- 1Department of Laboratory Medicine, The First Affiliated Hospital of Zhengzhou University, Zhengzhou, China
- 2Collaborative Innovation Center for Diagnosis and Treatment of Infectious Diseases, State Key Laboratory for Diagnosis and Treatment of Infectious Diseases, The First Affiliated Hospital, College of Medicine, Zhejiang University, Hangzhou, China
- 3Department of Structure and Morphology, Jinan Microecological Biomedicine Shandong Laboratory, Jinan, Shandong, China
- 4Research Units of Infectious Diseases and Microecology, Chinese Academy of Medical Sciences, Beijing, China
Purpose: To explore the genetic characteristics of the IMP-4 and SFO-1 co-producing multidrug-resistant (MDR) clinical isolates, Enterobacter hormaechei YQ13422hy and YQ13530hy.
Methods: MALDI-TOF MS was used for species identification. Antibiotic resistance genes (ARGs) were tested by PCR and Sanger sequencing analysis. In addition to agar dilution, broth microdilution was used for antimicrobial susceptibility testing (AST). Whole-genome sequencing (WGS) analysis was conducted using the Illumina NovaSeq 6000 and Oxford Nanopore platforms. Annotation was performed by RAST on the genome. The phylogenetic tree was achieved using kSNP3.0. Plasmid characterization was conducted using S1-pulsed-field gel electrophoresis (S1-PFGE), Southern blotting, conjugation experiments, and whole genome sequencing (WGS). An in-depth study of the conjugation module was conducted using the OriTFinder website. The genetic context of blaIMP-4 and blaSFO-1 was analyzed using BLAST Ring Image Generator (BRIG) and Easyfig 2.3.
Results: YQ13422hy and YQ13530hy, two MDR strains of ST51 E. hormaechei harboring blaIMP-4 and blaSFO-1, were identified. They were only sensitive to meropenem, amikacin and polymyxin B, and were resistant to cephalosporins, aztreonam, piperacillin/tazobactam and aminoglycosides, intermediate to imipenem. The genetic context surrounding blaIMP-4 was 5′CS-hin-1-IS26-IntI1-blaIMP-4-IS6100-ecoRII. The integron of blaIMP-4 is In823, which is the array of gene cassettes of 5′CS-blaIMP-4. Phylogenetic analysis demonstrated that E. hormaechei YQ13422hy and YQ13530hy belonged to the same small clusters with a high degree of homology.
Conclusion: This observation revealed the dissemination of the blaIMP-4 gene in E. hormaechei in China. We found that blaIMP-4 and blaSFO-1 co-exist in MDR clinical E. hormaechei isolates. This work showed a transferable IncN-type plasmid carrying the blaIMP-4 resistance gene in E. hormaechei. We examined the potential resistance mechanisms of pYQ13422-IMP-4 and pYQ13422-SFO-1, along with their detailed genetic contexts.
Introduction
Enterobacter cloacae complex (ECC) is the most common group of species among the genus Enterobacter, including six closely related species: E. cloacae, E. asburiae, E. hormaechei, E. kobei, E. ludwigii, and E. nimipressuralis (Mezzatesta et al., 2012). Enterobacter hormaechei can be widely found in different environments such as the nature (Osei Sekyere and Reta, 2021), feces of humans or animals. But it is also an important pathogenic bacteria in hospitals, which can be responsible for nosocomial infections, such as wounds, urinary tract, and soft tissue infections (Xu et al., 2015). The horizontal spread of bacterial resistance genes, especially the carbapenemase-encoding gene, has brought great difficulties to clinical treatment (Annavajhala et al., 2019).
Since blaIMP-1 was firstly declared in Japan in 1991 (Watanabe et al., 1991), IMP- producing ECC has been playing an increasingly significant role in the world antibiotic resistance stage, like Malaysia (Liew et al., 2018), Portugal (Goncalves et al., 2021), and Korea (Lee et al., 2017). As time progressed, more and more IMP variants appeared in China, including IMP-2 (Riccio et al., 2000), IMP-8 (Yan et al., 2001), IMP-4 (Chu et al., 2001), IMP-26 (Gou et al., 2020). In China, IMP-4-positive carbapenemase-producing Enterobacterales (CPE) have become important carbapenem-resistant bacteria (Hu et al., 2014), since it was first discovered in Hongkong (Chu et al., 2001) in 2001. The blaIMP-4 is mainly found in Pseudomonas aeruginosa, but has been gradually reported in Enterobacteriaceae (Matsumura et al., 2017), such as E. hormaechei (Chen et al., 2022). More importantly, the coincidence of blaIMP and other antibiotic resistance genes is becoming increasingly common, such as co-carrying blaIMP-4 and blaNDM-1 (Zhang et al., 2021a), further increasing the tremendous pressure of clinical treatment.
In 1999, a clinical E. cloacae 8009 isolate possessing a transferable plasmid harboring blaSFO-1 was reported in Japan (Matsumoto and Inoue, 1999). The reports of blaSFO-1 and coexisting antibiotic resistance genes have recently increased in China (Zhou et al., 2020). In comparison with other broad-spectrum-beta-lactamases, the blaSFO-1 gene has a low occurrence of antimicrobial resistance that has been ignored by routine monitoring. We found a carbapenem-resistant E. hormaechei clinical isolate co-harboring blaSFO-1 and blaIMP-4.
There are few studies on the transmission of blaSFO-1 and blaIMP-4 in ECC in China, especially E. hormaechei. Therefore, it is vital to further explore the genome and phenotypic characteristics of the blaSFO-1 and blaIMP-4 in E. hormaechei in China. We identified clinical isolates of E. hormaechei YQ13422hy and YQ13530hy co-producing blaIMP-4 and blaSFO-1, and described the detailed content of a conjugative IncN-plasmid. Furthermore, we revealed the underlying transmission mechanisms of blaIMP-4.
Materials and methods
Bacterial strains
We continuously collected ECC clinical isolates from a tertiary hospital affiliated to Wenzhou Medical University from 2015 to 2017 for routine surveillance. A total of eight carbapenemase producing ECC clinical isolates have been identified using the MALDI-TOF MS (Bruker, Bremen, Germany). Among them, the two isolates of IMP-4-producing E. hormaechei strains (25%) were identified using PCR and next-generation sequencing (NGS), designated as YQ13422hy and YQ13530hy.
Multilocus sequence typing and antimicrobial susceptibility testing
As described previously, multilocus sequence typing (MLST) was conducted (Gou et al., 2020). A new sequence type has been submitted to MLST and have been approved by PubMLST (http://pubmlst.org/ecloacae). Agar dilution and broth microdilution were used for antimicrobial susceptibility testing (AST), and Escherichia coli ATCC 25922 was used as control. AST results were interpreted based on the Clinical and Laboratory Standards Institute (CLSI) 2021 standards, while tigecycline and colistin clinical breakpoints were based on the 2022 EUCAST (http://www.eucast.org). Sixteen antimicrobial resistance genes were searched using PCR, including blaKPC, blaNDM, blaIMP, blaOXA-23, blaOXA-48, blaVIM, and mcr-1-10.
