- Division of Cellular and Applied Infection Biology, Institute of Zoology, RWTH Aachen University, Aachen, Germany
Members of the WD40-repeat protein family can be found in all eukaryotic proteomes where they usually serve as interaction platforms for the assembly of large protein complexes and are therefore essential for the integrity of these complexes. In the malaria parasite Plasmodium falciparum, the WD40-repeat protein PfWLP1 has been shown to interact with members of distinct adhesion protein complexes in the asexual blood stages and gametocyte stages. In this study, we demonstrate that the presence of PfWLP1 is crucial for both the stability of these gametocyte-specific adhesion complexes as well as for gametocyte maturation and gametogenesis. Using reverse genetics, we generated a PfWLP1-knockdown parasite line for functional characterization of the protein. Knockdown of PfWLP1 resulted in a slight reduction of gametocyte numbers and significantly the impaired ability of the gametocytes to exflagellate. PfWLP1-knockdown further led to reduced protein levels of the Limulus coagulation factor C-like (LCCL)-domain proteins PfCCp1 and PfCCp2, which are key components of the adhesion complexes. These findings suggest that the interaction of PfWLP1 with members of the PfCCp-based adhesion complex ensures complex stability and thereby contributes to gametocyte viability and exflagellation.
Introduction
The malaria parasite Plasmodium falciparum is transmitted from human to human through the bloodmeal of the mosquito vector. While symptoms in the human host are caused by asexual blood stage parasites, which multiply in the human red blood cells (RBCs), transmission to the mosquito is mediated by intraerythrocytic sexual precursor cells, the gametocytes. During their maturation in the human RBCs, the gametocytes pass through five morphologically distinguishable stages. When the mature gametocytes are taken up by the mosquito during its bloodmeal, they undergo sexual reproduction in the mosquito midgut and continue their life-cycle in the vector (reviewed in Kuehn and Pradel, 2010; Bennink et al., 2016).
The sexual phase of the malaria parasite goes along with the coordinated expression of stage-specific adhesion proteins, which assemble to complexes at the parasite plasma membrane. The complexes include members of the P. falciparum LCCL domain-containing protein family (PfCCp) (Pradel et al., 2004; Pradel et al., 2006; Scholz et al., 2008; Simon et al., 2009; Simon et al., 2016; reviewed in Kuehn et al., 2010). The six PfCCp proteins, which are highly conserved among the apicomplexan clade (Pradel et al., 2004; Becker et al., 2010; Bastos et al., 2013; reviewed in Dessens et al., 2004), interact by adhesion domain-mediated binding and assemble to a complex with adhesive properties. The PfCCp-based protein complex is localized in the lumen of the parasitophorous vacuole (Pradel et al., 2004) and is anchored to the gametocyte plasma membrane via interactions with Pfs230, which itself is binding to the GPI-anchored protein Pfs48/45 (Kumar, 1987; Kumar and Wizel, 1992).
Upon gametocyte activation, coupling of the complex to the membrane is enhanced through the proteolytic processing of Pfs230, additional protein-protein-interactions and the integration of the adhesion protein Pfs25 into the complex, which further connects the complex with the plasma membrane via a GPI-anchor (Simon et al., 2016). These adhesion complexes are particularly present on the surface of the extracellular macrogametes that have formed during gametogenesis. It has been hypothesized that the PfCCp-based adhesion complex might play a role in adhesive processes during fertilization such as mediating contact between the female macrogamete and male microgamete (reviewed in Kuehn et al., 2010).
The six PfCCp proteins exhibit a co-dependent expression so that the lack of one PfCCp protein leads to the loss of other members of the family, while Pfs230 expression is not affected (Simon et al., 2009). Further, the properly processed form of Pfs230 is necessary for the correct assembly of the complex, since incorrect processing or lack of Pfs230 leads to proteolysis of the PfCCp proteins and release from the complex in activated gametocytes (Brooks and Williamson, 2000; Simon et al., 2016).
Previously, the WD40-repeat protein-like protein PfWLP1 has been identified as a component of the PfCCp-based protein complex in P. falciparum gametocytes (von Bohl et al., 2015). WD40-repeat protein-like proteins are characterized by WD40 domains, which in general are composed of seven or multiple of seven WD40-repeats. The repeats are short repeating amino acid motifs with a length of approximately 44 to 60 amino acids which are terminated by a tryptophan-aspartate (WD) dipeptide (reviewed in e.g. Voorn and Ploegh, 1992; Neer et al., 1994; Xu and Min, 2011). WD40-domain containing proteins act as scaffolds for the formation of large protein complexes and are involved in numerous biological processes such as signal transduction, cell division or transcription regulation depending on their interaction partners (reviewed in Stirnimann et al., 2010; Jain and Pandey, 2018).
In the P. falciparum genome, 80 putative WD40-domain containing proteins have been identified in an in silico analysis, most of which remain uncharacterized until now (Chahar et al., 2015). PfWLP1, which has been demonstrated to be unique for the genus Plasmodium, has been shown to be expressed in the asexual schizont stage and during gametocyte development (von Bohl et al., 2015). In schizonts, the protein initially accumulates underneath the plasma membrane and subsequently relocalizes underneath the micronemes, when the merozoites have formed. In merozoites, PfWLP1 then interacts with the transmembrane protein PfAMA1 (apical membrane antigen 1) – a part of the AMA1/RON (rhoptry neck protein)-complex, which is involved in tight junction formation during RBC invasion (Lamarque et al., 2011; reviewed in Boucher and Bosch, 2015). In the sexual stages, PfWLP1 primarily localizes underneath the plasma membrane and associates with PfCCp1 and Pfs230 in maturing and activated gametocytes (von Bohl et al., 2015).
In this study we aimed to analyse in more detail the link between PfWLP1 and the PfCCp-based adhesion complex and to unveil the role of PfWLP1 for gametocytes. To address the question, we generated a glmS-based PfWLP1-knockdown (KD) line and functionally characterized the protein using biochemical and cell-based assays.
Methods
Gene Identifiers
The following PlasmoDB gene identifiers are assigned to the proteins investigated in this study: Pf39 [PF3D7_1108600], PfCCp1 [PF3D7_1475500], PfCCp2 [PF3D7_1455800], Pfs230 [PF3D7_0209000], PfWLP1 [PF3D7_1443400].
Antibodies
The following antisera were used in this study: mouse polyclonal antisera against PfCCp1rp1 (Scholz et al., 2008), Pfs230 region C (Williamson et al., 1995), Pf39rp1 (Simon et al., 2009) and PfWLP1rp1 (von Bohl et al., 2015); rabbit polyclonal antisera against PfCCp2 (Simon et al., 2009) and Pfs230 (Biogenes); and polyclonal rabbit anti-HA antibody (Sigma-Aldrich).
Parasite Lines
In this study, the Plasmodium falciparum wild-type (WT) strain NF54 (ATCC) was used. The generation of the PfWLP1-KD line is described below.
Parasite Culture
The asexual blood stage parasites and gametocytes of the WT strain NF54 (WT NF54) and the PfWLP1-KD line were cultivated in human A+ RBCs in RPMI1640/HEPES medium (Gibco) supplemented with 10% v/v heat-inactivated human serum (A+). The medium was further complemented with 50 µg/ml hypoxanthine (Sigma-Aldrich) and 10 µg/ml gentamicin (Gibco). For cultivation of the PfWLP1-KD line, the selection drug WR99210 (Jacobus Pharmaceutical Company) was added in a final concentration of 2.5 nM. The cultures were kept at 37°C in an atmosphere of 5% O2 and 5% CO2 in N2. Human A+ RBCs and sera were purchased from the Institute of Transfusion Medicine, University Hospital Aachen, Germany. The donors remained anonymous and the RBC and serum samples were pooled. The work on human blood was approved by the Ethics Commission of RWTH Aachen University. Synchronization of ring stage parasites was performed using sorbitol treatment as described (Lambros and Vanderberg, 1979). Gametocyte stages were enriched by Percoll gradient centrifugation (GE Healthcare Life Science) as previously described (Kariuki et al., 1998). In vitro induction of gametogenesis was performed by incubating mature gametocytes in 100 µM xanthurenic acid dissolved in 1% v/v 0.5 M NH4OH/ddH2O for 15 min at RT.
