- 1Department of Zoology, College of Science, King Saud University, Riyadh, Saudi Arabia
- 2Department of Biology, College of Science, Princess Nourah Bint Abdulrahman University, Riyadh, Saudi Arabia
- 3Department of Science and Technology, Al-Nairiyah University College, University of Hafr Al-Batin, Hafr Al-Batin, Saudi Arabia
- 4Department of Zoology and Entomology, Faculty of Science, Helwan University, Cairo, Egypt
Herbal extracts are promising agents against various parasitic diseases, such as malaria. This study aimed to evaluate the ameliorative action of Eucalyptus camaldulensis extract (ECE) against hepatic damage caused by Plasmodium chabaudi infection. Mice were allocated into five groups as follows: two groups served as the control non-infected groups that received distilled water and ECE, respectively; subsequent three groups were infected with 106 P. chabaudi parasitized erythrocytes; the last two groups were infected with the parasite and then treated with ECE and chloroquine. On day 8 post-infection, the parasite count increased inside erythrocytes (59.4% parasitemia in the infected group). Parasitemia was successfully reduced to 9.4% upon ECE treatment. Phytochemical screening using GC mass spectrometry revealed that ECE contained 23 phytochemical components. Total phenolics and flavonoids in ECE were 104 ± 2 and 7.1± 3 µg/mL, respectively, with 57.2% antioxidant activity. ECE ameliorated changes in liver histopathology and enzymatic activity of alanine aminotransferase, aspartate aminotransferase, and alkaline phosphatase. In addition, ECE prevented oxidative damage induced by the parasite in the liver, as evidenced by the change in the liver concentrations of glutathione, nitric oxide, malondialdehyde, and catalase. Moreover, ECE was able to regulate the expression of liver cytokines, interleukins-1β and 6, as well as IFN-γ mRNA. ECE possesses antiplasmodial, antioxidant, and anti-inflammatory activity against liver injury induced by the parasite P. chabaudi.
Introduction
Malaria is the most common parasitic disease in humans, and is transmitted through the bite of an infected female Anopheles mosquito. It remains a major cause of illness and death worldwide where 229 million cases and 409,000 deaths were estimated in 2019 (WHO, 2020).
Malaria pathogenesis research is primarily conducted using rodent malaria parasites such as Plasmodium chabaudi, owing to its genome sequence and pathological similarities to the human parasite (Carlton et al., 2002).
Consequently, new, affordable, and effective treatment strategies are needed to reduce the incidence of malaria in endemic countries. Natural products, such as medicinal plants, have long been used as promising and reliable sources for treating parasitic infections, including malaria. The structural diversity of natural products and their ability to interact with therapeutic targets justify their use in the search for new drugs. More than 40% of the authorized drugs on the market are of natural or semi-synthetic origin (Newman and Cragg, 2016). Malaria has been treated with medicinal plants since ancient times. Hence, plant sources could be useful in discovering potential candidates for new antimalarial drugs (Taherkhani et al., 2013).
Eucalyptus camaldulensis belongs to the family Myrtaceae and is considered a source of biologically active compounds (Ghalem and Mohamed, 2014). The leaf extract of E. camaldulensis (ECE) is known to possess antioxidant (Singab et al., 2011), antimicrobial (Ghalem and Mohamed, 2008), larvicidal (Medhi et al., 2010), pesticidal (Batish et al., 2008), and antiparasitic properties (Hassani et al., 2013). Hence, this study aimed to investigate the antiplasmodial and hepatoprotective properties of ECE against blood-stage malaria caused by P. chabaudi in mice by evaluating their oxidative and inflammatory status.
Materials and methods
Preparation of Eucalyptus camaldulensis extracts
Leaves were collected from plants grown in Qassim, Saudi Arabia (26°04’57.0”N, 43°39’42.2”E). The plant was identified by a specialist at the herbarium of King Saud University. The leaves were air-dried and then ground into a powder. The constituents of the powdered leaves were extracted with 70% methanol (Lubbad et al., 2015). ECE was diluted with distilled water and used for subsequent experiments.
Determination of total phenolics in ECE
The total phenolic content of ECE was determined using a modified Folin-Ciocalteu method in which 12.5 µL of ECE (1 mg/mL) was mixed with 125 µL of Folin–Ciocalteu reagent (25%) then incubated for 5 min. After that, Na2CO3 was added and the mixture was kept in dark for 1.5 h. At 760 nm, samples were measured using a microplate reader (Thermo Fisher Scientific, Waltham, MA, USA). A gallic acid standard curve was used to calculate the total phenolic content. (Al-Zharani et al., 2019).
Determination of total flavonoids in ECE
The total flavonoid content was determined using a colorimetric assay with aluminium chloride. This is by mixing 100 µL of ECE (1 mg/mL) with 100 µL of aluminum chloride (2%). In brief, 100 µL of ECE (1 mg/mL) and 100 µL of aluminum chloride (2%). The absorbance was measured at 368 nm. The flavonoids in the samples were estimated using a calibration curve of quercetin, a standard flavonoid (Ghosh et al., 2013).
Gas chromatography-mass spectrometry analysis for ECE
The phytochemical analysis of ECE was performed according to the protocol recommended by Kanthal et al. (2014). Gas chromatography-mass spectrometry (GC-MS) was performed using a 7000D GC/MS Triple Quad GC-MS unit (Agilent Technologies, USA).
DPPH radical scavenging method for antioxidant activity
The free radical scavenging activity of extracts was determined using 2,2-diphenyl-1-picrylhydrazyl (DPPH). In brief, 20 µL of ECE (1 mg/mL) was mixed with 80 µL of a methanolic solution of DPPH (100 mM) and then incubated in the dark for 30 min at 25°C. Absorption was read at 517 nm and radical scavenging activity was calculated (Ghosh et al., 2013).
Animals and infection
Female C57BL/6 mice (9–11 weeks old) were bred and provided standard diet and water ad libitum. The experiments were approved by the Research Ethics Committee for Laboratory Animal Care at King Saud University (approval no.: KSU-Se-21-77).
Cryopreserved P. chabaudi parasites were passaged in donor C57BL/6 mice and intraperitoneally injected into experimental mice as previously described (Wunderlich et al., 1982). A Neubauer chamber was used to calculate the injected dose (106 parasitized erythrocytes). The P. chabaudi-parasitized erythrocytes were injected intraperitoneally into mice in 100 µL phosphate buffer (Wunderlich et al., 1982). To determine P. chabaudi induced parasitemia, Giemsa-stained smears from tail blood of mice were prepared.
