- 1Instituto Multidisciplinario de Investigaciones en Patologías Pediátricas (IMIPP), Consejo Nacional de Investigaciones Científicas y Tecnológicas (CONICET)-Gobierno de la Ciudad de Buenos Aires (GCBA), Servicio de Parasitología y Enfermedad de Chagas, Hospital de Niños “Dr. Ricardo Gutiérrez, Ministerio de Salud, Buenos Aires, Argentina
- 2Instituto de Investigaciones Biomédicas (INBIOMED), Facultad de Medicina Universidad de Buenos Aires (UBA) – CONICET, Buenos Aires, Argentina
- 3Instituto Nacional de Parasitología (INP) ‘Dr. Mario Fatala Chaben’-Administración Nacional de Laboratorios e Institutos de Salud (ANLIS) ‘Dr. Carlos G. Malbrán’, CONICET, Buenos Aires, Argentina
- 4Instituto de Microbiología y Parasitología Médica (IMPaM), Universidad de Buenos Aires, Buenos Aires, Argentina
- 5Departamento de Ciencias Básicas, Universidad Nacional de Luján, Buenos Aires, Argentina
- 6Division of Pediatric Clinical Pharmacology, Department of Pediatrics, Schulich School of Medicine & Dentistry, University of Western Ontario, London, ON, Canada
Drug repurposing and combination therapy have been proposed as cost-effective strategies to improve Chagas disease treatment. Miltefosine (MLT), a synthetic alkylphospholipid initially developed for breast cancer and repositioned for leishmaniasis, is a promising candidate against Trypanosoma cruzi infection. This study evaluates the efficacy of MLT as a monodrug and combined with benznidazole (BZ) in both in vitro and in vivo models of infection with T. cruzi (VD strain, DTU TcVI). MLT exhibited in vitro activity on amastigotes and trypomastigotes with values of IC50 = 0.51 µM (0.48 µM; 0,55 µM) and LC50 = 31.17 µM (29.56 µM; 32.87 µM), respectively. Drug interaction was studied with the fixed-ration method. The sum of the fractional inhibitory concentrations (ΣFICs) resulted in ∑FIC= 0.45 for trypomastigotes and ∑FIC= 0.71 for amastigotes, suggesting in vitro synergistic and additive effects, respectively. No cytotoxic effects on host cells were observed. MLT efficacy was also evaluated in a murine model of acute infection alone or combined with BZ. Treatment was well tolerated with few adverse effects, and all treated animals displayed significantly lower mean peak parasitemia and mortality than infected non-treated controls (p<0.05). The in vivo studies showed that MLT led to a dose-dependent parasitostatic effect as monotherapy which could be improved by combining with BZ, preventing parasitemia rebound after a stringent immunosuppression protocol. These results support MLT activity in clinically relevant stages from T. cruzi, and it is the first report of positive interaction with BZ, providing further support for evaluating combined schemes using MLT and exploring synthetic alkylphospholipids as drug candidates.
Introduction
Chagas disease, caused by the hemoflagellate Trypanosoma cruzi, is considered a neglected tropical disease due to the scarcity of safe and effective treatments 1. Current therapeutic options are limited to nifurtimox (NFX) and benznidazole (BZ), both developed over 40 years ago.
Etiological treatment during the acute stage results in high rates of parasitemia clearance and serological negativity, while treatment success during the chronic phase is currently arguable. Adverse events are usual, especially in adults, forcing treatment discontinuation (Pérez-Molina and Molina, 2018).
Despite the urgent need to develop safer drugs, the pharmaceutical industry has shown limited interest in research for new chemical entities, probably due to the socioeconomic condition of most affected patients (Paulino et al., 2005).
Drug repurposing (i.e., the study of drugs already developed and tested for other diseases) (Chatelain and Ioset, 2011) and combination therapy arise as cost-effective strategies to fill the gap in the drug discovery pipeline (Keenan et al., 2013a; Andrews et al., 2014). Additionally, a positive drug combination increases drug efficacy by targeting multiple metabolic pathways, minimizing the risk of drug resistance, and reducing the frequency and intensity of dose-dependent adverse reactions (Field et al., 2017).
Initially developed for treating skin metastases in breast tumors, miltefosine (MLT), also known as hexadecylphosphocholine, is the first and to date only oral drug for visceral and cutaneous leishmaniasis (Dorlo et al., 2012). Extensive experience with MLT in leishmaniasis clinical trials suggests an excellent safety profile for patients, including pediatric patients, with low to moderate adverse events (Singh et al., 2006; Mbui et al., 2019). Still, some concerns remain regarding MLT effects on the gastrointestinal tract and potential teratogenicity (Dorlo et al., 2012).
MLT is considered a protein kinase B (PKB) inhibitor with a key role in intracellular signaling for cell viability (Dorlo et al., 2012). However, the mechanisms of action related to its anti-protozoal activity are not fully clarified yet. Some evidence suggests an inhibitory effect on phosphatidylcholine and sphingomyelin synthesis, triggering apoptosis (Maya et al., 2007; Apt, 2010) or inhibiting the mitochondrial cytochrome c oxidase and altering the organelle membrane (Dorlo et al., 2012; Ghosh et al., 2013). Similarly, it was proposed that MLT inhibits the parasite phosphatidylcholine biosynthesis through the transmethylation pathway (Lira et al., 2001).
Recently, it was proposed that MLT targeted the sphingosine-activated plasma membrane directly Ca+2 channel, both on Leishmania donovani and T. cruzi, affecting the intracellular calcium homeostasis (Benaim et al., 2020).
Previous reports indicated that MLT exhibits both in vitro (Croft et al., 1996; Santa-Rita et al., 2000) and in vivo activity (Saraiva et al., 2002; Martinez-Peinado et al., 2021) against T. cruzi.
However, in vivo efficacy was evaluated by different monotherapy treatment schemes and cure criteria hampering the assessment of the MLT ability to eradicate T. cruzi infection. Therefore, we conducted a set of in vitro and in vivo experiments based on previous guidelines (Romanha et al., 2010) to evaluate MLT efficacy alone and in combination with BZ.
Materials and Methods
In Vitro Studies
Cell culture
Vero-C76 cells (obtained from Asociación Banco Argentino de Células, an ATCC-certified cell bank) were cultured in T25 or T75 flasks with RPMI-1640 medium (Life Technologies Corporation, Grand Island, New York, USA) at 37°C in an atmosphere of 5% CO2, supplemented with 2.5 g/L sodium bicarbonate and 5% fetal bovine serum (FBS) (Natocor, Córdoba, Argentina) and penicillin (100 UI/mL) - streptomycin (50 μg/mL) or gentamicin (25 μg/mL).
Parasite Strain
The VD strain of T. cruzi (DTU TcVI) was previously characterized (Gulin et al., 2018), and it was used throughout these studies. Bloodstream trypomastigotes were obtained from infected CF-1 outbred mice at parasitemia peak, isolated as previously described (Brossas et al., 2017), and maintained in cell culture for in vitro assays or used directly for in vivo studies.
Trypanocidal Activity
The trypanocidal activity was assayed on cell culture-derived trypomastigotes. Experiments were carried out in 96-well microplates containing 106 parasites/mL, in 100 µL as final volume. Serially diluted (160-0.156 µM) pure drugs (MLT, BZ, and NFX) were added to the wells, and the plates were incubated at 37°C for 24 h. After incubation, reduction in T. cruzi viability was determined by counting motile trypomastigotes in Kova International, Inc., Garden Grove, CA, USA. Each drug concentration was evaluated in triplicate, and control cultures were maintained without the drug. The lytic concentration 50% (LC50), defined as the drug concentration that resulted in a 50% reduction compared to the untreated control, was estimated by non-linear regression analysis plotting the percentage of viable trypomastigotes against the log of drug concentration (Rodrigues et al., 2014).
Inhibition of Trypomastigotes Egress Test
Egress of trypomastigote forms from host cells was used as an indirect method to assess the arrest of amastigote development. Vero C-76 cells, plated at a density of 104 cells/well in 96-well plates, were infected with 106 parasites/mL and incubated at 37°C and 5% CO2 for 24 h. The wells were washed with PBS 1X to remove non-attached parasites, and the infected cell cultures were treated with serially diluted (160-0.156 µM) pure drugs (MLT, BZ, NFX) by triplicate. Control cell cultures were maintained without treatment. Trypomastigotes’ egress from host cells was evaluated on the 5th day post-infection (dpi) as previously described (Polanco-Hernández et al., 2012). The number of parasites egressed was determined by counting viable trypomastigotes in the supernatant using the KOVA Glasstic slides®. The inhibitory concentration 50% (IC50), defined as the drug concentration resulting in a 50% reduction of trypomastigotes’ egress from infected cells, was estimated by non-linear regression analysis plotting the percentage of viable trypomastigotes against the log of drug concentration (da Silva et al., 2010; Polanco-Hernández et al., 2012).
Determination of Cytotoxicity
Vero C-76 cells were plated at a density of 104 cells in 96-wells plates and maintained at 37°C in an atmosphere of 5% CO2 overnight. Then, they were incubated in the presence of the compounds in serially diluted concentrations (160-0.156 µM). Each drug concentration was evaluated in triplicate, and control cultures were maintained without the drug. After 5 days, plates were washed and 100 μL of RPMI-5% SFB with 10% of a 3 mM resazurin solution (Sigma Aldrich, Saint Louis, MO, USA), were added to each well. The plate was incubated at 37°C with a 5% CO2 atmosphere for 4 h, and the reduction of resazurin was read in an ELISA reader (Thermo Fisher Scientific Oy, Vantaa, Finland) at 570-595 nm. Optical densities were analyzed to obtain the dose-response curve and the cytotoxic concentration 50 (CC50), defined as the concentration of compound that reduced the cell viability by 50% compared to untreated controls. Finally, the selectivity index (SI) was calculated as the CC50 for Vero cells/IC50 for T. cruzi parasites (Miranda et al., 2015).
