- 1Institut für Medizinische Mikrobiologie und Krankenhaushygiene, Medizinische Fakultät der Otto-von-Guericke Universität Magdeburg, Magdeburg, Germany
- 2Universitätsklinik für Plastische, Ästhetische und Handchirurgie Medizinische Fakultät der Otto-von-Guericke Universität Magdeburg, Magdeburg, Germany
- 3Landesamt für Verbraucherschutz Sachsen-Anhalt, Halle (Saale), Germany
Erysipelothrix rhusiopathiae is a facultative anaerobic, environmentally stable, Gram-positive rod that causes swine and avian erysipelas as a zoonotic pathogen. In humans, the main manifestations described are circumscribed erysipeloid, generalized erysipeloid, and endocarditis. Here, we report a 46-year-old female patient who presented to the physician because of redness and marked functio laesa of the hand, in terms of a pain-related restricted range of motion, and was treated surgically. E. rhusopathiae was detected in tissue biopsy. The source of infection was considered to be a pond in which both swine and, later, her dog bathed. The genome of the isolate was completely sequenced and especially the presumptive virulence associated factors as well as the presumptive antimicrobial resistance genes, in particular a predicted homologue to the multiple sugar metabolism regulator (MsmR), several predicted two-component signal transduction systems, three predicted hemolysins, two predicted neuraminidases, three predicted hyaluronate lyases, the surface protective antigen SpaA, a subset of predicted enzymes that potentially confer resistance to reactive oxygen species (ROS), several predicted phospholipases that could play a role in the escape from phagolysosomes into host cell cytoplasm as well as a predicted vancomycin resistance locus (vex23-vncRS) and three predicted MATE efflux transporters were investigated in more detail.
Introduction
A 46-year-old female patient presented to our clinic with swelling, redness, and pain on the right thumb that had been progressive for two days. The patient reported that she suffered a minor lesion of the thumb pad from cracking walnuts. On inspection, a blister approximately 1 cm in diameter was found on the palmar end phalanx of the right thumb, still covered by intact skin. A marked swelling with semicircular redness extended down to the proximal phalanx. On the extensor surface, a well-demarcated striated lymphangitis reached across the wrist down to the distal forearm. Clinical inspection did not allow a clear distinction between erysipelas and cellulitis. To explore, whether a foreign body may have remained from walnut cracking, an incision was made under local anesthesia, which revealed no pus, no indurated tissue, and no foreign body.
Background
The genus Erysipelothrix
E. rhusiopathiae belongs to the Erysipelotrichaceae family and is the only human pathogenic microbial species of the genus Erysipelothrix. Further microbial species of the genus have been described more recently and include Erysipelothrix tonsillarum (Takahashi et al., 1987), Erysipelothrix inopinata (Verbarg et al., 2004), Erysipelothrix muris (Chen et al., 2006), Erysipelothrix larvae (Bang et al., 2015; Bang et al., 2016), Erysipelothrix piscisicarius (Pomaranski et al., 2020), Erysipelothrix anatis sp. nov., Erysipelothrix aquatica sp. nov., Erysipelothrix urinaevulpis (Eisenberg et al., 2022) and the as yet undesignated Erysipelothrix species 1, Erysipelothrix species 2, and Erysipelothrix species 3 (Takahashi et al., 2008).
Historical classification of the species Erysipelothrix rhusiopathiae
The species designation changed a number of times. Robert Koch first isolated a bacterium of the genus Erysipelothrix in 1876 from a mouse that he had previously inoculated with putrid blood. He designated this pathogen as the bacterium of mouse septicemia, E. mursiseptica (Wang et al., 2010). Friedrich Löffler isolated a similar organism, under the name Bacillus of swine erysipelas (latinized by Kitt in 1893 as Bacillus rhusiopathiae suis), from the skin blood vessels of a pig that had died of swine erysipelas in 1886 and he was the first to describe the pathogen and the disease caused by it in swine (Wang et al., 2010). Friedrich Julius Rosenbach isolated a bacterium similar to Robert Koch’s bacterium from a patient with localized skin lesions in 1909, so that Erysipelothrix was now established as a human pathogen after initial case reports since 1870. Rosenbach coined the term “erysipeloid” to distinguish between the streptococcal infection “erysipelas” and the efflorescence he had observed. Rosenbach still distinguished three different microbial species: E. muriseptica, E. porci, and E. erysiploides, depending on their origin of isolation from mice, swine, or humans (Wang et al., 2010). Later it was discovered that they were three nearly identical isolates of the same microbial species, which was named E. insidiosa, as originally proposed by Trevisan in 1885. This name, as well as all other 36 names circulating for this bacterium at the time, were discarded in 1966 in favor of E. rhusiopathiae, a combination of genus and species that had been coined as early as 1918 (Wang et al., 2010). According to comparative genomic analyses, the species E. rhusiopathiae can be further subdivided into three clades, as well as a fourth clade phylogenetically intermediate between clades 2 and 3 (Forde et al., 2020). In addition, Erysipelothrix spp. can be divided into at least 28 known serovars (Opriessnig et al., 2020). Serovar 1 is considered to be the most virulent and therefore of greatest veterinary importance (Selbitz et al., 2011).
Culture and morphology
The cell wall of E. rhusiopathiae consists among others of lysine and glycine, which distinguishes it from Listeria and Corynebacteria. The negative catalase test differentiates Erysipelothrix spp. from Kurthia spp. In the Gram stain, Gram-positive rods are recognizable in chain formation, but with a highly variable single cell length, from 0.8 µm to 2.5 µm (Figure 1A). Occasionally, even filaments of up to 60 µm in length can be seen. E. rhusiopathiae is readily decolorized during Gram staining, and Gram-labile or even Gram-negative staining behavior may be apparent (Figure 1B) (Carroll et al., 2019).
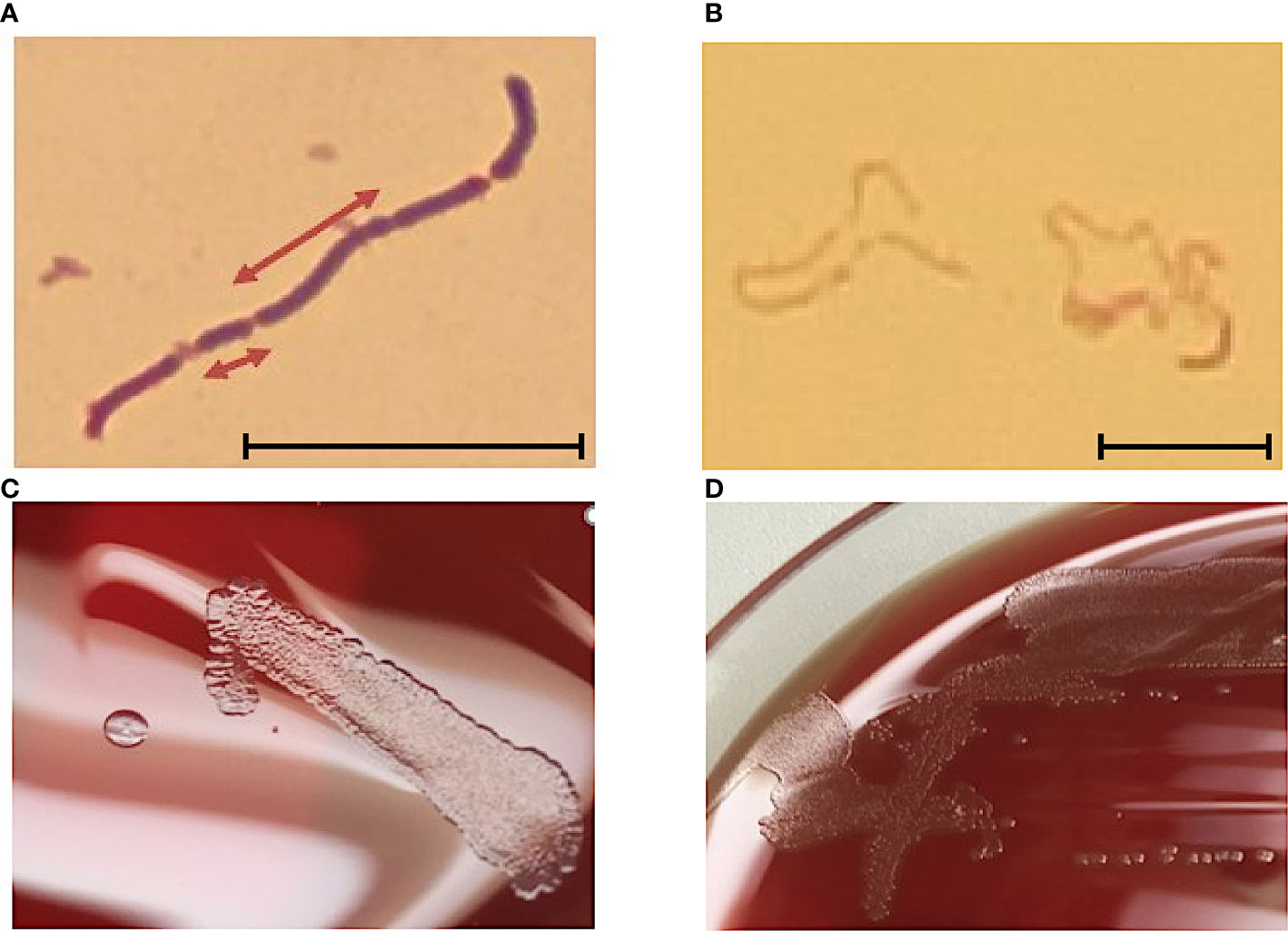
Figure 1 (A) E. rhusopathiae Gram stain (magnification 1:1000, scale bar = 5 µm). The arrows indicate two Gram-positive rods with distinctly different lengths. (B) Gram stained slide with E. rhusopathiae (magnification 1:1000, scale bar = 5 µm). A decolorized (pseudo-) Gram-negative sample is shown. This phenomenon occurs regularly when preparing the microscopic specimen with colonies appearing “rough” on agar plates. (C) Growth of E. rhusopathiae on Schaedler KV agar. On the left, a smooth clearly circumscribed pinpoint colony can be seen, next to a bed of confluent colonies (right). (D) smooth and rough colonies on Columbia sheep blood agar.