Generation of PfWLP1-KD and Induction of Knockdown
The PfWLP1-KD line was generated by single-crossover homologous recombination using the pARL-HA-glmS vector (Flammersfeld et al., 2020). A 1053-bp fragment homologous to the 3’ end of the pfwlp1 gene was amplified via PCR using forward primer 5’-TAGCGCGGCCGCGAATTCTAAAAATATGGCTACCTAC-3’ and reverse primer 5’-TACGCCCTAGGAAAAGCCACAAACGCCCAGAG-3’. Ligation of insert and vector backbone was mediated by NotI and AvrII restriction sites that were added to the PCR product via the primers. A synchronized WT NF54 ring stage culture was electroporated with 100 µg of the plasmid in transfection buffer (310 V, 950 µF, 12 ms; Bio-Rad gene pulser Xcell) as described (Wirth et al., 2014). WR99210 was added to the culture in a final concentration of 2.5 nM starting 6 h after transfection. Once resistant parasites appeared in the culture, they were checked for plasmid integration into the genome by diagnostic PCR. Therefore, genomic DNA of the transfected cultures was isolated using the NucleoSpin Blood Kit (Macherey-Nagel) and used as a template in the PCR. The following primers were used in the PCR (see Figure S1A for regions of primer binding): PfWLP1-KD-5’ integration forward primer 5’-CAATATATTAATGACAAGCGGTTATGATGG-3’ (primer 1), PfWLP1-KD-3’ integration reverse primer 5’-GTATATAATTTTCATGTTTTTAATATTGTACTCTC-3’ (primer 2), pARL-HA-glmS forward primer 5’-GCTTTACACTTTATGCTTCCGGCTCG-3’ (primer 3) and pARL-HA-glmS reverse primer 5’-CCTTAGAGCTCGGCATAATCTGG-3’ (primer 4). To induce the glmS-ribozyme and thereby the knockdown of pfwlp1 gene expression, the culture was treated with 2.5 mM glucosamine hydrochloride (GlcN; D-(+)-glucosamine hydrochloride; Sigma-Aldrich) as described (Prommana et al., 2013).
Growth Assays and Exflagellation Assay
To investigate the asexual blood stage replication and gametocyte development, cultures of the WT NF54 strain or the PfWLP1-KD line were synchronized and set to an initial parasitemia of 0.25% or 2%, respectively. The parasites were cultivated either in the presence or absence of 2.5 mM GlcN. For the asexual growth assay, samples were taken and Giemsa-stained thin blood smears were prepared every 12 h over a time period of 96 h. For the gametocyte development assay, smears were prepared at five or six different time points over a time period of 13 to 15 d. The smears were evaluated microscopically at 1,000-fold magnification and parasitemia and gametocytemia were calculated by counting the percentage of parasites or gametocytes in 1,000 RBCs in triplicate, respectively. For the comparative exflagellation assay, gametocytes of the WT NF54 strain and the PfWLP1-KD line were cultivated in presence or absence of 2.5 mM GlcN for 12 to 14 days. Two days before the execution of the assay, GlcN was withdrawn from the cultures, since no exflagellation can be observed in GlcN-containing medium. The gametocytemia was adjusted between the samples to be compared and 100 µl of each culture was activated in vitro as described above. At 15 min post-activation, numbers of exflagellation centers were counted microscopically at 400-fold magnification in 30 optical fields.
Western Blot Analysis
While asexual blood stage parasites from WT NF54 and the PfWLP1-KD line were harvested from either mixed or synchronized cultures, gametocytes were enriched via Percoll gradient centrifugation (see above). Parasites were released from the enveloping RBC membrane by incubation in 0.05% w/v saponin/PBS for 10 min. Parasites were pelleted and resuspended in lysis buffer (150 mM NaCl, 0.1% v/v Triton X-100, 0.5% w/v sodium deoxycholate, 0.1% w/v SDS, 50 mM Tris-HCl pH 8.0) supplemented with protease inhibitor cocktail (complete EDTA-free, Roche). The lysates were supplemented with 5x SDS-PAGE loading buffer containing 25 mM dithiothreitol and heat-denatured at 95°C for 10 min. The protein lysates were separated via SDS-PAGE and subsequently transferred to a Hybond ECL nitrocellulose membrane (Amersham Biosciences). Blocking of non-specific binding sites was performed by incubation of the membrane in Tris-buffered saline containing 5% w/v skim milk, pH 7.5. For immunodetection, the membrane was incubated with polyclonal mouse anti-Pf39 antisera (dilution 1:2,500), mouse anti-PfCCp1 antisera (dilution 1:1,000), rabbit anti-PfCCp2 antisera (dilution 1:1,000) or rabbit anti-HA antibody (1:5,000) diluted in blocking solution at 4°C overnight. The membrane was washed and further incubated for 1 h at RT with a goat anti-mouse or anti-rabbit alkaline phosphatase-coupled secondary antibody (dilution 1:10,000, Sigma-Aldrich) and developed in a solution of nitroblue tetrazolium chloride (NBT) and 5-bromo-4-chloro-3-indoxylphosphate (BCIP, Sigma-Aldrich) for 5-15 min at RT. After being scanned, Western blots (WBs) were processed and band intensities were measured using the ImageJ 1.51f software.
Indirect Immunofluorescence Assay
Untreated and GlcN-treated gametocytes of the PfWLP1-KD line were air-dried as cell monolayers on glass slides and fixed in methanol at -80°C for 10 min. Membrane permeabilization and blocking of non-specific binding sites was performed by incubation in 0.01% w/v saponin/0.5% w/v BSA/PBS with 1% v/v neutral goat serum for 30 min at RT. For immunostaining, the preparations were incubated for 2 h at 37°C with polyclonal mouse anti-PfWLP1 antiserum (dilution 1:50), polyclonal mouse anti-PfCCp1 antiserum (dilution 1:50) or polyclonal rabbit anti-PfCCp2 antiserum (dilution 1:200) diluted in blocking solution. After washing, binding of the primary antibody was detected by incubation with Alexa Flour 488-conjugated goat anti-mouse or goat anti-rabbit secondary antibody (Invitrogen) diluted 1:1,000 in PBS for 45 min at 37°C. Counterstaining of the gametocyte plasma membrane was performed by incubation with polyclonal rabbit anti-Pfs230 antiserum (dilution 1:500) or polyclonal mouse anti-Pfs230 antiserum (dilution 1:300) in blocking solution for 1 h at 37°C, followed by incubation for 45 min at 37°C with polyclonal Alexa Flour 594-conjugated goat anti-rabbit or goat anti-mouse secondary antibody (Invitrogen) diluted 1:1,000 in PBS. Parasite nuclei were highlighted by treatment with Hoechst 33342 nuclear stain (Invitrogen) for 1 min at RT. Cells were mounted with anti-fading solution AF2 (CitiFlour™) and sealed with nail varnish. Microscopic evaluation was performed using a Leica DM 5500 B fluorescence microscope and fluorescence intensity was quantified using ImageJ 1.51f. Images were processed using Adobe Photoshop CS software.
