Corrigendum: Gut microbiota: Linking nutrition and perinatal depression
- 1Affiliated Wuhan Mental Health Center, Tongji Medical College, Huazhong University of Science and Technology, Wuhan, China
- 2Nutrilite Health Institute, Shanghai, China
- 3Jinyintan Hospital, Wuhan, China
- 4Ingredion Incorporated, Bridgewater, NJ, United States
Perinatal depression is a mood disorder that is reported in women during pregnancy (prenatal) and after childbirth (postnatal). The onset of perinatal depression is associated with changes in reproductive hormones, stress hormones and neurosteroids. These chemical compounds can be modulated by the gut microbiota, which may affect maternal mental health during the perinatal period via the gut-brain-axis. Recent studies suggest that nutritional and dietary interventions (vitamin D, ω-3 fatty acids, iron, and fiber) effectively prevent or mitigate maternal depression and anxiety, but their efficacy is confounded by various factors, including the gut microbiota. Probiotics are efficacious in maintaining microbiota homeostasis, and thus, have the potential to modulate the development of perinatal mood disorders, despite no evidence in human. Therefore, clinical trials are warranted to investigate the role of probiotic supplementation in perinatal depression and behavioral changes. This article reviews the interplay between nutrition, gut microbiota and mood and cognition, and the evidence suggesting that probiotics affect the onset and development of perinatal depression.
Introduction
Perinatal depression is defined as the onset of a major or minor depressive episode during pregnancy (antenatal depression), after childbirth (postpartum depression), or both (Leung and Kaplan, 2009). Owing to the changes in circulating biochemicals, mood disturbances have been reported in up to 80% mothers within few days of delivery (Van Niel and Payne, 2020). If untreated, perinatal depression may result into serious complications, such as premature birth, preeclampsia, maternal morbidity, and even maternal suicide (Jarde et al., 2016). Therefore, it is critical for the general public to raise awareness about perinatal depression.
The human gut harbors trillions of microorganisms that form a complex and dynamic ecosystem (Clemente et al., 2012). The gut microbiome is a crucial factor that shapes and modulates immune responses, indicated by the link between gut microbial dysbiosis and multiple diseases (Li et al., 2018; Kan et al., 2020; Metwaly et al., 2022). In addition, the gut microbiota affects mood, cognition and brain health by regulating nervous, endocrine, and immune signaling mechanisms (Dinan and Cryan, 2017) because of its ability to modulate the activity of the hypothalamic-pituitary-adrenal (HPA) axis. Furthermore, an altered gut microbial profile has been strongly associated with anxiety and depression (Bear et al., 2020). However, dietary and nutritional interventions have potent effects on reducing depression (Yang et al., 2020), where microbiota may serve as important mediators (Dahl et al., 2020).
Probiotics include live bacteria and/or yeast intended to maintain or improve the normal microflora in the gut. Although probiotic supplements are increasingly consumed during pregnancy to enhance maternal health, their effects on perinatal depression have not been reviewed. Therefore, the objective of this article was to review the role of microbiota on the interactions between diet, nutrition, and maternal mental health, and the mechanism by which probiotic intake may alleviate perinatal depression.
Nutrition and perinatal depression
Vitamin D
The prevalence of vitamin D deficiency was prevalent in women during pregnancy and in newborns is a serious concern (Bodnar et al., 2007; Dijkstra et al., 2007; Lee et al., 2007), because vitamin D is associated with hippocampal learning and memory in mothers and neural cell growth in the offspring (Liang et al., 2018; Li et al., 2021). Previous studies suggest that vitamin D acts as a neuroactive hormone and modulates the concentration of neuronal calcium ions, which regulate neuroplasticity and mood (de Abreu et al., 2009; Berridge, 2017). A meta-analysis of nine longitudinal studies with 8,470 subjects revealed a significantly inverse association between serum 25(OH)D levels and the risk of postpartum depression, with a cutoff of 50 nmol/L (Wang et al., 2018). Nonetheless, a randomized controlled trial indicated that supplementation with 2000 IU vitamin D3 from 26-28 weeks of gestation up to childbirth significantly reduced depression scores, suggesting that vitamin D supplementation during late pregnancy was beneficial for diminishing perinatal depression.
ω-3 fatty acids
Several in vivo studies suggest that ω-3 fatty acids (FA) may modulate depression. Pusceddu et al. showed that supplementation of eicosapentaenoic acid (EPA) and docosahexaenoic acid (DHA) effectively restored altered gut microbiota induced by maternal separation-related stress (Pusceddu et al., 2015). In contrast, ω-3 FA deficiency in pregnant mice resulted in increased depression and fear-induced freezing behavior in their offspring, indicating that maternal ω-3 FA availability may affect neurobehavioral and cognitive development in the fetus (Robertson et al., 2017). Two meta-analyses have reported that ω-3 FA supplementation reduced the symptoms of depression in patients with mood disorders, but exhibited limited effects on normal populations (Appleton et al., 2010; Grosso et al., 2014). Mechanistic studies showed that both EPA and DHA can prevent inflammation-induced reduction in neurogenesis and apoptosis, which is mediated by lipoxygenase and cytochrome P460 EPA/DHA metabolites in hippocampal neurons (Borsini et al., 2021).
Iron
Evidence suggest that iron deficiency is associated with psychiatric morbidity, including depression. A storehouse of iron, the basal ganglia in the brain responds rapidly to abnormal iron levels, which, in turn, affects the ability of the brain to process emotions. A recent study revealed that patients suffering from iron-deficiency anemia exhibited significantly higher incidence of sleep disorders, anxiety disorders, and depression compared to healthy controls (Lee et al., 2020). Similarly, Shariatpanaahi et al. reported that the odds of depressive symptoms was significantly increased in patients with low ferritin levels compared to healthy ones (Vahdat Shariatpanaahi et al., 2007).
Fiber
Dietary fiber is a vital component of healthy diet. A meta-analysis of four case-control studies indicated that compared to healthy controls, patients with depression exhibited significantly lower fiber intake. This investigation also pooled five cross-sectional studies and revealed that dietary fiber consumption was inversely associated with the odds of depression (Fatahi et al., 2021). However, these observational studies did not determine the causality of fiber intake and the onset of depression. Moreover, the benefits of fiber consumption against perinatal depression were increasingly evident in obese rats, whose fiber-enriched diets significantly attenuated cognitive effects and maternal depression (Liu et al., 2020). In addition, an increased dietary fiber intake has been associated with reduced depression in premenopausal, but not in postmenopausal women (Kim et al., 2021).
Fructo-oligosaccharides (FOS) and galacto-oligosaccarides (GOS) are considered as prebiotics as they selectively elevate the populations of health-promoting bacteria, whereas other fibers, which facilitate the growth of all gut bacteria, including pathogenic bacteria, are not deemed as prebiotics (Gibson et al., 2017). Inulin-type FOS have been implicated in the inhibition of mild stress-induced depression and intestinal epithelial damages by lowering corticosterone levels (Chi et al., 2020). In humans, supplementation of GOS effectively mitigated anxiety, but the effects were not clinically significant (Johnstone et al., 2021). Therefore, clinical trials are required to validate the efficacy of FOS and GOS in alleviating stress and mood disorders.
Nutrition and microbiota
The gut microbial profile can be modulated by altering lifestyle, such as nutrition and diet. As mentioned previously, the intake of vitamin D, ω-3 FA, and fiber intakes has been associated with depression. Notably, these nutrients and fiber are also involved in modulating the gut microbiota, which may consequently mediate their efficacy as anti-depressants (Table 1).
Vitamin D and ω-3 FA deficiencies have been linked to gut dysbiosis and gastrointestinal (GI) inflammation, where both vitamin D and ω-3 FA can enhance gut microbial diversity and decrease the Firmicutes to Bacteroidetes ratio (F/B ratio) (Robertson et al., 2017; Singh et al., 2020). In addition, vitamin D and ω-3 FA supplementation may promote the abundance of Bifidobacterium; vitamin D intake may increase the abundance of Akkermansia (Charoenngam et al., 2020; Singh et al., 2020), Coprococcus (Naderpoor et al., 2019), and Lactococcus (Kanhere et al., 2017) and decrease the abundance of Porphyromonas (Charoenngam et al., 2020), Ruminococcus (Naderpoor et al., 2019), Veillonella and Erysipelotrichaceae (Kanhere et al., 2017), whereas ω-3 FA may increase the abundance of Lactobacillus and decrease the abundance of Anaeroplasma, Clostridium, and Peptostreptococcaceae members, as shown in an in vivo study (Robertson et al., 2017), and enhance Bifidobacterium, Lactobacillus, and Roseburia, as shown in a clinical trial (Watson et al., 2018). The role of Firmicutes has been well-documented in the development of depression. An in vivo study reported that reduced F/B ratio was associated with depression-like behaviors in mice that underwent chronic stress (Bailey et al., 2011). Similarly, decreased abundance of Firmicutes has been associated with the progression of depression (Naseribafrouei et al., 2014; Kelly et al., 2016; Jiang et al., 2018). Moreover, lipopolysaccharide (LPS) derived from the gut microbiota are considered as major endotoxins that can trigger inflammation and systemic endotoxemia (Lin et al., 2020). Nevertheless, LPS play a complicated role in depression. Although LPS overproduction can lead to inflammation, two studies have reported that LPS produced by gut bacteria might promote immunity in depressed patients (Maes et al., 2010; Maes et al., 2012). Therefore, further studies are necessary to determine the role of LPS homeostasis in depression.
Compared to iron surplus, iron deficiency exhibited increased influence on gut microbiota dysbiosis (Rusu et al., 2020). According to a clinical trial on children, iron supplementation at a high dosage of 50 mg per day (4 days/week) for 38 weeks did not alter short chain fatty acids in the feces (Dostal et al., 2014). However, iron supplementation may increase the abundance of Enterobacteriaceae members such as the pathogenic strains of Escherichia coli. In addition, iron surplus may reduce the abundance of Bifidobacterium and Lactobacillus (Paganini and Zimmermann, 2017), whereas iron deficiency may decrease the Bifidobacteriaceae/Enterobacteriaceae ratio (Muleviciene et al., 2018). Therefore, a balanced iron intake is critical to maintain normal abundance of Bifidobacteria to address depression (Altaib et al., 2021).
Dietary fibers are either polysaccharides with a minimum of 10 sugar moieties or oligosaccharides with 3-10 sugar residues. Although fiber cannot be metabolized by humans, they can be utilized by certain species in the gut microbiota through anaerobic fermentation, thereby modulating the gut microbial profile. The final products of anaerobic fermentation include short chain fatty acids (SCFA), namely acetate, propionate, and butyrate. The supplementation of FOS resulted in reduced levels of depression-associated bacteria (e.g., Anaerostipes, Oscillibacter, Proteobacteria, and Streptococcus) and increased the abundance of the phylum Cyanobacteria, which is a group of bacteria that can produce H2S, a metabolite that exhibits antidepressant-like properties (Chi et al., 2020). Moreover, both FOS and GOS can promote the abundance of Bifidobacterium (Liu et al., 2017), while the effect of FOS in affecting butyrate-producing microbes, including Phascolarctobacterium and Ruminococcus were inconsistently reported (Liu et al., 2017; Tandon et al., 2019).
In addition to single nutrients or nutritional compounds, dietary patterns exhibited significant association with depressive symptoms. Poor diets have been reported to significantly alter the abundance and diversity of gut microbes. Several studies also suggest pathophysiological links between unhealthy diet and susceptibility of depression. Additionally, dietary patterns characterized by high consumption of processed meat, refined carbohydrates, simple sugars, high-fat dairy products (e.g., butter), and gravy and low consumption of fruits and vegetables are positively correlated with the risk of depression (Li et al., 2017). Such dietary patterns exhibit major features of a western diet that contains excessive saturated fat, simple sugars, salt, and food additives. In contrast, a multicenter, randomized, primary prevention field trial on patients with type 2 diabetes revealed that a Mediterranean diet supplemented with nuts a significantly reduced the risk of depression (Sánchez-Villegas et al., 2013).
