- 1Department of Internal Medicine, Kangwon National University Hospital, Kangwon National University School of Medicine, Chuncheon, South Korea
- 2Division of Allergy and Clinical Immunology, Department of Internal Medicine, School of Medicine, Kyungpook National University Chilgok Hospital, Kyungpook National University, Daegu, South Korea
- 3Department of Surgery, Kangwon National University Hospital, Kangwon NationalUniversity School of Medicine, Chuncheon, South Korea
- 4Department of Pathology, Kangwon National University School of Medicine, Chuncheon, South Korea
- 5Department of Hospital Medicine, Kangwon National University School of Medicine, Chuncheon, South Korea
Background and Aim: Current therapeutic strategies for Clostridioides difficile infections (CDI), including oral vancomycin, metronidazole and fecal microbial transplantation, have limited efficacy and treatment failure may occur in as many as one- third of cases. Recent studies have reported that lower concentrations of 25-hydroxyvitamin D are associated with CDI severity and recurrence. However, there have been no studies on microbiota composition after the administration of vitamin D in patients with CDI. Therefore, our study aimed to compare the microbiota composition between the two groups, including eight CDI-positive patients with vitamin D supplementation and ten CDI-positive patients without vitamin D supplementation by using 16S rRNA microbial profiling.
Methods: Twenty subjects were enrolled in this prospective randomized controlled study. One subject dropped out due to lack of contact with the guardian after discharge and one subject dropped out due to withdrawal of consent. Thus, 18 patients with CDI and vitamin D insufficiency (vitamin D level < 17 ng/mL) were divided into two groups: CDI with vitamin D supplementation (n = 8) and CDI without vitamin D supplementation (control: n = 10). Subjects with vitamin D insufficiency were randomized to receive 200,000 IU intramuscular cholecalciferol whereas patients in the control group received only oral vancomycin. Stool samples were obtained twice before vancomycin was administered and eight weeks after treatment; the V3-V4 16S rRNA metagenomic sequencing was performed using EzBioCloud.
Results: The alpha diversity of the gut microbiota in the recovery state was significantly higher than that in the CDI state. Analysis of bacterial relative abundance showed significantly lower Proteobacteria and higher Lachnospiraceae, Ruminococcaceae, Akkermansiaceae, and Bifidobacteriaceae in the recovery state. When comparing the control and vitamin D treatment groups after eight weeks, increase in alpha diversity and, abundance of Lachnospiraceae, and Ruminococcaceae exhibited the same trend in both groups. A significant increase in Bifidobacteriaceae and Christensenellaceae was observed in the vitamin D group; Proteobacteria abundance was significantly lower in the vitamin D treatment group after eight weeks than that in the control group.
Conclusion: Our study confirmed that the increase in the abundance of beneficial bacteria such as Bifidobacteriaceae, and Christensenellaceae were prominently evident during recovery after administration of a high dose of cholecalciferol. These findings indicate that vitamin D administration may be useful in patients with CDI, and further studies with larger sample sizes are required.
Introduction
Clostridioides difficile is a spore-forming, and toxin-producing, gram-positive anaerobic bacterium. C. difficile infection (CDI) is caused by the colonization of C. difficile due to changes in the composition of the normal intestinal flora of hospitalized patients receiving antibiotics (Guh and Kutty, 2018). CDI is one of the most common causes of nosocomial infections, and its incidence and mortality rates are increasing worldwide (Guh et al., 2020). In addition, antibiotic use is associated with the recurrence and emergence of antibiotic-resistant bacteria. Current therapies using oral vancomycin or metronidazole are inappropriate for treating intractable severe CDI and preventing recurrent CDI (Surawicz and Alexander, 2011). In recent years, attention has been focused on treatments for the preservation and restoration of intestinal flora and the optimization of the immune response to CDI (Johnson et al., 2021).
Over the past several years, experimental studies have reported the association of vitamin and trace element deficiencies with systemic inflammation and multi-organ failure (Holick, 2007). In particular, vitamin D is involved in the maintenance of bone growth, calcium and phosphorus metabolism, and immune system functions (Chang and Lee 2019). Vitamin D-related epidemiological studies have also reported that vitamin D deficiency increases the risk of systemic infection and is associated with a poor disease course and greater disease activity in patients with chronic inflammatory diseases (Jørgensen et al., 2010; Kempker and Martin, 2013). Recent studies have provided evidence that lower concentrations of 25-hydroxyvitamin D [25(OH)D] (vitamin D level < 20 ng/mL) are associated with CDI severity and recurrence (Sahay and Ananthakrishnan, 2014; Abdelfatah et al., 2015). In another study, vitamin D protect against CDI by restoring melanocyte inducing transcription factor expression and lysosomal function in mice (Chan et al., 2022). However, to date, there have been no studies on changes in microbiota composition after the administration of vitamin D in patients with CDI.
In this prospective observational study, we aimed to compare the microbiota composition by conducting 16S rRNA microbial profiling of two groups: CDI-positive with vitamin D supplementation and CDI-positive without vitamin D supplementation.
Materials and Methods
Study Population
This was a prospective, randomized, controlled, and interventional pilot study on 20 patients diagnosed with CDI (defined as ≥3 loose stools in 24 hours without any other cause and a positive glutamate dehydrogenase antigen test, positive Toxin A and Toxin B test or positive polymerase chain reaction) and vitamin D deficiency (serum 25(OH)D levels <17 ng/mL) at Kangwon National University Hospital between October 2019 and June 2021. Twenty subjects were randomly classified into two groups. Exclusion criteria included the use of immunosuppressants, pregnancy or plans to become pregnant in the next 3 months, disorders associated with hypercalcemia, current hypercalcemia (10.8 mg/dL albumin-corrected serum calcium. or 5.2 mg/dL ionized calcium), history of nephrolithiasis, chronic kidney disease worse than stage III, current substantial hepatic dysfunction (2.5 mg/dL total bilirubin, 1.0 mg/dL, direct bilirubin), use of probiotics (within four weeks from the date of CDI diagnosis), and inflammatory bowel disease. The study was approved by the Institutional Review Board of Kangwon National University Hospital (KNUH B-2019-04-005-011). Written informed consent was obtained from all the patients. This research was registered at the Clinical Research Information Service (identifier KCT0004335). Patients in the vitamin D treatment group were administered high-dose vitamin D3 (200,000 IU) via intramuscular injection once and were treated with oral vancomycin (125 mg, qid, for 14 days), whereas patients in the control group received only oral vancomycin (Figure 1).
Data Collection
Stool samples were collected from all patients at baseline and at eight weeks after treatment. Stool samples were immediately stored on ice and frozen at −80°C. DNA was extracted from the collected samples using a sterile container, and the composition of the microorganisms was analyzed via 16S rRNA sequencing using the extracted DNA.
