- 1Group of Translational Research in Digestive Diseases, Institute for Health Research Aragón (IIS Aragón), Zaragoza, Spain
- 2Department of Biochemistry and Molecular & Cellular Biology, University of Zaragoza, Zaragoza, Spain
- 3Department of Medicine, Psychiatry and Dermatology, University of Zaragoza, Zaragoza, Spain
- 4Biomedical Research Networking Center in Hepatic and Digestive Diseases (CIBERehd), Madrid, Spain
- 5Digestive Diseases Service, University Clinic Hospital Lozano Blesa, Zaragoza, Spain
Two-component regulatory systems (TCRS) are ubiquitous signal transduction mechanisms evolved by bacteria for sensing and adapting to the constant changes that occur in their environment. Typically consisting of two types of proteins, a membrane sensor kinase and an effector cytosolic response regulator, the TCRS modulate via transcriptional regulation a plethora of key physiological processes, thereby becoming essential for bacterial viability and/or pathogenicity and making them attractive targets for novel antibacterial drugs. Some members of the phylum Campylobacterota (formerly Epsilonproteobacteria), including Helicobacter pylori and Campylobacter jejuni, have been classified by WHO as “high priority pathogens” for research and development of new antimicrobials due to the rapid emergence and dissemination of resistance mechanisms against first-line antibiotics and the alarming increase of multidrug-resistant strains worldwide. Notably, these clinically relevant pathogens express a variety of TCRS and orphan response regulators, sometimes unique among its phylum, that control transcription, translation, energy metabolism and redox homeostasis, as well as the expression of relevant enzymes and virulence factors. In the present mini-review, we describe the signalling mechanisms and functional diversity of TCRS in H. pylori and C. jejuni, and provide an overview of the most recent findings in the use of these microbial molecules as potential novel therapeutic targets for the development of new antibiotics.
Introduction
Phylum Campylobacterota, previously known as Epsilonproteobacteria (Oren and Garrity, 2021), is a metabolically and physiologically diverse group of prokaryotes comprising both pathogenic and commensal heterotrophic genera such as Helicobacter, Campylobacter, Wolinella, and Arcobacter, but also free-living inhabitants of sulphidic ecosystems that are considered important primary producers (Waite et al., 2017). Among pathogenic members of Campylobacterota, some species constitute the etiological agents of relevant infections and serious associated-diseases in humans.
Helicobacter pylori colonizes human stomach of more than half of the global population (Hooi et al., 2017) inducing chronic inflammation of the gastric epithelium that in some cases progresses to atrophic gastritis, peptic ulcer disease, gastric adenocarcinoma, and mucosa associated lymphoid tissue (MALT) lymphoma (Kao et al., 2016; Chmiela and Kupcinskas, 2019). This microorganism has been associated with 90% of non-cardia gastric cancer worldwide (Moss, 2017) and constitutes the only bacterial pathogen classified as a class I carcinogen (IARC et al., 1994). Campylobacter jejuni is recognized as the major cause of bacterial food-borne gastroenteritis worldwide, being responsible of 80-90% of all cases of diagnosed campylobacteriosis (Elmi et al., 2020). The bacterium is part of the commensal microbiota of different avian species and other wild and domestic animals (Burnham and Hendrixson, 2018; Thepault et al., 2020), and it is transmitted to humans mainly by consumption of contaminated meat and other foods (Facciola et al., 2017). Despite the fact that infection in humans usually produces only mild and self-limited bloody diarrhea, severe complications and even death could occur in very young children, elderly people and immunocompromised patients (Same and Tamma, 2018; Igwaran and Okoh, 2019).
Antibiotic chemotherapy is crucial for treatment of H. pylori infection and mitigation of gastric cancer incidence (Rokkas et al., 2017; Leung et al., 2018; Nam et al., 2019); but also to treat invasive or extra-gastrointestinal infections by C. jejuni and reducing the risk of severe complications of campylobacteriosis in more susceptible populations (Arguello et al., 2015; Mizuno et al., 2022). However, the increasing development of antibiotic resistance and the rapid propagation of multidrug-resistant strains of these clinically relevant pathogens worldwide have contributed to a concerning detriment of the efficacy of current antibiotic therapies (Boyanova et al., 2019; Mourkas et al., 2019; Kuo et al., 2021; Sciortino et al., 2021). As a consequence, several studies have been focused on the identification and validation of new potential therapeutic targets in these microorganisms that lead to the development of novel and effective antibacterial strategies (Hossain et al., 2018; Deblais et al., 2019; González et al., 2019b; González et al., 2020; Roszczenko-Jasinska et al., 2020; Salillas and Sancho, 2020).
TCRS: A bacterial signal transduction mechanism to sense, respond, and adapt to environmental changes
Two component regulatory systems (TCRS) are ubiquitous signal transduction mechanisms among bacteria that enable microbial cells to perceive both environmental changes and internal information and subsequently trigger a rapid physiological response in order to better adapt to the new conditions. Typically, a TCRS comprises two different proteins that act in concert, a membrane-anchored histidine kinase (HK) and a cognate soluble response regulator (RR), which mostly acts as a DNA binding transcription factor (Figure 1). Chemical or physical stimuli are perceived by the HK, which subsequently undergoes autophosphorylation and transduces the signal via phosphoryl group to the REC domain of the RR. Phosphorylation usually induces conformational changes in the RR structure that activate or promote their effector functions (Gao et al., 2019). Notably, some RRs appear to act with independence of a cognate HK, which are known as “orphan” RRs. In some cases, these atypical unpaired RRs could naturally lack the conserved Asp and/or other residues required for the phosphotransfer reaction or retain their effector function upon substitution of these residues in the receiver domain, suggesting the existence of phosphorylation-independent mechanisms of regulation (Beier, 2012).
