- 1Department of Zoology, Abdul Wali Khan University Mardan, Mardan, Pakistan
- 2Department of Chemistry, Abdul Wali Khan University Mardan, Mardan, Pakistan
- 3King Abdulaziz City for Science and Technology, Riyadh, Saudi Arabia
- 4Department of Pediatrics, Yale School of Medicine Yale University, New Haven, CT, United States
- 5Centro de Biotecnologia and Faculdade de Veterinária, Universidade Federal do Rio Grande do Sul, Porto Alegre, Brazil
- 6Laboratory of Infectious Diseases, Joint Faculty of Veterinary Medicine, Kagoshima University, Kagoshima, Japan
Ticks are blood-feeding ecto-parasites that have a cosmopolitan distribution in tropical and subtropical regions of the world. Ticks cause economic losses in the form of reduced blood, meat and dairy products, as well as pathogen transmission. Different acaricides such as organochlorines, organophosphates, formamidines (e.g. amitraz), synthetic pyrethroids, macrocyclic lactones, fipronil, and fluazuron are currently used sequentially or simultaneously to control tick infestations. Most acaricide treatments now face increasingly high chances of failure, due to the resistance selection in different tick populations against these drugs. Acaricide resistance in ticks can be developed in different ways, including amino acid substitutions that result in morphological changes in the acaricide target, metabolic detoxification, and reduced acaricide entry through the outer layer of the tick body. The current literature brings a plethora of information regarding the use of different acaricides for tick control, resistance selection, analysis of mutations in target sites, and resistance mitigation. Alternatives such as synergistic use of different acaricides, plant-derived phytochemicals, fungi as biological control agents, and anti-tick vaccines have been recommended to avoid and mitigate acaricide resistance. The purpose of this review was to summarize and discuss different acaricides applied for tick control, their mechanisms of action and resistance selection, genetic polymorphisms in their target molecules, as well as the approaches used for diagnosis and mitigation of acaricide resistance, specifically in Rhipicephalus microplus ticks.
Introduction
Ticks are hematophagous ecto-parasites with global geographical distribution, especially in tropical and subtropical regions, where they parasitize terrestrial and semi-terrestrial vertebrates (Ali et al., 2019; Guglielmone et al., 2020). Ticks also serve as vector reservoirs for the spread of pathogens among their vertebrate hosts (Parola and Raoult, 2001). In the livestock industry, this results in significant losses due to host blood depletion, general discomfort and irritation, reduced dairy and meat production, suppression of immune functions, and damage to hides, among other negative impacts (Desta, 2016; Tabor et al., 2017).
Before the introduction of synthetic acaricides, different chemicals such as cotton-seed oil, fish oil, beaumont crude oil, the combination of lard oil with sulfur as well as lard oil with kerosene oil have been used on the host’s body surface to control tick infestations. In cattle, for example, the combination of kerosene oil with cotton-seed oil and sulfur, kerosene oil with cotton-seed oil, and crude petroleum alone have shown effectiveness against ticks (Mohler, 1906). Arsenic, introduced in 1895 (National Research Council, 1977; Angus, 1996), was the first acaricide widely used to control ticks and tick-borne diseases until the introduction of organochlorines (OCs), because it was cheap, stable, and water-soluble (Graham and Hourrigan, 1977; George et al., 2004). Arsenic was used to control tick infestations in different countries like Australia (Angus, 1996), South Africa (Matthewson and Baker, 1975), Mexico, and the USA (Drummond et al., 1964). In 1937, treatment failure due to the selection of arsenic resistant Rhipicephalus microplus was reported for the first time in Australia (Newton, 1967), and arsenic resistant Rhipicephalus decoloratus (Whitehead, 1958) as well as Rhipicephalus appendiculatus and Rhipicephalus evertsi (Matthewson and Baker, 1975) were documented in South Africa in 1940 and 1975, respectively.
The OCs, organophosphates (OPs), amitraz, synthetic pyrethroids (SPs), macrocyclic lactones (MLs), and fipronil are commonly used acaricides that act on the tick central nervous system through various mechanisms, affecting gamma-aminobutyric acid (GABA)-gated chloride channels, inhibitors of acetylcholine-esterase (AChEs), octopamine tyramine receptors, voltage-gated sodium channels, glutamate gated chloride channels (Glu-Cl), and GABA-receptors, respectively (Chen et al., 2007). Different acaricides have specific targets and different modes of action, which affects the reproduction, growth and survival of various tick species (Klafke et al., 2017; Klafke et al., 2019). Spraying, washing, pouring, and injections are the different methods for the application of acaricides on host animals (Food and Agriculture Organization, 2004; Higa et al., 2019). Incorrect dilution, inappropriate application, persistent use and overdosing are the main factors that hasten acaricide resistance selection in ticks (Aguilar-Tipacamu et al., 2011; Abbas et al., 2014). Certain species may be even more prone to develop acaricide resistance, due to favorable conditions created by their broad distribution and/or particularities of their life cycle (Guerrero et al., 2012a).
Rhipicephalus annulatus, R. microplus, and Rhipicephalus australis undergo rapid selection in response to selective pressure and higher concentration of acaricides (Burger et al., 2014). Different populations of R. microplus have been found resistant to all acaricides that have been used for their control, including: arsenics (Newton, 1967), OCs e.g. dichloro-diphenyl-trichloroethane (DDT) (Stone and Webber, 1960; Bandara and Karunaratne, 2017), OPs (Li et al., 2003; Miller et al., 2005), MLs e.g. ivermectin (Klafke et al., 2006; Perez-Cogollo et al., 2010a), formamidines e.g. amitraz (Miller et al., 2002), SPs (Guerrero et al., 2001; Kumar et al., 2013; Ghosh et al., 2015; Klafke et al., 2019), fipronil (Castro-Janer et al., 2010), and fluazuron (Reck et al., 2014). The surveillance of acaricide resistance in various regions is essential in order to adopt adequate treatment approaches. Globally the acaricide-resistant ticks identified through different diagnostic tests have been reported (Table 1).
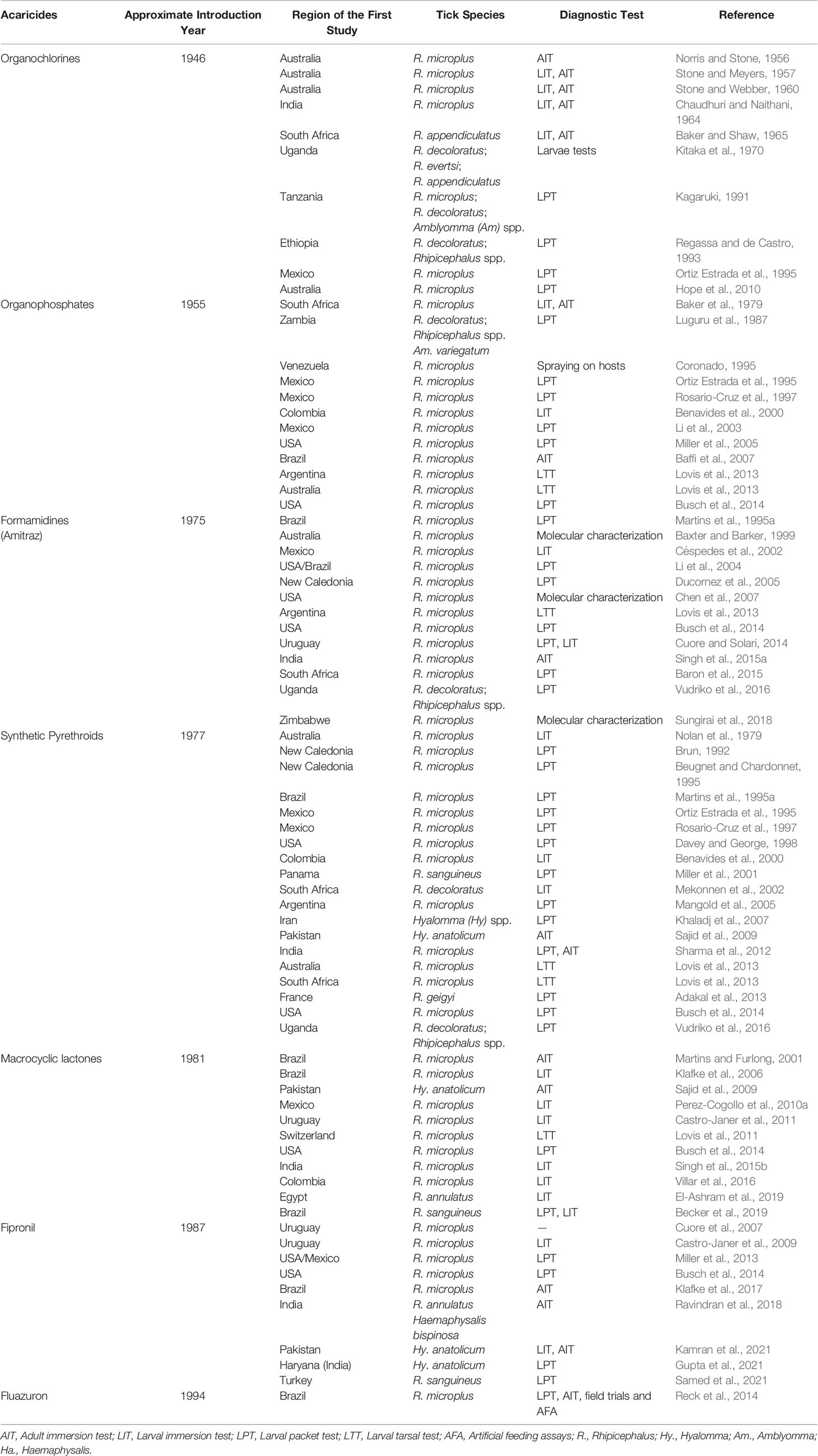
Table 1 Historical and regional aspects of different acaricides, resistant ticks and diagnostic approaches.
In recent years, a number of reviews have discussed different aspects of acaricide resistance, including identification of resistant populations, development of novel diagnostic methods, effects of resistance in tick metabolism, environment pollution, evolution and spread of resistant populations, and actions to mitigate risks related to acaricide resistance (George, 2000; George et al., 2004; Abbas et al., 2014; Benelli et al., 2017; Banumathi et al, 2017; Nwanade et al., 2020; Agwunobi et al., 2021). Genetic mutations in ticks frequently result in the selection of acaricide resistance (de Oliveira Souza Higa et al., 2015), causing conformational changes in the target site, enhanced acaricide metabolism, or reduced ability by the drug to penetrate the tick’s outer defensive layers (Guerrero et al., 2012a). A comprehensive understanding on acaricides regarding their mode of action, resistance development, and single nucleotide polymorphisms (SNPs) in the target sites is needed to mitigate acaricides resistance in different tick species. This review paper aims to summarize and critically discuss different acaricides applied for tick control, with focus on mode of action, genetic polymorphisms on target sites, diagnostic approaches, and mitigation strategies regarding acaricides resistance.
Definition and Types of Acaricide Resistance
Tick resistance against acaricides is described as “the specific heritable trait(s) in a population of ticks selected as a result of the population’s interaction with an acaricide”. The number of ticks that remain alive after exposure to the specific applied concentration of acaricides increases significantly (Rodriguez-Vivas et al., 2018). The mutated genes, inherited from the survivor ticks, are initially uncommon and rarely occur in the tick population, but increase in frequency with time. Acquired resistance is thus defined as “a resistance that results from heritable declines in drug susceptibility with the passage of time” (Chapman, 1997), which in turn results in phenotypic resistance (Esteve-Gasent et al., 2020). The term tolerance refers to the ability of a parasite to survive exposure to a specific drug dose that would usually be considered effective. Resistance across different active chemical ingredients having similar mechanisms of actions is known as cross-resistance (Li et al., 2003, Li et al., 2005a).
Three main forms of acaricide resistance are generally known (Figure 1). Metabolic resistance is achieved through acaricide detoxification by the metabolic activity of enzymes such as cytochrome P-450s (CYP), esterases, and glutathione S-transferase (GST) (Hemingway and Ranson, 2000; Guerrero et al., 2012a). Resistance developed through conformational changes in the acaricide target site in neuronal enzymes and receptors, leading to impaired interaction between the drug and the target, is termed “target site modification resistance” (Coles and Dryden, 2014). The decreased access of acaricides to the internal body environment due to modifications in the tick outer layer (exoskeleton) is called reduced penetration resistance (Schnitzerling et al., 1983; Guerrero et al., 2012a).
Historical Perspective
Figure 2 presents a general timeline showing different acaricides regarding their year of introduction and the first reports of resistance. Organochlorines (OCs) were the first commercially viable synthetic acaricides, and were introduced in 1946. Dichloro-diphenyl-trichloroethane (DDT) and benzene hexachloride (BHC) were the first OCs used as acaricides (Maunder, 1949; Whitnall et al., 1951), and resistance against both drugs was documented in R. microplus as early as 1960 in Australia (Stone and Webber, 1960). The organophosphates (OPs) were introduced approximately in 1955 (dioxathion), and resistance against this acaricide was recorded for the first time in 1987 in R. decoloratus and Amblyomma variegatum from Zambia (Luguru et al., 1987). Formamidines were introduced in the early 1960s (Hollingworth, 1976), while amitraz was registered for the first time in 1975, just 2 decades before the first amitraz-resistant R. microplus ticks were reported in Brazil (Martins et al., 1995a). Amitraz-resistant R. microplus ticks were confirmed in 2001 in Mexico (Céspedes et al., 2002). Synthetic pyrethroids (SPs) were introduced in 1977, while resistance against SPs was observed for the first time just 2 years later, in R. microplus from Australia (Nolan et al., 1979). Macrocyclic lactones (MLs), in turn, were introduced in 1981, and resistance against MLs was recorded for the first time in 2001 in R. microplus from Brazil (Martins and Furlong, 2001). Fipronil was introduced in 1987, and resistant R. microplus ticks were reported for the first time in 2007 in Uruguay (Cuore et al., 2007). The growth regulator fluazuron was introduced in 1994 (Junquera et al., 2019), and similar to other acaricides, first reports of resistant ticks arised 20 years later, in 2014, in R. microplus from Brazil which were already resistant to all six types of available acaricides (Reck et al., 2014).
Diagnostic Tests
Monitoring acaricide resistance in the field tick population is necessary for optimal and strategic use, slowing resistance selection, and surveillance of different acaricide resistant ticks. Diagnosis of resistance against different acaricides is essential and urgently required through efficient and inexpensive tests which should be easily followed (Sabatini et al., 2001).
The larval packet test (LPT), developed in 1962, uses acaricide-treated filter paper packets to incubate the tick larvae (Stone and Haydock, 1962). This test has been used to survey and diagnose resistance against OPs and SPs (Guerrero et al., 2014). The LPT takes 5–6 weeks to be completed, thus it is restricted by the lead time until results are available. The larval immersion test (LIT) was developed in 1966 (Shaw, 1966). In this assay, larvae are immersed in the test acaricide solution prepared at the applicable concentrations, to check the mortality rate and to obtain resistant ticks. This test is usually employed to characterize resistance against amitraz and MLs (Rodriguez-Vivas et al., 2006b; Perez-Cogollo et al., 2010a). In adult immersion test (AIT), which has been developed in 1973 (Drummond et al., 1973) and is currently applied in different studies, engorged female ticks are immersed in acaricide solutions prepared according to the tested concentrations (Whitnall et al., 1951). Despite broadly used for different acaricides, the AIT is not suitable to test for resistance against amitraz, which is one of the most commonly used acaricides in Mexico (Jonsson and Hope, 2007). The larval tarsal test (LTT) was established for the first time in Switzerland in 2011. It is a highly sensitive and time-efficient in vitro test, and has been used to determine resistance levels in R. microplus, as well as other ixodid ticks (Lovis et al., 2011).