Drug Combination Assays
In vitro tests were carried out to identify the LC50 and IC50 of each drug separately (see above). For combination studies, dilutions were made by the fixed-ratio method, where the LC50 or the IC50 of one of the drugs remained constant, and the other drug was diluted in fixed ratios of the obtained LC50 or IC50 (Fivelman et al., 2004).
To assess trypanocidal activity, tested MLT concentrations (30.85; 7.71; 1.93 µM) were added to the pre-determined LC50 value of BZ at a fixed concentration. Similarly, the MLT LC50 value was added to serially diluted BZ (9.43; 2.36; 0.59 µM).
To study the inhibitory amastigote effect, selected MLT concentrations (0.51; 0.25; 0.12; 0.06; 0.03) were added to the pre-determined BZ IC50 value at fixed concentration. Similarly, the MLT IC50 was added to serial dilutions of BZ (0.72; 0.36; 0.18; 0.09; 0.04 µM).
For both cases, the Fractional Inhibitory Concentration Index (FICI) was calculated according to Cantin and Chamberland (1993) where,
LC50 or IC50 of A in combination LC50 or IC50 of B in combination
LC50 or IC50 of A alone LC50 or IC50 of B alone
FICI values were interpreted as follows: ≤ 0.5, synergistic; 0.5 > 4, additive and ≥ 4 antagonistic interaction (Odds, 2003).
In Vivo Assays
Experimental Animals
Twenty-one-day-old female BALB/cJ mice (16 ± 2 grams) were purchased from the Faculty of Veterinary Sciences, University of Buenos Aires, Argentina, and housed under conventional closed barriers at Ricardo Gutiérrez Children’s Hospital animal facilities (Buenos Aires, Argentina).
Animals were kept at five mice per cage in 600 cm2 polycarbonate cages, including plastic tubes as environmental enrichment and nesting material. Animals were individually identified, and cages were properly labeled. Cages were filled with irradiated chip-bedding, which was changed once a week. Mice had access to food (Rata-Ratón Cooperación®, Buenos Aires, Argentina) and filtered water ad libitum. Macroenvironmental conditions included a 12:12-h light: dark cycle (starting at 6 a.m.), controlled temperature (20 ± 2°C), and humidity (55 ± 10%).
Procedures for housing and handling animals followed international guidelines for animal care and welfare (National Research Council, 2011). The study protocol was approved by the Institutional Animal Care and Use Committee from the Faculty of Veterinary Sciences (University of Buenos Aires) (Protocol #2014/04).
Infection and Treatment Schedules
Five-week-old mice were infected by intraperitoneal (ip) injection with 500 bloodstream trypomastigotes from the VD strain of T. cruzi. After the infection was established, mice with patent parasitemia were assigned by simply randomization to treatment groups as indicated in Table 1 (monotherapy) and Table 2 (combinatory therapy).
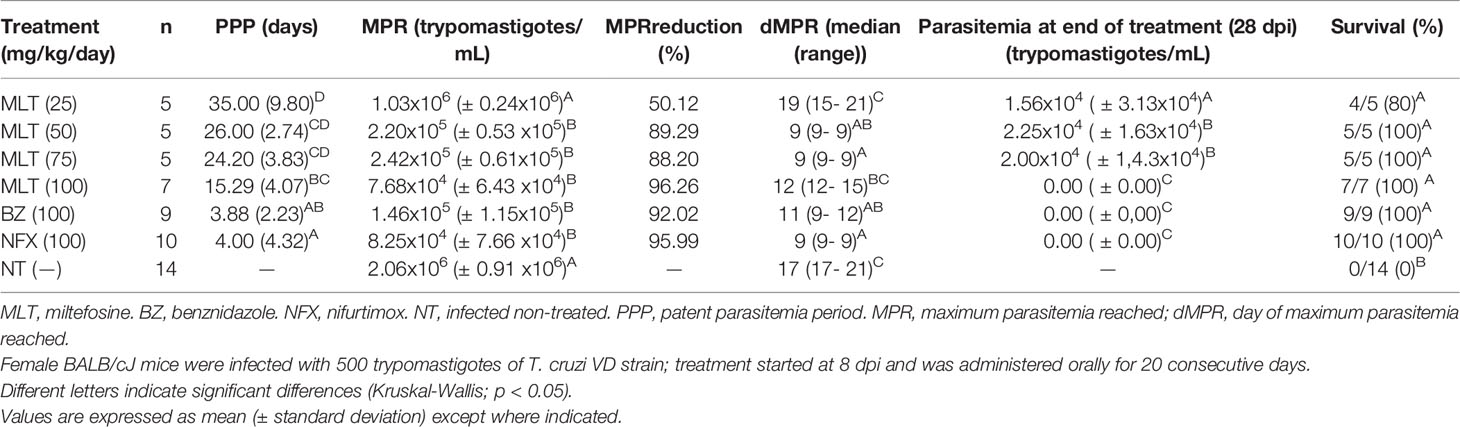
Table 1 Effect of miltefosine, benznidazole, or nifurtimox on parasitemia parameters in BALB/cJ mice infected with Trypanosoma cruzi (VD strain) a.
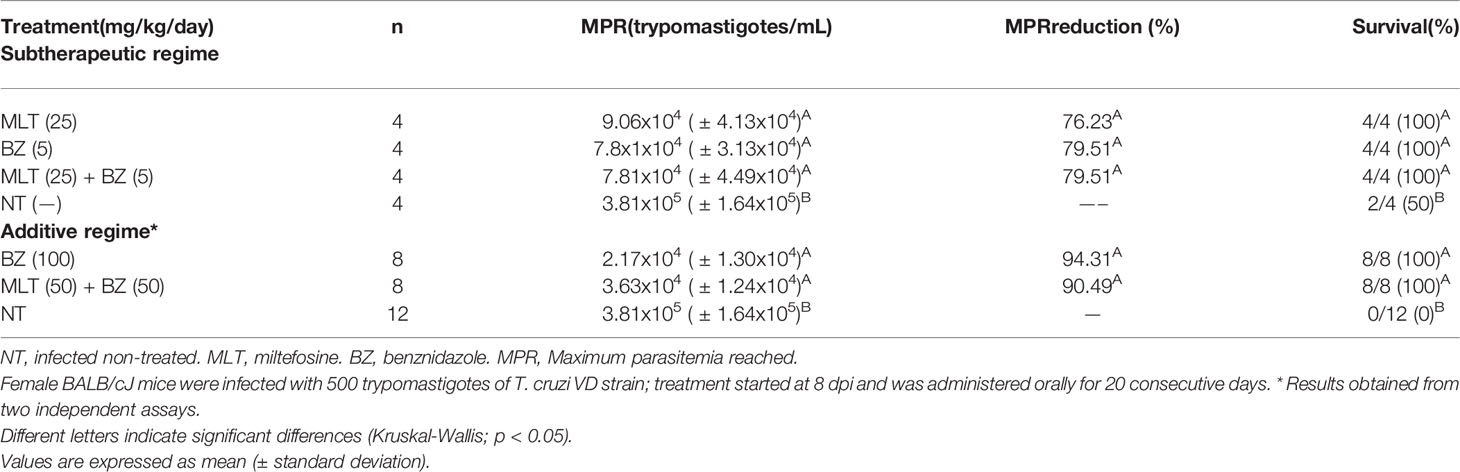
Table 2 Effect of miltefosine alone or combined with benznidazole on parasitemia parameters in BALB/cJ mice infected with Trypanosoma cruzi (VD strain).
MLT (pure product provided by Laboratorio Dr. Lazar & Cía. S.A.Q. e I, Buenos Aires, Argentina) was prepared in a 100 mg/mL work solution and dissolved in sterile distilled water. BZ and NFX (Radanil, Roche, Buenos Aires, Argentina and Lampit, Bayer, Buenos Aires, Argentina) were used as reference treatments in this study. Commercial tablets were crushed and suspended in 0.25% carboxymethylcellulose solution (Sigma Aldrich, Saint Louis, MO, USA). To avoid potential pharmaceutical interactions between drugs, BZ was administered at least an hour before MLT in the combination treatment.
Treatment started immediately upon onset of parasitemia in all animals and it was administered daily for 20 consecutive days by oral route in 50 µL as final volume. Doses, length of treatment, and route of administration for monotherapy schemes were chosen based on published data (Saraiva et al., 2002; Romanha et al., 2010). For combinatory treatments, doses were carefully chosen to obtain sub-therapeutic (i.e., doses that are administered separately do not lead to parasitemia suppression) (Gulin et al., 2020) or additive effects (i.e., doses that are administered together add up to the therapeutic dose that leads to parasitemia suppression).
Evaluation of Treatment Response
Clinical condition, including physical appearance and behavior based on previously established parameters (Olfert and Godson, 2000), as well as body weight and temperature, were recorded weekly.