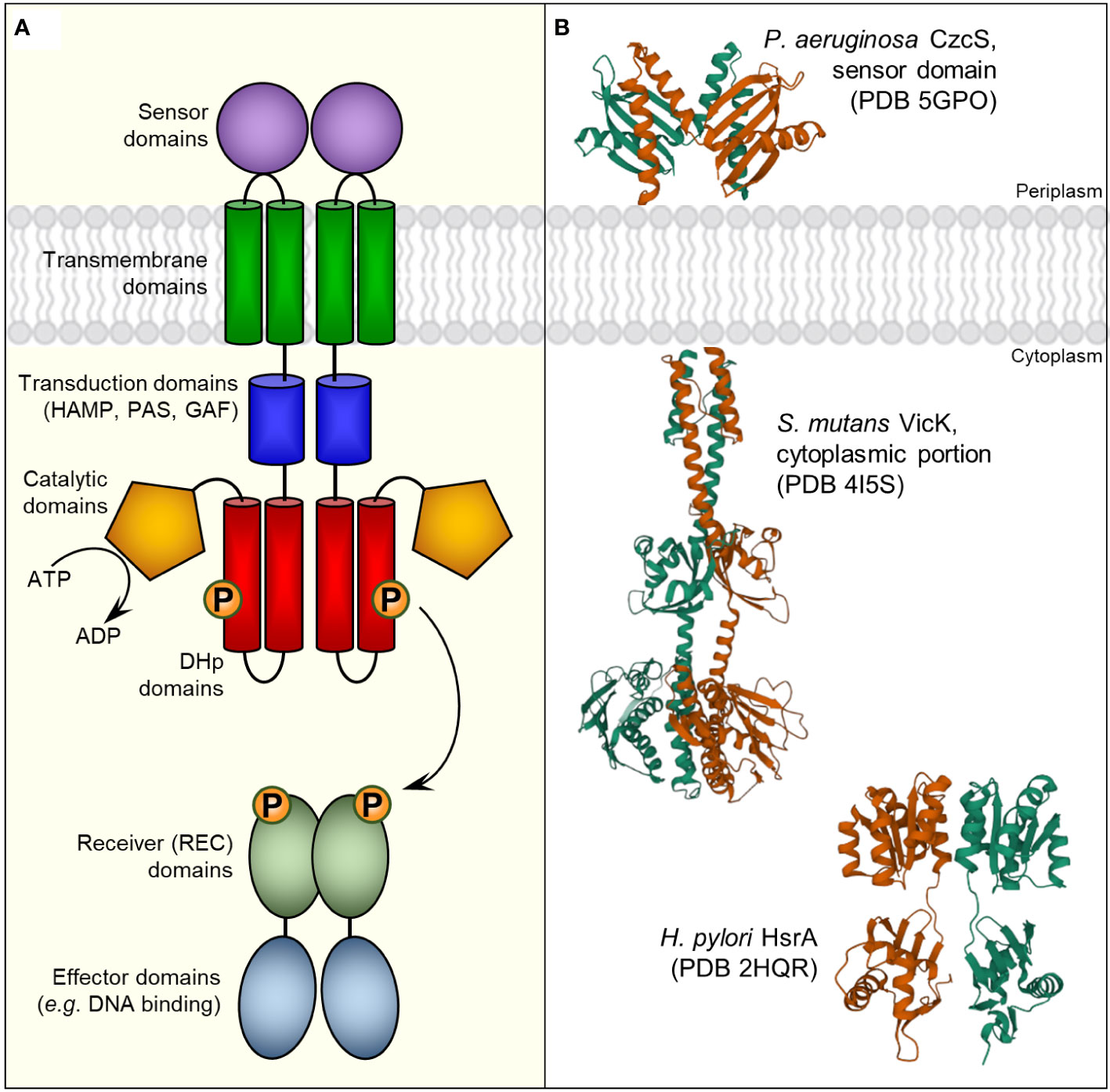
Figure 1 Bacterial two-component regulatory systems (TCRS). (A) Schematic representation of a typical TCRS comprising a membrane-anchored sensor histidine kinase (HK) and its cognate cytoplasmic response regulator (RR). Although microbial HKs can exhibit a considerable variety in their architectures (Jacob-Dubuisson et al., 2018), a canonical HK consists in a multi-domain homodimeric protein with extracellular sensors that perceives specific stimuli and undergoes autophosphorylation in a conserved His residue through a cytosolic catalytic-ATP binding domain. Phosphoryl group is subsequently transferred to a conserved Asp residue of the cognate RR promoted mainly by intermolecular interactions between the dimerization and histidine phosphotransfer (DHp) domains of the HK and the receiver (REC) domain of the RR (Yamada et al., 2009). Thus, the specificity of the interaction between a given HK and its cognate RR appears to be primarily defined by the nature and positions of contacting residues at the interface between the DHp (HK) and the REC (RR) domains, though additional HK domains could also be involved in RR binding (Buschiazzo and Trajtenberg, 2019). (B) 3D structures of different HK- and RR-functional domains. Corresponding Protein Data Bank (PDB) entries appear in parentheses.
Structural and functional diversity of TCRS in H. pylori and C. jejuni
Although the physiological roles of most HKs and RRs predicted in whole-genome sequencing analyses of major pathogenic species of Campylobacterota remain poorly understood, significant advances in the comprehension of signalling transduction mechanisms and regulatory functions of certain TCRS have been achieved. As we detail below, strains of H. pylori and C. jejuni express a variety of TCRS and atypical RRs that control the on/off switch of a plethora of genes and operons involved in relevant physiological processes and virulence mechanisms (Table 1). Notably, the amount and complexity of TCRS in these host-associated Campylobacterota species are far from those observed in predicted signaling systems of free-living microorganisms included in the same phylum such as Sulfurospirillum spp. or Sulfurimonas spp., which exhibit a broader ecological breath. Thus, the extensive adaptation of H. pylori and C. jejuni to the restricted ecological niches of the gastro-intestinal tracts of their host led to streamlined genomes with a very small number of TCRS and minimal complexity in the signal transduction mechanisms (Mo et al., 2022).
Helicobacter pylori
One of the best-studied TCRS in this gastric pathogen contributes to acid acclimation and is required for gastric colonization. The ArsRS signal transduction mechanism is composed of the sensor ArsS HK and its cognate OmpR-like ArsR RR, which are co-transcribed and negatively auto-regulated by phosphorylated ArsR (Dietz et al., 2002). ArsS-dependent phosphorylation increases the affinity of ArsR by promoters of a variety of genes involved in acid acclimation, acting in all of these cases as a transcriptional activator (Pflock et al., 2005; Pflock et al., 2006; Wen et al., 2007; Loh et al., 2010). Deletion of ArsS impairs colonization of the murine stomach, but this HK is not essential for cell viability under neutral pH (Panthel et al., 2003; Loh and Cover, 2006). In contrast, ArsR appears essential for in vitro growth of H. pylori, suggesting that the expression of certain essential genes depends on the non-phosphorylated form of ArsR (Beier and Frank, 2000; Gupta et al., 2009). ArsRS is also involved in the acid-dependent negative regulation of the expression of adhesins and biofilm formation (Goodwin et al., 2008; Servetas et al., 2016; Acio-Pizzarello et al., 2017; Servetas et al., 2018), and contributes to acetone metabolism, resistance to oxidative stress and quorum-sensing (Loh et al., 2010).
H. pylori expresses two other TCRS involving two-partner proteins that play relevant roles for the in vitro growth and pathogenicity of the microorganism. Thus, the CrdRS system, composed of the CrdS HK and the OmpR-like CrdR RR appears to be involved in copper homeostasis (Waidner et al., 2005) and survival of the pathogen under nitrosative stress (Hung et al., 2015). On the other hand, the regulatory partner composed of the cytoplasmic sensor FlgS HK and the NtrC-like FlgR RR mediates the expression of the σ54-dependent flagellar genes that encode structural proteins of the basal body and hook, which are essential for H. pylori motility (Spohn and Scarlato, 1999; McDaniel et al., 2001; Brahmachary et al., 2004). The internal signal sensed by FlgS that triggers its autophosphorylation and subsequent activation of FlgR remains unknown (Alvarado et al., 2019).
Along with the aforementioned typical TCRS, the regulatory machinery of H. pylori includes two orphan OmpR-like RRs, the proteins HP1043 (also known as HsrA) and HP1021 (Beier and Frank, 2000). HsrA is a homodimeric essential RR that lacks the conserved phosphorylation site and appears to act as a phosphorylation-independent transcriptional activator (Schar et al., 2005; Hong et al., 2007; Pelliciari et al., 2017; Zannoni et al., 2021). The protein regulates its own expression (Delany et al., 2002), but also the expression of a plethora of other genes involved in crucial cellular processes such as translation, transcription, energy metabolism, nitrogen metabolism, chemotaxis, and redox homeostasis (Olekhnovich et al., 2013; Olekhnovich et al., 2014; Pelliciari et al., 2017).
