- Department of Microbiology and Environmental Toxicology, University of California, Santa Cruz, Santa Cruz, CA, United States
Streptococcus pneumoniae (Spn) is the primary agent of community-acquired pneumonia. Neutrophils are innate immune cells that are essential for bacterial clearance during pneumococcal pneumonia but can also do harm to host tissue. Neutrophil migration in pneumococcal pneumonia is therefore a major determinant of host disease outcomes. During Spn infection, detection of the bacterium leads to an increase in proinflammatory signals and subsequent expression of integrins and ligands on both the neutrophil as well as endothelial and epithelial cells. These integrins and ligands mediate the tethering and migration of the neutrophil from the bloodstream to the site of infection. A gradient of host-derived and bacterial-derived chemoattractants contribute to targeted movement of neutrophils. During pneumococcal pneumonia, neutrophils are rapidly recruited to the pulmonary space, but studies show that some of the canonical neutrophil migratory machinery is dispensable. Investigation of neutrophil migration is necessary for us to understand the dynamics of pneumococcal infection. Here, we summarize what is known about the pathways that lead to migration of the neutrophil from the capillaries to the lung during pneumococcal infection.
Introduction
Streptococcus pneumoniae (Spn) is a Gram-positive bacterium and regular member of the microbiota in the upper respiratory tract of about 0-40% of adults (Esposito et al., 2016; Smith et al., 2020) and 27-65% of children (Weiser et al., 2018). Though usually an asymptomatic colonizer in the upper respiratory tract, Spn can also cause clinical syndromes including pneumonia, bacteremia and meningitis. Pneumonia is the most common outcome of Spn infection (Brooks and Mias, 2018) and can cause disruption of lung integrity, leading to further invasion of the pathogen to the bloodstream and brain, causing bacteremia and meningitis, respectively. Although the severity of infection depends partly on the specific strain and serotype of Spn (Melin et al., 2010; Hyams et al., 2013), the host’s immune response also plays a critical role in pathogenesis and disease outcome. Initial detection of Spn in the lung is mediated by resident alveolar macrophages (Cole et al., 2014; Dockrell and Brown, 2015) and epithelial cells (Yamamoto et al., 2014), leading to significant infiltration of neutrophils into the lung. Recent work has also shown the importance of complement (Agarwal and Blom, 2015) and T cells (Ivanov et al., 2014) in modulating the innate immune response to Spn in the lung (Kadioglu and Andrew, 2004). Following infection, a rapid return to homeostasis and resolution of inflammation is crucial for host outcome (Kumar, 2020).
A hallmark of pneumococcal pneumonia is the rapid influx of neutrophils which play a crucial role in controlling Spn burden. Neutrophils can kill Spn intracellularly through phagocytosis but are better recognized for their diverse and highly specialized extracellular antimicrobial defenses mediated by degranulation (Amulic et al., 2012). Degranulation is a regulated process by which activated neutrophils release effectors, including cationic antimicrobial peptides, serine proteases, myeloperoxidase, and reactive oxygen species (ROS) (Effah et al., 2021). Neutrophils can also release DNA to trap bacteria in neutrophil extracellular traps (NETs) through a process known as NETosis (Effah et al., 2021). Finally, neutrophils play a critical role in regulation of inflammation through the production of cytokines which recruit additional leukocytes to the site of infection (Tecchio et al., 2014). These defenses can be very effective against Spn and are critical for control of symptomatic infection. Neutropenic patients are at increased risk for pneumonia (Rolston, 2001) and patients with a deficiency in degranulation experience recurrent infection and have a diminished ability to kill Spn (Ganz et al., 1988), a finding recapitulated in mouse models (Borsa et al., 2019).
However, like most successful pathogens, Spn has evolved myriad strategies to evade neutrophil-mediated immunity, which we will highlight in this review. Furthermore, because neutrophil defenses occur largely through the release of highly damaging compounds into the extracellular milieu, neutrophilic inflammation almost always causes immunopathology which can increase disease severity. Neutrophil recruitment and activity must be tightly controlled. Significant evidence exists indicating these two features can be coupled - the signals neutrophils receive during recruitment can influence effector functions at the site of infection. This review centers on the first phenomenon - mechanisms of neutrophil recruitment during pulmonary Spn infection.
Neutrophil Dynamics in Murine Models
Murine models have been a powerful tool to understand the dynamics of neutrophil recruitment and will be the focus of this review, though it is important to note that all findings in murine systems must be validated to assess relevance to human disease. Pulmonary infection can be established through intranasal or intratracheal inoculation of Spn (Jeong et al., 2011). Pathogenesis is dependent on the bacterial strain (Mizrachi-Nebenzahl et al., 2003; Melin et al., 2010; Seyoum et al., 2011; Naucler et al., 2013; Paton and Trappetti, 2019) and is highly influenced by murine background (Gingles et al., 2001; Ripoll et al., 2010), age (Boyd et al., 2012; Williams et al., 2015; Janesch et al., 2018), and sex (Kadioglu et al., 2011a). Neutrophil infiltration into the lung occurs within the first day of infection and the infection is typically resolved (either by clearance of bacteria or death of mice) within a week. An ~100 fold increase in pulmonary neutrophils occurs following infection with Spn TIGR4, peaking at 18 hours post-infection (Bou Ghanem et al., 2015). The early recruitment of neutrophils is critical to bacterial clearance and host survival. Delays in recruitment lead to increased bacterial loads in the lung (Nieminen et al., 2008; Kadioglu et al., 2011b) and systemic neutrophil depletion results in higher bacterial burden and increased mortality (McNamee and Harmsen, 2006; Bou Ghanem et al., 2015). Although neutrophil influx is often correlated with neutrophil function in pneumococcal pneumonia, the link between these two phenomena requires more robust research.
Prolonged or excessive neutrophil recruitment, however, can cause damage to the pulmonary barrier and increase Spn invasion into the blood (Domon and Terao, 2021). In contrast to the detrimental effect of neutrophil depletion at the onset of infection, a protective effect is observed if neutrophil depletion is initiated at 18 hours post-infection, when neutrophil recruitment has already reached its peak. Depletion at this point reduces bacterial burden in the blood and increases survival (Bou Ghanem et al., 2015). Thus, damage caused by uncontrolled neutrophil inflammation can outweigh the antimicrobial benefit of the neutrophils and therefore must be tightly regulated. IL-10 is a potent anti-inflammatory signal responsible for modulation of inflammation. IL-10-/- mice have increased expression of proinflammatory cytokines, exacerbated recruitment of neutrophils to the lungs, and increased susceptibility to Spn infection (Peñaloza et al., 2015). The regulation and dynamics of neutrophils recruitment to the pulmonary space is therefore a critical determinant of outcome following pneumococcal pneumonia.
Recognition of Spn
To initiate neutrophil recruitment, tissue-resident immune cells and pulmonary epithelial cells recognize pneumococcal components by pattern recognition receptors (PRRs) such as Toll-Like Receptors (TLRs) and NOD-Like Receptors (NLRs) which results in production of cytokine and chemokines (reviewed extensively (Calbo and Garau, 2010; Hartl et al., 2018; Koppe et al., 2012; MacCain and Tuomanen, 2020; Paterson and Mitchell, 2006). A major downstream output of PRR signaling is the activation of the master transcription regulator NF-κB, which is required for optimal recruitment of neutrophils during Spn infection (Alcamo et al., 2001). Mice lacking TLR-adaptor MyD88 have defects in neutrophil recruitment to the lung (Albiger et al., 2005), indicating TLR signaling is important for detection of Spn. Of the 12 TLRs that exist in mice, TLR1, TLR2, TLR3, TLR4, TLR7, TLR9, and TLR13 have been shown to detect Spn ligands (Branger et al., 2004; Schmeck et al., 2006; Spelmink et al., 2016; Famà et al., 2020). However, not all have a significant impact on neutrophil influx (Craig et al., 2009). Knockout of TLR2 (Knapp et al., 2004; Dessing et al., 2008a) leads to defects in neutrophil recruitment, while knockout of TLR9 (Albiger et al., 2007) or TLR4 does not (Branger et al., 2004; Srivastava et al., 2005; Dessing et al., 2008b). Triple knockout mice lacking TLR7/9/13 have defects in generation of neutrophil-attracting chemokines and increased susceptibility almost as severe as MyD88-/- mice. However, single deletion is not sufficient to cause susceptibility demonstrating these sensors show functional compensation in vivo (Famà et al., 2020). Another important family of PRR activated by Spn is cytosolic NOD1 and NOD2 that detect peptidoglycan (Zheng et al., 2018). Deletion of either does not affect neutrophil influx but does lead to bacterial burden increase in some tissues (Lysenko et al., 2007; Davis et al., 2011).
In addition to activation of PRRs, bacterial ligands can also serve as direct chemoattractants for neutrophils (Bloes et al., 2015). Neutrophils can sense N-formyl peptides (fMLP) produced by Spn via fMLP receptor. Administration of fMLP receptor antagonist prior to Spn infection decreased neutrophils in bronchoalveolar lavage (BALF) (Fillion et al., 2001). Pneumolysin, a major virulence factor produced by Spn, also has myriad effects on neutrophil migration. Recombinant pneumolysin is sufficient to induce neutrophil migration in transwell assays, demonstrating it can serve as a chemoattractant (Moreland and Bailey, 2006). Recent work has demonstrated that pneumolysin-induced neutrophil migration is mediated through the formation of pores by pneumolysin and subsequent generation of inflammatory lipids which act as chemoattractants (Adams et al., 2020). Additionally, pneumolysin is a pore-forming toxin and causes damage-induced inflammation in vivo (Rubins et al., 1992; Rubins et al., 1993; Rayner et al., 1995; Witzenrath et al., 2006; García-Suárez et al., 2007). Pulmonary inoculation of recombinant pneumolysin is sufficient to cause neutrophil influx in vivo and Spn lacking pneumolysin results in decreased neutrophil infiltration. However, as pneumolysin is a crucial virulence factor, this attenuated strain is rapidly cleared in many animal models which likely contributes to decreased inflammation (Rubins et al., 1995; Kadioglu et al., 2000).
