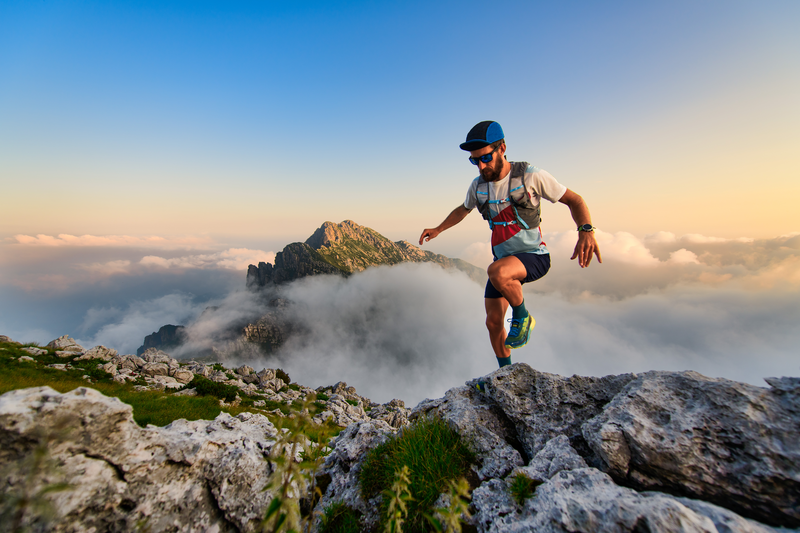
94% of researchers rate our articles as excellent or good
Learn more about the work of our research integrity team to safeguard the quality of each article we publish.
Find out more
ORIGINAL RESEARCH article
Front. Cell. Infect. Microbiol. , 20 June 2022
Sec. Parasite and Host
Volume 12 - 2022 | https://doi.org/10.3389/fcimb.2022.851903
This article is part of the Research Topic Insights in Parasite and Host: 2021 View all 9 articles
Parasites are important components of the immense n-dimensional trophic network that connects all living beings because they, among others, forge biodiversity and deeply influence ecological evolution and host behavior. In this sense, the influence of Trypanosomatidae remains unknown. The aim of this study was to determine trypanosomatid infection and richness in rats, opossums, and dogs in the semiarid Caatinga biome. We submitted DNA samples from trypanosomatids obtained through axenic cultures of the blood of these mammals to mini exon multiplex-PCR, Sanger, and next-generation sequencing targeting the 18S rDNA gene. Phylogenetic analyses were performed to identify genetic diversity in the Trypanosomatidae family. Shannon, Simpson, equability, and beta-diversity indices were calculated per location and per mammalian host. Dogs were surveyed for trypanosomatid infection through hemocultures and serological assays. The examined mammal species of this area of the Caatinga biome exhibited an enormous trypanosomatid species/genotypes richness. Ten denoised Operational Taxonomic Units (ZOTUs), including three species (Trypanosoma cruzi, Trypanosoma rangeli and Crithidia mellificae) and one Trypanosoma sp. five genotypes/lineages (T. cruzi DTU TcI, TcII, and TcIV; T. rangeli A and B) and four DTU TcI haplotypes (ZOTU1, ZOTU2, ZOTU5, and ZOTU10 merged), as well as 13 Amplicon Sequence Variants (ASVs), including five species (T. cruzi, T. rangeli, C. mellificae, Trypanosoma dionisii, and Trypanosoma lainsoni), five genotypes/lineages (same as the ZOTUs) and six DTU TcI haplotypes (ASV, ASV1, ASV2, ASV3, ASV5 and ASV13), were identified in single and mixed infections. We observed that trypanosomatids present a broad host spectrum given that species related to a single host are found in other mammals from different taxa. Concomitant infections between trypanosomatids and new host-parasite relationships have been reported, and this immense diversity in mammals raised questions, such as how this can influence the course of the infection in these animals and its transmissibility. Dogs demonstrated a high infection rate by T. cruzi as observed by positive serological results (92% in 2005 and 76% in 2007). The absence of positive parasitological tests confirmed their poor infectivity potential but their importance as sentinel hosts of T. cruzi transmission.