Regarding culture conditions, E. rhusiopathiae is relatively undemanding and can be grown on aerobically incubated sheep blood or chocolate blood agar. Nevertheless, a capnophilic atmosphere favors bacterial growth. When grown on Schaedler KV agar, chocolate agar, or Columbia sheep blood agar, E. rhusiopathiae exhibits two colony morphologies. On the one hand, it appears as a smooth (S) colony in the form of clearly delimited pin-points, on the other hand, as a rough (R) colony with irregular boundaries (Figures 1C, D) (Carroll et al., 2019). The S-form is particularly observable in initial culture from acute disease cases, but the R-form is particularly observable in culture from chronic disease cases and after more frequent passaging in vitro (Selbitz et al., 2011). Moreover, the facultative anaerobic bacterium can be cultivated in trypticase soy or Schaedler broth. To suppress possible accompanying flora, especially when isolating E. rhusiopathiae from environmental and marine locations, or from animal waste and products, numerous selective media were developed (Brooke and Riley, 1999; Fidalgo et al., 2000; Wang et al., 2010).
Identification
Recently, species identification of E. rhusiopathiae has become a minor issue with availability of MALDI-TOF mass spectrometry and modern biochemical methods such as VITEK® 2 GP ID card (Biomérieux, Nürtingen, Germany), API® Coryne (Biomérieux, Nürtingen, Germany), Rapid ID 32 STREP (Biomérieux, Nürtingen, Germany), or BD Phoenix™ PID Panel (BD Biosciences, Sparks, MD, USA). More reliable identification may have contributed to an observed increase of E. rhusiopathiae detection in human specimen since about 2008 (Farfour et al., 2012; Principe et al., 2016). Additionally, several PCR-based assays have been developed to detect E. rhusopathiae; to differentiate it from other Erysipelothrix species; and to subdifferentiate (serotype) within the microbial species (Fidalgo and Riley, 2004; Yamazaki, 2006; Pal et al., 2010; Shiraiwa et al., 2017; Shimoji et al., 2020). For epidemiological purposes, different subtyping methods have been developed based on multilocus sequence typing (MLST), pulsed-field gel electrophoresis (PFGE) (Janßen et al., 2015) and sequencing of the hypervariable region of the spaA gene (Nagai et al., 2008).
Epidemiology
E. rhusiopathiae is widespread among mammals, birds, and fish, but most commonly found in swine and their feces (Funke, 2009). In approximately 50% of healthy swine, E. rhusiopathiae can be detected in the tonsils or lymphoid tissue (Spiteri and Taylor-Robinson, 2018). Due to its high tenacity, the pathogen persists for several months in soils and water bodies as well as in decaying animals and fish (Selbitz et al., 2011). Human infection usually occurs in occupational groups exposed to appropriate animal products or excreta, such as farmers, veterinarians, furriers, butchers, fishermen, fishmongers, homemakers, cooks, and grocers.
Disease in swine and other animals
Susceptible to infection with E. rhusiopathiae are primarily swine between 3 and 12 months of age. After oral, conjunctival, or percutaneous infection, bacteremia occurs after three to five days of incubation, later resulting in the characteristic manifestations of the skin (Selbitz et al., 2011). Acute swine erysipelas, most commonly associated with serovar 1 - subtype 1a, manifests with high fever up to 42°C and the typical landmark redness of the skin. In the peracute form of the disease, the animals can also die before the pathognomonic symptoms have developed. This is referred to as “white erysipelas” (Selbitz et al., 2011). The subacute but also the acute course is characterized by pathognomonic raised rhomboid skin lesions of several centimeters in diameter that are called “diamond skin”. Chronicity of the symptoms of erysipelas, that this means a disease duration of more than four weeks, is possible. Chronic erysipelas may occur as a result of acute disease but may also be a direct consequence of persistent infection with low virulent strains of E. rhusiopathiae. Typical manifestations are skin necrosis, polyarthritides and/or endocarditis valvularis, which may be manifested by cauliflower-like thrombotic-ulcerative deposits on the atrioventricular valves (Selbitz et al., 2011). E. rhusiopathiae infections are not restricted to swine. It also occurs in sheep, in which the disease frequently manifests as chronic polyarthritis and rarely as septicemia, as well as in turkeys, ducks, chickens, mice, rats, beavers, cattle, rabbits, horses, minks, foxes, and dolphins (Selbitz et al., 2011). For prophylaxis, 17 different commercial vaccines are currently available in veterinary medicine (Opriessnig et al., 2020).
Clinical manifestations in humans
In contrast to swine, serotypes 2, 7, and 16 are the most commonly involved in the pathogenesis of human erysipeloid (Veraldi et al., 2009). If the pathogen enters the skin via a wound, there are basically three clinical manifestations: a localized skin lesion (so-called erysipeloid), a generalized skin lesion, and bloodstream infection. Local erysipeloid is characterized by sharply circumscribed, painful, reddish, non-repressible edema often accompanied by vesiculation and erosive lesions without pus formation (Wang et al., 2010). In addition, arthralgia, myalgia, lymphadenitis and mild fever may occur (Rostamian et al., 2022). Frequently, the back of the hand (as in our patient) or the extensor side of the fingers are affected, because the tendons form a row being wrapped around very tight (Veraldi et al., 2009). Usually, the spread of the pathogen is limited to a roundish area at the point of entry. Rarely, the so-called multiple or systemic erysipeloid is reported. This results in the radial spread of multiple erysipeloids from the original focus (Wang et al., 2010) particularly seen in immunocompromised individuals (Veraldi et al., 2009). Bloodstream infection is rare but associated with severe disease, e.g. endocarditis (Drekonja, 2013; Hofseth et al., 2017; Wang et al., 2020). Other complications include meningitis, osteomyelitis, or septic arthritis (Wang et al., 2010; Groeschel et al., 2019). Immunosuppression represents an important causal factor for systemic infection.
Methods
Culture, species identification and susceptibility testing
According to the diagnostic routine at the Department of Medical Microbiology and Hospital Hygiene of the Medical Faculty of Otto-von-Guericke University Magdeburg, Columbia CNA agar with 5% sheep blood (aerobic), Schaedler/Schaedler KV agar (anaerobic) and Schaedler broth (media obtained from Fisher Scientific GmbH, Schwerte, Gemany) were inoculated with the tissue biopsy taken.
Species identification was performed both biochemically, using a VITEK® 2 GP ID card and a VITEK® 2 XL device (Biomérieux, Nürtingen, Germany) as well as by MALDI-TOF MS (Vitek-MS, Biomérieux, Nürtingen, Germany).
Susceptibility testing was carried out using MIC test strips (Liofilchem S.r.l., Roseto degli Abruzzi (Teramo), Italy).
Whole genome sequencing
Prior to DNA isolation the E. rhusiopathiae isolate was cultivated on Columbia agar supplemented with 5% sheep blood (Becton Dickinson, Beckton-Dickinson, New Jersey, USA) and incubated at 37°C for 16h. DNA was isolated from agar colony material (ca. 5 C.F.U.) using the CTAB-lysozyme protocol by Larsen and coworkers (Larsen et al., 2007). Cells were harvested (10 min., 3,000 x g), resuspended in 450 µl GTE solution (10 mM EDTA and 50 mM Glucose in 25 mM Tris-CL) and digested for one hour at 37°C with lysozyme (adding 50 µl of 10 mg/ml lysozyme to a final concentration of 1 mg/ml). Then, the cell suspension was incubated after adding 150 µl proteinase K (10 mg/ml) in 10% SDS (30 min, 55°C). Treatment with 4 µl RNase A (Qiagen, Hilden Germany; 700 U/ml, 2 min, room temperature) was followed by addition of 200 µl NaCl (5 M). CTAB (4.1 g NaCl in 90 ml water + 10 g cetrimide, Sigma cat. No. H5882, in NaCl) was preheated to 65°C, 160 µl were added and the solution was incubated (10 min, 65°C). This is followed by 2 extraction steps of a chloroform:isoamyl alcohol extraction. After adding chloroform/isoamylalkohol (24:1, ca. 1 ml) the solution was centrifuged (10,000 x g, 5 min). Then the upper aqueous phase was transferred to a fresh tube and again chloroform/isoamylalkohol (24:1, ca. 0,9 ml) was added. The solution was centrifuged once again (10,000 x g, 5 min). The aqueous layer (800 µl) was transferred to a fresh tube, 560 µl isopropanol were added, and the tube was inverted until DNA precipitates. The suspension was incubated for 5 min at room temperature and subsequently centrifuged (10,000 x g, 10 min). Afterwards, the pellet was washed twice with ethanol (70%, 10,000 x g, 10 min). 50 µl TE-buffer were added after 15 min of air-drying. Library preparation was performed using the TruePrep DNA Library Prep Kit V2 for Illumina (1 ng) (Vazyme Biotech Co. Ltd., Nanjing, China) and samples were barcoded with the Nextera XT Index Kit (24 indexes, 96 samples, Illumina, San Diego, USA).
Bioinformatics
Data were analyzed with Ridom SeqSphere+ (Ridom™, Münster, Germany) using a custom made core genome with the Fujisawa strain (NC_015601.1) as seed genome. Nine different E. rhusiopathiae genomes were used as query genomes (SY1027, NC_021354.1; GXBY-1, NZ_CP014861.1; WH13013, NZ_CP017116.1; ML101, NZ_CP029804.1; KC-Sb-R1; NZ_CP033601.1; NCTC8163; NZ_LR134439.1; G4T10, NZ_CP011860.1; SE38; NZ_CP011861.1; ZJ, NZ_CP041995.1). The samples were analyzed after a de novo assembly with the SKESA (version 2.3.0) algorithm (Souvorov et al., 2018).
Screening for the presence of antimicrobial resistance genes and point mutations causing antimicrobial resistance was performed using Resfinder V4.1 (Zankari et al., 2012), PointFinder (Zankari et al., 2017), and ResFinderFG V1.0 (Sommer et al., 2009; Pehrsson et al., 2016).
