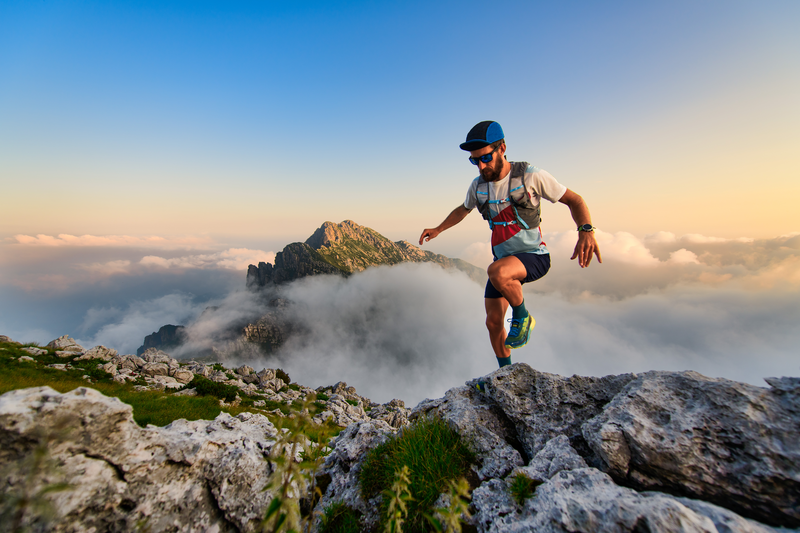
94% of researchers rate our articles as excellent or good
Learn more about the work of our research integrity team to safeguard the quality of each article we publish.
Find out more
ORIGINAL RESEARCH article
Front. Cell. Infect. Microbiol. , 22 July 2022
Sec. Microbiome in Health and Disease
Volume 12 - 2022 | https://doi.org/10.3389/fcimb.2022.922753
This article is part of the Research Topic Next-Generation Sequencing in Ophthalmology: The Microbiome in Ocular Health and Disease View all 9 articles
Purpose: To compare the ocular surface and meibum microbial communities of humans with Demodex Blepharitis (DB) and healthy controls.
Methods: Conjunctival sac and meibum samples from 25 DB patients and 11 healthy controls were analyzed using metagenomic next-generation sequencing (mNGS).
Results: The alpha-diversity of the conjunctival sac microbiome of the DB group (observed, Chao1, ACE) was lower than that of the control group, whereas all meibum diversity indicators were similar. In conjunctival samples, the relative abundance (RA) of the phylum Proteobacteria was significantly higher (p=0.023), and the RA of both phyla Actinobacteria and Firmicutes was significantly lower (p=0.002, 0.025, respectively) in the DB group than that in the control group. In meibum samples, the RA of the phyla Proteobacteria and Actinobacteria were similar, whereas that of the phylum Firmicutes was significantly lower in the DB group (p=0.019) than that in the control group. Linear discriminant analysis with effect size measurement of the conjunctival and meibum microbiomes showed that Sphingobium sp. YG1 and Acinetobacter guillouiae were enriched in the DB group. Sphingobium sp. YG1, Acinetobacter guillouiae and Pseudomonas putida in the DB group were related to more severe ocular surface clinical parameters. Discriminative genera’s principal coordinate analysis separated all control and DB microbiomes into two distinct clusters.
Conclusions: Proteobacteria’s increased prevalence may indicate ocular microbial community instability. The species Sphingobium sp. YG1 and Acinetobacter guillouiae are potentially pathogenic bacterial biomarkers in DB. Demodex infection mainly affects the ocular surface microbiome rather than penetrating deeper into the meibomian gland.
The ocular surface microbiome is an important component of the ocular surface. In healthy people, microbes coexisting in the ocular surface or other organs maintain a stable state of competition and cooperation, such as competition with each other or mutual use of resources, nutrition, and space (Lee et al., 2012; Li et al., 2019). The relative abundance (RA) of each member of the microbiome and interactions among microbial species are crucial for the homeostasis and sustainability of ecosystems (Wintermute and Silver, 2010; Kumar et al., 2016). Disruption of the stable state can lead to ocular surface diseases, such as dry eye, meibomian gland dysfunction (MGD), and blepharitis (Zhu et al., 2018; de Paula et al., 2019; Yan et al., 2020).
Blepharitis is a chronic inflammatory process of the eyelid margin that may result in tear film changes, eye irritation symptoms, clinically apparent inflammation, and ocular surface disease (Amescua et al., 2019). An important etiological factor of blepharitis is Demodex mites (Coston, 1967; Rodríguez et al., 2005; Zhao et al., 2012). D. folliculorum and D. brevis are the most common ectoparasites on the ocular surface (Cheng et al., 2015). D. folliculorum primarily inhabits lash follicles, whereas D. brevis infests lash sebaceous and meibomian glands (MGs). The probable pathogenic role of Demodex in blepharitis includes direct mechanical abrasion by mite claws and MG orifice obstructions, inflammatory responses elicited by mite debris or waste, and bacteria carried by Demodex mites (Zhu et al., 2018). Therefore, the identification of microbial communities on the ocular surface of Demodex blepharitis (DB) patients can help to clarify the pathological mechanisms and provide valuable information for prevention or treatment.
