- 1Department of Food Safety, Nutrition and Veterinary Public Health, Istituto Superiore di Sanità, Rome, Italy
- 2Core Facilities, Istituto Superiore di Sanità, Rome, Italy
Free-living amoebae (FLA) are widely distributed protozoa in nature, known to cause severe eye infections and central nervous system disorders. There is growing attention to the potential role that these protozoa could act as reservoirs of pathogenic bacteria and, consequently, to the possibility that, the persistence and spread of the latter may be facilitated, by exploiting internalization into amoebae. Shiga toxin-producing strains of Escherichia coli (STEC) are zoonotic agents capable of causing serious diseases, such as hemorrhagic colitis (HC) and hemolytic uremic syndrome (HUS). Cattle represent the main natural reservoir of STEC, which are frequently found also in other domestic and wild ruminants, often without causing any evident symptoms of disease. The aspects related to the ecology of STEC strains in animal reservoirs and the environment are poorly known, including the persistence of these microorganisms within niches unfavorable to survival, such as soils or waters. In this study we investigated the interaction between STEC strains of serotype O157: H7 with different virulence gene profiles, and a genus of a wild free-living amoeba, Acanthamoeba sp. Our results confirm the ability of STEC strains to survive up to 20 days within a wild Acanthamoeba sp., in a quiescent state persisting in a non-cultivable form, until they reactivate following some stimulus of an unknown nature. Furthermore, our findings show that during their internalization, the E. coli O157 kept the set of the main virulence genes intact, preserving their pathogenetic potential. These observations suggest that the internalization in free-living amoebae may represent a means for STEC to resist in environments with non-permissive growth conditions. Moreover, by staying within the protozoa, STEC could escape their detection in the vehicles of infections and resist to the treatments used for the disinfection of the livestock environment.
Introduction
Free-living amoebae (FLA) are ubiquitous and widely distributed Protozoa commonly found in terrestrial and aquatic environments where they feed on bacteria, algae, and fungi.
These organisms have also been isolated in various man-made constructions such as drinking water supplies, hospital water networks, eyewash stations, swimming pools, spas, homes, dental unit’s waterlines, cooling towers and wastewater water plants. Despite their wide distribution, only some genera of FLA are known to be potentially pathogenic to humans.
FLA are characterized by two life cycle stages: the trophozoite and the resistant cyst. The former represents the actively feeding stage, preying on bacteria, algae, viruses, yeast (Siddiqui and Khan, 2012). Encystment is triggered by adverse environmental conditions: indeed, on cystic stage, the amoebae are preserved against food shortage, high/low temperatures, pH extremes, gamma and UV radiation, and chemicals used for disinfection in health care settings and drinking water production (Nataro and Kaper, 1998; Tozzi et al., 2003; Caprioli et al., 2005; Khan, 2006; Loret and Greub, 2010; Thomas et al., 2010; Siddiqui and Khan, 2012).
In addition to their pathogenicity, there is growing attention about the potential role that FLA could play as reservoirs of pathogenic bacteria and, consequently, to the possibility that, their persistence and spread may be facilitated, by exploiting internalization into amoebae. The relationship between bacteria and amoeba may serve (i) to protect bacteria from hostile conditions; and (ii) to support bacteria to adapt to persistence in mammalian phagocytic cells, indicating that amoeba-bacteria relationships are involved in complex interactions (Greub and Raoult, 2004). In addition, the ability of bacteria to resist to grazing by amoeba may have led to their evolution to cause human diseases, i.e., evade human immune cells such as macrophages (Greub and Raoult, 2004). Recent studies have shown FLA could represent a niche for many human bacterial pathogens (Scheid, 2018), including food-related pathogens (Vaerewijck et al., 2014; Lambrecht et al., 2015), to survive and replicate in, therefore representing an efficient vehicle to increase the persistence and diffusion of amoeba resistant bacteria (ARBs) in the environment.
Acanthamoeba is a free-living amoeba that plays an important role in controlling bacterial populations in a variety of terrestrial environments, and it serves as a model for the study of protozoa–bacteria predator–prey interactions (Henriquez et al., 2021). It is one of the most common FLA genera found in soil and water samples (Page, 1988) having a cosmopolitan distribution and a wide variety of habitats. Its wide distribution in nature brings frequently humans in contact with this amoeba, and evidence is found in the extensive presence of related antibodies in human and animal populations.
In addition to causing severe ocular and brain infections in humans, Acanthamoeba has been shown to harbor many bacterial, viral, and fungal species, although not always to their own advantage (Rayamajhee et al., 2021).
Escherichia coli O157:H7 is considered the archetype Shiga toxin encoding E. coli (STEC) strain (Gyles, 2007). STEC are characterized by the production of Shiga toxins (stx), potent cytotoxins that inhibit the protein synthesis within eukaryotic cells (Melton-Celsa, 2014), which are encoded by the stx genes harbored on lysogenic bacteriophages. Stxs are the major virulence factor of STEC and comprise a family of structurally related cytotoxins with similar biological activity. Two main types of stx genes have been described, stx1 and stx2, which, as of now, have been subtyped in three and eleven subtypes, respectively (Scheutz et al., 2012; Lacher et al., 2016; Bai et al., 2018; Yang et al., 2019).
STEC are zoonotic pathogens, causing foodborne infections with symptoms ranging from mild diarrhea to hemolytic uremic syndrome (HUS), sometimes with fatal outcomes (Caprioli et al., 2005). Infection is transmitted to humans through the ingestion of contaminated food, but person to person transfer and direct contact with infected animals or contaminated environment, especially water is also reported (Caprioli et al., 2005).
Cattle represent the main natural reservoir of STEC, which are, however, frequently found also in other domestic and wild ruminants, often without causing any evident symptoms of disease (Caprioli et al., 2005).
The aspects related to the ecology of STEC strains in animal reservoirs and the environment, as well as the related biological mechanisms are poorly known, including the persistence of these microorganisms within niches unfavorable to survival, such as soils or waters.
In the present work, we used a combined approach to investigate the nature of interaction between STEC and the free-living amoeba Acanthamoeba.
We (i) investigated the internalization ability of a panel of STEC O157: H7, with different virulence gene profiles, in a field isolate of Acanthamoeba sp.; (ii) determined whether the bacterium remains quiescent or in a state of active growth within the protozoan; (iii) studied the integrity and genomic stability of the STEC strains internalized in Acanthamoeba sp. in comparison with the same isolates grown in permissive culture conditions.