Mechanisms of perinatal depression
Reproductive hormones
Using positron emission tomography and functional magnetic resonance imaging, researchers have revealed that gonadal steroids hormones modulate neurocircuitry, neuroplasticity, and cortical activity during normal and pathological affective states (Rubinow and Girdler, 2011; Schiller et al., 2015). Estrogen is an ovarian hormone that can modulate serotonergic functions by regulating tryptophan hydroxylase and serotonin reuptake transporter in raphe nuclei (Shors and Leuner, 2003). Additionally, reduced serotonin levels are associated with depression and arousal. The administration of an estrogen receptor (ER) antagonist to hippocampus resulted in anxiety and depression behavior in female rats. Once activated, ERs, such as ERα and Erβ, may display anxiolytic-like effects, but the onset of postpartum depression has been associated only with changes in ERα and ERα-related system (Furuta et al., 2013). In women with low estrogen levels, estrogen administration may improve mood and alleviate depressive symptoms (Gregoire et al., 1996; Ahokas et al., 2001). Nevertheless, several risks and adverse effects, such as increased production of coagulation factors and pro-inflammatory cytokines, hypertriglyceridemia, venous thromboembolism, and gallstones, are associated with long-term estrogen administration (Mehta et al., 2021).
Similar to estrogen, progesterone is a critical hormone for sexual and reproductive development in females. During pregnancy, progesterone facilitates uterus growth and lactation. Studies have also shown the ability of progesterone to stimulate GABA and brain-derived neurotrophic factor (BDNF) in the hippocampus and cerebral cortex. However, in a study by Osborne et al., progesterone levels in the second or third trimesters were not associated with the risk of postpartum depression (Osborne et al., 2017). Considering the small sample size of this study, these observations could not be verified. Thus, further large-scale studies on the role of progesterone in postpartum depression are warranted.
Oxytocin, a hormone produced in the hypothalamus and involved in childbirth and lactation, exhibited a significant inverse relationship with postpartum depression (Thul et al., 2020). Mechanistically, oxytocin modulates depression by activating a MAP kinase (MAPK) cascade, subsequently phosphorylating cAMP response element-binding protein (CREB), leading to enhanced BDNF expression (Matsuzaki et al., 2012). Furthermore, enhanced estrogen and progesterone levels increase oxytocin transcription in hypothalamus, which primarily regulates maternal behavior and lactation (Schiller et al., 2015).
Stress hormones
Cortisol, a glucocorticoid, is a key physiological inducer of stress response (Lancaster et al., 2010). In a study on 44 pregnant women, researchers observed that the hair cortisol levels during the first and third trimesters were positively correlated with pregnancy-related stress, indicating that hair cortisol levels could serve as a potential predictor or indicator of maternal depression (Caparros-Gonzalez et al., 2017). However, cortisol secretion depends on corticotropin releasing factor (CRF), which regulates the response of the HPA axis and is produced in response to real or imagined cognitive stressors (Seth et al., 2016). Moreover, CRF can stimulate the release of adrenocorticotropic hormone (ACTH), leading to the production of cortisol in the adrenal cortex. Since human placenta contains biologically active CRF, hypothalamic CRF production is increased during pregnancy, resulting in high levels of cortisol and hypercortisolemia.
Neurosteroids
Neurosteroids refer to endo- and exogenous steroids produced in the brain to regulate neuronal excitability by regulating nuclear steroid receptors (Reddy, 2010). They also play an important role in the development of mood disorders, cognitive disfunctions, and memory deficits (Ratner et al., 2019). Allopregnanolone (3α-hydroxy-5α-pregnan-20-one) is a progesterone metabolite that can enhance GABAergic signaling by interacting with the GABAA receptor. Low levels of allopregnanolone have been associated with increased risk of mood disorders, including depression during late pregnancy (Hellgren et al., 2014). Typically, the concentration of serum allopregnanolone ranges from 0.5-5 nmol/L, varying with the stages of the menstrual cycle. However, serum allopregnanolone concentration first increases to more than 10 times during the antenatal period, and then rapidly decreases to 1-2 nmol/l after parturition (Nappi et al., 2001; Pearson Murphy et al., 2001), suggesting a potential mechanism of postnatal depression.
Inflammation
Women at different trimesters of pregnancy exhibit distinct inflammatory profiles, with the first, second, and third being majorly pro-inflammatory, anti-inflammatory, and pro-inflammatory states, respectively (Mor and Cardenas, 2010). Individuals responds to inflammatory cytokines vary based on history of depression, medical history, body mass index, and psychosocial status (Sawyer, 2021). According to large-scale meta-analyses, higher levels of IL-6, IL-12, IL-13, IL-18 and TNFα were observed in patients with depression (Köhler et al., 2017a). A prospective study with a follow-up period of 12 years also suggested a predictive role of proinflammatory cytokines including C-reactive protein (CRP) and IL-6, in depression (Gimeno et al., 2009). These findings were conspicuous because pro-inflammatory cytokines have been reported to affect serotonin metabolism, increase neurodegeneration by impairing BDNF release, and interfere with monoamine, glutamate and neuropeptide metabolism, resulting in altered neurotransmitter levels and the development of mood disorders (Felger and Lotrich, 2013).
Microbiota affects depression level via the microbiota-gut-brain-axis
The microbiota-gut-brain-axis (MGBA) was identified based on the findings that linked the gastrointestinal endocrine system with alterations in neurons and brain cells (Track, 1980). Subsequently, this concept was supported by studies that reported the contribution of gut microbiota to the development of various cognitive diseases (Chang et al., 2022). Significant differences have been observed in the gut microbial profile of patients with depression and healthy controls (Naseribafrouei et al., 2014; Jiang et al., 2018). Moreover, disturbances in cognition and mood have been reported in patients with gut inflammation (Masand et al., 1995; Addolorato et al., 1997; Kurina et al., 2001). Previous in vivo studies revealed that GI inflammation induced anxiety-like behavior (less exploration and more behavioral inhibition) and altered the central nervous system (Lyte et al., 1998; Bercik et al., 2010). To determine causality, researchers leveraged germ-free (GF) animals and observed that the absence of gut microbiota promoted anxiety and neuroendocrine response to a stressful environment (Desbonnet et al., 2008; Crumeyrolle-Arias et al., 2014). Compared to GF mice, gnotobiotic mice with normal specific pathogen-free microbiota were less anxious and were more active (Neufeld et al., 2011; Nishino et al., 2013). Well-designed prospective studies have also depicted the impact of dysbiosis on mental health disorders. In both adults and infants, dose- and time-dependent associations have been reported between antibiotic usage and depression (Lurie et al., 2015; Köhler et al., 2017b; Slykerman et al., 2017b), indicating that alterations in gut microbiota may have a substantial impact on mental health disorders or behavioral changes throughout life.
Intriguingly, studies have shown that depressive symptoms can be transmitted to recipients of fecal microbiota transplantation (FMT). In a study by Zheng et al., after transplanting the fecal microbiota of depressed individuals and healthy controls in GF mice, the recipients of fecal microbiota of depressed patients exhibited more severe depressive symptoms compared to those who received the fecal microbiota of healthy subjects (Zheng et al., 2016). Similarly, Kelly et al. conducted FMT by oral gavage of the fecal microbiota from depressed patients or controls in a microbiota-deficient rat model, and reported that the recipients of fecal microbiota from depressed patients developed more severe anhedonia and anxiety-like behavior, with disrupted tryptophan metabolism. However, these alterations were not observed in the recipients of fecal microbiota from healthy controls (Kelly et al., 2016). These findings demonstrated a causal role of gut microbiota in the development of depressive symptoms.
Mechanistically, the abundance and profile of microbiota greatly influence GI health by modulating the epithelial layer and mucosa (Valdes et al., 2018). Several factors, such as medication, infection, and lifestyle (e.g., diet), can substantially alter the composition of the gut microbiota, thereby affecting GI health and the progression of various diseases (Cheng et al., 2021; Durmusoglu et al., 2021). As the gut microbiota and brain communicate through multiple routes, including the MGBA. MGBA is gaining increased attention in fields investigating mood and cognition disorders because the gut microbiota is linked to hormonal changes, which may be implicated in psychiatric disorders (Cryan et al., 2019) (Figure 1).
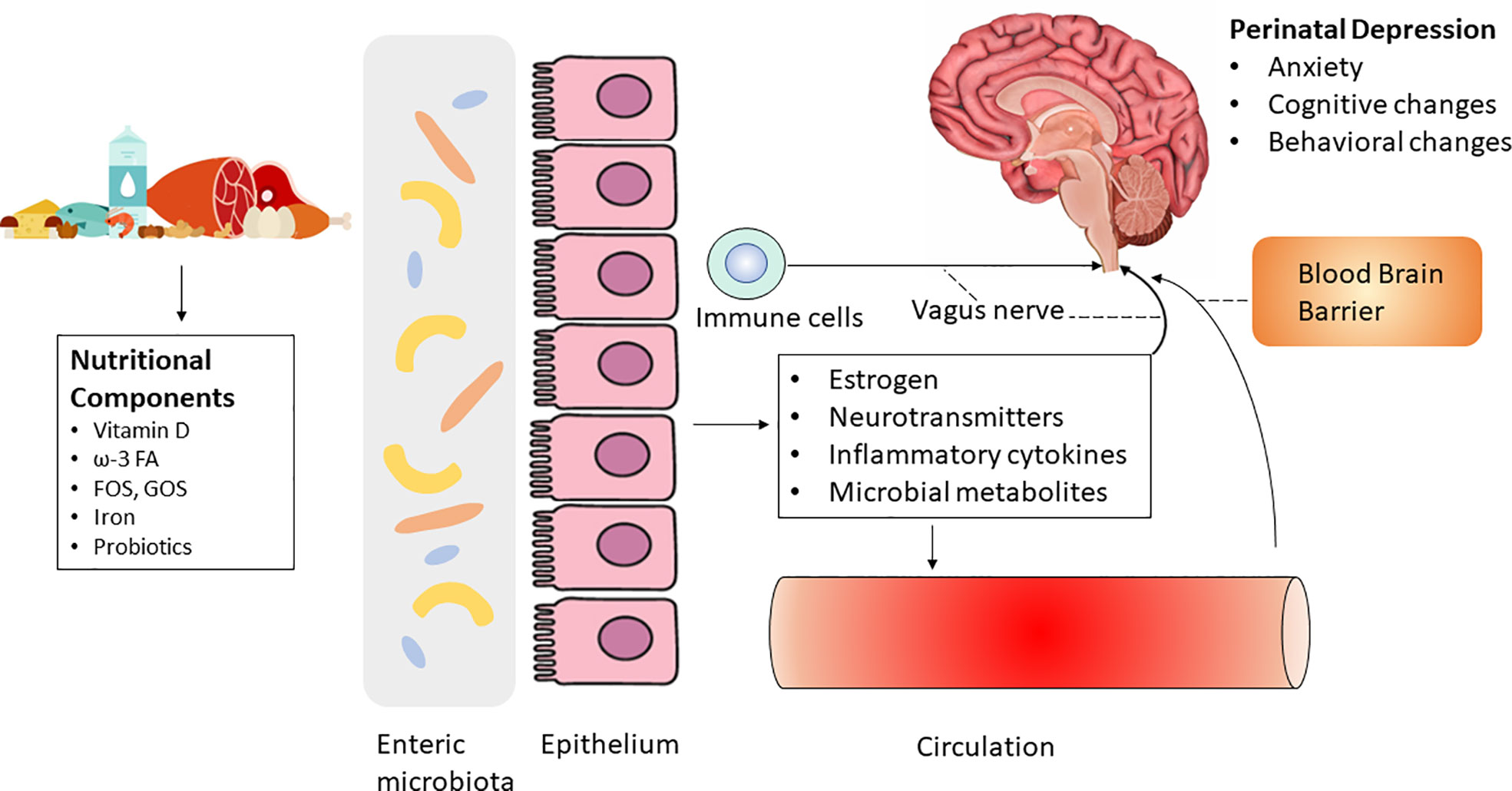
Figure 1 Diet and nutrition may regulate perinatal depression via the microbiota-gut-brain axis by influencing systemic biomarkers including estrogen, neurotransmitters, inflammatory cytokines and microbial metabolites. The role of probiotics on perinatal depression is controversial.
