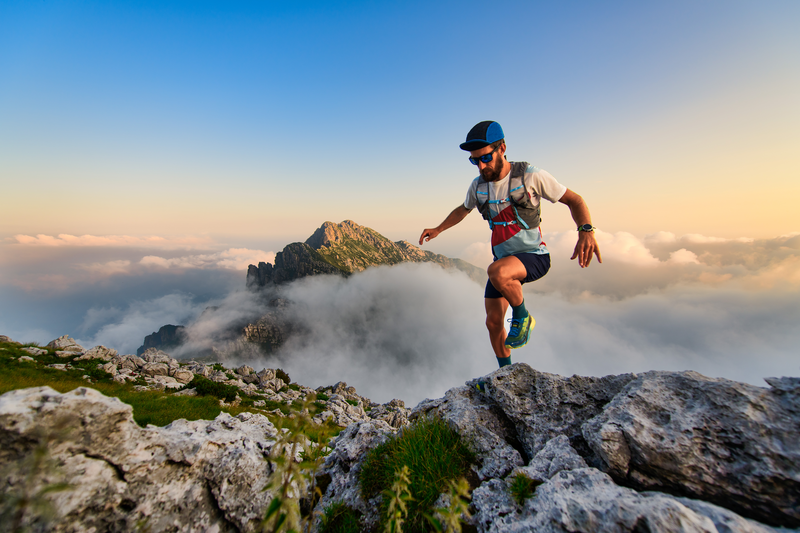
95% of researchers rate our articles as excellent or good
Learn more about the work of our research integrity team to safeguard the quality of each article we publish.
Find out more
ORIGINAL RESEARCH article
Front. Cell. Infect. Microbiol. , 02 September 2022
Sec. Molecular Bacterial Pathogenesis
Volume 12 - 2022 | https://doi.org/10.3389/fcimb.2022.981792
This article is part of the Research Topic Mobile Genetic Elements as Dissemination Drivers of Multidrug-Resistant Gram-Negative Bacteria View all 5 articles
Ceftazidime/Avibactam (CAZ/AVI) is frequently used to treat KPC-producing Pseudomonas aeruginosa (KPC-PA) and Enterobacterales. CAZ/AVI resistance is driven by several mechanisms. In P. aeruginosa this mainly occurs through alteration of AmpC, porins, and/or efflux pump overexpression, whereas in Enterobacterales it frequently occurs through D179Y substitution in the active site of KPC enzyme. This aminoacid change abolishes AVI binding to the KPC active site, hence inhibition is impaired. However, this substitution also decreases KPC-mediated resistance to carbapenems (“see-saw” effect). The goal of this work was to characterize the in vivo acquisition of CAZ/AVI resistance through D179Y substitution in a KPC-PA isolated from a hospitalized patient after CAZ/AVI treatment. Two KPC-PA isolates were obtained. The first isolate, PA-1, was obtained before CAZ/AVI treatment and was susceptible to CAZ/AVI. The second isolate, PA-2, was obtained after CAZ/AVI treatment and exhibited high-level CAZ/AVI resistance. Characterization of isolates PA-1 and PA-2 was performed through short and long-read whole genome sequencing analysis. The hybrid assembly showed that PA-1 and PA-2A had a single plasmid of 54,030 bp, named pPA-1 and pPA-2 respectively. Each plasmid harbored two copies of the blaKPC-containing Tn4401b transposon. However, while pPA-1 carried two copies of blaKPC-2, pPA-2 had one copy of blaKPC-2 and one copy of blaKPC-33, the allele with the D179Y substitution. Interestingly, isolate PA-2 did not exhibit the “see-saw” effect. The blaKPC-33 allele was detected only through hybrid assembly using a long-read-first approach. The present work describes a KPC-PA isolate harboring a plasmid-borne CAZ/AVI resistance mechanism based on two copies of blaKPC-2-Tn4401b and D179Y mutation in one of them, that is not associated with loss of resistance to carbapenems. These findings highlight the usefulness of a fine-tuned combined analysis of short and long-read data to detect similar emerging resistance mechanisms.
Pseudomonas aeruginosa is one of the most frequent causative agents of healthcare-associated infections and poses a major concern due to its outstanding ability to develop resistance to antimicrobial agents (Botelho et al., 2019). Carbapenemases are the most important acquired resistance in P. aeruginosa because it confers resistance to all beta-lactams and because some of them are encoded in mobile genetic elements that facilitate their dissemination. The most frequent carbapenemase in P. aeruginosa worldwide is VIM (Karlowsky et al., 2018; Karlowsky et al., 2019; Kiwei et al., 2021). In a recent report including more than 400 beta-lactam resistant P. aeruginosa isolates in Europe, 37% were VIM positive, followed by NDM and IMP (Torrens et al., 2022). In contrast to the worldwide distributed class B beta-lactamase-producing P. aeruginosa, KPC-producing P. aeruginosa (KPC-PA) has been mostly reported in American countries like Argentina (Pasterán et al., 2012; Ramírez et al., 2013), Brazil (Luna et al., 2012), Trinidad y Tobago (Akpaka et al., 2009), United States (Poirel et al., 2010), Chile (Enberg et al., 2020; Costa et al., 2021) Colombia (Villegas et al., 2007; Rada et al., 2021) and also in China (Kiwei et al., 2021). A recent Chilean study showed that among 61 carbapenemase-producing P. aeruginosa isolates, 54% harbored KPC, and the remaining 46% produced VIM (Costa et al., 2021).