Organochlorines
Organochlorines include ethane-derived chlorates such as DDT, cyclodienes (chlordane, aldrin, dieldrin, endrin, heptachlor, and toxaphene), and compounds related to hexa-chlorocyclohexane such as lindane (Jayaraj et al., 2016). Organochlorines were demonstrated to cause mortality, suppression of fertility as well as inhibition of egg hatching in R. annulatus and Haemaphysalis bispinosa engorged female ticks (Ravindran et al., 2018). Resistance against toxaphene in R. decoloratus, R. appendiculatus, and R. evertsi has been documented (Kitaka et al., 1970). Organochlorines have a wide range of activity against arthropods (ticks and insects), but have harmful impacts as may be found in the environment, milk, meat as well as in the fat of animal hosts after their application (Beugnet and Franc, 2012). This lipophilic property was the main drawback of OCs, for which its use against ticks was banned (Graf et al., 2004).
Organochlorines Mechanism of Action and Resistance
The target site for DDT is the voltage-gated sodium channels of the axon, and resistance is caused by mutations in the amino acid at the binding site (Ware and Whitacre, 2004). The targets for dieldrin are GABA-gated chloride channel receptors (Hope et al., 2010). The drug modifies the channel properties, causing hyperexcitation by inhibiting the entry of chloride ions into the nerve, ultimately leading to the death of ticks (Ozoe et al., 2010). Cyclodienes cause mutations (single nucleotide polymorphisms, or SNPs within the GABA-gated chloride channel gene that result in the selection of tick resistance (Ffrench-Constant et al., 2000). Nucleotide mutations (non-synonymous and synonymous) in R. microplus genes targeted by or associated with specific acaricides have been identified. Mutations related to OCs use in R. microplus were different from the observed for certain insects, however they were located in the same second transmembrane protein domain of the GABA-gated channel (TM-2). The effect of this mutation was unknown, but the modified TM-2 may alter the channel properties, inhibiting the entry of chloride ions into the nerve (Corley et al., 2012), and resulting in the insensitivity of the dieldrin’s target site (Ffrench-Constant et al., 2000). Polymorphisms had also been identified in the voltage-gated sodium channels of nerve axons, a target of DDT, which causes the channel’s closure after depolarization and results in a consecutive leakage of sodium ions across the membrane of the neuron (Holan, 1969) (Table 2).
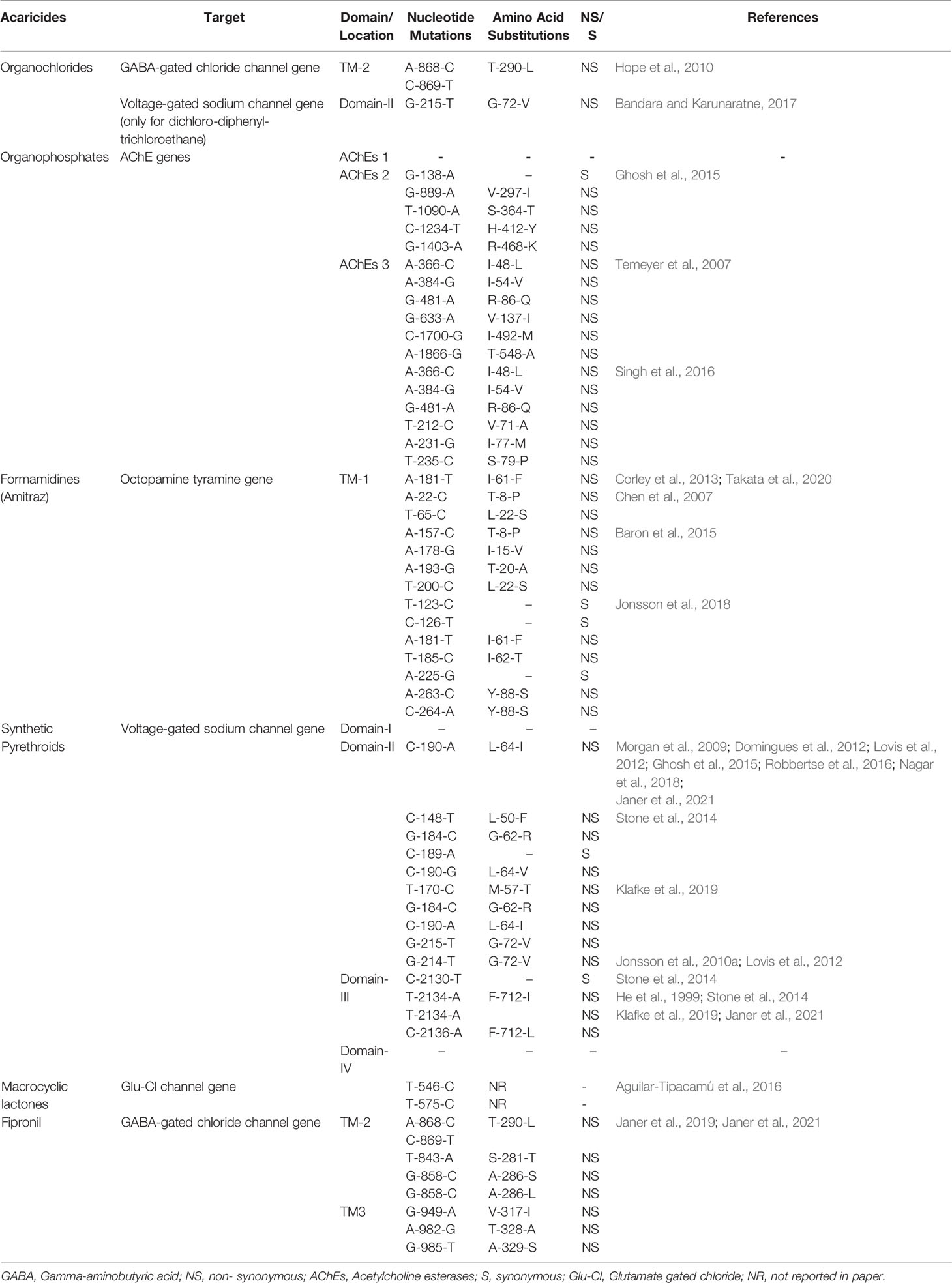
Table 2 Nucleotide and amino acid substitutions in acaricide target genes in Rhipicephalus microplus ticks.
Organophosphates
Organophosphates acaricides were introduced in the market mainly for the control of OC-resistant Rhipicephalus ticks, which were alarmingly increasing in numbers (Shaw, 1970). This class of acaricides include coumaphos, chlorpyrifos, chlorfenvinphos, diazinon, dioxathion, and ethion (Abbas et al., 2014; Adeyinka et al., 2018). From 1950s to the early 1970s, the OPs were used for tick control in Australia. They were used in large amounts in the national tick eradication program in Mexico between 1974 and 1984 (Trapaga, 1989).
Rhipicephalus microplus resistance against different OPs (coumaphos, chlorpyrifos, and chlorfenvinphos) have been recorded (Rosario-Cruz et al., 2009), with the first observations coming from South Africa in 1979 (Baker et al., 1979), then Mexico in 1980 (Ortiz Estrada et al., 1995). Organophosphates resistance in R. microplus is attributed to increased esterase enzyme activity (Rosario-Cruz et al., 2005), and different microarray techniques have been used to detect resistance against OPs, including coumaphos, in R. microplus (Saldivar et al., 2008). Resistant homozygous R. microplus (strain Tuxpan) were experimentally obtained after the application of OP over seven successive generations (Foil et al., 2004).
Organophosphates do not accumulate in fatty tissues of animals like OCs, however, OPs are more toxic to humans than OCs (Chattopadhyay et al., 2004). The use of OPs poses health risks especially to agricultural and manufacturing workers, and children. Organophosphates target the central nervous system (CNS) and cause restlessness, headache, drowsiness, confusion, slurred speech, emotional lability, psychosis, ataxia (Kwong, 2002). Cardiovascular, respiratory, gastrointestinal, sensory, CNS, and motor systems are the most affected by OPs, with life-threatening outcomes (Collombet, 2011).
Organophosphates Mechanism of Action and Resistance
A key enzyme (AChEs), necessary for nervous system functions in R. microplus, is the target site for OPs (Figure 3), as well as carbamates (carbaryl and promacyl) (Li et al., 2003; Temeyer et al., 2013). In the presence of a cholinesterase inhibitor, the enzyme would not be available to break down acetylcholine, resulting in a continuous neuron firing, which in turn causes overstimulation of the nervous system and lead to death (Temeyer et al., 2007).
Mechanisms of resistance to OPs include the modifications of the target site in the AChEs gene, carboxylesterase (CE) gene, and metabolic detoxification (Kumar, 2019). Organophosphates may interact with esterases found in the integument layers of R. microplus, resulting in the overexpression of esterases in larvae as well as in adult R. microplus, and consequent resistance to OPs (Villarino et al., 2001). Even though tick resistance to OPs is known to occur mostly through changes in metabolic activities and conformational changes in AChEs (Pruett, 2002; de Oliveira Souza Higa et al., 2015). Post-translational modifications in AChEs proteins have also been suggested as resistance mechanisms (Baxter and Barker, 1998).
The SNPs associated with OPs could be detected in three genes potentially coding for AChEs in R. microplus ticks: BmAChE-1 (Baxter and Barker, 1998), BmAChE-2 (Baxter and Barker, 2002), and BmAChE-3 (Temeyer et al., 2009). Searches for mutations in BmAChE-1 and BmAChE-2 associated with OP-resistance could not reveal a clear link to any of the identified amino acid substitutions. All six detected mutations were found in combination with each other except R-86-Q (Temeyer et al., 2007). These mutations are responsible for the insensitivity of R. microplus ticks to OPs (Table 2).
Formamidines
Formamidines were introduced as alternatives to OPs after their failure. This class of acaricides includes chlordimeform, clenpyrin, chloromethiuron, amitraz, and cymiazole (Hollingworth, 1976). Chlordimeform was used in cattle’s vats to restore the effectiveness of OPs against OP-resistant R. microplus in Australia. Formamidine acaricides have been suggested to have a harmful impact on tick CNS, by targeting octopamine tyramine receptors (Dudai et al., 1987), and also inhibit the activity of monoamine oxidase enzymes in R. microplus (Schuntner and Thompson, 1977). The effectiveness of amitraz as an acaricide has been proven for several tick species including R. microplus and R. annulatus (Davey et al., 1984). The control efficacy of amitraz was more than 97%, and resulted in weight loss as well as reduced egg production in female ticks. Amitraz was used for rapid removal (within hours) of larvae, nymphs as well as adult ticks from the host (Davey et al., 1984).
In insects, octopamine is present in peripheral and central nervous systems, being involved in proprioception, vision and complex behaviors like flying, walking, and learning (Blenau and Baumann, 2001). In ticks, the putative octopamine receptor has been identified (Chen et al., 2007), and classified as OCT/Tyr receptor in phylogenetic studies (Corley et al., 2013; Baron et al., 2015). The examined gene was initially described as an octopamine-like G-protein-coupled receptor (GPCR) (Baxter and Barker, 1999; Baron et al., 2015; Robbertse et al., 2016). The GPCRs are known to have a wide range of important roles in eukaryotes, including physiology, reproduction, growth and development, stress responses, feeding, and behavior. They have seven-transmembrane proteins which relay signals in the extracellular environment to the intracellular second messenger system by binding to heterotrimeric G-proteins, adenylate cyclase, cAMPs, and protein kinases (Liu et al., 2021). Thus, these receptors transfer signals to second messenger pathways upon interaction with octopamine (Blenau and Baumann, 2001). Among all acaricides used for tick control in Uganda, 37% of the formulations were composed of amitraz (Vudriko et al., 2016), while this acaricide was the control method of choice in 27% of the surveyed farms in Zambia (Muyobela et al., 2015). Amitraz was effective in controlling ticks, however, resistance against amitraz was detected in R. microplus and other Rhipicephalus species in Australia, South America, and South Africa (Li et al., 2004, Li et al., 2005a; Jonsson and Hope, 2007; Vudriko et al., 2016). The selection of tick resistance against amitraz is a serious problem that attracted urgent attention. It has been observed that the chances of resistance selection become greater due to the excessive use of amitraz against R. microplus (Chen et al., 2007), and Hyalomma anatolicum (Jyoti et al., 2019). Research on the ovicidal activity of amitraz against R. sanguineus eggs has shown that one-day old eggs are more sensitive to amitraz than 10-12-day old eggs (Davey et al., 1989).
Drowsiness in animals, dry skin, and a harsh hair coat are frequent side effects of amitraz applications. It can cause toxicity in both animals and humans when swallowed, inhaled, or applied to the animal’s skin in high doses (Aydin et al., 1997; Phetudomsinsuk et al., 2014). It is carcinogenic in nature and was removed from the market in 1976 (Ware and Whitacre, 2004).
Formamidines Mechanism of Action and Resistance
Octopamine receptors are found in acari, which makes it a suitable specific target site for acaricides (Figure 3), but no octopamine receptors have been described in mammalian cell membrane (Evans and Gee, 1980; Chen et al., 2007). The mechanism of action of amitraz is based on its toxicity-inducing interaction with octopamine receptors. Resistance in R. microplus might occur via conformational changes caused by mutations in the gene encoding octopamine receptor (Chen et al., 2007). Four putative amitraz resistance mechanisms have been described, including (1) insensitivity of octopamine tyramine receptor, (2) insensitive β-adrenergic octopamine receptors (BAOR), (3) enhanced expression of monoamine oxidase, and (4) the activity of ATP binding transporters (ABC-transporters) (Jonsson et al., 2018). The ABC-transporters act by pumping toxins out of the cell, and serving as a defense mechanism against acaricides in R. microplus (Pohl et al., 2011).
Amitraz resistance has been described as a complicated multigenic trait, with recessive resistance alleles (Li et al., 2004, Li et al., 2005a). Nucleotide mutations were identified in the octopamine tyramine receptor gene among resistant R. microplus ticks. All the polymorphisms in GPCR target sites were connected with amitraz resistance in R. microplus, and resulted in the insensitivity of the target (Baron et al., 2015) (Table 2).
Synthetic Pyrethroids
Synthetic pyrethroids are chlorinated or brominated halogenated esters of a chrysanthemic acid isomer and synthetic alcohol molecule. Synthetic pyrethroids were introduced in the late 1970s, after the identification of resistance against formamidines in R. microplus. Permethrin was registered and authorized for use by the US Environmental Protection Agency (US Environmental Protection Agency [USEPA], 2006). Synthetic pyrethroids comprised about 17% of the total acaricides used at the beginning of the 21st century (Davies et al., 2007). Different SPs can be categorized as type-I and type-II, according to the presence or absence of a cyano group in the alcohol portion (Soderlund and Bloomquist, 1989). Cypermethrin, flumethrin, cyhalothrin, and cyfluthrin are other common SPs used to control ticks (Bonnefoy et al., 2008). Permethrins were the first SPs used as acaricides, followed by cypermethrin and deltamethrin (Davies et al., 2007).
The CYP enzyme family controls the metabolism and detoxification of endogenous and exogenous cell damaging chemicals such as plant toxins, drugs, and pesticides in many arthropods (Kasai, 2004). A study regarding gene expression of CYP and CE related to detoxification of SPs in acaricide-resistant R. microplus showed higher levels of CYP expression in pyrethroid-resistant ticks as compared to susceptible ticks (Cossío-Bayúgar et al., 2018). Resistance against pyrethroid and propetamphos was observed in R. bursa in Iran, and found to be related to the metabolic activities of esterases, CYP, and GST enzymes (Enayati et al., 2010).
Commercially available SPs like deltamethrin and cypermethrin did not strongly induce resistance in Hy. anatolicum and R. microplus in India (Sharma et al., 2018). Reduction in egg masses of R. microplus upon the use of different concentrations of deltamethrin and cypermethrin was observed (Godara et al., 2019). A low to moderate level of resistance to λ-cyhalothrin and cypermethrin, respectively, in R. annulatus from Iran (Ziapour et al., 2017). Further, R. sanguineus eggs were more sensitive to cis-cypermethrin and cypermethrin than to coumaphos (Bicalho et al., 2001), although when nymphs and unfed females were treated with coumaphos, cis-cypermethrin, deltamethrin, amitraz, or cypermethrin in a comparative study, they showed sensitivity to cis-cypermethrin and cypermethrin only. Ovicidal activity of cis-cypermethrin, deltamethrin, and cypermethrin was assessed, and results showed that one day old eggs were more sensitive to these acaricides than 10-12 days old eggs. Additionally, different life stages (eggs, larvae, nymph, and adult) of R. sanguineus were also evaluated, with larval stages showing almost 100% mortality (Davey et al., 1989).