Culture-based and culture-independent approaches, including polymerase chain reaction, 16S rRNA sequencing, and denaturing gradient gel electrophoresis, have been applied to study ocular microbial communities (Zhang et al., 2017; Jiang et al., 2018; Zhu et al., 2018; Kang et al., 2021). Development of culture-independent approaches, such as 16S rRNA comparison, has demonstrated that the ocular surface microbiota is more diverse in composition than as deduced from culture-based methods (Yan et al., 2020; Kang et al., 2021). Nowadays, a few studies had investigated the effect of Demodex mites on ocular surface flora by 16S rRNA or culture methods, but the results were quite different (Zhu et al., 2018; Yan et al., 2020; Liang et al., 2021). Compared to 16s rRNA sequencing, metagenomic next-generation sequencing (mNGS) has the advantage of sequencing all genomic DNA in a given sample, leading to a higher resolution and detection of more species, and also including taxa of the viruses, eukaryotes, and superkingdoms archea (Ranjan et al., 2016). To our best knowledge, mNGS had never been used to investigate the microbial communities in the ocular surface and meibum of DB patients. Here, we applied mNGS technology to compare the diversity and interactions of microbial communities in conjunctival swabs and meibum obtained from DB patients and healthy controls.
Twenty-five DB patients who visited the Eye Hospital, Wenzhou Medical University for ophthalmic examinations between June 2019 and May 2020 and 11 healthy controls without blepharitis or MGD were enrolled in our study. Informed consent was obtained from all the participants. This study was approved by the Ethical Committee of the Eye Hospital of Wenzhou Medical University and registered at ClinicalTrials.gov: NCT04451122. All methods were conducted in accordance with the tenets of the Declaration of Helsinki. Demodex blepharitis was diagnosed based on the diagnostic criteria (presence of at least one symptom, such as redness, eye itching, foreign body sensation, abnormal eyelashes with cylindrical dandruff in both eyes, and positive results on light microscopic examination of the eyelashes) and age >20 years (Liang et al., 2017). According to the diagnostic criteria, three lashes with retained cylindrical dandruff were removed from each lid and placed separately on each end of a glass slide for a total of 12 lashes on four slides. If at least three or more Demodex bodies, including adult, larva, protonymph, or nymph stage of D. folliculorum or D. brevis, were found on any of the four slides, the result was considered positive. Control participants had never been diagnosed with blepharitis or MGD, were aged >20 years, and had clean eyelashes without cylindrical dandruff or squamous cell debris (collarette). Participants wearing contact lenses, with chalazion, active ocular or nasolacrimal infections, severe systemic diseases, a history of probiotic treatment within the previous 6 months, systemic antibiotic drugs within the previous 3 months, or topical antibiotics within the previous week were excluded from the study. Participants were asked to withhold any topical medications for 48 h before sample collection. To avoid contamination during sample collection, the samples were collected in an ophthalmic treatment room sterilized with ultraviolet light twice a day for at least one hour each time, and for more than half an hour before each sampling. Conjunctival swab samples were collected using sterile transport swabs (IngeniGen XMK Biotechnologies Inc. Zhejiang, China) and were taken from the lower conjunctival sac and MGs. MG secretions from the first squeeze were discarded to avoid contamination from eyelid margins. Another sterile swab was used to collect the secretions from the second squeeze, which was thought to be the meibum in the deep segment of MGs The sterile swabs containing the samples were immediately placed into a sterile tube containing preservation solution and were stored in an ultralow temperature freezer at -80°C before DNA extraction.
Clinical assessments were performed sequentially as follows: SPEED questionnaire, fluorescein tear break-up time (TBUT), corneal fluorescein staining (CFS) (Song et al., 2013), lid abnormality (LAM) (Fu et al., 2021), meibum expressibility, and meibography. We assessed the meibum expressibility of 15 glands in each lower eyelid (0–45) (Lane et al., 2012). Images of upper and lower MGs were captured using Keratograph 5M (K5M; Oculus, Wetzlar, Germany). The meiboscore was determined as follows: 0, no MG atrophy; 1, MG atrophy of <1/3 of the total lid area; 2, MG atrophy of 1/3 to 2/3 of the total lid area; 3, MG atrophy of > 2/3 of the total lid area. The upper and lower eyelids’ scores were added to yield the final meiboscore (range, 0–6) (Arita et al., 2008).
DNA was extracted from the swabs using a DNA extraction kit (IngeniGen XMK Biotechnologies Inc., Zhejiang, China), according to the manufacturer’s instructions. Before DNA extraction, an internal control bacterium was added to the samples. DNA concentration was measured using a Qubit® 4.0 Fluorometer (Thermo Fisher Scientific, Waltham, MA). DNA libraries were constructed using the Ingenigen XMKbio DNA-seq Library Prep Kit (IngeniGen XMK Biotechnologies, Inc.) using the Tn5 transposase method. DNA library concentrations were measured using a Qubit® 4.0 Fluorometer (Thermo Fisher Scientific), and their quality was evaluated using an Agilent 4200 TapeStation system (Agilent Technologies, Santa Clara, CA). Qualified libraries with different barcodes were then pooled accordingly. Blank tubes with unused swabs and sterile water were used as blank extraction-negative controls during DNA extraction and library preparation to filter reagent and laboratory environmental contamination taxa. The “environmental” species with a frequency of more than 10% in the negative controls (pre-determined by ingeniSeq-MG V1.0 mNGS software) over the past 100 runs were considered as contaminants and filtered out from the final results. Sequencing was performed on an Illumina Nextseq550 using a 75-bp single-end sequencing mode.
Raw metagenomic shotgun reads were quality-checked and trimmed using fastp (Chen et al., 2018). Sequences were aligned with the human reference genome (GRCh38) using bowtie2 (Langmead and Salzberg, 2012) to remove human genome sequences; thus, the unaligned reads were recovered. Background sequences from run processing were also filtered from the recovered unaligned reads. All non-host reads were assumed to be microbe-related. Kraken2 was used for taxonomic classification (Wood et al., 2019). HUMAnN3 (Beghini et al., 2021) was used for KEGG pathway analysis. Permutational multivariate analysis of variance (PERMANOVA) between HC and DB groups was done with adonis in vegan with a similarity index using 9999 permutations. All further bioinformatics analyses, data visualization, and statistical analyses were performed in R (version 4.0) (Team R Development Core, 2018) using the vegan (Dixon, 2003), ggplot2, and microeco (Liu et al., 2021) packages.