Blood parasitemia was evaluated twice a week with a hemocytometer, a technique with a detection limit of 12,500 parasites/mL. Briefly, 5 μL of blood obtained from the vein tail was diluted 1:5 in lysis buffer (Tris-NH4Cl 0.83%, pH=7.2), and parasites were counted in a Neubauer chamber at 400X magnification.
Additional parameters were calculated to assess drug efficacy: patent parasitemia period (PPP), defined as the number of days in which parasitemia can be detected by direct observation; the maximum parasitemia reached (MPR), considered as the maximum parasitemia value achieved in each infected animal; and day of MPR (dMPR). Mortality was registered daily. Pre-established anticipated endpoints were used to avoid unnecessary pain and stress, and animals were euthanized if they fulfilled any of these criteria (i.e., 20% weight loss from initial body weight, body temperature lower than 33.5°C, or parasitemia ≥2x106 trypomastigotes/mL). Euthanasia was performed with CO2 inhalation in a saturated chamber or with sodium pentothal overdose (300 mg/kg, ip).
Cyclophosphamide-Induced Immune Suppression and Assessment of Cure
After the treatment schemes were completed, mice with negative parasitemia were submitted to an immunosuppression protocol with cyclophosphamide (CYP). CYP (Filaxis®; Martínez, Buenos Aires, Argentina) was diluted in sterile sodium chloride 0.45% and administered by ip route at 200 mg/kg once a week for 28 days. During the immunosuppression cycle, the presence of blood trypomastigotes was assessed periodically. If parasitemia did not rebound, animals were euthanized and blood, heart, and skeletal muscle samples were collected for parasite DNA quantification by qPCR and histology evaluation.
PCR Sampling and Preparation
Blood samples were obtained by submandibular vein puncture (Golde et al., 2005) and collected in sterile cryotubes with 1:3 guanidine-HCl 6M-EDTA 0.2M pH 8 buffer. Skeletal muscle from the rear legs and heart were obtained and rinsed with sterile distilled water before being collected in separate sterile cryotubes. Samples were stored at -20°C until processing.
DNA was extracted with High Pure PCR Template Preparation Kit (Roche Diagnostics GmbH, Mannheim, Germany) according to the manufacturer’s protocol. To discard inhibitions, an internal amplification control (0.2 ng) was included in each sample before starting the DNA extraction procedure (Duffy et al., 2013). Extracted DNA was quantified by spectrophotometry at 260 nm wavelength in a Nanodrop 1000 spectrophotometer (Thermo Fisher Scientific, Wilmington, DE, USA) and stored at -20°C until use.
Quantitative PCR
Amplification was performed using oligonucleotides targeted to a 166 bp T. cruzi satellite DNA fragment and IAC on a StepOne PCR system (Applied Biosystems, Foster City, California, USA) as described elsewhere (Duffy et al., 2013). qPCR conditions and standard parasite curve for data analysis were performed as previously described (Gulin et al., 2018). The efficiency of amplification was determined using the following calculation: Efficiency (E) = 10(−1/slope), using Step One Software 2.1 (Applied Biosystems, Foster City, California, USA).
Positive and negative blood or tissue samples and reagent controls were run in each assay. DNA extraction, mixing, and qPCR reaction were performed in separate areas to avoid contamination. The cycle threshold (Ct) allowed interpolating the parasite equivalents of each sample from the standard curve.
Tissue Samples Preparation and Histological Analysis
Segments of heart and skeletal muscle were fixed in buffered 10% formaldehyde, dehydrated, and embedded in paraffin. Then, 5 mm thick sections were stained with hematoxylin and eosin (H&E). A single-blind evaluation of the specimens was performed by light microscopy, and the parasite load was expressed as the presence or absence of amastigote nests. The degree of myocardial and skeletal muscle inflammation was scored as previously reported (Tarleton et al., 1994; Solana et al., 2012).
Statistical Analysis
For in vivo studies, data distribution and homoscedasticity were evaluated. If the distribution was normal and variance was uniform, analysis of variance (ANOVA) and correction for multiple comparisons with Bonferroni test, when necessary, were performed. Data with distributions other than normal were analyzed by non-parametric Kruskal-Wallis test and compared in pairs. Survival analysis was performed using the Kaplan-Meier test.
In all cases, p-values <0.05 were considered statistically significant. Statistical analyses for in vivo tests were performed with InfoStat/P 2014 program, while analysis of the in vitro data and graphics were prepared with GraphPad Prism 5.03 (GraphPad Software, San Diego, California, USA). Values in tables and graphs are expressed in mean values with standard deviation unless otherwise indicated.
Results
In Vitro Anti-T. cruzi Activity of MLT Alone and Combined With BZ
The in vitro anti-T. cruzi activity and cytotoxicity of MLT, BZ, and NFX alone are summarized in Table 3. The MLT trypanolytic effect was significantly less potent than BZ or NFX, obtaining an LC50 value near the high micromolar range. However, MLT exhibited an in vitro amastigote inhibitory activity similar to BZ and NFX.
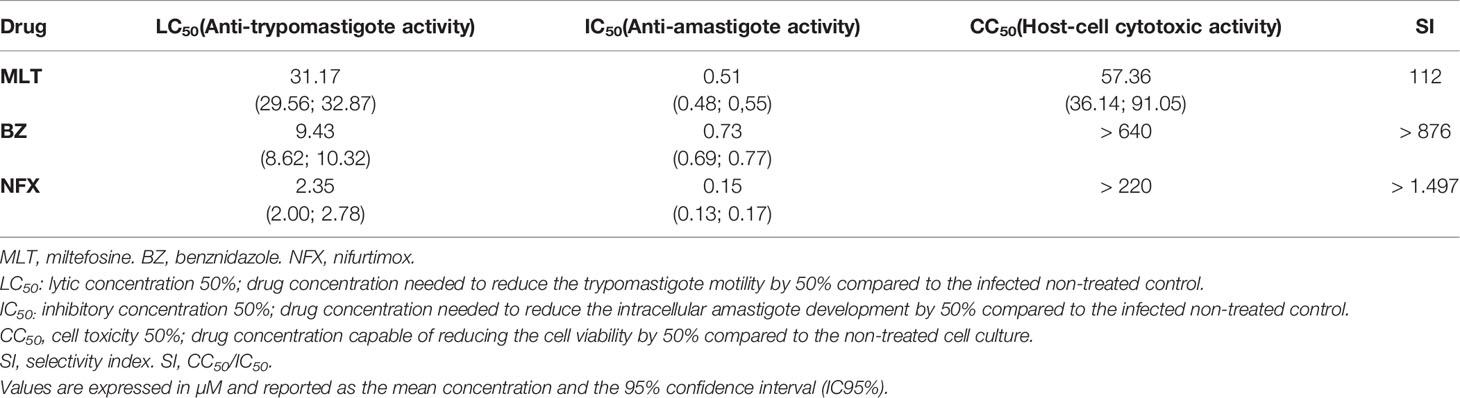
Table 3 In vitro activity of miltefosine, benznidazole, and nifurtimox on trypomastigote and intracellular amastigote stages of Trypanosoma cruzi (VD strain) and their cytotoxic effects on Vero cells.
The in vitro activity of MLT and BZ combination on trypomastigotes and amastigotes stages of T. cruzi is summarized in Table 4, and isobolograms are shown in Figure 1. The MLT and BZ association led to a significant decrease in trypomastigotes viability, assessed as a drop in the LC50 value. Moreover, the ΣFICI suggested that this combination would have a synergic effect on trypomastigotes lysis in culture. Similarly, the in vitro MLT and BZ effect on the amastigote stage reduced the IC50 value compared to the IC50 of each drug separately. Thus, the ΣFICI suggested an additive interaction.

Table 4 In vitro fractional inhibitory concentration index from miltefosine and benznidazole on trypomastigotes and amastigotes stages of Trypanosoma cruzi (VD strain).