Synthetic pyrethroids are poorly soluble in water and highly lipid soluble. They have limited toxic effects against terrestrial animals because of the short life-span in the environment (Schleier III and Peterson, 2011). SPs have been shown to be carcinogenic when animals were exposed to them on a daily basis (US Environmental Protection Agency [USEPA], 2006).
Synthetic Pyrethroids Mechanism of Action and Resistance
Voltage-gated sodium channels are integral transmembrane proteins, responsible for electrical propagation in most excitable cells. Sodium ions enter the cell by keeping the voltage-gated sodium channels open (activated) and cause membrane depolarization. The closure of voltage-gated sodium channels for just a few milliseconds after channel activation (fast inactivation) is responsible for the action potential’s falling phase (Dong, 2007). The mechanism of action of different SPs have been suggested to involve adhesion to the target site (voltage-gated sodium channels), causing these channels to remain open for a longer period, and resulting in a higher influx of sodium ions into the cell, with aperiodic neuronal discharge (Lees and Bowman, 2007). The voltage-gated sodium channel (containing four domains (I–IV) each having six segments) is a known SP target, where point mutations may be the cause of resistance in many tick species (He et al., 1999; Dong, 2007).
Type-I SPs cause the repetitive release of sodium ions in response to even a single stimulus, while SPs type-II cause membrane depolarization by interfering with membrane action potential. Type-II pyrethroids also delay the inactivation of voltage-gated sodium channels for longer periods compared with type-I SPs (Shafer et al., 2005).
Synthetic pyrethroids resistance is linked to mutations in voltage-gated sodium channel genes of R. microplus, with polymorphisms being identified in two of the four domains (domain-II and domain-III) as shown in Table 2 (Morgan et al., 2009) (Table 2).
Macrocyclic Lactones
Due to the unusual ability to eliminate both external and internal parasites, macrocyclic lactones are referred to as “endectocide” (Shoop et al., 1995). Avermectin, doramectin, selamectin, abamectin, ivermectin, eprinomectin, milbemycins, moxidectin, milbemycin oxime, and spinosyns are the MLs most commonly used in cattle (Rodriguez-Vivas et al., 2018). Different MLs have been used for the control of internal (gastrointestinal nematodes and microfilariae) and external (ticks, mites, and mange) parasites (Vercruysse and Rew, 2002). The use of a generic version of MLs, or excessive use of MLs in tropical and subtropical countries have influenced the selection of resistance in R. microplus (Perez-Cogollo et al., 2010a).
Environmental, biological, and management factors influence the selection of resistance against these acaricides in R. microplus populations (Perez-Cogollo et al., 2010b). The rise in potential resistance against ivermectin in R. microplus was observed after 10 generations in four different settings: larvae treated with ivermectin, larvae obtained from ivermectin-treated engorged female ticks, larvae obtained from ivermectin-treated engorged female ticks on an ivermectin-treated host, and larvae obtained from ivermectin-treated engorged female ticks that had high oviposition potential (Klafke et al., 2010). In R. microplus ticks collected from red deers, resistance against ivermectin was recorded for the first time in Mexico (Rodríguez-Vivas et al., 2014). In R. annulatus, resistance against ivermectin was studied for the first time in Egypt (El-Ashram et al., 2019). Rhipicephalus microplus ticks resistant against ivermectin have been reported from India (Singh et al., 2015b), while ivermectin-resistant Hy. anatolicum ticks were documented from Pakistan (Sajid et al., 2009; Kamran et al., 2021). Different MLs have different lipophilic properties, and human handlers should be careful to avoid contact with these drugs. Local swelling or pruritis at the injection site in animals occurs in response to subcutaneous administration of MLs. Additionally, the MLs treated animals suffer weight loss, increased body temperature and muscle tremors. Macrocyclic lactones, specifically ivermectin, become harmful to aquatic life when applied in water or when ivermectin-treated animals enter lakes, ponds, or streams shortly after application (Macrocyclic Lactones Veterinary-Systemic, 2006).
Macrocyclic Lactones Mechanism of Action and Resistance
Protostome invertebrates, including ticks, express Glu-Cl channels in neurons and muscle, the target sites of MLs (Rodríguez-Vivas et al., 2010) (Figure 3). The mechanism of action of ivermectin was characterized by its high binding affinity to Glu-Cl receptors. These proteins control chloride ions movement across the channels located in the muscle and nervous cells (Cully et al., 1994), and thus their impairment can cause muscular paralysis and death of ticks by inhibition of neuronal impulses (Martin et al., 2002). When chloride gated channels remain open for a long period, conductivity of the membrane becomes irreversibly higher, which causes hyperpolarization as well as paralysis of tick muscles (Rodríguez-Vivas et al., 2010). Several species express efflux transporters called ABC-transporters (a super-family of integral membrane proteins). Extracellular and intracellular membranes can pump out the xenobiotic (drug, chemical, toxins) and endogenous metabolites through these protein channels, which reduces the concentrations of harmful chemicals in the cell. In R. microplus, these efflux pumps work as a defense mechanism against ivermectin (Pohl et al., 2011; El-Ashram et al., 2019), while esterases can also have a role in the detoxification of ivermectin (El-Ashram et al., 2019).
Macrocyclic lactones have been shown to be more effective against single host ticks than multi-host ticks (McKellar and Benchaoui, 1996). Different studies (Martins and Furlong, 2001; Klafke et al., 2006; Castro-Janer et al., 2011) reported that R. microplus ticks are resistant to MLs, and mutations were observed in the Glu-Cl gene of R. microplus ticks (Table 2) (Aguilar-Tipacamú et al., 2016) (Table 2).
Fipronil
Fipronil is a member of the phenylpyrazolic family of insecticides and has been used to control R. microplus infestations in cattle (Cole et al., 1993). While the use of fipronil was introduced in the late 1990s, resistance was only recently detected after the development of stable diagnostic tests (Cuore et al., 2007; Castro-Janer et al., 2009). It was persistently used in many countries and has been shown to be highly effective against ticks (Castro-Janer et al., 2009).
Fipronil was not widely utilized at first because of its higher costs relative to other chemicals. Its usage and demand increased in the early 2000s due to a decrease in costs and an increase in R. microplus tick populations resistant to other acaricides (Reck et al., 2014). Rhipicephalus microplus ticks collected in Mexico have developed resistance against fipronil, however, the extensive use of permethrin in the 1980s may have contributed to the selection of this resistance (Miller et al., 2013). Rhipicephalus microplus ticks resistant against fipronil have been reported from different regions such as Uruguay (Castro-Janer et al., 2009; Castro-Janer et al., 2010; Cuore and Solari, 2014), Switzerland (Lovis et al., 2011), and Brazil (Klafke et al., 2017; Becker et al., 2019). Other species of the genus Rhipicephalus (R. sanguineus) were also found to be resistant to fipronil (Samed et al., 2021). Fipronil-resistant Hy. anatolicum ticks were reported from Pakistan (Kamran et al., 2021). Resistance against fipronil may have developed at lower rates in R. microplus compared with Hy. anatolicum (Gupta et al., 2021). Fipronil is effective for tick control, but it is also toxic for honeybees, birds, fish and some other beneficial invertebrates. During fipronil applications, animals including humans (workers) can be exposed through their skin, eyes, or by breathing, resulting in skin irritation, sweating, nausea, vomiting, headache, stomach pain, dizziness, weakness, and seizures (Jackson et al., 2009).
Fipronil Mechanism of Action and Resistance
Similar to OCs (e.g. lindane, dieldrin), fipronil acts by blocking GABA-receptors (Castro-Janer et al., 2009), and inhibiting the passage of chloride ions through GABA-gated chloride channels (Figure 3). It may cause mortality when used in high concentrations, while smaller doses may disrupt nervous functions (Cole et al., 1993). Fipronil is classified as a selective GABA antagonist because it binds effectively to the GABA-receptor chloride channels of ticks (Food and Agriculture Organization, 2009).
Mutations were also identified within the second transmembrane (TM-2) and third transmembrane (TM-3) domain of the GABA-gated chloride channel gene in fipronil resistant R. microplus ticks (Janer et al., 2019; Janer et al., 2021) (Table 2).
Fluazuron
The fluazuron is a benzoylphenyl urea compound that inhibits chitin formation in R. microplus. Other benzoylphenyl urea compounds such as diflubenzuron, lufenuron, and flufenoxuron are effective against a wide range of insects except the fluazuron, which is effective against tick species (Junquera et al., 2019). Because of its narrow spectrum of activity and lack of lethal impact on established tick infestations, the fluazuron utilization was low to moderate after its introduction. However, its utilization has steadily increased due to the increasing resistance of R. microplus and R. decoloratus to numerous acaricides such as synthetic pyrethroids and amitraz (Food and Agriculture Organization, 2004), ivermectin (Martins and Furlong, 2001), and fipronil (Miller et al., 2013).
Fluazuron has shown higher efficacy against ticks than against other arthropods (Junquera et al., 2019). In Brazil, the field trials of fluazuron resulted in 99% effectiveness after treatment for 21 days (Martins et al., 1995b). Only larval stages of R. microplus ticks could be observed after the host was treated with fluazuron, and no ticks were found that had reached further developmental stages. Reduction in egg viability also occurred due to fluazuron application. Fluazuron has the ability to accumulate in fat, and may be released from the body through milk, thus protecting calves from ticks without direct application (Bull et al., 1996). It has been suggested that fluazuron affected solely the hatchability of larvae from treated female ticks, and that a synergistic effect of fluazuron with MLs negatively impacted reproductive parameters in R. microplus (Cruz et al., 2014). Fluazuron affected nymphs and larvae, when they were attempting molting, and did not harm any adult ticks (Junquera et al., 2019).
Fluazuron showed the highest efficacy (100%) than any other used acaricides against R. microplus ticks, and no resistance was reported against fluazuron, although it was suggested that its inappropriate use may lead to resistance development (Raynal et al., 2013). In a later study, resistance against fluazuron was recorded in R. microplus ticks (Reck et al., 2014).
Fluazuron Mechanism of Action and Resistance
Fluazuron inhibits tick growth (Bull et al., 1996) by interfering with the formation of chitin through inhibition of enzymes involved in the molting process, and results in the hardening of the tick exoskeleton. Fluazuron treatment causes damage to several chitinous structures (smaller hypostome and chelicerae, scutum, sensilla, pores, anal plaque) in R. sanguineus nymphs, structures that play essential roles in tick survival (Calligaris et al., 2013).
Although the use of fluazuron was necessary for tick control and for cattle management, the careless use of this drug may weaken the immune system of the host, which in turn carries huge risks for diseases (Ribeiro et al., 2019). Although SNPs associated with fluazuron have not been reported, further research is essential to characterize its target site.
Cross and Multi-Resistance against Different Acaricides
Cross-resistance between dieldrin (an OC) and fipronil has been reported, which affects the GABA-gated chloride channels of many insects (Le Corronc et al., 2002). The selection of cross-resistance against OCs led to a reduction in the use of these acaricides against different tick populations including R. microplus, R. decoloratus, and R. appendiculatus (Stone and Meyers, 1957; Baker and Shaw, 1965; George et al., 2004). It has been reported that there was a significant pattern of cross-resistance in many R. microplus populations against OCs (DDT) and SPs (cypermethrin) (Food and Agriculture Organization, 2004). Cross-resistance between two OPs (diazinon and coumaphos) and carbamate acaricide (carbaryl) has also been observed in R. microplus ticks (Li et al., 2005b). Cross-resistance between fipronil and lindane was examined in R. microplus ticks in Brazil and Uruguay, because both acaricides have the same target (Castro-Janer et al., 2010). Rhipicephalus microplus populations from Mexico were studied with multiple resistance to OCs, OPs and other acaricides (Foil et al., 2004; Alonso-Díaz et al., 2006).
Mitigation of Acaricide Resistance and Alternative Control Methods
Strategies must be developed to minimize the selection of acaricide resistance in ticks. Different acaricides have different modes of action, thus alternate use may help in the reduction of resistance selection. Rotation of acaricides such as pyrethroids with coumaphos (OPs) has been used, and rotation of amitraz with other groups of acaricides may delay a further spread of amitraz resistance (Thullner et al., 2007). The alternate use of acaricides, especially amitraz with spinosad, may help in the minimization of resistance selection (Jonsson et al., 2010b). It has been recommended to perform further trials of experiments to finalize the conclusions about beneficial aspects of acaricide rotation in the field (Adakal et al., 2013).
Synergistic use of acaricide combinations may reduce the chances of resistance selection (Thullner et al., 2007), however, due to the lack of appropriate field work, this assumption remains to be confirmed. Nevertheless, the combination of fipronil with permethrin was recommended after showing effectiveness against Dermacentor reticulatus (Dumont et al., 2015b), Ixodes ricinus and R. sanguineus ticks (Dumont et al., 2015a). The combination of OPs (chlorfenvinphos and ethion) with SPs (deltamethrin and cypermethrin) has been successfully applied for the control of R. microplus (George et al., 2004). The combination of OPs (e.g. dichlorvos) with tetrachlorvinphos was successfully used against OP-resistant R. microplus (Davey et al., 2013). The combination of different acaricides such as flumethrin with cyfluthrin, chlorpyriphos with permethrin, and cypermethrin with cymiazole were available in the market, and were used for tick control in Mexico (Rodriguez-Vivas et al., 2006a). Satisfactory results (high larvae mortality rate) were observed from the combined effects of permethrin and amitraz, while there was zero larvae mortality when permethrin was used alone (Fernández-Salas et al., 2012). The application of combined acaricides may help in delaying the resistance selection, because individual ticks are very unlikely to have resistant alleles of two or more genes for different acaricides with different modes of action (Lovis et al., 2013). Deltamethrin, thymol, and essential oil derived from Eucalyptus have shown synergistic acaricidal efficacy against R. microplus (Arafa et al., 2021).
Besides resistance development, excessive use of acaricides has negative impacts on the environment, animal health, and animal-derived products. For these reasons, environmentally friendly alternative control strategies are needed, which would also be important for the mitigation of acaricide resistance. Numerous plant-derived products have been used to aid tick control. Rhipicephalus microplus ticks were killed at a nearly 100% rate by applying the essential oils of Eucalyptus citriodora and Eu. staigeriana at 10% concentration, while Eu. globulus showed 100% efficacy at 20% concentration (Chagas et al., 2002). In another study, Eucalyptus spp. leaf extracts showed 96% effectiveness against female R. microplus ticks (Costa et al., 2008). Larval mortality of 100% and 98%, and reproductive efficiency inhibition of 100% and 91.5%, were observed in R. microplus treated with Melia azedarach chloroformic and hexanic extracts, respectively (Borges et al., 2003). The acaricidal activity of environment friendly essential oils from different plant species has been tested against different ticks. Essential oils obtained from Origanum bilgeri and Cunila species have shown acaricidal activity against Rhipicephalus ticks (Apel et al., 2009; Koc et al., 2013). Thymol, a major component of essential oils, has shown 100% acaricidal efficacy against R. microplus and R. sanguineus ticks (Daemon et al., 2009). Essential oils extracted from Allium sativum were 90-100% effective in killing R. microplus larvae (Martinez-Velázquez et al., 2011). Essential oils extracted from Tagetes minuta have shown 95% efficacy against larvae of Amblyomma cajennense and Argas miniatus (Garcia et al., 2012). The application of essential oils extracted from Azadirachta indica seeds affected egg hatching and caused 100% larval mortality in Hy. anatolicum ticks (Abdel-Shafy and Zayed, 2002). Oils extracted from leaves of Hesperozygis ringens caused 95% inhibition of larval hatchability and 100% larval mortality in R. microplus (Ribeiro et al., 2010). Two monoterpenes (carvacrol and thymol) were used alone or in combination with cypermethrin to control R. microplus. It was observed that thymol enhances cypermethrin toxicity (Tavares et al., 2022b), and that both monoterpenes increased the activity of antioxidant and detoxifying enzymes (glutathione-S-transferase, catalase, superoxide dismutase, and glutathione peroxidase) in the R. microplus ticks (Tavares et al., 2022a). The combination of essential oils from Cinnamomum zeylanicum, Cuminum cyminum, and Pimenta dioica in emulsion preparations showed a synergic effect against R. microplus (Lazcano Díaz et al., 2019). Furthermore, the combination of essential oils extracted from Laurus nobilis and Copaifera officinalis showed higher efficacy than the isolated oils against R. microplus, indicating that the synergic action could increase the effect of environment-friendly compounds (Vinturelle et al., 2021). Some of the mechanisms of acaricidal activity by plant products include inhibition of egg development, suppression of chitin formation, inhibition of growth regulator hormones, and disruption of the mating communications. These products have different modes of action, thereby slowing down the selection of resistant ticks (De Souza Chagas et al., 2012; Pavela et al., 2016). Factors such as identification of suitable phyto-metabolites, extraction time of phytochemicals, the concentration of extracts, odors of plant-derived extracts, extracted plant age, specie of ticks, and exposure time of ticks may have undermined the research on phytotherapeutic alternatives. During many years, various studies were focused on the identification of natural resources, but recent research has focused on chemical standardization, quality of phytochemicals for tick control, and other issues like long-term stability, storage, and transportation of different plant extracts, which are essential for the commercial availability of these products (Benelli and Pavela, 2018; Selles et al., 2021).