The contaminant filtering step removed samples with insufficient sequences (<10,000) as well as operational taxonomic units present at <0.0001% RA. Data were statistically analyzed using SPSS software (version 20.0; IBM SPSS Corp., Chicago, IL). Independent-sample t-tests, chi-square tests, and Mann–Whitney U tests were used to compare the differences in age, sex, and clinical examination results between DB patients and controls. The Mann–Whitney U test was performed to analyze the alpha-diversity indices and relative abundances of dominant phyla, genera, and species between groups. The generalized estimating equation was used to assess the relationship between ocular surface parameters and relative abundances of top species. Statistical significance was set at p<0.05.
Fifty eyes from 25 DB patients and 22 eyes from 11 healthy adults were enrolled in the study. The clinical parameters of DB patients and healthy controls are shown in Table 1. The SPEED scores, TBUT, CFS, LAM, meiboscores, and meibum expressibility were significantly higher in DB patients than in controls (Table 1).
Whole metagenomes were generated from the conjunctival swab and meibum of control (n=11) and DB (n=25) patients in this study. Overall, 1249.17 million reads were generated for the 72 samples, with an average of 19.8 million reads per sample. The average RAs of bacteria, fungi, viruses, and unclassified bacteria were 94.9%, 1.0%, 3.8%, and 0.18%, respectively, in conjunctival swabs and 95.5%, 0.8%, 3.2%, and 0.36%, respectively, in meibum groups. Bacterial taxonomic assignment and hierarchical classification of the reads revealed 12 phyla, 222 genera, and 575 species in conjunctival swabs and 11 phyla, 221 genera, and 508 species in meibum in both groups.
Figure 1 shows the comparisons of alpha-diversity of conjunctival swab and meibum samples between the two groups. The conjunctival sac microbiome community population of the DB group (observed, Chao1, ACE) was lower than that of the control group, whereas their community diversities (Shannon and Simpson) were similar (Figure 1). In the meibum samples, there was no significant difference in the alpha-diversity between the two groups. The conjunctival sac had lower diversity (observed, Chao1, ACE, Shannon) than the meibum in both groups. Rarefaction curves were plotted for all bacterial microbiomes, and most samples showed a tendency towards saturation, indicating that sufficient depth and coverage had been achieved during sampling.
Figure 1 The comparisons of the alpha-diversity indices (A, Observed ; B, Chao1 ; C, ACE ; D, Shannon ; E, Simpson) in the conjunctival sac and meibum microbiome between the two groups. HC, Healthy control; DB, Demodex blepharitis.
Table 2 shows that there were five bacterial flora phyla (Proteobacteria, Actinobacteria, Firmicutes, Bacteroidetes, and Deinococcus-Thermus), one phylum from the eukaryotic microbiome (Apicomplexa), and one virus phylum (Uroviricota), which had a RA>1%, in each group. Proteobacteria, Actinobacteria, and Firmicutes were predominant. The differences in the mean abundances of Proteobacteria, Actinobacteria, and Firmicutes between the DB and control groups’ conjunctival swab samples were significant (p<0.05, Table 2). Similarly, the difference in the mean abundance of Firmicutes between the DB and control groups’ meibum samples was significant (p<0.05, Table 2); however, those of Proteobacteria and Actinobacteria were not significant (Table 2).
Table 2 Phyla in the conjunctival swab or meibum samples of controls and Demodex blepharitis groups.
At the genus level, 16 genera in conjunctival swab samples and 18 genera in meibum samples with >1% average RA are shown in Table 3. In conjunctival samples, the top-five genera (in the order of RA) of controls and DB groups were Pseudomonas, Cutibacterium, Acinetobacter, Sphingomonas, Staphylococcus, and Acinetobacter, Delftia, Pseudomonas, Sphingobium, Chryseobacterium, respectively (Figure 2; Table 3); and the RA of the above genera, except for Pseudomonas, was statistically significantly different between the two groups (Table 3). Similar to the conjunctival swab samples, the top-five genera of meibum samples (in the order of RA) of controls and DB were Pseudomonas, Cutibacterium, Acinetobacter, Staphylococcus, Stenotrophomonas, and Acinetobacter, Delftia, Pseudomonas, Sphingobium, Chryseobacterium, respectively (Figure 3; Table 3); and the RA of these genera was statistically significantly different between the two groups (Table 3).
Table 3 Genera in the conjunctival swab or meibum samples of controls and Demodex blepharitis groups (Relative Abundance>1%).
Figure 2 Differences in the relative abundances of microbial genera in the conjunctival sac samples of the control and Demodex blepharitis groups. HC, Healthy control; DB, Demodex blepharitis.
Figure 3 Differences in the relative abundance of microbial genera in the meibum samples of the control and Demodex blepharitis groups. HC, Healthy control; DB, Demodex blepharitis.
Table 4 shows the species with an average RA>1% in the conjunctival swab or meibum samples of the control and DB groups. In conjunctival swab samples, the top-five species (in the order of RA) of the control and Demodex blepharitis groups were Cutibacterium acnes, Toxoplasma gondii, Moraxella osloensis, Staphylococcus hominis, Stenotrophomonas maltophilia, and Delftia tsuruhatensis, Acinetobacter johnsonii, Pseudomonas putida, Acinetobacter guillouiae, Acinetobacter sp. MYb10, respectively; and the RA of the above species, except that of Toxoplasma gondii, showed statistically significant differences between the two groups. In meibum samples, the top-five species (in the order of RA) in the controls and Demodex blepharitis patients were Cutibacterium acnes, Stenotrophomonas maltophilia, Moraxella osloensis, Staphylococcus hominis, Pseudomonas veronii, and Delftia tsuruhatensis, Sphingobium sp. YG1, Acinetobacter johnsonii, Acinetobacter guillouiae, Pseudomonas putida, respectively; and the RAs of these species were statistically significantly different between the two groups.
