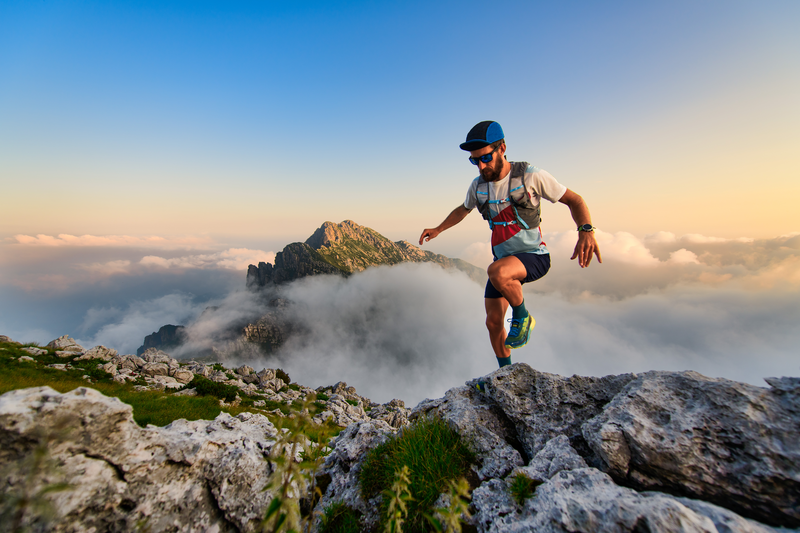
94% of researchers rate our articles as excellent or good
Learn more about the work of our research integrity team to safeguard the quality of each article we publish.
Find out more
ORIGINAL RESEARCH article
Front. Cell. Infect. Microbiol. , 13 September 2022
Sec. Clinical Microbiology
Volume 12 - 2022 | https://doi.org/10.3389/fcimb.2022.979219
Extensively drug-resistant and hypervirulent Klebsiella pneumoniae (XDR-hvKp) is a new problem for patients in Intensive Care Unit (ICU) and can become an even more severe threat if resistant to tigecycline, considered one of the ‘last lines of defense’ drugs. This study collected seven non-replicated tigecycline-resistant XDR-hvKp from seven patients and performed genome analysis and epidemiological investigation using whole genome equencing (WGS) and other methods. All strains in this study were identified as ST11-KL64 and showed high resistance to antibiotics such as β-lactams, aminoglycosides, quinolones, and tigecycline, and one strain was also resistant to colistin. All strains were determined to be hvKp by the results of serum resistance assay and Galleria mellonella infection models. All strains had resistance genes blaCTX-M-65,blaKPC-2,blaLAP-2,blaTEM-1B, rmtB, and qnrS1 and virulence factors such as rmpA, rmpA2, and aerobactin (iucABCD, iutA). The expression of the AcrAB-TolC efflux pump was upregulated in all strains, and the expression levels of the gene pmrK was significantly upregulated in colistin-resistant strain DP compared to colistin-sensitive strain WT in this study. In conclusion, we described an outbreak caused by tigecycline-resistant XDR-hvKp in the ICU of a teaching hospital in southwest China. The spread of these superbugs poses a great threat to patients and therefore requires us to closely monitor these XDR-hvKp and develop relevant strategies to combat them.
Klebsiella pneumoniae (K. pneumoniae) is one of the most common opportunistic pathogens in hospitals, which causes pneumonia, sepsis, urinary tract infections, and other life-threatening diseases (Bengoechea and Sa Pessoa, 2019). At the same time, K. pneumoniae has attracted growing attention due to its rapid drug resistance and virulence evolution (Chang et al., 2021).
Classical K. pneumoniae (cKp) and hvKp are commonly divided into two pathogenic types for research based on their virulence, with hvKp being more virulent than cKp (Russo and Marr, 2019). HvKp can infect healthy individuals of any age, and it can cause hepatic abscesses in the absence of biliary tract infection or other symptoms; and can metastatically spread to multiple sites of the body, causing severe invasive infections were firstly reported in Taiwan and now prevalent mainly in the Asian Pacific Rim (Liu et al., 1986; Russo and Marr, 2019).The main virulence factors affecting hvKp are rmpA, rmpA2, aerobactin (iucABCD, iutA), salmochelin (iroBCDNE), rmpC, rmpD, and peg344. Briefly, rmpA, rmpA2 regulate capsular polysaccharide (CPS) synthesis and mucoid phenotype; the siderophores aerobactin (iucABCD, iutA) and salmochelin (iroBCDNE) play an important role in infection; rmpC, rmpD and peg344 are involved in virulence enhancement (Choby et al., 2020). Lately, some studies have found that mutations in the capsule-biosynthesis genes can also affect strains’ virulence (Ernst et al., 2020a; Morales-León et al., 2021).
HvKp can acquire antibiotic resistance by acquiring mobile genetic elements carrying antibiotic resistance genes or mutations in chromosomal genes under antibiotic pressure (Lee et al., 2017; Russo and Marr, 2019). At the same time multidrug-resistant (MDR) K. pneumoniae can enhance virulence by acquiring virulence or hybrid plasmids mediating multidrug-resistance and hypervirulence (Magiorakos et al., 2012; Lan et al., 2021). These conditions led to the emergence of carbapenem-resistant hypervirulent K. pneumoniae (CR-hvKp) which has both hypervirulent and multidrug-resistant phenotypes (Gu et al., 2018).
Colistin and tigecycline are among the few antibiotics treatments for carbapenem-resistant K. pneumoniae (CRKP) infections (Sheu et al., 2019). Unfortunately, pathogens can resist colistin and tigecycline through alterations of mgrB, mutations in the two-component regulatory systems (pmrAB and phoPQ), or disruption of regulatory genes encoding the efflux pumps (e.g., ramR, ramA, and rarA) (Cannatelli et al., 2014; Galani et al., 2021). If XDR-hvKp, poses a great threat to the patients in ICU (Xiong et al., 2021), were to become resistant to tigecycline, this would have even more severe consequences. We found that very little information is available on tigecycline-resistant CR-hvKp, and our search in PubMed resulted in only several papers about tigecycline-resistant CR-hvKp (Huang et al., 2018; Chen et al., 2021; Jin et al., 2021; Zhang et al., 2021). Hence, we need to keep an eye on these “superbugs”.
In this study, an outbreak of tigecycline-resistant XDR-hvKp in an ICU of a teaching hospital in southwest China, was investigated to elucidate the antibiotic resistance mechanisms and virulence factors of the strains, explore the evolution and transmission of XDR-hvKp in the clinical environment, and provide valuable information for monitoring and controlling these “superbugs”.