Materials and methods
Amoebal strain and culture conditions
An environmental isolate of Acanthamoeba sp. belonging to the T4 genotype, isolated from thermal waters (T = 40°C), was kindly obtained from Dr. David Di Cave and Dr. Federica Berrilli (Parasitology Unit, University of Rome “Tor Vergata”, Italy). The amoebae were grown without shaking in proteose peptone-yeast-glucose (PYG – 0,75%, w/v, proteose peptone; 0,75%, w/v, yeast extract; 1,5%, w/v, glucose) medium as monolayers in 75 cm2 tissue culture flasks at 37°C and subsequently, were sub-cultured weekly by tapping flasks to detach cells before diluting 1:5 in fresh medium (Berk and Garduno, 2012). Stationary phase (5-10 days) cultures were used throughout this study (95% trophozoite form).
Bacterial strains and culture conditions
Three STEC O157: H7 strains from the culture collection at National Reference Laboratory for E. coli (Istituto Superiore di Sanità, Italy) and a commensal strain of E. coli used as a non-pathogenic control were used in this study (Table 1).
All bacteria were grown in Luria–Bertani (LB) broth overnight. Prior to the intracellular survival assays, all the strains were checked for their ability to grow in presence of the antibiotic used in the internalization experiments. For co-culture experiments, the overnight cultures of bacteria in LB broth were refreshed with the ratio of 1:10 into fresh LB broth and incubated at 37℃ with continuous shaking until the absorbance at 590 nm reached 0.5 to 0.6.
Intracellular survival assays
To study STEC interactions with Acanthamoeba sp., intracellular survival assays were performed in triplicates, as previously described (Jung et al., 2007; Jacquier et al., 2013; Yousuf et al., 2013). Briefly, free-living amoebae were inoculated in 48-well plates in PYG medium. The plates were incubated at 37℃ for 24-48 h to obtain confluent cultures (at least 50%). After this incubation, media were aspirated, and wells were washed once with phosphate buffered saline (PBS). Subsequently, E. coli strains were added (106 CFU ml-1), and plates incubated for 120 min at room temperature. Following this incubation, gentamicin (150 μg/ml) was added to each well to kill extracellular bacteria and eventually the plates were incubated at 37°C for 25 days. At 12 h, 24 h, 72 h, 6 days, 10 days, 15 days, 20 days and 25 days intervals, the medium in the single wells was carefully checked for bacterial contamination and for amoeba integrity. The protozoa were eventually washed three times with PBS and the amoebae lysed by adding SDS (0.5% final conc.) to each well for 40 min and the presence of live bacteria was assessed by plating onto MacConkey agar plates, with and without sorbitol and the resulting CFU enumerated. Randomly picked single bacterial colonies were used as template in a specific PCR for the detection of stx1, stx2 and eae genes (Paton and Paton, 1998).
Analysis of the expression of rpoS gene
The resuscitation of several species of Viable But Not Culturable (VBNC) bacteria upon being internalized by the amoebae has been documented (Steinert et al., 1997; García et al., 2007; Epalle et al., 2014; Casini et al., 2018; Dey et al., 2020). The biological reason of this shift is unknown and largely unexplored. To verify whether the lack of bacterial growth registered at certain sampling times during the invasion experiment was due to the present of VBNC forms of the internalized strains, we conducted an analysis of the of rpoS gene expression. This gene encodes a conserved alternative sigma factor that regulates the expression of many stress response genes in E. coli. The expression of rpoS gene was analyzed using the total RNA prepared from the amoebic SDS (0.5%) lysates, sampled in a replicated intracellular assay were the protozoa were sampled at 24 h, 72 h and 10 days. Additionally, the total RNA was extracted from one ml of overnight broth cultures grown at 37°C of each E. coli strains used in this internalization experiment to be used as calibrator in the expression experiments. The RNA was extracted using the Norgen RNA/Protein Purification Kit, according to the manufacturer’s instructions. In detail, 1µg of RNA was used for DNA removal and retro-transcription with the QuantiTect Reverse Transcription (Qiagen, Germantown, MD, USA). Two µl of the cDNA solutions were used in Real Time PCR reactions targeting rpoS gene in 40 cycles of a two steps thermal profile (10 s at 95°C and 1 min at 55°C) using the following primers and probes: rpoS_FWD: TTCGTTTGCCGATTCACATC; rpoS_REV: TCTCTTCCGCACTTGGTTCA; rpoS_probe: TTACCTGCGAACAGCAC (Liu et al., 2010). The transcription of the housekeeping gene gapA (Fitzmaurice et al., 2004) was measured and used to normalize the amount of template used, while the level of transcription recorded in the RNA preparations from the strains grown in broth cultures was used as calibrator to calculate the relative fold change in gene expression by the 2−ΔΔCT threshold cycle (CT) method (Schmittgen, 2001).
Whole genome sequencing of the internalized E. coli strains
All bacterial strains internalized within Acanthamoeba and grown on the plates were subjected to whole genome sequencing (WGS) to investigate their genome’s integrity and stability. Total DNA was extracted from a 2 mL overnight culture of each strain grown in TSB at 37°C, with the GRS Genomic DNA Kit Bacteria (GRISP Research Solutions, Porto, Portugal). The genomes’ sequences were produced with an Ion Torrent S5 sequencing platform (Thermo Fisher Scientific, MA, USA). Libraries of about 400 bp from 100 ng of total DNA were prepared using the NEBNext® Fast DNA Fragmentation & Library Prep Set for Ion Torrent™ (#E6285L, New England BioLabs, MA, USA). Following preparation, library qualities and amounts were assessed using the Tape Station System 4200 with the High Sensitivity D1000 Reagents Kit (Agilent Technologies #5067-5585). Libraries were enriched on the Ion Chef System using the Ion 510™ & 520™ & 530™ Kit (#A34018, #A27754, #A27755, Ion Chef Supplies/Solutions, Thermo Fisher Scientific, MA, USA), and eventually subjected to sequencing.
Genomic characterization of the strains used in the internalization experiments
The bioinformatic analyses were carried out using the tools present in the Galaxy public server ARIES (https://www.iss.it/site/aries) (Knijn et al., 2020). The virulence genes content of the genomes was determined with the Patho_typing tool (https://github.com/B-UMMI/patho_typing) developed by the INNUENDO project (Llarena et al., 2018) using the E. coli virulence genes database (Joensen et al., 2014). Antimicrobial resistance genes were detected through ABRicate v0.8.13 using the ResFinder database (https://github.com/tseemann/abricate). Phylogenomics analysis was performed by determining the core genome MLST (cgMLST) using the chewBBACA tool (Silva et al., 2018) and the scheme developed by the INNUENDO project, which comprises 2,360 loci in total (Llarena et al., 2018; Silva et al., 2018). The distances between strains were calculated by pairwise comparison of the allelic profiles through the chewTree tool (https://www.iss.it/site/aries). The pairwise comparison was considered reliable when >80% of loci for each sample were assigned to an allele. For each pair of samples, the alleles which were not found, only partially found, or not correctly assigned to any locus were excluded from the analysis, as previously described (Gigliucci et al., 2021). The resulting dendrogram was visualized and modified using iTOL (https://itol.embl.de/ - Letunic and Bork, 2007).