Experimental design
To determine the suitable dose and peak parasitemia level, the mice were divided into six groups (n = 10). The first group consisted of uninfected control mice that were given only water by gavage. The second to the seventh groups were infected intraperitoneally with 1 × 106 P. chabaudi–infected erythrocytes. After 1 h, the third, fourth, and fifth groups of mice were administered ECE at doses of 100, 200, and 400 mg/kg daily for 8 days. The last group was administered 10 mg/kg chloroquine phosphate (CQ) (Sigma-Aldrich, St. Louis, USA) daily for 4 days (Abay et al., 2015). Based on parasitemia results (Figure S1), we studied the effect of ECE by repeating the experiment using five groups of mice (n = 5/group): group 1, non-infected control group; group 2, non-infected treated group (treated with 100 mg/kg ECE); group 3, infected group; group 4, infected group + 100 mg/kg ECE; group 5, infected group + 10 mg/kg chloroquine. The dose of ECE was selected based on the study by Anigboro et al. (2020) and results of a preliminary experiment (Figure S1). All animals were CO2 asphyxiated and dissected on day 8 post infection for sample collection.
Sample collection
Heparinized tubes were used to collect the blood from the heart. The livers of mice were isolated and cut into small pieces. Parts were preserved in neutral buffered formalin for histopathological examination while others were stored at -80°C to study the antioxidant activity of E. camaldulensis, and to extract RNA for the subsequent study of gene expression.
Biochemical studies
Blood plasma was separated and kept at -20°C until use. Plasma was then analyzed according to the manufacturer’s instructions using commercial kits (Biomerieux, Marcy l’Etoil, France) for alanine aminotransferase (ALT), aspartate aminotransferase (AST), and alkaline phosphatase (ALP).
Histopathological studies
Freshly prepared liver samples were fixed in 10% neutral buffered formalin and then embedded in paraffin. Hematoxylin and eosin were used for staining after the samples were cut into 5 µm thick sections (Drury and Wallington, 1980). The liver histological scoring was carried out in accordance with the guidelines outlined by Ishak et al. (1995). In summary, this quantification measure for determining the histopathological changes in the liver is based on the examination of liver sections at various magnifications, yielding scores of 1-3, 4-8, 9-12, and 13-18 for minimal, mild, moderate, and severe liver injury, respectively.
Liver oxidative stress
Malondialdehyde (MDA) was measured in liver homogenates using thiobarbituric acid (Ohkawa et al., 1979). Nitric oxide (NO), glutathione (GSH), and catalase levels were measured as described by Ellman (1959); Green et al. (1982), and Aebi (1984).
Gene expression
To carry out real-time quantitative polymerase chain reaction (RT-qPCR), liver RNA was isolated using TRIzol (QIAGEN, Hilden, Germany). DNase was used to prepare RNA (Applied Biosystems, Darmstadt, Germany), and a reverse transcription kit was used to convert the samples into cDNA (QIAGEN, Hilden, Germany). The ABI Prism 7500HT sequence detection system (Applied Biosystems, Darmstadt, Germany) was used for PCR along with SYBR green PCR master mix (QIAGEN, Hilden, Germany). Levels of interleukin-1β (IL-1β), interleukin-6 (IL-6), and interferon gamma (INF-γ) were investigated using primers from Macrogen Inc. (Seoul, South Korea) (Table 1). PCR was performed as described by Dkhil et al. (2019). The fold change in mRNA expression was determined using the 2−ΔΔCT method (Livak and Schmittgen, 2001).
Statistical analysis
Significance was evaluated using one-way analysis of variance, and statistical comparisons between the groups were performed using Duncan’s test using a statistical package program (SPSS version 17.0). All values are expressed as the mean and standard error of the mean. All p-values were two-tailed, and p ≤ 0.05 was considered significant for all statistical analyses.
Results
The total phenolic and flavonoid contents in ECE reached 104 ± 2 and 7.1± 3 µg/mL, respectively while the antioxidant activity of ECE reached 57.2% (Table 2).
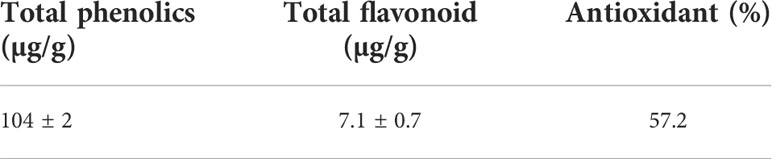
Table 2 Total phenolic, flavonoid contents and radical scavenging activity of E. camaldulensis extract.
Phytochemical screening using GC mass spectrometry revealed that ECE contained 23 phytochemical components (Table 3, Figure 1). The compounds benzene, 1,2,4,5-tetramethyl-, naphthalene, 2,4-Di-tert-butylphenol, hexadecanoic acid, methyl ester (palmitic acid), methyl stearate, hexadecanoic acid, and 2-hydroxy-1- (hydroxymethyl)ethyl ester appeared in Figure 1 with elevated peaks.