Estrogen
The gut microbiota has been implicated in the regulation of circulating estrogens. Mechanistically, gut microbiota regulates estrogen by secreting β-glucuronidase (GUS), an enzyme that deconjugates estrogens into their active forms (Baker et al., 2017). Till date, more than three thousand total and 279 unique microbiome-encoded microbiome-encoded β-glucuronidase (GUS) enzymes have been identified (Sui et al., 2021). However, only 60 bacterial genera in the human GI tract encode GUS, including Bifidobacterium, Lactobacillus, Roseburia, and Clostridium (Kwa et al., 2016). The microbial species that encode GUS or GUS candidates have been detailed in (Sui et al., 2021). The component of the gut microbiota expressing GUS is particularly important as these species play a role in recycling inactivated GUS by either metabolizing it and releasing the metabolites into the circulating system, or releasing the parent compound into the GI lumen (Figure 2). Thus, disruption in the gut microbiome, or dysbiosis, may reduce circulating estrogens, resulting in estrogen-mediated pathologies including cognition deficits (Sherwin, 2012).
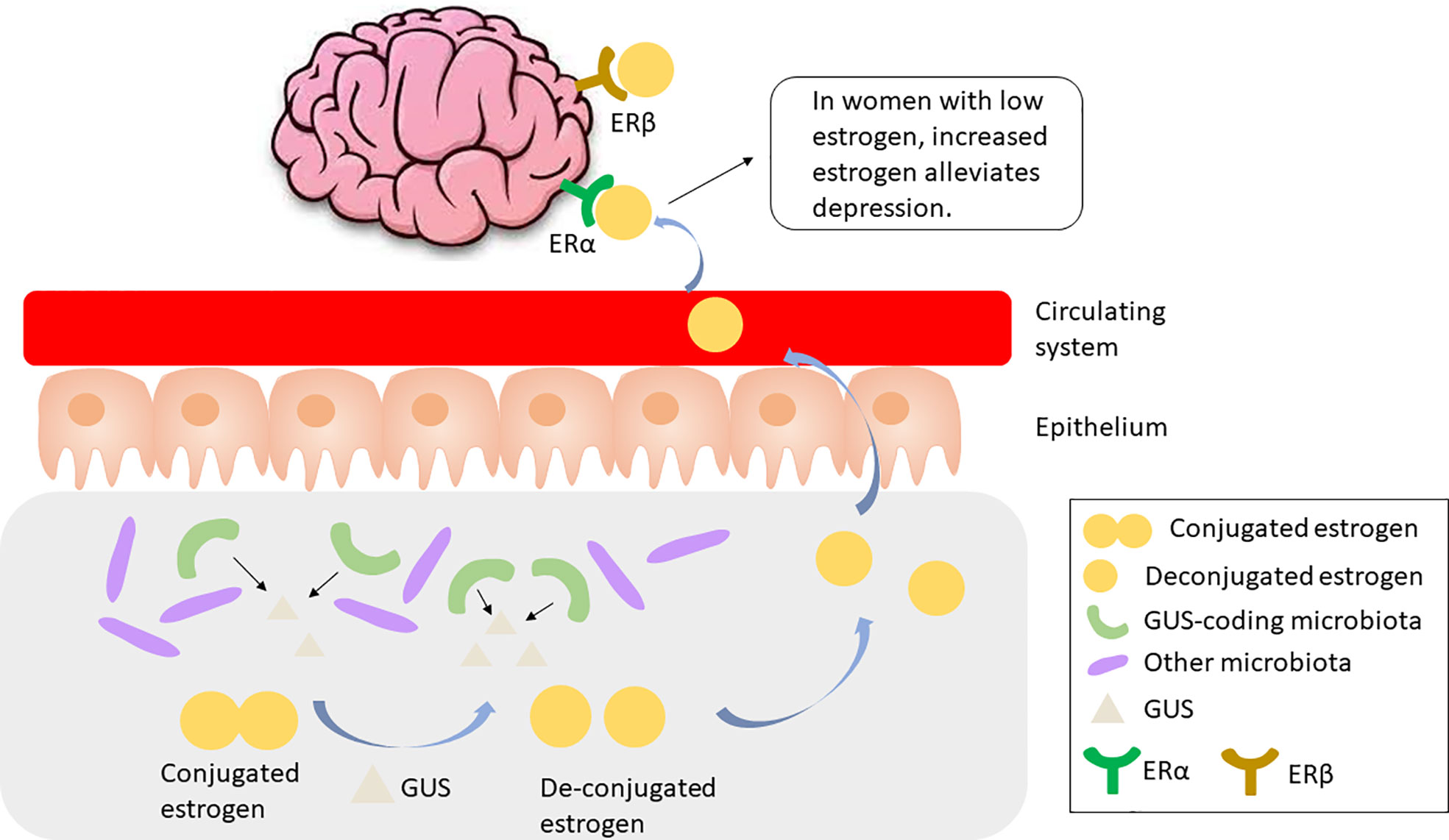
Figure 2 GUS-coding microbiota produces GUS to cleave and activate conjugated estrogen, which is released to the circulating system and binds to ERα, resulting in alleviated depression in women with low estrogen. GUS, β-glucuronidase; ER, estrogen receptor.
Neurotransmitters
Various neurotransmitters such as GABA, serotonin, glutamate, histamine, and acetylcholine (Ach), are synthesized by the gut microbiota (Oleskin et al., 2016). Several Lactobacillus species produce a wide range of neurotransmitters (Yong et al., 2019) (Table 2). GABA can be synthesized by certain Bifidobacterium species (Barrett et al., 2012), Bacteroides fragilis (Strandwitz et al., 2019), and L. plantarum (An et al., 2021). Furthermore, microbial species, including L. plantarum, B. subtilis, and S. aureus, can produce Ach (Horiuchi et al., 2003; Koussoulas et al., 2018; O’Donnell et al., 2020). In addition, Staphylococcus can synthesize and release tyramine, serotonin and tryptamine (Luqman et al., 2018) (Table 2). As neurotransmitters are transported between neurons for communicating and regulating cognition and emotion, microbiota can promote the crosstalk between the gut and brain by producing these chemical messengers.
Inflammation
The gut microbiota plays an important role in inflammatory diseases by modulating the levels of pro- and anti-inflammatory cytokines. Although microorganisms are primarily confined to the gut by the epithelial barrier, microbial metabolites can penetrate the barrier and enter this circulating system. Notably, increased circulating LPS may result in enhanced levels of TNFα, MCP-1, IL-1β, and NF-κB p65 in the brain, resulting in neuroinflammation, neurodegeneration, and behavioral changes (Qin et al., 2007). An elevated level of circulating proinflammatory cytokines may compromise the blood-brain barrier (BBB), and long-term exposure to these pro-inflammatory cytokines may also lead to neuropsychiatric disorders and depression (Felger and Lotrich, 2013). Evidence from rodent experiments explain the mechanism by which microbiota affects depression by altering inflammatory markers. Zhang et al. reported that stressed mice exhibited significantly more severe oscillopsia and reduced F/B ratio. Nonetheless, intracerebroventricular administration of an anti-mouse IL-6 receptor antibody (MR16-1) effectively restored F/B ratio and improved oscillopsia, suggesting that the interaction between inflammatory cytokines and microbiota was associated with depressive symptoms (Zhang et al., 2017). Compared to the control group, depressive patients treated with antidepressant exhibited substantially decreased Caspase-1 levels (Alcocer-Gómez et al., 2014), which cleaves IL-1β. The loss of Caspase-1 activity increased the abundance of Akkermansia spp. and restabilized the gut microbiota profile. Therefore, modulating the gut microbiota by blocking inflammatory cytokine receptors may serve as a promising therapeutic approach for depression.
Peripheral insults that result in systemic inflammation may facilitate the recruitment of peripheral immune cells to the brain and cause the overactivation of neuroimmune response, leading to neurodegenerative diseases and mood disorders (Cheng et al., 2018; Wendeln et al., 2018; Peng et al., 2022). Schiweck et al. reported a higher level of circulating T helper (Th) cells, especially Th17 cells, in the patients with severe depression (Schiweck et al., 2020). Th17 cells mainly present and polarize in the gut, exhibiting high plasticity. Microbiota dysbiosis has been reported to promote the differentiation of CD4+ T-cells into Th17 cells and their pathogenicity (Shao et al., 2020; Su et al., 2020). Although an imbalanced Th1/Th2 ratio, with increased Th1 phenotype, was observe in depressive patients (Myint et al., 2005), the induction of Th1 cytokines is mainly through parasite, not dysbiosis. In summary, microbiota dysbiosis may facilitate the development of depression by increasing the release of pro-inflammatory cytokines and impairing the Treg/Th17 balance (Figure 3).
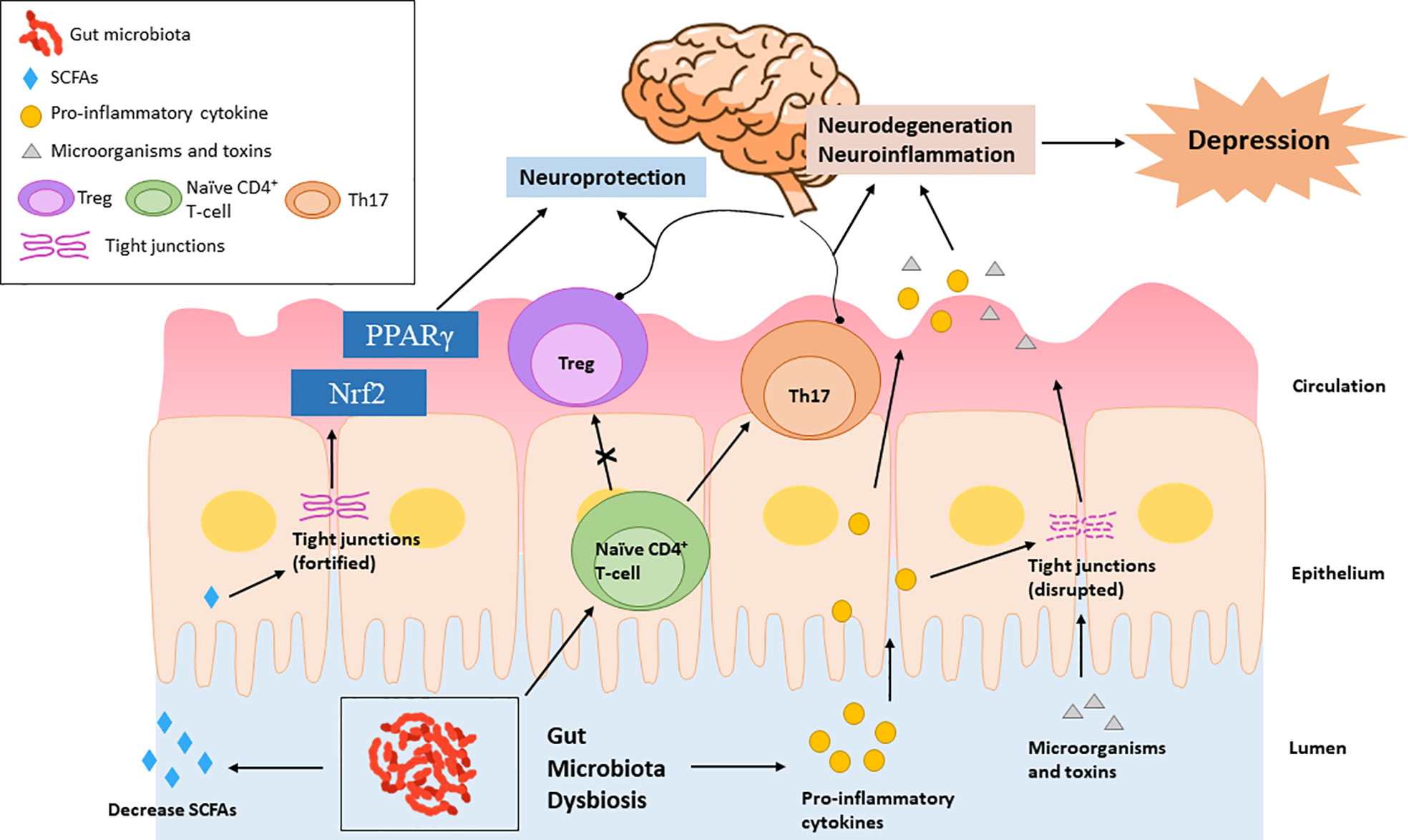
Figure 3 Microbiota dysbiosis increases pro-inflammatory cytokines, which promote the differentiation of naïve autoreactive CD4+ T-cells in Th17 cells and subsequently cause neurodegeneration. Elevated pro-inflammatory cytokines can also impair the tight junctions, leading to the intestinal transport of microorganisms and bacterial toxins, causing neuroinflammation. Microbiota dysbiosis decreases SCFAs, which exhibit neuroprotective functions by enhancing the intestinal tight junction and up-regulating the PPARγ and Nrf2 signaling pathways.
