- 1Department of Rheumatology and Immunology, Tianjin Medical University General Hospital, Tianjin, China
- 2Discipline Construction Office, Tianjin Medical University, Tianjin, China
- 3Department of Biochemistry and Molecular Biology, Department of Immunology, Key Laboratory of Immune Microenvironment and Disease (Ministry of Education), Key Laboratory of Cellular and Molecular Immunology in Tianjin, Excellent Talent Project, The Province and Ministry Co-sponsored Collaborative Innovation Center for Medical Epigenetics, School of Basic Medical Science, Tianjin Medical University, Tianjin, China
As a set of inflammatory disorders, spondyloarthritis (SpA) exhibits distinct pathophysiological, clinical, radiological, and genetic characteristics. Due to the extra-articular features of this disorder, early recognition is crucial to limiting disability and improving outcomes. Gut dysbiosis has been linked to SpA development as evidence grows. A pathogenic SpA process is likely to occur when a mucosal immune system interacts with abnormal local microbiota, with subsequent joint involvement. It is largely unknown, however, how microbiota alterations predate the onset of SpA within the “gut-joint axis”. New microbiome therapies, such as probiotics, are used as an adjuvant therapy in the treatment of SpA, suggesting that the modulation of intestinal microbiota and/or intestinal barrier function may contribute to the prevention of SpA. In this review, we highlight the mechanisms of SpA by which the gut microbiota impacts gut inflammation and triggers the activation of immune responses. Additionally, we analyze the regulatory role of therapeutic SpA medication in the gut microbiota and the potential application of probiotics as adjunctive therapy for SpA.
Introduction
Spondyloarthritis (SpA) is a family of clinical disorders with some featured manifestations, etiopathological characteristics and genetic factors (Moz et al., 2017; Duba and Mathew, 2018; Terenzi et al., 2018). SpA primarily features sacroiliitis, namely, axial SpA (axSpA), and the peripheral joint can also be involved (pSpA) (Sieper et al., 2009; Rudwaleit et al., 2011; Stolwijk et al., 2012; Dubreuil and Deodhar, 2017; van der Heijde et al., 2017). Back pain is a major symptom of axSpA, and exercise can improve pain and stiffness (Rudwaleit and Sieper, 2012; Bidad et al., 2017). Additionally, there are other musculoskeletal manifestations (e.g., arthritis, enthesitis, dactylitis, etc.) and extra-articular manifestations (e.g., psoriasis, anterior uveitis, etc.) for axSpA (Duba and Mathew, 2018; Magrey et al., 2020). In recent years, the term “axSpA” has been used more commonly to cover this group of SpA diseases (Sieper and Poddubnyy, 2017; Ritchlin and Adamopoulos, 2021; Robinson et al., 2021; Danve and Deodhar, 2022).
Based on the sacroiliac joint radiological results, axSpA can be classified further into radiologically positive axSpA with definite imaging damage (r-axSpA) and radiologically negative axSpA, namely, non-radiographic axSpA (nr-axSpA) (Sieper and Poddubnyy, 2017; Ritchlin and Adamopoulos, 2021; Robinson et al., 2021; Danve and Deodhar, 2022). There are some patients with nr-axSpA who progress to r-axSpA (Protopopov and Poddubnyy, 2018). Ankylosing spondylitis (AS) is the best studied subtype of axSpA at the later stage (Dougados and Baeten, 2011; Sieper and Poddubnyy, 2017). Enteropathic arthritis (EPA), reactive arthritis (ReA), and psoriatic arthritis (PsA) are the common types of pSpA (Wilson and Folzenlogen, 2012; Taams et al., 2018). New imaging modalities, biomarkers, and genetic data may be available to update the classification criteria of SpA (Sieper et al., 2009; Rudwaleit et al., 2011; Dubreuil and Deodhar, 2017; van der Heijde et al., 2017). Emerging evidence supports the idea that SpA and inflammatory bowel disease (IBD) share similarities in their genetic predisposition and pathogenesis (Olivieri et al., 2014; Karreman et al., 2017; Fragoulis et al., 2019; Qaiyum et al., 2021). Thus, IBD-associated SpA was a concern in this study.
Based on the most recent research on the relationship between SpA and intestinal inflammation, we summarize the evidence that supports intestinal dysbiosis in SpA. Specifically, the mechanisms by which dysbiosis contributes to subclinical intestinal inflammation and immune response activation to SpA will help us to understand the idea of the “gut-joint axis”. To this end, we outline the effects of current SpA medication treatment options on the gut microbiota with a focus on research findings in the field of microbiota-targeted precision therapy. Lastly, some thoughts were provided on the underlying mechanisms and modulation of the intestinal microbiota as potential new treatment approaches to SpA.
Intestinal microbial dysbiosis in SpA
The human microbiota contains a plethora of parasitic or symbiotic microorganisms, such as bacteria, viruses, and fungi (Miller et al., 2021). The intestine is crucial for maintaining homeostasis between the microbiota and the host, which involves the effective coordination of different immune cells (Tiffany and Bäumler, 2019). The number of cells in the typical flora of the human colon has been estimated to reach 1012 colony forming units (CFU) (Sekirov et al., 2010). The intestinal flora primarily consist of Bacteroidetes, Firmicutes, Actinobacteria, and Proteobacteria (Honda and Littman, 2012). The gut microbiota is associated with human metabolic and physiological activities, including balancing the immune response and regulating intestinal endocrine function and amino acid metabolism (Honda and Littman, 2012; Lynch and Pedersen, 2016; Dupont et al., 2020). Dysbiosis means the occurrence of a microbiome imbalance when environmental factors or host-related factors alter the composition and function of microbial communities, which is related to a series of clinical disorders (Brandl and Schnabl, 2015; Tiffany and Bäumler, 2019).
Emerging publications report the relationship between the gut microbiota and SpA. We summarized the recent findings on the changes in the gut microbiota under SpA in Table 1. There is no consensus on which bacterial species are linked to the development of SpA. Several candidate microbes were identified as potential drivers of gut inflammation in experimental SpA induced by HLAB27 (Gill et al., 2019). Prevotella and Blautia (Lewis rats) and Akkermansia muciniphila, rc4-4, Lachnospira, and Lachnospiraceae (Fischer rats) were found to be closely related to the dysregulated inflammatory pathways (Gill et al., 2019). The emergence of joint damage resembling Reiter’s syndrome in a ship’s crew sick with bacterial dysentery prompted researchers to investigate the link between the intestine and arthritis (Noer, 1966). Mounting evidence supports the functional links of SpA and gastrointestinal inflammation (Rizzo et al., 2018), which are closely related to intestinal microbial dysbiosis (Ni et al., 2017). Animal experiments have revealed that transgenic rats harboring HLA-B27 displayed clinical symptoms comparable to human SpA but without further development under sterile conditions, which indicates that intestinal microbes are essential for the onset of SpA (Taurog et al., 1994).
Mechanisms of microbiota-derived intestinal inflammation in SpA
Microbial dysbiosis of gut commensals has been implicated in SpA and is highly related to gut inflammation (Gill et al., 2015). Patients with SpA frequently suffer from IBD (Fragoulis et al., 2019), and there are similarities in the aberrations of the gut microbiota under IBD and AS (Klingberg et al., 2019). The mechanism underlying how intestinal inflammation causes immune damage to peripheral organs is currently inconclusive. Three points are under consideration. (1) There may be no inherent relationship, but rather a simple overlap of clinical characteristics, between axSpA with subclinical intestinal inflammation and IBD with sacroiliitis. (2) A-specific factor causes SpA to experience both intestinal and joint inflammation in parallel. Genetic, immune, and environmental factors all have a direct impact on the immune system, which in turn induces the clinical immune feature of SpA. This may partly explain why some individual SpA cases have only joint immune changes but no intestinal symptoms. (3) The “gut-joint axis” hypothesis, which is currently very popular, indicates possible links between intestinal and joint pathology. Genetic, immune, and environmental factors first affect the gut and induce dysbiosis in the microbiota through a series of regulatory mechanisms (e.g., arthritic peptide recognition, clonal expansion, etc., and ultimately lead to the inflammation of other sites, such as joints (Gracey et al., 2020; Zaiss et al., 2021).
