- 1Department of Gastroenterology, The Third Xiangya Hospital, Central South University, Changsha, China
- 2Hunan Key Laboratory of Non-resolving Inflammation and Cancer, Central South University, Changsha, China
Background and Aims: The impacts of Helicobacter pylori (H. pylori) eradication on the gastrointestinal microbiota are controversial, and whether the short-term and long-term changes in the gastrointestinal microbiota following different eradication regimens are consistent remains inconclusive. This study aimed to examine the effects of various eradication regimens on the gastrointestinal microflora at follow-up evaluations within 7 days, at 1–3 months, and over 6 months changes in the gastrointestinal microbiota.
Materials and Methods: Studies reported on the PubMed, Embase, Cochrane Library, Web of Science, and ClinicalTrails.gov databases before March 2022 were collected. Data analysis and visualization were conducted using Review Manager 5.4.1. The tool of the Cochrane Collaboration to assess the risk of bias was suitable for randomized controlled trials with the Newcastle–Ottawa scale for nonrandomized controlled trials. In addition, the process was conducted in accordance with the Preferred Reporting Items for Systematic Reviews and Meta-Analyses (PRISMA) statement.
Results: After a series of rigorous screenings, a total of 34 articles with 1,204 participants were included for this review analysis. The results showed changes in the gut microflora at the phylum level or the family and genus levels. After metronidazole-containing triple therapy, the number of Enterobacteriaceae increased at 1–3 months follow-up. After Metronidazole-free triple therapy, Actinobacteria decreased significantly, and this trend lasted for more than 6 months. Within 7 days after eradication treatment, the follow-up results showed a decrease in the number of Lactobacillus. After Bismuth-containing quadruple therapy, the changes in Actinobacteria fluctuated with the follow-up time. The changes in Proteobacteria showed a downward trend lasting for 1–3 months after eradication but returned to baseline levels over 6 months after eradication. Subgroup analyses indicated that host age could influence changes in the gut microbiota.
Conclusion: Different eradication regimens had varied effects on the short-term and long-term abundance of the gastrointestinal microbiota, but the decreasing trend of the microbiota diversity was the same for all regimens at the short-term follow-up. This study summarizes the changes of gut microbiota at different stages after different eradication regimens and hope to provide some references for supplementing probiotics, while further studies is needed to support these findings.
Systematic Review Registration: https://www.crd.york.ac.uk/PROSPERO/, identifier: CRD42021292726
1 Introduction
Helicobacter pylori (H. pylori) is a microaerophilic gram-negative bacillus and a pathogenic bacterium that colonizes the gastric mucosa (McNulty, 1999). H. pylori infection is associated with a variety of digestive diseases, such as atrophic gastritis, peptic ulcer, and gastric cancer (Kuipers et al., 1995). The eradication of H. pylori can partially reverse atrophy and intestinal metaplasia and reduce the risk of gastric cancer (Choi et al., 2018; Hwang et al., 2018; Ford et al., 2020). In 2015, H. pylori infection was officially identified as an infectious disease in the Kyoto global consensus report, and it was recommended that all patients should be treated (Sugano et al., 2015). Conventional eradication regimens include triple therapy with two antibiotics and a proton pump inhibitor (PPI), quadruple therapy with bismuth, and dual therapy of one antibiotic and a PPI (Rokkas et al., 2021). Symptoms of gastrointestinal discomfort after eradication of H. pylori have also been reported occasionally (Madden et al., 2005; Blaabjerg et al., 2017). In addition, it has been reported that the gastrointestinal flora changes greatly after eradication of H. pylori, and these changes are likely related to the drugs used in the eradication regimen (Ianiro et al., 2016). Therefore, the effect of different eradication regimens on the gastrointestinal microbiota is worth studying.
The gastrointestinal microbiota is constantly changing from infancy to adulthood. It plays a vital role in human health and can perform essential functions in the body’s immune, metabolic, and nervous systems (Adak and Khan, 2019). When the gastrointestinal microbiota is dysregulated, it is accompanied by changes in the abundance and diversity of the microbial community, which can affect a variety of the host’s physiological functions, leading to the occurrence of various diseases. The effect of H. pylori on the gastrointestinal microbiota is reflected in both the bacteria themselves and the eradication regimen that is used (Chen et al., 2021). On the one hand, colonization by H. pylori affects the gastric microenvironment, thereby influencing the types and populations of gastric microbiota and may also affect the gut microbiota. In the presence of H. pylori, the interaction of the microbiota in the stomach is greatly reduced (Coker et al., 2018). Clinical studies have reported that the dysbacteriosis associated with H. pylori infection is associated with a variety of digestive system diseases, such as gastric ulcers, gastritis, and gastric cancer (Ferreira et al., 2018; Guo et al., 2020; Rajilic-Stojanovic et al., 2020). On the other hand, the drugs selected for H. pylori eradication also cause changes in the gut microbiota. Accepted opinion had shown that metronidazole can inhibit the presence of anaerobic flora in the gut, which is considered to be the normally dominant gut flora, which has a protective effect on the overall gut environment (Freeman et al., 1997). A number of previous reports found that after H. pylori eradication, the diversity of the microbiota significantly decreased in a short period, and this change may last for 1 month or more than 6 months (Jakobsson et al., 2010; Liou et al., 2019; Kakiuchi et al., 2020; Guillemard et al., 2021). It has also been reported that the diversity of the intestinal flora increased during the 1-year follow-up of H. pylori eradication (Sung et al., 2020). On the basis of previous studies, short-term and long-term changes in the gastrointestinal microbiota after H. pylori eradication are unclear.
Changes in the gastrointestinal microbiota after eradication of H. pylori are controversial. On the basis of the high infection rate of H. pylori and the important role of the gastrointestinal microbiota, this study conducted a meta-analysis to analyze whether the short-term and long-term effects of different treatment regimens on the gastrointestinal microbiota following H. pylori eradication were consistent and whether there were differences among the various treatments regarding specific impacts on the gastrointestinal microbial community. This study was divided into groups according to eradication regimen, and the short-term and long-term changes in the gastrointestinal microbiota under each regimen were recorded and analyzed. Changes resulting from the different treatment regimens were compared. In addition, factors such as age and race were analyzed to comprehensively analyze the changes in the flora.
2 Materials and methods
2.1 Literature search
A literature search was conducted through five databases: PubMed, Embase, the Cochrane library, Web of Science, and ClinicalTrails.gov. The time limit was before March 2022, and nonhuman studies and non-English studies were excluded. To search the literature as comprehensively as possible, the following search terms were combined: (Gastrointestinal Microbiome OR Gastrointestinal Microbiomes OR Microbiome OR Gastrointestinal OR Gut Microbiome* OR Microbiome, Gut OR Gut Microflora OR Microflora, Gut OR Gut Microbiota* OR Microbiota, Gut OR Gastrointestinal Flora OR Flora, Gastrointestinal OR Gut Flora OR Flora, Gut OR Gastrointestinal Microbiota* OR Microbiota, Gastrointestinal OR Gastrointestinal Microbial Community* OR Microbial Community, Gastrointestinal OR Gastrointestinal Microflora OR Microflora, Gastrointestinal OR Gastric Microbiome* OR Microbiome, Gastric OR Intestinal Microbiome* OR Microbiome, Intestinal OR Intestinal Microbiota* OR Microbiota, Intestinal OR Intestinal Microflora OR Microflora, Intestinal OR Intestinal Flora OR Flora, Intestinal OR Enteric Bacteria OR Bacteria, Enteric) AND (Helicobacter pylori OR H. pylori OR Hp OR Helicobacter nemestrinae OR Campylobacter pylori* OR Campylobacter pylori subsp. Pylori) AND (Therapeutics OR Therapeutic OR Therapy* OR Treatment* OR eradicate* OR regimen*). The detailed retrieval process is shown in Supplementary Table 1. The literature retrieved from the above five databases was managed through EndNote 20, and the literature was screened according to the inclusion and exclusion criteria in the next step.