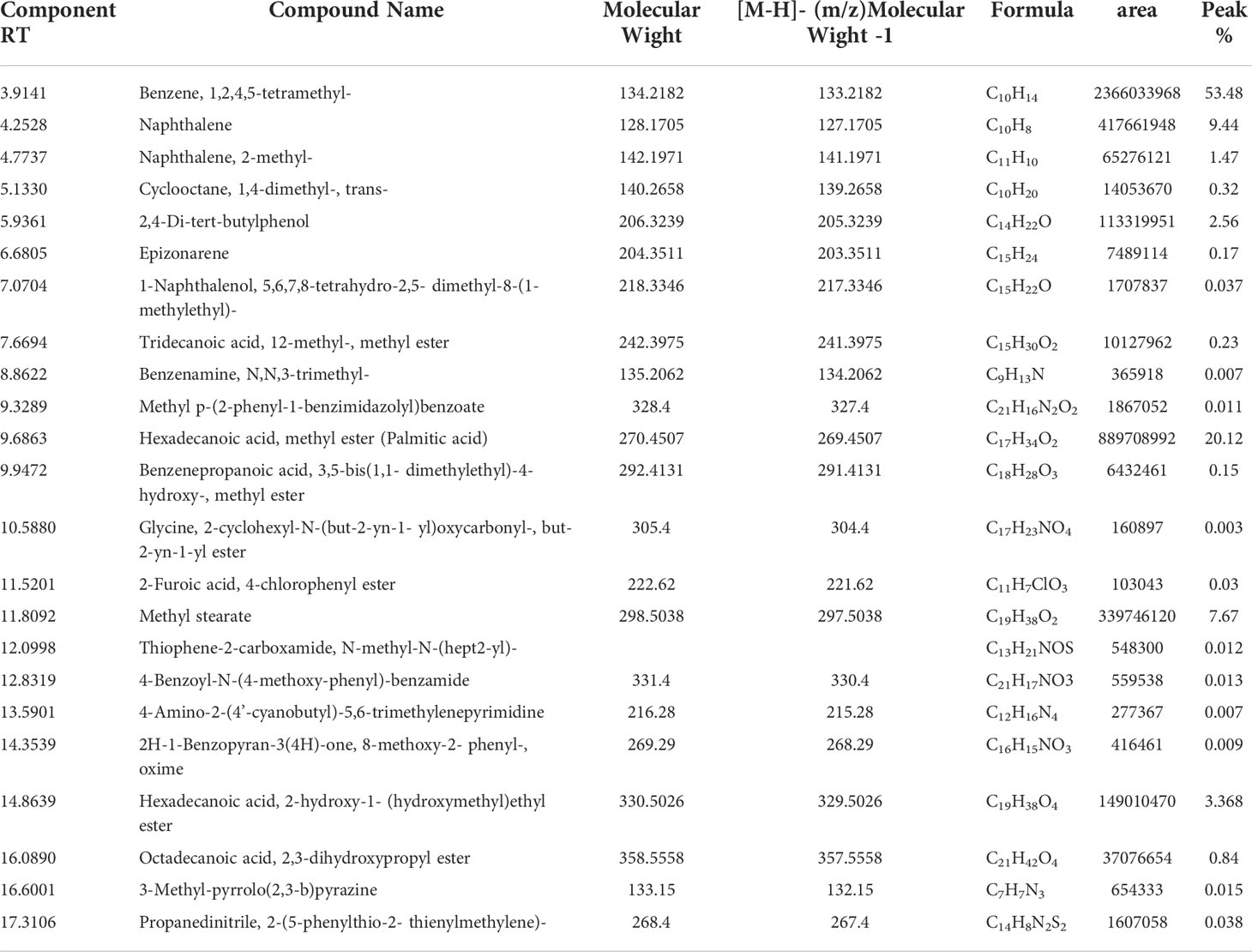
Table 3 Identification of phytochemical compounds by GC-Mass in Eucalyptus camaldulensis leaf extracts.
Figure 2 shows the parasitemia in the infected (59.4 ± 8.5%) and ECE-treated mice (9.4 ± 0.6%) compared to mice administered the reference drug chloroquine (13.6 ± 2%).
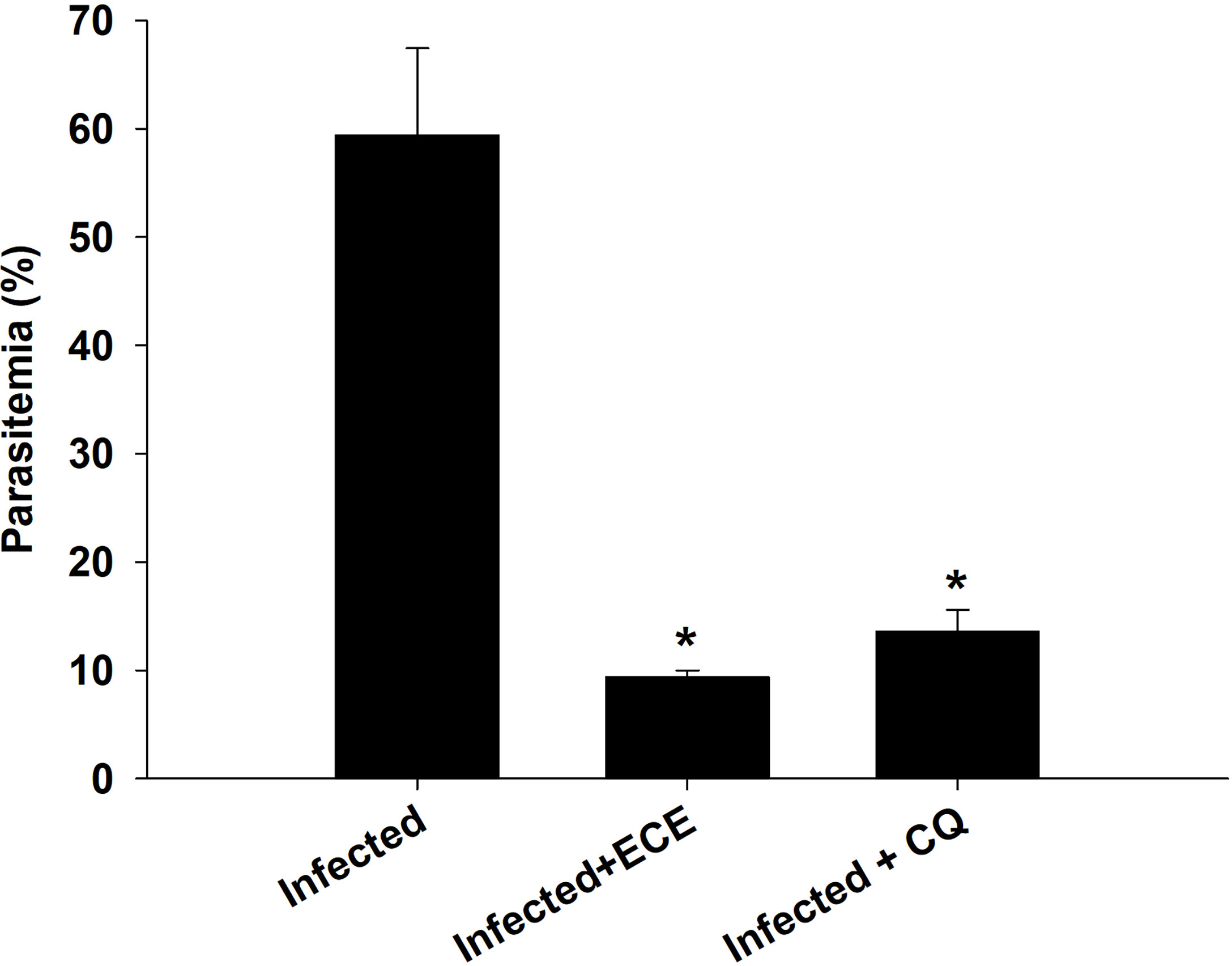
Figure 2 Percentage of parasitemia in mice infected with P. chabaudi and treated with Eucalyptus camaldulensis extract (ECE) and Chloroquine (CQ). Data are presented as mean ± SD at p ≤ 0.001. *, Significance against infected group.
