- 1Department of Biosciences, The Commission on Science and Technology for Sustainable Development in the South (COMSATS) University Islamabad (CUI), Islamabad, Pakistan
- 2Department of Physical Chemistry and Polymers Technology, Silesian University of Technology, Gliwice, Poland
- 3Joint Doctoral School, Silesian University of Technology, Gliwice, Poland
- 4Department of Microbiology and Immunology, Faculty of Pharmacy, Zagazig University, Zagazig, Egypt
- 5Department of Bacteriology, Immunology, and Mycology, Faculty of Veterinary Medicine, Suez Canal University, Ismailia, Egypt
- 6Department of Pharmacology, Faculty of Pharmacy, Delta University for Science and Technology, Gamasa, Egypt
- 7Faculty of Medicine and Pharmacy, University of Oradea, Oradea, Romania
The coronavirus disease 2019 (COVID-19) pandemic has prompted a lot of questions globally regarding the range of information about the virus’s possible routes of transmission, diagnostics, and therapeutic tools. Worldwide studies have pointed out the importance of monitoring and early surveillance techniques based on the identification of viral RNA in wastewater. These studies indicated the presence of the severe acute respiratory syndrome coronavirus 2 (SARS-CoV-2) RNA in human feces, which is shed via excreta including mucus, feces, saliva, and sputum. Subsequently, they get dumped into wastewater, and their presence in wastewater provides a possibility of using it as a tool to help prevent and eradicate the virus. Its monitoring is still done in many regions worldwide and serves as an early “warning signal”; however, a lot of limitations of wastewater surveillance have also been identified.
Introduction
Economic stability and the security of human health are considerably affected by infectious diseases as they cause one-fourth of the mortalities around the world. Even with the development of advanced healthcare systems, viral infections still continue to emerge (Bloom and Cadarette, 2019). The recent outbreak of coronavirus disease 2019 (COVID-19) appeared to be highly pathogenic and life-threatening, with high mortality rates, affecting almost every country in the world (Ming, 2020). The symptoms include fatigue, fever, dry cough, muscle pain, and shortness of breath (Chen et al., 2020; Huang et al., 2020; Muhammad et al., 2021; Egbuna et al., 2022). Initially, the disease was believed to be pneumonia by healthcare officials; however, a thorough analysis of the patient’s throat sample detected a novel coronavirus (CoV) (Ulhaq et al., 2021). On January 7, another CoV pathogen in humans was discovered; provisionally, it was named novel coronavirus 2019 (nCoV-19) by the World Health Organization (WHO). Because of its genetic similarity with severe acute respiratory syndrome coronavirus (SARS-CoV), it was then recognized as SARS-CoV-2 (severe acute respiratory syndrome coronavirus 2) according to the viral nomenclature scheme (Basit et al., 2021). The disease caused by SARS-CoV-2 was initially named viral pneumonia; the WHO later gave it the name coronavirus disease 2019 (COVID-19) due to the presence of a number of unique disease symptoms of the lower respiratory tract, such as difficulty in breathing (WHO 2019-nCoV Situation Report-22). Moreover, patients also suffer from mental dissatisfaction, acute kidney failure, and dysfunction of various other organs in extreme cases of COVID-19 (Basit et al., 2021). However, the incubation period fluctuates based on the patient’s health status and immunity, where it can be shorter in older people and in those with a weak immune system. COVID-19 is rapidly transmitted through respiratory droplets due to its more contagious characteristic (Jiang et al., 2020). More than 213 countries have reported over 40 million confirmed cases, with nationwide lockdowns imposed to prevent the spread of the disease (Kataki et al., 2021).
SARS-CoV-2 had been detected in the blood and in anal and oropharyngeal swabs, along with urine samples of patients who tested positive (Chen et al., 2020; Ren et al., 2020), indicating the presence of this deadly viral RNA in the streamlets of wastewater treatment plants (WWTPs) (Foladori et al., 2020). The swab protocols are mostly used for the collection of target pathogens from the environment and from patients (Colaneri et al., 2020; Moore et al., 2021), but with various disadvantages. These include the inability to perform collection from living environments and the possibility of getting false-positive signals due to the free RNA fragments of lysed viruses from the environment and patients, as they can remain stable for long periods of time. The European Union (EU) Commission suggested a systematic surveillance approach for SARS-CoV-2 in EU wastewaters (Commission, E, 2021). Patients infected with SARS-CoV-2 do not always have the typical symptoms (Nishiura et al., 2020), as almost 40%–50% of infections do not show any symptoms (Oran and Topol, 2020), but can still spread the virus (Gandhi et al., 2020). Both asymptomatic and symptomatic individuals shed SARS-CoV-2 in the urine, saliva, feces, and nasal fluids (Cevik et al., 2021). The analysis of fecal samples is more sensitive for the detection of SARS-COV-2 as feces contain a higher viral load (Yuan et al., 2021). Reports showed that the presence of SARS-CoV-2 RNA in human feces (Holshue et al., 2020; Song et al., 2020) and urine leads to the shedding of the viral RNA in wastewater (Ding et al., 2020; Gao et al., 2020; Holshue et al., 2020; Jiehao et al., 2020; Wang et al., 2020; Woelfel et al., 2020). Several studies have identified the viral RNA in wastewater (Ahmed et al., 2020a; Medema et al., 2020). COVID-19 consequently causes alarming situations worldwide, posing a cluster of questions for the scientific community regarding the contemporaneous exploration of epidemiological studies such as wastewater surveillance. Therefore, methodologies need to be developed using wastewater for epidemiological studies of human diseases such as COVID-19.
The epidemiology of human viruses in wastewater can be beneficial for population-based analysis of the epidemiology of SARS-CoV-2 to prevent its further transmission (Hillary et al., 2021). Viral detection of CoVs in wastewater was first developed in 2013 (Wong et al., 2013). The genome of the human coronavirus HKU1 (HCoV-HKU1) was identified in sewage (Bibby and Peccia, 2013), and animal CoV had also been found in surface water (Blanco et al., 2019). Environmental surveillance (ES) based on wastewater sampling enables the prediction of the condition of drainage areas with little effort compared to conducting clinical surveys (Agrawal et al., 2021). Pre-symptomatic and asymptomatic infected individuals normally are not included in clinical surveys. Sample collection and testing are usually expensive and time-consuming. A lot of studies have shown that the monitoring of wastewater can detect the outbreak of viruses such as norovirus and poliovirus faster than clinical surveys (Hovi et al., 2012; Hellmér et al., 2014; Daughton, 2020; Hata and Honda, 2020). Its potential advantage is the possibility to conduct screening of a large population using a few samples without depending on the availability of clinical testing (Thompson et al., 2020), with transmission collections in large geographic regions that can assist in rapid efforts by public health authorities (Stadler et al., 2020).
Evidence of viral RNA in fecal material
Coronavirus get into fecal material through the swallowing of secretions from the upper respiratory tract, which is then mixed with food when it is not affected by gastric acid. Viral replication in intestinal cells or infected immune cells plays a role in the presence of CoV in feces (Ding et al., 2020; Gu et al., 2020). Its replication in intestinal samples had been identified, determining the gastrointestinal tract (GIT) of humans as a site of SARS-CoV-2 infection (Ding et al., 2004). SARS-Cov-2 replication can be confirmed by the presence of the viral RNA in feces (Wang et al., 2020). A study showed that the rectal mucosa is altered by infection with SARS-CoV-2 and exhibited a replication. The virus was replicated in the rectum during the period of incubation, while the viral particles showed in the epithelial cells of the patient’s intestine (Qian et al., 2021). Occasionally, urine samples of infected individuals contain viral RNA, but the swabs from the throat result as negative (Xu et al., 2020). The SARS-CoV-2 RNA has been reported earlier from throat and nasal swabs compared to fecal samples (Zhang et al., 2020c). A lot of studies have shown this fact, as in one case that revealed the SARS-CoV-2 RNA being contained in throat and nasal swabs (TS and NS, respectively) for only 9 days after ailment, while the fecal RNA remained for more than 20 days (Cai et al., 2020). Another report showed the viral RNA in fecal material for up to 4–5 weeks (Cai et al., 2020; Zou et al., 2020). Generally, infected patients are discharged from hospital when the NS and TS samples are negative, even if the fecal samples are still positive for SARS-CoV-2 RNA; consequently, the oral–fecal route implication needs to be highlighted, particularly in the context of the stability of the SARS-CoV-2 virus in sewage water. Moreover, its fecal shedding recommends demonstrating the decontamination practices for toilets and the observation of hygiene and sanitation practices (Parasa et al., 2020).
Gastrointestinal symptoms of COVID-19 and shedding of SARS-CoV-2 in excreta
The presence of ACE2 in the intestinal cells confirms the entry of SARS-CoV-2 in the GIT, with the presence of SARS-CoV-2 in the stool and diarrhea having been reported (Muhammad et al., 2021). Examination of the esophagus revealed lymphocyte intrusion in the squamous epithelium, stomach lamina propria, duodenum, and interstitial edema, along with various gastrointestinal symptoms of COVID-19 such as nausea, vomiting, and diarrhea (Leung et al., 2003; Memish et al., 2020). These symptoms were shown by nearly 2%–10% of patients (Wang et al., 2005; Gao et al., 2020; Wang et al., 2020). The virus usually infects epithelial cells of the GIT (Ding et al., 2020); moreover, the RNA of SARS-CoV2 has been detected in 40%–85% of fecal samples, indicating a similar frequency of the detection of SARS-CoV-2 RNA in feces to respiratory secretions (Natarajan et al., 2022). According to Ling et al. (2020), 81.8% of studies on CoV reported the detection of SARS-CoV-2 in stool samples. The viral load is nearly 108 copies/g of feces (Lescure et al., 2020; Pan et al., 2020; Woelfel et al., 2020), 107 copies/ml when there is diarrhea, and 2.5 × 104 copies/ml in the case of urine (Hung et al., 2004). Viral shedding in fecal material continues for almost 7 weeks after the onset of the first symptom (Ding et al., 2020; Jiehao et al., 2020). Globally, there are various reports on the replication and infection of SARS-CoV-2 in the GIT, as shown in Table 1. With the rapid growth of the global population, the emergence and reemergence of human pathogenic viruses have prompted a demand for surveillance systems that understand the dynamics of infection in populations (Mao et al., 2020). The widespread circulation of SARS-CoV-2 variants calls for wastewater-based epidemiology to examine its magnitude and distribution in a community by examining its biomarker levels in a sewage network. Detection of the presence of SARS-CoV-2 in wastewater can be utilized as a disease surveillance tool, which has been supported worldwide by many researchers (Ahmed et al., 2020a; Lodder and de Roda Husman, 2020).
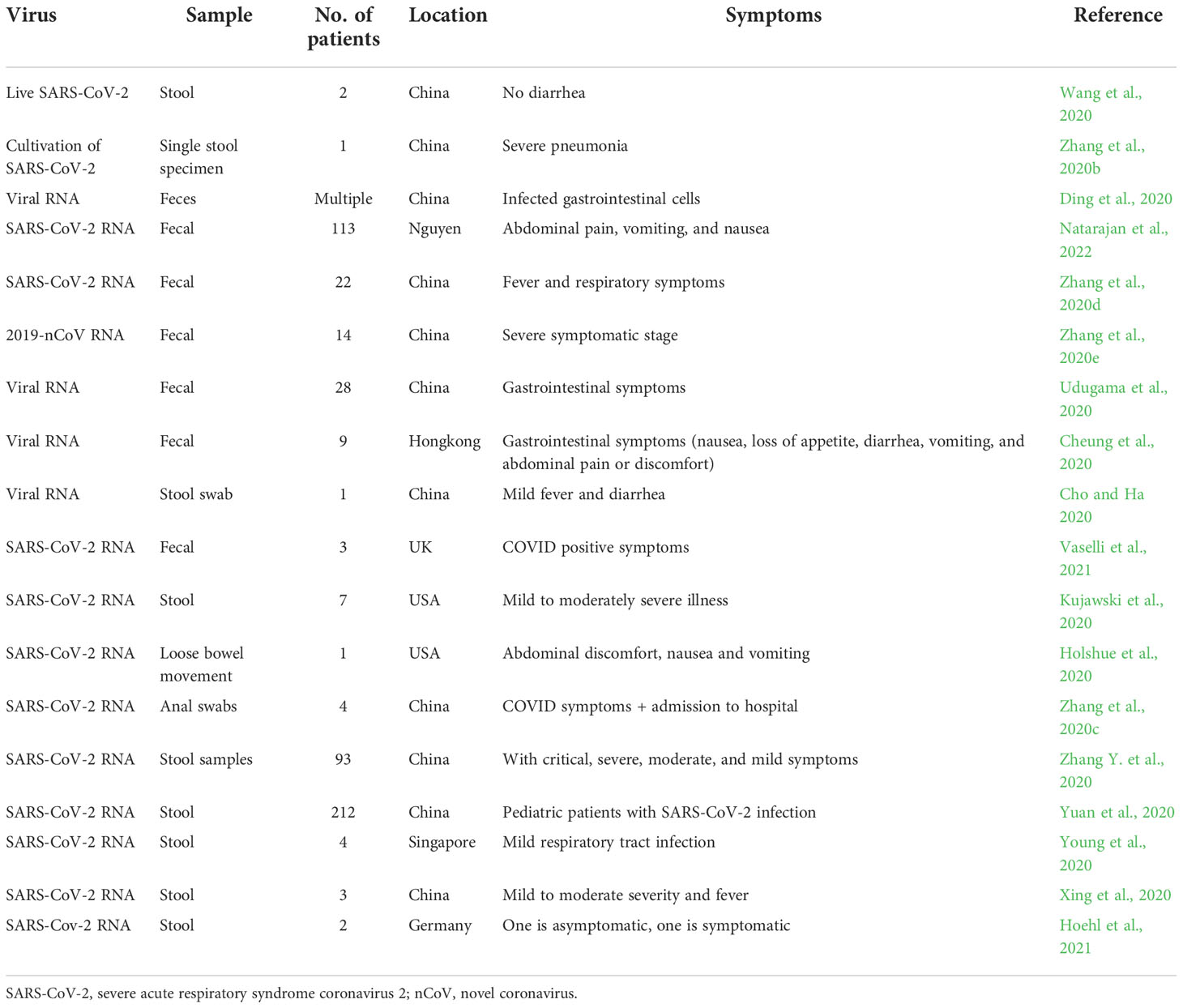
Table 1 Worldwide studies on the replication and infection of SARS-COV-2 in stool and fecal samples.
The SARS-CoV-2 RNA has been reported in groundwater, surface water, wastewater, sludge, and other hospital-related water systems during the low- and middle-risk periods of COVID-19 (Zhao et al., 2020). The concentration and the molecular detection of SARS-CoV-2 RNA in wastewater isolated from different localities throughout the world are shown in Table 2. The necessity for surface hygiene can be explained by the detection SARS-CoV-2 in the toilets of infected patients (Ding et al., 2020). Moreover, SARS-CoV-2 was also found in natural waters derived from areas with poor sanitation, which has serious consequences on health and the environment (Guerrero-Latorre et al., 2020). However, RNA detection in wastewater lacks an appropriate standard protocol. The method used to calculate the concentration of viruses is not that effective for the recovery of these viruses (Haramoto et al., 2009; Ye et al., 2016).
