- 1Department of Pharmacy, The Second Xiangya Hospital, Central South University, Changsha, China
- 2Institute of Clinical Pharmacy, Central South University, Changsha, China
- 3Institute of Clinical Medicine, The First Affiliated Hospital, University of South China, Hengyang, China
Alterations in the composition and function of the gut microbiota have been reported in patients with type 2 diabetes mellitus (T2DM). Emerging studies show that prescribed antidiabetic drugs distort the gut microbiota signature associated with T2DM. Even more importantly, accumulated evidence provides support for the notion that gut microbiota, in turn, mediates the efficacy and safety of antidiabetic drugs. In this review, we highlight the current state-of-the-art knowledge on the crosstalk and interactions between gut microbiota and antidiabetic drugs, including metformin, α-glucosidase inhibitors, glucagon-like peptide-1 receptor agonists, dipeptidyl peptidase-4 inhibitors, sodium-glucose cotransporter 2 inhibitors, traditional Chinese medicines and other antidiabetic drugs, as well as address corresponding microbial-based therapeutics, aiming to provide novel preventative strategies and personalized therapeutic targets in T2DM.
Introduction
Type 2 diabetes mellitus (T2DM) is a highly prevalent metabolic disorder characterized by elevated blood glucose levels, primarily caused by insulin secretion disturbance, insulin resistance, or both (Cersosimo et al., 2000). In recent years, gut microbiota, which refers to a complicated assembly of trillions of microbes, is reported to be involved in the pathogenesis and treatment responses of T2DM (Bouter et al., 2017; Koropatkin and Martens, 2017; Vázquez-Baeza et al., 2018). Additionally, emerging evidence has indicated that the gut microbiota affects the pharmacology of antidiabetic drugs, and drug-induced metabolites transform the structure of gut microbiota in turn (Gu et al., 2017; Koropatkin and Martens, 2017).
Gut microbiota is predominated by bacterial phyla Firmicutes and Bacteroidetes, followed by other phyla such as Actinobacteria, Proteobacteria and Verrucomicrobia (Woting and Blaut, 2016). With the growing recognition of gut microbiome as the second human genome, pharmacomicrobiomics has been introduced as the expansion of pharmacogenomics, which facilitates the investigation of the interaction between microbiome variation and drugs response (Doestzada et al., 2018). On the one hand, various studies have shown that antidiabetic drugs can affect the composition and function of gut microbiota (Forslund et al., 2015; Wu et al., 2017). On the other hand, the gut microbiota can influence an individual’s response to a specific drug by altering the drug’s bioactivity, bioavailability or toxicity (Koppel et al., 2017). A recent study showed that two-thirds of 271 tested drugs were subject to gut microbiota metabolism (Zimmermann et al., 2019), in which microbial enzymes transformed them into inactive or even toxic drug metabolites (Spanogiannopoulos et al., 2016). Despite the fact that interaction between gut microbiota and antidiabetic agents is increasingly being understood, the role of gut microbiota in the drug efficacy and safety is not fully clarified.
In the present review, we clarify the interaction between gut microbiota and antidiabetic agents, such as metformin, α-glucosidase inhibitors, glucagon-like peptide-1 (GLP-1) receptor agonists, dipeptidyl peptidase-4 (DPP4) inhibitors, sodium-glucose cotransporter 2 (SGLT2) inhibitors, traditional Chinese medicines (TCMs) and other antidiabetic drugs, as well as address the therapeutics based on gut microbiota, aiming to develop personalized treatments and potential individualized preventative and therapeutic strategies.
Interaction Between Gut Microbiota and Antidiabetic Drugs
Lines of evidence have suggested that gut microbiota can not only be influenced by antidiabetic drugs (Table 1), but also in turn affect an individual’s response to those drugs. Furthermore, the interaction between gut microbiota and antidiabetic drugs is complex and bidirectional (Figure 1).
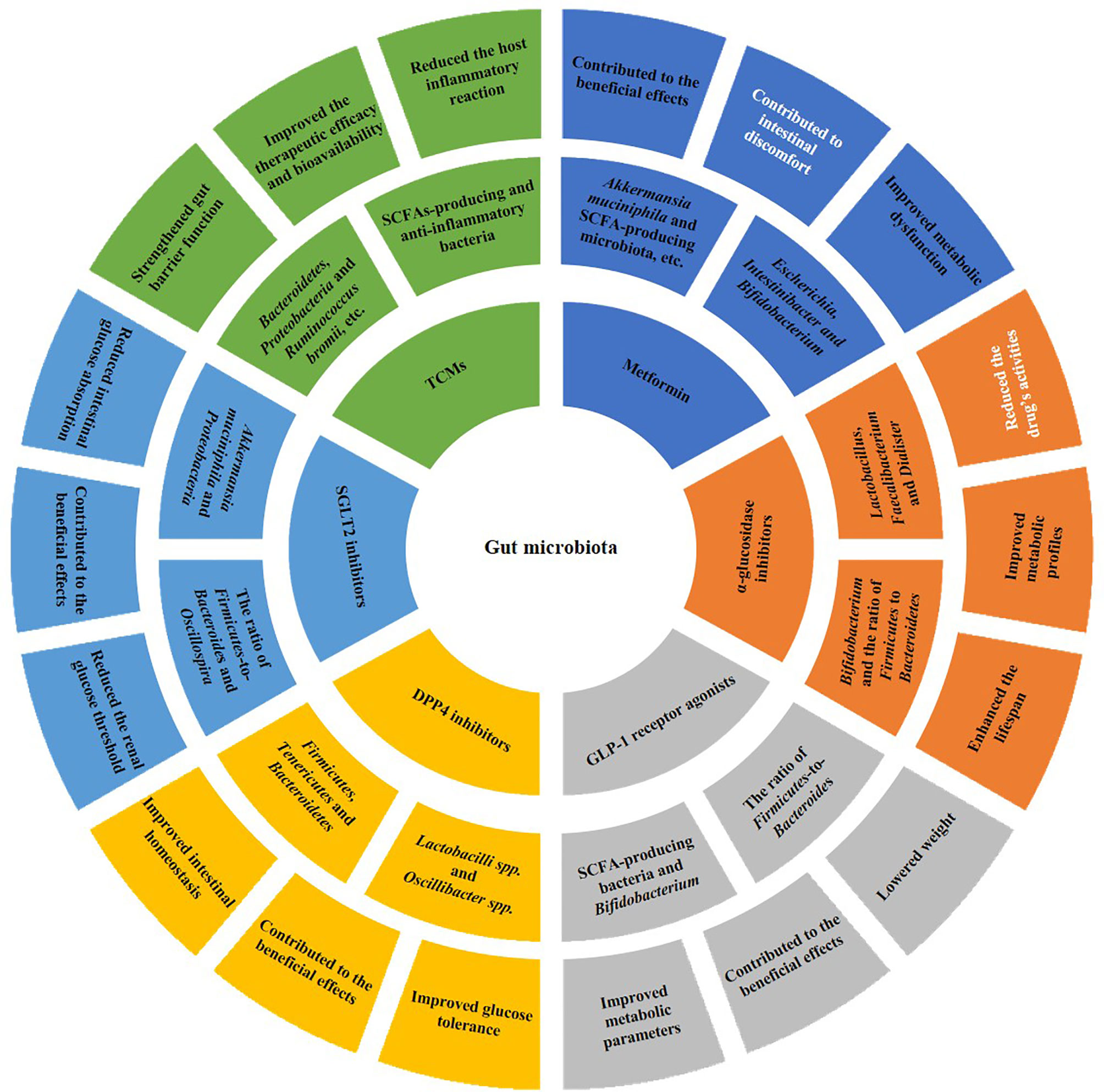
Figure 1 Bidirectional interaction between gut microbiota and antidiabetic drugs. On the one hand, antidiabetic drugs, including metformin, α-glucosidase inhibitors, GLP-1 receptor agonists, DPP4 inhibitors, SGLT2 inhibitors and TCMs, can affect the composition of gut microbiota (the second outermost circle). On the other hand, the gut microbiota can reduce the inflammatory reaction and alter the drug’s bioactivity, bioavailability or toxicity, thus influencing drug’s efficacy and safety, as well as improving metabolic dysfunction (the outermost circle). In the outermost ring, white fonts represent negative impacts of the antidiabetic drugs, while black fonts represent positive impacts. SCFA, short-chain fatty acid; GLP-1, glucagon-like peptide-1; DPP4, dipeptidyl peptidase-4; SGLT2, sodium-glucose cotransporter 2; TCMs, traditional Chinese medicines.
Antidiabetic Drugs Influencing the Gut Microbiota
Metformin is the most commonly used glucose-lowering drug for the treatment of T2DM, especially T2DM associated with obesity. Previous studies indicated that intravenous administration of metformin did not lower glucose in contrast to oral metformin (Bonora et al., 1984), and the bioactivity of metformin originated in the intestine (Bailey et al., 2008). There is evidence to suggest that metformin changes microbiota composition in not only T2DM patients (Forslund et al., 2015; Wu et al., 2017; Nakajima et al., 2020), but also in healthy people (Bryrup et al., 2019; Ejtahed et al., 2019). Metagenomic analysis of microbiota suggested that metformin influenced antidiabetic effect through short-chain fatty acids (SCFAs) production, as well as potential microbial genes and pathways (Lee and Ko, 2014; Forslund et al., 2015; de la Cuesta-Zuluaga et al., 2017; Wu et al., 2017; Bauer et al., 2018). In addition, increase in the production of SCFAs, especially butyrate and propionate, activated intestinal gluconeogenesis, which improved glycemic control and reduced hepatic glucose production, appetite and body weight (Ejtahed et al., 2016). To elucidate the mechanism by which gut microbiota mediated the antidiabetic effects of metformin, a further study investigated metformin-microbiota interactions and showed that metformin affected pathways with biological functions in species from mucin-degradation bacteria and SCFA production, and related genes in these species encoded metalloproteins or metal transporters (Wu et al., 2017). A systematic review stressed that the changes of gut microbiota were associated with metformin, and T2DM patients receiving metformin showed increases in Enterobacteriales and Akkermansia muciniphila, a mucin-degrading bacteria that has been shown to reverse metabolic disorders (Cao et al., 2020). On the other hand, a randomized trial reported that metformin shifted long-term gut microbiota composition, increasing E. coli and R. torques and decreasing I. bartlettii and R. intestinalis at 6th and 12nd month in overweight and obese cancer survivors, respectively (Mueller et al., 2021). Furthermore, in healthy subjects without changes in glycemic control, metformin led to an increased abundance of Escherichia/Shigella spp. and Bilophila wadsworthia, as well as a reduced abundance of Clostridium spp. and Intestinibacter spp. (Bryrup et al., 2019). These results suggest that the changes in microbiota were caused by metformin itself, rather than simply reflecting improved glycemic control.