Table 4 Species in the conjunctival swab or meibum samples of controls and Demodex blepharitis groups (Relative Abundance>1%).
Linear discriminant analysis (LDA) combined with effect size measurement (LEfSe) analysis of the tax showed that both conjunctival swab and meibum microbiota of DB were enriched in Sphingobium sp. YG1 (species-level)-Sphingobium (genus-level)-Proteobacteria (phylum-level) and Acinetobacter guillouiae (species-level)-Acinetobacter (genus-level)-Proteobacteria (phylum-level), suggesting that these bacteria could be classified as the potential pathogenic bacterial biomarkers for Demodex blepharitis. Cutibacterium acnes (species-level)-Cutibacterium (genus-level)-Actinobacteria (phylum-level), and Pseudomonas (genus-level)-Proteobacteria (phylum-level) were more plentiful in the healthy control group’s conjunctival swab and meibum samples than in the DB group (Figure 4). Principal coordinate analysis of the discriminative genera separated all control and DB microbiomes into two distinct clusters both in conjunctival swab samples (p = 0.001, PERMANOVA, Figure 5) and meibum samples (p = 0.001, PERMANOVA, Figure 5).
Figure 4 Bacterial biomarkers identified with the linear discriminant analysis effect size (LEfSe) algorithm. Linear discriminant analysis (LDA) scores with the LEfSe tool for taxa, with LDA scores>4 and p<0.05 shown in the histogram (A, C). Cladogram displaying the relations between taxa at different taxonomic levels (B, D). Each circle represents a hierarchy, followed by phylum, class, order, family, and genus. Different phyla are marked with different colors. The size of the nodes represents the taxon abundance. HC, Healthy control; DB, Demodex blepharitis.
Figure 5 Principal coordinate analysis of two groups of conjunctival sac microbiome (A) and meibum microbiome (B). HC, Healthy control; DB, Demodex blepharitis.
To investigate the correlation between microbe abundance and the ocular surface parameters in the DB groups, we chose the species (RA >2.0%) to perform GEE analysis, taking age and gender into account. Correlations between the top species and the ocular surface parameters were shown in Table 5. It could be considered that higher RAs of species Sphingobium sp. YG1, Acinetobacter guillouiae, and Pseudomonas putida in both conjunctival swab and meibum samples, and species Delftia tsuruhatensis in conjunctival swab samples, were related to a more severe disease with higher scores of SPEED, LAM, Meiboscore and/or lower scores of FBUT, C,FS and meibum. In contrast, higher RAs of species Acinetobacter johnsonii, Acinetobacter sp. MYb10, and Cutibacterium acnes in both conjunctival swab samples and meibum samples, were related to the mild degree of disease with lower scores of SPEED, LAM, Meiboscore, and/or higher scores of FBUT, meibu,m and CFS. Only Toxoplasma gondii had no significant effect on any ocular surface parameters.
Table 5 Associations of ocular surface parameters and the species (RA>2.0%) in the conjunctival swab and meibum samples using generalized estimating equations analysis.
KEGG pathway analysis of both conjunctival swab and meibum samples of DB and control groups revealed enrichment of functions related to infectious diseases, the immune system, and signal transduction.
In conjunctival swab samples, LDA with LEfSe showed that nine pathways were significantly different between the control and DB groups (|LDA|>4). Three pathways (ribosome, oxidative phosphorylation, and bacterial secretion system) were more abundant in the control group, and six pathways (purine metabolism, pyrimidine metabolism, homologous recombination, DNA replication, base excision repair, and nucleotide excision repair) were more abundant in the DB group (Figure 6).
Figure 6 Discriminative Kyoto encyclopedia of genes and Genomes pathways in the conjunctival sac microbiome (A) and meibum microbiome (B) of the controls and Demodex blepharitis groups. HC, Healthy control; DB, Demodex blepharitis.
LDA combined with LEfSe analysis showed eleven pathways that were significantly different between the control and DB groups (|LDA|>4). Five pathways (ribosome, RNA degradation, plant-pathogen interaction, oxidative phosphorylation, cell cycle–caulobacter) were relatively more abundant in the control group, and six pathways (purine metabolism, pyrimidine metabolism, homologous recombination, DNA replication, base excision repair, nucleotide excision repair) were relatively more abundant in the DB group (Figure 6).
Blepharitis can arise from inflammation caused by Demodex mites on the ocular surface and from secondary bacterial infections (Lee et al., 2010). A comprehensive understanding of the ocular microbiome characteristics associated with Demodex mites is essential for understanding the pathogenesis, prevention, and treatment of blepharitis. Demodex infests lash follicles and inhabits the sebaceous glands and MGs. The activities of Demodex in the MG and their carried pathogens may cause changes in the flora and microenvironment of the conjunctival sac and MG, which causes changes in the meibum composition and aggravates inflammation. At present, there are few studies on the bacterial community of meibum in DB patients. In this study, we used metagenome sequencing to obtain a more comprehensive understanding of the taxonomic and functional complications of the ocular surface microbiome in DB patients.
Alpha-diversity analysis showed that the meibum microbiome diversity (Chao1, ACE, observed, Shannon) in DB and controls was significantly higher than that in the conjunctival swab samples (Figure 1). Interestingly, there was no significant difference in the alpha-diversity indices of the meibum microbiome between the two groups, while the conjunctival swab microbiome community population (Chao1, ACE, observed) from DB was significantly lower than that in the controls (p=0.002, 0.001, 0.047, respectively). Demodex infestation seems to change mainly the diversity of the microbiome in the conjunctival sac rather than that in the meibum. This may have occurred since we discarded the outer segment of the meibum in the MG and collected the deeper meibum, which might have been minimally affected by Demodex mites. This means that even in patients with Demodex infection, the microbiome in the meibum did not change significantly as compared with the normal controls since Demodex activity was limited to the ocular surface and the proximal opening of the MG, and it did not penetrate the deeper parts of the MG. Moreover, D. brevis parasites more obviously influence the MGs, making it more difficult to detect from epilated eyelashes than D. folliculorum that reside in eyelashes and follicles. In this study, we also diagnosed DB by detecting mites on the eyelashes; however, the number or absence of D. brevis was not clear. Although there was no significant difference in the alpha-diversity of the meibum between the two groups, there were significant differences in the dominant flora and their relative abundances at different taxonomic levels.
