- 1Department of Bacteriology, Capital Institute of Pediatrics, Beijing, China
- 2Department of Pulmonology, The Affiliated Children’s Hospital, Capital Institute of Pediatrics, Beijing, China
- 3Institute of Hepatology, Chinese People Liberation Army General Hospital, Beijing, China
The Burkholderia cepacia complex (BCC) is a group of opportunistic pathogens, including Burkholderia cepacia, Burkholderia multivorans, Burkholderia vietnamiensis and Burkholderia ambifaria, which can cause severe respiratory tract infections and lead to high mortality rates among humans. The early diagnosis and effective treatment of BCC infection are therefore crucial. In this study, a novel and rapid recombinase-aided amplification (RAA) assay targeting the 16S rRNA gene was developed for BCC detection. The protocol for this RAA assay could be completed in 10 min at 39°C, with a sensitivity of 10 copies per reaction and no cross-reactivity with other pathogens. To characterize the effectiveness of the RAA assay, we further collected 269 clinical samples from patients with bacterial pneumonia. The sensitivity and specificity of the RAA assay were 100% and 98.5%, respectively. Seven BCC-infected patients were detected using the RAA assay, and three BCC strains were isolated from the 269 clinical samples. Our data showed that the prevalence of BCC infection was 2.60%, which is higher than the 1.40% reported in previous studies, suggesting that high sensitivity is vital to BCC detection. We also screened a patient with B. vietnamiensis infection using the RAA assay in clinic, allowing for appropriate treatment to be initiated rapidly. Together, these data indicate that the RAA assay targeting the 16S rRNA gene can be applied for the early and rapid detection of BCC pathogens in patients with an uncharacterized infection who are immunocompromised or have underlying diseases, thereby providing guidance for effective treatment.
Introduction
The Burkholderia cepacia complex (BCC) is a group of over 20 phenotypically similar but genetically different Gram-negative, non-fermenting, bacteria that includes Burkholderia cepacia, Burkholderia multivorans, Burkholderia vietnamiensis and Burkholderia ambifaria (Depoorter et al., 2016; Sfeir, 2018; Tavares et al., 2020). Members of the BCC are opportunistic pathogens that can cause severe respiratory infections in immunocompromised patients (such as those with congenital immunodeficiency, HIV, and cancer patients receiving chemotherapy) or patients with underlying diseases such as cystic fibrosis (CF) and chronic granulomatous (Kalish et al., 2006; Greenberg et al., 2009; Tavares et al., 2020). According to a previous study in Nepal, the infection rate of BCC was 1.4% in patients who received more than 48 hours of mechanical ventilation (Baidya et al., 2021). The 2017 CF Foundation Annual Report on Patient Registrations showed that the occurrence of BCC was 2.4% in CF patients (Blanchard and Waters, 2019). Although the BCC only accounts for a small proportion of lung infections in CF patients, BCC infection accelerates lung function deterioration, which leads to a poor prognosis and high mortality (Whiteford et al., 1995; Stephenson et al., 2015). This high mortality is generally caused by the progression of “cepacia syndrome”, which is clinically characterized by necrotizing pneumonia, sepsis, hyperthermia, and even severe progressive respiratory failure (Lord et al., 2020; Lauman and Dennis, 2021). In view of the significant morbidity and mortality associated with BCC infection, rapid and accurate detection is vital to initiate timely and effective treatment. Currently, BCC identification mainly involves isolation on selective media and DNA-based detection methods such as PCR and qPCR (De Volder et al., 2021). However, novel methods that offer high sensitivity and specificity, while being quick and easy to perform, are much needed (Ahn et al., 2020).
Recombinase-aided amplification (RAA) is a rapid, specific, sensitive and reliable isothermal gene amplification technology (Wang et al., 2021). At present, RAA has been widely used in the detection of various pathogens such as SARS-CoV-2, adenovirus, hepatitis B virus, Escherichia coli, Klebsiella pneumoniae, Mycoplasma pneumoniae, Salmonella and Vibrio parahaemolyticus (Zhang et al., 2017; Shen et al., 2019; Wang et al., 2019; Xue et al., 2020; Mu et al., 2021; Zhang et al., 2021; Zheng et al., 2021; Feng et al., 2022). RAA achieves DNA amplification by employing recombinase UvsX, DNA polymerase and single-stranded DNA binding protein at 35°C–42°C, replacing the traditional thermal cycling process (Fan et al., 2020). With the addition of 6-carboxyfluorescein (FAM)-labeled probes and biotin-labeled primers, double-labeled amplification products can be obtained, making the assay sensitive and specific (He et al., 2021).
In this study, pairs of primers and probes targeting the 16S rRNA gene, which is highly conserved and specific for BCC species, were designed, and a rapid and intuitive RAA assay with high sensitivity and specificity for BCC detection was established. To assess the applicability of the RAA assay in clinic, 269 clinical samples were detected, and conventional PCR was also performed for comparison.
Material and methods
Ethical approval
This study was performed in compliance with the Helsinki Declaration (Ethical Principles for Medical Research Involving Human Subjects) and was approved by the research board of the Ethics Committee of the Capital Institute of Pediatrics, Beijing, China (SHERLLM2022004). All specimens used in this study are part of routine patient management without any additional collection, and all patient data were anonymously reported.
Bacterial strains
The RAA assay was evaluated with 12 clinically-common pathogens, including Haemophilus influenzae, Mycobacterium tuberculosis, Staphylococcus aureus, Pseudomonas aeruginosa, K. pneumoniae, E. coli, M. pneumoniae, B. cepacia, B. multivorans, B. vietnamiensis, B. ambifaria and B. gladioli. The details of these strains are shown in Table S1.