In February 2022, an outbreak of K. pneumoniae occurred in the ICU of a teaching hospital in southwest China. The antibiotic resistance mechanisms, virulence factors, and genetic correlates of strains isolated from seven non-replicated strains of K. pneumoniae obtained clinical specimens from seven patients from January 26, 2022, to February 24, 2022, were included in this study. The strains were identified by Matrix-assisted laser desorption/ionization-time of flight (MALDI-TOF) mass spectrometry (MS) (Bruker, Germany). The patient’s electronic medical record collected information on the patient’s gender, age, admission, diagnosis, antimicrobial therapy, and treatment outcome.
The Minimal inhibitory concentrations (MIC) of amikacin, gentamicin, cefepime, ceftazidime-avibactam, chloramphenicol, imipenem, meropenem, tetracycline, tigecycline, and colistin were determined using the microbroth dilution method. The susceptibility of the strains to other antibiotics was measured using the MicroScan Walkaway-96 system (Siemens, West Sacramento, CA, USA). Pseudomonas aeruginosa ATCC 27853 and E. coli ATCC 25922 were used as quality control strains. The strains’ susceptibility to tigecycline was interpreted according to the breakpoints set by the U.S. Food and Drug Administration (FDA) (≤2 mg/L for sensitive, 4 mg/L for intermediate, and ≥8 mg/L for resistant), and the remaining antibiotic results were interpreted according to the 2022 Clinical and Laboratory Standards Association (CLSI-M100-2022) guidelines (Pillar et al., 2008).
The strains were transferred to blood agar plates and incubated overnight. The colonies were touched with an inoculation loop and stretched outward, and the length of the sticky filaments pulled out was measured. A length larger than 5 mm was defined as a positive result, and the above operation was repeated three times for each strain (Yang et al., 2022).
The assay was performed according to the published experiment (Hughes et al., 1982; Podschun et al., 1993). Briefly, 25ul of a bacterial suspension at a concentration of 1.5×106 CFU/mL was mixed with 75ul of healthy human serum and the mixture was incubated at 35°C. The baseline and the 1, 2 and 3 hour mixtures were diluted and inoculated on nutrient agar plates overnight. The number of colonies at each timepoint was counted, and the strains were graded (1, 2 for “highly sensitive”; 3, 4 for “moderately sensitive”; 5, 6 for “resistant”) as specified in the experimental method (Hughes et al., 1982; Podschun et al., 1993), depending on the results. Survival curves for serosensitivity were made according to the survival rate at each timepoint.
The experiments were performed according to a previously published method (Insua et al., 2013). Briefly, a suspension of 1×108 CFU/mL was prepared from PBS-washed overnight cultures, and the suspension was diluted in a gradient to obtain a series of concentrations from 108 to 105. Ten microliters of suspension were injected into the Galleria mellonella larvae using a Hamilton syringe with a 30-gauge needle (15 biological replicates for each concentration). The larvaes were placed in the dark at 37°C with food and observed every 12 hours for three days and the number of surviving larvae was recorded. The experimental data were used to calculate the lethal dose 50 (LD50) according to the formula of Reed and Muench, and the results are expressed as log10 LD50 (Thakur and Fezio, 1981).
The hypervirulent K. pneumoniae NTUH-K2044 and the low-virulence ATCC 700603 were used as comparators. Both of the above experiments were repeated independently in triplicate.
PFGE experiments were performed as described in the previous report (Han et al., 2013). Briefly, the strains in this study were inoculated on nutrient agar plates and incubated overnight at 37°C. We first digested bacterial suspensions (turbidity of 3.8-4.2) with proteinase K (TAKARA, Beijing) and then added agarose to obtain DNA gel blocks. Subsequently, slices of DNA gel blocks were digested with the restriction enzyme Xba I (TAKARA, Beijing). We performed electrophoresis at a voltage of 6 v/cm, with pulse parameters of 6–36s for 19 hours. We analyzed the results using BioNumerics (Version8.1, Applied maths, Inc.).
Extraction and purification of total DNA from isolates incubated overnight in LB broth to logarithmic growth using the MagPure Bacterial DNA KF Kit (Magen). Quantification of total DNA was completed using the Qubit™ dsDNA HS Assay Kit (ThermoFisher) and Hieff NGS™ DNA Selection Beads (Shanghai). Library construction was completed using the NEB Next® Ultra™ DNA Library Prep Kit for Illumina® (NEB), followed by sequencing via the Illumina (NovaSeq 6000,USA) platform. Quality assessment of sequencing data was performed using FastQC (v 0.11.2), and validated data was obtained by trimming the sequencing data with Trimmomatic (v 0.36).
The Virulence Factor Database (VFDB) (http://www.mgc.ac.cn/VFs/) and BIGSdb Pasteur database (http://bigsdb.pasteur.fr) were used to obtain strains’ capsular serotypes and virulence genes. The IS finder (https://www-is.biotoul.fr/blast.php) was used to identify the type of insertion sequence (IS). The sequencing data were uploaded to the Center for Genomic Epidemiology (http://www.genomicepidemiology.org/) to obtain multilocus sequence typing (MLST), resistance genes, pore proteins, and plasmid replicon types for the strains. The genomes were compared using the Basic Local Alignment Search Tool (BLAST, https://blast.ncbi.nlm.nih.gov/Blast.cgi) and OAT software. A phylogenetic tree was constructed based on the Orthologous average nucleotide identity (OrthoANI) between the genomes.
Chromosomal mutations were identified by comparison with K. pneumoniae ATCC 13883 (accession number: JOOW01). K. pneumoniae genome (accession numbers LT174540 and JCMB01, respectively) was also used as a reference to identify mutations in the rcsAB and lon protease genes.
The strains were mixed as donors with the recipient bacteria (sodium azide-resistant E. coli J53) in LB broth in a 2:1 ratio and the mixture was incubated at 36°C for 24 hours. The transconjugants were screened on nutrient agar plates containing sodium azide (180 mg/L) and meropenem (4 mg/L) (Borgia et al., 2012). Transformants with blaKPC (Supplementary Table 1) and resistance to meropenem were defined as transduction conjugates.
In the presence of efflux pump inhibitor 1-(1-naphthylmethyl)-piperazine (NMP, 100mg/L), we determined the MIC of all strains to tigecycline. If the MIC value drops by a factor of 4 or more in the presence of epi, the efflux pump of the strain is significantly inhibited (Deng et al., 2014).