Plasmid characterization and conjugation assays
Pulsed-field gel electrophoresis (PFGE) was used to determine the homology between strains YQ13422hy and YQ13530hy. PFGE was undertaken on the CHEF-DR III system (Bio-Rad. Hercules, CA, United States), and patterns were evaluated and interpreted according to the published guidelines (Xu et al., 2018). The profiles of plasmids in strains YQ13422hy and YQ13530hy were analyzed by the S1-PFGE, as previously described (Wang et al., 2019). Then we used a digoxigenin-labeled blaIMP-4 probe made by a dig-high prime DNA Labeling and Detection Starter Kit II (Roche Diagnostics) to determine the location of plasmid harboring blaIMP-4 via southern blotting and hybridization. The transferability of plasmids was investigated by using E. coli J53, a NaN3-resistant standard strain, as a receptor for conjugation assays. Subsequently, transconjugants carrying blaIMP-4 were first selected using Mueller-Hinton agar (OXOID, Hampshire, United Kingdom) plates containing both 1 mg/L meropenem and 200 mg/L NaN3. Further, the selected conjugates were confirmed by MALDI-TOF/MS, PCR identified the blaIMP-4 gene, and AST was used to confirm the expression of drug resistance genes.
Whole genome sequencing and in silico analyses
Genomic DNA was extracted using a Genomic DNA Isolation Kit (QIAGEN, Hilden, Germany) and sequenced using Illumina Novaseq 6000 (Illumina, San Diego, CA, United States) and Oxford Nanopore platforms (Oxford Nanopore Technologies, Oxford, United Kingdom). RAST 2.0 was used to annotate the draft genomes obtained by SPAdes version 3.9.1 (Aziz et al., 2008) (http://rast.nmpdr.org/). ISfinder and INTEGRALL were used to detect insertion sequence elements and integrons (https://www-is.biotoul.fr/). Antimicrobial resistance genes (ARGs) were identified by Resfinder (https://cge.cbs.dtu.dk/services/ResFinder/). Different plasmid genome sequences were compared by BLAST Ring Image Generator (Alikhan et al., 2011) (BRIG). The figures about the genetic context surrounding the antibiotic resistance genes were drawn by Easyfig 2.3 (Sullivan et al., 2011). To verify whether the plasmids pYQ13422-IMP-4, pYQ13530-IMP-4, pYQ13422-SFO-1 and pYQ13530-SFO-1 were conjugative plasmids, we used the OriTFinder website (https://tool-mml.sjtu.edu.cn/oriTfinder/oriTfinder.html).
Phylogenetic analysis
We downloaded all available IMP-carrying ECC from the NCBI genome database in May 2022 to study the phylogenetic relationships of YQ13422hy and YQ13530hy with other ECC. KSNP3.0 (Gardner et al., 2015) was used to construct the phylogenetic tree based on the previously-mentioned downloaded data via SNPS. ITOL was used to visualize and modify the phylogenetic tree (https://itol.embl.de/).
Results
Species confirmation and homology analysis
The YQ13422hy strain was isolated from a sputum specimen of a 36-year-old male suffering from hypoxic encephalopathy on March 12, 2017. YQ13530hy was isolated from a sputum specimen of a 60-year-old male with brain herniation on April 2, 2017. The patient carrying YQ13422hy was hospitalized for 3 months, from March 01, 2017 to June 10, 2022. The patient carrying YQ13530hy was hospitalized for 1 month from April 01, 2017 to April 19, 2022. Patient carrying YQ13422hy was treated with intravenous vancomycin, Imipenem and Cilastatin Sodium, as well as Cefoperazone Sodium and Sulbactam Sodium. Patient carrying YQ13530hy was treated with vancomycin, meropenem and levofloxacin. Both patients were hospitalized in the same ward. ANI analysis (Figure S2 and Table S2) and WGS showed that the two isolates were highly homologous, and the blaIMP-4-bearing plasmids had 99.97% similarity, indicating the isolates’ clonal spread. In fact, it is not clear how the clonal spread happened, but we suspect that it may have been through contact or through the air, because both strains were detected in sputum.
AST of Enterobacter hormaechei YQ13422hy and YQ13530hy
The isolates YQ13422hy and YQ13530hy both displayed resistance to aztreonam, ceftriaxone, cefotaxime, ceftazidime, levofloxacin, ciprofloxacin, gentamicin, piperacillin/tazobactam, chloromycin, amoxicillin-clavulanate, cefepime, with sensitivity to meropenem, amikacin, and polymyxin B. For imipenem, YQ13422hy and YQ13530hy were determined as intermediate. In the case of YQ13422hy, it exhibited intermediate resistance to fosfomycin, and susceptibility to trimethoprim/sulfamethoxazole and tigecycline. On the other hand, YQ13530hy showed resistance to trimethoprim/sulfamethoxazole and tigecycline, while it was susceptible to fosfomycin. AST results revealed that both strains were MDR E. hormaechei. The results of AST of E. hormaechei YQ13422hy and YQ13530hy are shown in Table 1.
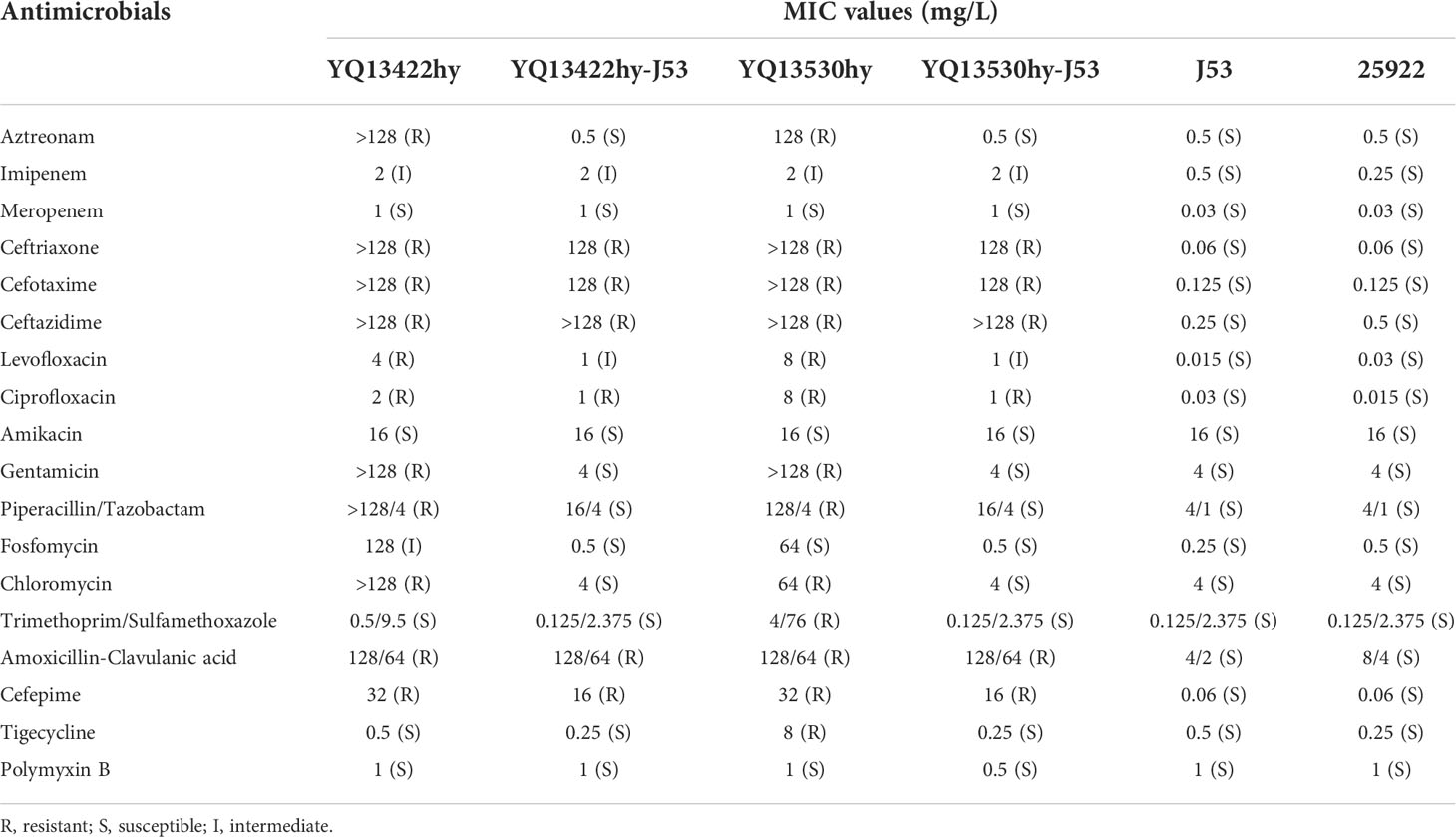
Table 1 MIC values of antimicrobials for E. hormaechei YQ13422hy andYQ13530hy, recipient strain J53, transconjugants YQ13422hy-J53 and YQ13530hy-J53, and control strain E. coli 25922.