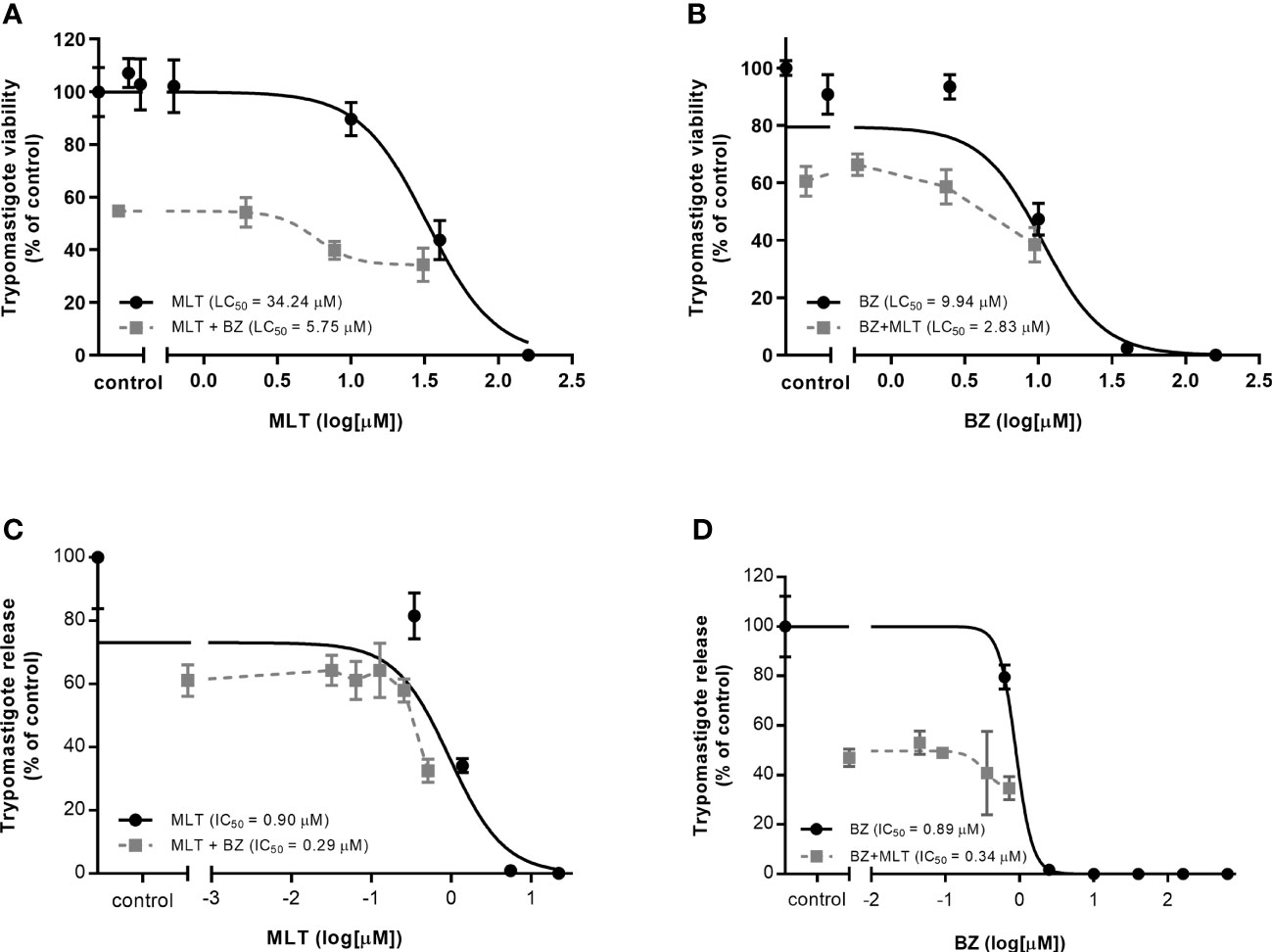
Figure 1 In vitro activity of miltefosine (MLT) and benznidazole (BZ) combination on (A-B) trypomastigotes and intracellular amastigote development (C-D) of Trypanosoma cruzi (VD strain). (A) LC50 value from BZ combined with variable concentrations of MLT.(B) LC50 value from MLT combined with variable concentrations of BZ. (C) IC50 value from BZ combined with variable concentrations of MLT. (D) IC50 value from MLT combined with variable concentrations of BZ. Values are expressed in µM and reported as the mean concentration and the 95% confidence interval (IC95%).
In Vivo Evaluation of MLT as Monotherapy
Clinical Response
Mice treated with MLT exhibited slight signs of discomfort during oral administration, denoted by retching, anxious appearance, and repeated grooming of lips and cheeks immediately after treatment. These signs lasted 15-20 minutes and then the animals returned to their usual behavior. These changes were not observed in the non-treated infected group administered the same volume of vehicle (i.e., distilled water or CMC 0.25%), nor in the mice infected and treated with BZ or NFX.
Throughout MLT treatment, the mice experienced a decrease in body weight, although these differences were not statistically significant compared with the other experimental groups (Supplementary Material, Figure S1.A). In the groups treated with MLT at 50 or 75 mg/kg/day, the bodyweight remained between the initial and an average increase of 20%, while the mice treated with 100 mg/kg/day of MLT showed a marked weight decrease from 11 dpi, but they recovered at 21 dpi and increased by an average of 9.4% compared to the initial weight by the end of the treatment (28 dpi).
Body temperatures remained close to the lower limit of the normal range for the murine species, without significant differences between the treatment groups, possibly due to the wide dispersion of the obtained values (Supplementary Material, Figure S1.B).
However, the NT group showed a significant decrease in body temperature as the acute course of the infection progressed, which was accompanied by a reduction in body weight, lethargic behavior, and ruffled coat.
Treatment Response During the Acute Phase
MLT treatment led to suppressive effects on parasitemia and associated parameters in a dose-dependent manner (Figure 2 and Table 1). The patent parasitemia period (PPP) was statistically different between the treatment groups (p <0.0001). MLT administered at 100 mg/kg/day significantly reduced the PPP compared to MLT at 25 mg/kg/day. In contrast, groups treated with MLT or BZ at 100 mg/kg/day did not lead to significant differences in PPP during the acute phase of infection.
Moreover, treatment with MLT in doses ≥50 mg/kg/day reduced maximum parasitemia reached (MPR) without significant differences compared to the reduction achieved with BZ or NFX. Animals treated with 100 mg/kg/day of BZ, NFX, or MLT had undetectable blood trypomastigotes at the end of the treatment (Figure 2).
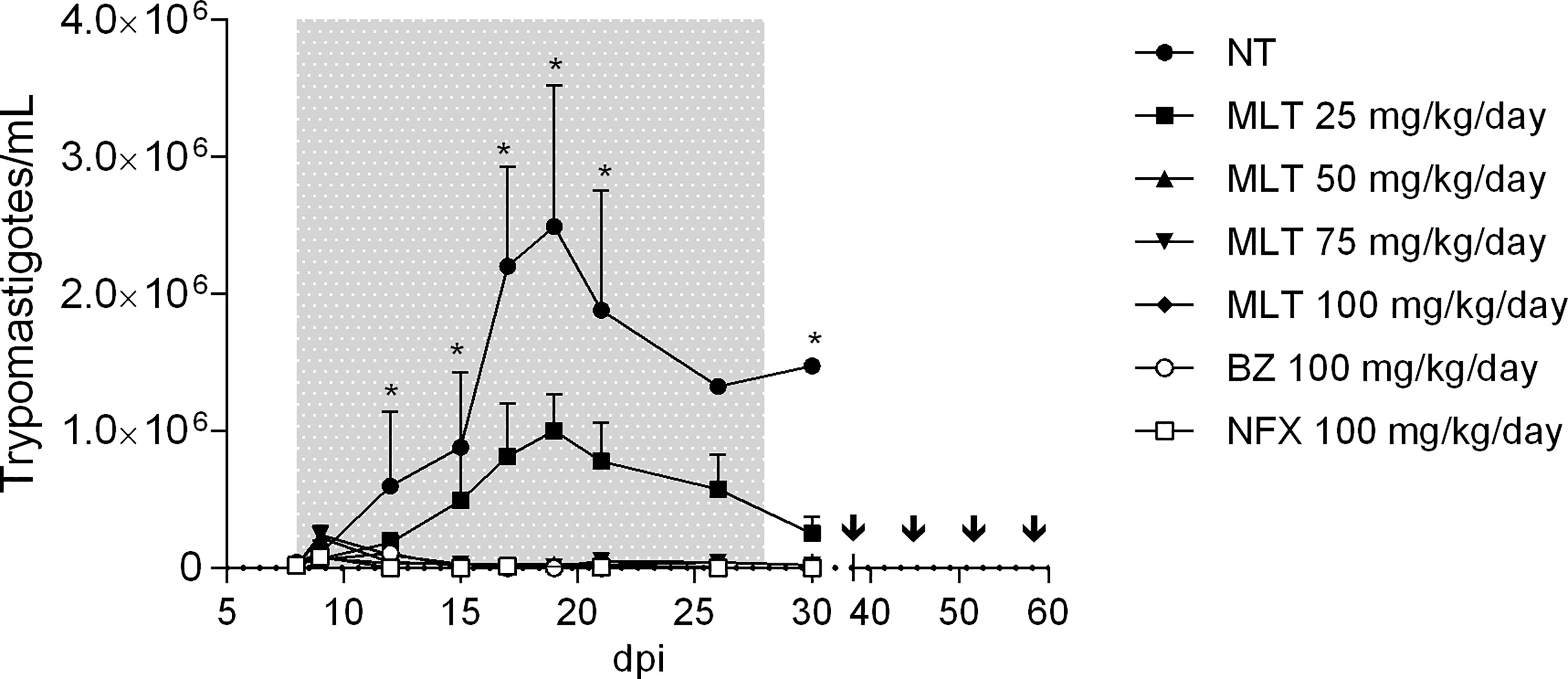
Figure 2 Effect of miltefosine, benznidazole, or nifurtimox on parasitemia course in BALB/cJ mice infected with Trypanosoma cruzi (VD strain). Values are expressed as mean trypomastigotes/mL (± SD) in peripheral blood from experimental groups according to the days after infection (dpi). Mice were inoculated with 500 trypomastigotes of the VD strain of Trypanosoma cruzi, and treatment started at parasitemia onset (8th dpi). During treatment and up to 10 days post-treatment, parasitemia was evaluated by fresh blood examination (FBE) to determine parasitemia rebound. Animals with negative parasitemia were submitted to immunosuppression consisting of four doses of cyclophosphamide (CYP; 200 mg/kg; ip route), separated by one week. Parasitemia was evaluated during the CYP cycle and up to 7 days after the last dose. The asterisks indicate significant differences compared to NT and MLT 25 mg/kg/day groups (Kruskal-Wallis, p < 0.05). Grey shading indicates the treatment period. Arrows indicate CYP administration cycle. NT, infected non-treated. MLT, miltefosine. BZ, benznidazole. NFX, nifurtimox.
