- 1Physical Chemistry I and Research Center of Micro- and Nanochemistry and (Bio)Technology (Cμ), Department of Chemistry and Biology, University of Siegen, Siegen, Germany
- 2INSERM, Univ Brest, EFS, UMR 1078, GGB-GTCA, Brest, France
- 3CHRU de Brest, Service de Génétique Médicale et de Biologie de la Reproduction, Centre de Référence des Maladies Rares “Maladies Neuromusculaires”, Brest, France
Women with cystic fibrosis (CF) have a significantly lower life expectancy compared to men, which is indicated by an earlier impairment of lung function due to chronic colonization with biofilm formed by Pseudomonas aeruginosa. There is growing evidence that blood serum concentrations of the steroid sex hormone estradiol (E2) correlate with the occurrence of pulmonary exacerbations in CF but also play a role in the mucoid switch of P. aeruginosa. This study aims to shed light on possible microbiological reasons for sexual dimorphism in CF by investigating the influence of E2 on biofilm formation of P. aeruginosa CF isolates. For this purpose, 10 CF isolates of the respiratory tract derived from different CF patients have been treated with E2 in a microtiter plate biofilm model. Biofilms have been examined by crystal violet assays, field emission scanning electron microscopy (FE-SEM), 3D laser scanning microscopy (LSM), and quorum sensing (QS) reporter assays of the supernatants taken from biofilms. This allowed us to simultaneously investigate the effects of E2 on attached biofilm mass, biofilm ultrastructure, and QS activity. Upon E2 treatment, six out of 10 investigated CF isolates showed an increase of attached biofilm mass, whereas biofilms from two tested non-CF laboratory strains (PAO1 and ATCC19660) did not. Moreover, FE-SEM and 3D LSM analyses of the E2 responsive CF biofilms revealed ultrastructural remodeling of biofilm structure at different scales with increased formation of prominent biofilm spots, enhanced coverage with extracellular polymeric substance (EPS), and extended average surface roughness. QS activity measurements performed in biofilm supernatants via luminescence acyl homoserine lactone (AHL) reporter assays further showed that E2 treatment may also modulate QS signaling, as shown in an E2 sensitive CF isolate. Together, our results suggest the biofilm modulating effects of E2 on various clinical CF isolates that are documented by both biomass and ultrastructural changes of biofilms. The gained new insight into the influence of steroid hormones on P. aeruginosa biofilm phenotypes might pave the way for novel future approaches in personalized medicine based on the patients’ sex and hormonal status.
Introduction
CF is a hereditary orphan disease that affects 70,000 people worldwide. It is characterized by the production of a viscous mucus, especially in the lung, and caused by a mutation in the Cystic Fibrosis Transmembrane Conductance Regulator (CFTR) gene resulting in malfunction of the chloride channel encoded by this gene. The CF mucus offers perfect conditions for the colonization of biofilm-forming lung pathogens such as P. aeruginosa, which can lead to life-threatening lung infections and destruction of respiratory airways (Bhagirath et al., 2016; Bell et al., 2020). Although Pseudomonas lung infections have been gradually reduced over the past 10 years, still more than 50% of adult CF patients at 30 years of age exhibit a chronic colonization with P. aeruginosa according to recent CF registry reports from UK, Germany, and US (Cystic Fibrosis Trust, 2020; Nährlich et al., 2020; Cystic Fibrosis Foundation, 2021).
Several studies report on a prominent sexual dimorphism in chronical respiratory diseases associated with colonization and persistence of bacterial pathogens. These differences between women and men in CF patients and patients with non-CF-bronchiectasis apply to disease prevalence, severity, and outcome that means an earlier impairment of lung function in women (Vidaillac et al., 2018). Moreover, women have a significantly lower life expectancy than men, a phenomenon that has been defined as the ‘CF-Gender Gap’ (Harness-Brumley et al., 2014; Saint-Criq and Harvey, 2014; Sweezey and Ratjen, 2014). This observation is underlined by the UK Cystic Fibrosis Registry Annual Data Report 2019 by showing a 6 years lower median predicted survival for female CF patients compared to male CF patients when considering the years 2015-2019 (Cystic Fibrosis Trust, 2020). In spite of the high medical relevance for pathogenesis and patient treatment, there is still a lack of understanding the underlying mechanisms and key drivers (Silveyra et al., 2021). Notably, women on average develop chronic infections with mucoid P. aeruginosa 1.7 years earlier than men (Demko et al., 1995). Mucoid P. aeruginosa produces extraordinary large amounts of the polysaccharide alginate, and is known to be associated with frequent pulmonary exacerbations and resistance to the host’s immune defense and antibiotic treatments (Chotirmall et al., 2012).
Reasons for this sex disparity have not been fully clarified and are discussed controversially (Lam et al., 2021). They seem to be multifactorial, ranging from anatomic to nutrition status differences to hormonal differences between men and women as well as fluctuating sex hormonal serum levels affected by the season, day, age, weight, pregnancy, female cycle, the onset of menopause, or the use of hormonal contraceptives. In particular, the sex hormone 17β-estradiol ((17β)-estra-1,3,5(10)-triene-3,17-diol, hereinafter: E2) contributes to more unfavorable clinical prognosis for women with CF (Chotirmall et al., 2012; Saint-Criq and Harvey, 2014; Sweezey and Ratjen, 2014; Sutton et al., 2015).
The following modes of E2 action in CF disease progression are postulated: 1) an effect on calcium-controlled chloride ion channels (Imberti et al., 2018), 2) the modulation of the immune response during defense against infection (Abid et al., 2017), and 3) an E2 induced increase in specialized mucus-producing epithelial cells of the bronchial mucosa (Tam et al., 2014). From a microbiological point of view, there is evidence that the switch to a mucoid P. aeruginosa phenotype is also influenced by the patient’s blood serum E2 level (Chotirmall et al., 2012).
Very recently, it was shown that E2 can have a direct impact on P. aeruginosa, inducing bacterial motility, production of the virulence factor pyocyanin, as well as adhesion to and invasion into bronchoepithelial cells (Tyrrell and Harvey, 2020). Tyrrell et al. further investigated whether the addition of selective estrogen receptor modulators (SERMs) can reverse these E2 induced effects. The authors found that the mobility of P. aeruginosa in a semi-solid medium, meaning the swarming behavior, as well as the movement on surfaces promoted via type IV pili, meaning the twitching behavior, were significantly increased after adding E2 to the culture. The study resulted in a first hint that E2 modulates biofilm growth of the non-CF laboratory strain PAO1, but not for the two CF isolates from one patient they tested.
However, the underlining molecular mechanisms for such E2 induced physiological changes on bacterial pathogens like P. aeruginosa are still uncovered and looking at the bioavailability and metabolism of E2 by bacteria, there are many open questions. In 1992, it was first reported that P. aeruginosa expresses a cytosolic estrogen-binding protein with lower affinities also for other steroids (Rowland et al., 1992). Apart from that, it was reported that at least some environmental bacteria, including P. aeruginosa, are able to degrade and metabolize steroids for survival and proliferation, although it was observed that passive diffusion might be impeded by the lipopolysaccharide (LPS) leaflet on the outer surface of Gram-negative bacteria (Plésiat and Nikaido, 1992). Just recently, the degradation of E2 via 3-oxoacyl-(acyl-carrier protein) reductase (OAR) from Pseudomonas citronellolisa was reported (Fu et al., 2022). OARs have been also described in P. aeruginosa as an essential enzyme of the fatty acid biosynthetic pathway to produce QS signals and virulence factors like pyoverdine (Guo et al., 2019) that could be another link to how E2 can indirectly influence these relevant virulence processes just via its metabolism.
Until now, there is very limited knowledge on how E2 regulates biofilm development of P. aeruginosa in the context of CF and with respect to growth extent and its phenotype. Therefore, studies reporting on the direct impact of E2 on a broader collection of CF-P. aeruginosa isolates, which are known for their wide variation in phenotype and responsiveness to environmental conditions (Thöming et al., 2020), are needed.
Around 80% of microorganisms in the environment and in the body involved in infections, including P. aeruginosa, reside in biofilms (Jamal et al., 2018). They are attached to mucosal epithelia surfaces and built up by accumulated microorganisms that are encapsulated via a protective and highly hydrated mucous layer consisting of polysaccharides, proteins, and nucleic acids, and called the extracellular polymeric substance (EPS). The EPS facilitates optimal nutrient diffusion and protection against antibiotic treatments via biofilm-induced tolerance, recently proven for P. aeruginosa clinical isolates (Thöming and Häussler, 2022), or the survival of ‘persister cells’, a small subpopulation of non-dividing multi-drug resistant bacterial cells remaining after antibiotic treatment (Yin et al., 2019).
At the beginning of the biofilm’s life cycle a stronger irreversible attachment is established, e.g., mediated via type IV pili, which results in multilayered biofilm structures (Boudarel et al., 2018). During maturation, a spot-like aggregation of cells into microcolonies and a simultaneous production of EPS lead to matured biofilms with unique structures, which can be diverse amongst P. aeruginosa. Finally, a subpopulation of bacteria can actively leave the biofilm or passively detach due to shear forces to initiate a new colonization with subsequent formation of a new biofilm (Yin et al., 2019).
Microbial infections are controlled by a complex bidirectional pathogen-host crosstalk, involving inter-kingdom signaling molecules including hormones. QS systems mediate microbial communication and are essential for the regulation of the biofilm life cycle, including the formation of EPS and virulence factors. The link between the QS system and biofilm formation is best investigated for P. aeruginosa that uses acyl homoserine lactones (AHLs) (Babatunde et al., 2018; Mukherjee and Bassler, 2019). Recent studies found that host factors can also interfere with QS regulated biofilm growth (Blackledge et al., 2013). Biofilms are also known to adapt easily to environmental changes. Microbial infections are controlled by a complex bidirectional intra- and inter-kingdom crosstalk between such biofilm-forming pathogens and the host, which includes microbial signals like QS molecules and virulence factors from bacteria but also signaling molecules originating from the mammalian immune and hormonal system (Freestone, 2013).
P. aeruginosa, for instance, performs a series of adaptive processes during CF lung infections, which are influenced by specific factors in the host’s environment. This microbial adaptation mostly affects the biofilm phenotype or virulence, which in case of P. aeruginosa is reflected by the switch to mucoid phenotypes and an increased production of pyocyanin, both leading to a boost in pathogenicity and decline in lung function (Ryall et al., 2014; Thöming et al., 2020).
Considering the lack of knowledge in understanding how E2 impacts biofilm growth of CF- P. aeruginosa isolates, this study was devoted to performing a systematic investigation of P. aeruginosa isolates from several CF patients. Complementary approaches of biofilm analyses were used to get insights into the impact of the sex steroid hormone estradiol on biofilms of this CF lung pathogen. In doing so, we were able to show that estradiol not only enhances growth of biofilm but also induces ultrastructural changes in biofilms from a subset of CF isolates.
Materials and Methods
Source of Estradiol and Common Chemical Reagents, Media, and Buffer
17β-estradiol (here: E2), β-estradiol-water soluble (cyclodextrin-encapsulated, here: WSE2, 1 mg contains 48 mg E2, rest is 2-Hydroxypropyl-β-cyclodextrin), 2-Hydroxypropyl-β cyclodextrin (here: β-cyclodextrin), Dimethyl sulfoxide (DMSO) and crystal violet (CV) were purchased from Sigma-Aldrich (Steinheim, Germany). Ethanol (EtOH) was purchased from Carl Roth (Karlsruhe, Germany). LB (Lysogeny broth) was prepared by dissolving 7 g/l Sodium Chloride (Th Geyer), 5 g/l Yeast Extract (Carl Roth) and 10 g/l Tryptone/Peptone (granulated, Carl Roth) in distilled water.
Dulbecco’s phosphate buffered saline (DPBS, without calcium or magnesium; Lonza Walkersville, MD USA), buffers, Milli-Q water (Millipore Elix® Advantage 3, Millipak® Filter) and media were sterilized via autoclaving (121°C, 1.2 bar for 15 min) before usage.
Non-CF P. aeruginosa Strains and CF P. aeruginosa Isolates
The bacteria included in this study and their origins are listed in Supplementary Table S1. P. aeruginosa isolates from CF patients have been described and analyzed in previous studies (Berchel et al., 2011; Le Gall et al., 2013; Mottais et al., 2018; Hornischer et al., 2019; Khaledi et al., 2020; Thöming and Häussler, 2022).