Microbial metabolites
A substantial body of evidence supports that SCFAs play a pivotal role in the development of depression. The levels of acetic acid and isocaproic acid are significantly lower in the depressive women than the matched healthy subjects (Skonieczna-Żydecka et al., 2018). Moreover, the concentrations of acetate and propionate are negatively associated with the depression score (Skonieczna-Żydecka et al., 2018), indicating that circulating SCFA levels might serve as an indicator of the severity of depression. SCFAs can be transferred through BBB (Kekuda et al., 2013; Vijay and Morris, 2014) and fortify the BBB integrity by regulating the expression of tight junction proteins (Braniste et al., 2014). Although the mechanistic evidence on how SCFAs modulate neurological disorders is not well-documented, several signaling pathways including the peroxisome proliferator-activated receptor gamma (PPARγ) pathway and the Nuclear factor erythroid 2-related factor 2 (Nrf2) redox pathway might be involved, since the activation of both signaling pathways are related to the alleviation of depression and stress (Yao et al., 2016; Gold, 2021).
Effects of probiotics on perinatal depression
Recently, probiotics have been used to modulate the gut microbiome and improve cognition, as they target the MGBA. Considering the impact of microbiota dysbiosis on mental health disorders including anxiety and depression, probiotic intake may be an effective strategy for preventing and alleviating perinatal depression.
Probiotics can modulate depression by altering the systemic biomarkers discussed in the previous section. Several in vivo studies have revealed that supplementation with Lactobacillus probiotics promotes estrogen levels and alleviates diseases resulting from reduced estrogen levels (Itoh et al., 2011; Britton et al., 2014; Guo et al., 2016). In a clinical trial on postmenopausal osteopenic women, probiotic and isoflavone aglycone supplementation significantly enhanced the ratio of urinary 2-hydroxyestrone (2-OH) to 16α-hydroxyestrone (16α-OH), favoring estrogen metabolite profile (Lambert et al., 2017). In addition to reproductive hormones, probiotics also regulate stress hormones. Yang et al. suggested that probiotic supplementation effectively enhanced serum CRF levels, thereby relieving anxiety in patients before surgery (Yang et al., 2016). Similarly, in rats, oral administration of L. farciminis substantially decreased circulating ACTH and corticosterone, as well as reduced hypothalamic CRF and pro-inflammatory cytokine expression (Ait-Belgnaoui et al., 2012). In mice, the administration of lactic acid bacteria including B. longum subsp. infantis E41 and B. breve M2CF22M7 substantially enhanced the secretion of 5-hydroxytryptophan (5-HTP) and mitigated depressive behavior (Tian et al., 2019). Furthermore, oral administration of Bifidobacterium altered serotonin metabolism in the brain stem (Desbonnet et al., 2008). Although diet is a well-known determinant of the gut microbiota profile, a study on rats indicated that probiotic supplementation markedly mitigated depression and skewed the production of pro-inflammatory cytokines, independent of diet (Abildgaard et al., 2017). These studies suggest the efficacy of probiotic supplementation in altering reproductive hormones, stress related hormones, and inflammatory cytokines, all of which are closely linked to the development of depression (Figure 4), as discussed in section 2.
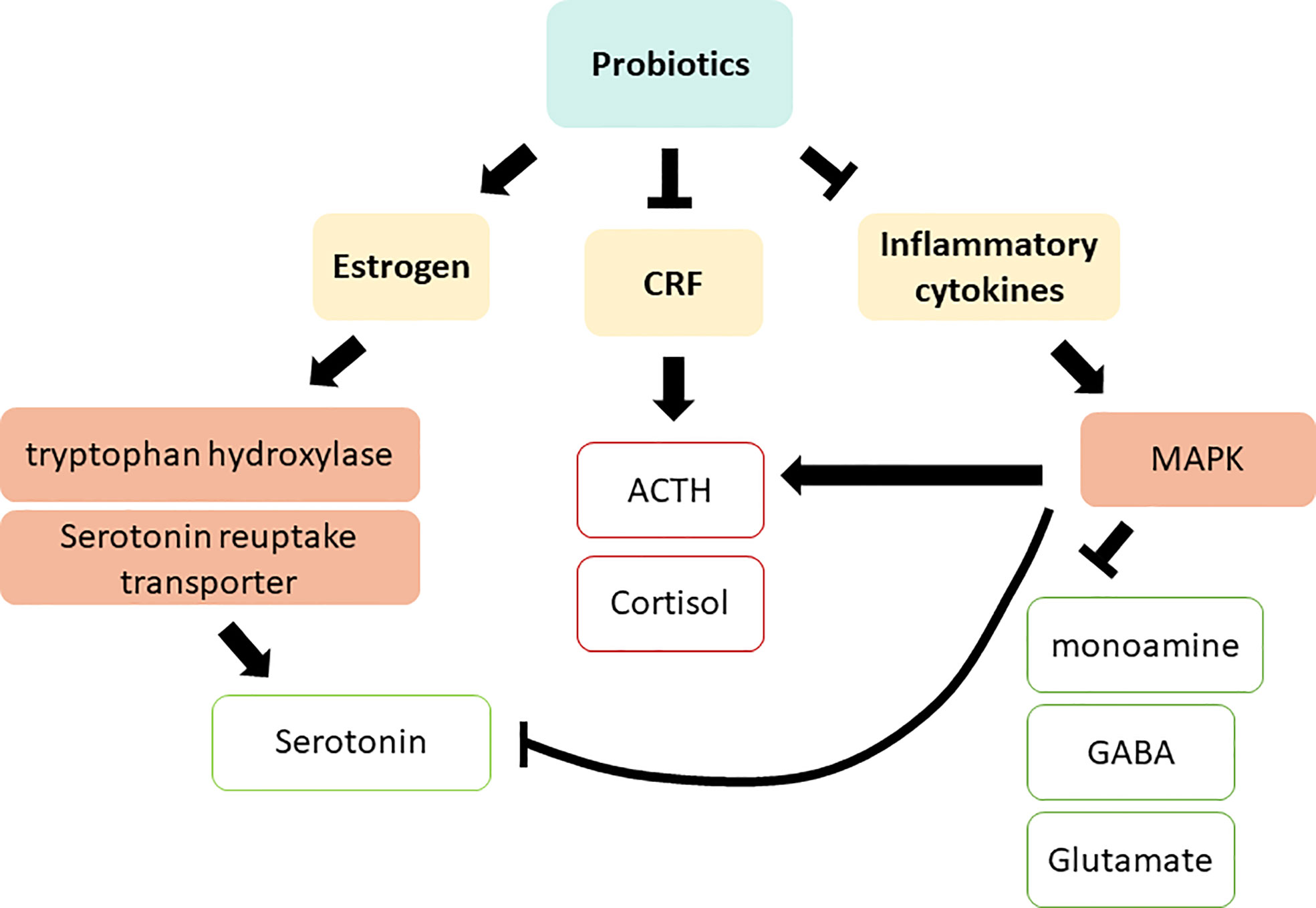
Figure 4 Action mechanisms of probiotics on depression via regulating systemic biomarkers. CRF, Corticotropin releasing factor; ACTH, adrenocorticotropic hormone; MAPK, MAP kinase; GABA, γ-Aminobutyric acid. Red squares indicate an increased level in depressive patients; green squares indicate a decreased level in depressive patients.
In humans, the consumption of a beverage high in L. casei strain Shirota, B. breve strain Yakult, L. lactis, and Streptococcus thermophilus, twice a day for 28 days significantly improved stress resilience and reduced anxiety (Kato et al., 2021). Mi et al. revealed that L. reuteri supplementation at a dose of 108 [colony-forming units (CFU)] significantly reduced infant colic and mitigated maternal depression during infantile colic (Mi et al., 2015).
Nevertheless, the effects of probiotics on mitigating perinatal depression have been controversial. A study by Slykerman et al. on 423 females, who were at 14-16 weeks pregnant and received either placebo or L. rhamnosus HN001 (6 x 109 CFU/g) from enrolment until six-months postpartum indicated that probiotic supplementation significantly reduced depression and anxiety scores during the postpartum period (Slykerman et al., 2017a). However, using the same L. rhamnosus strain, in addition to B. lactis, Dawe et al. did not observe clinically significant effects of the probiotic mixture (6.5 × 109 CFU) on anxiety and mental health scores of 230 obese pregnant women y (Dawe et al., 2020). Similarly, a recent clinical trial (n = 40) reported that the intake of a probiotic mixture of several species (2.5 × 109 CFU; B. bifidum, B. lactis, L. acidophilus, L. brevis, L. casei, L. salivarius, and L. lactis) did not effectively alleviate maternal stress and anxiety, or improve the mother-infant bonding (Browne et al., 2021). In contrast, in another clinical trial on overweight and obese women, daily probiotic supplementation (L. rhamnosus and B. animalis ssp. Lactis; each 1010 CFU) increased depression scores at 12-months postpartum (Table 3). Therefore, whether probiotics are beneficial for perinatal depression is still debatable.
Discussion
Extensive evidence suggest that nutritional and dietary interventions are associated with perinatal depression, as changes in dietary habits and nutrition can alter microbiota profile within a short period (Klimenko et al., 2018). Therefore, microbiota may modulate the efficacy of nutritional and dietary interventions on perinatal depression.
Despite sevαal studies on the mechanism by which the gut microbiota might impact mood and cognition, particularly by targeting the MGBA, the role of probiotic supplementation in alleviating perinatal depression is unclear. There is also a dearth of clinical trials describing how probiotic interventions may mitigate maternal depression, anxiety, and arousal.
Although several studies have reported the benefits of probiotic supplementation for reducing antenatal depression, they have been limited by the absence of any significant effects. Firstly, the relatively small sample size and short intervention period reduced the potential to observe a statistically significantly difference between the control and the supplemented groups. Secondly, most of the participants exhibited a high socio-economic status and healthy lifestyle (less smoking), suggesting that they might have undergone less stressful situations in daily life, and were more aware of their mental and psychological health. This might diminish the difference between the treatment and control groups.