Migration of Neutrophils to the Lung
Neutrophils are bone marrow-derived cells that circulate in the bloodstream until recruited into tissue. Inflammation induces vasodilation to slow blood flow, allowing neutrophils to sequentially migrate through the endothelium, interstitium, basement membrane and the epithelium (Figure 1). Each of these steps is orchestrated through interactions between the neutrophil and membrane-bound ligands or soluble factors (Adams et al., 2021). Some chemoattractants are known to play a role in specific movements across the endothelium, epithelium or through a specific space. For example, CD73, which plays a role in adenosine production, contributes to transendothelial but not transepithelial migration. We will highlight these nuances during our discussion of specific factors.
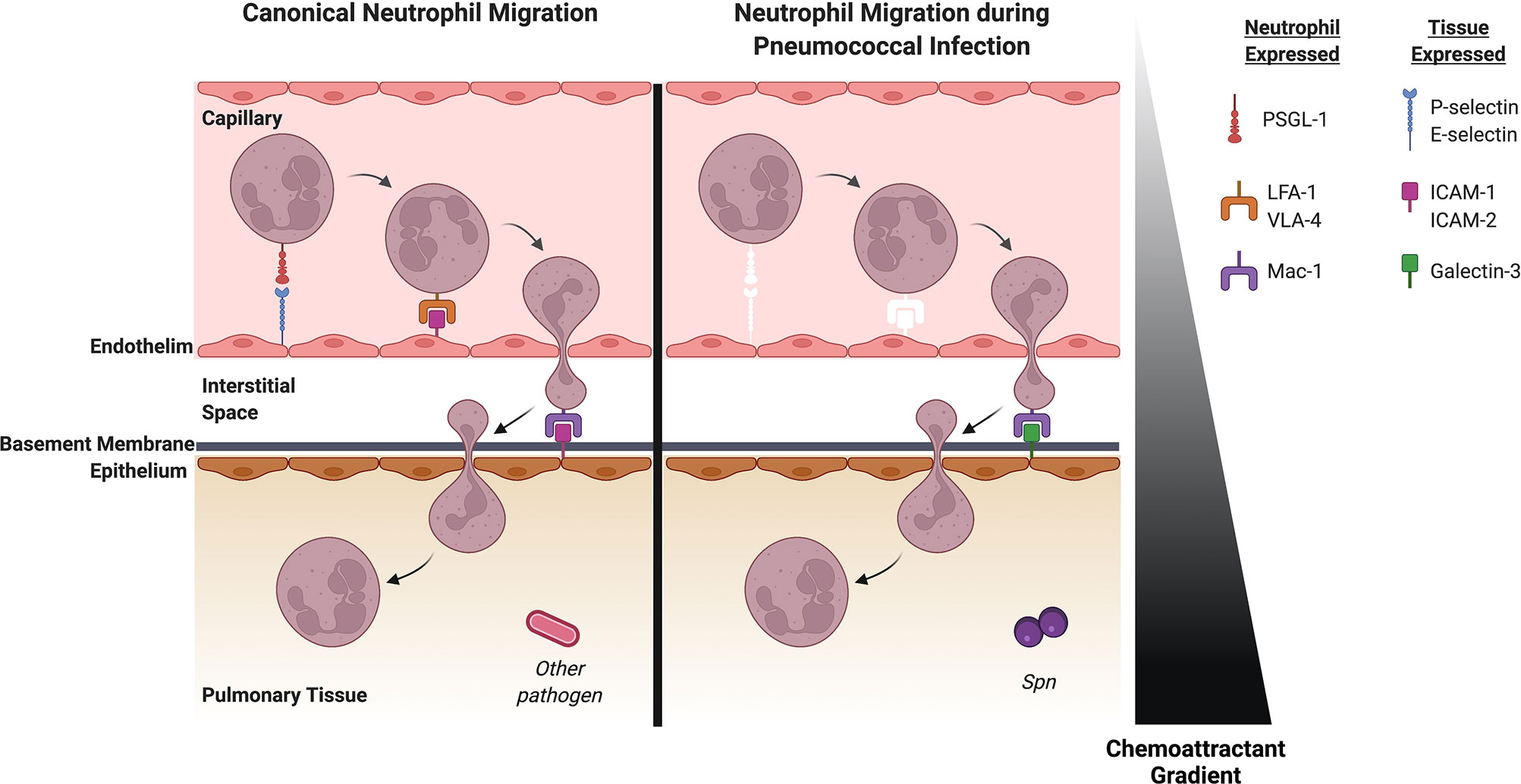
Figure 1 Neutrophil recruitment in the lung during pneumococcal pneumonia differs from canonical neutrophil recruitment to the lung. Left: Canonical neutrophil recruitment into pulmonary tissue. Selectins impart weak interactions between the endothelium and neutrophil which allows tethering by the integrin PSGL-1. LFA-1 and VLA-4 on the neutrophil then interact with ICAM-1 and ICAM-2 on the endothelial surface allowing the neutrophil to cross the endothelium. ICAM-1 and ICAM-2 also allows the neutrophil to bind to the epithelium via the integrin Mac-1 and subsequently migrate into the pulmonary space. Right: Neutrophil recruitment to the lung during Spn infection. White molecules represent factors that are important for canonical neutrophil recruitment but do not play a role in pneumococcal pneumonia. Selectins are dispensable for neutrophil recruitment for pneumococcal pneumonia. Mechanical entrapment in the thinner vessels likely plays a role in initial attraction of the neutrophil to the endothelium. The canonical integrin-ligand pairs that allow the neutrophil to bind to the endothelium seem to be non-essential in pneumococcal pneumonia, so unknown ligands and integrins on the endothelium and the neutrophil may play a role here. Once the neutrophil is in the interstitial space, ligands on the epithelium capture the neutrophil. Although canonical ligands like ICAM-1 and ICAM-2 have been shown to be indispensable here, Galectin-3 has a significant role in subsequent movement of the neutrophil into the pulmonary space. Spn, Streptococcus pneumoniae. Created with BioRender.com.
Transendothelial migration from the blood begins when inflammatory cytokines upregulate adhesion molecules on the endothelial surface. In most tissues, neutrophil migration occurs in post-capillary venules. Migration into the alveolar space, however, occurs in the capillaries which have a smaller diameter and it is thought that mechanical entrapment may be important for neutrophil migration (Doyle et al., 1997). Capture of neutrophils is typically mediated by E-selectin (CD62E) and P-selectin (CD62P). However, pulmonary neutrophil migration during Spn uniquely happens independently of these interactions (Mizgerd et al., 1996). Consistent with this, mice lacking neutrophilic P-selectin glycoprotein ligand-1 (PSGL-1), which interacts with endothelial E- and P-selectin, show no defect in pulmonary migration during Spn (Ramos-Sevillano et al., 2016). However, PGSL-1 knockout mice show increased bacterial burden in the lung and blood indicating PGSL-1 has other roles in the prevention of invasive disease. Bacteria lacking a protease that can degrade PGSL-1 (Spn ΔzmpC) stimulate increased neutrophil inflammation (Surewaard et al., 2013), pointing towards a potential role for PGSL-1 or similar integrins during infection. Surface expression of other adhesion molecules involved in neutrophil recruitment including CD54 (ICAM-1), CD102 (ICAM-2), CD106 (VCAM-1), and E-selectin is not upregulated on pulmonary endothelium following exposure to two different serotypes of Spn (Bullard et al., 1995; Moreland et al., 2004), suggesting these are not major drivers regulator of neutrophil influx during Spn infection.
Integrins are heterodimers consisting of an α and β subunit and are upregulated during inflammation to promote neutrophil attachment to the endothelial surface. CD18 is a β subunit that can dimerize with four different α subunits (CD11a, CD11b, CD11c, and CD11d). CD11a/CD18 (LFA-1) is canonically an important integrin for neutrophil arrest and has a known role in several bacterial pneumonias (Maas et al., 2018). However, inhibition or deletion of CD11a or CD18 does not affect neutrophil recruitment during Spn infection (Mizgerd et al., 1997; Mizgerd et al., 1999; Maas et al., 2018). Similarly, ICAM-1 (CD54), the canonical binding partner of CD11a/CD18, does not contribute to pulmonary recruitment during infection. ICAM-1 expression is not upregulated on pulmonary epithelium by Spn (Burns et al., 1994) and mutation of ICAM-1 lead to defects in peritoneal, but not pulmonary recruitment during Spn infection (Bullard et al., 1995). Therefore, ICAM-1 plays a tissue-specific role in neutrophil recruitment. Another β integrin, very-late antigen 4 (VLA-4), is increased on neutrophils during in vitro Spn infection (Kubes et al., 1995). Studies in mice, however, did not observe this increase and antibody blockade of VLA-4 has no effect during Spn infection (Tasaka et al., 2002). Redundancy in adhesion molecules likely contributes, at least in part, to modest phenotypes in recruitment defects. However, a double-mutant lacking both P-selectin and ICAM-1 still shows no defect in Spn-mediated neutrophil recruitment (Bullard et al., 1995) indicating mechanisms of neutrophil recruitment are unique to Spn compared to other bacterial pneumonias.
Following sequestration on the endothelial surface, neutrophil movement is canonically mediated by the neutrophilic integrin L-selectin (CD62L) and gap junction protein connexin 43. Knockout of L-selectin does not affect neutrophil recruitment in response to Spn in mice (Doyle et al., 1997) and work in the rabbit lung associated Spn infection with downregulation of L-selectin on neutrophils (Burns and Doerschuk, 1994). While connexin 43 plays an important role in pneumococcal meningitis (Bello et al., 2020), it has not been well studied in pneumonia. Once the neutrophil migrates through the endothelium, it must travel through the interstitium and cross the basement membrane. Overall, these processes are not well-characterized, but attention has been brought to glycosaminoglycans as a key component of the extracellular space and as modulators of the inflammatory response (Souza-Fernandes et al., 2006). Studies in nasopharyngeal tissue implicate glycosaminoglycans in Spn attachment to epithelial cells (Tonnaer et al., 2006). Expression of certain glycosaminoglycans may therefore benefit the bacterium more than mediate neutrophil recruitment; however these processes are not well studied in the lung and therefore more research is needed to understand how neutrophils cross the endothelium, interstitium and basement membrane.