To figure out the association between diabetes and gut microbiota modified by metformin, Cuesta-Zuluaga et al. performed a retrospective study and found that patients with diabetes taking metformin had higher relative abundance of mucin-degradation Akkermansia muciniphila and several sorts of SCFA-producing microbiota compared with participants without diabetes (de la Cuesta-Zuluaga et al., 2017). Conversely, for diabetic patients not taking metformin, relative abundance was higher in Clostridiaceae 02d06 and lower in Enterococcus casseliflavus (de la Cuesta-Zuluaga et al., 2017).
α-glucosidase inhibitors, including acarbose, voglibose and miglitol, are the first-line drugs in noninsulin-dependent T2DM characterized by their high efficacy in postponing the digestion of carbohydrates and reducing postprandial hyperglycemia (Montandon and Jornayvaz, 2017), those medications inhibit carbohydrate hydrolysis by binding to human intestinal maltase-glucoamylase (MGAM) and sucrase-isomaltase (SI), and consequently delay and reduce the absorption of glucose. Furthermore, there is growing evidence that α-glucosidase inhibitors impact microbiota composition. For instance, T2DM patients treated with acarbose showed increased abundance of Bifidobacterium longum and decreased concentration of lipopolysaccharides (Su et al., 2015). Another clinical trial suggested that Butyricicoccus, Phascolarctobacterium, and Ruminococcus decreased while Lactobacillus, Faecalibacterium, and Dialister increased in patients with prediabetes after acarbose treatment (Zhang X. et al., 2017). Interestingly, Smith et al. observed that there were notable changes in microbial communities and the concentrations of SCFAs in the mice treated with acarbose compared with those of control mice, and microbial communities and fecal SCFAs increased the lifespan of the mice treated with acarbose (Smith et al., 2019). Recently, a study from Chinese population provided that α-glucosidase inhibitors contributed to the plentitude of Bifidobacterium and Lactobacillus, as well as promoted several amino acid pathways (Zhang F. et al., 2019). Also, 12-week voglibose administration decreased the ratio of Firmicutes to Bacteroidetes and improved metabolic profiles including those of blood glucose and lipid metabolism (Do et al., 2016). Therefore, it has been suggested that α-glucosidase inhibitors may have beneficial effects on glycemic control partly through gut microbiota in T2DM.
GLP-1 is an incretin hormone secreted by intestinal endocrine cells (L cells) in response to food ingestion (Drucker and Nauck, 2006). It can enhance glucose-induced insulin from pancreatic β-cells and suppress glucagon secretion; in addition, it also contributes to the inhibition of appetite and gastric emptying (Drucker and Nauck, 2006; Baggio and Drucker, 2014). Numbers of studies have shown that gut microbiota modulates satiety and glucose homoeostasis by inducing the secretion of GLP-1 in mice (Tolhurst et al., 2012; Kimura et al., 2013; Vettorazzi et al., 2016; Aoki et al., 2017). Meanwhile, GLP-1 receptor agonists, a new class of antidiabetic drugs, were also reported to affect the intestinal environment and, indeed, changes in the gut microbiota had been linked to GLP-1 receptor agonists (Wang et al., 2016; Zhang et al., 2018; Zhao et al., 2018; Charpentier et al., 2021; Shang et al., 2021). Generally, the Firmicutes to Bacteroidetes ratio is regarded to be of significant relevance in human gut microbiota composition. Wang et al. demonstrated that liraglutide could modulate the gut microbiota to a more lean-related composition in diabetic mice with normal weight, and they also found a higher Firmicutes-to-Bacteroides ratio after liraglutide treatment (Wang et al., 2016). Inconsistently, another study argued that liraglutide increased the Bacteroides-to-Firmicutes ratio to lower weight significantly regardless of the glycemic status in both simple obese and diabetic obese subjects (Zhao et al., 2018). This discrepancy may be attributed to the different level of hyperglycemia and model systems used. By constructing diabetic animal model, researches showed that administration of GLP-1 receptor agonists profoundly changed the composition of gut microbiota in diabetic male rats (Yuan et al., 2018; Zhang et al., 2018). In particular, several SCFAs-producing bacteria, including Bacteroides, Lachnospiraceae, and probiotic bacteria, including Bifidobacterium, were selectively enhanced in liraglutide-treated diabetic male rats (Zhang et al., 2018). In parallel, liraglutide increased the Bacteroidetes-to-Firmicutes ratio by reducing the Th1 cell frequency and enhancing certain immune cells, such as regulatory T (Treg) cell, innate lymphoid cell 1 (ILC1) and ILC3, which was linked to the nitrogen or the purine metabolism pathways, thus improving glucose-induced insulin secretion (Charpentier et al., 2021). Crucially, GLP-1 receptor agonists could at least partially restore the balance of gut microbiota (Yuan et al., 2018).
DPP4 inhibitors have been proposed to lower blood glucose primarily through inhibiting the degradation of GLP-1 and are recommended as a first-line hypoglycemic treatment in T2DM by the American Association of Clinical Endocrinologists (Drucker and Nauck, 2006; Handelsman et al., 2015). A previous study proposed the DPP-4-like activity of the gut microbiota as a target of DPP-4 inhibition, which could open new therapeutics uses of DPP4 inhibitors to regulate gut microbiota dysbiosis (Olivares et al., 2018b). Liao et al. demonstrated that DDP4 inhibitors improved glucose metabolism by increasing the abundance of Bacteroidetes, and substantially reversing the changes in the gut microbiota induced by high fat diet (HFD) (Liao et al., 2019). An investigation into the effect of sitagliptin on gut microbiota indicated that the phyla Bacteroidetes decreased, while Firmicutes and Tenericutes increased; in addition, sitagliptin partially corrected the dysbiosis of microbiota and altered the population of SCFA-producing bacteria in HFD-fed rats with T2DM (Yan et al., 2016). Similarly, another experiment showed that vildagliptin treatment was associated with increased Bacteroidetes and decreased Firmicutes along with decreased Firmicutes/Bacteroidetes ratio in the diabetic rats (Zhang Q. et al., 2017). In parallel, vildagliptin was proposed to exert beneficial effects through the modulation of gut microbiota, and was linked with increased Lactobacilli spp. and propionate production along with decreased Oscillibacter spp. (Olivares et al., 2018a). To explain these changes, Olivares and his colleagues performed experiments and found that vildagliptin reduced Toll-like receptor (TLR) ligands in caecal content, as well as restored the expression of antimicrobial peptides (AMPs) and the depth of the crypts in the ileum (Olivares et al., 2018a). Furthermore, they also explored that vildagliptin indirectly reduced gene expression of proinflammatory cytokines in liver. These findings demonstrate an important effect of DPP4 inhibitors on the gut microbiota, revealing a potential strategy for improving glucose homeostasis.
SGLT2 inhibitors, a novel class of anti-diabetic substances, are used to achieve the glucose-lowering effect by increasing urinary glucose excretion (Tahrani et al., 2013). After 8 weeks of treatment with dapagliflozin, diabetic mice displayed lower arterial stiffness and blood glucose level, and even more importantly, decreased Firmicutes-to-Bacteroidetes ratio and Oscillospira, as well as increased Akkermansia muciniphila (Lee et al., 2018). Notably, another study demonstrated that dapagliflozin and metformin have similar glucose-lowering effect, but they differentially affected the composition of fecal microbiota in type 2 diabetic rats (Yang et al., 2020). The dapagliflozin group mainly increased the relative abundance of Proteobacteria (especially Desulfovibrionaceae) and did not influence the Firmicutes-to-Bacteroidetes ratio. Conversely, several studies considered that SGLT2 inhibitors had almost no effect on gut bacteria (Du et al., 2018; van Bommel et al., 2020). Nevertheless, it was essential to emphasize that all the study participants had been treated with metformin, which could have overshadowed the potential effects of dapagliflozin on the gut microbiota (van Bommel et al., 2020). In short, further research is needed to figure out the influence of SGLT2 inhibitors on gut microbiota.