Microtiter Plate Biofilm Model and E2 Treatment
P. aeruginosa glycerol stocks stored at -80°C were streaked onto Columbia agar with sheep blood plus (Oxoid Deutschland GmbH, Wesel, Germany). Overnight cultures were prepared by inoculating 3 ml of LB with a single colony and incubated at 180 rpm and 37°C (incubator MaxQ6000, Fisher Scientific, Hampton, NH, USA) for 17 h +/- 2 h.
After adjusting the overnight culture in semi-micro cuvettes (1.6 ml Rotilabo single-use, Carl Roth) to an OD600 of 0.5 in LB, cultures were diluted 250-fold in LB. P. aeruginosa isolates were treated with E2 or EtOH, DMSO or β-cyclodextrin (solvent controls). E2 was dissolved in EtOH and DMSO, respectively while the WSE2 was dissolved in H2O with a final concentration of 10 nM (complexed with 50 nM β-cyclodextrin), if not indicated otherwise. Concentrations of EtOH and DMSO solvent controls were 0.0001% (v/v) and 0.00001% (v/v), respectively. Final concentration for β-cyclodextrin control was 50 nM. Biofilm formation was assessed in a Serocluster™ 96-well U bottom plate, polyvinyl chloride (PVC) (Corning®, Kennebunk, ME, USA) and sealed with an air-permeable membrane AeroSeals™ (Kisker Biotech GmbH & Co. KG). Isolates were placed in a humid stainless-steel chamber to avoid media evaporation and incubated for 48 h or, in case of slow growing isolates, C21-C2 and MHH0985 for 72 h at 37°C without shaking. After 24 h and additionally after 48 h for slow growing isolates 60 µL of medium containing solvent controls and E2 at the above indicated final concentrations was added to the microtiter plate according to an established biofilm model for susceptibility screenings (Thöming and Häussler, 2022) that was adjusted for this study. Biofilms were further analyzed as depicted in Figure 1.
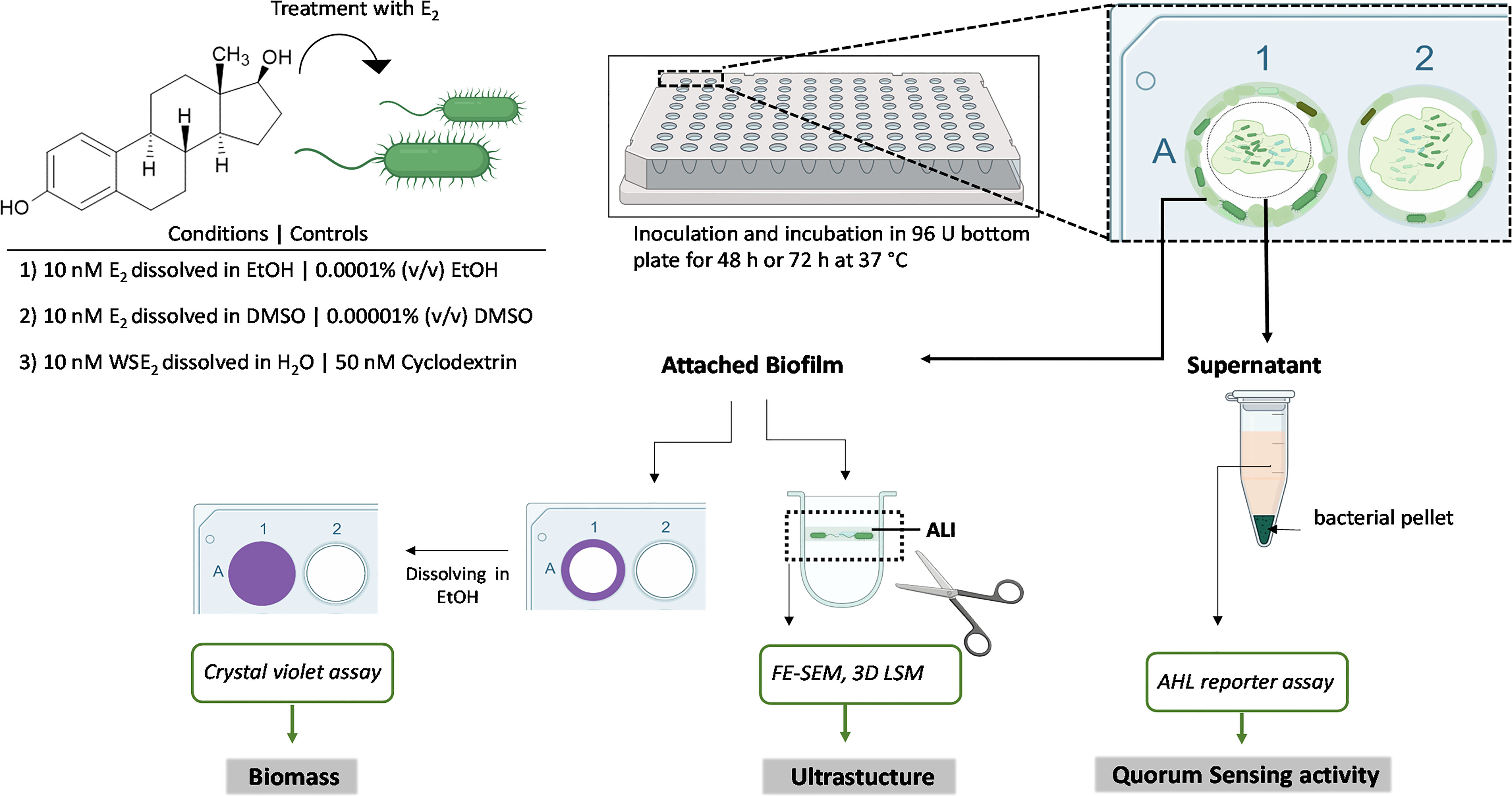
Figure1 Integrated analysis of applied biofilm model. Biofilms of CF isolates were grown under static conditions on a PVC 96-well plate for 48 h or 72 h (in case of slow growing isolates) and both the attached biofilm mass as well as the QS activity in supernatants were analyzed. The attached biofilm was stained with crystal violet to quantify the biomass and sections of the PVC wells containing the biofilm at the air-liquid interface (ALI) were cut out and microscopically analyzed.
Crystal Violet Assay for Quantification of Adherent Biofilm
Non-adherent bacteria were washed off gently by placing the pipette tip in the middle of the well (biofilm mainly adhered to the walls of the wells), and washed thrice with 200 μl sterile H2O at 120 rpm for 5 min at 37°C (incubator MaxQ6000). Adherent bacterial biomass was determined via CV assay as previously described with slight adjustments (O’Toole, 2011). Briefly, biofilms were stained with 180 μl of 0.1% (v/v) CV dissolved in water and filtered to remove crystals. Plates were incubated for 30 min at room temperature (RT). The wells were washed again thrice with H2O while shaking them at 120 rpm for 5 min for each washing step at RT and the plates were tipped on tissues to remove excess stain. To dissolve CV bound to the biofilm 200 μl of 95% (v/v) EtOH was added to each well for 30 min at RT. Solutions were pipetted up and down thrice before transferring the solutions to a transparent flat bottom 96-well plate (Sarstedt AG & Co. KG, Nümbrecht, Germany) in order to measure the absorbance at OD580 with a multiplate reader (Tecan Infinite® M200, Tecan Austria GmbH, Grödig/Salzburg, AUT).
Samples were diluted in a way, that final absorbence values are less than 1.0. For each treatment condition a minimum of six (up to eight) technical replicates (wells) were considered. Percentage of attached biofilm mass was calculated by subtracting the LB sterile control’s background from each sample. E2 induced changes of the percentage of attached biofilm mass was determined by dividing the absorbance at 580 nm as CV assay readout (after sterile LB background subtraction) of E2 treated biofilms by the absorbance of the corresponding solvent control and then multiplied with 100.
Experiments were repeated at least three times. Responsiveness measured via CV assay was defined as 10% change relative to control (> 110%, or <90%), for at least three independent experiments regulated in the same direction for an E2 treatment condition. Another requirement was that the arithmetic mean of all experiments had to exceed this threshold. CF isolates were defined to show no effect on a certain E2 treatment condition, if less than three independent experiments showed a regulation (more than 10%) in the same direction.
FE-SEM Biofilm Sample Preparation and Analysis
PVC plates with biofilms were washed three times with PBS and fixed with 2.5% Glutaraldehyde/PBS over night at 4°C. After the plates were washed again three times with PBS, 200 µL of the following solutions were added to the plates and incubated at 20°C for 10 min for dehydration of samples: 50% EtOH, 70% EtOH, 80% EtOH, 90% EtOH. Afterward, plates were incubated with 100% EtOH for 10 min. Finally, 200 µL of hexamethyldisilazane (Sigma Aldrich, Steinheim, Germany) was added to each well for 30-60 s and plates were air dried before gold sputtering.
Sections of the well including the air-liquid interface (ALI) of the 96-well U bottom plates were cut out with a scalpel (first the bottom, then the upper part). A cut was then made on the side of each well to flatten it on a heated plate at 45°C by gently pressing it down with a tweezer on both sides. Samples were placed on a specimen stub containing carbon adhesive discs. The samples were sputter-coated with a thin gold layer (8 –10 nm) in a sputter coater (S150B, BOC Edwards, Bolton, UK) to increase the conductivity of the sample surfaces at a pressure of 0.2 mbar in an argon atmosphere for 2 min at a voltage of 1.0 kV.
The FE-SEM (Zeiss Ultra 55 cv, Oberkochen, Germany) measurements were performed with an operating voltage of 5.0 kV and the detector was the Inlens secondary electron detector. SmartSEM software was used for the collection and processing of the FE-SEM images. Documented areas of the ALI were defined to be positioned maximally 500 µm below the upper visible border of the biofilm.
Because the FE-SEM analysis lacks information about the Z-axis and therefore three-dimensional structure, the gold-sputtered biofilm samples were also analyzed via the 3D-laser scanning microscope LEXT OLS4000 (Olympus, Hamburg, Germany). Topographical images were measured based on the reflection from the surface of the biofilm samples. The data were processed and analyzed by using SPIP software (Image Metrology, Lyngby, Denmark) to create 3D pictures and determine the roughness parameter Sq. The tilt and curvature of the PVC plates was compensated by using 4th order flattening and measurement noise was removed by interpolation of individual pixel outliers. The topography is shown as 3D representation and the average area roughness Sq of the surface was evaluated in three areas (240x240 µm²) that are free of scratches/artefacts on the surface. The maximum roughness height Rt was determined via the Software Gwyddion 2.60 using linear interpolation and is based on 10 representative profiles over a minimal length of 0.25 mm and a maximal length of 0.7 mm.
Quorum Sensing Reporter Assay
After growth of biofilms as described above supernatants were pooled from 6 wells and centrifuged at 26452 x g for 10 min and transferred to a new Eppendorf tube, sterile filtered (Rotilabo-syringe filter, PES, sterile, pore size 0.20 µm, Carl Roth) and kept on ice until measurement.
For the measurement of QS activity, that indicates the presence of AHLs in the biofilm supernatant, reporter strains Escherichia coli JM109 luxP LuxCDABE pSB401 and Escherichia coli JM109 luxP LuxCDABE pSB1075 (Winson et al., 1998) were used that detect different spectra of AHLs in the supernatant. AHLs N-Hexanoyl-DL-homoserine lactone (C6-HSL) and N-(3-Oxododecanoyl)-L-homoserine lactone (3-Oxo-C12-HSL) purchased from Sigma Aldrich were used as positive controls at a concentration of 10-6 M.
AHL reporter bacteria were overnight cultured by adding one colony to 5 ml LB containing a final concentration of either 10 µg/ml Tetracycline (pSB401) or 100 µg/ml Ampicillin (pSB1075) for 16 h at 200 rpm and 37°C. Afterwards, reporter bacteria suspensions were adjusted to an OD600nm of 0.2 in LB.
Ninety µL of reporter bacteria E. coli pSB401 or E. coli pSB1075 were pipetted in triplicates into a white, sterile 96-well flat bottom microtiter plate (Greiner Bio-one, Frickenhausen, Germany). Thirty µL of supernatant, LB control, or LB containing 10-6 M synthetic AHLs were added to the well and sealed with transparent Ampliseal™ (Greiner Bio-One). Luminescence activity was measured via the multiplate reader (Tecan Infinite® M200) for 20 h with a kinetic interval of 20 min at 30°C. The percentage of QS activity change upon E2 treatment was calculated based on the luminescence activity at timepoint 15 h (for pSB401) or 2 h (for pSB1075). After subtracting the luminescence background caused by the reporter strain for each measurement, mean resulting luminescence of the technical triplicates after E2 treatment was divided by the corresponding luminescence of the mean control condition and multiplied with 100.