Results
E. coli survival within Acanthamoeba sp. in view of long-term interactions
Our findings revealed that all E. coli strains tested were internalized and remained viable inside Acanthamoeba sp. for the entire length of the experiments, with various growth rates, depending on the strain (Table 2). In addition, Acanthamoeba sp. remained intact throughout all the incubation period without added nutrients at 37°C as assessed by microscope observation. All internalized STEC strains maintained their stx1; stx2; eae virulence genes profile (see Table 1 – Materials and Methods section) during all time (12h – 25 days). Interestingly, STEC strains did not show growth on plate at 20 days, (Table 2) whereas an intense bacterial growth was observed at 25 days (Table 2).
The gene rpoS is expressed in sampling times that did not show bacterial growth
As we could not see growth of bacterial cells in some sampling times (Table 2), an additional short-time internalization experiment was carried out to investigate the expression of rpoS gene in the internalized bacteria where we sampled the wells at 24 and 72 hours and at 10 days (Table 3). The rpoS is a conserved alternative sigma factor that regulates the expression of many stress response genes and is generally used a selective marker for the quantification of VBNC form of E. coli O157:H7 (Liu et al., 2010). In detail, our results showed that the gene rpoS was expressed at all sampling times, even without visible bacterial growth on the plates (Table 3 and Figure 1). The quantitative analysis showed that the level of rpoS expression ranged form 0.3-fold to 4.58-fold compared with the level of expression of this gene in the same strains recovered from broth cultures (Figure 1 and Supplementary Table 1). Strain ED638 showed the lowest levels of rpoS expression compared to all the other strains investigated, below the threshold of the calibrator at all sampling times (Figure 1). Strains EDL933 displayed the maximum level of rpoS transcription at 24 and 72 hours while strain ED605 produced the highest amount of rpoS mRNA at 72 hours, which declined slightly at ten days. The commensal E. coli strain showed a peculiar pattern of transcription of the rpoS gene reaching a peak of 4.58-fold at ten days while maintaining a basal level of transcription at the other two sampling points. The expression of rpoS gene in the absence of growth on plates suggests that the strains analyzed, could have remained in a quiescent state (VBNC) induced by activation of the cellular stress response.

Table 3 E. coli (CFU) intracellular of Acanthamoeba sp. at 24 hours, 72 hours and 10 days (short time intracellular assay).
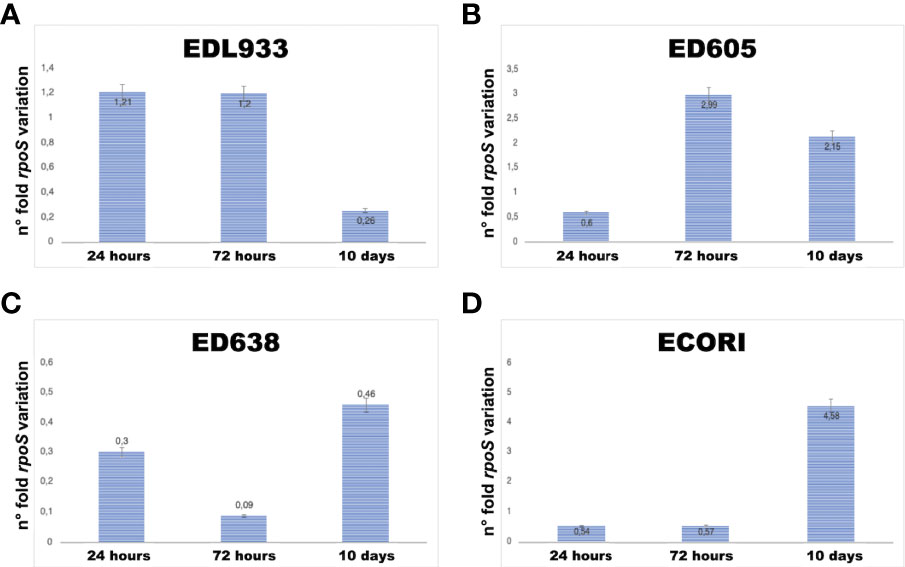
Figure 1 rpoS’ gene transcription profiles (A–D). The mRNA of the housekeeping gene gapA was used normalize the template (normalizator), while the rpoS mRNA of the same strains grown in broth at permissive conditions was used to calculate the relative fold change in gene expression by the 2−ΔΔCT threshold cycle (CT) method (calibrator).
Genomic characterization of the internalized E. coli strains
The median number of contigs obtained in the generated assemblies was 128 and the median N50 value was 121115 bp. Among the three STEC strains tested, two (EDL933 and ED638) belonged to ST11 and ED605 to ST1804, while ECORI was typed as ST10. As expected, all STEC strains harbored stx genes, subtype stx1a, alone (ED605) or in combination with stx2a (EDL933) and subtype stx2c in ED638. In addition, STEC strains showed the presence of the virulence genes eae, espA, espB and tir, encoded on the LEE locus, as well as nleB, a non-LEE encoded effector of the Type Three Secretion System (T3SS). Regarding the antimicrobial resistance genes, all the analyzed strains were positive for the presence of blaEC genes, encoding Class C β-lactamases. Interestingly, we have noticed in the genome retrieved from one colony of the internalized non-pathogenic ECORI strain (remained in the protozoa for 6 days – see Table 2) the presence of one astA gene’ allele encoding a thermostable toxin in different pathogenic E. coli strains, including STEC strains (Veilleux and Dubreuil, 2006); this gene was not present in the ECORI strain prior to the internalization experiments, and the observed allele (asta_4_ab042002) was different from that observed in the STEC strains used in the invasion assays (asta_8_hm099897) (Supplementary Table 2). The analysis of the genome of the ECORI strain carrying the asta_4_ab042002 allele (Acc. No. AB042002.1) showed that this sequence was part of a contig of 16.1 Kb carrying also the fae operon encoding the K88 fimbrial antigen of enterotoxigenic E. coli (Klemm, 1981), together with other genes, including some related with insertion sequences (data not shown). This contig was searched in the genome of the same ECORI sequenced before the internalization experiments using the BLASTn algorithm, with negative results.