Location of blaIMP-4 and blaSFO-1 and the conjugation assays
S1-PFGE and hybridization experiments on YQ13422hy and YQ13530hy (Figure S1) showed that the plasmid harboring the blaIMP-4 resistance gene was about 53 kb and it was named pYQ13422-IMP-4. The plasmid carrying blaSFO-1 resistance gene was designated as pYQ13422-SFO-1.
The transconjugant was identified as E. coli by MALDI-TOF/MS. Then PCR and Sanger sequencing were performed to determine that the transconjugant was carrying the blaIMP-4 resistance gene. The results of AST also indicated that the plasmid pYQ13422-IMP-4 was successfully transferred into recipient J53. A comparison of AST results between YQ13422hy and YQ13422-J53, YQ13530hy and YQ13530-J53 showed that the transconjugant was resistant to ceftriaxone, cefotaxime, ciprofloxacin, ceftazidime, amoxicillin-clavulanic acid and cefepime, sensitive to aztreonam, gentamicin, piperacillin/tazobactam, chloromycin and fosfomycin, and intermediate to levofloxacin and imipenem, but significantly increased the MIC value of the transconjugant to levofloxacin. Through the analysis by the OriTFinder website, the complete conjugative modules on the plasmid pYQ13422-IMP-4, pYQ13530-IMP-4, pYQ13422-SFO-1, and pYQ13530-SFO-1 were identified, including the origin of transfer site (oriT), gene cluster for bacterial type IV secretion system (T4SS), gene encoding type IV coupling protein (T4CP), and relaxase gene (Table S4). Based on these results, it appears they are MDR plasmids that can be horizontal transferred (Figure 1). Because pYQ13422-IMP-4 and pYQ13530-IMP-4 are exactly the same, we only show pYQ13422-IMP-4 in Figure 1.
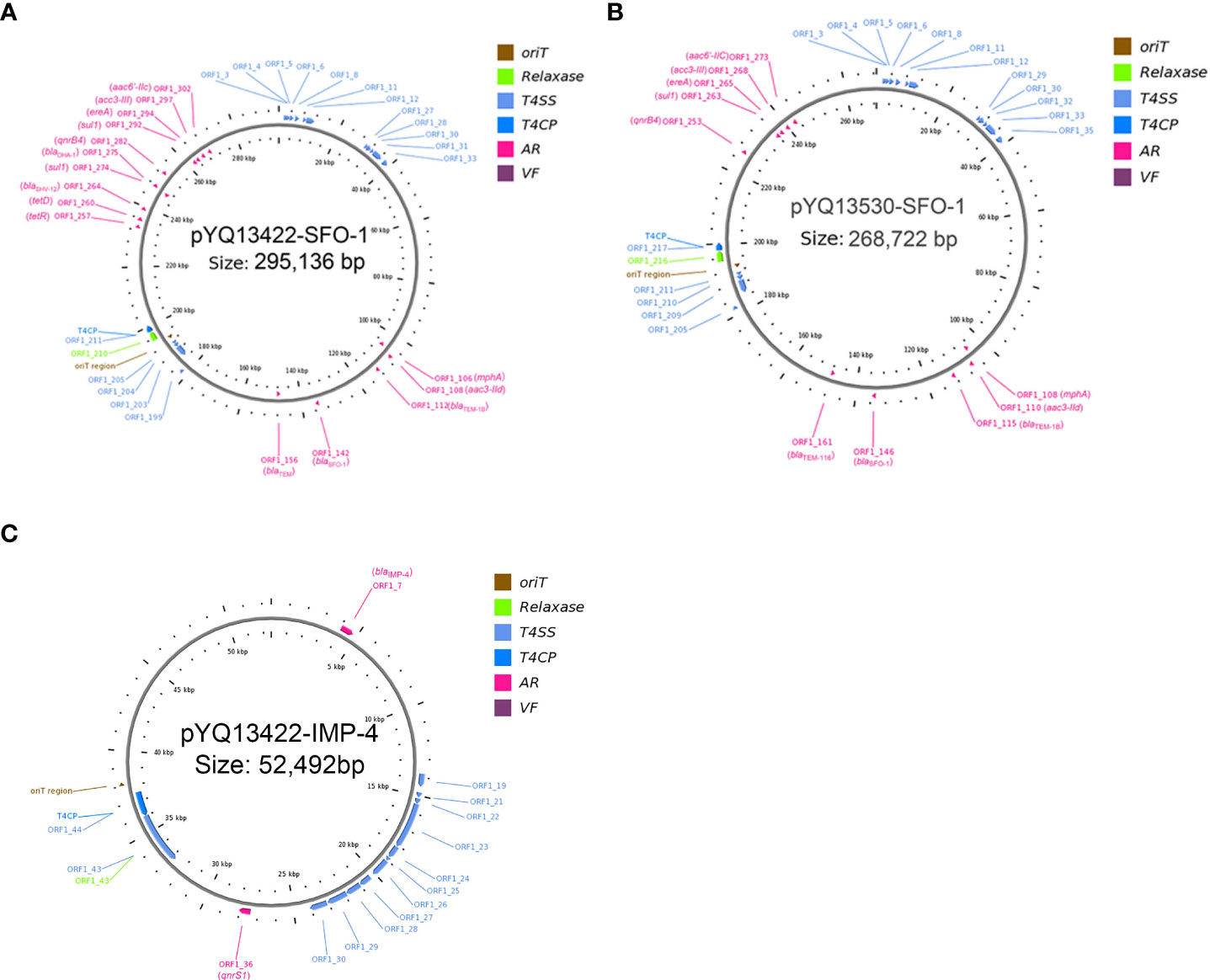
Figure 1 Three conjugative plasmids pYQ13422-SFO-1 (A) and pYQ13530-SFO-1 (B) and pYQ13422-IMP-4 (C). AR (ARGs), acquired antibiotic resistance determinant genes; VF, virulence factors.
Characterization of the genome of E. hormaechei YQ13422hy and YQ13530hy
The result of S1-PFGE showed that YQ13422hy and YQ13530hy both carried three plasmids of different sizes, as mentioned above. WGS showed that YQ13422hy and YQ13530hy both carried four plasmids of different sizes. The plasmid pYQ13422hy-3 and Pyq13530hy-3 are not visible in the S1-PFGE result due to its small size; 4995bp.
