- 1Key Laboratory of Integrated Pest Management of Crop in Central China, Ministry of Agriculture, Hubei Key Laboratory of Crop Diseases, Insect Pests and Weeds Control, Institute of Plant Protection and Soil Fertility, Hubei Academy of Agricultural Sciences, Wuhan, China
- 2Institute of Chinese Herbal Medicines, Hubei Academy of Agricultural Sciences, Enshi, China
- 3The Sainsbury Laboratory, Norwich Research Park, Norwich, United Kingdom
- 4State Key Laboratory for Rice Biology, Institute of Biotechnology, Zhejiang University, Hangzhou, China
The discovery of natural bioactive compounds from endophytes or medicinal plants against plant diseases is an attractive option for reducing the use of chemical fungicides. In this study, three compounds, indole-3-carbaldehyde, indole-3-carboxylic acid (3-ICA), and jasmonic acid (JA), were isolated from the EtOAc extract of the culture filtrate of the endophytic fungus Lasiodiplodia pseudotheobromae LPS-1, which was previously isolated from the medicinal plant, Ilex cornuta. Some experiments were conducted to further determine the antifungal activity of these compounds on wheat powdery mildew. The results showed that JA was much more bioactive than indole-3-carbaldehyde and 3-ICA against Blumeria graminis, and the disease severity caused by B. graminis decreased significantly with the concentration increase of JA treatment. The assay of the interaction of 3-ICA and JA indicated that there was a significant synergistic effect between the two compounds on B. graminis in each of the ratios of 3-ICA to JA (3-ICA:JA) ranging from 1:9 to 9:1. When the compound ratio of 3-ICA to JA was 2:8, the synergistic coefficient was the highest as 22.95. Meanwhile, a histological investigation indicated that, under the treatment of JA at 500 μg/ml or 3-ICA:JA (2:8) at 40 μg/ml, the appressorium development and haustorium formation of B. graminis were significantly inhibited. Taken together, we concluded that JA plays an important role in the infection process of B. graminis and that 3-ICA as a synergist of JA enhances the antagonism against wheat powdery mildew.
Introduction
Powdery mildews (Ascomycota) encompass a category of widespread fungal pathogens that negatively impact a broad range of crops (Glawe, 2008). These fungi are obligate biotrophs, being completely dependent on living tissue for life, and are often host specific, associating with only one or a few species. For example, Blumeria graminis forma specialis tritici (Bgt) is known to be specific to wheat (Triticum aestivum) (Dean et al., 2012; Manser et al., 2021). Although B. graminis f. sp. tritici–resistant wheat cultivars have been developed, complete control of the disease has not been demonstrated and exogenous fungicide application is still necessary (Yang et al., 2008). Unfortunately, decades of application of agrochemical fungicides not only have produced pervasive environmental pollution but also have increased fungicide tolerance among fungal pathogen populations (Gong et al., 2013; Yang et al., 2013). An emerging alternative to the continued overuse of agrochemical fungicides is the adoption of naturally produced molecules, particularly those produced by plant endophytic microbes (Aly et al., 2010; Kharwar et al., 2011).
Endophytic microorganisms, both bacteria and fungi, carry out some part of their life cycle within living plant tissue and cause no disease symptoms (Tan and Zou, 2001; Rodriguez et al., 2009). Although most endophytes are of exogenous environmental original, some may be transmitted vertically in seeds or other generative tissue (Siegel et al., 1995; Rodriguez et al., 2009; Wei et al., 2014). Many endophytes form mutualistic relationships with their hosts, often considerably enhancing growth, defense, and adaptation to stress (Tan and Zou, 2001; Schardl et al., 2004; Rosenblueth and Martínez-Romero, 2006). In addition, endophytes may synthesize their own biologically active products, which can be applied as biocontrol agents either directly or indirectly, to stimulate induced resistance (Staniek et al., 2008; Aly et al., 2010; Kharwar et al., 2011; Vieira et al., 2014). Specifically, in some cases, the presence of endophytic microbes can produce amounts of bioactive secondary metabolites, including hormones, autocoids, and defense-related substances (Tudzynski, 1997; Pirttilä et al., 2004; Tanaka et al., 2005).
Endophytic microbes gained some notability in 1993 with the isolation of taxol, a multifunctional alkaloid, from the endophytic fungus Taxomyces andreanae, although endophytes have been mentioned in the literature since at least 1904 (Stierle et al., 1993; Qian et al., 2014). Since then, several other important compounds have been discovered to be produced by endophytic microbes, including isocoumarin (Findlay et al., 1995), camptothecin (Puri et al., 2005), podophyllotoxin (Eyberger et al., 2006), cochlioquinone A and isocochlioquinone A (Campos et al., 2008), and others (Schulz et al., 2002; Aly et al., 2010; Kharwar et al., 2011; Alvin et al., 2014). In Lasiodiplodia pseudotheobromae F2, isolated from Illigera rhodantha (Hernandiaceae) flower, six sulfureous diketopiperazines, lasiodiplines A–F, were characterized, and lasiodipline E was a potent antibacterial compound against the clinical strains Streptococcus sp., Peptostreptococcus sp., Bacteroides vulgates, and Veillonella parvula, respectively (Wei et al., 2014). In L. pseudotheobromae #1048 AMSTITYEL, isolated from stem of Aegle marmelos, two new compounds, lasdiplactone and lasdiploic acid, were isolated, which showed high xanthine oxidase inhibitory activity. In addition, the endophytic fungus #1048 AMSTITYEL showed maximum in vitro proteolytic and fibrinolytic activity (Meshram and Saxena, 2016; Kumar et al., 2019). In L. pseudotheobromae FKI-4499, isolated from soil collected in Okinawa Prefecture, Japan, aldsulfin, an anti-mannheimiosis agent, was identified, which displayed antibacterial activity against Mannheimia haemolytica and Pasteurella multocida (Sakai et al., 2021). In L. pseudotheobromae IBRL OS-64, isolated from the leaf of Ocimum sanctum, the fungal extract displayed significant antibacterial and anti-biofilm activities against methicillin-resistant Staphylococcus aureus (MRSA) and could be a candidate for antibacterial and antibiofilm drugs (Jalil and Ibrahim, 2021). In L. pseudotheobromae C1136, isolated from Tridax procumbens leaves, the active metabolites produced by the bioherbicidal isolates have bioherbicidal activity against Amaranthus hybridus L. and Echinochloa crus-galli weeds, and rhamnolipid, a biosurfactant produced by the bacterial Pseudomonas aeruginosa C1501, can serve as an adjuvant to improve the penetrability of bioherbicide active components from C1136 for controlling weeds (Adetunji and Oloke, 2013; Adetunji et al., 2017; Adetunji et al., 2018; Adetunji et al., 2020). However, the endophytic L. pseudotheobromae species have not been used in controlling agricultural diseases. Although natural products produced by endophytes are biodegradable, have low environmental toxicity, and show broad-spectrum bioactivity, these microorganisms remain an underutilized resource (Gunatilaka, 2006; Suryanarayanan et al., 2012). Such a class of safe, effective, and environment-friendly biocontrol agents would be particularly well suited for modern integrated management programs, aimed at reducing agrochemical use (Balandrin et al., 1985; Yang et al., 2008).
In our previous work, we isolated the endophytic fungus L. pseudotheobromae LPS-1 from the medicinal plant Ilex cornuta and found that the culture filtrate of the fungal isolate controlled Bgt infection more effectively than the broad-spectrum fungicide triadimefon (10 μg/ml) (Xiang et al., 2016). In this study, we sought to isolate and identify the specific secondary metabolites produced by L. pseudotheobromae LPS-1 that are antagonistic toward B. graminis on wheat. Here, we report the isolation and identification of three such compounds: indole-3-carbaldehyde (A2-5-6-1), indole-3-carboxylic acid (3-ICA; A2-5-6-2), and jasmonic acid (JA; A2-5-6-3). We further demonstrated that JA is antagonistic to appressorium development and haustorium formation during the B. graminis infection process, and 3-ICA synergistically enhances the antagonism of JA against B. graminis on wheat.
Materials and Methods
Plant Preparation, Fungal Strains, and Culture Conditions
Seeds of the powdery mildew disease-susceptible wheat variety “Zheng 98” were planted in 20-cm-diameter plastic pots, at a density of 10 plants per pot, and grew the seedlings out in a growth chamber for 10 days at 70% relative humidity, 18 ± 1°C, and under constant light (72 μmol m−2 s−1) conditions.
The B. graminis isolate E21 was obtained from Yilin Zhou of the Institute of Plant Protection (IPP), Chinese Academy of Agricultural Sciences (CAAS). Prior to inoculation of wheat plants, conidia were induced on excised segments of wheat leaf in a growth chamber for 10 days at 18 ± 1°C and under constant light (72 μmol m−2 s−1) conditions. L. pseudotheobromae LPS-1 was previously isolated from the medicinal plant, Ilex cornuta, at the Wuhan Botanical Garden in China. The internal transcribed spacer (ITS) and translation elongation factor 1α (TEF-1α) sequences were uploaded to GenBank under accession numbers KU180477 and KU180478, respectively (Xiang et al., 2016). LPS-1 was subcultured on potato dextrose agar (PDA) plates and then incubated at 25 ± 1°C for 3 days.
Collection of LPS-1 Culture Filtrate
To collect the bioactive compounds produced by LPS-1, 300 ml of pre-sterilized potato dextrose broth (PDB) was poured into a 1-L Erlenmeyer flask and was aseptically inoculated with three 6-mm LPS-1 mycelial plugs and statically incubated at 25 ± 1°C for 7 days. When incubation was finished, the mycelial material was removed from the broth by Whatman paper filtration (Z240567, Sigma Aldrich, USA). The remaining broth was centrifuged for 10 min at 12,000 rpm. A total of 120 L of culture filtrate was collected for analysis.