There are reports of 58 species of fungi found infecting 73 different acari, and several fungi species have shown potential anti-tick efficacy. Beauveria bassiana, Metarhizium anisopliae, Isaria farinosa, Isaria famosorosea, and Lecanicillium lecanii have been shown to infect various ixodid ticks (Chandler et al., 2000; Jackson and Jaronski, 2009). Environmental factors that affect the persistence and virulence of fungi against ticks include high or low environmental temperature, low humidity, UV radiation, precipitation, soil type, composition and pH (in case of direct application on soil) (Jackson et al., 2009; Jaronski, 2010; Wu et al., 2020). The stability of some fungal products is very limited, which is why its large-scale production can be expensive. Most manufacturers would recommend exactly the same application method for fungal products as used for other chemical pesticides (Shelton and Roush, 2000).
To minimize the need for acaricides and delay resistance selection, the strategic use of anti-tick vaccines has been strongly and preferably recommended as suitable alternatives for tick control (Guerrero et al., 2012b). The first commercial anti-tick vaccine was developed by expressing gut glycoproteins (Bm86) of diversely distributed R. microplus (Willadsen and Kemp, 1988). The effectiveness of this anti-tick vaccine varies in different regions against different ticks, therefore the identification of novel antigens as vaccine candidates are underway (Freeman et al., 2010). Since Bm86, numerous novel antigens from several tick species have been tested for potential anti-tick vaccine development, however, none has been approved for the market so far (Parizi et al., 2012; de la Fuente et al., 2017; Rego et al., 2019; Ali et al., 2022).
The development of alternative approaches to mitigate tick resistance against available acaricides, such as the implementation of non-chemical control methods, application of biosafety protocols for the prevention of resistant ticks, development of novel environment-friendly acaricides, suitable diagnostic methods, and anti-tick vaccines, are in continuous and pressing demand.
Conclusion
All of the available acaricides have faced failure due to the selection of tick resistance. The repeated use of a single acaricide is often responsible for the selection of fast-developing acaricide resistance. Regular surveillance of ticks for resistance against different acaricides may play a vital role in the minimization of resistance selection. Livestock owners and workers must be trained on tick control and resistance mitigation measures. Environmentally friendly, stable, cheaper, easily accessible, and novel acaricides, as well as anti-tick vaccines should be developed to overcome tick resistance against acaricides.
Author Contributions
MKO, NI, AAlo, AZK and AA searched and collected the literature. ISV, TT, AA supervised the overall investigations. All authors equally contributed to the formulation of the whole manuscript.
Funding
We acknowledge the financial support provided by Pakistan Science Foundation and Higher Education Commission Islamabad Pakistan, researchers supporting project number (RSP2022R494), and the CNPq and CAPES – Brazil for providing research facilities. This work was supported by JSPS KAKENHI Grant Number JP22H02522.
Conflict of Interest
The authors declare that the research was conducted in the absence of any commercial or financial relationships that could be construed as a potential conflict of interest.
Publisher’s Note
All claims expressed in this article are solely those of the authors and do not necessarily represent those of their affiliated organizations, or those of the publisher, the editors and the reviewers. Any product that may be evaluated in this article, or claim that may be made by its manufacturer, is not guaranteed or endorsed by the publisher.
References
Abbas, R. Z., Zaman, M. A., Colwell, D. D., Gilleard, J., Iqbal, Z. (2014). Acaricide Resistance in Cattle Ticks and Approaches to Its Management: The State of Play. Vet. Parasitol. 203, 6–20. doi: 10.1016/j.vetpar.2014.03.006
Abdel-Shafy, S., Zayed, A. A. (2002). In Vitro Acaricidal Effect of Plant Extract of Neem Seed Oil (Azadirachta Indica) on Egg, Immature, and Adults Stages of Hyalomma Anatolicum Excavatum (Ixodoidea: Ixodidae). Vet. Parasitol. 106, 89–96. doi: 10.1016/s0304-4017(02)00023-7
Adakal, H., Stachurski, F., Chevillon, C. (2013). Tick Control Practices in Burkina Faso and Acaricide Resistance Survey in Rhipicephalus (Boophilus) Geigyi (Acari: Ixodidae). Exp. Appl. Acarol. 59, 483–491. doi: 10.1007/s10493-013-9706-6
Adeyinka, A., Muco, E., Pierre, L. (2018). Organophosphates (Treasure Island (FL: StatPearls Publishing).
Aguilar-Tipacamú, G., Mosqueda-Gualito, J., Cantó-Alarcón, G. J., Klafke, G. M., Arellano-Carvajal, F., Alonso-Díaz, M. M., et al. (2016). Identification of Mutations in the Glutamate-Dependent Chlorine Channel in Rhipicephalus Microplus Resistant and Susceptible to Ivermectins. Sci. Work. Chiapas. 11, 20–26.
Aguilar-Tipacamu, G., Rosario-Cruz, R., Miller, R. J., Guerrero, F. D., Rodriguez-Vivas, R. I., Garcia-Vazquez, Z. (2011). Phenotype Changes Inherited by Crossing Pyrethroid Susceptible and Resistant Genotypes From the Cattle Tick Riphicephalus (Boophilus) Microplus. Exp. Appl. Acarol. 54, 301–311. doi: 10.1007/s10493-011-9441-9
Agwunobi, D. O., Yu, Z., Liu, J. (2021). A Retrospective Review on Ixodid Tick Resistance Against Synthetic Acaricides: Implications and Perspectives for Future Resistance Prevention and Mitigation. Pestic. Biochem. Physiol. 173, 104776. doi: 10.1016/j.pestbp.2021.104776
Ali, A., Khan, M. A., Zahid, H., Yaseen, P. M., Qayash Khan, M., Nawab, J., et al. (2019). Seasonal Dynamics, Record of Ticks Infesting Humans, Wild and Domestic Animals and Molecular Phylogeny of Rhipicephalus Microplus in Khyber Pakhtunkhwa Pakistan. Front. Physiol. 10. doi: 10.3389/fphys.2019.00793
Ali, A., Zeb, I., Alouffi, A., Zahid, H., Almutairi, M. M., Ayed Alshammari, F., et al. (2022). Host Immune Responses to Salivary Components-A Critical Facet of Tick-Host Interactions. Front. Cell Infect. Microbiol. 171. doi: 10.3389/fcimb.2022.809052
Alonso-Díaz, M. A., Rodríguez-Vivas, R. I., Fragoso-Sánchez, H., Rosario-Cruz, R. (2006). Resistencia De La Garrapata Boophilus Microplus a Los Ixodicidas. Arch. Med. Vet. 38, 105–113. doi: 10.4067/S0301-732X2006000200003
Angus, B. M. (1996). The History of the Cattle Tick Boophilus Microplus in Australia and Achievements in Its Control. Int. J. Parasitol. 26, 1341–1355. doi: 10.1016/S0020-7519(96)00112-9
Apel, M. A., Sardá-Ribeiro, V. L., Bordignon, S. A. L., Henriques, A. T., von Poser, G. (2009). Chemical Composition and Toxicity of the Essential Oils From Cunila Species (Lamiaceae) on the Cattle Tick Rhipicephalus (Boophilus) Microplus. Parasitol. Res. 105, 863–868. doi: 10.1007/s00436-009-1455-4
Arafa, W. M., Aboelhadid, S. M., Moawad, A., Shokeir, K. M., Ahmed, O., de León, A. A. P. (2021). Control of Rhipicephalus Annulatus Resistant to Deltamethrin by Spraying Infested Cattle With Synergistic Eucalyptus Essential Oil-Thymol-Deltamethrin Combination. Vet. Parasitol. 290, 109346. doi: 10.1016/j.vetpar.2021.109346
Aydin, K., Kurtoglu, S., Poyrazoglu, M. H., Üzüm, K., Üstünbas, H. B., Hallaç, I. K. (1997). Amitraz Poisoning in Children: Clinical and Laboratory Findings of Eight Cases. Hum. Exp. Toxicol. 16, 680–682. doi: 10.1016/j.toxac.2019.12.001
Baffi, M. A., de Souza, G. R. L., Vieira, C. U., de Sousa, C. S., Gourlart, L. R., Bonetti, A. M. (2007). Identification of Point Mutations in a Putative Carboxylesterase and Their Association With Acaricide Resistance in Rhipicephalus (Boophilus) Microplus (Acari: Ixodidae). Vet. Parasitol. 148, 301–309. doi: 10.1016/j.vetpar.2007.06.016
Baker, J. A. F., Janet, O., Robertson, W. D. (1979). Ixodicidal Resistance in Boophilus Microplus (Canestrini) in the Republic of South Africa and Transkei. J. S. Afr. Vet. Assoc. 50, 296–301.
Baker, J. A. F., Shaw, R. D. (1965). Toxaphene and Lindane Resistance in Rhipicephalus Appendiculatus, the Brown Ear Tick of Equatorial and Southern Africa. J. S. Afr. Vet. Assoc. 36, 321–330.
Bandara, K. J., Karunaratne, S. P. (2017). Mechanisms of Acaricide Resistance in the Cattle Tick Rhipicephalus (Boophilus) Microplus in Sri Lanka. Pestic. Biochem. Physiol. 139, 68–72. doi: 10.1016/j.pestbp.2017.05.002
Banumathi, B., Vaseeharan, B., Rajasekar, P., Prabhu, N. M., Ramasamy, P., Murugan, K., et al. (2017). Exploitation of Chemical, Herbal and Nanoformulated Acaricides to Control the Cattle Tick, Rhipicephalus (Boophilus) Microplus–A Review. Vet. Parasitol. 244, 102–110. doi: 10.1016/j.vetpar.2017.07.021
Baron, S., van der Merwe, N. A., Madder, M., Maritz-Olivier, C. (2015). SNP Analysis Infers That Recombination Is Involved in the Evolution of Amitraz Resistance in Rhipicephalus Microplus. PLos One 10, e0131341. doi: 10.1371/journal.pone.0131341
Baxter, G. D., Barker, S. C. (1998). Acetylcholinesterase cDNA of the Cattle Tick, Boophilus Microplus: Characterisation and Role in Organophosphate Resistance. Insect Biochem. Mol. Biol. 28, 581–589. doi: 10.1016/S0965-1748(98)00034-4
Baxter, G. D., Barker, S. C. (1999). Isolation of a cDNA for an Octopamine-Like, G-Protein Coupled Receptor From the Cattle Tick, Boophilus Microplus. Insect Biochem. Mol. Biol. 29, 461–467. doi: 10.1016/S0965-1748(99)00023-5
Baxter, G. D., Barker, S. C. (2002). Analysis of the Sequence and Expression of a Second Putative Acetylcholinesterase cDNA From Organophosphate-Susceptible and Organophosphate-Resistant Cattle Ticks. Insect Biochem. Mol. Biol. 32, 815–820. doi: 10.1016/S0965-1748(01)00168-0
Becker, S., Webster, A., Doyle, R. L., Martins, J. R., Reck, J., Klafke, G. M. (2019). Resistance to Deltamethrin, Fipronil and Ivermectin in the Brown Dog Tick, Rhipicephalus Sanguineus Sensu Stricto, Latreille (Acari: Ixodidae). Ticks. Tick. Borne. Dis. 10, 1046–1050. doi: 10.1016/j.ttbdis.2019.05.015
Benavides, E., Rodríguez, J. L., Romero, A. (2000). Isolation and Partial Characterization of the Montecitos Strain of Boophilus Microplus (Canestrin) Multiresistant to Different Acaricides. Ann. N. Y. Acad. Sci. 916, 668–671. doi: 10.1111/j.1749-6632.2000.tb05358.x
Benelli, G., Maggi, F., Romano, D., Stefanini, C., Vaseeharan, B., Kumar, S., et al. (2017). Nanoparticles as Effective Acaricides Against Ticks—a Review. Ticks. Tick. Borne. Dis. 8, 821–826. doi: 10.1016/j.ttbdis.2017.08.004
Benelli, G., Pavela, R. (2018). Repellence of Essential Oils and Selected Compounds Against Ticks-A Systematic Review. Acta Trop. 179, 47–54. doi: 10.1016/j.actatropica.2017.12.025
Beugnet, F., Chardonnet, L. (1995). Tick Resistance to Pyrethroids in New Caledonia. Vet. Parasitol. 56 (4), 325–338. doi: 10.1016/0304-4017(94)00686-7
Beugnet, F., Franc, M. (2012). Insecticide and Acaricide Molecules and/or Combinations to Prevent Pet Infestation by Ectoparasites. Trends Parasitol. 28, 267–279. doi: 10.1016/j.pt.2012.04.004
Bicalho, K. A., Ferreira, F., Borges, L. M. F., Ribeiro, M. F. B. (2001). In Vitro Evaluation of the Effects of Some Acaricides on Life Stages of Rhipicephalus Sanguineus (Acari: Ixodidae). Arq. Bras. Med. Vet. Zootec. 53, 548–552. doi: 10.1590/S0102-09352001000500006
Blenau, W., Baumann, A. (2001). Molecular and Pharmacological Properties of Insect Biogenic Amine Receptors: Lessons From Drosophila Melanogaster and Apis Mellifera. Arch. Insect Biochem. Physiol.: Published. Collaboration. Entomol. Soc. America 48, 13–38. doi: 10.1002/arch.1055
Bonnefoy, X., Kampen, H., Sweeney, K. (2008). Public Health Significance of Urban Pests (US: World Health Organization).