2.2 Study selection
The inclusion criteria of the articles were as follows: (a) full text of research papers in English; (b) human beings as research subjects; (c) samples available for microbiota analysis; (d) successful application of different therapies, such as dual therapy, triple therapy, and quadruple therapy; (e) data on microbiota changes after eradication; and (f) prospective study. Animal experiments were not included in this study, and all clinical data were derived from published studies. Articles that did not meet the inclusion criteria and were repeatedly retrieved from different databases were excluded. Two authors independently completed the above process. When differences of opinion arose, a third author was required to re-evaluate the differences and come to the final result in addition; the process was conducted in accordance with the Preferred Reporting Items for Systematic Reviews and Meta-Analyses (PRISMA) statement (Liberati et al., 2009).
2.3 Data extraction
When the included articles were identified, the following main study characteristics were extracted and recorded. Information extraction tables were designed in advance and included the author, year of publication, number of included research subjects, and basic characteristics of the research subjects (gender, age, ethnicity, etc.), the specific eradication strategy, the time of sample evaluation, and the changes in the types and quantities of gastrointestinal microbes before and after eradication. According to the different measurement standards of the outcome indicators of different studies, the analysis of gastrointestinal microbiota abundance and diversity was divided into the phylum level obtained by next-generation sequencing and the family and genus level obtained by microbial culture. At the phylum level, representative microbial flora genera such as Bacteroidetes, Firmicutes, Actinobacteria, and Proteobacteria would serve as our measure of the microbiota, at the family and genus level, Bifidobacterium, Lactobacillus, Enterobacteriaceae, and Enterococcus were planned to be used as our observation indicators of the gut microbiota (Ye at al., 2020). Data on bacterial diversity in the findings should also be listed. The data extraction process required two evaluators to cross-check independently. In addition, pre-extraction was conducted before the data were formally extracted to evaluate whether the data extraction table was reasonable and the degree of consistency of understanding of the same problem among different evaluators. If there was any disagreement in the process, it was communicated and resolved first, and a third evaluator intervened.
2.4 Quality assessment
Randomized controlled trials were assessed by the Cochrane Collaboration tool for risk of bias assessment, while nonrandomized controlled trials were assessed for quality using the Newcastle–Ottawa scale (Lo et al., 2014).
2.5 Data analysis
By calculating the desired outcome based on the data provided, we included as many articles and data from randomized controlled trials and prospective studies as possible for the meta-analysis. Continuous variables in the study results were expressed as the mean ± standard deviation (SD), including the mean relative abundance (%) (NGS, Next Generation Sequencing), mean counts (log colony forming units (CFUs)/g fecal wet weight) (conventional microbial culture). Weighted mean differences (WMDs) with 95% confidence intervals were selected for continuous variable results. Statistical heterogeneity was assessed using the I2 statistic and Cochran’s Q-test. In addition, pooled estimates were obtained using the fixed model (Mantel and Haenszel) method (I2 ≤ 50%, P >.1) or the stochastic model (M–H anomaly) method (I2 > 50%, P ≤.1). Subgroup analysis was performed to compare differences in gut flora after H. pylori eradication in different age groups. In addition, we used sensitivity analysis to assess whether the meta-analysis results were stable or dependable. The software used for data analysis in this study was Review Manager (RevMan) (Version 5.4.1, The Cochrane Collaboration, 2020).
3 Results
3.1 Study selection and characteristics
By searching the five databases, a total of 2,311 results were identified; finally, 34 studies and 1,204 participants were included (Adamsson et al., 1998; Adamsson et al., 1999; Buehling et al., 2001; Madden et al., 2005; Plummer et al., 2005; Shimbo et al., 2005; Myllyluoma et al., 2007; Imase et al., 2008; Jakobsson et al., 2010; Wang and Huang, 2014; Oh et al., 2016b; Yap et al., 2016; Wang et al., 2017; Yanagi et al., 2017; Chen et al., 2018; Gotoda et al., 2018; Hsu et al., 2018; Yildiz et al., 2018; Cornejo-Pareja et al., 2019; He et al., 2019; Hsu et al., 2019; Liou et al., 2019; Olekhnovich et al., 2019; Guo et al., 2020; Kakiuchi et al., 2020; Martín-Núñez et al, 2020. The specific process is shown in Figure 1. The criterion for evaluating successful H. pylori eradication was the results of 13/14C-UBT or biopsies. The main information of the included literature has been listed in Supplementary Table 2, including the key findings and specific comments of the study. After grouping according to the treatment regimen, the specific information is displayed in Supplementary Tables 3–5. All included studies provided definite follow-up time, including 23 studies within 7-day short-term follow-up, 22 studies with mid-term follow-up in 1–3 months, and 9 studies with long-term follow-up over 6 months (Supplementary Tables 6–8). Sensitivity analysis showed that the listed results were stable. The quality assessment is shown in Supplementary Figure 1 and Table 9.
3.2 Short-term and long-term alterations in gastric microbiota after eradication
Of the 34 included studies, a total of six studies presented gastric microbiota analysis, four of which included only gastric data (Adamsson et al., 1998; Adamsson et al., 1999; He et al., 2019; Guo et al., 2020; Sung et al., 2020; Yuan et al., 2021). After arranging the provided data, it was found that all six studies only concerned whether the changes in the microbiota before and after treatment were statistically different, without specific data, so meta-analysis could not be carried out (Supplementary Table 10). Besides, inconsistent conclusions in different studies regarding changes in the diversity.
3.3 Short-term and long-term alterations in gut microbiota after eradication
3.3.1 Alterations in gut microbiota after dual therapy
Only one of the included articles used dual therapy to eradicate H. pylori and only provided significant differences without specific available data (Supplementary Table 3). Due to the small number of available studies and the limitation of unanalyzable outcome data in the literature, meta-analysis of the short-term or long-term effects of dual regimens on gut microbes could not be carried out, and the effect of dual regimens on gut microbes still needs further and high-quality research to illustrate.
3.3.2 Alterations in gut microbiota after triple therapy
There were a total of 21 articles on the changes in microbiota after triple therapy, and the main information is listed in Supplementary Table 4. At the phylum level, gut microbiota are expressed by relative abundance (%), and at the family and genus levels, gut microbiota are expressed by log CFU/g. Considering the impact of antibiotic types on the flora, the triple therapy regimens were further divided into Metronidazole-containing regimen and Metronidazole-free regimen. Antibiotic regimens administered in individual studies are listed in Supplementary Table 4.
3.3.2.1 Alterations in gut microbiota after Metronidazole-containing triple therapy
Five of the included studies used Metronidazole-containing triple therapy, but only two studies provided data on the changes in the microbiota at the family and genus levels 1–3 months after eradication treatment. The analysis results are shown in Figure 2. The results showed that the number of Enterobacteriaceae (WMD = −0.22[−0.45, 0.00]) increased at follow-up 1–3 months after treatment (Figure 2A). Meanwhile, the number of Enterococcus did not differ significantly (Figure 2B). Analysis could not be performed due to a lack of microbiota data at the phylum level and other follow-up times. In addition, the results of these two studies did not involve statistics related to bacterial diversity.