Phylogenomics investigation
To investigate the genomes’ integrity and stability of the E. coli strains internalized in Acanthamoeba sp., a phylogenetic analysis was performed based on cgMLST. In addition, the genomes of the same bacterial strains’ grown in broth culture for 15 days were included in the analysis. The resulting dendrogram is shown in Figure 2.
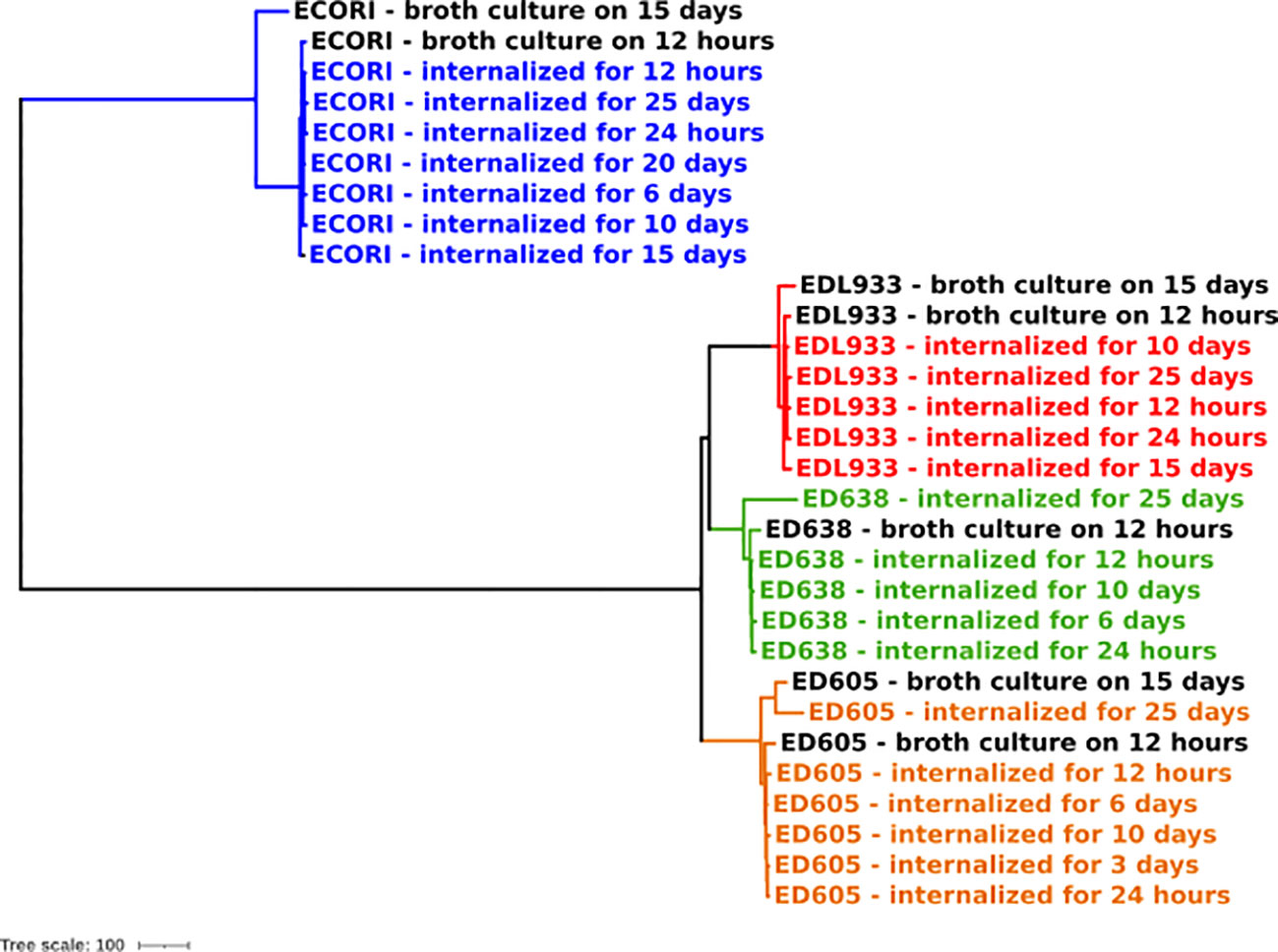
Figure 2 Phylogenomic analysis of E. coli strains internalized in Acanthamoeba sp. through cgMLST. The colors indicate the clade attributed to each bacterial strain.
The analysis showed four main clades, corresponding to each bacterial strain here analyzed (EDL933 – clade, ED605 – clade, ED638 – clade and ECORI - clade). Each clade was characterized by a very low variability pointing out a higher genome stability of E. coli strains internalized in Acanthamoeba sp., comparing to same strains grown in broth for 15 days (>100 allelic differences).
The maximum number of allelic distances within clades was much lower in some clades than in others. In detail, the maximum number of allelic differences (AD) detected between the strains’ internalized at the 25 days’ time point, were 23 AD in EDL933 clade, 110 AD in ED605 clade, 42 AD in ED638 clade, and 19 AD in ECORI clade.
Discussion
It is well known that free-living amoebae, especially Acanthamoeba spp., interact with many bacterial species and can play a role as their host. Despite that the mechanisms used by certain microorganisms to enter and remain vital in the amoeba are not completely clear, it is known that happens in nature and that this process may last few days or months (Rayamajhee et al., 2021). The fact that Acanthamoeba is a ubiquitous protozoan that can be found in many natural and/or man-made environments is relevant, making it necessary to understand the mechanisms underlying these interactions.
Several studies demonstrated that Acanthamoeba can interact with different pathogenic bacteria, including food-related pathogens, such as Legionella pneumophila, Vibrio cholerae, Listeria monocytogenes, Salmonella spp., Campylobacter sp., Helicobacter pylori, Yersinia enterocolitica, Bacillus anthracis and Staphylococcus aureus (incl. MRSA) (Balczun and Scheid, 2017; Scheid, 2018; Rayamajhee et al., 2021).
Unlike these well-studied interactions, association studies between FLA and STEC are scarce. Intracellular survival and multiplication of E. coli O157:H7 inside Acanthamoeba was first described by Barker et al., (1999). In this study, microscopical analysis revealed that several Acanthamoeba trophozoites contained ten or more E. coli cells within membrane bound vacuoles and that only in some of them bacterial digestion took place. Other authors also noted a reduced amoebal growth rate, and an increased amoebal mortality, mediated by Shiga toxins produced by E. coli in the cocultures. The same authors also showed that the Pho regulon was required for E. coli O157:H7 growth when cocultured with Acanthamoeba, whereas Shiga toxins as such, were not essential for bacterial survival in cocultures (Chekabab et al., 2012). The exact mechanisms by which the Pho regulon enhances intra-amoebal survival is not known, yet. Contradictory reports can be found on the Shiga toxins’ role of the internalized E. coli O157:H7; as a matter of fact, other studies showed that Stxs produced by internalized bacteria, were responsible for the killing of Acanthamoeba trophozoites (Chekabab et al., 2013; Arnold and Koudelka, 2014). Apart from the Pho regulon, little is known about the mechanisms involved in the interaction of STEC with FLA. Carruthers et al. (2010) described that during E. coli O157:H7 internalization in Acanthamoeba, several virulence genes, including Stxs and T3SS, and genes involved in the response to various stressful conditions (iron deprivation and oxidative stress) were upregulated.