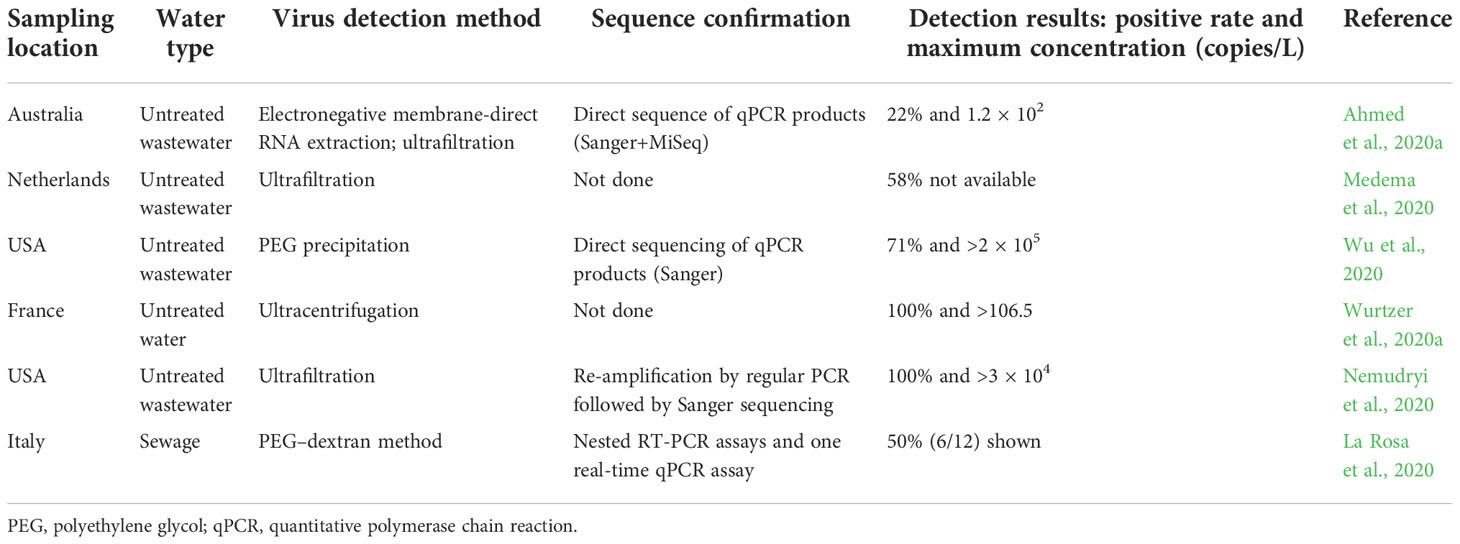
Table 2 Details of the reported molecular detection of severe acute respiratory syndrome coronavirus 2 (SARS-CoV-2) in wastewater.
Approaches used in wastewater-based epidemiology
Surveillance provides insights into the condition of the outbreak in an area by testing the wastewater samples with time. The surveillance data are used in three ways: to monitor the presence of infections in a community, to track the infection trends in a community, and to perform targeted screening of the infections for moderation measures (CDC, 2022). There are various types of wastewater-based epidemiology (WBE), which require research for validation. The qualitative approach examines the minimum level of infection, which detects sensitivity, the semi-qualitative approach can indicate the comparative level of infection in a community, while the quantitative approach can detect the absolute infection level and is capable of performing comparisons across communities. Initially, these approaches were used as tools to assess illegal drug use within a community (Daughton, 2001) by quantifying them with specific human metabolites in wastewater (Feng et al., 2018). Sewage RNA collection can both indicate past and active infections when considering the duration of RNA excretion through the stool (Chen et al., 2020; Wu et al., 2020). Viral shedding can occur right after infection; however, an infected patient being identified through this system must show symptoms and start treatments before clinical diagnosis (Larsen and Wigginton, 2020). Surveillance via wastewater can be used as an indicator of disease transmission and its ratios increasing and decreasing, such as, traditionally, deaths, hospitalizations, serological data, and test positivity (Rowe et al., 2009). Similarly, daily surveillance of SARS-CoV-2 RNA in wastewater can provide evidence, similar to daily individual testing in a community, but with a less invasive and cost-effective method reconfirming the already diagnosed cases. Population size is important in the use of the quantitative approach, which can determine the infected population (Daughton, 2012; Daughton, 2018). Calibration of the monitoring of WBE is important so that the data will be related to the infection rates, which can be conducted utilizing quantitative PCR (qPCR) with reverse regression of the known rates of infection (Bar-Or et al., 2021). This will help in the estimation of the total number of infected people, reliably enabling a comparison of the infection rates in communities. Moreover, the monitoring information should be presented and interpreted to the public for awareness; experts and scientific authors can play an important role. The involvement of policy makers, government health officials, and leaders would also help in this regard (Daughton, 2020).
SARS-CoV-2 surveillance in wastewater
The wastewater surveillance approach is presently utilized all over the world as an effective tool for SARS-CoV-2 RNA monitoring (Bivins et al., 2020). It is not only limited to the detection of COVID-19, but was also previously utilized for viruses such as hepatitis A and poliovirus (Asghar et al., 2014; La Rosa et al., 2014; Bivins et al., 2020). This surveillance method is currently utilized in almost 55 countries across the world with a declaration from over 250 organizations to analyze wastewater samples for SARS-CoV-2 RNA from more than 2,690 sites, including surface waters and WWTPs (Naughton et al., 2021). Some of these observations were done even before the appearance of the first positive case clinically (Medema et al., 2020). Therefore, this surveillance technique is useful in indicating the presentation of SARS-COV-2 and other viruses in communities along with the estimation of its effectiveness in the healthcare field (Kitajima et al., 2020). Wastewater can be a factor in the outbreak of SARS viruses because of improper sewage systems (McKinney et al., 2006). Retrospectively, in 2004, wastewater epidemiology was used for SARS-CoV, which found that almost 30% of disinfected wastewater and 100% of untreated water contained the virus during the first outbreak in China (Wang et al., 2005). The prevalence of SARS-CoV-2 was determined by detecting the RNA copies of the virus in the Australian sewage basin using reverse transcription quantitative PCR (RT-qPCR). This surveillance method was also used in the USA to study the strains of SARS-CoV-2, their phylogeny, ancestry, and the effectiveness of interventions for public health in reference to the outbreak (Nemudryi et al., 2020). Wastewater surveillance for COVID-19 around the world is shown in Table 3.
In Australia, wastewater surveillance detected the SARS-CoV-2 RNA using sequencing and qPCR (Agrawal et al., 2021); however, further studies are needed to understand the reliability of the analysis. A lot of studies have described the detection of SARS-CoV-2 within sewage or wastewater in USA, Australia, France, and Netherlands (Lodder and de Roda Husman, 2020; Medema et al., 2020; Nemudryi et al., 2020; Wu et al., 2020; Wurtzer et al., 2020b). Most of these studies were done without treatments and had a maximum concentration of 106 copies/L (Wurtzer et al., 2020a). These studies, along with several other continuous efforts in different regions of the world, were conducted to provide updates on the community prevalence of SARS-CoV-2 and its epidemiology. This surveillance method can also be used as a “warning signal” to alleviate the spread of infection in communities, as an outline describing the ethical issues in relation to the basic approach to sanitation (Murakami et al., 2020).
ES can help provide information on the transmission of infection within a community before clinical surveillance is done. This serves as an early warning system, as the shedding of SARS-CoV-2 begins even before the onset of symptoms in an infected person (Jones et al., 2020). It can also highlight the underreported characteristics from clinical surveillance due to several testing policies; thus, ES can assist in better monitoring of the incidences of COVID-19 (Choi et al., 2018). It can also be beneficial in international airports, voyage ships, and aircraft for monitoring the SARS-CoV-2 drifts among travelers (Medema et al., 2020). Analysis at the molecular level in a community can paint a picture of the existing and emerging variants of the virus (Izquierdo-Lara et al., 2021). An alarming fact is that, in many poor countries, the virus is transported to treatment plants for the dumping of human waste, which accounts for almost 900 million public globally (WHO, 2017). Underground water or soil can also become contaminated due to lack of sanitation. A number of previous outbreaks also showed viral shedding via human excreta (Goh et al., 2013; Zhou et al., 2017; Yeo et al., 2020). The consequences of COVID-19 indication in wastewater were given by Nghiem et al. (2020), with a description of the fecal–oral transmission routes by Heller et al. (2020).
Understanding COVID-19 epidemiology through wastewater surveillance
The presence of the viral RNA of SARS-CoV-2 in wastewater creates the possibility of employing it as a tool to study viral genomics, epidemiology, and prevalence, as well as possible eradication from the community (Kitajima et al., 2020). The transmission routes of SARS-CoV-2 include seepage wastewater as reusable water, biosolid products from sludge, thrusting, mixing, and microbial vaporizer. Viruses need to be viable to cause infection through these systems. According to updated information, the viability of CoVs decreases in wastewater, and their infectivity should also be diminished upon transfer from feces to effluent and then to treatment plants and the environment (La Rosa et al., 2020). The epidemiology of wastewater can be an important method to trace the viral circulation in a community in order to evaluate the prevalence and genomic diversity (Sinclair et al., 2008; Xagoraraki and O’Brien, 2020).
Wastewater systems can provide an opportunity to detect the virus extracted from feces (Carducci et al., 2006; La Rosa and Muscillo, 2013). With this method, monitoring the epidemiology of the virus has become possible, with the traditional techniques being somehow limited due to viral infections that do not cause any symptoms (Johansson et al., 2014; Qi et al., 2018). Figure 1 shows the surveillance process related to sludge and wastewater.
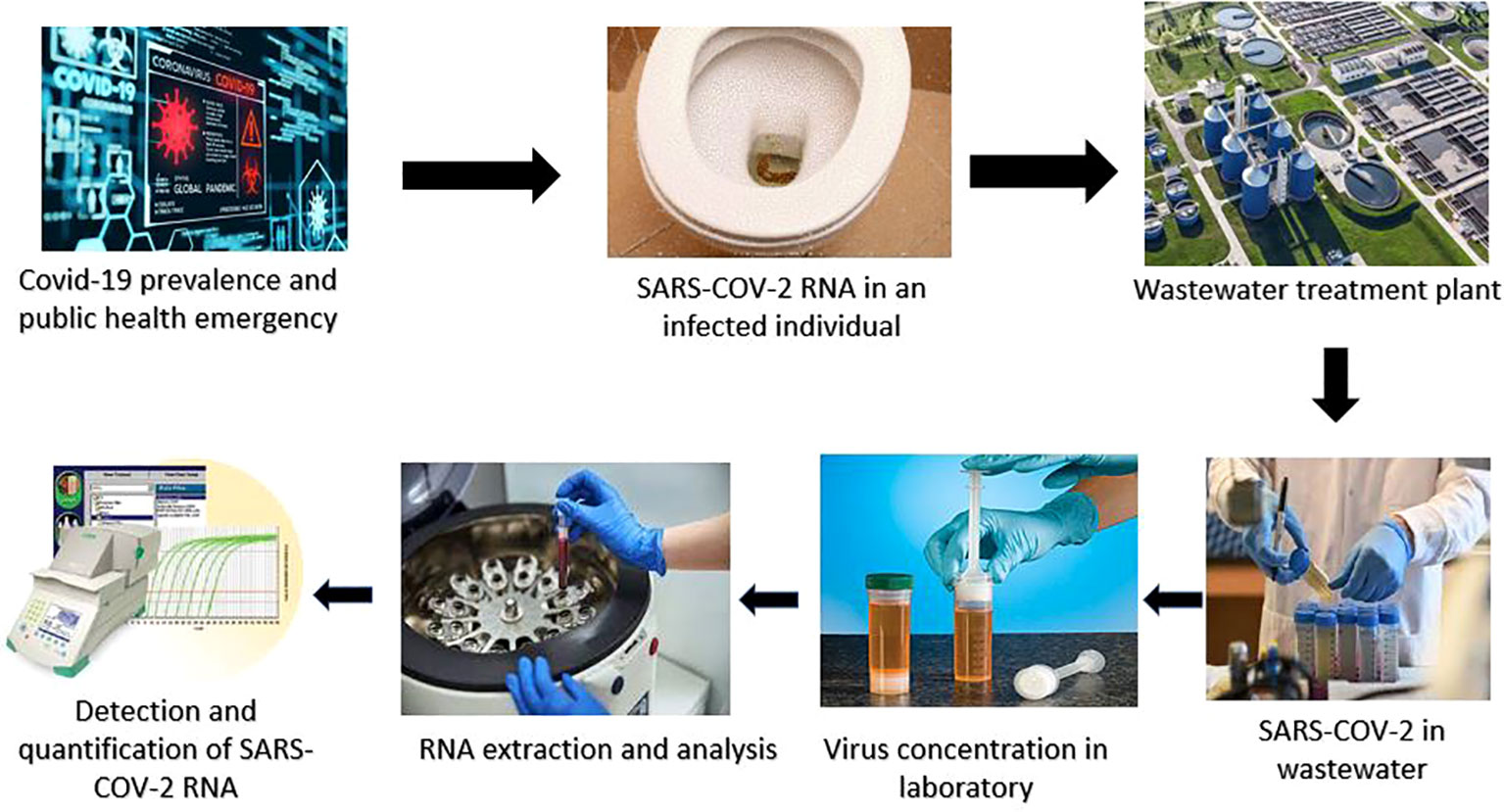
Figure 1 Steps of wastewater-based epidemiology (WBE) by testing wastewater to monitor the outbreak of SARS-CoV-2..
It is rather difficult to determine the circulation of a virus in a community through comparisons between regions with varied capabilities of viral diagnosis (Ortiz-Ospina and Hasell, 2020). Viral surveillance is an impartial method of evaluating the viral spread in different regions, even when there is a limitation in resources and diagnosis. Monitoring can also enable the detection of changes in the viral strains with genomic analysis from an evolution perspective (Lodder et al., 2013; La Rosa et al., 2014).
Wastewater surveillance can detect viruses even at low levels, such as when there is a decrease in cases due to healthcare interventions (Asghar et al., 2014). Moreover, it is important when a novel virus or strain is introduced into a population (Sinclair et al., 2008; Savolainen-Kopra et al., 2011) due to seasonal changes or fluctuations (Sedmak et al., 2003; Hellmér et al., 2014; Prevost et al., 2015). Therefore, these strategies can be useful as an “early warning” system (Xagoraraki and O’Brien, 2020) to determine any alterations in public health involvement, as in the case of social isolation and lockdowns with the recurrence of SARS-CoV-2. A lot of preventive measures can be implemented by performing wastewater analysis for viruses and by being able to detect any novel viruses earlier than clinical detection (Ng et al., 2012; Fernandez-Cassi et al., 2018; Bibby et al., 2019). The total number of affected individuals can be estimated by detecting the RNA copy numbers of SARS-CoV-2 in untreated wastewater via catchment site and by using the Monte Carlo simulation. This statistical method is employed to resolve complex issues and determine possible outcomes using estimates (Sin and Espuña, 2020), which is a simple and easy-to-use concept. The estimation of almost 1,090 infected patients with clinical observations was conducted using this method (Ahmed et al., 2020a). Studies have pointed out the need for more strategies and molecular-based assays to validate the presence of viruses in water, which will increase the accuracy of wastewater surveillance.
Limitations in wastewater surveillance
In diagnostics, various factors can affect the reliability of the results (Bustin et al., 2009; Medema et al., 2020; Pecson et al., 2021). False-positive errors can result from high sensitivity developed via the analysis of wastewater samples using poor RT-PCR assays (Ahmed et al., 2022). The application of WBE can be a bit difficult due to poor sanitation and the lack of treatment plants for wastewater (Ahmed et al., 2021; Jakariya et al., 2021). Therefore, currently, it is not likely to entirely alter the viral RNA levels in wastewater for its prevalence (Larsen and Wigginton, 2020). Several limitations of wastewater surveillance have been identified, such as in relating the identified infected cases and the virus levels. As viral excretion can be altered during the infection period, delays can occur due to different time frames, capturing altered population distributions due to traveling, and fluid dilution from precipitation, among others (La Rosa et al., 2020). Moreover, the risk of genome stability in wastewater, alterations in the sampling type, poor efficiency of the viral concentration technique, and unavailability of a sensitive screening assay can limit the quantification of viral detection, especially when the concentration is low. The pandemic can become worse when appropriate testing trials fail to efficiently determine patients infected with SARS-CoV-2 (Mercer and Salit, 2021). Additionally, these patients are extremely contagious either at the pre-asymptomatic or the asymptomatic stage. Hence, without a proper screening assay, they can infect healthy individuals earlier than they can be identified for isolation or hospitalization (Oran and Topol, 2020). Consequently, for the accurate detection of COVID-19, new molecular-based methods have been recently developed. These include a microarray-based method, loop-mediated isothermal amplification (LAMP) method, RNA targeting CRISPR (clustered regularly interspaced short palindromic repeats) diagnosis, rolling circle amplification-based method, and nanopore targeted sequencing (NTS) (Basit et al., 2021).