Results
Generation of a PfWLP1-KD Parasite Line
PfWLP1 was previously shown to be a 96-kDa cytosolic protein comprising five WD40 motifs that accumulates underneath the plasma membrane in gametocytes and here associates with members of the PfCCp-based adhesion protein complex (von Bohl et al., 2015). So far, the generation of a PfWLP1-knockout (KO) parasite line remains unsuccessful, although genetic manipulation of the pfwlp1 gene locus is possible (von Bohl et al., 2015). To characterize the role of PfWLP1 in the formation of the gametocyte specific adhesion protein complex in more detail, an inducible PfWLP1-KD line was generated, using the previously described pARL-HA-glmS vector (Flammersfeld et al., 2020). The pARL-HA-glmS-WLP1 vector was electroporated into ring stage parasites and WR99210 resistant parasites were obtained after six weeks. Integration of the vector into the pfwlp1 gene locus was confirmed by integration PCR (Figures S1A, B). Successful downregulation of pfwlp1 gene expression and in consequence reduced protein levels were confirmed by WB. For this, mixed asexual blood parasites of the PfWLP1-KD line were cultivated in the presence or absence of 2.5 mM GlcN for three days. Subsequent WB using a polyclonal anti-HA antibody confirmed the presence of the PfWLP1-HA fusion protein in lysates of the mutant line. Further, treatment with GlcN led to a reduction of PfWLP1-HA protein levels by approximately 45% (Figures S1C, D). Immunoblotting with polyclonal mouse anti-Pf39 antisera was used as a loading control and for normalization of band intensities. To confirm the downregulation of PfWLP1 in the gametocyte stages, gametocytes of the PfWLP1KD-line were cultivated in the presence or absence of 2.5 mM GlcN for 14 d, followed by WB analysis and quantification of the PfWLP1-HA-specific band intensities was performed as described above. Similar to the asexual blood stages, GlcN-treatment in gametocytes led to a significant reduction of PfWLP1-HA protein levels by approximately 40% (Figures 1A, B). Reduction of PfWLP1-HA protein levels in gametocytes upon GlcN-treatment was further confirmed via immunofluorescence assay (IFA), where even a reduction of the protein levels by approximately 75% was detected (Figures 1C, D). To investigate, if the reduction of the PfWLP1-HA protein levels affect the morphology of the parasites, thin blood smears were prepared from WT NF54 and PfWLP1-KD cultures that were cultivated either in the presence or absence of 2.5 mM GlcN. The smears were Giemsa-stained and evaluated by light microscopy. The treatment with GlcN did not have a visible effect on the morphology of either WT NF54 or PfWLP1-KD blood stage parasites. Both, asexual blood stages and gametocytes at different stages of maturity exhibited normal morphologies after GlcN-treatment (Figure 1E).
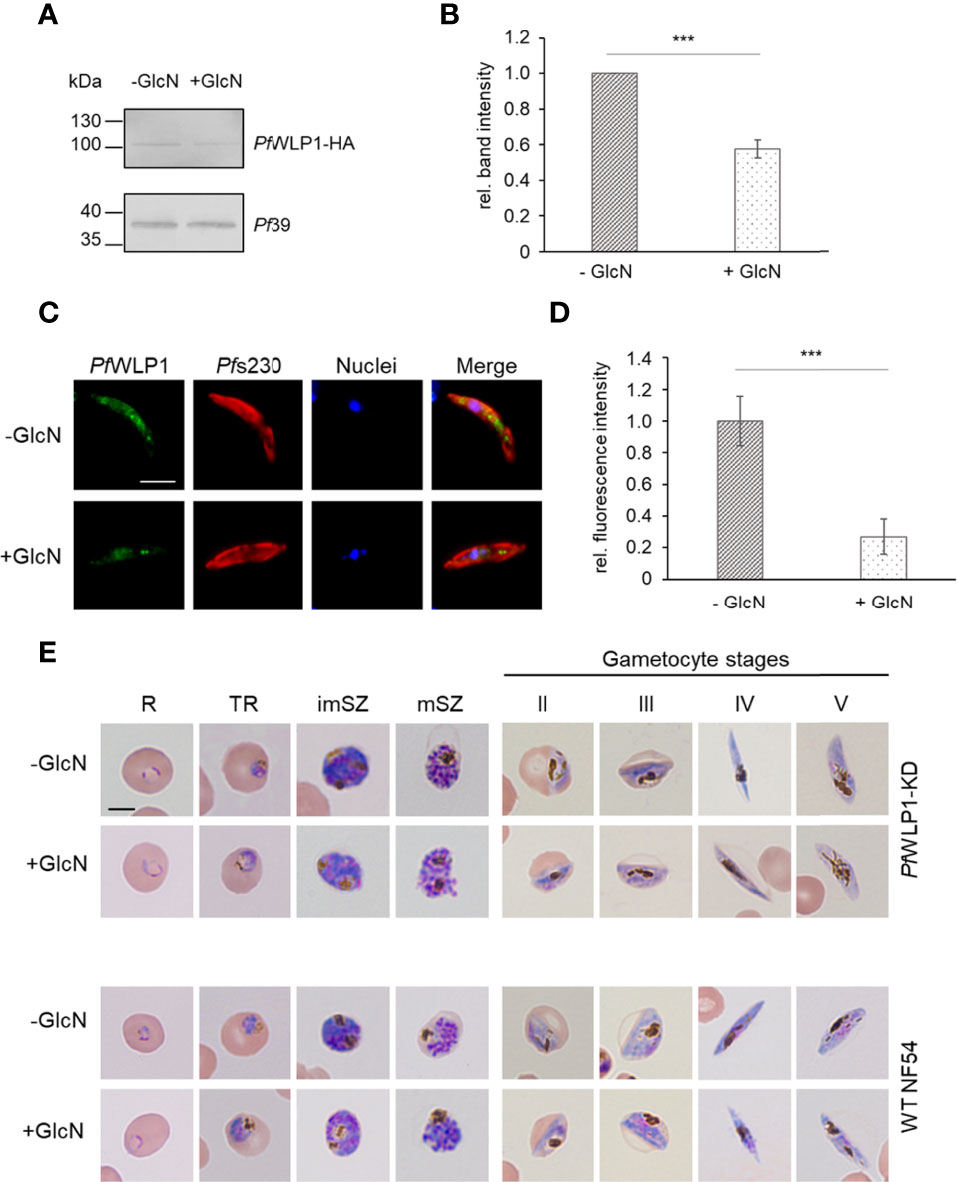
Figure 1 Knockdown of PfWLP1 does not affect parasite morphology. (A) Downregulation of PfWLP1-HA expression in PfWLP1-KD gametocytes. Gametocytes of the PfWLP1-KD line were cultivated either in the presence or absence of 2.5 mM GlcN for 14 d. Protein lysates were subjected to WB using rabbit anti-HA antibody to detect PfWLP1-HA (~108 kDa). Equal loading was confirmed using a polyclonal mouse anti-Pf39 antiserum (~39 kDa). (B) Quantification of the PfWLP1-HA expression in PfWLP1-KD gametocytes. The intensities of the PfWLP1-HA specific protein bands of three independent WB (performed as described in A) were quantified using ImageJ 1.51f and normalized to the respective band intensities for Pf39 (mean ± SD; untreated set to 1). ***p < 0.001 (Student’s t-test). (C) Immunolabeling of PfWLP1-HA in gametocytes of the PfWLP1-KD line. Gametocytes of the PfWLP1-KD line were cultivated as described in (A) Untreated and GlcN-treated gametocytes were immunolabeled with polyclonal mouse anti-PfWLP1 antiserum (green) and counterstained with polyclonal rabbit anti-Pfs230 antiserum (red). Nuclei were highlighted with Hoechst 33342 nuclear stain (blue). Bar, 5 µm. (D) Quantification of the PfWLP1-HA fluorescence signal in PfWLP1-KD gametocytes in the presence or absence of GlcN. IFAs were performed as described in (C) The fluorescence intensity of the PfWLP1-HA signal was quantified in 20 gametocytes using ImageJ 1.51f (mean ± SD; untreated set to 1). ***p < 0.001 (Student’s t-test). (E) Morphology of PfWLP1-KD blood stage parasites. Asexual blood stage parasites and gametocytes of WT NF54 and PfWLP1-KD were cultivated either in the presence or absence of 2.5 mM GlcN. Cultures were treated with GlcN for 3 d (asexual blood stages) or 5-14 d (gametocyte stages). The morphology was analyzed via Giemsa staining of blood smears. R, ring; TR, trophozoite; imSZ, immature schizont; mSZ, mature schizont. Bar, 5 µm. The results (A, C, E) are representative for three independent experiments.