Administration of MLT at doses ≥50 mg/kg/day prevented mortality in 100% of the mice. Groups treated with MLT at any dose, BZ or NFX, were statistically more likely to survive than NT controls (p<0.0001 for log-rank Mantel-Cox test).
Assessment of Parasitological Cure
At the end of the treatment, at least one mouse from groups receiving MLT at 25, 50, and 75 mg/kg/day exhibited patent parasitemia (1/4, 4/5, and 4/5, respectively). Conversely, all mice treated with 100 mg/kg/day of MLT, BZ, or NFX had negative parasitemia by optical microscopy. Through the following 10 days, parasitemia remained negative in all animals, regardless of the treatment previously administered.
The CYP immunosuppression cycle was initiated 10 days after treatment completion. The parasitemia rebound occurred throughout the follow-up period in all animals from MLT treated groups, usually between the second and fourth CYP dose.
Animals from BZ and NFX groups displayed parasitemia rebound in 44% (4/9) and 60% (6/10) of cases, respectively. qPCR on blood samples yielded the same positivity rate as microscopy.
Histopathology
Inflammation scoring in skeletal muscle samples was significantly different between treatment groups (p= 0.0025) (Supplementary Material, Figure S2.A). Inflammation in skeletal muscle was significantly lower in animals treated with MLT at 50 mg/kg/day than the NT group, similarly to animals treated with BZ or NFX. The other MLT doses studied had a wide range of variability in inflammation scores. Regarding the inflammation degree in the myocardium, there were no significant differences among treatment groups (Supplementary Material, Figure S2.B). The proportion of animals with amastigote nests was significantly higher in the NT group than in other treatment groups, in skeletal and myocardial muscle (Chi-square, p=0.0003 and 0.0044, respectively). No significant differences were detected between the groups receiving MLT, BZ, or NFX, both in skeletal muscle and myocardium (Figure 3). Microphotographs of representative skeletal and cardiac muscle samples from different treatment groups can be observed as Supplementary Material (Figures S3, S4, respectively).
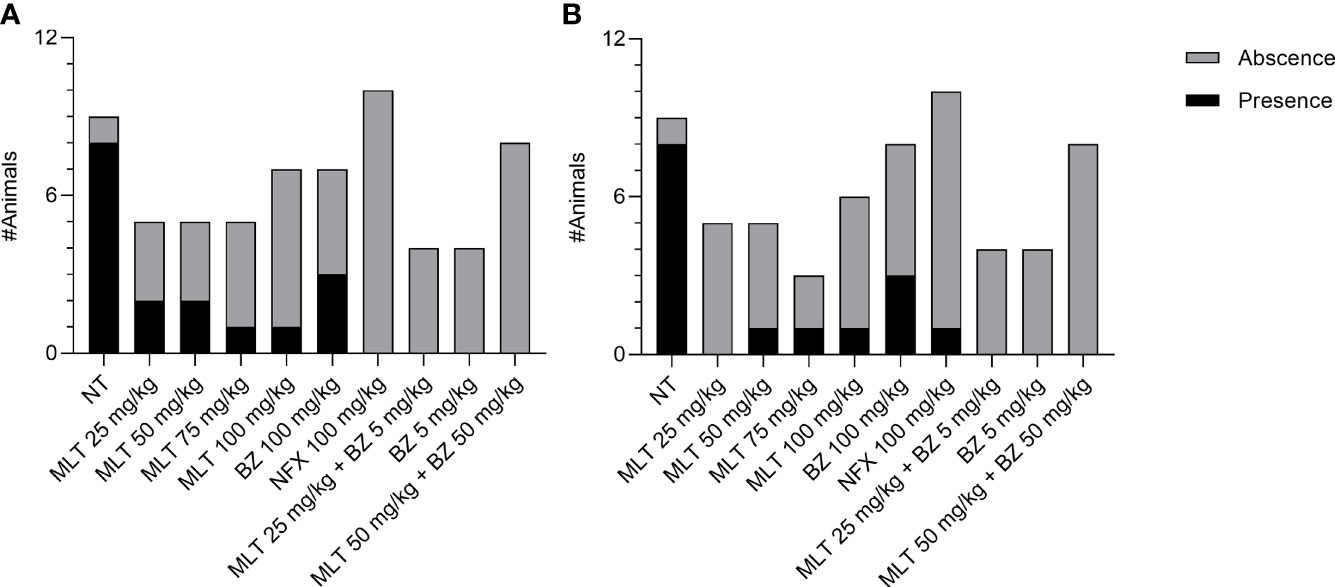
Figure 3 Effect of miltefosine (alone or in combined regimes), benznidazole, or nifurtimox on amastigote nest burden in BALB/cJ mice infected Trypanosoma cruzi, (VD strain). Mice with the presence or absence of amastigote nests in skeletal muscle (A) and heart samples (B). NT, infected non-treated. MLT, miltefosine. BZ, benznidazole. NFX, nifurtimox.
In Vivo Evaluation of MLT Combined With BZ
Clinical Response
Similar to the monotherapy trials, MLT administration in combined regimes produced a significant variation of the average weight gain from day 13 post-infection (6 days of treatment), with a decrease relative to the initial weight of between 10 and 15% in the animals treated with the MLT and BZ combination, which was then partially reversed toward the end of treatment. However, the initial weight was not recovered. Body temperature values were close to the lower limit of the normal range for the species recorded, without significant differences between the treatment groups (Supplementary Material, Figures S5, S6).
Treatment Response
During the acute course of the infection, treatments lead to a significant decrease in parasitemia. MLT and BZ administration in sub-therapeutic doses given separately or in combination diminished the MPR between 76.23 to 79.51%, which was significantly higher in the NT infected group compared to the treatment groups (p < 0.01) (Table 2). Likewise, administration of sub-therapeutic doses of MLT and BZ alone or in combination prevented mortality (Supplementary Material, Figures S7).
For the additive treatment regime, the combination of BZ+MLT at half the effective doses (i.e., 50 mg/kg/day each) significantly reduced MPR compared to NT animals, but there were no significant differences when compared with the group receiving BZ 100 mg/kg/day. Finally, 100% of the mice treated with MLT either alone or combined with BZ survived (Supplementary Material, Figure S8).
Assessment of Parasitological Cure
The treatment with sub-therapeutic doses of MLT or BZ, alone or in combination, was not able to eliminate circulating parasites and therefore the estimated parasitic load by qPCR was not significantly different (Table 5).
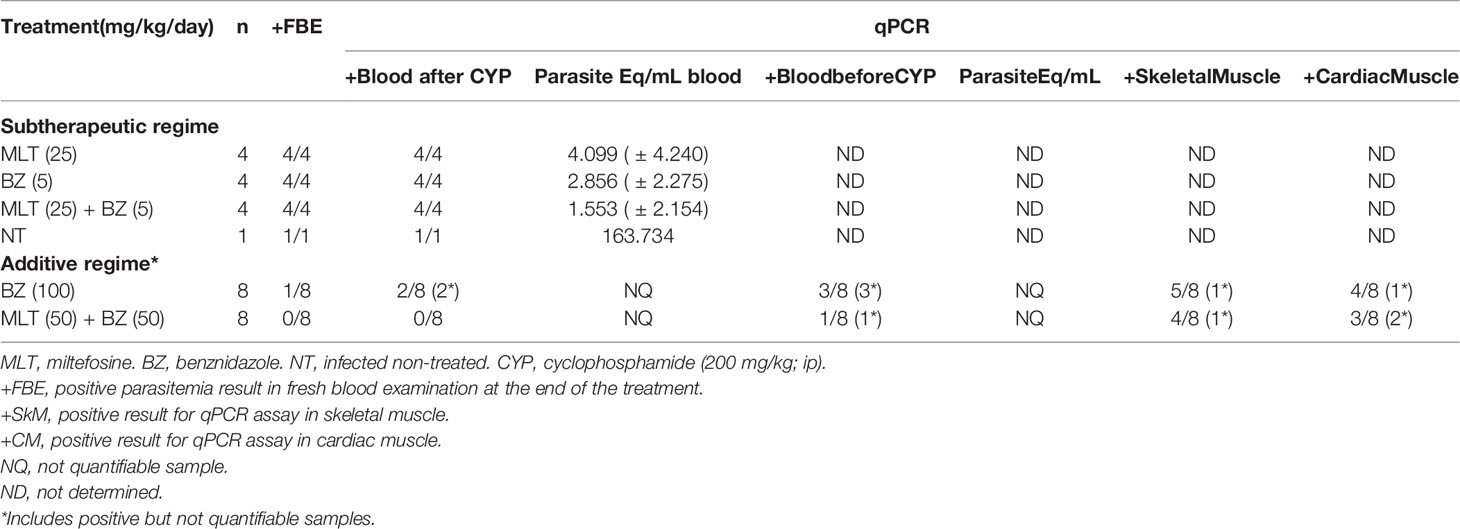
Table 5 Effect of miltefosine alone or combined with benznidazole at different sub-therapeutic or additive regimes on parasite burden at the end of the treatment in BALB/cJ mice infected with Trypanosoma cruzi (VD strain).
Regarding the additive treatment regime, mice receiving BZ at 100 mg/kg/day or MLT+BZ at 50 mg/kg/day showed negative parasitemia by fresh blood examination after 20 consecutive days of treatment. The experimental groups were kept under observation from that moment and the following 10 days, and parasitemia remained negative in all subjects, regardless of the administered treatment.