Some of these diagnostic methods have not been independently assessed or approved by authorities of healthcare systems. The iAMP COVID-19 detection kit (Atila BioSystems, Mountain View, CA, USA) based on isothermal amplification (LAMP) technology has been approved by the U.S. Food and Drug Administration (FDA) with 100% sensitivity (BioSystems, A, 2020). Another kit, Sherlock™ CRISPR (Sherlock Biosciences, Boston, MA, USA), which is based on CRISPR technology, can detect SARS-CoV-2 with a specificity of 100% (Biosciences, S, 2020), while the microarray method allows the detection of various microbial agents through the amplification of nucleic acids with high specificity (Vora et al., 2004) and previously showed results of the detection of CoVs (Shi et al., 2003). The limit of detection and the clinical sensitivity are considered crucial criteria for commercial molecular diagnostics in the case of COVID-19 diagnosis (Bachelet, 2020; Tang et al., 2020). Currently, there are only two kinds of commercial diagnostic tests: serological tests to detect CoV-2 antibodies in serum and molecular diagnostics to determine viral RNA in respiratory specimens (Sheridan, 2020). The next-generation sequencing-based method Swab-Seq is another technique utilizing RT-PCR primers, which has exclusive molecular codes for the sequencing of many samples (Bloom et al., 2020). Decentralized wastewater samples are quite difficult to manage due to the rigorous sample collection process for households (Takeda et al., 2021). Analysis of the high-risk points is needed as a solution to this problem, which includes quarantine areas, hospitals, and healthcare services. Furthermore, samples from sludge plants (collected from decentralized systems) should be collected (UNDP, 2020). Various shareholders need to be involved in the processes of a monitoring framework, including sampling, analysis, information feedback, and any subsequent action or decision-making. Sample analysis would require equipment for the health and research sectors involving national and international platforms for wastewater surveillance (UN Women, 2020). Budgets should be established for prevention and response, along with the establishment of committees to meet regional and international goals (Takeda et al., 2021). The calibration of viral RNA needs to be performed against the exact number of cases presented by marking and targeting biomarkers (Daughton, 2020). The future of wastewater surveillance needs to be assessed more in light of vigorous epidemiological data, as enormous limitations of testing potential have been observed over time, especially in communities where delays in testing can occur.
SARS-CoV-2 diagnosis from wastewater samples
The presence of SARS-CoV-2 in wastewater samples can be confirmed by determining the viral RNA sequence with qPCR (WHO, 2020). In this regard, the positive control can be shown by determining the plasmid with the complete nucleocapsid gene of SARS-CoV-2 (Wu et al., 2020). Different pretreatment techniques are usually performed during the sampling of wastewater to ensure efficient detection with maximum viral concentration. A lot of approaches for the enrichment of different viruses have been recently used, including PCR assays; however, there is still a lack of a standard protocol. The initial diagnostic test was based on the specific primers and sequences for PCR. This test was developed based on previous research works on the detection of SARS-CoV-2 (Udugama et al., 2020). Several companies have started commercializing them for a faster supply of tests (Vandenberg et al., 2021).
The active sampling approach has been demonstrated for the quantification of SARS-CoV-2 in wastewater, along with the trends of infection (Graham et al., 2020; Castro-Gutierrez et al., 2022), with several methods to determine concentrations having been advised for wastewater (Rusiñol et al., 2020; Barril et al., 2021; Cervantes-Aviles et al., 2021; Chik et al., 2021; Philo et al., 2021). Most of these focused on extrinsically sourced viral controls in water (Kantor et al., 2021). Filtration, nuclease treatment, and freeze–thaw methods are among the reputable and rapid procedures for viral RNA separation from host RNA (Marston et al., 2013; Dupinay et al., 2014; Hall et al., 2014). The main aim is to avoid the reduction of the overall viral RNA quantity during separation from the host RNA (Victoria et al., 2008; Hall et al., 2014). In this regard, microfluidic devices are far more dependable compared to the traditional methods because of their requirement of less volume of biological samples for disease biomarker testing within a short time. Moreover, parallel analysis assays based on a single microfluidic device can provide the best statistical results (Verpoorte and De Rooij, 2003; Zhang et al., 2013). The gold standard for SARS-CoV-2 diagnostic is RT-PCR (Carter et al., 2020), which is specific, reliable, and sensitive. Numerous costly instruments and highly trained professionals are needed to perform the tests, which is a concern in developing countries (Afzal, 2020). On the contrary, the RT-LAMP test reduces the detection time to almost 30 min, enabling rapid detection (Lamb et al., 2020). The available microfluidic kits offer a rapid, cost-effective, and precise detection overall; therefore, they are appropriate in settings where resources are scarce for point-of-care testing (POCT). Integrated microfluidic devices have applications in whole-genome sequencing, COVID-19 progression, and intratracheal neutralization of a virus (Jamiruddin et al., 2022).
Polymerase chain reaction
In molecular biology, PCR is the quick and broadly used method to make DNA copies from thousands to millions containing the fragment of a gene. A very small quantity of DNA (genetic material) can be amplified by PCR and provide adequate evidence of the DNA or gene segment for comprehensive study. Most viral diseases are diagnosed using PCR due to its broader impact and reliability, indicating its value for routine use in the diagnosis of various infections such as COVID-19 (Basit et al., 2021). The etiological agent of COVID-19 is SARS-CoV-2, which is an RNA virus that can be converted to complementary DNA (cDNA) by the process of reverse transcription through the reverse transcriptase enzyme. Subsequently, with the use of specific primers, the DNA sample is amplified by PCR and then further processed by gel visualization and gene sequencing (Setianingsih et al., 2019). However, for the diagnosis of COVID-19, PCR is the most recommended test. Microfluidics coupled with PCR (MFQPCR) results can be accelerated, providing fast (up to 1 h) and accurate results (Zhou et al., 2014). However, there are certain limitations to the use of PCR. These include obtaining false-positive results, a long processing time, and low specificity and sensitivity. With quantitative microbial risk assessment, MFQPCR can quantitatively detect various pathogens in freshwater contaminated by waterfowl feces (Ishii et al., 2014) with high sensitivity. Wastewater contains a large number of pathogenic RNA viruses (Miura et al., 2015; Kobayashi et al., 2017), and microfluidic POCT has an advantage over traditional assays because it employs transportable devices and can carry out tests at diverse sampling sites (Telenti et al., 2021). However, conventional PCR assays are quite rigorous and labor-intensive (Le Guyader et al., 1994; Aw et al., 2009).
Real-time PCR
During the pandemic, the most widely used and most favored method for COVID-19 diagnosis was real-time RT-PCR, due to RNA being the genetic material and its several advantages, which significantly helped in the detection of infection in the early phases (Corman et al., 2020). Comparatively, real-time RT-PCR has high specificity and sensitivity compared to general PCR, and the procedure is simple and quantitative (Noh et al., 2017). Considerable efforts have been exerted toward the improvement of the real-time RTPCR method in order to overcome its disadvantages when carrying out the procedure (Basit et al., 2021).
Loop-mediated isothermal amplification method
Among the molecular-based diagnostic methods used for COVID-19, the most prominent during the recent outbreak has been the LAMP method. It has higher sensitivity and specificity rates and amplifies the nucleic acids (DNA/RNA) very rapidly. The method’s procedure involves DNA polymerase and specific primers that synthesize the targeted DNA. The temperature range for this method is 60–65°C, which is a change from that of the ordinary process as it did not denature the strands but only displaced them (Nagamine et al., 2002). At the end point of detection through the LAMP method, the amplified products are further analyzed using gel electrophoresis. Additionally, due to the exponential amplification feature of LAMP, four different primers can detect six different target sequences at the same time (Francois et al., 2011). Within a very short time or a maximum of an hour, up to more than 109 copies of the targeted sequence of loop-form DNA can be amplified by the LAMP assay, with the final product being in the form of many inverted repeats. The use of LAMP for the clinical diagnosis of COVID-19 appears to be extremely useful, reliable, and cost-effective as it does not require expensive instruments or reagents/chemicals. In this case, the ORF1b region of SARS-CoV-2 is targeted and amplified by six primers to establish effective viability. A schematic illustration of the combined reverse transcription LAMP and vertical flow visualization (RT-LAMP-VF) assay is shown in Figure 2.
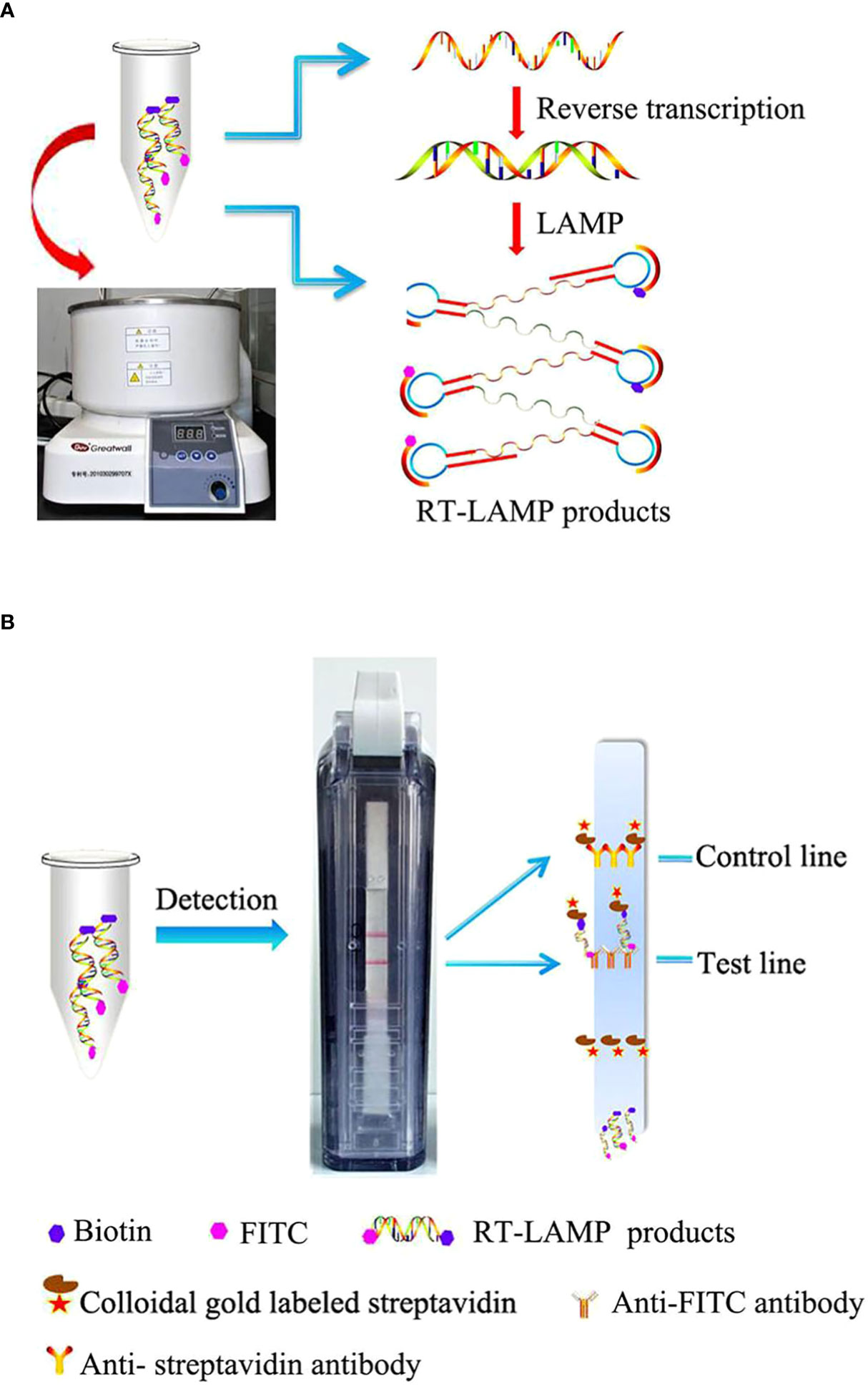
Figure 2 Schematic illustration of the reverse transcription loop-mediated isothermal amplification and vertical flow visualization (RT-LAMP-VF) assay. Figure adopted from Huang et al. (2018). (A) RT-LAMP was executed in a water bath at a constant temperature. (B) The products of RT-LAMP were detected by using a vertical flow visualization strip.
Enzyme-linked immunosorbent assay
The enzyme-linked immunosorbent assay (ELISA) technique utilizes enzyme immunoassay to detect the ligand in a liquid sample. The antibodies are used against the antigen (Alhajj and Farhana, 2022). This is commonly used in molecular research and diagnostics due to its high reproducibility and specificity, but the traditional ELISA has shown a lot of drawbacks, including difficult procedures, long assay time (4–6 h), and the high volume of reagent used (100 μl). These limitations affect its application in clinical diagnosis (Tan et al., 2017). However, these downsides can be overcome by combining ELISA with microfluidic technology (microfluidic-assisted ELISA). The sample volume can be reduced by almost 20-fold with the capillary force, which pulls the reagent into the reaction chamber during the immunoassay. This results in an overall reagent saving of 5- to 10-fold (Ghosh et al., 2020). The total assay time can also be lessened by 50%, which reduces the inclusive cost of labor (Thaitrong et al., 2013).
Coronavirus disease modeling using microfluidics
Microfluidic technology is based on the complexity of multicellular and cellular interactions on a microscopic scale, the reproduction of biochemical forces via tissue engineering, and the use of organ-on-a-chip technology (Li et al., 2012). The recreation of the cellular microenvironment is the biggest challenge in tissue engineering for CoV research (Prakash et al., 2012). Devices related to microfluidics have been successfully used so that cell-based virus assays could be developed (Berkenbrock et al., 2020b). Tissue engineering related to microfluidics can assist in elucidating the entry mechanism of CoV and the persistence of infection in cells. Antiviral drug discovery can also be accelerated in this manner (Tatara, 2020).
At present, public health communities around the world have been challenged by the high infection and death rates of the recently emerged COVID-19. Such scenarios require the infection to be diagnosed accurately and rapidly in order to control the COVID-19 epidemic, which is only possible with the use of molecular-based diagnostics coupled with microfluidics. Microfluidics can improve diagnostic methods so that a cost-effective and efficient strategy is developed (Udugama et al., 2020). RT-LAMP is an amplification technique for nucleic acids commonly utilized for clinical samples for the effective detection of SARS-CoV-2 (point-of-care) (Mautner et al., 2020). The field of microfluidics can be an alternative to time-consuming benchtop assays. Microfluidic devices capable of manipulating minute amounts of fluids and extracting information from them have been enabled by microelectronics and micro-electromechanical systems (MEMS) (Manz et al., 1990; Prakash et al., 2012).
Recently, a lot of microdevices have been devised to detect small-sized pathogens such as viruses (Yang and Yamamoto, 2016; Zhu et al., 2020). The WHO has recommended the use of qPCR with the combination of RT-PCR (qRT-PCR) for the detection of COVID-19 (Corman et al., 2020). The LAMP technique has now become a commonly used method for molecular diagnostics in benchtop assays, which are based on somewhat constant temperatures for amplification, i.e., between 60°C and 65°C (Notomi et al., 2000). This is compatible with the technique of reverse transcriptase for SARS-CoV-2 benchtop assay (Hong et al., 2004). The benchtop assay based on RT-LAMP showed similar positive results to the RT-qPCR-based protocol in the diagnosis of COVID-19, which was approved by the WHO (Lu et al., 2020). This new protocol can be used for home testing, being applied to microdevices with less risk of spreading the virus. Therefore, the LAMP protocol is now considered a simple method with enormous advantages to be used in microfluidic devices for testing (Nguyen et al., 2020).
The devices related to microfluidics utilize serological testing to detect Zika virus (ZIKV), Middle East respiratory syndrome coronavirus (MERS-CoV), and dengue virus (DENV). Hence, it is also a promising approach to detecting SARS-CoV-2 (Berkenbrock et al., 2020a). These devices are much more efficient and have taken advantage of well-established benchtop assays (implemented in macroscopic analysis tools rather than in miniaturized portable devices), which have been adapted to miniaturized lab-on-a-chip versions. One of the reasons for this is the recent concentrated research effort on SARS-CoV-2, which has unveiled its genetic material, proteins, and other molecules that form the virus, as well as the memory antibodies for the disease (Berkenbrock et al., 2020a; Cao et al., 2020; Li et al., 2020; Turoňová et al., 2020).