DNA extraction and BCC isolation of clinical samples
A total of 269 bronchoalveolar lavage fluid, throat swab or sputum samples from patients with bacterial pneumonia were randomly collected. Total DNA was extracted from the 269 collected samples using the QIAamp DNA Mini Kit (Qiagen, Hilden, Germany) and was stored at −80°C. BCC standard strains and the clinical samples were plated onto B. cepacia selective agar (BCSA) for 2 days at 35°C, followed by 3 days of incubation at room temperature (Martina et al., 2020).
Recombinant plasmid construction
The PCR amplification product of the 16S rRNA gene (BCC, GenBank accession number: LC496395) was cloned into the vector pGM-T (Tiangen Biotech, Beijing, China) according to the manufacturer’s instructions. The primers used for amplification are shown in Table 1. The concentration of plasmid was detected using a NanoDrop spectrophotometer. Then, the standard recombinant plasmids were prepared at 10-fold dilutions ranging from 106 copies/µL to 100 copies/µL for sensitivity analysis. The plasmid concentration and copy number were determined using the formula: DNA copy number (copy number/µL) = [6.02×1023 × plasmid concentration (ng/µL) ×10−9]/[DNA length (in nucleotides) × 660]. The length of the DNA (3226 bp) was the sum of the plasmid fragment length (211 bp) and the vector length (3015 bp).
RAA primer design
The sequence of the 16S rRNA gene was used for RAA primer design. According to the principles of RAA primer and probe design (primer length between 30 to 35 bp, probe length between 46 to 52 bp), four sets of primers and probes were manually designed in highly-conserved regions. Primer and probe specificities were analyzed using NCBI Primer-BLAST, and the formation of primer dimers and secondary structures (hairpins) was predicted using the Website OligoEvaluator (http://www.oligoevaluator.com/LoginServlet). The primers and probes were synthesized and purified using high-performance liquid chromatography (Sangon Biotech, Shanghai, China).
RAA assay
The RAA assay was performed in a 50 μL reaction volume using a commercial RAA kit (Qitian, Jiangsu, China). The reaction mixture included 2 μL extracted DNA template, 25 μL reaction buffer, 15.7 μL DNase-free water, 2.1 μL primer F/R, 0.6 μL of probe and 2.5 μL of magnesium acetate. The reaction mixture was added to the tube containing the RAA enzyme mixture in lyophilized form. The test tubes were placed in a B6100 shaking mixer (QT-RAA-B6100, Qitian), incubated for 4 min, mixed briefly, centrifuged, and finally transferred to a fluorescence detector (QT-RAA-1620, Qitian), and measured at 39°C for 10 min.
Sensitivity and specificity of the RAA assay
The sensitivity of the RAA assay was assessed using a series of diluted recombinant plasmids ranging from 106 to 100 copies/μL. The specificity was evaluated by detecting four strains of different BCC subspecies, one strain of B. gladioli (which does not belong to the BCC) and seven clinically-common pathogens, as presented in Table S1. The positive control was 16S rRNA-positive plasmid, and the negative control was nucleic acid-free water.
PCR assay
PCR primers were designed according to primer design principles (Table 1). The conventional PCR assay was performed in a 25 μL reaction system containing 12.5 μL 2 × Taq PCR MasterMix (Tiangen Biotech), 1.0 μL of each primer and 2 μL of extracted DNA template. The reaction program was set as 95°C for 10 min, followed by 35 cycles of 30 s at 95°C, 30 s at 55°C and 1 min at 72°C, and a final extension step for 10 min at 72°C. PCR products were visualized on a 1.5% agarose gel and stained with GeneGreen. Images were acquired with a Gel Doc EQ imaging system (Bio-Rad, Hercules, USA), and the amplified product was sequenced (Sangon Biotech).
Evaluating the RAA assay on clinical samples
Established RAA assay was evaluated using 269 clinical samples from patients with bacterial pneumoniae. The RAA assay results were compared to conventional PCR assay. The formulas for evaluating RAA assay were as follows: consistency between RAA and conventional PCR assays = (true positives + true negatives)/(true positives + true negatives + false positives + false negatives) × 100%, sensitivity of RAA assay = true positives/(true positives + false negatives) × 100%, specificity of RAA assay = true negatives/(false positives + true negatives) × 100%, and the conventional PCR method was used as gold standard for identification (Gautam et al., 2017). A case was also used for assessing the clinical application of RAA assay for detecting BCC infection in this study. The pus sample extracted from the patient’s neck abscess and sputum were used for BCC isolation, RAA and metagenomic sequencing (Vison Medicals, Guangzhou, China).
Genome sequencing and analysis
BCC isolates were subjected to DNA extraction using the Wizard Genome DNA Purification Kit (Promega, Madison, USA) and sequenced on the Illumina HiSeq PE150 platform (Novogene, Beijing, China). The genome sequences were assembled and annotated by software SOAP denovo and Prokka. Multilocus sequence typing (MLST) identification was performed using the Institute Pasteur database (https://bigsdb.pasteur.fr/klebsiella/klebsiella.html) by analyzing the sequences of seven housekeeping genes (atpD, gltB, gyrB, recA, lepA, phaC and trpB) (Diancourt et al., 2005). The core genome single-nucleotide polymorphisms (SNPs) of BCC strains were detected using the software Snippy and Gubbins, as previously described (Gan et al., 2022). The extracted SNPs were used for phylogenetic tree construction by software MEGA. Strains MSMB384WGS and FL_2_3_10_S3_D0 were used as reference strains for B. cepacia phylogenetic tree and B. vietnamiensis phylogenetic tree, respectively (Croucher et al., 2015). Strain ATCC10248 (B. gladioli) was included as an outgroup. All strains used for constructing the phylogenetic tree are listed in Table S2.
