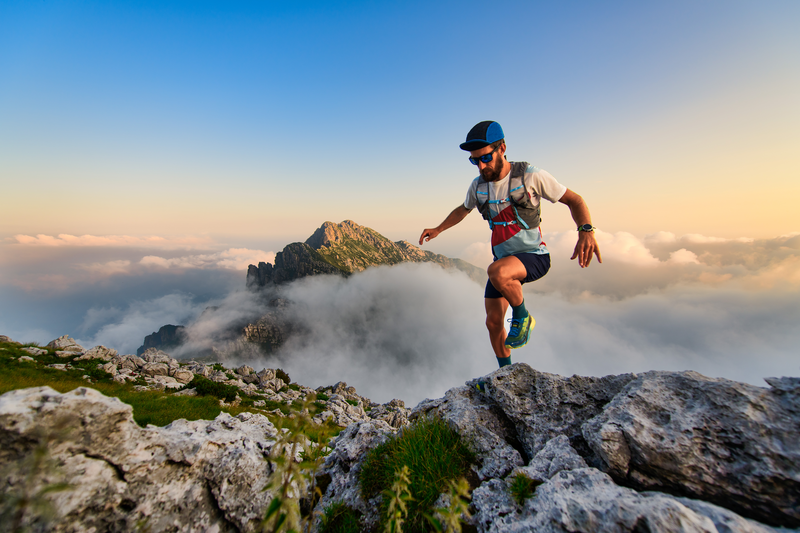
94% of researchers rate our articles as excellent or good
Learn more about the work of our research integrity team to safeguard the quality of each article we publish.
Find out more
OPINION article
Front. Cell. Infect. Microbiol. , 02 June 2022
Sec. Parasite and Host
Volume 12 - 2022 | https://doi.org/10.3389/fcimb.2022.939813
This article is part of the Research Topic Rising Stars in Parasite and Host: 2022 View all 9 articles
In the past decades, insect vector-borne parasitic diseases (VBDs), such as malaria, leishmaniasis, and lymphatic filariasis, have caused a devastating health problem, threatening for over 250 million lives worldwide (Wilson et al., 2020). While the causative parasites of VBDs together with their native insect vectors have been largely documented, much remains unknown. Global efforts are still needed to fully elucidate the parasites’ genes that are playing parts in the infectivity and mechanisms responsible in the modulation of host antiparasitic immune responses. In addition, characterization of important VBDs hallmarks and factors responsible in the vector and host susceptibility to parasitic transmission and infection remains underexplored. Once revealed, such information shall provide a vital role in the improvement of preventive actions to control the spread of VBDs and pharmacotherapeutic strategies to limit the disease burden.
Several insects such as mosquitoes, flies (sand flies, black flies, and tsetse flies), and triatomine bugs, have all been implicated in the transmission of parasites to human (Wilson et al., 2020). While much has been done in the identification of insect(s) suitable for the parasites’ life cycle prior to infection in the human, it remains challenging to carry out experimental investigations in the native insect vectors (Schneider, 2000; Zolfaghari Emameh et al., 2015). Complexities in the vector rearing and the lack of genetic toolbox available for carrying out experimental procedures in the native vectors are two of the most encountered obstacles for anyone working in this field (Schneider, 2000; Zolfaghari Emameh et al., 2015). To address this issue, the use of insect model such as fruit fly Drosophila melanogaster to study the parasitic biology and other important knowledge related to the transmission and vector-parasite interaction can be seen as one of the alternative endeavors. In this opinion paper, we would like to propose the use of D. melanogaster to study the biology, immunology, and pathogenic characteristics of human parasites, and to some extent other vertebrate parasites, to reflect the situation occurred either during parasites’ lifecycle in the vector or during their infectious phase in their definitive vertebrate host. However, it is important to note that the results obtained from the Drosophila works shall be interpreted with caution once the results are translated into either the parasites’ native vectors or their vertebrate host.
