- 1Manchester Fungal Infection Group, Division of Evolution, Infection and Genomics, Faculty of Biology, Medicine and Health, University of Manchester, Manchester, United Kingdom
- 2Department of Statistics, Athens University of Economics and Business, Athens, Greece
- 3MRC Centre for Medical Mycology, University of Exeter, Exeter, United Kingdom
- 4Division of Informatics, School of Heath Sciences, University of Manchester, Manchester, United Kingdom
Damage to the lung epithelium is a unifying feature of disease caused by the saprophytic fungus Aspergillus fumigatus. However, the mechanistic basis and the regulatory control of such damage is poorly characterized. Previous studies have identified A. fumigatus mediated pathogenesis as occurring at early (≤ 16 hours) or late (>16 hours) phases of the fungal interaction with epithelial cells, and respectively involve direct contact with the host cell or the action of soluble factors produced by mature fungal hyphae. Both early and late phases of epithelial damage have been shown to be subject to genetic regulation by the pH-responsive transcription factor PacC. This study sought to determine whether other transcriptional regulators play a role in modulating epithelial damage. In particular, whether the early and late phases of epithelial damage are governed by same or distinct regulators. Furthermore, whether processes such as spore uptake and hyphal adhesion, that have previously been documented to promote epithelial damage, are governed by the same cohorts of epithelial regulators. Using 479 strains from the recently constructed library of A. fumigatus transcription factor null mutants, two high-throughput screens assessing epithelial cell detachment and epithelial cell lysis were conducted. A total of 17 transcription factor mutants were found to exhibit reproducible deficits in epithelial damage causation. Of these, 10 mutants were defective in causing early phase damage via epithelial detachment and 8 mutants were defective in causing late phase damage via epithelial lysis. Remarkably only one transcription factor, PacC, was required for causation of both phases of epithelial damage. The 17 mutants exhibited varied and often unique phenotypic profiles with respect to fitness, epithelial adhesion, cell wall defects, and rates of spore uptake by epithelial cells. Strikingly, 9 out of 10 mutants deficient in causing early phase damage also exhibited reduced rates of hyphal extension, and culture supernatants of 7 out of 8 mutants deficient in late phase damage were significantly less cytotoxic. Our study delivers the first high-level overview of A. fumigatus regulatory genes governing lung epithelial damage, suggesting highly coordinated genetic orchestration of host-damaging activities that govern epithelial damage in both space and time.
Introduction
The lung is the major portal of human exposure to airborne particles, including the spores of various fungal species, some of which can cause severe infections. Spores of the saprophytic pathogen, Aspergillus fumigatus are small (2-3 μm) and ubiquitous in indoor and outdoor environments (Kwon-Chung and Sugui, 2013; van Rhijn et al., 2021). The spores upon inhalation, easily reach the alveolar regions of the human lung (Escobar et al., 2016). Interaction of A. fumigatus spores with the respiratory epithelium can sometimes cause disease, most often in hosts having immune dysfunction. The resultant spectrum of pulmonary syndromes, called aspergilloses, can be broadly categorised into allergic, chronic and invasive diseases. The most severe manifestation is invasive pulmonary aspergillosis (IPA) occurring in individuals with extreme immunocompromise such as prolonged neutropenia in haematological malignancy, post- stem cell or solid organ transplantation, or on immunosuppressive treatments (Kosmidis and Denning, 2015). IPA can also complicate the clinical course of COVID-19 and influenza and is associated with a significant increase in mortality, especially in critically ill patients admitted to intensive care units (Schauwvlieghe et al., 2018; Casalini et al., 2021). The annual burden of fatal disease is estimated at over 200,000 deaths per annum for IPA (Brown et al., 2012; Bongomin et al., 2017). Understanding the pathogenic mechanisms leading to lung damage, as well as identifying A. fumigatus genes and attributes involved in the process could lead to a more targeted therapeutic strategy that subverts adverse pathology.
The pathogenesis of A. fumigatus-mediated disease occurs in a stepwise fashion that involves the morphological transition of the inhaled fungal spore into a hyphal form. Broadly speaking, epithelial damage can be considered as occurring at early (spore) or late (hyphal) phases of the fungal interaction with epithelial cells. Contact-mediated damage leads to detachment of the epithelial cells within 16 hours of A. fumigatus infection in vitro, wherein even a mere contact with spores leads to loss of actin polymerisation, rounding and epithelial detachment (Kogan et al., 2004), or via activation of host immune signaling (Okaa et al., 2021). In vitro studies have shown that alveolar epithelial cells internalise 30-50% of encountered spores (Wasylnka and Moore, 2003) leading to fungal killing or intracellular occupancy that might aid immune evasion and dissemination (reviewed in Bertuzzi et al., 2018). Further, actin-mediated internalisation of fungal spores might also disrupt epithelial integrity by provoking the detachment of epithelial cells during early infection (Bertuzzi et al., 2014). If the spore form is not rapidly neutralized by the combined activities of epithelial cells and professional phagocytes, spore germination initiates with the breaking of dormancy and isotropic swelling of the spores (Baltussen et al., 2019). Spore germination leads to outgrowth of an apically extending cell called a primary hypha. Hyphal growth within, or close to, epithelial monolayers is associated with fungal invasion of epithelial cells, and transcriptomic analysis of this process both in vitro and in vivo suggests the involvement of fungal proteases and toxic secondary metabolites (Bertuzzi et al., 2014; Escobar et al., 2018). Any alterations in cell wall composition has been implied in deficient host-fungal interaction and altered virulence in invasive fungi (Bulawa et al., 1995; Valiante et al., 2015). In vitro infection studies have revealed specific cell wall adhesins mediating adhesion to the lung epithelium at the distinct morphological growth stages of the fungus (Levdansky et al., 2010; Liu et al., 2016; Voltersen et al., 2018). The secreted and hyphal exopolysaccharide galactosaminogalactan (GAG), that mediates adherence of A. fumigatus hyphae to host cells is also critical for host damage and for virulence in murine IPA models (Gravelat et al., 2013). A. fumigatus secreted proteases cause destruction of the mammalian F-actin cytoskeleton and loss of focal adhesion (Kogan et al., 2004; Namvar et al., 2015), and the secreted secondary metabolite gliotoxin has been shown to exert a directly cytotoxic and immune-modulatory effect on several host cells, including airway epithelial cells (Scharf et al., 2012; Zhang et al., 2019). However, strains lacking certain protease activities or deficient in gliotoxin biosynthesis still retain virulence in vivo, (Bok et al., 2006; Sharon et al., 2009a; Shemesh E. et al., 2017) suggesting that the coordinated activities of multiple fungal attributes combine to elicit fatal tissue damage in the host. Supporting this theory, Bertuzzi et al. (2014) revealed that A. fumigatus mutants lacking the pH-responsive transcription factor PacC fail to activate the expression of a multitude of secreted proteases and secondary metabolites during host colonization. In vitro dissection of this phenotype revealed a 50% reduction in detachment of A549 cells from cultured monolayers at an early stage of infection with a ΔpacC strain, as well as a reduction in epithelial cell lysis occurring at a later timepoint. Moreover, the mutants were unable to penetrate the lung epithelium and demonstrated reduced virulence in a murine model of aspergillosis, in comparison to the parental strain (Bertuzzi et al., 2014).
By negating the effects of redundancy of gene products involved in host-pathogen interactions of invasive fungi in the establishment of infection, transcription factor (TF) mutants have proven to be powerful tools for resolving the complexity of host-pathogen interactions that drive fungal diseases of humans (Nobile et al., 2012; Jung et al., 2015). Although multiple A. fumigatus TFs regulating fungal physiology and growth processes have been implicated in in vivo virulence (Bultman et al., 2017), no high throughput or genome wide studies on epithelial damage the host-pathogen interaction employing transcription factors have been previously carried out in the Aspergillus genus. The recently generated library of A. fumigatus transcription factor mutants (TFKOs) by (Furukawa et al., 2020) has provided a methodological toolkit that allowed, for the first time, a genome-scale census of A. fumigatus TFs driving epithelial damage. This study sought to identify the transcriptional regulators that are required for causation of epithelial damage and via phenotypic analyses to identify the underlying causal events during the host-pathogen interaction.
Material and Methods
A. fumigatus Strains and Growth Conditions
A collection of 479 A. fumigatus transcription factor knock-out mutants (Furukawa et al., 2020) constructed in the A1160p+ genetic background, also known as MFIG001 (Fraczek et al., 2013; Bertuzzi et al., 2021) was used in this study. The TFKO strains were stored at -80°C and cultured on Aspergillus Complete Media agar (ACM) (Pontecorvo et al., 1953), pH 6.5 containing Hygromycin B (Alfa Aesar, UK) at a concentration of 100 µg/ml for selective growth. The fungus was cultured on ACM at 37°C for 3-5 days and the spores were harvested in sterile distilled water and the spore suspensions were filtered through sterile miracloth (Calbiochem, UK) to remove mycelial fragments. Following two washes of the spore suspension with sterile water, the spores/ml were enumerated using OD600 measurement (Furukawa et al., 2020). Accuracy of spore counts in infecting inocula was assessed via serial dilution and enumeration of viable fungal colonies following culture on ACM solid agar.
Culture and Maintenance of Epithelial Cells
Commercially sourced human carcinomic alveolar basal epithelial A549 cells were used in this study (American type culture collection, CCL-185). A549 cells were routinely cultivated and maintained in RPMI-1640 medium with L-glutamine, (Sigma-Aldrich) supplemented with 10% fetal bovine serum (Gibco, UK) and 1% penicillin-streptomycin (Sigma-Aldrich) at 37°C with 5% CO2. Cells were seeded at 5x104/ml for a 24 well plate and at 7.5x104/ml for a 96 well plate and incubated for 2 days at 37°C and 5% CO2 for a 90-100% confluent A549 monolayer. On infection day, media was replaced, and all infections were performed in supplemented RPMI-1640 medium (as above, with additional trace elements (Na2B4O7.10H2O (0.04 mg/l), CuSO4. 5H2O (0.4 mg/l), FeCl3.6H2O (1.16 mg/l), MnSO4. 2H2O (0.8 mg/l), Na2MoO4. 2H2O (0.8 mg/l), ZnSO4. 7H2O (8.0 mg/l)) at 37°C and 5% CO2.
High-Throughput Screens for Epithelial Damage
The parental strain (A1160p+) and the PBS challenge were included as controls for each infection in both the detachment and cell lysis assays. To ensure the measurement of damage from the high-throughput screens was not an artefact of inoculum size, A549 cells were challenged with a 10 times serial dilution of spores of the A. fumigatus parental strain (A1160p+) to calculate dose-dependency of epithelial damage phenotypes. According to standard curves generated by plotting viable spore density versus epithelial detachment or lysis, the phenotypic data were retrospectively adjusted for small deviances from desired inoculum sizes.
Detachment Assay
As detailed in Rahman et al, 2021, A549 monolayers were cultured to confluence in 96 well glass bottom plates (Greiner Bio-one) and challenged with 20 μl of a 107 spores/ml suspension (200,000 spores per well), with five technical replicates (one technical replicate per plate). After 16 hours, the A549 monolayer was washed once with pre-warmed phosphate buffered saline (PBS) (Thermo-Fisher Scientific) to remove detached A549 cells. This was followed by fixing the remaining adherent A549 cells in the wells with 4% formaldehyde in PBS for 10 min (Alfa Aesar, UK), permeabilizing the cells with 0.2% Triton-X100 (VWR) for 2 min and staining nuclei of the adherent A549 cells with DAPI (Alfa Aesar) at 300 nM for 5 min, protected from direct light (Rahman et al., 2021). DAPI fluorescence was excited with a 405nm LED and its emission was captured on a HyD detector (405-600 nm). Image capture was performed in high throughput via automated confocal microscopy (Leica SP8x; Leica Microsystems, Germany) from 9 fields of view per well at 40x magnification using a HC PL APOCS 40x/0.85 DRY objective. The number of adherent A549 cells in each image was quantified using a cell segmentation macro written for FIJI (Rahman et al., 2021). Viable counts of inocula used for infection were determined by plating 103 spores on ACM agar for 48 hr. The difference between the observed and predicted counts was calculated for each mutant.
Cell Lysis Assay
Fully confluent A549 cells grown in 24 well plates (Greiner Bio-one) were challenged with 50 μl of 107 spores/ml suspension (500,000 spores per well) with at least 3 technical replicates in one plate. For fungal culture filtrates a 106 spores/ml culture of RPMI was incubated at 37°C and 5% CO2 for 48 hours and then filtered through five layers of Miracloth (Calbiochem, UK) and then a 0.45 µm syringe filter to remove any spores and hyphal fragments. Elimination of all live spores from the culture filtrate was confirmed by plating of the filtrates on solid ACM agar for up to 48 hours. Fully confluent A549 monolayers in 24 well plates were challenged with a 1:5 dilution (in supplemented RPMI) of the filtered culture filtrates for 24 hours. Following incubation with A. fumigatus spores or culture filtrates for 24 hours, cell culture supernatants were collected to measure LDH released by epithelial cells on lysis, using the Cytox 96 non-radioactive cytotoxicity assay kit (Promega), as per manufacturer’s instructions. The LDH assay was carried out in 96 or 384 well plate format and a recombinant porcine LDH enzyme (Sigma-Aldrich) was used in each LDH assay plate in a serial dilution to extrapolate a standard curve which was used to estimate LDH released on infection with A. fumigatus. Viable counts of inocula used for infection were determined by plating 100 spores on solid ACM agar for up to 48 hours.