According to the WGS results, YQ13422hy and YQ13530hy were shown by MLST to carry the genes fusA (4), leuS (6), rplB (4), rpoB (6), dnaA (4), gyrB (4), pyrG (37), confirming its typing as ST51. Specific genome information on plasmid sizes, Inc and MLST typing and resistance genes is displayed in Table 2.
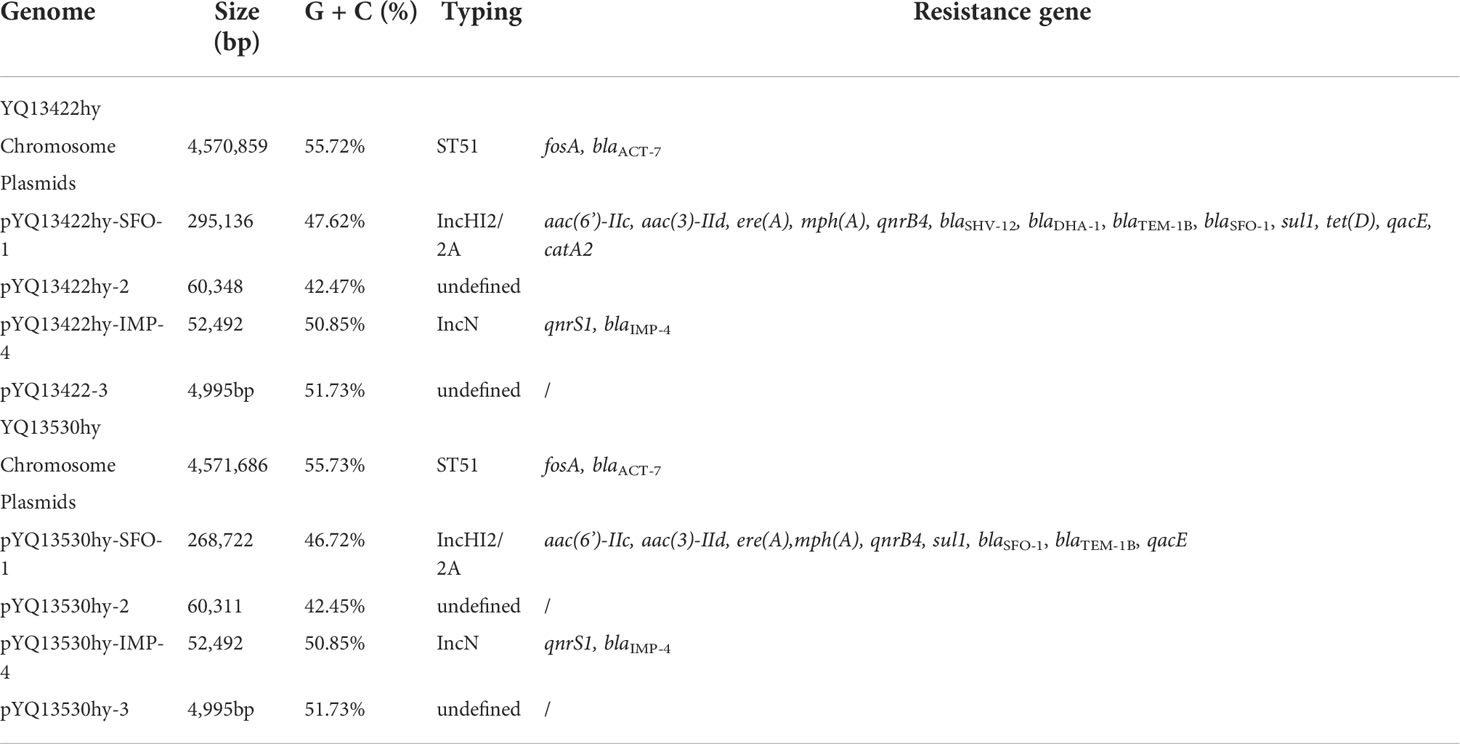
Table 2 Genome information and acquired antibiotic resistance genes of E. hormaechei YQ13422hy and YQ13530hy.
Structural characterization of the transferable plasmid
The sequence length of plasmid pYQ13422-IMP-4 is 52,492bp, including 92 protein-encoding genes, and its G + C content is 50.85%. This plasmid carries the blaIMP-4 gene and the qnrS1 gene, which is known from above. Its plasmid type is IncN by Plasmidfinder. The most similar plasmids (with 100% coverage and 99% identities) identified by NCBI blast are as follows: pIMP-GZ1517 (KT982618.1), pZHH-3 (CP059714), p128379-IMP (MF344559) and pIMP-GZ1058 (KU051709.1) from E. coli, and pIMP-HZ1 (KU886034) from K. pneumoniae. BLAST, Ring Image Generator (BRIG) generated the circular image of multiple plasmid comparisons, and the results were demonstrated in Figure 2. The plasmids carry multiple insertion sequences at different positions, such as IS6100, IS1X2, IS26 and ISKpn19. Further, we investigated the genetic environment of the IMP-4 resistance gene and found that it has an IntI1 upstream and also carries a group II intron reverse transcriptase/maturase gene downstream of it. Comparison with pIMP-GZ1517 (KT982618.1) and pIMP-GZ1058 (KU051709.1) revealed that an insertion sequence IS26 was missing on the YQ13422-IMP-4 plasmid (Figure S5). Integron In823 was identified by INTEGRALL, whose array of gene cassettes is 5′CS-blaIMP-4. YQ13422-SFO-1 is demonstrated in Figure 2B.