Fruit fly D. melanogaster has been proposed as one of the prominent models to uncover many aspects of infectious diseases (Harnish et al., 2021), including to unravel several inquiries related to parasite biology and vector competence (Table 1). However, the application of D. melanogaster in the field of parasitology remains strictly limited despite its huge potential. With its superior flexibility for genetic modification (Bier, 2005; Ugur et al., 2016) and high genetic similarities to other insects such as mosquitoes, sand flies, and other vector insects (Schneider, 2000; Zolfaghari Emameh et al., 2015), D. melanogaster shall allow a genetically tractable yet robust platform to assess the role of parasites’ proteins in the vector. Indeed, this insect has been extensively used in the exploration of cellular and molecular properties of human pathogens, such as bacteria and viruses, in both in vitro and in vivo conditions (Hughes et al., 2012; Nainu et al., 2020; Harnish et al., 2021). Based on such notion, it would be possible to conduct similar experiments to examine the pathological role of parasites’ proteins. This is particularly important to provide fundamental insights on the conserved pathogenic mechanisms of the parasites as well as antiparasitic immunity activated in the insect vectors. To achieve this, the use of an in vivo two-component GAL4/UAS system will provide a prompt yet efficient and economical platform to study the pathogenic function of parasites’ protein in Drosophila. The binary GAL4/UAS system is generally consist of the Gal4 gene and UAS (Upstream Activation Sequence) site. The Gal4 gene encodes Saccharomyces cerevisiae GAL4, a transcription factor that will bind to the UAS site which subsequently promote the expression of transgene of interest inserted downstream of the UAS site (Brand and Perrimon, 1993). With the help of this binary system, it is highly feasible to carry out experimental studies to investigate the pathological function of parasites’ protein at the cellular and organismal level at every developmental phases. This is particularly important to reveal how the parasites can interrupt host cellular and physiological function in the insect vectors or even, how the vectors can cope with such condition. Furthermore, the application of diverse genetic tools in Drosophila will help to reveal not only parasites’ protein but also insect cellular factors required for parasite replication. This, in the end, shall drive scientific endeavors to identify novel targets for anti-parasitic therapy by targeting the parasites’ protein or cellular factors present in the vector.
Drosophila melanogaster has several essential qualities of model organism required to address the important yet unrequited facets of VBDs, especially at the early stage of research. It has small size, rapid life cycle, and easily breed and maintained at lower cost (Pandey and Nichols, 2011). Moreover, with its relatively simple genetics, D. melanogaster offers a highly tractable solution yet economical for experiments that requires genetic modifications (Jennings, 2011). Even better, an improved list of mutant and transgenic fly lines relevant for human infectious disease models are already available upon request from multiple stock centers around the world (Pandey and Nichols, 2011; Ugur et al., 2016). Using these fly stocks, researchers may conduct an in-depth study on the function of parasites or host genes of interest in the in vitro and in vivo situations. These experimental features are surely important and advantageous, especially for researchers with limited access to animal infrastructure and funding.
Genetically, Drosophila shares more than 70% similarity with human diseased gene (Pandey and Nichols, 2011). With its close genetic and proteomic similarities to human, Drosophila might also serve as one of the prospective model organisms in the antiparasitic drug discovery. This is particularly important in the existence of ethical constraints to execute high-throughput investigational study on newly discovered drugs using vertebrate animal models. While this approach has been successfully used for the discovery of novel targets for antiviral drug candidates (Martins et al., 2016), such application remains to be seen in the antiparasitic drug discovery. Plausibly, a relatively straightforward assay to be first conducted is the proof-of-principle investigation for antiparasitic effect by using the already available drugs used in the treatment of respective VBDs. For example, one may assess whether primaquine can provide antimalarial protection to the plasmodium-infected Drosophila, similar to its effect to the human host. Once succeeds, pharmacological and toxicological assessments of new drug candidates as well as drug repurposing efforts to treat VBDs can be done using Drosophila model system.