Quantitative real-time PCR (RT-qPCR) was used to assess the expression levels of the ramA, marA, soxS, acrA, acrB, acrR, phoP, phoQ, pmrD, pmrA, pmrB, pmrC, and pmrK genes, using the primers in Supplementary Table 1. In brief, we extracted the RNA of strains in this study using the EasyPure RNA Kit (TransGen Biotech, Beijing, China). We used TransScript® All-in-One First-Strand cDNA Synthesis SuperMix for qPCR (TransGen Biotech, Beijing, China) for reverse transcription. We performed the RT-qPCR assays using PerfectStart® Green qPCR SuperMix (TransGen Biotech, Beijing, China). The 16S rRNA gene was used as an internal standard (Yang Y. et al., 2021), K. pneumoniae ATCC 13883 (expression = 1) was used as control (2-ΔΔCT method). All experiments were performed for three replicates.
Seven non-replicated pathogens were isolated from the sputum of seven patients from December 26, 2021, to February 24, 2022, and identified as K. pneumoniae by MALDI-TOF MS. All patients had received invasive procedures (including tracheal intubation, catheter drainage, surgery, and puncture), and some had underlying diseases, such as tumors, hepatic impairment, and diabetes mellitus (Table 1). All patients had severe respiratory symptoms such as pulmonary infections. The first strain was isolated from the sputum specimen from patient WT on January 26, 2022. Patient WT was hospitalized in ICU on January 22, 2022, transferred to the Respiratory ICU on January 26, and was still under treatment in critical condition as of March 25. The last isolate was collected from the sputum of patient YF on February 23, 2022. A total of seven non-replicated strains from seven patients were obtained in this study, and all strains were isolated for the first time from each patient. As of March 25, 2022, among the seven patients, two had died, three abandoned treatment and were discharged from the hospital, and two were still undergoing treatment (Figure 1). Hospital workers took infection control measures promptly, so the outbreak was effectively controlled. Apart from these seven patients, no other K. pneumoniae-infected patients were found in the ICU and other departments for the time being. The follow-up epidemiological investigation is still in progress. The detailed molecular epidemiological investigation is described below.
Figure 1 Epidemiology of seven patients in this study. Each grey broadband represents the timeline of one patient, the figure indicates the date of transfer of the patient to the new ward and the date of isolation of the strain as well as the date of the patient’s first use of this type of antibiotic in this investigation. The outcome of each patient is represented at the end of each grey broadband. The names of patients/strains are next to the pink broadband.
The results showed that all seven strains were resistant to β-lactams, quinolones, aminoglycosides, tetracyclines, sulfonamides, nitrofurantoin, and fosfomycin with almost identical MICs (Table 2). All strains were defined as XDR based on the drug sensitivity results. Notably, all six of the seven strains were sensitive to colistin, except for strain DP, which was resistant to colistin (MIC = 4 mg/L). All strains were sensitive to chloramphenicol and resistant to tigecycline with the same MIC (MIC = 8 mg/L), and no strain was found to be resistant to ceftazidime-avibactam.
All strains could form a viscous filament larger than 5 mm. The serum resistance of strain WT was highly sensitive, whereas the other strains in this study were all resistant to serum (Figure 2B). The Galleria mellonella infection model showed an LD50 of 3.87 ± 0.17 (Mean ± SE) for K. pneumoniae NTHU-K2044 (Supplementary Table 2), which has no significant difference compared to the seven strains in this study (Supplementary Figure 1).
Figure 2 Virulence phenotypes of seven XDR-hvKp strains. (A) Survival curves of the Galleria mellonella larvae. (B) Activity of strains in serum from healthy human. The data are expressed as the Mean ± SE (standard error).In (A, B), K. pneumoniae NTUH-K2044 and ATCC 700603 were used as positive control and negative control, respectively.
The MLST of the seven non-replicated K. pneumoniae strains in this study was ST11, and the capsular serotype of all strains was KL64. The result of PFGE showed no difference in the bands of all strains (Supplementary Figure 2).The phylogenetic tree constructed by ANI showed that all strains were highly homologous (Figure 3). WGS analysis showed that all strains had blaCTX-M-65, blaKPC-2, blaLAP-2, blaTEM-1B (β-lactam resistance genes), rmtB (aminoglycoside resistance gene), fosA3 (fosfomycin resistance gene), qnrS1 (quinolone resistance gene), sul2 (sulphonamide resistance gene), dfrA14 (trimethoprim resistance gene), tet(A) (tetracycline resistance gene), ompk35, ompk37, ompk36 genes. Comparison with the sequence of K. pneumoniae ATCC 13883 (accession number: JOOW01) revealed that mutations in gyrA(Asp87Gly, Ser83Ile), parC(Ser80Ile) in the quinolone resistance determining region (QRDR) and fosA (Ile91Val) associated with fosfomycin resistance were present in all strains. We analyzed the gene sequences associated with tigecycline resistance including tet(A), ramR, ramA, acrR, acrA, acrB, acrD, marA, marR, rarA, robA, soxR, soxS, rpsj, tolc, and lon. Comparison with K. pneumoniae ATCC 13883 revealed a mutation of stop194Lys in the ramR gene. And we also found that the acrR of all strains was truncated by the insertion of ISKpn26 (Figure 4C). Comparison with Escherichia coli plasmid RP1 tetracycline resistance determinants (GenBank accession number X00006) revealed the mutations of type 1 (Ile5Arg, Val55Met, Ile75Val, Thr84Ala, Ser201Ala, Phe202Ser, and Val203Phe), and Ala370Val in tet(A) in all strains. No other mutations were detected on other genes, and we did not find the genes like tet(X) or tmexCD1-toprJ-family in this study. We also analyzed the genes pmrA, pmrB, pmrC, pmrD, phoP, phoQ, crrA, crrB, mgrB, and mcr, associated with colistin resistance. The results showed that pmrB mutations such as Arg256Gly and Thr246Ala were present on all strains, but only one amino acid substitution was detected on pmrB (Thr157Pro) of the colistin-resistant strain DP, which differed from the other six strains (Supplementary Table 3). No other mutations were detected in the above genes, no insertions were detected on mgrB, and we also did not find the mcr-family genes.
Figure 3 Phylogenetic tree of seven XDR-hvkp and K. pneumoniae ATCC 13883 based on OrthoANI values; K. pneumoniae ATCC 13883 was used as a control. The value on the branch, not the length of the branch in the graph, represents the real physical length of the branch.