Compound Extraction and Isolation
The culture filtrate (120 L) was evaporated to extractum under reduced pressure at 50°C and extracted with petroleum ether and ethyl acetate (EtOAc) successively. A total of 35.7 g of petroleum ether extract and 197.2 g of EtOAc extract were obtained. The bioactivity of the aqueous phase and the above two extracts against Bgt E21 was tested, and it was found that the EtOAc extract had the highest biological activity and was thus chosen for further isolation and purification. The EtOAc extract (197.2 g) was dissolved in EtOAc, mixed with 100 mesh silica gel, and loaded onto a silica gel (5 cm × 10 cm, 300–400 mesh) chromatography column eluted with a gradient of petroleum ether/EtOAc (v/v: 50:1, 20:1, 10:1, 5:1, and 1:1) based on F254 silica gel thin-layer chromatography (TLC) monitoring. The same components were combined and concentrated to extractum under reduced pressure, and 10 fractions were obtained (A1~A10). The fraction A2, with the highest bioactivity against Bgt E21, was separated on thin silica gel H (5 cm × 30 cm) eluted with a gradient of petroleum ether/acetone first (v/v: 5:1 and 3:1) and then with a gradient of dichloromethane/methanol (CH2Cl2/MeOH) (v/v: 50:1, 30:1, 20:1, and 10:1) elution based on F254 silica gel TLC monitoring, obtaining 11 subfractions (A2-1~A2-11). The subfraction A2-5, with the highest bioactivity against Bgt E21, was further isolated by Sephadex LH-20 column (5 cm × 100 cm) eluted with MeOH based on F254 silica gel TLC monitoring, obtaining 12 subfractions (A2-5-1~A2-5-12). The subfraction A2-5-6, with the highest bioactivity against Bgt E21, was purified by high-performance liquid chromatography (HPLC) using a C18 column (2 cm × 25 cm; YMC Co., Ltd., Japan), yielding three compounds: A2-5-6-1 (19.2 mg), A2-5-6-2 (13.3 mg), and A2-5-6-3 (18.1 mg). A MeOH-H2O mixture was used as the mobile phase, and the flow rate was 10 ml/min. The UV detector was set to 220 nm. Purified compounds were identified as indole-3-carbaldehyde (A2-5-6-1), 3-ICA (A2-5-6-2), and JA (A2-5-6-3) using mass spectrometry (MS) and 1H and 13C nuclear magnetic resonance (NMR) (Table 1).
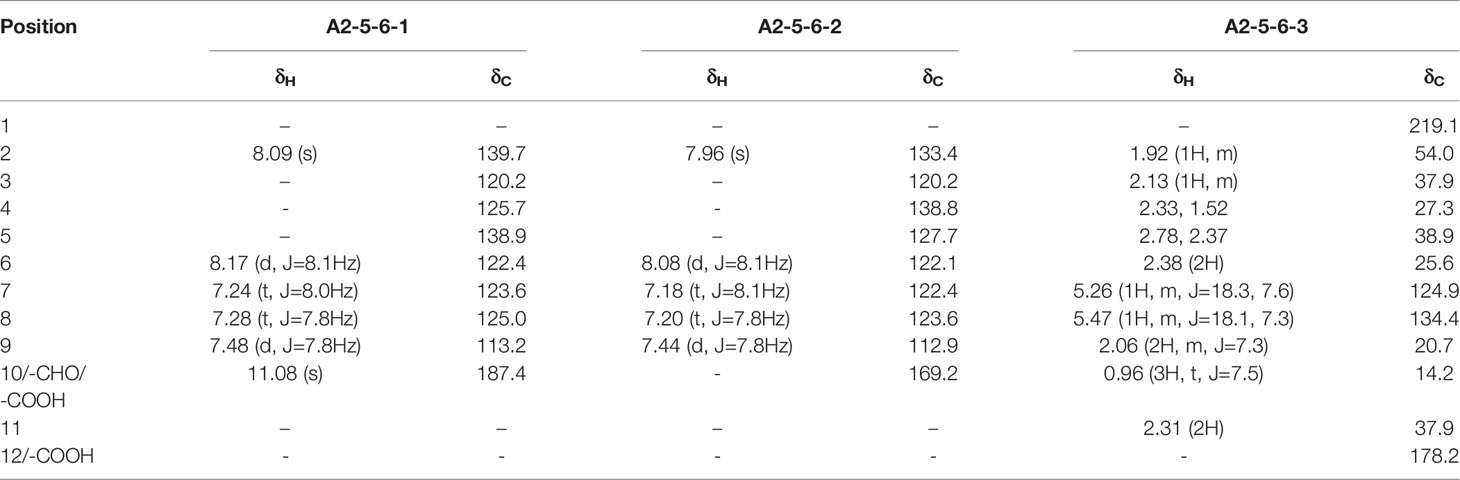
Table 1 1H (150 MHz, CDCl3) and 13C NMR (150 MHz, CDCl3) spectroscopic data of A2-5-6-1, A2-5-6-2, and A2-5-6-3.
Histological Investigation and Disease Severity Determination
To test their ability to control powdery mildew disease, the 3-ICA solution, the JA solution, and the 2:8 combination of 3-ICA:JA were applied to the wheat plants at the two-leaf stage with an automatic sprayer (PDE0012, Burkard Scientific Ltd., UK), with a spray volume of 350 ml/m2 and a pressure of 0.25 MPa. Treated wheat plants were air dried for 8 h and then inoculated with Bgt E21 at a density of 2 to 4 × 103 conidia/cm2. Inoculated plants were incubated in a growth chamber at 18 ± 1°C, with the first 12 h in total darkness and thereafter in constant light (72 μmol m−2 s−1) conditions. When the incubation period was complete, plants were divided into two sets, either for histological examination of the Bgt E21 infection process or for assessment of powdery mildew disease severity. All experiments were repeated three times and utilized a randomized design with 12 replications.
For histological examination, leaf segments (3 cm) were excised from the midsection of primary leaves and subsequently stained with alcoholic lactophenol trypan blue (Koch and Slusarenko, 1990). At 8, 24, and 48 h post-inoculation (hpi), leaf segments were examined at magnification of ×10, and both ungerminated conidia and germinated conidia with primary germ tubes (with either normal or deformed appressoria) were counted within 10 haphazardly chosen fields. Meanwhile, the number of primary or mature haustoria per Bgt E21 colony was randomly scored from 30 colonies. To assess the disease severity of wheat powdery mildew, the number of Bgt E21 colonies presented on each leaf was counted at 7 days post-inoculation (dpi). The formula is as follows: Disease severity (%) = (diseased leaf area)/(total leaf area) × 100.
Statistics and Assessment of Synergism
Dose-response regression was done by SPSS 22.0. The half maximal effective concentrations (EC50) of 3-ICA, JA, and their combinations were calculated according to the method of Gisi et al. (1985). Briefly, the calculated theoretical EC50 (EC50(th)) = (a + b)/(a/EC50(A) + b/EC50(B)), where A and B represent 3-ICA and JA, respectively, and a and b are the ratios of the two compounds in the combinations. To categorize the type of interaction between 3-ICA and JA, we utilized the following ratio: R = EC50(th)/EC50(ob), where EC50(ob) is the EC50 value calculated according to the observed data. An additive response is indicated by an R-value between 0.5 and 1.5, a synergistic response is indicated by an R-value of greater than 1.5, and an antagonistic response is indicated by an R-value of less than 0.5. Treatment means were compared using the Duncan’s multiple range test, with significance set at the P = 0.05 level.
Results
Isolation of the Indole-3-Carbaldehyde, Indole-3-Carboxylic Acid, and Jasmonic Acid From LPS-1
Our previous work found that the culture filtrate of the endophytic fungus L. pseudotheobromae LPS-1 provided control of Bgt infection more effectively than the commercially available fungicide triadimefon (10 μg/ml) (Xiang et al., 2016). Therefore, to determine which secondary metabolites play a major role in antagonizing wheat powdery mildew, the compounds produced by the strain LPS-1 cultured in PDB broth for 7 days were isolated and identified. Three compounds (A2-5-6-1, A2-5-6-2, and A2-5-6-3) with relatively high concentrations were isolated from the ethyl acetate (EtOAc) extract using silica gel column chromatography as detailed in the experimental methods and were identified as indole-3-carbaldehyde (Figure 1A), 3-ICA (Figure 1B), and JA (Figure 1C). The MS, 1H NMR, and 13C NMR data (Table 1) were further compared to the literature, to confirm out results.
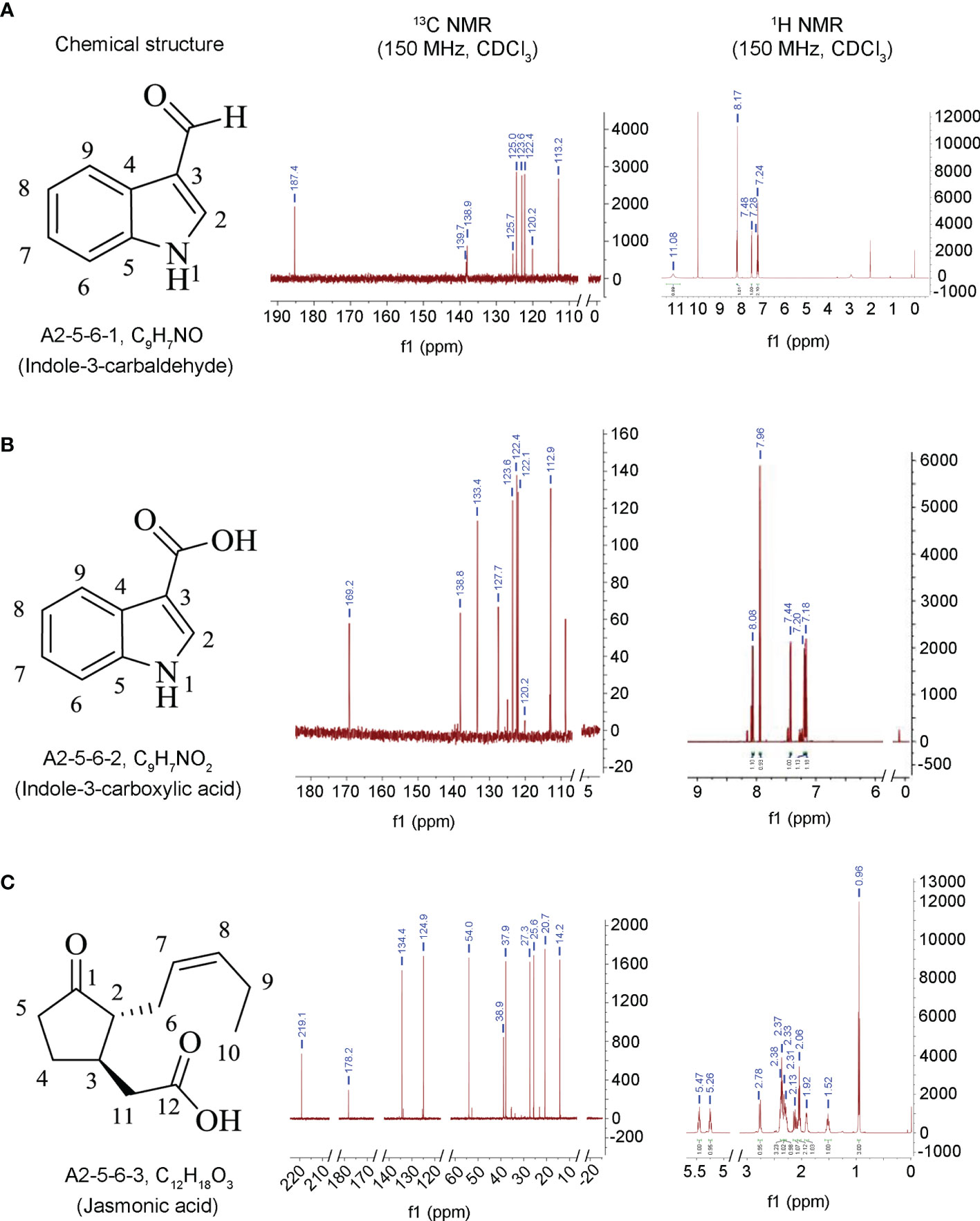
Figure 1 Chemical structure and NMR graphs of these compounds isolated from fermentation cultures of L. pseudotheobromae LPS-1. (A) A2-5-6-1, C9H7NO, indole-3-carbaldehyde. (B) A2-5-6-2, C9H7NO2, indole-3-carboxylic acid. (C) A2-5-6-3, C12H18O3, jasmonic acid.