Infection induced a significant increase in the liver enzymes ALT, AST, and ALT (Table 4). ECE ameliorated the changes in liver enzymes, similar to that observed after treatment with the reference drug.
Mice infected with P. chabaudi demonstrated extensive liver damage with inflammatory cells, whereas healthy controls and ECE-administered groups had normal liver architecture. Hepatic lesions were relieved, and the liver score was lowered in ECE-treated mice, which was linked to a reduction in inflammatory cell infiltration (Figure 3). Ishak’s score indicated that the infected group’s liver activity index ranged from 13 to 15. When mice were given ECE, the liver index dropped to 5-8 and when treated with chloroquine, the score reached 6-8 (Table 5).
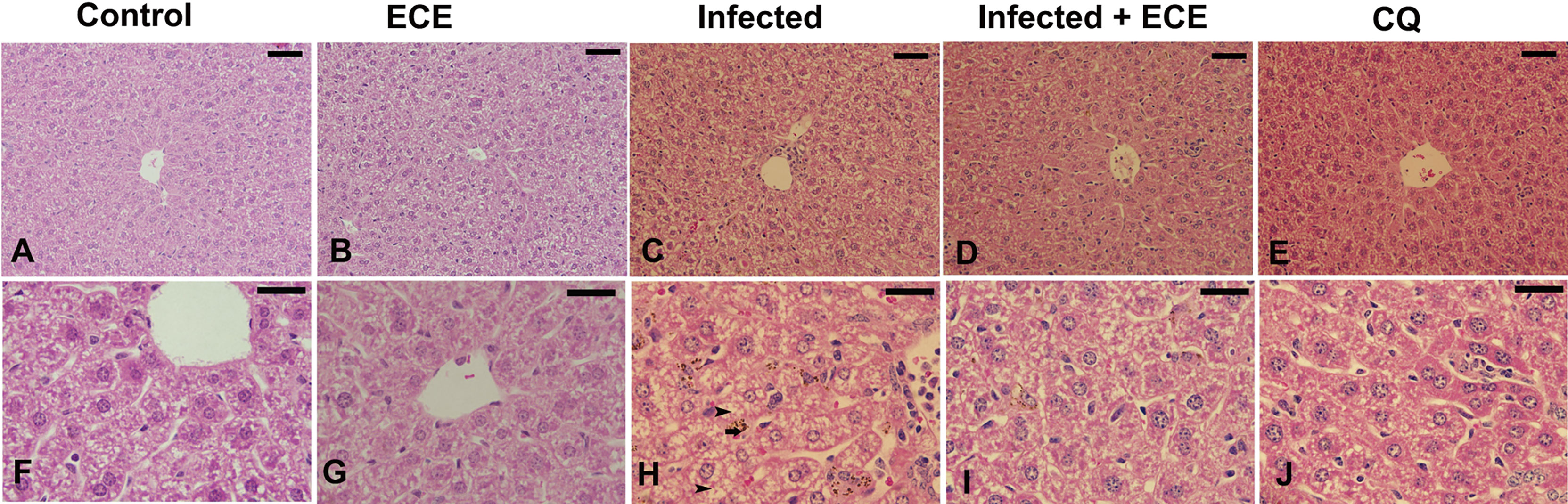
Figure 3 Effect of Eucalyptus camaldulensis extract (ECE) on the liver histology of mice infected with P. chabaudi. (A, F) non-infected group. (B, G) ECE treated group. (C, H) Infected group with inflammation, malaria pigment (arrow) and hepatocytic vacuolation (arrow head). (D, I) Infected-ECE treated group with improved structure. (E, J) infected-chloroquine treated group with improved structure. Scale bar = 25 µm.
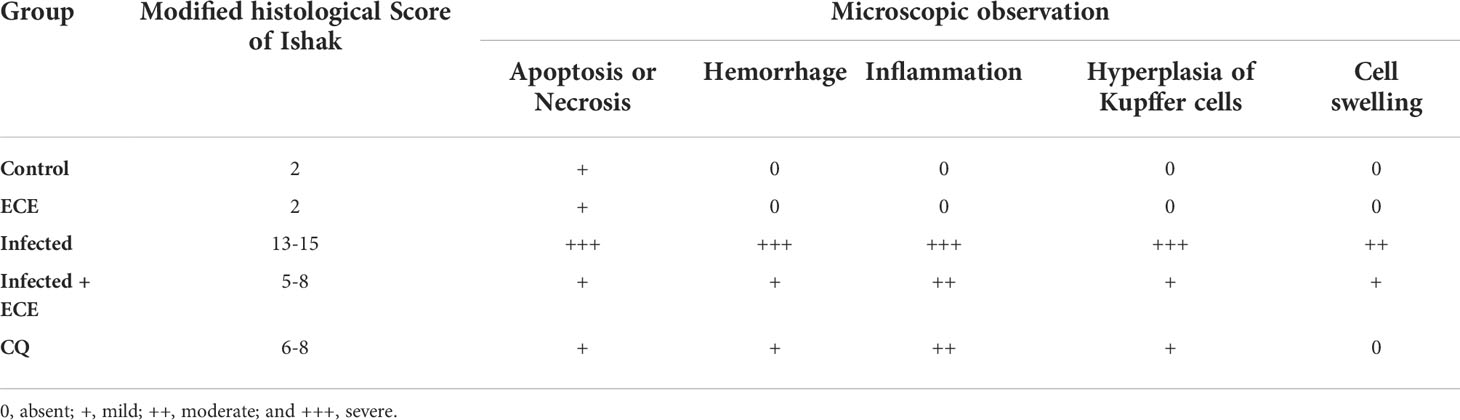
Table 5 Histology score demonstrating the protective effect of Eucalyptus camaldulensis extract (ECE) against P. chabaudi-parasitized erythrocytes in the liver of mice compared to chloroquine (CQ).