Recent advancements
National wastewater surveillance systems were introduced and implemented worldwide following the COVID-19 pandemic to better understand the extent of SARS-CoV-2 infection in communities. The quantitative monitoring of SARS-Cov-2 within raw sewage can be a good indicator of the progression of viral circulation in a population (Bonanno Ferraro et al., 2021). The Centers for Disease Control and Prevention (CDC) has provided many predictions using various models to estimate future deaths from COVID-19 (Wang et al., 2022). Nevertheless, several advanced techniques have been developed so that the population-level spread of the disease can be tracked statistically (Salathe et al., 2012). Some models have revealed predictive analytic expertise for deaths and hospitalizations (Kissler et al., 2020). Various research groups have been working on wastewater surveillance for COVID-19, including sewage monitoring with the SARS2-EWSP (SARS-CoV-2 Early Warning Wastewater Surveillance Platform), Utah State, in New York (Utah DEQ, 2022) and Tempe, Arizona’s COVID-19 Wastewater Dashboard (Tempe, 2021). The study by Peccia et al. concluded that delaying the sample processing of sludge can wear away the potential of this approach (Larsen and Wigginton, 2020). Recent findings from Bangladesh displayed many contamination results in surface water due to failure in fecal sludge management services (Amin et al., 2020). Risks of waterborne infections can increase due to viral contamination by groundwater recharge in rural areas (Organization, W. H and UniCeF, 2010). On the other hand, various wastewater studies from high-income areas with good sanitation systems are also well-documented (Ahmed et al., 2021; Ahmed et al., 2020b; Kumar et al., 2020; Medema et al., 2020; Randazzo et al., 2020; Rimoldi et al., 2020; Wurtzer et al., 2020a). A significant positive correlation was found between new COVID-19 cases and the viral load in wastewater, suggesting the potential value of clinical testing and wastewater data monitoring in cities (Hoar et al., 2022). Nevertheless, there is still inadequate progress made in the use of wastewater surveillance to monitor the trends in COVID-19, regardless of the applications of several techniques (Bivins et al., 2020; Zahedi et al., 2021). A systematic review reported the detection of almost 7,644 (29.2%) positive samples out of a total of 26,197 samples collected from 66 studies (Shah et al., 2022). Similarly, there are reports of wastewater surveillance done in different settings, including colleges, hospitals, nursing homes, and dormitories (Gonçalves et al., 2022). Reports from the USA showed high consistency between the results of clinical testing of COVID-19 and wastewater samples collected from college residents (Colosi et al., 2021). The viral RNA detected in wastewater from Arizona University campus was directed for specific clinical testing to isolate infected individuals. Eventually, positive wastewater samples provided an early warning of the presence of infection, preventing possible disease transmission (Betancourt et al., 2021). Another study from a university in the US identified asymptomatic COVID-19 cases that were not detected clinically (Gibas et al., 2021). A lot of infected cases were detected by following the SARS-CoV-2 presence in sewage in nursing places in Spain within a time frame of 5–19 days (Davó et al., 2021). The hospital wastewater in Slovenia was found to contain SARS-CoV-2 RNA with a low prevalence of COVID-19 reported at the time (José Gonçalves et al., 2021). In Spain, the estimation of a high proportion of active spillers from SARS-CoV-2 RNA in wastewater indicated infection in individuals who are asymptomatic (Chavarria-Miró et al., 2021). Moreover, positive signals at a minimum of 33 times within 3 months were detected in New South Wales, in which raw sewage inflowing accounted for most of the positive cases, followed by treated effluents after filtration, primary sludge, and river samples (Shah et al., 2022). High sample positivity was reported by Peccia et al., which resulted in positive detections in almost 17,661 samples (20.6%) from primary sludge (Peccia et al., 2020).
Discussion
Better management of the pandemic requires the precise and timely identification of individuals with SARS-CoV-2, which relies on using suitable testing in various clinical settings for better clinical decision-making (Daughton, 2020). The RNA level of SARS-CoV-2 in sewage correlated with the prevalence of COVID-19, highlighting the efficacy of sewage surveillance as a monitoring tool (Medema et al., 2020). Although there has been a significantly increase in testing capability in developed countries, obvious disproportions in some countries are still evident, especially in developing regions where there is a lack of testing facilities and clinical infrastructure (Abdullahi et al., 2020; Giri and Rana, 2020). This issue must prompt the WHO to encourage researchers to focus their exertions on developing point-of-care assays for community use (Organization, 2020). Advanced techniques such as isothermal amplification methods (e.g., RT-LAMP) are widely adopted because of their low cost and fast processing time, but a lot of false-positive results have also been reported due to nonspecific amplification. This raises concerns regarding their extensive implementation (Rolando et al., 2020). Modern diagnostics provides core diagnostic solutions to carry out large numbers of tests in a timely manner (Pfefferle et al., 2020). An accurate diagnosis of a disease is vital to containing its spread, as a study has estimated that 80% of positive cases of COVID-19 were originally spread by undetected infections (Peccia et al., 2020). Molecular assays are being developed so that the clinical sensitivity and ease-of-use of diagnostic tests can be enhanced (Chan et al., 2020).
With the official announcement of the COVID-19 pandemic by the WHO on March 11, 2020, millions of lives worldwide have been negatively affected by the virus (Franchi, 2020). The developmental timelines of the COVID-19 vaccines were quite long (Corey et al., 2020), along with the risk of SARS-CoV-2 immunity declining with time (Kissler et al., 2020). Therefore, a powerful, authentic, and simultaneous indicator can assist in timely interventions for public health in the case of outbreaks. Various tracking measures have been used for COVID-19, including death rates, confirmed cases, and hospitalizations. However, inefficiencies were discovered in the distribution processes, assembly, and data collection, including reporting delays (Lipsitch and Santillana, 2019). As an acknowledgment to fill such gaps, several recent works have revolved around the potential use of WBE corresponding to clinical testing (Thompson et al., 2020; Venugopal et al., 2020). WBE has been successfully used to track various uses of drugs and other factors. Recently, its popularity has increased in water-related areas with its practical implications via its ability to identify underreported and asymptomatic patients with infectious diseases (Sims and Kasprzyk-Hordern, 2020). The LAMP protocol is now considered a simple method with enormous advantages for use in microfluidic devices for testing. Combined with reverse transcription, it can be a better option for the monitoring of COVID-19 infection through WBE. Nevertheless, its practical applications in the surveillance of COVID-19 have only been associated with clinical testing capacity (Amoah et al., 2021). More research is needed to address its wide application.
Currently, a wide range of clinical examinations and molecular diagnostics for suspected cases can reduce the occurrence of false-negative results; moreover, a detailed and careful examination of the commercial tests must be conducted to identify errors and regulate the efficacy of the approved tests (Sharfstein et al., 2020). These would help in better understanding COVID-19 diagnostics to improve the monitoring of emerging infectious diseases in the future. The development of better laboratory assays should not delay the effort to develop rapid diagnostic tests, even with the low precision during the early phase of the outbreak, as rapid tests can play a significant role in the fight against infection in the initial days while highly efficient assays are in the development stages (Ramdas et al., 2020).
Future remarks
Independent assessments of emerging technologies can provide alternative diagnostic solutions with faster and better screening of individuals with suspected infection. The implementation of targeted, timely, and appropriate testing to reduce the effects of the epidemic is necessary. Generally, epidemic testing is hampered by the lack of options other than test applications for clinical diagnosis and the detection of infections. The wastewater surveillance approach needs to frequently increase the number of populations tested until the key level of detection of positive cases is achieved in order to directly indicate the infection spread in a community. Hence, more rigorous testing is needed to increase the ratio significantly. Nevertheless, diagnostic tests were never intended for mass surveillance. These tests are not only time-consuming and costly, but they can also pose serious exposure risks to those who administer them. Alternatives should be used to solve the problem of diagnostic tests being costly and time-consuming by increasing the quantity of conventional diagnostic tests and minimizing the number of tests mandatory to determine a positive case. Utilizing WBE as an early warning system can reduce the high demand for diagnostic testing by supplementing shortages in the supply chain due to limited manufacturing capacity. The usefulness of WBE can be extended by targeting endogenous biomarkers that were significantly increased during the disease outbreak. However, more research is needed with regard to virus detection in wastewater corresponding to its transmission by highlighting diverse geographic areas and collections from wastewater facilities. A comparison of sludge and inflow from the same wastewater plants can help in determining which approach is more sensitive to decrease the case numbers in a population.
Conclusion
Viral surveillance can be an impartial method for evaluating the viral spread in different regions, even when there is a limitation of resources and diagnosis. These surveillance strategies can be used as “early warning” systems. Studies have pointed out the need for additional strategies and molecular-based assays to validate the presence of enveloped viruses in water, which will increase the accuracy of wastewater surveillance.
Author contributions
SM and lH wrote the original draft, GY, AMA, SS, and SC contributed to revision,and fund acquisition. All the authors read the manuscript and participated in the review, editing and agreed to the published version of the manuscript.
Funding
GY is grateful to the Alexander - von - Humboldt Foundation (AVH) for granting the Return Fellowship EGY 1194703 GF - P.
Conflict of interest
The authors declare that the research was conducted in the absence of any commercial or financial relationships that could be construed as a potential conflict of interest.
Publisher’s note
All claims expressed in this article are solely those of the authors and do not necessarily represent those of their affiliated organizations, or those of the publisher, the editors and the reviewers. Any product that may be evaluated in this article, or claim that may be made by its manufacturer, is not guaranteed or endorsed by the publisher.
References
Abdullahi, I. N., Emeribe, A. U., Akande, A. O., Ghamba, P. E., Adekola, H. A., Ibrahim, Y., et al. (2020). Roles and challenges of coordinated public health laboratory response against COVID-19 pandemic in Africa. J. Infect. Dev. Ctries. 14 (07), 691–695. doi: 10.3855/jidc.12813
Afzal, A. (2020). Molecular diagnostic technologies for COVID-19: Limitations and challenges. J. Adv. Res. 26, 149–159. doi: 10.1016/j.jare.2020.08.002.
Agrawal, S., Orschler, L., Lackner, S. (2021). Long-term monitoring of SARS-CoV-2 RNA in wastewater of the Frankfurt metropolitan area in southern Germany. Sci. Rep. 11 (1), 5372. doi: 10.1038/s41598-021-84914-2
Ahmed, W., Angel, N., Edson, J., Bibby, K., Bivins, A., O'Brien, J. W., et al. (2020a). First confirmed detection of SARS-CoV-2 in untreated wastewater in Australia: a proof of concept for the wastewater surveillance of COVID-19 in the community. Sci. Total Environ. 728, 138764. Doi: 10.1016/j.scitotenv.2020.138764
Ahmed, W., Bertsch, P. M., Bivins, A., Bibby, K., Gathercole, A., Haramoto, E., et al. (2020b). Comparison of virus concentration methods for the RT-qPCR-based recovery of murine hepatitis virus, a surrogate for SARS-CoV-2 from untreated wastewater. Sci. Total Environ. 739, 139960. doi: 10.1016/j.scitotenv.2020.139960.
Ahmed, F., Islam, M. A., Kumar, M., Hossain, M., Bhattacharya, P., Islam, M. T., et al. (2021). First detection of SARS-CoV-2 genetic material in the vicinity of COVID-19 isolation centre in Bangladesh: Variation along the sewer network. Sci. Total Environ. 776, 145724. doi: 10.1016/j.scitotenv.2021.145724
Ahmed, W., Simpson, S. L., Bertsch, P. M., Bibby, K., Bivins, A., Blackall, L. L., et al. (2022). Minimizing errors in RT-PCR detection and quantification of SARS-CoV-2 RNA for wastewater surveillance. Sci. Total Environ. 805, 149877. doi: 10.1016/j.scitotenv.2021.149877
Alhajj, M., Farhana, A. (2022). “Enzyme linked immunosorbent assay,” in StatPearls (Treasure Island (FL: StatPearls Publishing LLC).
Allen, D., Krein, J., Sitaraman, G., Weyl, E. G., The Rockefeller Foundation (2020) National covid-19 testing action plan: pragmatic steps to reopen our workplaces and our communities. Available at: https://www.rockefellerfoundation.org/wp-content/uploads/2020/04/TheRockefellerFoundation_WhitePaper_Covid19_4_21_2020.pdf.
Amin, N., Liu, P., Foster, T., Rahman, M., Miah, M. R., Ahmed, G. B., et al. (2020). Pathogen flows from on-site sanitation systems in low-income urban neighborhoods, Dhaka: A quantitative environmental assessment. Int. J. Hyg. Environ. Health 230, 113619. doi: 10.1016/j.ijheh.2020.113619
Amoah, I., Mthethwa, N., Pillasy, L., Deepnarain, N., Pillay, K., Awolusi, O., et al. (2021). RTLAMP: A CheBentham science publisher aper, simpler and faster alternative for the detection of SARS-CoV-2 in wastewater. Food Environ. Virol. 13, 1–10. doi: 10.1007/s12560-02109489-7
Ampuero, M., Valenzuela, S., Valiente-Echeverría, F., Soto-Rifo, R., Barriga, G. P., Chnaiderman, J., et al. (2020). SARS-CoV-2 detection in sewage in Santiago, Chile - preliminary results. medRxiv, 2020.2007.2002.20145177. doi: 10.1101/2020.07.02.20145177
Asghar, H., Diop, O. M., Weldegebriel, G., Malik, F., Shetty, S., El Bassioni, L., et al. (2014). Environmental surveillance for polioviruses in the global polio eradication initiative. J. Infect. dis. 210 (suppl_1), S294–S303. doi: 10.1093/infdis/jiu384
Arora, S., Nag, A., Kalra, A., Sinha, V., Meena, E., Saxena, S., et al. (2022). Successful application of wastewater-based epidemiology in prediction and monitoring of the second wave of COVID-19 with fragmented sewerage systems-a case study of Jaipur (India). Environ. Monit Assess. 194 (5), 342. doi: 10.1007/s10661-022-09942-5
Aw, T. G., Gin, K. Y.-H., Ean Oon, L. L., Chen, E. X., Woo, C. H. (2009). Prevalence and genotypes of human noroviruses in tropical urban surface waters and clinical samples in Singapore. Appl. Environ. Microbiol. 75 (15), 4984–4992. doi: 10.1128/AEM.00489-09.
Bachelet, V. C. (2020). Do we know the diagnostic properties of the tests used in COVID-19? a rapid review of recently published literature. Medwave 20 (3), e7890. doi: 10.5867/medwave.2020.03.7891.
Bar-Or, I., Yaniv, K., Shagan, M., Ozer, E., Weil, M., Indenbaum, V., et al. (2021). Regressing SARS-CoV-2 sewage measurements onto COVID-19 burden in the population: A proof-of-Concept for quantitative environmental surveillance. Front. Public Health 9. doi: 10.3389/fpubh.2021.561710
Barril, P. A., Pianciola, L. A., Mazzeo, M., Ousset, M. J., Jaureguiberry, M. V., Alessandrello, M., et al. (2021). Evaluation of viral concentration methods for SARS-CoV-2 recovery from wastewaters. Sci. Total Environ. 756, 144105. doi: 10.1016/j.scitotenv.2020.144105.
Basit, A., Ulhaq, I., Hussain, F., Din, Z. U., Rahim, K. (2021). “Nucleic acid based detection of COVID-19,” in Coronavirus disease-19 (COVID-19): A perspective of new scenario COVID-19: Epidemiology, biochemistry, and diagnostics, vol. 405-419. (Netherlands | United Arab Emirates: Bentham Science Publisher). doi: 10.2174/9789814998932121010018
Berkenbrock, J. A., Grecco-Machado, R., Achenbach, S. (2020a). Arsenal of microfluidic testing devices may combat COVID-19 pandemic. Mrs Bulletin. 45 (7), 511–514. doi: 10.1557/mrs.2020.181.