The Trypanosomatidae family is a group composed of flagellate protozoa that infect invertebrates, vertebrates, and plant hosts (Hoare, 1966; Vickerman, 1976). Although it is a well-studied group, new findings are continuously reported given that new parasite-host interactions and species/genotype diversity inside this family have been increasingly observed, especially after the advancement of molecular biology tools (d’Avila-Levy et al., 2016; Dario et al., 2017a; Maslov et al., 2019; Rangel et al., 2019; Rodrigues et al., 2019; Dario et al., 2021a).
The parasite species of this family are traditionally classified according to their developmental forms in their hosts during their life cycle (Hoare, 1966). Monoxenous trypanosomatids are those that evolved in only one host, namely, invertebrates. An example within this group is the parasite species from the genus Crithidia, which were first described to infect the Anopheles maculipennis mosquito (Léger, 1902) and are now recognized to infect bees, reduviids, and hoverflies (Langride and McGhee, 1967; Lipa and Triggiani, 1988; Yurchenko et al., 2014; Ishemgulova et al., 2017). Monoxenic trypanosomatids were classically termed “inferior trypanosomatids”, demonstrating how much a reductionist and anthropocentric view can bypass important biological phenomena even from the sanitary point of view.
Trypanosomatid species that target an invertebrate, a vertebrate, or a plant host are named heteroxenous. The focus of this article is the Trypanosoma genus, which comprises species that infect vertebrates. However, the transmission cycle is only known for a minority of species, such as Trypanosoma brucei (sleeping sickness in humans), Trypanosoma evansi (surra disease in animals), and Trypanosoma cruzi (Chagas disease in humans) (Podlipaev, 2001). This finding reflects that the main studies are focused on human or animal parasites of economic interest, forgetting the profound interdependence between the health of all living beings and their environment.
Caatinga is a unique biome in Brazil that is found mainly in the northeast region and a small area in the southeast, namely, north of Minas Gerais state. The dominant climate is semiarid, and the term Caatinga means white forest in the indigenous language (Silva et al., 2004; Souza et al., 2015). Caatinga is the third most degraded biome in Brazil (Myers et al., 2000), and approximately 80% of the vegetation is completely modified due to extractivism and agriculture. Most of these areas are currently in the early or intermediate stages of ecological succession (Araújo Filho, 1996). As a result of these profound changes, the Caatinga presents large extensions where desertification is already noted (Souza et al., 2015). This biome, which represented an important endemic area for Chagas disease (Sousa et al., 2020) exhibits a diverse and unique fauna, comprising approximately 1307 animal species (Instituto Chico Mendes de Conservação da Biodiversidade, 2018). Caatinga harbors most of the triatomine species diversity in Brazil together with the Cerrado (Gurgel-Gonçalves et al., 2012), and T. cruzi infection is observed in mammals from five different orders in this biome: Artiodactyla, Carnivora, Cingulata, Didelphimorphia, and Rodentia (Jansen et al., 2018).
The order Didelphimorphia is one of the oldest and most important hosts of Trypanosoma species, including T. cruzi (Jansen et al., 2018; Rodrigues et al., 2019; Jansen et al., 2020), having as its main representative marsupials of the genus Didelphis (Jansen et al., 2015). The genus Didelphis presents a broad range distribution in nature due to its ability to adapt to different ecological niches, including anthropized environments (Olifiers et al., 2015). Therefore, it is considered a biomarker of degraded environments. These animals are nomadic, solitary, take refuge in holes and tree foliage, and are excellent climbers (Jansen et al., 2018). They are able to use all forest strata and are therefore in contact with the different transmission cycles of T. cruzi (Jansen et al., 2020).