Compound A2-5-6-1 was obtained as white powder, and the molecular formula was determined as C9H7NO. 1H NMR (150 MHz, CDCl3): δH 8.09 (s), 8.17 (d, J = 8.1Hz), 7.24 (t, J = 8.0Hz), 7.28 (t, J = 7.8Hz), 7.48 (d, J = 7.8Hz), 11.08 (s). 13C NMR (150 MHz, CDCl3): δC 139.7, 120.2, 125.7, 138.9, 113.2, 122.4, 123.6, 125.0, 187.4 (Table 1). The spectral data were similar to that reported in the literature (Burton et al., 1986; Qian et al., 2014), and the compound was identified as indole-3-carbaldehyde (Figure 1A).
Compound A2-5-6-2 was also obtained as white powder, and the molecular formula was determined as C9H7NO2. 1H NMR (150 MHz, CDCl3): δH 7.96 (s), 8.08 (d, J = 8.1Hz), 7.18 (t, J = 8.1Hz), 7.20 (t, J=7.8Hz), 7.44 (d, J = 7.8Hz). 13C NMR (150 MHz, CDCl3): δC 133.4, 120.2, 127.7, 138.8, 112.9, 122.1, 122.4, 123.6, 169.2 (Table 1). The spectral data were similar to that reported in the literature (Burton et al., 1986; Qian et al., 2014), and the compound was identified as 3-ICA (Figure 1B).
Compound A2-5-6-3 was also obtained as white powder, and the molecular formula was determined as C12H18O3. 1H NMR (150 MHz, CDCl3): δH 1.92 (1H, m), 2.13 (1H, m), 2.33, 1.52, 2.78, 2.37, 2.38 (2H), 5.26 (1H, m, J = 18.3, 7.6), 5.47 (1H, m, J = 18.1, 7.3), 2.06 (2H, m, J = 7.3), 0.96 (3H, t, J = 7.5), 2.31 (2H). 13C NMR (150 MHz, CDCl3): δC 219.1, 54.0, 37.9, 27.3, 38.9, 25.6, 124.9, 134.4, 20.7, 14.2, 37.9, 178.2 (Table 1). The spectral data were similar to that reported in the literature (Husain et al., 1993), and the compound was identified as JA (Figure 1C).
Disease Severity Determination
To further determine the antifungal activity of indole-3-carbaldehyde, 3-ICA, and JA against wheat powdery mildew, we tested the bioactivity of indole-3-carbaldehyde (with a concentration gradient of 2,500, 1,250, 625, 312.5, and 156.25 μg/ml), 3-ICA (with a concentration gradient of 2,500, 1,250, 625, 312.5, and 156.25 μg/ml), and JA (with a concentration gradient of 500, 250, 125, 62.5, and 31.25 μg/ml) against Bgt E21, respectively. Interestingly, there was no significant difference found in either disease severity or inhibition rate between either the indole-3-carbaldehyde or the 3-ICA treatments and the untreated control group (Mock). However, JA was much more bioactive than indole-3-carbaldehyde and 3-ICA against Bgt E21, and the disease severity decreased significantly, and inhibition rate increased significantly, with increasing JA concentration. When JA at 500 μg/ml was applied, the disease severity was 2.67 ± 0.53% and the inhibition rate was 96.95 ± 0.66% (Table 2), indicating that JA plays an important role in the infection process of wheat powdery mildew.
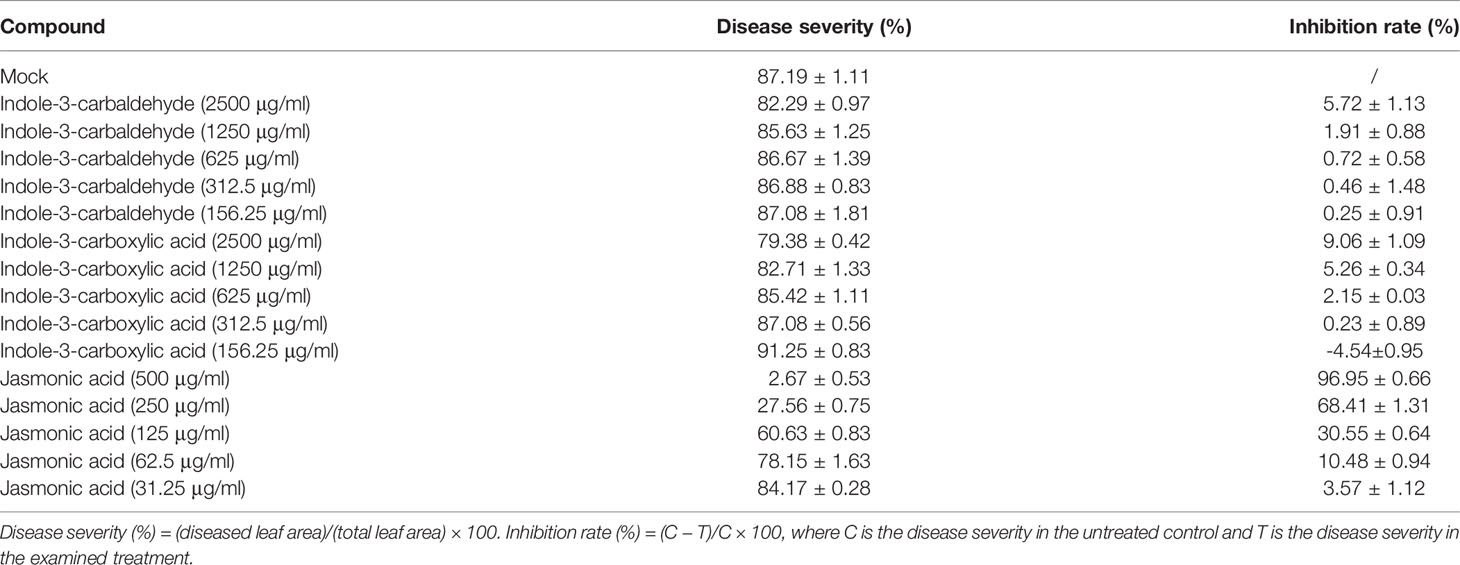
Table 2 The activities of indole-3-carbaldehyde, indole-3-carboxylic acid, and jasmonic acid against wheat powdery mildew in the laboratory.
Work by others has indicated that 3-ICA can be used as an antibiotic adjuvant of doxycycline toward a range of Gram-negative bacteria (Cadelis et al., 2021). Therefore, we wondered whether 3-ICA may interact with JA as an adjuvant to improve its bioactivity against wheat powdery mildew. We combined indole-3-carbaldehyde or 3-ICA with JA, and the compound ratio of indole-3-carbaldehyde in combination with JA (indole-3-carbaldehyde:JA) or 3-ICA in combination with JA (3-ICA:JA) was both 1:9, 2:8, 3:7, 4:6, 5:5, 6:4, 7:3, 8:2, and 9:1. Our results showed that the R-value (synergistic coefficient: theoretical EC50/observed EC50) of the combinations of 3-ICA and JA ranged from 2.1 to 22.95 for wheat powdery mildew. There was a synergistic effect when the combinations of the two compounds were at each ratio of 1:9-9:1 on this disease. Specifically, when 3-ICA and JA were combined in a ratio of 2:8, the EC50 was the lowest, at 9.05 μg/ml, and the synergistic coefficient was the highest, at 22.95 (Table 3). However, indole-3-carbaldehyde combined with JA did not produce significant synergism (Table S1). Meanwhile, we further tested the antagonism against Bgt E21 using the final concentration of 3-ICA at 2,500 μg/ml, JA at 500 μg/ml, and 3-ICA:JA (2:8) at 40 μg/ml, respectively. We found that Bgt E21 caused severe infection on susceptible wheat leaves in both the untreated control (Mock) and 3-ICA treatments. However, there were no visible disease symptoms observed on susceptible wheat leaves under the treatment of JA or 3-ICA:JA (2:8) (Figure 2). Overall, it appears that 3-ICA as a synergist of JA enhances the antagonism against wheat powdery mildew.
