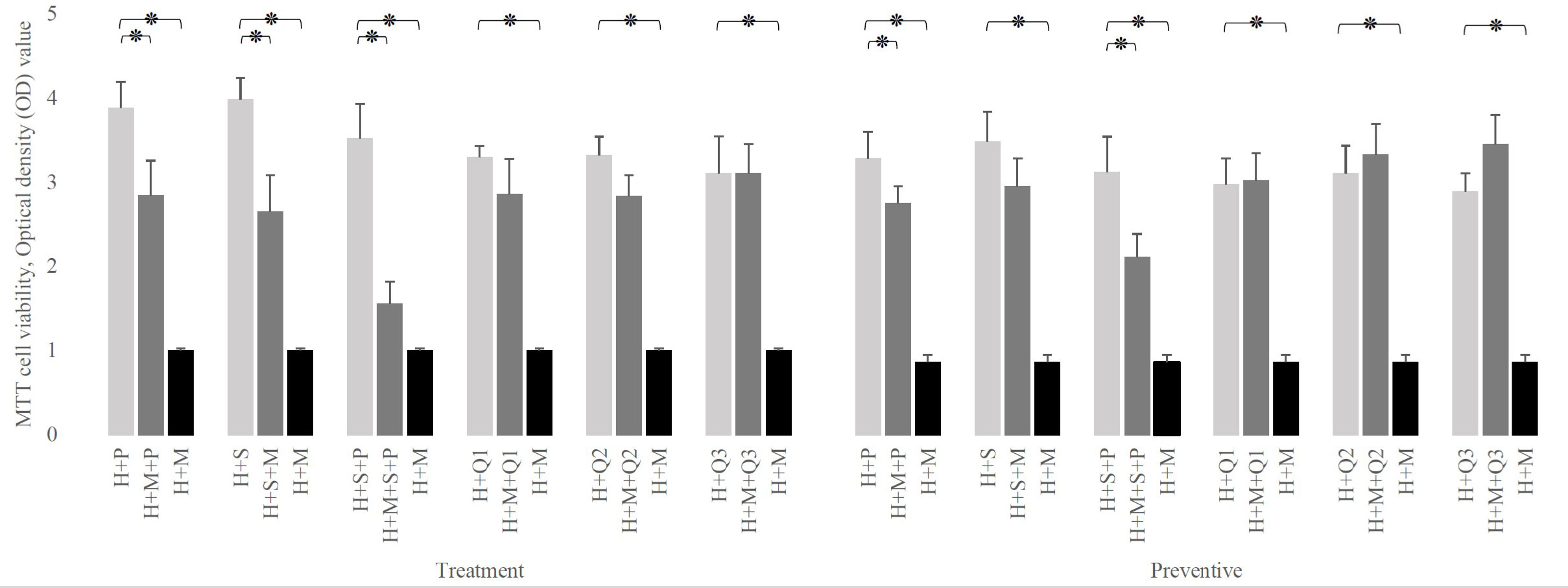
Figure 3 Comparison of H (HEp-2 cell) viability in the presence of P (L. plantarum), S (L. salivarius), and M (S. mutans) to the absence of one of them. The simulation’s presence of any doses of Q10 or L. salivarius preserves the viability of HEp-2 cells in both therapeutic and preventive settings. The values are the mean ± SD of these assays. Asterisk (*) indicates a significant difference (p-value<0.05) in the values when compared to the control.
Also, Figure 3 reveals that in the presence of Q10, at any doses and any setting, S. mutans infection does not affect the viability of HEp-2 cells (p-value > 0.05). Moreover, the simultaneous addition of Q10 and S. mutans to infected HEp-2 cells increased their survival rates from 1.007± 0.02 (S. mutans without Q10) to 2.859 ± 0.412 at 1 μg, 2.838 ± 0.241 at 5 μg, and 3.102± 0.352 at 10 μg (p-value: 0.0001). Similar data have been seen in preventive mode.
3.1.3 Effect of probiotics and Q10 co-addition on infected HEp-2 cells
As shown in Figure 4, regardless of the experimental setting, the presence of probiotics in a Q10-free well significantly increases the viability of infected HEp-2 cells compared to their absence. in the therapeutic setting, this increment was from 1.007± 0.02 to 2.843 ± 0.412 (in the presence of L. plantarum), 2.654 ± 0.432 (in the presence of L. salivarius), and 1.557± 0.265 (in presence of both probiotics). Similarly, in the preventive setting, it raises from 0.865± 0.08 to 2.749± 0.204, 2.954± 0.328, and 2.111± 0.275, in the presence of L. plantarum, L. salivarius, and both, respectively.