The HP1021 orphan RR acts as an inhibitor of DNA replication by binding to specific sequences within the oriC region, thereby avoiding DnaA-oriC interactions and the unwinding of duplex DNA (Donczew et al., 2015). The DNA binding activity of this RR is affected by both the redox state of its cysteine residues and the presence of divalent metal cations, especially Zn2+ (Szczepanowski et al., 2021). Hence, the regulator functions as a redox sensor that controls DNA replication, synthesis of Fe-S clusters, acetone metabolism, and response to oxidative stress (Pflock et al., 2007; Donczew et al., 2015; Szczepanowski et al., 2021). Deletion mutants of this dispensable protein exhibit a small-colony phenotype (McDaniel et al., 2001).
H. pylori exhibits chemotactic responses to a variety of chemical cues, which are perceived by a battery of chemoreceptors (Machuca et al., 2017; Johnson and Ottemann, 2018). The transduction of the signal from the receptors to the flagellar motor is mediated by two CheY response regulators, the protein CheY1 and the bifunctional protein CheY2/A, which consists of a CheY-like receiver domain (CheY2) fused to the CheA HK (Foynes et al., 2000; Jimenez-Pearson et al., 2005). Mutants in any one of these two proteins exhibited impaired chemotaxis and failed to colonize animal models (Foynes et al., 2000).
Campylobacter jejuni
C. jejuni is able to spatially discriminate among different regions of the host intestine by monitoring short-chain fatty acids (e.g. butyrate, acetate) and the lactate produced by the commensal microbiota of lower and upper intestinal tracks, respectively (Luethy et al., 2017; Goodman et al., 2020). The BumSR TCRS perceives butyrate levels and triggers a dual effect on gene expression, activating the transcription of a set of genes but repressing the transcription of other targets (Luethy et al., 2015; Goodman et al., 2020). BumR appears relevant for colonization of both avian hosts and humans (Crofts et al., 2018; Goodman et al., 2020). BumS lacks transmembrane domains and in vitro autokinase activity, but this cytoplasmic protein exhibited a robust and specific phosphatase activity for BumR (Goodman et al., 2020). Given that phosphorylation appears to increase the in vitro DNA-binding affinity of BumR by its target promoters, BumS could function as a negative modulator of its cognate RR (Goodman et al., 2020). BumSR modulates the expression of genes involved in basic metabolism, iron/heme acquisition, respiration, and some colonization factors (Luethy et al., 2015).
The RacSR TCRS modulates gene expression in response to changes in temperature, acting as both an activator and a repressor of transcription (Bras et al., 1999). Inactivation of both RacS and RacR significantly reduced chicken colonization and led to defective growth above 42°C; however, both mutants displayed only minimal defects in their in vitro growth at 42°C (Bras et al., 1999; Apel et al., 2012). Impairment in chicken colonization appeared to be a consequence of multiple phenotypic changes, including misregulation of several heat shock proteins but also alterations in cellular morphology that diminished motility and reduced epithelial cell invasion (Apel et al., 2012). RacSR has also been associated with glutamine catabolism (van der Stel et al., 2015b), and the induction of changes in the respiratory metabolism (van der Stel et al., 2015a).
CprRS is a TCRS composed of the essential OmpR-like response regulator CprR and the dispensable CprS HK. Mutants of CprS appeared defective in chick colonization with an increased susceptibility to osmotic and oxidative stress (Svensson et al., 2009). Little is known about the CprRS regulon (Svensson et al., 2009; Svensson et al., 2015), but the in vitro essentiality of the RR and the non-essential nature of its cognate HK resemble the regulatory scenario observed in the H. pylori TCRS ArsRS. However, a point mutation in the phosphoacceptor Asp52 residue of the CprR REC domain impaired viability, indicating that CprR phosphorylation is essential despite the dispensability of CprS (Svensson et al., 2015).
In DccRS, the OmpR-like DccR RR is specifically phosphorylated by its cognate DccS HK in the late stationary growth phase, probably using released metabolic products as triggering signals (Wosten et al., 2010). The activated RR enhances transcription of a handful of genes encoding putative periplasmic and membrane proteins that appeared relevant, but not essential for chicken colonization (Wosten et al., 2010). Deletion mutants of DccR and DccS exhibited reduced colonization in mice and chicks, but this TCRS is dispensable for in vitro growth (MacKichan et al., 2004).
The C. jejuni TCRS comprising the partners PhosS and PhosR enables survival of this food-borne pathogen outside their hosts, in phosphate-deprived environments such as surface waters (Wosten et al., 2006). Limitation of inorganic phosphate is perceived by the PhosS HK, which autophosphorylates itself and transfers the phosphoryl group to the receiver domain of PhosR. The activated RR enhances the expression of a phosphate-inducible regulon, mostly composed by genes encoding phosphate uptake mechanisms. Both the in vitro growth under phosphate-replete conditions and the chicken colonization capability were similar in a phosR mutant and its isogenic wild-type strain. (Wosten et al., 2006).
Similarly to H. pylori, transcription of genes encoding structural proteins of the basal body and hook of C. jejuni flagellum, but also the minor filament protein FlaB, is regulated by σ54 factor (RpoN), whose function is stimulated by the FlgSR TCRS (Wosten et al., 2004). A signal associated to the flagellar type III secretion system (T3SS) in conjunction with the flagellar proteins FliG (C ring) and FliF (MS ring) is presumed to trigger autophosphorylation of the cytoplasmic FlgS HK, which subsequently activates its cognate FlgR RR (Joslin and Hendrixson, 2009; Boll and Hendrixson, 2013). Curiously, the C. jejuni FlgR RR undergoes an unusual phase-variable production that affects σ54-dependent expression of flagellar genes and consequently the motility and colonization capabilities of the bacterial cell. By this mechanism, a small amount of colonizing C. jejuni cells become non-motile and they would be more readily shed into the environment, thereby promoting transmission and colonization of new naïve hosts (Hendrixson, 2006).
Besides the above-described typical TCRS, C. jejuni expresses two relevant Omp-like orphan RRs. Among them, it is worth noticing CosR, the essential orthologue of the H. pylori HsrA RR (Raphael et al., 2005; Muller et al., 2007). CosR appears to modulate the expression of several genes involved in a broad variety of cellular processes, including oxidative stress defenses, respiration, energy metabolism and biosynthesis, gene regulation, copper tolerance, flagellum biosynthesis, lipid metabolism, biofilm formation, among others (Hwang et al., 2011; Hwang et al., 2012; Turonova et al., 2015; Park et al., 2021). This RR exhibits a dual role on gene expression, repressing the transcription of several genes involved in the oxidative stress response such as SodB, Dps, Rrc and LuxS, but acting as an activator of AhpC (Hwang et al., 2011) and KatA (Hwang et al., 2012). Additionally, CosR represses the transcription of CmeABC (Hwang et al., 2012; Grinnage-Pulley et al., 2016), the major multidrug efflux pump of C. jejuni (Sharifi et al., 2021).