PfWLP1 Is Crucial for Exflagellation
Comparative phenotype analyses between WT NF54 and the PfWLP1-KD line cultivated either in the presence or absence of 2.5 mM GlcN showed that downregulation of PfWLP1-HA does not have a significant effect on the asexual blood stage replication (Figures 2A, Figure S1E). However, GlcN-treated PfWLP1-KD parasites showed a significant reduction in gametocyte numbers formed after 13 d of cultivation compared to untreated parasites (Figure 2B), while GlcN-treatment did not affect gametocyte formation in WT NF54 parasites (Figure S1F), indicating that PfWLP1 impacts gametocyte maturation. Hence, the ability of the parasites to form motile male microgametes was analyzed in exflagellation assays. Therefore, WT NF54 and PfWLP1-KD gametocytes were grown in GlcN-containing medium until maturity. Two days prior to gametocyte activation, GlcN was withdrawn from the cultures. After in vitro activation of the gametocytes, the numbers of exflagellation centers were evaluated by light microscopy. GlcN-treatment resulted in a significant inhibition of exflagellation in PfWLP1-KD gametocytes when compared to GlcN-treated WT NF54 gametocytes (Figure 2C), pointing to a crucial role of PfWLP1 in exflagellation. Untreated PfWLP1-KD and WT NF54 gametocytes on the other hand exhibited comparable numbers of exflagellation centers in this experiment (Figure 2C).
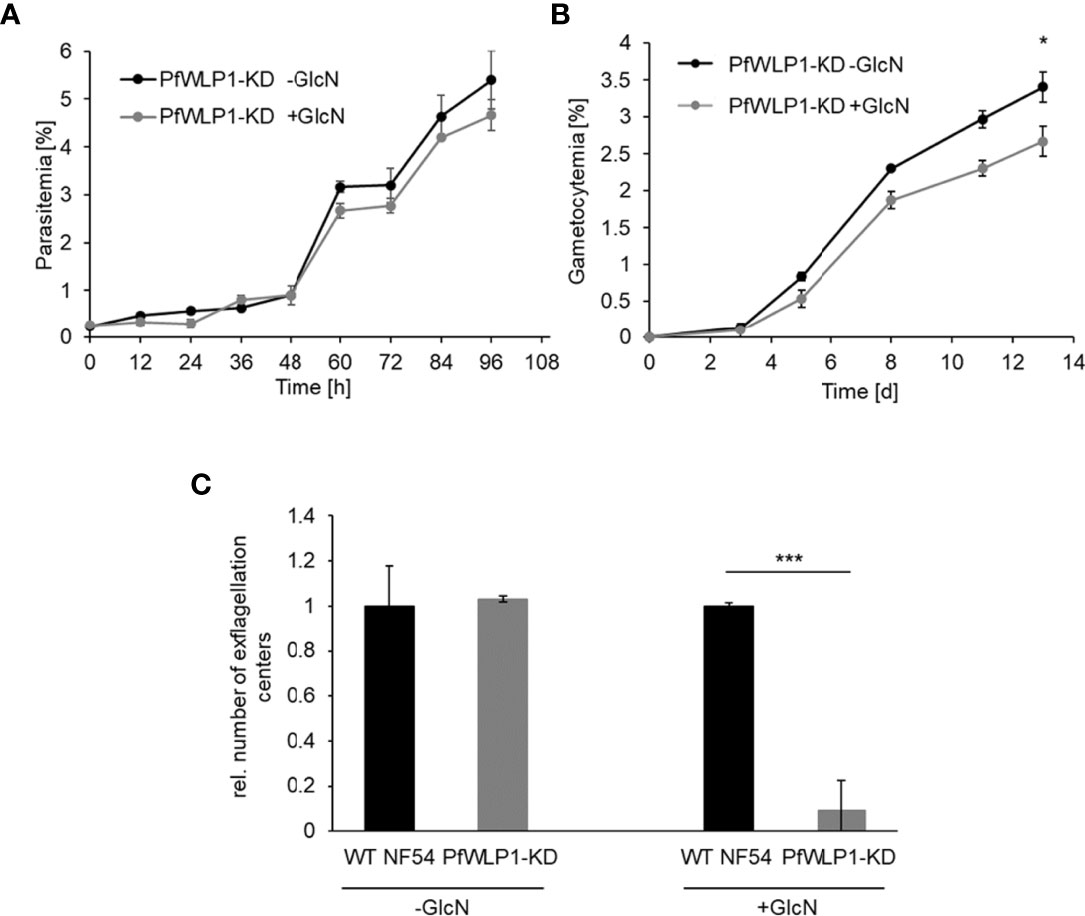
Figure 2 Parasite development and exflagellation in the PfWLP1-KD line. (A) Asexual blood stage replication of PfWLP1-KD parasites. Synchronized ring stage parasites of the PfWLP1-KD line with a starting parasitemia of 0.25% were cultivated either in the presence or absence of 2.5 mM GlcN for 96 h. The parasitemia was evaluated microscopically every 12 h using Giemsa smears. The experiment was performed in triplicate (mean ± SD). (B) Gametocyte formation of PfWLP1-KD parasites. Synchronized ring stage parasites of the PfWLP1-KD line with a starting parasitemia of 2% were cultivated either in the presence or absence of 2.5 mM GlcN over a time period of 13 d. The numbers of gametocytes were evaluated microscopically on 5 time points using Giemsa smears. The experiment was performed in triplicate (mean ± SD). The differences in gametocytemia between the treated and untreated culture on day 13 were investigated statistically (*p < 0.05; Student’s t-test). (C) Formation of exflagellation centers in the PfWLP1-KD line. WT NF54 and PfWLP1-KD gametocytes were cultivated in 2.5 mM GlcN. Two days before the exflagellation assay, GlcN was withdrawn from the culture. The gametocytes were induced in vitro and 15 min after activation the numbers of exflagellation centers were evaluated microscopically in 30 optical fields. Untreated cultures served as control. The experiment was performed in triplicate (mean ± SD; numbers of exflagellation centers in WT NF54 cultures were set to 1). ***p < 0.001 (Student’s t-test). The results (A–C) are representative for three independent experiments.