The Rodentia order is the most diverse order within the small wild mammal taxa, and these animals can be found in different environments, ranging from tropical forests to deserts, plateaus to plains, and wild to urban environments. In nature, they are able to circulate through different forest strata and are found in terrestrial, arboreal, and semiaquatic areas (Wilson and Reeder, 2005). In relation to T. cruzi transmission, some studies have reported T. cruzi infection in rodents in Latin America (Herrera et al., 2005; Yeo et al., 2005; Herrera et al., 2007b; Vaz et al., 2007; Roque et al., 2008; Ramsey et al., 2012; Charles et al., 2013; Orozco et al., 2014; Herrera et al., 2015; Jansen et al., 2015; Morales et al., 2017). Several species of this order are highly susceptible to experimental infection by T. cruzi, demonstrating high and long-lasting parasitemia (Roque et al., 2005). However, it was suggested that this taxon plays a secondary role as a reservoir in the wild environment (Jansen et al., 2018) because the infection rate of wild rodents by T. cruzi is low, potentially due to the restricted use of the environment (restricted home range), which leads to less contact with other infected mammals and vectors (Jansen et al., 2015).
The role of dogs in the transmission cycle of T. cruzi can vary according to each region. In Brazil, dogs are highly exposed to T. cruzi infection based on high rates of positive serological tests. However, the detection of the parasite in blood smears or parasite isolation by hemoculture and xenodiagnosis is rare (Roque and Jansen, 2008; Noireau et al., 2009; Xavier et al., 2012, Jansen et al., 2018). A distinct scenario was described in Argentina, especially in the Gran Chaco region, where dogs are one of the main T. cruzi reservoirs, as demonstrated by high transmissibility competence (Gürtler et al., 2007; Gürtler and Cardinal, 2015). In Brazilian regions that present triatomines and small wild mammals with high T. cruzi infection rates, dogs are usually also exposed to infection as observed by serological positive tests but primarily without positive parasitological tests to date. Therefore, monitoring these animals through parasitological and serological surveys is an indication of the presence of a parasite transmission cycle occurring among wild free-ranging mammals in the area (Roque et al., 2008; Roque and Jansen, 2008; Pineda et al., 2011; Xavier et al., 2012).
Mixed infections by trypanosomatids, mainly in wild mammals, are poorly understood and rarely studied in animals. However, these infections can provide important epidemiological data because coinfections can modify the course of infection of a parasitosis. As the one-parasite-one-disease concept generally predominates, the focus has always been on T. cruzi or Leishmania sp., and the possible coinfecting trypanosomatids have been relegated to the background. Mixed infections between different trypanosomatid species and genotypes are very common in nature (Barbosa et al., 2017; Dario et al., 2017a; Jansen et al., 2018; Patiño et al., 2021). The determination of mixed infections is essential for the understanding of parasitological events, especially the resulting interactions between the parasite and its hosts. The aim of this study was to determine the trypanosomatid infection and richness in the synantropic Rattus rattus, Didelphis albiventris, and dogs in the T. cruzi transmission cycle in this semiarid Caatinga biome region.
Jaguaruana municipality (4°50’02” S, 37°46’51” W) is in the Caatinga biome of Ceará state, northeast Brazil (Figure 1). According to the Brazilian Institute of Geography and Statistics (IBGE, 2010), Jaguaruana has an area of 867,251 km² and a total population of 32,239 inhabitants. DNA samples of the trypanosomatids isolated from hemocultures of R. rattus, D. albiventris, and triatomines (Rhodnius nasutus and Triatoma brasiliensis) were deposited in the DNA library of the Trypanosoma from wild and domestic mammals and vectors collection (COLTYP/Fiocruz). Forty-two deposited Trypanosoma spp. samples derived from R. rattus, D. albiventris, R. nasutus, and T. brasiliensis were submitted to DNA extraction (Table 1). The hemocultures were washed with phosphate-buffered saline solution, 100 µg/mL proteinase K (Invitrogen, Carlsbad, CA, USA), and 0.5% sodium dodecyl sulfate, and the samples were incubated at 56°C for 2 h. After this step, the DNA was extracted using the phenol–chloroform method (Sambrook and Russel, 2001). The trypanosomatid and DNA samples were obtained from opossums, rats, and triatomines collected in the following locations: Caatinguinha, Coberto, Córrego das Melancias, Figueiredo do Ivan, Figueiredo do Bruno, Figueiredo do Epifânio, Oiticica, Perímetro Irrigado, and Saquinho (Figure 1) in 2001, 2002, 2004, 2005, and 2006. These areas were considered periurban areas and were defined as transitional or surrounding regions where urban and rural activities overlapped and landscape characteristics were subject to rapid modifications due to anthropogenic activity (Lima et al., 2012).