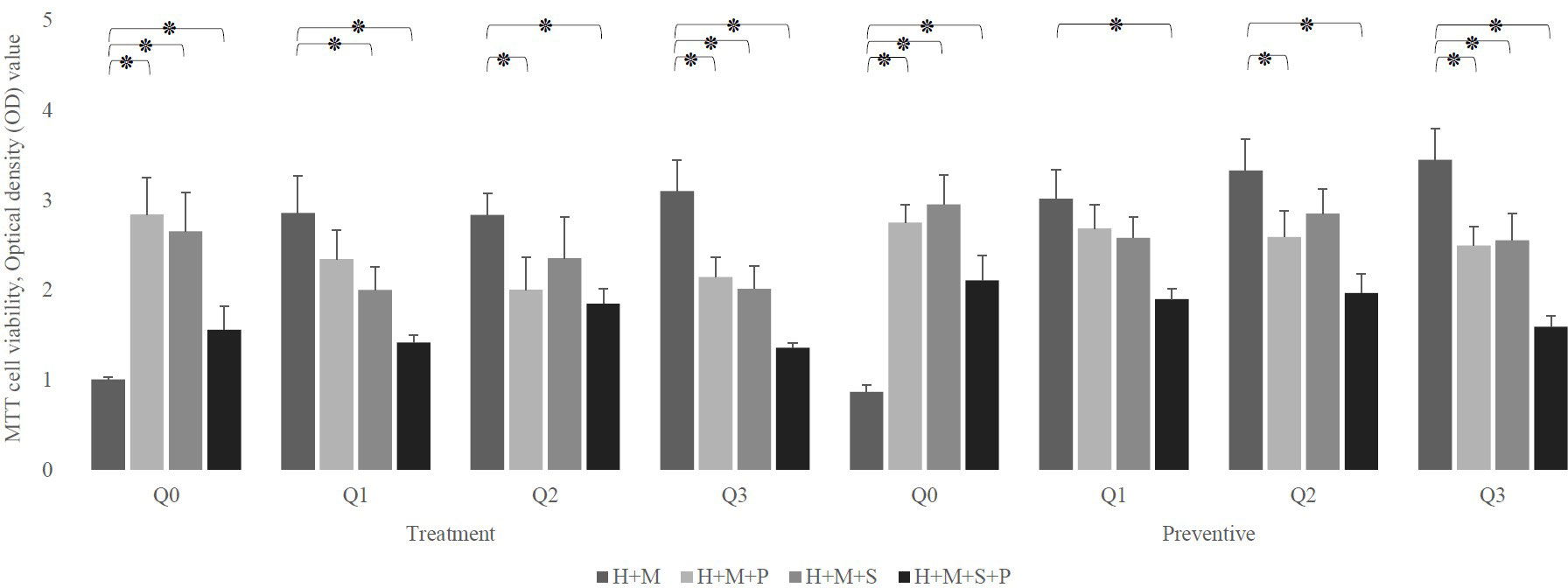
Figure 4 The effect of probiotics (P, L. plantarum; S, L. salivarius) on the viability of infected H (HEp-2 cell) treated with Q10 (Q0, absence Q10; Q1, 1μg Q10; Q2, 5μg Q10; and Q3, 10μg Q10) in the treatment and preventive mode. The existence of probiotics in the absence of Q10 preserves the viability of HEp-2 cells compared to the absence of probiotics. On the other hand, the addition of probiotics to a Q10-contained environment decreases the infected HEp-2 cells’ viability, regardless of setting, except for the coexistence of 1μg Q10 with L. plantarum and 5μg Q10 with L. salivarius in both settings and prevention with 1μg Q10 with L. salivarius. The values are the mean ± SD of these assays. Asterisk (*) indicates a significant difference (p-value<0.05) in the values when compared to the control.
Also, Figure 4, demonstrates that in both therapeutic and preventive modes, the addition of probiotics to Q10-contained wells reduces the viability of infected HEp-2 cells except in 5 conditions: the prevention and treatment of infected HEp-2 cells with 1 μg of Q10 and L. plantarum (p-value:0.2397 and 0.1625, respectively), the prevention and treatment of infected HEp-2 cells with 5 μg of Q10 and L. salivarius (p-value: 0.1364 and 0.1844, respectively) and prevention of S. mutans infection with 1 μg of Q10 and L. salivarius (p-value: 0.1276).
Despite the fact that all concentrations of Q10 preserve the viability of infected in absence of probiotics, (p-value less than 0.0001) our preventive data show that the co-addition of Q10 to probiotic-rich environments not only does not change the viability of infected cells, but also the copresence of 10 μg of Q10 with L. plantarum and L. salivarius in comparison to the coexistence of both probiotics decrease the viability of infected HEp-2 cells from 2.111± 0.275 to 1.596± 0.12 (p-value: 0.0410). Nevertheless, the therapeutic data demonstrated that the simultaneous addition of Q10 and probiotics to S. mutans-infected HEp-2 cells does not change the viability compared to the sole existence of probiotics unless in the presence of 5 μg of Q10 with L. plantarum (p-value=0.0578) (Figure 5). Also, the results of the microscopic counting of live HEp-2 are summarized in Table 1.