Certainly, a fair justification needs to be made regarding the use of D. melanogaster as an insect vector model or even infected host model in the study of human parasites. Several limitations may present hurdles to the experimental applications. One of the biggest constraints is the apparent differences in the anatomical and physiological profiles between Drosophila and humans (Panayidou et al., 2014; Nainu et al., 2020), which might prevent researchers from finding the most precise answers for their research questions. However, this discrepancy also typical for other animal models, even the vertebrate (mammalian) ones. To cautiously limit such drawback, findings obtained from Drosophila studies shall be subjected for confirmation in the mammalian models prior to verification in humans. This has been done, in part, by several researchers in other relevant studies (Pandey and Nichols, 2011; Panayidou et al., 2014; Martins et al., 2016; Nainu et al., 2017), suggesting that biological functions are conserved from D. melanogaster to mammals, despite their anatomical differences. Furthermore, Drosophila lacks adaptive immune responses thus limit its application in the vaccination-related studies. However, it is worth noting that many of the Drosophila’s innate immune responses against pathogens, particularly viruses and bacteria, have been confirmed to be exist in humans (Buchon et al., 2014; Xu and Cherry, 2014; Harnish et al., 2021). Such pathogens-mediated immune responses can serve as an appropriate basis for further investigations, whether the same immune pathways also play roles in the antiparasitic immunity. Despite its limitations, Drosophila shall be a promising complementary model system to explore vector competence, parasites biology and their related behaviors inside the vector, and hopefully at the same time might provide a glimpse of evidence for uncharted antiparasitic immunity of the metazoan host. Using this approach, one may present scientific solutions and encourage the achievement of the 3rd goal of sustainable developmental goals (SDGs), improvement of healthy lives and wellbeing for all people, in an affordable and systematic manner. Nevertheless, careful considerations shall be taken when one decides to use fruit fly in their research to accurately reflect the appropriate use of scientific knowledge and potential clinical application of drug candidates.
At present, D. melanogaster has played a prominent role in the comprehensive investigation of pathogenic human viruses and bacteria, including the outcomes of infection on the metazoan host cells and scientific approaches to mitigate the infection. However, the use of D. melanogaster in the study of human parasites remain underexplored. With the accessibility of advanced yet cost-effective research toolbox in Drosophila (Pandey and Nichols, 2011; Ugur et al., 2016) and feasibility of research using human parasites (Schneider and Shahabuddin, 2000; Peltan et al., 2012; Okuda et al., 2016), much information will be gained in no time. Indeed, it is in our belief that D. melanogaster shall become a versatile model to explore less-studied topics in the field of human/veterinary parasitology. For instance, by examining the expression pattern of anti-parasitic innate immune genes in Drosophila, upon parasitic challenge, one may help to reveal the function of similar genes in the insect vector. Alternatively, Drosophila can help to reveal parasites genes and/or host factors accountable to the susceptibility of host to infection, as shown in the case of malaria, leishmaniasis, and lymphatic filariasis (Table 1). Systematic revelation of parasite pathogenic components (proteins and others) and the interactions of such components with host immunity shall lead to a better approach in diseases management and/or parasite/vector controlling. Surely, with proper research inquiries, D. melanogaster will offer a tremendous help in the life-threatening battle against VBDs.
FN designed the outline of the manuscript. FN and ES drafted the manuscript. All authors carried out the revision of the manuscript and given the approval for submission.
The authors declare that the research was conducted in the absence of any commercial or financial relationships that could be construed as a potential conflict of interest.
All claims expressed in this article are solely those of the authors and do not necessarily represent those of their affiliated organizations, or those of the publisher, the editors and the reviewers. Any product that may be evaluated in this article, or claim that may be made by its manufacturer, is not guaranteed or endorsed by the publisher.
We would like to offer our gratitude to the members of UNHAS Fly Research Group that provide tremendous help in the manuscript preparation. This study was supported by PDUPT Research Grant 2022 from the Directorate General of Higher Education, Ministry of Education, Culture, Research, and Technology, Indonesia.