Lots of previous studies have shown that Proteobacteria (3.90% – 74%), Actinobacteria (5.00% – 64.8%), Firmicutes (3.90% – 41.71%), and Bacteroidetes(1.73% – 41%) were the most dominant phyla in the ocular surface of healthy subjects, dry eye disease (DED), and MGD (Lu and Liu, 2016; Cavuoto et al., 2019a; Yan et al., 2020; Zhang et al., 2021; Zysset-Burri et al., 2021). In this study, the dominant phyla were consistent with previous studies’ results, and the most abundant phylum was Proteobacteria. The average RA of the phyla Proteobacteria and Actinobacteria was significantly higher and that of the phylum Firmicutes was significantly lower in DB patients than in the controls (Table 1). Yan et al.’s study demonstrated different results, which reported a higher RA of Firmicutes in the DB group than the healthy controls, and no significant differences in Proteobacteria and Actinobacteria between two groups (Yan et al., 2020). However, some other studies reported similar results to our study, with a higher RA of the phyla Proteobacteria in MGD or patients with Demodex infestation than in healthy controls (Lee et al., 2012; Dong et al., 2019; Liang et al., 2021). Combined with the results of the LEfSe analysis of conjunctival swab and meibum samples, an increased prevalence of the Proteobacteria phylum may be an indicator of an unstable ocular microbial community, as also found in gut microbiota (Shin et al., 2015; Litvak et al., 2017).
Bacterial microbiota assessments of the conjunctival swab and meibum from both healthy controls and Demodex blepharitis patients identified several of the most common genera on the ocular surface of patients with Demodex infestation, MGD, DED, and healthy controls as previous reported, such as Pseudomonas (Ozkan et al., 2017; Wen et al., 2017; Ozkan et al., 2019; Borroni et al., 2019; Li et al., 2019; Andersson et al., 2021), Cutibacterium (Ozkan et al., 2017; Wen et al., 2017; Ozkan et al., 2019; Borroni et al., 2019; Dong et al., 2019), Acinetobacter (Dong et al., 2011; Ozkan et al., 2017; Li et al., 2019; Andersson et al., 2021), Sphingomonas (Ozkan et al., 2017; Dong et al., 2019; Andersson et al., 2021), and Staphylococcus (Dong et al., 2011; Ozkan et al., 2017; Wen et al., 2017; Dong et al., 2019). In our study, the RAs of genera Pseudomonas (meibum, 19.78% vs. 12.86%) and Cutibacterium (conjunctival swabs, 13.04% vs. 2.24%; meibum, 10.52% vs. 2.72%) were significantly higher in controls than in the Demodex blepharitis group. Andersson et al. also demonstrated that the RA of the genus Pseudomonas was markedly higher in healthy controls than in DED groups (24% versus 6%) (Andersson et al., 2021), and they identified Pseudomonas as a bacterial biomarker for healthy controls. In contrast, the RAs of the genera Acinetobacter, Sphingobium, and Delftia in Demodex blepharitis patients were significantly higher than that in healthy controls. Lee et al. investigated bacterial 16S rRNA genes of eyelash and tear samples from 7 blepharitis patients with Demodex infestation and 4 healthy controls using a pyrosequencing method (Lee et al., 2012); and they reported that an increase of Staphylococcus, Streptophyta, Corynebacterium, and Enhydrobacter, and a decrease of Cutibacterium were observed from blepharitis subjects, in terms of the relative abundances. Liang et al. used 16S rRNA sequencing to analyze the conjunctival swab samples of 14 MGD patients with ocular Demodex infestation and 17 healthy people, and reported that Pseudomonas, Acinetobacter, and Bacillus were the top three genera in all subjects, and there were more Acinetobacter, Novosphingobium, and Anoxybacillus in the Demodex infestation subjects and fewer Novosphingobium, Lactobacillus, and Candidatus Microthrix in the healthy control group (Liang et al., 2021). Yan et al. also analyzed bacterial 16S rRNA genes of conjunctival swab samples from 30 Demodex blepharitis patients and 14 healthy controls, and demonstrated a significantly higher RA of genus Lactobacillus and Bifidobacterium in Demodex blepharitis patients, while the RAs of genera Cutibacterium and Streptococcus were not statistically different from normal controls (Yan et al., 2020). A culture-based study found that the total colony counts and the incidences of Cutibacterium acnes and Staphylococcus aureus from the eyelashes of blepharitis with Demodex infestation were significantly higher than that of the controls (Zhu et al., 2018). When comparing the above results, we found that there was no consistent conclusion, which may be due to the relatively small samples, inconsistent diagnostic criteria, inconsistent flora detection methods, and sample types, etc.