Internalisation of Spores by Epithelial Cells
A. fumigatus spores were stained with fluorescein isothiocyanate (FITC, Sigma) for 30 min at 37°C while shaking. After three washes with PBS, 3x105 spores were added onto fully confluent A549 monolayers in 24 well glass bottom plates. Following 4 hours of infection, the wells were washed two times with PBS to remove non-adherent spores, and the non-internalized spores were stained with 0.1 mg/ml of Calcofluor White in PBS (CFW; Sigma) for 5 min at 37°C. The wells were washed again twice with PBS, fixed for 15 min at 37°C using 5% formaldehyde and stored in PBS protected from light. Fluorescence images from 9 fields of view were captured in each well using a 1.5 pin hole and a 40 x objective of a Leica SP8x confocal microscope (Leica Microsystems, Germany). External CFW stained spore fluorescence was excited with a 405nm LED and collected on a HyD detector (410-450 nm) and all spore FITC fluorescence was excited with a 488nm Argon laser and collected on a HyD detector (495-570nm). The number of external and internal spores within the total population in each field of view of pixel size (388.26 µm x 388.26 µm) were counted manually in FIJI (Schindelin et al., 2012) for all strains and expressed as % spores internalized. The % uptake relative to the parental strain was calculated of each strain.
Germination Efficiency and Hyphal Extension
Fungal growth was assessed by time-lapse transmitted light imaging (Leica SP8; Leica Microsystems, Germany) of strains cultured in supplemented RPMI (as described above) at 37°C. Approximately 5x104 spores were inoculated per well of a 24 well glass bottom plate (Greiner Bio-one) and allowed to settle for 45-60 min at 37°C. Transmitted light images were captured using a 10x/0.4NA lens and a 514nm argon laser, in each well of the plate every hour up to 48 hours. Approximately 100 spores were captured in each image. The total number of germinated spores were counted at every time point using FIJI and the germination rate was calculated. The lengths of individual hyphae were measured at each time-point starting from germination, by using the segmented line measurement tool in FIJI (Schindelin et al., 2012). Hyphae were measured up until the moment they grew out of the optical plane, of approximately 16-18 hours for the parental strain.
Adhesion to Epithelial Cells
Germlings were generated by growth of 5x104 spores/ml in supplemented RPMI (as described above) for 7 hours at 37°C and added to fully confluent A549 cells in a 24-well glass bottom plate (Greiner Bio-one). Germlings for the TF mutants with a delay in germination were grown for a longer length of time until they achieve approximately similar visual hyphal growths as the parental strain. Following 30 min of incubation, non-adherent germlings were removed by a PBS rinse and the remaining adherent germlings were stained with 10 µg/ml of CFW for 5 min, prior to fixation with 4% formaldehyde. A 1441 µm2 field of view was captured in each well using a 10x lens objective for CFW germling fluorescence using a confocal microscope (Leica SP8x; Leica Microsystems, Germany). Fluorescence was excited with a 405nm LED and collected on a HyD detector (410-450nm). The number of adherent germlings remaining in each well were counted manually in FIJI (Schindelin et al., 2012).
Cell Wall Sensitivity Assay
Sensitivity to the cell wall destabilizing agent, CFW, was tested by inoculating serial spore concentrations (106, 105, 104, 103) onto solid aspergillus minimal media agar (Bertuzzi et al., 2014) with CFW (200 ug/ml).
Statistics
Log2 transformed LDH data were filtered for assay plate-dependent batch effects and analyzed using a Bayesian modelling approach (Kruschke, 2013) that describes data using a Student’s t distribution and performs posterior inference by Markov chain Monte Carlo sampling. We compared the posterior distributions for each TFKO mutant to the corresponding parental strain of each assay plate. Significant difference between the two means, based on a 95% Bayesian credible interval, was deemed “significant” and is denoted by TRUE in Table S2. Data are presented as the mean value ± standard deviation (SD). Other statistical tests were performed using Prism 9.00 software (GraphPad Software, La Jolla, CA). Quantitative differences between groups were tested using ANOVA one way or two-way analysis. To correct for multiple testing, Fishers LSD t-test (comparisons of selected groups to the parental strain) was applied. Data was also analysed using multiple t-tests or two-way ANOVA Sidak’s comparisons test of means. p < 0.01 was considered statistically significant for these tests.
Results
Distinct Cohorts of Transcriptional Regulators Drive Early and Late Phases of A. fumigatus-Mediated Epithelial Damage
In order to identify transcriptional regulators required for epithelial damage during A. fumigatus infection 479 A. fumigatus knock-out mutants (TFKOs), each lacking an individual transcription factor-encoding gene (Furukawa et al., 2020), were incubated with A549 alveolar epithelial monolayers. Damage was measured at 16 hours and 24 hours of infection, respectively using high throughput quantitation of epithelial cell detachment and epithelial cell lysis (Bertuzzi et al., 2014; Okaa et al., 2021; Rahman et al., 2021) as indicators of host cell damage. Assay formats were optimised for high throughput use by assessing damage caused by A. fumigatus A1160p+ versus that of a ΔpacC null mutant which is deficient in causing both early and late stages of the host damage (Figure S1).
Detachment of epithelial cells was quantified following a 16-hour infection of A549 monolayers with A. fumigatus spores (Figure 1A). Of the 479 TFKO mutants initially screened (Table S1), 18 and 28 TFKOs exhibited an increased and decreased capacity, respectively, to cause detachment of epithelial cells during a 16-hour infection (Figure 1B). For the purposes of this study, our interest focused upon those TFKOs demonstrating reduced epithelial detachment. In a follow-up low throughput assay, 10 of the 28 originally identified TFKOs that were identified as being defective in eliciting epithelial cell detachment exhibited reproducible phenotypes (Figure 1C and Table 1). Among these, 6 TFs have been previously characterised, including the pH-responsive transcription factor PacC (AFUB_037210) previously reported to regulate A. fumigatus epithelial invasion (Bertuzzi et al., 2014), CreA (AFUB_027530) the broad domain regulator of carbon catabolism (Beattie et al., 2017), AreA (AFUB_096370) the broad domain regulator of nitrogen metabolism (Hensel et al., 1998; Krappmann and Braus, 2005), a C6 zinc cluster family TF (AFUB_033930) located in the uncharacterised small peptide secondary metabolite gene cluster 12 (Bignell et al., 2016) and two TFs NsdD and NsdC (AFUB_035330 and AFUB_089440) that repress the asexual sporulation programme in Aspergillus species (Wu et al., 2018; de Castro et al., 2021).
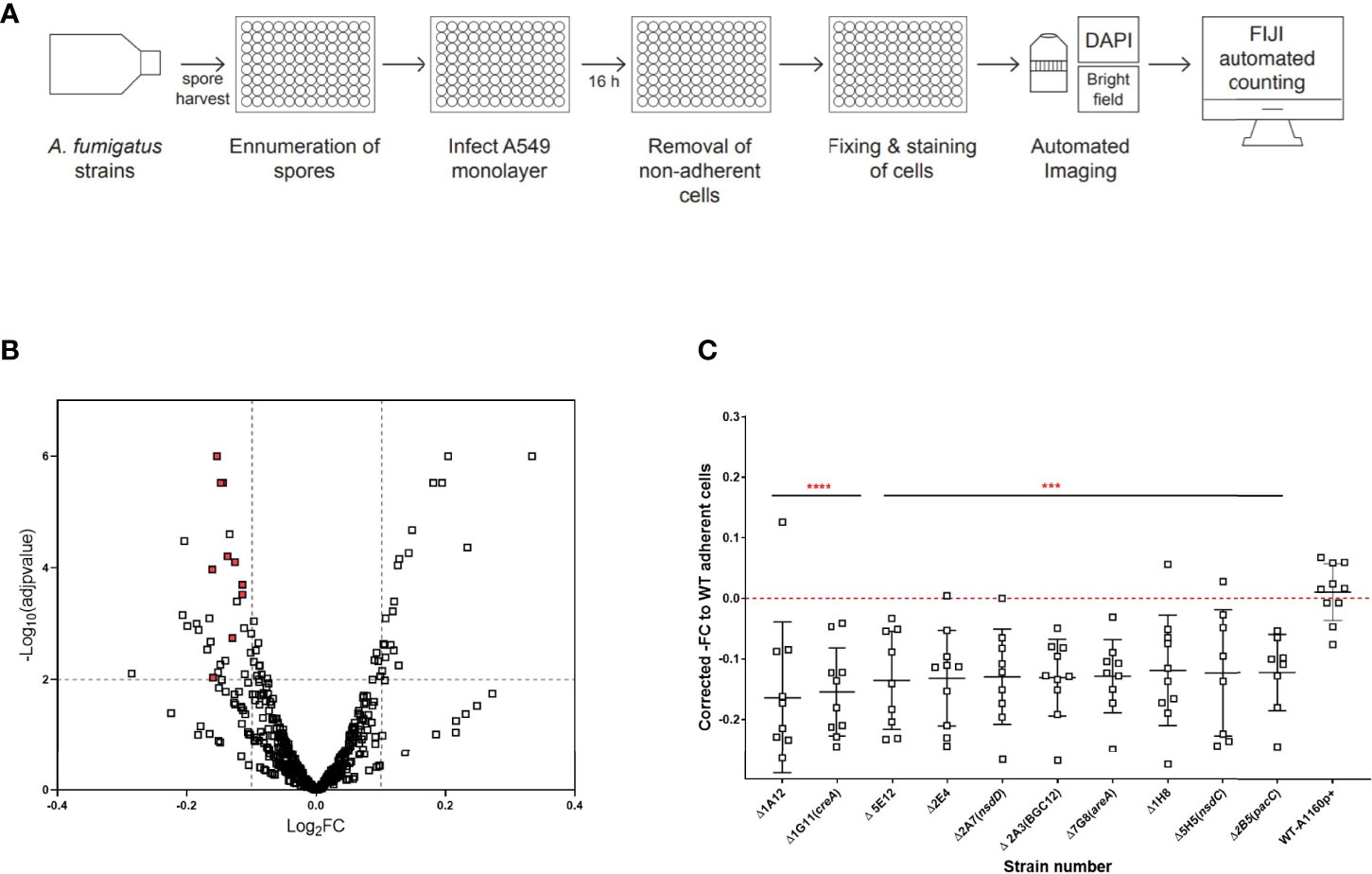
Figure 1 High throughput screening identifies A. fumigatus transcription factors required for pneumocyte detachment. (A) Experimental design to analyse detachment of epithelial cells in high throughput. Harvested spores of A. fumigatus TFKOs were counted using OD600 measurement and incubated with A549 cell monolayers for 16 hours, followed by automated imaging and quantification of remaining number of A549 cells in the monolayer to determine epithelial cell damage via detachment of cells. (B) Volcano plot showing the output from the detachment screen. Data is transformed as -fold change number of adherent cells for 479 TF mutants. The cut-off (dotted line) on the y-axis signifies p value<0.1 from Fisher’s LSD multiple comparison of one-way ANOVA relative to the parental strain. The cut-off on the x axis of the plot was based upon the average fold change and between the controls (parental strain and the ΔpacC). (C) Fold change number of adherent cells, relative to the parental strain for the 10 cell detachment mutants. The 10 cell detachment mutants showing reproducibility of phenotypes when assay was conducted in low throughput. Data shown is technical replicates of two biological replicates. Error bars show ± SD. Data was analysed by one-way ANOVA with Fisher’s LSD multiple comparisons test relative to the parental strain (WT-A1160p+). ***P<0.001, ****P<0.0001.
Epithelial cell lysis was measured by infecting fully confluent A549 alveolar monolayers with spores from the TFKO null mutants for 24 hours, after which LDH released by the epithelial cells lysed because of hyphal mediated damage was quantified (Figure 2A). A. fumigatus was not found to secrete endogenous LDH enzyme at this time-point, therefore confirming the sole source of LDH as being epithelial cells (Figure S1). The quantification of LDH was performed via a standard curve generated from a serial dilution of the standard LDH enzyme for each assay plate. A Bayesian approach for calculation of the posterior probability that mutants deviate from the wild type was applied to overcome batch-related variations in LDH quantitation (Table S2). After filtering for plate and batch effects, 34 TFKOs caused a decreased LDH release by the A549 epithelial monolayers after a 24-hour infection (Figure 2B and Table S1). Of these 34, only 8 TFs demonstrated reproducible reductions in LDH activity with a reproducibility of 2/3 or 2/2 without one Standard deviation of each other (Figure 2C and Table 1). Among these 8 TFKOs, only 4 TF-encoding genes have been previously characterised. This includes HapX (AFUB_052420), a component of the CCAAT-binding complex (Hortschansky et al., 2017) which is involved in iron acquisition and metabolism in Aspergillus (Gsaller et al., 2014), PacC (AFUB_037210) previously reported to regulate A. fumigatus epithelial invasion (Bertuzzi et al., 2014), Ace1/SltA (AFUB_041100) which regulates conidial formation including cell wall architecture and secretion of mycotoxins and secondary metabolites (Liu et al., 2021) and AtfD (AFUB_078150) which is involved in conidial stress responses (Silva et al., 2021).