Ceftazidime-avibactam (CAZ/AVI) is a novel antimicrobial combination that gathers ceftazidime, a well-known cephalosporin, with avibactam, a beta-lactamase inhibitor highly active against class A carbapenemases such as KPC. Notably, while avibactam also inhibits class C and some D enzymes, it does not exhibit activity against class B carbapenemases (Ehmann et al., 2012), Currently, CAZ/AVI is one of the main antibiotic alternatives used to treat infections by KPC-producing bacteria, including KPC-PA (Temkin et al., 2017) and KPC-producing Enterobacterales (Shields et al., 2017a). Unfortunately, CAZ/AVI resistance in P. aeruginosa is not uncommon, with reported resistance rates of 10-16% among isolates of carbapenem-resistant P. aeruginosa not harboring class B beta-lactamases (Kazmierczak et al., 2018; Karlowsky et al., 2018; Karlowsky et al., 2019). Globally, CAZ/AVI resistance occurs through several mechanisms: changes in the omega-loop of the KPC or AmpC enzymes; porin mutations; increased copy number and/or expression of blaKPC genes; and increased expression of efflux pumps (Botelho et al., 2019; Coppi et al., 2020). D179Y substitution in the omega-loop of KPC-2 (designated KPC-33 variant) has been associated with CAZ/AVI resistance in Enterobacterales (Wang et al., 2020). While the specific mechanism of CAZ/AVI resistance conferred by the D179Y mutation has not been completely characterized, some studies suggest it may be related to hydrogen bonds stabilizing the interaction of the enzymatic active site with CAZ, decreasing its affinity to AVI (Winkler et al., 2015; Barnes et al., 2017). Other studies have reported that D179Y alters the acylation of KPC, decreasing the inactivation constant of AVI by ~70,000-fold (Compain and Arthur, 2017). Indeed, experiments with purified KPC-33 demonstrated a 20-fold increase in the concentration of AVI needed to inhibit 50% of CAZ hydrolysis. Also, KPC-33 exhibited a 10-fold higher efficiency hydrolyzing CAZ as compared to wild-type (WT) KPC-2 (Tsivkovski and Lomovskaya, 2020). Interestingly, the D179Y substitution restores the susceptibility to carbapenems in Enterobacterales, known as the “see-saw” effect (Shields et al., 2017a; Haidar et al., 2017; Shields et al., 2017b). In contrast to Enterobacterales, CAZ/AVI resistance in P. aeruginosa has been mostly associated with point mutations in chromosomal genes coding for AmpC and OXA-539 beta-lactamases (Fraile-Ribot et al., 2017; Wang et al., 2020). In addition, several changes in chromosomal genes have been reported in CAZ/AVI resistant clinical isolates of P. aeruginosa: ftsI, nalD, dnaK, ctpA, together with increased expression of MexAB-OprM efflux pump system (Castanheira et al., 2019). Herein, we report the in vivo emergence of high-level CAZ/AVI resistance in a clinical isolate of KPC-PA bearing two copies of blaKPC, one of which developed the D179Y substitution. Interestingly, the development of CAZ-AVI resistance did not result in concomitant carbapenem susceptibility. The finding of KPC-33 was detected through a multi-step hybrid assembly pipeline using long and short-read whole-genome sequencing (WGS) platforms.
This work was approved by the Ethical Committee of Pontificia Universidad Católica de Chile. Isolates were obtained from a 77-year-old male patient admitted in February 2019 due to a dissecting abdominal aortic aneurysm. Two days after surgical repair, a urine culture performed due to tachycardia and abdominal pain, yielded a KPC-PA (isolate PA-1). The isolate was susceptible to amikacin, colistin and CAZ/AVI. Therapy with colistin plus CAZ/AVI was started immediately. After 16 days of therapy, fever and clinical worsening occurred and the patient developed respiratory failure and shock, requiring vasoactive support and mechanical ventilation. A culture from an endotracheal aspirate obtained at that moment yielded a CAZ/AVI resistant KPC-PA isolate (isolate PA-2). Therefore CAZ/AVI was replaced by a combination of colistin plus amikacin. The patient developed renal impairment with hypernatremia and hypokalemia that were managed with hypotonic fluids and intravenous loads, respectively. Finally, the patient exhibited a good clinical response after 48 days of colistin therapy, with disappearance of the fever and decreasing inflammatory parameters, and was discharged after 127 days of hospitalization.
Antimicrobial susceptibility was determined through the agar dilution method as per Clinical Laboratory Standards Institute (CLSI) recommendations (CLSI, 2019). Susceptibility to CAZ/AVI, ceftolozane/tazobactam and aztreonam were determined using the broth microdilution method using SensiTitre Antimicrobial Susceptibility Testing System according to manufacturer`s instructions (Thermo Fisher Scientific, United States). Susceptibility categorization was performed using the breakpoints proposed by the CLSI guidelines (CLSI, 2019).
Isolates were initially assessed for carbapenemase activity through Carba-NP testing as per CLSI recommendations (CLSI, 2019). The presence of KPC, OXA-48, IMP, VIM and NDM was determined using the immunochromatographic test NG-CARBA 5 (NG-BIOTECH®, France). Further, molecular confirmation was performed using PCR targeting carbapenemase genes blaKPC, blaOXA-48, blaNDM, blaIMP, blaIMI, blaGES and blaVIM, as previously described (Wozniak et al., 2012). DNA from carbapenemase-producing clinical isolates previously characterized in our laboratory was used as positive controls (Wozniak et al., 2012).
SDS-PAGE analyses of the insoluble outer-membrane fraction were performed as described (Wozniak et al., 2012). Briefly, 2mL of overnight culture were centrifuged, resuspended in 10mM Tris HCl pH8, sonicated for 2min and centrifuged at 7,000g for 5min. The supernatant obtained was centrifuged at 13,000g for 45min, and the pellet was resuspended in 10mM Tris-HCl pH8 with 2% Triton X-100 and incubated at 37°C for 30min. The suspension was centrifuged at 13,000g for 45min and the pellet containing outer membrane proteins was resuspended in 100mM Tris-HCl pH8 with 2% SDS. A volume containing 50µg of protein was incubated at 98°C for 5min and analyzed in a 12.5% polyacrylamide gel at 100V. Gels were stained overnight in 0.1% Coomassie blue and washed with 1% acetic acid. Susceptible P. aeruginosa ATCC 27853 was included as control.
Isolates PA-1 and PA-2 were sequenced using both short-read and long-read WGS. For short-read sequencing, a 350 bp insert DNA library was prepared using Illumina DNA Prep Kit (formerly Nextera Flex), using the Hackflex protocol (Gaio et al., 2022). Sequencing was performed in an Illumina Platform PE150, at the University of Technology Sydney’s Bioscience Laboratory (Sydney, Australia). The Q30 obtained was > 90% for both isolates. De novo assembly was performed using SPADES version 3.7 package (Bankevich et al., 2012). Genomic annotation of the recovered draft genomes was performed with Prokka tool 1.11 (Seemann, 2014). Final visualization was made using Geneious™. For long-read sequencing, an Oxford Nanopore platform was used. A Ligation Library was prepared and sequenced on a GridION device at the Garvan Institute of Medical Research (Sydney, Australia).