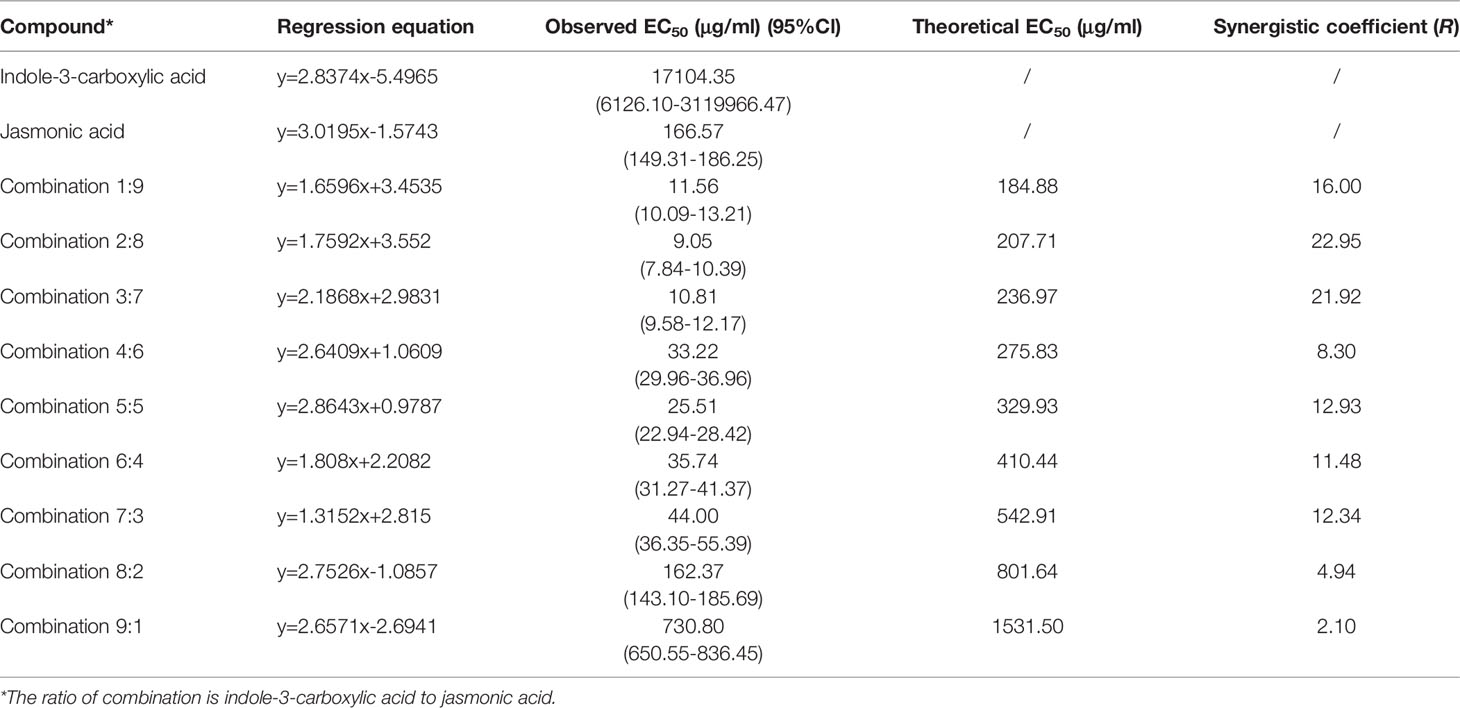
Table 3 The activities of indole-3-carboxylic acid, jasmonic acid, and their combinations on wheat powdery mildew in the laboratory.
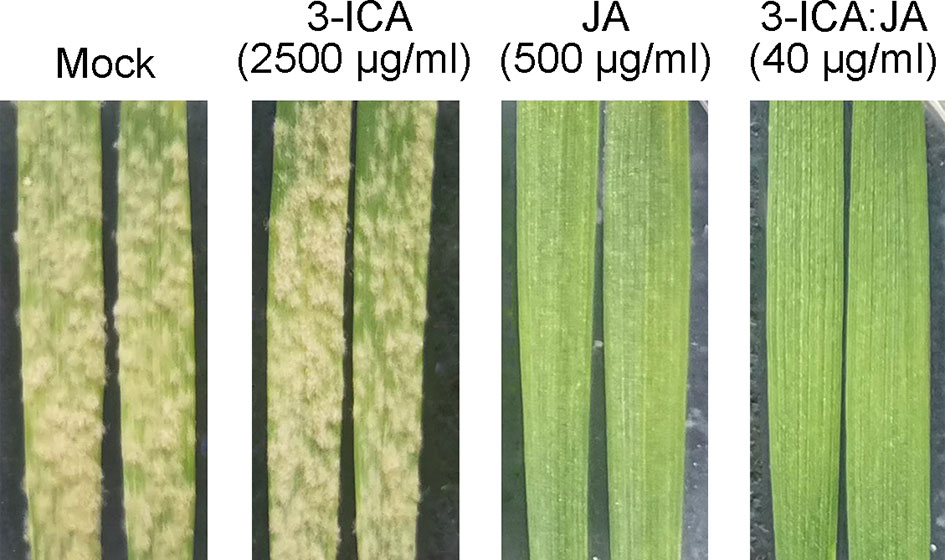
Figure 2 The activity of 3-ICA, JA, and their combinations against wheat powdery mildew at 2,500, 500, and 40 μg/ml, respectively. Mock, untreated control; 3-ICA, indole-3-carboxylic acid; JA, jasmonic acid; 3-ICA:JA, the 2:8 combination of indole-3-carboxylic acid and jasmonic acid.
Histological Investigation of B. graminis Infection
To determine the roles of 3-ICA and JA in the Bgt E21 infection process, we treated wheat leaves with either 3-ICA at 2,500 μg/ml, JA at 500 μg/ml, or 3-ICA : JA (2:8) at 40 μg/ml, 8 h prior to inoculation. The results showed that, in the untreated control (Mock) and 3-ICA treatment, wheat leaves were successfully infected and colonized by Bgt E21. By 8 hpi, appressoria were formed from germinated conidia. Primary haustoria were formed by 24 hpi and matured by 48 hpi, and then, secondary haustoria and hypha were formed by 72 hpi. The base of conidiophores emerged by 96 hpi and abundant fresh conidia reproduced by 120 hpi. However, in both the JA and 3-ICA:JA (2:8) treatments, the germinated conidia did not form normal appressoria, and the percentages of deformed appressoria were significantly higher (JA, 90.53 ± 0.91%; 3-ICA:JA, 89.68 ± 1.36%) than either the 3-ICA (5.85 ± 1.14%) or untreated control (6.79 ± 1.62%) treatments. Furthermore, the deformed appressoria failed to penetrate the host cells, and no haustoria were formed (Figure 3; Table 4). Meanwhile, at 24 hpi, conidia germinated at a rate of 83.47 ± 1.85% in the JA treatment, 83.44 ± 1.53% in the 3-ICA : JA treatment, 84.24 ± 1.65% in the 3-ICA treatment, and 86.43 ± 1.69% in the untreated control. In addition, at 24 hpi, appressoria were formed at a rate of 90.73 ± 0.78% in the JA treatment, 90.38 ± 1.85% in the 3-ICA:JA treatment, 91.22 ± 1.58% in the 3-ICA treatment, and 92.83 ± 0.60% in the untreated control. Overall, we found no significant difference in either the conidia germination rate or appressorium formation rate between the 3-ICA, JA, or 3-ICA : JA treatment and the untreated control (Table 4). These data suggest that JA plays important roles in appressorium development and haustorium formation against B. graminis.
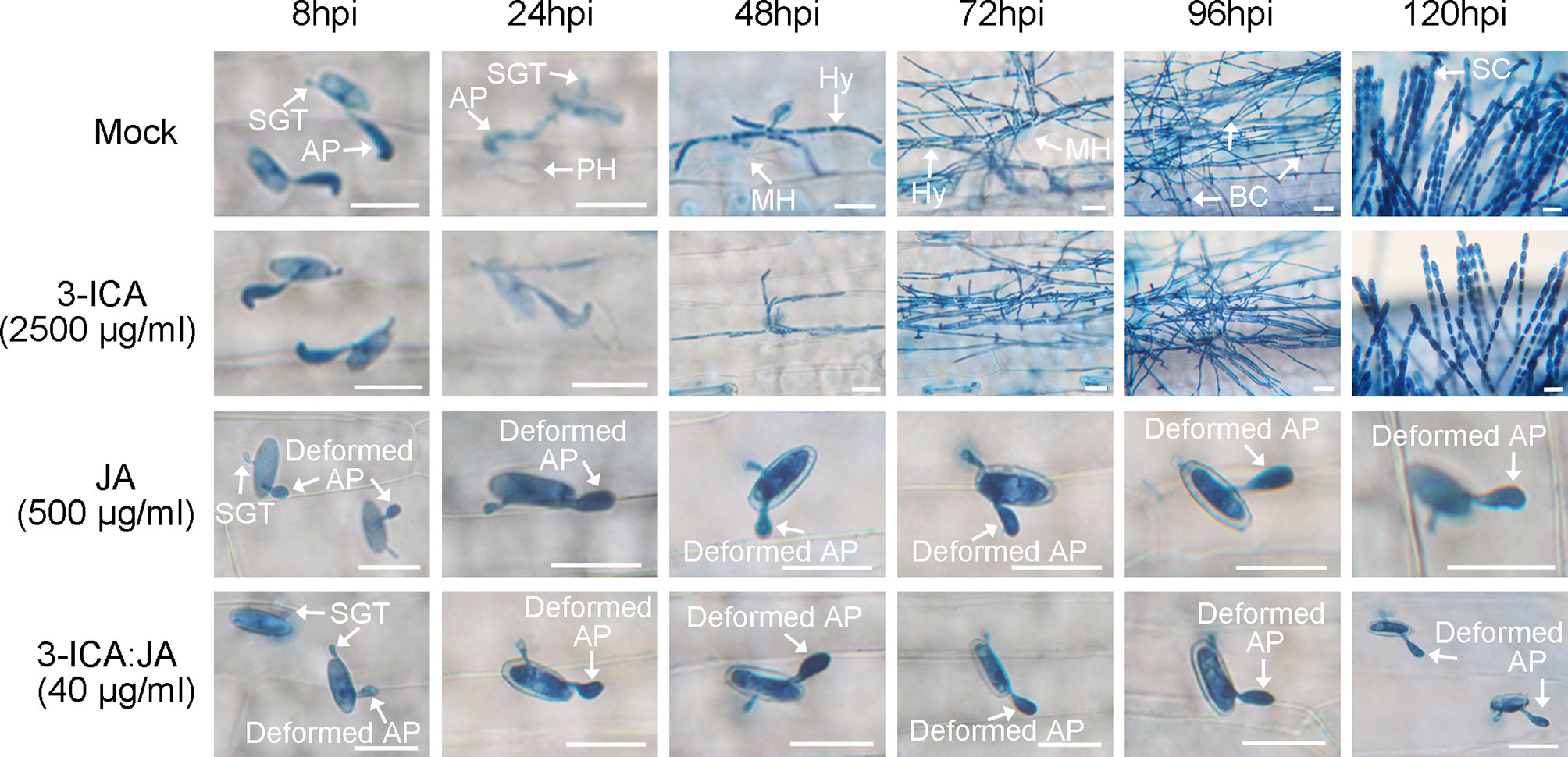
Figure 3 The development of B graminis on wheat leaves at different time courses in 3-ICA, JA, and their combination treatment. Mock, untreated control; 3-ICA, indole-3-carboxylic acid at 2,500 μg/ml; JA, jasmonic acid at 500 μg/ml; 3-ICA:JA, the 2:8 combination of indole-3-carboxylic acid and jasmonic acid at 40 μg/ml. Sampling time was 8, 24, 48, 72, 96, and 120 hpi, respectively. SGT, sticky germ tube; AP, appressorium; PH, primary haustorium; MH, mature haustorium; Hy, hypha; BC, base of conidiophores; SC, spore chain. The deformed appressoria failed to penetrate the host cells. Scale bar = 20 μm.