In the present study, we investigated the internalization of STEC O157:H7 isolated from human disease or the animal reservoir, with different virulence gene profiles, into a wild strain of Acanthamoeba sp. as the host model. As E. coli O157:H7 encounters protozoa in the environment throughout its life cycle, we chose to use the well-studied and ubiquitous protozoan Acanthamoeba for this study. We observed that Acanthamoeba can internalize E. coli including STEC and that the internalized E. coli strains (both pathogenic and non-pathogenic) were able to survive within amoebae for at least 25 days.
These results extend the observations reported on the STEC O157 internalization to field isolates from (i) different sources and (ii) with diverse virulence genes assets. This finding is compatible with a role of Acanthamoeba in hosting STEC and possibly contributing to their environmental persistence, as well as in assisting STEC O157:H7 for enhanced survival during its route through the rumen of cattle. This could be similar to the Acanthamoeba spp. role as hosts for V. cholerae, Vibrio parahaemolyticus and Salmonella typhi in the aquatic environments of endemic areas (Laskowski-Arce and Orth, 2008; Abd et al., 2010; Sandstrom et al., 2010; Douesnard-Malo and Daigle, 2011; Matz et al., 2011). It’s interesting to point out that the survival within Acanthamoeba sp. was evidenced for STEC but also for the non-pathogenic commensal E. coli strain (ECORI). There are no reports describing this condition, however a non-invasive E. coli K-12 strain has been shown to be present intracellularly in amoebas, even if, after a short time it was lysed by amoebas, indicating E. coli K-12 as bacterial prey rather than an endosymbiont (Mungroo et al., 2021). We hypothesize that the strain used in this study, being a commensal in human intestine, may have acquired mechanisms allowing it to resist within the free-living amoebae. The intracellular assay’ results also showed that in different sampling times (15 days and 20 days) no bacterial growth was observed, but the vitality of the bacteria was restored at later times (25 days) (Table 2). This observation may be related with the internalized E. coli strains present in viable but non-cultivable (VBNC) forms, which is supported by the analysis of the expression of rpoS gene in the internalized STEC strains. As a matter of fact, our analysis exhibited the activation of the rpoS’ transcription during the bacterial permanence inside the protozoa, (Figure 1 A, B, D) except for the strain ED638, (Figure 1C) for which the expression of this gene remained at the basal level or below throughout the whole experiment’s duration. The lack of bacterial growth in this experiment (Table 3), together with the detection of rpoS transcription, suggests that E. coli strains were in a state of quiescence induced by the activation of the cellular stress response.
A similar finding was described for Aeromonas hydrophila. For this species, the shift to the VBNC form occurs on average twenty days earlier in the presence of Acanthamoeba than it does alone (Rahman et al., 2008). This would reduce the window for its detection using standard techniques and increases the likelihood of underreporting its presence in water systems, eventually augmenting the chances of human infection. Interestingly, the resuscitation of several species of VBNC bacteria upon being internalized by the amoebae has been documented by several authors (Steinert et al., 1997; García et al., 2007; Epalle et al., 2014; Casini et al., 2018; Dey et al., 2019; Dey et al., 2020) as it has been observed in this study (Table 2). To date, the mechanism of this shift is unknown and understanding the driving forces behind these interactions could allow developing more effective culture methods that might improve the detection of pathogens in their reservoirs and certain vehicles of infections.
Due to the high plasticity of the E. coli genome, the maintenance of bacterial quiescence state inside the amoebae would also have been indicated by a greater genomic stability. The cgMLST performed on the genomes of internalized E. coli at different sampling times (Table 2) showed a substantial genomic stability of the STEC strains kept inside Acanthamoeba (Figure 2) which adds evidence for the survival of the internalized strains in VBNC forms.
Furthermore, the analyses revealed that the STEC strains internalized in Acanthamoeba maintained the virulence genes asset intact, and therefore their genetic potential for pathogenicity. Even though the STEC virulence genes are mostly present on mobile genetic elements, all the strains internalized retained the presence of the bacteriophages-related Stx-coding genes and the plasmid encoded determinants associated with the STEC pathogenicity. The mobile genetic elements, and particularly phages, are frequently lost when the bacterial cells are subjected to stress. The retention of the Stx-phages in the internalized STEC is thus interesting. Nevertheless, this observation was not surprising in the light of the activation of the rpoS gene. As a matter of fact, the activation of the stress response and the overexpression of this gene has been associated with lower levels of Stx-converting bacteriophages induction, reducing their lytic activation, and conferring a better survival to the bacterial cells (Imamovic et al., 2016). Finally, the finding of the presence of the astA gene in the genome of the non-pathogenic ECORI strain growth after 6 days of permanence inside Acanthamoeba was noteworthy. This gene encodes a thermostable toxin produced by different pathogenic E. coli strains, including STEC (Veilleux and Dubreuil, 2006). In particular, the allele identified in this strain was identical to that described in an ETEC strain O42 (Acc. No. AB042002.1) and is not present in the genome of the ECORI strain used in the internalization experiments. The sequence analysis showed that the genome of the ECORI strain isolated at 6 days from the beginning of the internalization experiment possessed at least 16.1 Kb of DNA, comprised into a single contig, absent from the original ECORI isolate and containing also other genes characteristic of the ETEC pathotype, such as the genes faeG and faeE, part of an operon encoding the K88 fimbrial antigen (Klemm, 1981). The same contig also contained genes encoding IS-associated transposases (data not shown). The gene faeG has been described to be present in certain plasmids of hybrid ETEC-STEC hybrid strains (Michelacci et al., 2018), suggesting that a possible mechanism for its acquisition could be mediated by a plasmid, although, at this stage we cannot exclude that other mechanisms of horizontal gene transfer may have occurred. These observations could suggest a role for the protozoan as a “genes’ reservoir”. Whether the astA gene identified in the ECORI strain was derived from another E. coli strain simultaneously present in the Acanthamoeba or acquired in another different way, remains to be ascertained. Whatever the mechanism was, however, our findings suggest that the acquisition of exogenous genes by bacteria during their permanence into Acanthamoeba is possible and strengthen the role of this amoeba as a gene melting pot (Mungroo et al., 2021). In conclusion, our findings showed that E. coli, including STEC, can survive inside protozoan for extended periods of time. In addition, our results support the role of Acanthamoeba species in being potential host reservoir for STEC, putatively conferring to these microorganisms the ability to escape their detection in the animal reservoir and the vehicles of infection, while maintaining intact their pathogenic potential. Further research is currently ongoing to elucidate the complete pattern of mechanisms and strategies used by the two organisms to come to a balanced status where both gain advantages from the co-existence.