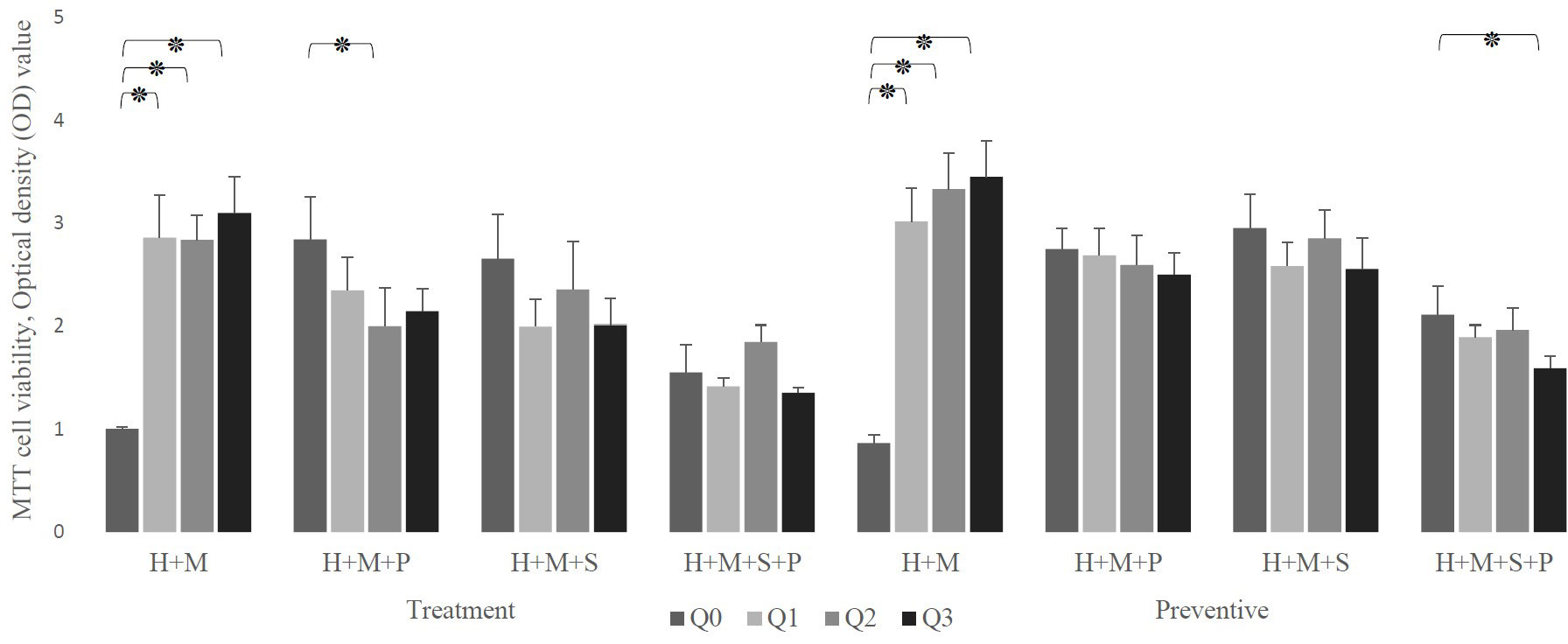
Figure 5 The effect of the presence of Q10 on the viability of infected H (HEp-2 cell) treated with probiotics (P, L. plantarum; S, L. salivarius) in the treatment and preventive mode. The presence of Q10 at all doses leads to the prevention and treatment of HEp-2 cells. Also, co-treatment of these cells with Q10 and probiotics at some doses and prevention at all doses preserves their viability. The values are the mean ± SD of these assays. Asterisk (*) indicates a significant difference (p-value<0.05) in the values when compared to the control. (Q0, absence Q10; Q1, 1μg Q10; Q2, 5μg Q10; and Q3, 10μg Q10).
3.2 Adhesion to HEp-2 cells
3.2.1 S. mutans adherence
Counting the mean number of adhered bacteria to 100 HEp-2 cells, revealed that aside from the effect of probiotics, the sole presence of Q10 in the HEp-2 cell culture medium decreased the number of HEp-2 cell-adhered S. mutans from 676 (in absence of any probiotics or Q10) to 633, 440, and 241 in presence of 1, 5, and 10 μg of Q10.
Also, the addition of probiotics without Q10 has similar results, On average, in absence of any substance, 676 bacteria adhered to each HEp-2 cell, but the presence of L. salivarius and L. plantarum decrease it to 328 and 454 bacteria per cell.
Our data showed that the co-addition of Q10 and probiotics compare to their sole existence vastly reduces the number of adhered S. mutans. The best results have been shown in the presence of L. plantarum and 1μg of Q10 (160 bacteria per HEp-2 cell), L. plantarum and 10μg of Q10 (150 bacteria per HEp-2 cell), and L. salivarius and 5μg of Q10 (96 bacteria per HEp-2 cell) (Figure 6).