After crossing the basement membrane, neutrophils undergo transepithelial migration which consists of basolateral adhesion, paracellular transit and alveolar entry. Like transendothelial migration, migration of neutrophils across the epithelium is orchestrated by ligand-integrin binding. The best-characterized receptor for basolateral adhesion of neutrophils is CD11b/CD18 (Mac-1). During Spn infection, blockade or knockout of CD11b decreased neutrophil influx and increased bacterial lung burden (Kadioglu et al., 2011b). ICAM-1 is a ligand for CD11b/CD18 (as well as the aforementioned CD11a/CD18 endothelial transmigration integrin), but knockout or antibody blockade of ICAM-1 did not affect neutrophil infiltration (Moreland et al., 2004; Kadioglu et al., 2011b). The role of ICAM-1 in canonical transepithelial and transendothelial migration has been reported, but this ligand does not seem to be necessary for pneumococcal pneumonia. Instead, galectin-3 is a ligand for CD11b/CD18 that is upregulated in lungs infected with Spn but not other bacterial species (Nieminen et al., 2008). Galectin-3 deficient mice showed decreased neutrophil influx and increased bacterial load in response to pneumococcal pneumonia, indicating galectin-3 is a Spn-specific integrin that aids in migration of neutrophils.
To cross the epithelial barrier during paracellular transit, neutrophils release serine proteases and matrix metalloproteases that degrade intercellular junctions. Neutrophil elastase, cathepsin G and proteinase 3 can all degrade junction proteins such as E-cadherin. Recent work in human lung tissue showed that pneumococcal infection reduced the junction components occludin, ZO-1, claudin-5 and VE-cadherin but did not change the presence of other claudins (Peter et al., 2017). Pneumolysin also increases the permeability of the pulmonary lining through disruption of intercellular junctions (Rubins et al., 1993; Rayner et al., 1995; Knippenberg et al., 2015) which may allow neutrophils to cross the epithelium more easily. Once neutrophils pass the intercellular junctions of the epithelium, they interact with the apical side of the epithelium and enter the pulmonary space.
Chemokines and Cytokines
Neutrophil migration is ultimately accomplished through the cooperation of integrin-ligand interactions and directed migration down a gradient of intermediate-target and end-target attractants. The gradient of chemokines, inflammatory lipids, serum proteins and bacterial components induce migration through activation of G-protein coupled receptors (GPCRs) on neutrophils. Intermediate-target attractants control directed neutrophil migration en route to the site of inflammation, whereas end-target attractants are preferred by neutrophils and determine local neutrophil activity once in the lung.
Chemokines are important intermediate-target chemoattractants that interact with the neutrophil en route to the lung. In mice, the chemokines CXCL1 (KC), CXCL2 (MIP-2), and CXCL5 (LIX) mediate the neutrophils’ basolateral adhesion to the lung epithelium (Adams et al., 2021). CXCL1 and CXCL2 are produced primarily by myeloid cells (Fillion et al., 2001; Craig et al., 2009), whereas epithelial cells are the predominant sources of CXCL5 (Yamamoto et al., 2014).
CXCL1 is produced in response to TLR-mediated NF-κB activation (Paudel et al., 2019) and plays a crucial role in the recruitment of neutrophils (McColl and Clark-Lewis, 1999) after Spn. Mice lacking CXCL1 showed decreased neutrophil abundance in the BALF as well as increased bacterial burden in the lung, blood and BALF. CXCL1 is also required for emergency granulopoiesis during Spn infection, which also contributes to decreased neutrophil numbers recruited to the lungs (Paudel et al., 2019). Once neutrophils reach the pulmonary space, they make CXCL2 upon stimulation by local cues produced by myeloid and epithelial cells (Kamata et al., 2016). CXCL2 is canonically a potent chemoattractant in bacterial pneumonia (Adams et al., 2021), but its role has not been well-studied with Spn. One study showed that the administration of the proinflammatory cytokine IL-12 could improve innate defense in the lung against Spn by inducing IFN-γ production, enhancing CXCL2 expression, and thereby increasing neutrophil recruitment to the lung after infection (Sun et al., 2007). This suggests a direct role for CXCL2 in neutrophil migration during pneumococcal pneumonia.
In addition to the myeloid-derived CXCL1 and CXCL2, epithelial-derived CXCL5 enhances neutrophil recruitment (Gibbs et al., 2014; Yamamoto et al., 2014). Mice with previous Spn infection had faster bacterial clearance upon secondary infection in part to prolonged stability of the chemokine ligand CXCL5 transcripts by IL-17a (Shenoy et al., 2020) resulting in more rapid neutrophil recruitment to the lung. Mice with heightened levels of CXCL5 had increased neutrophil influx, better control of pulmonary burden and increased survival upon Spn infection (Mancuso et al., 2018).
CXCL1, CXCL2 and CXCL5 all bind to CXCR1 and CXCR2 receptors on neutrophils and therefore these chemokines and receptors are emerging as exciting therapeutic targets to control neutrophil influx (Planagumà et al., 2015; Sundaresh et al., 2021). The role of CXCR1 has not been explored in pneumococcal pneumonia, but CXCR2 is important for optimal bacterial clearance. Spn infection of CXCR2 knockout mice or mice given a CXCR2 antagonist resulted in a defect in neutrophil influx and an associated increase in bacterial counts in BALF and lungs (Eash et al., 2010; Herbold et al., 2010).
Production of proinflammatory cytokines such as TNF-α, IL-1, and IL-17 are also important for neutrophil regulation (Bergeron et al., 1998). Antibody blockade (Takashima et al., 1997) or knockout (Jeong et al., 2015) of TNF-α lead to increased bacterial burden and mortality, though it did not directly affect neutrophil recruitment. Mice with defects in IL-1β but not IL-1α showed significantly worse immunopathology, bacterial burden and mortality following pulmonary infection with Spn (Kafka et al., 2008). A triple mouse mutant deficient in TNF-α receptors (TNFR1, TNFR2) and IL-1 receptor (IL-1RI) also had reduced neutrophil influx to the lung (Jones et al., 2005). The cytokine IL-17, typically associated with neutrophilic responses, acts in concert with TNF-α and IL-1 to promote inflammation. IL-17A can be either beneficial or detrimental depending on the strain of Spn, a process attributed to the abundance and function of recruited neutrophils (Ritchie et al., 2018). For example, mice lacking IL-17a had decreased neutrophil influx following infection with several strains of Spn, but had strain-dependent correlation with mouse survival. Together proinflammatory cytokines influence neutrophil migration at multiple levels including activation of myeloid cell effector function, upregulation of epithelial, endothelial, and neutrophil adhesion molecules, and activation of neutrophil extravasation. As such, the contribution of each of these factors may not directly reflect on neutrophil numbers, but interruption of their signaling may alter neutrophil migration and function.
Non-Chemokine Chemoattractants
Cleavage or processing of host molecules like extracellular matrix, complement proteins and phospholipids also produces a variety of chemoattractants. Matrikines are degraded components of the extracellular matrix that can act as intermediate chemoattractants for neutrophils during pulmonary inflammation (Adams et al., 2021). These components have not been well-studied in pneumococcal infection, but producers of the canonical matrikine proline-glycine-proline (PGP) are upregulated in response to Spn (Akthar et al., 2015). Activation of the host complement system generates classical neutrophil chemoattractants including the end-target attractant C3a and C5a, which increase in lungs during Spn infection (Angel et al., 1994; Paterson and Mitchell, 2006). The role of C3a has not been directly tested during Spn, but C5a neutralization did not affect neutrophil numbers in BALF (Müller-Redetzky et al., 2020) suggesting complement is not a main driver of neutrophil influx.
Inflammatory lipids are another important mediator which are produced upon phospholipid cleavage by phospholipase A2 to generate arachidonic acid (Rubins et al., 1994). Arachidonic acid modification by lipoxygenases produces chemoattractants leukotriene B4 (LTB4) and hepoxilin A3 (HXA3) (Dobrian et al., 2011). LTB4 is an intermediate stage chemoattractant produced by the lipoxygenase 5-LOX and is canonically important for transepithelial migration (Palmblad et al., 1981). Mice lacking 5-LOX exhibited increased susceptibility to pneumococcal pneumonia which could be reversed by exogenous administration of LTB4 (Mancuso et al., 2010). Interestingly, the pneumococcal virulence factor pneumolysin increases activity of phospholipase A2 and production of LTB4 in human neutrophils (Cockeran et al., 2001a; Cockeran et al., 2001b; Bhowmick et al., 2012; Bhowmick et al., 2017). HXA3 is another phospholipase A2-derived end-target chemoattractant that acts mostly on the apical side of the lung epithelium. HXA3 robustly attracts neutrophils across the lung epithelium during Spn infection (Bhowmick et al., 2013; Adams et al., 2020) and induced pulmonary inflammation (Bhowmick et al., 2017).
Another important host-derived modulator of neutrophil function is purinergic signaling. Purine nucleotides such as ATP, ADP, and adenosine differentially activate purinergic receptors including P2X (activated by ATP), P2Y (activated by ATP and ADP) and P1 receptors (activated by adenosine) (Huang et al., 2021). In homeostatic conditions, extracellular ATP is minimal due to rapid hydrolysis by surface associated nucleases including CD39 (converts ATP to AMP) and CD73 (converts AMP to adenosine), and extracellular nucleosides (degrade adenosine) (Baron et al., 2015). During inflammation, extracellular ATP can be acutely increased by secretion via connexins or pannexins (Muñoz et al., 2021) or upon loss of membrane integrity due to cell death. In the context of Spn, the virulence factor pneumolysin colocalizes with and transcriptionally upregulates P2X7R on neutrophils (Domon et al., 2016). A separate study (Cuypers et al., 2020) showed that experimental augmentation of extracellular ATP can protect from pneumolysin-induced neutrophil degranulation, though the effect was not dependent on P2X7R, suggesting a role for other P2 receptors or downstream signaling. For example, extracellular adenosine contributes to bacterial clearance and survival of mice following Spn infection. This effect is mediated in part by CD73-dependent generation of adenosine from AMP and can be pharmacologically augmented by preventing adenosine degradation (Bou Ghanem et al., 2015). Additionally, the role of CD73-dependent neutrophil attraction is specific to transendothelial migration and does not have a role in transepithelial migration. The mechanism of adenosine-based protection is not well-defined in Spn, but several P1 adenosine receptors (A1, A2B, A3) have immunomodulatory roles in endotoxin-induced pulmonary inflammation, and thus remains an open area for exploration during Spn infection (Ngamsri et al., 2010; Schingnitz et al., 2010; Wagner et al., 2010).
Discussion
Neutrophils are critical mediators of pneumococcal pneumonia and their rapid influx from the bloodstream into the pulmonary space is required for clearance of Spn. Once recruited to the lungs, neutrophils produce proinflammatory cytokines, perform phagocytosis, NETosis or degranulation and can cause considerable damage to host tissue if infection is not resolved in a reasonable time. Therefore, neutrophil influx to the lung is a critical factor in pneumococcal pneumonia pathogenesis.