Figure 1 Jaguaruana municipality map, Ceará state, northeast Brazil. The red area represents Ceará state, and the blue area represents Jaguaruana municipality. The red area represents Ceará state, and the blue area represents Jaguaruana municipality. Each dot represents locations where DNA samples were obtained. CE, Ceará state; PI, Piauí state; RN, Rio Grande do Norte state; PB, Paraíba state. Data sources: Instituto Brasileiro de Geografia e Estatística – IBGE (www.ibge.gov.br); Google Earth (https://www.google.com.br/intl/pt-BR/earth/). The map was constructed using QGIS software v.2.18.15. Unfortunately, the location of the two rats included in this study could not be identified.
Table 1 DNA sample origin and identification in Jaguaruana municipality, Ceará state, northeast Brazil.
To identify T. cruzi infection, the DNA samples were submitted to multiplex PCR to amplify the nontranscribed spacer of the miniexon gene (Fernandes et al., 2001) for the identification of TcI (DTU I – 200 bp), TcII (DTU II/V/VI – 250 bp), zymodeme 3 (DTU III/IV – 150 bp), and T. rangeli (100 bp) as well as mixed infections. All reactions included distilled water as a negative control. The following T. cruzi strains served as positive controls: TcI-SylvioX/10cl1; TcII-Esmeraldocl3; TcIII-M5631cl5; TcIV-92122102R; and TcV/VI-SC43cl1. One T. rangeli isolate (Choco) also served as a positive control. The PCR products were subjected to electrophoresis in a 2% agarose gel, which was stained with ethidium bromide solution and visualized under UV light. Restriction fragment length polymorphism (RFLP) analysis of the nuclear 1f8 gene and DNA digestion by the Alw21I enzyme were performed to distinguish DTUs TcII from TcV and TcVI (Rozas et al., 2007). The digested products were electrophoresed in a 3% agarose gel, stained with ethidium bromide solution, and visualized under ultraviolet light.
Additionally, the samples were submitted to nested-PCR for amplification of the small subunit (SSU) rDNA gene (650 bp) (Noyes et al., 1999; Smith et al., 2008). The amplified products were subjected to electrophoresis as described for mini-exon multiplex PCR and purified using the Illustra GFX PCR DNA and gel band purification kit (GE Healthcare Life Sciences, Little Chalfont, Buckinghamshire, UK). The forward and reverse DNA strands of samples were sequenced using BigDye Terminator v3.1 Cycle Sequencing Kit (Applied Biosystems, Foster City, CA, USA) on an ABI 3730 DNA sequencer available on the PDTIS/FIOCRUZ sequencing platform.
For NGS, DNA samples were amplified using the primers S825F (5’-ACCGTTTCGGCTTTTGTTGG-3’) and S662R (5’-GACTACAATGGTCTCTAATC-3’) (Maslov et al., 1996; Barbosa et al., 2017) of the 18S rDNA gene for partial Trypanosomatidae gene sequences. These primers contained Illumina MiSeq adapter sequences. For the polymerase chain reaction (PCR), the 2X Phusion Flash High Fidelity Master Mix (Thermo Fisher, Waltham, MA, USA) was used based on the following reaction parameters: 10 µl of the master mix, 0.8 μM of each primer, and 2 to 5 µl of DNA in a final volume of 20 µl. The DNA amplification was adapted from the cycling recommended by the manufacturer as follows: an initial denaturation step at 98°C for 10 s; 30 cycles of denaturation at 98°C for 1 s, annealing at 55°C for 10 s, and extension at 72°C for 15 s; and a final extension step at 72°C for 1 min. The amplified products were subjected to electrophoresis in a 2% agarose gel containing SYBR Safe Gel Stain (Invitrogen, Waltham, MA, USA) and visualized under ultraviolet light to observe the band of interest (~350 base pairs). The amplified samples were purified (Illustra GFX PCR DNA and gel band purification kit, GE Healthcare Life Sciences, Little Chalfont, Buckinghamshire, UK) and quantified by fluorimetry (Qubit 2.0, Life Technologies, Carlsbad, CA, USA).