Figure 4 (A) Comparison of sequences surrounding blaKPC-2 between strains in this study and strain PKPHS2 (CP003224). (B) Comparison of sequences related to virulence among strains in this study, Pkp12-vir (CP082766), and NTUH-K2044 (AP006726). (C) Schematic representation of the insertion of the acrR gene by ISkpn26. In (A–C), as all strains in this study showed almost identical results to those of the studies mentioned above, we show only a graphical representation of one of the seven strains at random. Grey shading indicates >99% identity between sequences.
All strains carried the virulence factors rmpA, rmpA2, rmpC, rmpD, aerobactin (iucABCD, iutA), entsiderophore, iroE, yersiniabactin. Besides, they also carried type III fimbriae, type I fimbriae, type IV pili and type VI secretion systems (T6SSs) related to colonization, adhesion, and microbial antagonism. No strains carried iroBCD, but all strains carried iroN truncated by ISKpn26. Comparison with the genome of the K. pneumoniae (accession numbers LT174540 and JCMB01, respectively) revealed the presence of an amino acid substitution in rcsA (ser35Asn) in all strains, and no mutation was detected in the lon protease gene. Notably, the remaining six strains in this study had a shift mutation in the wzc region on the CPS gene cluster (Asn724Lys, Asn725Stop) compared to strain WT, which resulted in premature transcriptional termination.
For the gene environment of blaKPC-2, blaKPC-2 and the insertion sequence around blaKPC-2 together form a ISKpn27- blaKPC-2- ISKpn6 structure, but no TN4401 was found. Comparison with the NCBI data showed that blaKPC-2 and insertions upstream and downstream of blaKPC-2 in all strains formed the non-Tn4401 element (NTEKPC-Ib) (Figure 4A). For the gene environment of rmpA, rmpC and rmpD and peg-344 were surrounding rmpA, and there are two ISKpn26 at positions upstream of rmpA and downstream of peg-344 respectively, iroN was truncated due to the insertion of ISKpn26 (Figure 4B). This region also had the same structure as the reported strain Pkp12-vir (GenBank accession number CP082766) by comparison with the NCBI data.
In the presence of NMP, the MIC of all strains for tigecycline decreased to 0.5 mg/L (Table 1). The results showed that NMP reversed the resistance of all strains to tigecycline in this study. Using K. pneumoniae ATCC 13883 as the control (expression = 1), RT-qPCR results showed that the expression of acrA, acrB, and ramA genes were significantly upregulated in all strains, acrR gene’s expression was significantly down-regulated, while the expression levels of soxS and marA were not statistically different compared to the control strain (Table 3). Expression levels of pmrA, pmrB, pmrC, and pmrK genes were significantly upregulated in colistin-resistant strain DP compared to colistin-sensitive strain WT, while the expression levels of phoP, phoQ, and pmrD did not show significant differences between strain WT and strain DP (Figure 5).
Figure 5 Comparison between the relative expression levels of the phoP, phoQ, pmrC, pmrA, pmrB, pmrK, and pmrD genes in strain DP and strain WT (Relative expression compared with K. pneumoniae ATCC13883, expression = 1). Values of relative expression are expressed as means and the standard deviation from experiments which were repeated in triplicate independently. *P<0.05; **P<0.01; ***P<0.001 by two-tailed unpaired t test; ns, no significance by two-tailed unpaired t test.
According to the results obtained from the Center for Genomic Epidemiology, all strains carried plasmids of the same plasmid type, IncFII, IncR, repB, ColRNAI, and IncHI1B (Supplementary Table 3). In this study, the plasmids of the tested strains were not transferred to E. coli J53 after several experiments.
Our study reported an outbreak of XDR-hvKp, which to our knowledge was the first report of an outbreak of XDR-hvKp in a hospital ICU in southwest China. The seven non-replicated strains isolated from the sputum of seven patients showed highly similar drug resistance (Table 2) and virulence phenotypes (Figures 2A, B), which, when combined with the results of PFGE (Supplementary Figure 2) and phylogenetic tree (Figure 3), suggested that the seven strains were closely related (Tenover et al., 1995; Jain et al., 2018). According to the chronological order of the patient’s ward and the patient’s infection, patient WT was in the ICU for only three days; therefore, the patient who may have cross-infected with patient WT during these three days was either patient FY or patient DP (Figure 1). Therefore, we speculated that strain WT might have been transmitted to patient FY or DP. Then strain FY or strain DP was transferred in an unknown manner to the remaining patients in this study. Another possibility is that strain FY or strain DP was transferred to patient WT and the rest of the patients in this study, and that patient WT left the ICU with strain WT to the respiratory ICU. However, the cause and the route of transmission of the outbreak in this study were still unknown and investigations were still ongoing.
The MLST of all strains in this study was ST11, the dominant clonal group of CRKP commonly found in China, and ST11 CRKP is also a high-risk clonal group with the ability to obtain hypervirulence-encoding plasmids (Liao et al., 2020). Recent studies had shown that ST11-KL64 was one of the most common types of ST11, the dominant clonal group of CR-hvKp in China, and was gradually replacing KL47. Furthermore, ST11-K64 CR-hvKp exhibited worrisome virulence and environmental survival rates (Liao et al., 2020; Zhou et al., 2020).