Data Processing and Statistical Analysis
Standard deviations were calculated via Excel (STDEV.P) and further calculations with mean values were performed according to standard deviation propagation. Statistical analyses of CV assay data and QS reporter assays were performed using GraphPad Prism to compare E2 induced changes (in terms of attached biofilm mass or QS activity in %) with control condition for a minimum of three independent experiments. One sample t-test compared to a theoretical mean of 100% was performed for each condition. Significant induction or reduction of attached biofilm was defined as p ≤ 0.05. Non-significant response (here induction) means that 10% more attached biofilm mass was measured relative to control for at least three independent experiments as well as the arithmetic mean of all performed experiments. The remaining CF isolates with effects lower than this 10% were dedicated as “no effect”.
Results
The effect of E2 on biofilm development of CF isolates was tested as shown in the experimental setup in Figure 1. Because E2 is barely water soluble we used EtOH for comparison with studies of others (Tyrrell and Harvey, 2020), and DMSO as solvents to carry out experiments under aqueous conditions. As a third condition we used a water soluble E2 that contains 50 nM β-cyclodextrin for complexation of E2 allowing its solubility in H2O to obtain a final concentration of 10 nM E2. All these treatment conditions were compared to the corresponding solvent controls in order to normalize result to possible solvent effects on bacteria. Our integrative approach allows to perform several readouts, including biofilm mass quantification via CV assay, characterization of ultrastructure via FE-SEM, height profile, and roughness via 3D LSM on the same biofilm substrate. Additionally, QS activity of biofilm culture supernatants was determined via AHL reporter assays (Figure 1).
CF Isolates Respond to E2 With Increased Attachment of Biofilm Mass In Vitro
From the 10 investigated CF isolates, six were found responsive to E2 at least for one of the tested E2 treatment in at least three of four performed independent experiments (Figure 2).
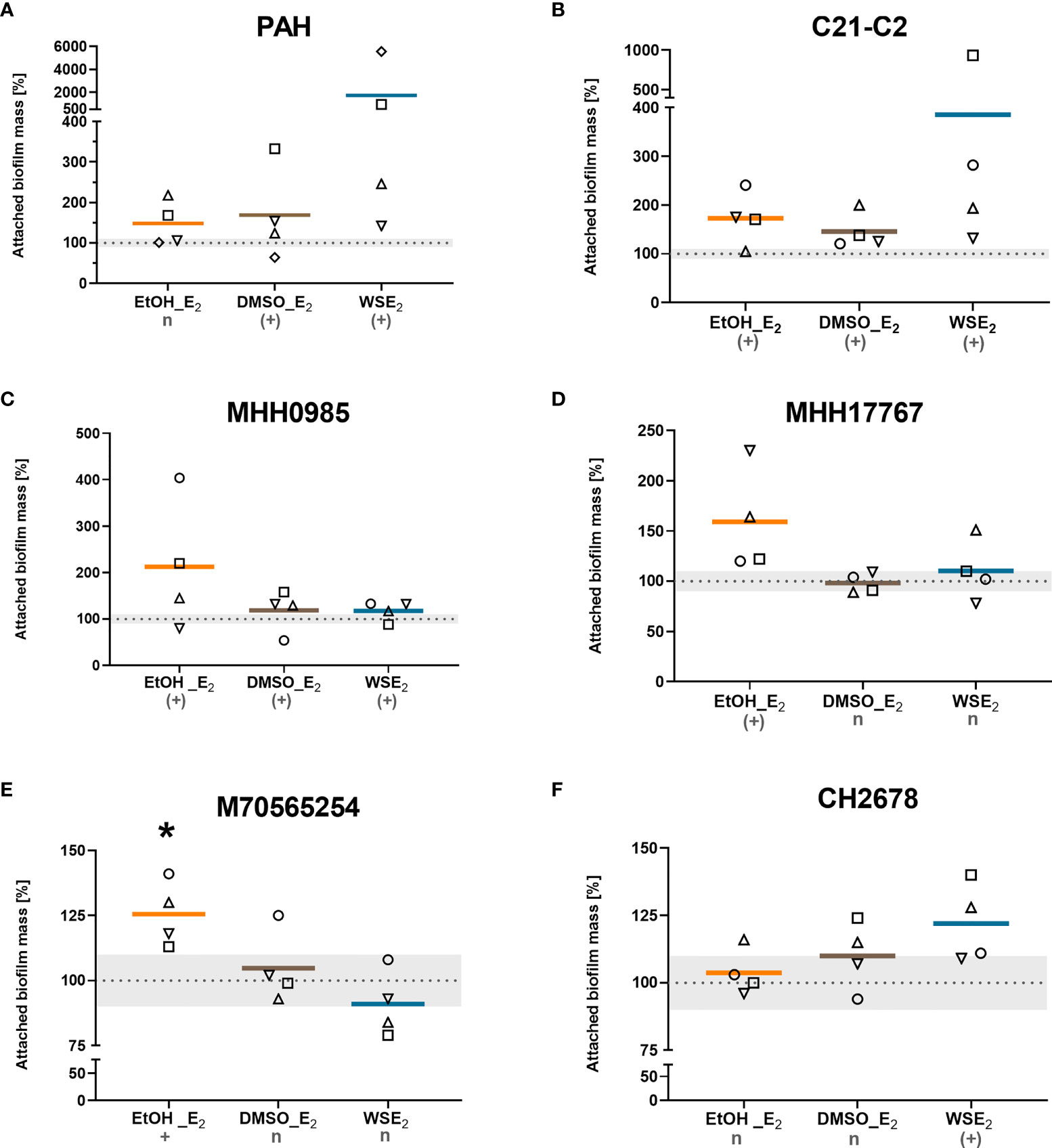
Figure 2 CF isolates that showed E2 induced attached biofilm mass. CF isolates PAH (A), C21-C2 (B), MHH0985 (C), MHH17767 (D), M70565254 (E), and CH2678 (F) were treated either with 10 nM E2 in EtOH (orange), E2 in DMSO (brown), WSE2 (blue), or corresponding solvent controls (see Figure 1) for 48 h or 72 h in case of (B, C). Attached biofilm mass was quantified via CV assay. Absorbance of the corresponding solvent control was set to 100% (see dotted line). Colored horizontal lines indicate mean values of four independent experiments (◯ = experiment 1, ⬜ = experiment 2, △ = experiment 3, ▽ = experiment 4). E2 responsiveness was defined as more than 10% change (indicated by the grey zone between 90% and 110%). *: p ≤ 0.05 (n=4). n: no effect, (+): induction, +: significantly induced (see Materials/Methods for detailed description).
Two isolates (C21-C2 and MHH0985) showed E2 induced biofilm attachment for all three conditions tested that use different approaches to bring E2 in solution (EtOH, DMSO, and β-cyclodextrin in water).
Further, we identified a PAH increase in attached biofilm for both E2 in DMSO and WSE2. MHH17767 and M70565254 responded with an increase in attached biofilm mass to E2 in EtOH with the latter exhibiting a significant effect compared to control, while CH2678 only responded to WSE2. Treatment with WSE2 resulted in the strongest mean attached biofilm mass induction of more than 17-fold for PAH (Figure 2A), followed by treatment with E2 in EtOH, exceeding a mean increase of around 2-fold for isolate MHH0985 (Figure 2C).
To investigate if the induction of attached biofilm mass is indirectly caused by an overall enhanced planktonic growth kinetics, four of the E2 responsive P. aeruginosa isolates were analyzed under the six different growth conditions for 24 h (E2 treatment and corresponding solvent control). Growth dynamics did not result in any relevant changes in doubling times or maximal endpoint OD600 nm upon E2 treatment for any of the tested CF isolates (Supplementary Figures S4A, B). There was also no concentration dependent effect on planktonic growth detectable for WSE2 concentrations ranging from 10-9 to 10-6 M (Supplementary Figure S4C).
Supplementary Figure S5 illustrates that four other CF isolates (C4-C1, MHH15204, MHH16563, and MHH2419) were considered non-E2 responsive to any of the E2 treatment conditions according to the above-mentioned criteria.
To answer the question if E2 causes the same effect on broadly used non-CF P. aeruginosa strains in our biofilm model that are isolated from other human tissue than the respiratory tract, we tested the non-CF strains ATCC19660 and PAO1.
Non-CF strains did not show any E2 responses for most of the tested conditions. Only the treatment of P. aeruginosa ATCC 19660 with E2 in EtOH resulted in a significant decrease in biofilm attachment that means a contrary effect compared to E2 responsive CF isolates. PAO1 turned out to be non-responsive toward E2 regarding a change in attached biofilm mass (Figure 3).
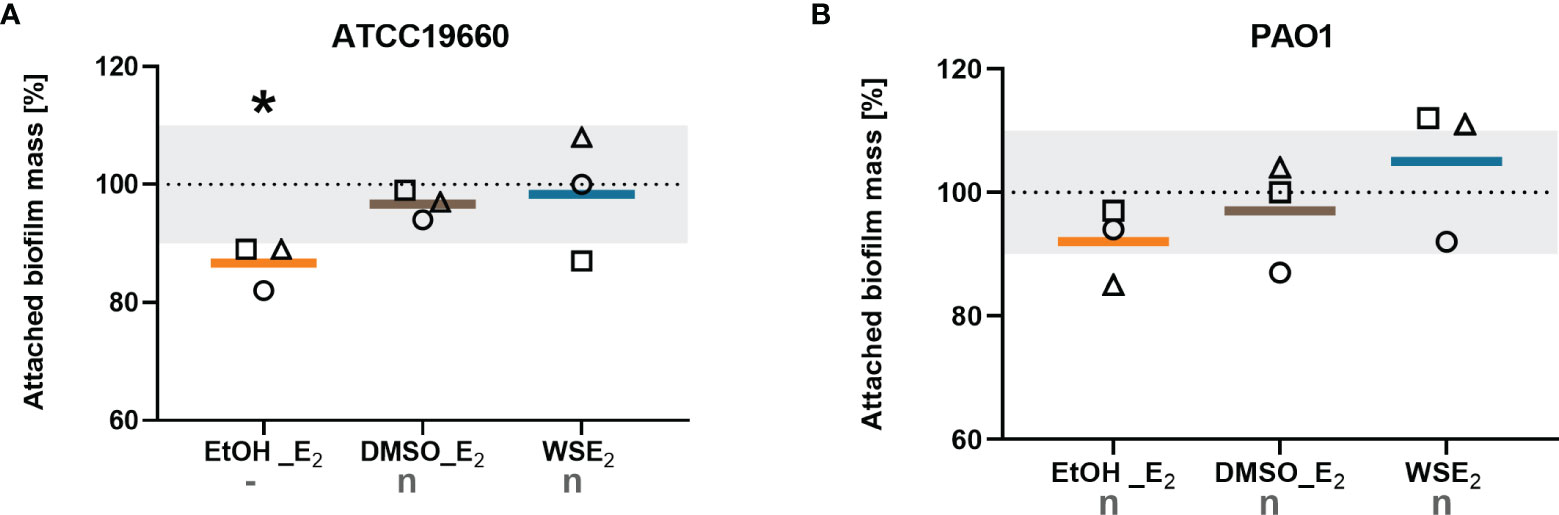
Figure 3 Non-CF strains did not show increase in attached biofilm mass upon any E2 treatment. P. aeruginosa strains ATCC19660 (A) and PAO1 (B) were treated with either 10 nM E2 in EtOH (orange), E2 in DMSO (brown), WSE2 (blue), or corresponding solvent controls for 48 h (see Figure 1). Attached biofilm mass was quantified via CV assay. Solvent controls were set to 100% (see dotted line). Colored horizontal lines indicate mean values of three independent experiments (◯ = experiment 1, ⬜ = experiment 2, △ = experiment 3) with each single experiment based on at least six technical replicates. E2 responsiveness was defined as more than 10% change (indicated by the grey zone between 90% and 110%). *: p ≤ 0.05 (n=3). n: no effect, -: significantly reduced (see Materials/Methods for detailed description).