For the DNA library preparation, a second PCR was performed to introduce Illumina indices (Nextera XT Index Kit v2, Illumina, San Diego, CA, USA). The 2X Phusion Flash High Fidelity Master Mix (Thermo Fisher, Waltham, MA, USA) was also used, and 100 ng of each amplified product was applied. The PCR cycling in this step was as follows: an initial denaturation step at 98°C for 10 s; 8 cycles of denaturation at 98°C for 1 s, annealing at 55°C for 10 s and extension at 72°C for 15 s; and a final extension step at 72°C for 1 min. After PCR, samples were purified using magnetic beads (Ampure Beads Beckman Coulter) and quantified (Qubit 2.0, Life Technologies, Carlsbad, CA, USA). The samples were pooled to equimolar concentrations, and sequencing was performed on a MiSeq sequencer (Illumina, San Diego, CA, USA) using the MiSeq Reagent v2 kit (500 cycles) (2 x 250 paired-end reads).
SSU rDNA analysis: To obtain the consensus sequences, each forward and reverse sample was assembled and edited using the SeqMan program (DNASTAR Lasergene). The obtained sequences were aligned and corrected using MegaX software (Kumar et al., 2018). The sequences were compared with nucleotide sequences deposited in GenBank using the BLAST (Basic Local Alignment Search Tool – https://blast.ncbi.nlm.nih.gov/Blast.cgi - algorithm for trypanosomatid species identification).
Denoised Operational Taxonomic Unit (ZOTU) NGS analysis: To obtain reads with base calling quality greater than or equal to 99.9%, the reads generated were filtered and edited using the Sickle program (Joshi and Fass, 2011). The quality-selected reads were mapped against a trypanosomatid sequence database built from 18S rDNA gene sequences (Supplementary Material S1) available in the SILVA v132 database project (Quast et al., 2013) using the Bowtie 2 program (Langmead and Salzberg, 2012). To obtain a consensus read, forward and reverse reads were overlapped using the Flash 1.2.11 program (Magoč and Salzberg, 2011). The reads for which the forward sequence did not overlap with the reverse sequence were submitted to the fastq_mergepairs command available at USEARCH v11 (drive5.com/usearch/). For ZOTU identification, unique reads were identified using the command dereplication in VSEARCH 2.17.1 (Rognes et al., 2016). The UNOISED (Edgar and Flyvbjerg, 2015; Edgar 2016) algorithm was used to cluster the correct biological sequences in the reads, and an OTU table was constructed using USEARCH v11 (drive5.com/usearch/). The identification of trypanosomatid species and their genotypes was initially determined using the Basic Local Alignment Search Tool (BLAST) algorithm available on the National Center for Biotechnology Information (NCBI) website. As a read cutoff for determining the species/genotype occurrence per sample, the total reads per sample obtained in the ZOTU table were normalized to 100,000 reads, and ZOTUs that presented ≤ 150 reads in the sample were excluded from the analysis.
Amplicon Sequence Variant NGS analysis: The NGS-generated data were imported into the R v3.6.2 environment, wherein all the analyses were performed (R Development Core, 2021). Sequences were analyzed using the DADA2 package v1.14.0 following the analysis pipeline as given in the tutorial (https://benjjneb.github.io/dada2/tutorial.html) (Callahan et al., 2016). Furthermore, the same sequence database used for the ZOTU analysis was used in the ASV analysis (Supplementary Material S1). The ASV table and assigned taxonomy and sample metadata information were combined as a phyloseq object (phyloseq package version 1.30.0) (McMurdie & Holmes, 2013).