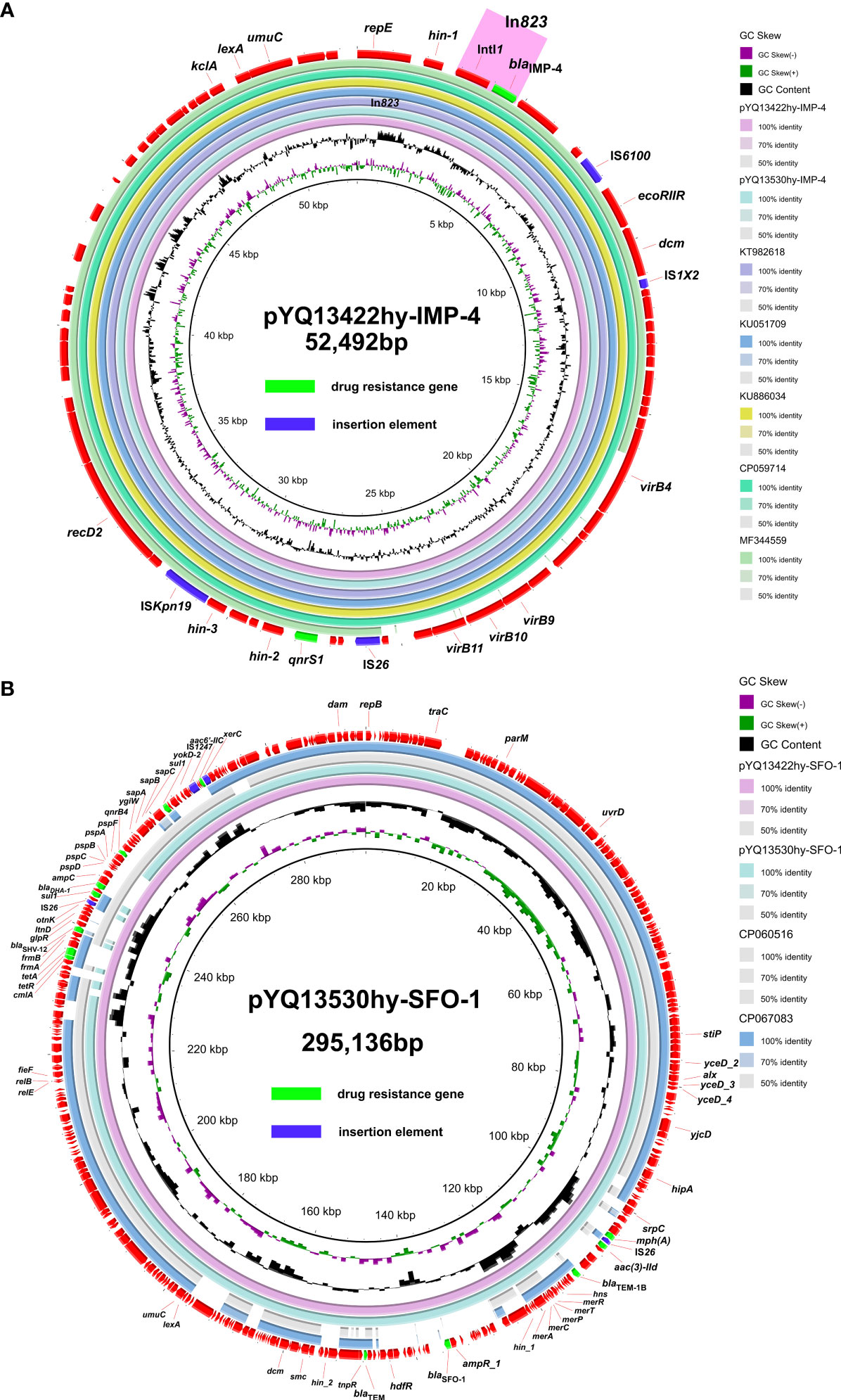
Figure 2 Genomic analyses of plasmid pYQ13422-IMP-4 (A) and pYQ13422-SFO-1 (B). The comparative plasmid circular map of pYQ13422-IMP-4 and pYQ13422-SFO-1, generated using BLAST Ring Image Generator (BRIG), shows the genes and their locations.
In addition to analyzing MDR plasmid characteristics, we also examined mobile elements flanking the resistant genes (Figure S5). The blaSFO−1 was detected on a Tn3 unit (TnAS3-IS5075-traX-ΔTn3-ampR-blaSFO-1-ΔIS3). According to the genetic mapping of blaSFO-1, ampR was upstream of blaSFO−1. Regulation of SFO-1 is carried out by the regulator ampR, which is inversely oriented upstream (Fernandez et al., 2011). Tn3 and IS5075 were located upstream of ampR, and genetic mapping also showed that the transposon Tn3 was interrupted.
Phylogenetic analysis
We downloaded all genomic data of the blaIMP-carrying ECC isolates (n = 167) from NCBI publicly available data and performed a phylogenetic analysis with YQ13422hy and YQ13530hy (Table S1). The data showed that the vast majority of bacteria carrying the blaIMP resistance genes in the ECC are E. hormaechei, with 154 strains accounting for 91.12% of all strains. The results revealed that the blaIMP resistance genes carried by the ECC were blaIMP-1 (n = 66), blaIMP-4 (n = 77), blaIMP-8 (n = 8), blaIMP-13 (n = 2), blaIMP-16 (n = 1), blaIMP-26 (n = 3), and blaIMP-70 (n = 12). Of these, 158 strains were isolated from humans, and only 11 strains had no host information. The majority of isolates were from Japan, China and Australia. The source of these strains is almost exclusively clinical, mainly blood, urine, sputum, and screening swab. YQ13422hy and YQ13530hy form a small cluster alone, and a larger cluster with GCA_015684015, GCA_021165665, GCA_015683815, but GCA_015684015 and GCA_015683815 are isolated from Australia, while GCA_021165665.1 is recovered from Ireland. They are both E. hormaechei and carry the drug resistance gene blaIMP-4. More specific information is shown in Figure S6.
Discussion
ECC is increasingly being isolated from clinical specimens and is now one of the world’s most critical nosocomial infectious pathogens (Bolourchi et al., 2022). The ECC carrying blaIMP has emerged in six countries, including United Kingdom, the United States, Ireland, Japan, China, and Australia. Thus, the prevalence of the blaIMP gene worldwide should be given sufficient attention.
According to our susceptibility results, strains carrying blaIMP-4 are intermediate to imipenem and sensitive to meropenem. There is evidence suggesting that IMP-4 enzyme has much stronger hydrolytic activity for imipenem than meropenem, which is consistent with previous findings (Chu et al., 2001). A considerable amount of literature now exists suggesting that multiple different species of bacteria carrying blaIMP-4 are intermediate or sensitive to imipenem and meropenem (Chu et al., 2001; Lee et al., 2017; Tarabai et al., 2021; Zhang et al., 2021b). However, the exact mechanism is still unclear. Intermediate susceptibility to imipenem and susceptibility to meropenem in strains carrying blaIMP-4 possible mechanism could be: i) related to the activity of efflux pumps (Zhang et al., 2021b), or (ii) It is possibly that the organisms had little or no expression of their blaIMP-4 gene (Chu et al., 2001), or (iii) It seems that IMP enzymes confer carbapenem resistance only in members of the family Enterobacteriaceae with concomitant permeability lesions (Chu et al., 2001).
Plasmids play a major role in the dissemination of antibiotic resistance genes among Enterobacteriaceae (Huang et al., 2013). Although there have been many studies on IncN-type plasmids, few studies have found that IncN-type plasmids carrying the IMP-4 resistance gene in E. hormaechei. IncN-type plasmids carrying genes such as blaKPC (Gomez-Simmonds et al., 2022) and blaNDM (Hirabayashi et al., 2021) have been found in E. coli (Dorr et al., 2022) and Citrobacter (Yao et al., 2021). Also, a lot of IncN blaIMP-4-carrying plasmids were described in Enterobacterales, including one study showing the isolation of Klebsiella pneumoniae carrying an IncN-type plasmid with blaKPC-2 from dogs (Sellera et al., 2021). Wang and colleagues have already reported that an IncN ST7 plasmid carrying blaIMP-4 is disseminated in a variety of enterobacterial species originating from patients with epidemiological links in remote areas of China (Wang et al., 2017). The plasmids carrying the blaIMP-4 gene of YQ13422hy and YQ13530hy are entirely identical. Besides, it’s worth noting that we collected these two bacteria from different patients in the same ward at different times in the same hospital. In addition, based on the INTEGRALL database, blaIMP-4 is located on a class 1 integron In823, which is rare in E. hormaechei, with the array of gene cassettes 5′CS-blaIMP-4. It has become a consensus that the proliferation of integrons has exacerbated the prevalence of drug-resistant genes, especially class 1 integrons (Souque et al., 2021). The 3′CS of most class I integrons include three open reading frames (ORFs): sulfa resistance gene (sul1), quaternary ammonium compound and ethidium bromide tolerance gene (qacEΔ1) and an ORF of unknown function. However, unlike the classical class 1 integron, the 3’CS of the class 1 integron of YQ13422-IMP-4, sul1 and qacEΔ1 was not found.