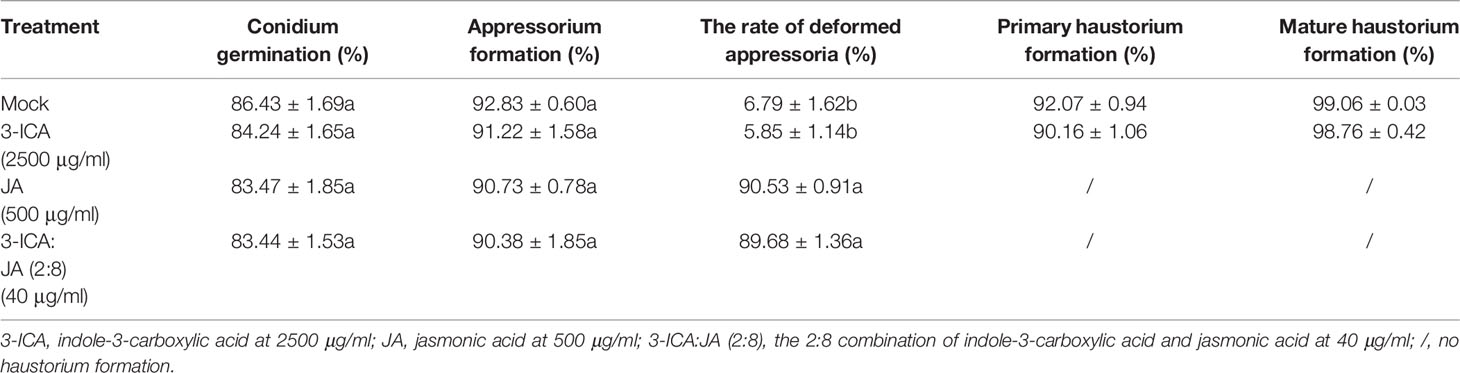
Table 4 Histological investigation of the infection process of B. graminis after treatment with 3-ICA, JA, and their combinations.
Discussion
In this study, the endophytic fungus L. pseudotheobromae LPS-1 isolated from the medicinal plant, Ilex cornuta, can produce three agriculturally relevant bioactive compounds: indole-3-carbaldehyde, 3-ICA, and JA. Among them, both indole-3-carbaldehyde and 3-ICA are commonly present in microorganisms and plants and present antimicrobial and antitumor activities (Yue et al., 2000; Anderton et al., 2004; Kavitha et al., 2010; Mujahid et al., 2011; Qian et al., 2014; Liu et al., 2017; Zhou et al., 2019; Duong et al., 2021). Some studies have showed that indole-3-carbaldehyde, isolated from Pseudomonas sp. ST4, Streptomyces sp. CT37, Aeromicrobium ponti LGMB491, Janthinobacterium lividum, Clitocybe nuda LA82, Angelica sinensis callus, and Marinomonas sp., has inhibitory effects on the growth of Sporisorium scitamineum, Ustilago maydis, Candida albicans ATCC 10231, Staphylococcus aureus, and Batrachochytrium dendrobatidis JEL215, on zoospore germination of Phytophthora capsici PCM81, on neuroinflammation, and on the biofilm formation of Vibrio cholerae O1, respectively (Brucker et al., 2008; Chen et al., 2012; Rajalaxmi et al., 2016; Gos et al., 2017; Zhou et al., 2017; Liu et al., 2020; Fang et al., 2020). However, other reports about indole-3-carbaldehyde did not indicate its antimicrobial activity against pathogens. In this study, we also demonstrated that indole-3-carbaldehyde has no antifungal activity against Bgt E21 (Table 2). This indicates that indole-3-carbaldehyde may exhibit different extents of antimicrobial activity against different pathogens. Therefore, whether indole-3-carbaldehyde, isolated from L. pseudotheobromae LPS-1, has antimicrobial activity against other plant pathogens will be investigated in the future. For 3-ICA, in plants, it is regarded as an inactive auxin (IAA; indole-3-acetic acid) analog, and IAA is also implicated in plant defense (Bari and Jones, 2009; Borgati and Boaventura, 2011; Petti et al., 2012). Some studies indicate that 3-ICA, a plant cell wall–bound metabolite that could mediate accelerating callose accumulation in response to pathogens, may perform important functions as a mediator of induced resistance in plant basal defense against biotic stress. However, its function may be regulatory or signaling because it has no direct antifungal effect on pathogens (Forcat et al., 2010; Gamir et al., 2012; Iven et al., 2012; Gamir et al., 2014a; Gamir et al., 2014b; Frerigmann et al., 2016; Gamir et al., 2018; Pastor-Fernández et al., 2019). Similarly, in this study, we also found that there was no significant difference in disease severity and pathogenicity of Bgt E21 on susceptible wheat leaves between 3-ICA treatment and the untreated group (Mock) (Figure 2; Table 2), which preliminarily indicated that 3-ICA had no inhibitory effect on the infection process of wheat powdery mildew. Contrarily, JA was much more bioactive than indole-3-carbaldehyde and 3-ICA against Bgt E21, and the disease severity of Bgt E21 decreased significantly with the concentration increase of JA treatment (Table 2). In plants, the plant hormone JA and its derivatives are involved in regulating a diverse set of processes including cellular development, defense, and resistance to both abiotic and biotic stress (Ruan et al., 2019; Gomi, 2021). For instance, when applied to plants, the JA-derivative lasiojasmonate A (LasA), isolated from Lasiodiplodia mediterranea, a pathogen of grapes, induces many JA-regulated response in planta at both the genetic and physical level (Chini et al., 2018). The synthetic JA-Ile-macrolactone 5b acts to stimulate induced resistance against pests and pathogens in tea (Lin et al., 2020). In apple trees, JA has been shown to be more effective at stimulating induced resistance to Tetranychus urticae than the fungicide acibenzolar-S-methyl (BTH: benzothiadiazole) (Warabieda et al., 2020). Exogenous treatment with JA can enhance chilling tolerance of peach fruit and reduce the severity of internal flesh browning (Zhao et al., 2021). Some studies have also shown that JA signaling can induce the transcription of several defense-related transcription factors (TFs), such as MYC, MYB, NAC, ERF, and WRKY (Lorenzo et al., 2003; Delessert et al., 2005; Skibbe et al., 2008; Fernández-Calvo et al., 2011; Gao et al., 2011; Qi et al., 2011), and can also induce the MAP kinase cascade (Li et al., 2017), calcium channel activation (Kenton et al., 1999), and other processes that, along with other plant hormones like abscisic acid, salicylic acid, and ethylene, regulate plant growth, development, and stress response (Santner and Estelle, 2009; Ruan et al., 2019). JA is also present in other fungi in addition to L. pseudotheobromae LPS-1, such as Acremonium sp. D212, Botryodiplodia theobromae, L. theobromae strain 2334, and L. iranensis (Husain et al., 1993; Eng et al., 2016; Han et al., 2020; Shen et al., 2022). However, in this study, we do not know the molecular mechanism of 3-ICA and JA antagonizing B. graminis at present. Therefore, whether 3-ICA and JA served as signal molecules to induce the resistance of wheat against the infection of B. graminis will be further elucidated in the future.
Work by others has indicated that 3-ICA can be effectively employed as an antibiotic adjuvant of doxycycline against a range of Gram-negative bacteria (Cadelis et al., 2021). However, 3-ICA as a synergist of JA against plant pathogens has not been reported. Therefore, we wondered whether 3-ICA may interact with JA as an adjuvant to improve its bioactivity against fungal infection. We found that, in this study, there was indeed a synergistic effect between 3-ICA and JA against Bgt E21 on wheat in each of the ratios of 3-ICA to JA (3-ICA:JA) ranging from 1:9 to 9:1. When the compound ratio of 3-ICA to JA was 2:8, the synergistic coefficient was the highest as 22.95 (Table 3). Therefore, we chose the compound ratio of 3-ICA to JA as 2:8 for the following study. Similar to the treatment of JA at 500 μg/ml, there was no symptom on susceptible wheat leaves, and the appressorium and haustorium formation of Bgt E21 was significantly inhibited under the treatment of 3-ICA:JA (2:8) at 40 μg/ml (Figures 2, 3; Table 4). This indicated that treatment with 3-ICA:JA (2:8) at 40 μg/ml was just as affective at controlling Bgt E21 infection as treatment with JA alone at 500 μg/ml, a 12.5× greater concentration of JA. It appears that 3-ICA significantly enhances the antagonistic efficiency of JA against wheat powdery mildew. In addition, to avoid the possibility of pathogenic resistance, antibiotic adjuvants should preferably lack their own antibiotic activity (Bernal et al., 2013), and we found that 3-ICA alone showed no direct activity against Bgt E21. Thus, 3-ICA can be employed as a synergist of JA to enhance the antagonism against B. graminis, and we suggest that the combination of JA and 3-ICA is potentially suitable for modern integrated disease management programs, aimed at reducing agrochemical use. These data further evince the untapped capacity of endophytic microorganisms to contribute useful compounds to agriculture and beyond.
Data Availability Statement
The original contributions presented in the study are included in the article/Supplementary Material. Further inquiries can be directed to the corresponding authors.
Author Contributions
Conceived and designed the experiments: YQ, LY, and LX. Performed the experiments: YQ, DH, SG, XZ, BY, MX, WS, FZ, ML, TC, DY, LY, and LX. Analyzed the data: YQ, LY and LX. Wrote the paper: YQ, XY, ZW, and LX.
Funding
This work was supported by the Natural Science Foundation of China (Grant No. 31701834), the Natural Science Foundation of Hubei Province (Grant No. 2021CFB403), the China Agriculture Research System of MOF and MARA (Grant No. CARS-03), the Youth Science Foundation of Hubei Academy of Agricultural Sciences (Grant No. 2022NKYJJ08) and the Youth Science Foundation of Institute of Plant Protection and Soil Fertility, Hubei Academy of Agricultural Sciences (Grant No. 2021ZTSQJ13). The funders had no role in study design, data collection and analysis, decision to publish, or preparation of the manuscript.