The ZOTU and ASV reads were aligned to other trypanosomatid 18S rDNA retrieved from the GenBank database using the L-INS-i algorithm in MAFFT v.7.0 (Katoh, 2013). The alignment was visualized and manually edited on MegaX (Kumar et al., 2018). Maximum likelihood (ML) estimation and Bayesian inference (BI) were performed for trypanosomatid species/genotype identification and genetic clustering. For each phylogenetic analysis, the best base substitution models were chosen according to the corrected Akaike information criterion (cAIC) in ModelFinder (Kalyaanamoorthy et al., 2017). ML reconstruction was performed in the IQ-Tree program (Nguyen et al., 2015) on PhyloSuite v.1.2.2 (Zhang et al., 2020). Ultrafast bootstrapping (Hoang et al., 2018) was performed with 5000 replicates with 1000 maximum interactions and 0.99 minimum correlation coefficients for branch support. To validate this result, the SH-aLRT branch test with 5000 replicates was also applied. Bayesian tree reconstruction was performed in Bayesian Evolutionary Analysis Sampling Trees (BEAST) v2.6.2 (Bouckaert et al., 2019) using the Bayesian Markov chain Monte Carlo (MCMC) method to assign trypanosomatid species/genotypes prior to information. The birth-death model specification was used in genotype tree reconstruction, and the Yule model specification was used for species tree reconstruction. Three independent runs were performed for 20 M with sampling every 2000 generations. The runs converged and the effective sample size (ESS) were calculated after 25% of each run was excluded (burn-in) from each run in TRACER v.1.6 (Rambaut et al., 2018). Parameters greater than 500 were considered appropriate. The final tree was generated with maximum clade credibility (MCC) based on 16878 trees (burn-in = 5625) and a 0.6 posterior probability limit (PP) in Tree Annotator. The ML and BI reconstruction trees were visualized in Figtree v.1.4.3.
In addition, haplotype networks based on T. cruzi DTU TcI samples were generated in Network software version 5.0.1.1 (fluxus-engineering.com) to define evolutionary relationships and to observe the intraspecificity among these DTUs. The network was built using median-joining (Bandelt et al., 1999) and maximum parsimony (Polzin and Daneschmand, 2003) postprocessed clean-up procedures.
Trypanosoma cruzi DTU TcI haplotype diversity was calculated using the Shannon, Simpson, evenness, and beta-diversity indices per location and per mammalian host. All statistical analyses were performed in R version 4.0.3 (vegan and betapart packages).
DNA samples were obtained from wild mammals and dogs in the surrounding areas and were investigated for T. cruzi infection using parasitological and serological methods. Blood samples were collected using Vacutainer® tubes containing EDTA by puncturing the dog’s brachial vein with the informed consent of their owners. A questionnaire was used to record the name, age, sex, size, primary function (hunting, companionship, or protection), and anatomical peculiarities. The dogs were investigated in 2004 and 2005 in a previous study (Lima et al., 2012), and another investigation was performed in 2007 by our group.
Blood (0.3–0.6 µL) was seeded in two tubes containing Novy-Mc Neal-Nicole medium (NNN) overlaid with 2 mL liver infusion tryptose medium (LIT). Fresh blood smears were examined by optical microscopy. The remaining blood was centrifuged, and the serum was stored at −20°C. Hemocultures were examined every 2 weeks for 5 months, and the positive samples were amplified for molecular characterization and cryopreservation. The amplified samples were deposited in the Coleção de Trypanosoma de Mamíferos Silvestres, Domésticos e Vetores (COLTRYP/Fiocruz).
A serological survey for the detection of anti-T. cruzi antibodies was performed using an indirect immunofluorescence antibody test (IFAT) to evaluate infection in wild mammals and dogs (Camargo, 1966). The sera were tested with anti-dog IgG conjugate (FICT, Sigma, St. Louis, MO, USA), and a cutoff value of 1:40 was adopted. To avoid misinterpretation due to cross reactions and detect mixed infections, the dogs were also screened for Leishmania infection using an IFI-Leishmania Bio-Manguinhos Kit (Fiocruz). Dogs with 2x higher serologic titers for Leishmania than T. cruzi were not considered infected by T. cruzi. When both titers were similar and greater than the cutoff, mixed infection by both parasites was considered (Roque et al., 2008).