The process of directed neutrophil migration from the blood across the endothelial and epithelial barriers depends on integrin-ligand interactions between the neutrophil and barrier cells as well as GPCR-mediated sensing of a gradient of chemoattractants. After detection of Spn through PRRs, resident myeloid cells and epithelial cells generate cytokines that signal to endothelial and epithelial cells to upregulate ligands with which to attract and tether neutrophils. The canonical neutrophil chemokines in mice, CXCL1, CXCL2, and CXCL5 as well as other chemoattracts created by the host (C5a, C3a, LTB4, HXA3) and Spn (fMLP, pneumolysin), guide the neutrophil to the lung through GPCR recognition by the neutrophil and subsequent movement. Neutrophils receive cytokine signals to express integrins and produce granules that aid in migration. Some of the canonical integrins that mediate neutrophil migration are dispensable in Spn infection and more research is needed to characterize mediators that dictate neutrophil movement from the bloodstream (Table 1). In particular, the necessary ligand-integrin pairs for transendothelial migration are not known for pneumococcal pneumonia and further studies in this particular process are needed.
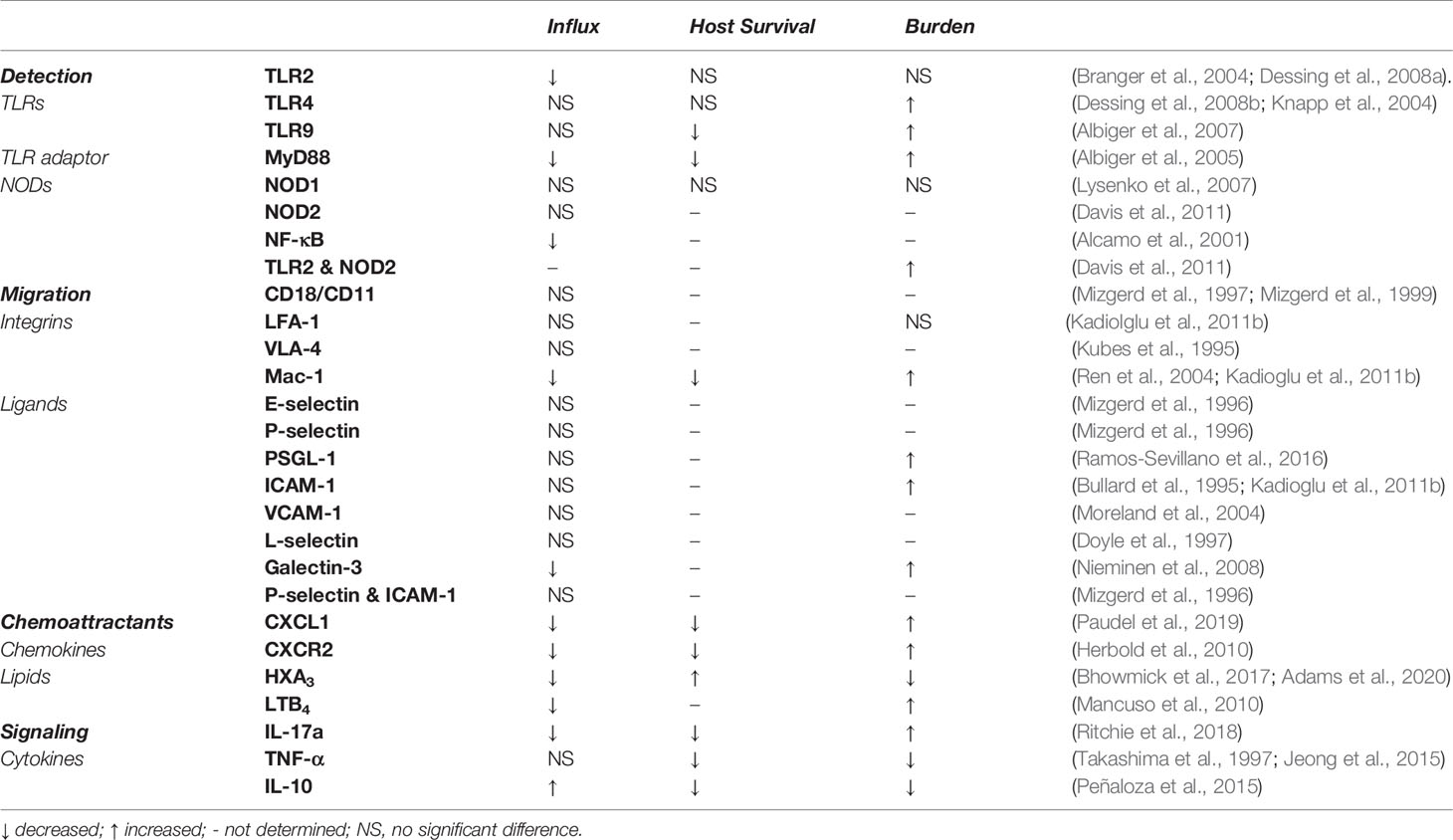
Table 1 Effect of in vivo depletion or knockout of key neutrophil migration mediators on neutrophil influx, survival and bacterial burden in pneumococcal pneumonia.
Improved models of neutrophil recruitment throughout infection may help elucidate our understanding of the contribution of these immune effectors to the pathophysiology of Spn infection. Although murine models have allowed us to gain a better understanding of neutrophil recruitment, the application of other physiologically relevant models such as human organoids (Gkatzis et al., 2018), intravital microscopy (Alizadeh-Tabrizi et al., 2021), and humanized mice (Zheng et al., 2022) to the study of pneumococcal pneumonia will be indispensable in developing our understanding of human infection.
Furthermore, host changes such as age (Tseng and Liu, 2014), neutrophil age (Simmons et al., 2021), time of day (Scheiermann et al., 2018), and co-infection (Jochems et al., 2018) modulate neutrophil recruitment and but their contribution to the pathophysiology of Spn infection of the lung is not well-studied and requires more attention. For example, neutrophils in aged hosts show aberrant chemotaxis and reduced NETosis. Pneumococcal pneumonia is particularly prevalent in older patients, but the precise interactions that lead to this dysfunction require more research (Grudzinska et al., 2020). The respiratory microbiome is also an increasingly compelling contributor to pulmonary disease which may be necessary for understanding neutrophil recruitment in pneumococcal pneumonia (Li et al., 2019). Additionally, it has been increasingly recognized in the field of neutrophil biology that not all neutrophils are alike but rather that there are subpopulations of neutrophils. Definitions of N1 and N2 neutrophil subsets as pro-inflammatory and anti-inflammatory respectively have emerged out of cancer biology (Ohms et al., 2020) and these classifications have not been explored with regard to pneumococcal pneumonia but may provide insight to the complex role of neutrophils in Spn infection.
The precise inflammatory landscape caused by excessive recruitment of neutrophils is of interest for therapeutic reasons (Németh et al., 2020). For example, dampening neutrophil recruitment with a CXCR2 antagonist has been shown to alleviate respiratory inflammation in patients with COPD (Rennard et al., 2015). Therefore, further studies defining mediators of neutrophil recruitment that are active in pneumococcal pneumonia may allow for the development of clinical therapeutics.
Author Contributions
CP: conceptualization and writing for original draft preparation. CP and JK: writing, review, editing, and visualization. All authors contributed to the article and approved the submitted version.
Funding
JK received funding from UC Santa Cruz which was used to support authors’ effort in writing this manuscript as well as publication fees.
Conflict of Interest
The authors declare that the research was conducted in the absence of any commercial or financial relationships that could be construed as a potential conflict of interest.
Publisher’s Note
All claims expressed in this article are solely those of the authors and do not necessarily represent those of their affiliated organizations, or those of the publisher, the editors and the reviewers. Any product that may be evaluated in this article, or claim that may be made by its manufacturer, is not guaranteed or endorsed by the publisher.
References
Adams, W., Bhowmick, R., Bou Ghanem, E. N., Wade, K., Shchepetov, M., Weiser, J. N., et al. (2020). Pneumolysin Induces 12-Lipoxygenase-Dependent Neutrophil Migration During Streptococcus Pneumoniae Infection. J. Immunol. 204, 101–111. doi: 10.4049/jimmunol.1800748
Adams, W., Espicha, T., Estipona, J. (2021). Getting Your Neutrophil: Neutrophil Transepithelial Migration in the Lung. Infect. Immun., 89. doi: 10.1128/IAI.00659-20
Agarwal, V., Blom, A. M. (2015). Roles of Complement C1q in Pneumococcus-Host Interactions. Crit. Rev. Immunol. 35, 173–184. doi: 10.1615/critrevimmunol.2015012177
Akthar, S., Patel, D. F., Beale, R. C., Peiró, T., Xu, X., Gaggar, A., et al. (2015). Matrikines are Key Regulators in Modulating the Amplitude of Lung Inflammation in Acute Pulmonary Infection. Nat. Commun. 6, 8423. doi: 10.1038/ncomms9423
Albiger, B., Dahlberg, S., Sandgren, A., Wartha, F., Beiter, K., Katsuragi, H., et al. (2007). Toll-Like Receptor 9 Acts at an Early Stage in Host Defence Against Pneumococcal Infection. Cell Microbiol. 9, 633–644. doi: 10.1111/j.1462-5822.2006.00814.x
Albiger, B., Sandgren, A., Katsuragi, H., Meyer-Hoffert, U., Beiter, K., Wartha, F., et al. (2005). Myeloid Differentiation Factor 88-Dependent Signalling Controls Bacterial Growth During Colonization and Systemic Pneumococcal Disease in Mice. Cell Microbiol. 7, 1603–1615. doi: 10.1111/j.1462-5822.2005.00578.x
Alcamo, E., Mizgerd, J. P., Horwitz, B. H., Bronson, R., Beg, A. A., Scott, M., et al. (2001). Targeted Mutation of TNF Receptor I Rescues the RelA-Deficient Mouse and Reveals a Critical Role for NF-κb in Leukocyte Recruitment. J. Immunol. 167, 1592–1600. doi: 10.4049/jimmunol.167.3.1592
Alizadeh-Tabrizi, N., Hall, S., Lehmann, C. (2021). Intravital Imaging of Pulmonary Immune Response in Inflammation and Infection. Front. Cell Dev. Biol. 8. doi: 10.3389/fcell.2020.620471
Amulic, B., Cazalet, C., Hayes, G. L., Metzler, K. D., Zychlinsky, A. (2012). Neutrophil Function: From Mechanisms to Disease. Annu. Rev. Immunol. 30, 459–489. doi: 10.1146/annurev-immunol-020711-074942
Angel, C. S., Ruzek, M., Hostetter, M. K. (1994). Degradation of C3 by Streptococcus Pneumoniae. J. Infect. Dis. 170, 600–608. doi: 10.1093/infdis/170.3.600
Baron, L., Gombault, A., Fanny, M., Villeret, B., Savigny, F., Guillou, N., et al. (2015). The NLRP3 Inflammasome is Activated by Nanoparticles Through ATP, ADP and Adenosine. Cell Death Dis. 6, e1629–e1629. doi: 10.1038/cddis.2014.576
Bello, C., Smail, Y., Sainte-Rose, V., Podglajen, I., Gilbert, A., Moreira, V., et al. (2020). Role of Astroglial Connexin 43 in Pneumolysin Cytotoxicity and During Pneumococcal Meningitis. PloS Pathog. 16, e1009152. doi: 10.1371/journal.ppat.1009152
Bergeron, Y., Ouellet, N., Deslauriers, A. M., Simard, M., Olivier, M., Bergeron, M. G. (1998). Cytokine Kinetics and Other Host Factors in Response to Pneumococcal Pulmonary Infection in Mice. Infect. Immun. 66, 912–922. doi: 10.1128/IAI.66.3.912-922.1998
Bhowmick, R., Clark, S., Bonventre, J. V., Leong, J. M., McCormick, B. A. (2017). Cytosolic Phospholipase A2α Promotes Pulmonary Inflammation and Systemic Disease During Streptococcus Pneumoniae Infection. Infection Immun. 85, e00280–17. doi: 10.1128/IAI.00280-17
Bhowmick, R., Leong, J., McCormick, B. (2012). Phospholipase A2 Plays a Key Role in PMN Transepithelial Migration During S. Pneumoniae Infection (55.18). J. Immunol. 188, 55.18–55.18.