Mounting evidence supports the functional link between the gut dysbiosis-related immune system response and SpA. Th17 cells are vital in host defense against external microbes and fungi, and IL-23 signaling is required for the maturation and stability of the pro-inflammatory Th17 phenotype (Schinocca et al., 2021). The IL-23/IL-17 axis and associated cytokines have been implicated in the etiology of SpA (Tsukazaki and Kaito, 2020; Lukasik et al., 2021). In addition to Th17 cells, some innate-like T-cell subsets that express the IL-23 receptor, such as mucosa-associated invariant T (MAIT) cells, T cells, invariant natural killer T cells (iNKT), and type 3 innate lymphocytes (ILC3), boost the type 3 immune response and play a role in the pathogenesis of SpA (Sherlock et al., 2012; Mauro et al., 2019). For instance, upon stimulation with bacterial products, ILC3s can induce an inflammatory response through the production of IL-17 and link the gut microbiota and local/systemic immunity (Annunziato et al., 2015; Asquith et al., 2019). Other immune cells, including mast cells and macrophages, can further amplify the inflammatory effect by secreting cytokines such as IL-23, IL-17, IL-1, IL-22, and tumor necrosis factor (TNF)-α (Annunziato et al., 2015). MAIT cells are capable of producing pro-inflammatory cytokines, such as IL-17, IL-22, TNF, or interferon (IFN)-γ (Gracey et al., 2016; Toussirot and Saas, 2018; Li et al., 2022). Taken together, these findings show that various immune cell-mediated IL-23/IL-17 axes contribute to the migration of blood vessels.
Here, we propose an IL-23/IL-17 axis-based mechanistic model regarding intestinal inflammation driving immune damage in peripheral joints. As shown in Figure 1, our model includes three parts, namely, “immunological changes in the gut”, “cytokine cascades initiated in blood vessels”, and “targeting migration and immune response of extraintestinal joint sites”. Detailed information is shown in the legend of Figure 1. Accumulating evidence suggests that impaired intestinal barrier structure and function, as characterized by altered tight junction protein density and high intestinal permeability, may increase the entry of bacterial and/or microbiota-derived inflammatory components into the circulatory system (Vaile et al., 1999; Gill et al., 2015; Ciccia et al., 2017). For instance, as a modulator of high intestinal permeability, zonulin protein is highly expressed and implicated in damage to the intestinal mucosal barrier and gut vascular barrier (Ciccia et al., 2017). Fluctuations in the amount, variety, and function of the core gut microbiome can cause increased gut permeability (Brandl and Schnabl, 2015). Herein, IL-23/IL-17 axis-mediated gut bacteria and metabolites influence gut immune mechanisms and disrupt the gut barrier integrity. The “aberrant cell trafficking hypothesis (recirculation)” mimics the recruitment of mucosal-derived cells to joints, in which T cells and macrophages in the gut activate and then recirculate to the joints (Qaiyum et al., 2021). Evidence on adhesion molecules (e.g., integrin, etc.) that determine the homing pattern of circulating lymphocytes may be involved in the mechanism and process of immune-competent cell migration from the intestinal mucosa. For instance, MAIT cells have been isolated from the synovial fluid of AS patients (Gracey et al., 2016). Integrin-expressing T cells proliferate in the inflamed joints of AS patients, and α4β7 integrin promotes T lymphocyte migration from the gut to the synovium (Qaiyum et al., 2019). Thus, cytokine cascades of IL-17 are initiated in blood vessels and migrate to the extraintestinal axial/peripheral joint sites for immune interference, probably through an adhesion molecular-involved mechanism.
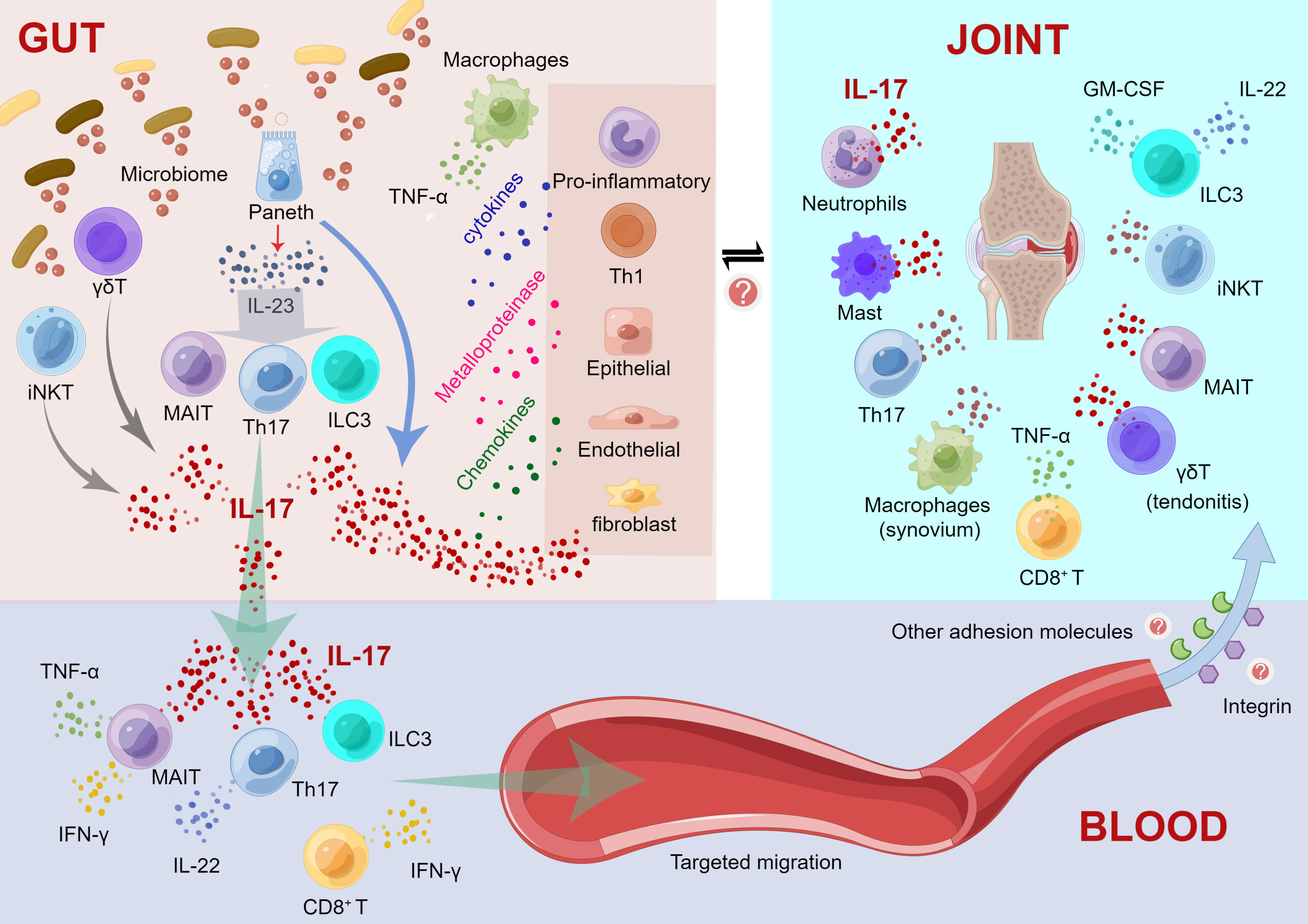
Figure 1 Mechanistic model regarding the intestinal inflammation driving immune damage in peripheral joints. (1) Immunological changes in the gut. a) Activated Paneth cells produce IL-23 and IL-17 after recognizing the altered microbiome; IL-23 causes the differentiation of Th17, ILC3 and MAIT cells; and these activated cells foster the production of elevated levels of IL-17. IL-17 acts as an inflammatory mediator to stimulate the production of cytokines by other proinflammatory cells and Th1 cells and promotes the production of metalloproteinases and chemokines by macrophages, epithelial cells, endothelial cells, and fibroblasts, thereby triggering and maintaining inflammation. b) γδ T and iNKT cells can recognize microbial antigens and release IL-17. c) Simultaneously, macrophage recruitment promoted the secretion of TNF-α. (2) Cytokine cascades initiated in blood vessels. a) IL-17, Th17 cells, ILC3 and MAIT cells can migrate through the intestinal mucosal barrier to the blood, and these cells may transfer inflammation to the joints. b) Th17, γδT, ILC3 cells and MAIT cells are induced to produce IL-17 in the bloodstream; Th17 cells also produce IL-22. c) MAIT cells also contribute to the production of TNF-α and IFN-γ. d) CD8+ T cells produce IFN-γ. (3) Targeting migration and immune response of extraintestinal joint sites. a) Activated cytokines migrate to inflammatory sites, such as the axial/peripheral joints, for immune interference. b) Some adhesion molecules, such as integrins, may contribute to the target migration of these immune cells circulating in the blood to peripheral joints. c) In peripheral joints, neutrophils, mast cells, Th17 cells, CD8+ T cells, MAIT cells and iNKT cells induce IL-17 production. ILC3 cells produce IL-22 and GM-CSF, whereas IL-17 is only produced in axial joints. d) γδT cells promote tendonitis through elevated IL-17. e) Macrophages induce TNF-α production in the synovium. This figure was drawn by Figdraw.