Meanwhile, we confirmed the presence of a transposon TnAS3 carrying the blaSFO-1 gene, which belongs to the transposon family Tn3. Studies on the Tn3 family of transposons have been relatively extensive. Previous studies indicate that the most characteristic resolvases of the Tn3 transposon family are members of the serine recombinase (S recombinase) family, but rarely are members of the tyrosine recombinase (Y recombinase) family (Nicolas et al., 2015). The plasmid YQ13422hy-SFO-1 carries tyrosine recombinase xerC. Meanwhile, through our study on the structure of the YQ13422-IMP-4 plasmid and comparative analysis with other plasmids, we found that the blaIMP-4 genes all contain a group II intron reverse transcriptase/maturase downstream, and speculated that this gene might be associated with the transfer and spread of blaIMP-4. Compared with plasmids pIMP-GZ1517 (KT982618) and pIMP-GZ1517 (KU051709), pYQ13422-IMP-4 and pZHH-3 (CP059714) have no insert sequence IS26, which suggests that IntI1 can transfer blaIMP-4 independently and IS26 may not be the critical gene for blaIMP-4 gene transfer. In pIMP-GZ1517 (KT982618) and pIMP-GZ1517 (KU051709), the IntI1 gene was interrupted by an IS26 element, but blaIMP-4 could still be transferred. We believed that the truncated IntI1 was out of its function, and the transfer was achieved by IS26. We also found that p128379-IMP does not have the integrase IntI1, but contains IS26. We discovered the entire complete conjugative modules on the plasmids pYQ13422-IMP-4, pYQ13422-SFO-1 and pYQ13530-SFO-1.
The studies on blaIMP-4 in E. hormaechei are rare worldwide, with significant differences between countries. A prospective cohort study (Roberts et al., 2020a) in Australia showed that the primary ST type of ECC carrying blaIMP-4 was ST90, and the plasmid carrying blaIMP-4 was IncHI2-type. Currently, blaIMP-4 is Australia’s most common resistance gene (Sidjabat et al., 2015), and our phylogenetic analysis based on published data from NCBI confirmed this. Another study (Roberts et al., 2020b) also supported a similar view. Furthermore, we found that all the integrons of E. hormaechei carrying blaIMP-4 in the published studies rarely contain In823. This further indicates that the context of the blaIMP-4 gene may be different in China.
In China, few reports described the detection of blaIMP-4 gene in E. hormaechei (Chen et al., 2022). Kai Zhou et al. found a strain of E. hormaechei of ST418 carrying blaNDM-1, mcr-9.1, and blaIMP-4 (Zhou et al., 2017). According to its research, the plasmid carrying blaIMP-4 was IncHI2-type, which is consistent with the global trend. The blaSFO-1 gene is not routinely monitored, but it could be an important weapon against antibiotics. So, the coexistence of blaSFO-1 and other antibiotic resistance genes should not be ignored. A previous study reported the co-producing of SFO-1 and IMP-4 in Klebsiella pneumoniae clinical isolate (Zhou et al., 2017). Moreover, in our work, we not only found the co-producing of SFO-1 and IMP-4 in E. hormaechei, but also found they are located at two different transferable plasmids. Antibiotic resistance may be increased by the presence of blaSFO-1. The research of AST results on IMP-producing ECC (Hickey et al., 2021) also suggested that IMP metalloenzymes production in ECC infections is serious, and our work also validated the study. E. hormaechei carrying the blaIMP-4 gene spread rapidly, with enhanced drug resistance and changes in the genetic environment. Therefore, the coexistence of blaSFO-1 and blaIMP-4 undoubtedly complicates the treatment of E. hormaechei infections. The limitation of our work is that only two samples were studied, and there were no more samples to further elaborate on the prevalence of IncN-plasmid carrying IMP-4 in E. hormaechei.
Conclusion
Our study found the co-production of IMP-4 and SFO-1 in E. hormaechei. Besides, it revealed the IncN-type plasmid carrying blaIMP-4 in E. hormaechei, which indicated the potential horizontal transformation of ARGs. In conclusion, our work supplemented the studies of E. hormaechei carrying blaIMP-4 and blaSFO-1 in China, and also suggested that focusing on E. hormaechei will be important in future studies.
Data availabilitiy statement
The datasets presented in this study can be found in online repositories. The names of the repository/repositories and accession number(s) can be found in the article/Supplementary Material.
Ethics statement
Written informed consent was obtained from the individual(s) for the publication of any potentially identifiable images or data included in this article.
Author contributions
The experiments were conceived and designed by JG and BZ. The samples and experiments were collected and performed by JQ, HG, RL, CL and RC. The data was analyzed by HX and XG. The manuscript was written by JQ. All authors contributed to the article and approved the submitted version.
Funding
This work was supported by research grants from Henan Science and Technology Department (No. 192102310059), the National Natural Science Foundation of China (82072314), the Research Project of Jinan Microecological Biomedicine Shandong Laboratory (JNL-2022011B), the Fundamental Research Funds for the Central Universities (2022ZFJH003), CAMS Innovation Fund for Medical Sciences (2019-I2M-5-045), and Henan Province Medical Science and Technology Research Project Joint Construction Project (No. LHGJ20190232).
Conflict of interest
The authors declare that the research was conducted in the absence of any commercial or financial relationships that could be construed as a potential conflict of interest.
Publisher’s note
All claims expressed in this article are solely those of the authors and do not necessarily represent those of their affiliated organizations, or those of the publisher, the editors and the reviewers. Any product that may be evaluated in this article, or claim that may be made by its manufacturer, is not guaranteed or endorsed by the publisher.
Supplementary material
The Supplementary Material for this article can be found online at: https://www.frontiersin.org/articles/10.3389/fcimb.2022.998578/full#supplementary-material
Supplementary Figure 1 | Plasmid profiles of (E) hormaechei YQ13422hyh and YQ13530hy. (A) Plasmid size determination by S1-PFGE, with Salmonella enterica serotype Braenderup H9812 as the size marker. (B) Southern blotting hybridization with an IMP-4-specific probe.
Supplementary Figure 2 | The ANI analysis between YQ13422hy and YQ13530hy showed that there is a high level of similarity between the two genomes.
Supplementary Figure 3 | Genomic analyses of plasmid pYQ13422-IMP-4. The comparative plasmid circular map of pYQ13422-IMP-4 generated using BLAST Ring Image Generator (BRIG), shows the genes and their locations.
Supplementary Figure 4 | YQ13422hy and YQ13530hy ‘s genomes contain a wealth of information
Supplementary Figure 5 | Genetic context of blaIMP-4 on pYQ13422-IMP-4 and blaSFO-1 on pYQ13422-SFO-1. Genes are denoted by arrows. Genes, mobile elements, and other features are colored based on their functional classification.
Supplementary Figure 6 | The phylogenetic tree of 167 strains ECC based on the blaIMP resistance genes, generated by kSNP3.0 plus E. hormaechei YQ13422hy (Biosample SAMN28918927) and YQ13530hy (Biosample SAMN28919657). The sources of strains are identified as clinical. We used different colors to represent different meanings. The seven circles around the phylogenetic tree indicate the species (inner circle), ST type, IMP-type, host, location, year, and source (outer circle) of these strains. We marked YQ13422hy and YQ13530hy in red.