After that period, the immunosuppression cycle started in all mice (CYP, 200 mg/kg/week, ip route). Mice initially treated with MLT+BZ exhibited negative parasitemia at the end of the immunosuppression cycle, while only one animal from group BZ (100 mg/kg/day) showed parasitemia rebound.
Later, blood qPCR showed that 3/8 animals treated with BZ 100 mg/kg/day presented detectable non-quantifiable DNA. Whereas only one mouse treated with MLT+BZ combination exhibited detectable but non-quantifiable parasitic DNA in blood after immunosuppression (Table 5). Parasitic DNA was detected in low quantity in skeletal and cardiac muscle samples, and it could not be quantified with the previously established curve. The proportion of positive samples in skeletal and cardiac muscle was not statistically significant between treatment groups (Table 5).
Histopathology
Regarding the combinatory therapy, inflammation scores in skeletal muscle and cardiac muscle were not significantly different among treatment groups. A scarce inflammatory infiltrate was observed, assigning scores ranging from 0 to 3 for skeletal muscle and between 0 and 2 for cardiac muscle, with no significant differences (Supplementary Material, Figure S2.A, S2.B). Moreover, amastigotes nests could not be recorded in any sample from treatment groups (Figure 3). Microphotographs of representative skeletal and cardiac muscle samples from different treatment groups can be observed as Supplementary Material (Figure S3, S4, respectively).
Discussion
MLT is a lipophospholipid analog developed originally as an antineoplastic agent (Dorlo et al., 2012). Based on previous in vitro and in vivo studies and results from oncology clinical trials, MLT was repositioned for visceral and cutaneous leishmaniasis treatment. Since its registration in 2002, MLT remains the only oral drug available for leishmaniasis (Reimão et al., 2020).
MLT and other alkyl-lipophospholipids have proven activity on T. cruzi (Croft et al., 1996; Luna et al., 2009). Our results support the MLT effect on inhibiting amastigote development in vitro and the low efficacy on trypomastigote stage (Croft et al., 1996; Santa-Rita et al., 2000). Moreover, MLT high selectivity index suggests a specific antiparasitic mechanism of action without affecting the host cell.
To date, only three studies have assessed the in vivo efficacy of MLT (Croft et al., 1996; Saraiva et al., 2002; Martinez-Peinado et al., 2021) in mice models of acute infection. While Croft et al. observed transient parasitemia suppression without a curative effect or reduction of mortality in mice treated for 5 days at up to 30 mg/kg/day by oral route, Saraiva et al. reported parasitic sterilization in all mice treated with 25 mg/kg/day, determined by direct blood observation at 120 dpi and spleen culture for 15 days. Recently, Martinez-Peinado et al. studied MLT efficacy by giving 30 mg/kg/day for 10 days, using the Brazil strain expressing firefly luciferase, which yielded statistical differences in luciferase activity compared to infected non-treated mice.
It is well known that several experimental variables affect the infection course and the main outcomes in animal models of T. cruzi infection (Chatelain and Konar, 2015), and their heterogeneity make difficult the direct comparison between results.
In this work, we evaluated the in vivo efficacy of MLT using state-of-the-art methodology based on previous guidelines for drug screening (Romanha et al., 2010) by using qPCR and immunosuppression cycle to elucidate the possible reemergence of parasites from tissues.
The murine model of acute infection with T. cruzi was previously standardized (Gulin et al., 2018), using the VD strain (TcVI), originally isolated from a patient infected by a congenital route. This model becomes relevant since mother-to-child infection is the main transmission mode in vector-free areas within and outside Latin America (Carlier et al., 2019). Furthermore, the VD strain displays high parasitemia levels and mortality rates in infected non-treated mice and a moderate BZ and NFX effect to eradicate the infection.
Despite a temporary animal discomfort observed in MLT oral administration, the treatment was well tolerated by the animals. Also, the weight loss and ruffled fur agree with previously reported effects (Saraiva et al., 2002). The body weight loss could be explained by the gastrointestinal discomfort caused by MLT at all assayed doses, an adverse effect widely reported in clinical trials (Jha et al., 1999; Sundar et al., 2002).
As described by Saraiva et al., the parasitemia course throughout the acute phase of infection was not suppressed with 25 mg/kg/day of MLT. However, in our infection model, the days of patent parasitemia and the MPR were lowered in a dose-dependent manner by MLT, and the 100 mg/kg/day dose was able to reduce blood trypomastigotes circulation to undetectable levels by optical microscopy.
When administered alone, MLT at lower doses leads to a parasitemia reduction, and this could be explained in part due to the widespread drug distribution and the slow clearance rate. In mice, oral MLT is absorbed slowly but extensively and accumulates mainly in the kidneys, liver, and lungs (Breiser et al., 1987). Clinical pharmacokinetic studies indicate that, due to its prolonged half-life, MLT accumulates during treatment reaching a steady-state concentration in the last week of a 28-day treatment schedule (Kip et al., 2018).
Although the drug failed to produce parasitic sterilization, it was able to maintain the burden of amastigotes at low levels, which was reactivated by CYP-induced immunosuppression. Moreover, animals treated with MLT showed lower rates of amastigote nests in the myocardium and in skeletal muscle compared to NT, which could be explained by the MLT extensive distribution in these tissues, as previously documented in mice and rats (Breiser et al., 1987; Marschner et al., 1992).
Failure to reach parasitological sterilization was also detected in a percentage of the animals treated with BZ or NFX. These results coincide with the previously published characterization of the VD strain (Gulin et al., 2018), and with other T. cruzi strains from the same DTU, usually classified as BZ-sensitive (Fernández et al., 2010; Keenan et al., 2013b).
MLT effect on Leishmania spp. was also studied in combination with reference drugs or with repositioned compounds. In vitro additive synergism with sodium stibogluconate and in vivo potentiate synergism potentiation combined with paromomycin or amphotericin B were reported (Seifert and Croft, 2006). Costa et al. also reported the parasiticidal effect of efavirenz on L. infantum and a lower IC50 adding MLT (Costa et al., 2016).
The combined effect of MLT with other compounds on T. cruzi viability was previously described by in vitro combination with ketoconazole, both on trypomastigotes and intracellular amastigotes (Santa-Rita et al., 2005). However, this is the first study of the anti-T cruzi effect of the MLT and BZ combination.
The analysis of the MLT+BZ in vitro combination suggests a synergistic effect on trypomastigotes viability and an additive interaction on intracellular amastigote development. This association was not evaluated previously, although similar in vitro interactions were reported when combining MLT with paromomycin (de Morais-Texeira et al., 2014) or nitazoxanide (Mesquita et al., 2014) on Leishmania spp.
We furthered the evaluation of the MLT and BZ association on a murine model of acute infection with T. cruzi. MLT+BZ combination at sub-therapeutic doses could not achieve an effect different from that obtained with the drugs separately. However, it decreased parasite loads in blood and prevented mortality in treated mice. Nevertheless, MLT+BZ at half the total dose (i.e., 50mg/kg) produced negative parasitemia and prevented the reactivation after the immunosuppression protocol.
The qPCR yielded disparate results in target organs such as skeletal muscle and myocardium in both experimental groups, with detection of parasitic DNA in low-loading or even non-quantifiable cases. These results could then question qPCR as a method to establish parasitological cure in cases where T. cruzi DNA is not detected in the blood.
Residual DNA in tissues and phagocytic cells has been reported in experimentally infected mice treated with BZ and considered cured by different methods (Guarner et al., 2001; Martins et al., 2008), which would indicate the presence of non-viable parasites or even cross-contamination with parasitic DNA.
However, recently described spontaneous dormancy in T. cruzi (Sánchez-Valdéz et al., 2018) could also explain the presence of parasitic DNA in tissues due to the non-replicative amastigote stage in the absence of bloodstream trypomastigotes, which would deserve further investigation to fully understand therapeutic failure both in preclinical studies and clinical trials.
Although our results demonstrate the inability of MLT to achieve a complete parasitological cure in an acute murine model of T. cruzi infection, the positive interaction with BZ both in vitro and in vivo opens a promising alternative in drug repurposing and combinatory therapy approach for Chagas disease treatment. MLT and other synthetic alkylphospholipids arise as drug candidates for combined treated schemes and serve as chemical scaffolds for new compound entities.
Data Availability Statement
The raw data supporting the conclusions of this article will be made available by the authors, without undue reservation.
Ethics Statement
The animal study was reviewed and approved by Institutional Animal Care and Use Committee from the Faculty of Veterinary Sciences (University of Buenos Aires) (Protocol #2014/04).
Author Contributions
JENG: Writing - original draft, Writing -review & editing, Investigation, Validation, Formal analysis. MMCB: Writing -review & editing, Investigation, Validation, Formal analysis. DR and MES: Investigation, Validation, Formal analysis. JA: Conceptualization, Methodology, Funding acquisition, Resources, Supervision. FG-B: Conceptualization, Methodology, Writing - review & editing, Funding acquisition, Resources, Supervision. All authors contributed to the article and approved the submitted version.
Funding
This work was supported by Agencia Nacional de Promoción de la Investigación, el Desarrollo Tecnológico y la Innovación, Ministerio de Ciencia y Técnica, Argentina (Grant: Proyectos de Investigación Cientı́fica y Tecnológica PICTO-GLAXO 2012-035).
Conflict of Interest
The authors declare that the research was conducted in the absence of any commercial or financial relationships that could be construed as a potential conflict of interest.