Bhowmick, R., Maung, N., Hurley, B. P., Bou Ghanem, E., Gronert, K., McCormick, B. A., et al. (2013). Systemic Disease During Streptococcus Pneumoniae Acute Lung Infection Requires 12-Lipoxygenase-Dependent Inflammation. J. Immunol. 191, 5115–5123. doi: 10.4049/jimmunol.1300522
Bloes, D. A., Kretschmer, D., Peschel, A. (2015). Enemy Attraction: Bacterial Agonists for Leukocyte Chemotaxis Receptors. Nat. Rev. Microbiol. 13, 95–104. doi: 10.1038/nrmicro3390
Borsa, N., Pasquale, M. D., Restrepo, M. I. (2019). Animal Models of Pneumococcal Pneumonia. Int. J. Mol. Sci. 20 (17), 4220. doi: 10.3390/ijms20174220
Bou Ghanem, E. N., Clark, S., Roggensack, S. E., McIver, S. R., Alcaide, P., Haydon, P. G., et al. (2015). Extracellular Adenosine Protects Against Streptococcus Pneumoniae Lung Infection by Regulating Pulmonary Neutrophil Recruitment. PloS Pathog. 11, e1005126. doi: 10.1371/journal.ppat.1005126
Boyd, A. R., Shivshankar, P., Jiang, S., Berton, M. T., Orihuela, C. J. (2012). Age-Related Defects in TLR2 Signaling Diminish the Cytokine Response by Alveolar Macrophages During Murine Pneumococcal Pneumonia. Exp. Gerontology 47, 507–518. doi: 10.1016/j.exger.2012.04.004
Branger, J., Knapp, S., Weijer, S., Leemans, J. C., Pater, J. M., Speelman, P., et al. (2004). Role Oftoll-Like Receptor 4 in Gram-Positive and Gram-Negative Pneumonia Inmice. Infect. Immun. 72 (2), 788–94. doi: 10.1128/IAI.72.2.788-794.2004
Brooks, L. R. K., Mias, G. I. (2018). Streptococcus Pneumoniae’s Virulence and Host Immunity: Aging, Diagnostics, and Prevention. Front. Immunol. 9. doi: 10.3389/fimmu.2018.01366
Bullard, D. C., Qin, L., Lorenzo, I., Quinlin, W. M., Doyle, N. A., Bosse, R., et al. (1995). P-Selectin/ICAM-1 Double Mutant Mice: Acute Emigration of Neutrophils Into the Peritoneum Is Completely Absent But Is Normal Into Pulmonary Alveoli. J. Clin. Invest. 95, 1782–1788. doi: 10.1172/JCI117856
Burns, A. R., Doerschuk, C. M. (1994). Quantitation of L-Selectin and CD18 Expression on Rabbit Neutrophils During CD18-Independent and CD18-Dependent Emigration in the Lung. J. Immunol. 153, 3177–3188.
Burns, A. R., Takei, F., Doerschuk, C. M. (1994). Quantitation of ICAM-1 Expression in Mouse Lung During Pneumonia. J. Immunol. 153, 3189–3198.
Calbo, E., Garau, J. (2010). Of Mice and Men: Innate Immunity in Pneumococcal Pneumonia. Int. J. Antimicrob. Agents 35, 107–113. doi: 10.1016/j.ijantimicag.2009.10.002
Cockeran, R., Steel, H. C., Mitchell, T. J., Feldman, C., Anderson, R. (2001a). Pneumolysin Potentiates Production of Prostaglandin E2 and Leukotriene B4 by Human Neutrophils. Infect. Immun. 69 (5), 3494–3496. doi: 10.1128/IAI.69.5.3494-3496.2001
Cockeran, R., Theron, A. J., Steel, H. C., Matlola, N. M., Mitchell, T. J., Feldman, C., et al. (2001b). Proinflammatory Interactions of Pneumolysin With Human Neutrophils. J. Infect. Dis. 183, 604–611. doi: 10.1086/318536
Cole, J., Aberdein, J., Jubrail, J., Dockrell, D. H. (2014). “Chapter Four - The Role of Macrophages in the Innate Immune Response to Streptococcus Pneumoniae and Staphylococcus Aureus: Mechanisms and Contrasts,” in Advances in Microbial Physiology Advances in Bacterial Pathogen Biology. Ed. Poole, R. K. (Academic Press), 125–202. doi: 10.1016/bs.ampbs.2014.08.004
Craig, A., Mai, J., Cai, S., Jeyaseelan, S. (2009). Neutrophil Recruitment to the Lungs During Bacterial Pneumonia. Infect. Immun. 77, 568–575. doi: 10.1128/IAI.00832-08
Cuypers, F., Klabunde, B., Gesell Salazar, M., Surabhi, S., Skorka, S. B., Burchhardt, G., et al. (2020). Adenosine Triphosphate Neutralizes Pneumolysin-Induced Neutrophil Activation. J. Infect. Dis. 222, 1702–1712. doi: 10.1093/infdis/jiaa277
Davis, K. M., Nakamura, S., Weiser, J. N. (2011). Nod2 Sensing of Lysozyme-Digested Peptidoglycan Promotes Macrophage Recruitment and Clearance of S. Pneumoniae Colonization in Mice. J. Clin. Invest. 121, 3666. doi: 10.1172/JCI57761
Dessing, M. C., Florquin, S., Paton, J. C., van der Poll, T. (2008a). Toll-Like Receptor 2 Contributes to Antibacterial Defence Against Pneumolysin-Deficient Pneumococci. Cell. Microbiol. 10, 237. doi: 10.1111/j.1462-5822.2007.01035.x
Dessing, M. C., Schouten, M., Draing, C., Levi, M., von Aulock, S., van der Poll, T. (2008b). Role Played by Toll-Like Receptors 2 and 4 in Lipoteichoic Acid-Induced Lung Inflammation and Coagulation. J. Infect. Dis. 197, 245–252. doi: 10.1086/524873
Dobrian, A. D., Lieb, D. C., Cole, B. K., Taylor-Fishwick, D. A., Chakrabarti, S. K., Nadler, J. L. (2011). Functional and Pathological Roles of the 12- and 15-Lipoxygenases. Prog. Lipid Res. 50, 115–131. doi: 10.1016/j.plipres.2010.10.005
Dockrell, D. H., Brown, J. S. (2015). “Chapter 21 - Streptococcus Pneumoniae Interactions With Macrophages and Mechanisms of Immune Evasion,” in Streptococcus Pneumoniae. Eds. Brown, J., Hammerschmidt, S., Orihuela, C. (Amsterdam: Academic Press), 401–422. doi: 10.1016/B978-0-12-410530-0.00021-1
Domon, H., Oda, M., Maekawa, T., Nagai, K., Takeda, W., Terao, Y. (2016). Streptococcus Pneumoniae Disrupts Pulmonary Immune Defence via Elastase Release Following Pneumolysin-Dependent Neutrophil Lysis. Sci. Rep. 6, 38013. doi: 10.1038/srep38013
Domon, H., Terao, Y. (2021). The Role of Neutrophils and Neutrophil Elastase in Pneumococcal Pneumonia. Front. Cell. Infection Microbiol. 11. doi: 10.3389/fcimb.2021.615959
Doyle, N. A., Bhagwan, S. D., Meek, B. B., Kutkoski, G. J., Steeber, D. A., Tedder, T. F., et al. (1997). Neutrophil Margination, Sequestration, and Emigration in the Lungs of L-Selectin-Deficient Mice. J. Clin. Invest. 99, 526–533. doi: 10.1172/JCI119189
Eash, K. J., Greenbaum, A. M., Gopalan, P. K., Link, D. C. (2010). CXCR2 and CXCR4 Antagonistically Regulate Neutrophil Trafficking From Murine Bone Marrow. J. Clin. Invest. 120, 2423–2431. doi: 10.1172/JCI41649
Effah, C. Y., Drokow, E. K., Agboyibor, C., Ding, L., He, S., Liu, S., et al. (2021). Neutrophil-Dependent Immunity During Pulmonary Infections and Inflammations. Front. Immunol. 12. doi: 10.3389/fimmu.2021.689866
Esposito, S., Mari, D., Bergamaschini, L., Orenti, A., Terranova, L., Ruggiero, L., et al. (2016). Pneumococcal Colonization in Older Adults. Immun. Ageing 13, 2. doi: 10.1186/s12979-016-0057-0
Famà, A., Midiri, A., Mancuso, G., Biondo, C., Lentini, G., Galbo, R., et al. (2020). Nucleic Acid-Sensing Toll-Like Receptors Play a Dominant Role in Innate Immune Recognition of Pneumococci. mBio 11 (2), e00415–20. doi: 10.1128/mBio.00415-20
Fillion, I., Ouellet, N., Simard, M., Bergeron, Y., Sato, S., Bergeron, M. G. (2001). Role of Chemokines and Formyl Peptides in Pneumococcal Pneumonia-Induced Monocyte/Macrophage Recruitment. J. Immunol. 166, 7353–7361. doi: 10.4049/jimmunol.166.12.7353
Ganz, T., Metcalf, J. A., Gallin, J. I., Boxer, L. A., Lehrer, R. I. (1988). Microbicidal/cytotoxic Proteins of Neutrophils are Deficient in Two Disorders: Chediak-Higashi Syndrome and. J. Clin. Invest. 82, 552–556. doi: 10.1172/JCI113631
García-Suárez, M., del, M., Flórez, N., Astudillo, A., Vázquez, F., Villaverde, R., et al. (2007). The Role of Pneumolysin in Mediating Lung Damage in a Lethal Pneumococcal Pneumonia Murine Model. Respir. Res. 8, 3. doi: 10.1186/1465-9921-8-3
Gibbs, J., Ince, L., Matthews, L., Mei, J., Bell, T., Yang, N., et al. (2014). An Epithelial Circadian Clock Controls Pulmonary Inflammation and Glucocorticoid Action. Nat. Med. 20, 919–926. doi: 10.1038/nm.3599