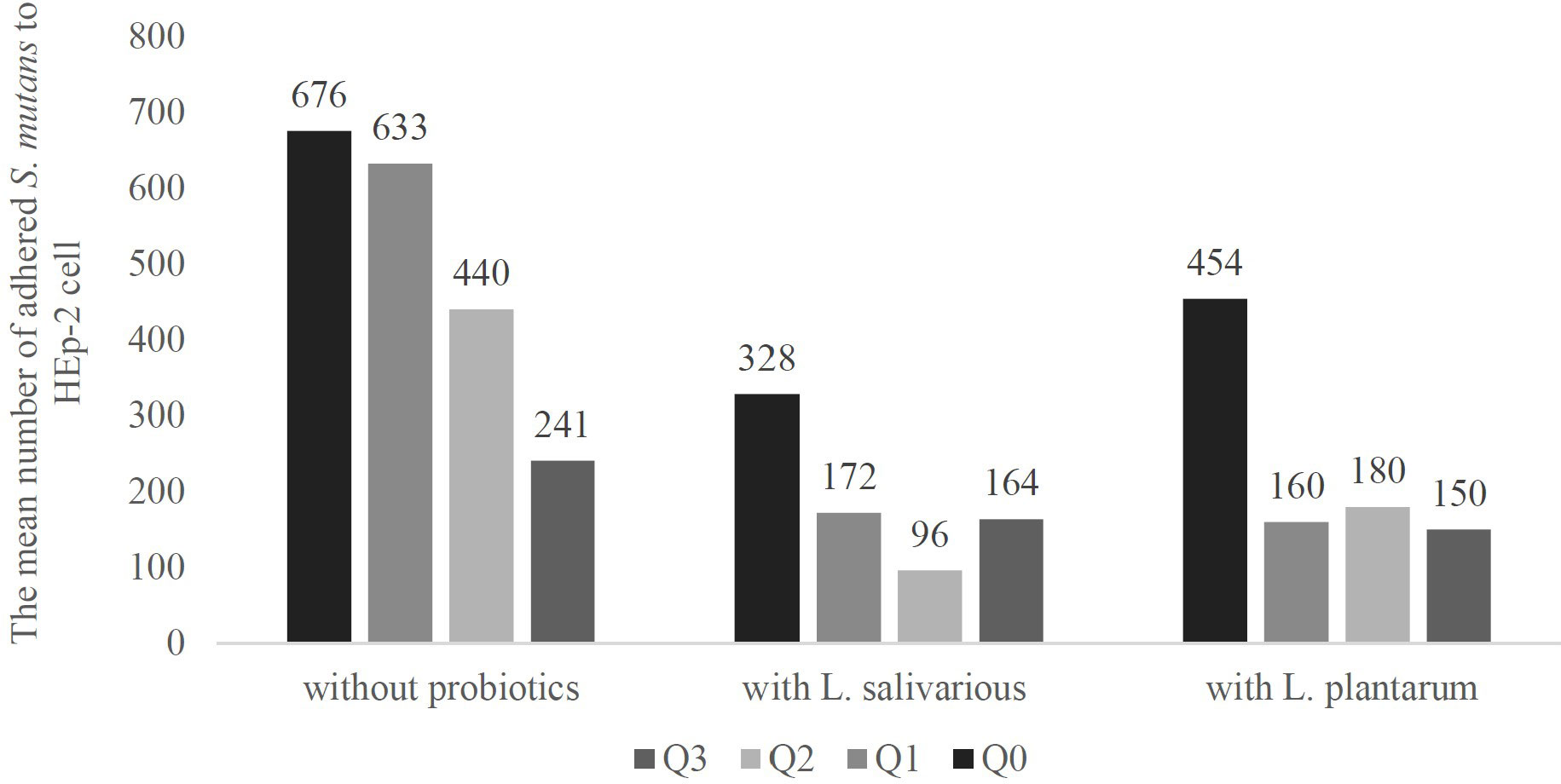
Figure 6 The adherence of S. mutans to in presence and absence of probiotics and Q10. S. mutants adhesion is an important step in the pathophysiology of various diseases, and higher adherence may lead to lower viability of HEp-2 cells and other dental complications. The adherence was measured using a microscope and observing 100 Hep-2 cells in 5 fields. The results indicate the mean number of adhered S. mutans. Q0, absence Q10; Q1, 1μg Q10; Q2, 5μg Q10; and Q3, 10μg Q10.
The indirect method results (Table 2) showed that preseance of L. plantarum decreases the number of cell-attached S. mutans from 105 CFU to 104 CFU. However, the cocontaminant addition of probiotics with any dose of Q10 has extensive impact. The top results were seen in preseance of L. salivarius with 5 μg of Q10 (102 CFU).
3.2.2 Probiotic adherence
Table 3, demonstrating the mean number of probiotic bacteria adhering to each epithelial cell, showed that on one hand, L. salivarius has a higher adherence rate than L. plantarum (198 vs. 140), and on the other hand, the adherence of probiotics to HEp-2 cells decreases in the presence of S. mutans. This reduction was from 140 to 120 in the presence of L. plantarum and from 198 to 124 in the existence of L. salivarius. As a matter of fact, in the sole presence of S. mutans the adherence rate of L. salivarius was almost the same as L. plantarum.
Also, our data revealed that Q10 decreases the probiotics adherence in all concentrations. In absence of Q10, an average of 140 L. plantarum bacteria were attached to each HEp-2 cell, but adding 1, 5, and 10 μg Q10 reduce it to 128, 94, and 94 bacteria, respectively. Likewise, 198 L. salivarius bacteria adhered to each epithelial cell, and the addition of 1, 5, and 10 μg of Q10 decrease it to 93, 120, and 80 cells.