SpA therapeutic medication and gut microbiome
Currently, non-steroidal anti-inflammatory drugs (NSAIDs) are the first choice for pharmacological treatment of axSpA, according to the guideline panel (Sieper and Poddubnyy, 2017; Ritchlin and Adamopoulos, 2021; Robinson et al., 2021; Danve and Deodhar, 2022). Disease-modifying anti-rheumatic drugs (DMARDs) have some effect on axSpA patients with peripheral arthritis, but their efficacy in most SpA patients remains debatable (Ward et al., 2019). DMARDs can be further divided into several categories, including traditional DMARDs or conventional synthetic DMARDs (csDMARDs), biologic DMARDs (bDMARDs), and targeted synthetic DMARDs (tsDMARDs) (Buer, 2015; Smolen et al., 2020). bDMARDs and tsDMARDs are recommended for adults with active AS despite treatment with NSAIDs (Ward et al., 2019). Considering the close links between SpA and the gut microbiota (Table 1), we reviewed the potential association between different SpA therapeutic medications and the gut microbiome.
NSAIDs
For SpA patients, NSAIDs can effectively alleviate the symptoms of SpA, such as morning stiffness and joint pain (Queiro-Silva et al., 2021). Even so, different NSAID treatments can cause a distinct alteration in the bacterial composition, which contributes to intestinal damage during NSAID treatment (Rogers and Aronoff, 2016; Otani et al., 2017). A reduction in mouse NSAID-induced enteropathy can be achieved by inhibiting bacterial β-glucuronidase enzyme activity (Saitta et al., 2014).
csDMARDs
Even though csDMARDs [e.g., methotrexate (MTX), sulfasalazine (SSZ), hydroxychloroquine (HCQ), etc.] are not the first choice for axSpA, the subtypes of peripheral joint manifestations for PsA and EPA involve csDMARDs (Mease, 2012; Chimenti et al., 2019; Scher et al., 2020; Jacobs et al., 2021). The treatment efficacy of the MTX and SSZ combination was observed for active axSpA patients in a prospective cohort study (Ganapati et al., 2021). Mounting evidence supports the functional links of csDMARDs and gut microbiota. For instance, the gut microbiome is essential for the treatment effect and prognostic evaluation of MTX for patients with rheumatoid arthritis (RA) (Scher et al., 2020; Artacho et al., 2021). Restored gut dysbiosis symptoms were observed in a rat model of experimental colitis after treatment with SSZ (Zheng et al., 2017). Following treatment with a short-term high dose of HCQ, female C57BL/6J mice showed altered gut microbiota rather than immunological responses (Pan et al., 2021). However, there are few studies on the effect of csDMARDs on the intestinal flora of SpA, which merits further investigation.
bDMARDs
Tumor necrosis factor inhibitors (TNFi) and interleukin-17 inhibitors (IL-17i), two main types of bDMARDs, can be considered for patients who have failed or are intolerant to NSAID therapy (van der Heijde et al., 2017). TNF-α antagonists have specific therapeutic effects on SpA patients (Navarro-Compán et al., 2021) and exhibit considerable microbiota recovery (Chen et al., 2021; Ditto et al., 2021). Furthermore, there is a cross-influence between TNFi treatment and intestinal microbiota. For instance, SpA patients may respond to anti-TNF-α treatment more effectively if they exhibit a specific fecal microbiota signature (Bazin et al., 2018). Some microbes can serve as indicators of the therapeutic responsiveness of TNFi treatment for AS patients (Zhang et al., 2020). The lowering of bacterial arthritic peptides has been demonstrated to contribute to the improvement of the gut microbiome following TNFi treatment (Yin et al., 2020). Recent studies targeting IL-17 have provided new ideas for treating refractory SpA that has not responded to previous treatments (Gladman et al., 2019; van der Heijde et al., 2020; Schett et al., 2021). The IL-17 axis has a great deal of promise in terms of enhancing SpA therapy options (Smith and Colbert, 2014), and the potential role of gut dysbiosis can be considered.
tsDMARDs
tsDMARDs, such as Janus kinase (JAK) inhibitors, have begun to be used in clinical trials with promising outcomes (Gladman et al., 2017; Toussirot, 2022). There have been limited studies of longitudinal changes in the gut microbiome with tsDMARD treatment in SpA. JAK inhibition has been found to have an indirect effect on the production of critical cytokines implicated in the pathogenesis of SpA as well as the triggering and maintenance of immunological responses (Veale et al., 2019; Ritchlin and Adamopoulos, 2021).
New microbiome therapies such as probiotics
Probiotics have emerged as a new topic of SpA study as research into the involvement of gut microorganisms in the pathophysiology of the disease continues to intensify. Probiotics are described as living microorganisms that provide health advantages to the host when given in sufficient amounts (Hill et al., 2014). Lactobacillus, Bifidobacterium, and yeast are the most prevalent probiotics (Ashraf and Shah, 2014; Cunningham et al., 2021). Probiotics can be made up of a single strain, a mixture of strains, or a combination of both.
Mechanism of action of probiotics
Probiotics have been researched in vivo, in vitro, and in animal models for their anti-inflammatory properties. Probiotics have been shown to improve the microbiota by modifying the gut environment, suppressing harmful bacterial growth, and preventing further immune system damage caused by inflammatory diseases (Shamoon et al., 2019). The mechanism of action of probiotics is usually strain-specific, interacting with the host and microbiome primarily through molecular effectors present on cell structures or secreted as metabolites (Cunningham et al., 2021). The basic mechanisms of action include promoting the growth of beneficial microorganisms in the intestinal microbiota, influencing immunological function, strengthening the intestinal barrier, competing with harmful microbes in the gut, and producing organic acids and antimicrobial compounds (Plaza-Diaz et al., 2019).
The immune response can be modulated by influencing cells involved in innate and adaptive immunity. Epithelial cells, dendritic cells (DCs), natural killer cells (NKs), macrophages, and lymphocytes are all affected by probiotics through the Toll-like activation of signaling pathways that regulate cell proliferation and cytokine production (Bermudez-Brito et al., 2012). The ability of probiotics to modulate the cytokine profile of DCs is strain-specific (Borchers et al., 2009; Cristofori et al., 2021)Furthermore, some probiotics can mediate the differentiation from B cells to IgA-producing plasma cells, and secretory IgA protects against infections by restricting bacterial attachment to the epithelium and inhibiting the penetration of host tissue (Liu et al., 2018).
Probiotics can also engage with the host immune system indirectly. Through the manipulation of the gut epithelial barrier and mucus layer properties, the release of antimicrobial compounds, and the management of competition with pathogenic bacteria, specific probiotic metabolites may exert anti-inflammatory and antibacterial effects. Immune responses and systemic inflammation are also influenced by probiotic-driven metabolites such as short-chain fatty acids (SCFAs), which modulate immune cell activity (Oliviero and Spinella, 2020). This characteristic may contribute to the correction of intestinal wall hyperpermeability in the “gut-joint axis”. The major mechanism of probiotic action is illustrated in Figure 2.