Compared to those in the control group, mice in the infected group exhibited significantly higher liver MDA and NO levels, lower GSH levels, and a significant decrease in antioxidant catalase enzyme activity (Figure 4). Notably, ECE as CQ supplementation restored the balance between oxidants and antioxidants in the hepatic tissue following infection by decreasing the production of MDA and NO and enhancing the examined antioxidant proteins (Figure 3).
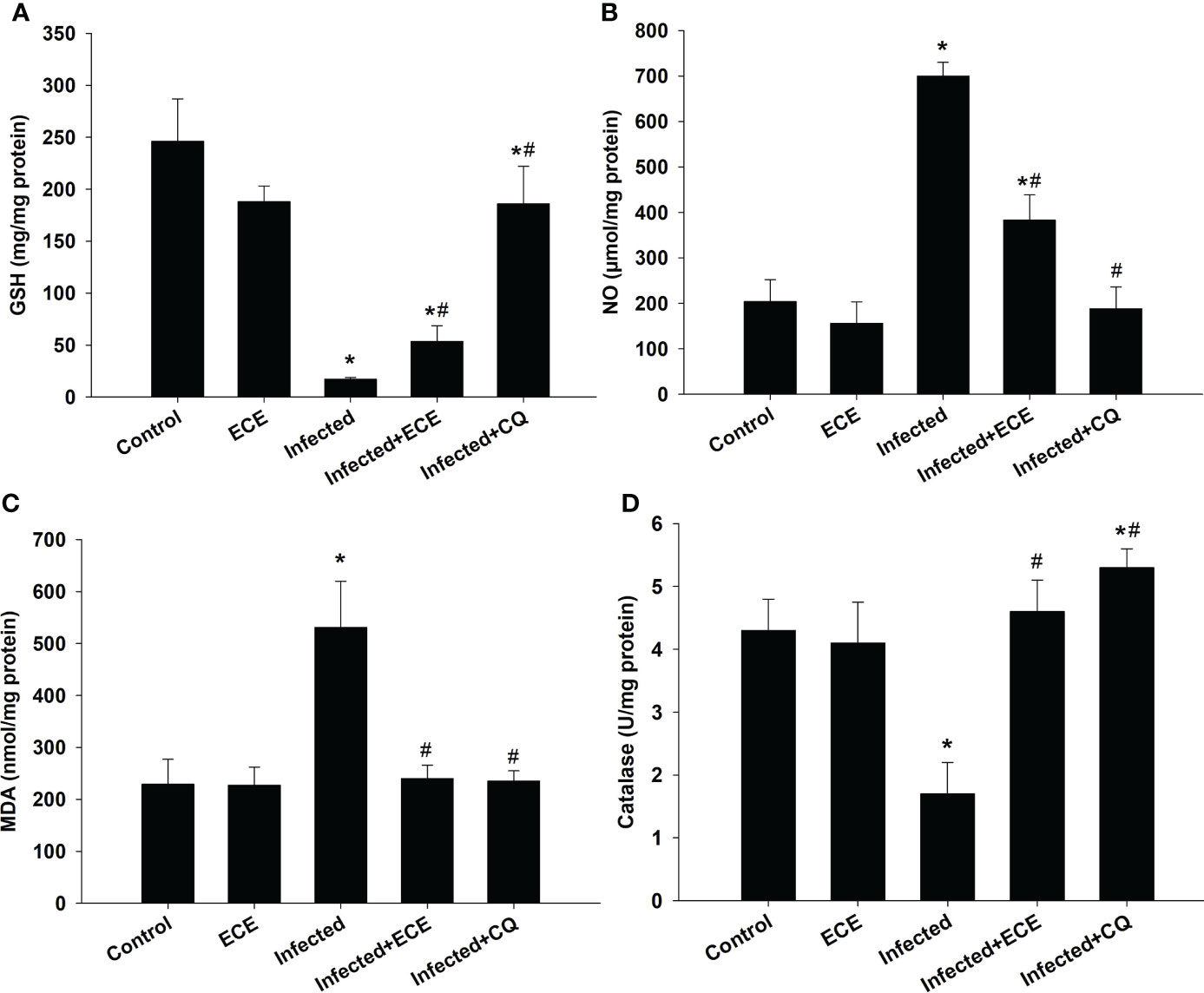
Figure 4 Effect of Eucalyptus camaldulensis extract (ECE) on the level of (A) glutathione (GSH), (B) nitric oxide (NO), (C) malondialdehyde (MDA), and (D) catalase in liver of mice infected with P. chabaudi. Data are presented as mean ± SD at p ≤ 0.001. * and #: Significance against the control and infected groups, respectively.
P. chabaudi infection induced hepatic tissue inflammation, as reflected in a significant elevation (p < 0.05) in the pro-inflammatory cytokine levels of IL-1β, IL-6, and IFN-γ (Figure 5). However, this inflammatory response was significantly suppressed following ECE or CQ administration, reflecting the protective impact of ECE as CQ against inflammatory events triggered during the development of malaria.
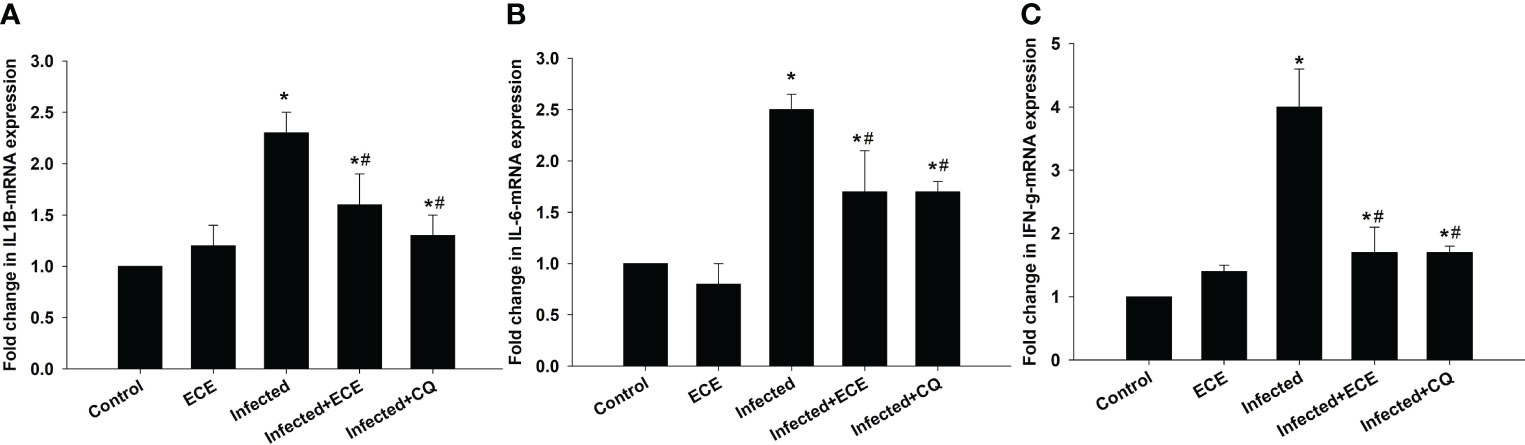
Figure 5 Effect of Eucalyptus camaldulensis extract (ECE) on (A) IL1β, (B) IL-6, and (C) IFN-γ-mRNA expression in liver of mice infected with P. chabaudi. Data are presented as mean ± SD at p ≤ 0.001. * and #: Significance against the control and infected groups, respectively.