A second C. jejuni orphan RR, the protein CbrR, is an atypical RR that lacks a DNA-binding domain, but contains a C-terminal GGDEF motif commonly observed in diguanylate cyclase enzymes (Raphael et al., 2005; Cox et al., 2022). Deletion mutants of CbrR exhibited increased sensitivity to sodium deoxycholate, enhanced motility and reduced ability to colonize chickens (Raphael et al., 2005; Cox et al., 2022), whereas CbrR overexpression resulted in loss of flagella and a significant reduction in biofilm formation (Cox et al., 2022). Yeast two-hybrid screenings have showed that CbrR interacts with CheA (Parrish et al., 2007), which seems to indicate a putative role in chemotaxis.
TCRS as targets for novel antimicrobials against H. pylori and C. jejuni
Bacterial TCRS have been proposed as promising therapeutic targets due to their validated essentiality for growth and pathogenicity of most clinical relevant pathogens (Bem et al., 2015; González et al., 2018; Hirakawa et al., 2020; Roncarati et al., 2022). TCRS become attractive targets for drug development against H. pylori and C. jejuni, where these regulatory proteins modulate essential physiological processes such as transcription, translation, energy metabolism and redox homeostasis, but also relevant mechanisms for host colonization and persistence of in vivo infection including cell morphology, motility, chemotaxis, acid acclimation as well as the expression of several virulence factors.
At least two RRs appear essential for H. pylori viability, ArsR from the pH-sensitive ArsRS TCRS, and the orphan OmpR-like effector protein HsrA. Similarly, two RRs have proved to be indispensable for C. jejuni survival, CprR from the CprSR TCRS, and the orphan regulator CosR. In addition, a group of other HKs and RRs are essential for optimal host colonization such as ArsS in H. pylori, which is indispensable for acid acclimation and gastric colonization, or the FlgSR TCRS in both pathogens, whose deletion results in loss of motility and chemotaxis. However, even though the expression of a variety of essential TCRS-related proteins in H. pylori and C. jejuni opens the door for the promising use of novel effective therapeutic targets in drug development; studies focused on this topic are relatively scarce.
To date, some investigations have been carried out in order to validate HsrA and ArsR as effective therapeutic targets against H. pylori infection as well as discovering low-molecular weight inhibitors of these RRs exhibiting strong bactericidal activities against antibiotic-resistant strains of this pathogen (González et al., 2019a; González et al., 2019b; González et al., 2020; Antoniciello et al., 2022; González et al., 2022). Notably, some of the novel antibacterial candidates evaluated in these studies, including natural flavonoids and 1,4-dihydropiridines, have shown in vitro antimicrobial activities in the range of those observed with first-line conventional antibiotics (González et al., 2021; González et al., 2022). In some cases, these novel antimicrobial candidates exhibited synergistic effects in combination with conventional antibiotics, leading to reversion of drug-resistant phenotypes (González et al., 2019b), but also showed minor antimicrobial actions on representative members of human normal microbiota (González et al., 2022).
Future perspectives
The rapid emergence and dissemination of multidrug-resistant strains worldwide has effectively diminished the efficacy of current antibiotic therapies against most clinically relevant pathogens, including H. pylori and C. jejuni. A major challenge in the R&D of new effective antibiotics is the identification of novel molecular targets that allow for overcoming the current circulating resistome. The use of essential TCRS as targets for novel antimicrobials and antivirulence strategies against major pathogenic species of Campylobacterota could be a gateway to novel personalized combinatory therapies that can make it possible to cope with the increasing concern of multidrug resistance. Targeting TCRS-related proteins uniquely found in this group of microorganisms would enable the development of next-generation precision antibiotics, with highly selective antimicrobial action and minor risk of dysbiosis.
Author Contributions
JC, AL, and AG wrote the manuscript. All authors contributed to the article and approved the submitted version.
Funding
This work has been supported by the Government of Aragon, Spain (B25_20R) and University of Zaragoza (2018/0420).
Conflict of interest
The authors declare that the research was conducted in the absence of any commercial or financial relationships that could be construed as a potential conflict of interest.
Publisher’s note
All claims expressed in this article are solely those of the authors and do not necessarily represent those of their affiliated organizations, or those of the publisher, the editors and the reviewers. Any product that may be evaluated in this article, or claim that may be made by its manufacturer, is not guaranteed or endorsed by the publisher.
References
Acio-Pizzarello, C. R., Acio, A. A., Choi, E. J., Bond, K., Kim, J., Kenan, A. C., et al. (2017). Determinants of the regulation of Helicobacter pylori adhesins include repeat sequences in both promoter and coding regions as well as the two-component system ArsRS. J. Med. Microbiol. 66 (6), 798–807. doi: 10.1099/jmm.0.000491
Alvarado, A., Behrens, W., Josenhans, C. (2019). Protein activity sensing in bacteria in regulating metabolism and motility. Front. Microbiol. 10. doi: 10.3389/fmicb.2019.03055
Antoniciello, F., Roncarati, D., Zannoni, A., Chiti, E., Scarlato, V., Chiappori, F. (2022). Targeting the essential transcription factor HP1043 of Helicobacter pylori: a drug repositioning study. Front. Mol. Biosci. 9. doi: 10.3389/fmolb.2022.887564
Apel, D., Ellermeier, J., Pryjma, M., Dirita, V. J., Gaynor, E. C. (2012). Characterization of Campylobacter jejuni RacRS reveals roles in the heat shock response, motility, and maintenance of cell length homogeneity. J. Bacteriol 194 (9), 2342–2354. doi: 10.1128/JB.06041-11
Arguello, E., Otto, C. C., Mead, P., Babady, N. E. (2015). Bacteremia caused by Arcobacter butzleri in an immunocompromised host. J. Clin. Microbiol. 53 (4), 1448–1451. doi: 10.1128/JCM.03450-14
Beier, D. (2012). “Deviations from the rule: orphan and atypical response regulators,” in Two-component systems in bacteria. Eds. Gross, R., Beier, D. (UK: Caister Academic Press).