As expected, the co-presence of Q10 and S. mutans compared to the sole presence of S. mutans lowers the number of adhered probiotics. However, the co-addition of L. salivarius and 5μg Q10, L. plantarum, and 10 μg Q10 almost preserve the probiotic adhesion compared to the absence of Q10.
4 Discussion
Our current in vitro study reveals that L. plantarum and L. salivarius protect epithelial cells against S. mutans in both therapeutic and preventive settings, although not completely. In contrast, Q10, at all concentrations, entirely preserves the viability of infected HEp-2 cells. The effect of the co-presence of Q10 and probiotics was contrasting. Among them, L. salivarius and 5μg Q10 form the best results. The viability of infected HEp-2 cells entirely preserves in the presence of this combination in both therapeutic and preventive settings. Also, in their presence, we witnessed the lowest S. mutans adherence and complete prevention of S. mutans’ effect in reducing the number of attached probiotics.
Our data agree with numerous in vitro and in vivo studies that suggest using one of these two probiotics against S. mutans (Caglar et al., 2005; Bonifait et al., 2009; Ogawa et al., 2011; Dodoo et al., 2020). Probiotic bacteria combat periodontal disease with different mechanisms. First of all, they affect both oral microbiota and immune responses; in this regard, a study by Mehrabani et al. showed that using probiotics as a dietary supplement, local treatment, or therapeutic alternative to antibiotic treatment is a promising way to boost the host immune system and decrease pathobionts adherence (Mehrabani Natanzi et al., 2018). Second, some probiotics can create a biofilm that replaces biofilm-growing bacteria such as S. mutans. Studies have suggested different probiotics with this effect, but L. plantarum and L. salivarius have had the most promising impacts on S. mutans and related diseases such as halitosis, periodontitis, and plaque. For instance, Lim et al. showed that L. plantarum could inhibit biofilm formation and glucan-related gene expression levels in S. mutans. Therefore it can be used as a biofilm inhibitor and an oral probiotic in functional foods (Lim et al., 2020). Also, Ogawa et al. demonstrated that L. salivarius inhibits biofilm formation by streptococci in the in vitro assay (Ogawa et al., 2011). Third, it has been suggested that these probiotics and S. mutans begin to compete for adhesions to HEp-2 cells, and as a result of this competition, fewer S. mutans can attach to target cells, leading to a significant reduction in the pathogenicity of these bacteria (Keller et al., 2011; Lee et al., 2019). In support of this hypothesis, our microscopic study clearly showed that increasing L. plantarum and L. salivarius adhesion leads to a lower adhesion rate of S. mutans; thus, HEp-2 cells’ survival rate improves. Nowadays, this is widely used in transforming and preserving normal microbiota.
Although Q10 is known as an intracellular antioxidant, studies have shown that local Q10 therapy is promising. In this regard, a double-blind study conducted by Matsumura et al. reported that oral hygiene combined with Q10 therapy could improve treatment results in certain patients with periodontitis (Matsumura et al., 1973). Also, Hanioka et al. observed that patients who received topical Q10 with subgingival debridement showed significant improvements in the plaque index and gingival index compared to patients who received only subgingival debridement (Hanioka et al., 1994). Similarly, Manthena et al. noted a significant reduction in gingival inflammation in the Q10 group compared to patients who received only scaling and root planning (Manthena et al., 2015).
However, the story does not end here; studies revealed that the Q10 activity is not limited to treatment but also may play a role in the etiology of periodontal disease. For example, Studies by Littaru, Henson, and Nakamura support that periodontal disease is significantly associated with Q10 deficiency (Littarru et al., 1971; Nakamura et al., 1974; Hansen et al., 1976). Likewise, our study showed that HEp-2 cell viability increased in the presence of different concentrations of Q10. Also, HEp-2 cells exposed to Q10 3 hours before infection had higher viability.
Different studies suggest various mechanisms for this co-enzyme in treating periodontal disease, including stimulating the immune system, increasing oxygen supply, reducing inflammation, or acting as an antioxidant on HEp-2 cells. For example, Hanioka et al. suggested that inflamed cells need higher oxygenation, so the administration of Q10 improves oxygen utilization and, thus, free radicals in inflamed gingival tissue. They concluded that the antioxidant activity of Q10 is the main mechanism of tissue healing (Hanioka et al., 1994). In contrast, Denny et al. assessed the antioxidant and anti-inflammatory effects of Q10 in 10 non-smoking periodontally healthy volunteers. They also demonstrated reductions in gingival bleeding after 28 days of supplementation but found no changes in gingival crevicular fluid total antioxidant capacity, indicating that the potential utility of Q10 may be independent of its antioxidant activity (Denny et al., 1999).
The anti-bacterial efficacy of this co-enzyme has been repeatedly reported in numerous studies (Nakamura et al., 1974; Wilkinson et al., 1975). For instance, McRee et al. report that oral administration of Q10 significantly reduced subgingival bacteria (MCREE et al., 1993); also, Block et al. found that 25 mg/kg of Q10 protects mice from some experimental bacterial infections (Block et al., 1978). These in vivo studies suggest that Q10 administration stimulates the immune system and suppresses bacterial growth. However, as our study was in vitro, This explanation is not just inadequate.