Results
Primers and probes designed for the RAA assay
Four sets of RAA primers and probes named 16S-1 (Figure 1 and Table 1), 16S-2, 16S-3 and 16S-4 (Table S3) were manually designed against the conserved region of the 16S rRNA gene of BCC. No cross-reactivity with other species was predicted by the BLAST tool. The amplification efficiencies of the four sets of primers and probes were compared, and the recombinant 16S rRNA plasmid was used as the positive template and nucleic acid-free water was used as the negative template. All reactions were performed in triplicate. Under the same reaction condition and system, 16S-1 exhibited the highest amplification efficiency compared to other sets (Supplementary Figure 1). Therefore, 16S-1 was chosen as the primers and probe for the subsequent analysis.
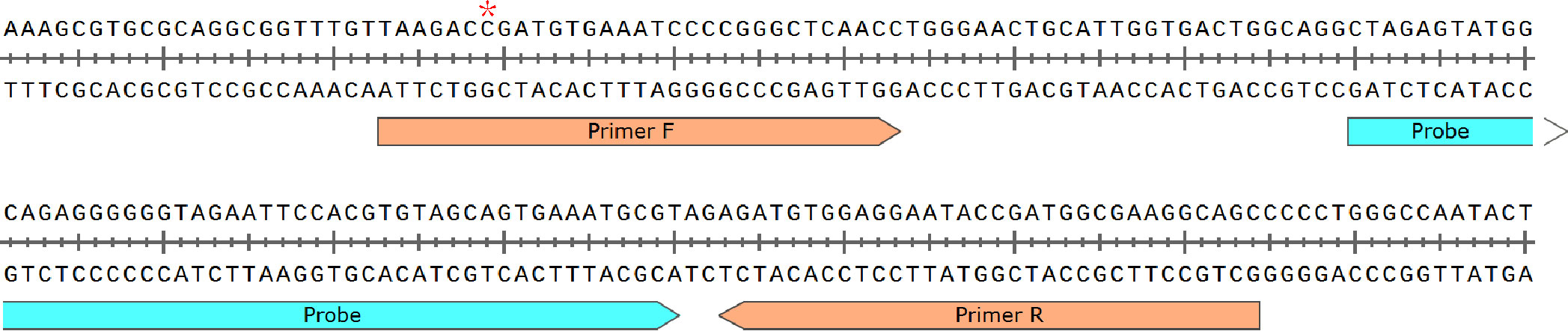
Figure 1 Primer and probe sequences used in RAA detection. * denotes the site with multiple subtypes between BCC species, Burkholderia cepacia is C, Burkholderia multivorans is A or C, Burkholderia vietnamiensis is C, and Burkholderia ambifaria is C.
Sensitivity of the RAA assay
The template concentration of the recombinant plasmid determined by the NanoDrop spectrophotometer was 153.6 ng/µL, and the converted copy number was 4.34×1010 copies/µL. The 10-fold serially diluted recombinant plasmids ranged in concentration from 106 to 100 copies/µL. The results of sensitivity analysis are shown in Figure 2A. The RAA assay detected the 16S rRNA gene within 10 min at 39°C at a sensitivity of 10 copies/µL. The minimum concentration of conventional PCR was 103 copies/µL.
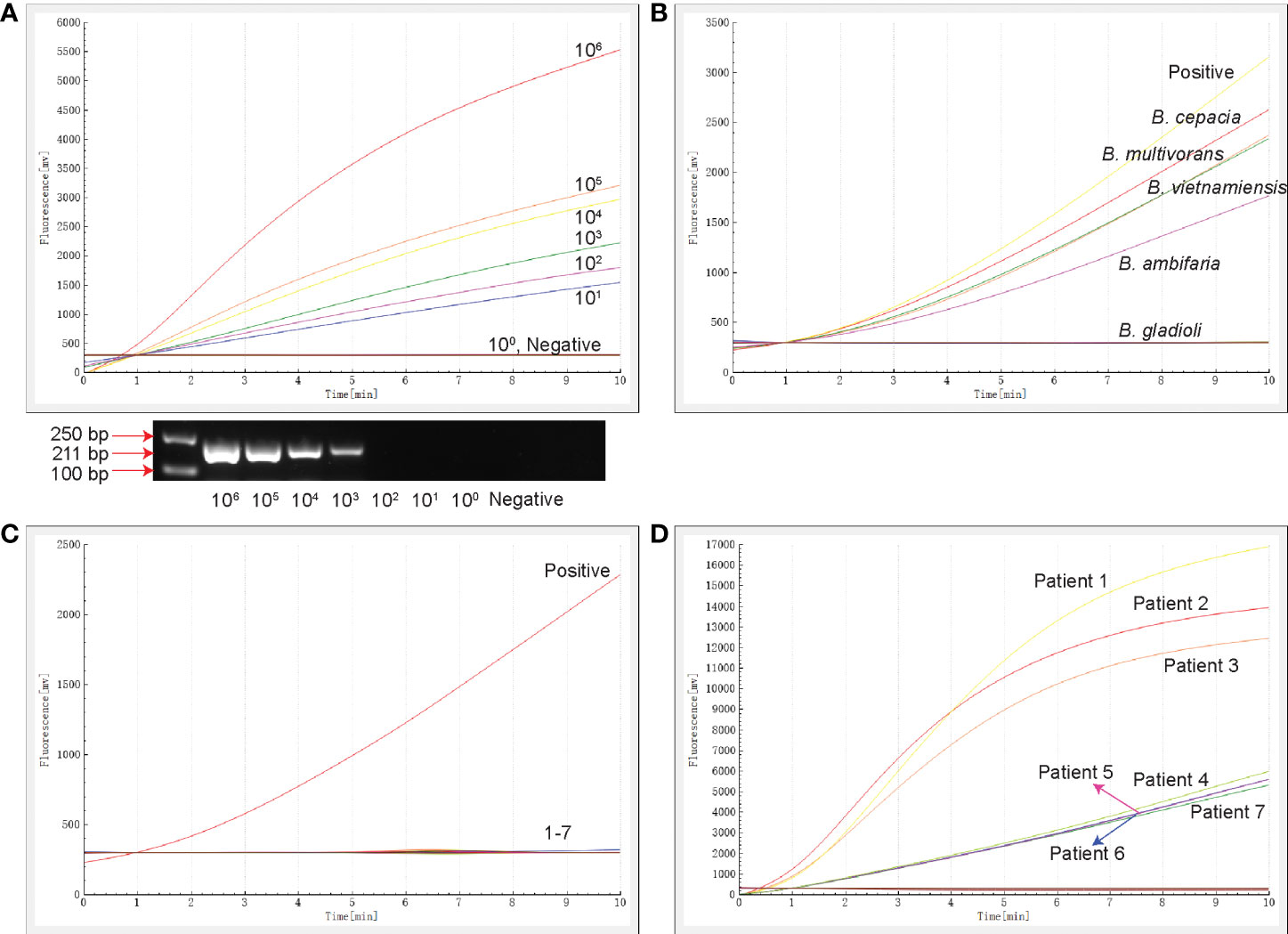
Figure 2 The sensitivity, specificity and clinical testing of the RAA assay. (A) The sensitivity of RAA and conventional PCR assays. The results of RAA are shown by an amplification curve, and the PCR amplification products are shown following separation on a 1.5% agarose gel. (B) The specificity of the RAA assay for members of the Burkholderia family. (C) The specificity of RAA for clinically-common pathogens. 1: Haemophilus influenzae ATCC10211, 2: M. tuberculosis ATCC25618, 3: Staphylococcus aureus ATCC29213, 4: Pseudomonas aeruginosa ATCC27853, 5: K. pneumoniae ATCC BAA-2146, 6: E. coli ATCC25922, 7: Mycoplasma pneumoniae M129 ATCC 29342. (D) Seven samples tested positive by the RAA assay. The positive control was 16S rRNA-positive plasmid, and the negative control was nucleic acid-free water.
Specificity of the RAA assay