Short-read and long-read sequences were assembled using two different hybrid assembly approaches: Unicylcler and Trycycler (Wick et al., 2017; Wick et al., 2021). Of note, Unicycler uses a short-read-first approach and Trycycler uses a long-read-first approach. For Trycycler assembly, a set of 12 assemblies made with three different assemblers, using different random subsets as input, were generated. The three assemblers used were Flye (Kolmogorov et al., 2019), Miniasm (Heng Li, 2016) and Raven (Vaser and Šikić, 2021). The clustering of assemblies was evaluated manually in a phylogenetic tree, and after confirming that the three assemblies were concordant in the plasmids and chromosomes generated, a consensus sequence was generated. The resistome characterization, genome annotation, and visualization of the hybrid assemblies were performed using the Comprehensive Antibiotic Resistance Database (CARD) Resistance Gene Identifier (RGI) v1.0.0 (McArthur et al., 2013), Prokka v1.11 (Seemann, 2014) and CGView Builder v1.0.0 (Grant and Stothard, 2008).
Raw sequence data were deposited in BioProject No. PRJNA839103 in the Sequence Read Archive of the National Center for Biotechnology Information with BioSample accession numbers SAMN28535839, SAMN28535840 for PA-1 and PA-2 respectively. Final assembly of chromosomes were deposited in Genbank with accession numbers CP097709 and CP097710, for PA-1 and PA-2 respectively. Final assembly of plasmids were deposited in Genbank with accession numbers CP097845 and CP097844 for pPA-1 and pPA-2 respectively.
To confirm the KPC D179Y substitution, a forward primer that included the nucleotide change G532T at the 3’ end was designed. The same primer with the WT nucleotide was also designed. The primers used were: WT-F: GCGCGCGGCGATGAGGTATC; Mut-F: GCGCGCGGCGATGAGGTATA; Rev: CTTGCCGCTCGGTGATAATC. Two PCR reactions were done for each isolate: one with primers WT-F and WT-Rev, and the other one with primers Mut-F and Mut-Rev; both PCR reactions produced a 640 bp amplicon. It was expected that PCR with primers Mut-F and Mut-Rev produced a 640 bp amplicon for PA-2, and no amplicon for PA-1. PCR with primers WT-F and WT-Rev was expected to produce a 640 bp amplicon for both isolates.
A summary of the susceptibility profile of PA-1 and PA-2 is presented in Table 1. Briefly, PA-1 exhibited resistance to all tested antimicrobials tested except for colistin, amikacin, and CAZ/AVI with minimum inhibitory concentrations (MIC) of ≤2, ≤8 and 4 μg/mL, respectively. PA-2 exhibited the same susceptibility profile of PA-1, except for CAZ/AVI, which resulted in a MIC of >256 μg/mL (Table 1). Both isolates exhibited carbapenemase activity as indicated by a positive Carba-NP test. Immunochromatographic detection of carbapenemases showed that both isolates produced a KPC enzyme, which was later confirmed through PCR targeting the blaKPC gene (data not shown). Since altered production of the OprD porin may also affect CAZ/AVI activity, the porin profile was analyzed through SDS-PAGE of the insoluble outer membrane fraction. As shown in Figure 1, the band corresponding to OprD was absent in both clinical isolates as compared to the susceptible control. Therefore, an OprD-mediated resistance to CAZ/AVI in PA-2 was unlikely.
Figure 1 SDS-PAGE analysis of outer membrane protein extraction of isolates PA-1 and PA-2. Weight (kDa) of the Molecular Weight Standard (Std MW) bands used in protein electrophoresis is indicated next to the bands. P. aeruginosa ATCC 27853 was used as a control isolate. OprD was located based in its molecular weight of 48,4 kDa and according to previous reports (Rodríguez-Beltrán et al., 2015).
Our genomic in-silico MLST analysis revealed both isolates belonged to ST654 and they harbored a blaKPC-2 gene embedded in a typical Tn4401b transposon. Mutations in several chromosomally-encoded genes frequently associated with CAZ/AVI resistance were analyzed; no differences were found between both isolates. However, several mutations in genes associated with CAZ/AVI resistance were present in both isolates respect to WT strain PAO-1 (Table 2). An 8 bp deletion at nucleotide 235 was observed in mexT, which resulted in a frameshift from amino acid 80. In addition, mexZ had a 2 bp deletion at positions 550 and 551, which produced a frameshift from amino acid 184. Both changes in MexT and MexZ were predicted to result in a loss of protein function according to PROVEAN analysis (Choi et al., 2012). Other changes observed in chromosomal genes were predicted to produce minor or no changes in protein function according to PROVEAN analysis (Table 2). Both PA-1 and PA-2 had the same allele of the endogenous ampC gene (PDC-3), WT alleles of mexAB-oprM operon, the same polymorphisms in transcriptional regulators MexT, MexD, MexZ, NalC and NalD, and the same variant of OprD porin. Despite OprD porin had a deletion of two amino acids and 29 amino acid substitutions respect to PAO-1 strain, these alterations were neutral according to PROVEAN analysis. This OprD protein variant was reported as functional (Suresh et al., 2020). According to these data, no evident differences were found between both isolates that could explain CAZ/AVI resistance in PA-2.
Table 2 Sequence analysis of PA-1 and PA-2 acquired resistance genes and chromosomal genes associated with CAZ/AVI resistance compared to PAO-1 control strain.