Reports indicate that different probiotic strains may differently regulate mood and behavior. For example, colonization with B. infantis inhibited locomotor activity and did not affect anxiety levels in mice (Nishino et al., 2013; Tian et al., 2019), whereas monoassociation with Brautia coccoides significantly decreased anxiety levels, with only a slight impact on locomotor activity (Nishino et al., 2013).
In conclusion, preventing or alleviating perinatal depression by targeting the MGBA using probiotic supplements is a promising approach. However, increased studies are warranted to validate the efficacy of certain probiotic strains in antenatal and postnatal depression and depression-related behavioral changes. Thus, further investigations on this theme will enable suggesting recommendations and guidelines on probiotic supplementation to mitigate perinatal depression.
Author contributions
JS, BZ, YY and XL designed the study. JS, BZ, JK and GL reviewed literatures. SZ, LS, XZ, XY, JM, and JC extracted information. JS, BZ, JK, GL, SZ, LS and XZ wrote the first draft of the manuscript. XY, JM and JC provided figures. All the authors revised the manuscript and provided the final version of the manuscript.
Conflict of interest
Author JC was employed by company Ingredion Incorporated.
The remaining authors declare that the research was conducted in the absence of any commercial or financial relationships that could be construed as a potential conflict of interest.
Publisher’s note
All claims expressed in this article are solely those of the authors and do not necessarily represent those of their affiliated organizations, or those of the publisher, the editors and the reviewers. Any product that may be evaluated in this article, or claim that may be made by its manufacturer, is not guaranteed or endorsed by the publisher.
References
Abildgaard, A., Elfving, B., Hokland, M., Wegener, G., Lund, S. (2017). Probiotic treatment reduces depressive-like behaviour in rats independently of diet. Psychoneuroendocrinology 79, 40–48. doi: 10.1016/j.psyneuen.2017.02.014
Addolorato, G., Capristo, E., Stefanini, G., Gasbarrini, G. (1997). Inflammatory bowel disease: a study of the association between anxiety and depression, physical morbidity, and nutritional status. Scand J. Gastroenterol. 32 (10), 1013–1021. doi: 10.3109/00365529709011218
Ahokas, A., Kaukoranta, J., Wahlbeck, K., Aito, M. (2001). Estrogen deficiency in severe postpartum depression: successful treatment with sublingual physiologic 17beta-estradiol: a preliminary study. J. Clin. Psychiatry 62 (5), 332–336. doi: 10.4088/JCP.v62n0504
Ait-Belgnaoui, A., Durand, H., Cartier, C., Chaumaz, G., Eutamene, H., Ferrier, L., et al. (2012). Prevention of gut leakiness by a probiotic treatment leads to attenuated HPA response to an acute psychological stress in rats. Psychoneuroendocrinology 37 (11), 1885–1895. doi: 10.1016/j.psyneuen.2012.03.024
Alcocer-Gómez, E., de Miguel, M., Casas-Barquero, N., Núñez-Vasco, J., Sánchez-Alcazar, J. A., Fernández-Rodríguez, A., et al. (2014). NLRP3 inflammasome is activated in mononuclear blood cells from patients with major depressive disorder. Brain behavior Immun. 36, 111–117. doi: 10.1016/j.bbi.2013.10.017
Altaib, H., Badr, Y., Suzuki, T. (2021). Bifidobacteria and psychobiotic therapy: Current evidence and future prospects. Rev. Agric. Sci. 9, 74–91. doi: 10.7831/ras.9.0_74
An, J., Seok, H., Ha, E. M. (2021). GABA-producing lactobacillus plantarum inhibits metastatic properties and induces apoptosis of 5-FU-resistant colorectal cancer cells via GABA(B) receptor signaling. J. Microbiol. 59 (2), 202–216. doi: 10.1007/s12275-021-0562-5
Appleton, K. M., Rogers, P. J., Ness, A. R. (2010). Updated systematic review and meta-analysis of the effects of n– 3 long-chain polyunsaturated fatty acids on depressed mood. Am. J. Clin. Nutr. 91 (3), 757–770. doi: 10.3945/ajcn.2009.28313
Bailey, M. T., Dowd, S. E., Galley, J. D., Hufnagle, A. R., Allen, R. G., Lyte, M. (2011). Exposure to a social stressor alters the structure of the intestinal microbiota: implications for stressor-induced immunomodulation. Brain behavior Immun. 25 (3), 397–407. doi: 10.1016/j.bbi.2010.10.023
Baker, J. M., Al-Nakkash, L., Herbst-Kralovetz, M. M. (2017). Estrogen-gut microbiome axis: Physiological and clinical implications. Maturitas 103, 45–53. doi: 10.1016/j.maturitas.2017.06.025
Barrett, E., Ross, R. P., O'Toole, P. W., Fitzgerald, G. F., Stanton, C. (2012). γ-aminobutyric acid production by culturable bacteria from the human intestine. J. Appl. Microbiol. 113 (2), 411–417. doi: 10.1111/j.1365-2672.2012.05344.x
Bear, T. L. K., Dalziel, J. E., Coad, J., Roy, N. C., Butts, C. A., Gopal, P. K. (2020). The role of the gut microbiota in dietary interventions for depression and anxiety. Adv. Nutr. 11 (4), 890–907. doi: 10.1093/advances/nmaa016
Bercik, P., Verdu, E. F., Foster, J. A., Macri, J., Potter, M., Huang, X., et al. (2010). Chronic gastrointestinal inflammation induces anxiety-like behavior and alters central nervous system biochemistry in mice. Gastroenterology 139 (6), 2102–2112. e2101. doi: 10.1053/j.gastro.2010.06.063
Berridge, M. J. (2017). Vitamin d and depression: Cellular and regulatory mechanisms. Pharmacol. Rev. 69 (2), 80–92. doi: 10.1124/pr.116.013227
Bodnar, L. M., Simhan, H. N., Powers, R. W., Frank, M. P., Cooperstein, E., Roberts, J. M. (2007). High prevalence of vitamin d insufficiency in black and white pregnant women residing in the northern united states and their neonates. J. Nutr. 137 (2), 447–452. doi: 10.1093/jn/137.2.447
Borsini, A., Nicolaou, A., Camacho-Muñoz, D., Kendall, A. C., Di Benedetto, M. G., Giacobbe, J., et al. (2021). Omega-3 polyunsaturated fatty acids protect against inflammation through production of LOX and CYP450 lipid mediators: relevance for major depression and for human hippocampal neurogenesis. Mol. Psychiatry 26 (11), 6773–6788. doi: 10.1038/s41380-021-01160-8
Braniste, V., Al-Asmakh, M., Kowal, C., Anuar, F., Abbaspour, A., Tóth, M., et al. (2014). The gut microbiota influences blood-brain barrier permeability in mice. Sci. Trans. Med. 6 (263), 263ra158–263ra158. doi: 10.1126/scitranslmed.3009759
Britton, R. A., Irwin, R., Quach, D., Schaefer, L., Zhang, J., Lee, T., et al. (2014). Probiotic l. reuteri treatment prevents bone loss in a menopausal ovariectomized mouse model. J. Cell Physiol. 229 (11), 1822–1830. doi: 10.1002/jcp.24636
Browne, P. D., Bolte, A. C., Besseling-van der Vaart, I., Claassen, E., de Weerth, C. (2021). Probiotics as a treatment for prenatal maternal anxiety and depression: a double-blind randomized pilot trial. Sci. Rep. 11 (1), 3051. doi: 10.1038/s41598-021-81204-9
Caparros-Gonzalez, R. A., Romero-Gonzalez, B., Strivens-Vilchez, H., Gonzalez-Perez, R., Martinez-Augustin, O., Peralta-Ramirez, M. I. (2017). Hair cortisol levels, psychological stress and psychopathological symptoms as predictors of postpartum depression. PloS One 12 (8), e0182817. doi: 10.1371/journal.pone.0182817
Chang, L., Wei, Y., Hashimoto, K. (2022). Brain–gut–microbiota axis in depression: A historical overview and future directions. Brain Res. Bull. 182, 44–56. doi: 10.1016/j.brainresbull.2022.02.004
Charoenngam, N., Shirvani, A., Kalajian, T. A., Song, A., Holick, M. F. (2020). The effect of various doses of oral vitamin D(3) supplementation on gut microbiota in healthy adults: A randomized, double-blinded, dose-response study. Anticancer Res. 40 (1), 551–556. doi: 10.21873/anticanres.13984
Cheng, J., Balbuena, E., Miller, B., Eroglu, A. (2021). The role of β-carotene in colonic inflammation and intestinal barrier integrity. Front. Nutr. 8. doi: 10.3389/fnut.2021.723480
Cheng, Y., Desse, S., Martinez, A., Worthen, R. J., Jope, R. S., Beurel, E. (2018). TNFα disrupts blood brain barrier integrity to maintain prolonged depressive-like behavior in mice. Brain behavior Immun. 69, 556–567. doi: 10.1016/j.bbi.2018.02.003
Chi, L., Khan, I., Lin, Z., Zhang, J., Lee, M. Y. S., Leong, W., et al. (2020). Fructo-oligosaccharides from morinda officinalis remodeled gut microbiota and alleviated depression features in a stress rat model. Phytomedicine 67, 153157. doi: 10.1016/j.phymed.2019.153157
Clemente, J. C., Ursell, L. K., Parfrey, L. W., Knight, R. (2012). The impact of the gut microbiota on human health: an integrative view. Cell 148 (6), 1258–1270. doi: 10.1016/j.cell.2012.01.035
Crumeyrolle-Arias, M., Jaglin, M., Bruneau, A., Vancassel, S., Cardona, A., Daugé, V., et al. (2014). Absence of the gut microbiota enhances anxiety-like behavior and neuroendocrine response to acute stress in rats. Psychoneuroendocrinology 42, 207–217. doi: 10.1016/j.psyneuen.2014.01.014
Cryan, J. F., O'Riordan, K. J., Cowan, C. S. M., Sandhu, K. V., Bastiaanssen, T. F. S., Boehme, M., et al. (2019). The microbiota-Gut-Brain axis. Physiol. Rev. 99 (4), 1877–2013. doi: 10.1152/physrev.00018.2018
Dahl, W. J., Rivero Mendoza, D., Lambert, J. M. (2020). Diet, nutrients and the microbiome. Prog. Mol. Biol. Transl. Sci. 171, 237–263. doi: 10.1016/bs.pmbts.2020.04.006
Dawe, J. P., McCowan, L. M. E., Wilson, J., Okesene-Gafa, K. A. M., Serlachius, A. S. (2020). Probiotics and maternal mental health: A randomised controlled trial among pregnant women with obesity. Sci. Rep. 10 (1), 1291. doi: 10.1038/s41598-020-58129-w
de Abreu, D. F., Eyles, D., Feron, F. (2009). Vitamin d, a neuro-immunomodulator: implications for neurodegenerative and autoimmune diseases. Psychoneuroendocrinology 34, S265–S277. doi: 10.1016/j.psyneuen.2009.05.023
Desbonnet, L., Garrett, L., Clarke, G., Bienenstock, J., Dinan, T. G. (2008). The probiotic bifidobacteria infantis: an assessment of potential antidepressant properties in the rat. J. Psychiatr. Res. 43 (2), 164–174. doi: 10.1016/j.jpsychires.2008.03.009
Dijkstra, S., Van Beek, A., Janssen, J., De Vleeschouwer, L., Huysman, W., Van Den Akker, E. (2007). High prevalence of vitamin d deficiency in newborn infants of high-risk mothers. Arch. Dis. Childhood 92 (9), 750–753. doi: 10.1136/adc.2006.105577
Dinan, T. G., Cryan, J. F. (2017). Brain–gut–microbiota axis–mood, metabolism and behaviour. Nat. Rev. Gastroenterol. Hepatol. 14 (2), 69–70. doi: 10.1038/nrgastro.2016.200
Dostal, A., Baumgartner, J., Riesen, N., Chassard, C., Smuts, C. M., Zimmermann, M. B., et al. (2014). Effects of iron supplementation on dominant bacterial groups in the gut, faecal SCFA and gut inflammation: a randomised, placebo-controlled intervention trial in south African children. Br. J. Nutr. 112 (4), 547–556. doi: 10.1017/s0007114514001160