Table 1 summarizes the E2 effects determined for every strain in the various conditions studied, thus distinguishing between E2 responsive that react with induction or reduction of attached biofilm mass to at least one E2 treatment condition, and E2 non-responsive strains according to the criteria considered.
In summary, our data show that E2 does specifically enhance growth of attached biofilms for more than half of the tested CF isolates, which is independent from planktonic growth behavior. Such E2 effect was not observed with the non-CF strains PAO1 and ATCC19660.
E2 Induces Changes in Biofilm Structure of E2 Responsive CF Isolates
The quality of the biofilm was also investigated microscopically with a focus on surface coverage, surface ultrastructure, height profile, and roughness. We analyzed those CF isolates more in detail that responded to E2 based on the CV assay (PAH, C21-C2, and MHH17767, Figure 2) as well as PAO1 as lab strain that did not show any change in attached biofilm mass (see Figure 3). The strongest effect on ultrastructure upon E2 treatment was observed for the isolate PAH already on a low magnified overview of the overall biofilm for all used solvents showing the extent of biofilm formation and the arrangement of microcolonies (Figures 4, 5). β-cyclodextrin alone had an inhibitory effect on biofilm attachment compared to the other solvent controls. WSE2 could recover biofilm attachment, as shown in Figure 4 and as determined by CV assay indicating on average a more than 17-fold higher attached biofilm mass (Figure 2A). In addition, quantification of surface coverage based on low magnified SEM pictures showed seven times higher biofilm surface coverage compared to β-cyclodextrin control (Supplementary Figure S6C).
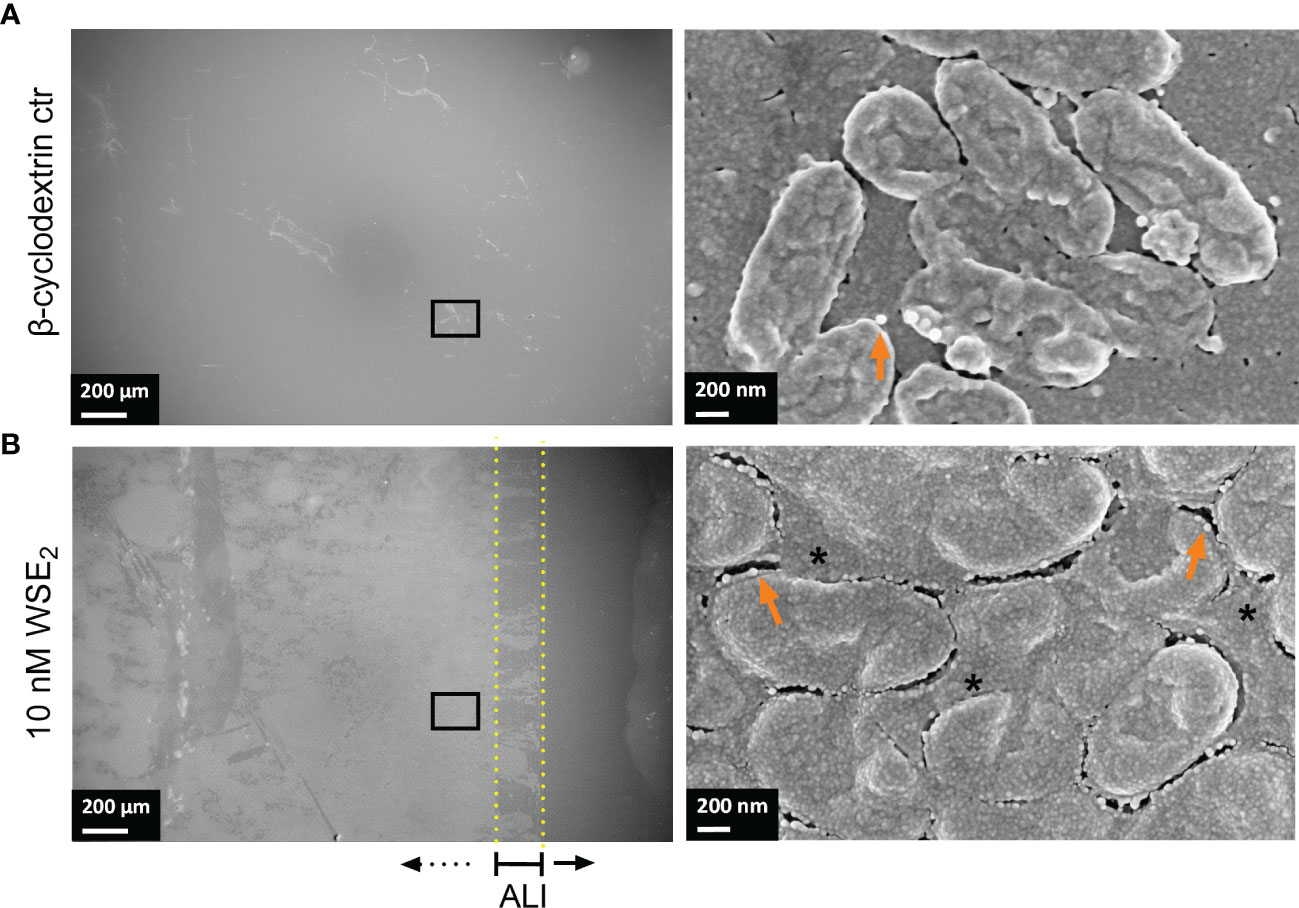
Figure 4 WSE2 strongly induces growth of attached PAH biofilm. Overview of exemplary PAH biofilms grown in 50 nM β-cyclodextrin (CD) as solvent controls (A) or 10 nM WSE2 (B). Biofilm samples were cut out from PVC well plates and analyzed via FE-SEM. Black rectangle selection indicates area chosen for further magnification to visualize single bacteria (right). ALI: Air-liquid-interface (yellow dotted line, not visible for A). Orange arrows: outer membrane vesicles; *: compact EPS. Scale bar: 200 µm (left), 200 nm (right). Additional measurements of the sample: attached biofilm mass (Figure 2A, experiment No. 4◊), biofilm surface coverage (Supplementary Figure S6C) and height profile/roughness (Figure 7).
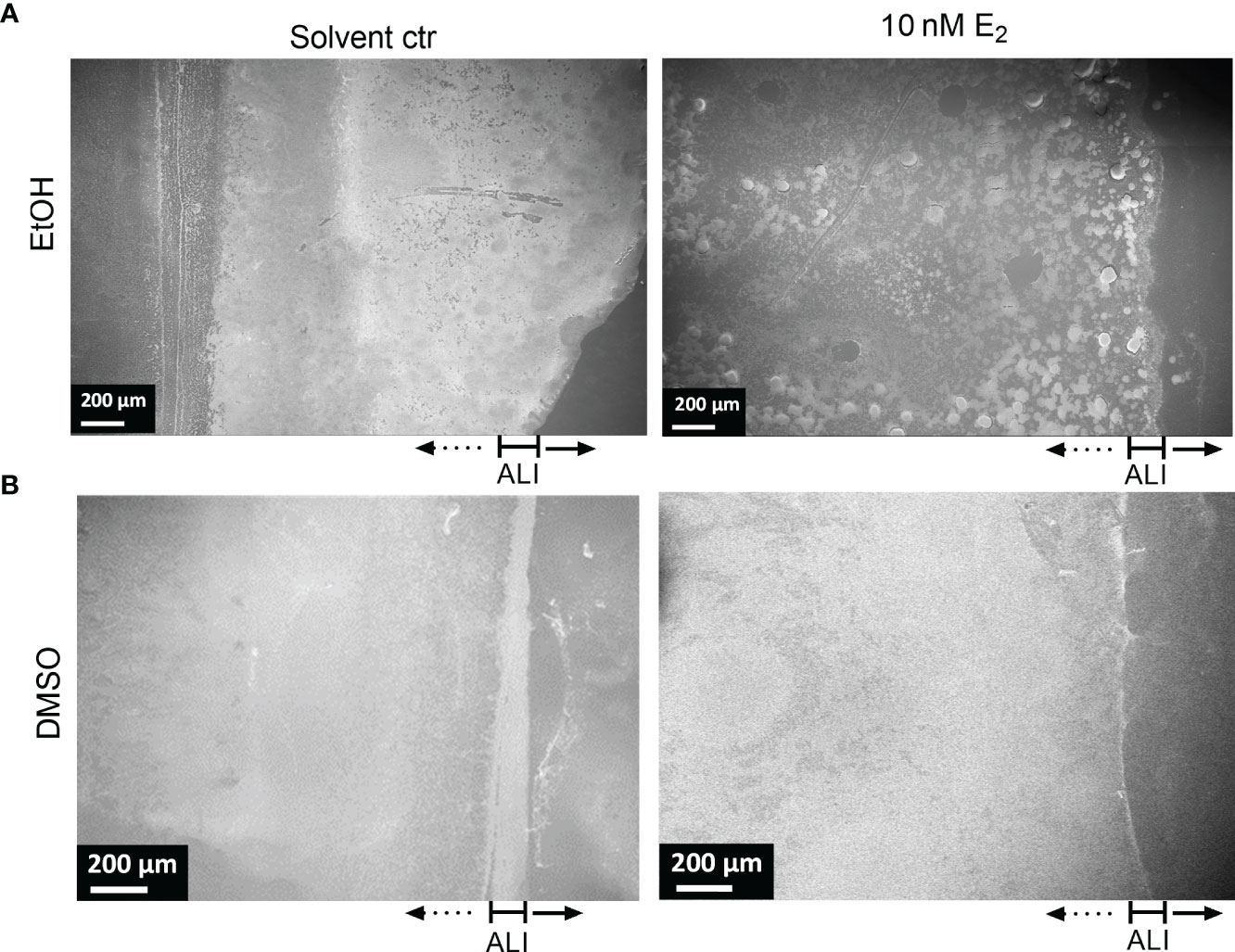
Figure 5 Structure of PAH biofilms treated with E2 dissolved in EtOH and DMSO. Overview of exemplary PAH biofilms treated with either 10 nM E2 in EtOH (A, right) or in DMSO (B, right) in comparison to the corresponding solvent controls (left). Biofilm samples were cut out from PVC well plates and analyzed via FE-SEM. ALI, Air-liquid interface. Scale bar: 200 µm. Additional measurements of the sample: attached biofilm mass (Figure 2A, experiment No. 4◊), biofilm surface coverage (Supplementary Figure S6) and height profile/roughness (Figure 7).
The most obvious structural difference of the overall PAH biofilm was observed for E2 in EtOH. Throughout the overall biofilm, but especially at the air-liquid interface, a structural difference toward prominent microcolonies were observed compared to the more homogeneously grown biofilm under solvent control conditions (Figure 5).
Also, such a tendency of bacterial agglomeration into microcolonies embedded in a complex network of EPS could be detected for PAH biofilms grown on transwell insert substrates under the influence of E2 dissolved in EtOH (Supplementary Figure S7). Treatment with E2 dissolved in DMSO resulted in a different distribution of the overall biomass. For the DMSO control biofilm density was higher at the air-liquid interface (Figure 5B, left) while the E2 treated biofilm seemed to build up a dense biofilm including submerged areas of the well substrate (Figure 5B, right). Similar observations were reflected by isolate MHH17767 that was also accompanied by the appearance of elongated cells (Supplementary Figure S10). This influence of E2 dissolved in DMSO on the overall biofilm growth is underpinned by a slight increase of the biofilm-covered area based on the image analysis of SEM pictures, where E2 in DMSO induced a higher coverage area compared to control condition for PAH and C21-C2 (Supplementary Figure S6).
The highest magnification with single bacteria resolution illustrates the surface pattern of bacteria and differences in the EPS covering the bacteria and filling the extracellular gaps between bacteria (Figures 4, 6). This can be clearly differentiated between the WSE2 and the β-cyclodextrin control condition for PAH biofilms. For the control condition only single aggregates of few bacteria disconnected via EPS are visible, while bacteria within the dense biofilm at the air-liquid interface are embedded in a homogeneously distributed EPS (Figure 4, right) similar to the PAH biofilms treated with E2 dissolved in EtOH or DMSO (Figure 6, lower row).