Gingles, N. A., Alexander, J. E., Kadioglu, A., Andrew, P. W., Kerr, A., Mitchell, T. J., et al. (2001). Role of Genetic Resistance in Invasive Pneumococcal Infection: Identification and Study of Susceptibility and Resistance in Inbred Mouse Strains. Infect. Immun. 69 (1), 426–434. doi: 10.1128/IAI.69.1.426-434.2001
Gkatzis, K., Taghizadeh, S., Huh, D., Stainier, D. Y. R., Bellusci, S. (2018). Use of Three-Dimensional Organoids and Lung-on-a-Chip Methods to Study Lung Development, Regeneration and Disease. Eur. Respir. J. 52, 1800876. doi: 10.1183/13993003.00876-2018
Grudzinska, F. S., Brodlie, M., Scholefield, B. R., Jackson, T., Scott, A., Thickett, D. R., et al. (2020). Neutrophils in Community-Acquired Pneumonia: Parallels in Dysfunction at the Extremes of Age. Thorax 75, 164–171. doi: 10.1136/thoraxjnl-2018-212826
Hartl, D., Tirouvanziam, R., Laval, J., Greene, C. M., Habiel, D., Sharma, L., et al. (2018). Innate Immunity of the Lung: From Basic Mechanisms to Translational Medicine. J. Innate Immun. 10, 487–501. doi: 10.1159/000487057
Herbold, W., Maus, R., Hahn, I., Ding, N., Srivastava, M., Christman, J. W., et al. (2010). Importance of CXC Chemokine Receptor 2 in Alveolar Neutrophil and Exudate Macrophage Recruitment in Response to Pneumococcal Lung Infection. Infect. Immun. 78, 2620–2630. doi: 10.1128/IAI.01169-09
Huang, Z., Xie, N., Illes, P., Di Virgilio, F., Ulrich, H., Semyanov, A., et al. (2021). From Purines to Purinergic Signalling: Molecular Functions and Human Diseases. Sig Transduct. Target Ther. 6, 1–20. doi: 10.1038/s41392-021-00553-z
Hyams, C., Trzcinski, K., Camberlein, E., Weinberger, D. M., Chimalapati, S., Noursadeghi, M., et al. (2013). Streptococcus Pneumoniae Capsular Serotype Invasiveness Correlates With the Degree of Factor H Binding and Opsonization With C3b/Ic3b. Infect. Immun. 81, 354–363. doi: 10.1128/IAI.00862-12
Ivanov, S., Paget, C., Trottein, F. (2014). Role of non-Conventional T Lymphocytes in Respiratory Infections: The Case of the Pneumococcus. PloS Pathog. 10, e1004300. doi: 10.1371/journal.ppat.1004300
Janesch, P., Stulik, L., Rouha, H., Varga, C., Steinhäuser, J., Zerbs, M., et al. (2018). Age-Related Changes in the Levels and Kinetics of Pulmonary Cytokine and Chemokine Responses to Streptococcuspneumoniae in Mouse Pneumonia Models. Cytokine 111, 389–397. doi: 10.1016/j.cyto.2018.09.012
Jeong, D.-G., Jeong, E.-S., Seo, J.-H., Heo, S.-H., Choi, Y.-K. (2011). Difference in Resistance to Streptococcus Pneumoniae Infection in Mice. Lab. Anim. Res. 27, 91–98. doi: 10.5625/lar.2011.27.2.91
Jeong, D.-G., Seo, J.-H., Heo, S.-H., Choi, Y.-K., Jeong, E.-S. (2015). Tumor Necrosis Factor-Alpha Deficiency Impairs Host Defense Against Streptococcus Pneumoniae. Lab. Anim. Res. 31, 78–85. doi: 10.5625/lar.2015.31.2.78
Jochems, S. P., Marcon, F., Carniel, B. F., Holloway, M., Mitsi, E., Smith, E., et al. (2018). Inflammation Induced by Influenza Virus Impairs Human Innate Immune Control of Pneumococcus. Nat. Immunol. 19, 1299–1308. doi: 10.1038/s41590-018-0231-y
Jones, M. R., Simms, B. T., Lupa, M. M., Kogan, M. S., Mizgerd, J. P. (2005). Lung NF-κB Activation and Neutrophil Recruitment Require IL-1 and TNF Receptor Signaling During Pneumococcal Pneumonia. J. Immunol. 175, 7530–7535. doi: 10.4049/jimmunol.175.11.7530
Kadioglu, A., Andrew, P. W. (2004). The Innate Immune Response to Pneumococcal Lung Infection: The Untold Story. Trends Immunol. 25, 143–149. doi: 10.1016/j.it.2003.12.006
Kadioglu, A., Cuppone, A. M., Trappetti, C., List, T., Spreafico, A., Pozzi, G., et al. (2011a). Sex-Based Differences in Susceptibility to Respiratory and Systemic Pneumococcal Disease in Mice. J. Infect. Dis. 204, 1971–1979. doi: 10.1093/infdis/jir657
Kadioglu, A., Filippo, K. D., Bangert, M., Fernandes, V. E., Richards, L., Jones, K., et al. (2011b). The Integrins Mac-1 and α4β1 Perform Crucial Roles in Neutrophil and T Cell Recruitment to Lungs During Streptococcus Pneumoniae Infection. J. Immunol. 186, 5907–5915. doi: 10.4049/jimmunol.1001533
Kadioglu, A., Gingles, N. A., Grattan, K., Kerr, A., Mitchell, T. J., Andrew, P. W. (2000). Host Cellular Immune Response to Pneumococcal Lung Infection in Mice. Infect. Immun. 68, 492–501. doi: 10.1128/IAI.68.2.492-501.2000
Kafka, D., Ling, E., Feldman, G., Benharroch, D., Voronov, E., Givon-Lavi, N., et al. (2008). Contribution of IL-1 to Resistance to Streptococcus Pneumoniae Infection. Int. Immunol. 20, 1139–1146. doi: 10.1093/intimm/dxn071
Kamata, H., Yamamoto, K., Wasserman, G. A., Zabinski, M. C., Yuen, C. K., Lung, W. Y., et al. (2016). Epithelial Cell-Derived Secreted and Transmembrane 1a Signals to Activated Neutrophils During Pneumococcal Pneumonia. Am. J. Respir. Cell Mol. Biol. 55, 407–418. doi: 10.1165/rcmb.2015-0261OC
Knapp, S., Wieland, C. W., van ’t Veer, C., Takeuchi, O., Akira, S., Florquin, S., et al. (2004). Toll-Like Receptor 2 Plays a Role in the Early Inflammatory Response to Murine Pneumococcal Pneumonia But Does Not Contribute to Antibacterial Defense. J. Immunol. 172, 3132–3138. doi: 10.4049/jimmunol.172.5.3132
Knippenberg, S., Ueberberg, B., Maus, R., Bohling, J., Ding, N., Tarres, M. T., et al. (2015). Streptococcus Pneumoniae Triggers Progression of Pulmonary Fibrosis Through Pneumolysin. Thorax 70, 636–646. doi: 10.1136/thoraxjnl-2014-206420
Koppe, U., Suttorp, N., Opitz, B. (2012). Recognition of Streptococcus Pneumoniae by the Innate Immune System. Cell. Microbiol. 14, 460–466. doi: 10.1111/j.1462-5822.2011.01746.x
Kubes, P., Niu, X.-F., Smith, C. W., Kehrli, M. E., Jr., Reinhardt, P. H., Woodman, R. C. (1995). A Novel β1-Dependent Adhesion Pathway on Neutrophils: A Mechanism Invoked by Dihydrocytochalasin B or Endothelial Transmigration. FASEB J. 9, 1103–1111. doi: 10.1096/fasebj.9.11.7544310
Kumar, V. (2020). Pulmonary Innate Immune Response Determines the Outcome of Inflammation During Pneumonia and Sepsis-Associated Acute Lung Injury. Front. Immunol. 11. doi: 10.3389/fimmu.2020.01722
Li, K., Chen, Z., Huang, Y., Zhang, R., Luan, X., Lei, T., et al. (2019). Dysbiosis of Lower Respiratory Tract Microbiome are Associated With Inflammation and Microbial Function Variety. Respir. Res. 20, 272. doi: 10.1186/s12931-019-1246-0
Lysenko, E. S., Clarke, T. B., Shchepetov, M., Ratner, A. J., Roper, D. I., Dowson, C. G., et al. (2007). Nod1 Signaling Overcomes Resistance of S. Pneumoniae to Opsonophagocytic Killing. PloS Pathog. 3, e118. doi: 10.1371/journal.ppat.0030118
Maas, S. L., Soehnlein, O., Viola, J. R. (2018). Organ-Specific Mechanisms of Transendothelial Neutrophil Migration in the Lung, Liver, Kidney, and Aorta. Front. Immunol. 9. doi: 10.3389/fimmu.2018.02739
MacCain, W. J., Tuomanen, E. I. (2020). Mini-Review: Bioactivities of Bacterial Cell Envelopes in the Central Nervous System. Front. Cell Infect. Microbiol. 10. doi: 10.3389/fcimb.2020.588378
Mancuso, P., Lewis, C., Serezani, C. H., Goel, D., Peters-Golden, M. (2010). Intrapulmonary Administration of Leukotriene B4 Enhances Pulmonary Host Defense Against Pneumococcal Pneumonia. Infect. Immun. 78 (5), 2264–2271. doi: 10.1128/IAI.01323-09
Mancuso, R. I., Miyaji, E. N., Silva, C. C. F., Portaro, F. V., Soares-Schanoski, A., Ribeiro, O. G., et al. (2018). Impaired Expression of CXCL5 and Matrix Metalloproteinases in the Lungs of Mice With High Susceptibility to Streptococcus Pneumoniae Infection. Immun. Inflamm. Dis. 6, 128–142. doi: 10.1002/iid3.205
McColl, S. R., Clark-Lewis, I. (1999). Inhibition of Murine Neutrophil Recruitment In Vivo by CXC Chemokine Receptor Antagonists. J. Immunol. 163, 2829–2835.