DNA Extraction, PCR Amplification and Sequencing
Total DNA was extracted using the Maxwell® RSC PureFood GMO and Authentication Kit (Promega, USA), according to the manufacturer’s instructions. PCR amplification was performed using fusion primers targeting the V3-V4 region of the 16S rRNA gene. For bacterial amplification, the fusion primers 341F (5’-AATGATACGGCGACCACCGAGATCTACAC-XXXXXXXX-TCGTCGGCAGCGTC-AGATGTGTATAAGAGACAG-CCTACGGGNGGCWGCAG-3’; underlined sequence indicates the target region primer) and 805R (5’- CAAGCAGAAGACGGCATACGAGAT-XXXXXXXX-GTCTCGTGGGCTCGG-AGATGTGTATAAGAGACAG-GACTACHVGGGTATCTAATCC-3’) were used. The fusion primers were constructed in the following order: P5 (P7) graft-binding, i5 (i7) index, Nextera consensus, sequencing adaptor, and target region sequence. The amplification conditions were as follow: initial denaturation at 95°C for 3 min, followed by 25 cycles of denaturation at 95°C for 30 s, annealing at 55°C for 30 s, extension at 72°C for 30 s, and a final elongation at 72°C for 5 min. The PCR product was confirmed using 1% agarose gel electrophoresis and visualized using a Gel Doc system (Bio-Rad, Hercules, CA, USA). The amplified products were purified using CleanPCR (CleanNA). Equal concentrations of the purified products were pooled and short fragments (non-target products) were removed using CleanPCR (CleanNA). Quality and product size were assessed on the Bioanalyzer 2100 (Agilent, Palo Alto, CA, USA) using a DNA 7500 chip. Mixed amplicons were pooled and sequencing was performed by Chunlab, Inc. (Seoul, Korea), using the Illumina MiSeq Sequencing system (Illumina, USA) according to the manufacturer’s instructions. Sequence data were deposited in the National Center for Biotechnology Information as Bio Project ID: PRJNA824324.
Data Analysis Pipeline
The processing of raw reads started with quality check and filtering of low quality (<Q25) reads by Trimmomatic ver. 0.321. After QC pass, paired-end sequence data were merged together using the fastq_mergepairs command of VSEARCH version 2.13.42 with default parameters. Primers were then trimmed using the alignment algorithm of Myers and Miller3 at a similarity cutoff of 0.8. Non-specific amplicons that do not encode 16S rRNA were detected using nhmmer (Wheeler and Eddy, 2013) in the HMMER software package ver. 3.2.1 with hmm profiles. Unique reads were extracted and redundant reads were clustered with the unique reads by the derep_fulllength command of VSEARCH (Rognes et al., 2016). The EzBioCloud 16S rRNA database5 was used for taxonomic assignment using usearch global command of VSEARCH2 followed by more precise pairwise alignment3. Chimeric reads were filtered on reads with <97% similarity by reference based chimeric detection using the UCHIME algorithm6 and the non-chimeric 16S rRNA database from EzBioCloud. After chimeric filtering, reads that are not identified at the species level (with <97% similarity) in the EzBioCloud database were compiled and cluster_fast command2 was used to perform de-novo clustering to generate additional Operational taxonomic units. Finally, OTUs with single reads (singletons) were omitted from further analyses. The secondary analysis which includes diversity calculation and biomarker discovery was conducted using in-house programs of Chunlab, Inc (Seoul, South Korea). Alpha diversity information was confirmed through the Chao1 value, and Shannon. The relationship between samples was visualized through principal coordinate analysis (PCoA) using the Bray-Curtis dissimilarity and beta diversity distances were calculated using the Bray-Curtis dissimilarity index.
Linear discriminant Effect Size (LEfSe) analysis was performed to identify bacteria that were significantly different; the degree of difference was expressed as a linear discriminant analysis (LDA) score with α = 0.05 and LDA score threshold-2. At this time, 1% or more of the genus level was analyzed. All analyses mentioned above were performed in the EzBioCloud 16S-based MTP, which is a Chunlab bioinformatics cloud platform.
Statistical Analysis
Continuous variables were analyzed using the Mann–Whitney U test. Fisher’s exact test was used to compare the categorical variables between the control and experimental group. Paired data were analyzed using the paired Wilcoxon signed-rank test. In the statistical analysis, continuous variables were analyzed using t-test when P ≥ 0.05 and the Shapiro-Wilk and the Mann-Whitney U tests when P < 0.05. Statistical analyses were performed using SPSS for Windows (version 19.0; IBM Co., Armonk, NY, USA). Statistical significance was set at P < 0.05. GraphPad Prism 9.0 software (GraphPad Inc., San Diego, CA, USA) was used to generate the graphs.
Results
Demographic and Clinical Characteristics
The demographic and clinical characteristics of the patients are summarized in Table 1. Twenty subjects who met the inclusion criteria were enrolled; however, one subject dropped out due to no contact with the guardian after discharge, and one subject dropped out due to withdrawal of consent. Thus, a total of 18 subjects was analyzed. No differences in age, sex, BMI, presence of hypertension, presence of diabetes, cardiovascular disease, liver disease, chronic kidney disease, or chronic respiratory disease were observed between the vitamin D treatment and control groups. In addition, no statistically significant difference between the two groups in any of the tests performed were observed, except for blood urea nitrogen in the hematological examination (Table 1).
Changes in the Gut Microbiota at the Time of CDI and Recovery After Eight Weeks
Alpha diversity, particularly in the Shannon index was increased (Figure 2A). The beta diversity using principal coordinate analysis was higher in terms of weighted UniFrac distance in CDI than in the recovery state (Figure 2B). When looking at the changes in individual species, the abundance of Proteobacteria (Enterobacteriaceae and Sutterellaceae) and Enterococcaceae, which are generally known to increase during CDI or antibiotic treatment, increased during CDI compared to those after recovery. In particular, a significant reduction in Proteobacteria (47.61 ± 37.20% in CDI vs. 13.63 ± 16.79% in recovery, P = 0.002) was evident during the recovery period. Conversely, the numbers of commensal bacteria and beneficial strains increased during the recovery period. A statistically significant increase in the abundance of short-chain fatty acid (SCFA)-producing clostridia such as Lachnospiraceae (3.50 ± 8.17% in CDI vs. 22.92 ± 19.66% in recovery, P = 0.001 and Ruminococcaceae (0.49 ± 0.80% in CDI vs. 11.12 ± 15.27% in recovery, P < 0.001) was observed (Figure 1C). Beneficial strains such as Akkermanssiaceae (0.03 ± 0.13% in CDI vs. 3.35 ± 9.71% in recovery, P = 0.020), Bifidobacteriaceae (0.79 ± 3.27% in CDI vs. 2.44 ± 3.79% in recovery, P = 0.017) and Christensenellaceae (0.13 ± 0.04% in CDI vs. 1.02 ± 2.69% in recovery, P = 0.022) also showed increased abundance. The decrease in Proteobacteria abundance and the increase in Lachnospiraceae and Ruminococcaceae abundance during the recovery period showed a statistically strong association (Figure 2C).