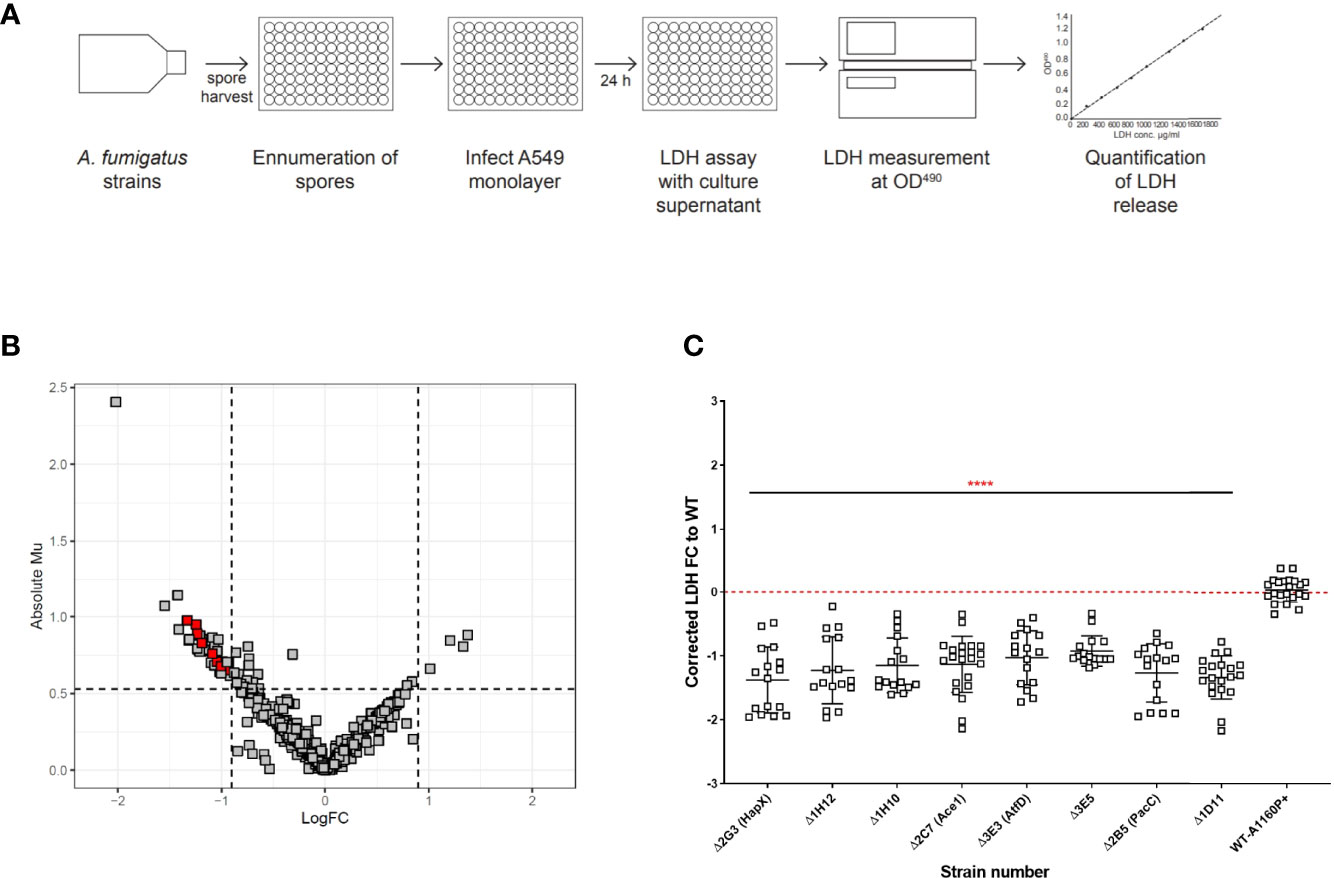
Figure 2 High throughput screening identifies A. fumigatus transcription factors required for cytolytic death of pneumocytes. (A) Experimental design to analyse cell lysis of epithelial monolayers in high throughput using LDH release assay. Harvested spores of A. fumigatus TF mutants were counted using OD600 measurement and incubated with A549 cell monolayers for 24 hours, followed by measurement of LDH in the supernatant to determine epithelial cell damage via cell lysis. Volcano plot showing the output from the cell lysis screen. (B) Data is filtered to remove plate/batch errors and shows the Absolute Mu (derived from Bayesian Modelling) versus log Fold change for each mutant relative to the parental strain. The cut-offs for the plot were based upon the average fold change and the average Mu difference between the controls (parental strain and the ΔpacC) throughout the screen. (C) LDH fold change relative to the parental strain for the 8 cell lysis mutants. The 8 cell lysis mutants showing reproducibility of phenotypes when assay conducted in low throughput. Data shown is technical replicates of at least two biological replicates. Error bars show ± SD. Data was analysed by one-way ANOVA with Fishers’ LSD multiple comparisons test relative to the parental strain (WT-A1160p+). ****P<0.0001.
TFKOs with defects in detachment did not exhibit a reduced ability to cause cell lysis relative the parental strain and vice versa. PacC (Bertuzzi et al., 2014) was found to be the only transcription factor that is required for causation of both epithelial cell detachment and cell lysis (Table 1). Conclusively, our analysis identified distinct cohorts of transcriptional regulators involved in the early and late causation of epithelial damage.
Hyphal Growth Rates Distinguish Between A. fumigatus TFKO Mutants Deficient in Epithelial Detachment and Cell Lysis
An obvious explanation for the observed deficits in epithelial detachment and cytolysis might be reduced fitness of the respective TFKOs. At early time points of host interaction this might derive from germination defects, at later time points from reductions in hyphal growth rates, or both. To explore this possibility, the germination efficiencies and hyphal growth rates of the TFKOs were measured at hourly intervals using time-lapse confocal imaging. Of the 17 mutants identified as having significant deficits in epithelial damage, four (ΔpacC, ΔcreA, ΔhapX, and ΔsltA) exhibited at least 50% reductions in germination and/or hyphal growth rates relative to the parental strain (Figure 3 and Figure S2). Enumeration of spore germination in the parental isolate A1160p+ revealed that spore germination commenced at 4 hours reaching 100% by 10-11 hours. Amongst the TFKOs causing reduced epithelial cell detachment (Table 1), most exhibited similar germination efficiencies to that of the parental strain (Figures S2A, C). Notable exceptions are the ΔpacC and ΔcreA strains that exhibited 70% germination at 11 hours (Figure S2), achieving 100% germination by 16 hours and the ΔhapX, and ΔsltA mutants where only 20- 30% of spores had germinated at 11 hours (Figure 3). Even after 20 hours, germination efficiencies fell well short of 100% for both the ΔhapX, and ΔsltA mutants.
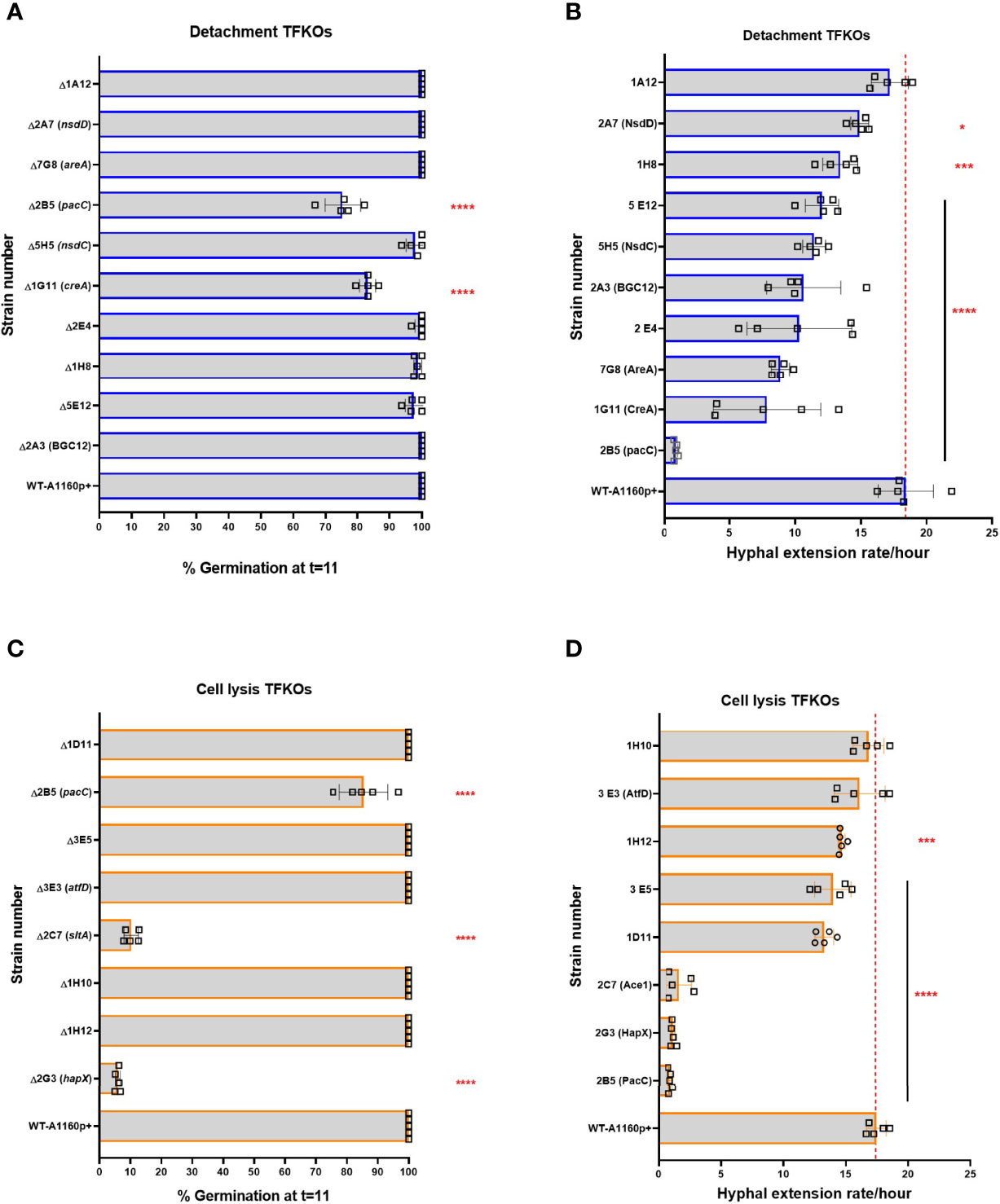
Figure 3 Percentage germination and hyphal extension rates of A. fumigatus TFKOs measured by assessing growth using time-lapse confocal microscopy. Mutants of the cell damage TFKOs were grown in supplemented RPMI-1640 for up to 24 hours. Imaged were captured every hour at 40x magnification. Percentage germination was calculated by enumerating total germinated spores in at least three fields of view. Hyphae were measured from the images at each hour, using FIJI. (A) Percentage germination at 11 hours of growth for the cell detachment TFKOs. (B) Hyphal extension rate per hour of cell detachment mutants. (C) Percentage germination at 11 hours of growth for the cell lysis TFKOs. (D) Hyphal extension rate per hour of the cell lysis mutants. WT=A1160p+. Error bars show ±SD. Data was analysed by one-way ANOVA with uncorrected Fisher’s LSD multiple comparisons test relative to the parental strain (WT-A1160p+). *P<0.1, ***P<0.001, ****P<0.0001.
The parental strain achieved hyphal lengths of ~ 150 µm by 16 hours of growth in supplemented RPMI, representing a mean rate of hyphal extension of 20 μm/hour of growth (Figure 3). Hyphal extension rates of TFKOs defective in epithelial cell detachment were reduced with 8 of the 10 TFKOs exhibiting hyphal extension rates of 15-20 μm/hour or less (Figures 3 and S2). Consistent with previous reports of hyper-branching growth (Bertuzzi et al., 2014), the ΔpacC strain achieved less than 20 μm in hyphal length by 16 hours (Figure S2). In summary, analyses of germination and hyphal growth rates revealed that the spores of most mutants germinated as efficiently as those of the parental strain, but hyphal extension rates frequently differed from those of the parental isolate. Categorically, we find that the phenotype that associates most robustly with reductions in epithelial detachment is that of reduced hyphal extension rates.