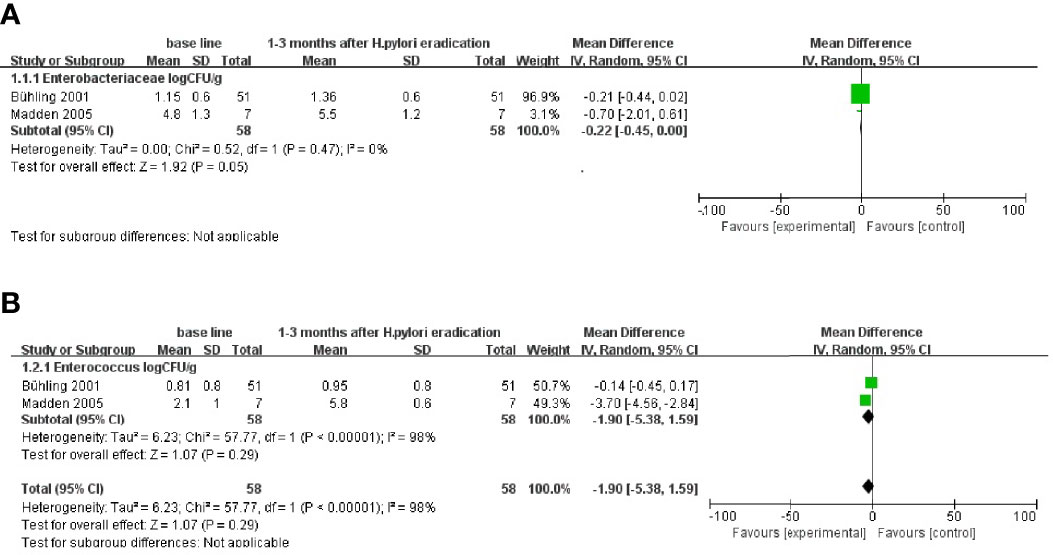
Figure 2 Follow-up results at the family and genus levels after Metronidazole-containing triple therapy of (H) pylori. (A) Changes in Enterobacteriaceae at 1–3 months after eradication treatment follow-up. (B) Changes in Enterococcus at 1–3 months after eradication treatment follow-up.
3.3.2.2 Alterations in gut microbiota after Metronidazole-free triple therapy
At the phylum level, the data of Actinobacteria and Bacteroidetes at 1–3 months after eradication treatment follow-up were analyzed. The results showed that Actinobacteria decreased (WMD = 0.51 [0.46, 0.56] %) (Figure 3A). However, the difference in the change in Bacteroidetes was not statistically significant (Figure 3B). The results after more than 6 months after eradication treatment follow-up showed that Actinobacteria decreased (WMD = 2.22 [0.45, 3.99] %), while Bacteroidetes, Firmicutes, and Proteobacteria showed no significant difference (Figures 3C–F), which was consistent with the 1–3 month follow-up. Age-based subgroup analysis results also showed differences in Actinobacteria changes between adults and children (Figure 3G).
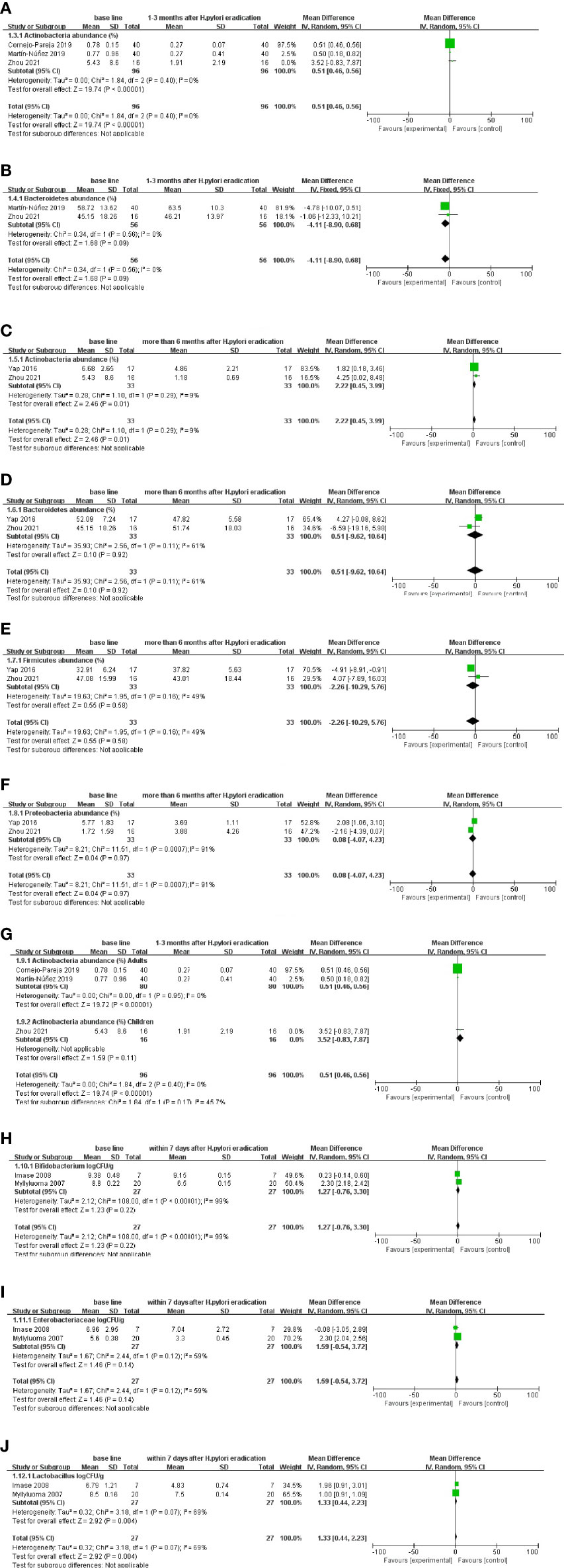
Figure 3 Follow-up results after Metronidazole-free triple therapy of H. pylori. (A, B) At the phylum level, changes in gut microbiota abundance (%) at 1–3 months after eradication treatment follow-up. (C–F) At the phylum level, changes in gut microbiota abundance (%) more than 6 months after eradication treatment follow-up. (G) At the phylum level, changes in gut microbiota abundance (%) between adults and children at age-based subgroup analysis. (H–J) At the family and genus levels, changes in the number (CFU/g) of gut microbiota within 7 days after eradication treatment follow-up.
At the family and genus levels, within 7 days after eradication treatment, the follow-up results showed a decrease in the number of Lactobacillus (WMD = 1.10 [0.39, 1.80] log CFU/g), whereas there was no significant difference in Bifidobacterium and Enterobacteriaceae (Figures 3H–J).
A total of nine articles in Supplementary Table 4 recorded whether the diversity change was significantly different, but there was no consistent result in the diversity change among different research results, so meta-analysis could not be conducted.
In addition, when subgroup analysis was performed on the two major categories of triple therapy regimens, the available data showed that the gut microbiota at the family and genus levels was not significantly different within 7 days after eradication treatment follow-up with the two triple regimens, but other follow-up time points were due to the lack of data that could not be analyzed temporarily (Supplementary Figures 2A–C).
3.3.3 Alterations in gut microbiota after quadruple therapy
A total of 11 studies are listed in Supplementary Table 5, four of which described the microbiota at the phylum level, gut microbiota expressed by relative abundance (%), and two described the microbiota at the species level, gut microbiota expressed by log CFU/g. Another five had no data available for analysis. Quadruple regimens are divided into Bismuth-containing quadruple and Bismuth-free quadruple based on whether the regimen contains bismuth. Only three studies used a Bismuth-free quadruple regimen and two had the specific data, the specific information about the antibiotic regimens administered in individual studies is listed in Supplementary Table 5.