Beier, D., Frank, R. (2000). Molecular characterization of two-component systems of Helicobacter pylori. J. Bacteriol. 182 (8), 2068–2076. doi: 10.1128/JB.182.8.2068-2076.2000
Bem, A. E., Velikova, N., Pellicer, M. T., Baarlen, P., Marina, A., Wells, J. M. (2015). Bacterial histidine kinases as novel antibacterial drug targets. ACS Chem. Biol. 10 (1), 213–224. doi: 10.1021/cb5007135
Boll, J. M., Hendrixson, D. R. (2013). A regulatory checkpoint during flagellar biogenesis in Campylobacter jejuni initiates signal transduction to activate transcription of flagellar genes. mBio 4 (5), e00432–e00413. doi: 10.1128/mBio.00432-13
Boyanova, L., Hadzhiyski, P., Kandilarov, N., Markovska, R., Mitov, I. (2019). Multidrug resistance in Helicobacter pylori: Current state and future directions. Expert Rev. Clin. Pharmacol. 12 (9), 909–915. doi: 10.1080/17512433.2019.1654858
Brahmachary, P., Dashti, M. G., Olson, J. W., Hoover, T. R. (2004). Helicobacter pylori FlgR is an enhancer-independent activator of sigma54-RNA polymerase holoenzyme. J. Bacteriol 186 (14), 4535–4542. doi: 10.1128/JB.186.14.4535-4542.2004
Bras, A. M., Chatterjee, S., Wren, B. W., Newell, D. G., Ketley, J. M. (1999). A novel Campylobacter jejuni two-component regulatory system important for temperature-dependent growth and colonization. J. Bacteriol 181 (10), 3298–3302. doi: 10.1128/JB.181.10.3298-3302.1999
Burnham, P. M., Hendrixson, D. R. (2018). Campylobacter jejuni: collective components promoting a successful enteric lifestyle. Nat. Rev. Microbiol. 16 (9), 551–565. doi: 10.1038/s41579-018-0037-9
Buschiazzo, A., Trajtenberg, F. (2019). Two-component sensing and regulation: how do histidine kinases talk with response regulators at the molecular level? Annu. Rev. Microbiol. 73, 507–528. doi: 10.1146/annurev-micro-091018-054627
Chmiela, M., Kupcinskas, J. (2019). Review: Pathogenesis of Helicobacter pylori infection. Helicobacter 24 Suppl 1, e12638. doi: 10.1111/hel.12638
Cox, C. A., Bogacz, M., El Abbar, F. M., Browning, D. D., Hsueh, B. Y., Waters, C. M., et al. (2022). The Campylobacter jejuni response regulator and cyclic-di-GMP binding CbrR is a novel regulator of flagellar motility. Microorganisms 10 (1), 86. doi: 10.3390/microorganisms10010086
Crofts, A. A., Poly, F. M., Ewing, C. P., Kuroiwa, J. M., Rimmer, J. E., Harro, C., et al. (2018). Campylobacter jejuni transcriptional and genetic adaptation during human infection. Nat. Microbiol. 3 (4), 494–502. doi: 10.1038/s41564-018-0133-7
Deblais, L., Helmy, Y. A., Kumar, A., Antwi, J., Kathayat, D., Acuna, U. M., et al. (2019). Novel narrow spectrum benzyl thiophene sulfonamide derivatives to control Campylobacter. J. Antibiot (Tokyo) 72 (7), 555–565. doi: 10.1038/s41429-019-0168-x
Delany, I., Spohn, G., Rappuoli, R., Scarlato, V. (2002). Growth phase-dependent regulation of target gene promoters for binding of the essential orphan response regulator HP1043 of Helicobacter pylori. J. Bacteriol 184 (17), 4800–4810. doi: 10.1128/JB.184.17.4800-4810.2002
Dietz, P., Gerlach, G., Beier, D. (2002). Identification of target genes regulated by the two-component system HP166-HP165 of Helicobacter pylori. J. Bacteriol 184 (2), 350–362. doi: 10.1128/jb.184.2.350-362.2002
Donczew, R., Makowski, L., Jaworski, P., Bezulska, M., Nowaczyk, M., Zakrzewska-Czerwinska, J., et al. (2015). The atypical response regulator HP1021 controls formation of the Helicobacter pylori replication initiation complex. Mol. Microbiol. 95 (2), 297–312. doi: 10.1111/mmi.12866
Elmi, A., Nasher, F., Dorrell, N., Wren, B., Gundogdu, O. (2020). Revisiting Campylobacter jejuni virulence and fitness factors: Role in sensing, adapting, and competing. Front. Cell Infect. Microbiol. 10. doi: 10.3389/fcimb.2020.607704
Facciola, A., Riso, R., Avventuroso, E., Visalli, G., Delia, S. A., Lagana, P. (2017). Campylobacter: from microbiology to prevention. J. Prev. Med. Hyg 58 (2), E79–E92.
Foynes, S., Dorrell, N., Ward, S. J., Stabler, R. A., McColm, A. A., Rycroft, A. N., et al. (2000). Helicobacter pylori possesses two CheY response regulators and a histidine kinase sensor, CheA, which are essential for chemotaxis and colonization of the gastric mucosa. Infect. Immun. 68 (4), 2016–2023. doi: 10.1128/IAI.68.4.2016-2023.2000
Gao, R., Bouillet, S., Stock, A. M. (2019). Structural basis of response regulator function. Annu. Rev. Microbiol. 73, 175–197. doi: 10.1146/annurev-micro-020518-115931
González, A., Casado, J., Chueca, E., Salillas, S., Velázquez-Campoy, A., Espinosa Angarica, V., et al. (2019a). Repurposing dihydropyridines for treatment of Helicobacter pylori infection. Pharmaceutics 11 (12), 681. doi: 10.3390/pharmaceutics11120681
González, A., Casado, J., Chueca, E., Salillas, S., Velázquez-Campoy, A., Sancho, J., et al. (2020). Small molecule inhibitors of the response regulator ArsR exhibit bactericidal activity against Helicobacter pylori. Microorganisms 8 (4), 503. doi: 10.3390/microorganisms8040503
González, A., Casado, J., Gündüz, M. G., Santos, B., Velázquez-Campoy, A., Sarasa-Buisan, C., et al. (2022). 1,4-dihydropyridine as a promising scaffold for novel antimicrobials against Helicobacter pylori. Front. Microbiol. 13. doi: 10.3389/fmicb.2022.874709
González, A., Casado, J., Lanas, A. (2021). Fighting the antibiotic crisis: flavonoids as promising antibacterial drugs against Helicobacter pylori infection. Front. Cell Infect. Microbiol. 11. doi: 10.3389/fcimb.2021.709749
González, A., Fillat, M. F., Lanas, A. (2018). Transcriptional regulators: valuable targets for novel antibacterial strategies. Future Med. Chem. 10 (5), 541–560. doi: 10.4155/fmc-2017-0181
González, A., Salillas, S., Velázquez-Campoy, A., Espinosa Angarica, V., Fillat, M. F., Sancho, J., et al. (2019b). Identifying potential novel drugs against Helicobacter pylori by targeting the essential response regulator HsrA. Sci. Rep. 9 (1), 11294. doi: 10.1038/s41598-019-47746-9
Goodman, K. N., Powers, M. J., Crofts, A. A., Trent, M. S., Hendrixson, D. R. (2020). Campylobacter jejuni BumSR directs a response to butyrate via sensor phosphatase activity to impact transcription and colonization. Proc. Natl. Acad. Sci. U.S.A. 117 (21), 11715–11726. doi: 10.1073/pnas.1922719117