TCMs, generally also known as botanical medicine or phytomedicine, have been shown to effectively reduce blood glucose for many years (Lian et al., 2015; Hu et al., 2016). Although TCMs have significant effects on the treatment of T2DM, the mechanisms underlying the therapeutics effects remain elusive. In recent decades, accumulating evidence confirmed that TCMs could improve T2DM by modulation of gut microbiota (Xu et al., 2015; Nie et al., 2019; Zhang B. et al., 2019; Zheng et al., 2020a; Zheng et al., 2020b). Yao et al. observed that Berberine reduced the blood glucose levels and improved glucose tolerance and serum lipid parameters in type 2 diabetic rats (Yao et al., 2020). Further analysis found that the relative abundance was increased for Bacteroidetes and decreased for Proteobacteria and Verrucomicrobia after Berberine treatment (Yao et al., 2020). Likewise, after 30 days of administration, Bereris kansuensis extract increased the abundance of phyla Bacteroidetes and Akkermansia, while reduced the abundance of Proteobacteria and several harmful bacteria (e.g., Enterococcus and Fusobacterium), which was related to its antidiabetic effect in T2DM rats (Xu et al., 2021). To investigate the potential microbial-related mechanism underlying the hypoglycemic effect of Berberine, Zhang et al. found that the inhibition of deoxycholic acid (DCA) biotransformation by Ruminococcus bromii might be involved in the hypoglycemic effect of Berberine (Zhang Y. et al., 2020). Moreover, a recent study demonstrated that the glucose-lowering effect of Gegen Qinlian Decoction could be attributed to Berberine, and both of them significantly modulated the overall gut microbiota structure and enriched butyrate-producing bacteria, including Faecalibacterium and Roseburia (Xu et al., 2020). Additionally, two TCM prescriptions, Xiexin Tang and Huang-Lian-Jie-Du-Decoction were reported to increase SCFAs-producing and anti-inflammatory bacteria (e.g., Parabacteroides, Blautia, Akkermansia, and Adlercreutzia) in T2DM rats (Chen et al., 2018; Wei et al., 2018), providing novel insights into the mechanism and clinical treatment for T2DM from the perspective of gut microbiota. Another two TCMs, JinQi Jiangtang tablets and Andrographolide ameliorated glucose intolerance and insulin resistance in T2DM mice by enriching microbial species of Akkermansia muciniphila and increasing SCFAs level (Cao et al., 2019; Su et al., 2020). The mechanism was related to regulating the gut barrier integrity and reducing the host inflammation. Consistent with the abovementioned results, Chen et al. found that Ge-Gen-Jiao-Tai-Wan formula could reduce blood glucose levels and improve glucose tolerance by regulating the composition of the gut microbiota (Chen et al., 2021). Correspondingly, Ge-Gen-Jiao-Tai-Wan formula up-regulated the beneficial phylum Firmicutes and bile-acid-related genus Lactobacillus, promoting the production of primary bile acids (PBAs) and activating the PBA- farnesoid X receptor (FXR)-GLP-1 pathway (Chen et al., 2021).
In addition to the above-mentioned antidiabetic drugs, the influence of sulfonylurea, peroxisome proliferative activated receptor (PPARG) agonists and insulin on microbiota composition and the consequent metabolic benefits has also been emphasized (Huo et al., 2015; Zhang F. et al., 2019; Madsen et al., 2021). Insulin increased the abundance of Fusobacterium, which up-regulated the genes involved in triglyceride and arachidonic acid metabolism (Zhang F. et al., 2019). Previous studies reported that hippurate was a component of urine and mainly generated from the breakdown of plant phenols and aromatic amino acids by gut microbiota (Williams et al., 2002; Mulder et al., 2005). Metabonomic analysis investigated that levels of those aromatic amino acids (phenylalanine and tryptophan) were decreased, and hippurate was increased in the urine of T2DM patients after the treatment of sulfonylurea, which might be mediated via gut microbiota (Huo et al., 2015). Recently, Madsen et al. revealed that rosiglitazone, a PPARG agonist, improved glucose homeostasis without influencing local gut microbiome in diabetic db/db mice by using full-length bacterial 16S rRNA sequencing (Madsen et al., 2021).
The Impact of Gut Microbiota on Antidiabetic Drug’s Efficacy and Safety
Although the changes in gut microbiota caused by antidiabetic drugs were not simply reflecting improved glycemic control, the antidiabetic effect and safety of antidiabetic agents depended partly on certain groups of gut microbiota (Table 2). Wu et al. transferred the fecal samples from metformin-treated donors (treated with metformin for 4 months) to germ-free mice and indicated that glucose tolerance was improved mainly through increasing the production of SCFAs or altering plasma bile acid composition, suggesting that increased growth of SCFA-producing bacterial species could potentially contribute to the antidiabetic effect of metformin (Wu et al., 2017). Another study revealed that the level of bile acid glycoursodeoxycholic acid (GUDCA) was increased and Bacteroides fragilis was decreased in newly diagnosed T2DM treated with metformin for 3 days (Sun et al., 2018). Further experiments confirmed that B. fragilis–GUDCA–intestinal FXR axis mediated the glucose-lowering effect of metformin.
α-glucosidase inhibitors, which were not absorbed in the small intestine or not metabolized before excretion, created a chance for unintended cross-interaction with gut microbiota. Previous studies identified that the sequence and structural active sites of human intestinal α-glucosidases (MGAM and SI) and microbial α-glucosidases (from Blaubia obeum) were highly homologous, and microbial α-glucosidases could process dietary carbohydrates as well as be inhibited by α-glucosidase inhibitors with comparable strengths (Kuriyama et al., 2008; Natori et al., 2011). Thus, the location and any changes of these active sites might affect the access and specificity of these α-glucosidases to α-glucosidase inhibitors (Tan et al., 2018), mediating their therapeutic effect.
GLP-1 resistance has been reported to seriously impair the effect of GLP-1 receptor agonists (Knop et al., 2012). Grasset et al. identified a specific set of ileum bacteria impairing the GLP-1-activated gut brain axis for the control of insulin secretion and gastric emptying, hence inducing GLP-1 resistance (Grasset et al., 2017). Intriguingly, fecal samples from DDP4 inhibitors-treated T2DM patients transferred to HFD-fed mice improved the glucose intolerance of the recipients, suggesting that the altered gut microbiota contributed to hypoglycemic effects of DDP4 inhibitors even in the absence of additional treatments (Liao et al., 2019). In addition, the gut microbiota might improve the therapeutic efficacy and bioavailability of TCMs by affecting their transformation and absorption (Wang et al., 2017).
In addition to the impact on drug efficacy, gut microbiota can also contribute to the side effects of antidiabetic drugs. It is well known that gastrointestinal side effects are reported in up to one-third of patients taking metformin, and these side effects can be attributed to the identified metabolism genes (mainly derived from an increase of E. coli species) and the increase of virulence factors (Forslund et al., 2015). Because of the high homology of the active sites of human α-glucosidases and gut bacterial α-glucosidases, one proposed theory was that α-glucosidase inhibitors could affect the bacterial α-glucosidases in human gut and exert beneficial effects or create adverse gastrointestinal symptoms (Tan et al., 2018).
New Insights for Developing Personalized Treatments
Given the interplay between gut microbiota and antidiabetic drugs, there is increasing awareness that altering microbiota can impact metabolic phenotype and provide a rational basis for targeting gut microbiota to develop personalized treatments in T2DM (Aron-Wisnewsky et al., 2019; Ghorbani et al., 2021; Huda et al., 2021). Several new insights including fecal microbiota transplantation (FMT), probiotics or prebiotics, and intermittent-fasting could contribute to the desired drug response and personalized medicine (Table 3 and Figure 2).
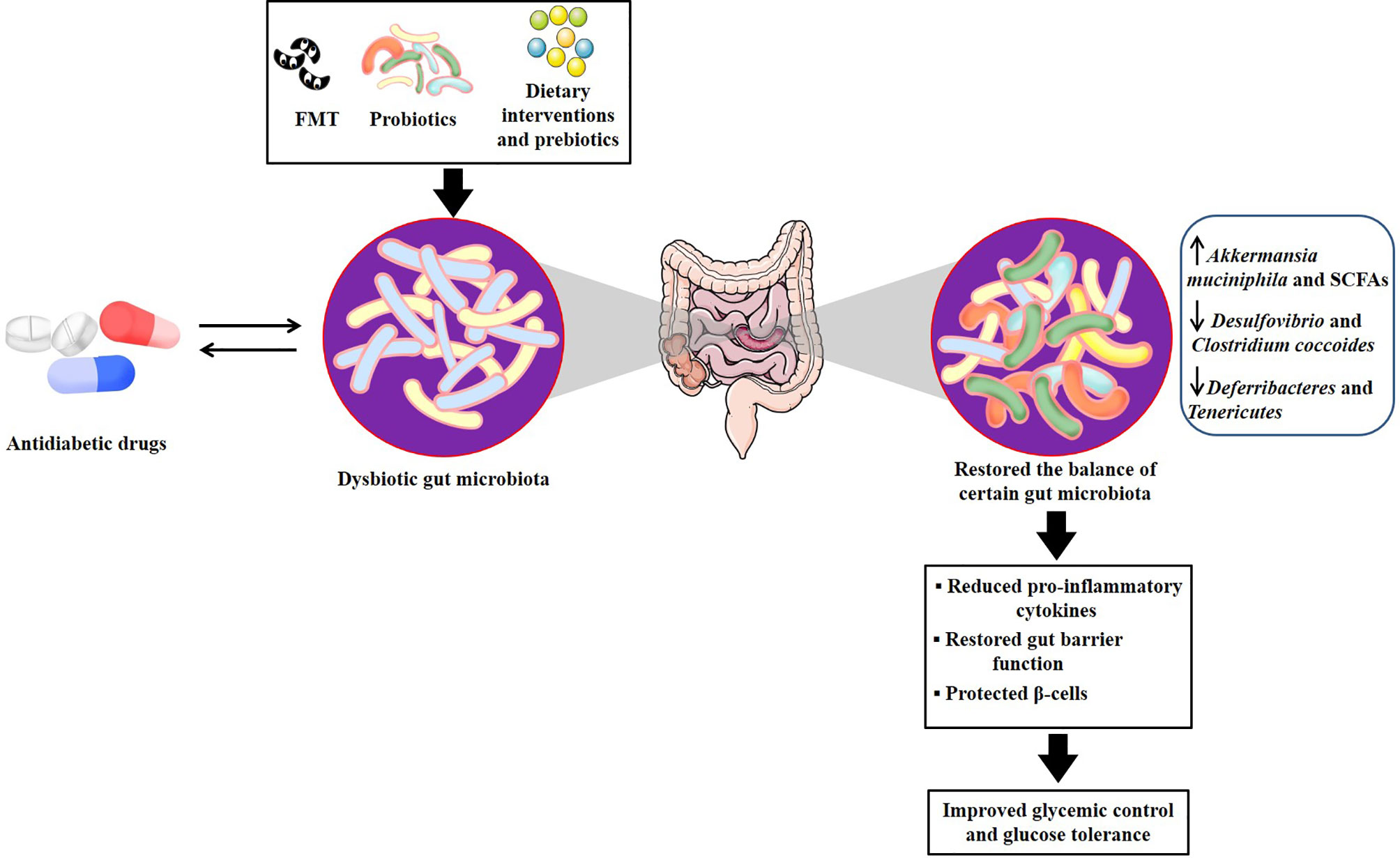
Figure 2 Potential mechanisms of microbial-based therapeutics for developing personalized treatments in T2DM. There are several relevant mechanisms through which antidiabetic drugs treat T2DM by regulating the gut microbiota. Microbial-based therapeutics, including FMT, probiotics and dietary interventions and prebiotics, could directly target gut microbiota or act as an adjunctive to antidiabetic drugs to restore the balance of several certain dysbiotic gut microbiota, which contributed to reducing pro-inflammatory cytokines, restoring gut barrier function, as well as protecting β cells, therefore improving glycemic control and glucose tolerance. FMT, fecal microbiota transplantation; T2DM, type 2 diabetes mellitus.