As mentioned above, the main results of our MTT assay were quite like the previous studies. However, we conducted the microscopic and adhesion assay to gain a better insight. These tests manifest a noteworthy observation that adding Q10 in the presence of probiotics decreases probiotics’ adhesion to HEp-2 cells (Figure 6), which is probably the reason for the reduced survival rate of infected HEp-2 after the simultaneous addition of probiotics and Q10 compared to each one alone. Also, we observed that Q10 alone decreases the adhesion of S. mutans to HEp-2 cells. Co-administration of Q10 and probiotics had a similar result. As shown in Figure 4, the simultaneous presence of this co-enzyme and any probiotics leads to a decrease in S. mutans adhesion. On the other hand, Table 3 shows that the presence of Q10 has a negative impact on the adhesion of probiotics to HEp-2 cells.
As a matter of fact, our data illustrated that the presence of Q10 decreases the adhesion of all bacteria to HEp-2 cells, regardless of their pathogenicity and, obviously, the immune system. To explain this observation, we suggest a previously unmentioned mechanism that Q10s’ cell protection is not only for its antioxidant or immunomodulation activity but also Q10 may inhibit bacterial adhesion, which leads to a bacterial reduction in the in vitro and probably in vivo environment.
The effect of Q10 on cell wall stability has been illustrated in previous studies, mostly involving LDL biosynthesis; for instance, Eriksson et al. illustrated that Q10 stabilizes the cell membranes by its lipid ordering and condensing activity (Eriksson et al., 2018). Although tracing the exact mechanism demands extensive scrutiny, a review of involved pathways suggests the role of phosphatidylinositol-4,5-bisphosphate 3-kinase catalytic subunit alpha/beta/delta (PI3K). Tsai et al. demonstrated that Q10 suppressed the oxLDL-induced pathways, which affect Rho-associated protein kinase 2, and eventually PI3K, a key enzyme in focal adhesion.
Another significant point of this study is the improvement of L. salivarius adhesion and reduction of S. mutans adhesion to HEp-2 cells in the presence of 5μg of Q10. This probably shows that 5μg of Q10 is a suiTable concentration that prevents S. mutans adhesion as much as possible and, at the same time, has a minor negative impact on L. salivarius adhesion.
Despite the significant results, this study had some limitations that should be investigated in further studies, such as conducting qPCR on functional genes involved in adhesion or viability, investigating other concentrations of probiotics, and exploring the effect of Q10, L. plantarum, and L. salivarius on the proliferation of HEp-2 cells.
In conclusion, this in vitro study showed that the presence of L. plantarum, L. salivarius, and Q10 can significantly decrease the adhesion rate of S. mutans and improve the survival rate of infected HEp-2 cells in both therapeutic and preventive settings. Although the survival rates improved in the simultaneous presence of the two probiotics or Q10 with either, this improvement was not as significant as the presence of either of these substances alone. Our study suggests that they affect the survival of HEp-2 cells through very different mechanisms. As a result, there is a possibility that the simultaneous use of probiotics and Q10 in the oral environment will improve oral and dental health, respectively, through the improvement of oral microbiota and the overall reduction of bacterial adhesion. As a result, further investigations are required to ascertain the clinical efficacy of the co-administration of Q10 and these probiotics.
Data availability statement
The raw data supporting the conclusions of this article will be made available by the authors, without undue reservation.
Author contributions
SM drafted the manuscript and participated in experiments. PM conducted the statistical analysis, participated in structural editing, and revised the manuscript critically. ZK and MM equally supervised the project and resaved the manuscript. All authors contributed to the article and approved the submitted version.
Conflict of interest
The authors declare that the research was conducted in the absence of any commercial or financial relationships that could be construed as a potential conflict of interest.
Publisher’s note
All claims expressed in this article are solely those of the authors and do not necessarily represent those of their affiliated organizations, or those of the publisher, the editors and the reviewers. Any product that may be evaluated in this article, or claim that may be made by its manufacturer, is not guaranteed or endorsed by the publisher.
References
Block, L. H., Georgopoulos, A., Mayer, P., Drews, J. (1978). Nonspecific resistance to bacterial infections. enhancement by ubiquinone-8. J. Exp. Med. 148 (5), 1228–1240.
Bonifait, L., Chandad, F., Grenier, D. (2009). Probiotics for oral health: myth or reality? J. Can. Dental Assoc. 75 (8), 585–90.
Caglar, E., Kargul, B., Tanboga, I. (2005). Bacteriotherapy and probiotics’ role on oral health. Oral. diseases. 11 (3), 131–137.
Chugh, P., Dutt, R., Sharma, A., Bhagat, N., Dhar, M. S. (2020). A critical appraisal of the effects of probiotics on oral health. J. Funct. foods. 70, 103985.