Data availability statement
The genome of ECORI strain internalized in the protozoa for 6 days together with the genome the of ECORI sequenced before the internalization experiments can be found in online repositories. The name of the repository and accession numbers can be found below: NCBI; PRJNA864586, SAMN30074962 - SAMN30074963.
Author contributions
MMDF isolated and characterized the wild type amoebae strain, set up the experimental settings, and drafted the manuscript. LC performed the invasion assays and the analysis of the expression of rpoS. AB, PC, and FM contributed to the setup of experiments, to the scientific discussion and helped with the revision of the draft manuscript. MM and MC performed the Ion Torrent library preparation and sequencing. AK developed the tools used for the bioinformatic analysis and maintain the ARIES webserver. SM conceived the study, contributed to the scientific discussion and thoroughly revised the manuscript. All authors contributed to the article and approved the submitted version.
Funding
The study was supported by intramural funds of the Istituto Superiore di Sanità.
Conflict of interest
The authors declare that the research was conducted in the absence of any commercial or financial relationships that could be construed as a potential conflict of interest.
Publisher’s note
All claims expressed in this article are solely those of the authors and do not necessarily represent those of their affiliated organizations, or those of the publisher, the editors and the reviewers. Any product that may be evaluated in this article, or claim that may be made by its manufacturer, is not guaranteed or endorsed by the publisher.
Supplementary material
The Supplementary Material for this article can be found online at: https://www.frontiersin.org/articles/10.3389/fcimb.2022.926127/full#supplementary-material
Supplementary Table 1 | For each tested strain, the table includes the statistics of the rpoS’ gene expression.
Supplementary Table 2 | For each tested strain, the table includes the statistics of the assembled contigs, details on sequencing technology used and the virulence genes content of the genomes. Highlighted in yellow the astA gene’ allele encoding a thermostable toxin not present in the other ECORI strain.
References
Abd, H., Valeru, S. P., Sami, S. M., Saeed, A., Raychaudhuri, S., Sandstrom, G. (2010). Interaction between Vibrio mimicus and Acanthamoeba castellanii. Environ. Microbiol. Rep. 2, 166–171. doi: 10.1111/j.1758-2229.2009.00129.x
Arnold, J. W., Koudelka, G. B. (2014). The Trojan horse of the microbiological arms race: phage-encoded toxins as a defence against eukaryotic predators. Environ. Microbiol. 16, 454–466. doi: 10.1111/1462-2920.12232
Bai, X., Fu, S., Zhang, J., Fan, R., Xu, Y., Sun, H., et al. (2018). Identification and pathogenomic analysis of an Escherichia coli strain producing a novel shiga toxin 2 subtype. Sci. Rep. 8 (1), 6756. doi: 10.1038/s41598-018-25233-x
Balczun, C., Scheid, P. L. (2017). Free-living amoebae as hosts for and vectors of intracellular microorganisms with public health significance. Viruses 9 (4), 65. doi: 10.3390/v9040065
Barker, J., Humphrey, T. J., Brown, M. W. (1999). Survival of Escherichia coli 0157 in a soil protozoan: implications for disease. FEMS Microbiol. Lett. 173 (2), 291–295. doi: 10.1111/j.1574-6968.1999.tb13516.x
Berk, S., Garduno, R. (2012). The Tetrahymena and Acanthamoeba model systems. Methods Mol. Biol. 954, 393–416. doi: 10.1007/978-1-62703-161-5_25
Caprioli, A., Morabito, S., Brugere, H., Oswald, E. (2005). Enterohaemorrhagic Escherichia coli: Emerging issues on virulence and modes of transmission. Vet. Res. 36, 289–311. doi: 10.1051/vetres:2005002
Carruthers, M. D., Bellaire, B. H., Minion, F. C. (2010). Exploring the response of Escherichia coli O157:H7 EDL933 within Acanthamoeba castellanii by genome-wide transcriptional profiling. FEMS Microbiol. Lett. 312, 15–23. doi: 10.1111/j.1574-6968.2010.02098.x
Casini, B., Baggiani, A., Totaro, M., Mansi, A., Costa, A. L., Aquino, F., et al. (2018). Detection of viable but non-culturable legionella in hospital water network following monochloramine disinfection. J. Hosp. Infect. 98, 46–52. doi: 10.1016/j.jhin.2017.09.006
Chekabab, S. M., Daigle, F., Charette, S. J., Dozois, C. M., Harel, J. (2012). Survival of enterohemorrhagic Escherichia coli in the presence of Acanthamoeba castellanii and its dependence on pho regulon. Microbiol. Open 1 (4), 427–437. doi: 10.1002/mbo3.40
Chekabab, S. M., Daigle, F., Charette, S. J., Dozois, C. M., Harel, J. (2013). Shiga toxins decrease enterohaemorrhagic Escherichia coli survival within Acanthamoeba castellanii. FEMS Microbiol. Lett. 344, 86–93. doi: 10.1111/1574-6968.12158
Dey, R., Rieger, A., Banting, G., Ashbolt, N. J. (2020). Role of amoebae for survival and recovery of “non-culturable” Helicobacter pylori cells in aquatic environments. FEMS Microbiol. Ecol. 96, 1–11. doi: 10.1093/femsec/fiaa182