McNamee, L. A., Harmsen, A. G. (2006). Both Influenza-Induced Neutrophil Dysfunction and Neutrophil-Independent Mechanisms Contribute to Increased Susceptibility to a Secondary Streptococcus Pneumoniae Infection. Infect. Immun. 74, 6707–6721. doi: 10.1128/IAI.00789-06
Melin, M., Trzciński, K., Meri, S., Käyhty, H., Väkeväinen, M. (2010). The Capsular Serotype of Streptococcus Pneumoniae Is More Important Than the Genetic Background for Resistance to Complement. Infect. Immun. 78, 5262–5270. doi: 10.1128/IAI.00740-10
Mizgerd, J. P., Horwitz, B. H., Quillen, H. C., Scott, M. L., Doerschuk, C. M. (1999). Effects of CD18 Deficiency on the Emigration of Murine Neutrophils During Pneumonia. J. Immunol. 163, 995–999.
Mizgerd, J. P., Kubo, H., Kutkoski, G. J., Bhagwan, S. D., Scharffetter-Kochanek, K., Beaudet, A. L., et al. (1997). Neutrophil Emigration in the Skin, Lungs, and Peritoneum: Different Requirements for CD11/CD18 Revealed by CD18-Deficient Mice. J. Exp. Med. 186, 1357–1364. doi: 10.1084/jem.186.8.1357
Mizgerd, J. P., Meek, B. B., Kutkoski, G. J., Bullard, D. C., Beaudet, A. L., Doerschuk, C. M. (1996). Selectins and Neutrophil Traffic: Margination and Streptococcus Pneumoniae-Induced Emigration in Murine Lungs. J. Exp. Med. 184, 639–645. doi: 10.1084/jem.184.2.639
Mizrachi-Nebenzahl, Y., Lifshitz, S., Teitelbaum, R., Novick, S., Levi, A., Benharroch, D., et al. (2003). Differential Activation of the Immune System by Virulent Streptococcus Pneumoniae Strains Determines Recovery or Death of the Host. Clin. Exp. Immunol. 134, 23–31. doi: 10.1046/j.1365-2249.2003.02261.x
Moreland, J. G., Bailey, G. (2006). Neutrophil Transendothelial Migration In Vitro to Streptococcus Pneumoniae is Pneumolysin Dependent. Am. J. Physiology-Lung Cell. Mol. Physiol. 290, L833–L840. doi: 10.1152/ajplung.00333.2005
Moreland, J. G., Bailey, G., Nauseef, W. M., Weiss, J. P. (2004). Organism-Specific Neutrophil-Endothelial Cell Interactions in Response to Escherichia Coli, Streptococcus Pneumoniae, and Staphylococcus Aureus. J. Immunol. 172, 426–432. doi: 10.4049/jimmunol.172.1.426
Müller-Redetzky, H., Kellermann, U., Wienhold, S.-M., Gutbier, B., Lienau, J., Hellwig, K., et al. (2020). Neutralizing Complement C5a Protects Mice With Pneumococcal Pulmonary Sepsis. Anesthesiology 132, 795–807. doi: 10.1097/ALN.0000000000003149
Muñoz, M. F., Griffith, T. N., Contreras, J. E. (2021). Mechanisms of ATP Release in Pain: Role of Pannexin and Connexin Channels. Purinergic Signal 17, 549–561. doi: 10.1007/s11302-021-09822-6
Naucler, P., Darenberg, J., Morfeldt, E., Ortqvist, A., Henriques Normark, B. (2013). Contribution of Host, Bacterial Factors and Antibiotic Treatment to Mortality in Adult Patients With Bacteraemic Pneumococcal Pneumonia. Thorax 68, 571–579. doi: 10.1136/thoraxjnl-2012-203106
Németh, T., Sperandio, M., Mócsai, A. (2020). Neutrophils as Emerging Therapeutic Targets. Nat. Rev. Drug Discov. 19, 253–275. doi: 10.1038/s41573-019-0054-z
Ngamsri, K.-C., Wagner, R., Vollmer, I., Stark, S., Reutershan, J. (2010). Adenosine Receptor A1 Regulates Polymorphonuclear Cell Trafficking and Microvascular Permeability in Lipopolysaccharide-Induced Lung Injury. J. Immunol. 185, 4374–4384. doi: 10.4049/jimmunol.1000433
Nieminen, J., St-Pierre, C., Bhaumik, P., Poirier, F., Sato, S. (2008). Role of Galectin-3 in Leukocyte Recruitment in a Murine Model of Lung Infection by Streptococcus Pneumoniae. J. Immunol. 180, 2466–2473. doi: 10.4049/jimmunol.180.4.2466
Ohms, M., Möller, S., Laskay, T. (2020). An Attempt to Polarize Human Neutrophils Toward N1 and N2 Phenotypes In Vitro. Front. Immunol. 11. doi: 10.3389/fimmu.2020.00532
Palmblad, J., Malmsten, C. L., Udén, A.-M., Rådmark, O., Engstedt, L., Samuelsson, B. (1981). Leukotriene B4 Is a Potent and Stereospecific Stimulator of Neutrophil Chemotaxis and Adherence. Blood 58, 658–661. doi: 10.1182/blood.V58.3.658.658
Paterson, G. K., Mitchell, T. J. (2006). Innate Immunity and the Pneumococcus. Microbiology 152, 285–293. doi: 10.1099/mic.0.28551-0
Paton, J. C., Trappetti, C. (2019). Streptococcus Pneumoniae Capsular Polysaccharide. Microbiol. Spectr. 7 (2). doi: 10.1128/microbiolspec.GPP3-0019-2018
Paudel, S., Baral, P., Ghimire, L., Bergeron, S., Jin, L., DeCorte, J. A., et al. (2019). CXCL1 Regulates Neutrophil Homeostasis in Pneumonia-Derived Sepsis Caused by Streptococcus Pneumoniae Serotype 3. Blood 133, 1335–1345. doi: 10.1182/blood-2018-10-878082
Peñaloza, H. F., Nieto, P. A., Muñoz-Durango, N., Salazar-Echegarai, F. J., Torres, J., Parga, M. J., et al. (2015). Interleukin-10 Plays a Key Role in the Modulation of Neutrophils Recruitment and Lung Inflammation During Infection by Streptococcus Pneumoniae. Immunology 146, 100–112. doi: 10.1111/imm.12486
Peter, A., Fatykhova, D., Kershaw, O., Gruber, A. D., Rueckert, J., Neudecker, J., et al. (2017). Localization and Pneumococcal Alteration of Junction Proteins in the Human Alveolar-Capillary Compartment. Histochem. Cell Biol. 147, 707–719. doi: 10.1007/s00418-017-1551-y
Planagumà, A., Domènech, T., Pont, M., Calama, E., García-González, V., López, R., et al. (2015). Combined Anti CXC Receptors 1 and 2 Therapy is a Promising Anti-Inflammatory Treatment for Respiratory Diseases by Reducing Neutrophil Migration and Activation. Pulm Pharmacol. Ther. 34, 37–45. doi: 10.1016/j.pupt.2015.08.002
Ramos-Sevillano, E., Urzainqui, A., de Andrés, B., González-Tajuelo, R., Domenech, M., González-Camacho, F., et al. (2016). PSGL-1 on Leukocytes Is a Critical Component of the Host Immune Response Against Invasive Pneumococcal Disease. PloS Pathog. 12, e1005500. doi: 10.1371/journal.ppat.1005500
Rayner, C. F., Jackson, A. D., Rutman, A., Dewar, A., Mitchell, T. J., Andrew, P. W., et al. (1995). Interaction of Pneumolysin-Sufficient and -Deficient Isogenic Variants of Streptococcus Pneumoniae With Human Respiratory Mucosa. Infect. Immun. 63 (2), 442–447. doi: 10.1128/iai.63.2.442-447.1995
Ren, B., McCrory, M. A., Pass, C., Bullard, D. C., Ballantyne, C. M., Xu, Y., et al. (2004). The Virulence Function of Streptococcus Pneumoniae Surface Protein A Involves Inhibition of Complement Activation and Impairment of Complement Receptor-Mediated Protection. J. Immunol. 173, 7506–7512. doi: 10.4049/jimmunol.173.12.7506
Rennard, S. I., Dale, D. C., Donohue, J. F., Kanniess, F., Magnussen, H., Sutherland, E. R., et al. (2015). CXCR2 Antagonist MK-7123. A Phase 2 Proof-Of-Concept Trial for Chronic Obstructive Pulmonary Disease. Am. J. Respir. Crit. Care Med. 191, 1001–1011. doi: 10.1164/rccm.201405-0992OC
Ripoll, V. M., Kadioglu, A., Cox, R., Hume, D. A., Denny, P. (2010). Macrophages From BALB/c and CBA/Ca Mice Differ in Their Cellular Responses to Streptococcus Pneumoniae. J. Leukocyte Biol. 87, 735–741. doi: 10.1189/jlb.0509359
Ritchie, N. D., Ritchie, R., Bayes, H. K., Mitchell, T. J., Evans, T. J. (2018). IL-17 can be Protective or Deleterious in Murine Pneumococcal Pneumonia. PloS Pathog. 14, e1007099. doi: 10.1371/journal.ppat.1007099
Rolston, K. V. (2001). The Spectrum of Pulmonary Infections in Cancer Patients. Curr. Opin. Oncol. 13, 218–223. doi: 10.1097/00001622-200107000-00002
Rubins, J. B., Charboneau, D., Paton, J. C., Mitchell, T. J., Andrew, P. W., Janoff, E. N. (1995). Dual Function of Pneumolysin in the Early Pathogenesis of Murine Pneumococcal Pneumonia. J. Clin. Invest. 95, 142–150. doi: 10.1172/JCI117631