Conflict of Interest
The authors declare that the research was conducted in the absence of any commercial or financial relationships that could be construed as a potential conflict of interest.
Publisher’s Note
All claims expressed in this article are solely those of the authors and do not necessarily represent those of their affiliated organizations, or those of the publisher, the editors and the reviewers. Any product that may be evaluated in this article, or claim that may be made by its manufacturer, is not guaranteed or endorsed by the publisher.
Acknowledgments
This work was supported by the Natural Science Foundation of China (Grant No. 31701834), the Natural Science Foundation of Hubei Province (Grant No. 2021CFB403), the China Agriculture Research System of MOF and MARA (Grant No. CARS-03), the Youth Science Foundation of Hubei Academy of Agricultural Sciences (Grant No. 2022NKYJJ08), and the Youth Science Foundation of Institute of Plant Protection and Soil Fertility, Hubei Academy of Agricultural Sciences (Grant No. 2021ZTSQJ13). The funders had no role in study design, data collection and analysis, decision to publish, or preparation of the manuscript.
Supplementary Material
The Supplementary Material for this article can be found online at: https://www.frontiersin.org/articles/10.3389/fcimb.2022.898500/full#supplementary-material
Supplementary Table 1 | The activities of indole-3-carbaldehyde, jasmonic acid, and their combinations on wheat powdery mildew in the laboratory.
References
Adetunji, C. O., Oloke, J. K. (2013). Efficacy of Freshly Prepared Pesta Granular Formulations From the Multi-Combination of Wild and Mutant Strain of Lasiodiplodia Pseudotheobromae and Pseudomonas Aeruginosa. Agric. Univ. Tirana 12 (4), 555–563.
Adetunji, C. O., Oloke, J. K., Kumar, A., Swaranjit, S., Akpor, B. (2017). Synergetic Effect of Rhamnolipid From Pseudomonas Aeruginosa C1501 and Phytotoxic Metabolite From Lasiodiplodia Pseudotheobromae C1136 on Amaranthus Hybridus L. And Echinochloa Crus-Galli Weeds. Environ. Sci. pollut. Res. Int. 24 (15), 13700–13709. doi: 10.1007/s11356-017-8983-8
Adetunji, C. O., Oloke, J. K., Osemwegie, O. O. (2018). Environmental Fate and Effects of Granular Pesta Formulation From Strains of Pseudomonas Aeruginosa C1501 and Lasiodiplodia Pseudotheobromae C1136 on Soil Activity and Weeds. Chemosphere 195, 98–107. doi: 10.1016/j.chemosphere.2017.12.056
Adetunji, C. O., Oloke, J. K., Phazang, P., Sarin, N. B. (2020). Influence of Eco-Friendly Phytotoxic Metabolites From Lasiodiplodia Pseudotheobromae C1136 on Physiological, Biochemical, and Ultrastructural Changes on Tested Weeds. Environ. Sci. pollut. Res. Int. 27 (9), 9919–9934. doi: 10.1007/s11356-020-07677-9
Alvin, A., Miller, K. I., Neilan, B. A. (2014). Exploring the Potential of Endophytes From Medicinal Plants as Sources of Antimycobacterial Compounds. Microbiol. Res. 169 (7-8), 483–495. doi: 10.1016/j.micres.2013.12.009
Aly, A. H., Debbab, A., Kjer, J., Proksch, P. (2010). Fungal Endophytes From Higher Plants: A Prolific Source of Phytochemicals and Other Bioactive Natural Products. Fungal Divers. 41 (1), 1–16. doi: 10.1007/s13225-010-0034-4
Anderton, M. J., Manson, M. M., Verschoyle, R. D., Gescher, A., Lamb, J. H., Farmer, P. B., et al. (2004). Pharmacokinetics and Tissue Disposition of Indole-3-Carbinol and its Acid Condensation Products After Oral Administration to Mice. Clin. Cancer Res. 10(15), 5233–5241. doi: 10.1158/1078-0432.CCR-04-0163
Balandrin, M. F., Klocke, J. A., Wurtele, E. S., Bollinger, W. H. (1985). Natural Plant Chemicals: Sources of Industrial and Medicinal Materials. Science 228 (4704), 1154–1160. doi: 10.1126/science.3890182
Bari, R., Jones, J. D. G. (2009). Role of Plant Hormones in Plant Defence Responses. Plant Mol. Biol. 69 (4), 473–488. doi: 10.1007/s11103-008-9435-0
Bernal, P., Molina-Santiago, C., Daddaoua, A., Llamas, M. A. (2013). Antibiotic Adjuvants: Identification and Clinical Use. Microb. Biotechnol. 6 (5), 445–449. doi: 10.1111/1751-7915.12044
Borgati, T. F., Boaventura, M. A. D. (2011). Effects of Indole Amides on Lettuce and Onion Germination and Growth. Z. Naturforsch. C. J. Biosci. 66 (9-10), 485–490. doi: 10.1515/znc-2011-9-1008
Brucker, R. M., Harris, R. N., Schwantes, C. R., Gallaher, T. N., Flaherty, D. C., Lam, B. A., et al. (2008). Amphibian Chemical Defense: Antifungal Metabolites of the Microsymbiont Janthinobacterium Lividum on the Salamander Plethodon Cinereus. J. Chem. Ecol. 34 (11), 1422–1429. doi: 10.1007/s10886-008-9555-7
Burton, G., Ghini, A. A., Gros, E. G. (1986). 13c NMR Spectra of Substituted Indoles. Ref. Data 24 (9), 829–831. doi: 10.1002/mrc.1260240919
Cadelis, M. M., Li, S. A., Bourguet-Kondracki, M. L., Blanchet, M., Douafer, H., Brunel, J. M., et al. (2021). Spermine Derivatives of Indole-3-Carboxylic Acid, Indole-3-Acetic Acid and Indole-3-Acrylic Acid as Gram-Negative Antibiotic Adjuvants. ChemMedChem 16 (3), 513–523. doi: 10.1002/cmdc.202000359
Campos, F. F., Rosa, L. H., Cota, B. B., Caligiorne, R. B., Rabello, A. L. T., Alves, T. M. A., et al. (2008). Leishmanicidal Metabolites From Cochliobolus Sp., an Endophytic Fungus Isolated From Piptadenia Adiantoides (Fabaceae). PloS Negl. Trop. Dis. 2 (12), e348. doi: 10.1371/journal.pntd.0000348
Chen, J., Su, H., Huang, J. (2012). Isolation and Identification of Secondary Metabolites of Clitocybe Nuda Responsible for Inhibition of Zoospore Germination of Phytophthora Capsici. J. Agric. Food Chem. 60 (30), 7341–7344. doi: 10.1021/jf301570y
Chini, A., Cimmino, A., Masi, M., Reveglia, P., Nocera, P., Solano, R., et al. (2018). The Fungal Phytotoxin Lasiojasmonate A Activates the Plant Jasmonic Acid Pathway. J. Exp. Bot. 69 (12), 3095–3102. doi: 10.1093/jxb/ery114
Dean, R., Van Kan, J. A. L., Pretorius, Z. A., Hammond-Kosack, K. E., Pietro, A. D., Spanu, P. D., et al. (2012). The Top 10 Fungal Pathogens in Molecular Plant Pathology. Mol. Plant Pathol. 13 (4), 414–430. doi: 10.1111/j.1364-3703.2011.00783.x
Delessert, C., Kazan, K., Wilson, I. W., Straeten, D. V. D., Manners, J., Dennis, E. S., et al. (2005). The Transcription Factor ATAF2 Represses the Expression of Pathogenesis-Related Genes in Arabidopsis. Plant J. 43 (5), 745–757. doi: 10.1111/j.1365-313X.2005.02488.x
Duong, T. T., Nguyen, T. T. L., Dinh, T. H. V., Hoang, T. Q., Vu, T. N., Doan, T. O., et al. (2021). Auxin Production of the Filamentous Cyanobacterial Planktothricoides Strain Isolated From a Polluted River in Vietnam. Chemosphere 284, 131242. doi: 10.1016/j.chemosphere.2021.131242
Eng, F., Haroth, S., Feussner, K., Meldau, D., Rekhter, D., Ischebeck, T., et al. (2016). Optimized Jasmonic Acid Production by Lasiodiplodia Theobromae Reveals Formation of Valuable Plant Secondary Metabolites. PloS One 11 (12), e0167627. doi: 10.1371/journal.pone.0167627
Eyberger, A. L., Dondapati, R., Porter, J. R. (2006). Endophyte Fungal Isolates From Podophyllum Peltatum Produce Podophyllotoxin. J. Nat. Prod 69 (8), 1121–1124. doi: 10.1021/np060174f
Fang, Q., Maglangit, F., Mugat, M., Urwald, C., Kyeremeh, K., Deng, H. (2020). Targeted Isolation of Indole Alkaloids From Streptomyces Sp. CT37. Molecules 25 (5), 1108. doi: 10.3390/molecules25051108
Fernández-Calvo, P., Chini, A., Fernández-Barbero, G., Chico, J. M., Gimenez-Ibanez, S., Geerinck, J., et al. (2011). The Arabidopsis bHLH Transcription Factors MYC3 and MYC4 Are Targets of JAZ Repressors and Act Additively With MYC2 in the Activation of Jasmonate Responses. Plant Cell 23 (2), 701–715. doi: 10.1105/tpc.110.080788
Findlay, J. A., Buthelezi, S., Lavoie, R., Peña-Rodriguez, L., Miller, J. D. (1995). Bioactive Isocoumarins and Related Metabolites From Conifer Endophytes. J. Nat. Prod 58 (11), 1759–1766. doi: 10.1021/np50125a021
Forcat, S., Bennett, M., Grant, M., Mansfield, J. W. (2010). Rapid Linkage of Indole Carboxylic Acid to the Plant Cell Wall Identified as a Component of Basal Defence in Arabidopsis Against Hrp Mutant Bacteria. Phytochemistry 71 (8-9), 870–876. doi: 10.1016/j.phytochem.2010.03.010