FMT
FMT is a process of transferring stool from a healthy donor to another patient’s intestinal tract, which can not only reverse gut microbiota dysbiosis (He et al., 2015), but also rebuild the recipient’s intestinal microbial ecosystem (Groen and Nieuwdorp, 2017). Over the past few years, T2DM has been suggested responsive to FMT (Khoruts and Sadowsky, 2016), which also has attracted increased attention.
In the FMT-treated mice with diabetes, Desulfovibrio and Clostridium coccoides levels were significantly decreased, and the fecal levels of Akkermansia muciniphila were increased (Zhang P.P. et al., 2020). Moreover, Akkermansia muciniphila led to increased expression of HDAC3, which remarkably improved glycolipid metabolism. Likewise, glucose tolerance was improved by transfer of fecal samples from patients treated with metformin to germ-free mice (Wu et al., 2017). Metagenomics analysis indicated that metformin promoted functional shifts in gut microbiota of fecal samples, including lipopolysaccharide biosynthesis and SCFA metabolism. Notably, another study investigated the effects of lean donor-FMT versus self-FMT on patients with metabolic syndrome and found that insulin sensitivity was not changed at 18 weeks after self-FMT, but was significantly improved at 6 weeks after lean donor-FMT (Kootte et al., 2017). Moreover, a recent review considered that whether FMT was a future therapeutic option needed further evaluation (Aron-Wisnewsky et al., 2019). Collectively, FMT may be an interesting option to modify certain gut microbiota and a potential target for developing personalized treatments.
Probiotics
Probiotics are live microorganisms, which have a beneficial effect on human health when administered in adequate amounts (Hill et al., 2014; Kesika et al., 2019). A number of studies revealed that multi-strain probiotic supplement, including Lactobacillus plantarum HAC01 and Probioglu™, lowered blood glucose and HbA1c levels, as well as improved glucose tolerance by protecting β-cells and restoring the gut microbiota and SCFAs in streptozotocin-induced diabetic rat models with HFD (Hsieh et al., 2021; Lee et al., 2021). Furthermore, a randomized clinical trial performed by Toejing et al. demonstrated that probiotic supplementation L. paracasei HII01 significantly decreased fasting blood glucose level by increasing beneficial bacteria and decreasing pathogenic bacteria, thus suggesting a potential role of this probiotic as an adjuvant treatment in T2DM (Toejing et al., 2021). Another randomized controlled pilot study showed that participants taking metformin in combination with probiotics had higher concentration of plasma butyrate and SCFA-producing bacteria after the 12-week intervention, lower fasting plasma glucose and weaker insulin resistance, which suggested that probiotic might act as an adjunctive to metformin and thus enhanced glucose management at the individual level (Palacios et al., 2020). Consistent with this result, a recent study showed that the probiotic supplementation improved the glycemic parameters in T2DM patients and thus could be recommended as a potential adjuvant treatment alongside medicine for T2DM therapy (Bock et al., 2021). Mechanistically, these probiotics exerted antidiabetic effect and ameliorated the symptom of T2DM, as well as restored gut barrier function via reducing pro-inflammatory cytokines and intestinal permeability, and activating antioxidant enzymes (Sharma et al., 2016; Kim et al., 2018; Wang et al., 2020).
Dietary Interventions and Prebiotics
Dietary interventions and prebiotics are the ingredients that beneficially affect the host by selectively promoting the growth and the activity of certain bacterial species (Gibson and Roberfroid, 1995; Wu et al., 2011). Although gut microbiota played an important role in human by interacting with host diet, there was large inter-individual variation in the response to diet (Lampe et al., 2013), and studies displayed that the gut microbial composition could be used to identify those participants who would benefit from dietary interventions or prebiotics (Korpela et al., 2014; Salonen et al., 2014; Kovatcheva-Datchary et al., 2015). A meta-analysis of randomized controlled trials concluded that dietary interventions supplemented with either prebiotics or synbiotics resulted in improvements in glucose homeostasis in patients with T2DM (Mahboobi et al., 2018). Moreover, Yu et al. argued that different dietary supplements might exert synergistic protective effects against T2DM via reducing the blood glucose levels and effectively improving some beneficial bacterium (Yu et al., 2021).
Additionally, prebiotic inulin was conducive to alleviate T2DM by modulating gut microbiota (Li et al., 2019; Birkeland et al., 2020). Further analysis found that dietary inulin increased the relative abundance of Cyanobacteria and Bacteroides, as well as reduced the relative abundance of Ruminiclostridium, Deferribacteres, and Tenericutes via suppressing inflammation (Li et al., 2019). Noteworthily, a symbiotic mixture of prebiotics and probiotics supplementation could be more beneficial compared to prebiotic or probiotic alone (Morshedi et al., 2020). A recent randomized trial also demonstrated that administration of berberine with probiotics improved blood glucose levels compared to the group treated with berberine alone (Zhang Y. et al., 2020). Therefore, there will be a promising synergistic approach in the future involving both diet and prebiotics in the personalized prevention and treatment of T2DM. Currently, a prominent study integrated clinical and microbial data and devised machine learning algorithms for postprandial glycemic response (PPGR) prediction (Shilo et al., 2022), which implied that personally tailored treatments could be customized for individuals in the future.
Conclusions and Future Perspectives
With the bidirectional interaction between gut microbiota and antidiabetic agents is increasingly being understood, targeting gut microbiota can contribute to increasing drug efficacy and safety, and thus enable a personalized medicine approach for the treatment and management of T2DM.
It is noteworthy that pharmacomicrobiomics play an essential role in combing personal microbiome and genetic profiles to better predict individual’s drug response and efficacy at the individual level. Mapping and modeling human microbiome drug metabolism with genome-scale and meta-omics analyses could improve our understanding of the roles of drugs and microbial communities in personalized medicine (Zimmermann et al., 2019; Javdan et al., 2020; Heinken et al., 2021). With the advance of multi-omics in gut microbiota research, microbiota-based personalized treatment is expected to be achieved by integration of multi-omics data with microbiome data in T2DM patients. Excitingly, thanks to current technologies, around 80% of gut microbes are readily available using bacterial culture (Lagier et al., 2016), which helps to mimic the intestinal environment and makes it possible to conduct individual-based drug testing on cultured bacteria, thus developing novel preventative strategies and personalized therapeutic targets.
Finally, applying the abovementioned novel approaches may contribute to a better understanding of the interactions between gut microbiota and antidiabetic drugs in T2DM, ultimately leading to future potential major advances in personalized medicine.
Author Contributions
WL, ZL and JZ wrote the original draft. WL and ZL reviewed and edited the draft. BS revised and supervised overall project. All authors read and approved the final version of manuscript. All authors contributed to the article and approved the submitted version.
Funding
This work was supported by grants from National Natural Science Foundation of China (No. 82104307, 82003872), Natural Science Foundation of Hunan Province (No. 2021JJ40865, 2021JJ40847, 2020JJ5513).
Conflict of Interest
The authors declare that the research was conducted in the absence of any commercial or financial relationships that could be construed as a potential conflict of interest.
Publisher’s Note
All claims expressed in this article are solely those of the authors and do not necessarily represent those of their affiliated organizations, or those of the publisher, the editors and the reviewers. Any product that may be evaluated in this article, or claim that may be made by its manufacturer, is not guaranteed or endorsed by the publisher.
Abbreviations
T2DM, Type 2 diabetes mellitus; GLP-1, glucagon-like peptide-1; DPP4, dipeptidyl peptidase-4; SGLT2, sodium-glucose cotransporter 2; TCMs, traditional Chinese medicines; SCFAs, short-chain fatty acids; PPARG, peroxisome proliferative activated receptor; MGAM, maltase-glucoamylase, SI, sucrase-isomaltase; Treg, regulatory T; ILC1, innate lymphoid cell 1; HFD, high fat diet; TLR, Toll-like receptor; AMPs, antimicrobial peptides; DCA, deoxycholic acid; PBAs, primary bile acids; FXR, farnesoid X receptor; GUDCA, glycoursodeoxycholic acid; FMT, fecal microbiota transplantation; PPGR, postprandial glycemic response.