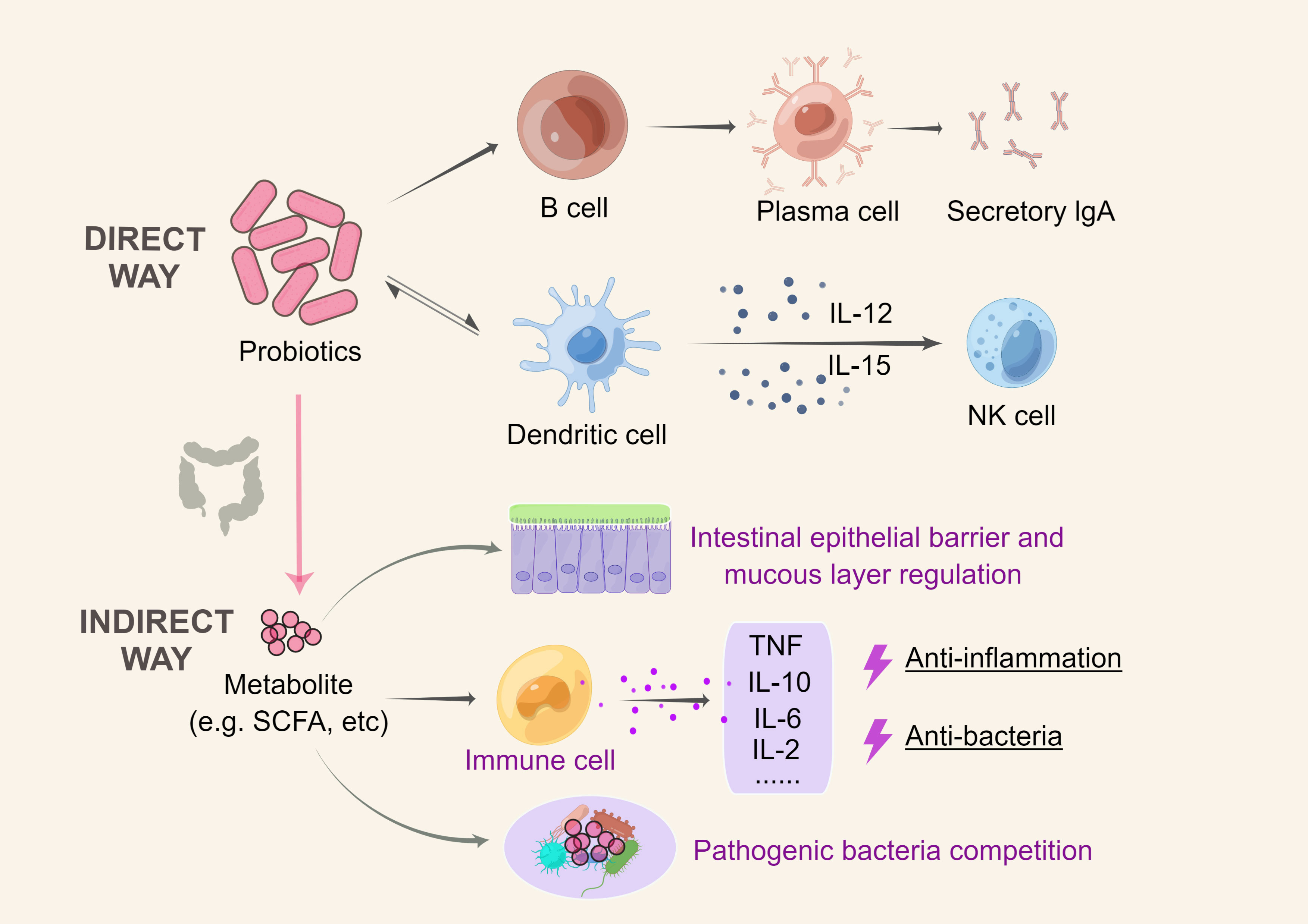
Figure 2 Mechanism of action of probiotics. Direct mechanism: probiotics can activate sentinel cells through the Toll-like activation of signaling pathways; DCs can drive NK cell activation by secreting cytokines such as IL-12 and IL-15, and probiotics can impact this pathway; probiotics can cause B cells to differentiate into IgA-producing plasma cells; and probiotics also interact with antigen-presenting cells to influence the reduction of proinflammatory cytokines, thereby triggering an adaptive response. Indirect mechanism: Through the manipulation of the gut epithelial barrier and mucus layer properties, probiotic release of antimicrobial compounds, and management of competition with pathogenic bacteria, specific probiotic metabolites may exert anti-inflammatory and antibacterial effects. This figure was drawn by Figdraw.
Clinical studies of probiotics as adjunctive therapy in SpA
Probiotics can theoretically be used to manipulate the microbiome as a promising adjunct therapy for SpA. As mentioned above, there are multiple extraintestinal manifestations of IBD, of which arthritis is one of the most common and is defined as EPA, an important member of the SpA disease spectrum. In this study, we provide a summary of current clinical trials using probiotics for IBD in Table 2. The number of controlled studies on probiotics for SpA is small, and the results of the few studies that have been conducted are not encouraging (Sanchez et al., 2022). Sanges et al. reported that a probiotic mixture containing Lactobacillus acidophilus and Lactobacillus salivarius is able to reduce arthritis disease scores in SpA patients with quiescent colitis, which may help in the management of SpA in patients with ulcerative colitis (Sanges et al., 2009). The findings of Lowe et al. suggest that combining Bifidobacterium and Lactobacillus preparations may have benefits in terms of pain relief, lower C-reactive protein (CRP), and improved quality of life. However, compared to RA patients, SpA patients did not benefit as much from CRP reduction (Lowe et al., 2020).
The efficacy of probiotics in SpA is currently uncertain. There are several causes for this outcome. First, the quality of all current clinical data is poor, sample sizes are small, and the risk of bias and imprecision is significant, necessitating the performance of more randomized controlled trials. Second, the type of inflammatory change, illness severity, microbiota features, and potential confounding factors, such as age, sex, food, and individual microbiological characteristics, must all be considered. Lastly, the anti-inflammatory efficacy of probiotics is incredibly reliant on the dose and strain. It is possible that different bacterial strains have different functions in relation to their hosts.
Due to limited survival rates and/or competition with the indigenous gut microbiome, delivering live bacteria via probiotics is difficult. Probiotic effects can be mediated via their metabolites or biological components, such as postbiotics. Amino acid derivatives altered by the gut microbiota could be a type of postbiotic that has anti-inflammatory properties by attaching to specific receptors on intestinal epithelial cells (Żółkiewicz et al., 2020). Because of their stability, synbiotics, such as “nonviable” microbial cells or crude cell extracts, can benefit both humans and animals if present in sufficient amounts (Vallejo-Cordoba et al., 2020). Probiotic bacteria can be manipulated in an unlimited manner with emerging biological engineering tools. In response to externally supplied substrates, genetically modified bacterial/probiotic strains can be employed to detect early inflammatory markers as well as to distribute and generate therapeutic compounds to the mucosal surface, hence boosting the overall efficiency of the system. It is also worthy of in-depth investigation for other gut microbiota-targeted adjuvant therapies of SpA, such as fecal microbiota transplantation (FMT) and dietary treatment.
Some thoughts
1. It is time to rethink the heterogeneity of the SpA disease class and its underlying mechanisms. We only have a rudimentary understanding of the known associations with the microbiota in SpA-related diseases right now. However, the exact mechanisms through which the microbiome contributes to SpA in the axial or peripheral skeleton are still unknown, and the mechanisms by which the combined effects of the gut microbiome, immune system, and host genetics contribute to tissue damage will continue to be a hot topic for future research.
2. There is both commonality and specificity in dysbiosis among key disease subtypes, such as AS, IBD, and PsA, in SpA, implying a complicated relationship in the etiology of these heterogeneous disorders. The genetic background of these diseases has a high degree of overlap, but the clinical manifestations are significantly heterogeneous. It is not clear whether there is a connection between gut microbiota and joint lesions and whether there are other factors involved, weaving a relatively complex network of infection, immunity, and injury. Using SpA as an example to identify microbes that influence human disease susceptibility and phenotype will be a considerable challenge.
3. Does gut flora research have the potential to aid us in treating SpA with precision? Early diagnosis, early intervention, early treatment, and effective management of SpA have remained unsolved challenges in clinical practice, often leading to delayed diagnosis and precise individualized treatment. Advances in detection technologies such as sequencing and multiomics approaches such as metabolomics have allowed us to delve deeper into the links between ecological dysregulation, bacterial metabolites, and disease development and will provide new insights into the unraveling of this diverse group of diseases. Further research into mechanisms such as the ‘gut-joint’ axis may allow physicians to characterize SpA and disease progression with specific biomarkers to aid early diagnosis and provide individualized and precise treatment. The gut microbiome’s plasticity has also prompted researchers to assess the viability of precision therapy based on the gut microbiome’s SpA and to design new targeted therapies.
Conclusions
The gut microbiota has emerged as a major focus of investigation into the pathogenesis of SpA. Although definitive proof of causality is still lacking, dysbiosis is linked to the pathogenesis of HLA-B27-associated SpAs. Notably, there is an interaction between the gut flora and the efficacy of current conventional therapeutic agents, and detailed studies on the gut-joint axis suggest that more targeted bDMARDs are a promising therapeutic area. Large-scale longitudinal studies and cross-sectional clinical trials are required to investigate the microbiota as a potential biomarker and its role in the prevention or treatment of SpA. According to the available literature, probiotics are extensively researched for use in SpA as adjunctive therapy. Nevertheless, the high heterogeneity in study design due to the use of different strains, quantities, and timing of supplementation makes it difficult to conclude whether probiotics are effective at this time. Engineered microbes, however, could be a more promising topic. Future microbiomics investigations and new analytical tools, such as bioinformatics, will allow for the more extensive study of host-microbiota interactions, providing new insights into the pathophysiology of SpA and, ideally, translating into therapeutically useful therapies.
Author contributions
XL, JC, XG, and JY conceived and drafted the study. JY screened abstracts, XL, JC, and XG collected all data. XL and JC drafted the manuscript. JY performed critical revisions of the manuscript. All authors have approved the final draft of the manuscript.
Funding
This work was supported by grants from National Nature Science Foundation of China (31870747, 32070724), Tianjin Natural Science Foundation Project (20JCYBJC00470).
Conflict of interest
The authors declare that the research was conducted in the absence of any commercial or financial relationships that could be construed as a potential conflict of interest.
Publisher’s note
All claims expressed in this article are solely those of the authors and do not necessarily represent those of their affiliated organizations, or those of the publisher, the editors and the reviewers. Any product that may be evaluated in this article, or claim that may be made by its manufacturer, is not guaranteed or endorsed by the publisher.