The specificity of the RAA assay was confirmed by the detection of four strains of different BCC subspecies and eight clinically-common pathogens (Table S1). Only B. cepacia, B. multivorans, B. vietnamiensis and B. ambifaria produced fluorescence signals (Figure 2B), while the other strains tested, including B. gladioli that does not belong to the BCC, were negative (Figures 2B, C). No cross-reactivity with strains of other species being detected in this RAA assay targeted 16S rRNA.
Evaluation of the RAA assay using clinical samples
A total of 269 specimens collected from patients with bacterial pneumonia were simultaneously detected by RAA and conventional PCR assays (Table 2). Seven samples (2.6%) tested positive and 262 samples tested negative for the BCC by the RAA assay (Figure 2D), whereas three cases (1.1%) tested positive and 266 cases tested negative in conventional PCR assay. Among them, four samples were positive by RAA and negative by the conventional PCR assay. The percentage consistency between RAA and PCR assays was 98.5%. Compared with the gold standard PCR method, the RAA assay had a sensitivity of 100% and a specificity of 98.5%.
To investigate the value of the RAA assay in terms of clinical application, we also analyzed a patient with B. vietnamiensis infection in detail. The 7-year-old girl was diagnosed with chronic granulomatous disease. To determine the cause of infection, cervical lymph node puncture sample was collected for metagenomic sequencing, and the results indicated B. vietnamiensis infection. Meanwhile, BCC infection was further confirmed by the RAA assay targeting the 16S rRNA gene, and a BCC isolate was successfully isolated from a pus sample, confirming the reliability of RAA assay for clinical diagnosis.
The prevalence of BCC infection among patients with pneumonia was 2.60%, and three strains were isolated from these positive samples. Isolates BC1 (ST608, Genome accession number SAMN29388125) and BC2 (ST608, Genome accession number SAMN29388126) belonged to B. cepacia, and isolate Vit1 (Genome accession number SAMN29388124) belonged to B. vietnamiensis (atpD type 27, gltB type 231, gyrB type 16, recA type 22, lepA type 12, phaC 5 type 6 and trpB type 268, which was a new sequence type). In the phylogenetic tree of B. cepacia, isolates BC1 and BC2 clustered with strains collected from patients in China (toggle2 and toggle3), suggesting that these four strains from China were closely related phylogenetically and potentially shared epidemiological characteristics (Figure 3A). In the B. vietnamiensis phylogenetic tree, isolate Vit1 was separate from the Chinese strains, indicating that it shared no phylogenetic or epidemiological characteristics (Figure 3B).