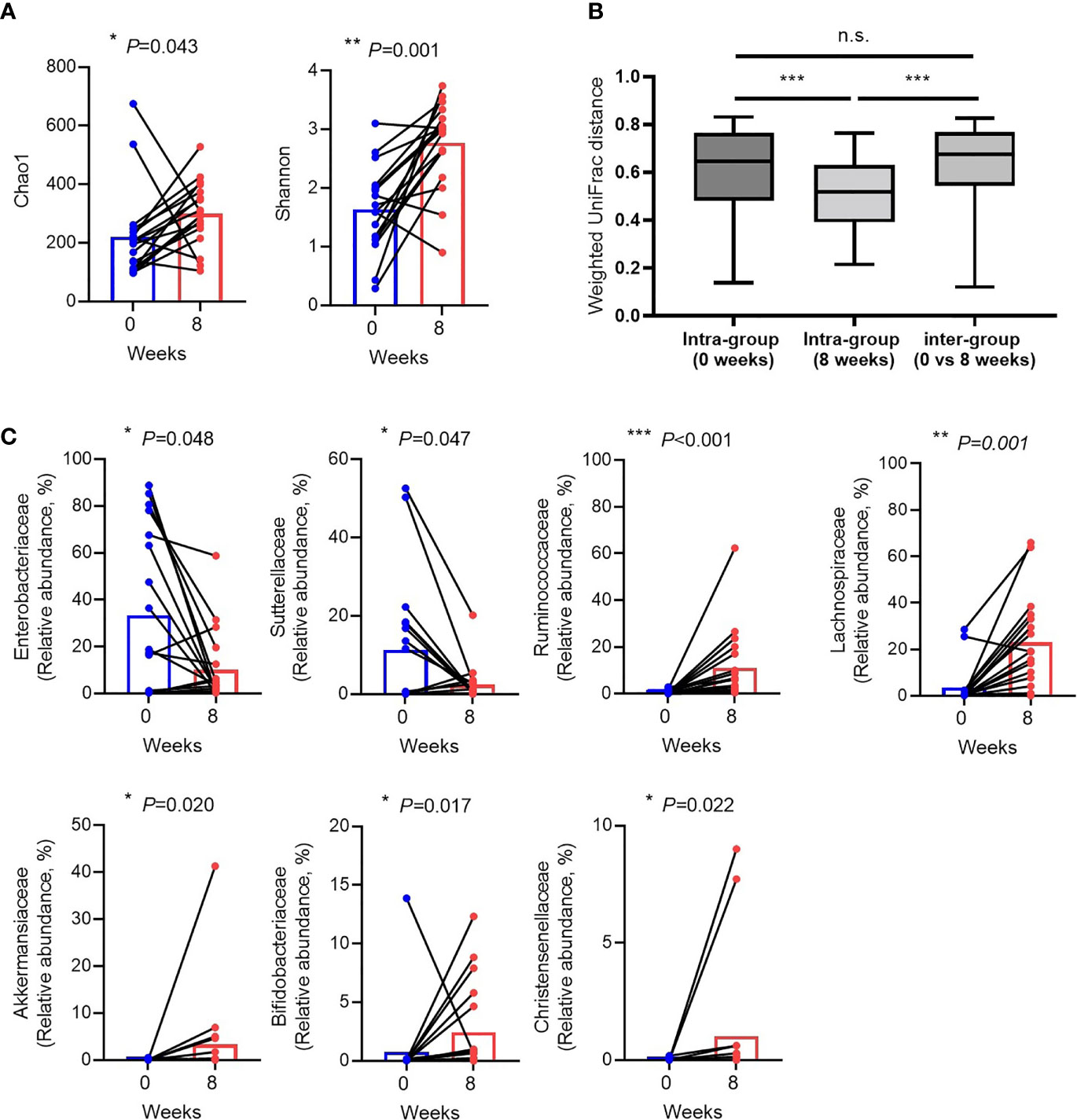
Figure 2 Changes in the gut microbiota between Clostridioides difficile infection and recovery after eight weeks. (A) Alpha diversity. (B) Weighted UniFrac distance. (C) Relative abundances of individual bacteria that were significantly different between Clostridioides difficile infection and recovery. Blue dots indicate C. difficile infection state (0 week), red dots indicate recovery after eight weeks, and black lines represent changes in the same patient. n.s, not significant; *P < 0.05, **P < 0.01 and ***P < 0.001 (Wilcoxon signed rank test and Mann-Whitney U test).
Changes in the Gut Microbiota in the Control and Vitamin D Treatment Groups
No significant difference in the alpha diversity between the vitamin D treatment and control groups were observed (Figure 3A). Beta diversity was not significantly different between the vitamin D treatment and control groups (Figures 3B, 3C). In addition, no statistically significant differences at the phylum level were observed between the two groups (Supplementary figure 1).