References
Żółkiewicz, J., Marzec, A., Ruszczyński, M., Feleszko, W. (2020). Postbiotics-a step beyond pre- and probiotics. Nutrients 12 (8), 2189. doi: 10.3390/nu12082189
Alam, M. T., Amos, G. C. A., Murphy, A. R. J., Murch, S., Wellington, E. M. H., Arasaradnam, R. P. (2020). Microbial imbalance in inflammatory bowel disease patients at different taxonomic levels. Gut Pathog. 12, 1. doi: 10.1186/s13099-019-0341-6
Annunziato, F., Romagnani, C., Romagnani, S. (2015). The 3 major types of innate and adaptive cell-mediated effector immunity. J. Allergy Clin. Immunol. 135 (3), 626–635. doi: 10.1016/j.jaci.2014.11.001
Artacho, A., Isaac, S., Nayak, R., Flor-Duro, A., Alexander, M., Koo, I., et al. (2021). The pretreatment gut microbiome is associated with lack of response to methotrexate in new-onset rheumatoid arthritis. Arthritis Rheumatol. 73 (6), 931–942. doi: 10.1002/art.41622
Ashraf, R., Shah, N. P. (2014). Immune system stimulation by probiotic microorganisms. Crit. Rev. Food. Sci. Nutr. 54 (7), 938–956. doi: 10.1080/10408398.2011.619671
Asquith, M., Sternes, P. R., Costello, M. E., Karstens, L., Diamond, S., Martin, T. M., et al. (2019). HLA alleles associated with risk of ankylosing spondylitis and rheumatoid arthritis influence the gut microbiome. Arthritis Rheumatol. 71 (10), 1642–1650. doi: 10.1002/art.40917
Bazin, T., Hooks, K. B., Barnetche, T., Truchetet, M. E., Enaud, R., Richez, C., et al. (2018). Microbiota composition may predict anti-tnf alpha response in spondyloarthritis patients: an exploratory study. Sci. Rep. 8 (1), 5446. doi: 10.1038/s41598-018-23571-4
Bermudez-Brito, M., Plaza-Diaz, J., Munoz-Quezada, S., Gomez-Llorente, C., Gil, A. (2012). Probiotic mechanisms of action. Ann. Nutr. Metab. 61 (2), 160–174. doi: 10.1159/000342079
Bibiloni, R., Fedorak, R. N., Tannock, G. W., Madsen, K. L., Gionchetti, P., Campieri, M., et al. (2005). VSL3 probiotic-mixture induces remission in patients with active ulcerative colitis. Am. J. Gastroenterol. 100 (7), 1539–1546. doi: 10.1111/j.1572-0241.2005.41794.x
Bidad, K., Gracey, E., Hemington, K. S., Mapplebeck, J. C. S., Davis, K. D., Inman, R. D. (2017). Pain in ankylosing spondylitis: a neuro-immune collaboration. Nat. Rev. Rheumatol. 13 (7), 410–420. doi: 10.1038/nrrheum.2017.92
Bjarnason, I., Sission, G., Hayee, B. (2019). A randomised, double-blind, placebo-controlled trial of a multi-strain probiotic in patients with asymptomatic ulcerative colitis and crohn’s disease. Inflammopharmacology 27 (3), 465–473. doi: 10.1007/s10787-019-00595-4
Borchers, A. T., Selmi, C., Meyers, F. J., Keen, C. L., Gershwin, M. E. (2009). Probiotics and immunity. J. Gastroenterol. 44 (1), 26–46. doi: 10.1007/s00535-008-2296-0
Bousvaros, A., Guandalini, S., Baldassano, R. N., Botelho, C., Evans, J., Ferry, G. D., et al. (2005). A randomized, double-blind trial of lactobacillus GG versus placebo in addition to standard maintenance therapy for children with crohn’s disease. Inflamm. Bowel. Dis. 11 (9), 833–839. doi: 10.1097/01.mib.0000175905.00212.2c
Brandl, K., Schnabl, B. (2015). Is intestinal inflammation linking dysbiosis to gut barrier dysfunction during liver disease? Expert. Rev. Gastroenterol. Hepatol. 9 (8), 1069–1076. doi: 10.1586/17474124.2015.1057122
Breban, M., Tap, J., Leboime, A., Said-Nahal, R., Langella, P., Chiocchia, G., et al. (2017). Faecal microbiota study reveals specific dysbiosis in spondyloarthritis. Ann. Rheumatol. Dis. 76 (9), 1614–1622. doi: 10.1136/annrheumdis-2016-211064
Buer, J. K. (2015). A history of the term “DMARD”. Inflammopharmacology 23 (4), 163–171. doi: 10.1007/s10787-015-0232-5
Chen, Z. N., Zheng, X. Q., Wu, X. Y., Wu, J. L., Li, X. M., Wei, Q. J., et al. (2021). Adalimumab therapy restores the gut microbiota in patients with ankylosing spondylitis. Front. Immunol. 12. doi: 10.3389/fimmu.2021.700570
Chimenti, M. S., Conigliaro, P., Triggianese, P., Canofari, C., Cedola, F., Onali, S., et al. (2019). Use of synthetic and biological DMARDs in patients with enteropathic spondyloarthritis: a combined gastro-rheumatological approach. Clin. Exp. Rheumatol. 37 (5), 723–730.
Ciccia, F., Guggino, G., Rizzo, A., Alessandro, R., Luchetti, M. M., Milling, S., et al. (2017). Dysbiosis and zonulin upregulation alter gut epithelial and vascular barriers in patients with ankylosing spondylitis. Ann. Rheumatol. Dis. 76 (6), 1123–1132. doi: 10.1136/annrheumdis-2016-210000
Codoner, F. M., Ramirez-Bosca, A., Climent, E., Carrion-Gutierrez, M., Guerrero, M., Perez-Orquin, J. M., et al. (2018). Gut microbial composition in patients with psoriasis. Sci. Rep. 8 (1), 3812. doi: 10.1038/s41598-018-22125-y
Costello, M. E., Ciccia, F., Willner, D., Warrington, N., Robinson, P. C., Gardiner, B., et al. (2015). Brief report: Intestinal dysbiosis in ankylosing spondylitis. Arthritis Rheumatol. 67 (3), 686–691. doi: 10.1002/art.38967
Cristofori, F., Dargenio, V. N., Dargenio, C., Miniello, V. L., Barone, M., Francavilla, R. (2021). Anti-inflammatory and immunomodulatory effects of probiotics in gut inflammation: A door to the body. Front. Immunol. 12. doi: 10.3389/fimmu.2021.578386
Cunningham, M., Azcarate-Peril, M. A., Barnard, A., Benoit, V., Grimaldi, R., Guyonnet, D., et al. (2021). Shaping the future of probiotics and prebiotics. Trends Microbiol. 29 (8), 667–685. doi: 10.1016/j.tim.2021.01.003
Danve, A., Deodhar, A. (2022). Treatment of axial spondyloarthritis: an update. Nat. Rev. Rheumatol. 18 (4), 205–216. doi: 10.1038/s41584-022-00761-z
Ditto, M. C., Parisi, S., Landolfi, G., Borrelli, R., Realmuto, C., Finucci, A., et al. (2021). Intestinal microbiota changes induced by TNF-inhibitors in IBD-related spondyloarthritis. RMD Open 7 (3), e001755. doi: 10.1136/rmdopen-2021-001755
Dougados, M., Baeten, D. (2011). Spondyloarthritis. Lancet 377 (9783), 2127–2137. doi: 10.1016/S0140-6736(11)60071-8
Duba, A. S., Mathew, S. D. (2018). The seronegative spondyloarthropathies. Prim. Care 45 (2), 271–287. doi: 10.1016/j.pop.2018.02.005
Dubreuil, M., Deodhar, A. A. (2017). Axial spondyloarthritis classification criteria: the debate continues. Curr. Opin. Rheumatol. 29 (4), 317–322. doi: 10.1097/BOR.0000000000000402
Dupont, H. L., Jiang, Z. D., Dupont, A. W., Utay, N. S. (2020). The intestinal microbiome in human health and disease. Trans. Am. Clin. Climatol. Assoc. 131, 178–197.