Goodwin, A. C., Weinberger, D. M., Ford, C. B., Nelson, J. C., Snider, J. D., Hall, J. D., et al. (2008). Expression of the Helicobacter pylori adhesin SabA is controlled via phase variation and the ArsRS signal transduction system. Microbiol. (Reading) 154 (Pt 8), 2231–2240. doi: 10.1099/mic.0.2007/016055-0
Grinnage-Pulley, T., Mu, Y., Dai, L., Zhang, Q. (2016). Dual repression of the multidrug efflux pump CmeABC by CosR and CmeR in Campylobacter jejuni. Front. Microbiol. 7 1097. doi: 10.3389/fmicb.2016.01097
Gupta, S. S., Borin, B. N., Cover, T. L., Krezel, A. M. (2009). Structural analysis of the DNA-binding domain of the Helicobacter pylori response regulator ArsR. J. Biol. Chem. 284 (10), 6536–6545. doi: 10.1074/jbc.M804592200
Hendrixson, D. R. (2006). A phase-variable mechanism controlling the Campylobacter jejuni FlgR response regulator influences commensalism. Mol. Microbiol. 61 (6), 1646–1659. doi: 10.1111/j.1365-2958.2006.05336.x
Hirakawa, H., Kurushima, J., Hashimoto, Y., Tomita, H. (2020). Progress overview of bacterial two-component regulatory systems as potential targets for antimicrobial chemotherapy. Antibiotics (Basel) 9 (10), 635. doi: 10.3390/antibiotics9100635
Hong, E., Lee, H. M., Ko, H., Kim, D. U., Jeon, B. Y., Jung, J., et al. (2007). Structure of an atypical orphan response regulator protein supports a new phosphorylation-independent regulatory mechanism. J. Biol. Chem. 282 (28), 20667–20675. doi: 10.1074/jbc.M609104200
Hooi, J. K. Y., Lai, W. Y., Ng, W. K., Suen, M. M. Y., Underwood, F. E., Tanyingoh, D., et al. (2017). Global prevalence of Helicobacter pylori infection: systematic review and meta-analysis. Gastroenterology 153 (2), 420–429. doi: 10.1053/j.gastro.2017.04.022
Hossain, M. U., Omar, T. M., Alam, I., Das, K. C., Mohiuddin, A. K. M., Keya, C. A., et al. (2018). Pathway based therapeutic targets identification and development of an interactive database CampyNIBase of Campylobacter jejuni RM1221 through non-redundant protein dataset. PloS One 13 (6), e0198170. doi: 10.1371/journal.pone.0198170
Hung, C. L., Cheng, H. H., Hsieh, W. C., Tsai, Z. T., Tsai, H. K., Chu, C. H., et al. (2015). The CrdRS two-component system in Helicobacter pylori responds to nitrosative stress. Mol. Microbiol. 97 (6), 1128–1141. doi: 10.1111/mmi.13089
Hwang, S., Kim, M., Ryu, S., Jeon, B. (2011). Regulation of oxidative stress response by CosR, an essential response regulator in Campylobacter jejuni. PloS One 6 (7), e22300. doi: 10.1371/journal.pone.0022300
Hwang, S., Zhang, Q., Ryu, S., Jeon, B. (2012). Transcriptional regulation of the CmeABC multidrug efflux pump and the KatA catalase by CosR in Campylobacter jejuni. J. Bacteriol 194 (24), 6883–6891. doi: 10.1128/JB.01636-12
IARC, W.H.O, I.W.G.o.t.E.o.C.R.t. Humans (1994). “Schistosomes, liver flukes and helicobacter pylori,” in IARC monographs on the evaluation of carcinogenic risks to humans (Lyon: World Health Organization), 178–240.
Igwaran, A., Okoh, A. I. (2019). Human campylobacteriosis: A public health concern of global importance. Heliyon 5 (11), e02814. doi: 10.1016/j.heliyon.2019.e02814
Jacob-Dubuisson, F., Mechaly, A., Betton, J. M., Antoine, R. (2018). Structural insights into the signalling mechanisms of two-component systems. Nat. Rev. Microbiol. 16 (10), 585–593. doi: 10.1038/s41579-018-0055-7
Jimenez-Pearson, M. A., Delany, I., Scarlato, V., Beier, D. (2005). Phosphate flow in the chemotactic response system of Helicobacter pylori. Microbiol. (Reading) 151 (Pt 10), 3299–3311. doi: 10.1099/mic.0.28217-0
Johnson, K. S., Ottemann, K. M. (2018). Colonization, localization, and inflammation: the roles of H. pylori chemotaxis in vivo. Curr. Opin. Microbiol. 41, 51–57. doi: 10.1016/j.mib.2017.11.019
Joslin, S. N., Hendrixson, D. R. (2009). Activation of the Campylobacter jejuni FlgSR two-component system is linked to the flagellar export apparatus. J. Bacteriol 191 (8), 2656–2667. doi: 10.1128/JB.01689-08
Kao, C. Y., Sheu, B. S., Wu, J. J. (2016). Helicobacter pylori infection: An overview of bacterial virulence factors and pathogenesis. BioMed. J. 39 (1), 14–23. doi: 10.1016/j.bj.2015.06.002
Kuo, C. J., Lee, C. H., Chang, M. L., Lin, C. Y., Lin, W. R., Su, M. Y., et al. (2021). Multidrug resistance: The clinical dilemma of refractory Helicobacter pylori infection. J. Microbiol. Immunol. Infect. 54 (6), 1184–1187. doi: 10.1016/j.jmii.2021.03.006
Lertsethtakarn, P., Ottemann, K. M., Hendrixson, D. R. (2011). Motility and chemotaxis in Campylobacter and Helicobacter. Annu. Rev. Microbiol. 65, 389–410. doi: 10.1146/annurev-micro-090110-102908
Leung, W. K., Wong, I. O. L., Cheung, K. S., Yeung, K. F., Chan, E. W., Wong, A. Y. S., et al. (2018). Effects of Helicobacter pylori treatment on incidence of gastric cancer in older individuals. Gastroenterology 155 (1), 67–75. doi: 10.1053/j.gastro.2018.03.028
Loh, J. T., Cover, T. L. (2006). Requirement of histidine kinases HP0165 and HP1364 for acid resistance in Helicobacter pylori. Infect. Immun. 74 (5), 3052–3059. doi: 10.1128/IAI.74.5.3052-3059.2006
Loh, J. T., Gupta, S. S., Friedman, D. B., Krezel, A. M., Cover, T. L. (2010). Analysis of protein expression regulated by the Helicobacter pylori ArsRS two-component signal transduction system. J. Bacteriol 192 (8), 2034–2043. doi: 10.1128/JB.01703-08
Luethy, P. M., Huynh, S., Parker, C. T., Hendrixson, D. R. (2015). Analysis of the activity and regulon of the two-component regulatory system composed by Cjj81176_1484 and Cjj81176_1483 of Campylobacter jejuni. J. Bacteriol 197 (9), 1592–1605. doi: 10.1128/JB.02564-14
Luethy, P. M., Huynh, S., Ribardo, D. A., Winter, S. E., Parker, C. T., Hendrixson, D. R. (2017). Microbiota-derived short-chain fatty acids modulate expression of Campylobacter jejuni determinants required for commensalism and virulence. mBio 8 (3), e00407–e00417. doi: 10.1128/mBio.00407-17
Machuca, M. A., Johnson, K. S., Liu, Y. C., Steer, D. L., Ottemann, K. M., Roujeinikova, A. (2017). Helicobacter pylori chemoreceptor TlpC mediates chemotaxis to lactate. Sci. Rep. 7 (1), 14089. doi: 10.1038/s41598-017-14372-2