Knockdown of PfWLP1 Leads to Reduced Expression of Members of the PfCCp-Complex
Previous studies unveiled a co-localization and interaction of PfWLP1 with members of the PfCCp-complex (von Bohl et al., 2015). With the help of the PfWLP1-KD line we now aimed at investigating a potential effect of PfWLP1 on the integrity and stability of the PfCCp-based adhesion protein complex. Therefore, gametocytes of the PfWLP1-KD line were treated with GlcN to induce KD of PfWLP1 and protein levels of selected members of the PfCCp-complex (i.e. PfCCp1 and PfCCp2) were evaluated. In IFA, immunolabelling for both, PfCCp1 and PfCCp2 was significantly reduced in GlcN-treated PfWLP1-KD gametocytes compared to untreated gametocytes (Figures 3A, B). However, the expression pattern analysis showed a typical punctuated localization of the remaining PfCCp1 and PfCCp2 on the gametocyte plasma membrane, as it was previously described (Pradel et al., 2004; Pradel et al., 2006). Noteworthy, the immunolabeling for Pfs230, which was used for counterstaining, did not alter between the treated and untreated gametocytes, although it is also part of the PfCCp-protein complex (Scholz et al., 2008; Simon et al., 2009; reviewed in Kuehn et al., 2010). In WT NF54 gametocytes, GlcN-treatment did not have an effect on the intensity of PfCCp1- and PfCCp2-fluorescence signals (Figures S2A, B). PfCCp1 and PfCCp2 protein levels were further compared in protein lysates of GlcN-treated and untreated PfWLP1-KD gametocytes via WB followed by quantification of band intensities. Immunoblotting and quantification revealed that the PfCCp1- and PfCCp2-specific protein bands were significantly less intense in the GlcN-treated samples compared to the untreated samples (Figures 3C, D). Immunoblotting with antisera against Pf39 served as a loading control and was used for normalization of the band intensities. The combined data demonstrate that the lack of PfWLP1 results in a reduction of PfCCp proteins on the gametocyte plasma membrane.
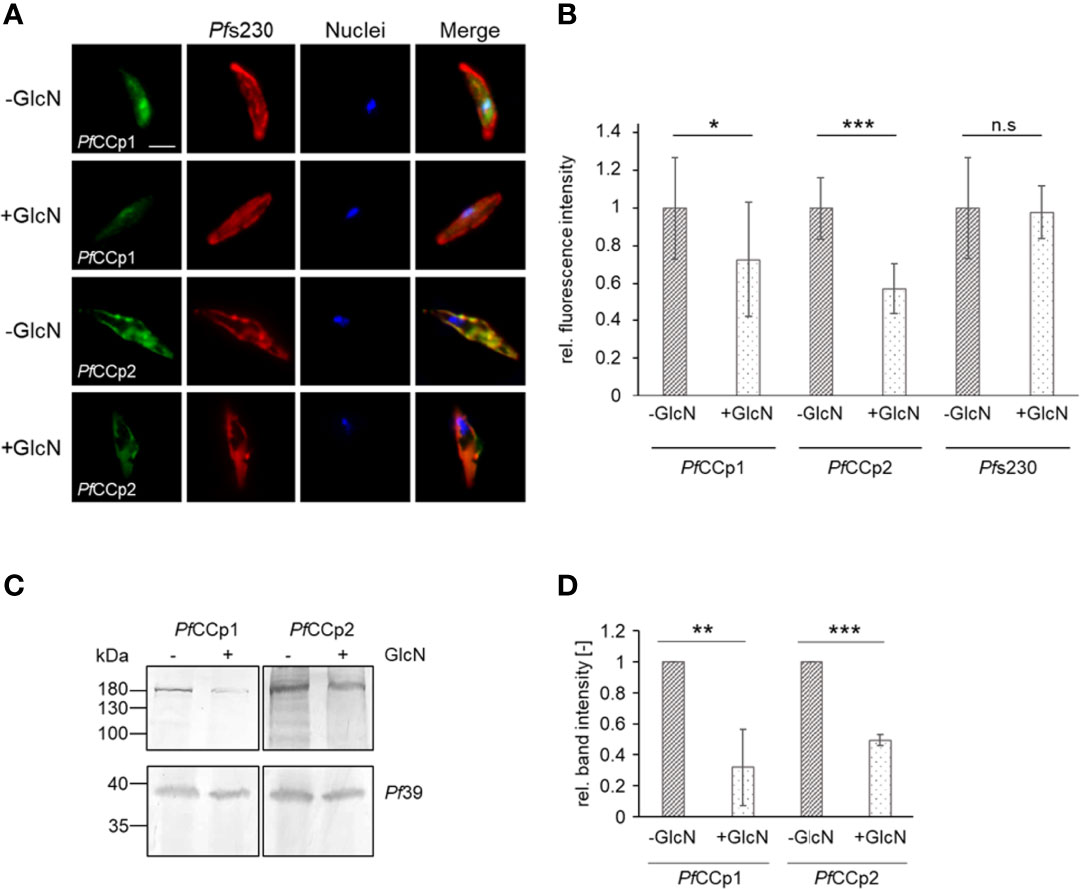
Figure 3 PfWLP1-KD affects protein abundance of PfCCp1 and PfCCp2. (A) Immunolabeling of PfCCp1 and PfCCp2 in gametocytes of the PfWLP1-KD line. Gametocytes of the PfWLP1-KD line were cultivated either in the presence or absence of GlcN for 14 d. Untreated and GlcN-treated gametocytes were immunolabeled with polyclonal mouse anti-PfCCp1 antiserum or polyclonal rabbit anti-PfCCp2 antiserum (green) and counterstained with polyclonal rabbit or mouse anti-Pfs230 antisera (red). Nuclei were highlighted with Hoechst 33342 nuclear stain (blue). Bar, 5 µm. (B) Immunolabeling has been performed as described in (A) The fluorescence intensity of PfCCp1, PfCCp2 and Pfs230 signals was quantified in 20 gametocytes using ImageJ 1.51f (mean ± SD; untreated set to 1). *p < 0.05; ***p < 0.001; n.s., not significant (Student’s t-test). The results are representative for two independent experiments. (C) Gametocytes of the PfWLP1-KD line were cultivated as described in (A) Gametocyte protein lysates were subjected to WB and PfCCp1 (~185 kDa) and PfCCp2 (~185 kDa) were immunolabeled with polyclonal mouse anti-PfCCp1 and rabbit anti-PfCCp2 antisera, respectively. Equal loading was confirmed using a polyclonal mouse anti-Pf39 antiserum (~39 kDa). (D) Quantification of the PfCCp1 and PfCCp2 expression in PfWLP1-KD gametocytes. The intensities of the PfCCp1 and PfCCp2 specific protein bands of three independent WB (performed as described in C) were quantified using ImageJ 1.51f and normalized to the respective band intensities for Pf39 (mean ± SD; untreated set to 1). **p < 0.01; ***p < 0.001 (Student’s t-test). The results (A, C, D) are representative for three independent experiments.
Discussion
Apicomplexan parasites exhibit various types of adhesive protein complexes on the cell membranes, which exert distinct functions and which depend on the life-cycle stage of the parasite. These adhesive multi-protein complexes are utilized by the parasites for different types of intercellular contact, ranging from gliding and attachment to target cells to host cell invasion and cytoadherence of infected RBCs. In the malaria parasite P. falciparum, membrane-coupled protein complexes composed of transmembrane proteins as well as GPI-anchored and peripheral adhesion proteins are known for the different life-cycle stages of the parasite. One example is the merozoite surface protein (MSP)-based protein complex, which is essential for the successful attachment of the merozoites to RBCs prior to invasion (reviewed in Cowman and Crabb, 2006). Merozoites further express the PfAMA1/RON complex, which defines the merozoite-RBC moving junction (e.g. Collins et al., 2009; Richard et al., 2010; Srinivasan et al., 2011). PfAMA1 is stored in the micronemes and translocated to the merozoite surface before invasion, while at the same time, the RON-complex is inserted into the host cell membrane (Cao et al., 2009; Riglar et al., 2011; reviewed in Shen and Sibley, 2012).