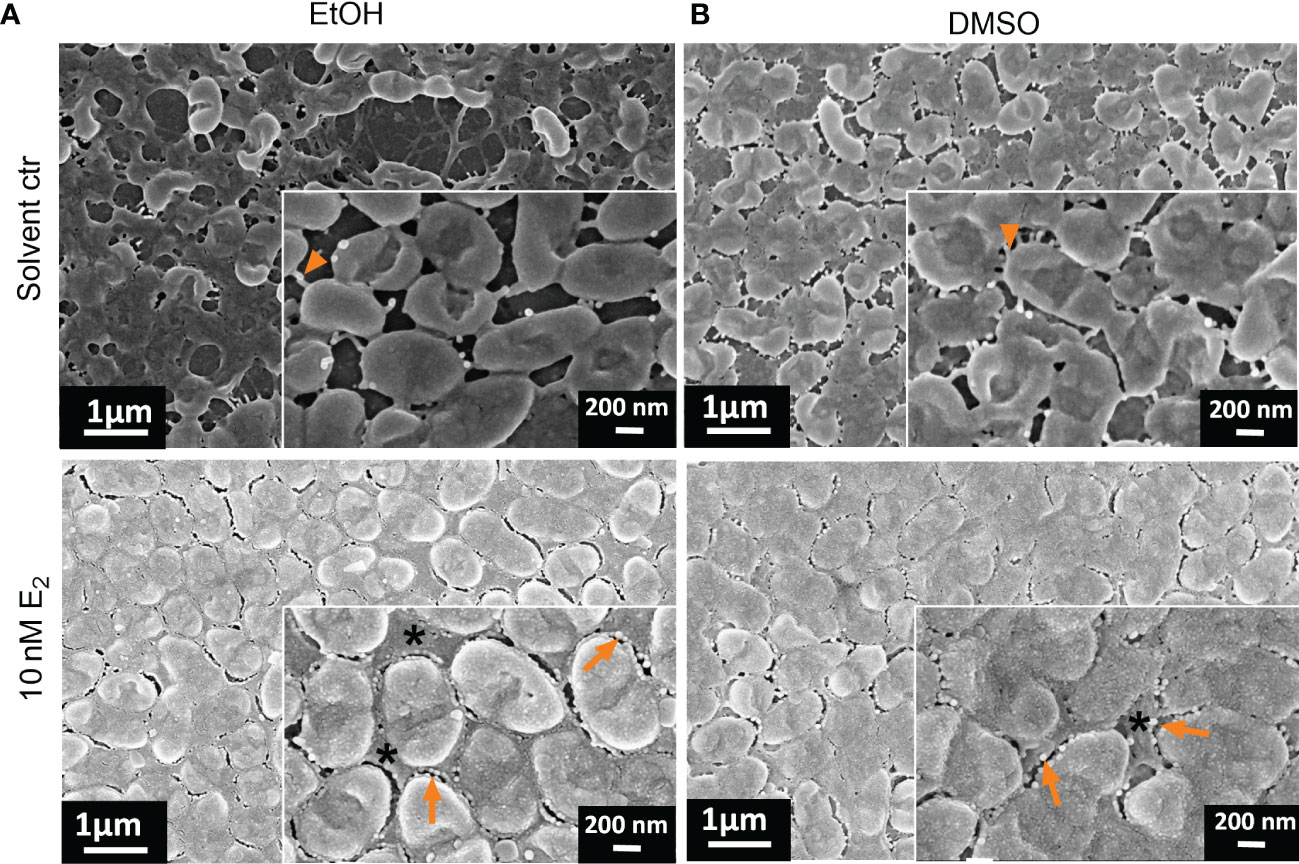
Figure 6 Ultrastructural changes of PAH biofilms treated with E2 dissolved in EtOH or DMSO. Overview of exemplary PAH biofilms treated with either 10 nM E2 in EtOH (A, down) or in DMSO (B, down) in comparison to the corresponding solvent controls [(A, B) up]. Biofilm samples were cut out and analyzed via FE-SEM. Arrowheads: visible bacterial cell-cell connecting appendages; arrows: outer membrane vesicles; *: compact EPS; scale bar: 200 µm; scale bar inserts: 200 nm. Additional measurements of the sample: biofilm mass (Figure 2A, experiment No. 4◊), biofilm surface coverage (Supplementary Figure S6) and height profile/roughness (Figure 7).
This EPS is so condensed that visible defined extracellular bacterial cell surface appendages probably reflecting pili and cup fimbriae or amyloid fibrils under solvent control conditions (Figure 6, arrowhead in magnified inserts) are not visible anymore after E2 treatment, where the intercellular space between the bacteria seemed to be homogenously filled with EPS embedding the bacteria.
Apart from PAH we also analyzed the E2 responsive isolate C21-C2, where FE-SEM analysis did not reveal any ultrastructural changes aside a slight increase of bacterial extracellular appendages that were more visible after treatment with E2 dissolved in DMSO compared to DMSO control (Supplementary Figure S8B). However, the lab strain PAO1 that already exhibited a strong biofilm forming phenotype under control conditions, showed some slight structural changes upon E2 treatment (Supplementary Figure S9) although attached biofilm mass was unchanged (Figure 3B). Especially, under treatment with E2 in EtOH and WSE2 bacterial appendages mediating cell-cell connections seemed to degenerate and form more extracellular vesicles compared to control.
Next, we analyzed the structure of PAH biofilms with the same gold-sputtered samples using 3D LSM to gain insights in the 3D structure, height profile and roughness, which confirmed the E2 induced changes in biofilm structure. Considering the height profile, it turned out that E2 dissolved in EtOH induced the formation of prominent microcolonies with a height of around 10 µm, indicating that these colonies contain several layers of bacteria (Figure 7).
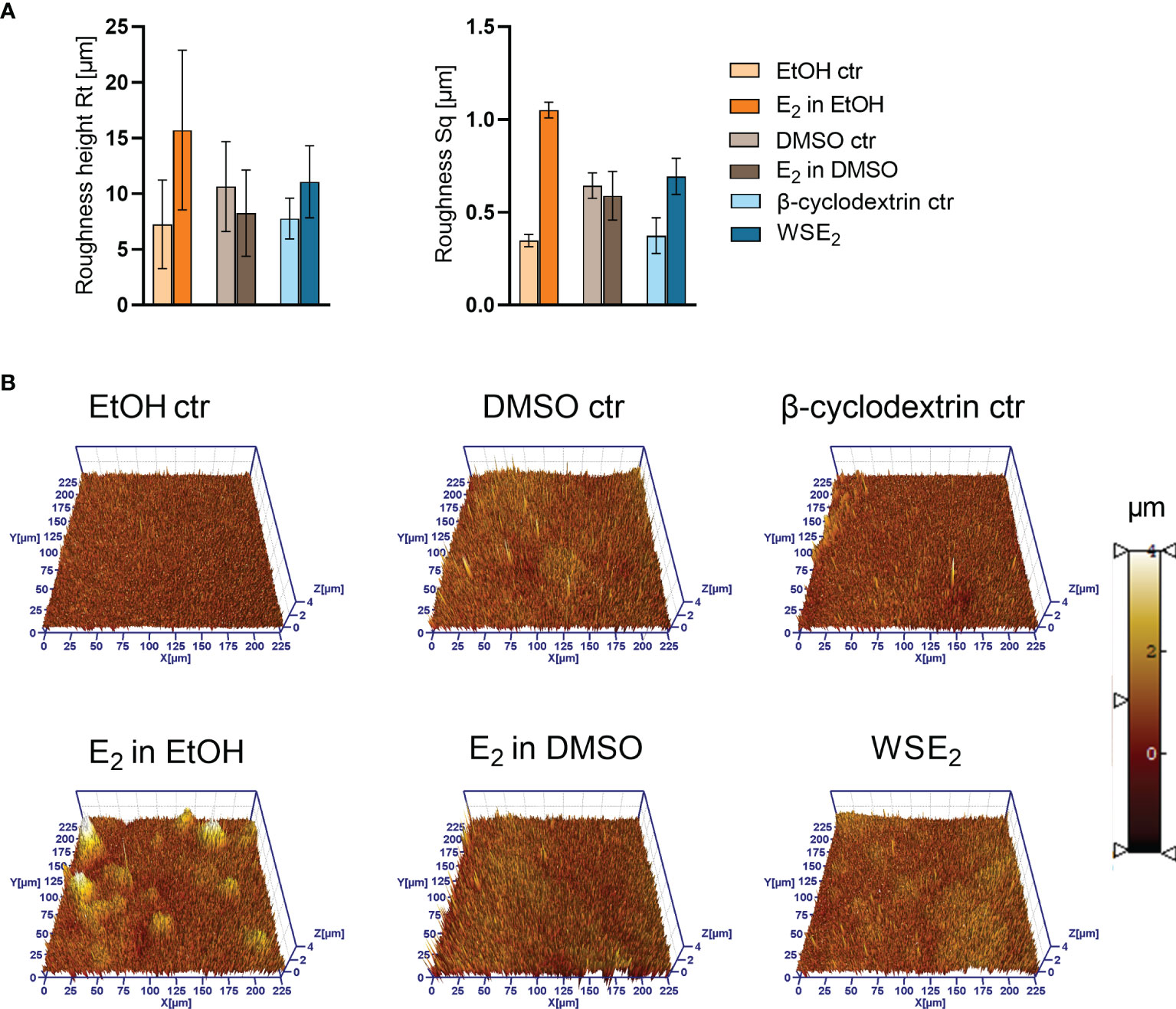
Figure 7 Height profile and roughness of PAH biofilms is changing upon E2 treatment as measured via 3D LSM. Same samples have been analyzed via FE-SEM as indicated in Figures 5, 6. A Roughness height Rt (A, left) and RMS (root mean square average roughness Sq) (A, right) have been determined based on the three 3D LSM pictures (example shown in B). Compare overview pictures in Supplementary Figure S11).
The roughness measurement revealed an increase in roughness for PAH biofilms treated with E2 in EtOH or WSE2, indicating a rearrangement of the biofilm architecture. However, when considering the resolution at the applied magnification on level of bacterial aggregates, this finding may also indicate a remodeling of the EPS.
E2 modulates Quorum Sensing Activity in E2 Responsive CF Isolates
Since QS plays a major role in the control of biofilm formation (Brindhadevi et al., 2020), we further collected supernatants from P. aeruginosa biofilms treated with E2 or the corresponding solvent control. QS activity was evaluated using the bioluminescence reporter strains E. coli pSB401 and E. coli pSB1075 as AHL biosensors (Winson et al., 1998), respectively, that have different sensitivity and responsiveness to long chain and short chain AHLs as illustrated in the Supplementary Figure S12. QS activity of supernatants from non-treated control biofilms of CF isolates PAH and C21-C2 was indicated by both reporter bacteria with C21-C2 supernatant resulting in a 60 times stronger luminescence signal for the pSB401 reporter strain than with PAH, which might be a hint to a higher concentration of short length AHLs (Supplementary Figure S12D).
Due to the prominent QS activity of C21-C2 biofilms measured by the pSB401 reporter bacteria, we investigated supernatants of biofilms treated with solvent control or E2 and found a prominent upregulation of QS activity on average two times higher for E2 in EtOH compared to EtOH control. In comparison WSE2 rather tends to reduce QS activity compared to β-cyclodextrin (Figure 8).
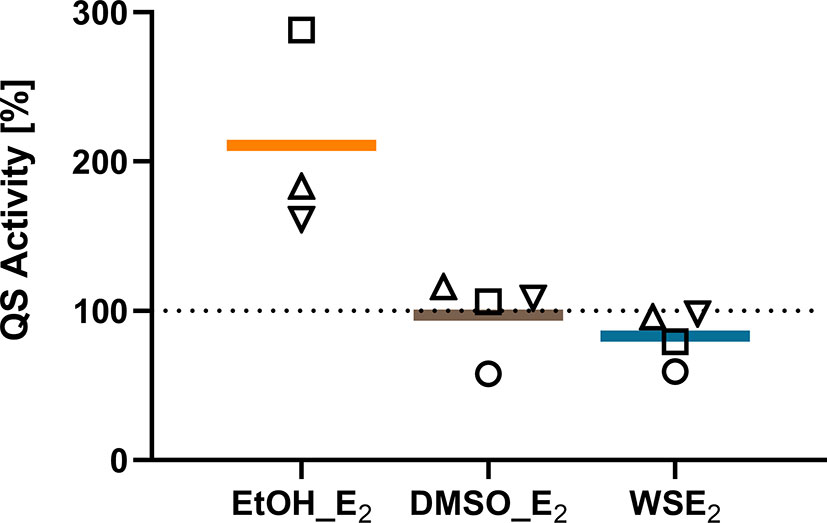
Figure 8 QS activity of C21-C2 biofilm supernatants measured by the luminescence reporter bacterium E. coli pSB401 is induced by E2 in EtOH. QS activity of supernatants from C21-C2 biofilms treated with 10 nM E2 in EtOH (orange), E2 in DMSO (brown), or WSE2 (blue) is compared relative to corresponding solvent control, which was set to 100% (dotted line). Each symbol represents an independent performed biofilm experiment with supernatants collection as biological replicate (○ = experiment 1, □ = experiment 2, ▵ = experiment 3). QS reporter assays have been performed for each supernatant and condition in technical triplicates.