At the phylum level, data analysis within 7 days after eradication treatment follow-up showed that Actinobacteria (WMD = 2.52 [0.25, 4.80] %) and Bacteroidetes (WMD = 24.17 [20.00, 28.33] %) decreased, Proteobacteria (WMD = −52.10 [−63.74, −40.45] %) increased, and Firmicutes showed no significant difference (Figures 4A–D). The results of 1–3 months after eradication treatment follow-up showed that Bacteroidetes (WMD = 11.24 [0.68, 21.81] %) decreased and Proteobacteria (WMD = −9.48 [−15.98, −2.98] %) increased, while Actinobacteria and Firmicutes changed with no difference (Figures 4E–H). More than 6 months after eradication treatment, the follow-up results showed that Actinobacteria (WMD = 1.55 [0.03, 3.08] %) decreased, whereas other flora basically recovered to the baseline level (Figures 4I–L).
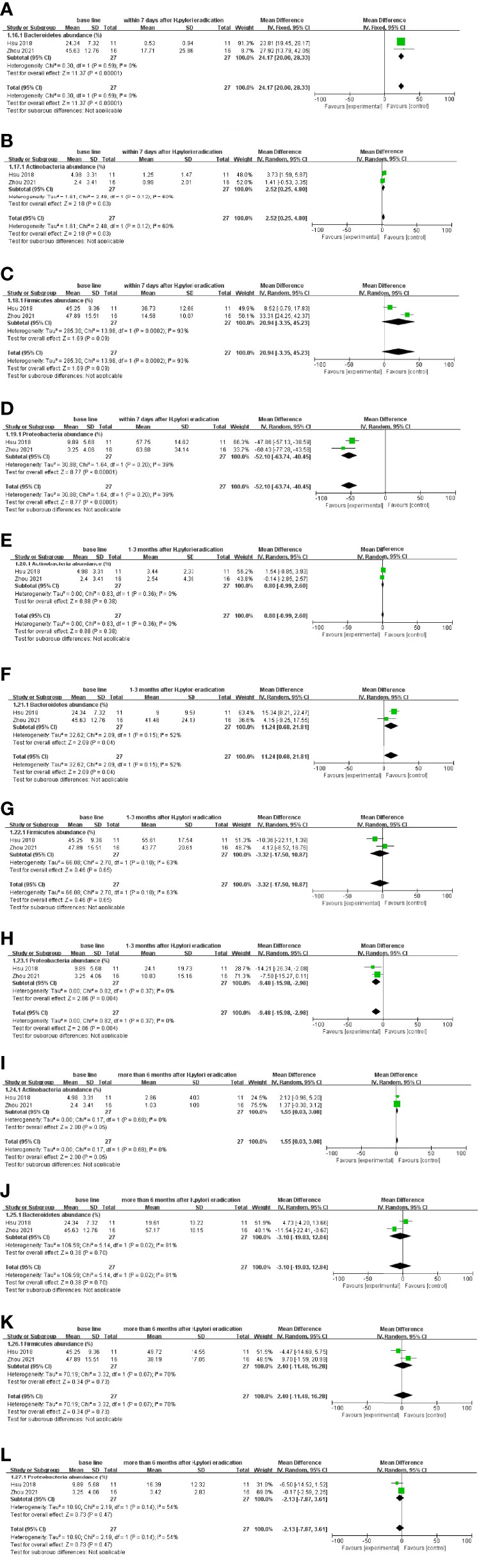
Figure 4 Follow-up results after Bismuth-containing quadruple therapy of H. pylori at the phylum level. (A–D) Alterations in gut microbiota within 7 days after eradication treatment follow-up. (E–H) Alterations at 1–3 months after eradication treatment follow-up. (I–L) Changes more than 6 months after eradication treatment follow-up.
At the phylum level, within 7 days, compared with 1–3 months after eradication treatment, the follow-up results. It was presented as Actinobacteria (WMD = −1.98 [−3.32, −0.64] %) and Firmicutes (WMD = −24.51[−34.57, −14.44] %) increased, Proteobacteria (WMD = −42.41 [23.66, 61.15] %) decreased, and there was no significant difference in Bacteroidetes (Figures 5A–D). Within 7 days compared with more than 6 months after eradication treatment follow-up results, Bacteroidetes (WMD = −28.13 [−47.98, −8.28] %) and Firmicutes (WMD = −18.74 [−29.11, −8.37] %) increased, Proteobacteria (WMD = 49.75 [31.34, 68.15] %) decreased, and there was no significant difference in the changes in Actinobacteria (Figures 5E–H). When 1–3 months were compared with more than 6 months after eradication treatment follow-up results, an increase in Bacteroidetes (WMD = −12.12 [−20.21, −4.04] %), a decrease in Proteobacteria (WMD = 7.48 [0.86, 14.10] %), and changes in Actinobacteria and Firmicutes were not significantly different (Figures 5I–L).
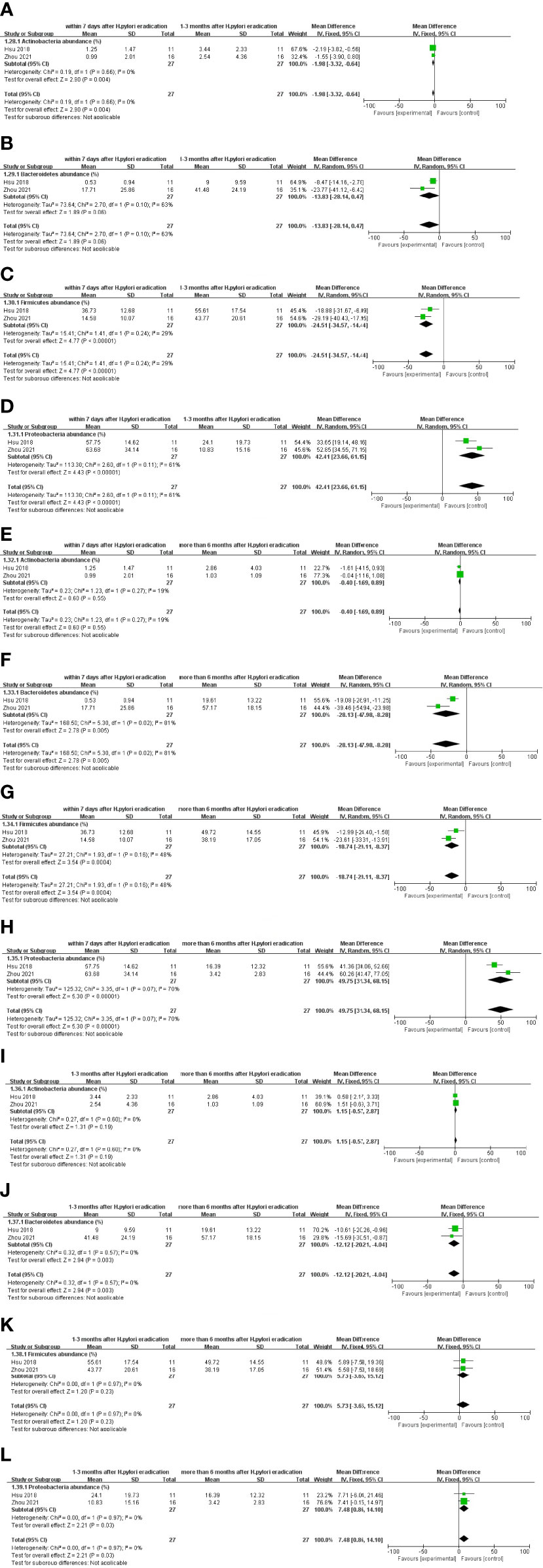
Figure 5 Comparison of results at different follow-up times after Bismuth-containing quadrupole therapy of H. pylori at the phylum level. (A–D) The alterations in gut microbiota within 7 days and 1–3 months after eradication treatment follow-up. (E–H) Alterations compared within 7 days and more than 6 months after eradication treatment follow-up. (I–L) Changes compared by 1–3 months and more than 6 months after eradication treatment follow-up.