Frerigmann, H., Pislewska-Bednarek, M., Sánchez-Vallet, A., Molina, A., Glawischnig, E., Gigolashvili, T., et al. (2016). Regulation of Pathogen-Triggered Tryptophan Metabolism in Arabidopsis Thaliana by MYB Transcription Factors and Indole Glucosinolate Conversion Products. Mol. Plant 9 (5), 682–695. doi: 10.1016/j.molp.2016.01.006
Gamir, J., Pastor, V., Cerezo, M., Flors, V. (2012). Identification of Indole-3-Carboxylic Acid as Mediator of Priming Against Plectosphaerella Cucumerina. Plant Physiol. Biochem. 61, 169–179. doi: 10.1016/j.plaphy.2012.10.004
Gamir, J., Pastor, V., Kaever, A., Cerezo, M., Flors, V. (2014a). Targeting Novel Chemical and Constitutive Primed Metabolites Against Plectosphaerella Cucumerina. Plant J. 78 (2), 227–240. doi: 10.1111/tpj.12465
Gamir, J., Pastor, V., Sánchez-Bel, P., Agut, B., Mateu, D., García-Andrade, J., et al. (2018). Starch Degradation, Abscisic Acid and Vesicular Trafficking Are Important Elements in Callose Priming by Indole-3-Carboxylic Acid in Response to Plectosphaerella Cucumerina Infection. Plant J. 96 (3), 518–531. doi: 10.1111/tpj.14045
Gamir, J., Sánchez-Bel, P., Flors, V. (2014b). Molecular and Physiological Stages of Priming: How Plants Prepare for Environmental Challenges. Plant Cell Rep. 33 (12), 1935–1949. doi: 10.1007/s00299-014-1665-9
Gao, Q. M., Venugopal, S., Navarre, D., Kachroo, A. (2011). Low Oleic Acid-Derived Repression of Jasmonic Acid-Inducible Defense Responses Requires the WRKY50 and WRKY51 Proteins. Plant Physiol. 155 (1), 464–476. doi: 10.1104/pp.110.166876
Gisi, U., Binder, H., Rimbach, E. (1985). Synergistic Interactions of Fungicides With Different Mode of Action. Trans. Brit. Mycol. Soc. 85 (2), 299–306. doi: 10.1016/S0007-1536(85)80192-3
Glawe, D. A. (2008). The Powdery Mildews: A Review of the World’s Most Familiar (Yet Poorly Known) Plant Pathogens. Annu. Rev. Phytopathol. 46, 27–51. doi: 10.1146/annurev.phyto.46.081407.104740
Gomi, K. (2021). Jasmonic Acid Pathway in Plants 2.0. Int. J. Mol. Sci. 22(7), 3506–3508. doi: 10.3390/ijms22073506
Gong, S. J., Yang, L. J., Xiang, L. B., Zeng, F. S., Zhang, X. J., Xue, M. F., et al. (2013). Baseline Sensitivity of Blumeria Graminis F. Sp. Tritic to Metrafenone, Quinoxyfen and Bioactivities of These Fungicides. Chin. J. Pest. Sci. 15 (5), 511–515. doi: 10.3969/j.issn.1008-7303.2013.05.05
Gos, F. M. W. R., Savi, D. C., Shaaban, K. A., Thorson, J. S., Aluizio, R., Possiede, Y. M., et al. (2017). Antibacterial Activity of Endophytic Actinomycetes Isolated From the Medicinal Plant Vochysia Divergens (Pantanal, Brazil). Front. Microbiol. 8. doi: 10.3389/fmicb.2017.01642
Gunatilaka, A. L. (2006). Natural Products From Plant-Associated Microorganisms: Distribution, Structural Diversity, Bioactivity, and Implications of Their Occurrence. J. Nat. Prod 69 (3), 509–526. doi: 10.1021/np058128n
Han, L., Zhou, X., Zhao, Y. T., Zhu, S. S., Wu, L. X., He, Y. L., et al. (2020). Colonization of Endophyte Acremonium Sp. D212 in Panax Notoginseng and Rice Mediated by Auxin and Jasmonic Acid. J. Integr. Plant Biol. 62 (9), 1433–1451. doi: 10.1111/jipb.12905
Husain, A., Ahmad, A., Agrawal, P. K. (1993). (-)-Jasmonic Acid, a Phytotoxic Substance From Botryodiplodia Theobromae: Characterization by NMR Spectroscopic Methods. J. Nat. Prod 56 (11), 2008–2011. doi: 10.1021/np50101a025
Iven, T., König, S., Singh, S., Braus-Stromeyer, S. A., Bischoff, M., Tietze, L. F., et al. (2012). Transcriptional Activation and Production of Tryptophan-Derived Secondary Metabolites in Arabidopsis Roots Contributes to the Defense Against the Fungal Vascular Pathogen Verticillium Longisporum. Mol. Plant 5 (6), 1389–1402. doi: 10.1093/mp/sss044
Jalil, M. T. M., Ibrahim, D. (2021). Fungal Extract of Lasiodiplodia Pseudotheobromae IBRL OS-64 Inhibits the Growth of Skin Pathogenic Bacterium and Attenuates Biofilms of Methicillin-Resistant Staphylococcus Aureus. Malays. J. Med. Sci. 28 (4), 24–36. doi: 10.21315/mjms2021.28.4.4
Kavitha, A., Prabhakar, P., Vijayalakshmi, M., Venkateswarlu, Y. (2010). Purification and Biological Evaluation of the Metabolites Produced by Streptomyces Sp. TK-Vl_333. Res. Microbiol. 161 (5), 335–345. doi: 10.1016/j.resmic.2010.03.011
Kenton, P., Mur, L. A. J., Draper, J. (1999). A Requirement for Calcium and Protein Phosphatase in the Jasmonate-Induced Increase in Tobacco Leaf Acid Phosphatase Specific Activity. J. Exp. Bot. 50 (337), 1331–1341. doi: 10.1093/jexbot/50.337.1331
Kharwar, R. N., Mishra, A., Gond, S. K., Stierle, A., Stierle, D. (2011). Anticancer Compounds Derived From Fungal Endophytes: Their Importance and Future Challenges. Nat. Prod. Rep. 28 (7), 1208–1228. doi: 10.1039/c1np00008j
Koch, E., Slusarenko, A. (1990). Arabidopsis is Susceptible to Infection by a Downy Mildew Fungus. Plant Cell 2 (5), 437–445. doi: 10.1105/tpc.2.5.437
Kumar, S., Pagar, A. D., Ahmad, F., Dwibedi, V., Wani, A., Bharatam, P. V., et al. (2019). Xanthine Oxidase Inhibitors From an Endophytic Fungus Lasiodiplodia Pseudotheobromae. Bioorg. Chem. 87, 851–856. doi: 10.1016/j.bioorg.2018.12.008
Lin, S. B., Dong, Y. N., Li, X. W., Xing, Y. X., Liu, M. M., Sun, X. L. (2020). JA-Ile-Macrolactone 5b Induces Tea Plant (Camellia Sinensis) Resistance to Both Herbivore Ectropis Oblique and Pathogen Colletotrichum Camelliae. Int. J. Mol. Sci. 21 (5), 1828–1841. doi: 10.3390/ijms21051828
Li, Y. Z., Qin, L., Zhao, J. J., Muhammad, T., Cao, H. H., Li, H. L., et al. (2017). SlMAPK3 Enhances Tolerance to Tomato Yellow Leaf Curl Virus (TYLCV) by Regulating Salicylic Acid and Jasmonic Acid Signaling in Tomato (Solanum Lycopersicum). PloS One 12 (2), e0172466. doi: 10.1371/journal.pone.0172466
Liu, S. Y., He, F., Lin, N. Q., Chen, Y. M., Liang, Z. B., Liao, L. S., et al. (2020). Pseudomonas Sp. ST4 Produces Variety of Active Compounds to Interfere Fungal Sexual Mating and Hyphal Growth. Microb. Biotechnol. 13 (1), 107–117. doi: 10.1111/1751-7915.13289
Liu, T. T., Wan, Y. C., Liu, R. S., Ma, L., Li, M. Y., Fang, H. (2017). Design, Synthesis and Preliminary Biological Evaluation of Indole-3-Carboxylic Acid-Based Skeleton of Bcl-2/Mcl-1 Dual Inhibitors. Bioorg. Med. Chem. 25 (6), 1939–1948. doi: 10.1016/j.bmc.2017.02.014
Lorenzo, O., Piqueras, R., Sánchez-Serrano, J. J., Solano, R. (2003). Ethylene Response Factor1 Integrates Signals From Ethylene and Jasmonate Pathways in Plant Defense. Plant Cell 15 (1), 165–178. doi: 10.1105/tpc.007468
Manser, B., Koller, T., Praz, C. R., Roulin, A. C., Zbinden, H., Arora, S., et al. (2021). Identification of Specificity-Defining Amino Acids of the Wheat Immune Receptor Pm2 and Powdery Mildew Effector Avrpm2. Plant J. 106 (4), 993–1007. doi: 10.1111/tpj.15214
Meshram, V., Saxena, S. (2016). Potential Fibrinolytic Activity of an Endophytic Lasiodiplodia Pseudotheobromae Species. 3 Biotech. 6 (1), 114. doi: 10.1007/s13205-016-0428-4
Mujahid, M., Sasikala, C., Ramana, C. V. (2011). Production of Indole-3-Acetic Acid and Related Indole Derivatives From L-Tryptophan by Rubrivivax Benzoatilyticus JA2. Appl. Microbiol. Biotechnol. 89 (4), 1001–1008. doi: 10.1007/s00253-010-2951-2
Pastor-Fernández, J., Pastor, V., Mateu, D., Gamir, J., Sánchez-Bel, P., Flors, V. (2019). Accumulating Evidences of Callose Priming by Indole-3-Carboxylic Acid in Response to Plectospharella Cucumerina. Plant Signal. Behav. 14 (7), 1608107. doi: 10.1080/15592324.2019.1608107
Petti, C., Reiber, K., Ali, S. S., Berney, M., Doohan, F. M. (2012). Auxin as a Player in the Biocontrol of Fusarium Head Blight Disease of Barley and Its Potential as a Disease Control Agent. BMC Plant Biol. 12, 224. doi: 10.1186/1471-2229-12-224
Pirttilä, A. M., Joensuu, P., Pospiech, H., Jalonen, J., Hohtola, A. (2004). Bud Endophytes of Scots Pine Produce Adenine Derivatives and Other Compounds That Affect Morphology and Mitigate Browning of Callus Cultures. Physiol. Plant 121 (2), 305–312. doi: 10.1111/j.0031-9317.2004.00330.x
Puri, S. C., Verma, V., Amna, T., Qazi, G. N., Spiteller, M. (2005). An Endophytic Fungus From Nothapodytes Foetida That Produces Camptothecin. J. Nat. Prod 68 (12), 1717–1719. doi: 10.1021/np0502802