In terms of drug resistance of all strains, the patterns of antibiotic resistance in seven XDR strains were almost identical (Supplementary Table 3). The genes blaCTX-M-65, blaKPC-2, blaLAP-2, and blaTEM-1B provide strains with β-lactams resistance (Galani et al., 2021). Among these genes blaKPC-2 was one of the most common carbapenemase genes in ST11 CRKP in China (Liao et al., 2020). The NTEKPC-Ib carried by all strains in this study (Figure 4A) was consistent with the NTEKPC possessed by strain PKPHS2 (CP003224) as previously reported (Chen et al., 2014), a mobile element that, like Tn4401, could play an important role in the transmission of blaKPC, more common in China and Brazil than Tn4401, the most prevalent in Europe and the United States (Yang X et al., 2021). None of the blaKPC-bearing plasmids from all strains in this study was successfully transferred into E. coli J53, which may be related to the host range and growth conditions of the strains, but did not exclude that the plasmids in this study could be transferred under other circumstances (Carattoli, 2009). In addition, mutations on fosA (Ile91Val) combined with the presence of fosA3 in all strains could explain the resistance to Fosfomycin (Ito et al., 2017; Wang et al., 2022). The strains in this study were resistant to quinolones, caused by mutations in gyrA (Asp87Gly, Ser83Ile) and parC (Ser80Ile) of QRDR. All strains carried qnrS1 which reduces susceptibility to quinolones (Fàbrega et al., 2009). All strains also carried rmtB, a common 16S rRNA methyltransferases (16S-RMTases) in Enterobacteriaceae, which could lead to a high-level of resistance to aminoglycosides, one of the therapeutic tools for CRKP (Doi et al., 2016). Unfortunately, tigecycline, one of the last resort treatments for XDR strains, was ineffective against all strains in this study (Table 1). Mutations in tet(A) (type 1 and Ala370Val) had all been shown to reduce the susceptibility of the strains to tigecycline (Chiu et al., 2017; Xu et al., 2021). Moreover, the acrR of the strains was also inserted by ISKpn26 (Figure 4C), which has been previously reported to activate the AcrAB-TolC efflux pump and reduce the strain’s susceptibility to tigecycline (Yang Y. et al., 2021). NMP can effectively inhibit the activity of the AcrAB-TolC efflux pump (Schuster et al., 2014). So, combining the results of RT-qPCR (Table 3) and efflux pump activity assays (Table 2), we can infer that the combination of ramA overexpression and the truncation of acrR by ISKpn26, which resulted in the upregulation of AcrAB-TolC efflux pump, was the main cause of resistance to tigecycline in all strains in this study (Chiu et al., 2017). Incidentally, the mechanism of tigecycline resistance in all strains resulted from the accumulation of multiple mutations and was consistent, indirectly indicating a high degree of genetic relatedness between all strains. Notably, strain DP in this study was resistant to colistin, while the other strains were not; therefore, we speculate that the factor causing this phenomenon may be the development of resistance under the selective pressure of colistin (Huang et al., 2021). The strain DP showed amino acid substitutions in pmrB (Thr157Pro) that were not present in the remaining six strains. According to a previous study, Thr157Pro in pmrB could cause overexpression of pmrCAB and pmrHFIJKLM operons, resulting in colistin resistance (Jayol et al., 2014), which is consistent with the situation in this study (Figure 5). In addition, all strains had amino acid substitutions in pmrB (Arg256Gly and Thr246Ala) and although a previous study had shown that such mutations did not cause strains to be resistant to colistin, their effects might differ by genetic backgrounds, so more research is needed to demonstrate whether these mutations have other unknown promotive effects on the strains’ resistance to colistin (Cheng et al., 2015; Aires et al., 2016). Fortunately, all strains were susceptible to ceftazidime-avibactam (Table 2), and avibactam is a β-lactamase inhibitor that allows ceftazidime to retain its antibacterial activity in Klebsiella pneumoniae carbapenemase-producing Enterobacteriaceae by inhibiting Ambler class A β-lactamases so that ceftazidime-avibactam could be an option for treatment against the pathogens identified in this study (Zasowski et al., 2015).
In terms of virulence factors, all strains possess rmpA, rmpA2, rmpC, and rmpD capable of regulating the hypermucoviscosity (HMV) phenotype and CPS synthesis, which could explain the HMV of all strains (Wacharotayankun et al., 1993; Russo and Marr, 2019; Walker et al., 2020). The isolates in this study all possessed the siderophore genes aerobactin (iucABCD, iutA) and iroE, which help the strains to acquire iron in the human host (Choby et al., 2020), and peg-344, an inner membrane transporter whose role in virulence is not yet clear (Bulger et al., 2017). Besides, aerobactin plays a decisive role in the virulence of hvKp, and peg-344, iucA, rmpA, and rmpA2 as markers to identify hvKp has been shown to have high accuracy (Russo et al., 2015; Russo et al., 2018). Therefore, based on the LD50, which was not statistically different from NTUH-K2044, and the virulence factors described above, it can be judged that all strains in this study were hvKp (Choby et al., 2020). In addition, the loss of iroBCD might be due to the insertion of ISKpn26, which has been similarly reported previously (Kong et al., 2021). The amino acid substitution in rcsA (ser35Asn) of all strains was identical to the mutation reported in a Chilean’s study, such a condition could affect the synthesis of CPS, thereby enhancing the virulence of the strains (Morales-León et al., 2021). We also observed that the serum resistance of strain WT was different from other strains (Figure 2B). Previous studies had shown that mutations in the wzc gene affect the virulence of strains, so we hypothesized that mutations in the wzc of strain WT are responsible for the difference in serum resistance of strain WT from other strains, but further studies are needed to confirm this (Ernst et al., 2020b).
In conclusion, the seven tigecycline-resistant XDR-hvKp strains in this study showed worrying results regarding antibiotic resistance and virulence. We used WGS techniques to reveal the virulence and antibiotic resistance mechanisms of the strains in this study and to explore possible mechanisms of microevolution of the different strains and possible routes of transmission of the strains between patients in this outbreak. Our study provided a reliable basis for the developing infection control and prevention measures in the hospital and valuable information for clinical management. Our results also suggested the need for continued close surveillance of these superbugs and the need for healthcare workers to practice strict hand hygiene to prevent such events from occurring (Vermeil et al., 2019).
The datasets presented in this study can be found in online repositories. The names of the repository/repositories and accession number(s) can be found in the article/Supplementary Material.
JL and ZZ conceived of and designed the study. SL, YX, and YD wrote this paper and contributed equally to this work. SL, YX, YD, and ZL performed the experiments. JL, ZZ, SL, and YX analyzed the data. All authors contributed to the article and approved the submitted version.
This work was supported by the Science and Technology Project of Science & Technology Department of Sichuan Province under Grant [No. 2021YFS0329, No. 2022YFQ0093], Luxian and Southwest Medical University Cultivation Project [No. 2020LXXNYKD 04].
We thank the curators of the Institute Pasteur MLST system (Paris, France) for the information about novel alleles, profiles, and/or isolates available at http://bigsdb.web.pasteur.fr. We thank the entire team of curators of GenBank databases for curating the data and making it publicly available at https://www.ncbi.nlm.nih.gov/.
The authors declare that the research was conducted in the absence of any commercial or financial relationships that could be construed as a potential conflict of interest.
All claims expressed in this article are solely those of the authors and do not necessarily represent those of their affiliated organizations, or those of the publisher, the editors and the reviewers. Any product that may be evaluated in this article, or claim that may be made by its manufacturer, is not guaranteed or endorsed by the publisher.