To obtain a more detailed genomic analysis, hybrid assemblies were performed using short and long-read data for each isolate. Using Unicycler (short-read-first approach), isolate PA-1 generated two circular scaffolds of 7,046,950 bp and 54,030 bp long, and isolate PA-2 generated two linear scaffolds of 6,969,342 bp and 71,243 bp, and a circular scaffold of 40,443 bp. Scaffolds of 54,030 bp (PA-1) and 40,443 bp (PA-2) corresponded to plasmids based on the presence of plasmid replication control genes and were named pPA-1 and pPA-2, respectively. Plasmid pPA-1 harbored two copies of the blaKPC gene, each one contained in a Tn4401b transposon separated by 8,687 bp, whereas plasmid pPA-2 had only one copy of the Tn4401b transposon containing blaKPC-2 according to Unicycler assembly. In contrast, using Trycycler (long-read-first approach) both isolates generated the same two circular scaffolds of 7,048,060 bp and 54,030 bp long. The Trycycler assembly showed both plasmids, pPA-1 and pPA-2 carried two copies of blaKPC, both of which were harbored in a Tn4401b transposon structure. Interestingly, while both copies in PA-1 were WT blaKPC-2, in isolate PA-2 one of the copies was a WT blaKPC-2 gene and the other copy had a G532T base change that produced D179Y substitution in the omega-loop of KPC-2 and was therefore a blaKPC-33 allele. To further corroborate this point mutation, the short reads of PA-1 and PA-2 were mapped against both blaKPC alleles. No reads of PA-1 aligned with the blaKPC-33 sequence. In contrast, analysis of PA-2 showed a balanced mapping of reads aligning with blaKPC-2 and blaKPC-33, respectively (Figure 2). A pair-wise alignment between the chromosomes of PA-1 and PA-2 demonstrated they were identical except for four 1-bp insertions/deletions and five 1-bp substitutions (99.99% identity) (Figure 3). Pair-wise alignment of plasmids pPA-1 and pPA-2 showed they were identical, except for nucleotide change in position G532T of the blaKPC gene, which resulted in the D179Y amino acid substitution (Figure 3).
Figure 2 Short reads of PA-1 (A, B) and PA-2 (C, D) mapped against blaKPC-2 (A, C) and blaKPC-33 sequences (B, D). Position 532 is indicated in a vertical rectangle, and it has “G” in blaKPC-2 and “A” in blaKPC-33..
Figure 3 Chromosomes of isolates PA-1 (A) and PA-2 (B) and plasmids pPA-1 and pPA-2 C. Red arrowheads in A and B represent genes that are associated with antimicrobial resistance. The resistome of plasmids pPA-1 and pPA-2 C is composed only by two blaKPC-2 genes in pPA-1 and both blaKPC-2 and blaKPC-33 genes in pPA-2. Grey bars represent coding sequences (CDS) whose gene names are shown for annotated genes or not shown for hypothetical proteins (hp). Transposase genes: associated with transposition events; hin_1, hin_2, dinG, soj: genes associated with DNA processing and repair; xerC: gene associated with plasmid replication control.
The single nucleotide substitution of blaKPC-33 with respect blaKPC-2 was further confirmed by PCR using primers specific to each of the alleles. To do so, we designed two identical forward primers with a single nucleotide difference between them in the 3`end, which corresponded to the G532T change associated to the blaKPC-33 allele. This nucleotide variation prevented the complete 3`end annealing of the primer against WT blaKPC-2. PCR with primers directed to blaKPC-2 amplified a 640 bp fragment as expected in both isolates PA-1 and PA-2, while primers directed to blaKPC-33 only amplified the 640 bp fragment in PA-2 (Figure 4). Therefore, the presence of blaKPC-33 was confirmed only in isolate PA-2.
Figure 4 Agarose gel electrophoresis of PCR products obtained in the amplification of PA-1 and PA-2 DNA using primers for amplification of blaKPC-2 (left) and blaKPC-33 (right). Both primer pairs amplify a fragment of 640 bp. Band sizes (bp) of the Molecular Weight Standard (Ld 100 bp) are indicated next to the bands.
In the present work, we characterized a KPC-PA clinical isolate that developed CAZ/AVI resistance in vivo through D179Y mutation in one of two blaKPC-2 genes that were harbored in a plasmid. Isolate PA-2 had >64-fold increase in CAZ/AVI MIC with respect to PA-1, but did not exhibit a decrease in carbapenem resistance (“see-saw” effect) as observed in Enterobacterales (Shields et al., 2017a; Shields et al., 2017b; Tsivkovski and Lomovskaya, 2020). The presence of both KPC-2 and KPC-33 is most likely responsible for the absence of the “see-saw” effect. This “all in one” resistant phenotype includes resistance to CAZ/AVI and to carbapenems, since the cost associated with the acquisition of blaKPC-33 alone is offset by blaKPC-2. In a previous work from our group, we described a D179Y mutation in the blaKPC-2 gene of a clinical isolate of P. aeruginosa, but CAZ/AVI resistance was not addressed that time (Wozniak et al., 2019). This KPC-PA isolate had a negative immunochromatographic test, therefore, it is unlikely that it had an additional WT blaKPC-2 copy. However, this isolate was resistant to meropenem and imipenem, therefore no “see-saw” effect was observed. According to these findings, the “see-saw” effect may be observable in Enterobacterales, but not necessarily in P. aeruginosa. This could be explained because Enterobacterales are much more dependent on carbapenemase function than P. aeruginosa, which has a variety of additional mechanisms for carbapenem resistance, e.g., efflux pump overexpression, porin alteration, AmpC and OXA enzymes alterations, among others (Botelho et al., 2019). In fact, several mutations in chromosomal genes associated with CAZ/AVI resistance were observed in both PA-1 and PA-2. Notably, isolate PA-1 was susceptible to CAZ/AVI, despite having these alterations. Most of the mutations observed have been previously reported in CAZ/AVI resistant isolates. NalC is a repressor of the positive regulator of MexAB-OprM and G71E mutation was described in aztreonam resistant isolates (Braz et al., 2016). NalD is a repressor of MexAB-OprM operon, and the mutation observed in this work has been observed in CAZ/AVI resistant P. aeruginosa (Castanheira et al., 2019). MexT is a transcriptional regulator that activates the expression of MexEF-OprN multidrug efflux system. The mutation observed in MexT has been reported in multi-drug resistant P. aeruginosa with decreased expression of OprD porin (Epp et al., 2001). MexZ is a repressor of the MexXY operon and alterations in mexZ have been described in CAZ/AVI resistant P. aeruginosa isolates (Castanheira et al., 2019). Mutation T105A in AmpC (PDC-3 allele) confers reduced susceptibility to imipenem, ceftazidime, and cefepime (Rodríguez-Martínez et al., 2009). Our results suggest that these mutations may not confer CAZ/AVI resistance alone, but they may contribute to overall resistance.