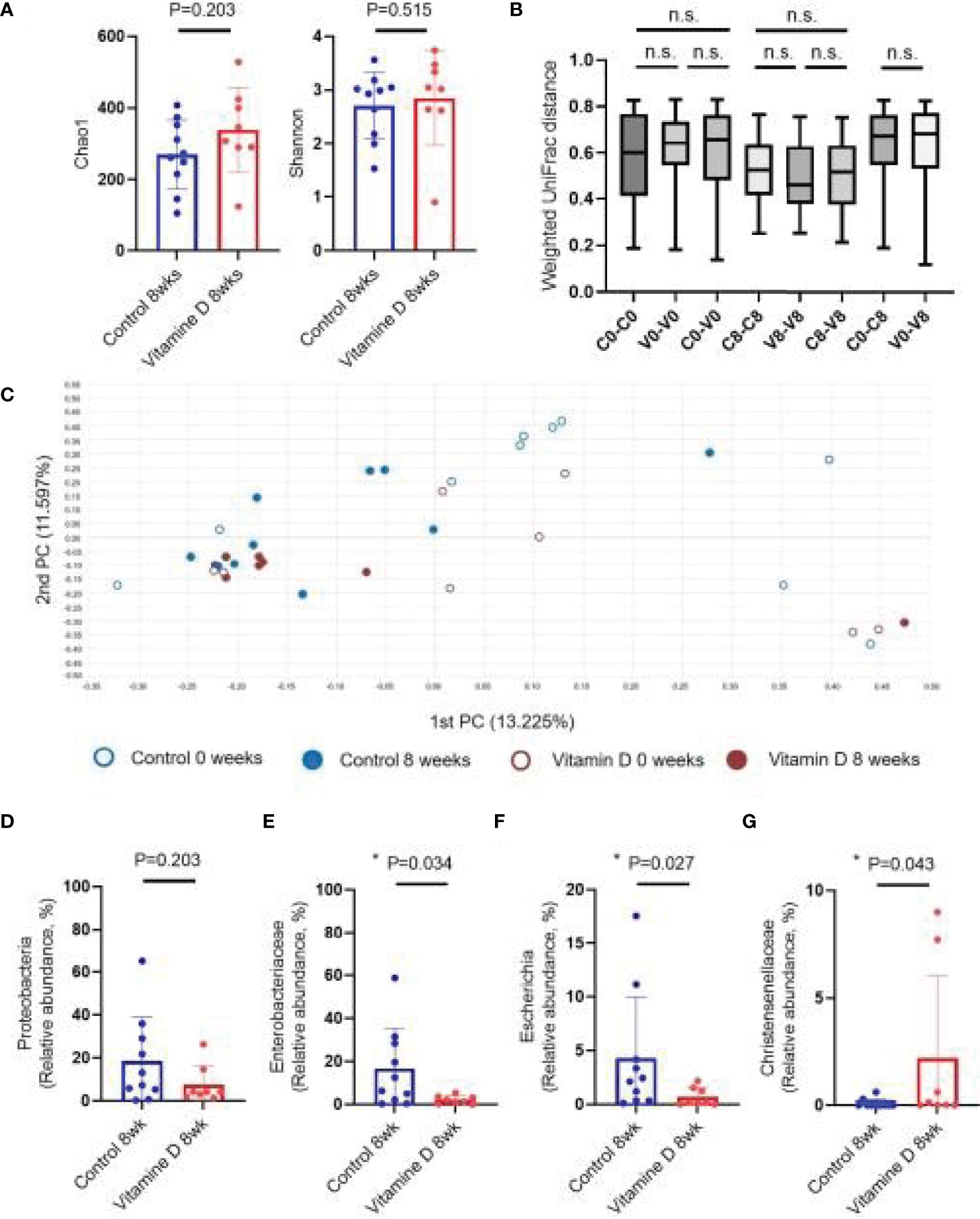
Figure 3 Effect of vitamin D supplementation on the gut microbiome. (A) Alpha diversity. (B) Weighted UniFrac distance. (C) Principal coordinates analysis (Bray–Curtis dissimilarity). (D) Relative abundances of Proteobacteria. (E) Relative abundances of Enterobacteriaceae. (F) Relative abundances of Escherichia. (G) Relative abundances of Christensenellaceae. n.s, not significant; *P < 0.05 (Wilcoxon signed rank test and Mann-Whitney U test).
With respect to individual taxon, the abundance of Proteobacteria, which significantly increased during CDI, showed a downward trend in the vitamin D treatment group, but the difference was not statistically significant. At the family and genus levels, the vitamin D treatment group showed a significant decrease in Enterobacteriaceae (16.50 ± 18.69% in CDI vs. 2.16 ± 1.88% in recovery, P = 0.034) and Escherichia (4.29 ± 5.68% in CDI vs. 0.69 ± 0.85% in recovery, P = 0.027) compared to those in the control group (Figures 3D–F and Supplementary Figure 2). Furthermore, the abundance of Christensenellaceae and Sutterellaceae was higher in the vitamin D treatment group. However, the vitamin D treatment group decreased more effectively than CDI and recovery groups (Figure 3G, Supplementary Figure 2 and Supplementary Figure 3).
The increase in Lachnospiraceae, Ruminococcaceae, Bifidobacteriaceae, and Christensenellaceae were evident in the vitamin D treatment group, whereas the abundance of Proteobacteria decreased (Figures 4A, B). Changes in Proteobacteria, Lachnospiraceae, and Ruminococcaceae abundance were also observed in both the vitamin D treatment group and the control group (Figures 4C–E). However, the increase in Bifidobacteriaceae and Christensenellaceae abundance was more prominent in the vitamin D treatment group than that in the control group (Figures 4F, G).
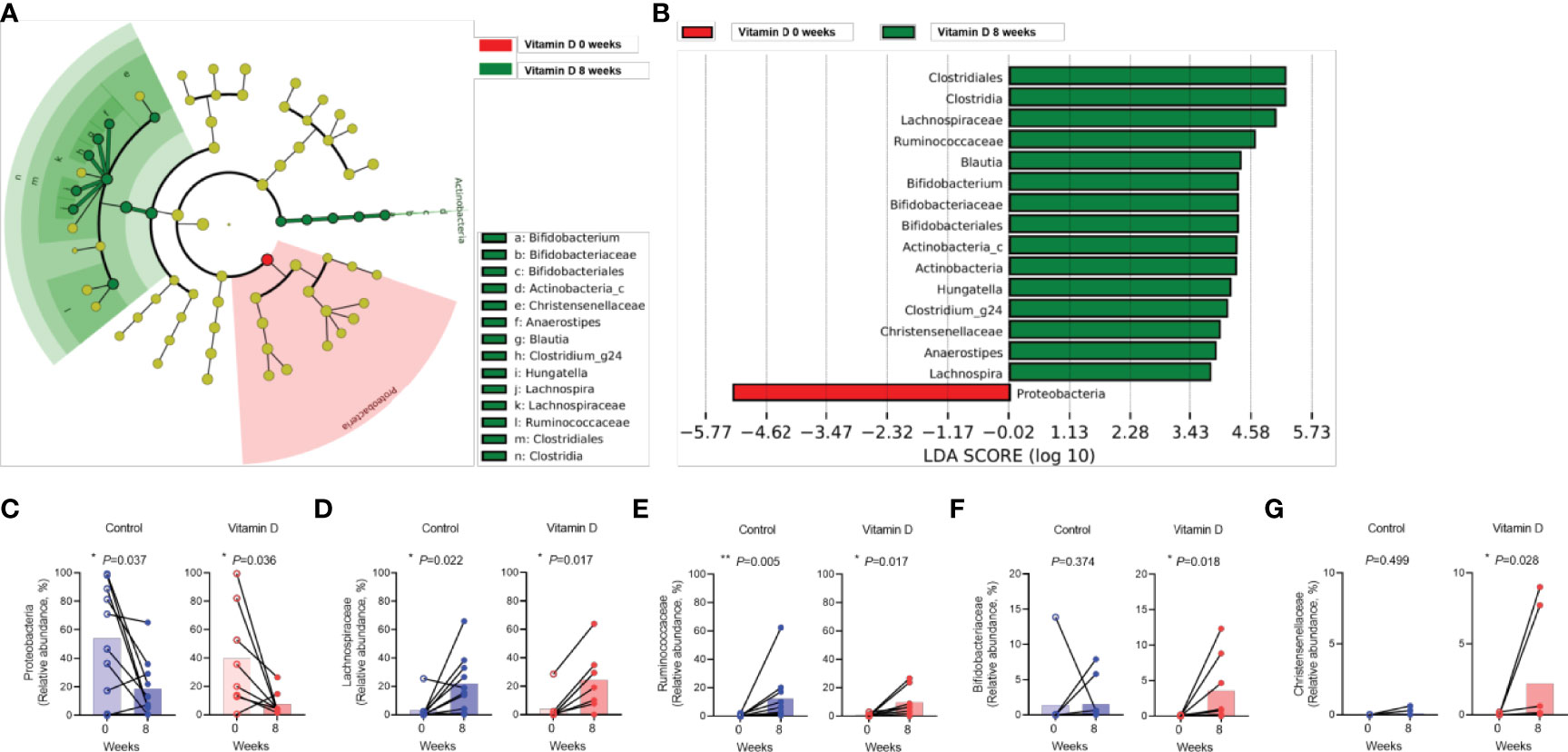
Figure 4 Effect of vitamin D supplementation on the individual gut taxa. A, B. Cladogram (A) and linear discriminant analysis scores (B) using LEfSe analysis of the fecal microbiota in Clostridioides difficile infection versus recovery after eight weeks in the vitamin D treatment group. (C–G). Differences in the abundance of individual bacteria between the control group and vitamin (D) supplement group. (C) Proteobacteria. (D) Lachnospiraceae. (E) Ruminococcaceae. (F) Bifidobacteriaceae. (G) Christensenellaceae. *P < 0.05, **P < 0.01 (Wilcoxon signed rank test).
Discussion
This study investigated the changes in the gut microbiota between CDI and recovery in patients with and without vitamin D supplementation. Specifically, we found a significant increase in the abundance of Proteobacteria during CDI, and an increase in the abundance of Lachnospiraceae, Ruminococcaceae, Akkermansiaceae, Bifidobacteriaceae, and Christensenellaceae after recovery. The vitamin D treatment group showed a significant increase in the abundance of beneficial bacteria, such as Bifidobacteriaceae and Christensenellaceae, and the abundance of Enterobacteriaceae in the recovery state was significantly lower than that in the group without vitamin D treatment.
To date, the overall incidence of CDI and severe or fulminant CDI have increased, in association with an increased infection by highly virulent strains, such as NAP1/BI/027, and the increased use of antibiotics, anticancer drugs, and gastric acid inhibitors (Pépin et al., 2004; Ricciardi et al., 2007). Another problem is the increased frequency of CDI recurrence that may be caused by the reactivation of previous bacteria or re-infection with new bacteria. According to previous studies, up to 25% of patients experience recurrent CDI within 30 days of treatment (Kelly, 2012). The risk of subsequent recurrence in patients was 45% (McFarland et al., 2002). In the treatment of severe, refractory, or recurrent CDI, vancomycin has shown limitations in enhancing the effectiveness of treating or preventing recurrence. Recently, many studies have investigated fecal microbiota transplantation (FMT) as a treatment for CDI. Conventional CDI treatment with vancomycin disrupts the balance of normal colonic flora and weakens the resistance of normal flora to other proliferating bacteria. In FMT, this imbalance is restored by transplanting donor feces containing normal flora into patients with CDI (Kim, 2012). However, FMT has procedural complications and risks the transmission of infectious agents (Reumkens et al., 2016; Zellmer et al., 2021).