Berkenbrock, J. A., Grecco-Machado, R., Achenbach, S. (2020b). Microfluidic devices for the detection of viruses: aspects of emergency fabrication during the COVID-19 pandemic and other outbreaks. Proc. R. Soc. A: Math. Phys. Eng. Sci. 476 (2243), 20200398. doi: 10.1098/rspa.2020.0398
Betancourt, W. Q., Schmitz, B. W., Innes, G. K., Prasek, S. M., Brown, K. M. P., Stark, E. R., et al. (2021). COVID-19 containment on a college campus via wastewater-based epidemiology, targeted clinical testing and an intervention. Sci. Total Environ. 779, 146408. doi: 10.1016/j.scitotenv.2021.146408
Bibby, K., Crank, K., Greaves, J., Li, X., Wu, Z., Hamza, I. A., et al. (2019). Metagenomics and the development of viral water quality tools. NPJ Clean Water. 2 (1), 1–13. doi: 10.1038/s41545-019-0032-3
Bibby, K., Peccia, J. (2013). Identification of viral pathogen diversity in sewage sludge by metagenome analysis. Environ. Sci. technol. 47 (4), 1945–1951. doi: 10.1021/es305181x
Biosciences, S (2020). Instructions for use: SherlockTM CRISPR SARS-CoV-2 kit (Boston, MA 02135, USA: Sherlock Biosciences, Inc.).
BioSystems, A (2020). iAMP COVID-19 detection kit (Mountain View, CA 94043, USA: Atila BioSystems, Inc.).
Bivins, A., North, D., Ahmad, A., Ahmed, W., Alm, E., Been, F., et al. (2020). Wastewater-based epidemiology: global collaborative to maximize contributions in the fight against COVID-19 (Washington, DC: ACS Publications).
Blanco, A., Abid, I., Al-Otaibi, N., Pérez-Rodríguez, F. J., Fuentes, C., Guix, S., et al (2019). Glass wool concentration optimization for the detection of enveloped and nonenveloped waterborne viruses. Food Environ. virol. 11 (2), 184–192. doi: 10.1007/s12560-019-09378-0
Bloom, D. E., Cadarette, D. (2019). Infectious disease threats in the twenty-first century: strengthening the global response. Front. Immunol. 10, 549. doi: 10.3389/fimmu.2019.00549
Bloom, J. S., Sathe, L., Munugala, C., Jones, E. M., Gasperini, M., Lubock, N. B., et al. (2020). Swab-seq: A high-throughput platform for massively scaled up SARS-CoV-2 testing. MedRxiv. doi: 10.1101/2020.08.04.20167874
Bonanno Ferraro, G., Veneri, C., Mancini, P., Iaconelli, M., Suffredini, E., Bonadonna, L., et al. (2021). A state-of-the-Art scoping review on SARS-CoV-2 in sewage focusing on the potential of wastewater surveillance for the monitoring of the COVID-19 pandemic. Food Environ. Virol.14 (4), 315–354. doi: 10.1007/s12560-021-09498-6
Bustin, S. A., Benes, V., Garson, J. A., Hellemans, J., Huggett, J., Kubista, M., et al. (2009). The MIQE guidelines: Minimum information for publication of quantitative real-time PCR experiments (Oxford, United Kingdom: Oxford University Press).
Cai, J., Xu, J., Lin, D. (2020). A case series of children with 2019 novel coronavirus infection: clinical and epidemiological features. Clin. Infect. Dis 71 (6), 1547–1551. doi: 10.1093/cid/ciaa198
Cao, Y., Su, B., Guo, X., Sun, W., Deng, Y., Bao, L., et al. (2020). Potent neutralizing antibodies against SARS-CoV-2 identified by high-throughput single-cell sequencing of convalescent patients’ b cells. Cell 182 (1), 73–84.e16. doi: 10.1016/j.cell.2020.05.025
Carducci, A., Verani, M., Battistini, R., Pizzi, F., Rovini, E., Andreoli, E., et al. (2006). Epidemiological surveillance of human enteric viruses by monitoring of different environmental matrices. Water Sci. Technol. 54 (3), 239–244. doi: 10.2166/wst.2006.475
Carter, L. J., Garner, L. V., Smoot, J. W., Li, Y., Zhou, Q., Saveson, C. J., et al. (2020). Assay techniques and test development for COVID-19 diagnosis (Washington, DC: ACS Publications).
Castro-Gutierrez, V., Hassard, F., Vu, M., Leitao, R., Burczynska, B., Wildeboer, D., et al. (2022). Monitoring occurrence of SARS-CoV-2 in school populations: a wastewater-based approach. PloS One 17 (6), e0270168. doi: 10.1371/journal.pone.0270168
CDC (2022). “Public health interpretation and use of wastewater surveillance data,” in Overview of wastewater surveillance data interpretation and use (1600 Clifton Road Atlanta, GA 30329-4027 USA: Centers for Disease Control and Prevention).
Cervantes-Aviles, P., Moreno-Andrade, I., Carrillo-Reyes, J. (2021). Approaches applied to detect SARS-CoV-2 in wastewater and perspectives post-COVID-19. J. Water Process Eng. 40, 101947. doi: 10.1016/j.jwpe.2021.101947
Cevik, M., Tate, M., Lloyd, O., Maraolo, A. E., Schafers, J., Ho, A. (2021). SARS-CoV-2, SARS-CoV, and MERS-CoV viral load dynamics, duration of viral shedding, and infectiousness: a systematic review and meta-analysis. Lancet Microbe 2 (1), e13–e22. doi: 10.1016/S2666-5247(20)30172-5
Chan, J. F.-W., Yip, C. C.-Y., To, K. K.-W., Tang, T. H.-C., Wong, S. C.-Y., Leung, K.-H., et al. (2020). Improved molecular diagnosis of COVID-19 by the novel, highly sensitive and specific COVID-19-RdRp/Hel real-time reverse transcription-PCR assay validated in vitro and with clinical specimens. J. Clin. Microbiol. 58 (5), e00310–e00320. doi: 10.1128/JCM.00310-20
Chavarria-Miró, G., Anfruns-Estrada, E., Martínez-Velázquez, A., Vázquez-Portero, M., Guix, S., Paraira, M., et al. (2021). Time evolution of severe acute respiratory syndrome coronavirus 2 (SARS-CoV-2) in wastewater during the first pandemic wave of COVID-19 in the metropolitan area of Barcelona, Spain. Appl. Environ. Microbiol. 87 (7), e02750–e02720. doi: 10.1128/AEM.02750-20
Chen, Y., Chen, L., Deng, Q., Zhang, G., Wu, K., Ni, L., et al. (2020). The presence of SARS-CoV-2 RNA in the feces of COVID-19 patients. J. Med. virol. 92 (7), 833840. doi: 10.1002/jmv.25825
Chen, C., Gao, G., Xu, Y., Pu, L., Wang, Q., Wang, L., et al. (2020). SARS-CoV-2–positive sputum and feces after conversion of pharyngeal samples in patients with COVID-19. Ann. Internal Med. 172 (12), 832–834. doi: 10.7326/M20-0991
Chen, N., Zhou, M., Dong, X., Qu, J., Gong, F., Han, Y., et al. (2020). Epidemiological and clinical characteristics of 99 cases of 2019 novel coronavirus pneumonia in wuhan, China: a descriptive study. Lancet 395 (10223), 507–513. doi: 10.1016/S0140-6736(20)30211-7
Cheung, K. S., Hung, I. F. N., Chan, P. P. Y., Lung, K. C., Tso, E., Liu, R., et al. (2020). Gastrointestinal manifestations of SARS-CoV-2 infection and virus load in fecal samples from a Hong Kong cohort: Systematic review and meta-analysis. Gastroenterology 159 (1), 81–95. doi: 10.1053/j.gastro.2020.03.065
Chik, A. H., Glier, M. B., Servos, M., Mangat, C. S., Pang, X.-L., Qiu, Y., et al. (2021). Comparison of approaches to quantify SARS-CoV-2 in wastewater using RT-qPCR: Results and implications from a collaborative inter-laboratory study in Canada. J. Environ. Sci. 107, 218–229. doi: 10.1016/j.jes.2021.01.029
Choi, P. M., Tscharke, B. J., Donner, E., O'Brien, J. W., Grant, S. C., Kaserzon, S. L., et al. (2018). Wastewater-based epidemiology biomarkers: past, present and future. TrAC Trends Anal. Chem. 105, 453–469. doi: 10.1016/j.trac.2018.06.004
Cho, S.-M., Ha, G. Y. (2020). A case of COVID-19 in a 45-day-old infant with persistent fecal virus shedding for more than 12 weeks. Yonsei Med. J. 61 (10), 901.
Colaneri, M., Seminari, E., Novati, S., Asperges, E., Biscarini, S., Piralla, A., et al. (2020). Severe acute respiratory syndrome coronavirus 2 RNA contamination of inanimate surfaces and virus viability in a health care emergency unit. Clin. Microbiol. Infect. 26 (8), 1094.e1091–e1094. doi: 10.1016/j.cmi.2020.05.009
Colosi, L. M., Barry, K. E., Kotay, S. M., Porter, M. D., Poulter, M. D., Ratliff, C., et al. (2021). Development of wastewater pooled surveillance of severe acute respiratory syndrome coronavirus 2 (SARS-CoV-2) from congregate living settings. Appl. Environ. Microbiol. 87 (13), e00433–e00421. doi: 10.1128/AEM.00433-21
Commission, E (2021). Commission recommendation on a common approach to establish a systematic surveillance of SARS-CoV-2 and its variants in wastewaters in the EU (Luxembourg Europe: Commission Recommendation, Official Journal of the European Union).
Corey, L., Mascola, J. R., Fauci, A. S., Collins, F. S. (2020). A strategic approach to COVID19 vaccine R&D. Science 368 (6494), 948–950. doi: 10.1126/science.abc5312.
Corman, V., Landt, O., Kaiser, M., Molenkamp, R., Meijer, A., Chu, D. (2020). Detection of 2019 novel coronavirus, (2019-nCoV) by real-time RT-PCR. Euro Surveill. 25, 2000045. doi: 10.2807/1560-7917.ES.2020.25.3.2000045
COVID, I, Murray, C. J. (2020). Forecasting COVID-19 impact on hospital bed-days, ICU-days, ventilator-days and deaths by US state in the next 4 months. MedRxiv. doi: 10.1101/2020.03.27.20043752
Daughton, C. G. (2001). Illicit drugs in municipal sewage. Pharm. Care Prod. Environ. 791, 348–364. doi: 10.1021/bk-2001-0791.ch020
Daughton, C. G. (2012). Real-time estimation of small-area populations with human biomarkers in sewage. Sci. Total Environ. 414, 6–21. doi: 10.1016/j.scitotenv.2011.11.015
Daughton, C. G. (2018). Monitoring wastewater for assessing community health: Sewage chemical-information mining (SCIM). Sci. Total Environ. 619, 748–764. doi: 10.1016/j.scitotenv.2017.11.102
Daughton, C. G. (2020). Wastewater surveillance for population-wide covid-19: The present and future. Sci. Total Environ. 736, 139631. doi: 10.1016/j.scitotenv.2020.139631
Davó, L., Seguí, R., Botija, P., Beltrán, M. J., Albert, E., Torres, I., et al. (2021). Early detection of SARS-CoV-2 infection cases or outbreaks at nursing homes by targeted wastewater tracking. Clin. Microbiol. Infect. 27 (7), 1061–1063. doi: 10.1016/j.cmi.2021.02.003
Ding, Y., He, L., Zhang, Q., Huang, Z., Che, X., Hou, J., et al. (2004). Organ distribution of severe acute respiratory syndrome (SARS) associated coronavirus (SARS-CoV) in SARS patients: implications for pathogenesis and virus transmission pathways. J. Pathol. 203 (2), 622630. doi: 10.1002/path.1560
Ding, Z., Qian, H., Xu, B., Huang, Y., Miao, T., Yen, H.-L., et al. (2020). Toilets dominate environmental detection of SARS-CoV-2 virus in a hospital. medRxiv 753, 141710. doi: 10.1101/2020.04.03.20052175
Dupinay, T., Pounder, K. C., Ayral, F., Laaberki, M.-H., Marston, D. A., Lacôte, S., et al. (2014). Detection and genetic characterization of Seoul virus from commensal brown rats in France. Virol. J. 11 (1), 1–9. doi: 10.1186/1743-422X-11-32
Egbuna, C., Chandra, S., Awuchi, C. G., Saklani, S., Ulhaq, I., Akram, M., et al. (2022). “Myth surrounding the FDA disapproval of hydroxychloroquine sulfate and chloroquine phosphate as drugs for coronavirus disease 2019,” in Coronavirus drug discovery (Netherlands: Elsevier), 153168.
Feng, L., Zhang, W., Li, X. (2018). Monitoring of regional drug abuse through wastewater-based epidemiology–a critical review. Sci. China Earth Sci. 61 (3), 239–255. doi: 10.1007/s11430-017-9129-x
Fernandez-Cassi, X., Timoneda, N., Martínez-Puchol, S., Rusiñol, M., Rodriguez-Manzano, J., Figuerola, N., et al. (2018). Metagenomics for the study of viruses in urban sewage as a tool for public health surveillance. Sci. Total Environ. 618, 870–880. doi: 10.1016/j.scitotenv.2017.08.249
Fernández-de-Mera, I. G., Rodríguez del-Río, F. J., de la Fuente, J., Pérez-Sancho, M., Hervás, D., Moreno, I., et al. (2021). Detection of environmental SARS-CoV-2 RNA in a high prevalence setting in Spain. Transboundary Emerging Dis. 68 (3), 1487–1492. doi: 10.1111/tbed.13817
Foladori, P., Cutrupi, F., Segata, N., Manara, S., Pinto, F., Malpei, F., et al. (2020). SARSCoV-2 from faeces to wastewater treatment: What do we know? A review. Sci. Total Environ. 743, 140444. doi: 10.1016/j.scitotenv.2020.140444
Fongaro, G., Stoco, P. H., Souza, D. S. M., Grisard, E. C., Magri, M. E., Rogovski, P., et al. (2021). The presence of SARS-CoV-2 RNA in human sewage in Santa catarina, Brazil, November 2019. Sci. Total Environ. 778, 146198. doi: 10.1016/j.scitotenv.2021.146198
Franchi, T. (2020). The impact of the covid-19 pandemic on current anatomy education and future careers: A student's perspective. Anat Sci. Educ. 13 (3), 312–315. doi: 10.1002/ase.1966
Francois, P., Tangomo, M., Hibbs, J., Bonetti, E. J., Boehme, C. C., Notomi, T., et al. (2011). Robustness of a loop-mediated isothermal amplification reaction for diagnostic applications. FEMS Immunol. Med. Microbiol. 62 (1), 41–48. doi: 10.1111/j.1574-695X.2011.00785.x
Gandhi, M., Yokoe, D. S., Havlir, D. V. (2020). Asymptomatic transmission, the achilles’ heel of current strategies to control covid-19 Vol. 382 (United States: Mass Medical Soc), 2158–2160.