Results and discussion
Microbiological results and clinical course
E. rhusiopathiae was cultured from the wound biopsy. The microbial species was identified using both VITEK® 2 GP ID card with 98% likelihood and MALDI ToF/Vitek MS (99.9%). Susceptibility testing using MIC test strips revealed the minimum inhibitory concentrations (MIC) listed in Table 1. For rarely isolated species such as E. rhusiopathiae, no specific breakpoints currently exist according to the EUCAST (European Committee on Antimicrobial Susceptibility Testing) guidelines, therefore the interpretation of MIC values was according to the non-species-specific EUCAST PK-PD breakpoints. Following the EUCAST guidance document for the use of PK-PD breakpoints, the results of susceptibility testing cannot be reported in a categorical terms, but only in the form of a guidance for treatment. Accordingly, antimicrobials listed as S “may be used for treatment”, and substances listes as R “should not be used for therapy”. Therefore, due to the non-species-specific EUCAST PK-PD breakpoints, the use of benzylpenicillin, the antibiotic of choice, aminopenicillins cephalosoprines, carbapenems, and fluoroquinolones can be encouraged. In contrast, the U.S. Clinical Laboratory Standards Institute (CLSI) defines E. rhusiopathiae specific breakpoints in its document M45 “Methods for Antimicrobial Dilution and Disk Susceptibility Testing of Infrequently Isolated or Fastidious Bacteria”, according to which benzylpenicillin, ampicillin, ceftriaxone, meropenem, imipenem, ciprofloxacin, and levofloxacin have been tested susceptible (Table 1). Other potential therapy options due to the CLSI breakpoints were macrolides (such as erthromycin) and clindamycin. However, no EUCAST PK-PD breakpoints are defined for these antimicrobials. The (intrinsic) resistance to vancomycin and aminoglycosides described in the literature was confirmed in the measured MIC values.
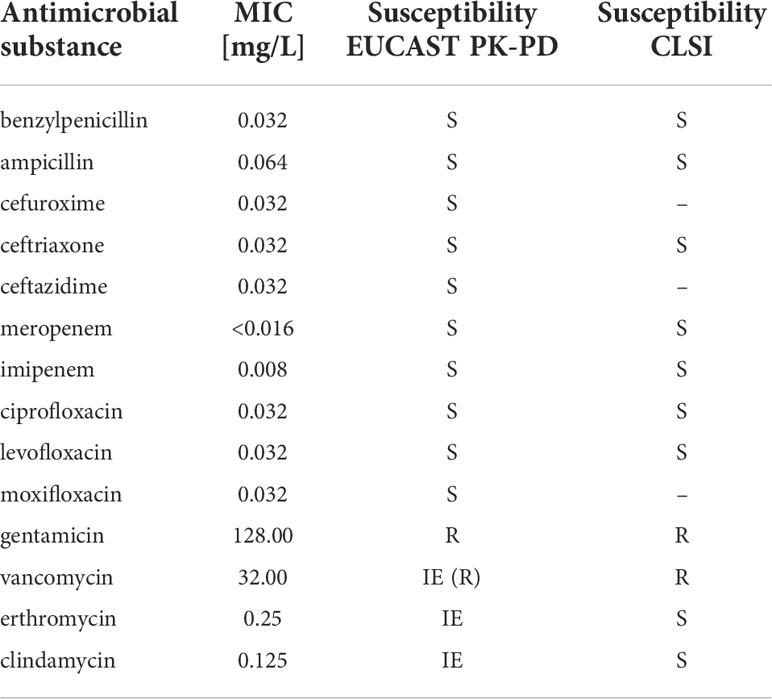
Table 1 Susceptibility of E. rhusiopathiae 319078 to various antimicrobials and assessment according to EUCAST PK-PD breakpoints.
Postoperative therapy was initial administration of cefazolin 2 g intravenously, followed by ampicillin/sulbactam (Unacid) orally (375 mg q6h) for 5 days (Table 2). The follow-up treatment was according to the standard of the “University Clinic for Plastic and Hand Surgery”. The intraoperative wound flap was removed on the first postoperative day. By the fifth postoperative day, there was complete regression of redness, including lymphangitis and swelling. The skin in the wound area appeared non-irritant with contiguous wound edges and splint immobilization of the thumb was terminated. The suture material was removed on the 14th postoperative day.
The origin of the pathogen could not be clarified with complete certainty. The patient denied any direct contact to pigs, including handling of raw pork. She suspected that the source of infection could be a pond in which pigs usually bathe. The pond had no connection to other waters. It was located near several farms and functioned as a watering and bathing place for a number of animals. Fish that could potentially be another source of E. rhusiopathiae were not observed in the water body and it is also very unlikely that this water body was a suitable habitat for fish. Her dog bathed in the pond and she petted and dried him afterwards. We attempted to culture E. rhusiopathiae from pond water unsuccessfully.
Genome analysis
SKESA analysis of the Illumina sequences resulted in 50 continuous long reads (CLR) with an average (total) length of 34,401 base pairs. We additionally sequenced long-reads on a MinION (Oxford Nanopore technologies ltd., Oxford, United Kingdom), and performed hybrid assembly with unicycler (v0.4.8, https://github.com/rrwick/Unicycler). This resulted in a single polished contig of 1,780,614 base pairs. The G+C content of the contig was 36.5% and the top species match identity by Ridom Seqsphere+ was E. rhusiopathiae by 99%. No extrachromosomal elements were detected.
Application of the NCBI-annotation pipeline resulted in 1,714 genes. Of this total number of genes, 1,621 are protein-coding genes, 13 are pseudo-genes, and 80 are RNA-coding genes. Of the 80 RNA-coding genes, 55 encode tRNAs, 4 encode ncRNAs, 7 encode 5S rRNAs, 7 encode 16S rRNAs, and 7 encode 23S rRNAs. The genome harboured neither prophages nor transposable elements.
Additional application of the RAST-annotation pipeline yielded 1152 predicted coding sequences and 508 predicted hypothetical proteins. Based on sequence identity, motif analysis, and structural homology to proteins of known function (mostly from other microbial species), a functional prediction of protein function is made as part of the annotation process, which usually requires further experimental verification. The functional categorization of the predicted coding sequences is based on a pure in silico analysis that would have to be confirmed in vitro or in vivo to be considered certain. The RAST subsystem coverage was 25% (413 of 1660 genes). “Protein metabolism” (107 of 558 terms, 19.2%), “carbohydrate metabolism” (104 terms, 18.6%), “nuceloside/nucleotide synthesis” (48 terms, 8.6%), “amino acid metabolism” (47 terms, 8.4%), and “Cofactors, Vitamins, Prosthetic Groups, Pigments” (42 terms, 7.5%) form the largest functional categories in terms of number (Figure 2). In order to classify this subsystem category distribution, the distribution of our human isolate was compared to a bovine, a dolphin and a porcine isolate (Table 3). However, it must be taken into account that the porcine isolate was also present as a closed genome (1 contig), the dolphin isolate was present as an incomplete genome consisting of 109 contigs, and the bovine isolate genome consisted of 240 contigs from a bovine ruminal metagenome project. Comparison showed that the subsystem category distributions were nearly identical in the human, the porcine, and the dolphin isolate. In the dolphin isolate, one category stood out in comparison to the human and the porcine isolate: “phages, prophages, transposable elements, plasmids”. The genome of the dolphin isolate contained a temperate phage, as indicated by the corresponding genes for a phage terminase, a phage portal protein, a phage-associated type III restriction enzyme, and various bacteriophage hypothetical proteins, among others. The incomplete E. rhusiopathiae genome of bovine origin diverged most significantly from the genomes of the other three isolates in subsystem category distribution (Table 3). However, these discrepancies were most likely due to the fact that the contigs were derived from a metagenome analysis.
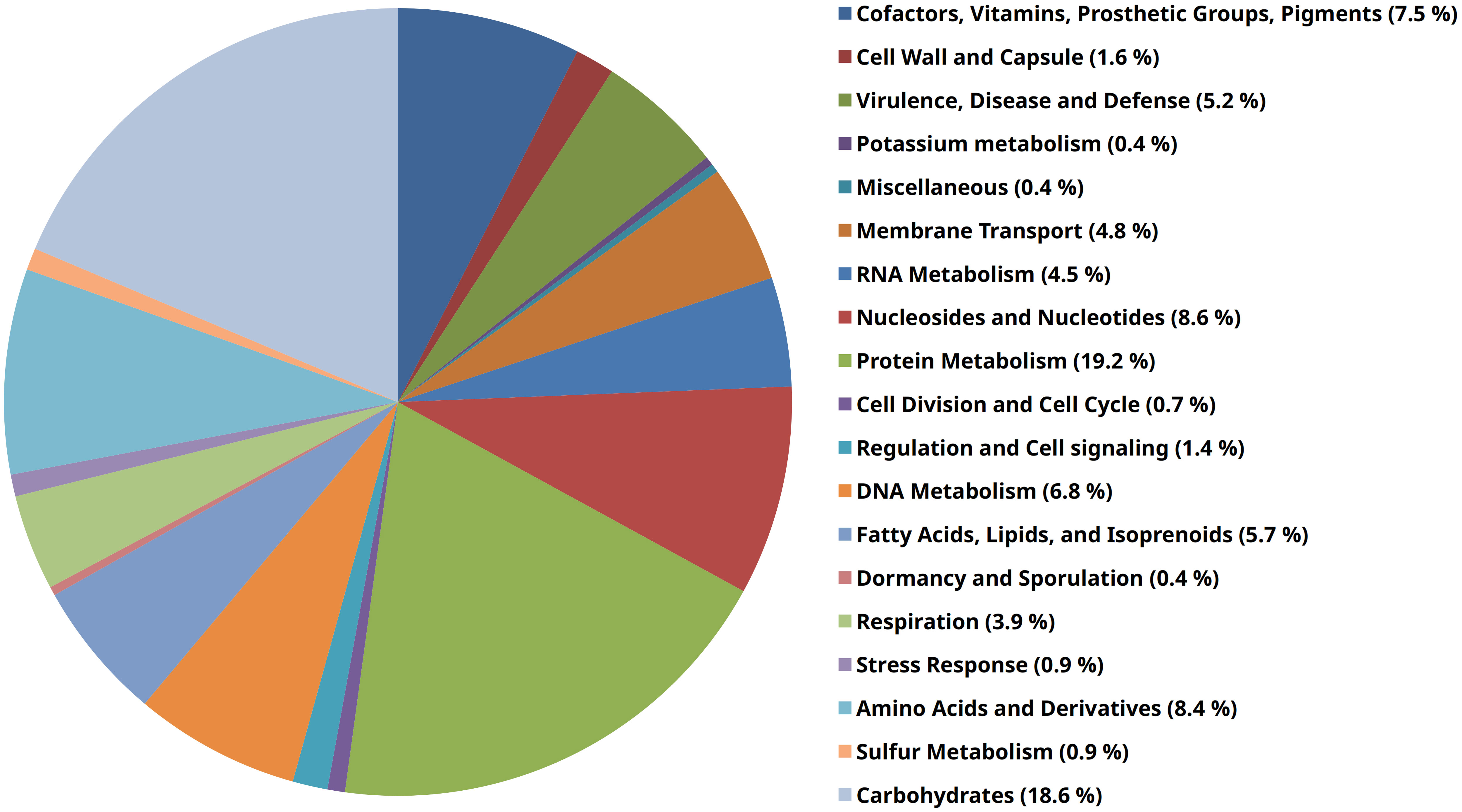
Figure 2 Pie chart of RAST subsystems that were identifiable in the genome of E. rhusiopathiae 319078. The 19 most abundant subsystems at the “category” level identified by RAST are represented by a specific color in the legend on the right side of the figure.