The Supplementary Material for this article can be found online at: https://www.frontiersin.org/articles/10.3389/fcimb.2022.979219/full#supplementary-material
Aires, C. A., Pereira, P. S., Asensi, M. D., Carvalho-Assef, A. P. (2016). mgrB mutations mediating polymyxin b resistance in klebsiella pneumoniae isolates from rectal surveillance swabs in Brazil. Antimicrob. Agents Chemother. 60, 6969–6972. doi: 10.1128/AAC.01456-16
Bengoechea, J. A., Sa Pessoa, J. (2019). Klebsiella pneumoniae infection biology: living to counteract host defences. FEMS Microbiol. Rev. 43, 123–144. doi: 10.1093/femsre/fuy043
Borgia, S., Lastovetska, O., Richardson, D., Eshaghi, A., Xiong, J., Chung, C., et al. (2012). Outbreak of carbapenem-resistant enterobacteriaceae containing blaNDM-1, Ontario, Canada. Clin. Infect. Dis. 55, e109–e117. doi: 10.1093/cid/cis737
Bulger, J., Macdonald, U., Olson, R., Beanan, J., Russo, T. A. (2017). Metabolite transporter PEG344 is required for full virulence of hypervirulent klebsiella pneumoniae strain hvKP1 after pulmonary but not subcutaneous challenge. Infect. Immun. 85, e00093–17. doi: 10.1128/IAI.00093-17
Cannatelli, A., Giani, T., D’andrea, M. M., Di Pilato, V., Arena, F., Conte, V., et al. (2014). MgrB inactivation is a common mechanism of colistin resistance in KPC-producing klebsiella pneumoniae of clinical origin. Antimicrob. Agents Chemother. 58, 5696–5703. doi: 10.1128/AAC.03110-14
Carattoli, A. (2009). Resistance plasmid families in enterobacteriaceae. Antimicrob. Agents Chemother. 53, 2227–2238. doi: 10.1128/AAC.01707-08
Chang, D., Sharma, L., Dela Cruz, C. S., Zhang, D. (2021). Clinical epidemiology, risk factors, and control strategies of klebsiella pneumoniae infection. Front. Microbiol. 12, 750662. doi: 10.3389/fmicb.2021.750662
Cheng, Y. H., Lin, T. L., Pan, Y. J., Wang, Y. P., Lin, Y. T., Wang, J. T. (2015). Colistin resistance mechanisms in klebsiella pneumoniae strains from Taiwan. Antimicrob. Agents Chemother. 59, 2909–2913. doi: 10.1128/AAC.04763-14
Chen, L., Mathema, B., Chavda, K. D., Deleo, F. R., Bonomo, R. A., Kreiswirth, B. N. (2014). Carbapenemase-producing klebsiella pneumoniae: molecular and genetic decoding. Trends Microbiol. 22, 686–696. doi: 10.1016/j.tim.2014.09.003
Chen, J., Zeng, Y., Zhang, R., Cai, J. (2021). In vivo emergence of colistin and tigecycline resistance in carbapenem-resistant hypervirulent klebsiella pneumoniae during antibiotics treatment. Front. Microbiol. 12, 702956. doi: 10.3389/fmicb.2021.702956
Chiu, S. K., Huang, L. Y., Chen, H., Tsai, Y. K., Liou, C. H., Lin, J. C., et al. (2017). Roles of ramR and tet(A) mutations in conferring tigecycline resistance in carbapenem-resistant klebsiella pneumoniae clinical isolates. Antimicrob. Agents Chemother. 61, e00391–17. doi: 10.1128/AAC.00391-17
Choby, J. E., Howard-Anderson, J., Weiss, D. S. (2020). Hypervirulent klebsiella pneumoniae - clinical and molecular perspectives. J. Intern. Med. 287, 283–300. doi: 10.1111/joim.13007
Deng, M., Zhu, M. H., Li, J. J., Bi, S., Sheng, Z. K., Hu, F. S., et al. (2014). Molecular epidemiology and mechanisms of tigecycline resistance in clinical isolates of acinetobacter baumannii from a Chinese university hospital. Antimicrob. Agents Chemother. 58, 297–303. doi: 10.1128/AAC.01727-13
Doi, Y., Wachino, J. I., Arakawa, Y. (2016). Aminoglycoside resistance: The emergence of acquired 16S ribosomal RNA methyltransferases. Infect. Dis. Clin. North Am. 30, 523–537. doi: 10.1016/j.idc.2016.02.011
Ernst, C. M., Braxton, J. R., Rodriguez-Osorio, C. A., Zagieboylo, A. P., Li, L., Pironti, A., et al. (2020a). Adaptive evolution of virulence and persistence in carbapenem-resistant klebsiella pneumoniae. Nat. Med. 26, 705–711. doi: 10.1038/s41591-020-0825-4
Ernst, C. M., Braxton, J. R., Rodriguez-Osorio, C. A., Zagieboylo, A. P., Li, L., Pironti, A., et al. (2020b). Author correction: Adaptive evolution of virulence and persistence in carbapenem-resistant klebsiella pneumoniae. Nat. Med. 26, 1307. doi: 10.1038/s41591-020-0974-5
Fàbrega, A., Madurga, S., Giralt, E., Vila, J. (2009). Mechanism of action of and resistance to quinolones. Microb. Biotechnol. 2, 40–61. doi: 10.1111/j.1751-7915.2008.00063.x
Galani, I., Karaiskos, I., Giamarellou, H. (2021). Multidrug-resistant klebsiella pneumoniae: mechanisms of resistance including updated data for novel β-lactam-β-lactamase inhibitor combinations. Expert Rev. Anti Infect. Ther. 19, 1457–1468. doi: 10.1080/14787210.2021.1924674
Gu, D., Dong, N., Zheng, Z., Lin, D., Huang, M., Wang, L., et al. (2018). A fatal outbreak of ST11 carbapenem-resistant hypervirulent klebsiella pneumoniae in a Chinese hospital: a molecular epidemiological study. Lancet Infect. Dis. 18, 37–46. doi: 10.1016/S1473-3099(17)30489-9
Han, H., Zhou, H., Li, H., Gao, Y., Lu, Z., Hu, K., et al. (2013). Optimization of pulse-field gel electrophoresis for subtyping of klebsiella pneumoniae. Int. J. Environ. Res. Public Health 10, 2720–2731. doi: 10.3390/ijerph10072720
Huang, P. H., Cheng, Y. H., Chen, W. Y., Juan, C. H., Chou, S. H., Wang, J. T., et al. (2021). Risk factors and mechanisms of in vivo emergence of colistin resistance in carbapenem-resistant klebsiella pneumoniae. Int. J. Antimicrob. Agents 57, 106342. doi: 10.1016/j.ijantimicag.2021.106342