This study was the first time to use mNGS to determine the species in conjunctival swab samples and meibum samples, to our best knowledge. Delftia tsuruhatensis and Sphingobium sp. YG1 was the most abundant species in the Demodex blepharitis samples, while Cutibacterium acnes was the most abundant species in the control samples. Delftia tsuruhatensis was first reported as the most abundant conjunctival flora in patients with conjunctival lymphoma, which is an emerging opportunistic healthcare-associated pathogen that can affect immunocompromised patients (Ranc et al., 2018), and it may change the conjunctival environment through its ability to degrade and utilize glucose oxidatively (Asao et al., 2019). Sphingobium sp. YG1 is a lignin model dimer-metabolizing bacterium newly isolated from sediment and has never been reported on the ocular surface (Ohta, 2018). However, the genus Sphingobium had been reported to be abundant in the MGD meibum (Zhao et al., 2020). Interestingly, the correlation between species abundances and clinical parameters in the DB group showed higher RAs of Delftia tsuruhatensis, Sphingobium sp. YG1, Acinetobacter guillouiae, and Pseudomonas putida were related to the worse ocular surface condition, while Cutibacterium acnes, Acinetobacter johnsonii, and Acinetobacter sp. MYb10 was related to better ocular surface condition. Combined with the LEfSe results, Sphingobium sp. YG1 and Acinetobacter guillouiae could be classified as the potential pathogenic bacterial biomarkers for Demodex blepharitis, while Cutibacterium acnes could be regarded as a friendlier flora for the healthy ocular surface.
The main difference in KEGG pathway analysis of both conjunctival swab and meibum samples between the groups focused on pathways for energy metabolism, genetic information processing, and environmental information processing. Purine metabolism, pyrimidine metabolism, homologous recombination, DNA replication, base excision repair, and nucleotide excision repair were more common in Demodex blepharitis patients than in controls. This suggests that the ocular surface of Demodex blepharitis patients might have higher levels of DNA damage and repair and a higher frequency of cellular activity in the host than in controls.
This study had some limitations. One of the limitations is the long duration of this study, and we did not collect sampling environment-negative controls, although we adopted strict environmental sterilization during each sample collection. Second, previous studies had revealed that the microbiota diversity on the ocular surface of healthy participants changes with age (Suzuki et al., 2020; Katzka et al., 2021). Participants’ age ranged from 20 to 60 years in this study, and this age range may have affected the results. Thirdly, this is a single-center research and the enrolled population was likely homogeneous to provide generalized conclusions.
Despite these limitations, this study provides novel insights into the ocular surface microbiota in Demodex blepharitis patients. The effect of Demodex on the ocular surface microbiome was more significant than that on the meibum microbiome. The increasing of phylum Proteobacteria might be an indicator of an unstable ocular microbial community.
The data presented in the study are deposited in the NCBI repository, accession number PRJNA856121. Further inquiries can be directed to the corresponding author.
The studies involving human participants were reviewed and approved by Ethical Committee of the Eye Hospital of Wenzhou Medical University. The patients/participants provided their written informed consent to participate in this study.
QD conceptualized the structure. YF, JW wrote the first draft. DW, TL, XS, LL, MZ, ZZ, and XY made a substantial contribution to the content. All authors approved the final version of the manuscript.
This study was supported by the Zhejiang Provincial Medical and Health Science, Technology Program of Health and Family Planning Commission (Grant number: 2019RC220)
The authors declare that the research was conducted in the absence of any commercial or financial relationships that could be construed as a potential conflict of interest.
All claims expressed in this article are solely those of the authors and do not necessarily represent those of their affiliated organizations, or those of the publisher, the editors and the reviewers. Any product that may be evaluated in this article, or claim that may be made by its manufacturer, is not guaranteed or endorsed by the publisher.
Amescua, G., Akpek, E. K., Farid, M., Garcia-Ferrer, F. J., Lin, A., Rhee, M. K., et al. (2019). Blepharitis preferred practice pattern®. Ophthalmology 126, P56–P93. doi: 10.1016/j.ophtha.2018.10.019
Andersson, J., Vogt, J. K., Dalgaard, M. D., Pedersen, O., Holmgaard, K., Heegaard, S. (2021). Ocular surface microbiota in patients with aqueous tear-deficient dry eye. Ocul. Surf. 19, 210–217. doi: 10.1016/j.jtos.2020.09.003
Arita, R., Itoh, K., Inoue, K., Amano, S. (2008). Noncontact infrared meibography to document age-related changes of the meibomian glands in a normal population. Ophthalmology 115, 911–915. doi: 10.1016/j.ophtha.2007.06.031