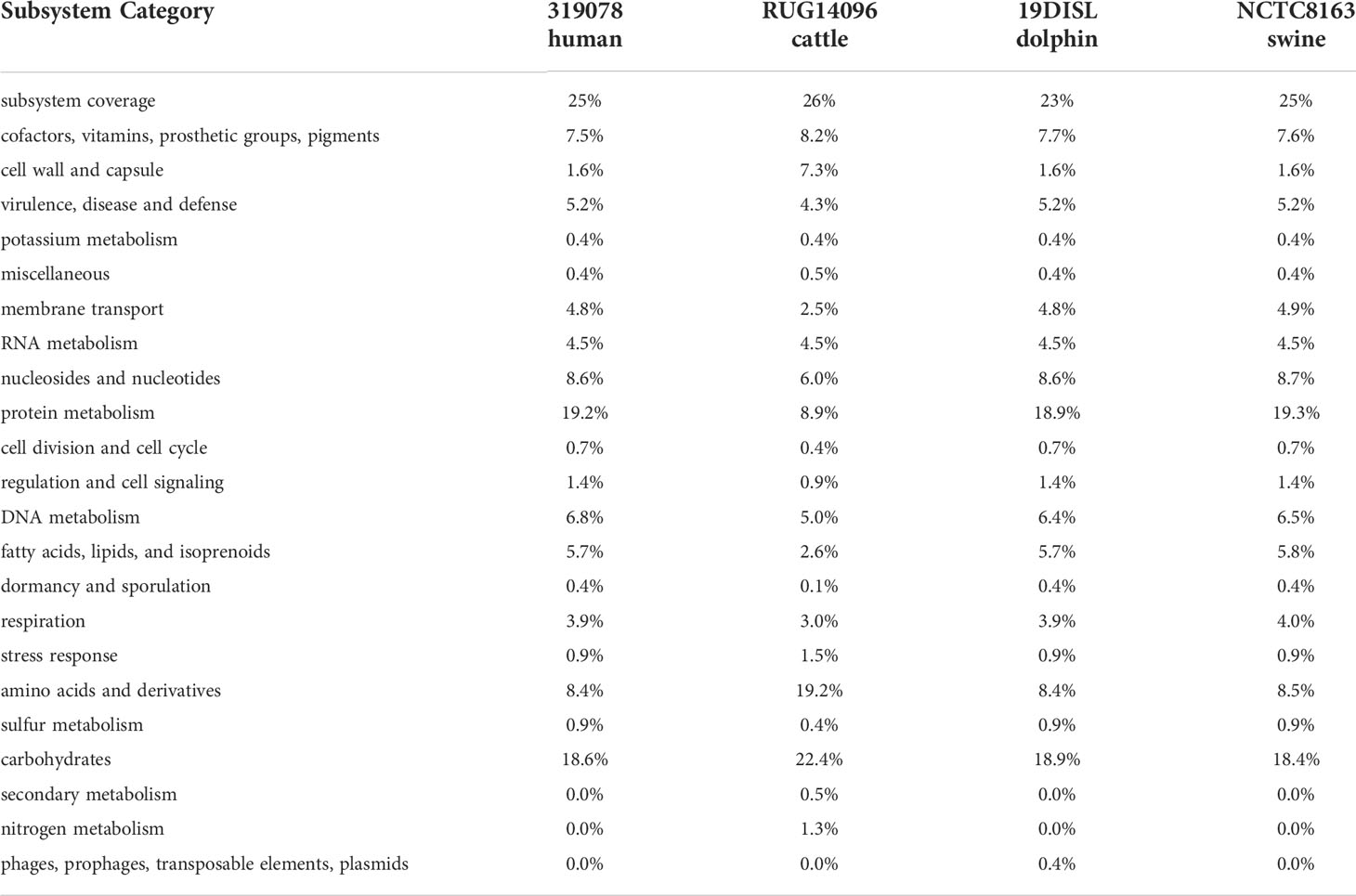
Table 3 Comparison of functional subsystem category distribution of E. rhusiopathiae isolates of different origins.
Antimicrobial resistance genes
Analysis of the genome using Resfinder V4.1, PointFinder, and ResFinderFG V1.0 (Selected %ID threshold 50% & Selected minimum length 40% for both tools) did not reveal any acquired antimicrobial resistance genes. Genes encoding gyrase (gyrA/B) and topoisomerase IV (parC/parE) were identified as potential determinants of quinolone resistance, but since no quinolone resistance was detected phenotypically, it was assumed that these genes were present in the wild-type form and did not contain point mutations that cause quinolone resistance. Furthermore, the genome of E. rhusiopathiae 319078 contained three genes encoding for proteins with homology to a MATE (Multidrug And Toxic Compound Extrusion) family MDR efflux pump (Table 4). This family of multidrug efflux transporter pumps was associated with fluoroquinolone resistance in Bacteroides thetaiotaomicron (Miyamae et al., 2001) as well as with fluoroquinolone, ethidium, and aminoglycoside resistance in Vibrio parahaemolyticus (Morita et al., 1998). However, the presence of these genes encoding proteins with a predicted function of MATE family efflux transporters did not confer phenotypic quinolone resistance but could be a factor accounting for the phenotypically observed aminoglycoside resistance in E. rhusiopathiae 319078.
A gene cluster homologous to the “Streptococcus pneumoniae vancomycin tolerance locus” was identified as a presumptive factor for intrinsic vancomycin resistance/tolerance in E. rhusiopathiae. The “Streptococcus pneumoniae vancomycin tolerance locus” (vex123-pep27-vncRS locus) consists of an ABC transporter formed by the gene products of vex1, vex2, and vex3, the two-component response regulator VncR and it’s associated sensor histidine kinase VncS as well as Pep27, a secreted peptide sensed by VncR/S (Novak et al., 1999; Mitchell and Tuomanen, 2002). In contrast, the homologous gene cluster of E. rhusiopathiae 319078 lacked the homologue of the secreted peptide gene pep27 and the homologous gene to the Vex1 subunit of the ABC transporter, a transmenbrane protein (Figure 3 and Tables 4, 5). While it has been shown that knock-out of pep27 has no effect on vancomycin-induced autolysis of S. pneumoniae (Robertson et al., 2002; Haas et al., 2004), the role of Vex1 has not yet been investigated in detail, neither in S. pneumoniae nor in E. rhusiopathiae. Vex1 and Vex3 are proposed to form a transmembrane protein channel while Vex2 is an ATP-binding cassette protein. It may be that in E. rhusiopathiae the Vex3 homolgue alone would able to shape a sufficient transmembrane channel. Since vancomycin resistance of E. rhusiopathiae is considered a characteristic resistance of this microbial species, further investigation of this four-gene gene cluster provides an interesting starting point for future experiments on vancomycin resistance. On the other hand, this gene cluster may also be completely non-functional, mainly due to the absence of a gene with predicted function of the Vex1 protein. The vex23-vncRS locus of E. rhusiopathiae is highly conserved in the available genome sequences. Among the 10 E. rhusiopathiae genomes deposited at NCBI, vex2 has 99.84% to 100% sequence identity at a 100% coverage, vex3 has 99.64% to 100% sequence identity at a 100% coverage, vncS has 99.64% to 99.86% sequence identity at a 100% coverage, and vncR (luxR) has 99.55% to 100% sequence identity at a 99% to 100% coverage.