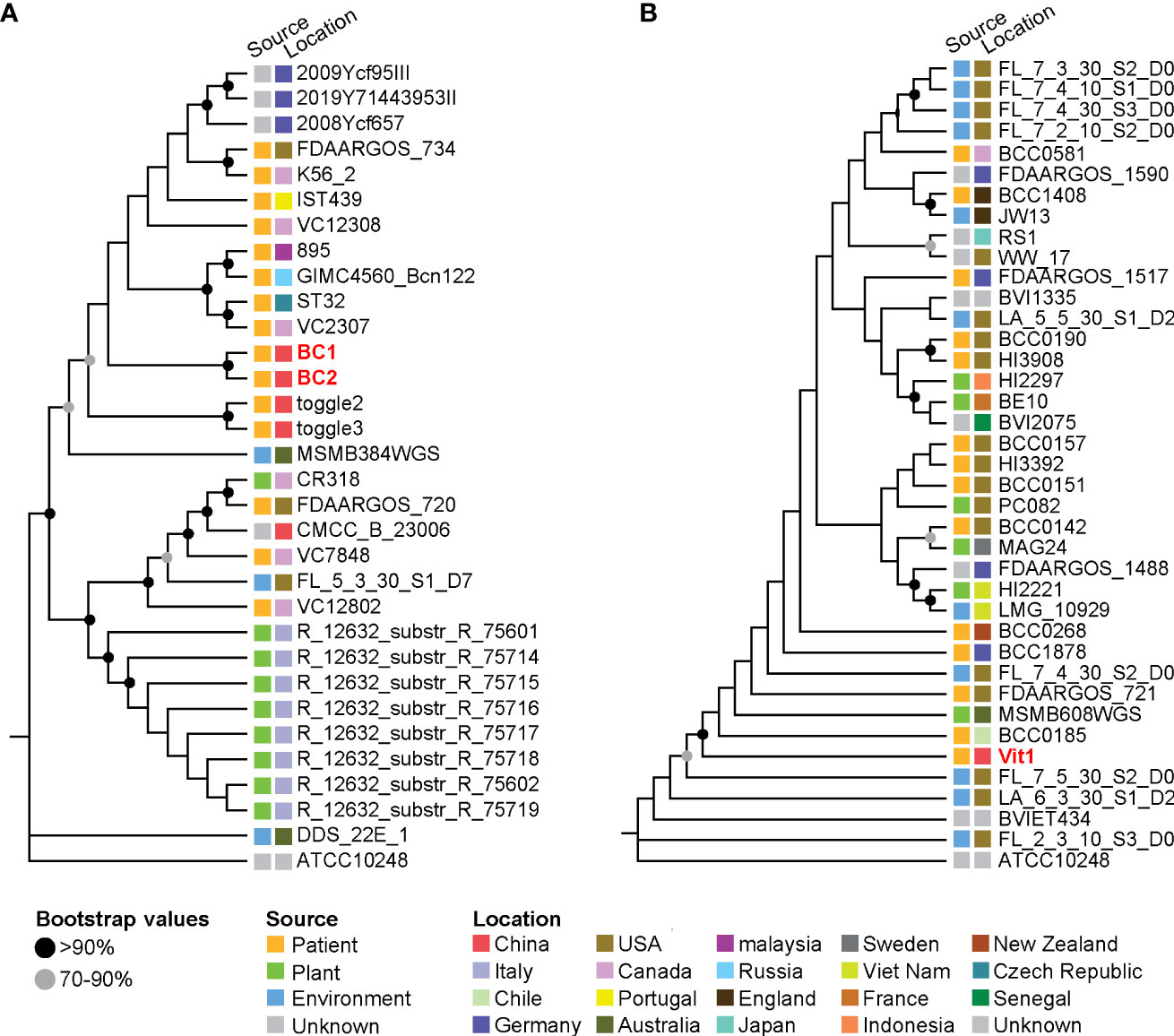
Figure 3 Phylogenetic trees of Burkholderia strains based on core single-nucleotide polymorphisms. (A) Phylogenetic tree of B. cepacia strains. (B) Phylogenetic tree of B. vietnamiensis strains. The squares beside the strains denote the source and location of the strains.
Discussion
BCC pathogens are an important cause of secondary severe pulmonary infection in patients with a congenital immunodeficiency or existing underlying disease, and earlier identification of pathogens means more rapid intervention and therefore better clinical outcomes. However, BCC pathogens typically require 48 to 72 hours of incubation for colonies to appear on selective media (Brown and Govan, 2007). Currently, BCC identification mainly depends on molecular methods (Drevinek et al., 2008), but the sensitivity of PCR assays varies widely. Moreover, BCC diagnosis also faces challenges posed by non-BCC Burkholderia species, such as B. gladioli (Brown and Govan, 2007), and a detection method is urgently needed to distinguish these organisms. In recent years, RAA has been successfully applied to the detection of many pathogens and SNPs (Qin et al., 2021). Because of its fast speed, low cost and high sensitivity, RAA is potentially suitable for clinical application.
Multiple copies of rRNA genes are present throughout the bacterial genome. Therefore, 16S rRNA gene-based PCR assays generally have higher sensitivity than PCR assays targeting single-copy genes such as recA (Brown and Govan, 2007). In this study, the gene 16S rRNA of BCC was selected through detailed analysis of conserved regions in multiple sequence alignments. The 16S rRNA gene shows no cross-homology and meets the requirements for designing BCC-specific RAA primers. The specificity and sensitivity of the RAA primers were verified by BLAST analysis and subsequent experiments.
The RAA assay targeting the 16S rRNA gene of BCC has the ability to detect a minimum template of 10 copies/µL, and the sensitivity is similar to that of RAA detection of other pathogens (Xiao et al., 2021), which was more sensitive than conventional PCR, qPCR and LAMP assays of BCC (Daddy Gaoh et al., 2021). Compared with conventional PCR ($45/sample, >2 h detection speed) (Pliakos et al., 2018), RAA has a lower cost ($5/sample), faster detection speed (10 min) and simpler reaction conditions, not requiring complex instruments. For samples with 10 copies/µL of BCC, a positive result can be obtained within 10 min under a constant temperature of 39°C. The RAA assay in this study did not cross-react with eight other species of pathogens tested, including one species of non-BCC Burkholderia and seven clinically-common species. Of note, we employed four species of the BCC and one non-BCC Burkholderia species (B. gladioli) to verify the specificity of the method, and the results showed that this method can effectively distinguish between BCC and non-BCC species.