Gao, Q. Y., Chen, Y. X., Fang, J. Y. (2020). 2019 novel coronavirus infection and gastrointestinal tract. J. dig. dis. 21 (3), 125. doi: 10.1111/1751-2980.12851
Ghosh, S., Aggarwal, K., Vinitha, T. U., Nguyen, T., Han, J., Ahn, C. H. (2020). A new microchannel capillary flow assay (MCFA) platform with lyophilized chemiluminescence reagents for a smartphone-based POCT detecting malaria. Microsyst Nanoeng. 6, 5. doi: 10.1038/s41378-019-0108-8
Gibas, C., Lambirth, K., Mittal, N., Juel, M. A. I., Barua, V. B., Brazell, L. R., et al. (2021). Implementing building-level SARS-CoV-2 wastewater surveillance on a university campus. Sci. Total Environ. 782, 146749. doi: 10.1016/j.scitotenv.2021.146749
Giri, A. K., Rana, D. R. (2020). Charting the challenges behind the testing of COVID-19 in developing countries: Nepal as a case study. Chin. Med. Journals Publ. House Co. 2, 53–56. doi: 10.1016/j.bsheal.2020.05.002
Goh, G. K.-M., Dunker, A. K., Uversky, V. (2013). Prediction of intrinsic disorder in MERSCoV/HCoV-EMC supports a high oral-fecal transmission. PloS curr. 5. doi: 10.1371/currents.outbreaks.22254b58675cdebc256dbe3c5aa6498b
Gonçalves, J., Koritnik, T., Mioč, V., Trkov, M., Bolješič, M., Berginc, N., et al. (2021). Detection of SARS-CoV-2 RNA in hospital wastewater from a low COVID-19 disease prevalence area. Sci. Total Environ. 755, 143226. doi: 10.1016/j.scitotenv.2020.143226
Gonçalves, J., Torres-Franco, A., Rodriguéz, E., Diaz, I., Koritnik, T., Silva, P. G. D., et al. (2022). Centralized and decentralized wastewater-based epidemiology to infer COVID-19 transmission - a brief review. One Health 15, 100405. doi: 10.1016/j.onehlt.2022.100405
Graham, K. E., Loeb, S. K., Wolfe, M. K., Catoe, D., Sinnott-Armstrong, N., Kim, S., et al. (2020). SARS-CoV-2 RNA in wastewater settled solids is associated with COVID-19 cases in a large urban sewershed. Environ. Sci. technol. 55 (1), 488–498. doi: 10.1021/acs.est.0c06191
Guerrero-Latorre, L., Ballesteros, I., Villacrés, I. M., Granda, M. G., Freire, B. P., Ríos-Touma, B. (2020). First SARS-CoV-2 detection in river water: implications in low sanitation countries. medRxiv 743, 140832. doi: 10.1101/2020.06.14.20131201
Gu, J., Han, B., Wang, J. (2020). COVID-19: Gastrointestinal manifestations and potential fecal-oral transmission. Gastroenterology 158 (6), 1518–1519. doi: 10.1053/j.gastro.2020.02.054
Hall, R. J., Wang, J., Todd, A. K., Bissielo, A. B., Yen, S., Strydom, H., et al. (2014). Evaluation of rapid and simple techniques for the enrichment of viruses prior to metagenomic virus discovery. J. virol. Methods 195, 194–204. doi: 10.1016/j.jviromet.2013.08.035
Haramoto, E., Kitajima, M., Katayama, H., Ito, T., Ohgaki, S. (2009). Development of virus concentration methods for detection of koi herpesvirus in water. J. fish dis. 32 (3), 297–300. doi: 10.1111/j.1365-2761.2008.00977.x
Hata, A., Honda, R. (2020). Potential sensitivity of wastewater monitoring for SARS-CoV-2: comparison with norovirus cases (Washington, DC: ACS Publications).
Heller, L., Mota, C. R., Greco, D. B. (2020). COVID-19 faecal-oral transmission: Are we asking the right questions? Sci. Total Environ. 729, 138919. doi: 10.1016/j.scitotenv.2020.138919
Hellmér, M., Paxéus, N., Magnius, L., Enache, L., Arnholm, B., Johansson, A., et al. (2014). Detection of pathogenic viruses in sewage provided early warnings of hepatitis a virus and norovirus outbreaks. Appl. Environ. Microbiol. 80 (21), 67716781. doi: 10.1128/AEM.01981-14
Hillary, L. S., Farkas, K., Maher, K. H., Lucaci, A., Thorpe, J., Distaso, M. A., et al. (2021). Monitoring SARS-CoV-2 in municipal wastewater to evaluate the success of lockdown measures for controlling COVID-19 in the UK. Water Res. 200, 117214. doi: 10.1016/j.watres.2021.117214
Hoar, C., Chauvin, F., Clare, A., McGibbon, H., Castro, E., Patinella, S., et al. (2022). Monitoring SARS-CoV-2 in wastewater during new York city's second wave of COVID-19: sewershed-level trends and relationships to publicly available clinical testing data. Environ. Sci.: Water Res. Technol. 8 (5), 1021–1035. doi: 10.1039/D1EW00747E
Hoehl, S., Kreutzer, E., Schenk, B., Westhaus, S., Foppa, I., Herrmann, E., et al. (2021). Longitudinal testing for respiratory and gastrointestinal shedding of severe acute respiratory syndrome coronavirus 2 (SARS-CoV-2) in day care centers in hesse, Germany. Clin. Infect. Dis. 73 (9), e3036–e3041. doi: 10.1093/cid/ciaa1912
Holshue, M. L., DeBolt, C., Lindquist, S., Lofy, K. H., Wiesman, J., Bruce, H., et al. (2020). First case of 2019 novel coronavirus in the united states. New Engl. J. Med 382 (10), 929–936. doi: 10.1056/NEJMoa2001191
Hong, T. C., Mai, Q. L., Cuong, D. V., Parida, M., Minekawa, H., Notomi, T., et al. (2004). Development and evaluation of a novel loop-mediated isothermal amplification method for rapid detection of severe acute respiratory syndrome coronavirus. J Clin Microbiol. 42 (5), 1956–61. doi: 10.1128/JCM.42.5.1956-1961.2004
Hovi, T., Shulman, L., van der Avoort, H., Deshpande, J., Roivainen, M., De Gourville, E. (2012). Role of environmental poliovirus surveillance in global polio eradication and beyond. Epidemiol. Infect. 140 (1), 1–13. doi: 10.1017/S095026881000316X
Huang, P., Wang, H., Cao, Z., Jin, H., Chi, H., Zhao, J., et al. (2018). A rapid and specific assay for the detection of MERS-CoV. Front. Microbiol. 9, 1101. doi: 10.3389/fmicb.2018.01101
Huang, C., Wang, Y., Li, X., Ren, L., Zhao, J., Hu, Y., et al. (2020). Clinical features of patients infected with 2019 novel coronavirus in wuhan, China. Lancet 395 (10223), 497–506. doi: 10.1016/S0140-6736(20)30183-5
Hung, I., Cheng, V., Wu, A., Tang, B., Chan, K., Chu, C., et al. (2004). Viral loads in clinical specimens and SARS manifestations. Emerg. Infect. dis. 10 (9), 1550. doi: 10.3201/eid1009.040058
Ihtisham, U., Basit, A., Ali, I., Hussain, F., Ali, Z., Siddique, F., et al. (2021). “Epidemiology, coronavirus disease-19 (COVID-19): A perspective of new scenario,” in COVID- 19: Epidemiology, biochemistry, and diagnostics, vol. 41. (Netherlands | United Arab Emirates: Bentham Science Publisher), 1–41. doi: 10.2174/9789814998932121010004
Iglesias, N. G., Gebhard, L. G., Carballeda, J. M., Aiello, I., Recalde, E., Terny, G., et al. (2021). SARS-CoV-2 surveillance in untreated wastewater: detection of viral RNA in a low-resource community in Buenos Aires, Argentina. Rev. Panam Salud Publica 45, e137. doi: 10.26633/rpsp.2021.137
Ishii, S., Nakamura, T., Ozawa, S., Kobayashi, A., Sano, D., Okabe, S. (2014). Water quality monitoring and risk assessment by simultaneous multipathogen quantification. Environ. Sci. Technol. 48 (9), 4744–4749. doi: 10.1021/es500578s
Izquierdo-Lara, R., Elsinga, G., Heijnen, L., Munnink, B. B. O., Schapendonk, C. M., Nieuwenhuijse, D., et al. (2021). Monitoring SARS-CoV-2 circulation and diversity through community wastewater sequencing, the Netherlands and Belgium. Emerg. Infect. Dis. 27 (5), 1405. doi: 10.3201/eid2705.204410
Jakariya, M., Ahmed, F., Islam, M. A., Ahmed, T., Al Marzan, A., Hossain, M., et al. (2021). Wastewater based surveillance system to detect SARS-CoV-2 genetic material for countries with on-site sanitation facilities: an experience from Bangladesh. MedRXiv 311, 119679. doi: 10.1101/2021.07.30.21261347
Jamiruddin, M. R., Meghla, B. A., Islam, D. Z., Tisha, T. A., Khandker, S. S., Khondoker, M. U., et al. (2022). Microfluidics technology in SARS-CoV-2 diagnosis and beyond: A systematic review. Life (Basel) 12 (5), 649. doi: 10.3390/life12050649
Jiang, X., Rayner, S., Luo, M. H. (2020). Does SARS-CoV-2 have a longer incubation period than SARS and MERS. J. Med. virol. 92 (5), 476–478. doi: 10.1002/jmv.25708
Jiehao, C., Jin, X., Daojiong, L., Zhi, Y., Lei, X., Zhenghai, Q., et al. (2020). A case series of children with 2019 novel coronavirus infection: clinical and epidemiological features. Clin. Infect. Dis. 71 (6), 1547–1551. doi: 10.1093/cid/ciaa198
Johansson, M. A., Vasconcelos, P. F., Staples, J. E. (2014). The whole iceberg: estimating the incidence of yellow fever virus infection from the number of severe cases. Trans. R. Soc. Trop. Med. Hyg. 108 (8), 482–487. doi: 10.1093/trstmh/tru092
Jones, D. L., Baluja, M. Q., Graham, D. W., Corbishley, A., McDonald, J. E., Malham, S. K., et al. (2020). Shedding of SARS-CoV-2 in feces and urine and its potential role in person-to-person transmission and the environment-based spread of COVID-19. Sci. Total Environ. 749, 141364. doi: 10.1016/j.scitotenv.2020.141364
Kantor, R. S., Nelson, K. L., Greenwald, H. D., Kennedy, L. C. (2021). Challenges in measuring the recovery of SARS-CoV-2 from wastewater. Environ. Sci. technol. 55 (6), 3514–3519. doi: 10.1021/acs.est.0c08210
Kataki, S., Chatterjee, S., Vairale, M. G., Sharma, S., Dwivedi, S. K. (2021). Concerns and strategies for wastewater treatment during COVID-19 pandemic to stop plausible transmission. Resour Conserv. Recycl. 164, 105156. doi: 10.1016/j.resconrec.2020.105156
Kissler, S. M., Tedijanto, C., Goldstein, E., Grad, Y. H., Lipsitch, M. (2020). Projecting the transmission dynamics of SARS-CoV-2 through the postpandemic period. Science 368 (6493), 860–868. doi: 10.1126/science.abb5793
Kitajima, M., Ahmed, W., Bibby, K., Carducci, A., Gerba, C. P., Hamilton, K. A., et al. (2020). SARS-CoV-2 in wastewater: State of the knowledge and research needs. Sci. Total Environ. 739, 139076. doi: 10.1016/j.scitotenv.2020.139076.
Kobayashi, N., Oshiki, M., Ito, T., Segawa, T., Hatamoto, M., Kato, T., et al. (2017). Removal of human pathogenic viruses in a down-flow hanging sponge (DHS) reactor treating municipal wastewater and health risks associated with utilization of the effluent for agricultural irrigation. Water Res. 110, 389–398. doi: 10.1016/j.watres.2016.10.054.
Kujawski, S. A., Wong, K. K., Collins, J. P., Epstein, L., Killerby, M. E., Midgley, C. M., et al. (2020). Clinical and virologic characteristics of the first 12 patients with coronavirus disease 2019 (COVID-19) in the united states. Nat. Med. 26 (6), 861–868. doi: 10.1038/s41591-020-0877-5
Kumar, M., Patel, A. K., Shah, A. V., Raval, J., Rajpara, N., Joshi, M., et al. (2020). First proof of the capability of wastewater surveillance for COVID-19 in India through detection of genetic material of SARS-CoV-2. Sci. Total Environ. 746, 141326. doi: 10.1016/j.scitotenv.2020.141326
Lamb, L. E., Bartolone, S. N., Ward, E., Chancellor, M. B. (2020). Rapid detection of novel coronavirus (COVID-19) by reverse transcription-loop-mediated isothermal amplification. PLoS One 15 (6), e0234682. doi: 10.1371/journal.pone.0234682
La Rosa, G., Della Libera, S., Iaconelli, M., Ciccaglione, A. R., Bruni, R., Taffon, S., et al. (2014). Surveillance of hepatitis a virus in urban sewages and comparison with cases notified in the course of an outbreak, Italy 2013. BMC Infect. Dis. 14 (1), 1–11. doi: 10.1186/1471-2334-14-419.
La Rosa, G., Iaconelli, M., Mancini, P., Ferraro, G. B., Veneri, C., Bonadonna, L., et al. (2020). First detection of SARS-CoV-2 in untreated wastewaters in Italy. Sci. Total Environ. 736, 139652. doi: 10.1016/j.scitotenv.2020.139652
La Rosa, G., Muscillo, M. (2013). “Molecular detection of viruses in water and sewage,” in Viruses in food and water (Netherlands: Elsevier), 97–125.
Larsen, D. A., Wigginton, K. R. (2020). Tracking COVID-19 with wastewater. Nat. Biotechnol. 38 (10), 1151–1153. doi: 10.1038/s41587-020-0690-1
Le Guyader, F., Dubois, E., Menard, D., Pommepuy, M. (1994). Detection of hepatitis a virus, rotavirus, and enterovirus in naturally contaminated shellfish and sediment by reverse transcription-seminested PCR. Appl. Environ. Microbiol. 60 (10), 3665–3671. doi: 10.1128/aem.60.10.3665-3671.1994
Lescure, F.-X., Bouadma, L., Nguyen, D., Parisey, M., Wicky, P.-H., Behillil, S., et al. (2020). Clinical and virological data of the first cases of COVID-19 in Europe: A case series. Lancet Infect. Dis. 20 (6), 697–706. doi: 10.1016/S1473-3099(20)30200-0
Leung, W. K., To, K.-F., Chan, P. K., Chan, H. L., Wu, A. K., Lee, N., et al. (2003). Enteric involvement of severe acute respiratory syndrome-associated coronavirus infection. Gastroenterology 125 (4), 1011–1017. doi: 10.1016/j.gastro.2003.08.001
Ling, Y., Xu, S. B., Lin, Y. X., Tian, D., Zhu, Z. Q., Dai, F. H., et al. (2020). Persistence and clearance of viral RNA in 2019 novel coronavirus disease rehabilitation patients. Chin. Med. J. 133 (09), 1039–1043. doi: 10.1097/CM9.0000000000000774
Lipsitch, M., Santillana, M. (2019). Enhancing situational awareness to prevent infectious disease outbreaks from becoming catastrophic. Global Catastrophic Biol. Risks. 424, 59–74. doi: 10.1007/82_2019_172
Li, X. J., Valadez, A. V., Zuo, P., Nie, Z. (2012). Microfluidic 3D cell culture: potential application for tissue-based bioassays. Bioanalysis 4 (12), 1509–1525. doi: 10.4155/bio.12.133
Li, Z., Yi, Y., Luo, X., Xiong, N., Liu, Y., Li, S., et al. (2020). Development and clinical application of a rapid IgM-IgG combined antibody test for SARS-CoV-2 infection diagnosis. J. Med. virol. 92 (9), 1518–1524. doi: 10.1002/jmv.25727
Lodder, W., de Roda Husman, A. M. (2020). SARS-CoV-2 in wastewater: potential health risk, but also data source. Lancet Gastroenterol. Hepatol. 5 (6), 533–534. doi: 10.1016/S2468-1253(20)30087-X
Lodder, W. J., Rutjes, S. A., Takumi, K., de Roda Husman, A. M. (2013). Aichi virus in sewage and surface water, the Netherlands. Emerg. Infect. dis. 19 (8), 1222. doi: 10.3201/eid1908.130312
Lu, R., Wu, X., Wan, Z., Li, Y., Zuo, L., Qin, J., et al. (2020). Development of a novel reverse transcription loop-mediated isothermal amplification method for rapid detection of SARS-CoV-2. Virol. Sinica. 35 (3), 344–347. doi: 10.1007/s12250-020-00218-1
Manz, A., Graber, N., Widmer, H. A. (1990). Miniaturized total chemical analysis systems: a novel concept for chemical sensing. Sensors actuators B: Chemical. 1 (1-6), 244–248. doi: 10.1016/0925-4005(90)80209-I
Mao, K., Zhang, K., Du, W., Ali, W., Feng, X., Zhang, H. (2020). The potential of wastewater-based epidemiology as surveillance and early warning of infectious disease outbreaks. Curr. Opin. Environ. Sci. Health 17, 1–7. doi: 10.1016/j.coesh.2020.04.006
Marston, D. A., McElhinney, L. M., Ellis, R. J., Horton, D. L., Wise, E. L., Leech, S. L., et al. (2013). Next generation sequencing of viral RNA genomes. BMC Genomics 14 (1), 1–12. doi: 10.1186/1471-2164-14-444
Mautner, L., Baillie, C.-K., Herold, H. M., Volkwein, W., Guertler, P., Eberle, U., et al. (2020). Rapid point-of-care detection of SARS-CoV-2 using reverse transcription loopmediated isothermal amplification (RT-LAMP). Virol. J. 17 (1), 1–14. doi: 10.1186/s12985-020-01435-6.