Hybrid assembly using short and long-read data is an excellent approach to predict putative plasmids, resistance genes, and beta-lactamase gene variants (Khezri et al., 2021). The G532T mutation in blaKPC gene responsible for D179Y substitution, was observable only in the hybrid assembly obtained with Trycycler; it was not observable in the short-read assembly, nor in the hybrid assembly made with Unicycler. The short reads (350 bp long) are unable to cover both KPC-Tn4401 copies (28,699 bp) present in plasmids pPA-1 and pPA-2, and Unicycler considered both blaKPC-2 and blaKPC-33 alleles as a single gene. In contrast, long reads (with an average length of 40,000 bp) can cover both KPC-Tn4401 copies. However, Trycycler requires manual intervention from the user, it is more time-consuming and therefore is more challenging for high-throughput assembly. In a previous report a short-read WGS analysis of a CAZ/AVI resistant clinical isolate of KPC-producing K. pneumoniae determined that 46% of blaKPC reads were blaKPC-33 and 54% were blaKPC-2 (Gaibani et al., 2020). A similar short-read WGS analysis of a clinical isolate of K. pneumoniae that developed high-level CAZ/AVI resistance after antibiotic treatment showed that 28% of the reads covering blaKPC gene were blaKPC-2 and 72% were blaKPC-33 (Sun et al., 2021). Both articles mentioned above concluded that these isolates consisted of a mixed population containing both CAZ/AVI-resistant and CAZ-AVI-susceptible bacteria, which is commonly interpreted as heteroresistance. Our results show that interpretation of short-read data must be done with caution because this strategy not always allows discrimination between mixed populations and gene duplication with subsequent mutation. These findings underscore the actual value of long-read WGS methods and hybrid assembly combining short and long-read data.
The plasmids described in this work, pPA-1 and pPA-2, were similar to pPA2047 a 43,660 bp plasmid from P. aeruginosa recently reported in Argentina (>65% identity) (Cejas et al., 2022), and to pPae-13, a plasmid that was recently reported in a KPC-PA clinical isolate in the same Institutional Hospital as the present work (>60% identity) (Wozniak et al., 2021). It is possible that replicative transposition of blaKPC-containing Tn4401b transposon in pPae-13 plasmid had produced pPA-1, and pPA-2 subsequently evolved through point mutation.
Development of high-level CAZ/AVI resistance upon CAZ/VI treatment greatly compromises the usefulness of this antibiotic combination for the treatment of KPC-PA infections, being colistin the most plausible option, with the subsequent associated renal impairment, like the one described in the present case. The lack of better therapeutic options together with the genetic characteristics of KPC-PA, i.e., location of blaKPC genes in Tn4401b transposon, carriage by the high-risk clone ST654, make this finding highly concerning, particularly in regions where KPC-PA is frequent.
The datasets presented in this study can be found in online repositories. The names of the repository/repositories and accession number(s) can be found below: https://www.ncbi.nlm.nih.gov/genbank/, CP097709 https://www.ncbi.nlm.nih.gov/genbank/, CP097710 https://www.ncbi.nlm.nih.gov/, SAMN28535839 https://www.ncbi.nlm.nih.gov/, SAMN28535840.
The information about human patients were reviewed and approved by the Institutional Review Board of the Ethics and Biosafety Committee of Pontificia Universidad Católica de Chile.
AW, PG and BB conceived the idea and designed the experiments. JO-P, MA-R and JMu supervised the work. VQ, AW and BB performed the experiments in the study. BB, JMa, MA-R and AW performed bioinformatic analysis. PG, AW, BB, and MA-R wrote the paper. All authors read and approved the final manuscript.
This work was supported by research funds from SENTRY (Antimicrobial Resistance Surveillance Program), the Red de Salud UC-Christus and the Department of Clinical Laboratories at the School of Medicine of Pontificia Universidad Católica de Chile. Funding for whole-genome sequencing was obtained through the Key Technology Partnership Program from the University of Technology Sydney. This work was partially funded by the Agencia Nacional de Investigation y Desarrollo (FONDECYT regular award #1211947; JMu), and the Millennium Science Initiative, Millennium Initiative for Collaborative Research on Bacterial Resistance, Government of Chile (award number NCN17_081; JMM)
We thank the staff of the Microbiology Laboratory of the Red de Salud UC-CHRISTUS for their help in the technical aspects of this work. We also thank the Millennium Science Initiative of the Ministry of Economy, Development and Tourism, Government of Chile. We would like to express our gratefulness to the “Pseudomonas group”, a collaborative team including researchers from various Chilean Universities: Pontificia Universidad Católica de Chile, Pontificia Universidad Católica de Valparaíso, Universidad de Concepción; Universidad del Desarrollo.
The authors declare that the research was conducted in the absence of any commercial or financial relationships that could be construed as a potential conflict of interest.
The handling editor AO-C declared a past collaboration with the authors AW, PG, MA-R, JM, JO-P.
All claims expressed in this article are solely those of the authors and do not necessarily represent those of their affiliated organizations, or those of the publisher, the editors and the reviewers. Any product that may be evaluated in this article, or claim that may be made by its manufacturer, is not guaranteed or endorsed by the publisher.