Borges, L. M. F., Ferri, P. H., Silva, W. J., Silva, W. C., Silva, J. G. (2003). In Vitro Efficacy of Extracts of Melia Azedarach Against the Tick Boophilus Microplus. Med. Vet. Entomol. 17, 228–231. doi: 10.1046/j.1365-2915.2003.00426.x
Brun, L. O. (1992). Resistance to Deltamethrin in Boophilus Microplus (Canestrini) (Acarina: Ixodidae) in New Caledonia. Aust. J. Entomol. 31, 301–302. doi: 10.1111/j.1440-6055.1992.tb00510.x
Bull, M. S., Swindale, S., Overend, D., Hess, E. A. (1996). Suppression of Boophilus Microplus Populations With Fluazuron–an Acarine Growth Regulator. Aust. Vet. J. 74, 468–470. doi: 10.1111/j.1751-0813.1996.tb07575.x
Burger, T. D., Shao, R., Barker, S. C. (2014). Phylogenetic Analysis of Mitochondrial Genome Sequences Indicates That the Cattle Tick, Rhipicephalus (Boophilus) Microplus, Contains a Cryptic Species. Mol. Phylogenet. Evol. 76, 241–253. doi: 10.1016/j.ympev.2014.03.017
Busch, J. D., Stone, N. E., Nottingham, R., Araya-Anchetta, A., Lewis, J., Hochhalter, C., et al. (2014). Widespread Movement of Invasive Cattle Fever Ticks (Rhipicephalus Microplus) in Southern Texas Leads to Shared Local Infestations on Cattle and Deer. Parasitol. Vectors 7, 1–16. doi: 10.1186/1756-3305-7-188
Calligaris, I. B., De Oliveira, P. R., Roma, G. C., Bechara, G. H., Camargo-Mathias, M. I. (2013). Action of the Insect Growth Regulator Fluazuron, the Active Ingredient of the Acaricide Acatak®, in Rhipicephalus Sanguineus Nymphs (Latreille 1806) (Acari: Ixodidae). Micros. Res. Tech. 76, 1177–1185. doi: 10.1002/jemt.22282
Castro-Janer, E., Martins, J. R., Mendes, M. C., Namindome, A., Klafke, G. M., Schumaker, T. T. S. (2010). Diagnoses of Fipronil Resistance in Brazilian Cattle Ticks (Rhipicephalus (Boophilus) Microplus) Using In Vitro Larval Bioassays. Vet. Parasitol. 173, 300–306. doi: 10.1016/j.vetpar.2010.06.036
Castro-Janer, E., Rifran, L., Gonzalez, P., Niell, C., Piaggio, J., Gil, A., et al. (2011). Determination of the Susceptibility of Rhipicephalus (Boophilus) Microplus (Acari: Ixodidae) to Ivermectin and Fipronil by Larval Immersion Test (LIT) in Uruguay. Vet. Parasitol. 178, 148–155. doi: 10.1016/j.vetpar.2010.12.035
Castro-Janer, E., Rifran, L., Piaggio, J., Gil, A., Miller, R. J., Schumaker, T. T. S. (2009). In Vitro Tests to Establish LC50 and Discriminating Concentrations for Fipronil Against Rhipicephalus (Boophilus) Microplus (Acari: Ixodidae) and Their Standardization. Vet. Parasitol. 162, 120–128. doi: 10.1016/j.vetpar.2009.02.013
Céspedes, N. S., Vargas, M. S., Sánchez, H. F., Vázquez, Z. G. (2002). First Case of Resistance to Amitraz in the Cattle Tick Boophilus Microplus in Mexico. Livestock. Technique. Mexico. 40, 81–92.
Chagas, A. C. D. S., Passos, W. M., Prates, H. T., Leite, R. C., Furlong, J., Fortes, I. C. P. (2002). Acaricide Effect of Eucalyptus Spp Essential Oils and Concentrated Emulsion on Boophilus Microplus. Braz. J. Vet. Res. Anim. Sci. 39, 247–253. doi: 10.1590/S1413-95962002000500006
Chandler, D., Davidson, G., Pell, J. K., Ball, B. V., Shaw, K., Sunderland, K. D. (2000). Fungal Biocontrol of Acari. Biocontrol Sci. Technol. 10, 357–384. doi: 10.1080/09583150050114972
Chapman, H. D. (1997). Biochemical, Genetic and Applied Aspects of Drug Resistance in Eimeria Parasites of the Fowl. Avian. Pathol. 26, 221–244. doi: 10.1080/03079459708419208
Chattopadhyay, A., Bhatnagar, N. B., Bhatnagar, R. (2004). Bacterial Insecticidal Toxins. Crit. Rev. Microbiol. 30, 33–54. doi: 10.1080/10408410490270712
Chaudhuri, R. P., Naithani, R. C. (1964). Resistance to BHC in the Cattle Tick Boophillus Microplus (Can.) in India. Bull. Entomol. Res. 55, 405–410. doi: 10.1017/S0007485300049555
Chen, A. C., He, H., Davey, R. B. (2007). Mutations in a Putative Octopamine Receptor Gene in Amitraz-Resistant Cattle Ticks. Vet. Parasitol. 148, 379–383. doi: 10.1016/j.vetpar.2007.06.026
Cole, L. M., Nicholson, R. A., Casida, J. E. (1993). Action of Phenylpyrazole Insecticides at the GABA-Gated Chloride Channel. Pestic. Biochem. Physiol. 46, 47–54. doi: 10.1006/pest.1993.1035
Coles, T. B., Dryden, M. W. (2014). Insecticide/acaricide Resistance in Fleas and Ticks Infesting Dogs and Cats. Parasi. Vectors 7, 1–10. doi: 10.1186/1756-3305-7-8
Collombet, J. M. (2011). Nerve Agent Intoxication: Recent Neuropathophysiological Findings and Subsequent Impact on Medical Management Prospects. Toxicol. Appl. Pharmacol. 255, 229–241. doi: 10.1016/j.taap.2011.07.003
Corley, S. W., Jonsson, N. N., Piper, E. K., Cutullé, C., Stear, M. J., Seddon, J. M. (2013). Mutation in the Rmβaor Gene Is Associated With Amitraz Resistance in the Cattle Tick Rhipicephalus Microplus. Proc. Natl. Acad. Sci. U.S.A. 110, 16772–16777. doi: 10.1073/pnas.1309072110
Corley, S. W., Piper, E. K., Jonsson, N. N. (2012). Generation of Full-Length cDNAs for Eight Putative GPCnR From the Cattle Tick, R. Microplus Using a Targeted Degenerate PCR and Sequencing Strategy. PLos One 7, e32480. doi: 10.1371/journal.pone.0032480
Coronado, A. (1995). Current Status of the Tropical Cattle Tick Boophilus Microplus in Venezuela. Publ. Especial., 22–29.
Cossío-Bayúgar, R., Martínez-Ibañez, F., Aguilar-Díaz, H., Miranda-Miranda, E. (2018). Pyrethroid Acaricide Resistance Is Proportional to P-450 Cytochrome Oxidase Expression in the Cattle Tick Rhipicephalus Microplus. BioMed. Res. Int. 2018, 1–6. doi: 10.1155/2018/8292465
Costa, F. B., Vasconcelos, P. S. D. S., Silva, A. M. M., Brandão, V. M., Da Silva, I. A., Teixeira, W. C., et al. (2008). Eficácia De Fitoterápicos Em Fêmeas Ingurgitadas De Boophilus Microplus, Provenientes Da Mesorregião Oeste do Maranhão, Brasil. Rev. Bras. Parasitol. Vet. 17, 83–86.
Cruz, B. C., Teixeira, W. F. P., Maciel, W. G., Felippelli, G., Fávero, F. C., Cruz, A. C., et al. (2014). Effects of Fluazuron (2.5 Mg/Kg) and a Combination of Fluazuron (3.0 Mg/Kg)+ Abamectin (0.5 Mg/Kg) on the Reproductive Parameters of a Field Population of Rhipicephalus (Boophilus) Microplus on Experimentally Infested Cattle. Res. Vet. Sci. 97, 80–84. doi: 10.1016/j.rvsc.2014.04.012
Cully, D. F., Vassilatis, D. K., Liu, K. K., Paress, P. S., van der Ploeg, L. H., Schaeffer, J. M., et al. (1994). Cloning of an Avermectin-Sensitive Glutamate-Gated Chloride Channel From Caenorhabditis Elegans. Nature 371, 707–711. doi: 10.1038/371707a0
Cuore, U., Solari, M. A. (2014). Multiresistant Population of Rhipicephalus (Boophilus) Microplus Ticks in Uruguay. Veter. (Montevideo). 50, 4–13.
Cuore, U., Trelles, A., Sanchís, J., Gayo, V., Solari, M. A. (2007). First Diagnosis of Resistance to Fipronil in the Common Cattle Tick Boophilus Microplus. Veter. (Montevideo). 42, 35–41.
Daemon, E., Monteiro, C. M. O., Rosa, L. S., Clemente, M. A., Arcoverde, A. (2009). Evaluation of the Acaricide Activity of Thymol on Engorged and Unengorged Larvae of Rhipicephalus Sanguineus (Latreill) (Acari: Ixodidae). Parasitol. Res. 105, 495–497. doi: 10.1007/s00436-009-1426-9
Davey, R. B., Ahrens, E. H., George, J. E. (1984). Efficacy of Sprays of Amitraz Against Boophilus Ticks on Cattle. Prev. Vet. Med. 2, 691–698. doi: 10.1016/01675877(84)90014-X
Davey, R. B., Ahrens, E. H., George, J. E. (1989). Ovicidal Activity of Topically Applied Acaricides Against Eggs of the Southern Cattle Tick (Acari: Ixodidae). J. Med. Entomol. 82, 539–542. doi: 10.1093/jee/82.2.539
Davey, R. B., George, J. E. (1998). In Vitro and In Vivo Evaluations of a Strain of Boophilus Microplus (Acari: Ixodidae) Selected for Resistance to Permethrin. J. Med. Entomol. 35, 1013–1019. doi: 10.1093/jmedent/35.6.1013
Davey, R. B., Thomas, D. B., Pound, J. M., Lohmeyer, K. H., Miller, R. J. (2013). Efficacy of an Organophosphate Mixture Against an Organophosphate-Resistant Strain of Rhipicephalus (Boophilus) Microplus (Acari: Ixodidae). Entomol. Sci. 48, 306–316. doi: 10.18474/0749-8004-48.4.306
Davies, T. G. E., Field, L. M., Usherwood, P. N. R., Williamson, M. S. (2007). DDT, Pyrethrins, Pyrethroids and Insect Sodium Channels. IUBMB Life 59, 151–162. doi: 10.1080/15216540701352042
de la Fuente, J., Contreras, M., Estrada-Peña, A., Cabezas-Cruz, A. (2017). Targeting a Global Health Problem: Vaccine Design and Challenges for the Control of Tick-Borne Diseases. Vaccine 35, 5089–5094. doi: 10.1016/j.vaccine.2017.07.097
de Oliveira Souza Higa, L., Garcia, M. V., Barros, J. C., Koller, W. W., Andreotti, R. (2015). Acaricide Resistance Status of the Rhipicephalus Microplus in Brazil: A Literature Overview. Med. Chem. 5, 326–333. doi: 10.4172/2161-0444.1000281
de Souza Chagas, A. C., de Barros, L. D., Cotinguiba, F., Furlan, M., Giglioti, R., de Sena Oliveira, M. C., et al. (2012). In Vitro Efficacy of Plant Extracts and Synthesized Substances on Rhipicephalus (Boophilus) Microplus (Acari: Ixodidae). Parasitol. Res. 110, 295–303. doi: 10.1007/s00436-011-2488-z
Desta, B.G.E.A.H. (2016). Review on the Impact of Ticks on Livestock Health and Productivity. J. Bio. Agr. Health 6:1–7.
Domingues, L. N., Brasil, B.D.S.A.F., de Paiva Bello, A. C. P., da Cunha, A. P., de Barros, A. T. M., Leite, R. C., et al. (2012). Survey of Pyrethroid and Organophosphate Resistance in Brazilian Field Populations of Rhipicephalus (Boophilus) Microplus: Detection of C190A Mutation in Domain II of the Para-Type Sodium Channel Gene. Vet. Parasitol. 189, 327–332. doi: 10.1016/j.vetpar.2012.04.031
Dong, K. (2007). Insect Sodium Channels and Insecticide Resistance. Invert. Neurosci. 7, 17–30. doi: 10.1007/s10158-006-0036-9
Drummond, R. E. A., Ernst, S. E., Trevino, J. L., Gladney, W. J., Graham, O. H. (1973). Boophilus Annulatus and B. Microplus: Laboratory Tests of Insecticides. J. Econ. Entomol. 66, 130–133. doi: 10.1093/jee/66.1.130
Drummond, R. O., Graham, O. H., Meleney, W. P., Diamant, G. (1964). Field Tests in Mexico With New Insecticides and Arsenic for the Control of Boophilus Ticks on Cattle. J. Econ. Entomol. 57, 340–346. doi: 10.1093/jee/57.3.340
Ducornez, S., Barré, N., Miller, R. J., de Garine-Wichatitsky, M. (2005). Diagnosis of Amitraz Resistance in Boophilus Microplus in New Caledonia With the Modified Larval Packet Test. Vet. Parasitol. 130, 285–292. doi: 10.1016/j.vetpar.2005.04.018
Dudai, Y., Buxbaum, J., Corfas, G., Ofarim, M. (1987). Formamidines Interact With Drosophila Octopamine Receptors, Alter the Flies' Behavior and Reduce Their Learning Ability. J. Comp. Physiol. 161, 739–746. doi: 10.1007/BF00605015
Dumont, P., Chester, T. S., Gale, B., Soll, M., Fourie, J. J., Beugnet, F. (2015a). Acaricidal Efficacy of a New Combination of Fipronil and Permethrin Against Ixodes Ricinus and Rhipicephalus Sanguineus Ticks. Parasitol. Vectors 8, 1–5. doi: 10.1186/s13071-015-0681-0
Dumont, P., Fourie, J. J., Soll, M., Beugnet, F. (2015b). Repellency, Prevention of Attachment and Acaricidal Efficacy of a New Combination of Fipronil and Permethrin Against the Main Vector of Canine Babesiosis in Europe, Dermacentor Reticulatus Ticks. Parasitol. Vectors 8, 1–6. doi: 10.1186/s13071-015-0682-z
El-Ashram, S., Aboelhadid, S. M., Kamel, A. A., Mahrous, L. N., Fahmy, M. M. (2019). First Report of Cattle Tick Rhipicephalus (Boophilus) Annulatus in Egypt Resistant to Ivermectin. Insects 10, 404. doi: 10.3390/insects10110404
Enayati, A. A., Asgarian, F., Amouei, A., Sharif, M., Mortazavi, H., Boujhmehrani, H., et al. (2010). Pyrethroid Insecticide Resistance in Rhipicephalus Bursa (Acari, Ixodidae). Pestic. Biochem. Physiol. 97, 243–248. doi: 10.1016/j.pestbp.2010.03.003
Esteve-Gasent, M. D., Rodríguez-Vivas, R. I., Medina, R. F., Ellis, D., Schwartz, A., Cortés Garcia, B., et al. (2020). Research on Integrated Management for Cattle Fever Ticks and Bovine Babesiosis in the United States and Mexico: Current Status and Opportunities for Binational Coordination. Pathogens 9, 871. doi: 10.3390/pathogens9110871
Evans, P. D., Gee, J. D. (1980). Action of Formamidine Pesticides on Octopamine Receptors. Nature 287, 60–62. doi: 10.1038/287060a0
Fernández-Salas, A., Rodríguez-Vivas, R. I., Alonso-Díaz, M.Á. (2012). Resistance of Rhipicephalus Microplus to Amitraz and Cypermethrin in Tropical Cattle Farms in Veracruz, Mexico. J. Parasitol. Res. 98, 1010–1014. doi: 10.1645/GE-3074.1
Ffrench-Constant, R. H., Anthony, N., Aronstein, K., Rocheleau, T., Stilwell, G. (2000). Cyclodiene Insecticide Resistance: From Molecular to Population Genetics. Annu. Rev. Entomol. 45, 449–466. doi: 10.1146/annurev.ento.45.1.449
Foil, L. D., Coleman, P., Eisler, M., Fragoso-Sanchez, H., Garcia-Vazquez, Z., Guerrero, F. D., et al. (2004). Factors That Influence the Prevalence of Acaricide Resistance and Tick-Borne Diseases. Vet. Parasitol. 125, 163–181. doi: 10.1016/j.vetpar.2004.05.012
Food and Aagricultural Oorganization of U.S (2009) Specifications and Evaluation for Agricultural Pesticides (Fipronil). Available at: http://www.fao.org/fileadmin/templates/agphome /documents/Pests_Pesticides/Specs/fipronil09.pdf (Accessed April 05, 2022).
Food and Agriculture Organization of the United Nations (2004) Guidelines Resistance Management and Integrated Parasite Control in Ruminants. Available at: http://www.fao.org/publications/card/en/c/AG014E (Accessed April 05, 2022).