Publisher’s Note
All claims expressed in this article are solely those of the authors and do not necessarily represent those of their affiliated organizations, or those of the publisher, the editors and the reviewers. Any product that may be evaluated in this article, or claim that may be made by its manufacturer, is not guaranteed or endorsed by the publisher.
Acknowledgments
We would like to thank Dr. Daniel Lombardo (Universidad de Buenos Aires, Facultad de Ciencias Veterinarias) for providing support in histology samples preparation and images acquisition.
Supplementary Material
The Supplementary Material for this article can be found online at: https://www.frontiersin.org/articles/10.3389/fcimb.2022.855119/full#supplementary-material
Footnotes
- ^ https://www.who.int/chagas/en/. Last access: Dec. 12, 2021.
References
Andrews, K., Fisher, G., Skinner-Adams, T. (2014). Drug Repurposing and Human Parasitic Protozoan Diseases. Int. J. Parasitol. Drugs Drug Resist. 4, 95–111. doi: 10.1016/j.ijpddr.2014.02.002
Apt, W. (2010). Current and Developing Therapeutic Agents in the Treatment of Chagas Disease. Drug Des. Devel. Ther. 4, 243–253. doi: 10.2147/dddt.s8338
Benaim, G., Paniz-Mondolfi, A., Sordillo, E., Martinez-Sotillo, N. (2020). Disruption of Intracellular Calcium Homeostasis as a Therapeutic Target Against Trypanosoma Cruzi. Front. Cell Infect. Microbiol. 10. doi: 10.3389/fcimb.2020.00046
Breiser, A., Kim, D., Fleer, E., Damenz, W., Drube, A., Berger, M., et al. (1987). Distribution and Metabolism of Hexadecylphosphocholine in Mice. Lipids 22, 925–926. doi: 10.1007/BF02535556
Brossas, J., Gulin, J., Bisio, M., Chapelle, M., Marinach-Patrice, C., Bordessoules, M., et al. (2017). Secretome Analysis of Trypanosoma Cruzi by Proteomics Studies. PloS One 12, e0185504. doi: 10.1371/journal.pone.0185504
Cantin, L., Chamberland, S. (1993). In Vitro Evaluation of the Activities of Azithromycin Alone and Combined With Pyrimethamine Against Toxoplasma Gondii. Antimicrob. Agents Chemother. 37, 1993–1996. doi: 10.1128/AAC.37.9.1993
Carlier, Y., Altcheh, J., Angheben, A., Freilij, H., Luquetti, A. O., Schijman, A. G., et al. (2019). Congenital Chagas Disease: Updated Recommendations for Prevention, Diagnosis, Treatment, and Follow-Up of Newborns and Siblings, Girls, Women of Childbearing Age, and Pregnant Women. PloS Negl. Trop. Dis. 13, 1–9. doi: 10.1371/journal.pntd.0007694
Chatelain, E., Ioset, J. (2011). Drug Discovery and Development for Neglected Diseases: The DNDi Model. Drug Des. Devel. Ther. 5, 175–181. doi: 10.2147/DDDT.S16381
Chatelain, E., Konar, N. (2015). Translational Challenges of Animal Models in Chagas Disease Drug Development: A Review. Drug Des. Devel. Ther. 19, 4807–4823. doi: 10.2147/DDDT.S90208
Costa, S., Machado, M., Cavadas, C., do Céu Sousa, M. (2016). Antileishmanial Activity of Antiretroviral Drugs Combined With Miltefosine. Parasitol. Res. 115, 3881–3887. doi: 10.1007/s00436-016-5153-8
Croft, S., Snowdon, D., Yardley, V. (1996). The Activities of Four Anticancer Alkyllysophospholipids Against Leishmania Donovani, Trypanosoma Cruzi and Trypanosoma Brucei. J. Antimicrob. Chemother. 38, 1041–1047. doi: 10.1093/jac/38.6.1041
da Silva, C., da Silva, P., Batista, M., Daliry, A., Tidwell, R., Soeiro, M. N. (2010). The Biological In Vitro Effect and Selectivity of Aromatic Dicationic Compounds on Trypanosoma Cruzi. Mem. Inst. Oswaldo. Cruz. 105, 239–245. doi: 10.1590/S0074-02762010000300001
de Morais-Texeira, E., Gallupo, M., Fonseca Rodrigues, L., Romanha, A., Rabello, A. (2014). In Vitro Interaction Between Paromomycin Sulphate and Four Drugs With Leishmanicidal Activity Against Three New World Leishmania Species. J. Antimicrob. Chemother. 69, 150–154. doi: 10.1093/jac/dkt318
Dorlo, T., Balasegaram, M., Beijnen, J., de Vries, P. (2012). Miltefosine: A Review of its Pharmacology and Therapeutic Efficacy in the Treatment of Leishmaniasis. J. Antimicrob. Chemother. 67, 2576–2597. doi: 10.1093/jac/dks275
Duffy, T., Cura, C., Ramirez, J., Abate, T., Cayo, N., Parrado, R., et al. (2013). Analytical Performance of a Multiplex Real-Time PCR Assay Using TaqMan Probes for Quantification of Trypanosoma Cruzi Satellite DNA in Blood Samples. PloS Negl. Trop. Dis. 7, e2000. doi: 10.1371/journal.pntd.0002000
Fernández, M., González-Cappa, S., Solana, M. (2010). Trypanosoma Cruzi : Immunological Predictors of Benznidazole Efficacy During Experimental Infection. Exp. Parasitol. 124, 172–180. doi: 10.1016/j.exppara.2009.09.006
Field, M., Horn, D., Fairlamb, A., Ferguson, M., Gray, D., Read, K., et al. (2017). Anti-Trypanosomatid Drug Discovery: An Ongoing Challenge and a Continuing Need. Nat. Rev. Microbiol. 15, 217–231. doi: 10.1038/nrmicro.2016.193
Fivelman, Q., Adagu, I., Warhurst, D. (2004). Modified Fixed-Ratio Isobologram Method for Studying In Vitro Interactions Between Atovaquone and Proguanil or Dihydroartemisinin Against Drug-Resistant Strains of Plasmodium Falciparum. Antimicrob. Agents Chemother. 48, 4097–4102. doi: 10.1128/AAC.48.11.4097-4102.2004
Ghosh, M., Roy, K., Roy, S. (2013). Immunomodulatory Effects of Antileishmanial Drugs. J. Antimicrob. Chemother. 68, 2834–2838. doi: 10.1093/jac/dkt262
Golde, W., Gollobin, P., Rodriguez, L. (2005). A Rapid, Simple, and Humane Method for Submandibular Bleeding of Mice Using a Lancet. Lab. Anim. 34, 39–43. doi: 10.1038/laban1005-39
Guarner, J., Bartlett, J., Zaki, S., Colley, D., Grijalva, M., Powell, M. (2001). Mouse Model for Chagas Disease: Immunohistochemical Distribution of Different Stages of Trypanosoma Cruzi in Tissues Throughout Infection. Am. J. Trop. Med. Hyg. 65, 152–158. doi: 10.4269/ajtmh.2001.65.152
Gulin, J., Bisio, M., Rocco, D., Altcheh, J., Solana, M., García-Bournissen, F. (2018). Molecular and Biological Characterization of a Highly Pathogenic Trypanosoma Cruzi Strain Isolated From a Patient With Congenital Infection. Exp. Parasitol. 186, 50–58. doi: 10.1016/j.exppara.2018.02.002
Gulin, J., Eagleson, M., López-Muñoz, R., Solana, M., Altcheh, J., García-Bournissen, F. (2020). In Vitro and In Vivo Activity of Voriconazole and Benznidazole Combination on Trypanosoma Cruzi Infection Models. Acta Trop. 211. doi: 10.1016/j.actatropica.2020.105606
Jha, T., Sundar, S., Thakur, C., Bachmann, P., Karbwang, J., Fischer, C., et al. (1999). Miltefosine, an Oral Agent, for the Treatment of Indian Visceral Leishmaniasis. N. Engl. J. Med. 341, 1795–1800. doi: 10.1056/NEJM199912093412403
Keenan, M., Alexander, P., Chaplin, J., Abbott, M., Diao, H., Wang, Z., et al. (2013a). Selection and Optimization of Hits From a High-Throughput Phenotypic Screen Against Trypanosoma Cruzi. Futur. Med. Chem. 5, 1733–1752. doi: 10.4155/fmc.13.139
Keenan, M., Chaplin, J., Alexander, P., Abbott, M., Best, W., Khong, A., et al. (2013b). Two Analogues of Fenarimol Show Curative Activity in an Experimental Model of Chagas Disease. J. Med. Chem. 56, 10158–10170. doi: 10.1021/jm401610c
Kip, A., Schellens, J., Beijnen, J., Dorlo, T. (2018). Clinical Pharmacokinetics of Systemically Administered Antileishmanial Drugs. Clin. Pharmacokinet. 57, 151–176. doi: 10.1007/s40262-017-0570-0
Lira, R., Contreras, L., Rita, R., Urbina, J. (2001). Mechanism of Action of Anti-Proliferative Lysophospholipid Analogues Against the Protozoan Parasite Trypanosoma Cruzi: Potentiation of In Vitro Activity by the Sterol Biosynthesis Inhibitor Ketoconazole. J. Antimicrob. Chemother. 47, 537–546. doi: 10.1093/jac/47.5.537