Deficits in Epithelial Uptake and Adhesion Are Common Amongst A. fumigatus TFKOs Defective in Epithelial Detachment and Cell Lysis
Uptake of spores by epithelial cells is complex, starting with contact mediated recognition via host receptors, followed by a dynamic assembly of the actin cytoskeleton that induces the envelopment of A. fumigatus spores by the epithelial cell membrane (Bertuzzi et al., 2018). Further, epithelial cells were found to internalise the non-invasive ΔpacC mutants significantly lesser than the invasive parental isolate (Bertuzzi et al., 2014), in a manner dependent upon the Dectin-1 receptor that recognizes the β-glucan fungal cell surface polysaccharide (Bertuzzi et al., 2014). To assess the contribution of spore internalisation on epithelial cell damage, the uptake of TFKO spores was quantified by differential fluorescence imaging (Figure 4A) following incubation of FITC-stained spores with A549 cells from 30 minutes to 6 hours, followed by CFW staining of externally adherent spores (Figure S3). The proportion of infecting A1160p+ spores internalised by A549 cells after a 4-hour infection was 20% (Figures 4B, C). Of the 10 TFKOs causing reduced epithelial detachment, five (ΔpacC, ΔnsdD, ΔnsdC, ΔAFUB_031000-Δ1H8 and ΔAFUB_019830-Δ5 E12) showed half the spore uptake relative to the parental isolate. Of the 8 TFKOs defective for epithelial lysis, 6 mutants (ΔpacC, ΔhapX, ΔsltA, ΔatfD, ΔAFUB_015750-Δ1D11, ΔAFUB_078520-Δ3 E5) demonstrated significantly reduced rates of spore uptake relative to the parental strain. Of these, spores of the ΔpacC, ΔhapX, and ΔsltA mutants exhibited ≥ 50% reduction in epithelial uptake relative to the parental isolate.
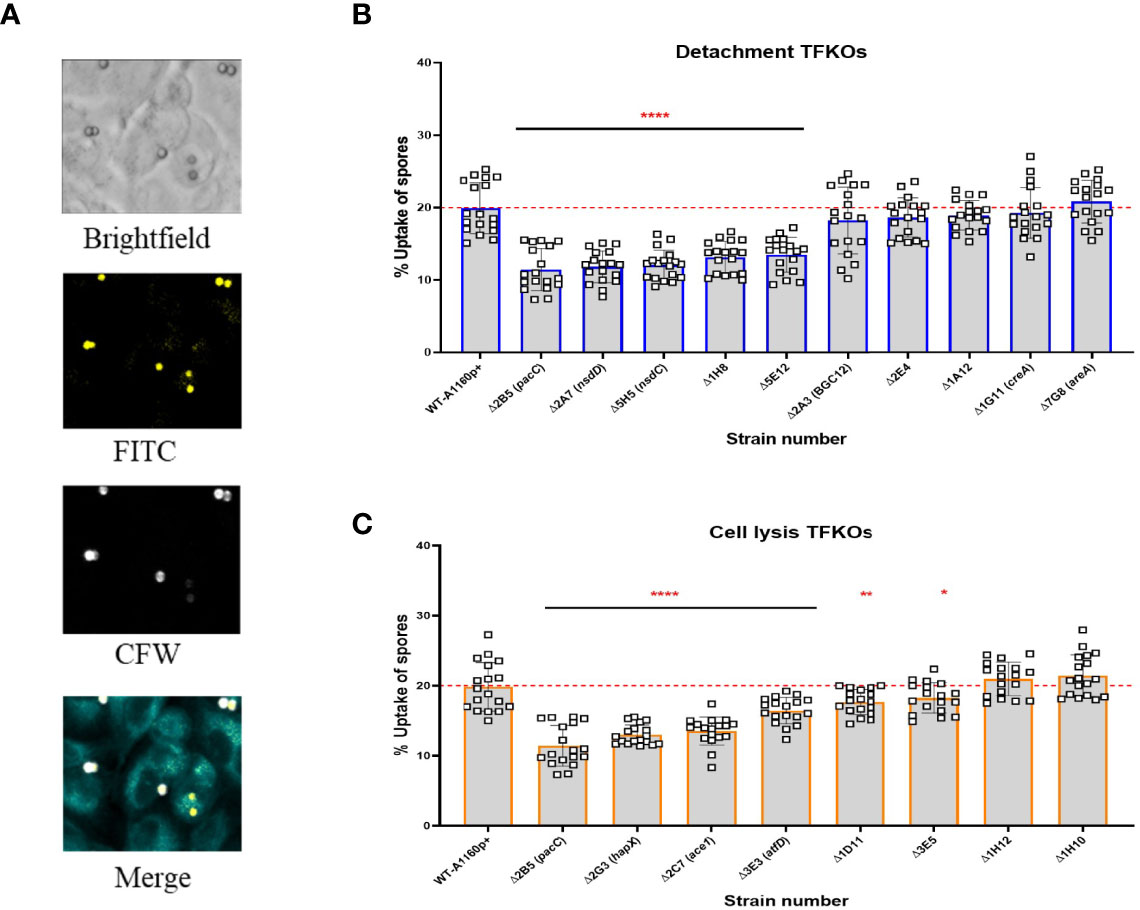
Figure 4 Uptake capacity of A549 cells to cell detachment and cell lysis A. fumigatus TFKO spores. FITC stained spores incubated with A549 cells for 4 hours to enable internalisation were washed with PBS to stain with calcofluor white to visualise the internal and external spores (A) Representative images of brightfield, FITC (all spores), calcofluor white (external adherent spores). Images were captured using the TCS-SP8 confocal microscope at 40x magnification (B) Percentage spore uptake for the cell detachment TFKOs. (C) Percentage spore uptake for the cell lysis TFKOs. WT=parental strain A1160p+. Error bars show ± SD. Data was analysed by one-way ANOVA with Fisher’s LSD multiple comparisons test. UI=Un-infected. *P<0.1, **P<0.01, ****P<0.0001. Data shown is technical replicates of two biological replicates.
Adhesion of A. fumigatus to host pneumocytes is thought to prevent easy removal of A. fumigatus from the mucosal surfaces and provide a close contact for manipulation and invasion of host cells (Sheppard, 2011; Brunke et al., 2016). In vitro observations report A. fumigatus morphotypes adhere to epithelial cells, from as early as 30 minutes post-infection (DeHart et al., 1997). The expression of a hyphal exopolysaccharide, galactosaminogalactan (GAG) has been demonstrated as critical for hyphal adherence to epithelial cells. Moreover, a null mutant of a transcriptional regulator for GAG synthesis, MedA, is hypovirulent in murine models of IA (Gravelat et al., 2010) implying an important role for adherence to host cells during infection of the mammalian lung. To address the relevance of reduced hyphal adherence in TFKOs causing reduced epithelial damage, germlings of the 17 TFKOs of interest were incubated with A549 cells for 30 min, after which the non-adhered germlings were removed by rinsing and those remaining were CFW stained for visualisation and enumeration of adherence by differential fluorescence microscopy (Figure 5A). To obviate the confounding effects of fitness deficits, TFKOs defective in germination and/or hyphal elongation were cultured for longer durations to achieve similar morphogenic states to the parental strain. Amongst the 10 TFKOs defective in causing epithelial detachment, 4 mutants (ΔpacC, ΔcreA, ΔareA, ΔAFUB_005510-Δ1A12) exhibited significantly reduced adherence of germlings to epithelial cells, compared to the parental strain, with ΔcreA and ΔpacC strains exhibiting a 50% reduction in adherence relative to the parental strain (Figure 5B). Similarly, 5 out of the 8 mutants (ΔpacC, ΔAFUB_031980-Δ1H10, ΔsltA, ΔAFUB_015750-Δ1D11, ΔAFUB_078520-Δ3 E5) mutants defective in cell lysis displayed significantly reduced adherence compared to the parental strain (Figure 5C).
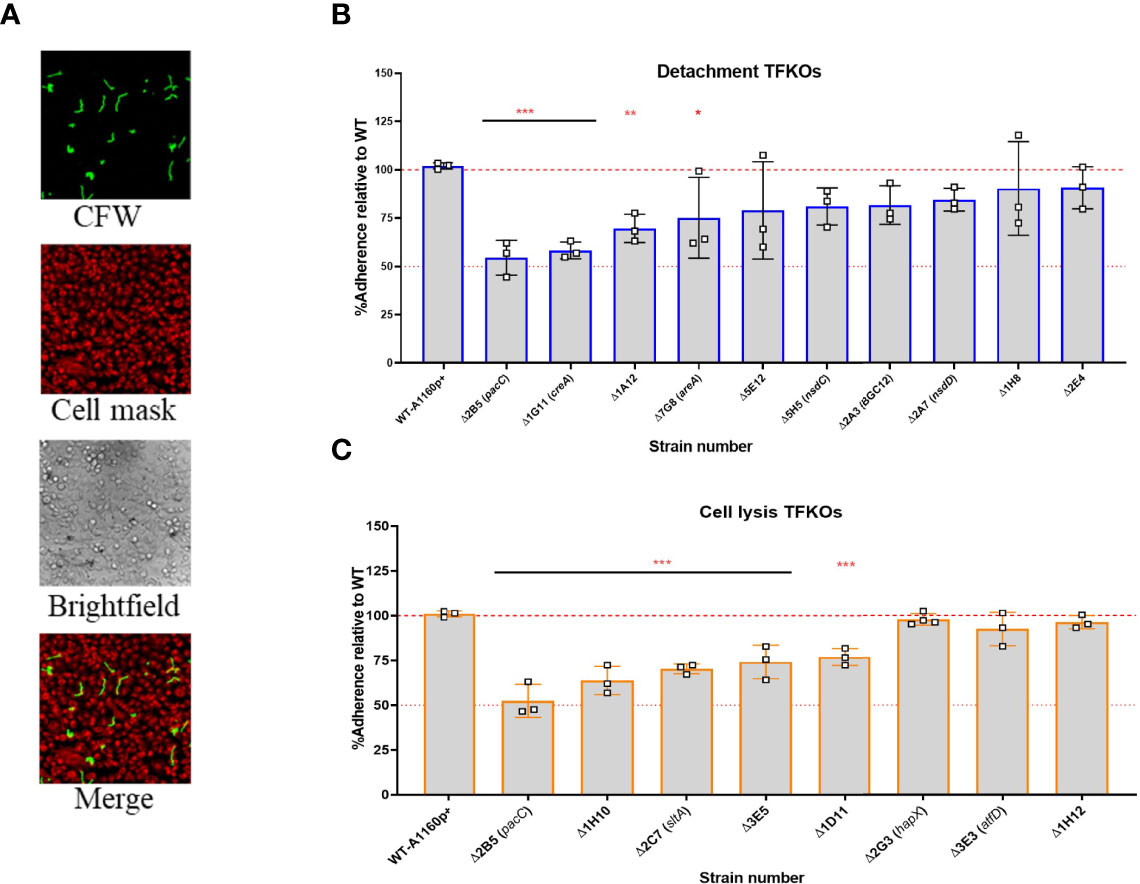
Figure 5 Adhesion capacity of the hyphal of A. fumigatus TFKOs to epithelial cells. Germlings of TFKOs incubated with A549 cells for 30 min to enable adhesion were rinsed with PBS and stained with calcofluor white to visualise only the adherent germlings (A) Representative images of calcofluor white stained germlings, cell mask stained A549 cells, brightfield and a merged image. Images captured with TCS-SP8 confocal microscope at 40x magnification (B) Percentage adherence of the cell detachment TFKOs (C) Percentage adherence of the cell lysis TFKOs. Error bars show ± SD. Data was analysed by one-way ANOVA with Fisher’s LSD multiple comparisons test. Data shown is technical reps of 3 biological reps. WT=A1160p+. *P<0.1, **P<0.01 ***P<0.0001.
Calcofluor White Sensitivity Associates Predominantly With A. fumigatus TFKOs Defective in Causation of Epithelial Damage
The cell wall of A. fumigatus is the outermost cellular structure that mediates host interactions including pathogen recognition and stimulation of the host immune response and pathogen adherence to host cells (Lee and Sheppard, 2016). Therefore, changes in cell wall structure and/or composition often affect pathogenicity. Previous studies have demonstrated that A. fumigatus spores and germlings are internalised by epithelial cells in a contact-, actin-, cell wall- and Dectin-1 dependent manner and ΔpacC mutants, which aberrantly remodel the cell wall during germinative growth, are less able than wild type counterparts to gain entry into epithelial cells (Bertuzzi et al., 2018). In fungal cells suffering cell wall aberrancies that involve chitin redistribution, sensitivity to the anionic dye Calcofluor white (CFW) that binds to chitin and interferes with the construction and stress tolerance of the cell wall, is a common phenotype (Roncero et al., 1988). To test the hypothesis that alterations in fungal cell wall contribute to differential damage in epithelial cells, the 17 TFKOs of interest were tested for sensitivity/resistance to CFW by growing serial dilutions (107,106,105,104) spores/ml of the mutants on solid AMM containing 200 µg/ml of CFW. Amongst the 10 mutants inducing reduced epithelial detachment (Figure 6), 9 TFKOs exhibited sensitivity to CFW relative to the parental strain. Furthermore, 6 of the 8 TFKOs isolates effecting reduced cytotoxicity to epithelial cells (Figure 6) exhibited sensitivity to CFW. The ΔsltA strain was resistant to CFW relative to the parental strain (Figure 6), as reported previously (Liu et al., 2021). Our findings demonstrate a robust association between cell wall aberrances and inability to cause epithelial damage.