At the family and genus levels, only one Bismuth-containing quadruple regimen was available with detailed data and therefore could not be analyzed.
Nine of the 11 studies listed provided significant differences in diversity, and 8 follow-up data suggested a reduction in diversity within 7 days after radical treatment, a change that was inconsistent between medium- and long-term follow-up (Supplementary Table 5).
3.4 Alterations in gut microbiota after different therapies during follow-up
After analyzing the changes of gut microbiota in different follow-up times after the same treatment, we re-analyzed whether there were differences in gut microbiota of different treatment regimens at the same follow-up time. All included studies provided definite follow-up time (Supplementary Tables 6–8) and the result was shown in Figure 6.

Figure 6 Comparison of results at different follow-up times after different treatments. (A–D) At the phylum level, alterations in gut microbiota within 7 days. (E–G) At the family and genus levels, alterations in gut microbiota within 7 days. (H–K) Alterations in gut microbiota at 1–3 months. (L–O) Alterations in gut microbiota more than 6 months.
3.4.1 Alterations in gut microbiota within 7 days after different therapies
At the phylum level, Actinobacteria decreased and Proteobacteria increased for both the Bismuth-containing quadruple therapy and Firmicutes showed a decreasing trend only after treatment with the Reverse hybrid therapy, which was inconsistent with the results for the Metronidazole-free triple therapy (Figures 6A, C, D). Bacteroidetes showed a decreasing trend only after treatment with Bismuth-containing quadruple regimen(Figure 6B). At the family and genus levels, Bifidobacterium tended to decrease after quadruple regimen treatment (with or without bismuth), but not after triple regimen (with or without metronidazole) (Figure 6E). Enterobacteriaceae showed an increasing trend after Metronidazole-containing triple therapy but no difference after Metronidazole-free triple therapy (Figure 6F). Lactobacillus declined after Metronidazole-free triple and Bismuth-containing quadruple regimen but unchanged in other regimens (Figure 6G).
3.4.2 Alterations in gut microbiota at 1–3 months after different therapies
At the phylum level, Actinobacteria showed a decreasing trend only after Metronidazole free triple therapy (Figure 6H). Bacteroidetes showed a decreasing and Proteobacteria showed an increasing trend only after Bismuth-containing quadruple regimen (Figures 6I, K). Firmicutes decreased only after Reverse hybrid therapy, which was inconsistent with other treatments (Figure 6J). At the family and genus levels, there was lack of studies to analyze.
3.4.3 Alterations in gut microbiota more than 6 months after different therapies
At the phylum level, Actinobacteria showed a decreasing trend after Metronidazole-free triple and Bismuth-containing quadruple regimens, which was inconsistent with other regimens (Figure 6L). The changes of Bacteroidetes and Proteobacteria after different treatment regimens were consistent, and there was no significant difference (Figures 6M, O). Firmicutes decreased only after Reverse hybrid therapy, which was inconsistent with results from other regimens (Figure 6N).
In summary, in order to easily see the changes in the gut microbiota at different follow-up times after different treatment regimens, the changes were listed in Table 1 in detail. The blank space in the table indicated lack of data or no differences in previous literature.
4. Discussion
The status of the gastrointestinal microbiota is a consideration that clinicians need to evaluate following H. pylori eradication, although the existing published studies remain inconclusive concerning the short-term and long-term effects of different eradication regimens on the gastrointestinal microbiota. This study selected the form of meta-analysis to summarize and analyze the prospective studies published thus far on changes in the abundance and diversity of gastrointestinal microbiota following H. pylori eradication treatment. This study was the first to analyze gastrointestinal microbiota data at different follow-up times according to the various eradication regimens.
Due to the lack of specific data on gastric microbiota, meta-analysis was not performed in this study, and more research is needed in the future to provide information on changes in gastric microbiota after H. pylori radical therapy.
After treatment with dual therapy, available follow-up data suggested that the gut microbiota had returned to baseline at 1–3 months after eradication follow-up. The findings of Horii et al. also suggest that Firmicutes decreased and Bacteroidetes
increased within 7 days after radical treatment. However, there was only 1 literature supported this conclusion, which is biased (Horii et al., 2021). Considering the pharmacological effects of antibiotics themselves, the triple regimens were divided into Metronidazole-containing triple therapy and Metronidazole-free triple therapy. After Metronidazole-containing triple therapy, the number of Enterobacteriaceae increased at 1–3 months after the treatment follow-up, but the number of Enterococcus did not differ significantly. Since few studies were retrieved, analysis could not be performed due to a lack of microbiota data at the phylum level and at follow-up times. In addition, the results did not involve statistics related to bacterial diversity. Therefore, more research is needed to explore the effect of metronidazole-containing regimens on the gastrointestinal microbiota. After Metronidazole-free triple therapy, Actinobacteria decreased significantly at the phylum level, and this trend lasted for more than 6 months, while at the family and genus levels, Lactobacillus decreased within 7 days after eradication. According to the drug types of the quadruple regimen, the quadruple regimen was divided into Bismuth-containing quadruple regimen and Bismuth-free quadruple regimen. After the Bismuth-containing quadruple regimen treatment, at the phylum level, the changes in Actinobacteria fluctuated with the follow-up time, showed a downward trend within 7 days after eradication, recovered to the baseline level at 1–3 months, and then decreased at more than 6 months of follow-up. Bacteroidetes, a decrease in abundance, was indicated at the 7-day and 1- to 3-month follow-ups, with no difference at more than 6 months of follow-up. When the data at different time points were compared, it was found that Bacteroidetes showed an upward trend, which also explained why the number of Bacteroidetes returned to baseline levels at more than 6 months of follow-up. The changes in Firmicutes did not show significant differences during the follow-up, but when the data at different follow-up time points were compared in pairs, it was found that the overall trend increased during the follow-up period. Although the available data showed that Firmicutes showed significant differences within 7 days, 1–3 months and over 6 months of follow-up, this upward trend may be confirmed at longer follow-up periods. The trend of changes in Proteobacteria was relatively simple; the downward trend lasted for 1–3 months after eradication and returned to baseline levels over 6 months. When the data at different time points were compared, it was found that Proteobacteria showed a downward trend. Subgroup analyses indicated that age could influence changes in the gastrointestinal microbiota, such as differences at the phylum level between adults and children at the 1- to 3-month follow-up after Metronidazole-free triple therapy. Only three studies used Bismuth-free quadruple regimen, and a meta-analysis could not be performed. Of the three studies, one was reverse hybrid therapy (PPI, amoxicillin for 14 days, clarithromycin and metronidazole in the initial 7 days) and two were concomitant therapy (PPI and three antibiotics) (Wang et al., 2017; Hsu et al., 2019; Liou et al., 2019). Direct analysis was not possible due to inconsistent units of microbiota data.
By analyzing different treatments at short-term follow-up, the results showed that the changes in Actinobacteria and Proteobacteria after Bismuth-containing quadruple regimen and reverse hybrid regimen were consistent. The change of Bacteroidetes was inconsistent that showed a decreasing trend only after treatment with Bismuth-containing quadruple regimen. The decreased trend in Bifidobacterium was consistent across the quadruple regimen, with or without bismuth. Enterobacteriaceae showed an increasing trend only after Metronidazole-containing triple therapy. Lactobacillus declined consistently after Metronidazole-free triple and Bismuth-containing quadruple regimen. At mid-term follow-up, the changes in the microbiota were not consistent, which may be caused by the limited number of included literatures. At long-term follow-up, the decreasing trend of Actinobacteria was consistent in Metronidazole-free triple and Bismuth-containing quadruple regimens.
Regarding the analysis of diversity, no matter which eradication method was chosen, the decreasing trend of diversity in the short term was consistent, but the results in the medium-term and long-term follow-ups were quite different, and further research is needed.