Luna, K., Hernández, I., Rueda, C., Zorro, M., Croft, S., Escobar, P. (2009). In Vitro Susceptibility of Trypanosoma Cruzi Strains From Santander, Colombia, to Hexadecylphosphocholine (Miltefosine), Nifurtimox and Benznidazole. Biomédica 29, 448–455 http://www.scielo.org.co/pdf/bio/v29n3/v29n3a13.pdf
Marschner, N., Kötting, J., Eibl, H., Unger, C. (1992). Distribution of Hexadecylphosphocholine and Octadecyl-Methyl-Glycero-3-Phosphocholine in Rat Tissues During Steady-State Treatment. Cancer Chemother. Pharmacol. 31, 18–22. doi: 10.1007/BF00695989
Martinez-Peinado, N., Cortes-Serra, N., Sherman, J., Rodriguez, A., Bustamante, J., Gascon, J., et al. (2021). Identification of Trypanosoma Cruzi Growth Inhibitors With Activity In Vivo Within a Collection of Licensed Drugs. Microorganisms 9. doi: 10.3390/microorganisms9020406
Martins, H., Figueiredo, L., Valamiel-Silva, J., Carneiro, C., Machado-Coelho, G., Vitelli-Avelar, D., et al. (2008). Persistence of PCR-Positive Tissue in Benznidazole-Treated Mice With Negative Blood Parasitological and Serological Tests in Dual Infections With Trypanosoma Cruzi Stocks From Different Genotypes. J. Antimicrob. Chemother. 61, 1319–1327. doi: 10.1093/jac/dkn092
Maya, J., Cassels, B., Iturriaga-Vásquez, P., Ferreira, J., Faúndez, M., Galanti, N., et al. (2007). Mode of Action of Natural and Synthetic Drugs Against Trypanosoma Cruzi and Their Interaction With the Mammalian Host. Comp. Biochem. Physiol. A Mol. Integr. Physiol. 146, 601–620. doi: 10.1016/j.cbpa.2006.03.004
Mbui, J., Olobo, J., Omollo, R., Solomos, A., Kip, A., Kirigi, G., et al. (2019). Pharmacokinetics, Safety and Efficacy of an Allometric Miltefosine Regimen for the Treatment of Visceral Leishmaniasis in Eastern African Children: An Open-Label, Phase-II Clinical Trial. Clin. Infect. Dis. 68, 1530–1538. doi: 10.1093/cid/ciy747
Mesquita, J., Tempone, A., Reimão, J. (2014). Combination Therapy With Nitazoxanide and Amphotericin B, Glucantime®, Miltefosine and Sitamaquine Against Leishimania (Leishmania) Infantum Intracellular Amastigotes. Acta Trop. 130, 112–116. doi: 10.1016/j.actatropica.2013.11.003
Miranda, C., Solana, M., Curto, M., Lammel, E., Schijman, A., Alba Soto, C. (2015). A Flow Cytometer-Based Method to Simultaneously Assess Activity and Selectivity of Compounds Against the Intracellular Forms of Trypanosoma Cruzi. Acta Trop. 152, 8–16. doi: 10.1016/j.actatropica.2015.08.004
National Research Council (2011). Guide for the Care and Use of Laboratory Animals. 8th (The National Academies Press Washington, D.C: The National Academic Press). doi: 10.17226/12910
Odds, F. (2003). Synergy, Antagonism, and What the Chequerboard Puts Between Them. J. Antimicrob. Chemother. 52, 1. doi: 10.1093/jac/dkg301
Olfert, E., Godson, D. (2000). Humane Endpoints for Infectious Disease Animal Models. ILAR. J. 41, 99–104. doi: 10.1093/ilar.41.2.99
Paulino, M., Iribarne, F., Dubin, M., Aguilera-Morales, S., Tapia, O., Stoppani, A. (2005). The Chemotherapy of Chagas’ Disease: An Overview. Mini Rev. Med. Chem. 5, 499–519. doi: 10.2174/1389557053765565
Pérez-Molina, J., Molina, I. (2018). Chagas Disease. Lancet 391, 82–94. doi: 10.1016/S0140-6736(17)31612-4
Polanco-Hernández, G., Escalante-Erosa, F., García-Sosa, K., Acosta-Viana, K., Chan-Bacab, M., Sagua-Franco, H., et al. (2012). In Vitro and In Vivo Trypanocidal Activity of Native Plants From the Yucatan Peninsula. Parasitol. Res. 110, 31–35. doi: 10.1007/s00436-011-2447-8
Reimão, J., Pita Pedro, D., Coelho, A. (2020). The Preclinical Discovery and Development of Oral Miltefosine for the Treatment of Visceral Leishmaniasis: A Case History. Expert Opin. Drug Discovery 15, 647–658. doi: 10.1080/17460441.2020.1743674
Rodrigues, J., Ueda-Nakamura, T., Corrêa, A., Sangi, D., Nakamura, C. (2014). A Quinoxaline Derivative as a Potent Chemotherapeutic Agent, Alone or in Combination With Benznidazole, Against Trypanosoma Cruzi. PloS One 9, e85706. doi: 10.1371/journal.pone.0085706
Romanha, A., Castro, S., Soeiro, M. N., Lannes-Vieira, J., Ribeiro, I., Talvani, A., et al. (2010). In Vitro and In Vivo Experimental Models for Drug Screening and Development for Chagas Disease. Mem. Inst. Oswaldo. Cruz. 105, 233–238. doi: 10.1590/s0074-02762010000200022
Sánchez-Valdéz, F., Padilla, A., Wang, W., Orr, D., Tarleton, R. (2018). Spontaneous Dormancy Protects Trypanosoma Cruzi During Extended Drug Exposure. Elife. 7 Mar 26;7:e34039 doi: 10.7554/eLife.34039
Santa-Rita, R., Lira, R., Barbosa, H., Urbina, J., de Castro, S. (2005). Anti-Proliferative Synergy of Lysophospholipid Analogues and Ketoconazole Against Trypanosoma Cruzi (Kinetoplastida: Trypanosomatidae): Cellular and Ultrastructural Analysis. J. Antimicrob. Chemother. 55, 780–784. doi: 10.1093/jac/dki087
Santa-Rita, R., Santos Barbosa, H., Meirelles, M., de Castro, S. (2000). Effect of the Alkyl-Lysophospholipids on the Proliferation and Differentiation of Trypanosoma Cruzi. Acta Trop. 75, 219–228. doi: 10.1016/s0001-706x(00)00052-8
Saraiva, V., Gibaldi, D., Previato, J., Mendonça-Previato, L., Bozza, M., Freire-De-Lima, C., et al. (2002). Proinflammatory and Cytotoxic Effects of Hexadecylphosphocholine (Miltefosine) Against Drug-Resistant Strains of Trypanosoma Cruzi. Antimicrob. Agents Chemother. 46, 3472–3477. doi: 10.1128/AAC.46.11.3472-3477.2002
Seifert, K., Croft, S. (2006). In Vitro and In Vivo Interactions Between Miltefosine and Other Antileishmanial Drugs. Antimicrob. Agents Chemother. 50, 73–79. doi: 10.1128/AAC.50.1.73-79.2006
Singh, U., Prasad, R., Mishra, O., Jayswal, B. (2006). Miltefosine in Children With Visceral Leishmaniasis: A Prospective, Multicentric, Cross-Sectional Study. Indian J. Pediatr. 73, 1077–1080. doi: 10.1007/BF02763048
Solana, M., Ferrer, M., Novoa, M., Song, W., Gómez, R. (2012). Decay-Accelerating Factor 1 Deficiency Exacerbates Trypanosoma Cruzi-Induced Murine Chronic Myositis. Muscle Nerve 46, 582–587. doi: 10.1002/mus.23347
Sundar, S., Jha, T., Thakur, C., Engel, J., Sindermann, H., Fischer, C., et al. (2002). Oral Miltefosine for Indian Visceral Leishmaniasis. N. Engl. J. Med. 347, 1739–1746. doi: 10.1056/NEJMoa021556
Keywords: Chagas disease, Trypanosoma cruzi, miltefosine, benznidazole, drug combination chemotherapy, drug repositioning
Citation: Gulin JEN, Bisio MMC, Rocco D, Altcheh J, Solana ME and García-Bournissen F (2022) Miltefosine and Benznidazole Combination Improve Anti-Trypanosoma cruzi In Vitro and In Vivo Efficacy. Front. Cell. Infect. Microbiol. 12:855119. doi: 10.3389/fcimb.2022.855119
Received: 14 January 2022; Accepted: 16 May 2022;
Published: 05 July 2022.
Edited by:
Vilma G. Duschak, Consejo Nacional de Investigaciones Científicas y Técnicas (CONICET), ArgentinaReviewed by:
Frederick S. Buckner, University of Washington, United StatesFabiane Matos Dos Santos, Federal University of Espírito Santo, Brazil
Higo Fernando Santos Souza, University of São Paulo, Brazil
Victor Monteon, Autonomous University of Campeche, Mexico
Copyright © 2022 Gulin, Bisio, Rocco, Altcheh, Solana and García-Bournissen. This is an open-access article distributed under the terms of the Creative Commons Attribution License (CC BY). The use, distribution or reproduction in other forums is permitted, provided the original author(s) and the copyright owner(s) are credited and that the original publication in this journal is cited, in accordance with accepted academic practice. No use, distribution or reproduction is permitted which does not comply with these terms.
*Correspondence: Facundo García-Bournissen, ZmFjdWdiMUBnbWFpbC5jb20=