McKinney, K. R., Gong, Y. Y., Lewis, T. G. (2006). Environmental transmission of SARS at amoy gardens. J. Environ. Health 68 (9), 26.
Medema, G., Been, F., Heijnen, L., Petterson, S. (2020). Implementation of environmental surveillance for SARS-CoV-2 virus to support public health decisions: Opportunities and challenges. Curr. Opin. Environ. Sci. Health 17, 49–71. doi: 10.1016/j.coesh.2020.09.006
Medema, G., Heijnen, L., Elsinga, G., Italiaander, R., Brouwer, A. (2020). Presence of SARSCoronavirus-2 RNA in sewage and correlation with reported COVID-19 prevalence in the early stage of the epidemic in the Netherlands. Environ. Sci. Technol. Letters. 7 (7), 511–516. doi: 10.1021/acs.estlett.0c00357
Memish, Z. A., Perlman, S., Van Kerkhove, M. D., Zumla, A. (2020). Middle East respiratory syndrome. Lancet 395 (10229), 1063–1077. doi: 10.1016/S0140-6736(19)33221-0
Mercer, T. R., Salit, M. (2021). Testing at scale during the COVID-19 pandemic. Nat. Rev. Genet. 22 (7), 415–426. doi: 10.1038/s41576-021-00360-w
Ming, W. (2020). Nanopore target sequencing for accurate and comprehensive detection of SARS-CoV-2 and other respiratory viruses. Small 16 (32), e2002169. doi: 10.1002/smll.202002169
Miura, T., Okabe, S., Nakahara, Y., Sano, D. (2015). Removal properties of human enteric viruses in a pilot-scale membrane bioreactor (MBR) process. Water Res. 75, 282–291. doi: 10.1016/j.watres.2015.02.046
MoE (2019). “Institute for global environmental strategies; hayama,” in Water environment partnership in Asia WEPA third phase final report.
Moore, G., Rickard, H., Stevenson, D., Aranega-Bou, P., Pitman, J., Crook, A., et al. (2021). Detection of SARS-CoV-2 within the healthcare environment: a multi-centre study conducted during the first wave of the COVID-19 outbreak in England. J. Hosp. Infect. 108, 189–196. doi: 10.1016/j.jhin.2020.11.024
Muhammad, A. M., Ulhaq, I., Rahim, K. (2021). “Histopathologic evaluation and scoring of SARSCoV- 2 infection,” in Coronavirus disease-19 (COVID-19): A perspective of new scenario coronavirus disease-19 (COVID-19): Different models and treatment strategies, vol. 20. (Netherlands | United Arab Emirates: Bentham Science Publisher), 52–71. doi: 10.2174/9781681089072121020006
Murakami, M., Hata, A., Honda, R., Watanabe, T. (2020). Wastewater-based epidemiology can overcome representativeness and stigma issues related to COVID-19. Environ. Sci. technol. 54 (9), 5311–5311. doi: 10.1021/acs.est.0c02172
Nagamine, K., Hase, T., Notomi, T. J. M. C. P. (2002). Accelerated reaction by loop-mediated isothermal amplification using loop primers. Mol. Cell. probes. 16 (3), 223229. doi: 10.1006/mcpr.2002.0415
Natarajan, A., Zlitni, S., Brooks, E. F., Vance, S. E., Dahlen, A., Hedlin, H., et al. (2022). Gastrointestinal symptoms and fecal shedding of SARS-CoV-2 RNA suggest prolonged gastrointestinal infection. Med 3 (6), 371–387. doi: 10.1016/j.medj.2022.04.001
Naughton, C. C., Roman, F. A., Alvarado, A. G. F., Tariqi, A. Q., Deeming, M. A., Bibby, K., et al. (2021). Show us the data: global COVID-19 wastewater monitoring efforts, equity, and gaps. MedRXiv. doi: 10.1101/2021.03.14.21253564
Neault, N., Baig, A. T., Graber, T., D’Aoust, P., Mercier, E., Alexandrov, I., et al. (2020). SARS-CoV-2 protein in wastewater mirrors COVID-19 prevalence. medRxiv.
Nemudryi, A., Nemudraia, A., Wiegand, T., Surya, K., Buyukyoruk, M., Cicha, C., et al. (2020). Temporal detection and phylogenetic assessment of SARS-CoV-2 in municipal wastewater. Cell Rep. Med. 1 (6), 100098. doi: 10.1016/j.xcrm.2020.100098.
Nghiem, L. D., Morgan, B., Donner, E., Short, M. D. (2020). The COVID-19 pandemic: Considerations for the waste and wastewater services sector. Case Stud. Chem. Environ. Eng. 1, 100006. doi: 10.1016/j.cscee.2020.100006
Ng, T. F. F., Marine, R., Wang, C., Simmonds, P., Kapusinszky, B., Bodhidatta, L., et al. (2012). High variety of known and new RNA and DNA viruses of diverse origins in untreated sewage. J. virol. 86 (22), 12161–12175. doi: 10.1128/JVI.00869-12
Nguyen, T., Duong Bang, D., Wolff, A. (2020). 2019 novel coronavirus disease (COVID-19): paving the road for rapid detection and point-of-care diagnostics. Micromachines 11 (3), 306. doi: 10.3390/mi11030306
Nishiura, H., Kobayashi, T., Miyama, T., Suzuki, A., Jung, S.-m., Hayashi, K., et al. (2020). Estimation of the asymptomatic ratio of novel coronavirus infections (COVID-19). Int. J. Infect. dis. 94, 154–155. doi: 10.1016/j.ijid.2020.03.020
Noh, J. Y., Yoon, S. W., Kim, D. J., Lee, M. S., Kim, J. H., Na, W., et al. (2017). Simultaneous detection of severe acute respiratory syndrome, middle East respiratory syndrome, and related bat coronaviruses by real-time reverse transcription PCR. Arch. virol. 162 (6), 1617–1623. doi: 10.1007/s00705-017-3281-9
Notomi, T., Okayama, H., Masubuchi, H., Yonekawa, T., Watanabe, K., Amino, N., et al. (2000). Loop-mediated isothermal amplification of DNA. Nucleic Acids Res. 28 (12), e63–e63. doi: 10.1093/nar/28.12.e63
OHCHR (2020) COVID-19 and the human rights of LGBTI people. Available at: https://www.ohchr.org/Documents/Issues/LGBT/LGBTIpeople.pdf (Accessed 28 July 2020).
Oran, D. P., Topol, E. J. (2020). Prevalence of asymptomatic SARS-CoV-2 infection: A narrative review. Ann. Internal Med. 173 (5), 362–367. doi: 10.7326/M20-3012
Organization, W. H. (2020). “COVID 19 public health emergency of international concern (PHEIC),” in Global research and innovation forum: Towards a research roadmap (Avenue Appia 20, 1211 Geneva, Switzerland: World Health Organisation).
Organization, W. H, UniCeF (2010). “Progress on sanitation and drinking-water: 2010 update,” in Progress on sanitation and drinking-water: 2010 update United Nations Office to support the International Decade for action ' Water for Life 2005-2015/UN Water Decade Programme on Advocacy and Communication (UNWDPAC) biennial report 2010 |2011, Avenida Cataluña 60, 50014 Zaragoza, Spain, 60–60.
Ortiz-Ospina, E., Hasell, J. (2020). “How many tests for COVID-19 are being performed around the world,” in Our world in data is a project of the Global Change Data Lab England, Wales.
Pan, Y., Zhang, D., Yang, P., Poon, L. L., Wang, Q. (2020). Viral load of SARS-CoV-2 in clinical samples. Lancet Infect. Dis. 20 (4), 411–412. doi: 10.1016/S1473-3099(20)30113-4
Parasa, S., Desai, M., Chandrasekar, V. T., Patel, H. K., Kennedy, K. F., Roesch, T., et al. (2020). Prevalence of gastrointestinal symptoms and fecal viral shedding in patients with coronavirus disease 2019: a systematic review and meta-analysis. JAMA network Open 3 (6), e2011335–e2011335. doi: 10.1001/jamanetworkopen.2020.11335
Peccia, J., Zulli, A., Brackney, D. E., Grubaugh, N. D., Kaplan, E. H., Casanovas-Massana, A., et al. (2020). Measurement of SARS-CoV-2 RNA in wastewater tracks community infection dynamics. Nat. Biotechnol. 38 (10), 1164–1167. doi: 10.1038/s41587-020-0684-z
Pecson, B. M., Darby, E., Haas, C. N., Amha, Y. M., Bartolo, M., Danielson, R., et al. (2021). Reproducibility and sensitivity of 36 methods to quantify the SARS-CoV-2 genetic signal in raw wastewater: findings from an interlaboratory methods evaluation in the US. Environ. science: Water Res. technol. 7 (3), 504–520. doi: 10.1039/D0EW00946F
Pfefferle, S., Reucher, S., Nörz, D., Lütgehetmann, M. (2020). Evaluation of a quantitative RT-PCR assay for the detection of the emerging coronavirus SARS-CoV-2 using a high throughput system. Eurosurveillance 25 (9), 2000152. doi: 10.2807/1560-7917.ES.2020.25.9.2000152
Philo, S. E., Keim, E. K., Swanstrom, R., Ong, A. Q., Burnor, E. A., Kossik, A. L., et al. (2021). A comparison of SARS-CoV-2 wastewater concentration methods for environmental surveillance. Sci. Total Environ. 760, 144215. doi: 10.1016/j.scitotenv.2020.144215
Prakash, S., Pinti, M., Bhushan, B. (2012). Theory, fabrication and applications of microfluidic and nanofluidic biosensors. Philos. Trans. R. Soc. A: Math. Phys. Eng. Sci. 370 (1967), 2269–2303. doi: 10.1098/rsta.2011.0498.
Prevost, B., Lucas, F., Goncalves, A., Richard, F., Moulin, L., Wurtzer, S. (2015). Large Scale survey of enteric viruses in river and waste water underlines the health status of the local population. Environ. Int. 79, 42–50. doi: 10.1016/j.envint.2015.03.004
Qian, Q., Fan, L., Liu, W., Li, J., Yue, J., Wang, M., et al. (2021). Direct evidence of active SARS-CoV-2 replication in the intestine. Clin. Infect. Dis. 73 (3), 361–366. doi: 10.1093/cid/ciaa925
Qi, R., Huang, Y.-T., Liu, J.-W., Sun, Y., Sun, X.-F., Han, H.-J., et al. (2018). Global prevalence of asymptomatic norovirus infection: a meta-analysis. EClinicalMedicine 2, 50–58. doi: 10.1016/j.eclinm.2018.09.001
Quality, U. D. O. E. (2022). SARS-CoV-2 sewage monitoring (195 North, 1950 West, Salt Lake City, Utah: Utah Department of Environmental Quality).
Ramdas, K., Darzi, A., Jain, S. (2020). ‘Test, re-test, re-test’: using inaccurate tests to greatly increase the accuracy of COVID-19 testing. Nat. Med. 26 (6), 810–811. doi: 10.1038/s41591-020-0891-7
Randazzo, W., Truchado, P., Cuevas-Ferrando, E., Simón, P., Allende, A., Sánchez, G. (2020). SARS-CoV-2 RNA in wastewater anticipated COVID-19 occurrence in a low prevalence area. Water Res. 181, 115942. doi: 10.1016/j.watres.2020.115942
Ren, J.-G., Li, D.-Y., Wang, C.-F., Wu, J.-H., Wang, Y., Sun, Y.-J., et al. (2020). Positive RTPCR in urine from an asymptomatic patient with novel coronavirus 2019 infection: a case report. Infect. Dis. 52 (8), 571–574. doi: 10.1080/23744235.2020.1766105
Rimoldi, S. G., Stefani, F., Gigantiello, A., Polesello, S., Comandatore, F., Mileto, D., et al. (2020). Presence and infectivity of SARS-CoV-2 virus in wastewaters and rivers. Sci. Total Environ. 744, 140911. doi: 10.1016/j.scitotenv.2020.140911
Rolando, J. C., Jue, E., Barlow, J. T., Ismagilov, R. F. (2020). Real-time kinetics and high-resolution melt curves in single-molecule digital LAMP to differentiate and study specific and non-specific amplification. Nucleic Acids Res. 48 (7), e42–e42. doi: 10.1093/nar/gkaa099
Rowe, A. K., Kachur, S. P., Yoon, S. S., Lynch, M., Slutsker, L., Steketee, R. W. (2009). Caution is required when using health facility-based data to evaluate the health impact of malaria control efforts in Africa. Malaria J. 8 (1), 1–3. doi: 10.1186/1475-2875-8-209
Rusiñol, M., Martínez-Puchol, S., Forés, E., Itarte, M., Girones, R., Bofill-Mas, S. (2020). Concentration methods for the quantification of coronavirus and other potentially pandemic enveloped virus from wastewater. Curr. Opin. Environ. Sci. Health 17, 21–28. doi: 10.1016/j.coesh.2020.08.002
Salathe, M., Bengtsson, L., Bodnar, T. J., Brewer, D. D., Brownstein, J. S., Buckee, C., et al. (2012). Digital epidemiology. PLOS Computational Biology, San Francisco, California, US.
Savolainen-Kopra, C., Paananen, A., Blomqvist, S., Klemola, P., Simonen, M.-L., Lappalainen, M., et al. (2011). A large Finnish echovirus 30 outbreak was preceded by silent circulation of the same genotype. Virus genes. 42 (1), 28–36. doi: 10.1007/s11262-010-0536-x
Sedmak, G., Bina, D., MacDonald, J. (2003). Assessment of an enterovirus sewage surveillance system by comparison of clinical isolates with sewage isolates from Milwaukee, Wisconsin, collected august 1994 to December 2002. Appl. Environ. Microbiol. 69 (12), 7181–7187. doi: 10.1128/AEM.69.12.7181-7187.2003
Setianingsih, T. Y., Wiyatno, A., Hartono, T. S., Hindawati, E., Dewantari, A. K., Myint, K. S., et al. (2019). Detection of multiple viral sequences in the respiratory tract samples of suspected middle East respiratory syndrome coronavirus patients in Jakarta, Indonesia 2015–2016. Int. J. Infect. Dis. 86, 102–107. doi: 10.1016/j.ijid.2019.06.022
Shah, S., Gwee, S. X. W., Ng, J. Q. X., Lau, N., Koh, J., Pang, J. (2022). Wastewater surveillance to infer COVID-19 transmission: A systematic review. Sci. Total Environ. 804, 150060. doi: 10.1016/j.scitotenv.2021.150060
Sharfstein, J. M., Becker, S. J., Mello, M. M. (2020). Diagnostic testing for the novel coronavirus. Jama 323 (15), 1437–1438. doi: 10.1001/jama.2020.3864
Sharif, S., Ikram, A., Khurshid, A., Salman, M., Mehmood, N., Arshad, Y., et al. (2020). Detection of SARs-CoV-2 in wastewater, using the existing environmental surveillance network: An epidemiological gateway to an early warning for COVID-19 in communities. medRxiv 2020, 2006.2003.20121426. doi: 10.1101/2020.06.03.20121426
Sheridan, C. (2020). Fast, portable tests come online to curb coronavirus pandemic. Nat. Biotechnol. 38 (5), 515–518. doi: 10.1038/d41587-020-00010-2
Shi, R., Ma, W., Wu, Q., Zhang, B., Song, Y., Guo, Q., et al. (2003). Design and application of 60mer oligonucleotide microarray in SARS coronavirus detection. Chin. Sci. Bulletin. 48 (12), 1165–1169. doi: 10.1007/BF03183928
Sims, N., Kasprzyk-Hordern, B. (2020). Future perspectives of wastewater-based epidemiology: monitoring infectious disease spread and resistance to the community level. Environ. Int. 139, 105689. doi: 10.1016/j.envint.2020.105689
Sinclair, R. G., Choi, C. Y., Riley, M. R., Gerba, C. P. (2008). Pathogen surveillance through monitoring of sewer systems. Adv. Appl. Microbiol. 65, 249. doi: 10.1016/S0065-2164(08)00609-6
Sin, G., Espuña, A. (2020). Editorial: Applications of Monte Carlo method in chemical, biochemical and environmental engineering. Front. Energy Res. 8. doi: 10.3389/fenrg.2020.00068
Song, Y., Liu, P., Shi, X., Chu, Y., Zhang, J., Xia, J., et al. (2020). SARS-CoV-2 induced diarrhoea as onset symptom in patient with COVID-19. Gut 69 (6), 1143–1144. doi: 10.1136/gutjnl-2020-320891
Stadler, L. B., Ensor, K., Clark, J. R., Kalvapalle, P., LaTurner, Z. W., Mojica, L., et al. (2020). Wastewater analysis of SARS-CoV-2 as a predictive metric of positivity rate for a major metropolis. MedRXiv. doi: 10.1101/2020.11.04.20226191
Takeda, T., Kitajima, M., Abeynayaka, A., Huong, N., Dinh, N., Sirikanchana, K., et al. (2021). “Governance of wastewater surveillance systems to minimize the impact of COVID-19 and future epidemics: cases across Asia-pacific,” in Environmental resilience and transformation in times of COVID-19 (Netherlands:Elsevier), 115–126.