Dey, R., Rieger, A. M., Stephens, C., Ashbolt, N. J. (2019). Interactions of Pseudomonas aeruginosa with Acanthamoeba polyphaga observed by imaging flow cytometry. Cytom. Part A 95, 555–564. doi: 10.1002/cyto.a.23768
Douesnard-Malo, F., Daigle, F. (2011). Increased persistence of Salmonella enterica serovar typhi in the presence of Acanthamoeba castellanii. Appl. Environ. Microbiol. 77, 7640–7646. doi: 10.1128/AEM.00699-11
Epalle, T., Girardot, F., Allegra, S., Maurice-Blanc, C., Garraud, O., Riffard, S. (2014). Viable but not culturable forms of Legionella pneumophila generated after heat shock treatment are infectious for macrophage-like and alveolar epithelial cells after resuscitation on Acanthamoeba polyphaga. Microb. Ecol. 69, 215–224. doi: 10.1007/s00248-014-0470-x
Fitzmaurice, J., Glennon, M., Duffy, G., Sheridan, J. J., Carroll, C., Maher, M. (2004). Application of real-time PCR and RT-PCR assays for the detection and quantitation of VT 1 and VT 2 toxin genes in E. coli O157:H7. Mol. Cell. Probes 18, 123–132. doi: 10.1016/j.mcp.2003.10.004
García, M. T., Jones, S., Pelaz, C., Millar, R. D., Abu Kwaik, Y. (2007). Acanthamoeba polyphaga resuscitates viable non-culturable Legionella pneumophila after disinfection. Environ. Microbiol. 9, 1267–1277. doi: 10.1111/j.1462-2920.2007.01245.x
Gigliucci, F., van Hoek, A. H. A. M., Chiani, P., Knijn, A., Minelli, F., Scavia, G., et al. (2021). Genomic characterization of hlyF-positive shiga toxin-producing Escherichia coli, Italy and the Netherlands 2000-2019. Emerg. Infect. Dis. 27 (3), 853–861. doi: 10.3201/eid2703.203110
Greub, G., Raoult, D. (2004). Microorganisms resistant to free-living amoebae. Clin. Microbiol. Rev. 17 (2), 413–433. doi: 10.1128/cmr.17.2.413-433.2004
Gyles, C. L. (2007). Shiga toxin-producing Escherichia coli: an overview. J. Anim. Sc. 85, 45–62. doi: 10.2527/jas.2006-508
Henriquez, F. L., Mooney, R., Bandel, T., Giammarini, E., Zeroual, M., Fiori, P. L., et al. (2021). Paradigms of Protist/Bacteria symbioses affecting human health: Acanthamoeba species and Trichomonas vaginalis. Front. Microbiol. 11. doi: 10.3389/fmicb.2020.616213
Imamovic, L., Ballesté, E., Martínez-Castillo, A., García-Aljaro, C., Muniesa, M. (2016). Heterogeneity in phage induction enables the survival of the lysogenic population. Environ. Microbiol. 18 (3), 957–969. doi: 10.1111/1462-2920.13151
Jacquier, N., Aeby, S., Lienard, J., Greub, G. (2013). Discovery of new intracellular pathogens by amoebal coculture and amoebal enrichment approaches. J. Vis. Exp. 27, 51055. doi: 10.3791/51055
Joensen, K. G., Scheutz, F., Lund, O., Hasman, H., Kaas, R. S., Nielsen, E. M., et al. (2014). Real-time whole-genome sequencing for routine typing, surveillance, and outbreak detection of verotoxigenic Escherichia coli. J. Clin. Microbiol. 52, 1501–1510. doi: 10.1128/JCM.03617-13
Jung, S. Y., Matin, A., Kim, K. S., Khan, N. A. (2007). The capsule plays an important role in Escherichia coli K1 interactions with Acanthamoeba. Int. J. Parasitol. 37, 417–423. doi: 10.1016/j.ijpara.2006.10.012
Khan, N. A. (2006). Acanthamoeba: Biology and increasing importance in human health. FEMS Microbiol. Rev. 30, 564–595. doi: 10.1111/j.1574-6976.2006.00023.x
Klemm, P. (1981). The complete amino-acid sequence of the K88 antigen, a fimbrial protein from Escherichia coli. Eur. J. Biochem. 117, 617–627. doi: 10.1111/j.1432-1033.1981.tb06382.x
Knijn, A., Michelacci, V., Orsini, M., Morabito, S. (2020). Advanced research infrastructure for experimentation in GenomicS (ARIES): A lustrum of galaxy experience. biorxiv 2020.05.14.095901, 1–16. doi: 10.1101/2020.05.14.095901
Lacher, D. W., Gangiredla, J., Patel, I., Elkins, C. A., Feng, P. C. (2016). Use of the Escherichia coli identification microarray for characterizing the health risks of shiga toxin-producing Escherichia coli isolated from foods. J. Food Prot. 79 (10), 1656–1662. doi: 10.4315/0362-028X.JFP-16-176
Lambrecht, E., Baré, J., Chavatte, N., Bert, W., Sabbe, K., Houf, K. (2015). Protozoan cysts act as a survival niche and protective shelter for foodborne pathogenic bacteria. Appl. Environ. Microbial. 81 (16), 5604–5612. doi: 10.1128/AEM.01031-15
Laskowski-Arce, M. A., Orth, K. (2008). Acanthamoeba castellanii promotes the survival of Vibrio parahaemolyticus. Appl. Environ. Microbiol. 74, 7183–7188. doi: 10.1128/AEM.01332-08
Letunic, I., Bork, P. (2007). Interactive tree of life (iTOL): An online tool for phylogenetic tree display and annotation. Bioinformatics 23 (1), 127–128. doi: 10.1093/bioinformatics/btl529
Liu, Y., Wang, C., Fung, C., Li, X. F. (2010). Quantification of viable but nonculturable Escherichia coli O157:H7 by targeting the rpoS mRNA. Anal. Chem. 82 (7), 2612–2615. doi: 10.1021/ac1003272
Llarena, A. K., Ribeiro-Gonçalves, B. F., Silva, D. N., Halkilahti, J., Machado, M. P., Da Silva, M. S., et al. (2018). INNUENDO: A cross-sectoral platform for the integration of genomics in the surveillance of food-borne pathogens. EFSA supporting publication 2018: EN-1498 15, 142 pp. doi: 10.2903/sp.efsa.2018.EN-1498.