MacKichan, J. K., Gaynor, E. C., Chang, C., Cawthraw, S., Newell, D. G., Miller, J. F., et al. (2004). The Campylobacter jejuni dccRS two-component system is required for optimal in vivo colonization but is dispensable for in vitro growth. Mol. Microbiol. 54 (5), 1269–1286. doi: 10.1111/j.1365-2958.2004.04371.x
McDaniel, T. K., Dewalt, K. C., Salama, N. R., Falkow, S. (2001). New approaches for validation of lethal phenotypes and genetic reversion in Helicobacter pylori. Helicobacter 6 (1), 15–23. doi: 10.1046/j.1523-5378.2001.00001.x
Mizuno, S., Yokoyama, K., Nukada, T., Ikeda, Y., Hara, S. (2022). Campylobacter jejuni bacteremia in the term infant a rare cause of neonatal hematochezia. Pediatr. Infect. Dis. J. 41 (4), e156–e157. doi: 10.1097/INF.0000000000003453
Mo, R., Liu, Y., Chen, Y., Mao, Y., Gao, B. (2022). Evolutionary principles of bacterial signaling capacity and complexity. mBio 13 (3), e0076422. doi: 10.1128/mbio.00764-22
Moss, S. F. (2017). The clinical evidence linking Helicobacter pylori to gastric cancer. Cell Mol. Gastroenterol. Hepatol. 3 (2), 183–191. doi: 10.1016/j.jcmgh.2016.12.001
Mourkas, E., Florez-Cuadrado, D., Pascoe, B., Calland, J. K., Bayliss, S. C., Mageiros, L., et al. (2019). Gene pool transmission of multidrug resistance among Campylobacter from livestock, sewage and human disease. Environ. Microbiol. 21 (12), 4597–4613. doi: 10.1111/1462-2920.14760
Muller, S., Pflock, M., Schar, J., Kennard, S., Beier, D. (2007). Regulation of expression of atypical orphan response regulators of Helicobacter pylori. Microbiol. Res. 162 (1), 1–14. doi: 10.1016/j.micres.2006.01.003
Nam, S. Y., Park, B. J., Nam, J. H., Kook, M. C. (2019). Effect of Helicobacter pylori eradication and high-density lipoprotein on the risk of de novo gastric cancer development. Gastrointest Endosc 90 (3), 448–456 e441. doi: 10.1016/j.gie.2019.04.232
Olekhnovich, I. N., Vitko, S., Chertihin, O., Hontecillas, R., Viladomiu, M., Bassaganya-Riera, J., et al. (2013). Mutations to essential orphan response regulator HP1043 of Helicobacter pylori result in growth-stage regulatory defects. Infect. Immun. 81 (5), 1439–1449. doi: 10.1128/IAI.01193-12
Olekhnovich, I. N., Vitko, S., Valliere, M., Hoffman, P. S. (2014). Response to metronidazole and oxidative stress is mediated through homeostatic regulator HsrA (HP1043) in Helicobacter pylori. J. Bacteriol 196 (4), 729–739. doi: 10.1128/JB.01047-13
Oren, A., Garrity, G. M. (2021). Valid publication of the names of forty-two phyla of prokaryotes. Int. J. Syst. Evol. Microbiol. 71 (10), 5056. doi: 10.1099/ijsem.0.005056
Panthel, K., Dietz, P., Haas, R., Beier, D. (2003). Two-component systems of Helicobacter pylori contribute to virulence in a mouse infection model. Infect. Immun. 71 (9), 5381–5385. doi: 10.1128/IAI.71.9.5381-5385.2003
Park, M., Hwang, S., Ryu, S., Jeon, B. (2021). CosR regulation of perR transcription for the control of oxidative stress defense in Campylobacter jejuni. Microorganisms 9 (6) 1281. doi: 10.3390/microorganisms9061281
Parrish, J. R., Yu, J., Liu, G., Hines, J. A., Chan, J. E., Mangiola, B. A., et al. (2007). A proteome-wide protein interaction map for Campylobacter jejuni. Genome Biol. 8 (7), R130. doi: 10.1186/gb-2007-8-7-r130
Pelliciari, S., Pinatel, E., Vannini, A., Peano, C., Puccio, S., De Bellis, G., et al. (2017). Insight into the essential role of the Helicobacter pylori HP1043 orphan response regulator: genome-wide identification and characterization of the DNA-binding sites. Sci. Rep. 7, 41063. doi: 10.1038/srep41063
Pflock, M., Bathon, M., Schar, J., Muller, S., Mollenkopf, H., Meyer, T. F., et al. (2007). The orphan response regulator HP1021 of Helicobacter pylori regulates transcription of a gene cluster presumably involved in acetone metabolism. J. Bacteriol 189 (6), 2339–2349. doi: 10.1128/JB.01827-06
Pflock, M., Finsterer, N., Joseph, B., Mollenkopf, H., Meyer, T. F., Beier, D. (2006). Characterization of the ArsRS regulon of Helicobacter pylori, involved in acid adaptation. J. Bacteriol. 188 (10), 3449–3462. doi: 10.1128/JB.188.10.3449-3462.2006
Pflock, M., Kennard, S., Delany, I., Scarlato, V., Beier, D. (2005). Acid-induced activation of the urease promoters is mediated directly by the ArsRS two-component system of Helicobacter pylori. Infect. Immun. 73 (10), 6437–6445. doi: 10.1128/IAI.73.10.6437-6445.2005
Raphael, B. H., Pereira, S., Flom, G. A., Zhang, Q., Ketley, J. M., Konkel, M. E. (2005). The Campylobacter jejuni response regulator, CbrR, modulates sodium deoxycholate resistance and chicken colonization. J. Bacteriol 187 (11), 3662–3670. doi: 10.1128/JB.187.11.3662-3670.2005
Rokkas, T., Rokka, A., Portincasa, P. (2017). A systematic review and meta-analysis of the role of Helicobacter pylori eradication in preventing gastric cancer. Ann. Gastroenterol. 30 (4), 414–423. doi: 10.20524/aog.2017.0144
Roncarati, D., Scarlato, V., Vannini, A. (2022). Targeting of regulators as a promising approach in the search for novel antimicrobial agents. Microorganisms 10 (1), 185. doi: 10.3390/microorganisms10010185
Roszczenko-Jasinska, P., Wojtys, M. I., Jagusztyn-Krynicka, E. K. (2020). Helicobacter pylori treatment in the post-antibiotics era-searching for new drug targets. Appl. Microbiol. Biotechnol. 104, 9891–9905. doi: 10.1007/s00253-020-10945-w
Salillas, S., Sancho, J. (2020). Flavodoxins as novel therapeutic targets against Helicobacter pylori and other gastric pathogens. Int. J. Mol. Sci. 21, 1881. doi: 10.3390/ijms21051881
Same, R. G., Tamma, P. D. (2018). Campylobacter infections in children. Pediatr. Rev. 39 (11), 533–541. doi: 10.1542/pir.2017-0285
Schar, J., Sickmann, A., Beier, D. (2005). Phosphorylation-independent activity of atypical response regulators of Helicobacter pylori. J. Bacteriol 187 (9), 3100–3109. doi: 10.1128/JB.187.9.3100-3109.2005
Sciortino, S., Arculeo, P., Alio, V., Cardamone, C., Nicastro, L., Arculeo, M., et al. (2021). Occurrence and antimicrobial resistance of arcobacter spp. recovered from aquatic environments. Antibiotics (Basel) 10 (3), 288. doi: 10.3390/antibiotics10030288
Servetas, S. L., Carpenter, B. M., Haley, K. P., Gilbreath, J. J., Gaddy, J. A., Merrell, D. S. (2016). Characterization of key Helicobacter pylori regulators identifies a role for ArsRS in biofilm formation. J. Bacteriol 198 (18), 2536–2548. doi: 10.1128/JB.00324-16