To evaluate the clinical applicability of this method, we further collected 269 clinical samples for BCC detection. The assay had a sensitivity of 100% and a specificity of 98.5%, which was superior to other reported assays (Daddy Gaoh et al., 2021). In particular, the four samples that tested negative for conventional PCR but positive for RAA also exhibited low peak values for the RAA assay. Moreover, previous studies have shown a low rate of infection with BCC (Baidya et al., 2021), but the results of the RAA assay in this study suggest a higher prevalence. BCC screening in specific populations may be strengthened through the application of the RAA assay in the future.
We carried out a case study of a child with B. vietnamiensis infection to test the applicability of RAA for clinical detection. The patient underwent recurrent infection as a result of congenital immunodeficiency, chronic granulomatous disease and the heavy use of antibiotics. The RAA assay in this study was able to detect B. multivorans, B. vietnamiensis and other common clinical BCC members (Rhodes and Schweizer, 2016). Thus, if patients with a similar course of disease were observed in clinic in the future, our RAA assay could be applied to quickly detect BCC infection. It is worth noting that the treatment of BCC infection relies on ceftazidime and other broad-spectrum cephalosporins (Rhodes and Schweizer, 2016). Although imipenem (a broad-spectrum β-lactam antibiotic) can be effective with clinical recovery occurring at a later stage, doctors often employ a large number of antibiotics to target a range of pathogens when the causative pathogen is unknown at an early stage. In the future, the aim would be to achieve early and rapid pathogen detection through RAA, enabling early targeted treatment and thereby avoiding the overuse of antibiotics.
Taken together, we established an RAA assay for BCC detection, which has high specificity and sensitivity. RAA has the advantages of simple reaction conditions, a short reaction time and low cost. The applicability of the assay was also verified in clinic. However, future studies are needed to analyze a greater number of clinical samples to further verify the effectiveness of the assay in the early detection of BCC infection.
Data availability statement
The datasets presented in this study can be found in online repositories. The names of the repository/repositories and accession number(s) can be found in the article/Supplementary Material.
Ethics statement
The studies involving human participants were reviewed and approved by This study was performed in compliance with the Helsinki Declaration (Ethical Principles for Medical Research Involving Human Subjects) and was approved by the research board of the Ethics Committee of the Capital Institute of Pediatrics, Beijing, China (SHERLLM2022004). All specimens used in this study are part of routine patient management without any additional collection, and all patient data were anonymously reported. Written informed consent to participate in this study was provided by the participants’ legal guardian/next of kin.
Author contributions
JY and LC designed the study and revised the manuscript. HF, LG, ZT, JH, BD, GX, YF, HZ, JC, CY, JF, ZF, TF, ZX, RZ, XC, SD, YZ and QZ performed the experiments. JH and YF collected the clinical samples. HF and LG analyzed the results. HF and LG wrote the manuscript. All authors contributed to the article and approved the submitted version.
Funding
This work was financially supported by grants from the National Natural Science Foundation (82002191, 82130065 and 32170201), Public service development and reform pilot project of Beijing Medical Research Institute (BMR2019-11) and Feng Foundation (FFBR 202103).
Conflict of interest
The authors declare that the research was conducted in the absence of any commercial or financial relationships that could be construed as a potential conflict of interest.
Publisher’s note
All claims expressed in this article are solely those of the authors and do not necessarily represent those of their affiliated organizations, or those of the publisher, the editors and the reviewers. Any product that may be evaluated in this article, or claim that may be made by its manufacturer, is not guaranteed or endorsed by the publisher.
Supplementary material
The Supplementary Material for this article can be found online at: https://www.frontiersin.org/articles/10.3389/fcimb.2022.984140/full#supplementary-material
Supplementary Figure 1 | Four sets of primers and probes used for RAA assay. The DNA template used in this assay was extracted from recombinant plasmid, and the negative control was nucleic acid-free water.