An adhesion complex similar in its structure to the PfMSP-based complex is found in gametocytes and gametes. The multi-protein complex is based on the six members of the LCCL-domain/PfCCp protein family and further includes the 6-cys proteins Pfs230 and Pfs48/45, the latter of which is linked to the membrane via a GPI anchor. In addition, the EGF-domain protein Pfs25 links the complex to the macrogamete surface (e.g. Simon et al., 2009; Simon et al., 2016; reviewed in Kuehn et al., 2010). The adhesive PfCCp-based protein complex on the surface of the gametes is crucial for sexual reproduction. Interestingly, (KO) of the PfCCp-proteins does not abolish exflagellation in the respective KO-lines, but impairs the transition of midgut sporozoites to the salivary glands (Pradel et al., 2004; Scholz et al., 2008). Pfs230-KO parasites are also able to egress from the host RBC and exflagellate, but are unable to interact with surrounding cells and form exflagellation centers (Eksi et al., 2006).
In addition to the adhesion proteins on the gametocyte plasma membrane, the cytosolic WD40-domain protein PfWLP1 has previously been described as an interaction partner of the PfCCp-protein complex (von Bohl et al., 2015). PfWLP1 is a member of the WD40-domain containing protein family which is one of the most abundant protein classes in eukaryotes and also includes prokaryotic proteins. Out of the 80 putative WD40-domain-containing proteins that have been identified in the P. falciparum genome (Chahar et al., 2015), only a few have been partially characterized so far, including for example the nuclear pore complex component PfSec13, which is also involved in the biogenesis of COPII-coated vesicles (Dahan-Pasternak et al., 2013), PfRACK, an ortholog of the receptor for activated C kinase (Madeira et al., 2003) and the histone chaperone PfRbAp46/48 (Kaushik et al., 2020). PfWLP1 was shown to be expressed in the maturing schizont as well as the gametocyte stages, where it accumulates underneath the plasma membrane and interacts with the stage-specific PfAMA1/RON- and PfCCp-complexes, respectively (von Bohl et al., 2015).
In this study we have successfully generated a PfWLP1-KD line, in which the PfWLP1 protein levels can be reduced by approximately 40-45% via GlcN-mediated transcript degradation, as detected in WB. The KD did not show any growth defect during the asexual replication cycle, pointing to a non-essential role of PfWLP1 in schizogony, contrary to assumptions in previous studies, in which a gene-KO remained unsuccessful (von Bohl et al., 2015) and a piggyBac transposon mutagenesis study that confirmed essentiality of PfWLP1 (Zhang et al., 2018). These contradictory data might be explained by the low KD rate, in asexual blood stages after 3 d of GlcN-treatment. Longer GlcN-treatment could lead to higher KD rates which might result in growth defects.
In the PfWLP1-KD, gametocyte maturation was affected and exflagellation was severely impaired when compared to WT NF54 parasites. Interestingly, the exflagellation ability is not affected in KOs of PfCCp1 – an interaction partner of PfWLP1 – or other PfCCp proteins. Noteworthy, antibodies against PfCCp1-4 and PfFNPA are able to inhibit exflagellation, however this effect is only measurable in active serum and not in heat-inactivated serum, pointing to a participation of active complement in the process (Scholz et al., 2008).
We further showed that the KD of PfWLP1 leads to reduced abundance of members of the PfCCp-complex, i.e. its interaction partners PfCCp1 and PfCCp2. Noteworthy, in the GlcN-treated PfWLP1-KD the remaining PfCCp1 and PfCCp2 proteins showed a similar subcellular localization as in the untreated culture, indicating that the PfCCp-complex still assembles and localizes correctly in the PfWLP1-KD line. However, low levels of PfCCp proteins are still present on the gametocyte surface, which might also be explained by the fact, that only roughly 45% of PfWLP1 was downregulated. Interestingly, the expression of Pfs230, which is also a member of the PfCCp-based protein complex, was not affected in the PfWLP1-KD. Similar results were obtained when the co-dependent expression of the PfCCp-proteins was analysed. While KO of one PfCCp-protein led to the complete or partial loss of other members of the protein family, the expression and localization of Pfs230 was not affected, indicating that Pfs230 expression is not dependent on the presence and assembly of all complex members (Pradel et al., 2006; Simon et al., 2009; reviewed in Kuehn et al., 2010).
Based on these data we suggest that the cytosolic PfWLP1 serves as an interaction platform for the PfCCp-based adhesion protein complex in gametocytes and gametes and is important for its assembly and/or integrity. We hypothesize that the intracellular PfWLP1 binds to the PfCCp-complex via an unknown membrane-spanning linker protein, which binds to one or more PfCCp-proteins in the lumen of the parasitophorous vacuole. The complex is bound to Pfs230 via interactions of Pfs230 and PfCCp4 (see Figure 4). As PfWLP1 was described to localize in close proximity with sub-pellicular proteins (von Bohl et al., 2015), it might connect the membrane-linked protein complex with the inner membrane complex of the parasite.
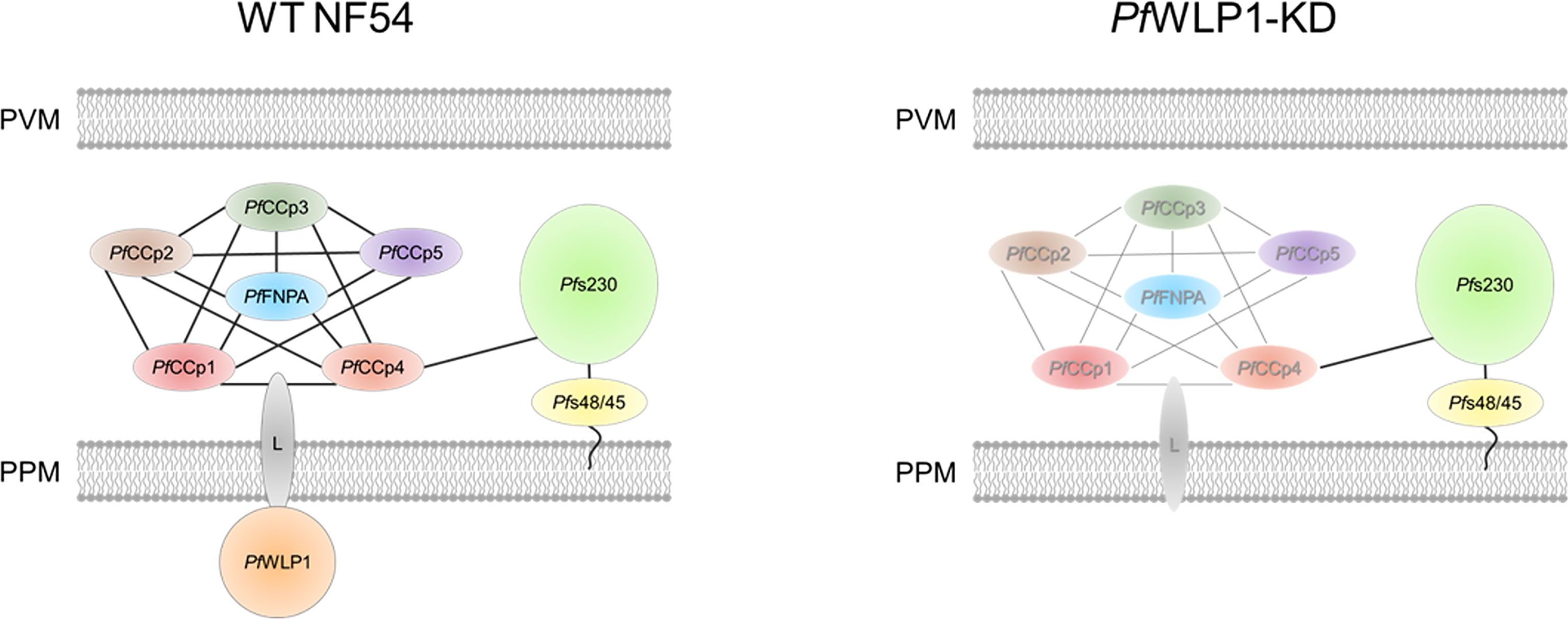
Figure 4 Model of the composition of the PfCCp-based protein complex in WT NF54 gametocytes and proposed changes in PfWLP1-KD gametocytes. Intracellular PfWLP1 probably binds to the PfCCp-complex via an unknown membrane-spanning linker protein (L). In the absence of PfWLP1, the PfCCp-complex is destabilized, while Pfs230 remains unaffected (destabilized complex shown in grey). PPM, parasite plasma membrane; PVM, parasitophorous vacuole membrane.