References
Alikhan, N. F., Petty, N. K., Ben Zakour, N. L., Beatson, S. A. (2011). BLAST ring image generator (BRIG): Simple prokaryote genome comparisons. BMC Genomics 12, 402. doi: 10.1186/1471-2164-12-402
Annavajhala, M. K., Gomez-Simmonds, A., Uhlemann, A.-C. (2019). Multidrug-resistant enterobacter cloacae complex emerging as a global, diversifying threat. Front. Microbiol. 10. doi: 10.3389/fmicb.2019.00044
Aziz, R. K., Bartels, D., Best, A. A., Dejongh, M., Disz, T., Edwards, R. A., et al. (2008). The RAST server: Rapid annotations using subsystems technology. BMC Genomics 9, 75. doi: 10.1186/1471-2164-9-75
Bolourchi, N., Giske, C. G., Nematzadeh, S., Mirzaie, A., Abhari, S. S., Solgi, H., et al. (2022). Comparative resistome and virulome analysis of clinical NDM-1-producing carbapenem-resistant enterobacter cloacae complex. J. Glob Antimicrob. Resist. 28, 254–263. doi: 10.1016/j.jgar.2022.01.021
Chen, W., Hu, Z., Wang, S., Huang, D., Wang, W., Cao, X., et al. (2022). Letter to the Editor: Characterization of a clinical enterobacter hormaechei strain belonging to epidemic clone ST418 Co-carrying blaNDM-1, blaIMP-4, and mcr-9.1. Microb. Drug Resist. 28, 180–184. doi: 10.1089/mdr.2020.0568
Chu, Y. W., Afzal-Shah, M., Houang, E. T., Palepou, M. I., Lyon, D. J., Woodford, N., et al. (2001). IMP-4, a novel metallo-beta-lactamase from nosocomial acinetobacter spp. collected in Hong Kong between 1994 and 1998. Antimicrob. Agents Chemother. 45, 710–714. doi: 10.1128/AAC.45.3.710-714.2001
Dorr, M., Silver, A., Smurlick, D., Arukha, A., Kariyawasam, S., Oladeinde, A., et al. (2022). Transferability of ESBL-encoding IncN and IncI1 plasmids among field strains of different salmonella serovars and escherichia coli. J. Glob Antimicrob. Resist. 30, 88–95. doi: 10.1016/j.jgar.2022.04.015
Fernandez, A., Pereira, M. J., Suarez, J. M., Poza, M., Trevino, M., Villalon, P., et al. (2011). Emergence in Spain of a multidrug-resistant enterobacter cloacae clinical isolate producing SFO-1 extended-spectrum beta-lactamase. J. Clin. Microbiol. 49, 822–828. doi: 10.1128/JCM.01872-10
Gardner, S. N., Slezak, T., Hall, B. G. (2015). kSNP3.0: SNP detection and phylogenetic analysis of genomes without genome alignment or reference genome. Bioinformatics 31, 2877–2878. doi: 10.1093/bioinformatics/btv271
Gomez-Simmonds, A., Annavajhala, M. K., Tang, N., Rozenberg, F. D., Ahmad, M., Park, H., et al. (2022). Population structure of blaKPC-harbouring IncN plasmids at a new York city medical centre and evidence for multi-species horizontal transmission. J. Antimicrob. Chemother. 77, 1873–1882. doi: 10.1093/jac/dkac114
Goncalves, D., Cecilio, P., Faustino, A., Iglesias, C., Branca, F., Estrada, A., et al. (2021). Intra- and extra-hospital dissemination of IMP-22-Producing klebsiella pneumoniae in northern Portugal: The breach of the hospital frontier toward the community. Front. Microbiol. 12, 777054. doi: 10.3389/fmicb.2021.777054
Gou, J. J., Liu, N., Guo, L. H., Xu, H., Lv, T., Yu, X., et al. (2020). Carbapenem-resistant enterobacter hormaechei ST1103 with IMP-26 carbapenemase and ESBL gene bla SHV-178. Infect. Drug Resist. 13, 597–605. doi: 10.2147/IDR.S232514
Hickey, C., Nguyen, S., Anes, J., Hurley, D., Donoghue, O., Fanning, S., et al. (2021). Differences in antimicrobial susceptibility testing complicating management of IMP carbapenemase-producing enterobacterales infection. J. Glob Antimicrob. Resist. 27, 284–288. doi: 10.1016/j.jgar.2021.09.010
Hirabayashi, A., Yahara, K., Mitsuhashi, S., Nakagawa, S., Imanishi, T., Ha, V. T. T., et al. (2021). Plasmid analysis of NDM metallo-β-lactamase-producing enterobacterales isolated in Vietnam. PLoS One 16, e0231119. doi: 10.1371/journal.pone.0231119
Huang, T. W., Wang, J. T., Lauderdale, T. L., Liao, T. L., Lai, J. F., Tan, M. C., et al. (2013). Complete sequences of two plasmids in a blaNDM-1-positive klebsiella oxytoca isolate from Taiwan. Antimicrob. Agents Chemother. 57, 4072–4076. doi: 10.1128/AAC.02266-12
Hu, L., Zhong, Q., Shang, Y., Wang, H., Ning, C., Li, Y., et al. (2014). The prevalence of carbapenemase genes and plasmid-mediated quinolone resistance determinants in carbapenem-resistant enterobacteriaceae from five teaching hospitals in central China. Epidemiol. Infect. 142, 1972–1977. doi: 10.1017/S0950268813002975
Lee, J. H., Bae, I. K., Lee, C. H., Jeong, S. (2017). Molecular characteristics of first IMP-4-Producing enterobacter cloacae sequence type 74 and 194 in Korea. Front. Microbiol. 8, 2343. doi: 10.3389/fmicb.2017.02343
Liew, S. M., Rajasekaram, G., Puthucheary, S. D., Chua, K. H. (2018). Detection of VIM-2-, IMP-1- and NDM-1-producing multidrug-resistant pseudomonas aeruginosa in Malaysia. J. Glob Antimicrob. Resist. 13, 271–273. doi: 10.1016/j.jgar.2018.01.026
Matsumoto, Y., Inoue, M. (1999). Characterization of SFO-1, a plasmid-mediated inducible class a beta-lactamase from enterobacter cloacae. Antimicrob. Agents Chemother. 43 (2), 307–313. doi: 10.1128/AAC.43.2.307
Matsumura, Y., Peirano, G., Motyl, M. R., Adams, M. D., Chen, L., Kreiswirth, B., et al. (2017). Global molecular epidemiology of IMP-producing enterobacteriaceae. Antimicrob. Agents Chemother. 72 (8), 2249–2258. doi: 10.1093/jac/dkx148
Mezzatesta, M. L., Gona, F., Stefani, S. (2012). Enterobacter cloacae complex: clinical impact and emerging antibiotic resistance. Future Microbiol. 7, 887–902. doi: 10.2217/fmb.12.61
Nicolas, E., Lambin, M., Dandoy, D., Galloy, C., Nguyen, N., Oger, C. A., et al. (2015). The Tn3-family of replicative transposons. Microbiol. Spectr. 3, 693–726. doi: 10.1128/microbiolspec.MDNA3-0060-2014
Osei Sekyere, J., Reta, M. A. (2021). Global evolutionary epidemiology and resistome dynamics of citrobacter species, enterobacter hormaechei, klebsiella variicola, and proteeae clones. Environ. Microbiol. 23, 7412–7431. doi: 10.1111/1462-2920.15387
Riccio, M. L., Franceschini, N., Boschi, L., Caravelli, B., Cornaglia, G., Fontana, R., et al. (2000). Characterization of the metallo-beta-lactamase determinant of acinetobacter baumannii AC-54/97 reveals the existence of bla(IMP) allelic variants carried by gene cassettes of different phylogeny. Antimicrob. Agents Chemother. 44, 1229–1235. doi: 10.1128/AAC.44.5.1229-1235.2000
Roberts, L. W., Catchpoole, E., Jennison, A. V., Bergh, H., Hume, A., Heney, C., et al. (2020a). Genomic analysis of carbapenemase-producing enterobacteriaceae in Queensland reveals widespread transmission of bla (IMP-4) on an IncHI2 plasmid. Microb. Genom 6 (1), e000321. doi: 10.1099/mgen.0.000321
Roberts, L. W., Harris, P. N. A., Forde, B. M., Ben Zakour, N. L., Catchpoole, E., Stanton-Cook, M., et al. (2020b). Integrating multiple genomic technologies to investigate an outbreak of carbapenemase-producing enterobacter hormaechei. Nat. Commun. 11, 466. doi: 10.1038/s41467-019-14139-5
Sellera, F. P., Fuga, B., Fontana, H., Esposito, F., Cardoso, B., Konno, S., et al. (2021). Detection of IncN-pST15 one-health plasmid harbouring bla(KPC-2) in a hypermucoviscous Klebsiella pneumoniae CG258 isolated from an infected dog, Brazil. Transbound Emerg Dis 68, 3083–3088. doi: 10.1111/tbed.14006.