Servetas, S. L., Doster, R. S., Kim, A., Windham, I. H., Cha, J. H., Gaddy, J. A., et al. (2018). ArsRS-dependent regulation of homB contributes to Helicobacter pylori biofilm formation. Front. Microbiol. 9 1497. doi: 10.3389/fmicb.2018.01497
Sharifi, S., Bakhshi, B., Najar-Peerayeh, S. (2021). Significant contribution of the CmeABC efflux pump in high-level resistance to ciprofloxacin and tetracycline in Campylobacter jejuni and Campylobacter coli clinical isolates. Ann. Clin. Microbiol. Antimicrob. 20 (1), 36. doi: 10.1186/s12941-021-00439-6
Spohn, G., Scarlato, V. (1999). Motility of Helicobacter pylori is coordinately regulated by the transcriptional activator FlgR, an NtrC homolog. J. Bacteriol 181 (2), 593–599. doi: 10.1128/JB.181.2.593-599.1999
Svensson, S. L., Davis, L. M., MacKichan, J. K., Allan, B. J., Pajaniappan, M., Thompson, S. A., et al. (2009). The CprS sensor kinase of the zoonotic pathogen Campylobacter jejuni influences biofilm formation and is required for optimal chick colonization. Mol. Microbiol. 71 (1), 253–272. doi: 10.1111/j.1365-2958.2008.06534.x
Svensson, S. L., Huynh, S., Parker, C. T., Gaynor, E. C. (2015). The Campylobacter jejuni CprRS two-component regulatory system regulates aspects of the cell envelope. Mol. Microbiol. 96 (1), 189–209. doi: 10.1111/mmi.12927
Szczepanowski, P., Noszka, M., Zyla-Uklejewicz, D., Pikula, F., Nowaczyk-Cieszewska, M., Krezel, A., et al. (2021). HP1021 is a redox switch protein identified in Helicobacter pylori. Nucleic Acids Res. 49 (12), 6863–6879. doi: 10.1093/nar/gkab440
Thepault, A., Rose, V., Queguiner, M., Chemaly, M., Rivoal, K. (2020). Dogs and cats: reservoirs for highly diverse Campylobacter jejuni and a potential source of human exposure. Anim. (Basel) 10 (5), 838. doi: 10.3390/ani10050838
Turonova, H., Briandet, R., Rodrigues, R., Hernould, M., Hayek, N., Stintzi, A., et al. (2015). Biofilm spatial organization by the emerging pathogen Campylobacter jejuni: comparison between NCTC 11168 and 81-176 strains under microaerobic and oxygen-enriched conditions. Front. Microbiol. 6. doi: 10.3389/fmicb.2015.00709
van der Stel, A. X., van Mourik, A., Heijmen-van Dijk, L., Parker, C. T., Kelly, D. J., van de Lest, C. H., et al. (2015a). The Campylobacter jejuni RacRS system regulates fumarate utilization in a low oxygen environment. Environ. Microbiol. 17 (4), 1049–1064. doi: 10.1111/1462-2920.12476
van der Stel, A. X., van Mourik, A., Laniewski, P., van Putten, J. P., Jagusztyn-Krynicka, E. K., Wosten, M. M. (2015b). The Campylobacter jejuni RacRS two-component system activates the glutamate synthesis by directly upregulating gamma-glutamyltranspeptidase (GGT). Front. Microbiol. 6. doi: 10.3389/fmicb.2015.00567
Waidner, B., Melchers, K., Stahler, F. N., Kist, M., Bereswill, S. (2005). The Helicobacter pylori CrdRS two-component regulation system (HP1364/HP1365) is required for copper-mediated induction of the copper resistance determinant CrdA. J. Bacteriol 187 (13), 4683–4688. doi: 10.1128/JB.187.13.4683-4688.2005
Waite, D. W., Vanwonterghem, I., Rinke, C., Parks, D. H., Zhang, Y., Takai, K., et al. (2017). Comparative genomic analysis of the class Epsilonproteobacteria and proposed reclassification to epsilonbacteraeota (phyl. nov.). Front. Microbiol. 8. doi: 10.3389/fmicb.2017.00682
Wen, Y., Feng, J., Scott, D. R., Marcus, E. A., Sachs, G. (2007). The HP0165-HP0166 two-component system (ArsRS) regulates acid-induced expression of HP1186 alpha-carbonic anhydrase in Helicobacter pylori by activating the pH-dependent promoter. J. Bacteriol 189 (6), 2426–2434. doi: 10.1128/JB.01492-06
Wosten, M. M., Parker, C. T., van Mourik, A., Guilhabert, M. R., van Dijk, L., van Putten, J. P. (2006). The Campylobacter jejuni PhosS/PhosR operon represents a non-classical phosphate-sensitive two-component system. Mol. Microbiol. 62 (1), 278–291. doi: 10.1111/j.1365-2958.2006.05372.x
Wosten, M. M., van Dijk, L., Parker, C. T., Guilhabert, M. R., van der Meer-Janssen, Y. P., Wagenaar, J. A., et al. (2010). Growth phase-dependent activation of the DccRS regulon of Campylobacter jejuni. J. Bacteriol 192 (11), 2729–2736. doi: 10.1128/JB.00024-10
Wosten, M. M., Wagenaar, J. A., van Putten, J. P. (2004). The FlgS/FlgR two-component signal transduction system regulates the fla regulon in Campylobacter jejuni. J. Biol. Chem. 279 (16), 16214–16222. doi: 10.1074/jbc.M400357200
Yamada, S., Sugimoto, H., Kobayashi, M., Ohno, A., Nakamura, H., Shiro, Y. (2009). Structure of PAS-linked histidine kinase and the response regulator complex. Structure 17 (10), 1333–1344. doi: 10.1016/j.str.2009.07.016
Zannoni, A., Pelliciari, S., Musiani, F., Chiappori, F., Roncarati, D., Scarlato, V. (2021). Definition of the binding architecture to a target promoter of HP1043, the essential master regulator of Helicobacter pylori. Int. J. Mol. Sci. 22 (15), 7848. doi: 10.3390/ijms22157848
Keywords: two-component regulatory system, Epsilonproteobacteria, Helicobacter pylori, Campylobacter jejuni, orphan response regulator, therapeutic target, Campylobacterota
Citation: Casado J, Lanas Á and González A (2022) Two-component regulatory systems in Helicobacter pylori and Campylobacter jejuni: Attractive targets for novel antibacterial drugs. Front. Cell. Infect. Microbiol. 12:977944. doi: 10.3389/fcimb.2022.977944
Received: 25 June 2022; Accepted: 08 August 2022;
Published: 24 August 2022.
Edited by:
Charles Martin Dozois, Institut National de la Recherche Scientifique, Université du Québec, CanadaReviewed by:
Marja-Liisa Hänninen, University of Helsinki, FinlandCopyright © 2022 Casado, Lanas and González. This is an open-access article distributed under the terms of the Creative Commons Attribution License (CC BY). The use, distribution or reproduction in other forums is permitted, provided the original author(s) and the copyright owner(s) are credited and that the original publication in this journal is cited, in accordance with accepted academic practice. No use, distribution or reproduction is permitted which does not comply with these terms.
*Correspondence: Andrés González, YW5kcmVzZ2xlenJvZEBnbWFpbC5jb20=
†These authors share senior authorship