Discussion
Natural products play a crucial role in the exploration of new leads for drug therapy against human diseases. Most medicinal agents used in the treatment of malaria are extracted from plants or natural products (Moyo et al., 2020). Moreover, there is always an urgent and continuing call to look for new antimalarial agents a most antimalarial drugs in current use have been rendered inefficient due to drug resistance.
Eucalyptus camaldulensis is considered a source of biologically active compounds rich in phenolics and flavonoids. These compounds could be the contributing factors towards the antimalarial effect and reduced parasitemia following the treatment of infected animals (Table 2). Recently, Anigboro et al. (2020) reported that E. camaldulensis aqueous leaf extracts could protect against P. berghei by significantly reducing malarial-induced physiological imbalances in liver and renal biomarkers, as well as serum electrolytes and this is owing to the presence of phytochemically active compounds in the extract by inducing malaria and dysfunction in the liver and kidney.
The GC mass analysis showed that ECE possesses several active compounds, including Naphthalene, an intestinal antiseptic and vermicide (IARC Working Group on the Evaluation of Carcinogenic Risks to Humans, 2002) as well as hexadecanoic acid and methyl ester (palmitic acid) are used in herbal medicine as antioxidant, anti-inflammatory, and anti-apoptotic agents (Hamed et al., 2020). In addition, methyl stearate is used to treat neural and cardiac diseases (Chen et al., 2020).
In our study, ECE treatment improved the health of host mice by reducing both weight loss and anemia relative to non-infected controls due to decreased parasitemia, which causes loss of appetite and decrease in erythrocyte count and hemoglobin content during infection. Similar results were obtained in our previous studies using different medicinal plants (see Dkhil et al., 2021).
Eucalyptus camaldulensis can generally reduce the activity of liver function enzymes (Anigboro et al., 2020). In this study, treatment of P. chabaudi-infected animals with ECE decreased blood plasma ALT, AST, and ALP levels. This demonstrates that plant extracts can improve the effects of malaria-induced liver damage.
The observed potential therapeutic effect of the extracts on liver enzyme activity could be attributed to parasite elimination and/or antioxidant effects of the plant extracts, which could be derived from its bioactive constituents (Gomes et al., 2011; Nutham et al., 2015).
The decrease in erythrocyte count and hemoglobin concentrations in P. chabaudi-infected mice indicated that the mice were anemic (Dkhil et al., 2019). Anemia causes changes in tissue physiology and histology, oxidative damage, and formation of free radicals. According to Omodeo-Salè et al. (2003), oxidative damage promotes the development of anemia during P. falciparum infection. This is due to an imbalance between oxidants and antioxidants, resulting in the formation of free radicals that cause oxidative injury to the liver (Guha et al., 2006). In addition, anemia is due to parasite usage of hemoglobin as a source of nutrition, thereby liberating heme, which promotes oxidative damage and produces histopathological changes in the liver (Kumar and Bandyopadhyay, 2005). These biochemical and histological findings are consistent with those of previous studies (Lubbad et al., 2015; Dkhil et al., 2019) that investigated the hepatoprotective effects of herbal extracts against P. chabaudi infection.
Our findings indicated that P. chabaudi-induced hepatic inflammation is accompanied by an inflammatory reaction in the liver. Furthermore, our results indicate that ECE not only kills Plasmodium parasites in animals but also has anti-inflammatory properties that protect the liver.
Infection with P. chabaudi could produce an inflammatory reaction in the mouse liver. This response is characterized by a change in liver structure as well as significant changes in oxidative stress biomarkers (GSH, NO, MDA, and catalase) and the inflammatory cytokines IFN-, IL-1β, and IL-6. Plasmodium infection is characterized by early and intensive cytokine-mediated effector mechanisms that kill or eliminate parasite-infected cells, and are linked to both acquired and innate immune responses (Gowda and Wu, 2018). In addition, an increase in IL-6 and IFN-γ levels is associated with hyperparasitemia (Angulo and Fresno, 2002). Moreover, inflamed liver cells, activated leukocytes, and macrophages generate excessive levels of pro-inflammatory cytokines and enzymes under inflammatory conditions, assisting in the initiation of the innate immune response (Wunderlich et al., 2014). IL-1 and IL-6 are proinflammatory cytokines that play a big role in inflammation and immunity, and they have been discovered to be upregulated following Plasmodium infection (Dkhil et al., 2021). Interestingly, ECE administration inhibited the development of inflammatory reactions following P. chabaudi infection by downregulating cytokines and controlling oxidative changes induced in the liver. All of these reduced inflammatory responses of ECE was originally due to the reduced number of parasitized erythrocytes as seen in Figure 1. Several reports support our findings that medicinal plants are able to reduce the parasitemia and protect the liver from inflammation (Wunderlich et al., 2014; Dkhil et al., 2019; Dkhil et al., 2021).
Here, we postulate that E. camaldulensis is capable of protecting the hepatic tissue following malaria-induced infection, as evidenced by the improved percentage of parasitemia and histological structure. In addition, E. camaldulensis restored the balance between oxidants and antioxidants, while suppressing inflammation in the liver. These findings indicate that E. camaldulensis is a promising hepatoprotective agent against malaria. Furthermore, additional studies are necessary to clarify the mechanisms underlying the liver’s capacity to respond to both P. chabaudi and E. camaldulensis.
Data availability statement
The original contributions presented in the study are included in the article/Supplementary Material, further inquiries can be directed to the corresponding author.