Akpaka, P., Swanston, W., Ihemere, H., Correa, A., Torres, J., Tafur, J., et al. (2009). Emergence of KPC-producing pseudomonas aeruginosa in Trinidad and Tobago. J. Clin. Microbiol. 47 (8), 2670–2671. doi: 10.1128/JCM.00362-09
Bankevich, A., Nurk, S., Antipov, D., Gurevich, A. A., Dvorkin, M., Kulikov, A. S., et al. (2012). SPAdes: A new genome assembly algorithm and its applications to single-cell sequencing. J. Comput. Biol. 19, 455–477. doi: 10.1089/cmb.2012.0021
Barnes, M. D., Winkler, M. L., Taracila, M. A., Page, M. G., Desarbre, E., Kreiswirth, B. N., et al. (2017). Klebsiella pneumoniae carbapenemase-2 (KPC-2), substitutions at ambler position Asp179, and resistance to ceftazidime-avibactam: unique antibiotic-resistant phenotypes emerge from beta-lactamase protein engineering. mBio 8, e00528-17. doi: 10.1128/mBio.00528-17
Botelho, J., Grosso, F., Peixe, L. (2019). Antibiotic resistance in pseudomonas aeruginosa - mechanisms, epidemiology and evolution. Drug Resist. Update 44, 100640. doi: 10.1016/j.drup.2019.07.002
Braz, V. S., Furlan, J. P., Fernandes, A. F., Stehling, E. G. (2016). Mutations in NalC induce MexAB-OprM overexpression resulting in high level of aztreonam resistance in environmental isolates of pseudomonas aeruginosa. FEMS Microbiol. Lett. 363 (16), fnw166. doi: 10.3390/microorganisms9122560
Castanheira, M., Doyle, T. B., Smith, C. J., Mendes, R. E., Sader, H. S. (2019). Combination of MexAB-OprM overexpression and mutations in efflux regulators, PBPs and chaperone proteins is responsible for ceftazidime/avibactam resistance in pseudomonas aeruginosa clinical isolates from US hospitals. J. Antimicrob. Chemother. 74 (9), 2588–2595. doi: 10.1093/jac/dkz243
Cejas, D., Elena, A., González-Espinosa, F., Pallecchi, L., Vay, C., Rossolini, G. M., et al. (2022). Characterization of blaKPC-2 harboring plasmids recovered from pseudomonas aeruginosa ST654 and ST235 high-risk clones. J. Glob. Antimicrob. Res. 29, 310–312. doi: 10.1016/j.jgar.2022.04.017
Choi, Y., Sims, G. E., Murphy, S., Miller, J. R., Chan, A. P. (2012). Predicting the functional effect of amino acid substitutions and indels. PLoS One 7 (10), e46688. doi: 10.1371/journal.pone.0046688
Clinical and Laboratory Standards Institute (2019). Performance standards for antimicrobial susceptibility testing: Twenty-ninth edition: M100 (Wayne, PA, USA: CLSI).
Compain, F., Arthur, M. (2017). Impaired inhibition by avibactam and resistance to the ceftazidime-avibactam combination due to the D179Y substitution in the KPC-2 β-lactamase. Antimicrob. Agents Chemother. 61 (7), e00451-17. doi: 10.1128/AAC.00451-17
Coppi, M., Di Pilato, V., Monaco, F., Giani, T., Conaldi, P. G., Rossolini, G. M. (2020). Ceftazidime-avibactam resistance associated with increased blaKPC-3 gene copy number mediated by pKpQIL plasmid derivatives in sequence type 258 klebsiella pneumoniae. Antimicrob. Agents Chemother. 64 (4), e01816-19. doi: 10.1128/AAC.01816-19
Costa, J. S. T. D., Lima, C. A., Vera-Leiva, A., San Martin, M. I., Bello-Toledo, H., Domínguez-Yévenes, M., et al. (2021). Carbapenemases produced by carbapenem-resistant pseudomonas aeruginosa isolated from hospitals in Chile. Rev. Chil. Infectol. 38 (1), 81–87. doi: 10.4067/S0716-10182021000100081
Ehmann, D. E., Jahić, H., Ross, P. L., Gu, R. F., Hu, J., Kern, G., et al. (2012). Avibactam is a covalent, reversible, non-β-lactam β-lactamase inhibitor. Proc. Natl. Acad. Sci. U.S.A. 109 (29), 11663–11668. doi: 10.1073/pnas.1205073109
Enberg, M. E., Puente, M., Wozniak, A., Castillo, C., Villagra, N., Labarca, J. (2020). Carbapenemases in pseudomonas aeruginosa with decreased susceptibility to carbapenems after a decade: from VIM to KPC. Rev. Chil. Infectol. 37 (4), 389–394. doi: 10.4067/S0716-10182020000400389
Epp, S. F., Köhler, T., Plésiat, P., Michéa-Hamzehpour, M., Frey, J., Pechère, J. C., et al. (2001). C-terminal region of pseudomonas aeruginosa outer membrane porin OprD modulates susceptibility to meropenem. Antimicrob. Agents Chemother. 45 (6), 1780–1787. doi: 10.1128/AAC.45.6.1780-1787.2001
Fraile-Ribot, P. A., Mulet, X., Cabot, G., del Barrio-Tofinão, E., Juan, C., Peírez, J. L., et al. (2017). In vivo emergence of resistance to novel cephalosporin–β-lactamase inhibitor combinations through the duplication of amino acid D149 from OXA-2 β-lactamase (OXA-539) in sequence type 235 pseudomonas aeruginosa. Antimicrob. Agents Chemother. 61, e01117-17. doi: 10.1128/AAC.01117-17
Gaibani, P., Re, M. C., Campoli, C., Viale, P. L., Ambretti, S. (2020). Bloodstream infection caused by KPC-producing klebsiella pneumoniae resistant to ceftazidime/avibactam: epidemiology and genomic characterization. Clin. Microbiol. Infect. 26 (4), 516.e1–516.e4. doi: 10.1016/j.cmi.2019.11.011
Gaio, D., Anantanawat, K., To, J., Liu, M., Monahan, L., Darling, A. E. (2022). Hackflex: low-cost, high-throughput, illumina nextera flex library construction. Microb. Genom. 8 (1), 744. doi: 10.1099/mgen.0.000744
Grant, J. R., Stothard, P. (2008). The CGView server: a comparative genomics tool for circular genomes. Nucleic Acids Res. 36 (Web Server issue), W181–W184. doi: 10.1093/nar/gkn179