The dominant microbiota of the gastrointestinal tract includes Actinobacteria, Bacteroidetes, Firmicutes, Proteobacteria, Bifidobacterium, and so forth (Bik et al., 2006; Sekirov et al., 2010). It has been reported that Bacteroidetes and Bifidobacterium can produce short-chain fatty acids such as butyrate, propionate and acetate to provide abundant energy for the host (Macfarlane and Macfarlane, 2003; Sartor, 2008). In addition, members of the genus Bacteroides, such as Bacteroides thetaiotaomicron, which mainly organizes carbohydrate metabolism, are closely related to body metabolism. Sharp decreases in the dominant microbiota after the eradication of H. pylori causes corresponding gastrointestinal symptoms, and the most obvious symptom is diarrhea (Blaabjerg et al., 2017).
The target flora of different antibiotics was different. For example, after treatment with amoxicillin, Clostridium perfringens and Eubacterium rectum in Firmicutes were reduced (Barc et al., 2004), Amoxicillin and clarithromycin can reduce the relative abundance of Firmicutes (Oh et al., 2016a), Actinobacteria were reduced by clarithromycin treatment (Williams et al., 1992; Jakobsson et al., 2010). Therefore, specific antibiotics must be considered when exploring the effect of different treatments on the microbiota.
Of the 34 included studies, 14 used the addition of probiotics as a control for conventional H. pylori eradication regimens to study changes in microbiota. In these studies, the conclusions showed that probiotics can alleviate the flora dysbiosis and improve gastrointestinal symptoms caused by H. pylori eradication to varying degrees, but the research also points out that it may not be necessary to take probiotics alone in young people with H. pylori infection (Yuan et al., 2021). This study summarizes the changes of gut flora at different stages after treatment with different regimens, so we hope to provide some references for supplementing probiotics.
Although our study yielded some significantly different results, there were some potential limitations. First, the sample size included in the meta-analysis was relatively small. Although we included as many articles as possible by broadening the search, most of the articles lacked analyzable data and provided only relevant significant differences, a problem that resulted in the availability of fewer data for actual analysis. The statistical results of gastrointestinal microbiota were also scattered, and the gastrointestinal microbiota obtained by different studies had diverse types, so it was difficult to merge the data. In addition, when grouped by different regimens, the short-term, mid-term, and long-term follow-up data may be incomplete, so some analyses could only discuss the differences at a certain time point. On the basis of the above points, small sample sizes could lead to unreliable measurement results. Second, there seems to be some bias in this study; for example, the choice of antibiotics in quadruple radical therapy was associated with bacterial resistance and susceptibility, which may have contributed to selection bias. The emergence of heterogeneity was largely due to bias. Due to the small sample size included in the study, bias could not be assessed well. Finally, subgroup analyses demonstrated that age influenced the microbiota results, but more quantitative results were lacking to confirm further analyses.
Conclusions
Different regimens used to eradicate H. pylori have varied effects on the short-term and long-term abundance of the gut microbiota, but a decrease of the microbial diversity was consistent across all eradication regimens at the short-term follow-up evaluations. This study provides some references for supplementing probiotics before and after H. pylori eradication, while further studies is needed to support these findings.
Author contributions
All the authors meet all criteria for authorship in the ICMJE recommendations. All authors were involved in the article retrieval, data acquisition, interpretation of data, and writing this paper. All the authors agreed to be accountable for all aspects of the work. All authors contributed to the article and approved the submitted version.
Funding
The present study was supported by the New Xiangya Talent Projects of the Third Xiangya Hospital of Central South University (no. 20180304), Hunan Provincial Natural Science Foundation of China (no. 2020JJ4853), Hunan Provincial Clinical Medical Technology Innovation Guidance Project (no. 2020SK53616), Scientific Research Project of Hunan Provincial Health Commission (no. 202103032097), and Hunan Provincial Natural Science Foundation of China for Youths(no. 2020JJ5609).
Data availability statement
The raw data supporting the conclusions of this article will be made available by the authors, without undue reservation.
Conflict of interest
The authors declare that the research was conducted in the absence of any commercial or financial relationships that could be construed as a potential conflict of interest.
Publisher’s note
All claims expressed in this article are solely those of the authors and do not necessarily represent those of their affiliated organizations, or those of the publisher, the editors and the reviewers. Any product that may be evaluated in this article, or claim that may be made by its manufacturer, is not guaranteed or endorsed by the publisher.
Supplementary material
The Supplementary Material for this article can be found online at: https://www.frontiersin.org/articles/10.3389/fcimb.2022.913384/full#supplementary-material
References
Adak, A., Khan, M. R. (2019). An insight into gut microbiota and its functionalities. Cell Mol. Life Sci. 76 (3), 473–493. doi: 10.1007/s00018-018-2943-4
Adamsson, I., Edlund, C., Seensalu, R., Sjöstedt, S., Nord, C. E. (1998). The normal gastric microflora and helicobacter pylori; before, during and after treatment with omeprazole and amoxycillin. Clin. Microbiol. Infect. 4 (6), 308–315. doi: 10.1111/j.1469-0691.1998.tb00064.x
Adamsson, I., Nord, C. E., Lundquist, P., Sjöstedt, S., Edlund, C. (1999). Comparative effects of omeprazole, amoxycillin plus metronidazole versus omeprazole, clarithromycin plus metronidazole on the oral, gastric and intestinal microflora in helicobacter pylori-infected patients. J. Antimicrob. Chemother. 44 (5), 629–640. doi: 10.1093/jac/44.5.629
Barc, M. C., Bourlioux, F., Rigottier-Gois, L., Charrin-Sarnel, C., Janoir, C., Boureau, H., et al. (2004). Effect of amoxicillin-clavulanic acid on human fecal flora in a gnotobiotic mouse model assessed with fluorescence hybridization using group-specific 16S rRNA probes in combination with flow cytometry. Antimicrob. Agents Chemother. 48 (4), 1365–1368. doi: 10.1128/aac.48.4.1365-1368.2004
Bik, E. M., Eckburg, P. B., Gill, S. R., Nelson, K. E., Purdom, E. A., Francois, F., et al. (2006). Molecular analysis of the bacterial microbiota in the human stomach. Proc. Natl. Acad. Sci. U.S.A. 103 (3), 732–737. doi: 10.1073/pnas.0506655103