Ethics statement
The experiments were approved by the Research Ethics Committee for Laboratory Animal Care at King Saud University (approval no.: KSU-Se-21-77).
Author contributions
HA, SA-Q, and MD designed the study; HA, RA-G, EA-S, FT, MQ, MM, MMM, TA-O, MA, and MD carried out the experiments and analyzed the data. All authors wrote and revised the manuscript. All authors read and approved the final manuscript.
Funding
This study was supported by Researchers Supporting Project (RSP2021/03), King Saud University, Riyadh, Saudi Arabia.
Conflict of interest
The authors declare that the research was conducted in the absence of any commercial or financial relationships that could be construed as a potential conflict of interest.
Publisher’s note
All claims expressed in this article are solely those of the authors and do not necessarily represent those of their affiliated organizations, or those of the publisher, the editors and the reviewers. Any product that may be evaluated in this article, or claim that may be made by its manufacturer, is not guaranteed or endorsed by the publisher.
Supplementary Material
The Supplementary Material for this article can be found online at: https://www.frontiersin.org/articles/10.3389/fcimb.2022.955042/full#supplementary-material
References
Abay, S. M., Lucantoni, L., Dahiya, N., Dori, G., Dembo, E. G., Esposito, F., et al. (2015). Plasmodium transmission blocking activities of vernonia amygdalina extracts and isolated compounds. Malaria J. 14, 288. doi: 10.1186/s12936-015-0812-2
Aebi, H. (1984). Catalase in vitro. Methods Enzymol. 105, 121–126. doi: 10.1016/S0076-6879(84)05016-3
Al-Zharani, M., Nasr, F. A., Abutaha, N., Alqahtani, A. S., Noman, O. M., Mubarak, M., et al. (2019). Apoptotic induction and anti-migratory effects of rhazya stricta fruit extracts on a human breast cancer cell line. Molecules 24 (21), 3968. doi: 10.3390/molecules24213968
Angulo, I., Fresno, M. (2002). Cytokines in the pathogenesis of and protection against malaria. Clin. Diag. Lab. Immunol. 9 (6), 1145–1152. doi: 10.1128/CDLI.9.6.1145-1152.2002
Anigboro, A. A., Avwioroko, O. J., Cholu, C. O. (2020). Phytochemical constituents, antimalarial efficacy, and protective effect of eucalyptus camaldulensis aqueous leaf extract in plasmodium berghei-infected mice. Prev. Mutrit. Food Sci. 25 (1), 58–64. doi: 10.3746/pnf.2020.25.1.58
Batish, D. R., Singh, H. P., Kohli, R. K., Kaur, S. (2008). Eucalyptus essential oil as a natural pesticide. For. Ecol. Manag 256, 2166–2174. doi: 10.1016/j.foreco.2008.08.008
Carlton, J. M., Angiuoli, S. V., Suh, B. B., Kooij, T. W., Pertea, M., Silva, J. C., et al. (2002). Genome sequence and comparative analysis of the model rodent malaria parasite plasmodium yoelii yoelii. Nature 419 (6906), 512–519. doi: doi.org/10.1038/nature01099
Chen, P. Y., Wu, C. Y., Clemons, G. A., Citadin, C. T., Couto E Silva, A., Possoit, H. E., et al. (2020). Stearic acid methyl ester affords neuroprotection and improves functional outcomes after cardiac arrest. Prostaglandins Leukot. Essen. Fatty Acids 159, 102138. doi: 10.1016/j.plefa.2020.102138
Dkhil, M. A., Al-Quraishy, S., Al-Shaebi, E. M., Abdel-Gaber, R., Thagfan, F. A., Qasem, M. A. (2021). Medicinal plants as a fight against murine blood-stage malaria. Saudi J. Biol. Sci. 28 (3), 1723. doi: 10.1016/j.sjbs.2020.12.014
Dkhil, M. A., Al-Shaebi, E., Al-Quraishy, S. (2019). Effect of indigofera oblongifolia on the hepatic oxidative status and expression of inflammatory and apoptotic genes in blood-stage murine malaria. Oxid. Med. Cell. Longev. 2019, 8264861. doi: 10.1155/2019/8264861
Drury, R. A. B., Wallington, E. A. (1980). Carleton’s histological technique (Oxford: Oxford University Press).
Ellman, G. L. (1959). Tissue sulfhydryl groups. Arch. Biochem. Biophysics 82 (1), 70–77. doi: 10.1016/0003-9861(59)90090-6
Ghalem, B. R., Mohamed, B. (2008). Antibacterial activity of leaf essential oils of eucalyptus globulus and eucalyptus camaldulensis. Afr. J. Pharm. Pharmacol. 2, 211–215.
Ghalem, B. R., Mohamed, B. (2014). Antibacterial activity of essential oil of north west Algerian eucalyptus camaldulensis against escherichia coli and staphylococcus aureus. J. Coast. Life Med. 2 (10), 799–804. doi: 10.12980/JCLM.2.201414B57
Ghosh, S., Derle, A., Ahire, M., More, P., Jagtap, S., Phadatare, S. D., et al. (2013). Phytochemical analysis and free radical scavenging activity of medicinal plants gnidia glauca and dioscorea bulbifera. PloS One 8, e82529. doi: 10.1371/journal.pone.0082529
Gomes, A. P., Vitorino, R. R., Costa, A., Mendonça, E. G., Oliveira, M. G. A., Siqueira, B. R. (2011). Severe plasmodium falciparum malaria. Rev. Bras. Ter. Intensive 23, 358–369. doi: 10.1590/S0103-507X2011000300015
Gowda, D. C., Wu, X. (2018). Parasite recognition and signaling mechanisms in innate immune responses to malaria. Front. Immunol. 9, 3006. doi: 10.3389/fimmu.2018.03006
Green, L. C., Wagner, D. A., Glogowski, J. (1982). Analysis of nitrate, nitrite, and [15N] nitrate in biological fluids. Analyt. Biochem. 126 (1), 131–138. doi: 10.1016/0003-2697(82)90118-X
Guha, M., Kumar, S., Choubey, V., Maity, P., Bandyopadhyay, U. (2006). Apoptosis in liver during malaria: role of oxidative stress and implication of mitochondrial pathway. FASEB J. 20, E439–E449. doi: 10.1096/fj.05-5338fje
Hamed, A. B., Mantawy, E. M., El-Bakly, W. M., Abdel-Mottaleb, Y., Azab, S. S. (2020). Putative anti-inflammatory, antioxidant, and anti-apoptotic roles of the natural tissue guardian methyl palmitate against isoproterenol-induced myocardial injury in rats. Futur. J. Pharm. Sci. 6, 31. doi: 10.1186/s43094-020-00044-y
Hassani, S., Asghari, G., Yousefi, H., Kazemian, A., Rafieiean, M., Darani, H. Y. (2013). Effects of different extracts of eucalyptus camaldulensis on trichomonas vaginalis parasite in culture medium. Advanced Biomed. Res. 2, 47. doi: 10.4103/2277-9175.114187
Hoffman, R. (2009). Hematology: Basic principles and practice (Philadelphia, PA: Churchill Livingstone/Elsevier).