Rats and opossums were demonstrated to maintain an impressive T. cruzi diversity, in addition to T. rangeli infection (Table 2). The results from 42 DNA samples that were submitted to mini-exon multiplex PCR (Table 2) showed that the majority (31) were T. cruzi TcI samples (26 D. albiventris and nine R. rattus). Moreover, one D. albiventris sample presented mixed infection by TcI and T. rangeli. Three samples derived from one D. albiventris specimen and two R. rattus specimens were identified as T. cruzi DTU TcII. One R. nasutus and the T. brasiliensis specimens presented infection by T. cruzi DTU TcI. Moreover, mixed infection by T. cruzi DTU TcI and T. rangeli was reported in the other R. nasutus specimen. SSU rDNA nested-PCR was performed in 42 DNA samples from rats (n=11), opossums (n=28), and triatomines (n=3). The results yielded electropherograms presenting double peaks, making it impossible to create consensus sequences and indicating mixed infections. Thirty-nine DNA samples (27 samples from D. albiventris, 11 from R. rattus, two from R. nasutus, and one from T. brasiliensis) were submitted to NGS for trypanosomatid species identification.
Table 2 Trypanosomatid molecular characterization of Rattus rattus, Didelphis albiventris, and triatomines by mini-exon multiplex-PCR, 18S rDNA Sanger sequencing, and next-generation sequencing.
Of the 35 samples (24 from D. albiventris, eight from R. rattus, two from R. nasutus, and one from T. brasiliensis) that were sequenced (Table 2), 10 ZOTUs were assigned to T. cruzi DTU TcI (ZOTU1, ZOTU2, ZOTU5, ZOTU10 merged); DTU TcII (ZOTU 10); DTU TcIV (ZOTU4); T. rangeli lineages A and B included ZOTU7 and ZOTU11, respectively; Trypanosoma sp. (ZOTU12) and C. mellificae (ZOTU3) (Figures 2 and 3). Eleven samples (31, 43%) presented single infection exclusively by T. cruzi TcI ZOTU1, and 24 samples (68.57%) presented mixed infection from two to five ZOTUS. ZOTU1 was predominant and was present in all samples. The DTU TcI was found in all mammalian hosts and vectors. DTU TcII was found in D. albiventris, and DTU TcIV was found in T. brasiliensis (Table 2). The sequence identified as Trypanosoma sp. (ZOTU12) found in mammals grouped together with sequences from the subgenus Schizotrypanum species (T. cruzi, T. c. marinkellei, T. dionisii, and T. erneyi) in a more basal branch (Figure 2). Crithidia mellificae was identified in two R. rattus specimen (Table 2). Trypanosoma rangeli was detected in both R. rattus and D. albiventris (Table 2).
Figure 2 Trypanosoma cruzi clade phylogenetic tree based on 465bp 18S rDNA fragment length from D. albiventris, R. rattus, R. nasutus, and T. brasiliensis culture samples. The tree was inferred with TPM3uf plus gamma distribution among sites (TPM3uf+G) for ML and BI. The number at nodes corresponds to ML (ultrabootstrap and SH-aLRT) and BI (posterior probability). The scale bar shows the number of nucleotide substitutions per site. The red bracket indicates the group formed by T. cruzi DTU TcI; the lilac bracket indicates the sequences grouped as T. cruzi DTU TcIV; the pink bracket indicates the sequences identified as T. cruzi DTU TcII; the blue bracket indicates the sequences identified as T. rangeli lineage A; and the yellow bracket indicates the sequences identified as T. rangeli lineage B.
Figure 3 Crithidia mellificae phylogenetic tree based on 333bp 18S rDNA fragment length from R. rattus hemoculture sample. The tree was inferred with Bayesian using Tamura-Nei with equal frequencies (TrNef) model for ML and BI. The number at nodes corresponds to ML (ultrabootstrap and SH-aLRT) and BI (posterior probability). The scale bar shows the number of nucleotide substitutions per site. The green square indicates the group formed by C. mellificae sequences from different hosts.
Thirteen ASVs were assigned to T. cruzi DTU TcI (ASV, ASV1, ASV2, ASV3, ASV5, ASV13), DTU TcII (ASV10), DTU TcIV (ASV4), T. rangeli lineages A (ASV7) and B (ASV11), T. dionisii (ASV9) (Figure 2), T. lainsoni (ASV14) (Figure 4) and C. mellificae (ASV15) (Figure 3). Thirteen samples (37, 14%) presented a single infection by T. cruzi TcI ASV1 (n=11) and TcI ASV2 (n=2), and 22 samples (62.85%) presented a mixed infection from two to four ASVs (Table 2). ASV1 was predominant, but seven samples (Table 2) of this amplicon sequence variant were not identified. Both D. albiventris and R. rattus presented infection by DTUs TcII and TcIV, T. rangeli A and B, C. mellificae, T. dionisii, and T. lainsoni (Table 2).