Vitamin D plays an important role in the maintenance of bone mineral density and has an important influence on the immune system, including the modulation of antiviral and antibacterial inflammatory immune responses (Chang and Lee 2019). Most studies emphasize maintaining vitamin D levels above 30 ng/ml to prevent osteoporosis and rickets (Dawson-Hughes et al., 2010; American Geriatrics Society Workgroup on Vitamin D Supplementation for Older Adults Workgroup on Vitamin, 2014). It also has an important influence on the immune system (Taha et al., 2021),. Vitamin D induces cathelicidin production, which can directly kill viruses and bacteria or bind to endotoxins (Liu et al., 2006). Studies on vitamin D deficiency and supplementation in inflammatory bowel disease (IBD) and inherited disorders, such as cystic fibrosis, have been published. When vitamin D was administered daily for one month to patients with IBD and vitamin D deficiency, a negative relationship between vitamin D levels and C-reactive protein was found (Jun et al., 2019). Kanhere et al. (2018) reported that in patients with cystic fibrosis and vitamin D deficiency, an imbalance in the intestinal microflora was observed, which improved when high doses of vitamin D were administered. Abdelfatah et al. (2015) suggested a significant relationship between vitamin D levels and CDI severity. In the present study, we investigated the effect of vitamin D by applying a strict standard of less than 17 ng/mL, which is lower than the vitamin D deficiency standard (Taha et al., 2021). When vitamin D3 (200,000 IU) was administered to patients with CDI at the initial stage of infection, vitamin D deficiency was quickly corrected, but the difference was not statistically significant compared to the control group in relieving microbiota dysbiosis.
Firmicutes and Bacteroidetes dominate the normal intestinal environment, whereas Proteobacteria, Actinobacteria, and Verrucomicrobia are less abundant (Backhed et al., 2005). An increased prevalence of Proteobacteria is a marker of an imbalance in the taxonomic composition of the gut microbiota and a potential diagnostic criterion for disease (Shin et al., 2015). In particular, an increase in Proteobacteria is evident when the gut microbiota changes, and metabolic homeostasis is disrupted by the use of antibiotics (Zarrinpar et al., 2018). In CDI, Proteobacteria and Enterococcus strains are the major strains that increase during dysbiosis owing to changes in the gut microbiota (Samarkos et al., 2018; Kim et al., 2020). Proteobacteria induces epithelial dysfunction and exacerbates intestinal inflammation, and the association between CDI and IBD is well known (Litvak et al., 2017; Caruso et al., 2020). In contrast, Bacteroides, Prevotella, and Clostridiales are commensal bacteria, and enterotyping is performed depending on which strain is dominant (Arumugam et al., 2011). Among them, SCFA-producing Clostridium, as well as Lachnospiraceae and Ruminococcaceae, play an important role in the anti-inflammatory action of intestinal immunity by increasing T reg activity (Guo et al., 2020; Park et al., 2020). In a previous study, patients with CDI showed a decrease in Lachnospiraceae and Ruminococcaceae (Antharam et al., 2013). In the present study, the increase in Proteobacteria abundance after CDI was substantial. Furthermore, during the recovery process, the decrease in Proteobacteria abundance and increase in the abundance of Lachnospiraceae and Ruminococcaceae, which are important for the secretion of metabolites such as SCFA with anti-inflammatory effects, particularly among normal flora were prominent. Therefore, methods that reduce Proteobacteria abundance and increase the abundance of commensal Clostridiales, such as Lachnospiraceae and Ruminococcaceae, could be used to treat CDI. Akkermansia is also a candidate probiotic that has recently received attention for its role in metabolic and systemic diseases (Plovier et al., 2017; Michalovich et al., 2019).