Bier, E. (2005). Drosophila, the Golden Bug, Emerges as a Tool for Human Genetics. Nat. Rev. Genet. 6, 9–23. doi: 10.1038/nrg1503
Brand, A. H., Perrimon, N. (1993). Targeted Gene Expression as a Means of Altering Cell Fates and Generating Dominant Phenotypes. Development 118, 401–415. doi: 10.1242/dev.118.2.401
Brandt, S. M., Jaramillo-Gutierrez, G., Kumar, S., Barillas-Mury, C., Schneider, D. S. (2008). Use of a Drosophila Model to Identify Genes Regulating Plasmodium Growth in the Mosquito. Genetics 180, 1671–1678. doi: 10.1534/genetics.108.089748
Buchon, N., Silverman, N., Cherry, S. (2014). Immunity in Drosophila Melanogaster — From Microbial Recognition to Whole-Organism Physiology. Nat. Rev. Immunol. 14, 796–810. doi: 10.1038/nri3763
Crossgrove, K., Maina, C. V., Robinson-Rechavi, M., Lochner, M. C. (2008). Orthologues of the Drosophila Melanogaster E75 Molting Control Gene in the Filarial Parasites Brugia Malayi and Dirofilaria Immitis. Mol. Biochem. Parasitol. 157, 92–97. doi: 10.1016/j.molbiopara.2007.08.010
Dos-Santos, G. R. R. M., Fontenele, M. R., Dias, F. D. A., Oliveira, P. L. D., Nepomuceno-Silva, J. L., De Melo, L. D. B., et al. (2015). Functional Studies of TcRjl, a Novel GTPase of Trypanosoma Cruzi, Reveals Phenotypes Related With MAPK Activation During Parasite Differentiation and After Heterologous Expression in Drosophila Model System. Biochem. Biophys. Res. Commun. 467, 115–120. doi: 10.1016/j.bbrc.2015.09.110
Harnish, J. M., Link, N., Yamamoto, S. (2021). Drosophila as a Model for Infectious Diseases. Int. J. Mol. Sci. 22, 2724. doi: 10.3390/ijms22052724
Hjerrild, K. A., Jin, J., Wright, K. E., Brown, R. E., Marshall, J. M., Labbé, G. M., et al. (2016). Production of Full-Length Soluble Plasmodium Falciparum RH5 Protein Vaccine Using a Drosophila Melanogaster Schneider 2 Stable Cell Line System. Sci. Rep. 6, 30357. doi: 10.1038/srep30357
Hughes, T. T., Allen, A. L., Bardin, J. E., Christian, M. N., Daimon, K., Dozier, K. D., et al. (2012). Drosophila as a Genetic Model for Studying Pathogenic Human Viruses. Virology 423, 1–5. doi: 10.1016/j.virol.2011.11.016
Jennings, B. H. (2011). Drosophila – a Versatile Model in Biology & Medicine. Mater. Today 14, 190–195. doi: 10.1016/S1369-7021(11)70113-4
Martins, N., Imler, J.-L., Meignin, C. (2016). Discovery of Novel Targets for Antivirals: Learning From Flies. Curr. Opin. Virol. 20, 64–70. doi: 10.1016/j.coviro.2016.09.005
Nainu, F., Rahmatika, D., Emran, T. B., Harapan, H. (2020). Potential Application of Drosophila Melanogaster as a Model Organism in COVID-19-Related Research. Front. Pharmacol. 11. doi: 10.3389/fphar.2020.588561
Nainu, F., Shiratsuchi, A., Nakanishi, Y. (2017). Induction of Apoptosis and Subsequent Phagocytosis of Virus-Infected Cells as an Antiviral Mechanism. Front. Immunol. 8. doi: 10.3389/fimmu.2017.01220
Okuda, K., Tong, M., Dempsey, B., Moore, K. J., Gazzinelli, R. T., Silverman, N. (2016). Leishmania Amazonensis Engages CD36 to Drive Parasitophorous Vacuole Maturation. PLoS Pathog. 12, e1005669. doi: 10.1371/journal.ppat.1005669
Panayidou, S., Ioannidou, E., Apidianakis, Y. (2014). Human Pathogenic Bacteria, Fungi, and Viruses in Drosophila: Disease Modeling, Lessons, and Shortcomings. Virulence 5, 253–269. doi: 10.4161/viru.27524