Loret, J. F., Greub, G. (2010). Free-living amoebae: Biological by-passes in water treatment. Int. J. Hyg Environ. Health 213 (3), 167–175. doi: 10.1016/j.ijheh.2010.03.004
Matz, C., Nouri, B., McCarter, L., Martinez-Urtaza, J. (2011). Acquired type III secretion system determines environmental fitness of epidemic Vibrio parahaemolyticus in the interaction with bacterivorous protists. PloS One 6, e20275. doi: 10.1371/journal.pone.0020275
Melton-Celsa, A. R. (2014). Shiga toxin (Stx) classification, structure, and function. Microbiol. Spectr. 2 (4), 1–21. doi: 10.1128/microbiolspec.EHEC-0024-2013
Michelacci, V., Maugliani, A., Tozzoli, R., Corteselli, G., Chiani, P., Minelli, F., et al. (2018). Characterization of a novel plasmid encoding F4-like fimbriae present in a shiga-toxin producing enterotoxigenic Escherichia coli isolated during the investigation on a case of hemolytic-uremic syndrome. Int. J. Med. Microbiol. 308, 947–955. doi: 10.1016/j.ijmm.2018.07.002
Mungroo, M. R., Siddiqui, R., Khan, N. A. (2021). War of the microbial world: Acanthamoeba spp. interactions with microorganisms. Folia Microbiol. (Praha). 66 (5), 689–699. doi: 10.1007/s12223-021-00889-7
Nataro, J. P., Kaper, J. B. (1998). Diarrheagenic Escherichia coli. Clin. Microbiol. Rev. 11, 142–201. doi: 10.1128/CMR.11.1.142
Page, F. C. (1988). A new key to freshwater and soil gymnamoebae. Culture Collection of Algae and Protozoa, Ambleside, 122pp. CCAP Publication. FBA
Paton, A. W., Paton, J. C. (1998). Detection and characterization of shiga toxigenic Escherichia coli by using multiplex PCR assays for stx1, stx2, eaeA, enterohemorrhagic E. coli hlyA, rfbO111, and rfbO157. J. Clin. Microbiol. 36 (2), 598–602. doi: 10.1128/JCM.36.2.598-602.1998
Rahman, M., Abd, H., Romling, U., Sandstrom, G., Möllby, R. (2008). Aeromonas-acanthamoeba interaction and early shift to a viable but nonculturable state of Aeromonas by Acanthamoeba. J. Appl. Microbiol. 104, 1449–1457. doi: 10.1111/j.1365-2672.2007.03687.x
Rayamajhee, B., Subedi, D., Peguda, H. K., Willcox, M. D., Henriquez, F. L., Carnt, N. (2021). A systematic review of intracellular microorganisms within Acanthamoeba to understand potential impact for infection. Pathogens 10, 225. doi: 10.3390/pathogens10020225
Sandstrom, G., Saeed, A., Abd, H. (2010). Acanthamoeba polyphaga is a possible host for Vibrio cholerae in aquatic environments. Exp. Parasitol. 126, 65–68. doi: 10.1016/j.exppara.2009.09.021
Scheid, P. (2018). Free-living amoebae as human parasites and hosts for pathogenic microorganisms. Proceedings 2 (11), 692. doi: 10.3390/proceedings2110692
Scheutz, F., Teel, L. D., Beutin, L., Piérard, D., Buvens, G., Karch, H., et al. (2012). Multicenter evaluation of a sequence-based protocol for subtyping shiga toxins and standardizing stx nomenclature. J. Clin. Microbiol. 50 (9), 2951–2963. doi: 10.1128/JCM.00860-12
Schmittgen, T. D. (2001). Real-time quantitative PCR. Methods 25, 383–385. doi: 10.1006/meth.2001.1260
Siddiqui, R., Khan, N. A. (2012). Acanthamoeba is an evolutionary ancestor of macrophages: a myth or reality? Exp. Parasitol. 130, 95–97. doi: 10.1016/j.exppara.2011.11.005
Silva, M., Machado, M. P., Silva, D. N., Rossi, M., Moran-Gilad, J., Santos, S., et al. (2018). chewBBACA: A complete suite for gene-by-Gene schema creation and strain identification. Microb. Genom. 4 (3), e000166. doi: 10.1099/mgen.0.000166
Steinert, M., Emödy, L., Amann, R., Hacker, J. (1997). Resuscitation of viable but nonculturable Legionella pneumophila philadelphia JR32 by Acanthamoeba castellanii. Appl. Environ. Microbiol. 63, 2047–2053. doi: 10.1128/aem.63.5.2047-2053.1997
Thomas, V., McDonnell, G., Denyer, S. P., Maillard, J. Y. (2010). Free-living amoebae and their intracellular pathogenic microorganisms: risks for water quality. FEMS Microbiol. Rev. 34 (3), 231–259. doi: 10.1111/j.1574-6976.2009.00190.x
Tozzi, A. E., Caprioli, A., Minelli, F., Gianviti, A., De Petris, L., Edefonti, A., et al. (2003). Shiga toxin-producing escherichia coli infections associated with hemolytic uremic syndrome, italy 1988-2000. Emerg. Infect. Dis. 9 (1), 106–108. doi: 10.3201/eid0901.020266
Vaerewijck, M., Baré, J., Lambrecht, E., Sabbe, K., Houf, K. (2014). Interactions of foodborne pathogens with free-living protozoa: potential consequences for food safety. Compr. Rev. Food Sci. Food Saf. 13, 924–944. doi: 10.1111/1541-4337.12100
Veilleux, S., Dubreuil, J. D. (2006). Presence of Escherichia coli carrying the EAST1 toxin gene in farm animals. Vet. Res. 37 (1), 3–13. doi: 10.1051/vetres:2005045
Yang, X., Bai, X., Zhang, J., Sun, H., Fu, S., Fan, R., et al. (2019). Escherichia coli strains producing a novel shiga toxin 2 subtype circulate in china. Int. J. Med. Microbiol. 10 (1), 151377. doi: 10.1016/j.ijmm.2019.151377
Keywords: Shiga toxin producing Escherichia coli (STEC), free- living amoebae (FLA), E. coli O157, food-safety, zoonosis
Citation: Montalbano Di Filippo M, Boni A, Chiani P, Marra M, Carollo M, Cristofari L, Minelli F, Knijn A and Morabito S (2022) Exploring the nature of interaction between shiga toxin producing Escherichia coli (STEC) and free-living amoeba - Acanthamoeba sp. Front. Cell. Infect. Microbiol. 12:926127. doi: 10.3389/fcimb.2022.926127
Received: 16 May 2022; Accepted: 15 August 2022;
Published: 09 September 2022.
Edited by:
Charles Martin Dozois, Université du Québec, CanadaReviewed by:
Jorge Alberto Giron, University of Puebla, MexicoDavid A. Montero, University of Chile, Chile
Lucia Galli, National University of La Plata, Argentina
Copyright © 2022 Montalbano Di Filippo, Boni, Chiani, Marra, Carollo, Cristofari, Minelli, Knijn and Morabito. This is an open-access article distributed under the terms of the Creative Commons Attribution License (CC BY). The use, distribution or reproduction in other forums is permitted, provided the original author(s) and the copyright owner(s) are credited and that the original publication in this journal is cited, in accordance with accepted academic practice. No use, distribution or reproduction is permitted which does not comply with these terms.
*Correspondence: Margherita Montalbano Di Filippo, bWFyZ2hlcml0YS5tb250YWxiYW5vZGlmaWxpcHBvQGlzcy5pdA==