Durmusoglu, D., Al’Abri, I. S., Collins, S. P., Cheng, J., Eroglu, A., Beisel, C. L., et al. (2021). In situ biomanufacturing of small molecules in the mammalian gut by probiotic saccharomyces boulardii. ACS Synthetic Biol. 10 (5), 1039–1052. doi: 10.1021/acssynbio.0c00562
Fatahi, S., Matin, S. S., Sohouli, M. H., Găman, M.-A., Raee, P., Olang, B., et al. (2021). Association of dietary fiber and depression symptom: A systematic review and meta-analysis of observational studies. Complementary Ther Med. 56, 102621. doi: 10.1016/j.ctim.2020.102621
Felger, J. C., Lotrich, F. E. (2013). Inflammatory cytokines in depression: neurobiological mechanisms and therapeutic implications. Neuroscience 246, 199–229. doi: 10.1016/j.neuroscience.2013.04.060
Furuta, M., Numakawa, T., Chiba, S., Ninomiya, M., Kajiyama, Y., Adachi, N., et al. (2013). Estrogen, predominantly via estrogen receptor α, attenuates postpartum-induced anxiety-and depression-like behaviors in female rats. Endocrinology 154 (10), 3807–3816. doi: 10.1210/en.2012-2136
Gibson, G. R., Hutkins, R., Sanders, M. E., Prescott, S. L., Reimer, R. A., Salminen, S. J., et al. (2017). Expert consensus document: The international scientific association for probiotics and prebiotics (ISAPP) consensus statement on the definition and scope of prebiotics. Nat. Rev. Gastroenterol. Hepatol. 14 (8), 491–502. doi: 10.1038/nrgastro.2017.75
Gimeno, D., Kivimäki, M., Brunner, E. J., Elovainio, M., De Vogli, R., Steptoe, A., et al. (2009). Associations of c-reactive protein and interleukin-6 with cognitive symptoms of depression: 12-year follow-up of the Whitehall II study. Psychol. Med. 39 (3), 413–423. doi: 10.1017/s0033291708003723
Gold, P. W. (2021). The PPARg system in major depression: Pathophysiologic and therapeutic implications. Int. J. Mol. Sci. 22 (17), 9248–9262. doi: 10.3390/ijms22179248
Gregoire, A., Kumar, R., Everitt, B., Studd, J. (1996). Transdermal oestrogen for treatment of severe postnatal depression. Lancet 347 (9006), 930–933. doi: 10.1016/s0140-6736(96)91414-2
Grosso, G., Pajak, A., Marventano, S., Castellano, S., Galvano, F., Bucolo, C., et al. (2014). Role of omega-3 fatty acids in the treatment of depressive disorders: a comprehensive meta-analysis of randomized clinical trials. PloS One 9 (5), e96905. doi: 10.1371/journal.pone.0096905
Guo, Y., Qi, Y., Yang, X., Zhao, L., Wen, S., Liu, Y., et al. (2016). Association between polycystic ovary syndrome and gut microbiota. PloS One 11 (4), e0153196. doi: 10.1371/journal.pone.0153196
Hellgren, C., Åkerud, H., Skalkidou, A., Bäckström, T., Sundström-Poromaa, I. (2014). Low serum allopregnanolone is associated with symptoms of depression in late pregnancy. Neuropsychobiology 69 (3), 147–153. doi: 10.1159/000358838
Horiuchi, Y., Kimura, R., Kato, N., Fujii, T., Seki, M., Endo, T., et al. (2003). Evolutional study on acetylcholine expression. Life Sci. 72 (15), 1745–1756. doi: 10.1016/S0024-3205(02)02478-5
Itoh, H., Sashihara, T., Hosono, A., Kaminogawa, S., Uchida, M. (2011). Lactobacillus gasseri OLL2809 inhibits development of ectopic endometrial cell in peritoneal cavity via activation of NK cells in a murine endometriosis model. Cytotechnology 63 (2), 205–210. doi: 10.1007/s10616-011-9343-z
Jarde, A., Morais, M., Kingston, D., Giallo, R., MacQueen, G. M., Giglia, L., et al. (2016). Neonatal outcomes in women with untreated antenatal depression compared with women without depression: a systematic review and meta-analysis. JAMA Psychiatry 73 (8), 826–837. doi: 10.1001/jamapsychiatry.2016.0934
Jiang, H.-y., Zhang, X., Yu, Z.-H., Zhang, Z., Deng, M., Zhao, J.-H., et al. (2018). Altered gut microbiota profile in patients with generalized anxiety disorder. J. Psychiatr. Res. 104, 130–136. doi: 10.1016/j.jpsychires.2018.07.007
Johnstone, N., Milesi, C., Burn, O., van den Bogert, B., Nauta, A., Hart, K., et al. (2021). Anxiolytic effects of a galacto-oligosaccharides prebiotic in healthy females (18–25 years) with corresponding changes in gut bacterial composition. Sci. Rep. 11 (1), 8302. doi: 10.1038/s41598-021-87865-w
Kan, J., Cheng, J., Xu, L., Hood, M., Zhong, D., Cheng, M., et al. (2020). The combination of wheat peptides and fucoidan protects against chronic superficial gastritis and alters gut microbiota: a double-blinded, placebo-controlled study. Eur. J. Nutr. 59 (4), 1655–1666. doi: 10.1007/s00394-019-02020-6
Kanhere, M., He, J., Chassaing, B., Ziegler, T. R., Alvarez, J. A., Ivie, E. A., et al. (2017). Bolus weekly vitamin D3 supplementation impacts gut and airway microbiota in adults with cystic fibrosis: A double-blind, randomized, placebo-controlled clinical trial. J. Clin. Endocrinol. Metab. 103 (2), 564–574. doi: 10.1210/jc.2017-01983
Kato, Y., Shimomura, Y., Takada, Y., Furuyashiki, T. (2021). Correlation between lactic acid bacteria beverage intake and stress resilience. Kobe J. Med. Sci. 67 (1), E1–E6. doi: 10.1016/s0024-3205(02)02478-5
Kekuda, R., Manoharan, P., Baseler, W., Sundaram, U. (2013). Monocarboxylate 4 mediated butyrate transport in a rat intestinal epithelial cell line. Digestive Dis. Sci. 58 (3), 660–667. doi: 10.1007/s10620-012-2407-x
Kelly, J. R., Borre, Y., O' Brien, C., Patterson, E., El Aidy, S., Deane, J., et al. (2016). Transferring the blues: Depression-associated gut microbiota induces neurobehavioural changes in the rat. J. Psychiatr. Res. 82, 109–118. doi: 10.1016/j.jpsychires.2016.07.019
Kim, Y., Hong, M., Kim, S., Shin, W.-Y., Kim, J.-H. (2021). Inverse association between dietary fiber intake and depression in premenopausal women: a nationwide population-based survey. Menopause 28 (2), 150–156. doi: 10.1097/GME.0000000000001711
Klimenko, N. S., Tyakht, A. V., Popenko, A. S., Vasiliev, A. S., Altukhov, I. A., Ischenko, D. S., et al. (2018). Microbiome responses to an uncontrolled short-term diet intervention in the frame of the citizen science project. Nutrients 10 (5), 576. doi: 10.3390/nu10050576
Köhler, C. A., Freitas, T. H., Maes, M., de Andrade, N. Q., Liu, C. S., Fernandes, B. S., et al. (2017a). Peripheral cytokine and chemokine alterations in depression: a meta-analysis of 82 studies. Acta Psychiatr. Scand. 135 (5), 373–387. doi: 10.1111/acps.12698
Köhler, O., Petersen, L., Mors, O., Mortensen, P., Yolken, R., Gasse, C., et al. (2017b). Infections and exposure to anti-infective agents and the risk of severe mental disorders: a nationwide study. Acta Psychiatrica Scand. 135 (2), 97–105. doi: 10.1111/acps.12671
Komatsuzaki, N., Nakamura, T., Kimura, T., Shima, J. (2008). Characterization of glutamate decarboxylase from a high gamma-aminobutyric acid (GABA)-producer, lactobacillus paracasei. Biosci. Biotechnol. Biochem. 72 (2), 278–285. doi: 10.1271/bbb.70163
Koussoulas, K., Swaminathan, M., Fung, C., Bornstein, J. C., Foong, J. P. P. (2018). Neurally released GABA acts via GABA(C) receptors to modulate Ca(2+) transients evoked by trains of synaptic inputs, but not responses evoked by single stimuli, in myenteric neurons of mouse ileum. Front. Physiol. 9. doi: 10.3389/fphys.2018.00097
Kurina, L., Goldacre, M., Yeates, D., Gill, L. (2001). Depression and anxiety in people with inflammatory bowel disease. J. Epidemiol. Community Health 55 (10), 716–720. doi: 10.1136/jech.55.10.716
Kwa, M., Plottel, C. S., Blaser, M. J., Adams, S. (2016). The intestinal microbiome and estrogen receptor-positive female breast cancer. J. Natl. Cancer Inst 108 (8), djw029. doi: 10.1093/jnci/djw029
Lambert, M. N. T., Thybo, C. B., Lykkeboe, S., Rasmussen, L. M., Frette, X., Christensen, L. P., et al. (2017). Combined bioavailable isoflavones and probiotics improve bone status and estrogen metabolism in postmenopausal osteopenic women: a randomized controlled trial. Am. J. Clin. Nutr. 106 (3), 909–920. doi: 10.3945/ajcn.117.153353
Lancaster, C. A., Gold, K. J., Flynn, H. A., Yoo, H., Marcus, S. M., Davis, M. M. (2010). Risk factors for depressive symptoms during pregnancy: a systematic review. Am. J. Obstet Gynecol 202 (1), 5–14. doi: 10.1016/j.ajog.2009.09.007
Lee, H.-S., Chao, H.-H., Huang, W.-T., Chen, S. C.-C., Yang, H.-Y. (2020). Psychiatric disorders risk in patients with iron deficiency anemia and association with iron supplementation medications: a nationwide database analysis. BMC Psychiatry 20 (1), 216. doi: 10.1186/s12888-020-02621-0
Lee, J. M., Smith, J. R., Philipp, B. L., Chen, T. C., Mathieu, J., Holick, M. F. (2007). Vitamin d deficiency in a healthy group of mothers and newborn infants. Clin. Pediatr. 46 (1), 42–44. doi: 10.1177/0009922806289311
Leung, B. M., Kaplan, B. J. (2009). Perinatal depression: prevalence, risks, and the nutrition link–a review of the literature. J. Am. Diet Assoc. 109 (9), 1566–1575. doi: 10.1016/j.jada.2009.06.368
Liang, Q., Cai, C., Duan, D., Hu, X., Hua, W., Jiang, P., et al. (2018). Postnatal vitamin d intake modulates hippocampal learning and memory in adult mice. Front. Neurosci. 12. doi: 10.3389/fnins.2018.00141
Li, C.-C., Liu, C., Fu, M., Hu, K.-Q., Aizawa, K., Takahashi, S., et al. (2018). Tomato powder inhibits hepatic steatosis and inflammation potentially through restoring SIRT1 activity and adiponectin function independent of carotenoid cleavage enzymes in mice. Mol. Nutr. Food Res. 62 (8), 1700738. doi: 10.1002/mnfr.201700738
Li, Y., Lv, M. R., Wei, Y. J., Sun, L., Zhang, J. X., Zhang, H. G., et al. (2017). Dietary patterns and depression risk: A meta-analysis. Psychiatry Res. 253, 373–382. doi: 10.1016/j.psychres.2017.04.020
Lin, T.-L., Shu, C.-C., Chen, Y.-M., Lu, J.-J., Wu, T.-S., Lai, W.-F., et al. (2020). Like cures like: Pharmacological activity of anti-inflammatory lipopolysaccharides from gut microbiome. Front. Pharmacol. 11. doi: 10.3389/fphar.2020.00554
Liu, F., Li, P., Chen, M., Luo, Y., Prabhakar, M., Zheng, H., et al. (2017). Fructooligosaccharide (FOS) and galactooligosaccharide (GOS) increase bifidobacterium but reduce butyrate producing bacteria with adverse glycemic metabolism in healthy young population. Sci. Rep. 7 (1), 11789. doi: 10.1038/s41598-017-10722-2
Liu, Z., Li, L., Ma, S., Ye, J., Zhang, H., Li, Y., et al. (2020). High-dietary fiber intake alleviates antenatal obesity-induced postpartum depression: Roles of gut microbiota and microbial metabolite short-chain fatty acid involved. J. Agric. Food Chem. 68 (47), 13697–13710. doi: 10.1021/acs.jafc.0c04290
Li, D., Wang, K., Yang, Z., Li, H., Wang, S. (2021). Vitamin d supplementation in mice with advanced maternal age and cognitive function of the offspring. Am. J. Trans. Res. 13 (7), 7641–7653.