References
Aoki, R., Kamikado, K., Suda, W., Takii, H., Mikami, Y., Suganuma, N., et al. (2017). A Proliferative Probiotic Bifidobacterium Strain in the Gut Ameliorates Progression of Metabolic Disorders via Microbiota Modulation and Acetate Elevation. Sci. Rep. 7, 43522. doi: 10.1038/srep43522
Aron-Wisnewsky, J., Clément, K., Nieuwdorp, M. (2019). Fecal Microbiota Transplantation: A Future Therapeutic Option for Obesity/Diabetes? Curr. Diabetes Rep. 19 (8), 51. doi: 10.1007/s11892-019-1180-z
Baggio, L. L., Drucker, D. J. (2014). Glucagon-Like Peptide-1 Receptors in the Brain: Controlling Food Intake and Body Weight. J. Clin. Invest. 124 (10), 4223–4226. doi: 10.1172/jci78371
Bailey, C. J., Wilcock, C., Scarpello, J. H. (2008). Metformin and the Intestine. Diabetologia 51 (8), 1552–1553. doi: 10.1007/s00125-008-1053-5
Bauer, P. V., Duca, F. A., Waise, T. M. Z., Rasmussen, B. A., Abraham, M. A., Dranse, H. J., et al. (2018). Metformin Alters Upper Small Intestinal Microbiota That Impact a Glucose-SGLT1-Sensing Glucoregulatory Pathway. Cell Metab. 27 (1), 101–117.e105. doi: 10.1016/j.cmet.2017.09.019
Birkeland, E., Gharagozlian, S., Birkeland, K. I., Valeur, J., Måge, I., Rud, I., et al. (2020). Prebiotic Effect of Inulin-Type Fructans on Faecal Microbiota and Short-Chain Fatty Acids in Type 2 Diabetes: A Randomised Controlled Trial. Eur. J. Nutr. 59 (7), 3325–3338. doi: 10.1007/s00394-020-02282-5
Bock, P. M., Telo, G. H., Ramalho, R., Sbaraini, M., Leivas, G., Martins, A. F., et al. (2021). The Effect of Probiotics, Prebiotics or Synbiotics on Metabolic Outcomes in Individuals With Diabetes: A Systematic Review and Meta-Analysis. Diabetologia 64 (1), 26–41. doi: 10.1007/s00125-020-05295-1
Bonora, E., Cigolini, M., Bosello, O., Zancanaro, C., Capretti, L., Zavaroni, I., et al. (1984). Lack of Effect of Intravenous Metformin on Plasma Concentrations of Glucose, Insulin, C-Peptide, Glucagon and Growth Hormone in Non-Diabetic Subjects. Curr. Med. Res. Opin. 9 (1), 47–51. doi: 10.1185/03007998409109558
Bouter, K. E., van Raalte, D. H., Groen, A. K., Nieuwdorp, M. (2017). Role of the Gut Microbiome in the Pathogenesis of Obesity and Obesity-Related Metabolic Dysfunction. Gastroenterology 152 (7), 1671–1678. doi: 10.1053/j.gastro.2016.12.048
Bryrup, T., Thomsen, C. W., Kern, T., Allin, K. H., Brandslund, I., Jørgensen, N. R., et al. (2019). Metformin-Induced Changes of the Gut Microbiota in Healthy Young Men: Results of a non-Blinded, One-Armed Intervention Study. Diabetologia 62 (6), 1024–1035. doi: 10.1007/s00125-019-4848-7
Cao, T. T. B., Wu, K. C., Hsu, J. L., Chang, C. S., Chou, C., Lin, C. Y., et al. (2020). Effects of Non-Insulin Anti-Hyperglycemic Agents on Gut Microbiota: A Systematic Review on Human and Animal Studies. Front. Endocrinol. (Lausanne) 11. doi: 10.3389/fendo.2020.573891
Cao, Y., Yao, G., Sheng, Y., Yang, L., Wang, Z., Yang, Z., et al. (2019). JinQi Jiangtang Tablet Regulates Gut Microbiota and Improve Insulin Sensitivity in Type 2 Diabetes Mice. J. Diabetes Res. 2019, 1872134. doi: 10.1155/2019/1872134
Cersosimo, E., et al. (2000)"Pathogenesis of Type 2 Diabetes Mellitus,". In: Endotext (South Dartmouth (MA: MDText.com, Inc) (Accessed 12 Mar 2019).
Charpentier, J., Briand, F., Lelouvier, B., Servant, F., Azalbert, V., Puel, A., et al. (2021). Liraglutide Targets the Gut Microbiota and the Intestinal Immune System to Regulate Insulin Secretion. Acta Diabetol. 58 (7), 881–897. doi: 10.1007/s00592-020-01657-8
Chen, M., Liao, Z., Lu, B., Wang, M., Lin, L., Zhang, S., et al. (2018). Huang-Lian-Jie-Du-Decoction Ameliorates Hyperglycemia and Insulin Resistant in Association With Gut Microbiota Modulation. Front. Microbiol. 9. doi: 10.3389/fmicb.2018.02380
Chen, H., Yao, Y., Wang, W., Wang, D. (2021). Ge-Gen-Jiao-Tai-Wan Affects Type 2 Diabetic Rats by Regulating Gut Microbiota and Primary Bile Acids. Evid. Based Complement. Alternat. Med. 2021, 5585952. doi: 10.1155/2021/5585952
de la Cuesta-Zuluaga, J., Mueller, N. T., Corrales-Agudelo, V., Velásquez-Mejía, E. P., Carmona, J. A., Abad, J. M., et al. (2017). Metformin Is Associated With Higher Relative Abundance of Mucin-Degrading Akkermansia Muciniphila and Several Short-Chain Fatty Acid-Producing Microbiota in the Gut. Diabetes Care 40 (1), 54–62. doi: 10.2337/dc16-1324
Doestzada, M., Vila, A. V., Zhernakova, A., Koonen, D. P. Y., Weersma, R. K., Touw, D. J., et al. (2018). Pharmacomicrobiomics: A Novel Route Towards Personalized Medicine? Protein Cell 9 (5), 432–445. doi: 10.1007/s13238-018-0547-2
Do, H. J., Lee, Y. S., Ha, M. J., Cho, Y., Yi, H., Hwang, Y. J., et al. (2016). Beneficial Effects of Voglibose Administration on Body Weight and Lipid Metabolism via Gastrointestinal Bile Acid Modification. Endocr. J. 63 (8), 691–702. doi: 10.1507/endocrj.EJ15-0747
Drucker, D. J., Nauck, M. A. (2006). The Incretin System: Glucagon-Like Peptide-1 Receptor Agonists and Dipeptidyl Peptidase-4 Inhibitors in Type 2 Diabetes. Lancet 368 (9548), 1696–1705. doi: 10.1016/s0140-6736(06)69705-5
Du, F., Hinke, S. A., Cavanaugh, C., Polidori, D., Wallace, N., Kirchner, T., et al. (2018). Potent Sodium/Glucose Cotransporter SGLT1/2 Dual Inhibition Improves Glycemic Control Without Marked Gastrointestinal Adaptation or Colonic Microbiota Changes in Rodents. J. Pharmacol. Exp. Ther. 365 (3), 676–687. doi: 10.1124/jpet.118.248575
Ejtahed, H. S., Soroush, A. R., Angoorani, P., Larijani, B., Hasani-Ranjbar, S. (2016). Gut Microbiota as a Target in the Pathogenesis of Metabolic Disorders: A New Approach to Novel Therapeutic Agents. Horm. Metab. Res. 48 (6), 349–358. doi: 10.1055/s-0042-107792
Ejtahed, H. S., Tito, R. Y., Siadat, S. D., Hasani-Ranjbar, S., Hoseini-Tavassol, Z., Rymenans, L., et al. (2019). Metformin Induces Weight Loss Associated With Gut Microbiota Alteration in non-Diabetic Obese Women: A Randomized Double-Blind Clinical Trial. Eur. J. Endocrinol. 180 (3), 165–176. doi: 10.1530/eje-18-0826
Forslund, K., Hildebrand, F., Nielsen, T., Falony, G., Le Chatelier, E., Sunagawa, S., et al. (2015). Disentangling Type 2 Diabetes and Metformin Treatment Signatures in the Human Gut Microbiota. Nature 528 (7581), 262–266. doi: 10.1038/nature15766
Ghorbani, Y., Schwenger, K. J. P., Allard, J. P. (2021). Manipulation of Intestinal Microbiome as Potential Treatment for Insulin Resistance and Type 2 Diabetes. Eur. J. Nutr. 60 (5), 2361–2379. doi: 10.1007/s00394-021-02520-4
Gibson, G. R., Roberfroid, M. B. (1995). Dietary Modulation of the Human Colonic Microbiota: Introducing the Concept of Prebiotics. J. Nutr. 125 (6), 1401–1412. doi: 10.1093/jn/125.6.1401
Grasset, E., Puel, A., Charpentier, J., Collet, X., Christensen, J. E., Tercé, F., et al. (2017). A Specific Gut Microbiota Dysbiosis of Type 2 Diabetic Mice Induces GLP-1 Resistance Through an Enteric NO-Dependent and Gut-Brain Axis Mechanism. Cell Metab. 25 (5), 1075–1090.e1075. doi: 10.1016/j.cmet.2017.04.013
Groen, A. K., Nieuwdorp, M. (2017). An Evaluation of the Therapeutic Potential of Fecal Microbiota Transplantation to Treat Infectious and Metabolic Diseases. EMBO Mol. Med. 9 (1), 1–3. doi: 10.15252/emmm.201607035
Gu, Y., Wang, X., Li, J., Zhang, Y., Zhong, H., Liu, R., et al. (2017). Analyses of Gut Microbiota and Plasma Bile Acids Enable Stratification of Patients for Antidiabetic Treatment. Nat. Commun. 8 (1), 1785. doi: 10.1038/s41467-017-01682-2