References
Ahn, Y., Gibson, B., Williams, A., Alusta, P., Buzatu, D., Lee, Y., et al. (2020). A comparison of culture-based, real-time PCR, droplet digital PCR and flow cytometric methods for the detection of burkholderia cepacia complex in nuclease-free water and antiseptics. J. Ind. Microbiol. Biotechnol. 47, 475–484. doi: 10.1007/s10295-020-02287-3
Baidya, S., Sharma, S., Mishra, S., Kattel, H., Parajuli, K., Sherchand, J. (2021). Biofilm formation by pathogens causing ventilator-associated pneumonia at intensive care units in a tertiary care hospital: An armor for refuge. BioMed. Res. Int. 2021, 8817700. doi: 10.1155/2021/8817700
Blanchard, A., Waters, V. (2019). Microbiology of cystic fibrosis airway disease. Semin. Respir. Crit. Care Med. 40, 727–736. doi: 10.1055/s-0039-1698464
Brown, A., Govan, J. (2007). Assessment of fluorescent in situ hybridization and PCR-based methods for rapid identification of burkholderia cepacia complex organisms directly from sputum samples. J. Clin. Microbiol. 45, 1920–1926. doi: 10.1128/JCM.00147-07
Croucher, N., Page, A., Connor, T., Delaney, A., Keane, J., Bentley, S., et al. (2015). Rapid phylogenetic analysis of large samples of recombinant bacterial whole genome sequences using gubbins. Nucleic Acids Res. 43, e15. doi: 10.1093/nar/gku1196
Daddy Gaoh, S., Kweon, O., Lee, Y., Lipuma, J., Hussong, D., Marasa, B., et al. (2021). Burkholderia cepaciaLoop-mediated isothermal amplification (LAMP) assay for detecting complex in non-sterile pharmaceutical products. Pathogens 10 (9), 1071. doi: 10.3390/pathogens10091071
Depoorter, E., Bull, M., Peeters, C., Coenye, T., Vandamme, P., Mahenthiralingam, E. (2016). Burkholderia: an update on taxonomy and biotechnological potential as antibiotic producers. Appl. Microbiol. Biotechnol. 100, 5215–5229. doi: 10.1007/s00253-016-7520-x
De Volder, A., Teves, S., Isasmendi, A., Pinheiro, J., Ibarra, L., Breglia, N., et al. (2021). Distribution of burkholderia cepacia complex species isolated from industrial processes and contaminated products in Argentina. Int. microbiol.: Off. J. Spanish Soc. Microbiol. 24, 157–167. doi: 10.1007/s10123-020-00151-z
Diancourt, L., Passet, V., Verhoef, J., Grimont, P., Brisse, S. (2005). Multilocus sequence typing of klebsiella pneumoniae nosocomial isolates. J. Clin. Microbiol. 43, 4178–4182. doi: 10.1128/JCM.43.8.4178-4182.2005
Drevinek, P., Baldwin, A., Dowson, C., Mahenthiralingam, E. (2008). Diversity of the parB and repA genes of the burkholderia cepacia complex and their utility for rapid identification of burkholderia cenocepacia. BMC Microbiol. 8, 44. doi: 10.1186/1471-2180-8-44
Fan, X., Li, L., Zhao, Y., Liu, Y., Liu, C., Wang, Q., et al. (2020). Clinical validation of two recombinase-based isothermal amplification assays (RPA/RAA) for the rapid detection of African swine fever virus. Front. Microbiol. 11, 1696. doi: 10.3389/fmicb.2020.01696
Feng, Z., Li, J., Zhang, J., Li, F., Guan, H., Zhang, R., et al. (2022). Development and evaluation of a sensitive recombinase aided amplification assay for rapid detection of vibrio parahaemolyticus. J. Microbiol. Methods 193, 106404. doi: 10.1016/j.mimet.2021.106404
Gan, L., Yan, C., Cui, J., Xue, G., Fu, H., Du, B., et al. (2022). Genetic diversity and pathogenic features in klebsiella pneumoniae isolates from patients with pyogenic liver abscess and pneumonia. Microbiol. Spectr. 10, e0264621. doi: 10.1128/spectrum.02646-21
Gautam, V., Sharma, M., Singhal, L., Kumar, S., Kaur, P., Tiwari, R., et al. (2017). MALDI-TOF mass spectrometry: An emerging tool for unequivocal identification of non-fermenting gram-negative bacilli. Indian J. Med. Res. 145, 665–672. doi: 10.4103/ijmr.IJMR_1105_15
Greenberg, D., Goldberg, J., Stock, F., Murray, P., Holland, S., Lipuma, J. (2009). Recurrent burkholderia infection in patients with chronic granulomatous disease: 11-year experience at a large referral center. Clin. Infect. dis.: an Off. Publ. Infect. Dis. Soc. America 48, 1577–1579.
He, Y., Chen, W., Fan, J., Fan, S., Ding, H., Chen, J., et al. (2021). Recombinase-aided amplification coupled with lateral flow dipstick for efficient and accurate detection of porcine parvovirus. Life (Basel) 11 (8), 762. doi: 10.3390/life11080762
Kalish, L., Waltz, D., Dovey, M., Potter-Bynoe, G., Mcadam, A., Lipuma, J., et al. (2006). Impact of burkholderia dolosa on lung function and survival in cystic fibrosis. Am. J. Respir. Crit. Care Med. 173, 421–425. doi: 10.1164/rccm.200503-344OC
Lauman, P., Dennis, J. (2021). Burkholderia cepaciaAdvances in phage therapy: Targeting the complex. Viruses. 13 (7), 1331. doi: 10.3390/v13071331
Lord, R., Jones, A., Horsley, A. (2020). Antibiotic treatment for burkholderia cepacia complex in people with cystic fibrosis experiencing a pulmonary exacerbation. Cochrane Database Syst. Rev. 4, CD009529. doi: 10.1002/14651858.CD009529.pub4
Martina, P., Martinez, M., Rivas, S., Leguizamón, L., Von Specht, M., Ferreras, J. (2020). Burkholderia cepacia complex: 11 years of surveillance in patients with cystic fibrosis in posadas, Argentina. Rev. Argent. Microbiol. 52, 176–182. doi: 10.1016/j.ram.2019.08.002
Mu, D., Zhou, D., Xie, G., Liu, J., Xiong, Q., Feng, X., et al. (2021). The fluorescent probe-based recombinase-aided amplification for rapid detection of escherichia coli O157:H7. Mol. Cell. Probes 60, 101777. doi: 10.1016/j.mcp.2021.101777
Pliakos, E., Andreatos, N., Shehadeh, F., Ziakas, P., Mylonakis, E. (2018). The cost-effectiveness of rapid diagnostic testing for the diagnosis of bloodstream infections with or without antimicrobial stewardship. Clin. Microbiol. Rev. 31 (3), e00095–17. doi: 10.1128/CMR.00095-17
Qin, Z., Xue, L., Cai, W., Gao, J., Jiang, Y., Yang, J., et al. (2021). Development of a recombinase-aided amplification assay for rapid detection of human norovirus GII.4. BMC Infect. Dis. 21, 248. doi: 10.1186/s12879-021-05942-x
Rhodes, K., Schweizer, H. (2016). Antibiotic resistance in burkholderia species. Drug resist. updates: Rev. commentaries antimicrob. Anticancer chemother. 28, 82–90.