Luqman, A., Nega, M., Nguyen, M. T., Ebner, P., Götz, F. (2018). SadA-expressing staphylococci in the human gut show increased cell adherence and internalization. Cell Rep. 22 (2), 535–545. doi: 10.1016/j.celrep.2017.12.058
Lurie, I., Yang, Y.-X., Haynes, K., Mamtani, R., Boursi, B. (2015). Antibiotic exposure and the risk for depression, anxiety, or psychosis: a nested case-control study. J. Clin. Psychiatry 76 (11), 825. doi: 10.4088/JCP.15m09961
Lyte, M., Varcoe, J. J., Bailey, M. T. (1998). Anxiogenic effect of subclinical bacterial infection in mice in the absence of overt immune activation. Physiol. Behav. 65 (1), 63–68. doi: 10.1016/S0031-9384(98)00145-0
Maes, M., Kubera, M., Leśkiewicz, M., Budziszewska, B., Basta-Kaim, A., Korzeniak, B., et al. (2010). The gut-brain barrier in major depression. Pharmacol. Rep. 62 (1), 52–52. doi: 10.1016/S1734-1140(10)71152-X
Maes, M., Kubera, M., Leunis, J. C., Berk, M. (2012). Increased IgA and IgM responses against gut commensals in chronic depression: further evidence for increased bacterial translocation or leaky gut. J. Affect. Disord. 141 (1), 55–62. doi: 10.1016/j.jad.2012.02.023
Masand, P. S., Kaplan, D. S., Gupta, S., Bhandary, A. N., Nasra, G. S., Kline, M. D., et al. (1995). Major depression and irritable bowel syndrome: is there a relationship? J. Clin. Psychiatry 56 (8), 363–367.
Matsuzaki, M., Matsushita, H., Tomizawa, K., Matsui, H. (2012). Oxytocin: a therapeutic target for mental disorders. J. Physiol. Sci. 62 (6), 441–444. doi: 10.1007/s12576-012-0232-9
Mazzoli, R., Pessione, E. (2016). The neuro-endocrinological role of microbial glutamate and GABA signaling. Front. Microbiol. 7 1934. doi: 10.3389/fmicb.2016.01934
Mehta, J., Kling, J. M., Manson, J. E. (2021). Risks, benefits, and treatment modalities of menopausal hormone therapy: current concepts. Front. Endocrinol. 12, 564781. doi: 10.3389/fendo.2021.564781
Metwaly, A., Reitmeier, S., Haller, D. (2022). Microbiome risk profiles as biomarkers for inflammatory and metabolic disorders. Nat. Rev. Gastroenterol. Hepatol 19 (6), 383–397. doi: 10.1038/s41575-022-00581-2
Mi, G.-L., Zhao, L., Qiao, D.-D., Kang, W.-Q., Tang, M.-Q., Xu, J.-K. (2015). Effectiveness of lactobacillus reuteri in infantile colic and colicky induced maternal depression: a prospective single blind randomized trial. Antonie van Leeuwenhoek 107 (6), 1547–1553. doi: 10.1007/s10482-015-0448-9
Mor, G., Cardenas, I. (2010). The immune system in pregnancy: a unique complexity. Am. J. Reprod. Immunol. 63 (6), 425–433. doi: 10.1111/j.1600-0897.2010.00836.x
Muleviciene, A., D'Amico, F., Turroni, S., Candela, M., Jankauskiene, A. (2018). Iron deficiency anemia-related gut microbiota dysbiosis in infants and young children: A pilot study. Acta Microbiol. Immunol. Hung 65 (4), 551–564. doi: 10.1556/030.65.2018.045
Myint, A. M., Leonard, B. E., Steinbusch, H. W., Kim, Y. K. (2005). Th1, Th2, and Th3 cytokine alterations in major depression. J. Affect. Disord. 88 (2), 167–173. doi: 10.1016/j.jad.2005.07.008
Naderpoor, N., Mousa, A., Fernanda Gomez Arango, L., Barrett, H. L., Dekker Nitert, M., de Courten, B. (2019). Effect of vitamin d supplementation on faecal microbiota: A randomised clinical trial. Nutrients 11 (12), 2888–2900. doi: 10.3390/nu11122888
Nappi, R. E., Petraglia, F., Luisi, S., Polatti, F., Farina, C., Genazzani, A. R. (2001). Serum allopregnanolone in women with postpartum “blues”11The authors are grateful to dr. e. casarosa (Department of obstetrics and gynecology, university of Pisa, Italy) and to dr. a. poma (Laboratory of endocrinology, institute for clinical and scientific research [IRCCS] mondino, university of pavia, Italy) for their expert technical assistance and to dr. r. h. purdy (Department of psychiatry, veterans administration hospital, San Diego, CA) for kindly providing allopregnanolone antisera. Obstetrics Gynecol 97 (1), 77–80. doi: 10.1016/S0029-7844(00)01112-1
Naseribafrouei, A., Hestad, K., Avershina, E., Sekelja, M., Linløkken, A., Wilson, R., et al. (2014). Correlation between the human fecal microbiota and depression. Neurogastroenterol. Motil. 26 (8), 1155–1162. doi: 10.1111/nmo.12378
Neufeld, K. M., Kang, N., Bienenstock, J., Foster, J. A. (2011). Reduced anxiety-like behavior and central neurochemical change in germ-free mice. Neurogastroenterol. Motil. 23 (3), 255–e119. doi: 10.1111/j.1365-2982.2010.01620.x
Nishino, R., Mikami, K., Takahashi, H., Tomonaga, S., Furuse, M., Hiramoto, T., et al. (2013). Commensal microbiota modulate murine behaviors in a strictly contamination-free environment confirmed by culture-based methods. Neurogastroenterol. Motil. 25 (6), 521–e371. doi: 10.1111/nmo.12110
O’Donnell, M. P., Fox, B. W., Chao, P.-H., Schroeder, F. C., Sengupta, P. (2020). A neurotransmitter produced by gut bacteria modulates host sensory behaviour. Nature 583 (7816), 415–420. doi: 10.1038/s41586-020-2395-5
Oleskin, A., El’-Registan, G., Shenderov, B. (2016). Role of neuromediators in the functioning of the human microbiota:”business talks” among microorganisms and the microbiota-host dialogue. Microbiology 85 (1), 1–22. doi: 10.1134/S0026261716010082
Oleskin, A. V., Zhilenkova, O. G., Shenderov, B. A., Amerhanova, A. M., Kudrin, V. S., Klodt, P. M. (2014). Lactic-acid bacteria supplement fermented dairy products with human behavior-modifying neuroactive compounds. J. Pharm. Nutr. Sci. 4 (3), 199–206. doi: 10.6000/1927-5951.2014.04.03.5
Osborne, L. M., Gispen, F., Sanyal, A., Yenokyan, G., Meilman, S., Payne, J. L. (2017). Lower allopregnanolone during pregnancy predicts postpartum depression: an exploratory study. Psychoneuroendocrinology 79, 116–121. doi: 10.1016/j.psyneuen.2017.02.012
Özogul, F. (2011). Effects of specific lactic acid bacteria species on biogenic amine production by foodborne pathogen. Int. J. Food Sci. Technol. 46 (3), 478–484. doi: 10.1111/j.1365-2621.2010.02511.x
Özoğul, F., Kuley, E., ÖZOĞUL, Y., ÖZOĞUL, İ. (2012). The function of lactic acid bacteria on biogenic amines production by food-borne pathogens in arginine decarboxylase broth. Food Sci. Technol. Res. 18 (6), 795–804.
Paganini, D., Zimmermann, M. B. (2017). The effects of iron fortification and supplementation on the gut microbiome and diarrhea in infants and children: a review. Am. J. Clin. Nutr. 106 (suppl_6), 1688S–1693S. doi: 10.3945/ajcn.117.156067
Pearson Murphy, B. E., Steinberg, S. I., Hu, F. Y., Allison, C. M. (2001). Neuroactive ring a-reduced metabolites of progesterone in human plasma during pregnancy: elevated levels of 5 alpha-dihydroprogesterone in depressed patients during the latter half of pregnancy. J. Clin. Endocrinol. Metab. 86 (12), 5981–5987. doi: 10.1210/jcem.86.12.8122
Peng, Z., Peng, S., Lin, K., Zhao, B., Wei, L., Tuo, Q., et al. (2022). Chronic stress-induced depression requires the recruitment of peripheral Th17 cells into the brain. J. Neuroinflamm. 19 (1), 186. doi: 10.1186/s12974-022-02543-6
Pokusaeva, K., Johnson, C., Luk, B., Uribe, G., Fu, Y., Oezguen, N., et al. (2017). GABA-producing bifidobacterium dentium modulates visceral sensitivity in the intestine. Neurogastroenterol. Motil. 29 (1), e12904. doi: 10.1111/nmo.12904
Pusceddu, M. M., El Aidy, S., Crispie, F., O’Sullivan, O., Cotter, P., Stanton, C., et al. (2015). N-3 polyunsaturated fatty acids (PUFAs) reverse the impact of early-life stress on the gut microbiota. PloS One 10 (10), e0139721.