Handelsman, Y., Bloomgarden, Z. T., Grunberger, G., Umpierrez, G., Zimmerman, R. S., Bailey, T. S., et al. (2015). American Association of Clinical Endocrinologists and American College of Endocrinology - Clinical Practice Guidelines for Developing a Diabetes Mellitus Comprehensive Care Plan - 2015. Endocr. Pract. 21 Suppl 1 (Suppl 1), 1–87. doi: 10.4158/ep15672.gl
Heinken, A., Basile, A., Hertel, J., Thinnes, C., Thiele, I. (2021). Genome-Scale Metabolic Modeling of the Human Microbiome in the Era of Personalized Medicine. Annu. Rev. Microbiol. 75, 199–222. doi: 10.1146/annurev-micro-060221-012134
He, C., Shan, Y., Song, W. (2015). Targeting Gut Microbiota as a Possible Therapy for Diabetes. Nutr. Res. 35 (5), 361–367. doi: 10.1016/j.nutres.2015.03.002
Hill, C., Guarner, F., Reid, G., Gibson, G. R., Merenstein, D. J., Pot, B., et al. (2014). Expert Consensus Document. The International Scientific Association for Probiotics and Prebiotics Consensus Statement on the Scope and Appropriate Use of the Term Probiotic. Nat. Rev. Gastroenterol. Hepatol. 11 (8), 506–514. doi: 10.1038/nrgastro.2014.66
Hsieh, P. S., Ho, H. H., Tsao, S. P., Hsieh, S. H., Lin, W. Y., Chen, J. F., et al. (2021). Multi-Strain Probiotic Supplement Attenuates Streptozotocin-Induced Type-2 Diabetes by Reducing Inflammation and β-Cell Death in Rats. PloS One 16 (6), e0251646. doi: 10.1371/journal.pone.0251646
Huda, M. N., Kim, M., Bennett, B. J. (2021). Modulating the Microbiota as a Therapeutic Intervention for Type 2 Diabetes. Front. Endocrinol. (Lausanne) 12. doi: 10.3389/fendo.2021.632335
Huo, T., Xiong, Z., Lu, X., Cai, S. (2015). Metabonomic Study of Biochemical Changes in Urinary of Type 2 Diabetes Mellitus Patients After the Treatment of Sulfonylurea Antidiabetic Drugs Based on Ultra-Performance Liquid Chromatography/Mass Spectrometry. BioMed. Chromatogr. 29 (1), 115–122. doi: 10.1002/bmc.3247
Hu, Y., Zhou, X., Liu, P., Wang, B., Duan, D. M., Guo, D. H. (2016). A Comparison Study of Metformin Only Therapy and Metformin Combined With Chinese Medicine Jianyutangkang Therapy in Patients With Type 2 Diabetes: A Randomized Placebo-Controlled Double-Blind Study. Complement. Ther. Med. 24, 13–18. doi: 10.1016/j.ctim.2015.11.005
Javdan, B., Lopez, J. G., Chankhamjon, P., Lee, Y. J., Hull, R., Wu, Q., et al. (2020). Personalized Mapping of Drug Metabolism by the Human Gut Microbiome. Cell 181 (7), 1661–1679.e1622. doi: 10.1016/j.cell.2020.05.001
Kesika, P., Sivamaruthi, B. S., Chaiyasut, C. (2019). Do Probiotics Improve the Health Status of Individuals With Diabetes Mellitus? A Review on Outcomes of Clinical Trials. BioMed. Res. Int. 2019, 1531567. doi: 10.1155/2019/1531567
Khoruts, A., Sadowsky, M. J. (2016). Understanding the Mechanisms of Faecal Microbiota Transplantation. Nat. Rev. Gastroenterol. Hepatol. 13 (9), 508–516. doi: 10.1038/nrgastro.2016.98
Kim, Y. A., Keogh, J. B., Clifton, P. M. (2018). Probiotics, Prebiotics, Synbiotics and Insulin Sensitivity. Nutr. Res. Rev. 31 (1), 35–51. doi: 10.1017/s095442241700018x
Kimura, I., Ozawa, K., Inoue, D., Imamura, T., Kimura, K., Maeda, T., et al. (2013). The Gut Microbiota Suppresses Insulin-Mediated Fat Accumulation via the Short-Chain Fatty Acid Receptor GPR43. Nat. Commun. 4, 1829. doi: 10.1038/ncomms2852
Knop, F. K., Aaboe, K., Vilsbøll, T., Vølund, A., Holst, J. J., Krarup, T., et al. (2012). Impaired Incretin Effect and Fasting Hyperglucagonaemia Characterizing Type 2 Diabetic Subjects are Early Signs of Dysmetabolism in Obesity. Diabetes Obes. Metab. 14 (6), 500–510. doi: 10.1111/j.1463-1326.2011.01549.x
Kootte, R. S., Levin, E., Salojärvi, J., Smits, L. P., Hartstra, A. V., Udayappan, S. D., et al. (2017). Improvement of Insulin Sensitivity After Lean Donor Feces in Metabolic Syndrome Is Driven by Baseline Intestinal Microbiota Composition. Cell Metab. 26 (4), 611–619.e616. doi: 10.1016/j.cmet.2017.09.008
Koppel, N., Maini Rekdal, V., Balskus, E. P. (2017). Chemical Transformation of Xenobiotics by the Human Gut Microbiota. Science 356 (6344), eaag2770. doi: 10.1126/science.aag2770
Koropatkin, N. M., Martens, E. C. (2017). Meds Modify Microbiome, Mediating Their Effects. Cell Metab. 26 (3), 456–457. doi: 10.1016/j.cmet.2017.08.022
Korpela, K., Flint, H. J., Johnstone, A. M., Lappi, J., Poutanen, K., Dewulf, E., et al. (2014). Gut Microbiota Signatures Predict Host and Microbiota Responses to Dietary Interventions in Obese Individuals. PloS One 9 (6), e90702. doi: 10.1371/journal.pone.0090702
Kovatcheva-Datchary, P., Nilsson, A., Akrami, R., Lee, Y. S., De Vadder, F., Arora, T., et al. (2015). Dietary Fiber-Induced Improvement in Glucose Metabolism Is Associated With Increased Abundance of Prevotella. Cell Metab. 22 (6), 971–982. doi: 10.1016/j.cmet.2015.10.001
Kuriyama, C., Kamiyama, O., Ikeda, K., Sanae, F., Kato, A., Adachi, I., et al. (2008). In Vitro Inhibition of Glycogen-Degrading Enzymes and Glycosidases by Six-Membered Sugar Mimics and Their Evaluation in Cell Cultures. Bioorg. Med. Chem. 16 (15), 7330–7336. doi: 10.1016/j.bmc.2008.06.026
Lagier, J. C., Khelaifia, S., Alou, M. T., Ndongo, S., Dione, N., Hugon, P., et al. (2016). Culture of Previously Uncultured Members of the Human Gut Microbiota by Culturomics. Nat. Microbiol. 1, 16203. doi: 10.1038/nmicrobiol.2016.203
Lampe, J. W., Navarro, S. L., Hullar, M. A., Shojaie, A. (2013). Inter-Individual Differences in Response to Dietary Intervention: Integrating Omics Platforms Towards Personalised Dietary Recommendations. Proc. Nutr. Soc. 72 (2), 207–218. doi: 10.1017/s0029665113000025
Lee, D. M., Battson, M. L., Jarrell, D. K., Hou, S., Ecton, K. E., Weir, T. L., et al. (2018). SGLT2 Inhibition via Dapagliflozin Improves Generalized Vascular Dysfunction and Alters the Gut Microbiota in Type 2 Diabetic Mice. Cardiovasc. Diabetol. 17 (1), 62. doi: 10.1186/s12933-018-0708-x
Lee, H., Ko, G. (2014). Effect of Metformin on Metabolic Improvement and Gut Microbiota. Appl. Environ. Microbiol. 80 (19), 5935–5943. doi: 10.1128/aem.01357-14
Lee, Y. S., Lee, D., Park, G. S., Ko, S. H., Park, J., Lee, Y. K., et al. (2021). Lactobacillus Plantarum HAC01 Ameliorates Type 2 Diabetes in High-Fat Diet and Streptozotocin-Induced Diabetic Mice in Association With Modulating the Gut Microbiota. Food Funct. 12 (14), 6363–6373. doi: 10.1039/d1fo00698c
Lian, F., Tian, J., Chen, X., Li, Z., Piao, C., Guo, J., et al. (2015). The Efficacy and Safety of Chinese Herbal Medicine Jinlida as Add-On Medication in Type 2 Diabetes Patients Ineffectively Managed by Metformin Monotherapy: A Double-Blind, Randomized, Placebo-Controlled, Multicenter Trial. PloS One 10 (6), e0130550. doi: 10.1371/journal.pone.0130550
Liao, X., Song, L., Zeng, B., Liu, B., Qiu, Y., Qu, H., et al. (2019). Alteration of Gut Microbiota Induced by DPP-4i Treatment Improves Glucose Homeostasis. EBioMedicine 44, 665–674. doi: 10.1016/j.ebiom.2019.03.057
Li, K., Zhang, L., Xue, J., Yang, X., Dong, X., Sha, L., et al. (2019). Dietary Inulin Alleviates Diverse Stages of Type 2 Diabetes Mellitus via Anti-Inflammation and Modulating Gut Microbiota in Db/Db Mice. Food Funct. 10 (4), 1915–1927. doi: 10.1039/c8fo02265h