Asao, K., Hashida, N., Ando, S., Motooka, D., Kurakami, H., Nakamura, S., et al. (2019). Conjunctival dysbiosis in mucosa-associated lymphoid tissue lymphoma. Sci. Rep. 9, 8424. doi: 10.1038/s41598-019-44861-5
Beghini, F., McIver, L. J., Blanco-Míguez, A., Dubois, L., Asnicar, F., Maharjan, S., et al. (2021). Integrating taxonomic, functional, and strain-level profiling of diverse microbial communities with biobakery 3. Elife 10, e65088. doi: 10.7554/eLife.65088
Borroni, D., Romano, V., Kaye, S. B., Somerville, T., Napoli, L., Fasolo, A., et al. (2019). Metagenomics in ophthalmology: Current findings and future prospectives. BMJ Open Ophthalmol. 4, e000248. doi: 10.1136/bmjophth-2018-000248
Cavuoto, K. M., Galor, A., Banerjee, S. (2019a). Anatomic characterization of the ocular surface microbiome in children. Microorganisms 7, 259. doi: 10.3390/microorganisms7080259
Cheng, A. M. S., Sheha, H., Tseng, S. C. G. (2015). Recent advances on ocular demodex infestation. Curr. Opin. Ophthalmol. 26, 295–300. doi: 10.1097/ICU.0000000000000168
Chen, S., Zhou, Y., Chen, Y., Gu, J. (2018). Fastp: An ultra-fast all-in-one FASTQ preprocessor. Bioinformatics 34, i884–i890. doi: 10.1093/bioinformatics/bty560
Coston, T. O. (1967). Demodex folliculorum blepharitis. Trans. Am. Ophthalmol. Soc 65, 361–392. doi: 10.1136/bjo.56.8.639
de Paula, A., Oliva, G., Barraquer, R. I., de la Paz, M. F. (2019). Prevalence and antibiotic susceptibility of bacteria isolated in patients affected with blepharitis in a tertiary eye centre in Spain. Eur. J. Ophthalmol. 30, 991–997. doi: 10.1177/1120672119854985
Dixon, P. (2003). VEGAN, a package of r functions for community ecology. J. Veg. Sci. 14, 927–930. doi: 10.1111/j.1654-1103.2003.tb02228.x
Dong, Q., Brulc, J. M., Iovieno, A., Bates, B., Garoutte, A., Miller, D., et al. (2011). Diversity of bacteria at healthy human conjunctiva. Investig. Ophthalmol. Vis. Sci. 52, 5408–5413. doi: 10.1167/iovs.10-6939
Dong, X., Wang, Y., Wang, W., Lin, P., Huang, Y. (2019). Composition and diversity of bacterial community on the ocular surface of patients with meibomian gland dysfunction. Investig. Ophthalmol. Vis. Sci. 60, 4774–4783. doi: 10.1167/iovs.19-27719
Fu, Y., Xiang, H., Hu, R., Huang, X., Lin, L., Jin, X. M. (2021). Prospective trial of a 2940 nm Er:YAG laser for the treatment of meibomian gland dysfunction. Graefe’s Arch. Clin. Exp. Ophthalmol. 259, 2269–2278. doi: 10.1007/s00417-021-05170-8
Jiang, X., Deng, A., Yang, J., Bai, H., Yang, Z., Wu, J., et al. (2018). Pathogens in the meibomian gland and conjunctival sac: Microbiome of normal subjects and patients with meibomian gland dysfunction. Infect. Drug Resist. 11, 1729–1740. doi: 10.2147/IDR.S162135
Kang, Y., Lin, S., Ma, X., Che, Y., Chen, Y., Wan, T., et al. (2021). Strain heterogeneity, cooccurrence network, taxonomic composition and functional profile of the healthy ocular surface microbiome. Eye Vis. 8, 6. doi: 10.1186/s40662-021-00228-4
Katzka, W., Dong, T. S., Luu, K., Lagishetty, V., Sedighian, F., Arias-Jayo, N., et al. (2021). The ocular microbiome is altered by sampling modality and age. Transl. Vis. Sci. Technol. 10, 24. doi: 10.1167/TVST.10.12.24
Kumar, V., Baweja, M., Singh, P. K., Shukla, P. (2016). Recent developments in systems biology and metabolic engineering of plant–microbe interactions. Front. Plant Sci. 7. doi: 10.3389/fpls.2016.01421
Lane, S. S., Dubiner, H. B., Epstein, R. J., Ernest, P. H., Greiner, J. V., Hardten, D. R., et al. (2012). A new system, the LipiFlow, for the treatment of meibomian gland dysfunction. Cornea 31, 396–404. doi: 10.1097/ICO.0b013e318239aaea
Langmead, B., Salzberg, S. L. (2012). Fast gapped-read alignment with bowtie 2. Nat. Methods 9, 357–359. doi: 10.1038/nmeth.1923
Lee, S. H., Chun, Y. S., Kim, J. H., Kim, E. S., Kim, J. C. (2010). The relationship between demodex and ocular discomfort. Investig. Ophthalmol. Vis. Sci. 51, 2906–2911. doi: 10.1167/iovs.09-4850
Lee, S. H., Oh, D. H., Jung, J. Y., Kim, J. C., Jeon, C. O. (2012). Comparative ocular microbial communities in humans with and without blepharitis. investig. Ophthalmol. Vis. Sci. 53, 5585–5593. doi: 10.1167/iovs.12-9922
Liang, L. Y., Li, J., Liu, Y. (2017). Diagnostic criteria of demodex blepharitis. Chin. J. Ophthalmol. 53, 648–652. doi: 10.3760/cma.j.issn.0412-4081.2017.09.003
Liang, X., Li, Y., Xiong, K., Chen, S., Li, Z., Zhang, Z., et al. (2021). Demodex infection changes ocular surface microbial communities, in which meibomian gland dysfunction may play a role. Ophthalmol. Ther. 10, 601–617. doi: 10.1007/s40123-021-00356-z
Li, Z. H., Gong, Y., Chen, S. Z., Li, S. Q., Zhang, Y., Zhong, H. M., et al. (2019). Comparative portrayal of ocular surface microbe with and without dry eye. J. Microbiol. 57, 1025–1032. doi: 10.1007/s12275-019-9127-2
Litvak, Y., Byndloss, M. X., Tsolis, R. M., Bäumler, A. J. (2017). Dysbiotic proteobacteria expansion: a microbial signature of epithelial dysfunction. Curr. Opin. Microbiol. 39, 1–6. doi: 10.1016/j.mib.2017.07.003
Liu, C., Cui, Y., Li, X., Yao, M. (2021). Microeco: An r package for data mining in microbial community ecology. FEMS Microbiol. Ecol. 97, fiaa255. doi: 10.1093/femsec/fiaa255
Ohta, Y., Shimane, Y., Nishi, S., Ichikawa, J., Kurosawa, K., Tsubouchi, T., et al (2018). Complete genome sequence of Sphingobium sp. strain YG1, a lignin model dimer- metabolizing bacterium isolated from sediment in Kagoshima Bay, Japan. Genome Announc. 6. , e00267–e00218. doi: 10.1128/genomeA.00267-18