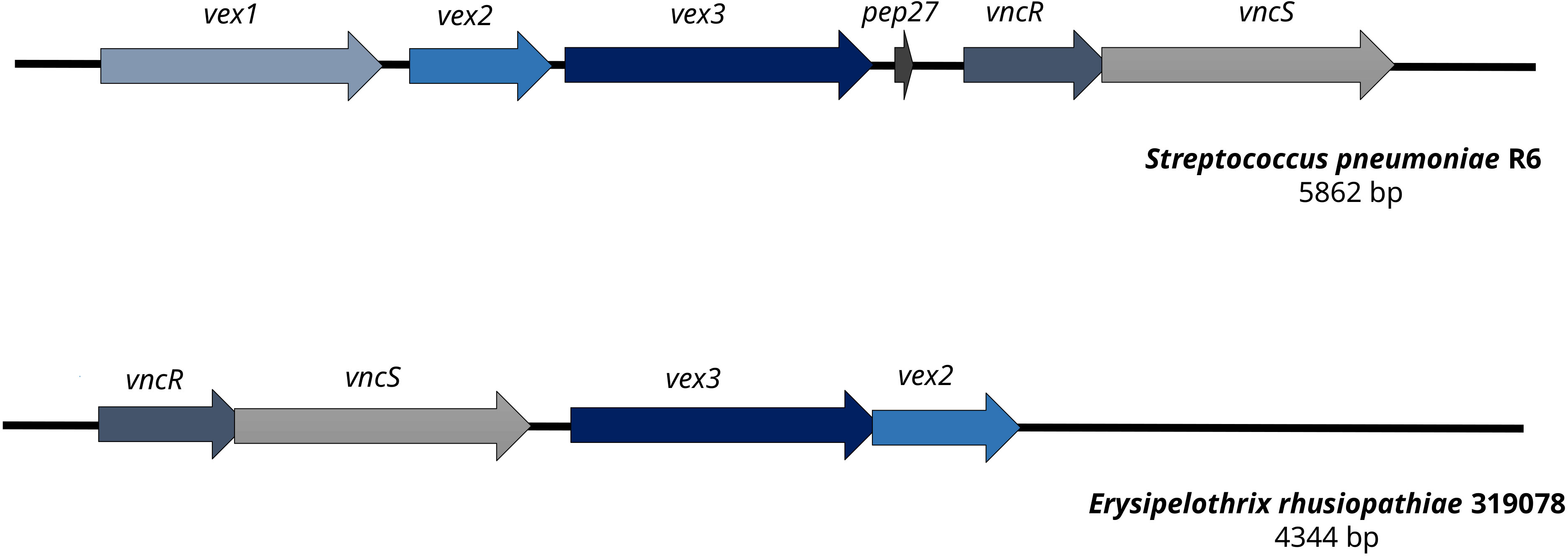
Figure 3 Comparison of the vancomycin tolerance locus of Streptococcus pneumoniae R6 and Erysipelothrix rhusiopathiae 319078. In contrast to the vancomycin tolerance locus of S. pneumoniae R6, E. rhusiopathiae 319078 lacks the pep27 and vex1 genes, yet the microbial isolate has a vancomycin MIC of 32.0 mg/L.
In addition, one gene encoding a protein with the predicted function of a class C beta-lactamase and two genes each encoding a protein with the predicted function of a metallo-beta-lactamase were also present in the genome of E. rhusiopathiae 319078 (Table 4). However, phenotypically, all penicillins, aminopenicillins, cephalosporins, and carbapenems were tested susceptible.
Peptidoglycan biosynthesis
E. rhusiopathiae possesses a complete set of genes encoding enzymes with a predicted function for peptidoglycan biosynthesis (Table 5). These genes are not organized in a cluster but are scattered throughout the whole genome. In previous writings, intrinsic vancomycin resistance of this microbial species was thought to be due to the termination of the peptide stem of the peptidoglycan with D-alanine-D-lactate. This would be similar to what was found in vancomycin-resistant enterococci of the vanA/vanB phenotype (Nelson, 1999). However, according to our annotation, this does not seem to be the case, since the predicted function of the murF gene (NBX27_03210) was a UDP-N-acetylmuramoyl-tripeptide–D-alanyl-D-alanine ligase, and thus the pentapeptide stem ending would be D-Ala-D-Ala. Nevertheless, E. rhusiopathiae has some peculiarities concerning the cell wall peptidoglycan structure. There was a discrepancy in the predicted function of the protein encoded by murC between the NCBI and RAST annotation piplines. While NCBI predicted here the function of a UDP-N-acetylmuramate–L-alanine ligase the predicted function according to RAST was UDP-N-acetylmuramate-L-serine ligase. Thus, instead of an L-Ala, there could be an L-Ser at position 1 of the pentapeptide as in Butyribacterium rettgeri (Vollmer et al., 2008). A second peculiarity exists at position 3 of the pentapeptide. Instead of an L-Lys, as found in most Gram-positive bacteria, E. rhusiopathiae has a D-Ala at this position (Vollmer et al., 2008), which was also in agreement with the predicted function of the murE gene product as a UDP-N-acetylmuramoylalanyl-D-glutamate-L-alanine ligase. The pentapeptide stem of E. rhusiopathiae therefore should have an amino acid sequence of D-Ala-D-Glu-D-Ala-D-Ala-D-Ala or possibly L-Ser-D-Glu-D-Ala-D-Ala-D-Ala. To what extent this specific structure of the pentapeptide is related to the intrinsic vancomycin resistance of the bacterium requires further investigation.
Virulence factors
The virulence factors of E. rhusiopathiae were systematically characterized as part of the first complete genome announcement by Ogawa and colleagues (Ogawa et al., 2011). After more than a decade of improvements in the annotation pipelines and since our isolate was of human origin we decided to reassess the virulence factors in the genome of our isolate.
Two-component signal transduction systems
Bacteria regulate the expression of a variety of genes, including those encoding virulence-associated factors, with two-component signal transduction systems integrating external signals. Ogawa and coworkers were able to identify a total of 15 genes that presumptively encode response regulators, and for 14 of them they were able to identify the corresponding presumptive sensor histidine kinase upstream or downstream. But unfortunately, they could assign a predicted function only for 4 two-component signal transduction systems (Ogawa et al., 2011). With the help of the annotation pipelines we employed (NCBI & RAST), we were able to assign a predicted function to all 14 two-component signal transduction systems (Table 6). As shown previously by Ogawa and coworkers, the two-component system NBX27_00670/NBX27_00665 exhibited homology to CssS & CssR and therefore its presumptive function could be the control of cellular responses to protein secretion stress (Hyyryläinen et al., 2001; Ogawa et al., 2011). Similarly, the two-component system, for which a function in the regulation of the phosphate regulon responsible for uptake of inorganic phosphate was predicted, had also been localized in the genome of E. rhusiopathiae 319078 (NBX27_04520/NBX27_04525) (Ogawa et al., 2011; Santos-Beneit, 2015). Due to their sequence identity, we could assign two further two-component systems (NBX27_01470/NBX27_01465 & NBX27_06020/NBX27_06025) to the LytTR family, which are potentially involved in regulating the expression of many virulence factors, e.g. extracellular polysaccharides, toxins and bacteriocins (Nikolskaya and Galperin, 2002; Ogawa et al., 2011). Three of the two-component systems (NBX27_01190/NBX27_01185, NBX27_07145/NBX27_07140, NBX27_07455/NBX27_07460) had response regulators with homologues to the LuxR family and could presumptively be involved in the regulation of quorum-sensing factors. The ComD/ComE system was shown to function as a negative transcriptional regulator of the capsular polysaccharide (cps) locus of S. pneumoniae (Zheng et al., 2017). A pair of homologous genes (NBX27_03955/NBX27_03960) was detected in E. rhusiopathiae 319078. The two-component signal transduction system NBX27_04290/NBX27_04285, which was homologous to vncS/vncR of S. pneumoniae, has already been discussed in the subsection “Antimicrobial resistance genes”, as it may presumptively play a role in tolerance to vancomycin via regulation of vex2 & vex3 expression. Furthermore, we could localize two-component signal transduction systems in the genome of E. rhusiopathiae 319078 for which a function in the regulation of thioredoxin reductase expression, adaptation to osmolality, invasin expression, as well as magnesium and cobalt transport was predictable (Table 5). In the vicinity of the response regulator localized at locus tag NBX27_06540, no sensor histidine kinase was found in the genome of E. rhusiopathiae 319078, so that this remains an orphan response regulator gene (Table 6).
Capsular polysaccharide synthesis
Another important virulence-associated factor is the ability of a bacterium to form a capsule, or capsular polysaccharide synthesis. A seven-gene capsular polysaccharide synthesis locus was identified in the genome of E. rhusiopathiae Fujisawa (Ogawa et al., 2011), which was also found in E. rhusiopathiae 319078 at 100% coverage and 99.24% sequence identity.
Surface-associated proteins
In the genome of E. rhusiopathiae Fujisawa, a total of 21 proteins was detected containing an LPTXTG-motif. Based on this motif, these proteins are predicted to be covalently linked to peptidoglycan chains by a specific sortase, and based on this pepdidoglycan linkage, it is assumed that these were surface-associated proteins (Ogawa et al., 2011). Both the sortase (NBX27_00075) and its potential 21 substrates were localized by us in the genome of E. rhusiopathiae 319078. Updates in the annotation are provided in Table 7. Of particular note is the surface protective antigen adhesin SpaA (NBX27_00545), which also functions as antigen in many subunit vaccines (Opriessnig et al., 2020). SpaA itself belongs to three surface proteins that bind to choline residues of teichoic acid and by this become membrane anchored (Ogawa et al., 2011; Borrathybay et al., 2015). It plays a significant role in virulence, adhesion to host cells, and serum resistance of E. rhusiopathiae (Borrathybay et al., 2015). In addition, two homologues to the Streptococcus pyogenes shaft pilin SpaA (Ramirez et al., 2020) were found in the genome (NBX27_00445 & NBX27_07175), which should not be confused with the surface protective antigen adhesin SpaA of E. rhusiopathiae and which possess an LPXTG motif and are therefore predicted to be membrane-anchored via the sortase already described. Hyaluronate lyases are considered to be a significant virulence factor, especially with regard to spreading in relatively hyaluronic acid-rich tissues such as the skin. Three coding sequences (CDSs) encoding proteins for which a hyaluronate lyase activity was predicted (NBX27_00835, NBX27_03750, & NBX27_06110) were found in the genome of E. rhusiopathiae 319078, representing potentially important factors in the pathogenesis of erysipeloid. Major virulence factors with complex action are bacterial neuraminidases (Soong et al., 2006). Both the E. rhusiopathiae Fujisawa (Ogawa et al., 2011) and E. rhusiopathiae 319078 genomes have two CDSs encoding for proteins for which a neuraminidase function was predicted. One carries the LPXTG motif and therefore should potentially be cell surface associated (NBX27_01575), the second apparently could act potentially as an extracellular enzyme (NBX27_03725).