Similar but less prominent effects on QS activity could be measured with the pSB1075 for C21-C2. However, PAH seems to be less responsive to QS activity changes, and QS regulation was just detected for WSE2 with the reporter pSB401 (Supplementary Figure S13).
Discussion
Microbial endocrinology is an emerging field relevant for understanding the impact of host environmental factors like hormones and how they influence infection progression on host mucosal surfaces (Neuman et al., 2015; Vemuri et al., 2019). With the recent insights into sex differences regarding the outcome of chronical lung diseases, specifically CF (Lam et al., 2021), sex steroid hormones moved into the spotlight of this research field (Silveyra et al., 2021). In the present work, the in vitro effects of E2 on P. aeruginosa biofilms were investigated on a panel of 10 clinical isolates each coming from a different CF patient (Supplementary Table S1).
We herein report a series of data characterizing possible effects toward P. aeruginosa biofilm growth. Considering the attached biofilm mass, the herewith investigated CF isolates can be divided into E2 responders or non-E2 responders. We found in the applied biofilm model that the attached biofilm mass is increased via E2 for six out of 10 analyzed CF isolates (Figure 2, Table 1, and Supplementary Figure S5). It turned out that the extent of this biofilm inducing effect is underlying high variations among the clinical isolates, which exhibits diverse phenotypes and growth characteristics on blood agar plates (see Supplementary Table S2; Supplementary Figures S1-2) but also differences in biofilm ultrastructure indicated via FE-SEM and 3D LSM analysis of this study (Figures 4-7).
Three E2 responsive CF isolates that showed an increase in biofilm mass (PAH, C21-C2, and MHH17767) were microscopically analyzed via FE-SEM. Strong structural differences between E2 treatment and corresponding control have been observed especially for PAH, therefore, these biofilms were analyzed additionally via 3D LSM to measure height profiles and surface roughness. WSE2 treatment condition revealed the strongest E2 effect indicated not only via CV assay results with over 17 times more attached biofilm mass (Figure 2A, experiment No. 4◊) but also seven times higher surface coverage (Supplementary Figure S6C). Thus, E2 could recover biofilm formation compared to the β-cyclodextrin control (Figure 4).
For EtOH a structural change of PAH biofilms toward growth into microcolonies was observed, which reduced homogeneity of the biofilm and enhanced microcolony thickness as well as surface roughness (Figure 7). Simultaneously, the number of bacteria between these microcolonies was reduced (Figure 5A). This was confirmed also on transwell insert substrates, which mediate slightly different biofilm growth conditions than PVC plates (Supplementary Figure S7). The visible E2 triggered change in morphotype might result from an early process during the initial steps of biofilm development. In other studies, it was reported that E2 influences the mobility of P. aeruginosa in a semi-solid medium, meaning the swarming behavior, as well as the movement on surfaces, called twitching behavior (Tyrrell and Harvey, 2020). These mobility modes are also determining biofilm phenotype and involve type IV pili, which can repetitively extend and retract to promote movements (Otton et al., 2017). A modulation of swarming or twitching behavior may therefore result in a migration of bacteria toward microcolonies (Rossi et al., 2018).
Other bacterial appendages that are not certainly distinguishable via FE-SEM from type IV pili are curli fibers (fab-based amyloid fibrils), which can enhance biofilms mechanically and protect them from desiccation (Erskine et al., 2018). Such bacterial appendages could be clearly seen under solvent control conditions for PAH in the intercellular space. After E2 treatment they disappeared due to degeneration or abundant filling of the intercellular space with EPS (Figure 6 and Supplementary Figure S7), which is likely composed of Pseudomonas specific exopolysaccharides such as Pel, Psl, and alginate or DNA (Wei and Ma, 2013).
According to Chotirmall et al., E2 can induce alginate production in P. aeruginosa after prolonged exposure in vitro. Based on the Irish Cystic Fibrosis Registry this study further revealed that P. aeruginosa found in CF patients undergoes two times more frequent mucoid conversion in female than male patients (Chotirmall et al., 2012).
This observation could be an explanation for the observed homogenous extracellular filling of PAH biofilm with EPS found in our study, although CF isolates were exposed for shorter time here.
Due to the complex composition of EPS such a hypothesis of EPS remodeling has to be further proven biochemically. In this regard, one needs to emphasize that an overproduction of alginate cannot be considered responsible for the increase of attached biofilm mass for all E2 responsive CF isolates investigated in this study because polysaccharide production can influence biofilm development but is dispensable for biofilm formation (Stapper et al., 2004).
Similar effects regarding the reduction of bacterial surface appendages after E2 treatment could be observed for the strain PAO1 although attached biofilm mass of this strain did not change (Figure 3) and PAO1 showed for all conditions a thick biofilm with homogeneous surface coverage (Supplementary Figure S9). The FE-SEM analysis revealed specific changes of the outer membrane/extracellular space triggered by E2. Bacterial surface appendages like pili or curli fibers seemed to be reduced for E2 in EtOH or WSE2 while outer membrane vesicles (OMV) with a diameter of around 50 nm was prevalent, a size in line with previous reports (Mozaheb and Mingeot-Leclercq, 2020). OMVs have been also visible for PAH and after E2 treatment (Figures 4, 6). Such OMV can indicate stress and mediate transfer of resistance or virulence factors as well as dynamic structural outer membrane changes.
When comparing the morphology changes of different CF isolates, we obtained heterogeneous results. Isolate MHH17767 exhibited a remarkable compact homogenous biofilm structure with no obvious changes upon E2 treatment apart from cell elongation that could be only observed for E2 in DMSO treatment condition (Supplementary Figure S10B). Also, we observed elongated cells with the EtOH solvent control condition for C21-C2, which were not present after treatment with E2 in EtOH (Supplementary Figure S8A). According to previous reports, cell elongation of P. aeruginosa seems to be a morphological change due to missing cell division under anaerobic growth, a strategy the pathogen uses to adapt to thickened mucus layers, as found for instance in CF patient airways (Yoon et al., 2011).
Our results can be compared with previous data from the literature. First, our observations contrast with the findings by Tyrrell et al. reporting no effect of E2 on biofilm growth of CF isolates (Tyrrell and Harvey, 2020). However, it is worth mentioning that (i) this previous study focused on two CF isolates collected from a single patient at different time of the disease progression and (ii) some CF isolates were also identified as non-E2 responders in our study (Supplementary Figure S5). Beside this, we did not find any induction of biofilm attachment for the reference strains PAO1 and ATCC19660 (Figure 3) as reported before (Tyrrell and Harvey, 2020). Such differences cannot only be caused by a slight difference in biofilm models and growth conditions but also deviating E2 treatment conditions, starting from the concentration of stock solution and corresponding final EtOH concentration in this case, what they used as the only condition to solve E2. Also, the different character of ATCC19660 with septicemia from a very different host environment may be one explanation for the opposite biofilm reducing response to E2 (Schook et al., 1976).
The mechanisms that are transducing the E2 effect are still not clarified. Therefore, we further tried to gain insights by investigating the planktonic growth as a driving force but also QS as a switching mechanism. The slight increase in total planktonic growth for PAH or the slightly reduced doubling time of MHH17767 (Supplementary Figures S4A, B) cannot alone explain the observed strong increase in attached biofilm mass (Figure 2). Building up on existing knowledge about the impact of the QS system on mucoid switch but also on biofilm formation dynamics of P. aeruginosa (Tahrioui et al., 2019; Brindhadevi et al., 2020), we performed QS assays indicating a different extent of QS activity amongst the tested CF isolates PAH and C21-C2 (Supplementary Figure S12D). Moreover, we obtained first indications for an E2 triggered mechanism via the QS system for CF isolate C21-C2, which showed increased QS activity after treatment with 10 nM E2 in EtOH (Figure 8). In contrast, another group showed that supraphysiological concentrations of E2 (367 µM), which are 3.7 x 10^4 times higher than the concentration used in this study, can inhibit the QS system of the non-CF lab strain PAO1 via binding to the LasR receptor and thereby interfering with QS (Beury-Cirou et al., 2013). This suggests that non-physiological, supraphysiological concentrations compared to the here used concentration (10 nM), which is closer to physiological E2 blood serum concentrations (0.1-1.6 nM) (Chotirmall et al., 2012), can result in very different outcomes. Apart from the E2 concentration aspect, differences in basal QS activities between the highly variable CF isolate and the non-CF strains PAO1 (Supplementary Table S1) used in this previous study may add to the observed discrepancy.
Due to the high variability of E2 response of CF isolates, it still has to be investigated in depth, which genetic background responsive CF isolates have in common, and which preconditioned morphotype is most susceptible for E2. Especially the switch from low adhesive to strong biofilm forming bacteria as well as the remodeling of the EPS that we could observe via FE-SEM needs to be uncovered in future studies. Also, the detailed mechanism of how E2 interacts with P. aeruginosa, whether it is directly mediated via an estradiol binding receptor, indirect effects of central signaling pathways (QS, c-GMP, etc.), or side products of the E2 metabolism are involved, is still an open question. Beside this, the high polymorphism reported in this study may imply that the basic starting condition and phenotype of the CF isolates are critical with respect to the effect of E2 on biofilm growth. These variations are not surprising, because phenotypes are known to be highly polymorphic amongst CF isolates and non-CF strains like PAO1 (Mowat et al., 2011; Thöming et al., 2020; Camus et al., 2021; Jurado-Martín et al., 2021). Especially for CF, it is known that P. aeruginosa undergoes evolutionary processes in the course of an infection and can adapt its phenotype to the host’s environment (Bragonzi et al., 2009; Fischer et al., 2021).
Strikingly, all six E2 responsive CF isolates were resistant toward at least two out of five antibiotic classes, PAH and C21-C2, two highly responsive CF isolates, that have been investigated in more detail in this study, lack susceptibility toward four out of five antibiotics (Supplementary Table S2). Due to the high prevalence of multi-drug resistant P. aeruginosa in the CF lung, more data are needed to prove if there is a correlation between E2 responsiveness and multi-drug resistance. Also, a small colony variants (SCVs) phenotype (Häußler et al., 1999) could precondition the impact of E2 on biofilm growth, when we consider that most of the E2 responsive CF isolates show a small colony morphotype
Finally, some methodical limitations of this study need to be mentioned. First, floating P. aeruginosa aggregates in the biofilm supernatant, which are physiologically relevant in CF regarding susceptibility toward antibiotics (Jennings et al., 2021), have not yet been investigated. Second, the solvent effect may have a strong impact and our results emphasize the relevance of the chosen condition how E2 is dissolved in in vitro biofilm models. Variations between the effect of different treatment conditions may be partly caused by possible side effects of the solvent used. Although the lowest solvent concentrations possible have been used in this work, there are certain reported effects EtOH (Lewis et al., 2019), DMSO (Singh et al., 2021), and β-cyclodextrin (Ikeda et al., 2002; Oishi et al., 2008) possibly have. Third, the here used E2 concentration was chosen for comparability with previous in vitro studies (Chotirmall et al., 2012; Tyrrell and Harvey, 2020) and was around 10 times higher than reported physiological concentrations in CF-sputum (Chotirmall et al., 2012), which need to be addressed by concentration gradients.