Qian, C. D., Fu, Y. H., Jiang, F. S., Xu, Z. H., Cheng, D. Q., Ding, B., et al. (2014). Lasiodiplodia Sp. ME4-2, an Endophytic Fungus From the Floral Parts of Viscum Coloratum, Produces Indole-3-Carboxylic Acid and Other Aromatic Metabolites. BMC Microbiol. 14, 297. doi: 10.1186/s12866-014-0297-0
Qi, T. C., Song, S. S., Ren, Q. C., Wu, D. W., Huang, H., Chen, Y., et al. (2011). The Jasmonate-ZIM-Domain Proteins Interact With the WD-Repeat/bHLH/MYB Complexes to Regulate Jasmonate-Mediated Anthocyanin Accumulation and Trichome Initiation in Arabidopsis Thaliana. Plant Cell 23 (5), 1795–1814. doi: 10.1105/tpc.111.083261
Rajalaxmi, M., Shafreen, R. B., Iyer, P. M., Vino, R. S., Balamurugan, K., Pandian, S. K. (2016). An In Silico, In Vitro and In Vivo Investigation of Indole-3-Carboxaldehyde Identified From the Seawater Bacterium Marinomonas Sp. As an Anti-Biofilm Agent Against Vibrio Cholerae O1. Biofouling 32 (4), 1–12. doi: 10.1080/08927014.2016.1154545
Rodriguez, R. J., White, J., Arnold, A. E., Redman, R. S. (2009). Fungal Endophytes: Diversity and Functional Roles. New Phytol. 182 (2), 314–330. doi: 10.1111/j.1469-8137.2009.02773.x
Rosenblueth, M., Martínez-Romero, E. (2006). Bacterial Endophytes and Their Interactions With Hosts. Mol. Plant Microbe Interact. 19 (8), 827–837. doi: 10.1094/MPMI-19-0827
Ruan, J. J., Zhou, Y. X., Zhou, M. L., Yan, J., Khurshid, M., Wang, W. F., et al. (2019). Jasmonic Acid Signaling Pathway in Plants. Int. J. Mol. Sci. 20 (10), 2479–2493. doi: 10.3390/ijms20102479
Sakai, K., Iwatsuki, M., Iizuka, M., Asami, Y., Nonaka, K., Masuma, R., et al. (2021). Aldsulfin, a Novel Unusual Anti-Mannheimiosis Epithiodiketopiperazine Antibiotic Produced by Lasiodiplodia Pseudotheobromae FKI-4499. J. Antibiot. 74 (6), 363–369. doi: 10.1038/s41429-021-00411-8
Santner, A., Estelle, M. (2009). Recent Advances and Emerging Trends in Plant Hormone Signaling. Nature 459 (7250), 1071–1078. doi: 10.1038/nature08122
Schardl, C. L., Leuchtmann, A., Spiering, M. J. (2004). Symbioses of Grasses With Seedborne Fungal Endophytes. Annu. Rev. Plant Biol. 55, 315–340. doi: 10.1146/annurev.arplant.55.031903.141735
Schulz, B., Boyle, C., Draeger, S., Römmert, A. K., Krohn, K. (2002). Endophytic Fungi: A Source of Novel Biologically Active Secondary Metabolites. Mycol. Res. 106 (9), 996–1004. doi: 10.1017/S0953756202006342
Shen, Z. Q., Zheng, P., Li, R. Y., Sun, X. Y., Chen, P. C., Wu, D. (2022). High Production of Jasmonic Acid by Lasiodiplodia Iranensis Using Solid-State Fermentation: Optimization and Understanding. Biotechnol. J. 17 (5), e2100550. doi: 10.1002/biot.202100550
Siegel, M. R., Schardl, C. L., Phillips, T. D. (1995). Incidence and Compatibility of Nonclavicipitaceous Fungal Endophytes in Festuca and Lolium Grass Species. Mycologia 87 (2), 196–202. doi: 10.1080/00275514.1995.12026521
Skibbe, M., Qu, N., Galis, I., Baldwin, I. T. (2008). Induced Plant Defenses in the Natural Environment: Nicotiana Attenuata WRKY3 and WRKY6 Coordinate Responses to Herbivory. Plant Cell 20 (7), 1984–2000. doi: 10.1105/tpc.108.058594
Staniek, A., Woerdenbag, H. J., Kayser, O. (2008). Endophytes: Exploiting Biodiversity for the Improvement of Natural Product-Based Drug Discovery. J. Plant Interact. 3, 75–93. doi: 10.1080/17429140801886293
Stierle, A., Strobel, G., Stierle, D. (1993). Taxol and Taxane Production by Taxomyces Andreanae, an Endophytic Fungus of Pacific Yew. Science 260 (5105), 214–216. doi: 10.1126/science.8097061
Suryanarayanan, T. S., Thirunavukkarasu, N., Govindarajulu, M. B., Gopalan, V. (2012). Fungal Endophytes: An Untapped Source of Biocatalysts. Fungal Divers. 54 (1), 19–30. doi: 10.1007/s13225-012-0168-7
Tanaka, A., Tapper, B. A., Popay, A., Parker, E. J., Scott, B. (2005). A Symbiosis Expressed non-Ribosomal Peptide Synthetase From a Mutualistic Fungal Endophyte of Perennial Ryegrass Confers Protection to the Symbiotum From Insect Herbivory. Mol. Microbiol. 57 (4), 1036–1050. doi: 10.1111/j.1365-2958.2005.04747.x
Tan, R. X., Zou, W. X. (2001). Endophytes: A Rich Source of Functional Metabolites. Nat. Prod. Rep. 18 (4), 448–459. doi: 10.1039/b100918o
Tudzynski, B. (1997). Fungal Phytohormones in Pathogenic and Mutualistic Associations. Plant Relat. 5, 167–184. doi: 10.1007/978-3-662-10370-8_10
Vieira, M. L. A., Johann, S., Hughes, F. M., Rosa, C. A., Rosa, L. H. (2014). The Diversity and Antimicrobial Activity of Endophytic Fungi Associated With Medicinal Plant Baccharis Trimera (Asteraceae) From the Brazilian Savannah. Can. J. Microbiol. 60 (12), 847–856. doi: 10.1139/cjm-2014-0449
Warabieda, W., Markiewicz, M., Wójcik, D. (2020). Mutual Relations Between Jasmonic Acid and Acibenzolar-S-Methyl in the Induction of Resistance to the Two-Spotted Spider Mite (Tetranychus Urticae) in Apple Trees. Exp. Appl. Acarol. 82 (1), 59–79. doi: 10.1007/s10493-020-00539-6
Wei, W., Jiang, N., Mei, Y. N., Chu, Y. L., Ge, H. M., Song, Y. C., et al. (2014). An Antibacterial Metabolite From Lasiodiplodia Pseudotheobromae F2. Phytochemistry 100, 103–109. doi: 10.1016/j.phytochem.2014.01.003
Xiang, L. B., Gong, S. J., Yang, L. J., Hao, J. J., Xue, M. F., Zeng, F. S., et al. (2016). Biocontrol Potential of Endophytic Fungi in Medicinal Plants From Wuhan Botanical Garden in China. Biol. Control 94, 47–55. doi: 10.1016/j.biocontrol.2015.12.002
Yang, X. J., Yang, L. J., Yu, D. Z., Ni, H. W. (2008). Effects of Physcion, a Natural Anthraquinone Derivative, on the Infection Process of Blumeria Graminis on Wheat. Can. J. Plant Pathol. 30 (3), 391–396. doi: 10.1080/07060660809507536
Yang, L., Zhou, Y. L., Duan, X. Y., Cao, X. R., Zou, Y. F. (2013). Sensitivity of Blumeria Graminis F. Sp. Tritici Isolates to Triadimefon and Fenpropidin in China. Acta Phytopathol. Sin. 43 (4), 430–434. doi: 10.3969/j.issn.0412-0914.2013.04.015
Yue, Q., Miller, C. J., White, J. F., Jr., Richardson, M. D. (2000). Isolation and Characterization of Fungal Inhibitors From Epichloë Festucae. J. Agric. Food Chem. 48 (10), 4687–4692. doi: 10.1021/jf990685q
Zhao, Y. Y., Song, C. C., Brummell, D. A., Qi, S. N., Lin, Q., Duan, Y. Q. (2021). Jasmonic Acid Treatment Alleviates Chilling Injury in Peach Fruit by Promoting Sugar and Ethylene Metabolism. Food Chem. 338, 128005. doi: 10.1016/j.foodchem.2020.128005
Zhou, Y., Zhang, X. X., Butcher, R. A. (2019). Tryptophan Metabolism in Caenorhabditis Elegans Links Aggregation Behavior to Nutritional Status. ACS Chem. Biol. 14 (1), 50–57. doi: 10.1021/acschembio.8b00872
Keywords: Lsiodiplodia pseudotheobromae LPS-1, indole-3-carboxylic acid, jasmonic acid, wheat powdery mildew, Blumeria graminis, synergist
Citation: Que Y, Huang D, Gong S, Zhang X, Yuan B, Xue M, Shi W, Zeng F, Liu M, Chen T, Yu D, Yan X, Wang Z, Yang L and Xiang L (2022) Indole-3-Carboxylic Acid From the Endophytic Fungus Lasiodiplodia pseudotheobromae LPS-1 as a Synergist Enhancing the Antagonism of Jasmonic Acid Against Blumeria graminis on Wheat. Front. Cell. Infect. Microbiol. 12:898500. doi: 10.3389/fcimb.2022.898500
Received: 17 March 2022; Accepted: 06 June 2022;
Published: 04 July 2022.
Edited by:
Hailing Jin, University of California, Riverside, United StatesReviewed by:
Ulrich Kück, Ruhr University Bochum, GermanyJianuan Zhou, South China Agricultural University, China
Copyright © 2022 Que, Huang, Gong, Zhang, Yuan, Xue, Shi, Zeng, Liu, Chen, Yu, Yan, Wang, Yang and Xiang. This is an open-access article distributed under the terms of the Creative Commons Attribution License (CC BY). The use, distribution or reproduction in other forums is permitted, provided the original author(s) and the copyright owner(s) are credited and that the original publication in this journal is cited, in accordance with accepted academic practice. No use, distribution or reproduction is permitted which does not comply with these terms.
*Correspondence: Libo Xiang, hbxlibo2008@163.com; Lijun Yang, yanglijun1993@163.com
†These authors have contributed equally to this work