IARC Working Group on the Evaluation of Carcinogenic Risks to Humans (2002). Some traditional herbal medicines, some mycotoxins, naphthalene and styreneIARC monographs on the evaluation of carcinogenic risks to humans, no. 82 (Lyon (FR: International Agency for Research on Cancer). Available at: https://www.ncbi.nlm.nih.gov/books/NBK326618/.
Ishak, K., Baptista, A., Bianchi, L., Callea, F., de Groote, J., Gudat, F., et al. (1995). Histological grading and staging of chronic hepatitis. J. Hepatol. 22, 696–699. doi: 10.1016/0168-8278(95)80226-6
Kanthal, L. K., Dey, A., Satyavathi, K., Bhojaraju, P. (2014). GC-MS analysis of bio-active compounds in methanolic extract of lactuca runcinata DC. Pharmacog. Res. 6 (1), 58–61. doi: 10.4103/0974-8490.122919
Kumar, S., Bandyopadhyay, U. (2005). Free heme toxicity and its detoxification systems in human. Toxicol. Lett. 157, 175–188. doi: 10.1016/j.toxlet.2005.03.004
Livak, K. J., Schmittgen, T. D. (2001). Analysis of relative gene expression data using real-time quantitative PCR and the 2– ΔΔCT method. Methods 25 (4), 402–408. doi: 10.1006/meth.2001.1262
Lubbad, M. Y., Al-Quraishy, S., Dkhil, M. A. (2015). Antimalarial and antioxidant activities of indigofera oblongifolia on plasmodium chabaudi-induced spleen tissue injury in mice. Parasitol. Res. 114 (9), 3431–3438. doi: 10.1007/s00436-015-4568-y
Medhi, S. M., Reza, S., Mahnaz, K., Reza, A. M., Abbas, H., Fatemeh, M., et al. (2010). Phytochemistry and larvicidal activity of eucalyptus camaldulensis against malaria vector, anopheles stephensi. Asian Pacif J. Trop. Med. 3, 841–845. doi: 10.1016/S1995-7645(10)60203-9
Moyo, P., Mugumbate, G., Eloff, J. N., Louw, A. I., Maharaj, V. J., Birkholtz, L. M. (2020). Natural products: A potential source of malaria transmission blocking drugs? Pharmaceuticals 13 (9), 251. doi: 10.3390/ph13090251
Newman, D. J., Cragg, G. M. (2016). Natural products as sources of new drugs from 1981 to 2014. J. Nat. Prod. 79 (3), 629–661. doi: 10.1021/acs.jnatprod.5b01055
Nutham, N., Sakulmettatham, S., Klongthalay, S., Chutoam, P., Somsak, V. (2015). Protective effects of tinospora crispa stem extract on renal damage and hemolysis during plasmodium berghei infection in mice. J. Pathog. 2015, 738608. doi: 10.1155/2015/738608
Ohkawa, H., Ohishi, N., Yagi, K. (1979). Assay for lipid peroxides in animal tissues by thiobarbituric acid reaction. Analyt. Biochem. 95 (2), 351–358. doi: 10.1016/0003-2697(79)90738-3
Omodeo-Salè, F., Motti, A., Basilico, N., Parapini, S., Olliaro, P., Taramelli, D. (2003). Accelerated senescence of human erythrocytes cultured with plasmodium falciparum. Blood 102, 705–711. doi: 10.1182/blood-2002-08-2437
Singab, A., Ayoub, N., Al-Sayed, E., Martiskainen, O., Sinkkonen, J., Pihlaja, K. (2011). Phenolic constituents of eucalyptus camaldulensis dehnh, with potential antioxidant and cytotoxic activities. Rec. Nat. Prod. 5, 271–280.
Taherkhani, M., Rustaiyan, A., Nahrevanian, H., Naeimi, S., Taherkhani, T. (2013). Comparison of antimalarial activity of artemisia turanica extract with current drugs in vivo. J. Vect. Borne Dis. 50, 51–56.
Wunderlich, F., Al-Quraishy, S., Dkhil, M. A. (2014). Liver-inherent immune system: its role in blood-stage malaria. Front. Microbiol. 5, 559. doi: 10.3389/fmicb.2014.00559
Keywords: Eucalyptus camaldulensis, malaria, mice, inflammation, oxidative damage, gene expression
Citation: Aljawdah HMA, Abdel-Gaber R, Al-Shaebi EM, Thagfan FA, Al-Quraishy S, Qasem MAA, Murshed M, Mares MM, Al-Otaibi T, Hawsah MA and Dkhil MA (2022) Hepatoprotective activity of Eucalyptus camaldulensis extract in murine malaria mediated by suppression of oxidative and inflammatory processes. Front. Cell. Infect. Microbiol. 12:955042. doi: 10.3389/fcimb.2022.955042
Received: 27 May 2022; Accepted: 25 July 2022;
Published: 12 August 2022.
Edited by:
Bernhard Biersack, University of Bayreuth, GermanyReviewed by:
Mohamed Z. M. Salem, Alexandria University, EgyptDiego Luis Costa, Faculty of Medicine of Ribeirão Preto, University of São Paulo, Brazil
Copyright © 2022 Aljawdah, Abdel-Gaber, Al-Shaebi, Thagfan, Al-Quraishy, Qasem, Murshed, Mares, Al-Otaibi, Hawsah and Dkhil. This is an open-access article distributed under the terms of the Creative Commons Attribution License (CC BY). The use, distribution or reproduction in other forums is permitted, provided the original author(s) and the copyright owner(s) are credited and that the original publication in this journal is cited, in accordance with accepted academic practice. No use, distribution or reproduction is permitted which does not comply with these terms.
*Correspondence: Mohamed A. Dkhil, mohameddkhil@yahoo.com