Blaabjerg, S., Artzi, D. M., Aabenhus, R. (2017). Probiotics for the prevention of antibiotic-associated diarrhea in outpatients-a systematic review and meta-analysis. Antibiot. (Basel) 6 (4). doi: 10.3390/antibiotics6040021
Buehling, A., Radun, D., Mueller, W. A., Malfertheiner, P. (2001). Influence of anti-helicobacter triple-therapy with metronidazole, omeprazole and clarithromycin on intestinal microflora. Aliment. Pharmacol. Ther. 15 (9), 1445–1452. doi: 10.1046/j.1365-2036.2001.01033.x
Chen, C. C., Liou, J. M., Lee, Y. C., Hong, T. C., El-Omar, E. M., Wu, M. S. (2021). The interplay between helicobacter pylori and gastrointestinal microbiota. Gut Microbes 13 (1), 1–22. doi: 10.1080/19490976.2021.1909459
Chen, L., Xu, W., He, J., Lee, A., Si, J., Chen, S. (2018). The impact of helicobacter pylori infection, eradication therapy, and probiotic supplementation on gut microenvironment homeostasis: An open-label, prospective clinical trial. J. Gastroenterol. Hepatol. 33, 75–75. doi: 10.1016/j.ebiom.2018.08.028
Choi, I. J., Kook, M. C., Kim, Y. I., Cho, S. J., Lee, J. Y., Kim, C. G., et al. (2018). Helicobacter pylori therapy for the prevention of metachronous gastric cancer. N Engl. J. Med. 378 (12), 1085–1095. doi: 10.1056/NEJMoa1708423
Coker, O. O., Dai, Z., Nie, Y., Zhao, G., Cao, L., Nakatsu, G., et al. (2018). Mucosal microbiome dysbiosis in gastric carcinogenesis. Gut 67 (6), 1024–1032. doi: 10.1136/gutjnl-2017-314281
Cornejo-Pareja, I., Martin-Nunez, G. M, Mar Roca-Rodriguez, M., Cardona, F., Coin-Araguez, L., Sanchez-Alcoholado, L., et al. (2019). H. pylori Eradication Treatment Alters Gut Microbiota and GLP-1 Secretion in Humans. J Of Clin Med 8 (4), 451. doi: 10.3390/jcm8040451
Ferreira, R. M., Pereira-Marques, J., Pinto-Ribeiro, I., Costa, J. L., Carneiro, F., Machado, J. C., et al. (2018). Gastric microbial community profiling reveals a dysbiotic cancer-associated microbiota. Gut 67 (2), 226–236. doi: 10.1136/gutjnl-2017-314205
Ford, A. C., Yuan, Y., Moayyedi, P. (2020). Helicobacter pylori eradication therapy to prevent gastric cancer: systematic review and meta-analysis. Gut 69 (12), 2113–2121. doi: 10.1136/gutjnl-2020-320839
Freeman, C. D., Klutman, N. E., Lamp, K. C. (1997). Metronidazole. a therapeutic review and update. Drugs 54 (5), 679–708. doi: 10.2165/00003495-199754050-00003
Gotoda, T., Takano, C., Kusano, C., Suzuki, S., Ikehara, H., Hayakawa, S., et al. (2018). Gut microbiome can be restored without adverse events after helicobacter pylori eradication therapy in teenagers. Helicobacter 23 (6), e12541. doi: 10.1111/hel.12541
Guillemard, E., Poirel, M., Schäfer, F., Quinquis, L., Rossoni, C., Keicher, C., et al. (2021). A randomised, controlled trial: Effect of a multi-strain fermented milk on the gut microbiota recovery after helicobacter pylori therapy. Nutrients 13 (9), 3171. doi: 10.3390/nu13093171
Guo, Y., Zhang, Y., Gerhard, M., Gao, J. J., Mejias-Luque, R., Zhang, L., et al. (2020). Effect of helicobacter pylori on gastrointestinal microbiota: a population-based study in linqu, a high-risk area of gastric cancer. Gut 69 (9), 1598–1607. doi: 10.1136/gutjnl-2019-319696
He, C., Peng, C., Wang, H., Ouyang, Y., Zhu, Z., Shu, X., et al. (2019). The eradication of helicobacter pylori restores rather than disturbs the gastrointestinal microbiota in asymptomatic young adults. Helicobacter 24 (4), e12590. doi: 10.1111/hel.12590
Horii, T., Suzuki, S., Takano, C., Shibuya, H., Ichijima, R., Kusano, C., et al. (2021). Lower impact of vonoprazan–amoxicillin dual therapy on gut microbiota for helicobacter pylori eradication. J. Gastroenterol. Hepatol. (Australia) 36 (12), 3314–3321. doi: 10.1111/jgh.15572
Hsu, P.-I., Pan, C.-Y., Kao, J. Y., Tsay, F.-W., Peng, N.-J., Kao, S.-S., et al. (2019). Short-term and long-term impacts of helicobacter pylori eradication with reverse hybrid therapy on the gut microbiota. J. Gastroenterol. Hepatol. 34 (11), 1968–1976. doi: 10.1111/jgh.14736
Hsu, P. I., Pan, C. Y., Kao, J. Y., Tsay, F. W., Peng, N. J., Kao, S. S., et al. (2018). Helicobacter pylori eradication with bismuth quadruple therapy leads to dysbiosis of gut microbiota with an increased relative abundance of Proteobacteria and decreased relative abundances of Bacteroidetes and Actinobacteria. Helicobacter 23 (4), e12498. doi: 10.1111/hel.12498
Hwang, Y. J., Kim, N., Lee, H. S., Lee, J. B., Choi, Y. J., Yoon, H., et al. (2018). Reversibility of atrophic gastritis and intestinal metaplasia after helicobacter pylori eradication - a prospective study for up to 10 years. Aliment. Pharmacol. Ther. 47 (3), 380–390. doi: 10.1111/apt.14424
Ianiro, G., Tilg, H., Gasbarrini, A. (2016). Antibiotics as deep modulators of gut microbiota: between good and evil. Gut 65 (11), 1906–1915. doi: 10.1136/gutjnl-2016-312297
Imase, K., Takahashi, M., Tanaka, A., Tokunaga, K., Sugano, H., Tanaka, M., et al. (2008). Efficacy of clostridium butyricum preparation concomitantly with helicobacter pylori eradication therapy in relation to changes in the intestinal microbiota. Microbiol. Immunol. 52 (3), 156–161. doi: 10.1111/j.1348-0421.2008.00026.x
Jakobsson, H. E., Jernberg, C., Andersson, A. F., Sjolund-Karlsson, M., Jansson, J. K., Engstrand, L. (2010). Short-term antibiotic treatment has differing long-term impacts on the human throat and gut microbiome. PLoS One 5 (3), e9836. doi: 10.1371/journal.pone.0009836
Kakiuchi, T., Mizoe, A., Yamamoto, K., Imamura, I., Hashiguchi, K., Kawakubo, H., et al. (2020). Effect of probiotics during vonoprazan-containing triple therapy on gut microbiota in helicobacter pylori infection: A randomized controlled trial. Helicobacter 25 (3), e12690. doi: 10.1111/hel.12690
Kuipers, E. J., Pérez-Pérez, G. I., Meuwissen, S. G., Blaser, M. J. (1995). Helicobacter pylori and atrophic gastritis: importance of the cagA status. J. Natl. Cancer Inst. 87 (23), 1777–1780. doi: 10.1093/jnci/87.23.1777
Liberati, A., Altman, D. G., Tetzlaff, J., Mulrow, C., Gøtzsche, P. C., Ioannidis, J. P., et al. (2009). The PRISMA statement for reporting systematic reviews and meta-analyses of studies that evaluate health care interventions: explanation and elaboration. PLoS Med. 6 (7), e1000100. doi: 10.1371/journal.pmed.1000100
Liou, J.-M., Chen, C.-C., Chang, C.-M., Fang, Y.-J., Bair, M.-J., Chen, P.-Y., et al. (2019). Long-term changes of gut microbiota, antibiotic resistance, and metabolic parameters after helicobacter pylori eradication: a multicentre, open-label, randomised trial. Lancet Infect. Dis. 19 (10), 1109–1120. doi: 10.1016/S1473-3099(19)30272-5
Lo, C. K., Mertz, D., Loeb, M. (2014). Newcastle-Ottawa Scale: comparing reviewers' to authors' assessments. BMC Med. Res. Methodol. 14, 45. doi: 10.1186/1471-2288-14-45