Although showing some relevance, it is obvious that the in vitro model used in this study is very simplified compared with in vivo settings in CF lung airways. It is noteworthy that this study rather considered the endpoint of the biofilm life cycle. Further complementary analysis of different biofilm formation stages could provide additional insights into the E2 action mode. Considering host parameters, this model may be further implemented (e.g., with adjacent lung epithelial cells, immune cells, patient mucus, and resident lung microbiota) in order to reproduce bidirectional pathogen/host crosstalk, involving inter-kingdom signaling molecules including hormones. It is likely that ‘host imprinting effects’ (i.e., the in vitro persistence of bacteria responsiveness to E2 due to in vivo specific conditioning host parameters) can also contribute to the variations observed. Broadly speaking, in vitro microbiology should be further accompanied by clinical studies about the medical relevance of the microbial endocrine effects of E2 on P. aeruginosa biofilms, E2 concentrations in the lung, and thereby confirm the relevance of new insights of a direct E2 effect on P. aeruginosa biofilm on the outcome of P. aeruginosa infection in CF. Chotirmall et al. did an extensive statistical analysis of the Irish CF patient registry showing that patients taking hormonal contraceptives had strikingly fewer pulmonary exacerbations and required less antibiotic treatment (Chotirmall et al., 2012). A complete understanding of the impact of the patient’s hormonal status and application of hormonal contraceptives on lung pathogens like P. aeruginosa could lead to a final clear advice regarding hormonal medications of CF patients that can make a game-changing difference in disease outcome. However, this study adds to few other studies that explicitly focused on the impact of E2 on biofilm formation. In the context of other bacterial pathogens like uropathogenic Escherichia coli, it was shown that biofilm formation and the overall virulence trait was increased when applying 5 pg/ml E2 (Engelsöy et al., 2021).
In summary, this study further emphasizes the complexity of the multifaceted hormonal E2 effects on P. aeruginosa biofilm growth, which is likely to be better addressed thanks to an integrated approach as that used in the present work. From this point of view, this study should be considered as a starting point for further investigation of the underlying mechanisms and qualitative changes of E2 modulated clinically relevant P. aeruginosa biofilms. Our findings provide one further tentative explanation for the sexual dimorphism in bacterial infections associated with biofilm formation in CF and other chronical respiratory diseases associated with P. aeruginosa lung infections.
Data Availability Statement
The original data supporting the findings of this study are included in the article/Supplementary Material. Further information can be provided by the corresponding author.
Author Contributions
JA: investigation (most of experimental work), methodology, data analysis and curation, manuscript drafting, final revision. FA: methodology, investigation, data analysis (SEM and growth curve analysis). AA: investigation, data analysis (FE-SEM). DN: investigation (SEM sample preparation), data analysis; AK: investigation (3D LSM), DW: data analysis and curation (3D LSM). TM: funding acquisition and manuscript revision. TLG: methodology, data interpretation, manuscript writing-revision and editing and funding acquisition. MM: Conceptualization, data interpretation, manuscript drafting/final revision and funding acquisition. All authors gave their specific input to the manuscript according to their contribution and finally approved the manuscript.
Funding
Mukoviszidose Institut gGmbH, Bonn, the research and development arm of the German Cystic Fibrosis Association Mukoviszidose e.V., the Christiane Herzog foundation; the University of Siegen (in particular the Open Access Publishing Fund, the Equality Office and the EaSE-2020 Fund); DAAD PPP Frankreich (Project-ID 55976814); the Hans-Böckler foundation; Ministère de l’Europe et des Affaires Etrangères (MEAE) and Ministère de l’Enseignement Supérieur, de la Recherche et de l’Innovation (MESRI; PHC PROCOPE 2017, Project no. 37733UM).
Conflict of Interest
The authors declare that the research was conducted in the absence of any commercial or financial relationships that could be construed as a potential conflict of interest.
Publisher’s Note
All claims expressed in this article are solely those of the authors and do not necessarily represent those of their affiliated organizations, or those of the publisher, the editors and the reviewers. Any product that may be evaluated in this article, or claim that may be made by its manufacturer, is not guaranteed or endorsed by the publisher.
Acknowledgments
The authors thank Susanne Häußler (University Hospital Copenhagen, Denmark; HZI, Braunschweig, Germany) and Toby Jenkins (University of Bath, UK) for kindly providing clinical CF isolates and the PAO1 strain, respectively, Bodo Philipps (Westphalian Wilhelms University Münster, Germany) for providing the luminescent AHL reporter bacteria, Qasim Alhusaini for help with FE-SEM measurements, and Hans Merzendorfer, Holger Schönherr (both University of Siegen, Germany) and Janne G. Thöming (University Hospital Copenhagen, Denmark) for valuable stimulating scientific discussions and methodological advice. Parts of this work have been presented at 44th European Cystic Fibrosis Conference, 2021 and the biofilms online conference, 2020.
Supplementary Material
The Supplementary Material for this article can be found online at: https://www.frontiersin.org/articles/10.3389/fcimb.2022.941014/full#supplementary-material
Abbreviations
3D LSM, Three-dimensional laser scanning microscopy; AHL, Acyl homoserine lactone; CV, Crystal violet; CD, β-cyclodextrin; CF, Cystic fibrosis; CF isolates, Pseudomonas aeruginosa clinical isolates from cystic fibrosis patients; CPM, Counts per minute; Ctr, Control; cGMP, Cyclic guanosine monophosphate; DMSO, Dimethyl sulfoxide; E2, Estradiol; EPS, Extracellular polymeric substance; FE-SEM, Field emission scanning electron microscopy; h, hour; OMV, Outer membrane vesicles; QS, Quorum sensing; RT, Room temperature; Rt, Roughness height; s, seconds; Sq, Average roughness; WSE2, Water soluble estradiol.
References
Abid, S., Xie, S., Bose, M. (2017). 17β-Estradiol Dysregulates Innate Immune Responses to Pseudomonas aeruginosa Respiratory Infection and Is Modulated by Estrogen Receptor Antagonism. Infect. Immun. 85, 1–15. doi: 10.1128/IAI.00422-17
Babatunde, A., Mar, A., Aung, M., Kim, I. S. (2018). Correlation Between Quorum Sensing Signal Molecules and Pseudomonas aeruginosa ‘ s Biofilm Development and Virulency. Curr. Microbiol. 75, 787–793. doi: 10.1007/s00284-018-1449-5
Bell, S. C., Mall, M. A., Gutierrez, H., Macek, M., Madge, S., Davies, J. C., et al. (2020). The Future of Cystic Fibrosis Care ;: A Global Perspective. Lancet Respir. Med. 8, 65–124. doi: 10.1016/S2213-2600(19)30337-6
Berchel, M., Le Gall, T., Denis, C., Le Hir, S., Quentel, F., Elléouet, C., et al. (2011). A Silver-Based Metal-Organic Framework Material as a “Reservoir” of Bactericidal Metal Ions. New J. Chem. 35, 1000–1003. doi: 10.1039/c1nj20202b
Beury-Cirou, A., Tannières, M., Minard, C., Soulère, L., Rasamiravaka, T., Dodd, R. H., et al. (2013). At a Supra-Physiological Concentration, Human Sexual Hormones Act as Quorum-Sensing Inhibitors. PloS One 8(12):e83564. doi: 10.1371/journal.pone.0083564
Bhagirath, A. Y., Li, Y., Somayajula, D., Dadashi, M., Badr, S., Duan, K. (2016). Cystic Fibrosis Lung Environment and Pseudomonas aeruginosa Infection. BMC Pulm Med. 16, 174. doi: 10.1186/s12890-016-0339-5
Blackledge, M. S., Worthington, R. J., Melander, C. (2013). Biologically Inspired Strategies for Combating Bacterial Biofilms. Curr. Opin. Pharmacol. 13, 699–706. doi: 10.1016/j.coph.2013.07.004
Boudarel, H., Mathias, J. D., Blaysat, B., Grédiac, M. (2018). Towards Standardized Mechanical Characterization of Microbial Biofilms: Analysis and Critical Review. NPJ Biofilms Microbiomes 4, 17. doi: 10.1038/s41522-018-0062-5
Bragonzi, A., Paroni, M., Nonis, A., Cramer, N., Montanari, S., Rejman, J., et al. (2009). Pseudomonas aeruginosa Microevolution During Cystic Fibrosis Lung Infection Establishes Clones With Adapted Virulence. Am. J. Respir. Crit. Care Med. 180, 138–145. doi: 10.1164/rccm.200812-1943OC
Brindhadevi, K., LewisOscar, F., Mylonakis, E., Shanmugam, S., Verma, T. N., Pugazhendhi, A. (2020). Biofilm and Quorum Sensing Mediated Pathogenicity in Pseudomonas aeruginosa. Process Biochem. 96, 49–57. doi: 10.1016/j.procbio.2020.06.001
Camus, L., Vandenesch, F., Moreau, K. (2021). From Genotype to Phenotype: Adaptations of Pseudomonas aeruginosa to the Cystic Fibrosis Environment. Microb. Genomics 7(3): mgen000513. doi: 10.1099/mgen.0.000513
Chotirmall, S., Smith, S., Gunaratnam, C., Cosgrove, S., Ph, D., Dimitrov, B., et al. (2012). Effect of Estrogen on Pseudomonas Mucoidy and Exacerbations in Cystic Fibrosis. N. Engl. J. Med. 24, 1978–1986. doi: 10.1056/NEJMoa1106126
Cystic Fibrosis Trust (2020). UK Cystic Fibrosis Registry 2019 Annual Data Report Cystic Fibrosis Registry.