Fragoulis, G. E., Liava, C., Daoussis, D., Akriviadis, E., Garyfallos, A., Dimitroulas, T. (2019). Inflammatory bowel diseases and spondyloarthropathies: From pathogenesis to treatment. World J. Gastroenterol. 25 (18), 2162–2176. doi: 10.3748/wjg.v25.i18.2162
Ganapati, A., Gowri, M., Antonisamy, B., Danda, D. (2021). Combination of methotrexate and sulfasalazine is an efficacious option for axial spondyloarthritis in a resource-limited, real-world clinical setting: a prospective cohort study. Clin. Rheumatol. 40 (5), 1871–1879. doi: 10.1007/s10067-020-05433-5
Gill, T., Asquith, M., Rosenbaum, J. T., Colbert, R. A. (2015). The intestinal microbiome in spondyloarthritis. Curr. Opin. Rheumatol. 27 (4), 319–325. doi: 10.1097/bor.0000000000000187
Gill, T., Brooks, S. R., Rosenbaum, J. T., Asquith, M., Colbert, R. A. (2019). Novel inter-omic analysis reveals relationships between diverse gut microbiota and host immune dysregulation in HLA-B27-Induced experimental spondyloarthritis. Arthritis Rheumatol. 71 (11), 1849–1857. doi: 10.1002/art.41018
Gladman, D. D., Orbai, A. M., Klitz, U., Wei, J. C., Gallo, G., Birt, J., et al. (2019). Ixekizumab and complete resolution of enthesitis and dactylitis: integrated analysis of two phase 3 randomized trials in psoriatic arthritis. Arthritis Res. Ther. 21 (1), 38. doi: 10.1186/s13075-019-1831-0
Gladman, D., Rigby, W., Azevedo, V. F., Behrens, F., Blanco, R., Kaszuba, A., et al. (2017). Tofacitinib for psoriatic arthritis in patients with an inadequate response to TNF inhibitors. N. Engl. J. Med. 377 (16), 1525–1536. doi: 10.1056/NEJMoa1615977
Gracey, E., Qaiyum, Z., Almaghlouth, I., Lawson, D., Karki, S., Avvaru, N., et al. (2016). IL-7 primes IL-17 in mucosal-associated invariant T (MAIT) cells, which contribute to the Th17-axis in ankylosing spondylitis. Ann. Rheumatol. Dis. 75 (12), 2124–2132. doi: 10.1136/annrheumdis-2015-208902
Gracey, E., Vereecke, L., McGovern, D., Frohling, M., Schett, G., Danese, S., et al. (2020). Revisiting the gut-joint axis: links between gut inflammation and spondyloarthritis. Nat. Rev. Rheumatol. 16 (8), 415–433. doi: 10.1038/s41584-020-0454-9
Groeger, D., O’Mahony, L., Murphy, E. F., Bourke, J. F., Dinan, T. G., Kiely, B., et al. (2013). Bifidobacterium infantis 35624 modulates host inflammatory processes beyond the gut. Gut Microbes 4 (4), 325–339. doi: 10.4161/gmic.25487
Hall, A. B., Yassour, M., Sauk, J., Garner, A., Jiang, X., Arthur, T., et al. (2017). A novel ruminococcus gnavus clade enriched in inflammatory bowel disease patients. Genome Med. 9 (1), 103. doi: 10.1186/s13073-017-0490-5
Hegazy, S. K., El-Bedewy, M. M. (2010). Effect of probiotics on pro-inflammatory cytokines and NF-kappaB activation in ulcerative colitis. World J. Gastroenterol. 16 (33), 4145–4151. doi: 10.3748/wjg.v16.i33.4145
Hill, C., Guarner, F., Reid, G., Gibson, G. R., Merenstein, D. J., Pot, B., et al. (2014). The international scientific association for probiotics and prebiotics consensus statement on the scope and appropriate use of the term probiotic. Nat. Rev. Gastroenterol. Hepatol. 11 (8), 506–514. doi: 10.1038/nrgastro.2014.66
Honda, K., Littman, D. R. (2012). The microbiome in infectious disease and inflammation. Annu. Rev. Immunol. 30, 759–795. doi: 10.1146/annurev-immunol-020711-074937
Ishikawa, H., Matsumoto, S., Ohashi, Y., Imaoka, A., Setoyama, H., Umesaki, Y., et al. (2011). Beneficial effects of probiotic bifidobacterium and galacto-oligosaccharide in patients with ulcerative colitis: a randomized controlled study. Digestion 84 (2), 128–133. doi: 10.1159/000322977
Jacobs, M. E., Pouw, J. N., Welsing, P., Radstake, T., Leijten, E. F. A. (2021). First-line csDMARD monotherapy drug retention in psoriatic arthritis: methotrexate outperforms sulfasalazine. Rheumatol. (Oxford) 60 (2), 780–784. doi: 10.1093/rheumatology/keaa399
Karreman, M. C., Luime, J. J., Hazes, J. M. W., Weel, A. (2017). The prevalence and incidence of axial and peripheral spondyloarthritis in inflammatory bowel disease: A systematic review and meta-analysis. J. Crohns Colitis 11 (5), 631–642. doi: 10.1093/ecco-jcc/jjw199
Klingberg, E., Magnusson, M. K., Strid, H., Deminger, A., Stahl, A., Sundin, J., et al. (2019). A distinct gut microbiota composition in patients with ankylosing spondylitis is associated with increased levels of fecal calprotectin. Arthritis Res. Ther. 21 (1), 248. doi: 10.1186/s13075-019-2018-4
Kruis, W., Fric, P., Pokrotnieks, J., Lukas, M., Fixa, B., Kascak, M., et al. (2004). Maintaining remission of ulcerative colitis with the probiotic escherichia coli nissle 1917 is as effective as with standard mesalazine. Gut 53 (11), 1617–1623. doi: 10.1136/gut.2003.037747
Li, M., Dai, B., Tang, Y., Lei, L., Li, N., Liu, C., et al. (2019). Altered bacterial-fungal interkingdom networks in the guts of ankylosing spondylitis patients. mSystems 4 (2), e00176-18. doi: 10.1128/mSystems.00176-18
Li, Y., Du, J., Wei, W. (2022). Emerging roles of mucosal-associated invariant T cells in rheumatology. Front. Immunol. 13. doi: 10.3389/fimmu.2022.819992
Liu, Y. Y., Tran, D. Q., Rhoads, J. M. (2018). Probiotics in disease prevention and treatment. J. Clin. Pharmacol. 58 (10), S164–S179. doi: 10.1002/jcph.1121
Lowe, J. R., Briggs, A. M., Whittle, S., Stephenson, M. D. (2020). A systematic review of the effects of probiotic administration in inflammatory arthritis. Complement. Ther. Clin. Pract. 40, 101207. doi: 10.1016/j.ctcp.2020.101207
Lukasik, Z., Gracey, E., Venken, K., Ritchlin, C., Elewaut, D. (2021). Crossing the boundaries: IL-23 and its role in linking inflammation of the skin, gut and joints. Rheumatol. (Oxford) 60 (Suppl 4), iv16–iv27. doi: 10.1093/rheumatology/keab385
Lynch, S. V., Pedersen, O. (2016). The human intestinal microbiome in health and disease. N. Engl. J. Med. 375 (24), 2369–2379. doi: 10.1056/NEJMra1600266
Magrey, M. N., Danve, A. S., Ermann, J., Walsh, J. A. (2020). Recognizing axial spondyloarthritis: A guide for primary care. Mayo Clin. Proc. 95 (11), 2499–2508. doi: 10.1016/j.mayocp.2020.02.007
Matsuoka, K., Uemura, Y., Kanai, T., Kunisaki, R., Suzuki, Y., Yokoyama, K., et al. (2018). Efficacy of bifidobacterium breve fermented milk in maintaining remission of ulcerative colitis. Dig. Dis. Sci. 63 (7), 1910–1919. doi: 10.1007/s10620-018-4946-2
Mauro, D., Macaluso, F., Fasano, S., Alessandro, R., Ciccia, F. (2019). ILC3 in axial spondyloarthritis: the gut angle. Curr. Rheumatol. Rep. 21 (7), 37. doi: 10.1007/s11926-019-0834-9
Mease, P. J. (2012). Spondyloarthritis: Is methotrexate effective in psoriatic arthritis? Nat. Rev. Rheumatol. 8 (5), 251–252. doi: 10.1038/nrrheum.2012.56
Miller, A. L., Bessho, S., Grando, K., Tükel, Ç. (2021). Microbiome or infections: Amyloid-containing biofilms as a trigger for complex human diseases. Front. Immunol. 12. doi: 10.3389/fimmu.2021.638867
Moz, S., Aita, A., Basso, D., Ramonda, R., Plebani, M., Punzi, L. (2017). Spondyloarthritis: Matrix metalloproteinasesas biomarkers of pathogenesis and response to tumor necrosis factor (TNF) inhibitors. Int. J. Mol. Sci. 18 (4), 830. doi: 10.3390/ijms18040830
Navarro-Compán, V., Sepriano, A., El-Zorkany, B., van der Heijde, D. (2021). Axial spondyloarthritis. Ann. Rheumatol. Dis. 80 (12), 1511–1521. doi: 10.1136/annrheumdis-2021-221035
Nishino, K., Nishida, A., Inoue, R., Kawada, Y., Ohno, M., Sakai, S., et al. (2018). Analysis of endoscopic brush samples identified mucosa-associated dysbiosis in inflammatory bowel disease. J. Gastroenterol. 53 (1), 95–106. doi: 10.1007/s00535-017-1384-4
Ni, J., Wu, G. D., Albenberg, L., Tomov, V. T. (2017). Gut microbiota and IBD: causation or correlation? Nat. Rev. Gastroenterol. Hepatol. 14 (10), 573–584. doi: 10.1038/nrgastro.2017.88
Noer, H. R. (1966). An “experimental” epidemic of reiter’s syndrome. Jama 198 (7), 693–698. doi: 10.1001/jama.1966.03110200049016
Olivieri, I., Cantini, F., Castiglione, F., Felice, C., Gionchetti, P., Orlando, A., et al. (2014). Italian Expert panel on the management of patients with coexisting spondyloarthritis and inflammatory bowel disease. Autoimmun. Rev. 13 (8), 822–830. doi: 10.1016/j.autrev.2014.04.003