Cosseau, C., Devine, D. A., Dullaghan, E., Gardy, J. L., Chikatamarla, A., Gellatly, S., et al. (2008). The commensal streptococcus salivarius K12 downregulates the innate immune responses of human epithelial cells and promotes host-microbe homeostasis. Infection immunity. 76 (9), 4163–4175.
Dahiya, P., Malhotra, A., Pajnoo, A., Gupta, R., Kamal, R. (2022). Comparative evaluation of efficacy of topical and intra-sulcular application of Co-enzyme Q10 in the non-surgical treatment of periodontal diseases. J. Indian Soc. Periodontology. 26 (6), 533–538.
Denny, N., Chapple, I., Matthews, J. (1999). Antioxidant and anti-inflammatory effects of coenzyme Q10: a preliminary study. J. Dent. Res. 78 (543), 00008.
Dodoo, C. C., Stapleton, P., Basit, A. W., Gaisford, S. (2020). The potential of streptococcus salivarius oral films in the management of dental caries: an inkjet printing approach. Int. J. Pharmaceutics. 591, 119962.
Eriksson, E. K., Hernández, V. A., Edwards, K. (2018). Effect of ubiquinone-10 on the stability of biomimetic membranes of relevance for the inner mitochondrial membrane. Biochim. Biophys. Acta (BBA)-Biomembranes. 1860 (5), 1205–1215.
Haeri, A., Khodaii, Z., Ghaderian, S. M. H., Tabatabaei Panah, A. S., Akbarzadeh Najar, R. (2012). Comparison of adherence patterns of a selection of probiotic bacteria to caco-2, HEp-2, and T84 cell lines. Ann. Microbiol. 62 (1), 339–344.
Hanioka, T., Tanaka, M., Ojima, M., Shizukuishi, S., Folkers, K. (1994). Effect of topical application of coenzyme Q10 on adult periodontitis. Mol. aspects Med. 15, s241–s2s8.
Hansen, I., Iwamoto, Y., Kishi, T., Folkers, K., Thompson, L. (1976). Bioenergetics in clinical medicine. IX. gingival and leucocytic deficiencies of coenzyme Q10 in patients with periodontal disease. Res. Commun. Chem. Pathol. Pharmacol. 14 (4), 729–738.
Hargreaves, I., Heaton, R. A., Mantle, D. (2020). Disorders of human coenzyme Q10 metabolism: an overview. Int. J. Mol. Sci. 21 (18), 6695.
Honda, K., Littman, D. R. (2016). The microbiota in adaptive immune homeostasis and disease. Nature. 535 (7610), 75–84.
Jose, J. E., Padmanabhan, S., Chitharanjan, A. B. (2013). Systemic consumption of probiotic curd and use of probiotic toothpaste to reduce streptococcus mutans in plaque around orthodontic brackets. Am. J. Orthodontics Dentofacial Orthopedics. 144 (1), 67–72.
Keller, M. K., Hasslöf, P., Stecksén-Blicks, C., Twetman, S. (2011). Co-Aggregation and growth inhibition of probiotic lactobacilli and clinical isolates of mutans streptococci: an in vitro study. Acta Odontologica Scandinavica. 69 (5), 263–268.
Khodaii, Z., Ghaderian, S. M. H., Natanzi, M. M. (2017). Probiotic bacteria against halitosis producing bacteria, in the presence of the hep 2 cells. Acta Med. Mediterranea. 33 (2), 301–304.
Kucia, M., Wietrak, E., Szymczak, M., Kowalczyk, P. (2020). Effect of ligilactobacillus salivarius and other natural components against anaerobic periodontal bacteria. Molecules. 25 (19), 4519.
Lee, K., Walker, A., Chakraborty, B., Kaspar, J., Nascimento, M., Burne, R. (2019). Novel probiotic mechanisms of the oral bacterium streptococcus sp. A12 as explored with functional genomics. Appl. Environ. Microbiol. 85 (21), e01335–e01319.
Lim, S.-M., Lee, N.-K., Paik, H.-D. (2020). Antibacterial and anticavity activity of probiotic lactobacillus plantarum 200661 isolated from fermented foods against streptococcus mutans. LWT. 118, 108840.
Littarru, G. P., Nakamura, R., Ho, L., Folkers, K., Kuzell, W. C. (1971). Deficiency of coenzyme Q10 in gingival tissue from patients with periodontal disease. Proc. Natl. Acad. Sci. 68 (10), 2332–2335.
Mack, D. R., Michail, S., Wei, S., McDougall, L., Hollingsworth, M. A. (1999). Probiotics inhibit enteropathogenic e. coli adherence in vitro by inducing intestinal mucin gene expression. Am. J. Physiology-Gastrointestinal Liver Physiol. 276 (4), G941–GG50.
Manthena, S., Rao, M. V. R., Penubolu, L. P., Putcha, M., Harsha, A. V. N. S. (2015). Effectiveness of CoQ10 oral supplements as an adjunct to scaling and root planing in improving periodontal health. J. Clin. Diagn. research: JCDR 9 (8), ZC26.