Huang, Y. H., Chou, S. H., Liang, S. W., Ni, C. E., Lin, Y. T., Huang, Y. W., et al. (2018). Emergence of an XDR and carbapenemase-producing hypervirulent klebsiella pneumoniae strain in Taiwan. J. Antimicrob. Chemother. 73, 2039–2046. doi: 10.1093/jac/dky164
Hughes, C., Phillips, R., Roberts, A. P. (1982). Serum resistance among escherichia coli strains causing urinary tract infection in relation to O type and the carriage of hemolysin, colicin, and antibiotic resistance determinants. Infect. Immun. 35, 270–275. doi: 10.1128/iai.35.1.270-275.1982
Insua, J. L., Llobet, E., Moranta, D., Pérez-Gutiérrez, C., Tomás, A., Garmendia, J., et al. (2013). Modeling klebsiella pneumoniae pathogenesis by infection of the wax moth galleria mellonella. Infect. Immun. 81, 3552–3565. doi: 10.1128/IAI.00391-13
Ito, R., Mustapha, M. M., Tomich, A. D., Callaghan, J. D., Mcelheny, C. L., Mettus, R. T., et al. (2017). Widespread fosfomycin resistance in gram-negative bacteria attributable to the chromosomal fosA gene. mBio 8, e00749–17. doi: 10.1128/mBio.00749-17
Jain, C., Rodriguez, R. L., Phillippy, A. M., Konstantinidis, K. T., Aluru, S. (2018). High throughput ANI analysis of 90K prokaryotic genomes reveals clear species boundaries. Nat. Commun. 9, 5114. doi: 10.1038/s41467-018-07641-9
Jayol, A., Poirel, L., Brink, A., Villegas, M. V., Yilmaz, M., Nordmann, P. (2014). Resistance to colistin associated with a single amino acid change in protein PmrB among klebsiella pneumoniae isolates of worldwide origin. Antimicrob. Agents Chemother. 58, 4762–4766. doi: 10.1128/AAC.00084-14
Jin, X., Chen, Q., Shen, F., Jiang, Y., Wu, X., Hua, X., et al. (2021). Resistance evolution of hypervirulent carbapenem-resistant klebsiella pneumoniae ST11 during treatment with tigecycline and polymyxin. Emerg. Microbes Infect. 10, 1129–1136. doi: 10.1080/22221751.2021.1937327
Kong, Y., Sun, Q., Chen, H., Draz, M. S., Xie, X., Zhang, J., et al. (2021). Transmission dynamics of carbapenem-resistant klebsiella pneumoniae sequence type 11 strains carrying capsular loci KL64 and rmpA/rmpA2 genes. Front. Microbiol. 12, 736896. doi: 10.3389/fmicb.2021.736896
Lan, P., Jiang, Y., Zhou, J., Yu, Y. (2021). A global perspective on the convergence of hypervirulence and carbapenem resistance in klebsiella pneumoniae. J. Glob. Antimicrob. Resist. 25, 26–34. doi: 10.1016/j.jgar.2021.02.020
Lee, C. R., Lee, J. H., Park, K. S., Jeon, J. H., Kim, Y. B., Cha, C. J., et al. (2017). Antimicrobial resistance of hypervirulent klebsiella pneumoniae: Epidemiology, hypervirulence-associated determinants, and resistance mechanisms. Front. Cell Infect. Microbiol. 7, 483. doi: 10.3389/fcimb.2017.00483
Liao, W., Liu, Y., Zhang, W. (2020). Virulence evolution, molecular mechanisms of resistance and prevalence of ST11 carbapenem-resistant klebsiella pneumoniae in China: A review over the last 10 years. J. Glob. Antimicrob. Resist. 23, 174–180. doi: 10.1016/j.jgar.2020.09.004
Liu, Y. C., Cheng, D. L., Lin, C. L. (1986). Klebsiella pneumoniae liver abscess associated with septic endophthalmitis. Arch. Intern. Med. 146, 1913–1916. doi: 10.1001/archinte.1986.00360220057011
Magiorakos, A. P., Srinivasan, A., Carey, R. B., Carmeli, Y., Falagas, M. E., Giske, C. G., et al. (2012). Multidrug-resistant, extensively drug-resistant and pandrug-resistant bacteria: an international expert proposal for interim standard definitions for acquired resistance. Clin. Microbiol. Infect. 18, 268–281. doi: 10.1111/j.1469-0691.2011.03570.x
Morales-León, F., Opazo-Capurro, A., Caro, C., Lincopan, N., Cardenas-Arias, A., Esposito, F., et al. (2021). Hypervirulent and hypermucoviscous extended-spectrum β-lactamase-producing klebsiella pneumoniae and klebsiella variicola in Chile. Virulence 12, 35–44. doi: 10.1080/21505594.2020.1859274
Pillar, C. M., Draghi, D. C., Dowzicky, M. J., Sahm, D. F. (2008). In vitro activity of tigecycline against gram-positive and gram-negative pathogens as evaluated by broth microdilution and etest. J. Clin. Microbiol. 46, 2862–2867. doi: 10.1128/JCM.00637-08
Podschun, R., Sievers, D., Fischer, A., Ullmann, U. (1993). Serotypes, hemagglutinins, siderophore synthesis, and serum resistance of klebsiella isolates causing human urinary tract infections. J. Infect. Dis. 168, 1415–1421. doi: 10.1093/infdis/168.6.1415
Russo, T. A., Marr, C. M. (2019). Hypervirulent klebsiella pneumoniae. Clin. Microbiol. Rev. 32, e00001–19. doi: 10.1128/CMR.00001-19
Russo, T. A., Olson, R., Fang, C. T., Stoesser, N., Miller, M., Macdonald, U., et al. (2018). Identification of biomarkers for differentiation of hypervirulent klebsiella pneumoniae from classical k. pneumoniae. J. Clin. Microbiol. 56, e00776–18. doi: 10.1128/JCM.00776-18
Russo, T. A., Olson, R., Macdonald, U., Beanan, J., Davidson, B. A. (2015). Aerobactin, but not yersiniabactin, salmochelin, or enterobactin, enables the growth/survival of hypervirulent (hypermucoviscous) klebsiella pneumoniae ex vivo and in vivo. Infect. Immun. 83, 3325–3333. doi: 10.1128/IAI.00430-15
Schuster, S., Kohler, S., Buck, A., Dambacher, C., König, A., Bohnert, J. A., et al. (2014). Random mutagenesis of the multidrug transporter AcrB from escherichia coli for identification of putative target residues of efflux pump inhibitors. Antimicrob. Agents Chemother. 58, 6870–6878. doi: 10.1128/AAC.03775-14