Oliviero, F., Spinella, P. (2020). Benefits of probiotics in rheumatic diseases. Front. Nutr. 7. doi: 10.3389/fnut.2020.00157
Otani, K., Tanigawa, T., Watanabe, T., Shimada, S., Nadatani, Y., Nagami, Y., et al. (2017). Microbiota plays a key role in non-steroidal anti-inflammatory drug-induced small intestinal damage. Digestion 95 (1), 22–28. doi: 10.1159/000452356
Palumbo, V. D., Romeo, M., Marino Gammazza, A., Carini, F., Damiani, P., Damiano, G., et al. (2016). The long-term effects of probiotics in the therapy of ulcerative colitis: A clinical study. Biomed. Pap. Med. Fac. Univ. Palacky Olomouc. Czech. Repub. 160 (3), 372–377. doi: 10.5507/bp.2016.044
Pan, Z. Y., Chang, Y. X., Han, N., Hou, F. Y., Lee, B. J. Y., Zhi, F. C., et al. (2021). Short-term high-dose gavage of hydroxychloroquine changes gut microbiota but not the intestinal integrity and immunological responses in mice. Life. Sci. 264, 118450. doi: 10.1016/j.lfs.2020.118450
Plaza-Diaz, J., Ruiz-Ojeda, F. J., Gil-Campos, M., Gil, A. (2019). Mechanisms of action of probiotics. Adv. Nutr. 10 (suppl_1), S49–S66. doi: 10.1093/advances/nmy063
Protopopov, M., Poddubnyy, D. (2018). Radiographic progression in non-radiographic axial spondyloarthritis. Expert. Rev. Clin. Immunol. 14 (6), 525–533. doi: 10.1080/1744666x.2018.1477591
Qaiyum, Z., Gracey, E., Yao, Y. C., Inman, R. D. (2019). Integrin and transcriptomic profiles identify a distinctive synovial CD8+T cell subpopulation in spondyloarthritis. Ann. Rheumatol. Dis. 78 (11), 1566–1575. doi: 10.1136/annrheumdis-2019-215349
Qaiyum, Z., Lim, M., Inman, R. D. (2021). The gut-joint axis in spondyloarthritis: immunological, microbial, and clinical insights. Semin. Immunopathol. 43 (2), 173–192. doi: 10.1007/s00281-021-00845-0
Queiro-Silva, R., García-Valle, A., Alonso-Castro, S., Alperi-López, M. (2021). Do NSAIDs take us away from treatment goals in axial spondyloarthritis: A story about dysbiosis or just a matter of bias? Front. Med. (Lausanne) 8. doi: 10.3389/fmed.2021.817884
Ritchlin, C., Adamopoulos, I. E. (2021). Axial spondyloarthritis: new advances in diagnosis and management. BMJ 372, m4447. doi: 10.1136/bmj.m4447
Rizzo, A., Guggino, G., Ferrante, A., Ciccia, F. (2018). Role of subclinical gut inflammation in the pathogenesis of spondyloarthritis. Front. Med. (Lausanne) 5. doi: 10.3389/fmed.2018.00063
Robinson, P. C., van der Linden, S., Khan, M. A., Taylor, W. J. (2021). Axial spondyloarthritis: concept, construct, classification and implications for therapy. Nat. Rev. Rheumatol. 17 (2), 109–118. doi: 10.1038/s41584-020-00552-4
Rogers, M. A. M., Aronoff, D. M. (2016). The influence of non-steroidal anti-inflammatory drugs on the gut microbiome. Clin. Microbiol. Infect. 22 (2), e171–178 e179. doi: 10.1016/j.cmi.2015.10.003
Rudwaleit, M., Sieper, J. (2012). Referral strategies for early diagnosis of axial spondyloarthritis. Nat. Rev. Rheumatol. 8 (5), 262–268. doi: 10.1038/nrrheum.2012.39
Rudwaleit, M., van der Heijde, D., Landewé, R., Akkoc, N., Brandt, J., Chou, C. T., et al. (2011). The assessment of SpondyloArthritis international society classification criteria for peripheral spondyloarthritis and for spondyloarthritis in general. Ann. Rheumatol. Dis. 70 (1), 25–31. doi: 10.1136/ard.2010.133645
Saitta, K. S., Zhang, C., Lee, K. K., Fujimoto, K., Redinbo, M. R., Boelsterli, U. A. (2014). Bacterial beta-glucuronidase inhibition protects mice against enteropathy induced by indomethacin, ketoprofen or diclofenac: mode of action and pharmacokinetics. Xenobiotica 44 (1), 28–35. doi: 10.3109/00498254.2013.811314
Sanchez, P., Letarouilly, J. G., Nguyen, Y., Sigaux, J., Barnetche, T., Czernichow, S., et al. (2022). Efficacy of probiotics in rheumatoid arthritis and spondyloarthritis: A systematic review and meta-analysis of randomized controlled trials. Nutrients 14 (2), 354. doi: 10.3390/nu14020354
Sanges, M., Valente, G., Rea, M., Della Gatta, R., De Franchis, G., Sollazzo, R., et al. (2009). Probiotics in spondyloarthropathy associated with ulcerative colitis: a pilot study. Eur. Rev. Med. Pharmacol. Sci. 13 (3), 233–234.
Scher, J. U., Nayak, R. R., Ubeda, C., Turnbaugh, P. J., Abramson, S. B. (2020). Pharmacomicrobiomics in inflammatory arthritis: gut microbiome as modulator of therapeutic response. Nat. Rev. Rheumatol. 16 (5), 282–292. doi: 10.1038/s41584-020-0395-3
Scher, J. U., Ubeda, C., Artacho, A., Attur, M., Isaac, S., Reddy, S. M., et al. (2015). Decreased bacterial diversity characterizes the altered gut microbiota in patients with psoriatic arthritis, resembling dysbiosis in inflammatory bowel disease. Arthritis Rheumatol. 67 (1), 128–139. doi: 10.1002/art.38892
Schett, G., Baraliakos, X., Van den Bosch, F., Deodhar, A., Ostergaard, M., Das Gupta, A., et al. (2021). Secukinumab efficacy on enthesitis in patients with ankylosing spondylitis: Pooled analysis of four pivotal phase III studies. J. Rheumatol. 48 (8), 1251–1258. doi: 10.3899/jrheum.201111
Schinocca, C., Rizzo, C., Fasano, S., Grasso, G., La Barbera, L., Ciccia, F., et al. (2021). Role of the IL-23/IL-17 pathway in rheumatic diseases: An overview. Front. Immunol. 12. doi: 10.3389/fimmu.2021.637829
Sekirov, I., Russell, S. L., Antunes, L. C., Finlay, B. B. (2010). Gut microbiota in health and disease. Physiol. Rev. 90 (3), 859–904. doi: 10.1152/physrev.00045.2009
Shamoon, M., Martin, N. M., O’Brien, C. L. (2019). Recent advances in gut microbiota mediated therapeutic targets in inflammatory bowel diseases: Emerging modalities for future pharmacological implications. Pharmacol. Res. 148, 104344. doi: 10.1016/j.phrs.2019.104344
Sherlock, J. P., Joyce-Shaikh, B., Turner, S. P., Chao, C. C., Sathe, M., Grein, J., et al. (2012). IL-23 induces spondyloarthropathy by acting on ROR-gammat+ CD3+CD4-CD8- entheseal resident T cells. Nat. Med. 18 (7), 1069–1076. doi: 10.1038/nm.2817
Sieper, J., Poddubnyy, D. (2017). Axial spondyloarthritis. Lancet 390 (10089), 73–84. doi: 10.1016/S0140-6736(16)31591-4
Sieper, J., Rudwaleit, M., Baraliakos, X., Brandt, J., Braun, J., Burgos-Vargas, R., et al. (2009). The assessment of SpondyloArthritis international society (ASAS) handbook: a guide to assess spondyloarthritis. Ann. Rheumatol. Dis. 68 Suppl 2, ii1–i44. doi: 10.1136/ard.2008.104018
Smith, J. A., Colbert, R. A. (2014). Review: The interleukin-23/interleukin-17 axis in spondyloarthritis pathogenesis: Th17 and beyond. Arthritis Rheumatol. 66 (2), 231–241. doi: 10.1002/art.38291
Smolen, J. S., Landewé, R. B., Bijlsma, J. W., Burmester, G. R., Dougados, M., Kerschbaumer, A., et al. (2020). EULAR recommendations for the management of rheumatoid arthritis with synthetic and biological disease-modifying antirheumatic drugs: 2019 update. Ann. Rheumatol. Dis. 79 (6), 685–699. doi: 10.1136/annrheumdis-2019-216655
Sood, A., Midha, V., Makharia, G. K., Ahuja, V., Singal, D., Goswami, P., et al. (2009). The probiotic preparation, VSL3 induces remission in patients with mild-to-moderately active ulcerative colitis. Clin. Gastroenterol. Hepatol. 7 (11), 1209.e1201. doi: 10.1016/j.cgh.2009.07.016
Stolwijk, C., Boonen, A., van Tubergen, A., Reveille, J. D. (2012). Epidemiology of spondyloarthritis. Rheumatol. Dis. Clin. North Am. 38 (3), 441–476. doi: 10.1016/j.rdc.2012.09.003
Taams, L. S., Steel, K. J. A., Srenathan, U., Burns, L. A., Kirkham, B. W. (2018). IL-17 in the immunopathogenesis of spondyloarthritis. Nat. Rev. Rheumatol. 14 (8), 453–466. doi: 10.1038/s41584-018-0044-2
Tamaki, H., Nakase, H., Inoue, S., Kawanami, C., Itani, T., Ohana, M., et al. (2016). Efficacy of probiotic treatment with bifidobacterium longum 536 for induction of remission in active ulcerative colitis: A randomized, double-blinded, placebo-controlled multicenter trial. Dig. Endosc. 28 (1), 67–74. doi: 10.1111/den.12553
Taurog, J. D., Richardson, J. A., Croft, J. T., Simmons, W. A., Zhou, M., Fernández-Sueiro, J. L., et al. (1994). The germfree state prevents development of gut and joint inflammatory disease in HLA-B27 transgenic rats. J. Exp. Med. 180 (6), 2359–2364. doi: 10.1084/jem.180.6.2359
Terenzi, R., Monti, S., Tesei, G., Carli, L. (2018). One year in review 2017: spondyloarthritis. Clin. Exp. Rheumatol. 36 (1), 1–14.