Ozkan, J., Nielsen, S., Diez-Vives, C., Coroneo, M., Thomas, T., Willcox, M. (2017). Temporal stability and composition of the ocular surface microbiome. Sci. Rep. 7, 9880. doi: 10.1038/s41598-017-10494-9
Ozkan, J., Willcox, M., Wemheuer, B., Wilcsek, G., Coroneo, M., Thomas, T. (2019). Biogeography of the human ocular microbiota. Ocul. Surf. 17, 111–118. doi: 10.1016/j.jtos.2018.11.005
Ranc, A., Dubourg, G., Fournier, P. E., Raoult, D., Fenollar, F. (2018). Delftia tsuruhatensis, an emergent opportunistic healthcare-associated pathogen. Emerg. Infect. Dis. 24, 594–596. doi: 10.3201/eid2403.160939
Ranjan, R., Rani, A., Metwally, A., McGee, H. S., Perkins, D. L. (2016). Analysis of the microbiome: Advantages of whole genome shotgun versus 16S amplicon sequencing. Biochem. Biophys. Res. Commun. 469. doi: 10.1016/j.bbrc.2015.12.083
Rodríguez, A. E., Ferrer, C., Alió, J. L. (2005). Chronic blepharitis and demodex. Arch. la Soc Española Oftalmol. 80, 635–642. doi: 10.4321/s0365-66912005001100004
Shin, N. R., Whon, T. W., Bae, J. W. (2015). Proteobacteria: Microbial signature of dysbiosis in gut microbiota. Trends Biotechnol. 33, 496–503. doi: 10.1016/j.tibtech.2015.06.011
Song, X., Zhao, P., Wang, G., Zhao, X. (2013). The effects of estrogen and androgen on tear secretion and matrix metalloproteinase-2 expression in lacrimal glands of ovariectomized rats. Investig. Ophthalmol. Vis. Sci. 55, 745–751. doi: 10.1167/iovs.12-10457
Suzuki, T., Sutani, T., Nakai, H., Shirahige, K., Kinoshita, S. (2020). The microbiome of the meibum and ocular surface in healthy subjects. Investig. Ophthalmol. Vis. Sci. 61, 18. doi: 10.1167/iovs.61.2.18
Team R Development Core (2018). A language and environment for statistical computing Vol. 2 (R Found. Stat. Comput, Vienna). Available at: https://www.R-project.org.
Wen, X., Miao, L., Deng, Y., Bible, P. W., Hu, X., Zou, Y., et al. (2017). The influence of age and sex on ocular surface microbiota in healthy adults. Investig. Ophthalmol. Vis. Sci. 58, 6030–6037. doi: 10.1167/iovs.17-22957
Wintermute, E. H., Silver, P. A. (2010). Dynamics in the mixed microbial concourse. Genes Dev. 24, 2603–2614. doi: 10.1101/gad.1985210
Wood, D. E., Lu, J., Langmead, B. (2019). Improved metagenomic analysis with kraken 2. bioRxiv. 20, 257. doi: 10.1101/762302
Yan, Y., Yao, Q., Lu, Y., Shao, C., Sun, H., Li, Y., et al. (2020). Association between demodex infestation and ocular surface microbiota in patients with demodex blepharitis. Front. Med. 7. doi: 10.3389/fmed.2020.592759
Zhang, S. D., He, J. N., Niu, T. T., Chan, C. Y., Ren, C. Y., Liu, S. S., et al. (2017). Bacteriological profile of ocular surface flora in meibomian gland dysfunction. Ocul. Surf. 15, 242–247. doi: 10.1016/j.jtos.2016.12.003
Zhang, Z., Zou, X., Xue, W., Zhang, P., Wang, S., Zou, H. (2021). Ocular surface microbiota in diabetic patients with dry eye disease. Investig. Ophthalmol. Vis. Sci. 62, 13. doi: 10.1167/iovs.62.12.13
Zhao, Y. E., Wu, L. P., Hu, L., Xu, J. R. (2012). Association of blepharitis with demodex: A meta-analysis. Ophthalmic Epidemiol. 19, 95–102. doi: 10.3109/09286586.2011.642052
Zhao, F., Zhang, D., Ge, C., Zhang, L., Reinach, P. S., Tian, X., et al. (2020). Metagenomic profiling of ocular surface microbiome changes in meibomian gland dysfunction. Investig. Ophthalmol. Vis. Sci. 61, 22. doi: 10.1167/IOVS.61.8.22
Zhu, M., Cheng, C., Yi, H., Lin, L., Wu, K. (2018). Quantitative analysis of the bacteria in blepharitis with demodex infestation. Front. Microbiol. 9. doi: 10.3389/fmicb.2018.01719
Keywords: Demodex blepharitis, meibum, metagenomic next-generation sequencing, microbial communities, ocular surface
Citation: Fu Y, Wu J, Wang D, Li T, Shi X, Li L, Zhu M, Zhang Z, Yu X and Dai Q (2022) Metagenomic profiling of ocular surface microbiome changes in Demodex blepharitis patients. Front. Cell. Infect. Microbiol. 12:922753. doi: 10.3389/fcimb.2022.922753
Received: 18 April 2022; Accepted: 30 June 2022;
Published: 22 July 2022.
Edited by:
Jerome Ozkan, University of New South Wales, AustraliaReviewed by:
Yuichiro Noiri, Niigata Universit, JapanCopyright © 2022 Fu, Wu, Wang, Li, Shi, Li, Zhu, Zhang, Yu and Dai. This is an open-access article distributed under the terms of the Creative Commons Attribution License (CC BY). The use, distribution or reproduction in other forums is permitted, provided the original author(s) and the copyright owner(s) are credited and that the original publication in this journal is cited, in accordance with accepted academic practice. No use, distribution or reproduction is permitted which does not comply with these terms.
*Correspondence: Qi Dai, ZHFAbWFpbC5leWUuYWMuY24=
†These authors share first authorship
Disclaimer: All claims expressed in this article are solely those of the authors and do not necessarily represent those of their affiliated organizations, or those of the publisher, the editors and the reviewers. Any product that may be evaluated in this article or claim that may be made by its manufacturer is not guaranteed or endorsed by the publisher.
Research integrity at Frontiers
Learn more about the work of our research integrity team to safeguard the quality of each article we publish.