Since several members of the PfCCp-complex have been proposed and investigated as putative antigens for the development of transmission-blocking vaccines, further analysis of the constituents and interactions of this complex is of particular importance.
Data Availability Statement
The original contributions presented in the study are included in the article/Supplementary Material. Further inquiries can be directed to the corresponding author.
Author Contributions
LR: methodology and data analysis. AF: methodology and data analysis. SB: data analysis and visualization. GP: conception and project administration. SB and GP: writing original draft. All authors contributed to the article and approved the submitted version.
Funding
This work was supported by the Deutsche Forschungsgemeinschaft (PR905/15-1 to GP).
Conflict of Interest
The authors declare that the research was conducted in the absence of any commercial or financial relationships that could be construed as a potential conflict of interest.
Publisher’s Note
All claims expressed in this article are solely those of the authors and do not necessarily represent those of their affiliated organizations, or those of the publisher, the editors and the reviewers. Any product that may be evaluated in this article, or claim that may be made by its manufacturer, is not guaranteed or endorsed by the publisher.
Supplementary Material
The Supplementary Material for this article can be found online at: https://www.frontiersin.org/articles/10.3389/fcimb.2022.942364/full#supplementary-material
References
Bastos, R. G., Suarez, C. E., Laughery, J. M., Johnson, W. C., Ueti, M. W., Knowles, D. P. (2013). Differential Expression of Three Members of the Multidomain Adhesion CCp Family in Babesia Bigemina, Babesia Bovis and Theileria Equi. PloS One 8, e67765. doi: 10.1371/journal.pone.0067765
Becker, C. A. M., Malandrin, L., Depoix, D., Larcher, T., David, P. H., Chauvin, A., et al. (2010). Identification of Three CCp Genes in Babesia Divergens: Novel Markers for Sexual Stages Parasites. Mol. Biochem. Parasitol. 174, 36–43. doi: 10.1016/j.molbiopara.2010.06.011
Bennink, S., Kiesow, M. J., Pradel, G. (2016). The Development of Malaria Parasites in the Mosquito Midgut. Cell. Microbiol. 18, 905–918. doi: 10.1111/cmi.12604
Boucher, L. E., Bosch, J. (2015). The Apicomplexan Glideosome and Adhesins – Structures and Function. J. Struct. Biol. 190, 93–114. doi: 10.1016/j.jsb.2015.02.008
Brooks, S. R., Williamson, K. C. (2000). Proteolysis of Plasmodium falciparum Surface Antigen, Pfs230, During Gametogenesis. Mol. Biochem. Parasitol. 106, 77–82. doi: 10.1016/S0166-6851(99)00201-7
Cao, J., Kaneko, O., Thongkukiatkul, A., Tachibana, M., Otsuki, H., Gao, Q., et al. (2009). Rhoptry Neck Protein RON2 Forms a Complex With Microneme Protein AMA1 in Plasmodium falciparum Merozoites. Parasitol. Int. 58, 29–35. doi: 10.1016/j.parint.2008.09.005
Chahar, P., Kaushik, M., Gill, S. S., Gakhar, S. K., Gopalan, N., Datt, M., et al. (2015). Genome-Wide Collation of the Plasmodium falciparum WDR Protein Superfamily Reveals Malarial Parasite-Specific Features. PloS One 10, e0128507. doi: 10.1371/journal.pone.0128507
Collins, C. R., Withers-Martinez, C., Hackett, F., Blackman, M. J. (2009). An Inhibitory Antibody Blocks Interactions Between Components of the Malarial Invasion Machinery. PloS Pathog. 5, e1000273. doi: 10.1371/journal.ppat.1000273
Cowman, A. F., Crabb, B. S. (2006). Invasion of Red Blood Cells by Malaria Parasites. Cell 124, 755–766. doi: 10.1016/j.cell.2006.02.006
Dahan-Pasternak, N., Nasereddin, A., Kolevzon, N., Pe’er, M., Wong, W., Shinder, V., et al. (2013). Pfsec13 Is an Unusual Chromatin-Associated Nucleoporin of Plasmodium falciparum That Is Essential for Parasite Proliferation in Human Erythrocytes. J. Cell Sci. 126, 3055–3069. doi: 10.1242/jcs.122119
Dessens, J. T., Sinden, R. E., Claudianos, C. (2004). LCCL Proteins of Apicomplexan Parasites. Trends Parasitol. 20, 102–108. doi: 10.1016/j.pt.2004.01.002
Eksi, S., Czesny, B., van Gemert, G.-J., Sauerwein, R. W., Eling, W., Williamson, K. C. (2006). Malaria Transmission-Blocking Antigen, Pfs230, Mediates Human Red Blood Cell Binding to Exflagellating Male Parasites and Oocyst Production. Mol. Microbiol. 61, 991–998. doi: 10.1111/j.1365-2958.2006.05284.x
Flammersfeld, A., Panyot, A., Yamaryo-Botté, Y., Aurass, P., Przyborski, J. M., Flieger, A., et al. (2020). A Patatin-Like Phospholipase Functions During Gametocyte Induction in the Malaria Parasite Plasmodium falciparum. Cell. Microbiol. 22, e:13146. doi: 10.1111/cmi.13146
Jain, B. P., Pandey, S. (2018). WD40 Repeat Proteins: Signalling Scaffold With Diverse Functions. Protein J. 37, 391–406. doi: 10.1007/s10930-018-9785-7
Kariuki, M. M., Kiaira, J. K., Mulaa, F. K., Mwangi, J. K., Wasunna, M. K., Martin, S. K. (1998). Plasmodium falciparum: Purification of the Various Gametocyte Developmental Stages From In Vitro-Cultivated Parasites. Am. J. Trop. Med. Hyg. 59, 505–508.