Inactivation of reactive oxygen species
Other significant virulence-associated factors are those that enable intracellular survival of the bacterium. Bacteria must protect themselves from reactive oxygen species (ROS) after the formation of the phagolysosome. Analysis of the E. rhusiopathiae Fujisawa genome identified 9 genes encoding enzymes with a predicted function indicating that they potentially play a role in the neutralization of ROS: a predicted superoxide dismutase, two predicted thioredoxins, two predicted thioredoxin-disulfide reductases, a predicted thiol peroxidase, a predicted glutaredoxin, and two predicted alkylhydroperoxide reductases (Ogawa et al., 2011). We were able to add three more CDSs to this funcional subgroup (Table 8): a predicted third thioredoxin gene (NBX27_00960), a predicted peptide methionine (S)-S-oxide reductase MsrA (NBX27_00585) that presumably reduces ROS-generated methionine sulfoxide in proteins back to methionine (Weissbach et al., 2002), and a predicted peroxide stress protein YaaA-homologue (NBX27_07905). YaaA was shown to reduce hydrogen peroxide induced damage by decreasing the fraction of intracellular unincorporated iron (Liu et al., 2011).
Phospholipases
Another group of enzymes that play a role in the intracellular life cycle of some bacteria are phospholipases. For example, it has been shown that patatin phospholipases of Rickettsia typhi contribute to open the phagosome or phagolysosome membrane and allow the bacterium to escape into the cytoplasm (Rahman et al., 2013; Smith and May, 2013). More recent studies demonstrated that phospholipases aid in the escape from vacuoles and phagosomes for Listeria monocytogenes, Shigella spp., Plasmodium berghei, Salmonella spp., and Legionella pneumophila (Bianchi and van den Bogaart, 2020; Petrišič et al., 2021; Srivastava and Mishra, 2022). Additionally, it was experimentally proven that Mycobacterium tuberculosis recruits the cytoplasmic phospholipase A2 to permeabilize the endosomal membrane in infected macrophages and to translocate to the cytosol (Jamwal et al., 2016). On the other it was shown that E. rhusiopathiae predominantly replicates in the cytoplasm of macrophages in the spatial vicinity of the entry site (Shimoji et al., 1996; Shimoji, 2000). Therefore, Ogawa and colleagues postulated that phospholipases also play a pivotal role in intracellular translocation of E. rhusiopathiae during phagosome opening. However, experimental evidence of this role remains to be provided for this microbial species. Ogawa and coworkers identified a total of 9 CDSs with homology to phospholipases in the genome of E. rhusiopathiae Fujisawa genome (Ogawa et al., 2011), which we also found in the genome of E. rhusiopathiae 319078 (Table 9).
Further virulence associated factors
One of the predicted virulence-associated factors additionally detected by RAST subsystem analysis in the genome of E. rhusiopathiae 319078 was a homologue to the multiple sugar metabolism regulator, (MsmR, NBX27_01505, Table 10). MsmR, an AraC/XylS type transcriptional regulator, is part of the Streptococcus pyogenes recombinatorial zone. In S. pyogenes this highly recombinatorial zone consists of genes encoding chaperonin, Hsp33; sortase, Spy0135; serum opacity factor, SOF; transcriptional regulator, RofA; negative transcriptional regulator, Nra; fibronectin-binding protein, PrtF; fibronectin-binding protein 2, PrtF2; collagen-binding adhesin, Cpa; multiple sugar metabolism regulator, MsmR; electron transfer flavoprotein 1A, EtfLS; and signal peptidase I, LepL. The gene products include several MSCRAMMs (microbial surface components recognizing adhesive matrix molecules) and play a crucial role in pili-production and mediate adhesion to human cells and tissues (Podbielski et al., 1999; Kreikemeyer et al., 2007). Transcriptome analysis in S. pyogenes serotype M49 showed that the MsmR regulon contains 24 genes under positive MsmR control and 36 genes repressed by MsmR (Nakata et al., 2005). It was demonstrated by electrophoretic mobility shift assay (EMSA) that MsmR binds directly to the promoter regions of the genes encoding fibronectin-binding protein 2 (prtF2), negative regulator of group A Streptococci (nra), collagen-binding protein (cpa), NAD-glycohydrolase (nga), and streptolysin O (slo) (Nakata et al., 2005). In Gram-positive bacteria such as group A streptococci, a cytolysin-mediated translocation (CMT) system replaces the “type III secretion machinery” commonly found in Gram-negative bacteria. Transcription of this CMT system, which plays an important role in host cell interaction, is regulated in particular by MsmR (Madden et al., 2001). However, only a MsmR homologue of this gene cluster is present in the genome of E. rhusiopathiae 319078. Whether a similar role in host cell adherence, internalization, and cytotoxicity exists in E. rhusiopathiae analogous to the role in S. pyogenes remains to be confirmed experimentally. The predicted MsmR was detectable in all of the 10 E. rhusiopathiae genomes deposited at NCBI with 99.84-99.92% sequence identity at a 100% coverage. Therefore, the MsmR homologue appears to be ubiquitous in E. rhusiopathiae. In addition, genes for a predicted type III hemolysin (NBX27_03180) and a predicted hemolysin-related protein (NBX27_02485) with a cystathionine-beta-synthase (CBS) domain exist in the E. rhusiopathiae 319078 genome that could be part of a potential CMT system. Another predicted hemolysin not previously described for E. rhusiopathiae is a homologue of the thermostable hemolysin delta-VPH (NBX27_08265), which was first described in Vibrio parahaemolyticus (Taniguchi et al., 1990).
Conclusions
In summary, we presented a patient with erysipeloid and lymphangitis. The site of entry was probably a minor trauma of the thumb. The source of infection could not be identified with certainty. A body of water in which pigs and the patient’s dog bathed consecutively was considered as potential reservoir. The genome of the E. rhusiopathiae isolate causative for the infection was whole-genome sequenced. A predicted vex23-vncRS locus homologous to the “S. pneumoniae vancomycin tolerance locus” and three predicted MATE family efflux transporters were identified as potential antimicrobial resistance determinants. Several genes encoding proteins with predicted functions that qualify them as potential virulence-associated factors have been identified, including a predicted homologue to the multiple sugar metabolism regulator, MsmR, which cloud play a role in host cell interaction, three predicted hemolysins, two predicted neuraminidases, three predicted hyaluronate lyases, the surface protective antigen adhesin SpaA, a subset of predicted enzymes that potentially play a role in intracellular survival, several predicted two-component signal transduction systems, two dozen predicted surface-associated proteins and a homologue to the capsular polysaccharide synthesis locus.
Data availability statement
The datasets presented in this study can be found in online repositories. The names of the repository/repositories and accession number(s) can be found below: https://www.ncbi.nlm.nih.gov/genbank/, CP098031.
Ethics statement
Ethical review and approval was not required for the study on human participants in accordance with the local legislation and institutional requirements. Written informed consent for participation was not required for this study in accordance with the national legislation and the institutional requirements.
Author contributions
Conceptualization, AK, AZ; methodology, AK, AZ; writing-original draft preparation, AZ, C-JS; writing-review and editing, AT, C-JS, PM, MR, MÐ, AD, DM, AK, AZ; visualization, AZ, MÐ; investigation, MÐ, DM; funding acquisition AK, whole genome sequencing and genome assembly, AT, PM, MR; data curation, annotation, patient treatment, AD; All authors contributed to the article and approved the submitted version.
Funding
The research of the authors was funded by the Deutsche Forschungsgemeinschaft (grant number ZA 697/6-1).
Acknowledgments
We are grateful to Nadja Schlüter, Francis Meier and Sabine Jürgenfeld for excellent technical assistance.
Conflict of interest
The authors declare that the research was conducted in the absence of any commercial or financial relationships that could be construed as a potential conflict of interest.
Publisher’s note
All claims expressed in this article are solely those of the authors and do not necessarily represent those of their affiliated organizations, or those of the publisher, the editors and the reviewers. Any product that may be evaluated in this article, or claim that may be made by its manufacturer, is not guaranteed or endorsed by the publisher.
Supplementary Material
The Supplementary Material for this article can be found online at: https://www.frontiersin.org/articles/10.3389/fcimb.2022.981477/full#supplementary-material
References
Bang, B.-H., Rhee, M.-S., Chang, D.-H., Park, D.-S., Kim, B.-C. (2015). Erysipelothrix larvae sp. nov., isolated from the larval gut of the rhinoceros beetle, Trypoxylus dichotomus (Coleoptera: Scarabaeidae). Antonie Van Leeuwenhoek 107, 443–451. doi: 10.1007/s10482-014-0342-x
Bang, B.-H., Rhee, M.-S., Chang, D.-H., Park, D.-S., Kim, B.-C. (2016). Erratum to: Erysipelothrix larvae sp. nov., isolated from the larval gut of the rhinoceros beetle, Trypoxylus dichotomus (Coleoptera: Scarabaeidae). Antonie Van Leeuwenhoek 109, 167–168. doi: 10.1007/s10482-015-0623-z
Bianchi, F., van den Bogaart, G. (2020). Vacuolar escape of foodborne bacterial pathogens. J. Cell Sci. 134, jcs247221. doi: 10.1242/jcs.247221
Borrathybay, E., Gong, F., Zhang, L., Nazierbieke, W. (2015). Role of surface protective antigen a in the pathogenesis of Erysipelothrix rhusiopathiae strain C43065. J. Microbiol. Biotechnol. 25, 206–216. doi: 10.4014/jmb.1407.07058
Brooke, C. J., Riley, T. V. (1999). Erysipelothrix rhusiopathiae: bacteriology, epidemiology and clinical manifestations of an occupational pathogen. J. Med. Microbiol. 48, 789–799. doi: 10.1099/00222615-48-9-789
Brzóstkowska, M., Raczkowska, A., Brzostek, K. (2012). OmpR, a response regulator of the two-component signal transduction pathway, influences inv gene expression in Yersinia enterocolitica O9. Front. Cell Infect. Microbiol. 2. doi: 10.3389/fcimb.2012.00153
Carroll, K. C., Pfaller, M. A., Landry, M. L., McAdam, A. J., Patel, R., Richter, S. S., et al. (2019). Manual of clinical microbiology. 12. Edition (Washington, DC: ASM Press).