Freeman, J. M., Davey, R. B., Kappmeyer, L. S., Kammlah, D. M., Olafson, P. U. (2010). Bm86 Midgut Protein Sequence Variation in South Texas Cattle Fever Ticks. Parasitol. Vectors 3, 1–8. doi: 10.1186/1756-3305-3-101
Garcia, M. V., Matias, J., Calvacante, J., Pires, D., da Silva, R., Andreotti, R. (2012). Chemical Identification of Tagetes Minuta Linnaeus (Asteraceae) Essential Oil and its Acaricidal Effect on Ticks. Rev. Bras. Parasitol. Vet. 4, 405–411. doi: 10.1590/S1984-29612012000400011
George, J. E. (2000). Present and Future Technologies for Tick Control. Ann. N. Y. Acad. Sci. 916, 583–588. doi: 10.1111/j.1749-6632.2000.tb05340.x
George, J. E., Pound, J. M., Davey, R. B. (2004). Chemical Control of Ticks on Cattle and the Resistance of These Parasites to Acaricides. Parasitology 129, 353–366. doi: 10.1017/S0031182003004682
Ghosh, S., Kumar, R., Nagar, G., Kumar, S., Sharma, A. K., Srivastava, A., et al. (2015). Survey of Acaricides Resistance Status of Rhipicephalus (Boophilus) Microplus Collected From Selected Places of Bihar, an Eastern State of India. Ticks. Tick. Borne. Dis. 6, 668–675. doi: 10.1016/j.ttbdis.2015.05.013
Godara, R., Katoch, R., Rafiqi, S. I., Yadav, A., Nazim, K., Sharma, R., et al. (2019). Synthetic Pyrethroid Resistance in Rhipicephalus (Boophilus) Microplus Ticks From North-Western Himalayas, India. Trop. Anim. Health Prod. 51, 1203–1208. doi: 10.1007/s11250-019-01810-8
Graf, J. F., Gogolewski, R., Leach-Bing, N., Sabatini, G. A., Molento, M. B., Bordin, E. L., et al. (2004). Tick Control: An Industry Point of View. Parasitology 129, S427–S442. doi: 10.1017/S0031182004006079
Graham, O. H., Hourrigan, J. L. (1977). Eradication Programs for the Arthropod Parasites of Livestock. J. Med. Entomol. 13, 629–658. doi: 10.1093/jmedent/13.6.629
Guerrero, F. D., Davey, R. B., Miller, R. J. (2001). Use of an Allele-Specific Polymerase Chain Reaction Assay to Genotype Pyrethroid Resistant Strains of Boophilus Microplus (Acari: Ixodidae). J. Med. Entomol. 38, 44–50. doi: 10.1603/0022-2585-38.1.44
Guerrero, F. D., Lovis, L., Martins, J. R. (2012a). Acaricide Resistance Mechanisms in Rhipicephalus (Boophilus) Microplus. Rev. Bras. Parasitol. Vet. 21, 1–6. doi: 10.1590/S1984-29612012000100002
Guerrero, F. D., Miller, R. J., de León, A. A. P. (2012b). Cattle Tick Vaccines: Many Candidate Antigens, But Will a Commercially Viable Product Emerge? Int. J. Parasitol. 42, 421–427. doi: 10.1016/j.ijpara.2012.04.003
Guerrero, F., Pérez de León, A., Rodriguez-Vivas, R., Jonsson, N., Miller, R., Andreotti, R. (2014). Acaricide Research and Development, Resistance and Resistance Monitoring 2, 353–374.
Guglielmone, A. A., Petney, T. N., Robbins, R. G. (2020). Ixodidae (Acari: Ixodoidea): Descriptions and Redescriptions of All Known Species From 1758 to December 31, 2019. Zootaxa 4871, 1–322. doi: 10.11646/zootaxa.4871.1.1
Gupta, S., Gupta, S., Kumar, S. (2021). Emergence of Fipronil Resistance in Cattle Ticks Rhipicephalus Microplus and Hyalomma Anatolicum Collected From Haryana, India. Int. J. Trop. Insect Sci. 41, 401–407. doi: 10.1007/s42690-020-00218-4
He, H., Chen, A. C., Davey, R. B., Ivie, G. W., George, J. E. (1999). Identification of a Point Mutation in the Para-Type Sodium Channel Gene From a Pyrethroid-Resistant Cattle Tick. Biochem. Biophys. Res. Commun. 261, 558–561. doi: 10.1006/bbrc.1999.1076
Hemingway, J., Ranson, H. (2000). Insecticide Resistance in Insect Vectors of Human Disease. Annu. Rev. Entomol. 45, 371–391. doi: 10.1146/annurev.ento.45.1.371
Higa, L. D. O. S., Garcia, M. V., Rodrigues, V. D. S., Junior, P. B., Barradas Pina, F. T., Barros, J. C., et al. (2019). Effects of Cypermethrin, Chlorpyrifos and Piperonyl Butoxide-Based Pour-on and Spray Acaricides on Controlling the Tick Rhipicephalus Microplus. Syst. Appl. Acarol. 24, 278–286. doi: 10.11158/saa.24.2.10
Holan, G. (1969). New Halocyclopropane Insecticides and the Mode of Action of DDT. Nature 221, 1025–1029. doi: 10.1038/2211025a0
Hollingworth, R. M. (1976). Chemistry, Biological Activity, and Uses of Formamidine Pesticides. Environ. Health Perspect. 14, 57–69. doi: 10.1289/ehp.761457
Hope, M., Menzies, M., Kemp, D. (2010). Identification of a Dieldrin Resistance-Associated Mutation in Rhipicephalus (Boophilus) Microplus (Acari: Ixodidae). J. Econ. Entomol. 103, 1355–1359. doi: 10.1603/EC09267
Jackson, D., Cornell, C. B., Luukinen, B., Buhl, K., Stone, D. (2009) Fipronil General Fact Sheet; National Pesticide Information Center, Oregon State University Extension Services. Available at: http://npic.orst.edu/factsheets/fipronil.html.
Jackson, M. A., Jaronski, S. T. (2009). Production of Microsclerotia of the Fungal Entomopathogen Metarhizium Anisopliae and Their Potential for Use as a Biocontrol Agent for Soil-Inhabiting Insects. Mycol. Res. 113, 842–850. doi: 10.1016/j.mycres.2009.03.004
Janer, E. C., Díaz, A., Fontes, F., Baraibar, F., Saporiti, T., Olhagaray, M. E. (2021). Molecular Survey of Pyrethroid and Fipronil Resistance in Isolates of Rhipicephalus Microplus in the North of Uruguay. Ticks. Tick. Borne. Dis. 12, 101747. doi: 10.1016/j.ttbdis.2021.101747
Janer, E. C., Klafke, G. M., Fontes, F., Capurro, M. L., Schumaker, T. S. S. (2019). Mutations in Rhipicephalus Microplus GABA Gated Chloride Channel Gene Associated With Fipronil Resistance. Ticks. Tick. Borne. Dis. 10, 761–765. doi: 10.1016/j.ttbdis.2019.03.009
Jaronski, S. T. (2010). Ecological Factors in the Inundative Use of Fungal Entomopathogens. BioControl 55, 159–185. doi: 10.1007/s10526-009-9248-3
Jayaraj, R., Megha, P., Sreedev, P. (2016). Organochlorine Pesticides, Their Toxic Effects on Living Organisms and Their Fate in the Environment. Interdiscip. Toxicol. 9, 90–100. doi: 10.1515/intox-2016-0012
Jonsson, N. N., Cutullè, C., Corley, S. W., Seddon, J. M. (2010a). Identification of a Mutation in the Para-Sodium Channel Gene of the Cattle Tick Rhipicephalus Microplus Associated With Resistance to Flumethrin But Not to Cypermethrin. Int. J. Parasitol. 40, 1659–1664. doi: 10.1016/j.ijpara.2010.07.006
Jonsson, N. N., Hope, M. (2007). Progress in the Epidemiology and Diagnosis of Amitraz Resistance in the Cattle Tick Boophilus Microplus. Vet. Parasitol. 146, 193–198. doi: 10.1016/j.vetpar.2007.03.006
Jonsson, N. N., Klafke, G., Corley, S. W., Tidwell, J., Berry, C. M., Koh-Tan, H. H. (2018). Molecular Biology of Amitraz Resistance in Cattle Ticks of the Genus Rhipicephalus. Front. Biosci. (Landmark). 23, 796–810. doi: 10.2741/4617
Jonsson, N. N., Miller, R. J., Kemp, D. H., Knowles, A., Ardila, A. E., Verrall, R. G., et al. (2010b). Rotation of Treatments Between Spinosad and Amitraz for the Control of Rhipicephalus (Boophilus) Microplus Populations With Amitraz Resistance. Vet. Parasitol. 169, 157–164. doi: 10.1016/j.vetpar.2009.12.026
Junquera, P., Hosking, B., Gameiro, M., Macdonald, A. (2019). Benzoylphenyl Ureas as Veterinary Antiparasitics. An Overview and Outlook With Emphasis on Efficacy, Usage and Resistance. Parasite 26, 1–26. doi: 10.1051/parasite/2019026
Jyoti, Singh, N. K., Singh, H., Rath, S. S. (2019). Modified Larval Packet Test Based Detection of Amitraz Resistance in Hyalomma Anatolicum Koch (Acari: Ixodidae) From Punjab Districts of India. Int. J. Acarol. 45, 391–394. doi: 10.1080/01647954.2019.1667432
Kagaruki, L. K. (1991). Tick (Acari: Ixodidae) Resistance to Organochlorine Acaricides in Tanzania. Int. J. Pest Manage. 37, 33–36. doi: 10.1080/09670879109371533
Kamran, K., Ali, A., Villagra, C. A., Bazai, Z. A., Iqbal, A., Sajid, M. S. (2021). Hyalomma Anatolicum Resistance Against Ivermectin and Fipronil is Associated With Indiscriminate Use of Acaricides in Southwestern Balochistan, Pakistan. Parasitol. Res. 120, 15–25. doi: 10.1007/s00436-020-06981-0
Kasai, S. (2004). Role of Cytochrome P450 in Mechanism of Pyrethroid Resistance. J. Pestic. Sci. 29, 234–239. doi: 10.1584/jpestics.29.234
Khaladj, M., Rahbari, S., Nabian, S., Laddonni, H., Atarod, V., Gerami Sadeghian, A., et al. (2007). Evaluation of Some Acaricide Agents Against Hard Ticks. J. Vet. Res. 62, 53–56.
Kitaka, F. X., Oteng, A. K., Kamya, E. P. (1970). Toxaphene-Resistant Ticks Occurring on Cattle in Uganda: Boophilus Decoloratus, Rhipicephalus Evertsi and Rhipicephalus Appendiculatus. Bull. Epizoot. Dis. Afr. 18, 137–142.
Klafke, G. M., de Albuquerque, T. A., Miller, R. J., Schumaker, T. T. S. (2010). Selection of an Ivermectin-Resistant Strain of Rhipicephalus Microplus (Acari: Ixodidae) in Brazil. Vet. Parasitol. 168, 97–104. doi: 10.1016/j.vetpar.2009.10.003
Klafke, G. M., Miller, R. J., Tidwell, J. P., Thomas, D. B., Sanchez, D., Arroyo, T. P. F., et al. (2019). High-Resolution Melt (HRM) Analysis for Detection of SNPs Associated With Pyrethroid Resistance in the Southern Cattle Fever Tick, Rhipicephalus (Boophilus) Microplus (Acari: Ixodidae). Int. J. Parasitol.: Drugs Drug Resist. 9, 100–111. doi: 10.1016/j.ijpddr.2019.03.001
Klafke, G. M., Sabatini, G. A., Thais, A., Martins, J. R., Kemp, D. H., Miller, R. J., et al. (2006). Larval Immersion Tests With Ivermectin in Populations of the Cattle Tick Rhipicephalus (Boophilus) Microplus (Acari: Ixodidae) From State of Sao Paulo, Brazil. Vet. Parasitol. 142, 386–390. doi: 10.1016/j.vetpar.2006.07.001
Klafke, G., Webster, A., Agnol, B. D., Pradel, E., Silva, J., de la Canal, L. H., et al. (2017). Multiple Resistance to Acaricides in Field Populations of Rhipicephalus Microplus From Rio Grande do Sul State, Southern Brazil. Ticks. Tick. Borne. Dis. 8, 73–80. doi: 10.1016/j.ttbdis.2016.09.019
Koc, S., Oz, E., Cinbilgel, I., Aydin, L., Cetin, H. (2013). Acaricidal Activity of Origanum Bilgeri P.H. Dais (Lamiaceae) Essential Oil and its Major Component, Carvacrol Against Adults Rhipicephalus Turanicus (Acari: Ixodidae). Vet. Parasitol. 193, 316–319. doi: 10.1016/j.vetpar.2012.11.010
Kumar, R. (2019). Molecular Markers and Their Application in the Monitoring of Acaricide Resistance in Rhipicephalus Microplus. Exp. Appl. Acarol. 78, 149–172. doi: 10.1007/s10493-019-00394-0
Kumar, R., Nagar, G., Sharma, A. K., Kumar, S., Ray, D. D., Chaudhuri, P., et al. (2013). Survey of Pyrethroids Resistance in Indian Isolates of Rhipicephalus (Boophilus) Microplus: Identification of C190A Mutation in the Domain II of the Para-Sodium Channel Gene. Acta Trop. 125, 237–245. doi: 10.1016/j.actatropica.2012.10.006
Kwong, T. C. (2002). Organophosphate Pesticides: Biochemistry and Clinical Toxicology. Ther. Drug Monit. 24, 144–149. doi: 10.1097/00007691-200202000-00022
Lazcano Díaz, E., Padilla Camberos, E., Castillo Herrera, G. A., Estarrón Espinosa, M., Espinosa Andrews, H., Paniagua Buelnas, N. A., et al. (2019). Development of Essential Oil-Based Phyto-Formulations to Control the Cattle Tick Rhipicephalus Microplus Using a Mixture Design Approach. Exp. Parasitol. 201, 26–33. doi: 10.1016/j.exppara.2019.04.008
Le Corronc, H., Alix, P., Hue, B. (2002). Differential Sensitivity of Two Insect GABA-Gated Chloride Channels to Dieldrin, Fipronil and Picrotoxinin. J. Insect Physiol. 48, 419–431. doi: 10.1016/S0022-1910(02)00061-6
Lees, K., Bowman, A. S. (2007). Tick Neurobiology: Recent Advances and the Post-Genomic Era. Invert. Neurosci. 7, 183–198. doi: 10.1007/s10158-007-0060-4
Li, A. Y., Davey, R. B., Miller, R. J., George, J. E. (2003). Resistance to Coumaphos and Diazinon in Boophilus Microplus (Acari: Ixodidae) and Evidence for the Involvement of an Oxidative Detoxification Mechanism. J. Med. Entomol. 40, 482–490. doi: 10.1603/0022-2585-40.4.482
Li, A. Y., Davey, R. B., Miller, R. J., George, J. E. (2004). Detection and Characterization of Amitraz Resistance in the Southern Cattle Tick, Boophilus Microplus (Acari: Ixodidae). J. Med. Entomol. 41, 193–200. doi: 10.1603/0022-2585-41.2.193
Li, A. Y., Davey, R. B., Miller, R. J., George, J. E. (2005a). Mode of Inheritance of Amitraz Resistance in a Brazilian Strain of the Southern Cattle Tick, Boophilus Microplus (Acari: Ixodidae). Exp. Appl. Acarol. 37, 183–198. doi: 10.1007/s10493-005-3260-9
Li, A. Y., Pruett, J. H., Davey, R. B., George, J. E. (2005b). Toxicological and Biochemical Characterization of Coumaphos Resistance in the San Roman Strain of Boophilus Microplus (Acari: Ixodidae). Pestic. Biochem. Physiol. 81, 145–153. doi: 10.1016/j.pestbp.2004.12.002
Liu, N., Wang, Y., Li, T., Feng, X. (2021). G-Protein Coupled Receptors (GPCRs): Signaling Pathways, Characterization, and Functions in Insect Physiology and Toxicology. Int. J. Mol. Sci. 22, 5260. doi: 10.3390/ijms22105260
Lovis, L., Guerrero, F. D., Miller, R. J., Bodine, D. M., Betschart, B., Sager, H. (2012). Distribution Patterns of Three Sodium Channel Mutations Associated With Pyrethroid Resistance in Rhipicephalus (Boophilus) Microplus Populations From North and South America, South Africa and Australia. Int. J. Parasitol.: Drugs Drug Resist. 2, 216–224. doi: 10.1016/j.ijpddr.2012.08.001
Lovis, L., Perret, J. L., Bouvier, J., Fellay, J. M., Kaminsky, R., Betschart, B., et al. (2011). A New In Vitro Test to Evaluate the Resistance Level Against Acaricides of the Cattle Tick, Rhipicephalus (Boophilus) Microplus. Vet. Parasitol. 182, 269–280. doi: 10.1016/j.vetpar.2011.06.004
Lovis, L., Reggi, J., Berggoetz, M., Betschart, B., Sager, H. (2013). Determination of Acaricide Resistance in Rhipicephalus (Boophilus) Microplus (Acari: Ixodidae) Field Populations of Argentina, South Africa, and Australia With the Larval Tarsal Test. J. Med. Entomol. 50, 326–335. doi: 10.1603/ME12127
Luguru, S. M., Chizyuka, H. G. B., Musisi, F. L. (1987). A Survey for Resistance to Acaricides in Cattle Ticks (Acari: Ixodidae) in Three Major Traditional Cattle Areas in Zambia. Bull. Entomol. Res. 77, 569–574. doi: 10.1017/S0007485300012062
MACROCYCLIC LACTONES (Veterinary-Systemic) (2006) (The United States Pharmacopeial Convention, Inc). Available at: https://cdn.ymaws.com/www.aavpt.resource/resmgr/imported/macrocyclicLactones.pdf.