Kaushik, M., Nehra, A., Gakhar, S. K., Gill, S. S., Gill, R. (2020). The Multifaceted Histone Chaperone RbAp46/48 in Plasmodium falciparum: Structural Insights, Production, and Characterization. Parasitol. Res. 119, 1753–1765. doi: 10.1007/s00436-020-06669-5
Kuehn, A., Pradel, G. (2010). The Coming-Out of Malaria Gametocytes. J. Biomed. Biotechnol. 2010, 976827. doi: 10.1155/2010/976827
Kuehn, A., Simon, N., Pradel, G. (2010). Family Members Stick Together: Multi-Protein Complexes of Malaria Parasites. Med. Microbiol. Immunol. 199, 209–226. doi: 10.1007/s00430-010-0157-y
Kumar, N. (1987). Target Antigens of Malaria Transmission Blocking Immunity Exist as a Stable Membrane Bound Complex. Parasite Immunol. 9, 321–335. doi: 10.1111/j.1365-3024.1987.tb00511.x
Kumar, N., Wizel, B. (1992). Further Characterization of Interactions Between Gamete Surface Antigens of Plasmodium falciparum. Mol. Biochem. Parasitol. 53, 113–120. doi: 10.1016/0166-6851(92)90013-a
Lamarque, M., Besteiro, S., Papoin, J., Roques, M., Vulliez-Le Normand, B., Morlon-Guyot, J., et al. (2011). The RON2-AMA1 Interaction Is a Critical Step in Moving Junction-Dependent Invasion by Apicomplexan Parasites. PloS Pathog. 7, e1001276. doi: 10.1371/journal.ppat.1001276
Lambros, C., Vanderberg, J. P. (1979). Synchronization of Plasmodium falciparum Erythrocytic Stages in Culture. J. Parasitol. 65, 418–420. doi: 10.2307/3280287
Madeira, L., DeMarco, R., Gazarini, M. L., Verjovski-Almeida, S., Garcia, C. R. S. (2003). Human Malaria Parasites Display a Receptor for Activated C Kinase Ortholog. Biochem. Biophys. Res. Commun. 306, 995–1001. doi: 10.1016/S0006-291X(03)01074-X
Neer, E. J., Schmidt, C. J., Nambudripad, R., Smith, T. F. (1994). The Ancient Regulatory-Protein Family of WD-Repeat Proteins. Nature 371, 297–300. doi: 10.1038/371297a0
Pradel, G., Hayton, K., Aravind, L., Iyer, L. M., Abrahamsen, M. S., Bonawitz, A., et al. (2004). A Multidomain Adhesion Protein Family Expressed in Plasmodium falciparum Is Essential for Transmission to the Mosquito. J. Exp. Med. 199, 1533–1544. doi: 10.1084/jem.20031274
Pradel, G., Wagner, C., Mejia, C., Templeton, T. J. (2006). Plasmodium falciparum: Co-Dependent Expression and Co-Localization of the PfCCp Multi-Adhesion Domain Proteins. Exp. Parasitol. 112, 263–268. doi: 10.1016/j.exppara.2005.11.010
Prommana, P., Uthaipibull, C., Wongsombat, C., Kamchonwongpaisan, S., Yuthavong, Y., Knuepfer, E., et al. (2013). Inducible Knockdown of Plasmodium Gene Expression Using the glmS Ribozyme. PloS One 8, e73783. doi: 10.1371/journal.pone.0073783
Richard, D., MacRaild, C. A., Riglar, D. T., Chan, J. A., Foley, M., Baum, J., et al. (2010). Interaction Between Plasmodium falciparum Apical Membrane Antigen 1 and the Rhoptry Neck Protein Complex Defines a Key Step in the Erythrocyte Invasion Process of Malaria Parasites. J. Biol. Chem. 285, 14815–14822. doi: 10.1074/jbc.M109.080770
Riglar, D. T., Richard, D., Wilson, D. W., Boyle, M. J., Dekiwadia, C., Turnbull, L., et al. (2011). Super-Resolution Dissection of Coordinated Events During Malaria Parasite Invasion of the Human Erythrocyte. Cell Host Microbe 9, 9–20. doi: 10.1016/j.chom.2010.12.003
Scholz, S. M., Simon, N., Lavazec, C., Dude, M. A., Templeton, T. J., Pradel, G. (2008). PfCCp Proteins of Plasmodium falciparum: Gametocyte-Specific Expression and Role in Complement-Mediated Inhibition of Exflagellation. Int. J. Parasitol. 38, 327–340. doi: 10.1016/j.ijpara.2007.08.009
Shen, B., Sibley, L. D. (2012). The Moving Junction, a Key Portal to Host Cell Invasion by Apicomplexan Parasites. Curr. Opin. Microbiol. 15, 449–455. doi: 10.1016/j.mib.2012.02.007
Simon, N., Kuehn, A., Williamson, K. C., Pradel, G. (2016). Adhesion Protein Complexes of Malaria Gametocytes Assemble Following Parasite Transmission to the Mosquito. Parasitol. Int. 65, 27–30. doi: 10.1016/j.parint.2015.09.007
Simon, N., Scholz, S. M., Moreira, C. K., Templeton, T. J., Kuehn, A., Dude, M.-A., et al. (2009). Sexual Stage Adhesion Proteins Form Multi-Protein Complexes in the Malaria Parasite Plasmodium falciparum. J. Biol. Chem. 284, 14537–14546. doi: 10.1074/jbc.M808472200
Srinivasan, P., Beatty, W. L., Diouf, A., Herrera, R., Ambroggio, X., Moch, J. K., et al. (2011). Binding of Plasmodium Merozoite Proteins RON2 and AMA1 Triggers Commitment to Invasion. Proc. Natl. Acad. Sci. USA 108, 13275–13280. doi: 10.1073/pnas.1110303108
Stirnimann, C. U., Petsalaki, E., Russel, R. B., Müller, C. W. (2010). WD40 Proteins Propel Cellular Networks. Trends Biochem. Sci. 35, 565–574. doi: 10.1016/j.tibs.2010.04.003
von Bohl, A., Kuehn, A., Simon, N., Ngongang, V. N., Spehr, M., Baumeister, S., et al. (2015). A WD40-Repeat Protein Unique to Malaria Parasites Associates With Adhesion Protein Complexes and Is Crucial for Blood Stage Progeny. Malar J. 14, 435. doi: 10.1186/s12936-015-0967-x
Voorn, L.V. D., Ploegh, H. L. (1992). The WD-40 Repeat. FEBS Lett. 307, 131–134. doi: 10.1016/0014-5793(92)80751-2
Williamson, K. C., Keister, D. B., Muratova, O., Kaslow, D. C. (1995). Recombinant Pfs230, a Plasmodium falciparum Gametocyte Protein, Induces Antisera That Reduce the Infectivity of Plasmodium falciparum to Mosquitoes. Mol. Biochem. Parasitol. 75, 33–42. doi: 10.1016/0166-6851(95)02507-3
Wirth, C. C., Glushakova, S., Scheuermayer, M., Repnik, U., Garg, S., Schaack, D., et al. (2014). Perforin-Like Protein PPLP2 Permeabilizes the Red Blood Cell Membrane During Egress of Plasmodium falciparum Gametocytes. Cell. Microbiol. 16, 709–733. doi: 10.1111/cmi.12288
Xu, C., Min, J. (2011). Structure and Function of WD40 Domain Proteins. Protein Cell 2, 202–214. doi: 10.1007/s13238-011-1018-1
Keywords: malaria, Plasmodium falciparum, gametocyte, WD40-repeat protein, adhesion protein complex, CCp proteins
Citation: Roling L, Flammersfeld A, Pradel G and Bennink S (2022) The WD40-Protein PfWLP1 Ensures Stability of the PfCCp-Based Adhesion Protein Complex in Plasmodium falciparum Gametocytes. Front. Cell. Infect. Microbiol. 12:942364. doi: 10.3389/fcimb.2022.942364
Received: 12 May 2022; Accepted: 23 June 2022;
Published: 18 July 2022.
Edited by:
Sudhir Kumar, Seattle Children’s Research Institute, United StatesCopyright © 2022 Roling, Flammersfeld, Pradel and Bennink. This is an open-access article distributed under the terms of the Creative Commons Attribution License (CC BY). The use, distribution or reproduction in other forums is permitted, provided the original author(s) and the copyright owner(s) are credited and that the original publication in this journal is cited, in accordance with accepted academic practice. No use, distribution or reproduction is permitted which does not comply with these terms.
*Correspondence: Sandra Bennink, bennink@bio2.rwth-aachen.de