In addition, we observed that the abundance of Bifidobacteriaceae and Christensenellaceae significantly increased with vitamin D supplementation. Bifidobacterium, a well-known probiotic, is effective against inflammatory diseases by reducing inflammatory substances including cytokines, protecting the intestinal epithelial barrier, and balancing the gut microbiota (Konieczna et al., 2012). The anti-inflammatory effects of Bifidobacterium in animal dextran sodium sulfate models and human IBD have also been consistently reported (Singh et al., 2020; Yao et al., 2021). In a recent study involving vitamin D supplementation in healthy controls, an increase in Bifidobacterium abundance was observed (Meng et al., 2020). Previous studies have reported that Bifidobacteriaceae reduces CDI through anti-inflammatory effects (Skraban et al., 2013; Singh et al., 2020). Meanwhile, the function of Christensenellaceae is relatively less known, but it is a commensal bacterium in the human gut that plays an important role in human health (Waters and Ley, 2019). Further research is needed on the effects of Bifidobacteriaceae and Christensenellaceae on CDI and their relationship with vitamin D. Fifth, it was not possible to evaluate whether there were intestinal changes in increased intestinal permeability following high-dose vitamin D supplementation. In our first study plan, we tried to perform biopsy by performing sigmoidoscopy at the time of initial diagnosis and 8 weeks after treatment for CDI. Through this biopsy, it was attempted to determine whether there were any changes in the tissues depending on whether or not high-dose vitamin D was administered, but it was not possible to obtain the consent of the patients and guardians.
This study has several limitations. First, since this was a pilot study, the sample size was small, which may be statistically underpowered. To overcome this limitation, we included a control group. Second, selection bias may be possible because only patients with CDI with a vitamin D level of less than 17 ng/mL were enrolled in our study. Third, other parameters such as diet were not evaluated. Further investigations, including dietary patterns and other predisposing factors that could affect the microbiome, would help deepen our understanding of the relationship between vitamin D and the microbiome. Fourth, the short-term effects of vitamin D supplementation could not be assessed. In this study, the control group also showed a significant change after eight weeks compared to the CDI group. Therefore, to observe the effects of vitamin D, additional analysis over a short period of one–two weeks may be necessary.
In conclusion, our study is the first to identify changes in the gut microbiota upon administration of high-dose vitamin D to patients with CDI and show that the administration of a high dose of cholecalciferol may play an adjuvant role in the treatment of CDI for the first time. Furthermore, our study confirmed that the increase in Christensenellaceae and Bifidobacteriaceae abundance was enhanced in vitamin D-deficient patients with CDI recovery after the administration of a high dose of cholecalciferol. Physicians should consider the potential role of vitamin D as replacement therapy in patients with CDI. These findings require further evaluation in a larger, multicenter study.
Data Availability Statement
The datasets presented in this study can be found in online repositories. The name of the repository and accession number can be found below: NCBI; PRJNA824324.
Ethics Statement
The studies involving human participants were reviewed and approved by the Institutional Review Board of Kangwon National University Hospital. The patients/participants provided their written informed consent to participate in this study.
Author Contributions
SL and H-KP: design of the study. SL, CK, DC and JP: data acquisition. H-KP, KL and HC: data analysis, and interpretation. S-JN, SP and GC: drafted the article and critically revised the manuscript. SL: gave final approval for the version to be submitted. All authors reviewed and approved the final version of the manuscript.
Funding
This study received funding from 2019 Hanmi Pharm. Co. The funder was not involved in the study design, collection, analysis, interpretation of data, the writing of this article or the decision to submit it for publication. All authors declare no other competing interests.
Conflict of Interest
The authors declare that the research was conducted in the absence of any commercial or financial relationships that could be construed as a potential conflict of interest.
Publisher’s Note
All claims expressed in this article are solely those of the authors and do not necessarily represent those of their affiliated organizations, or those of the publisher, the editors and the reviewers. Any product that may be evaluated in this article, or claim that may be made by its manufacturer, is not guaranteed or endorsed by the publisher.