Sidjabat, H. E., Townell, N., Nimmo, G. R., George, N. M., Robson, J., Vohra, R., et al. (2015). Dominance of IMP-4-producing enterobacter cloacae among carbapenemase-producing enterobacteriaceae in Australia. Antimicrob. Agents Chemother. 59, 4059–4066. doi: 10.1128/AAC.04378-14
Souque, C., Escudero, J. A., Maclean, R. C. (2021). Integron activity accelerates the evolution of antibiotic resistance. Elife, 10. doi: 10.7554/eLife.62474.
Sullivan, M. J., Petty, N. K., Beatson, S. A. (2011). Easyfig: A genome comparison visualizer. Bioinformatics 27, 1009–1010. doi: 10.1093/bioinformatics/btr039
Tarabai, H., Wyrsch, E. R., Bitar, I., Dolejska, M., Djordjevic, S. P. (2021). Epidemic HI2 plasmids mobilising the carbapenemase gene bla(IMP-4) in Australian clinical samples identified in multiple sublineages of escherichia coli ST216 colonising silver gulls. Microorganisms 9 (3), 567. doi: 10.3390/microorganisms9030567
Wang, Y., Lo, W. U., Lai, R. W., Tse, C. W., Lee, R. A., Luk, W. K., et al. (2017). IncN ST7 epidemic plasmid carrying blaIMP-4 in enterobacteriaceae isolates with epidemiological links to multiple geographical areas in China. J. Antimicrob. Chemother. 72, 99–103. doi: 10.1093/jac/dkw353
Wang, S., Xu, L., Chi, X., Li, Y., Kou, Z., Hou, P., et al. (2019). Emergence of NDM-1- and CTX-M-3-Producing raoultella ornithinolytica in human gut microbiota. Front. Microbiol. 10, 2678. doi: 10.3389/fmicb.2019.02678
Watanabe, M., Iyobe, S., Inoue, M., Mitsuhashi, S. (1991). Transferable imipenem resistance in pseudomonas aeruginosa. Antimicrob. Agents Chemother. 35, 147–151. doi: 10.1128/AAC.35.1.147
Xu, Y., Liu, Y., Liu, Y., Pei, J., Yao, S., Cheng, C. (2015). Bacteriophage therapy against enterobacteriaceae. Virol. Sin. 30, 11–18. doi: 10.1007/s12250-014-3543-6
Xu, H., Wang, X., Yu, X., Zhang, J., Guo, L., Huang, C., et al. (2018). First detection and genomics analysis of KPC-2-producing citrobacter isolates from river sediments. Environ. pollut. 235, 931–937. doi: 10.1016/j.envpol.2017.12.084
Yan, J. J., Ko, W. C., Wu, J. J. (2001). Identification of a plasmid encoding SHV-12, TEM-1, and a variant of IMP-2 metallo-beta-lactamase, IMP-8, from a clinical isolate of klebsiella pneumoniae. Antimicrob. Agents Chemother. 45, 2368–2371. doi: 10.1128/AAC.45.8.2368-2371.2001
Yao, Y., Falgenhauer, L., Falgenhauer, J., Hauri, A. M., Heinmüller, P., Domann, E., et al. (2021). Carbapenem-resistant citrobacter spp. as an emerging concern in the hospital-setting: Results from a genome-based regional surveillance study. Front. Cell Infect. Microbiol. 11, 744431. doi: 10.3389/fcimb.2021.744431
Zhang, Y., Gu, D., Yang, X., Wu, Y., Liu, C., Shen, Z., et al. (2021a). Emergence and genomic characterization of a KPC-2-, NDM-1-, and IMP-4-Producing klebsiella michiganensis isolate. Front. Microbiol. 12, 762509. doi: 10.3389/fmicb.2021.762509
Zhang, Y., Wang, X., Wang, Q., Chen, H., Li, H., Wang, S., et al. (2021b). Emergence of tigecycline nonsusceptible and IMP-4 carbapenemase-producing K2-ST65 hypervirulent klebsiella pneumoniae in China. Microbiol. Spectr. 9, e0130521. doi: 10.1128/Spectrum.01305-21
Zhou, K., Yu, W., Shen, P., Lu, H., Wang, B., Rossen, J. W. A., et al. (2017). A novel Tn1696-like composite transposon (Tn6404) harboring bla (IMP-4) in a klebsiella pneumoniae isolate carrying a rare ESBL gene bla (SFO-1). Sci. Rep. 7, 17321. doi: 10.1038/s41598-017-17641-2
Zhou, K., Zhou, Y., Zhang, C., Song, J., Cao, X., Yu, X., et al. (2020). Dissemination of a 'rare' extended-spectrum beta-lactamase gene blaSFO-1 mediated by epidemic clones of carbapenemase-producing enterobacter hormaechei in China. Int. J. Antimicrob. Agents 56, 106079. doi: 10.1016/j.ijantimicag.2020.106079
Keywords: Enterobacter hormaechei, ST51, blaIMP-4, blaSFO-1, IncN, multidrug-resistant, genomics
Citation: Qiao J, Ge H, Xu H, Guo X, Liu R, Li C, Chen R, Zheng B and Gou J (2022) Detection of IMP-4 and SFO-1 co-producing ST51 Enterobacter hormaechei clinical isolates. Front. Cell. Infect. Microbiol. 12:998578. doi: 10.3389/fcimb.2022.998578
Received: 20 July 2022; Accepted: 13 October 2022;
Published: 27 October 2022.
Edited by:
Milena Dropa, Faculty of Public Health, University of São Paulo, BrazilReviewed by:
Farzad Badmasti, Pasteur Institute of Iran, IranYancheng Yao, University of Giessen, Germany
Copyright © 2022 Qiao, Ge, Xu, Guo, Liu, Li, Chen, Zheng and Gou. This is an open-access article distributed under the terms of the Creative Commons Attribution License (CC BY). The use, distribution or reproduction in other forums is permitted, provided the original author(s) and the copyright owner(s) are credited and that the original publication in this journal is cited, in accordance with accepted academic practice. No use, distribution or reproduction is permitted which does not comply with these terms.
*Correspondence: Jianjun Gou, amlhbmp1bmdAenp1LmVkdS5jbg==; Beiwen Zheng, emhlbmdid0B6anUuZWR1LmNu
†These authors have contributed equally to this work and share first authorship