Mangold, A. J., Castelli, M. E., Nava, S., Aguirre, D. H., Guglielmone, A. A. (2005). Poblaciones De La Garrapata Rhipicephalus (Boophilus) Microplus Resistentes a Los Piretroides En Córdoba Y Salta, Argentina. Rev. FAVE. –. Cienc. Vet. 3, 55–59. doi: 10.14409/favecv.v3i1/2.1405
Martinez-Velázquez, M., Rosario-Cruz, R., Castillo-Herrera, G., Flores-Fernandez, J. M., Alvarez, A. H., Lugo-Cervantes, E. (2011). Acaricidal Effect of Essential Oils From Lipia Graveolens (Lamiales: Verbenaceae), Rosmarinus Officinalis (Lamiales: Lamiaceae), and Allium Sativum (Liliales: Liliaceae) Against Rhipicephalus (Boophilus) Microplus (Acari: Ixodidae). J. Med. Entomol. 48, 822–827. doi: 10.1603/ME10140
Martin, R. J., Robertson, A. P., Wolstenholme, A. J. (2002). Mode of Action of the Macrocyclic Lactones. Macrocyclic. Lactones. Antiparasit. Ther., 125–140. doi: 10.1079/9780851996172.0125
Martins, J. R., Corrêa, B.L., Ceresér, V.H. (1995b). Acaricide Action of Fluazuron, Pour-on Formulation, on the Cattle Tick Boophilus Microplus. SAGAR
Martins, J. R., Corrêa, B. L., Ceresér, V. H., Arteche C, C. P. (1995a). A Situation Reports on Resistance to Acaricides by the Cattle Tick Boophilus Microplus in the State of Rio Grande do Sul, Southern Brazil. Publ. Especial., 1–8.
Martins, J. R., Furlong, J. (2001). Avermectin Resistance of the Cattle Tick Boophilus Microplus in Brazil. Vet. Rec. 149, 64.
Matthewson, M. D., Baker, J. A. F. (1975). Arsenic Resistance in Species of Multi-Host Ticks in the Republic of South Africa and Swaziland. J. S. Afr. Vet. Assoc. 46, 341–344.
Maunder, J. C. J. (1949). Cattle Tick Control: Results Achieved in the Field With DDT and BHC. Qld. Agric. J., 1–8.
McKellar, Q. A., Benchaoui, H. A. (1996). Avermectins and Milbemycins. J. Vet. Pharmacol. Ther. 19, 331–351. doi: 10.1111/j.1365-2885.1996.tb00062.x
Mekonnen, S., Bryson, N. R., Fourie, L. J., Peter, R. J., Spickett, A. M., Taylor, R. J., et al. (2002). Acaricide Resistance Profiles of Single-and Multi-Host Ticks From Communal and Commercial Farming Areas in the Eastern Cape and North-West Provinces of South Africa. Onderstepoort J. Vet. Res. 69, 99–105.
Miller, R. J., Almazán, C., Ortíz-Estrada, M., Davey, R. B., George, J. E., De León, A. P. (2013). First Report of Fipronil Resistance in Rhipicephalus (Boophilus) Microplus of Mexico. Vet. Parasitol. 191, 97–101. doi: 10.1016/j.vetpar.2012.08.011
Miller, R. J., Davey, R. B., George, J. E. (2002). Modification of the Food and Agriculture Organization Larval Packet Test to Measure Amitraz-Susceptibility Against Ixodidae. J. Med. Entomol. 39, 645–651. doi: 10.1603/0022-2585-39.4.645
Miller, R. J., Davey, R. B., George, J. E. (2005). First Report of Organophosphate Resistant Boophilus Microplus (Acari: Ixodidae) Within the United States. J. Med. Entomol. 42, 912–917. doi: 10.1093/jmedent/42.5.912
Miller, R. J., George, J. E., Guerrero, F., Carpenter, L., Welch, J. B. (2001). Characterization of Acaricide Resistance in Rhipicephalus Sanguineus (Latreille) (Acari: Ixodidae) Collected From the Corozal Army Veterinary Quarantine Center, Panama. J. Med. Entomol. 38, 298–302. doi: 10.1603/0022-2585-38.2.298
Mohler, J. R. (1906). Texas or Tick Fever and its Prevention (No. 258) (US Department of Agriculture).
Morgan, J. A., Corley, S. W., Jackson, L. A., Lew-Tabor, A. E., Moolhuijzen, P. M., Jonsson, N. N. (2009). Identification of a Mutation in the Para-Sodium Channel Gene of the Cattle Tick Rhipicephalus (Boophilus) Microplus Associated With Resistance to Synthetic Pyrethroid Acaricides. Int. J. Parasitol. 39, 775–779. doi: 10.1016/j.ijpara.2008.12.006
Muyobela, J., Nkunika, P. O. Y., Mwase, E. T. (2015). Resistance Status of Ticks (Acari; Ixodidae) to Amitraz and Cypermethrin Acaricides in Isoka District, Zambia. Trop. Anim. Health Prod. 47, 1599–1605. doi: 10.1007/s11250-015-0906-4
Nagar, G., Sharma, A. K., Kumar, S., Saravanan, B. C., Kumar, R., Gupta, S., et al. (2018). Molecular Mechanism of Synthetic Pyrethroid and Organophosphate Resistance in Field Isolates of Rhipicephalus Microplus Tick Collected From a Northern State of India. Exp. Appl. Acarol. 75, 319–331. doi: 10.1007/s10493-018-0265-8
National Research Council (1977). Arsenic: Medical and Biologic Effects of Environmental Pollutants.
Newton, L. G. (1967). Acaricia E Resistance and Cattle Tick Control. Aust. Vet. J. 43, 389–394. doi: 10.1111/j.1751-0813.1967.tb04891.x
Nolan, J., Roulston, W. J., Schnitzerling, H. J. (1979). The Potential of Some Synthetic Pyrethroids for Control of the Cattle Tick (Boophilus Microplus). Aust. Vet. J. 55, 463–466. doi: 10.1111/j.1751-0813.1979.tb00369.x
Norris, K. R., Stone, B. F. (1956). Toxaphene-Resistant Cattle Ticks (Boophilus Microplus (Canestrini)) Occurring in Queensland. Aust. J. Agric. Res. 7, 211–226. doi: 10.1071/AR9560211
Nwanade, C. F., Wang, M., Wang, T., Yu, Z., Liu, J. (2020). Botanical Acaricides and Repellents in Tick Control: Current Status and Future Directions. Exp.Appl. Acarol. 81, 1–35. doi: 10.1007/s10493-020-00489-z
Ortiz Estrada, M., Santamaria Vargas, M., Ortiz Najera, A., Soberanes Cespedes, N., Osorio Miranda, J., Franco Bello, R., et al. (1995). Characterization of B. Microplus Resistance to Ixodicides in Mexico. Publ. Especial., 58–66.
Ozoe, Y., Asahi, M., Ozoe, F., Nakahira, K., Mita, T. (2010). The Antiparasitic Isoxazoline A1443 is a Potent Blocker of Insect Ligand-Gated Chloride Channels. Biochem. Biophys. Res. Commun. 391, 744–749. doi: 10.1016/j.bbrc.2009.11.131
Parizi, L. F., Githaka, N. W., Logullo, C., Konnai, S., Masuda, A., Ohashi, K., et al. (2012). The Quest for a Universal Vaccine Against Ticks: Cross-Immunity Insights. Vet. J. 194, 158–165. doi: 10.1016/j.tvjl.2012.05.023
Parola, P., Raoult, D. (2001). Ticks and Tickborne Bacterial Diseases in Humans: An Emerging Infectious Threat. Clin. Infect. Dis. 32, 897–928. doi: 10.1086/319347
Pavela, R., Canale, A., 004Dehlhorn, H., Benelli, G. (2016). Application of Ethnobotanical Repellents and Acaricides in Prevention, Control and Management of Livestock Ticks: A Review. Res. Vet. Sci. 109, 1–9. doi: 10.1016/j.rvsc.2016.09.001
Perez-Cogollo, L. C., Rodriguez-Vivas, R. I., Ramirez-Cruz, G. T., Miller, R. J. (2010a). First Report of the Cattle Tick Rhipicephalus Microplus Resistant to Ivermectin in Mexico. Vet. Parasitol. 168, 165–169. doi: 10.1016/j.vetpar.2009.10.021
Perez-Cogollo, L. C., Rodriguez-Vivas, R. I., Ramirez-Cruz, G. T., Rosado-Aguilar, J. A. (2010b). Survey of Rhipicephalus Microplus Resistance to Ivermectin at Cattle Farms With History of Macrocyclic Lactones Use in Yucatan, Mexico. Vet. Parasitol. 172, 109–113. doi: 10.1016/j.vetpar.2010.04.030
Phetudomsinsuk, K., Soontornsook, N., Phanusaweekul, N., Pathomsakulwong, W. (2014). Amitraz Toxicity in a Horse. Vet. Integr. Sci. 12, 233–238.
Pohl, P. C., Klafke, G. M., Carvalho, D. D., Martins, J. R., Daffre, S., da Silva Vaz, I., Jr., et al. (2011). ABC Transporter Efflux Pumps: A Defense Mechanism Against Ivermectin in Rhipicephalus (Boophilus) Microplus. Int. J. Parasitol. 41, 1323–1333. doi: 10.1016/j.ijpara.2011.08.004
Pruett, J. H. (2002). Comparative Inhibition Kinetics for Acetylcholinesterases Extracted From Organophosphate Resistant and Susceptible Strains of Boophilus Microplus (Acari: Ixodidae). J. Econ. Entomol. 95, 1239–1244. doi: 10.1603/0022-0493-95.6.1239
Ravindran, R., Jyothimol, G., Amithamol, K. K., Sunil, A. R., Chandrasekhar, L., Lenka, D. R., et al. (2018). In Vitro Efficacy of Amitraz, Coumaphos, Deltamethrin and Lindane Against Engorged Female Rhipicephalus (Boophilus) Annulatus and Haemaphysalis Bispinosa Ticks. Exp. Appl. Acarol. 75, 241–253. doi: 10.1007/s10493-018-0262-y
Raynal, J. T., Silva, A. A. B. D., Sousa, T. D. J., Bahiense, T. C., Meyer, R., Portela, R. W. (2013). Acaricides Efficiency on Rhipicephalus (Boophilus) Microplus From Bahia State North-Central Region. Rev. Bras. Parasitol. Vet. 22, 71–77. doi: 10.1590/S1984-29612013005000006
Reck, J., Klafke, G. M., Webster, A., Dall’Agnol, B., Scheffer, R., Souza, U. A., et al. (2014). First Report of Fluazuron Resistance in Rhipicephalus Microplus: A Field Tick Population Resistant to Six Classes of Acaricides. Vet. Parasitol. 201, 128–136. doi: 10.1016/j.vetpar.2014.01.012
Regassa, A., de Castro, J. J. (1993). Tick Resistance to Acaricides in Western Ethiopia. Trop. Anim. Health Prod. 25, 69–74. doi: 10.1007/BF02236506
Rego, R. O., Trentelman, J. J., Anguita, J., Nijhof, A. M., Sprong, H., Klempa, B., et al. (2019). Counterattacking the Tick Bite: Towards a Rational Design of Anti-Tick Vaccines Targeting Pathogen Transmission. Parasitol. Vectors. 12, 1–20. doi: 10.1186/s13071-019-3468-x
Ribeiro, V. L. S., Dos Santos, J. C., Bordignon, S. A., Apel, M. A., Henriques, A. T., von Poser, G. L. (2010). Acaricidal Properties of the Essential Oil From Hesperozygis Ringens (Lamiaceae) on the Cattle Tick Riphicephalus (Boophilus) Microplus. Bioresour. Technol. 101, 2506–2509. doi: 10.1016/j.biortech.2009.11.016
Ribeiro, J. G., Soares, A. S., Chaves, P. E. E., Limberger, J. T., da Rosa, E., Zuravski, L., et al. (2019). Novel Acaricidal Drug Fluazuron Causes Immunotoxicity via Selective Depletion of Lymphocytes T Cd8. Evid. -Based. Complement. Altern. Med. 2019, 1–7. doi: 10.1155/2019/2815461
Robbertse, L., Baron, S., van der Merwe, N. A., Madder, M., Stoltsz, W. H., Maritz-Olivier, C. (2016). Genetic Diversity, Acaricide Resistance Status and Evolutionary Potential of a Rhipicephalus Microplus Population From a Disease-Controlled Cattle Farming Area in South Africa. Ticks. Tick-borne. Dis. 7, 595–603. doi: 10.1016/j.ttbdis.2016.02.018
Rodriguez-Vivas, R. I., Alonso-Díaz, M. A., Rodríguez-Arevalo, F., Fragoso-Sanchez, H., Santaaria, V. M., Rosario-Cruz, R. (2006a). Prevalence and Potential Risk Factors for Organohosphate and Pyrethroid Resistance in Boophilus Microplus Ticks on Cattle Ranches From the State of Yucatan, Mexico. Vet. Parasitol. 136, 335–342. doi: 10.1016/j.vetpar.2005.05.069
Rodríguez-Vivas, R. I., Arieta-Román, R. J., Perez-Cogollo, L. C., Rosado-Aguilar, J. A., Ramírez-Cruz, G. T., Basto-Estrella, G. (2010). Uso De Lactonas Macrocíclicas Para El Control De La Garrapata Rhipicephalus (Boophilus) Microplus En El Ganado Bovino. Arch. Med. Vet. 42, 115–123. doi: 10.4067/S0301-732X2010000300002
Rodriguez-Vivas, R. I., Jonsson, N. N., Bhushan, C. (2018). Strategies for the Control of Rhipicephalus Microplus Ticks in a World of Conventional Acaricide and Macrocyclic Lactone Resistance. Parasitol. Res. 117, 3–29. doi: 10.1007/s00436-017-5677-6
Rodríguez-Vivas, R. I., Miller, R. J., Ojeda-Chi, M. M., Rosado-Aguilar, J. A., Trinidad-Martínez, I. C., de León, A. P. (2014). Acaricide and Ivermectin Resistance in a Field Population of Rhipicephalus Microplus (Acari: Ixodidae) Collected From Red Deer (Cervus Elaphus) in the Mexican Tropics. Vet. Parasitol. 200, 179–188. doi: 10.1016/j.vetpar.2013.11.025
Rodríguez-Vivas, R. I., Rodriguez-Arevalo, F., Alonso-Díaz, M. A., Fragoso-Sanchez, H., Santamaria, V. M., Rosario-Cruz, R. (2006b). Prevalence and Potential Risk Factors for Amitraz Resistance in Boophilus Microplus Ticks in Cattle Farms in the State of Yucatan, Mexico. Prev. Vet. Med. 75, 280–286. doi: 10.1016/j.prevetmed.2006.04.001
Rosario-Cruz, R., Almazan, C., Miller, R. J., Dominguez-Garcia, D. I., Hernandez-Ortiz, R., de la Fuente, J. (2009). Genetic Basis and Impact of Tick Acaricide Resistance. Front. Biosci. 14, 2657–2665. doi: 10.2741/3403
Rosario-Cruz, R., Guerrero, F. D., Miller, R. J., Rodriguez-Vivas, R. I., Dominguez-Garcia, D. I., Cornel, A. J., et al. (2005). Roles Played by Esterase Activity and by a Sodium Channel Mutation Involved in Pyrethroid Resistance in Populations of Boophilus Microplus (Acari: Ixodidae) Collected From Yucatan, Mexico. J. Med. Entomol. 42, 1020–1025. doi: 10.1093/jmedent/42.6.1020