Matsumura, T., Saji, S., Nakamura, R., Folkers, K. (1973). Evidence for enhanced treatment of periodontal disease by therapy with coenzyme q. international journal for vitamin and nutrition research internationale zeitschrift fur vitamin-und ernahrungsforschung. J. Int. vitaminologie Nutr. 43 (4), 537–548.
MCREE, J. T., Hanioka, T., Shizukuishi, S., Folkers, K. (1993). Therapy with Coenzyme Q10 for Patients with Periodontal Disease 1. Effect of Coenzyme Q 10 on Subgingival Microorganisms. J. Dental Health 43 (5), 659–666.
Mehrabani Natanzi, M., Emampour, M., Mirzaei, A., Kalantar, E., Khodaii, Z. (2018). Protective activity of probiotic bacteria against candida albicans: an In vitro study. Int. J. Enteric Pathog. 6 (4), 113–117.
Nakamura, R., Littarru, G. P., Folkers, K., Wilkinson, E. G. (1974). Study of CoQ10-enzymes in gingiva from patients with periodontal disease and evidence for a deficiency of coenzyme Q10. Proc. Natl. Acad. Sci. 71 (4), 1456–1460.
Negri, M., Gonçalves, V., Silva, S., Henriques, M., Azeredo, J., Oliveira, R. (2010). Crystal violet staining to quantify candida adhesion to epithelial cells. Br. J. Biomed. science. 67 (3), 120–125.
Nga, N., Ngoc, T., Trinh, N., Thuoc, T., Thao, D. (2020). Optimization and application of MTT assay in determining density of suspension cells. Analytical Biochem. 610, 113937.
Nordström, E. A., Teixeira, C., Montelius, C., Jeppsson, B., Larsson, N. (2021). Lactiplantibacillus plantarum 299v (LP299V®): three decades of research. Beneficial Microbes 12 (5), 441–465.
Ogawa, A., Furukawa, S., Fujita, S., Mitobe, J., Kawarai, T., Narisawa, N., et al. (2011). Inhibition of streptococcus mutans biofilm formation by streptococcus salivarius FruA. Appl. Environ. Microbiol. 77 (5), 1572–1580.
Ouwehand, A. C., Niemi, P., Salminen, S. J. (1999). The normal faecal microflora does not affect the adhesion of probiotic bacteria in vitro. FEMS Microbiol. Lett. 177 (1), 35–38.
Ranadheera, R., Baines, S., Adams, M. (2010). Importance of food in probiotic efficacy. Food Res. Int. 43 (1), 1–7.
Rasoolzadeh, E. A., Shidfar, F., Rasoolzadeh, R. A., Hezaveh, Z. S. (2022). The effect of coenzyme Q10 on periodontitis: a systematic review and meta-analysis of clinical trials. J. Evidence-Based Dental Pract. 22 (2), 101710.
Silva, L. M., Brenchley, L., Moutsopoulos, N. M. (2019). Primary immunodeficiencies reveal the essential role of tissue neutrophils in periodontitis. Immunol. Rev. 287 (1), 226–235.
Thaiss, C. A., Zmora, N., Levy, M., Elinav, E. (2016). The microbiome and innate immunity. Nature. 535 (7610), 65–74.
Wilkinson, E. G., Arnold, R., Folkers, K., Hansen, I., Kishi, H. (1975). Bioenergetics in clinical medicine. II. adjunctive treatment with coenzyme q in periodontal therapy. Res. Commun. Chem. Pathol. Pharmacol. 12 (1), 111–123.
Winkler, P., De Vrese, M., Laue, C., Schrezenmeir, J. (2005). Effect of a dietary supplement containing probiotic bacteria plus vitamins and minerals on common cold infections and cellular immune parameters. Int. J. Clin. Pharmacol. Ther. 43 (7), 318–26.
Keywords: co-enzyme Q10, probiotics, periodontal disease, ligilactobacillus salivarius, lactiplantibacillus plantarum
Citation: Khodaii Z, Mardi S, Mardi P and Natanzi MM (2023) Adjunctive role of Q10 with Ligilactobacillus salivarius, and Lactiplantibacillus plantarum probiotic Bacteria on the HEp-2 cells viability and adhesion of Streptococcus mutans. Front. Cell. Infect. Microbiol. 13:1053230. doi: 10.3389/fcimb.2023.1053230
Received: 25 September 2022; Accepted: 11 April 2023;
Published: 28 April 2023.
Edited by:
Daniel Belstrøm, University of Copenhagen, DenmarkReviewed by:
Divyashri Baraniya, Temple University, United StatesNilima Thosar, Sharad Pawar Dental College And Hospital (SPDC), India
Copyright © 2023 Khodaii, Mardi, Mardi and Natanzi. This is an open-access article distributed under the terms of the Creative Commons Attribution License (CC BY). The use, distribution or reproduction in other forums is permitted, provided the original author(s) and the copyright owner(s) are credited and that the original publication in this journal is cited, in accordance with accepted academic practice. No use, distribution or reproduction is permitted which does not comply with these terms.
*Correspondence: Mahboobeh Mehrabani Natanzi, mmehrabani95@gmail.com
†These authors have contributed equally to this work and share first authorship