Demko, C. A., Byard, P. J., Davis, P. B. (1995). Gender Differences in Cystic Fibrosis: Pseudomonas aeruginosa Infection. J. Clin. Epidemiol 48, 1041–1049. doi: 10.1016/0895-4356(94)00230-N
Engelsöy, U., Svensson, M. A., Demirel, I. (2021). Estradiol Alters the Virulence Traits of Uropathogenic Escherichia coli. Front. Microbiol. 12. doi: 10.3389/fmicb.2021.682626
Erskine, E., Macphee, C. E., Stanley-wall, N. R. (2018). Functional Amyloid and Other Protein Fibers in the Biofilm Matrix. J Mol Biol 430(20): 3642–3656. doi: 10.1016/j.jmb.2018.07.026
Fischer, S., Klockgether, J., Gonzalez Sorribes, M., Dorda, M., Wiehlmann, L., Tümmler, B. (2021). Sequence Diversity of the Pseudomonas aeruginosa Population in Loci That Undergo Microevolution in Cystic Fibrosis Airways. Access Microbiol. 3(12):000286. doi: 10.1099/acmi.0.000286
Freestone, P. (2013). Communication Between Bacteria and Their Hosts. Scientifica (Cairo) 2013, 1–15. doi: 10.1155/2013/361073
Fu, Y., Peng, W., Lin, S., Deng, Z., Liang, R. (2022). The 3-Oxoacyl-(Acyl-Carrier-Protein) Reductase HSD-X1 of Pseudomonas Citronellolis SJTE-3 Catalyzes the Conversion of 17β-Estradiol to Estrone. Protein Pept. Lett. 29(3):199–207. doi: 10.2174/0929866529666220113140721
Guo, Q. Q., Zhang, W.B., Zhang, C., Song, Y. L., Liao, Y. L., Ma, J. C., et al. (2019). Characterization of 3-Oxacyl-Acyl Carrier Protein Reductase Homolog Genes in Pseudomonas aeruginosa PAO1. Front. Microbiol. 10. doi: 10.3389/fmicb.2019.01028
Harness-Brumley, C. L., Elliott, A. C., Rosenbluth, D. B., Raghavan, D., Jain, R. (2014). Gender Differences in Outcomes of Patients With Cystic Fibrosis. J. Women’s Heal. 23, 1012–1020. doi: 10.1089/jwh.2014.4985
Häußler, S., Tümmler, B., Weißbrodt, H., Rohde, M., Steinmetz, I. (1999). Small-Colony Variants of Pseudomonas aeruginosa in Cystic Fibrosis. Clin. Infect. Dis. 29, 621–625. doi: 10.1086/598644
Hornischer, K., Khaledi, A., Pohl, S., Schniederjans, M., Pezoldt, L., Casilag, F., et al. (2019). BACTOME - a Reference Database to Explore the Sequence- and Gene Expression-Variation Landscape of Pseudomonas aeruginosa Clinical Isolates. Nucleic Acids Res. 47, D716–D720. doi: 10.1093/nar/gky895
Ikeda, T., Inoue, Y., Suehiro, A., Ikeshoji, H., Ishida, T., Takiguchi, N., et al. (2002). The Effects of Cyclodextrins on Autoinducer Activities of Quorum Sensing in Pseudomonas aeruginosa. J. Incl. Phenom 44, 381–382. doi: 10.1023/A:1023052810463
Imberti, R., Garavaglia, M. L., Verduci, I., Cannavale, G., Balduzzi, G., Papetti, S., et al. (2018). Antiestrogen- and Tamoxifen-Induced Effects on Calcium-Activated Chloride Currents in Epithelial Cells Carrying the ΔF508-CFTR Point Mutation. Respir. Res. 19, 198. doi: 10.1186/s12931-018-0901-1
Jamal, M., Ahmad, W., Andleeb, S., Jalil, F., Imran, M., Nawaz, M. A., et al. (2018). Bacterial Biofilm and Associated Infections. J. Chin. Med. Assoc. 81, 7–11. doi: 10.1016/j.jcma.2017.07.012
Jennings, L. K., Dreifus, J. E., Reichhardt, C., Storek, K. M., Secor, P. R., Wozniak, D. J., et al. (2021). Pseudomonas aeruginosa Aggregates in Cystic Fibrosis Sputum Produce Exopolysaccharides That Likely Impede Current Therapies. Cell Rep. 34, 108782. doi: 10.1016/j.celrep.2021.108782
Jurado-Martín, I., Sainz-Mejías, M., McClean, S. (2021). Pseudomonas aeruginosa: An Audacious Pathogen With an Adaptable Arsenal of Virulence Factors. Int. J. Mol. Sci. 22, 1–37. doi: 10.3390/ijms22063128
Khaledi, A., Weimann, A., Schniederjans, M., Asgari, E., Kuo, T., Oliver, A., et al. (2020). Predicting Antimicrobial Resistance in Pseudomonas aeruginosa With Machine Learning-Enabled Molecular Diagnostics. EMBO Mol. Med. 12:e10264. doi: 10.15252/emmm.201910264
Lam, G. Y., Goodwin, J., Wilcox, P. G., Quon, B. S. (2021). Sex Disparities in Cystic Fibrosis: Review on the Effect of Female Sex Hormones on Lung Pathophysiology and Outcomes. ERJ Open Res. 7, 00475–02020. doi: 10.1183/23120541.00475-2020
Le Gall, T., Berchel, M., Le Hir, S., Fraix, A., Salaün, J. Y., Férec, C., et al. (2013). Arsonium-Containing Lipophosphoramides, Poly-Functional Nano-Carriers for Simultaneous Antibacterial Action and Eukaryotic Cell Transfection. Adv. Healthc Mater. 2, 1513–1524. doi: 10.1002/adhm.201200478
Lewis, K. A., Baker, A. E., Chen, A. I., Harty, C. E., Kuchma, S. L., O’Toole, G. A., et al. (2019). Ethanol Decreases Pseudomonas aeruginosa Flagellar Motility Through the Regulation of Flagellar Stators. J. Bacteriol. 201, 1–17. doi: 10.1128/JB.00285-19
Mottais, A., Berchel, M., Sibiril, Y., Laurent, V., Gill, D., Hyde, S., et al. (2018). Antibacterial Effect and DNA Delivery Using a Combination of an Arsonium-Containing Lipophosphoramide With an N-Heterocyclic Carbene-Silver Complex – Potential Benefits for Cystic Fibrosis Lung Gene Therapy. Int. J. Pharm. 536, 29–41. doi: 10.1016/j.ijpharm.2017.11.022
Mowat, E., Paterson, S., Fothergill, J. L., Wright, E. A., Ledson, M. J., Walshaw, M. J., et al. (2011). Pseudomonas aeruginosa Population Diversity and Turnover in Cystic Fibrosis Chronic Infections. Am. J. Respir. Crit. Care Med. 183, 1674–1679. doi: 10.1164/rccm.201009-1430OC
Mozaheb, N., Mingeot-Leclercq, M. P. (2020). Membrane Vesicle Production as a Bacterial Defense Against Stress. Front. Microbiol. 11. doi: 10.3389/fmicb.2020.600221
Mukherjee, S., Bassler, B. L. (2019). Bacterial Quorum Sensing in Complex and Dynamically Changing Environments. Nat. Rev. Microbiol. 17, 371–382. doi: 10.1038/s41579-019-0186-5
Nährlich, L., Burkhart, M., Wosniok, J. (2020). German Cystic Fibrosis Registry Annual Data Report 2019.
Neuman, H., Debelius, J. W., Knight, R., Koren, O. (2015). Microbial Endocrinology: The Interplay Between the Microbiota and the Endocrine System. FEMS Microbiol. Rev. 39, 509–521. doi: 10.1093/femsre/fuu010
Oishi, K., Toyao, K., Kawano, Y. (2008). Suppression of Estrogenic Activity of 17β-Estradiol by β-Cyclodextrin. Chemosphere 73, 1788–1792. doi: 10.1016/j.chemosphere.2008.08.038
O’Toole, G. A. (2011). Microtiter Dish Biofilm Formation Assay. J. Vis. Exp. 47:2437. doi: 10.3791/2437
Otton, L. M., da Silva Campos, M., Meneghetti, K. L., Corção, G. (2017). Influence of Twitching and Swarming Motilities on Biofilm Formation in Pseudomonas Strains. Arch. Microbiol. 199, 677–682. doi: 10.1007/s00203-017-1344-7
Plésiat, P., Nikaido, H. (1992). Outer Membranes of Gram-Negative Bacteria are Permeable to Steroid Probes. Mol. Microbiol. 6, 1323–1333. doi: 10.1111/j.1365-2958.1992.tb00853.x
Rossi, E., Paroni, M., Landini, P. (2018). Biofilm and Motility in Response to Environmental and Host-Related Signals in Gram Negative Opportunistic Pathogens. J. Appl. Microbiol. 125, 1587–1602. doi: 10.1111/jam.14089
Rowland, S. S., Falkler, W. A., Bashirelahi, N. (1992). Identification of an Estrogen-Binding Protein in Pseudomonas aeruginosa. J. Steroid Biochem. Mol. Biol. 42, 721–727. doi: 10.1016/0960-0760(92)90113-W
Ryall, B., Carrara, M., Zlosnik, J. E. A., Behrends, V., Lee, X., Wong, Z. (2014). The Mucoid Switch in Pseudomonas aeruginosa Represses Quorum Sensing Systems and Leads to Complex Changes to Stationary Phase Virulence Factor Regulation. PLoS One. 9(5):e96166. doi: 10.1371/journal.pone.0096166
Saint-Criq, V., Harvey, B. J. (2014). Estrogen and the Cystic Fibrosis Gender Gap. Steroids 81, 4–8. doi: 10.1016/j.steroids.2013.11.023
Schook, L. B., Carrick, L., Berk, R. S. (1976). Murine Gastrointestinal Tract as a Portal of Entry in Experimental Pseudomonas aeruginosa Infections. Infect. Immun. 14, 564–570. doi: 10.1128/iai.14.2.564-570.1976
Silveyra, P., Fuentes, N., Rodriguez Bauza, D. (2021). Sex and Gender Differences in Lung Disease. Physiol. Behav. 1304:227–258. doi: 10.1007/978-3-030-68748-9_14
Singh, V. K., Almpani, M., Rahme, L. G. (2021). The Role of Common Solvents Against Pseudomonas aeruginosa-Induced Pathogenicity in a Murine Burn Site Infection Model. Microbiol. Spectr 9(1)e00233–21. doi: 10.1128/Spectrum.00233-21
Stapper, A. P., Narasimhan, G., Ohman, D. E., Barakat, J., Hentzer, M., Molin, S., et al. (2004). Alginate Production Affects Pseudomonas aeruginosa Biofilm Development and Architecture, But is Not Essential for Biofilm Formation. J. Med. Microbiol. 53, 679–690. doi: 10.1099/jmm.0.45539-0
Sutton, S., Rosenbluth, D., Raghavan, D., Zheng, J., Jain, R. (2015). Effects of Puberty on Cystic Fibrosis Related Pulmonary Exacerbations in Women Versus Men. Pediatr. Pulmonol. 49, 28–35. doi: 10.1002/ppul.22767
Sweezey, N. B., Ratjen, F. (2014). The Cystic Fibrosis Gender Gap: Potential Roles of Estrogen. Pediatr. Pulmonol. 49, 309–317. doi: 10.1002/ppul.22967
Tahrioui, A., Duchesne, R., Bouffartigues, E., Rodrigues, S., Maillot, O., Tortuel, D., et al. (2019). Extracellular DNA Release , Quorum Sensing , and PrrF1 / F2 Small RNAs are Key Players in Pseudomonas aeruginosa Tobramycin-Enhanced Biofilm Formation. NPJ Biofilms Microbiomes. 5(1):15. doi: 10.1038/s41522-019-0088-3
Tam, A., Wadsworth, S., Dorscheid, D., Man, S. P., Sin, D. D. (2014). Estradiol Increases Mucus Synthesis in Bronchial Epithelial Cells. PloS One 9, e100633. doi: 10.1371/journal.pone.0100633
Thöming, J. G., Häussler, S. (2022). Pseudomonas aeruginosa Is More Tolerant Under Biofilm Than Under Planktonic Growth Conditions: A Multi-Isolate Survey. Front. Cell. Infect. Microbiol. 12. doi: 10.3389/fcimb.2022.851784
Thöming, J. G., Tomasch, J., Preusse, M., Koska, M., Grahl, N., Pohl, S., et al. (2020). Parallel Evolutionary Paths to Produce More Than One Pseudomonas aeruginosa Biofilm Phenotype. NPJ Biofilms Microbiomes 6, 1–13. doi: 10.1038/s41522-019-0113-6
Tyrrell, J., Harvey, B. J. (2020). Sexual Dimorphism in the Microbiology of the CF ‘ Gender Gap ‘ ;: Estrogen Modulation of Pseudomonas aeruginosa Virulence. Steroids 156, 108575. doi: 10.1016/j.steroids.2019.108575
Vemuri, R., Sylvia, K. E., Klein, S. L., Forster, S. C., Plebanski, M., Eri, R., et al. (2019). The Microgenderome Revealed: Sex Differences in Bidirectional Interactions Between the Microbiota, Hormones, Immunity and Disease Susceptibility. Semin. Immunopathol. 41, 265–275. doi: 10.1007/s00281-018-0716-7
Vidaillac, C., Yong, V. F. L., Jaggi, T. K., Soh, M.-M., Chotirmall, S. H. (2018). Gender Differences in Bronchiectasis: A Real Issue? Breathe 14, 108–121. doi: 10.1183/20734735.000218
Wei, Q., Ma, L. Z. (2013). Biofilm Matrix and Its Regulation in Pseudomonas aeruginosa. Int J Mol Sci. 14(10):20983–21005. doi: 10.3390/ijms141020983
Winson, M. K., Swift, S., Fish, L., Throup, J. P., Jørgensen, F., Chhabra, S. R., et al. (1998). Construction and Analysis of luxCDABE-Based Plasmid Sensors for Investigating N-Acyl Homoserine Lactone-Mediated Quorum Sensing. FEMS Microbiol. Lett. 163, 185–192. doi: 10.1016/S0378-1097(98)00172-4
Yin, W., Wang, Y., Liu, L., He, J. (2019). Biofilms ;: The Microbial “ Protective Clothing “ in Extreme Environments. Int. J. Mol. Sci. 20(14):3423. doi: 10.3390/ijms20143423
Keywords: estradiol, Pseudomonas aeruginosa, biofilm growth, cystic fibrosis, clinical isolates, quorum sensing
Citation: Al-Zawity J, Afzal F, Awan A, Nordhoff D, Kleimann A, Wesner D, Montier T, Le Gall T and Müller M (2022) Effects of the Sex Steroid Hormone Estradiol on Biofilm Growth of Cystic Fibrosis Pseudomonas aeruginosa Isolates. Front. Cell. Infect. Microbiol. 12:941014. doi: 10.3389/fcimb.2022.941014
Received: 11 May 2022; Accepted: 08 June 2022;
Published: 13 July 2022.
Edited by:
Anders P. Hakansson, Lund University, SwedenReviewed by:
Maria V. Turkina, Linköping University, SwedenYuri Gogolev, Kazan Institute of Biochemistry and Biophysics (RAS), Russia
Copyright © 2022 Al-Zawity, Afzal, Awan, Nordhoff, Kleimann, Wesner, Montier, Le Gall and Müller. This is an open-access article distributed under the terms of the Creative Commons Attribution License (CC BY). The use, distribution or reproduction in other forums is permitted, provided the original author(s) and the copyright owner(s) are credited and that the original publication in this journal is cited, in accordance with accepted academic practice. No use, distribution or reproduction is permitted which does not comply with these terms.
*Correspondence: Mareike Müller, m.mueller@chemie-bio.uni-siegen.de