Macfarlane, S., Macfarlane, G. T. (2003). Regulation of short-chain fatty acid production. Proc. Nutr. Soc. 62 (1), 67–72. doi: 10.1079/pns2002207
Madden, J. A., Plummer, S. F., Tang, J., Garaiova, I., Plummer, N. T., Herbison, M., et al. (2005). Effect of probiotics on preventing disruption of the intestinal microflora following antibiotic therapy: a double-blind, placebo-controlled pilot study. Int. Immunopharmacol. 5 (6), 1091–1097. doi: 10.1016/j.intimp.2005.02.006
Martín-Núñez, G. M., Cornejo-Pareja, I., Roca-Rodríguez, M. D. M., Clemente-Postigo, M., Cardona, F., Fernández-García, J. C., et al. (2020). H. pylori Eradication Treatment Causes Alterations in the Gut Microbiota and Blood Lipid Levels. Front Med (Lausanne) 7, 417. doi: 10.3389/fmed.2020.00417.10.3389/fmed.2020.00417
McNulty, C. A. (1999). The discovery of campylobacter-like organisms. Curr. Top. Microbiol. Immunol. 241, 1–9. doi: 10.1007/978-3-642-60013-5_1
Myllyluoma, E., Ahlroos, T., Veijola, L., Rautelin, H., Tynkkynen, S., Korpela, R. (2007). Effects of anti-helicobacter pylori treatment and probiotic supplementation on intestinal microbiota. Int. J. Antimicrob. Agents 29 (1), 66–72. doi: 10.1016/j.ijantimicag.2006.08.034
Oh, B., Kim, J. W., Kim, B.-S. (2016b). Changes in the functional potential of the gut microbiome following probiotic supplementation during helicobacter pylori treatment. Helicobacter 21 (6), 493–503. doi: 10.1111/hel.12306
Olekhnovich, E. I., Manolov, A. I., Samoilov, A. E., Prianichnikov, N. A., Malakhova, M. V., Tyakht, A. V., et al. (2019). Shifts in the Human Gut Microbiota Structure Caused by Quadruple Helicobacter pylori Eradication Therapy. Front In Microbiol 10, 1902. doi: 10.3389/fmicb.2019.01902
Oh, B., Kim, B.-S., Kim, J. W., Kim, J. S., Koh, S.-J., Kim, B. G., et al. (2016a). The effect of probiotics on gut microbiota during the helicobacter pylori eradication: Randomized controlled trial. Helicobacter 21 (3), 165–174. doi: 10.1111/hel.12270
Plummer, S. F., Garaiova, I., Sarvotham, T., Cottrell, S. L., Le Scouiller, S., Weaver, M. A., et al. (2005). Effects of probiotics on the composition of the intestinal microbiota following antibiotic therapy. Int. J. Antimicrobial. Agents 26 (1), 69–74. doi: 10.1016/j.ijantimicag.2005.04.004
Rajilic-Stojanovic, M., Figueiredo, C., Smet, A., Hansen, R., Kupcinskas, J., Rokkas, T., et al. (2020). Systematic review: gastric microbiota in health and disease. Aliment. Pharmacol. Ther. 51 (6), 582–602. doi: 10.1111/apt.15650
Rokkas, T., Gisbert, J. P., Malfertheiner, P., Niv, Y., Gasbarrini, A., Leja, M., et al. (2021). Comparative effectiveness of multiple different first-line treatment regimens for helicobacter pylori infection: A network meta-analysis. Gastroenterology 161 (2), 495–507.e494. doi: 10.1053/j.gastro.2021.04.012
Sartor, R. B. (2008). Microbial influences in inflammatory bowel diseases. Gastroenterology 134 (2), 577–594. doi: 10.1053/j.gastro.2007.11.059
Sekirov, I., Russell, S. L., Antunes, L. C., Finlay, B. B. (2010). Gut microbiota in health and disease. Physiol. Rev. 90 (3), 859–904. doi: 10.1152/physrev.00045.2009
Shimbo, I., Yamaguchi, T., Odaka, T., Nakajima, K., Koide, A., Koyama, H., et al. (2005). Effect of clostridium butyricum on fecal flora in helicobacter pylori eradication therapy. World J. Gastroenterol. 11 (47), 7520–7524. doi: 10.3748/wjg.v11.i47.7520
Sugano, K., Tack, J., Kuipers, E. J., Graham, D. Y., El-Omar, E. M., Miura, S., et al. (2015). Kyoto Global consensus report on helicobacter pylori gastritis. Gut 64 (9), 1353–1367. doi: 10.1136/gutjnl-2015-309252
Sung, J. J. Y., Coker, O. O., Chu, E., Szeto, C. H., Luk, S. T. Y., Lau, H. C. H., et al. (2020). Gastric microbes associated with gastric inflammation, atrophy and intestinal metaplasia 1 year after helicobacter pylori eradication. Gut 69 (9), 1572–1580. doi: 10.1136/gutjnl-2019-319826
Wang, Z. J., Chen, X. F., Zhang, Z. X., Li, Y. C., Deng, J., Tu, J., et al. (2017). Effects of anti-helicobacter pylori concomitant therapy and probiotic supplementation on the throat and gut microbiota in humans. Microb. Pathog. 109, 156–161. doi: 10.1016/j.micpath.2017.05.035
Wang, Y. H., Huang, Y. (2014). Effect of lactobacillus acidophilus and bifidobacterium bifidum supplementation to standard triple therapy on helicobacter pylori eradication and dynamic changes in intestinal flora. World J. Microbiol. Biotechnol. 30 (3), 847–853. doi: 10.1007/s11274-013-1490-2
Williams, J. D., Maskell, J. P., Shain, H., Chrysos, G., Sefton, A. M., Fraser, H. Y., et al. (1992). Comparative in-vitro activity of azithromycin, macrolides (erythromycin, clarithromycin and spiramycin) and streptogramin RP 59500 against oral organisms. J. Antimicrob. Chemother. 30 (1), 27–37. doi: 10.1093/jac/30.1.27
Yanagi, H., Tsuda, A., Matsushima, M., Takahashi, S., Ozawa, G., Koga, Y., et al. (2017). Changes in the gut microbiota composition and the plasma ghrelin level in patients with helicobacter pylori-infected patients with eradication therapy. BMJ Open Gastroenterol. 4 (1), e000182. doi: 10.1136/bmjgast-2017-000182
Yap, T. W. C., Gan, H. M., Lee, Y. P., Leow, A. H. R., Azmi, A. N., Francois, F., et al. (2016). Helicobacter pylori eradication causes perturbation of the human gut microbiome in young adults. PLoS One 11 (3), e0151893. doi: 10.1371/journal.pone.0151893
Ye, Q., Shao, X., Shen, R., Chen, D., Shen, J.. (2020). Changes in the human gut microbiota composition caused by Helicobacter pylori eradication therapy: A systematic review and meta-analysis. Helicobacter 25 (4), e12713. doi: 10.1111/hel.12713713
Yildiz, S. S., Yalinay, M., Karakan, T.. (2018). Bismuth-based quadruple Helicobacter pylori eradication regimen alters the composition of gut microbiota. Le infezioni medicina 26 (2), 115–121.
Keywords: Helicobacter pylori, gastrointestinal microbiota, eradication regimen, dysbiosis, meta-analysis
Citation: Chen B, Li X-m, Cai T and Wang F (2022) Short-term and long-term alterations of gastrointestinal microbiota with different H. pylori eradication regimens: A meta-analysis. Front. Cell. Infect. Microbiol. 12:913384. doi: 10.3389/fcimb.2022.913384
Received: 05 April 2022; Accepted: 28 June 2022;
Published: 25 July 2022.
Edited by:
D. Scott Merrell, Uniformed Services University, United StatesReviewed by:
Peter Malfertheiner, University Hospital Magdeburg, GermanyJennifer Angeline Gaddy, Vanderbilt University Medical Center, United States
Copyright © 2022 Chen, Li, Cai and Wang. This is an open-access article distributed under the terms of the Creative Commons Attribution License (CC BY). The use, distribution or reproduction in other forums is permitted, provided the original author(s) and the copyright owner(s) are credited and that the original publication in this journal is cited, in accordance with accepted academic practice. No use, distribution or reproduction is permitted which does not comply with these terms.
*Correspondence: Fen Wang, d2Zlbi1qdWR5QGNzdS5lZHUuY24=