Madsen, M. S. A., Grønlund, R. V., Eid, J., Christensen-Dalsgaard, M., Sommer, M., Rigbolt, K., et al. (2021). Characterization of Local Gut Microbiome and Intestinal Transcriptome Responses to Rosiglitazone Treatment in Diabetic Db/Db Mice. BioMed. Pharmacother. 133, 110966. doi: 10.1016/j.biopha.2020.110966
Mahboobi, S., Rahimi, F., Jafarnejad, S. (2018). Effects of Prebiotic and Synbiotic Supplementation on Glycaemia and Lipid Profile in Type 2 Diabetes: A Meta-Analysis of Randomized Controlled Trials. Adv. Pharm. Bull. 8 (4), 565–574. doi: 10.15171/apb.2018.065
Montandon, S. A., Jornayvaz, F. R. (2017). Effects of Antidiabetic Drugs on Gut Microbiota Composition. Genes (Basel) 8 (10), 250. doi: 10.3390/genes8100250
Morshedi, M., Saghafi-Asl, M., Hosseinifard, E. S. (2020). The Potential Therapeutic Effects of the Gut Microbiome Manipulation by Synbiotic Containing-Lactobacillus Plantarum on Neuropsychological Performance of Diabetic Rats. J. Transl. Med. 18 (1), 18. doi: 10.1186/s12967-019-02169-y
Mueller, N. T., Differding, M. K., Zhang, M., Maruthur, N. M., Juraschek, S. P., Miller, E. R., 3rd, et al. (2021). Metformin Affects Gut Microbiome Composition and Function and Circulating Short-Chain Fatty Acids: A Randomized Trial. Diabetes Care 44 (7), 1462–1471. doi: 10.2337/dc20-2257
Mulder, T. P., Rietveld, A. G., van Amelsvoort, J. M. (2005). Consumption of Both Black Tea and Green Tea Results in an Increase in the Excretion of Hippuric Acid Into Urine. Am. J. Clin. Nutr. 81 (1 Suppl), 256s–260s. doi: 10.1093/ajcn/81.1.256S
Nakajima, H., Takewaki, F., Hashimoto, Y., Kajiyama, S., Majima, S., Okada, H., et al. (2020). The Effects of Metformin on the Gut Microbiota of Patients With Type 2 Diabetes: A Two-Center, Quasi-Experimental Study. Life (Basel) 10 (9), 195. doi: 10.3390/life10090195
Natori, Y., Imahori, T., Murakami, K., Yoshimura, Y., Nakagawa, S., Kato, A., et al. (2011). The Synthesis and Biological Evaluation of 1-C-Alkyl-L-Arabinoiminofuranoses, a Novel Class of α-Glucosidase Inhibitors. Bioorg. Med. Chem. Lett. 21 (2), 738–741. doi: 10.1016/j.bmcl.2010.11.112
Nie, Q., Chen, H., Hu, J., Fan, S., Nie, S. (2019). Dietary Compounds and Traditional Chinese Medicine Ameliorate Type 2 Diabetes by Modulating Gut Microbiota. Crit. Rev. Food Sci. Nutr. 59 (6), 848–863. doi: 10.1080/10408398.2018.1536646
Olivares, M., Neyrinck, A. M., Pötgens, S. A., Beaumont, M., Salazar, N., Cani, P. D., et al. (2018a). The DPP-4 Inhibitor Vildagliptin Impacts the Gut Microbiota and Prevents Disruption of Intestinal Homeostasis Induced by a Western Diet in Mice. Diabetologia 61 (8), 1838–1848. doi: 10.1007/s00125-018-4647-6
Olivares, M., Schüppel, V., Hassan, A. M., Beaumont, M., Neyrinck, A. M., Bindels, L. B., et al. (2018b). The Potential Role of the Dipeptidyl Peptidase-4-Like Activity From the Gut Microbiota on the Host Health. Front. Microbiol. 9, 1900. doi: 10.3389/fmicb.2018.01900
Palacios, T., Vitetta, L., Coulson, S., Madigan, C. D., Lam, Y. Y., Manuel, R., et al. (2020). Targeting the Intestinal Microbiota to Prevent Type 2 Diabetes and Enhance the Effect of Metformin on Glycaemia: A Randomised Controlled Pilot Study. Nutrients 12 (7), 2041. doi: 10.3390/nu12072041
Salonen, A., Lahti, L., Salojärvi, J., Holtrop, G., Korpela, K., Duncan, S. H., et al. (2014). Impact of Diet and Individual Variation on Intestinal Microbiota Composition and Fermentation Products in Obese Men. Isme J. 8 (11), 2218–2230. doi: 10.1038/ismej.2014.63
Shang, J., Liu, F., Zhang, B., Dong, K., Lu, M., Jiang, R., et al. (2021). Liraglutide-Induced Structural Modulation of the Gut Microbiota in Patients With Type 2 Diabetes Mellitus. PeerJ 9, e11128. doi: 10.7717/peerj.11128
Sharma, P., Bhardwaj, P., Singh, R. (2016). Administration of Lactobacillus Casei and Bifidobacterium Bifidum Ameliorated Hyperglycemia, Dyslipidemia, and Oxidative Stress in Diabetic Rats. Int. J. Prev. Med. 7, 102. doi: 10.4103/2008-7802.188870
Shilo, S., Godneva, A., Rachmiel, M., Korem, T., Kolobkov, D., Karady, T., et al. (2022). Prediction of Personal Glycemic Responses to Food for Individuals With Type 1 Diabetes Through Integration of Clinical and Microbial Data. Diabetes Care 45 (3), 502–511. doi: 10.2337/dc21-1048
Smith, B. J., Miller, R. A., Ericsson, A. C., Harrison, D. C., Strong, R., Schmidt, T. M. (2019). Changes in the Gut Microbiome and Fermentation Products Concurrent With Enhanced Longevity in Acarbose-Treated Mice. BMC Microbiol. 19 (1), 130. doi: 10.1186/s12866-019-1494-7
Spanogiannopoulos, P., Bess, E. N., Carmody, R. N., Turnbaugh, P. J. (2016). The Microbial Pharmacists Within Us: A Metagenomic View of Xenobiotic Metabolism. Nat. Rev. Microbiol. 14 (5), 273–287. doi: 10.1038/nrmicro.2016.17
Su, B., Liu, H., Li, J., Sunli, Y., Liu, B., Liu, D., et al. (2015). Acarbose Treatment Affects the Serum Levels of Inflammatory Cytokines and the Gut Content of Bifidobacteria in Chinese Patients With Type 2 Diabetes Mellitus. J. Diabetes 7 (5), 729–739. doi: 10.1111/1753-0407.12232
Su, H., Mo, J., Ni, J., Ke, H., Bao, T., Xie, J., et al. (2020). Andrographolide Exerts Antihyperglycemic Effect Through Strengthening Intestinal Barrier Function and Increasing Microbial Composition of Akkermansia Muciniphila. Oxid. Med. Cell Longev. 2020, 6538930. doi: 10.1155/2020/6538930
Sun, L., Xie, C., Wang, G., Wu, Y., Wu, Q., Wang, X., et al. (2018). Gut Microbiota and Intestinal FXR Mediate the Clinical Benefits of Metformin. Nat. Med. 24 (12), 1919–1929. doi: 10.1038/s41591-018-0222-4
Tahrani, A. A., Barnett, A. H., Bailey, C. J. (2013). SGLT Inhibitors in Management of Diabetes. Lancet Diabetes Endocrinol. 1 (2), 140–151. doi: 10.1016/s2213-8587(13)70050-0
Tan, K., Tesar, C., Wilton, R., Jedrzejczak, R. P., Joachimiak, A. (2018). Interaction of Antidiabetic α-Glucosidase Inhibitors and Gut Bacteria α-Glucosidase. Protein Sci. 27 (8), 1498–1508. doi: 10.1002/pro.3444
Toejing, P., Khampithum, N., Sirilun, S., Chaiyasut, C., Lailerd, N. (2021). Influence of Lactobacillus Paracasei HII01 Supplementation on Glycemia and Inflammatory Biomarkers in Type 2 Diabetes: A Randomized Clinical Trial. Foods 10 (7), 1455. doi: 10.3390/foods10071455
Tolhurst, G., Heffron, H., Lam, Y. S., Parker, H. E., Habib, A. M., Diakogiannaki, E., et al. (2012). Short-Chain Fatty Acids Stimulate Glucagon-Like Peptide-1 Secretion via the G-Protein-Coupled Receptor FFAR2. Diabetes 61 (2), 364–371. doi: 10.2337/db11-1019
van Bommel, E. J. M., Herrema, H., Davids, M., Kramer, M. H. H., Nieuwdorp, M., van Raalte, D. H. (2020). Effects of 12-Week Treatment With Dapagliflozin and Gliclazide on Faecal Microbiome: Results of a Double-Blind Randomized Trial in Patients With Type 2 Diabetes. Diabetes Metab. 46 (2), 164–168. doi: 10.1016/j.diabet.2019.11.005
Vázquez-Baeza, Y., Callewaert, C., Debelius, J., Hyde, E., Marotz, C., Morton, J. T., et al. (2018). Impacts of the Human Gut Microbiome on Therapeutics. Annu. Rev. Pharmacol. Toxicol. 58, 253–270. doi: 10.1146/annurev-pharmtox-042017-031849
Vettorazzi, J. F., Ribeiro, R. A., Borck, P. C., Branco, R. C., Soriano, S., Merino, B., et al. (2016). The Bile Acid TUDCA Increases Glucose-Induced Insulin Secretion via the cAMP/PKA Pathway in Pancreatic Beta Cells. Metabolism 65 (3), 54–63. doi: 10.1016/j.metabol.2015.10.021
Wang, L., Li, P., Tang, Z., Yan, X., Feng, B. (2016). Structural Modulation of the Gut Microbiota and the Relationship With Body Weight: Compared Evaluation of Liraglutide and Saxagliptin Treatment. Sci. Rep. 6, 33251. doi: 10.1038/srep33251