Sheu, C. C., Chang, Y. T., Lin, S. Y., Chen, Y. H., Hsueh, P. R. (2019). Infections caused by carbapenem-resistant enterobacteriaceae: An update on therapeutic options. Front. Microbiol. 10, 80. doi: 10.3389/fmicb.2019.00080
Tenover, F. C., Arbeit, R. D., Goering, R. V., Mickelsen, P. A., Murray, B. E., Persing, D. H., et al. (1995). Interpreting chromosomal DNA restriction patterns produced by pulsed-field gel electrophoresis: criteria for bacterial strain typing. J. Clin. Microbiol. 33, 2233–2239. doi: 10.1128/jcm.33.9.2233-2239.1995
Thakur, A. K., Fezio, W. L. (1981). A computer program for estimating LD50 and its confidence limits using modified behrens-Reed-Muench cumulant method. Drug Chem. Toxicol. 4, 297–305. doi: 10.3109/01480548109018136
Vermeil, T., Peters, A., Kilpatrick, C., Pires, D., Allegranzi, B., Pittet, D. (2019). Hand hygiene in hospitals: anatomy of a revolution. J. Hosp. Infect. 101, 383–392. doi: 10.1016/j.jhin.2018.09.003
Wacharotayankun, R., Arakawa, Y., Ohta, M., Tanaka, K., Akashi, T., Mori, M., et al. (1993). Enhancement of extracapsular polysaccharide synthesis in klebsiella pneumoniae by RmpA2, which shows homology to NtrC and FixJ. Infect. Immun. 61, 3164–3174. doi: 10.1128/iai.61.8.3164-3174.1993
Walker, K. A., Treat, L. P., Sepúlveda, V. E., Miller, V. L. (2020). The small protein RmpD drives hypermucoviscosity in klebsiella pneumoniae. mBio 11, e01750–20. doi: 10.1128/mBio.01750-20
Wang, Y. P., Chen, Y. H., Hung, I. C., Chu, P. H., Chang, Y. H., Lin, Y. T., et al. (2022). Transporter genes and fosA associated with fosfomycin resistance in carbapenem-resistant klebsiella pneumoniae. Front. Microbiol. 13, 816806. doi: 10.3389/fmicb.2022.816806
Xiong, L., Su, L., Tan, H., Zhao, W., Li, S., Zhu, Y., et al. (2021). Molecular epidemiological analysis of ST11-K64 extensively drug-resistant klebsiella pneumoniae infections outbreak in intensive care and neurosurgery units based on whole-genome sequencing. Front. Microbiol. 12, 709356. doi: 10.3389/fmicb.2021.709356
Xu, J., Zhu, Z., Chen, Y., Wang, W., He, F. (2021). The plasmid-borne tet(A) gene is an important factor causing tigecycline resistance in ST11 carbapenem-resistant klebsiella pneumoniae under selective pressure. Front. Microbiol. 12, 644949. doi: 10.3389/fmicb.2021.644949
Yang, X., Dong, N., Chan, E. W., Zhang, R., Chen, S. (2021). Carbapenem resistance-encoding and virulence-encoding conjugative plasmids in klebsiella pneumoniae. Trends Microbiol. 29, 65–83. doi: 10.1016/j.tim.2020.04.012
Yang, X., Sun, Q., Li, J., Jiang, Y., Li, Y., Lin, J., et al. (2022). Molecular epidemiology of carbapenem-resistant hypervirulent klebsiella pneumoniae in China. Emerg. Microbes Infect. 11, 841–49. doi: 10.1080/22221751.2022.2049458
Yang, Y., Yang, Y., Chen, G., Lin, M., Chen, Y., He, R., et al. (2021). Molecular characterization of carbapenem-resistant and virulent plasmids in klebsiella pneumoniae from patients with bloodstream infections in China. Emerg. Microbes Infect. 10, 700–709. doi: 10.1080/22221751.2021.1906163
Zasowski, E. J., Rybak, J. M., Rybak, M. J. (2015). The β-lactams strike back: Ceftazidime-avibactam. Pharmacotherapy 35, 755–770. doi: 10.1002/phar.1622
Zhang, Y., Wang, X., Wang, Q., Chen, H., Li, H., Wang, S., et al. (2021). Emergence of tigecycline nonsusceptible and IMP-4 carbapenemase-producing K2-ST65 hypervirulent klebsiella pneumoniae in China. Microbiol. Spectr. 9, e0130521. doi: 10.1128/Spectrum.01305-21
Keywords: Klebsiella pneumoniae, extensively drug-resistant, hypervirulent, tigecycline resistance, outbreak
Citation: Liu S, Ding Y, Xu Y, Li Z, Zeng Z and Liu J (2022) An outbreak of extensively drug-resistant and hypervirulent Klebsiella pneumoniae in an intensive care unit of a teaching hospital in Southwest China. Front. Cell. Infect. Microbiol. 12:979219. doi: 10.3389/fcimb.2022.979219
Received: 27 June 2022; Accepted: 25 August 2022;
Published: 13 September 2022.
Edited by:
Yi-Tsung Lin, Taipei Veterans General Hospital, TaiwanReviewed by:
Mingju Hao, Qianfoshan Hospital, Shandong University, ChinaCopyright © 2022 Liu, Ding, Xu, Li, Zeng and Liu. This is an open-access article distributed under the terms of the Creative Commons Attribution License (CC BY). The use, distribution or reproduction in other forums is permitted, provided the original author(s) and the copyright owner(s) are credited and that the original publication in this journal is cited, in accordance with accepted academic practice. No use, distribution or reproduction is permitted which does not comply with these terms.
*Correspondence: Jinbo Liu, bGl1amI3MjAzQHN3bXUuZWR1LmNu; Zhangrui Zeng, NzEwNjAxMTI0QHFxLmNvbQ==
†These authors have contributed equally to this work and share first authorship
Disclaimer: All claims expressed in this article are solely those of the authors and do not necessarily represent those of their affiliated organizations, or those of the publisher, the editors and the reviewers. Any product that may be evaluated in this article or claim that may be made by its manufacturer is not guaranteed or endorsed by the publisher.
Research integrity at Frontiers
Learn more about the work of our research integrity team to safeguard the quality of each article we publish.