Haidar, G., Clancy, C. J., Shields, R. K., Hao, B., Cheng, S., Nguyen, M. H. (2017). Mutations in blaKPC-3 that confer ceftazidime-avibactam resistance encode novel KPC-3 variants that function as extended-spectrum beta- lactamases. Antimicrob. Agents Chemother. 61, e02534-16. doi: 10.1128/AAC.02534-16
Heng, Li (2016). Minimap and miniasm: fast mapping and de novo assembly for noisy long sequences. Bioinformatics 32 (14), 2103–2110. doi: 10.1093/bioinformatics/btw152
Karlowsky, J. A., Kazmierczak, K. M., Bouchillon, S. K., de Jonge, B. L. M., Stone, G. G., Sahm, D. F. (2018). In vitro activity of ceftazidime-avibactam against clinical isolates of enterobacteriaceae and pseudomonas aeruginosa collected in Asia-pacific countries: Results from the INFORM global surveillance program 2012 to 2015. Antimicrob. Agents Chemother. 62 (7), e2517–e2569. doi: 10.1128/AAC.02569-17
Karlowsky, J. A., Kazmierczak, K. M., Bouchillon SK de Jonge, B. L. M., Stone, G. G., Sahm, D. F. (2019). In vitro activity of ceftazidime-avibactam against clinical isolates of enterobacteriaceae and pseudomonas aeruginosa collected in Latin American countries: Results from the INFORM global surveillance program 2012 to 2015. Antimicrob. Agents Chemother. 63 (4), e1814–e1818. doi: 10.1128/AAC.01814-18
Kazmierczak, K. M., de Jonge, B., Stone, G. G., Sahm, D. F. (2018). In vitro activity of Ceftazidime/Avibactam against isolates of pseudomonas aeruginosa collected in European countries: INFORM global surveillance 2012-15. J. Antimicrob. Chemother. 73 (10), 2777–2781. doi: 10.1093/jac/dky267
Khezri, A., Avershina, E., Ahmad, R. (2021). Hybrid assembly provides improved resolution of plasmids, antimicrobial resistance genes, and virulence factors in escherichia coli and klebsiella pneumoniae clinical isolates. Microorganisms 9, 2560. doi: 10.3390/microorganisms9122560
Kiwei, Z., Chen, J., Shen, H., Chen, Z., Yang, Q.-w., Zhu, J., et al. (2021). Emergence of ceftazidime- and avibactam-resistant klebsiella pneumoniae carbapenemase-producing pseudomonas aeruginosa in China. mSystems 6 (6), e00787-21. doi: 10.1128/mSystems.00787-21
Kolmogorov, M., Yuan, J., Lin, Y., Pevzner, P. (2019). Assembly of long, error-prone reads using repeat graphs. Nat. Biotechnol. 37, 540–546. doi: 10.1038/s41587-019-0072-8
Luna, P., Rodrigues, L., Borges, A., Lopes A and Vieira, M. (2012). First report of KPC-producing pseudomonas aeruginosa in Brazil. Antimicrob. Agents Chemother. 56 (9), 4990. doi: 10.1128/AAC.00699-12
McArthur, A. G., Waglechner, N., Nizam, F., Yan, A., Azad, M. A., Baylay, A. J., et al. (2013). The comprehensive antibiotic resistance database. Antimicrob Agents Chemother 57 (7), 3348–57. doi: 10.1128/AAC.00419-13
Pasterán, F., Faccone, D., Gomez, S., De Bunder, S., Spinelli, F., Rapoport, M., et al. (2012). Detection of an international multiresistant clone belonging to sequence type 654 involved in the dissemination of KPC-producing pseudomonas aeruginosa in Argentina. J. Antimicrob. Chemother. 67 (5), 1291–1293. doi: 10.1093/jac/dks032
Poirel, L., Nordmann, P., Lagrutta, E., Cleary T and Muñoz-Price, L. (2010). Emergence of KPC-producing pseudomonas aeruginosa in the united states. Antimicrob. Agents Chemother. 54, 3072. doi: 10.1128/AAC.00513-10
Rada, A. M., de la Cadena, E., Agudelo, C. A., Pallares, C., Restrepo, E., Correa, A., et al. (2021). Genetic diversity of multidrug-resistant pseudomonas aeruginosa isolates carrying blaVIM-2 and blaKPC-2 genes that spread on different genetic environment in Colombia. Front. Microbiol. 12, 663020. doi: 10.3389/fmicb.2021.663020
Ramírez, D. G., Nicola, F., Zarate, S., Relloso, S., Smayevsky, J., Arduino, S. (2013). Emergence of pseudomonas aeruginosa with KPC-type carbapenemase in a teaching hospital: an 8-year study. J. Med. Microbiol. 62 (10), 1565–1570. doi: 10.1099/jmm.0.059923-0
Rodríguez-Beltrán, J., Cabot, G., Valencia, E. Y., Costas, C., Bou, G., Oliver, A., et al. (2015). N-acetylcysteine selectively antagonizes the activity of imipenem in pseudomonas aeruginosa by an OprD-mediated mechanism. Antimicrob. Agents Chemother. 59 (6), 3246–3251. doi: 10.1128/AAC.00017-15
Rodríguez-Martínez, J. M., Poirel, L., Nordmann, P. (2009). Extended-spectrum cephalosporinases in pseudomonas aeruginosa. Antimicrob. Agents Chemother. 53 (5), 1766–1771. doi: 10.1128/AAC.01410-08
Seemann, T. (2014). Prokka: rapid prokaryotic genome annotation. Bioinformatics 30, 2068–2069. doi: 10.1093/bioinformatics/btu153
Shields, R. K., Nguyen, M. H., Press, E. G., Chen, L., Kreiswirth, B. N., Clancy, C. J. (2017a). Emergence of ceftazidime-avibactam resistance and restoration of car- bapenem susceptibility in klebsiella pneumoniae carbapenemase- producing K pneumoniae: a case report and review of literature. Open Forum Infect. Dis. 4, ofx101. doi: 10.1093/ofid/ofx101
Shields, R. K., Nguyen, M. H., Press, E. G., Chen, L., Kreiswirth, B. N., Clancy, C. J. (2017b). In vitro selection of meropenem resistance among ceftazidime- avibactam-resistant, meropenem-susceptible klebsiella pneumoniae iso- lates with variant KPC-3 carbapenemases. Antimicrob. Agents Chemother. 61, e00079-17. doi: 10.1128/AAC.00079-17
Sun, L., Li, H., Wang, Q., Liu, Y., Cao, B. (2021). Increased gene expression and copy number of mutated blaKPC lead to high-level ceftazidime/avibactam resistance in klebsiella pneumoniae. BMC Microbiol. 21 (1), 230. doi: 10.1093/femsle/fnw166