Supplementary Material
The Supplementary Material for this article can be found online at: https://www.frontiersin.org/articles/10.3389/fcimb.2022.904987/full#supplementary-material
References
Abdelfatah, M., Nayfe, R., Moftakhar, B., Nijim, A., Zoghbi, M. E., Donskey, C. J., et al. (2015). Low Vitamin D Level and Impact on Severity and Recurrence of Clostridium Difficile Infections. J. Investig. Med. 63, 17–21. doi: 10.1097/JIM.0000000000000117
American Geriatrics Society Workgroup on Vitamin D Supplementation for Older Adults Workgroup on Vitamin (2014). Recommendations Abstracted From the American Geriatrics Society Consensus Statement on Vitamin D for Prevention of Falls and Their Consequences. J. Am. Geriatr. Soc. 62, 147–152. doi: 10.1111/jgs.12631
Antharam, V. C., Li, E. C., Ishmael, A., Sharma, A., Mai, V., Rand, K. H., et al. (2013). Intestinal Dysbiosis and Depletion of Butyrogenic Bacteria in Clostridium Difficile Infection and Nosocomial Diarrhea. J. Clin. Microbiol. 51, 2884–2892. doi: 10.1128/JCM.00845-13
Arumugam, M., Raes, J., Pelletier, E., Paslier, D. L., Yamada, T., Mende, D. R., et al. (2011). Enterotypes of the Human Gut Microbiome. Nature 473, 174–180. doi: 10.1038/nature09944
Backhed, F., Sonnenburg, L. S., Peterson, D. A., Gordon, J. I.. (2005). Host-Bacterial Mutualism in the Human Intestine. Science 307, 1915–1920. doi: 10.1126/science.1104816
Caruso, R., Lo, B. C., Núñez, G. (2020). Host–microbiota Interactions in Inflammatory Bowel Disease. Nat. Rev. Immunol. 20, 411–426. doi: 10.1038/s41577-019-0268-7
Chan, H., Li, Q., Wang, X., Liu, W. Y., Hu, W., Zeng, J., et al. (2022). Vitamin D3 and Carbamazepine Protect Against Clostridioides Difficile Infection in Mice by Restoring Macrophage Lysosome Acidification. Autophagy 1–18. doi: 10.1080/15548627.2021.2016004
Chang, S.-W., Lee, H.-C.. (2019). Vitamin D and Health - The Missing Vitamin in Humans. Pediatr. Neonatol. 60 (3), 237–44. doi: 10.1016/j.pedneo.2019.04.007
Dawson-Hughes, B., Mithal, A., Bonjour, J. P., Boonen, S., Burckhardt, P., Yoshimura, N., et al. (2010). IOF Position Statement: Vitamin D Recommendations for Older Adults. Osteoporos. Int. 21, 1154. doi: 10.1007/s00198-010-1285-3
Guh, A. Y., Kutty, P. K. (2018). Clostridioides Difficile Infection. Ann. Intern. Med. 169, ITC49–ITC64. doi: 10.7326/AITC201810020
Guh, A. Y., Mu, Y., Winston, L. G., Johnston, H., Olson, D., Farley, M. M., et al. (2020). Trends in U.S. Burden of Clostridioides Difficile Infection and Outcomes. N. Engl. J. Med. 382, 1320–1330. doi: 10.1056/NEJMoa1910215
Guo, P., Zhang, K., Ma, X., He, P. (2020). Clostridium Species as Probiotics: Potentials and Challenges. J. Anim. Sci. Biotechnol. 11, 24. doi: 10.1186/s40104-019-0402-1
Holick, M. F. (2007). Vitamin D Deficiency. N. Engl. J. Med. 357, 266–281. doi: 10.1056/NEJMra070553
Jørgensen, S. P., Agnholt, J., Glerup, H., Lyhne, S., Villadsen, G. E., Hvas, C. L., et al. (2010). Clinical Trial: Vitamin D3 Treatment in Crohn’s Disease: A Randomized Double-Blind Placebo-Controlled Study. Aliment Pharmacol. Ther. 32, 377–383. doi: 10.1111/j.1365-2036.2010.04355.x
Johnson, S., Lavergne, V., Skinner, A. M., Gonzales-Luna, A. J., Garey, K. W., Kelly, C. P., et al. (2021). Clinical Practice Guideline by the Infectious Diseases Society of America (IDSA) and Society for Healthcare Epidemiology of America (SHEA): 2021 Focused Update Guidelines on Management of Clostridioides Difficile Infection in Adults. Clin. Infect. Dis. 73, e1029–e1044.
Jun, J. C., Yoon, H., Choi, Y. J., Shin, C. M., Park, Y. S., Kim, N., et al. (2019). When Daily Vitamin D Was Administered for One Month in IBD Patients With Vitamin D Deficiency, a Negative Relationship Between Vitamin D Levels and C-Reactive Protein Was Found. Intest Res. 17, 210–217. doi: 10.5217/ir.2018.00081
Kanhere, M., He, J., Chassaing, B., Ziegler, T. R., Lvie, E. A., Hao, L., et al. (2018). Bolus Weekly VitaminD3 Supplementation Impacts Gut and Airway Microbiota in Adults With Cystic Fibrosis: A Double-Blind, Randomized, Placebo-Controlled Clinical Trial. J. Clin. Endocrinol. Metab. 103, 564–574. doi: 10.1210/jc.2017-01983
Kelly, C. P. (2012). Can We Identify Patients at High Risk of Recurrent Clostridium Difficile Infection? Clin. Microbiol. Infect. 18 Suppl 6, 21.
Kempker, J. A., Martin, G. S. (2013). Vitamin D and Sepsis: From Associations to Causal Connections. Inflamm. Allergy Drug Targets 12, 246–252. doi: 10.2174/18715281113129990048
Kim, S. W. (2012). Treatment of Refractory or Recurrent Clostridium Difficile Infection. Korean J. Gastroenterol. 60, 71–78. doi: 10.4166/kjg.2012.60.2.71
Kim, J., Cho, Y., Seo, M.-R., Bae, M. H., Rho, M., Pai, H., et al. (2020). Quantitative Characterization of Clostridioides Difficile Population in the Gut Microbiome of Patients With C. Difficile Infection and Their Association With Clinical Factors. Sci. Rep. 10, 17608.
Konieczna, P., Akdis, C. A., Quigley, E. M., Shanahan, F., O'Mahony, L. (2012). Portrait of an Immunoregulatory Bifidobacterium. Gut Microbes 3, 261–266. doi: 10.4161/gmic.20358
Litvak, Y., Byndloss, M. X., Tsolis, R. M., Bäumler, A. J. (2017). Dysbiotic Proteobacteria Expansion: A Microbial Signature of Epithelial Dysfunction. Curr. Opin. Microbiol. 39, 1–6. doi: 10.1016/j.mib.2017.07.003
Liu, P. T., Stenger, S., Li, H., Li, H., Wenzel, L., Tan, B. H., Krutzik, S. R., et al. (2006). Toll-Like Receptor Triggering of a Vitamin D-Mediated Human Antimicrobial Response. Science 311, 1770–1773. doi: 10.1126/science.1123933
McFarland, L. V., Elmer, G. W., Surawicz, C. M. (2002). Breaking the Cycle: Treatment Strategies for 163 Cases of Recurrent Clostridium Difficile Disease. Am. J. Gastroenterol. 97, 1769–1775. doi: 10.1111/j.1572-0241.2002.05839.x