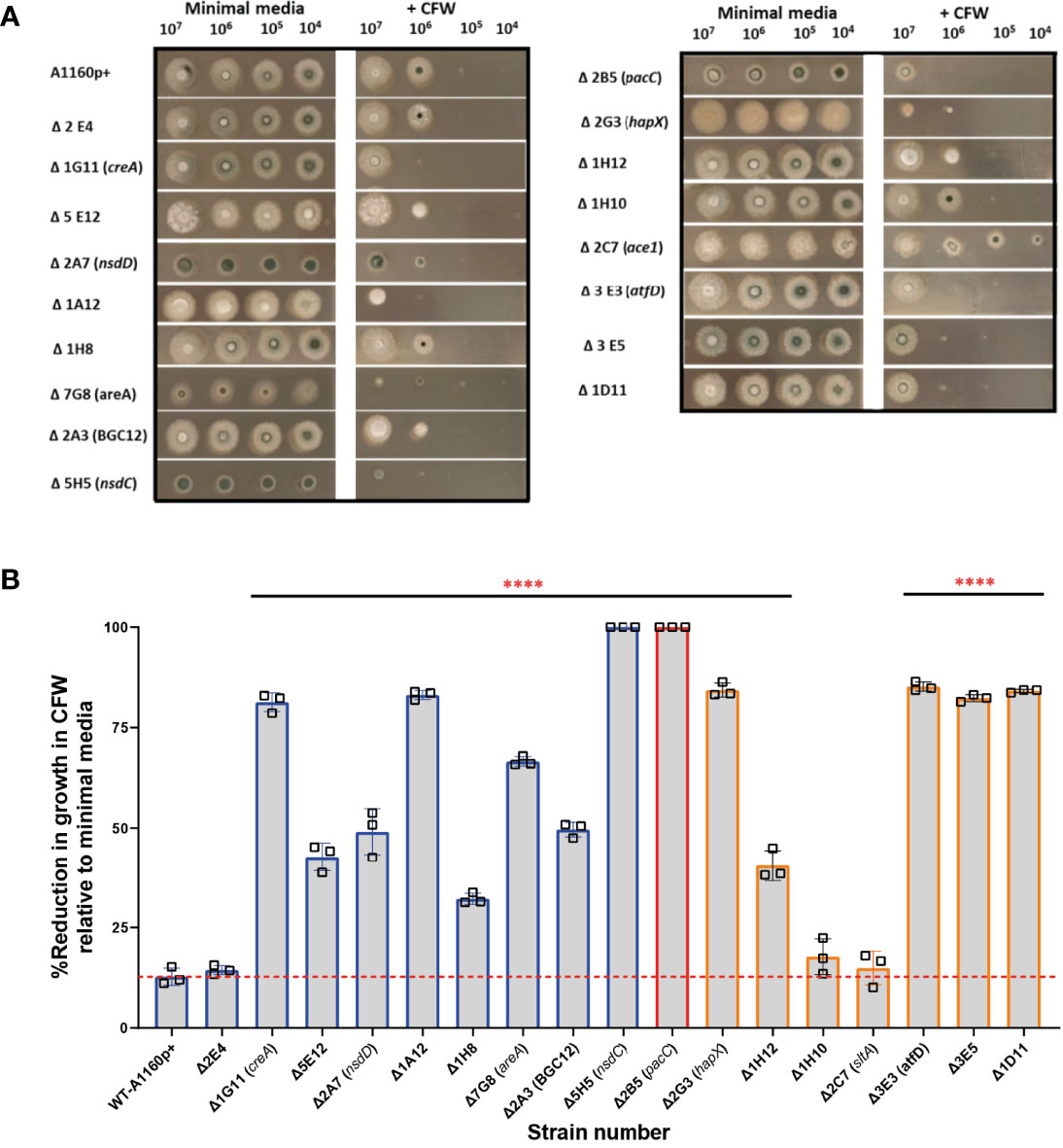
Figure 6 Sensitivity to calcofluor white in A.fumigatus TFKOs. Cell wall sensitivity was assessed in the A. fumigatus TFKOs by growing 106 TFKO spores on minimal media agar or minimal media agar containing CFW (200 mg/L) for 48 hours. (A) Images of the TFKO colonies grown in Aspergillus minimal media with and without CFW. 106 TFKO spores were inoculated on agar and images taken after 48 hours. (B) Percentage reduction in growth of TFKO in CFW calculated relative to growth in minimal media. Colony diameter was measured in three different regions of the colonies and percentage reduction in growth was analysed relative to the parental strain Data was analysed by one-way ANOVA with Fisher’s LSD multiple comparisons test. WT=A1160p+. ****P<0.0001.
Reduced Cytotoxicity of the A. fumigatus Culture Filtrates Associates Exclusively With A. fumigatus TFKOs Defective in Late Causation of Epithelial Damage
A. fumigatus secretes a wide range of proteases (serine proteases, metalloproteinases and aspartic proteases), and other enzymes, proteins and toxins during growth in the host environment (Latgé and Chamilos, 2020). Gliotoxin, one of the most widely studied secreted toxins of A. fumigatus is associated with induction of apoptotic cell death in several mammalian cell types (Kwon-Chung and Sugui, 2009; Raffa and Keller, 2019). Additionally, culture filtrates from mutants lacking the PrtT regulator that governs expression of six secreted proteases in A. fumigatus is associated with a reduced epithelial damage capacity in vitro (Sugui et al., 2007; Shemesh E. et al.,2017). Further, the host-adapting transcriptome of the non-invasive ΔpacC isolate revealed dysregulation of several genes encoding putatively secreted proteins suggesting profound importance of A. fumigatus secretions during epithelial invasion (Bertuzzi et al., 2014). To measure cytotoxicity of TFKO culture filtrates, epithelial cell lysis was measured after challenging A549 cells with the culture filtrates of the TFKO mutants. To align with previous studies that had identified the expression of cytotoxic factors at >16 hours of fungal culture (Bertuzzi et al., 2014), hyphae were grown to maturity (48 hours) before culture filtrates were harvested and co-incubated with A549 cells for 24 hours. Interestingly, with the exception of ΔpacC, none of the TFKOs defective in epithelial detachment exhibited significantly reduced epithelial damage relative to the parental strain (Figure 7A). However, in stark contrast, the culture filtrates of 7 out of 8 of the TFKOs defective in late causation of epithelial damage exhibited reduced capacity to cause damage relative to the parental strain (Figure 7B). These results corroborated soluble factors to be major contributors to epithelial damage during the late phase of epithelial damage infection.
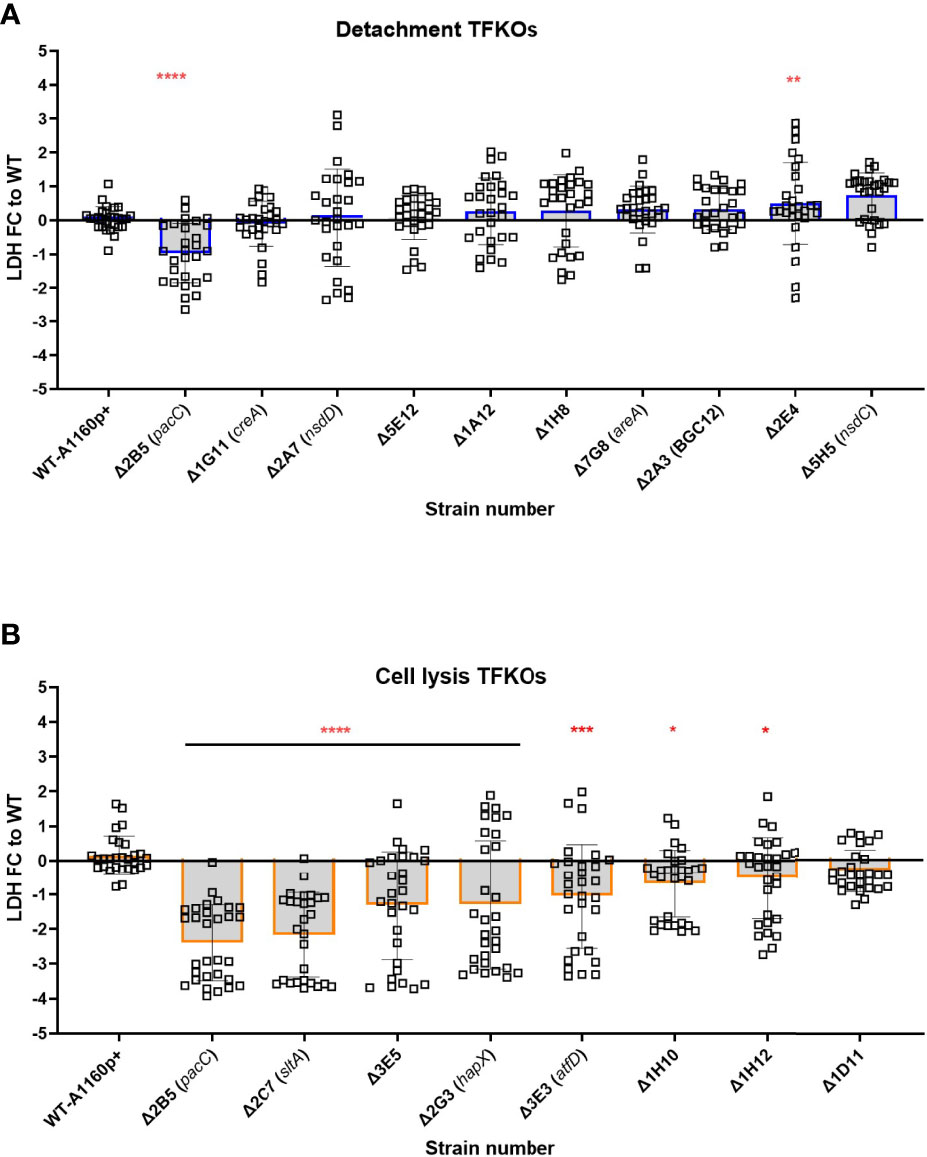
Figure 7 Culture filtrate damage caused by the A.fumigatus TFKOs. Damage caused by soluble factors of TFKOs to epithelial cells was assessed by measuring LDH release after 24-hour challenge of A549 cells with 48-hour culture filtrate of the TFKOs. (A) Fold change LDH of the cell detachment TFKOs (B) Fold change LDH of cell lysis TFKOs. WT=parental strain A1160p+. Error bars show ± SD. Data was analysed by one-way ANOVA with Fisher’s LSD multiple comparisons test. Data shown is 30 technical reps derived from 3 biological reps. WT=A1160p+. *P<0.1, **P<0.01 ***P<0.001, ****P<0.0001.
Discussion
Aspergillus fumigatus is a ubiquitous filamentous fungus and a potential cause of life-threatening lung disease in the immunocompromised. Interactions between the inhaled A. fumigatus spores and host lung pneumocytes are dynamic, complex, and poorly understood. Several recent reports identify important roles for lung epithelial cells during A. fumigatus infection, the nature of which can result in either expelling of the fungus or destruction of the lung (Croft et al., 2016; Bertuzzi et al., 2018). Previous invitro studies have identified a biphasic basis of fungus-induced lung epithelial damage, commencing with contact-dependent perturbation causing epithelial detachment, followed by hyphal mediated damage causing epithelial cell lysis, that in large part derives from the fungal secretome (Bertuzzi et al., 2014; Okaa et al., 2021). Despite the central importance of transcriptional regulation in driving expression of secreted fungal gene products, and the translational potential of inhibiting this process, very few transcriptional regulators have been identified as governing epithelial invasion.
To address this knowledge gap, a genome-scale census of A. fumigatus transcription factors was conducted (Figures 1, 2) by challenging a laboratory cultured pneumocyte cell line with 479 null mutants of A. fumigatus transcription factors (Furukawa et al., 2020). This identified 17 regulators of epithelial damage, 8 of which have not been previously characterised (Table 1). As with any high throughput screening approach, the inclusion of false positive or false negative data cannot be ruled out. To limit this, maximum stringency was adopted with reporting TFs with defects. The entire dataset is reported to aid the work of others seeking further potentially relevant TFKOs. A critical discovery is that distinct cohorts of A. fumigatus genetic regulators drive early acting epithelial detachment and late-acting cytolytic modes of epithelial damage. Only one A. fumigatus transcription factor, the pH-responsive transcription factor PacC, was found to govern causation of both early- and late-occurring damage. This finding is consistent with the idea that distinct genes could be employed at each phase of infection as the fungus establishes its niche in the host environment. A similar scenario has been reported for host kidney colonisation by C. albicans at 12, 24, and 48 hr post infection (Xu et al., 2015).