Suresh, M., Skariyachan, S., Narayanan, N., Pullampara Rajamma, J., Panickassery Ramakrishnan, M. K. (2020). Mutational variation analysis of oprD porin gene in multidrug-resistant clinical isolates of pseudomonas aeruginosa. Microb. Drug Resist. 26 (8), 869–879. doi: 10.1089/mdr.2019.0147
Temkin, E., Torre-Cisneros, J., Beovic, B., Benito, N., Giannella, M., Gilarranz, R., et al. (2017). Ceftazidime-avibactam as salvage therapy for infections caused by carbapenem resistant organisms. Antimicrob. Agents Chemother. 61, e01964-16. doi: 10.1128/AAC.01964-16
Torrens, G., van der Schalk, T. E., Cortes-Lara, S., Timbermont, L., Del Barrio-Tofiño, E., Xavier, B. B., et al. (2022). Susceptibility profiles and resistance genomics of pseudomonas aeruginosa isolates from European ICUs participating in the ASPIRE-ICU trial. J. Antimicrob. Chemother. 22, dkac122. doi: 10.1093/jac/dkac122
Tsivkovski, R., Lomovskaya, O. (2020). Potency of vaborbactam is less affected than that of avibactam in strains producing KPC-2 mutations that confer resistance to ceftazidime-avibactam. Antimicrob. Agents Chemother. 64, e01936-19. doi: 10.1128/AAC.01936-19
Vaser, R., Šikić, M. (2021). Time- and memory-efficient genome assembly with raven. Nat. Comput. Sci. 1, 332–336. doi: 10.1038/s43588-021-00073-4
Villegas, M., Lolans, K., Correa, A., Kattan, J., Lopez, J., Quinn, J., et al. (2007). First identification of pseudomonas aeruginosa isolates producing a KPC-type carbapenem-hydrolyzing β -lactamase. Antimicrob. Agents Chemother. 51 (4), 1553–1555. doi: 10.1128/AAC.01405-06
Wang, Y., Wang, J., Wang, R., Cai, Y. (2020). Resistance to ceftazidime-avibactam and underlying mechanisms. J. Glob. Antimicrob. Resist. 22, 18–27. doi: 10.1016/j.jgar.2019.12.009
Wick, R., Judd, L., Cerdeira, L., Hawkey, J., Méric, G., Vezina, B., et al. (2021). Trycycler: consensus long-read assemblies for bacterial genomes. Genome Biol. 22, 266. doi: 10.1186/s13059-021-02483-z
Wick, R. R., Judd, L. M., Gorrie, C. L., Holt, K. E. (2017). Unicycler: resolving bacterial genome assemblies from short and long sequencing reads. PloS Comput. Biol. 13 (6), e1005595. doi: 10.1371/journal.pcbi.1005595
Winkler, M. L., Papp-Wallace, K. M., Bonomo, R. A. (2015). Activity of ceftazidime/avibactam against isogenic strains of escherichia coli containing KPC and SHV β-lactamases with single amino acid substitutions in the Ω-loop. J. Antimicrob. Chemother. 70 (8), 2279–2286. doi: 10.1093/jac/dkv094
Wozniak, A., Figueroa, C., Moya-Flores, F., Guggiana, P., Castillo, C., Rivas, L., et al. (2021). A multispecies outbreak of carbapenem-resistant bacteria harboring the blaKPC gene in a non-classical transposon element. BMC Microbiol. 21 (1), 107. doi: 10.1186/s12866-021-02169-3
Wozniak, A., Paillavil, B., Legarraga, P., Zumarán, C., Prado, S., García, P. (2019). Evaluation of a rapid immunochromatographic test for detection of KPC in clinical isolates of enterobacteriaceae and pseudomonas species. Diagn. Microbiol. Infect. Dis. 95 (2), 131–133. doi: 10.1016/j.diagmicrobio.2019.05.009
Wozniak, A., Villagra, N. A., Undabarrena, A., Gallardo, N., Keller, N., Moraga, M., et al. (2012). Porin alterations present in non-carbapenemase-producing enterobacteriaceae with high and intermediate levels of carbapenem resistance in Chile. J. Med. Microbiol. 61, 1270–1279. doi: 10.1099/jmm.0.045799-0
Keywords: ceftazidime/avibactam resistance, pseudomonas aeruginosa, D179Y substitution, blaKPC-2 gene, Tn4401b transposon
Citation: García P, Brito B, Alcalde-Rico M, Munita JM, Martínez JRW, Olivares-Pacheco J, Quiroz V and Wozniak A (2022) Acquisition of resistance to ceftazidime-avibactam during infection treatment in Pseudomonas aeruginosa through D179Y mutation in one of two blaKPC-2 gene copies without losing carbapenem resistance. Front. Cell. Infect. Microbiol. 12:981792. doi: 10.3389/fcimb.2022.981792
Received: 29 June 2022; Accepted: 12 August 2022;
Published: 02 September 2022.
Edited by:
Andres Felipe Opazo-Capurro, University of Concepcion, ChileReviewed by:
Barbara Ghiglione, University of Buenos Aires, ArgentinaCopyright © 2022 García, Brito, Alcalde-Rico, Munita, Martínez, Olivares-Pacheco, Quiroz and Wozniak. This is an open-access article distributed under the terms of the Creative Commons Attribution License (CC BY). The use, distribution or reproduction in other forums is permitted, provided the original author(s) and the copyright owner(s) are credited and that the original publication in this journal is cited, in accordance with accepted academic practice. No use, distribution or reproduction is permitted which does not comply with these terms.
*Correspondence: Aniela Wozniak, YW5pZWxhLndvem5pYWtAZ21haWwuY29t; YXdvem5pYWtAdWMuY2w=
†These authors have contributed equally to this work
Disclaimer: All claims expressed in this article are solely those of the authors and do not necessarily represent those of their affiliated organizations, or those of the publisher, the editors and the reviewers. Any product that may be evaluated in this article or claim that may be made by its manufacturer is not guaranteed or endorsed by the publisher.
Research integrity at Frontiers
Learn more about the work of our research integrity team to safeguard the quality of each article we publish.