Sfeir, M. M. (2018). Burkholderia cepacia complex infections: More complex than the bacterium name suggest. J. Infect. 77, 166–170. doi: 10.1016/j.jinf.2018.07.006
Shen, X., Qiu, F., Shen, L., Yan, T., Zhao, M., Qi, J., et al. (2019). A rapid and sensitive recombinase aided amplification assay to detect hepatitis b virus without DNA extraction. BMC Infect. Dis. 19, 229. doi: 10.1186/s12879-019-3814-9
Stephenson, A., Sykes, J., Berthiaume, Y., Singer, L., Aaron, S., Whitmore, G., et al. (2015). Clinical and demographic factors associated with post-lung transplantation survival in individuals with cystic fibrosis. J. Heart Lung Transplant. 34, 1139–1145. doi: 10.1016/j.healun.2015.05.003
Tavares, M., Kozak, M., Balola, A., Sa-Correia, I. (2020). Burkholderia cepacia complex bacteria: a feared contamination risk in water-based pharmaceutical products. Clin. Microbiol. Rev. 33 (3), e00139–19. doi: 10.1128/CMR.00139-19
Wang, W., Wang, C., Zhang, Z., Zhang, P., Zhai, X., Li, X., et al. (2021). Recombinase-aided amplification-lateral flow dipstick assay-a specific and sensitive method for visual detection of avian infectious laryngotracheitis virus. Poultry Sci. 100, 100895. doi: 10.1016/j.psj.2020.12.008
Wang, R., Zhang, H., Zhang, Y., Li, X., Shen, X., Qi, J., et al. (2019). Development and evaluation of recombinase-aided amplification assays incorporating competitive internal controls for detection of human adenovirus serotypes 3 and 7. Virol. J. 16, 86. doi: 10.1186/s12985-019-1178-9
Whiteford, M., Wilkinson, J., Mccoll, J., Conlon, F., Michie, J., Evans, T., et al. (1995). Outcome of burkholderia (Pseudomonas) cepacia colonisation in children with cystic fibrosis following a hospital outbreak. Thorax 50, 1194–1198. doi: 10.1136/thx.50.11.1194
Xiao, X., Lin, Z., Huang, X., Lu, J., Zhou, Y., Zheng, L., et al. (2021). Vibrio vulnificusRapid and sensitive detection of using CRISPR/Cas12a combined with a recombinase-aided amplification assay. Front. Microbiol. 12, 767315. doi: 10.3389/fmicb.2021.767315
Xue, G., Li, S., Zhao, H., Yan, C., Feng, Y., Cui, J., et al. (2020). Use of a rapid recombinase-aided amplification assay for mycoplasma pneumoniae detection. BMC Infect. Dis. 20, 79. doi: 10.1186/s12879-019-4750-4
Zhang, W., Feng, Y., Zhao, H., Yan, C., Feng, J., Gan, L., et al. (2021). Klebsiella pneumoniaeA recombinase aided amplification assay for rapid detection of the carbapenemase gene and its characteristics in. Front. Cell. infect. Microbiol. 11, 746325. doi: 10.3389/fcimb.2021.746325
Zhang, X., Guo, L., Ma, R., Cong, L., Wu, Z., Wei, Y., et al. (2017). Rapid detection of salmonella with recombinase aided amplification. J. Microbiol. Methods 139, 202–204. doi: 10.1016/j.mimet.2017.06.011
Keywords: Burkholderia cepacia complex, recombinase-aided amplification, rapid detection, 16S rRNA, infection
Citation: Fu H, Gan L, Tian Z, Han J, Du B, Xue G, Feng Y, Zhao H, Cui J, Yan C, Feng J, Fan Z, Fu T, Xu Z, Zhang R, Cui X, Du S, Zhou Y, Zhang Q, Cao L and Yuan J (2022) Rapid detection of Burkholderia cepacia complex carrying the 16S rRNA gene in clinical specimens by recombinase-aided amplification. Front. Cell. Infect. Microbiol. 12:984140. doi: 10.3389/fcimb.2022.984140
Received: 01 July 2022; Accepted: 18 August 2022;
Published: 05 September 2022.
Edited by:
Zhanhui Wang, China Agricultural University, ChinaReviewed by:
Lei Ma, Anyang Institute of Technology, ChinaTodd Steck, University of North Carolina at Charlotte, United States
Copyright © 2022 Fu, Gan, Tian, Han, Du, Xue, Feng, Zhao, Cui, Yan, Feng, Fan, Fu, Xu, Zhang, Cui, Du, Zhou, Zhang, Cao and Yuan. This is an open-access article distributed under the terms of the Creative Commons Attribution License (CC BY). The use, distribution or reproduction in other forums is permitted, provided the original author(s) and the copyright owner(s) are credited and that the original publication in this journal is cited, in accordance with accepted academic practice. No use, distribution or reproduction is permitted which does not comply with these terms.
*Correspondence: Jing Yuan, eXVhbmppbmc2MjE2QDE2My5jb20=; Ling Cao, Y2FvbGluZzk5MTlAMTYzLmNvbQ==
†These authors have contributed equally to this work