Qin, L., Wu, X., Block, M. L., Liu, Y., Breese, G. R., Hong, J.-S., et al. (2007). Systemic LPS causes chronic neuroinflammation and progressive neurodegeneration. Glia 55 (5), 453–462. doi: 10.1002/glia.20467
Ratner, M. H., Kumaresan, V., Farb, D. H. (2019). Neurosteroid actions in memory and Neurologic/Neuropsychiatric disorders. Front. Endocrinol. 10. doi: 10.3389/fendo.2019.00169
Reddy, D. S. (2010). Neurosteroids: endogenous role in the human brain and therapeutic potentials. Prog. Brain Res. 186, 113–137. doi: 10.1016/B978-0-444-53630-3.00008-7
Robertson, R. C., Seira Oriach, C., Murphy, K., Moloney, G. M., Cryan, J. F., Dinan, T. G., et al. (2017). Omega-3 polyunsaturated fatty acids critically regulate behaviour and gut microbiota development in adolescence and adulthood. Brain Behavior Immun. 59, 21–37. doi: 10.1016/j.bbi.2016.07.145
Rubinow, D. R., Girdler, S. S. (2011). Hormones, heart disease, and health: individualized medicine versus throwing the baby out with the bathwater. Depress Anxiety 28 (4), 282–296. doi: 10.1002/da.20810
Rusu, I. G., Suharoschi, R., Vodnar, D. C., Pop, C. R., Socaci, S. A., Vulturar, R., et al. (2020). Iron supplementation influence on the gut microbiota and probiotic intake effect in iron deficiency-a literature-based review. Nutrients 12 (7), 1993. doi: 10.3390/nu12071993
Sánchez-Villegas, A., Martínez-González, M. A., Estruch, R., Salas-Salvadó, J., Corella, D., Covas, M. I., et al. (2013). Mediterranean Dietary pattern and depression: the PREDIMED randomized trial. BMC Med. 11 (1), 208. doi: 10.1186/1741-7015-11-208
Sawyer, K. M. (2021). The role of inflammation in the pathogenesis of perinatal depression and offspring outcomes. Brain Behavior Immun-Health 18, 100390. doi: 10.1016/j.bbih.2021.100390
Schiller, C. E., Meltzer-Brody, S., Rubinow, D. R. (2015). The role of reproductive hormones in postpartum depression. CNS spectrums 20 (1), 48–59. doi: 10.1017/S1092852914000480
Schiweck, C., Valles-Colomer, M., Arolt, V., Müller, N., Raes, J., Wijkhuijs, A., et al. (2020). Depression and suicidality: A link to premature T helper cell aging and increased Th17 cells. Brain Behav. Immun. 87, 603–609. doi: 10.1016/j.bbi.2020.02.005
Seth, S., Lewis, A. J., Galbally, M. (2016). Perinatal maternal depression and cortisol function in pregnancy and the postpartum period: a systematic literature review. BMC Pregnancy Childbirth 16 (1), 124. doi: 10.1186/s12884-016-0915-y
Shao, L., Li, M., Zhang, B., Chang, P. (2020). Bacterial dysbiosis incites Th17 cell revolt in irradiated gut. Biomed. Pharmacother. 131, 110674. doi: 10.1016/j.biopha.2020.110674
Sharafi, S., Nateghi, L. (2020). Optimization of gamma-aminobutyric acid production by probiotic bacteria through response surface methodology. Iran J. Microbiol. 12 (6), 584–591. doi: 10.18502/ijm.v12i6.5033
Sherwin, B. B. (2012). Estrogen and cognitive functioning in women: lessons we have learned. Behav. Neurosci. 126 (1), 123–127. doi: 10.1037/a0025539
Shors, T. J., Leuner, B. (2003). Estrogen-mediated effects on depression and memory formation in females. J. Affect. Disord. 74 (1), 85–96. doi: 10.1016/s0165-0327(02)00428-7
Singh, P., Rawat, A., Alwakeel, M., Sharif, E., Al Khodor, S. (2020). The potential role of vitamin d supplementation as a gut microbiota modifier in healthy individuals. Sci. Rep. 10 (1), 21641. doi: 10.1038/s41598-020-77806-4
Siragusa, S., De Angelis, M., Di Cagno, R., Rizzello, C. G., Coda, R., Gobbetti, M. (2007). Synthesis of γ-aminobutyric acid by lactic acid bacteria isolated from a variety of Italian cheeses. Appl. Environ. Microbiol. 73 (22), 7283–7290. doi: 10.1128/AEM.01064-07
Skonieczna-Żydecka, K., Grochans, E., Maciejewska, D., Szkup, M., Schneider-Matyka, D., Jurczak, A., et al. (2018). Faecal short chain fatty acids profile is changed in polish depressive women. Nutrients 10 (12), 1939–1952. doi: 10.3390/nu10121939
Slykerman, R. F., Hood, F., Wickens, K., Thompson, J. M. D., Barthow, C., Murphy, R., et al. (2017a). Effect of lactobacillus rhamnosus HN001 in pregnancy on postpartum symptoms of depression and anxiety: A randomised double-blind placebo-controlled trial. EBioMedicine 24, 159–165. doi: 10.1016/j.ebiom.2017.09.013
Slykerman, R. F., Thompson, J., Waldie, K. E., Murphy, R., Wall, C., Mitchell, E. A. (2017b). Antibiotics in the first year of life and subsequent neurocognitive outcomes. Acta Paediatric 106 (1), 87–94. doi: 10.1111/apa.13613
Stanaszek, P. M., Snell, J. F., O'Neill, J. J. (1977). Isolation, extraction, and measurement of acetylcholine from lactobacillus plantarum. Appl. Environ. Microbiol. 34 (2), 237–239. doi: 10.1128/aem.34.2.237-239.1977
Strandwitz, P., Kim, K. H., Terekhova, D., Liu, J. K., Sharma, A., Levering, J., et al. (2019). GABA-modulating bacteria of the human gut microbiota. Nat. Microbiol. 4 (3), 396–403. doi: 10.1038/s41564-018-0307-3
Sui, Y., Wu, J., Chen, J. (2021). The role of gut microbial β-glucuronidase in estrogen reactivation and breast cancer. Front. Cell Dev. Biol. 9. doi: 10.3389/fcell.2021.631552
Su, X., Yin, X., Liu, Y., Yan, X., Zhang, S., Wang, X., et al. (2020). Gut dysbiosis contributes to the imbalance of treg and Th17 cells in graves’ disease patients by propionic acid. J. Clin. Endocrinol. Metab. 105 (11), 3526–3547. doi: 10.1210/clinem/dgaa511
Tandon, D., Haque, M. M., Gote, M., Jain, M., Bhaduri, A., Dubey, A. K., et al. (2019). A prospective randomized, double-blind, placebo-controlled, dose-response relationship study to investigate efficacy of fructo-oligosaccharides (FOS) on human gut microflora. Sci. Rep. 9 (1), 5473. doi: 10.1038/s41598-019-41837-3
Thul, T. A., Corwin, E. J., Carlson, N. S., Brennan, P. A., Young, L. J. (2020). Oxytocin and postpartum depression: A systematic review. Psychoneuroendocrinology 120, 104793. doi: 10.1016/j.psyneuen.2020.104793
Tian, P., Wang, G., Zhao, J., Zhang, H., Chen, W. (2019). Bifidobacterium with the role of 5-hydroxytryptophan synthesis regulation alleviates the symptom of depression and related microbiota dysbiosis. J. Nutr. Biochem. 66, 43–51. doi: 10.1016/j.jnutbio.2019.01.007
Tsavkelova, E. A., Botvinko, I. V., Kudrin, V. S., Oleskin, A. V. (2000). Detection of neurotransmitter amines in microorganisms with the use of high-performance liquid chromatography. Dokl Biochem. 372 (1-6), 115–117.
Vahdat Shariatpanaahi, M., Vahdat Shariatpanaahi, Z., Moshtaaghi, M., Shahbaazi, S. H., Abadi, A. (2007). The relationship between depression and serum ferritin level. Eur. J. Clin. Nutr. 61 (4), 532–535. doi: 10.1038/sj.ejcn.1602542
Valdes, A. M., Walter, J., Segal, E., Spector, T. D. (2018). Role of the gut microbiota in nutrition and health. BMJ 361, k2179. doi: 10.1136/bmj.k2179
Van Niel, M. S., Payne, J. L. (2020). Perinatal depression: A review. Cleveland Clinic J. Med. 87 (5), 273–277. doi: 10.3949/ccjm.87a.19054
Vijay, N., Morris, M. E. (2014). Role of monocarboxylate transporters in drug delivery to the brain. Curr. Pharm. Design 20 (10), 1487–1498. doi: 10.2174/13816128113199990462
Wang, J., Liu, N., Sun, W., Chen, D., Zhao, J., Zhang, W. (2018). Association between vitamin d deficiency and antepartum and postpartum depression: a systematic review and meta-analysis of longitudinal studies. Arch. Gynecol Obstetrics 298 (6), 1045–1059. doi: 10.1007/s00404-018-4902-6
Watson, H., Mitra, S., Croden, F. C., Taylor, M., Wood, H. M., Perry, S. L., et al. (2018). A randomised trial of the effect of omega-3 polyunsaturated fatty acid supplements on the human intestinal microbiota. Gut 67 (11), 1974–1983. doi: 10.1136/gutjnl-2017-314968
Wendeln, A.-C., Degenhardt, K., Kaurani, L., Gertig, M., Ulas, T., Jain, G., et al. (2018). Innate immune memory in the brain shapes neurological disease hallmarks. Nature 556 (7701), 332–338. doi: 10.1038/s41586-018-0023-4
Yang, Y., Zhang, S., Zhang, X., Xu, Y., Cheng, J., Yang, X. (2020). The role of diet, eating behavior, and nutrition intervention in seasonal affective disorder: A systematic review. Front. Psychol. 11. doi: 10.3389/fpsyg.2020.01451
Yang, H., Zhao, X., Tang, S., Huang, H., Zhao, X., Ning, Z., et al. (2016). Probiotics reduce psychological stress in patients before laryngeal cancer surgery. Asia-Pacific J. Clin. Oncol. 12 (1), e92–e96. doi: 10.1111/ajco.12120
Yao, W., Zhang, J.-C., Ishima, T., Dong, C., Yang, C., Ren, Q., et al. (2016). Role of Keap1-Nrf2 signaling in depression and dietary intake of glucoraphanin confers stress resilience in mice. Sci. Rep. 6 (1), 30659. doi: 10.1038/srep30659
Yokoyama, S., Hiramatsu, J.-I., Hayakawa, K. (2002). Production of γ-aminobutyric acid from alcohol distillery lees by lactobacillus brevis IFO-12005. J. Biosci. Bioengineering 93 (1), 95–97. doi: 10.1016/S1389-1723(02)80061-5
Yong, S. J., Tong, T., Chew, J., Lim, W. L. (2019). Antidepressive mechanisms of probiotics and their therapeutic potential. Front. Neurosci. 13. doi: 10.3389/fnins.2019.01361
Zhang, J.C., Yao, W., Dong, C., Yang, C., Ren, Q., Ma, M., et al. (2017). Blockade of interleukin-6 receptor in the periphery promotes rapid and sustained antidepressant actions: a possible role of gut–microbiota–brain axis. Trans. Psychiatry 7 (5), e1138–e1138. doi: 10.1038/tp.2017.112
Keywords: fiber, perinatal depression, nutrition, microbiota, probiotics
Citation: Song J, Zhou B, Kan J, Liu G, Zhang S, Si L, Zhang X, Yang X, Ma J, Cheng J, Liu X and Yang Y (2022) Gut microbiota: Linking nutrition and perinatal depression. Front. Cell. Infect. Microbiol. 12:932309. doi: 10.3389/fcimb.2022.932309
Received: 09 May 2022; Accepted: 09 August 2022;
Published: 26 August 2022.
Edited by:
Lionel Ludovic Breton, IDEC therapeutic/CILIA Consult, FranceReviewed by:
Almagul Kushugulova, Nazarbayev University, KazakhstanJayanta Kumar Patra, Dongguk University Seoul, South Korea
Copyright © 2022 Song, Zhou, Kan, Liu, Zhang, Si, Zhang, Yang, Ma, Cheng, Liu and Yang. This is an open-access article distributed under the terms of the Creative Commons Attribution License (CC BY). The use, distribution or reproduction in other forums is permitted, provided the original author(s) and the copyright owner(s) are credited and that the original publication in this journal is cited, in accordance with accepted academic practice. No use, distribution or reproduction is permitted which does not comply with these terms.
*Correspondence: Yongde Yang, eWFuZ3lvbmdkZS50b25namlAZ21haWwuY29t; Xiaobo Liu, eGlhb2JvbGl1eGJsQGdtYWlsLmNvbQ==
†These authors share first authorship