Several fungal attributes have been acknowledged to contribute towards the pathogenesis of A. fumigatus during establishment of infection (Dagenais and Keller, 2009; Latgé and Chamilos, 2020). This study has probed the regulatory landscape during the temporal pathogenic profile of A. fumigatus mediated damage of the alveolar epithelium (Schematic in Figure 8A). Interestingly, almost every mutant deficient in causing epithelial damage exhibited a unique pattern of phenotypes (Summarised in Figures 8B, C). Nonetheless, phenotypic traits were identified that correlate with early- and/or late- causation of damage. For example, amongst 10 mutants exhibiting reduced epithelial detachment, all but one isolate exhibited reduced rates of hyphal extension (Figure 3). The hyphal forms of the fungus have been implied as critical for invasion in other invasive fungi such as C.albicans (Desai, 2018). An obvious explanation, negated by our data, is that reduced rates of hyphal extension reduce the capacity of extending hyphae to secrete soluble mediators of epithelial damage. If this were true, we might expect to have seen significant numbers of TFKOs defective in both early- and late-causation of epithelial damage rather than exclusivity of transcriptional regulators to governance of particular traits (Figure 7). An alternative explanation is that slower hyphal growth rates place a limitation upon the number of host cells that the nascent hyphae can come into direct contact with. The latter argument aligns well with previous observations that early-acting damage occurs in a contact-dependent manner (Bertuzzi et al., 2014), as well as with the phenotypic analyses conducted in this study that revealed deficits in rate of uptake into and/or adhesion to epithelial cells (Figures 4, 5). Aberrancies of cell wall organisation, as indicated by CFW sensitivity (Figure 6) might also contribute to reduced causation of epithelial detachment.
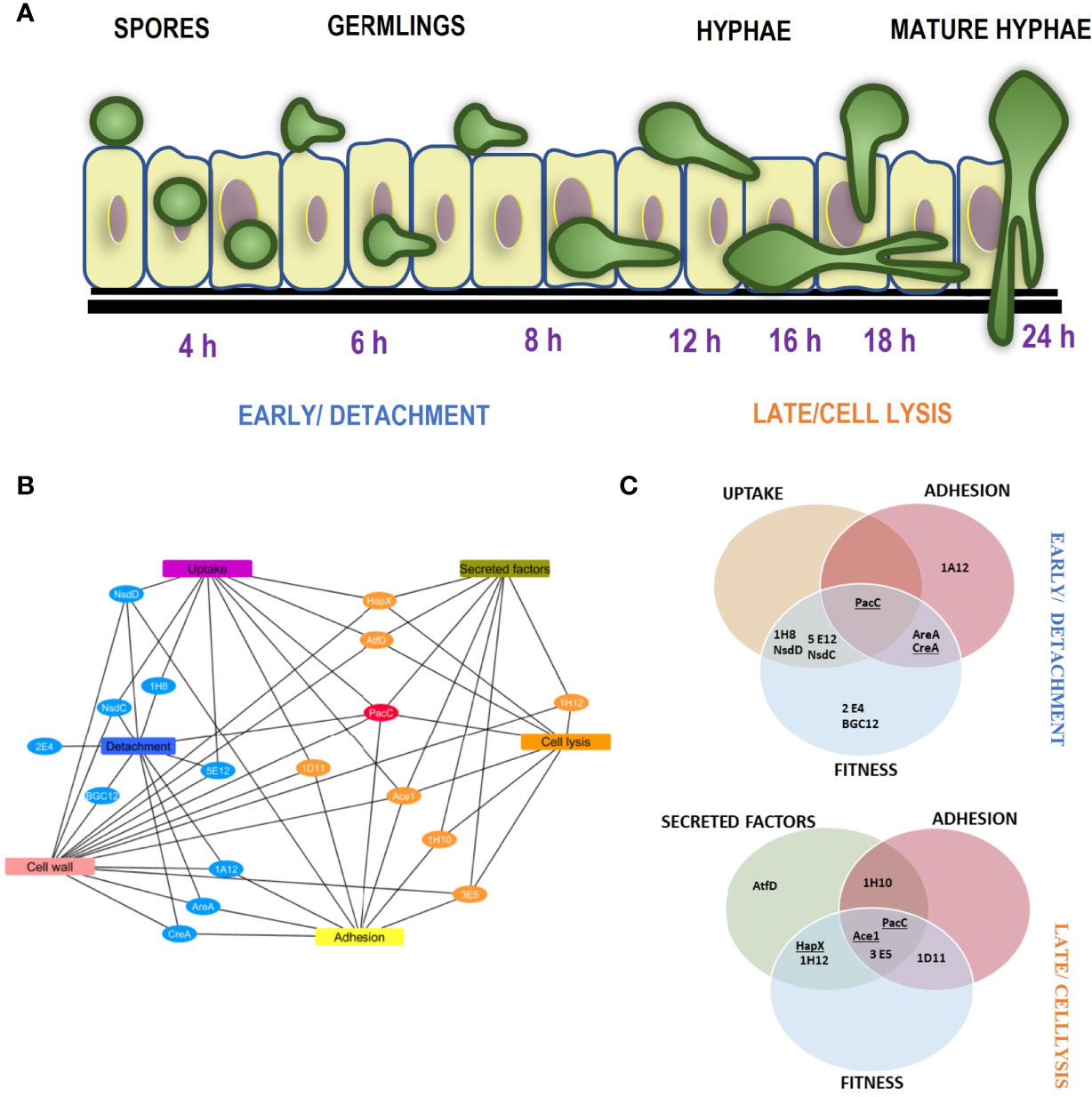
Figure 8 Temporal mechanistic profile of A. fumigatus mediated damage of alveolar epithelium regulated by the A. fumigatus transcription factors (A) Schematic showing the temporal mechanistic profile of A. fumigatus mediated damage of alveolar epithelium. A. fumigatus early interaction with the lung epithelium begins with spore uptake by the epithelial cells between 2-6 hours post infection. The spores germinate extracellular or intracellularly progressing infection between 8 and 12 hours. Adhesion of the germlings to the epithelium most likely enables close contact for invasion. Cell wall mediated damage occurs at the early stage directed by the detachment regulators. Following hyphal extension post 16 hours, the cell lysis regulators facilitate invasion via secretion of proteases and toxins, the cumulative effect of these epithelial interactions moderated by the A. fumigatus regulators leads to epithelial invasion. (B) and (C) Unique phenotypic and mechanistic profile of the A. fumigatus regulators, (B) shows a cytoscape network of the unique mechanistic attributes of the cell detachment and cell lysis regulators. (C) shows the attributes of the cell detachment and cell lysis mutants for uptake, adhesion, fitness and secreted factors (culture filtrates). The TFs underlined exhibited altered germination rates.
The characterised functions of previously studied transcription factors yield further clues to the mechanisms underlying the epithelial detachment phenomenon. Among the 8 TFs required for efficient pneumocyte detachment 4 TFs (PacC (AFUB_037210), AreA (AFUB_096370) NsdD and NsdC (AFUB_035330 and AFUB_089440) have been implicated in co-ordination of sporulation and secondary metabolism (Calvo et al., 2002; Bayram and Braus, 2012; de Castro et al., 2021). Consistent with such functionality, the colonial phenotypes of all four mutants exhibit compact morphology (Figures 3, 6). It is therefore feasible that the secondary metabolite composition of the spores of these mutants differs sufficiently from that of the parental isolate to cause deficits in epithelial detachment, either via aberrancies in one or several of contact-mediated toxicity, epithelial uptake of and phagolysomal fusion, the latter of which has recently been shown to determine efficiency of intracellular fungal killing (Ben-Ghazzi et al., 2021).
Remarkably, TFKOs that are deficient in causing epithelial cell lysis universally exhibit culture filtrates that are less cytolytic than the parental progenitor (Figure 7). In 3 out of 8 instances PacC, HapX and SltA (Bertuzzi et al., 2014; Gsaller et al., 2014; Liu et al., 2021), it might be argued that this occurs as a direct consequence of radically reduced hyphal growth. However, PacC mature hyphae (generated via extended incubation times) produce a cytolytically inert secretome, presumably due to loss of gliotoxin biosynthesis and multiple secreted fungal proteases (Bertuzzi et al., 2014). Of the remaining 5 TFKOs deficient in causing cytolytic damage to epithelial cells, the functionality of the TFs remains uncharacterised. It will be interesting to identify commonalities/distinctions between the secretomes of these mutants to pinpoint the causal agents of cytolytic host cell death. In summary, our finding supports the idea that virulence is multifactorial, hence there could be a combination of the fungal attributes that contributed to reduced epithelial invasion. For example, in vivo transcriptomic studies have shown PacC to regulate secreted and cell wall genes and deficit in causing uptake but not spore epithelial adhesion (Bertuzzi et al., 2014) and SltA regulates the expression of multiple secondary metabolite gene clusters and mycotoxin as well being sensitive to cell wall perturbing agents (Liu et al., 2021)
Surprisingly A. fumigatus transcription factor PrtT reported to regulate protease production (Sharon et al., 2009b) did not show significant differences in epithelial cytotoxicity compared to the parental strain. (Okaa et al., 2021). Further, the GliZ TF that regulates gliotoxin production (Bok et al., 2006; Schoberle et al., 2014) did not contribute to epithelial damage during our high throughput screening. Our study also did not identify TFKO mutants previously reported to have deficient adhesion and damage to epithelial cells namely DvrA, MedA and SomA (Ejzykowicz et al., 2010; Gravelat et al., 2010; Lin et al., 2015) to cause highly significant differences in epithelial damage. One plausible explanation is the disparate genetic backgrounds of the constructed mutants that could have an effect on their capacity to cause damage.
It remains to be seen, in the context of mammalian disease, whether the effects of early- and late-acting damage processes have mutually exclusive, additive or synergistic effects upon pathogenicity. Of note, the only TF that orchestrates both modes of damage, PacC, has been demonstrated to be a key regulator of epithelial invasion that is critical for mammalian pathogenicity (Bertuzzi et al., 2014). Given the broad regulatory activity of TFs, it is likely that the phenotypes associated with null mutants will be more pleiotropic in nature than we have discovered in this study, and it will therefore be necessary to identify the individual effectors of epithelial damage that function under their regulatory control. Nonetheless, the distinctive spatial and temporal contributions of TFs to epithelial damage indicate that early- and late-acting damage might be differentially significant to diseases caused predominantly by spore or hyphal forms of A. fumigatus. The use of immortalized cell line poses several risks and limitations, for example the pathogen is exposed to only one cell type that might exhibit altered responses relative to primary cell counterparts and/or the intact tissue environment where in multiple other cell types and secretions would be involved. It is important to note that in this study, we are inherently studying the interaction between the fungus and the epithelial cells, we are not considering whether host inflammatory responses dampen or worsen the damage elicited by A. fumigatus infection. Acknowledging that pathogenicity is a dual phenomenon moderated by both host and pathogen factors, it could be likely that the fungus must elicit host damage which serves as a signal to amplify residual host inflammatory responses, in turn driving pathogenicity.
In conclusion, the discovery of novel regulators of epithelial damage and characrerisation of their phenotypic and mechanistic profiles moderating damage, has allowed a birds-eye scan in understanding the highly dynamic host-pathogen turmoil. This is necessary to fully understand the pathogenesis of IPA and also facilitates new gene targets for therapeutics, both of which would help us overcome the morbidity and mortality caused by the human killer fungus A. fumigatus.
Data Availability Statement
The original contributions presented in the study are included in the article/Supplementary Material. Further inquiries can be directed to the corresponding author.
Author Contributions
Conceived and designed the experiments: SR, EB, and MR. Performed the experiments: SR, and ZC. Analyzed the data: SR, EB, PP, NVR, and MR. Prepared cell lines: RFG. Assisted with microscopy and technical issues: DDT. Wrote the paper: SR and EB. Critically revised the manuscript: SR, EB, NVR, MB, DDT, and PP. All authors contributed to the article and approved the submitted version.
Funding
This research work from this paper was funded from project grants MR/M02010X/1 to EB, MB, and MR; and MR/S001824/1 and MR/L000822/1 to EB and also supported by the Wellcome Trust grant number 219551/Z/19/Z to EB and MB and 208396/Z/17/Z to MB.
Conflict of Interest
MB is the director and shareholder of PIQ Laboratories Ltd.
The remaining authors declare that the research was conducted in the absence of any commercial or financial relationships that could be construed as a potential conflict of interest.
Publisher’s Note
All claims expressed in this article are solely those of the authors and do not necessarily represent those of their affiliated organizations, or those of the publisher, the editors and the reviewers. Any product that may be evaluated in this article, or claim that may be made by its manufacturer, is not guaranteed or endorsed by the publisher.
Acknowledgments
We would like to thank Lea Gregson for her technical assistance with preparation of the A549 cell line.
Supplementary Material
The Supplementary Material for this article can be found online at: https://www.frontiersin.org/articles/10.3389/fcimb.2022.907519/full#supplementary-material
Supplementary Table 1 | Output of the detachment (sheet 1) and cell lysis (sheet 2) screening for the TFKO mutants used for the generation of the volcano plot and the analysis.
Supplementary Table 2 | Output of the Boolean analysis performed for the LDH epithelial cell lysis screening for the TFKO mutants.