Chen, M., Huang, W., Li, J. (2006). The first report of Erysipelothrix muris sp. nov. Unpublished. Available at: https://www.ncbi.nlm.nih.gov/Taxonomy/Browser/wwwtax.cgi?id=380638.
Drekonja, D. M. (2013). Erysipelothrix bacteremia without endocarditis: rare event or under-reported occurrence? Diagn. Microbiol. Infect. Dis. 77, 280–281. doi: 10.1016/j.diagmicrobio.2013.07.002
Eisenberg, T., Mühldorfer, K., Erhard, M., Fawzy, A., Kehm, S., Ewers, C., et al. (2022). Erysipelothrix anatis sp. nov., erysipelothrix aquatica sp. nov. and Erysipelothrix urinaevulpis sp. nov., three novel species of the genus, and emended description of erysipelothrix. Int. J. Syst. Evol. Microbiol. 72:1–12. doi: 10.1099/ijsem.0.005454
Farfour, E., Leto, J., Barritault, M., Barberis, C., Meyer, J., Dauphin, B., et al. (2012). Evaluation of the andromas matrix-assisted laser desorption ionization–time of flight mass spectrometry system for identification of aerobically growing gram-positive bacilli. J. Clin. Microbiol. 50, 2702–2707. doi: 10.1128/JCM.00368-12
Fidalgo, S. G., Riley, T. V. (2004). Detection of Erysipelothrix rhusiopathiae in clinical and environmental samples. Methods Mol. Biol. 268, 199–205. doi: 10.1385/1-59259-766-1:199
Fidalgo, S. G., Wang, Q., Riley, T. V. (2000). Comparison of methods for detection of Erysipelothrix spp. and their distribution in some Australasian seafoods. Appl. Environ. Microbiol. 66, 2066–2070. doi: 10.1128/AEM.66.5.2066-2070.2000
Forde, T. L., Kollanandi Ratheesh, N., Harvey, W. T., Thomson, J. R., Williamson, S., Biek, R., et al. (2020). Genomic and immunogenic protein diversity of Erysipelothrix rhusiopathiae isolated from pigs in great Britain: Implications for vaccine protection. Front. Microbiol. 11. doi: 10.3389/fmicb.2020.00418
Funke, G. (2009). “Erysipelothrix rhusiopathiae,” in Mikrobiologische diagnostik. bakteriologie, mykologie, virologie, parasitologie (Thieme). Eds. Neumeister, B., Geiss, H. K., Braun, R., Kimmig, P., 368–369. Stuttgart (Germany): Georg Thieme Verlag.
Groeschel, M., Forde, T., Turvey, S., Joffe, A. M., Hui, C., Naidu, P., et al. (2019). An unusual case of Erysipelothrix rhusiopathiae prosthetic joint infection from the Canadian Arctic: whole genome sequencing unable to identify a zoonotic source. BMC Infect. Dis. 19, 282. doi: 10.1186/s12879-019-3913-7
Haas, W., Sublett, J., Kaushal, D., Tuomanen, E. I. (2004). Revising the role of the pneumococcal vex-vncRS locus in vancomycin tolerance. J. Bacteriol. 186, 8463–8471. doi: 10.1128/JB.186.24.8463-8471.2004
Hofseth, K., Dalen, H., Kibsgaard, L., Nebb, S., Kümmel, A., Mehl, A. (2017). Infectious tenosynovitis with bloodstream infection caused by Erysipelothrix rhusiopathiae, a case report on an occupational pathogen. BMC Infect. Dis. 17, 12. doi: 10.1186/s12879-016-2102-1
Hyyryläinen, H. L., Bolhuis, A., Darmon, E., Muukkonen, L., Koski, P., Vitikainen, M., et al. (2001). A novel two-component regulatory system in Bacillus subtilis for the survival of severe secretion stress. Mol. Microbiol. 41, 1159–1172. doi: 10.1046/j.1365-2958.2001.02576.x
Jamwal, S. V., Mehrotra, P., Singh, A., Siddiqui, Z., Basu, A., Rao, K. V. S. (2016). Mycobacterial escape from macrophage phagosomes to the cytoplasm represents an alternate adaptation mechanism. Sci. Rep. 6, 23089. doi: 10.1038/srep23089
Janßen, T., Voss, M., Kühl, M., Semmler, T., Philipp, H.-C., Ewers, C. (2015). A combinational approach of multilocus sequence typing and other molecular typing methods in unravelling the epidemiology of Erysipelothrix rhusiopathiae strains from poultry and mammals. Vet. Res. 46, 84. doi: 10.1186/s13567-015-0216-x
Kreikemeyer, B., Nakata, M., Köller, T., Hildisch, H., Kourakos, V., Standar, K., et al. (2007). The Streptococcus pyogenes serotype M49 nra-Ralp3 transcriptional regulatory network and its control of virulence factor expression from the novel eno ralp3 epf sagA pathogenicity region. Infect. Immun. 75, 5698–5710. doi: 10.1128/IAI.00175-07
Larsen, M. H., Biermann, K., Tandberg, S., Hsu, T., Jacobs, W. R. (2007). Genetic manipulation of Mycobacterium tuberculosis. Curr. Protoc. Microbiol. Chapter 10, Unit 10A.2. doi: 10.1002/9780471729259.mc10a02s6
Liu, Y., Bauer, S. C., Imlay, J. A. (2011). The YaaA protein of the Escherichia coli OxyR regulon lessens hydrogen peroxide toxicity by diminishing the amount of intracellular unincorporated iron. J. Bacteriol. 193, 2186–2196. doi: 10.1128/JB.00001-11
Madden, J. C., Ruiz, N., Caparon, M. (2001). Cytolysin-mediated translocation (CMT): a functional equivalent of type III secretion in gram-positive bacteria. Cell 104, 143–152. doi: 10.1016/s0092-8674(01)00198-2
Mitchell, L. S., Tuomanen, E. I. (2002). Molecular analysis of antibiotic tolerance in pneumococci. Int. J. Med. Microbiol. 292, 75–79. doi: 10.1078/1438-4221-00193
Miyamae, S., Ueda, O., Yoshimura, F., Hwang, J., Tanaka, Y., Nikaido, H. (2001). A MATE family multidrug efflux transporter pumps out fluoroquinolones in Bacteroides thetaiotaomicron. Antimicrobial. Agents Chemother. 45, 3341–3346. doi: 10.1128/AAC.45.12.3341-3346.2001
Morita, Y., Kodama, K., Shiota, S., Mine, T., Kataoka, A., Mizushima, T., et al. (1998). NorM, a putative multidrug efflux protein, of Vibrio parahaemolyticus and its homolog in Escherichia coli. Antimicrob. Agents Chemother. 42, 1778–1782. doi: 10.1128/AAC.42.7.1778
Nagai, S., To, H., Kanda, A. (2008). Differentiation of Erysipelothrix rhusiopathiae strains by nucleotide sequence analysis of a hypervariable region in the spaA gene: discrimination of a live vaccine strain from field isolates. J. Vet. Diagn. Invest. 20, 336–342. doi: 10.1177/104063870802000313
Nakata, M., Podbielski, A., Kreikemeyer, B. (2005). MsmR, a specific positive regulator of the Streptococcus pyogenes FCT pathogenicity region and cytolysin-mediated translocation system genes. Mol. Microbiol. 57, 786–803. doi: 10.1111/j.1365-2958.2005.04730.x
Nelson, R. R. (1999). Intrinsically vancomycin-resistant gram-positive organisms: clinical relevance and implications for infection control. J. Hosp. Infect. 42, 275–282. doi: 10.1053/jhin.1998.0605
Nikolskaya, A. N., Galperin, M. Y. (2002). A novel type of conserved DNA-binding domain in the transcriptional regulators of the AlgR/AgrA/LytR family. Nucleic Acids Res. 30, 2453–2459. doi: 10.1093/nar/30.11.2453
Novak, R., Henriques, B., Charpentier, E., Normark, S., Tuomanen, E. (1999). Emergence of vancomycin tolerance in Streptococcus pneumoniae. Nature 399, 590–593. doi: 10.1038/21202
Ogawa, Y., Ooka, T., Shi, F., Ogura, Y., Nakayama, K., Hayashi, T., et al. (2011). The genome of Erysipelothrix rhusiopathiae, the causative agent of swine erysipelas, reveals new insights into the evolution of firmicutes and the organism’s intracellular adaptations▿. J. Bacteriol. 193, 2959–2971. doi: 10.1128/JB.01500-10
Ogura, M., Ohsawa, T., Tanaka, T. (2008). Identification of the sequences recognized by the Bacillus subtilis response regulator YrkP. Biosci. Biotechnol. Biochem. 72, 186–196. doi: 10.1271/bbb.70548
Opriessnig, T., Forde, T., Shimoji, Y. (2020). Erysipelothrix spp.: Past, present, and future directions in vaccine research. Front. Veterinary Sci. 7. doi: 10.3389/fvets.2020.00174
Pal, N., Bender, J. S., Opriessnig, T. (2010). Rapid detection and differentiation of erysipelothrix spp. by a novel multiplex real-time PCR assay. J. Appl. Microbiol. 108, 1083–1093. doi: 10.1111/j.1365-2672.2009.04560.x
Pehrsson, E. C., Tsukayama, P., Patel, S., Mejía-Bautista, M., Sosa-Soto, G., Navarrete, K. M., et al. (2016). Interconnected microbiomes and resistomes in low-income human habitats. Nature 533, 212–216. doi: 10.1038/nature17672
Petrišič, N., Kozorog, M., Aden, S., Podobnik, M., Anderluh, G. (2021). The molecular mechanisms of listeriolysin O-induced lipid membrane damage. Biochim. Biophys. Acta Biomembr. 1863, 183604. doi: 10.1016/j.bbamem.2021.183604
Podbielski, A., Woischnik, M., Leonard, B. A., Schmidt, K. H. (1999). Characterization of nra, a global negative regulator gene in group a streptococci. Mol. Microbiol. 31, 1051–1064. doi: 10.1046/j.1365-2958.1999.01241.x
Pomaranski, E. K., Griffin, M. J., Camus, A. C., Armwood, A. R., Shelley, J., Waldbieser, G. C., et al. (2020). Description of erysipelothrix piscisicarius sp. nov., an emergent fish pathogen, and assessment of virulence using a tiger barb (Puntigrus tetrazona) infection model. Int. J. Syst. Evol. Microbiol. 70, 857–867. doi: 10.1099/ijsem.0.003838
Principe, L., Bracco, S., Mauri, C., Tonolo, S., Pini, B., Luzzaro, F. (2016). Erysipelothrix rhusiopathiae bacteremia without endocarditis: Rapid identification from positive blood culture by MALDI-TOF mass spectrometry. a case report and literature review. Infect. Dis. Rep. 8, 6368. doi: 10.4081/idr.2016.6368
Rahman, M. S., Gillespie, J. J., Kaur, S. J., Sears, K. T., Ceraul, S. M., Beier-Sexton, M., et al. (2013). Rickettsia typhi possesses phospholipase A2 enzymes that are involved in infection of host cells. PloS Pathog. 9, e1003399. doi: 10.1371/journal.ppat.1003399
Ramirez, N. A., Das, A., Ton-That, H. (2020). New paradigms of pilus assembly mechanisms in gram-positive actinobacteria. Trends Microbiol. 28, 999–1009. doi: 10.1016/j.tim.2020.05.008
Robertson, G. T., Zhao, J., Desai, B. V., Coleman, W. H., Nicas, T. I., Gilmour, R., et al. (2002). Vancomycin tolerance induced by erythromycin but not by loss of vncRS, vex3, or pep27 function in Streptococcus pneumoniae. J. Bacteriol. 184, 6987–7000. doi: 10.1128/JB.184.24.6987-7000.2002
Rostamian, M., Rahmati, D., Akya, A. (2022). Clinical manifestations, associated diseases, diagnosis, and treatment of human infections caused by Erysipelothrix rhusiopathiae: a systematic review. Germs 12, 16–31. doi: 10.18683/germs.2022.1303
Santos-Beneit, F. (2015). The pho regulon: A huge regulatory network in bacteria. Front. Microbiol. 6. doi: 10.3389/fmicb.2015.00402
Selbitz, H.-J., Truyen, U., Valentin-Weigand, P., Alber, G., Amtsberg, G., Bauer, J., et al. (2011). Tiermedizinische mikrobiologie, infektions- und seuchenlehre. 9., vollständig überarbeitete auflage (Thieme Verlag).