Tang, Y.-W., Schmitz, J. E., Persing, D. H., Stratton, C. W. (2020). Laboratory diagnosis of COVID-19: current issues and challenges. J. Clin. Microbiol. 58 (6), e00512–e00520. doi: 10.1128/JCM.00512-20
Tan, X., Oo, M., Gong, Y., Li, Y., Zhu, H., Fan, X. (2017). Glass capillary based microfluidic ELISA for rapid diagnostics. Analyst. 142, 2378–2385. doi: 10.1039/C7AN00523G
Tatara, A. M. (2020). Role of tissue engineering in COVID-19 and future viral outbreaks. Tissue Eng. Part A. 26 (9-10), 468–474. doi: 10.1089/ten.TEA.2020.0094
Telenti, A., Arvin, A., Corey, L., Corti, D., Diamond, M. S., García-Sastre, A., et al. (2021). After the pandemic: perspectives on the future trajectory of COVID-19. Nature 596 (7873), 495–504. doi: 10.1038/s41586-021-03792-w.
Tempe (2021). Innovation in advancing community health and fighting COVID-19 Arizona, US: City of Tempe.
Thaitrong, N., Charlermroj, R., Himananto, O., Seepiban, C., Karoonuthaisiri, N. (2013). Implementation of microfluidic sandwich ELISA for superior detection of plant pathogens. PloS One 8, e83231. doi: 10.1371/journal.pone.0083231
Thompson, J. R., Nancharaiah, Y. V., Gu, X., Lee, W. L., Rajal, V. B., Haines, M. B., et al. (2020). Making waves: wastewater surveillance of SARS-CoV-2 for population-based health management. Water Res. 184, 116181. doi: 10.1016/j.watres.2020.116181
Trottier, J., Darques, R., Ait Mouheb, N., Partiot, E., Bakhache, W., Deffieu, M. S., et al. (2020). Post-lockdown detection of SARS-CoV-2 RNA in the wastewater of montpellier, France. One Health 10 100157. doi: 10.1016/j.onehlt.2020.100157
Turoňová, B., Sikora, M., Schürmann, C., Hagen, W. J., Welsch, S., Blanc, F. E., et al. (2020). Situ structural analysis of SARS-CoV-2 spike reveals flexibility mediated by three hinges. Science 370 (6513), eabd5223. doi: 10.1126/science.abd5223
Udugama, B., Kadhiresan, P., Kozlowski, H. N., Malekjahani, A., Osborne, M., Li, V. Y. C., et al. (2020). Diagnosing COVID-19: The disease and tools for detection. ACS Nano 14 (4), 3822–3835. doi: 10.1021/acsnano.0c02624
Ulhaq, I., Basit, A., Ali, I., Hussain, F., Ali, Z., Siddique, F., et al. (2021). Coronavirus disease-2019 (COVID- 19) (Netherlands | United Arab Emirates: Bentham Books).
UNDP (2020) Brief #2: Putting the UN framework for socio-economic response to COVID-19 into action: Insights. Available at: https://www.undp.org/content/undp/en/home/coronavirus/socio-economic-impact-of-covid-19.html (Accessed 29 July).
Utah, D. E. Q. (2022) SARS-CoV-2 sewage monitoring. Utah department of environmental quality. Available at: https://deq.utah.gov/water-quality/sars-cov-2-sewage-monitoring.
WHO (2017). “WHO/UNICEF joint monitoring programme for water supply, sanitation and hygiene (JMP),” in WHO. novel coronavirus, (2019-nCoV) SituationReport-22 Geneva: WorldHealth Organization. Available at: https://www.who.int/docs/defaultsource/.
Vandenberg, O., Martiny, D., Rochas, O., van Belkum, A., Kozlakidis, Z. (2021). Considerations for diagnostic COVID-19 tests. Nat. Rev. Microbiol. 19 (3), 171183. doi: 10.1038/s41579020-00461-z
Vaselli, N. M., Setiabudi, W., Subramaniam, K., Adams, E. R., Turtle, L., Iturriza-Gómara, M., et al. (2021). Investigation of SARS-CoV-2 faecal shedding in the community: a prospective household cohort study (COVID-LIV) in the UK. BMC Infect. Dis. 21 (1), 784. doi: 10.1186/s12879-021-06443-7
Venugopal, A., Ganesan, H., Raja, S. S. S., Govindasamy, V., Arunachalam, M., Narayanasamy, A., et al. (2020). Novel wastewater surveillance strategy for early detection of coronavirus disease 2019 hotspots. Curr. Opin. Environ. Sci. Health 17, 8–13. doi: 10.1016/j.coesh.2020.05.003
Verpoorte, E., De Rooij, N. F. (2003). Microfluidics meets MEMS. Proc. IEEE. 91 (6), 930–953. doi: 10.1109/JPROC.2003.813570
Victoria, J. G., Kapoor, A., Dupuis, K., Schnurr, D. P., Delwart, E. L. (2008). Rapid identification of known and new RNA viruses from animal tissues. PloS pathog. 4 (9), e1000163. doi: 10.1371/journal.ppat.1000163
Vora, G. J., Meador, C. E., Stenger, D. A., Andreadis, J. D. (2004). Nucleic acid amplification strategies for DNA microarray-based pathogen detection. Appl. Environ. Microbiol. 70 (5), 3047–3054. doi: 10.1128/AEM.70.5.3047-3054.2004
Wang, X.-W., Li, J.-S., Guo, T.-K., Zhen, B., Kong, Q.-X., Yi, B., et al. (2005). Concentration and detection of SARS coronavirus in sewage from xiao tang shan hospital and the 309th hospital. J. virol. Methods 128 (1-2), 156–161. doi: 10.1016/j.jviromet.2005.03.022
Wang, W., Xu, Y., Gao, R., Lu, R., Han, K., Wu, G., et al. (2020). Detection of SARS-CoV2 in different types of clinical specimens. Jama 323 (18), 1843–1844. doi: 10.1001/jama.2020.3786.
Wang, H., Paulson, K. R., Pease, S. A., Watson, S., Comfort, H., Zheng, P., et al. (2022). Estimating excess mortality due to the COVID-19 pandemic: a systematic analysis of COVID-19-related mortalit -2021. Lancet 399 (10334), 1513–1536. doi: 10.1016/S0140-6736(21)02796-3
Watson, C., Cicero, A., Blumenstock, J., Fraser, M., Johns Hopkins School of Public Health Center for Health Security (2020) A national plan to enable comprehensive COVID-19 case finding and contact tracing in the US. Available at: https://www.centerforhealthsecurity.org/our-work/pubs_archive/pubs-pdfs/2020/a-national-plan-to-enable-comprehensive-COVID-19-case-finding-and-contact-tracing-in-the-US.pdf.
WHO (2020) Laboratory testing for 2019 novel coronavirus, (2019-nCoV) in suspected human cases. Available at: https://www.who.int/publications/i/item/10665-331501.
Woelfel, R., Corman, V. M., Guggemos, W., Seilmaier, M., Zange, S., Mueller, M. A., et al. (2020). Clinical presentation and virological assessment of hospitalized cases of coronavirus disease 2019 in a travel-associated transmission cluster. MedRxiv 2020 581 (7809), 465–469. doi: 10.1038/s41586-020-2196-x.
Wong, M. V., Hashsham, S. A., Gulari, E., Rouillard, J.-M., Aw, T. G., Rose, J. B. (2013). Detection and characterization of human pathogenic viruses circulating in community wastewater using multi target microarrays and polymerase chain reaction. J. Water Health 11 (4), 659670. doi: 10.2166/wh.2013.322
Wu, Y., Guo, C., Tang, L., Hong, Z., Zhou, J., Dong, X., et al. (2020). Prolonged presence of SARS-CoV-2 viral RNA in faecal samples. Lancet Gastroenterol. Hepatol. 5 (5), 434–435. doi: 10.1016/S2468-1253(20)30083-2
Wurtzer, S., Marechal, V., Mouchel, J.-M., Maday, Y., Teyssou, R., Richard, E., et al. (2020a). Evaluation of lockdown impact on SARS-CoV-2 dynamics through viral genome quantification in Paris wastewaters. MedRxiv 25 (50), 2000776. doi: 10.2807/1560-7917.ES.2020.25.50.2000776
Wurtzer, S., Marechal, V., Mouchel, J.-M., Moulin, L. (2020b). Time course quantitative detection of SARS-CoV-2 in Parisian wastewaters correlates with COVID-19 confirmed cases. MedRxiv. doi: 10.1101/2020.04.12.20062679
Wu, F., Zhang, J., Xiao, A., Gu, X., Lee, W. L., Armas, F., et al. (2020a). SARS-CoV-2 titers in wastewater are higher than expected from clinically confirmed cases. Msystems 5 (4), e00614–e00620. doi: 10.1128/mSystems.00614-20.
Xagoraraki, I., O’Brien, E. (2020). “Wastewater-based epidemiology for early detection of viral outbreaks,” in Women in water quality (Germany: Springer), 75–97.
Xing, Y. H., Ni, W., Wu, Q., Li, W. J., Li, G. J., Wang, W. D., et al. (2020). Prolonged viral shedding in feces of pediatric patients with coronavirus disease 2019. J. Microbiol. Immunol. Infect. 53 (3), 473–480. doi: 10.1016/j.jmii.2020.03.021
Xu, Y., Li, X., Zhu, B., Liang, H., Fang, C., Gong, Y., et al. (2020). Characteristics of pediatric SARS-CoV-2 infection and potential evidence for persistent fecal viral shedding. Nat. Med. 26 (4), 502–505. doi: 10.1038/s41591-020-0817-4.
Yang, L., Yamamoto, T. (2016). Quantification of virus particles using nanopore-based resistive-pulse sensing techniques. Front. Microbiol. 7, 1500. doi: 10.3389/fmicb.2016.01500
Ye, Y., Ellenberg, R. M., Graham, K. E., Wigginton, K. R. (2016). Survivability, partitioning, and recovery of enveloped viruses in untreated municipal wastewater. Environ. Sci. technol. 50 (10), 5077–5085. doi: 10.1021/acs.est.6b00876
Yeo, C., Kaushal, S., Yeo, D. (2020). Enteric involvement of coronaviruses: is faecal–oral transmission of SARS-CoV-2 possible? Lancet Gastroenterol. Hepatol. 5 (4), 335–337. doi: 10.1016/S2468-1253(20)30048-0.
Young, B. E., Ong, S. W. X., Kalimuddin, S., Low, J. G., Tan, S. Y., Loh, J., et al. (2020). Epidemiologic features and clinical course of patients infected with SARS-CoV-2 in Singapore. Jama 323 (15), 1488–1494.
Yuan, C., Zhu, H., Yang, Y., Cai, X., Xiang, F., Wu, H., et al. (2020). Viral loads in throat and anal swabs in children infected with SARS-CoV-2. Emerg. Microbes Infect. 9 (1), 1233–1237. doi: 10.1080/22221751.2020.1771219
Yuan, C., Wang, H., Li, K., Tang, A., Dai, Y., Wu, B., et al. (2021). SARS-CoV-2 viral shedding characteristics and potential evidence for the priority for faecal specimen testing in diagnosis. PloS One 16 (2), e0247367. doi: 10.1371/journal.pone.0247367
Zahedi, A., Monis, P., Deere, D., Ryan, U. (2021). Wastewater-based epidemiology–surveillance and early detection of waterborne pathogens with a focus on SARS-CoV-2, cryptosporidium and giardia. Parasitol. Res. 120 (12), 4167–4188. doi: 10.1007/s00436-020-07023-5
Zhang, Y., Chen, C., Song, Y., Zhu, S., Wang, D., Zhang, H., et al. (2020a). Excretion of SARSCoV-2 through faecal specimens. Emerg. Microbes Infect. 9 (1), 2501–2508. doi: 10.1080/22221751.2020.1844551
Zhang, Y., Chen, C., Zhu, S., Shu, C., Wang, D., Song, J., et al. (2020b). Isolation of 2019-nCoV from a stool specimen of a laboratory-confirmed case of the coronavirus disease 2019 (COVID-19). China CDC Wkly 2 (8), 123–124.
Zhang, W., Du, R.-H., Li, B., Zheng, X.-S., Yang, X.-L., Hu, B., et al. (2020c). Molecular and serological investigation of 2019-nCoV infected patients: implication of multiple shedding routes. Emerg. Microbes infect. 9 (1), 386–389. doi: 10.1080/22221751.2020.1729071
Zhang, H. T., Huang, M. X., Liu, X., Zheng, X. C., Li, X. H., Chen, G. Q., et al. (2020d). Evaluation of the adjuvant efficacy of natural herbal medicine on COVID-19: a retrospective matched case-control study. Am. J. Chin. Med. 48 (04), 779–792.
Zhang, J., Wang, S., Xue, Y. (2020e). Fecal specimen diagnosis 2019 novel coronavirus–infected pneumonia. J. Med. Virol. 92 (6), 680–682.
Zhao, L., Atoni, E., Du, Y., Zhang, H., Donde, O., Huang, D., et al. (2020). First study on surveillance of SARS-CoV-2 RNA in wastewater systems and related environments in wuhan: Post-lockdown. medRxiv. doi: 10.1101/2020.08.19.20172924
Zhou, J., Li, C., Zhao, G., Chu, H., Wang, D., Yan, H. H.-N., et al. (2017). Human intestinal tract serves as an alternative infection route for middle East respiratory syndrome coronavirus. Sci. adv. 3 (11), eaao4966. doi: 10.1126/sciadv.aao4966.
Zhou, W., Oh, J., Li, W., Kim, D., Yang, M. H., Jang, J., et al. (2014). Chemical constituents of the Korean endangered species rhododendron brachycarpum. Biochem. Systematics Ecol. 56, 231–236.
Zhu, H., Fohlerová, Z., Pekárek, J., Basova, E., Neužil, P. (2020). Recent advances in lab-on-achip technologies for viral diagnosis. Biosen. Bioelectron. 153, 112041. doi: 10.1016/j.bios.2020.112041
Keywords: wastewater surveillance, point-of-care diagnostics, COVID-19 pandemic, COVID-19 surveillance system, early warning system
Citation: Maryam S, Ul Haq I, Yahya G, Ul Haq M, Algammal AM, Saber S and Cavalu S (2023) COVID-19 surveillance in wastewater: An epidemiological tool for the monitoring of SARS-CoV-2. Front. Cell. Infect. Microbiol. 12:978643. doi: 10.3389/fcimb.2022.978643
Received: 26 June 2022; Accepted: 03 November 2022;
Published: 05 January 2023.
Edited by:
Yasir Waheed, Shaheed Zulfiqar Ali Bhutto Medical University (SZABMU), PakistanReviewed by:
Kashif Rahim, Cholistan University of Veterinary and Animal Sciences, PakistanMuhammad Nisar, Cholistan University of Veterinary and Animal Sciences, Pakistan
Kamal Niaz, Cholistan University of Veterinary and Animal Sciences, Pakistan
Copyright © 2023 Maryam, Ul Haq, Yahya, Ul Haq, Algammal, Saber and Cavalu. This is an open-access article distributed under the terms of the Creative Commons Attribution License (CC BY). The use, distribution or reproduction in other forums is permitted, provided the original author(s) and the copyright owner(s) are credited and that the original publication in this journal is cited, in accordance with accepted academic practice. No use, distribution or reproduction is permitted which does not comply with these terms.
*Correspondence: Sajida Maryam, sajida.maryam786@gmail.com; Ihtisham Ul Haq, ihtishamulhaq535@gmail.com