Supplementary Figure 1 | Optimisation of epithelial damage assays for high throughput screening. Performance of detachment assay in a low throughput (A, B) and high throughput plate format (C, D) (A) Number of adherent cells per image enumerated in a 24 well plate. The total number of adherent cells per field of view were enumerated using a FIJI macro counting DAPI stained cells after A. fumigatus A1160+ and ΔpacC strains infection. Data was analysed by multiple t-tests. ****P<0.0001. (B) Representative images A1160p+ or ΔpacC after 16-hour infection of A549 monolayer in a 24 well plate. Images captured manually at 20 x magnification using NIKON TE-2000E (C) Number of adherent cells per image enumerated in a 96 well plate. The total number of adherent cells per image were enumerated using a FIJI macro counting DAPI stained cells after A. fumigatus A1160+ and ΔpacC strains infection. Data was analysed by multiple t-tests. ****P<0.0001. (D) Representative images A1160p+ and ΔpacC strains after 16-hour infection of A549 monolayer in a 96 well plate. Images captured automatedly at 40x magnification using confocal microscope. Suitability of LDH assay for high-throughput cell cytotoxicity screening (E, F) (E) A. fumigatus does not produce measurable LDH enzyme at 24 hours. Absolute LDH released by 500,000 spores of A1160p+, ΔpacC and un-infected in a 24 well plate after 24 hours challenge with and without A549 cells. (F) Performance of LDH assay in 96 versus 384 well format. LDH assay performed in a 96 well and a 384 well plate showing LDH fold change. Error bars show ± SD. Data was analysed by one-way ANOVA with Fisher’s LSD multiple comparisons test. UI=Un-infected. ***P<0.001, ****P<0.0001.
Supplementary Figure 2 | Hyphal extension rate and germination potential of the A. fumigatus TFKOS measured by assessing growth using time-lapse confocal microscopy. (A) Percentage germination of the cell detachment mutants. (B) Hyphal extension rate of cell detachment mutants germination of the eRegs (cell detachment) mutants. (C) Percentage germination of the cell detachment mutants. (D) Hyphal extension rate of the cell lysis mutants. WT=A1160p+. Data was analysed by one-way ANOVA with Fisher’s LSD multiple comparisons test. Error bars show ± SD. *P<0.1, **P<0.01 ***P<0.001, ****P<0.0001.
Supplementary Figure 3 | Uptake capacity of A549 cells to the A. fumigatus parental strain (A1160p+) and ΔpacC strain over time. FITC stained spores incubated with A549 cells for 30min, 1 hour, 2 hours and 4 hours to enable A549 internalisation, after which the infected cells, were washed with PBS to stain the externally adherent spores with calcofluor white. Images were captured using the TCS-SP8 confocal microscope at 40x magnification Error bars show ± SD. Data was analysed by two-way ANOVA Sidak’s comparisons test of means. ***P<0.001, ****P<0.0001. Data shown is 3 technical reps with three biological replicates.
References
Baltussen, T. J. H., Zoll, J., Verweij, P. E., Melchers, W. J. G. (2019). Molecular Mechanisms of Conidial Germination in Aspergillus Spp. Microbiol. Mol. Biol. Rev. 84 (1), e00049–19. doi: 10.1128/mmbr.00049-19
Bayram, Ö., Braus, G. H. (2012). Coordination of Secondarymetabolism and Development in Fungi: The Velvet Familyof Regulatory Proteins. FEMS Microbiol. Rev. 36 (1), 1–24. doi: 10.1111/J.1574-6976.2011.00285.X
Beattie, S. R., Mark, K. M. K., Thammahong, A., Ries, L. N. A., Dhingra, S., Caffrey-Carr, A. K., et al. (2017). Filamentous Fungal Carbon Catabolite Repression Supports Metabolic Plasticity and Stress Responses Essential for Disease Progression. PLoS Pathog. 13 (4), e1006340. doi: 10.1371/journal.ppat.1006340
Ben-Ghazzi, N., Moreno-Velásquez, S., Seidel, C., Thomson, D., Denning, D. W., Read, N. D., et al. (2021). Characterisation of Aspergillus Fumigatus Endocytic Trafficking Within Airway Epithelial Cells Using High-Resolution Automated Quantitative Confocal Microscopy. J. fungi. (Basel Switzerland). 7 (6), 454. doi: 10.3390/JOF7060454
Bertuzzi, M., Schrettl, M., Alcazar-Fuoli, L., Cairns, T. C., Muñoz, A., Walker, L. A., et al. (2014). The pH-Responsive PacC Transcription Factor of Aspergillus Fumigatus Governs Epithelial Entry and Tissue Invasion During Pulmonary Aspergillosis. PLoS Pathog. 10 (10), e1004413. doi: 10.1371/journal.ppat.1004413
Bertuzzi, M., Hayes, G. E., Icheoku, U. J., van Rhijn, N., Denning, D. W., Osherov, N., et al. (2018). ‘Anti-Aspergillus Activities of the Respiratory Epithelium in Health and Disease’. J. Fungi. 4 (1), 8. doi: 10.3390/jof4010008
Bertuzzi, M., van Rhijn, N., Krappmann, S., Bowyer, P., Bromley, M. J., Bignell, E. M., et al. (2021). On the Lineage of Aspergillus Fumigatus Isolates in Common Laboratory Use. Med. Mycol. Oxford Acad. 59 (1), 7–13. doi: 10.1093/MMY/MYAA075
Bignell, E., Cairns, T. C., Throckmorton, K., Nierman, W. C., Keller, N. P. (2016). Secondary Metabolite Arsenal of an Opportunistic Pathogenic Fungus. Philos. Trans. R. Soc. London B 371 (1709), 20160023. http://rstb.royalsocietypublishing.org/content/371/1709/20160023.
Bok, J. W., Chung, D., Balajee, S. A., Marr, K. A., Andes, D., Nielsen, K. F., et al. (2006). GliZ, a Transcriptional Regulator of Gliotoxin Biosynthesis, Contributes to Aspergillus Fumigatus Virulence. Infect. Immun. 74 (12), 6761–6768. doi: 10.1128/IAI.00780-06
Bongomin, F., Gago, S., Oladele, R. O., Denning, D. W. (2017). Global and Multi-National Prevalence of Fungal Diseases—Estimate Precision. J. Fungi. 3 (4), 57. doi: 10.3390/jof3040057
Brown, G. D., Denning, D. W., Gow, N. A., Levitz, S. M., Netea, M. G., White, T. C. (2012). Hidden Killers: Human Fungal Infections. Sci. Trans. Med. 4 (165), 165rv13. doi: 10.1126/science.1222236
Brunke, S., Mogavero, S., Kasper, L., Hube, B. (2016). Virulence Factors in Fungal Pathogens of Man. Curr. Opin. Microbiol. 32, 89–95. doi: 10.1016/j.mib.2016.05.010
Bulawa, C. E., Miller, D. W., Henry, L. K., Becker, J. M. (1995). Attenuated Virulence of Chitin-Deficient Mutants of Candida Albicans’, Proceedings of the National Academy of Sciences of the United States of America. Proc. Natl. Acad. Sci. U.S.A. 92 (23), 10570–10574. doi: 10.1073/PNAS.92.23.10570
Bultman, K. M., Kowalski, C. H., Cramer, R. A. (2017). Aspergillus Fumigatus Virulence Through the Lens of Transcription Factors. Med. mycol. 55 (1), 24–38. doi: 10.1093/mmy/myw120
Shemesh, E., Hanf, B., Hagag, S., Attias, S., Shadkchan, Y., Fichtman, B., et al. (2017). Phenotypic and Proteomic Analysis of the Aspergillus Fumigatus PrtT,XprG and XprG/PrtT Protease-Deficient Mutants. Front Microbiol 8, 2490. doi: 10.3389/fmicb.2017.02490
Calvo, A. M., Wilson, R. A., Bok, J. W., Keller, N. P. (2002). Relationship Between Secondary Metabolism and Fungal Development’, Microbiology and Molecular Biology Reviews. Am. Soc. Microbiol. (ASM) 66 (3), 447. doi: 10.1128/MMBR.66.3.447-459.2002
Casalini, G., Giacomelli, A., Ridolfo, A., Gervasoni, C., Antinori, S. (2021). ‘Invasive Fungal Infections Complicating COVID-19: A Narrative Review’. J. Fungi. 2021 7 (11), 921. doi: 10.3390/JOF7110921
Croft, C. A., Culibrk, L., Moore, M. M., Tebbutt, S. J. (2016). Interactions of Aspergillus Fumigatus Conidia With Airway Epithelial Cells: A Critical Review. Front. Microbiol. 7. doi: 10.3389/fmicb.2016.00472
Dagenais, T. R. T., Keller, N. P. (2009). Pathogenesis of Aspergillus Fumigatus in Invasive Aspergillosis. Clin. Microbiol. Rev. 9 (7), 447–465. doi: 10.1128/CMR.00055-08
de Castro, P. A., Valero, C., Chiaratto, J., Colabardini, A. C., Pardeshi, L., Pereira Silva, L., et al. (2021). Novel Biological Functions of the NsdC Transcription Factor in Aspergillus Fumigatus. mBio. 12 (1), 1–25. doi: 10.1128/MBIO.03102-20
DeHart, D. J., Agwu, D. E., Julian, N. C., Washburn, R.G. (1997). Binding and Germination of Aspergillus Fumigatus Conidia on Cultured A549 Pneumocytes. J. Infect. Dis. 175 (1), 146–150. doi: 10.1093/infdis/175.1.146
Desai, J. V. (2018). Candida Albicans Hyphae: From Growth Initiation to Invasion. J. Fungi. 4 (1), 10. doi: 10.3390/JOF4010010
DeHart, D. J., Agwu, D. E., Julian, N. C., Washburn, R. G. (2018). Binding and Germination of Aspergillus Fumigatus Conidia on Cultured A549 Pneumocytes. Journal of Infectious Diseases 175 (1), 146–50.