Shimoji, Y. (2000). Pathogenicity of Erysipelothrix rhusiopathiae: virulence factors and protective immunity. Microbes Infect. 2, 965–972. doi: 10.1016/S1286-4579(00)00397-X
Shimoji, Y., Shiraiwa, K., Tominaga, H., Nishikawa, S., Eguchi, M., Hikono, H., et al. (2020). Development of a multiplex PCR-based assay for rapid serotyping of Erysipelothrix species. J. Clin. Microbiol. 58, e00315-20. doi: 10.1128/JCM.00315-20
Shimoji, Y., Yokomizo, Y., Mori, Y. (1996). Intracellular survival and replication of Erysipelothrix rhusiopathiae within murine macrophages: Failure of induction of the oxidative burst of macrophages. Infect. Immun. 64, 1789–1793. doi: 10.1128/iai.64.5.1789-1793.1996
Shiraiwa, K., Ogawa, Y., Nishikawa, S., Eguchi, M., Shimoji, Y. (2017). Multiplex PCR assay for the simultaneous detection and differentiation of clonal lineages of Erysipelothrix rhusiopathiae serovar 1a strains currently circulating in Japan. J. Veterinary Med. Sci. 79, 1318–1322. doi: 10.1292/jvms.17-0255
Smith, L. M., May, R. C. (2013). Mechanisms of microbial escape from phagocyte killing. Biochem. Soc. Trans. 41, 475–490. doi: 10.1042/BST20130014
Sommer, M. O. A., Dantas, G., Church, G. M. (2009). Functional characterization of the antibiotic resistance reservoir in the human microflora. Science 325, 1128–1131. doi: 10.1126/science.1176950
Soong, G., Muir, A., Gomez, M. I., Waks, J., Reddy, B., Planet, P., et al. (2006). Bacterial neuraminidase facilitates mucosal infection by participating in biofilm production. J. Clin. Invest. 116, 2297–2305. doi: 10.1172/JCI27920
Souvorov, A., Agarwala, R., Lipman, D. J. (2018). SKESA: strategic k-mer extension for scrupulous assemblies. Genome Biol. 19, 153. doi: 10.1186/s13059-018-1540-z
Spiteri, M., Taylor-Robinson, A. W. (2018). Erysipelothrix rhusiopathiae: An important cause of bacterial disease in farmed pigs and an occupational pathogen of humans. Int. J. Clin. Med. Microbiol. 3: 134. doi: 10.15344/2456-4028/2018/134
Srivastava, P. N., Mishra, S. (2022). Disrupting a plasmodium berghei putative phospholipase impairs efficient egress of merosomes. Int. J. Parasitol. 52, 547–558. doi: 10.1016/j.ijpara.2022.03.002
Takahashi, T., Fujisawa, T., Benno, Y., Tamura, Y., Sawada, T., Suzuki, S., et al. (1987). Erysipelothrix tonsillarum sp. nov. isolated from tonsils of apparently healthy pigs. Int. J. Systematic Evolutionary Microbiol. 37, 166–168. doi: 10.1099/00207713-37-2-166
Takahashi, T., Fujisawa, T., Umeno, A., Kozasa, T., Yamamoto, K., Sawada, T. (2008). A taxonomic study on erysipelothrix by DNA-DNA hybridization experiments with numerous strains isolated from extensive origins. Microbiol. Immunol. 52, 469–478. doi: 10.1111/j.1348-0421.2008.00061.x
Taniguchi, H., Kubomura, S., Hirano, H., Mizue, K., Ogawa, M., Mizuguchi, Y. (1990). Cloning and characterization of a gene encoding a new thermostable hemolysin from Vibrio parahaemolyticus. FEMS Microbiol. Lett. 55, 339–345. doi: 10.1016/0378-1097(90)90020-q
Veraldi, S., Girgenti, V., Dassoni, F., Gianotti, R. (2009). Erysipeloid: a review. Clin. Exp. Dermatol. 34, 859–862. doi: 10.1111/j.1365-2230.2009.03444.x
Verbarg, S., Rheims, H., Emus, S., Frühling, A., Kroppenstedt, R. M., Stackebrandt, E., et al. (2004). Erysipelothrix inopinata sp. nov., isolated in the course of sterile filtration of vegetable peptone broth, and description of Erysipelotrichaceae fam. nov. Int. J. Systematic Evolutionary Microbiol. 54, 221–225. doi: 10.1099/ijs.0.02898-0
Vollmer, W., Blanot, D., De Pedro, M. A. (2008). Peptidoglycan structure and architecture. FEMS Microbiol. Rev. 32, 149–167. doi: 10.1111/j.1574-6976.2007.00094.x
Wang, Q., Chang, B. J., Riley, T. V. (2010). Erysipelothrix rhusiopathiae. Vet. Microbiol. 140, 405–417. doi: 10.1016/j.vetmic.2009.08.012
Wang, T., Khan, D., Mobarakai, N. (2020). Erysipelothrix rhusiopathiae endocarditis. IDCases 22, e00958. doi: 10.1016/j.idcr.2020.e00958
Weissbach, H., Etienne, F., Hoshi, T., Heinemann, S. H., Lowther, W. T., Matthews, B., et al. (2002). Peptide methionine sulfoxide reductase: structure, mechanism of action, and biological function. Arch. Biochem. Biophys. 397, 172–178. doi: 10.1006/abbi.2001.2664
Yamazaki, Y. (2006). A multiplex polymerase chain reaction for discriminating Erysipelothrix rhusiopathiae from Erysipelothrix tonsillarum. J. Vet. Diagn. Invest. 18, 384–387. doi: 10.1177/104063870601800411
Zankari, E., Allesøe, R., Joensen, K. G., Cavaco, L. M., Lund, O., Aarestrup, F. M. (2017). PointFinder: A novel web tool for WGS-based detection of antimicrobial resistance associated with chromosomal point mutations in bacterial pathogens. J. Antimicrobial. Chemother. 72, 2764–2768. doi: 10.1093/jac/dkx217
Zankari, E., Hasman, H., Cosentino, S., Vestergaard, M., Rasmussen, S., Lund, O., et al. (2012). Identification of acquired antimicrobial resistance genes. J. Antimicrobial. Chemother. 67, 2640–2644. doi: 10.1093/jac/dks261
Keywords: Erysipelothrix rhusiopathiae, case report, genome, Vancomycin Resistance, erysipeloid, swine erysipelas, MSMR, vex23-vncRS
Citation: Zautner AE, Tersteegen A, Schiffner C-J, Ðilas M, Marquardt P, Riediger M, Delker AM, Mäde D and Kaasch AJ (2022) Human Erysipelothrix rhusiopathiae infection via bath water – case report and genome announcement. Front. Cell. Infect. Microbiol. 12:981477. doi: 10.3389/fcimb.2022.981477
Received: 29 June 2022; Accepted: 03 October 2022;
Published: 24 October 2022.
Edited by:
Percy Schröttner, Institut für Medizinische Mikrobiologie und Virologie, Universitätsklinikum Carl Gustav Carus, Technische Universität Dresden, GermanyReviewed by:
HanWei Jiao, Southwest University, ChinaAndrew Clark, University of Texas Southwestern Medical Center, United States
Copyright © 2022 Zautner, Tersteegen, Schiffner, Ðilas, Marquardt, Riediger, Delker, Mäde and Kaasch. This is an open-access article distributed under the terms of the Creative Commons Attribution License (CC BY). The use, distribution or reproduction in other forums is permitted, provided the original author(s) and the copyright owner(s) are credited and that the original publication in this journal is cited, in accordance with accepted academic practice. No use, distribution or reproduction is permitted which does not comply with these terms.
*Correspondence: Andreas E. Zautner, YXphdXRuZUBnd2RnLmRl