Rosario-Cruz, R., Miranda-Miranda, E., Garcia-Vasquez, Z., Ortiz-Estrada, M. (1997). Detection of Esterase Activity in Susceptible and Organophosphate Resistant Strains of the Cattle Tick Boophilus Microplus (Acari: Ixodidae). Bull. Entomol. Res. 87, 197–202. doi: 10.1017/S0007485300027358
Sabatini, G. A., Kemp, D. H., Hughes, S., Nari, A., Hansen, J. (2001). Tests to Determine LC50 and Discriminating Doses for Macrocyclic Lactones Against the Cattle Tick, Boophilus Microplus. Vet. Parasitol. 95, 53–62. doi: 10.1016/S0304-4017(00)00406-4
Sajid, M. S., Iqbal, Z., Khan, M. N., Muhammad, G. (2009). In Vitro and In Vivo Efficacies of Ivermectin and Cypermethrin Against the Cattle Tick Hyalomma Anatolicum Anatolicum (Acari: Ixodidae). Parasitol. Res. 105, 1133–1138. doi: 10.1007/s00436-009-1538-2
Saldivar, L., Guerrero, F. D., Miller, R. J., Bendele, K. G., Gondro, C., Brayton, K. A. (2008). Microarray Analysis of Acaricide-Inducible Gene Expression in the Southern Cattle Tick, Rhipicephalus (Boophilus) Microplus. Insect Mol. Biol. 17, 597–606. doi: 10.1111/j.1365-2583.2008.00831.x
Samed, K. O. C., AYDIN, L., CETIN, H. (2021). The First Study on Fipronil, Chlorpyrifos-Methyl and Permethrin Resistance in Rhipicephalus Sanguineus Sensu Lato Ticks From Turkey. Int. J. Trop. Insect Sci., 42, 597–602. doi: 10.1007/s42690-021-00578-5
Schleier, J. J., III, Peterson, R. K. (2011). Pyrethrins and Pyrethroid Insecticides. Green Trends Insect Control. 11, 94–131. doi: 10.1039/9781849732901-00094
Schnitzerling, H. J., Nolan, J., Hughes, S. (1983). Toxicology and Metabolism of Some Synthetic Pyrethroids in Larvae of Susceptible and Resistant Strains of the Cattle Tick Boophilus Microplus (Can.). Pestic. Sci. 14, 64–72. doi: 10.1002/ps.2780140110
Schuntner, C. A., Thompson, P. G. (1977). Inhibition of a Carbaryl Oxidising Enzyme as the Primary Lesion in the Lethal Action of Formamidines in Boophilus Microplus. Aust. J. Entomol. 15, 388–388. doi: 10.1111/j.1440-6055.1976.tb01721.x
Selles, S., Kouidri, M., González, M. G., González, J., Sánchez, M., González-Coloma, A., et al. (2021). Acaricidal and Repellent Effects of Essential Oils Against Ticks: A Review. Pathogens 10, 1379. doi: 10.3390/pathogens10111379
Shafer, T. J., Meyer, D. A., Crofton, K. M. (2005). Developmental Neurotoxicity of Pyrethroid Insecticides: Critical Review and Future Research Needs. Environ. Health Perspect. 113, 123–136. doi: 10.1289/ehp.7254
Sharma, A. K., Kumar, R., Kumar, S., Nagar, G., Singh, N. K., Rawat, S. S., et al. (2012). Deltamethrin and Cypermethrin Resistance Status of Rhipicephalus (Boophilus) Microplus Collected From Six Agro-Climatic Regions of India. Vet. Parasitol. 188, 337–345. doi: 10.1016/j.vetpar.2012.03.050
Sharma, N., Singh, V., KP, S., Solanki, V., Gupta, J. P. (2018). Comparative Resistance Status of Hyalomma Anatolicum and Rhipicephalus (Boophilus) Microplus Ticks Against Synthetic Pyrethroids (Deltamethrin and Cypermethrin) From Banaskantha, Gujarat, India. Int. J. Acarol. 44, 268–275. doi: 10.1080/01647954.2018.1513559
Shaw, R. D. (1966). Culture of an Organophosphorus-Resistant Strain of Boophilus Microplus (Can.) and an Assessment of its Resistance Spectrum. Bull. Entomol. Res. 56, 389–405. doi: 10.1017/S0007485300056480
Shaw, R. D. (1970). Tick Control on Domestic Animals. II. The Effect of Modern Methods of Treatment. Trop. Sci. 12, 29–36.
Shelton, A. M., Roush, R. T. (2000). “Resistance to Insect Pathogens and Strategies to Manage Resistance,” in Field Manual of Techniques in Invertebrate Pathology (Dordrecht: Springer), 829–845. doi: 10.1007/978-94-017-1547-8_37
Shoop, W. L., Mrozik, H., Fisher, M. H. (1995). Structure and Activity of Avermectins and Milbemycins in Animal Health. Vet. Parasitol. 59, 139–156. doi: 10.1016/0304-4017(94)00743-V
Singh, N. K., Gelot, I. S., Singh, V., Rath, S. S. (2015a). Detection of Amitraz Resistance in Rhipicephalus (Boophilus) Microplus From North Gujarat, India. J. Parasitol. Dis. 39, 49–52. doi: 10.1007/s12639-013-0280-y
Singh, N. K., Singh, H., Prerna, M., Rath, S. S. (2015b). First Report of Ivermectin Resistance in Field Populations of Rhipicephalus (Boophilus) Microplus (Acari: Ixodidae) in Punjab Districts of India. Vet. Parasitol. 214, 192–194. doi: 10.1016/j.vetpar.2015.09.014
Singh, N. K., Singh, H., Singh, N. K., Rath, S. S. (2016). Multiple Mutations in the Acetylcholinesterase 3 Gene Associated With Organophosphate Resistance in Rhipicephalus (Boophilus) Microplus Ticks From Punjab, India. Vet. Parasitol. 216, 108–117. doi: 10.1016/j.vetpar.2015.12.004
Soderlund, D. M., Bloomquist, J. R. (1989). Neurotoxic Mechanisms of Pyrethroid Action. Ann. Rev. Entomol. 34, 77. doi: 10.1146/annurev.en.34.010189.000453
Stone, B. F., Haydock, K. P. (1962). A Method for Measuring the Acaricide-Susceptibility of the Cattle Tick Boophilus Microplus (Can.). Bull. Entomol. Res. 53, 563–578. doi: 10.1017/S000748530004832X
Stone, B. F., Meyers, R. A. J. (1957). Dieldrin-Resistant Cattle Ticks, Boophilus Microplus (Canestrini) in Queensland. Aust. J. Agric. Res. 8, 312–317. doi: 10.1071/AR9570312
Stone, N. E., Olafson, P. U., Davey, R. B., Buckmeier, G., Bodine, D., Sidak-Loftis, L. C., et al. (2014). Multiple Mutations in the Para-Sodium Channel Gene are Associated With Pyrethroid Resistance in Rhipicephalus Microplus From the United States and Mexico. Parasitol. Vectors. 7, 1–14. doi: 10.1186/s13071-014-0456-z
Stone, B. F., Webber, L. G. (1960). Cattle Ticks, Boophilus Microplus, Resistant to DDT, BHC, and Dieldrin. Aust. J. Agric. Res. 11, 106–119. doi: 10.1071/AR9600106
Sungirai, M., Baron, S., Moyo, D. Z., De Clercq, P., Maritz-Olivier, C., Madder, M. (2018). Genotyping Acaricide Resistance Profiles of Rhipicephalus Microplus Tick Populations From Communal Land Areas of Zimbabwe. Ticks. Tick-borne. Dis. 9, 2–9. doi: 10.1016/j.ttbdis.2017.10.017
Tabor, A. E., Ali, A., Rehman, G., Rocha Garcia, G., Zangirolamo, A. F., Malardo, T., et al. (2017). Cattle Tick Rhipicephalus Microplus-Host Interface: A Review of Resistant and Susceptible Host Responses. Front. Cell. Infect. Microbiol. 7. doi: 10.3389/fcimb.2017.00506
Takata, M., Misato, S., Ozoe, F., Ozoe, Y. (2020). A Point Mutation in the β-Adrenergic-Like Octopamine Receptor: Possible Association With Amitraz Resistance. Pest Manage. Sci. 76, 3720–3728. doi: 10.1002/ps.5921
Tavares, C. P., Sabadin, G. A., Sousa, I. C., Gomes, M. N., Soares, A. M. S., Monteiro, C. M. O., et al. (2022a). Effects of Carvacrol and Thymol on the Antioxidant and Detoxifying Enzymes of Rhipicephalus Microplus (Acari: Ixodidae). Ticks. Tick-borne. Dis. 13, 101929. doi: 10.1016/j.ttbdis.2022.101929
Tavares, C. P., Sousa, I. C., Gomes, M. N., Miró, V., Virkel, G., Lifschitz, A., et al. (2022b). Combination of Cypermethrin and Thymol for Control of Rhipicephalus Microplus: Efficacy Evaluation and Description of an Action Mechanism. Ticks. Tick-borne. Dis. 13, 101874. doi: 10.1016/j.ttbdis.2021.101874
Temeyer, K. B., Olafson, P. U., Brake, D. K., Tuckow, A. P., Li, A. Y., de León, A. A. P. (2013). Acetylcholinesterase of Rhipicephalus (Boophilus) Microplus and Phlebotomus Papatasi: Gene Identification, Expression, and Biochemical Properties of Recombinant Proteins. Pestic. Biochem. Physiol. 106, 118–123. doi: 10.1016/j.pestbp.2013.01.005
Temeyer, K. B., Olafson, P. U., Miller, R. J. (2009). Genotyping Mutations in BmAChE3: A Survey of Organophosphate-Resistant and-Susceptible Strains of Rhipicephalus (Boophilus) Microplus. J. Med. Entomol. 46, 1355–1360. doi: 10.1603/033.046.0614
Temeyer, K. B., Pruett, J. H., Olafson, P. U., Chen, A. C. (2007). R86Q, a Mutation in BmAChE3 Yielding a Rhipicephalus Microplus Organophosphate-Insensitive Acetylcholinesterase. J. Med. Entomol. 44, 1013–1018. doi: 10.1093/jmedent/44.6.1013
Thullner, F., Willadsen, P., Kemp, D. (2007). Acaricide Rotation Strategy for Managing Resistance in the Tick Rhipicephalus (Boophilus) Microplus (Acarina: Ixodidae): Laboratory Experiment With a Field Strain From Costa Rica. J. Med. Entomol. 44, 817–821. doi: 10.1093/jmedent/44.5.817
Trapaga, B. J. (1989). The Campaign Against Boophilus Microplus in Mexico, Benefit, Problems and Prospects (Mexico: Animal production and health. Food and Agricultural Organization), 50–75.
US Environmental Protection Agency [USEPA] (2006). Reregistration Eligibility Decision (RED) for Permethrin.
Vercruysse, J., Rew, R. S. (2002). Macrocyclic Lactones in Antiparasitic Therapy (Belgium:CABI Pub), 1–432.
Villarino, M. A., Waghela, S. D., Wagner, G. G. (2001). Histochemical Localization of Esterases in the Integument of the Female Boophilus Microplus (Acari: Ixodidae) Tick. J. Med. Entomol. 38, 780–782. doi: 10.1603/0022-2585-38.6.780
Villar, D., Puerta, J., López, A., Chaparro, J. J. (2016). Ivermectin Resistance of Three Rhipicephalus Microplus Populations Using the Larval Immersion Test. Rev. Colomb. Cienc. Pecu. 29, 51–57. doi: 10.21162/PAKJAS/21.9849
Vinturelle, R., Mattos, C., Meloni, J., Lamberti, H. D., Nogueira, J., da Silva Vaz Júnior, I., et al. (2021). Evaluation of Essential Oils as an Ecological Alternative in the Search for Control Rhipicephalus Microplus (Acari: Ixodidae). Vet. Parasitol.: Reg. Stud. Rep. 23, 100523. doi: 10.1016/j.vprsr.2020.100523
Vudriko, P., Okwee-Acai, J., Tayebwa, D. S., Byaruhanga, J., Kakooza, S., Wampande, E., et al. (2016). Emergence of Multi-Acaricide Resistant Rhipicephalus Ticks and its Implication on Chemical Tick Control in Uganda. Parasitol. Vectors. 9, 1–13. doi: 10.1186/s13071-015-1278-3
Ware, G. W., Whitacre, D. M. (2004). An Introduction to Insecticides Vol. 6 (US:The pesticide book).
Whitehead, G. B. (1958). Acaricide Resistance in the Blue Tick, Boophilus Decoloratus (Koch). Part I. Bull. Entomol. Res. 49, 661–673. doi: 10.1017/S0007485300053943
Whitnall, A. B. M., McHARDY, W. M., Whitehead, G. B., Meerholz, F. (1951). Some Observations on the Control of the Bont Tick, Amblyomma Hebraeum Koch. Bull. Entomol. Res. 41, 577–591. doi: 10.1017/S0007485300027838
Willadsen, P., Kemp, D. H. (1988). Vaccination With ‘Concealed’antigens for Tick Control. Parasitol. Today 4, 196–198. doi: 10.1016/0169-4758(88)90084-1
Wu, S., Toews, M. D., Oliveira-Hofman, C., Behle, R. W., Simmons, A. M., Shapiro-Ilan, D. I. (2020). Environmental Tolerance of Entomopathogenic Fungi: A New Strain of Cordyceps Javanica Isolated From a Whitefly Epizootic Versus Commercial Fungal Strains. Insects 11, 711. doi: 10.3390/insects11100711
Keywords: acaricides, mitigation, resistance, SNPs, ticks
Citation: Obaid MK, Islam N, Alouffi A, Khan AZ, da Silva Vaz I Jr, Tanaka T and Ali A (2022) Acaricides Resistance in Ticks: Selection, Diagnosis, Mechanisms, and Mitigation. Front. Cell. Infect. Microbiol. 12:941831. doi: 10.3389/fcimb.2022.941831
Received: 11 May 2022; Accepted: 02 June 2022;
Published: 06 July 2022.
Edited by:
Fernando Ariel Genta, Oswaldo Cruz Foundation (Fiocruz), BrazilReviewed by:
Zhijun Yu, Hebei Normal University, ChinaRodrigo Rosario-Cruz, Autonomous University of Guerrero, Mexico
Copyright © 2022 Obaid, Islam, Alouffi, Khan, da Silva Vaz, Tanaka and Ali. This is an open-access article distributed under the terms of the Creative Commons Attribution License (CC BY). The use, distribution or reproduction in other forums is permitted, provided the original author(s) and the copyright owner(s) are credited and that the original publication in this journal is cited, in accordance with accepted academic practice. No use, distribution or reproduction is permitted which does not comply with these terms.
*Correspondence: Abid Ali, dW9wX2FsaUB5YWhvby5jb20=; Tetsuya Tanaka, azYxOTk0MzFAa2FkYWkuanA=