Tiffany, C. R., Bäumler, A. J. (2019). Dysbiosis: from fiction to function. Am. J. Physiol. Gastrointest. Liver Physiol. 317 (5), G602–G608. doi: 10.1152/ajpgi.00230.2019
Tito, R. Y., Cypers, H., Joossens, M., Varkas, G., Van Praet, L., Glorieus, E., et al. (2017). Dialister as a microbial marker of disease activity in spondyloarthritis. Arthritis Rheumatol. 69 (1), 114–121. doi: 10.1002/art.39802
Toussirot, E. (2022). The use of janus kinase inhibitors in axial spondyloarthritis: Current insights. Pharm. (Basel) 15 (3), 270. doi: 10.3390/ph15030270
Toussirot, E., Saas, P. (2018). MAIT cells: potent major cellular players in the IL-17 pathway of spondyloarthritis? RMD Open 4 (2), e000821. doi: 10.1136/rmdopen-2018-000821
Tsukazaki, H., Kaito, T. (2020). The role of the IL-23/IL-17 pathway in the pathogenesis of spondyloarthritis. Int. J. Mol. Sci. 21 (17), 6401. doi: 10.3390/ijms21176401
Vaile, J. H., Meddings, J. B., Yacyshyn, B. R., Russell, A. S., Maksymowych, W. P. (1999). Bowel permeability and CD45RO expression on circulating CD20+ b cells in patients with ankylosing spondylitis and their relatives. J. Rheumatol. 26 (1), 128–135.
Vallejo-Cordoba, B., Castro-Lopez, C., Garcia, H. S., Gonzalez-Cordova, A. F., Hernandez-Mendoza, A. (2020). Postbiotics and paraprobiotics: A review of current evidence and emerging trends. Adv. Food. Nutr. Res. 94, 1–34. doi: 10.1016/bs.afnr.2020.06.001
van der Heijde, D., Mease, P. J., Landewe, R. B. M., Rahman, P., Tahir, H., Singhal, A., et al. (2020). Secukinumab provides sustained low rates of radiographic progression in psoriatic arthritis: 52-week results from a phase 3 study, FUTURE 5. Rheumatol. (Oxford) 59 (6), 1325–1334. doi: 10.1093/rheumatology/kez420
van der Heijde, D., Ramiro, S., Landewé, R., Baraliakos, X., Van den Bosch, F., Sepriano, A., et al. (2017). 2016 update of the ASAS-EULAR management recommendations for axial spondyloarthritis. Ann. Rheumatol. Dis. 76 (6), 978–991. doi: 10.1136/annrheumdis-2016-210770
Veale, D. J., McGonagle, D., McInnes, I. B., Krueger, J. G., Ritchlin, C. T., Elewaut, D., et al. (2019). The rationale for janus kinase inhibitors for the treatment of spondyloarthritis. Rheumatol. (Oxford) 58 (2), 197–205. doi: 10.1093/rheumatology/key070
Ward, M. M., Deodhar, A., Gensler, L. S., Dubreuil, M., Yu, D., Khan, M. A., et al. (2019). 2019 update of the American college of Rheumatology/Spondylitis association of America/Spondyloarthritis research and treatment network recommendations for the treatment of ankylosing spondylitis and nonradiographic axial spondyloarthritis. Arthritis Care Res. (Hoboken) 71 (10), 1285–1299. doi: 10.1002/acr.24025
Wen, C., Zheng, Z., Shao, T., Liu, L., Xie, Z., Le Chatelier, E., et al. (2017). Quantitative metagenomics reveals unique gut microbiome biomarkers in ankylosing spondylitis. Genome Biol. 18 (1), 142. doi: 10.1186/s13059-017-1271-6
Wildt, S., Nordgaard, I., Hansen, U., Brockmann, E., Rumessen, J. J. (2011). A randomised double-blind placebo-controlled trial with lactobacillus acidophilus la-5 and bifidobacterium animalis subsp. lactis BB-12 for maintenance of remission in ulcerative colitis. J. Crohns Colitis 5 (2), 115–121. doi: 10.1016/j.crohns.2010.11.004
Wilson, G., Folzenlogen, D. D. (2012). Spondyloarthropathies: new directions in etiopathogenesis, diagnosis and treatment. Mo. Med. 109 (1), 69–74.
Yin, J., Sternes, P. R., Wang, M. B., Song, J., Morrison, M., Li, T., et al. (2020). Shotgun metagenomics reveals an enrichment of potentially cross-reactive bacterial epitopes in ankylosing spondylitis patients, as well as the effects of TNFi therapy upon microbiome composition. Ann. Rheumatol. Dis. 79 (1), 132–140. doi: 10.1136/annrheumdis-2019-215763
Zaiss, M. M., Joyce Wu, H. J., Mauro, D., Schett, G., Ciccia, F. (2021). The gut-joint axis in rheumatoid arthritis. Nat. Rev. Rheumatol. 17 (4), 224–237. doi: 10.1038/s41584-021-00585-3
Zhang, F., Ma, C., Zhang, B. (2020). Dynamic variations in gut microbiota in ankylosing spondylitis patients treated with anti-TNF-α for six months. Ann. Clin. Lab. Sci. 50 (1), 99–106.
Keywords: spondyloarthritis, inflammation, gut-joint axis, probiotics, gut dysbiosis
Citation: Lyu X, Chen J, Gao X and Yang J (2022) Emerging story of gut dysbiosis in spondyloarthropathy: From gastrointestinal inflammation to spondyloarthritis. Front. Cell. Infect. Microbiol. 12:973563. doi: 10.3389/fcimb.2022.973563
Received: 21 June 2022; Accepted: 08 August 2022;
Published: 22 August 2022.
Edited by:
Huang He, Tianjin University, ChinaReviewed by:
Patricia Ruiz Limón, Universidad de Málaga, SpainLiping Duan, Peking University Third Hospital, China
Copyright © 2022 Lyu, Chen, Gao and Yang. This is an open-access article distributed under the terms of the Creative Commons Attribution License (CC BY). The use, distribution or reproduction in other forums is permitted, provided the original author(s) and the copyright owner(s) are credited and that the original publication in this journal is cited, in accordance with accepted academic practice. No use, distribution or reproduction is permitted which does not comply with these terms.
*Correspondence: Jie Yang, yangj@tmu.edu.cn