Pandey, U. B., Nichols, C. D. (2011). Human Disease Models in Drosophila Melanogaster and the Role of the Fly in Therapeutic Drug Discovery. Pharmacol. Rev. 63, 411–436. doi: 10.1124/pr.110.003293
Peltan, A., Briggs, L., Matthews, G., Sweeney, S. T., Smith, D. F. (2012). Identification of Drosophila Gene Products Required for Phagocytosis of Leishmania Donovani. PLoS One 7, e51831–e51831. doi: 10.1371/journal.pone.0051831
Schneider, D. (2000). Using Drosophila as a Model Insect. Nat. Rev. Genet. 1, 218–226. doi: 10.1038/35042080
Schneider, D., Shahabuddin, M. (2000). Malaria Parasite Development in a Drosophila Model. Science 288, 2376–2379. doi: 10.1126/science.288.5475.2376
Tonk, M., Pierrot, C., Cabezas-Cruz, A., Rahnamaeian, M., Khalife, J., Vilcinskas, A. (2019). The Drosophila Melanogaster Antimicrobial Peptides Mtk-1 and Mtk-2 are Active Against the Malarial Parasite Plasmodium Falciparum. Parasitol. Res. 118, 1993–1998. doi: 10.1007/s00436-019-06305-x
Ugur, B., Chen, K., Bellen, H. J. (2016). Drosophila Tools and Assays for the Study of Human Diseases. Dis. Models Mech. 9, 235–244. doi: 10.1242/dmm.023762
Wilson, A. L., Courtenay, O., Kelly-Hope, L. A., Scott, T. W., Takken, W., Torr, S. J., et al. (2020). The Importance of Vector Control for the Control and Elimination of Vector-Borne Diseases. PLoS Neglect. Trop. Dis. 14, e0007831. doi: 10.1371/journal.pntd.0007831
Xu, J., Cherry, S. (2014). Viruses and Antiviral Immunity in Drosophila. Dev. Comp. Immunol. 42, 67–84. doi: 10.1016/j.dci.2013.05.002
Keywords: fruit fly, model organism, infection, parasite biology, drug discovery, drug repurposing
Citation: Nainu F, Salim E, Emran TB and Sharma R (2022) Drosophila melanogaster as a Versatile Model for Studying Medically Important Insect Vector-Borne Parasites. Front. Cell. Infect. Microbiol. 12:939813. doi: 10.3389/fcimb.2022.939813
Received: 09 May 2022; Accepted: 16 May 2022;
Published: 02 June 2022.
Edited by:
Sudhir Kumar, Seattle Children’s Research Institute, United StatesReviewed by:
Monalisa Mishra, National Institute of Technology Rourkela, IndiaCopyright © 2022 Nainu, Salim, Emran and Sharma. This is an open-access article distributed under the terms of the Creative Commons Attribution License (CC BY). The use, distribution or reproduction in other forums is permitted, provided the original author(s) and the copyright owner(s) are credited and that the original publication in this journal is cited, in accordance with accepted academic practice. No use, distribution or reproduction is permitted which does not comply with these terms.
*Correspondence: Firzan Nainu, ZmlyemFubmFpbnVAdW5oYXMuYWMuaWQ=; orcid.org/0000-0003-0989-4023
Disclaimer: All claims expressed in this article are solely those of the authors and do not necessarily represent those of their affiliated organizations, or those of the publisher, the editors and the reviewers. Any product that may be evaluated in this article or claim that may be made by its manufacturer is not guaranteed or endorsed by the publisher.
Research integrity at Frontiers
Learn more about the work of our research integrity team to safeguard the quality of each article we publish.