Meng, D., Sommella, E., Salviati, E., Campiglia, P., Ganguli, K., Djebali, K., et al. (2020). Indole-3-Lactic Acid, a Metabolite of Tryptophan, Secreted by Bifidobacterium Longum Subspecies Infantis Is Anti-Inflammatory in the Immature Intestine. Pediatr. Res. 88, 209–217. doi: 10.1038/s41390-019-0740-x
Michalovich, D., Rodriguez-Perez, N., Smolinska, S., Pirozynski, M., Mayhew, D., Uddin, S., et al. (2019). Obesity and Disease Severity Magnify Disturbed Microbiome-Immune Interactions in Asthma Patients. Nat. Commun. 10, 5711. doi: 10.1038/s41467-019-13751-9
Park, H. K., Choi, Y., Lee, D. H., Kim, S., Lee, J.-M., Choi, S. W., et al. (2020). Altered Gut Microbiota by Azithromycin Attenuates Airway Inflammation in Allergic Asthma. J. Allergy Clin. Immunol. 145, 1466–1469.e8. doi: 10.1016/j.jaci.2020.01.044
Pé́pin, J., Valiquette, L., Alary, M. E., Villemure, P., Pelletier, A., Forget, K., et al. (2004). Clostridium Difficile-Associated Diarrhea in a Region of Quebec From 1991 to 2003: A Changing Pattern of Disease Severity. CMAJ 171, 466–472. doi: 10.1503/cmaj.1041104
Plovier, H., Everard, A., Druart, C., Depommier, C., Hul, M. V., Geurts, L., et al. (2017). A Purified Membrane Protein From Akkermansia Muciniphila or the Pasteurized Bacterium Improves Metabolism in Obese and Diabetic Mice. Nat. Med. 23, 107–113. doi: 10.1038/nm.4236
Reumkens, A., Rondagh, E. J., Bakker, C. M., Winkens, B., Masclee, A. M., Sanduleanu, S.. (2016). Post-Colonoscopy Complications: A Systematic Review, Time Trends, and Meta-Analysis of Population-Based Studies. Am. J. Gastroenterol. 111, 1092–1101. doi: 10.1038/ajg.2016.234
Ricciardi, R., Rothenberger, D. A., Madoff, R. D., Baxter, N. N. (2007). Increasing Prevalence and Severity of Clostridium Difficile Colitis in Hospitalized Patients in the United States. Arch. Surg. 142, 624–631. doi: 10.1001/archsurg.142.7.624
Rognes, T., Flouri, T., Nichols, B., Quince, C., Mahé, F. (2016). VSEARCH: A Versatile Open Source Tool for Metagenomics. PeerJ 4, e2584. doi: 10.7717/peerj.2584
Sahay, T., Ananthakrishnan, A. N. (2014). Vitamin D Deficiency is Associated With Community-Acquired Clostridium Difficile Infection: A Case–Control Study. BMC Infect. Dis. 14, 661. doi: 10.1186/s12879-014-0661-6
Samarkos, M., Mastrogianni, E., Kampouropoulou, O. (2018). The Role of Gut Microbiota in Clostridium Difficile Infection. Eur. J. Intern. Med. 50, 28–32. doi: 10.1016/j.ejim.2018.02.006
Shin, N. R., Whon, T. W., Bae, J. W. (2015). Proteobacteria: Microbial Signature of Dysbiosis in Gut Microbiota. Trends Biotechnol. 33, 496–503. doi: 10.1016/j.tibtech.2015.06.011
Singh, S., Bhatia, R., Khare, P., Sharma, S., Rajarammohan, S., Bishnoi, M., et al. (2020). Anti-Inflammatory Bifidobacterium Strains Prevent Dextran Sodium Sulfate Induced Colitis and Associated Gut Microbial Dysbiosis in Mice. Sci. Rep. 10, 18597. doi: 10.1038/s41598-020-75702-5
Singh, P., Rawat, A., Alwakeel, M., Sharif, E., Al Khodor, S. (2020). The Potential Role of Vitamin D Supplementation as a Gut Microbiota Modifier in Healthy Individuals. Sci. Rep. 10, 21641. doi: 10.1038/s41598-020-77806-4
Skraban, J., Dzeroski, S., Zenko, B., Mongus, D., Gangl, S., Rupnik, M., et al. (2013). Gut Microbiota Patterns Associated With Colonization of Different Clostridium Difficile Ribotypes. PLos One 8, e58005. doi: 10.1371/journal.pone.0058005
Surawicz, C. M., Alexander, J. (2011). Treatment of Refractory and Recurrent Clostridium Difficile Infection. Nat. Rev. Gastroenterol. Hepatol. 8, 330–339. doi: 10.1038/nrgastro.2011.59
Taha, R., Abureesh, S., Alghamdi, S., Hassan, R. Y., Cheikh, M. M., Bagabir, R. A., et al. (2021). The Relationship Between Vitamin D and Infections Including COVID-19: Any Hopes? Int. J. Gen. Med. 14, 3849–3870. doi: 10.2147/IJGM.S317421
Waters, J. L., Ley, R. E. (2019). The Human Gut Bacteria Christensenellaceae Are Widespread, Heritable, and Associated With Health. BMC Biol. 17, 83. doi: 10.1186/s12915-019-0699-4
Wheeler, T. J., Eddy, S. R. (2013). Nhmmer: DNA Homology Search With Profile HMMs. Bioinformatics 29 (19), 2487–2489. doi: 10.1093/bioinformatics/btt403
Yao, S., Zhao, Z., Wang, W., Liu, X. (2021). Bifidobacterium Longum: Protection Against Inflammatory Bowel Disease. J. Immunol. Res. 2021, 8030297. doi: 10.1155/2021/8030297
Zarrinpar, A., Chaix, A., Xu, Z. Z., Chang, M. W., Marotz, C. A., Saghatelian, A., et al. (2018). Antibiotic-Induced Microbiome Depletion Alters Metabolic Homeostasis by Affecting Gut Signaling and Colonic Metabolism. Nat. Commun. 9, 2872. doi: 10.1038/s41467-018-05336-9
Keywords: clostridioides difficile infection, vitamin D, microbiota, cholecalciferol, bifidobacteriaceae, christensenellaceae
Citation: Lee SH, Park H-K, Kang CD, Choi DH, Park SC, Park JM, Nam S-J, Chae GB, Lee Ky, Cho H and Lee SJ (2022) High Dose Intramuscular Vitamin D3 Supplementation Impacts the Gut Microbiota of Patients With Clostridioides Difficile Infection. Front. Cell. Infect. Microbiol. 12:904987. doi: 10.3389/fcimb.2022.904987
Received: 26 March 2022; Accepted: 10 May 2022;
Published: 06 June 2022.
Edited by:
Sangappa B. Chadchan, Washington University in St. Louis, United StatesReviewed by:
Chandni Talwar, Baylor College of Medicine, United StatesRakesh Arya, Cleveland Clinic, United States
Copyright © 2022 Lee, Park, Kang, Choi, Park, Park, Nam, Chae, Lee, Cho and Lee. This is an open-access article distributed under the terms of the Creative Commons Attribution License (CC BY). The use, distribution or reproduction in other forums is permitted, provided the original author(s) and the copyright owner(s) are credited and that the original publication in this journal is cited, in accordance with accepted academic practice. No use, distribution or reproduction is permitted which does not comply with these terms.
*Correspondence: Sung Joon Lee, joon617@kangwon.ac.kr; orcid.org/0000-0002-6451-0400
†These authors have contributed equally to this work