Ejzykowicz, D. E., Solis, N. V., Gravelat, F. N., Chabot, J., Li, X., Sheppard, D. C., et al. (2010). Role of Aspergillus Fumigatus DvrA in Host Cell Interactions and Virulence. Eukaryotic Cell 9 (10), 1432–1440. doi: 10.1128/EC.00055-10
Escobar, N., Ordonez, S. R., Wösten, H. A., Haas, P. J., de Cock, H., Haagsman, H. P. (2016). Hide, Keep Quiet, and Keep Low: Properties That Make Aspergillus Fumigatus a Successful Lung Pathogen. Front. Microbiol. 7. doi: 10.3389/fmicb.2016.00438
Escobar, N., Valdes, I. D., Keizer, E. M., Ordonez, S. R., Ohm, R. A., Wösten, H. A. B., et al. (2018). Expression Profile Analysis Reveals That Aspergillus Fumigatus But Not Aspergillus Niger Makes Type II Epithelial Lung Cells Less Immunological Alert. BMC Genomics 19 (1), 534. doi: 10.1186/S12864-018-4895-3
Fraczek, M. G., Bromley, M., Buied, A., Moore, C. B., Rajendran, R., Richardson, R.-R., et al. (2013). The Cdr1b Efflux Transporter is Associated With Non-Cyp51a-Mediated Itraconazole Resistance in Aspergillus Fumigatus. J. Antimicrob. Chemother. 68 (7), 1486–1496. doi: 10.1093/JAC/DKT075
Furukawa, T., van Rhijn, N., Fraczek, M., Gsaller, F., Davies, E., Carr, P., et al. (2020). The Negative Cofactor 2 Complex Is a Key Regulator of Drug Resistance in Aspergillus Fumigatus. Nat. Commun. 11 (1), 1–16. doi: 10.1038/s41467-019-14191-1
Gravelat, F. N., Beauvais, A., Liu, H., Lee, M. J., Snarr, B. D., Chen, D., et al. (2013). Aspergillus Galactosaminogalactan Mediates Adherence to Host Constituents and Conceals Hyphal β-Glucan From the Immune System. PLoS Pathog. 9 (8), e1003575. doi: 10.1371/journal.ppat.1003575
Gravelat, F. N., Ejzykowicz, D. E., Chiang, L. Y., Chabot, J. C., Urb, M., Macdonald, K. D., et al. (2010). Aspergillus Fumigatus MedA Governs Adherence, Host Cell Interactions and Virulence. Cell. Microbiol. 12 (4), 473–488. doi: 10.1111/j.1462-5822.2009.01408.x
Gsaller, F., Hortschansky, P., Beattie, S. R., Klammer, V., Tuppatsch, K., Lechner, B. E., et al. (2014). The Janus Transcription Factor HapX Controls Fungal Adaptation to Both Iron Starvation and Iron Excess. EMBO J. 33 (19), 2261–2276. doi: 10.15252/embj.201489468
Hensel, M., Arst, H. N., Aufauvre-Brown, A., Holden, D. W. (1998). The Role of the Aspergillus Fumigatus A Gene in Invasive Pulmonary Aspergillosis. Mol. Gen. Genet. 258 (5), 553–557. doi: 10.1007/s004380050767
Hortschansky, P., Haas, H., Huber, E. M., Groll, M., Brakhage, A. A. (2017). The CCAAT-Binding Complex (CBC) in Aspergillus Species. Biochim. Biophys. Acta (BBA) - Gene Regul. Mechanisms. Elsevier 1860 (5), 560–570. doi: 10.1016/J.BBAGRM.2016.11.008
Jung, K.-W., Yang, D. H., Maeng, S., Lee, K. T., So, Y. S., Hong, J., et al. (2015). Systematic Functional Profiling of Transcription Factor Networks in Cryptococcus Neoformans. Nat. Commun. 6 (1), 6757. doi: 10.1038/ncomms7757
Kogan, T. V., Jadoun, J., Mittelman, L., Hirschberg, K., Osherov, N. (2004). Involvement of Secreted Aspergillus Fumigatus Proteases in Disruption of the Actin Fiber Cytoskeleton and Loss of Focal Adhesion Sites in Infected A549 Lung Pneumocytes. J. Infect. Dis. 189 (11), 1965–1973. doi: 10.1086/420850
Kosmidis, C., Denning, D. (2015). The Clinical Spectrum of Pulmonary Aspergillosis. Thorax 70 (3), 270–277. doi: 10.1136/thoraxjnl-2014-206291
Krappmann, S., Braus, G. H. (2005). Nitrogen Metabolism of Aspergillus and its Role in Pathogenicity. Med. Mycol. 43 (s1), 31–40. doi: 10.1080/13693780400024271
Kruschke, J. K. (2013). Bayesian Estimation Supersedes the T Test. J. Exp. Psychol. 142 (2), 573–603. doi: 10.1037/A0029146
Kwon-Chung, K. J., Sugui, J. A. (2009). What do We Know About the Role of Gliotoxin in the Pathobiology of Aspergillus Fumigatus? Med. Mycol. 47(Supplement I), S97–103. doi: 10.1080/13693780802056012
Kwon-Chung, K. J., Sugui, J. A. (2013). Aspergillus Fumigatus-What Makes the Species a Ubiquitous Human Fungal Pathogen? PLoS Pathog. 9 (12), 1–4. doi: 10.1371/journal.ppat.1003743
Latgé, J. P., Chamilos, G. (2020). Aspergillus Fumigatus and Aspergillosis in 2019. Clin. Microbiol. Rev. 33 (1), e00140–18. doi: 10.1128/CMR.00140-18
Lee, M. J., Sheppard, D. C. (2016). Recent Advances in the Understanding of the Aspergillus Fumigatus Cell Wall. J. Microbiol. (Seoul Korea) 54 (3), 232–242. doi: 10.1007/s12275-016-6045-4
Levdansky, E., Kashi, O., Sharon, H., Shadkchan, Y., Osherov, N. (2010). The Aspergillus Fumigatus cspA Gene Encoding a Repeat-Rich Cell Wall Protein Is Important for Normal Conidial Cell Wall Architecture and Interaction With Host Cells. Eukaryotic Cell 9 (9), 1403–1415. doi: 10.1128/EC.00126-10
Lin, C.-J., Sasse, C., Gerke, J., Valerius, O., Irmer, H., Frauendorf, H., et al. (2015). Transcription Factor SomA Is Required for Adhesion, Development and Virulence of the Human Pathogen Aspergillus Fumigatus. PLoS Pathog. 11 (11), e1005205. doi: 10.1371/journal.ppat.1005205
Liu, H., Lee, M. J, Solis, N. V., Phan, Q. T., Swidergall, M., Ralph, B., et al. (2016). Aspergillus Fumigatus CalA Binds to Integrin α5β1 and Mediates Host Cell Invasion. Nat. Microbiol. 2, 16211. doi: 10.1038/nmicrobiol.2016.211
Liu, H., Xu, W., Bruno, V. M., Phan, Q. T., Solis, N. V., Woolford, C. A., et al. (2021). Determining Aspergillus Fumigatus Transcription Factor Expression and Function During Invasion of the Mammalian Lung. PLoS pathog. 17 (3). doi: 10.1371/JOURNAL.PPAT.1009235
Liu, H., Lee, M. J., Solis, N. V., Phan, Q. T., Swidergall, M., Ralph, B., et al. (2016). Aspergillus Fumigatus CalA Binds to Integrin α5β1 and Mediates Host Cell Invasion. Nat Microbiol. 2. doi: 10.1038/nmicrobiol.2016.211
Liu, Z., Raj, S., van Rhijn, N., Fraczek, M., Michel, J. P., Sismeiro, O., et al. (2021). Functional Genomic and Biochemical Analysis Reveals Pleiotropic Effect of Congo Red on Aspergillus Fumigatus. mBio 12 (3), e00863–21. doi: 10.1128/MBIO.00863-21
Namvar, S., Warn, P., Farnell, E., Bromley, M., Fraczek, M., Bowyer, P., et al. (2015). Aspergillus Fumigatus Proteases, Asp F 5 and Asp F 13, are Essential for Airway Inflammation and Remodelling in a Murine Inhalation Model. Clin. Exp. Allergy 45 (5), 982–993. doi: 10.1111/cea.12426
Nobile, C. J., Fox, E. P., Nett, J. E., Sorrells, T. R., Mitrovich, Q. M., Hernday, A. D., et al (2012). A Recently Evolved Transcriptional Network Controls Biofilm Development in Candida Albicans. Cell 148 (1), 126–138. doi: 10.1016/j.cell.2011.10.048
Okaa, U. J., Bertuzzi, M., Fortune-Grant, R., Thomson, D. D., Moyes, D. L., Naglik, J. R, et al. (2021). Aspergillus Fumigatus Drives Tissue Damage via Iterative Assaults Upon Mucosal Integrity and Immune Homeostasis. bioRxiv. doi: 10.1101/2021.11.09.468003
Pontecorvo, G., Roper, JA, Chemmons, KD., Macdonald, AW, Bufton, WJ. (1953). The Genetics of Aspergillus Nidulans. Adv. Genet. 5 (C), 141–238. doi: 10.1016/S0065-2660(08)60408-3
Raffa, N., Keller, N. P. (2019). A Call to Arms: Mustering Secondary Metabolites for Success and Survival of an Opportunistic Pathogen’. PLoS Pathog. 15 (4), e1007606. doi: 10.1371/JOURNAL.PPAT.1007606
Rahman, S., Thomson, D. D., Bertuzzi, M. (2021). Automated Quantitative Analysis of Airway Epithelial Cell Detachment Upon Fungal Challenge. Methods Mol. Biol. (Clifton N.J.) 2260, 225–239. doi: 10.1007/978-1-0716-1182-1_16
Roncero, C., Valdivieso, M. H., Ribas, J. C., Durán, Á. (1988). Isolation and Characterization of Saccharomyces Cerevisiae Mutants Resistant to Calcofluor White. J. Bacteriol. 170 (4), 1950–1954. doi: 10.1128/JB.170.4.1950-1954.1988
Scharf, D. H., Heinekamp, T., Remme, N., Hortschansky, P., Brakhage, A. A., Hertweck, C. (2012). Biosynthesis and Function of Gliotoxin in Aspergillus Fumigatus. Appl. Microbiol. Biotechnol. 93 (2), 467–472. doi: 10.1007/s00253-011-3689-1
Schauwvlieghe, A. F. A. D., Rijnders, B. J. A., Philips, N., Verwijs, R., Vanderbeke, L., Van Tienen, C., et al. (2018). Invasive Aspergillosis in Patients Admitted to the Intensive Care Unit With Severe Influenza: A Retrospective Cohort Study. Lancet Respir. Med. 6 (10), 782–792. doi: 10.1016/S2213-2600(18)30274-1
Schindelin, J., Arganda-Carreras, I., Frise, E., Kaynig, V., Longair, M., Pietzsch, T., et al. (2012). Fiji: An Open-Source Platform for Biological-Image Analysis. Nat. Methods 9 (7), 676–682. doi: 10.1038/nmeth.2019
Schoberle, T. J., Nguyen-Coleman, C. K., Herold, J., Yang, A., Weirauch, M., Hughes, T. R, et al. (2014). ‘A Novel C2H2 Transcription Factor That Regulates gliA Expression Interdependently With GliZ in Aspergillus Fumigatus’. PLoS Genet. 10 (5), e1004336. doi: 10.1371/journal.pgen.1004336
Sharon, H., Hagag, S., Osherov, N. (2009a). Transcription Factor PrtT Controls Expression of Multiple Secreted Proteases in the Human Pathogenic Mold Aspergillus Fumigatus. Infect. Immun. 77 (9), 4051–4060. doi: 10.1128/IAI.00426-09
Sharon, H., Hagag, S., Osherov, N. (2009b). Transcription Factor PrtT Controls Expression of Multiple Secreted Proteases in the Human Pathogenic Mold Aspergillus Fumigatus. Infect. Immun. 77 (9), 4051–4060. doi: 10.1128/IAI.00426-09
Shemesh, E., Hanf, B., Hagag, S., Attias, S., Shadkchan, Y., Fichtman, B., et al (2017). Phenotypic and Proteomic Analysis of the Aspergillus fumigatus ΔPrtT, ΔXprG and ΔXprG/ΔPrtT Protease-Deficient Mutants. Front. Microbiol. 8, 2490. doi: 10.3389/fmicb.2017.02490
Sheppard, D. C. (2011). Molecular Mechanism of Aspergillus Fumigatus Adherence to Host Constituents. Curr. Opin. Microbiol. 14 (4), 375–379. doi: 10.1016/j.mib.2011.07.006
Silva, L. P., Horta, M. A. C., Goldman, G. H. (2021). Genetic Interactions Between Aspergillus Fumigatus Basic Leucine Zipper (bZIP) Transcription Factors AtfA, AtfB, AtfC, and Atfd. Front. Fungal Biol. 0. doi: 10.3389/FFUNB.2021.632048
Sugui, J. A., Pardo, J., Chang, Y. C., Zarember, K. A., Nardone, G., Galvez, E. M., et al. (2007). Gliotoxin is a Virulence Factor of Aspergillus Fumigatus: GliP Deletion Attenuates Virulence in Mice Immunosuppressed With Hydrocortisone. Eukaryotic Cell 6 (9), 1562–1569. doi: 10.1128/EC.00141-07
Valiante, V., Macheleidt, J., Föge, M., Brakhage, A. A. (2015). The Aspergillus Fumigatus Cell Wall Integrity Signaling Pathway: Drug Target, Compensatory Pathways, and Virulence. Front. Microbiol. 6. doi: 10.3389/fmicb.2015.00325
van Rhijn, N., Coleman, J., Collier, L., Moore, C., Richardson, M. D., Bright-Thomas, R. J., et al. (2021). Meteorological Factors Influence the Presence of Fungi in the Air; A 14-Month Surveillance Study at an Adult Cystic Fibrosis Center. Front. Cell. Infect. Microbiol. 11. doi: 10.3389/FCIMB.2021.759944/BIBTEX
Voltersen, V., Blango, M. G., Herrmann, S., Schmidt, F., Heinekamp, T., Strassburger, M., et al. (2018). Crossm Proteome Analysis Reveals the Conidial Surface Protein CcpA Essential for Virulence of the Pathogenic Fungus Aspergillus Fumigatus. mBio 9(5), 1–18. doi: 10.1128/mBio.01557-18
Wasylnka, J. A., Moore, M. M. (2003). Aspergillus Fumigatus Conidia Survive and Germinate in Acidic Organelles of A549 Epithelial Cells. J. Cell Sci. 116 (Pt 8), 1579–1587. doi: 10.1242/jcs.00329
Wu, M. Y., Mead, M. E., Lee, M., Ostrem Loss, E. M., Kim, S., Rokas, A., et al. (2018). Systematic Dissection of the Evolutionarily Conserved WetA Developmental Regulator Across a Genus of Filamentous Fungi. mBio. 9 (4). doi: 10.1128/MBIO.01130-18/ASSET/86C3E27C-5ADC-40B7-A7F7-5750E266D9FA/ASSETS/GRAPHIC/MBO0041840260008.JPEG
Xu, W., Solis, N. V., Ehrlich, R. L., Woolford, C. A., Filler, S. G., Mitchell, A. P. (2015). ‘Activation and Alliance of Regulatory Pathways in C. Albicans During Mammalian Infection. PLoS Biol. 13 (2), e1002076. doi: 10.1371/JOURNAL.PBIO.1002076
Keywords: epithelial damage, aspergillus fumigatus, transcription factors, pathogenesis, regulatory control, epithelial cells, high-throughput screening, virulence factors
Citation: Rahman S, van Rhijn N, Papastamoulis P, Thomson DD, Carter Z, Fortune-Grant R, Rattray M, Bromley MJ and Bignell E (2022) Distinct Cohorts of Aspergillus fumigatus Transcription Factors Are Required for Epithelial Damage Occurring via Contact- or Soluble Effector-Mediated Mechanisms. Front. Cell. Infect. Microbiol. 12:907519. doi: 10.3389/fcimb.2022.907519
Received: 29 March 2022; Accepted: 18 May 2022;
Published: 28 July 2022.
Edited by:
Samir Agrawal, Barts Health NHS Trust, United KingdomReviewed by:
Teresa OMeara, University of Michigan, United StatesAgostinho Carvalho, University of Minho, Portugal
Copyright © 2022 Rahman, van Rhijn, Papastamoulis, Thomson, Carter, Fortune-Grant, Rattray, Bromley and Bignell. This is an open-access article distributed under the terms of the Creative Commons Attribution License (CC BY). The use, distribution or reproduction in other forums is permitted, provided the original author(s) and the copyright owner(s) are credited and that the original publication in this journal is cited, in accordance with accepted academic practice. No use, distribution or reproduction is permitted which does not comply with these terms.
*Correspondence: Elaine Bignell, ZS5iaWduZWxsQGV4ZXRlci5hYy51aw==