Rubins, J. B., Duane, P. G., Charboneau, D., Janoff, E. N. (1992). Toxicity of Pneumolysin to Pulmonary Endothelial Cells In Vitro. Infect. Immun. 60, 1740–1746. doi: 10.1128/iai.60.5.1740-1746.1992
Rubins, J. B., Duane, P. G., Clawson, D., Charboneau, D., Young, J., Niewoehner, D. E. (1993). Toxicity of Pneumolysin to Pulmonary Alveolar Epithelial Cells. Infect. Immun. 61 (4), 1352–1358 doi: 10.1128/iai.61.4.1352-1358.1993
Rubins, J. B., Mitchell, T. J., Andrew, P. W., Niewoehner, D. E. (1994). Pneumolysin Activates Phospholipase A in Pulmonary Artery Endothelial Cells. Infect. Immun. 62 (9), 3829–3836. doi: 10.1128/iai.62.9.3829-3836.1994
Scheiermann, C., Gibbs, J., Ince, L., Loudon, A. (2018). Clocking in to Immunity. Nat. Rev. Immunol. 18, 423–437. doi: 10.1038/s41577-018-0008-4
Schingnitz, U., Hartmann, K., Macmanus, C. F., Eckle, T., Zug, S., Colgan, S. P., et al. (2010). Signaling Through the A2B Adenosine Receptor Dampens Endotoxin-Induced Acute Lung Injury. J. Immunol. 184, 5271–5279. doi: 10.4049/jimmunol.0903035
Schmeck, B., Huber, S., Moog, K., Zahlten, J., Hocke, A. C., Opitz, B., et al. (2006). Pneumococci Induced TLR- and Rac1-Dependent NF-κb-Recruitment to the IL-8 Promoter in Lung Epithelial Cells. Am. J. Physiol. Lung Cell. Mol. Physiol. 290, L730–L737. doi: 10.1152/ajplung.00271.2005
Seyoum, B., Yano, M., Pirofski, L. (2011). The Innate Immune Response to Streptococcus Pneumoniae in the Lung Depends on Serotype and Host Response. Vaccine 29, 8002–8011. doi: 10.1016/j.vaccine.2011.08.064
Shenoy, A. T., Wasserman, G. A., Arafa, E. I., Wooten, A. K., Smith, N. M. S., Martin, I. M. C., et al. (2020). Lung CD4 + Resident Memory T Cells Remodel Epithelial Responses to Accelerate Neutrophil Recruitment During Pneumonia. Mucosal Immunol. 13, 334–343. doi: 10.1038/s41385-019-0229-2
Simmons, S. R., Bhalla, M., Herring, S. E., Tchalla, E. Y. I., Bou Ghanem, E. N. (2021). Older But Not Wiser: The Age-Driven Changes in Neutrophil Responses During Pulmonary Infections. Infect. Immun. 89, e00653-20. doi: 10.1128/IAI.00653-20
Smith, E. L., Wheeler, I., Adler, H., Ferreira, D. M., Sá-Leão, R., Abdullahi, O., et al. (2020). Upper Airways Colonisation of Streptococcus Pneumoniae in Adults Aged 60 Years and Older: A Systematic Review of Prevalence and Individual Participant Data Meta-Analysis of Risk Factors. J. Infect. 81, 540–548. doi: 10.1016/j.jinf.2020.06.028
Souza-Fernandes, A. B., Pelosi, P., Rocco, P. R. (2006). Bench-To-Bedside Review: The Role of Glycosaminoglycans in Respiratory Disease. Crit. Care 10, 237. doi: 10.1186/cc5069
Spelmink, L., Sender, V., Hentrich, K., Kuri, T., Plant, L., Henriques-Normark, B. (2016). Toll-Like Receptor 3/TRIF-Dependent IL-12p70 Secretion Mediated by Streptococcus Pneumoniae RNA and Its Priming by Influenza A Virus Coinfection in Human Dendritic Cells. mBio. 7(2), e00168–16 doi: 10.1128/mBio.00168-16
Srivastava, A., Henneke, P., Visintin, A., Morse, S. C., Martin, V., Watkins, C., et al. (2005). The Apoptotic Response to Pneumolysin is Toll-Like Receptor 4 Dependent and Protects Against Pneumococcal Disease. Infect. Immun. 73, 6479–6487. doi: 10.1128/IAI.73.10.6479-6487.2005
Sundaresh, B., Xu, S., Noonan, B., Mansour, M. K., Leong, J. M., van Opijnen, T. (2021). Host-Informed Therapies for the Treatment of Pneumococcal Pneumonia. Trends Mol. Med. 27, 971–989. doi: 10.1016/j.molmed.2021.07.008
Sun, K., Salmon, S. L., Lotz, S. A., Metzger, D. W. (2007). Interleukin-12 Promotes Gamma Interferon-Dependent Neutrophil Recruitment in the Lung and Improves Protection Against Respiratory Streptococcus Pneumoniae Infection. Infect. Immun. 75, 1196–1202. doi: 10.1128/IAI.01403-06
Surewaard, B. G. J., Trzciński, K., Jacobino, S. R., Hansen, I. S., Vughs, M. M., Sanders, E. A. M., et al. (2013). Pneumococcal Immune Evasion: ZmpC Inhibits Neutrophil Influx. Cell. Microbiol. 15, 1753–1765. doi: 10.1111/cmi.12147
Takashima, K., Tateda, K., Matsumoto, T., Iizawa, Y., Nakao, M., Yamaguchi, K. (1997). Role of Tumor Necrosis Factor Alpha in Pathogenesis of Pneumococcal Pneumonia in Mice. Infect. Immun. 65 (1), 257–260. doi: 10.1128/iai.65.1.257-260.1997
Tasaka, S., Richer, S. E., Mizgerd, J. P., Doerschuk, C. M. (2002). Very Late Antigen-4 in CD18-Independent Neutrophil Emigration During Acute Bacterial Pneumonia in Mice. Am. J. Respir. Crit. Care Med. 166, 53–60. doi: 10.1164/rccm.2105034
Tecchio, C., Micheletti, A., Cassatella, M. A. (2014). Neutrophil-Derived Cytokines: Facts Beyond Expression. Front. Immunol. 5. doi: 10.3389/fimmu.2014.00508
Tonnaer, E. L. G. M., Hafmans, T. G., Van Kuppevelt, T. H., Sanders, E. A. M., Verweij, P. E., Curfs, J. H. A. J. (2006). Involvement of Glycosaminoglycans in the Attachment of Pneumococci to Nasopharyngeal Epithelial Cells. Microb. Infect. 8, 316–322. doi: 10.1016/j.micinf.2005.06.028
Tseng, C. W., Liu, G. Y. (2014). Expanding Roles of Neutrophils in Aging Hosts. Curr. Opin. Immunol. 29, 43–48. doi: 10.1016/j.coi.2014.03.009
Wagner, R., Ngamsri, K.-C., Stark, S., Vollmer, I., Reutershan, J. (2010). Adenosine Receptor A3 is a Critical Mediator in LPS-Induced Pulmonary Inflammation. Am. J. Physiol. Lung Cell Mol. Physiol. 299, L502–L512. doi: 10.1152/ajplung.00083.2010
Weiser, J. N., Ferreira, D. M., Paton, J. C. (2018). Streptococcus Pneumoniae : Transmission, Colonization and Invasion. Nat. Rev. Microbiol. 16, 355–367. doi: 10.1038/s41579-018-0001-8
Williams, A. E., José, R. J., Brown, J. S., Chambers, R. C. (2015). Enhanced Inflammation in Aged Mice Following Infection With Streptococcus Pneumoniae Is Associated With Decreased IL-10 and Augmented Chemokine Production. Am. J. Physiology-Lung Cell. Mol. Physiol. 308, L539–L549. doi: 10.1152/ajplung.00141.2014
Witzenrath, M., Gutbier, B., Hocke, A. C., Schmeck, B., Hippenstiel, S., Berger, K., et al. (2006). Role of Pneumolysin for the Development of Acute Lung Injury in Pneumococcal Pneumonia. Crit. Care Med. 34, 1947–1954. doi: 10.1097/01.CCM.0000220496.48295.A9
Yamamoto, K., Ahyi, A.-N. N., Pepper-Cunningham, Z. A., Ferrari, J. D., Wilson, A. A., Jones, M. R., et al. (2014). Roles of Lung Epithelium in Neutrophil Recruitment During Pneumococcal Pneumonia. Am. J. Respir. Cell Mol. Biol. 50, 253–262. doi: 10.1165/rcmb.2013-0114OC
Zheng, Y., Sefik, E., Astle, J., Solis, A. G., Öz, H. H., Jackson, R., et al. (2022). Human Neutrophil Development and Functionality are Enabled in a Humanized Mouse Model. bioRxiv 481352. doi: 10.1101/2022.02.25.481352
Keywords: neutrophil, pneumonia, Streptococcus pneumoniae (pneumococcus), lung, migration
Citation: Palmer CS and Kimmey JM (2022) Neutrophil Recruitment in Pneumococcal Pneumonia. Front. Cell. Infect. Microbiol. 12:894644. doi: 10.3389/fcimb.2022.894644
Received: 12 March 2022; Accepted: 18 April 2022;
Published: 13 May 2022.
Edited by:
Shumin Tan, Tufts University School of Medicine, United StatesReviewed by:
Elsa Bou Ghanem, University at Buffalo, United StatesWalter Adams, San Jose State University, United States
Copyright © 2022 Palmer and Kimmey. This is an open-access article distributed under the terms of the Creative Commons Attribution License (CC BY). The use, distribution or reproduction in other forums is permitted, provided the original author(s) and the copyright owner(s) are credited and that the original publication in this journal is cited, in accordance with accepted academic practice. No use, distribution or reproduction is permitted which does not comply with these terms.
*Correspondence: Jacqueline M. Kimmey, jkimmey@ucsc.edu