Wang, Y., Tong, Q., Shou, J. W., Zhao, Z. X., Li, X. Y., Zhang, X. F., et al. (2017). Gut Microbiota-Mediated Personalized Treatment of Hyperlipidemia Using Berberine. Theranostics 7 (9), 2443–2451. doi: 10.7150/thno.18290
Wang, Y., Wu, Y., Sailike, J., Sun, X., Abuduwaili, N., Tuoliuhan, H., et al. (2020). Fourteen Composite Probiotics Alleviate Type 2 Diabetes Through Modulating Gut Microbiota and Modifying M1/M2 Phenotype Macrophage in Db/Db Mice. Pharmacol. Res. 161, 105150. doi: 10.1016/j.phrs.2020.105150
Wei, X., Tao, J., Xiao, S., Jiang, S., Shang, E., Zhu, Z., et al. (2018). Xiexin Tang Improves the Symptom of Type 2 Diabetic Rats by Modulation of the Gut Microbiota. Sci. Rep. 8 (1), 3685. doi: 10.1038/s41598-018-22094-2
Williams, R. E., Eyton-Jones, H. W., Farnworth, M. J., Gallagher, R., Provan, W. M. (2002). Effect of Intestinal Microflora on the Urinary Metabolic Profile of Rats: A (1)H-Nuclear Magnetic Resonance Spectroscopy Study. Xenobiotica 32 (9), 783–794. doi: 10.1080/00498250210143047
Woting, A., Blaut, M. (2016). The Intestinal Microbiota in Metabolic Disease. Nutrients 8 (4), 202. doi: 10.3390/nu8040202
Wu, G. D., Chen, J., Hoffmann, C., Bittinger, K., Chen, Y. Y., Keilbaugh, S. A., et al. (2011). Linking Long-Term Dietary Patterns With Gut Microbial Enterotypes. Science 334 (6052), 105–108. doi: 10.1126/science.1208344
Wu, H., Esteve, E., Tremaroli, V., Khan, M. T., Caesar, R., Mannerås-Holm, L., et al. (2017). Metformin Alters the Gut Microbiome of Individuals With Treatment-Naive Type 2 Diabetes, Contributing to the Therapeutic Effects of the Drug. Nat. Med. 23 (7), 850–858. doi: 10.1038/nm.4345
Xu, X., Gao, Z., Yang, F., Yang, Y., Chen, L., Han, L., et al. (2020). Antidiabetic Effects of Gegen Qinlian Decoction via the Gut Microbiota Are Attributable to Its Key Ingredient Berberine. Genomics Proteomics Bioinf. 18 (6), 721–736 doi: 10.1016/j.gpb.2019.09.007
Xu, T., Ge, Y., Du, H., Li, Q., Xu, X., Yi, H., et al. (2021). Berberis Kansuensis Extract Alleviates Type 2 Diabetes in Rats by Regulating Gut Microbiota Composition. J. Ethnopharmacol. 273, 113995. doi: 10.1016/j.jep.2021.113995
Xu, J., Lian, F., Zhao, L., Zhao, Y., Chen, X., Zhang, X., et al. (2015). Structural Modulation of Gut Microbiota During Alleviation of Type 2 Diabetes With a Chinese Herbal Formula. Isme J. 9 (3), 552–562. doi: 10.1038/ismej.2014.177
Yan, X., Feng, B., Li, P., Tang, Z., Wang, L. (2016). Microflora Disturbance During Progression of Glucose Intolerance and Effect of Sitagliptin: An Animal Study. J. Diabetes Res. 2016, 2093171. doi: 10.1155/2016/2093171
Yang, M., Shi, F. H., Liu, W., Zhang, M. C., Feng, R. L., Qian, C., et al. (2020). Dapagliflozin Modulates the Fecal Microbiota in a Type 2 Diabetic Rat Model. Front. Endocrinol. (Lausanne) 11. doi: 10.3389/fendo.2020.00635
Yao, Y., Chen, H., Yan, L., Wang, W., Wang, D. (2020). Berberine Alleviates Type 2 Diabetic Symptoms by Altering Gut Microbiota and Reducing Aromatic Amino Acids. BioMed. Pharmacother. 131, 110669. doi: 10.1016/j.biopha.2020.110669
Yuan, X., Ni, H., Chen, X., Feng, X., Wu, Q., Chen, J. (2018). Identification of Therapeutic Effect of Glucagon-Like Peptide 1 in the Treatment of STZ-Induced Diabetes Mellitus in Rats by Restoring the Balance of Intestinal Flora. J. Cell Biochem. 119 (12), 10067–10074. doi: 10.1002/jcb.27343
Yu, L., Zhou, X., Duan, H., Chen, Y., Cui, S., Guo, R., et al. (2021). Synergistic Protective Effects of Different Dietary Supplements Against Type 2 Diabetes via Regulating Gut Microbiota. J. Med. Food 24 (3), 319–330. doi: 10.1089/jmf.2020.4759
Zhang, X., Fang, Z., Zhang, C., Xia, H., Jie, Z., Han, X., et al. (2017). Effects of Acarbose on the Gut Microbiota of Prediabetic Patients: A Randomized, Double-Blind, Controlled Crossover Trial. Diabetes Ther. 8 (2), 293–307. doi: 10.1007/s13300-017-0226-y
Zhang, Y., Gu, Y., Ren, H., Wang, S., Zhong, H., Zhao, X., et al. (2020). Gut Microbiome-Related Effects of Berberine and Probiotics on Type 2 Diabetes (the PREMOTE Study). Nat. Commun. 11 (1), 5015. doi: 10.1038/s41467-020-18414-8
Zhang, P. P., Li, L. L., Han, X., Li, Q. W., Zhang, X. H., Liu, J. J., et al. (2020). Fecal Microbiota Transplantation Improves Metabolism and Gut Microbiome Composition in Db/Db Mice. Acta Pharmacol. Sin. 41 (5), 678–685. doi: 10.1038/s41401-019-0330-9
Zhang, F., Wang, M., Yang, J., Xu, Q., Liang, C., Chen, B., et al. (2019). Response of Gut Microbiota in Type 2 Diabetes to Hypoglycemic Agents. Endocrine 66 (3), 485–493. doi: 10.1007/s12020-019-02041-5
Zhang, Q., Xiao, X., Li, M., Yu, M., Ping, F., Zheng, J., et al. (2017). Vildagliptin Increases Butyrate-Producing Bacteria in the Gut of Diabetic Rats. PloS One 12 (10), e0184735. doi: 10.1371/journal.pone.0184735
Zhang, Q., Xiao, X., Zheng, J., Li, M., Yu, M., Ping, F., et al. (2018). Featured Article: Structure Moderation of Gut Microbiota in Liraglutide-Treated Diabetic Male Rats. Exp. Biol. Med. (Maywood) 243 (1), 34–44. doi: 10.1177/1535370217743765
Zhang, B., Yue, R., Chen, Y., Yang, M., Huang, X., Shui, J., et al. (2019). Gut Microbiota, a Potential New Target for Chinese Herbal Medicines in Treating Diabetes Mellitus. Evid. Based Complement. Alternat. Med. 2019, 2634898. doi: 10.1155/2019/2634898
Zhao, L., Chen, Y., Xia, F., Abudukerimu, B., Zhang, W., Guo, Y., et al. (2018). A Glucagon-Like Peptide-1 Receptor Agonist Lowers Weight by Modulating the Structure of Gut Microbiota. Front. Endocrinol. (Lausanne) 9. doi: 10.3389/fendo.2018.00233
Zheng, Y., Ding, Q., Zhang, L., Gou, X., Wei, Y., Li, M., et al. (2020a). The Effect of Traditional Chinese Medicine on Gut Microbiota in Adults With Type 2 Diabetes: A Protocol for Systematic Review and Meta-Analysis. Med. (Baltimore) 99 (38), e22233. doi: 10.1097/md.0000000000022233
Zheng, Y., Gou, X., Zhang, L., Gao, H., Wei, Y., Yu, X., et al. (2020b). Interactions Between Gut Microbiota, Host, and Herbal Medicines: A Review of New Insights Into the Pathogenesis and Treatment of Type 2 Diabetes. Front. Cell Infect. Microbiol. 10. doi: 10.3389/fcimb.2020.00360
Keywords: gut microbiota, antidiabetic drugs, type 2 diabetes mellitus, efficacy and safety, personalized therapeutic targets
Citation: Liu W, Luo Z, Zhou J and Sun B (2022) Gut Microbiota and Antidiabetic Drugs: Perspectives of Personalized Treatment in Type 2 Diabetes Mellitus. Front. Cell. Infect. Microbiol. 12:853771. doi: 10.3389/fcimb.2022.853771
Received: 13 January 2022; Accepted: 04 May 2022;
Published: 31 May 2022.
Edited by:
Tommi Vatanen, The University of Auckland, New ZealandReviewed by:
Jian Tan, The University of Sydney, AustraliaJunguk Hur, University of North Dakota, United States
Copyright © 2022 Liu, Luo, Zhou and Sun. This is an open-access article distributed under the terms of the Creative Commons Attribution License (CC BY). The use, distribution or reproduction in other forums is permitted, provided the original author(s) and the copyright owner(s) are credited and that the original publication in this journal is cited, in accordance with accepted academic practice. No use, distribution or reproduction is permitted which does not comply with these terms.
*Correspondence: Bao Sun, scy_csu2016@csu.edu.cn
†These authors have contributed equally to this work and share first authorship