Figure 4 Trypanosoma lainsoni phylogenetic tree based on 492bp 18S rDNA fragment length from D. albiventris and R. rattus hemoculture samples. The tree was inferred with Bayesian using transition model with plus gamma distribution among sites (TIM3+G) model for ML and BI. The number at nodes corresponds to ML (ultrabootstrap and SH-aLRT) and BI (posterior probability). The scale bar shows the number of nucleotide substitutions per site.
We also observed a T. cruzi DTU TcI intraspecific diversity. According to the haplotype network, four ZOTU and six ASV TcI haplotypes were observed to infect D. albiventris, R. rattus, R. nasutus, and T. brasiliensis (Figure 5). In the analysis, we observed that three ZOTUs (ZOTU1, ZOTU2, and ZOTU5) and ASVs (ASV1, ASV2, and ASV5) were the same haplotypes. We also observed five new TcI haplotypes: ZOTU5-ASV5, ZOTU10 merged, ASV, ASV3, and ASV13 (Figure 5). ASV, ASV3, and ASV13 were exclusively detected in D. albiventris (Figure 5B).
Figure 5 Trypanosoma cruzi DTU TcI haplotype network from D. albiventris, R. rattus, R. nasutus and T. brasiliensis culture samples. Networks were constructed with 18S rDNA (A) The size of each blue node is proportional to the haplotype frequency. (B) ASV haplotype per mammal and triatomine hosts. (C) ZOTU haplotype distribution per mammal and triatomine hosts. The small black circle represents the median vector, which can be interpreted as an unsampled sequence or an extinct ancestral sequence.
According to the ZOTU-ASV distribution map (Figure 6), T. cruzi DTU TcI occurs in all the locations studied. In the ZOTU distribution, Caatinguinha presented the greatest diversity of T. cruzi DTU TcI, where the four haplotypes were observed and C. mellificae was identified. Trypanosoma rangeli and Trypanosoma sp. occurred exclusively in Saquinho and Figueiredo do Bruno; Córrego das Melancias, Coberto and Oiticica were the locations where DTU TcII and TcIV were identified, respectively (Figure 6A). Caatinginha presented the greatest trypanosomatid species distribution according to the ASV map followed by Córrego das Melancias (Figure 6B).
Figure 6 Trypanosomatid distribution map in opossum, rats, and triatomines in Jaguaruana municipality, Ceará state, Brazil. Each color represents a ZOTU (A) and ASV (B) identified. According to the ZOTU map (A), the Caatinguinha location exhibited the greatest occurrence of T. cruzi DTU TcI; Figueiredo do Bruno and Saquinho presented greater Trypanosoma spp. diversity. In the ASV map (B), Caatinguinha and Córrego das Melancias presented the greatest trypanosomatid diversity. Data sources: Instituto Brasileiro de Geografia e Estatística – IBGE (www.ibge.gov.br); Google Earth (https://www.google.com.br/intl/pt-BR/earth/). The map was constructed using QGIS software v.2.18.15.
For the ZOTU analysis of the T. cruzi DTU TcI, the Caatinguinha location showed the greatest diversity of T. cruzi TcI haplotypes. Rattus rattus and D. albiventris TcI infection displayed the same diversity – the four DTU TcI haplotype occurrence, however, in R. rattus, TcI presented a greater distribution in mixed infection. In the ASV, D. albiventris presented a greater TcI distribution, and R. rattus presented a greater trypanosomatid diversity. The Caatinguinha location presented the greatest trypanosomatid diversity, followed by Córrego das Melancias.
The dogs examined in 2004 and 2005 as well as those examined in 2007 (n= 195) at Matinho, Dió, Saquinho, Córrego das Melancias, Figueiredo do Bruno, Figueiredo do Ivan, Caatinguinha, and Perímetro Irrigado displayed one common trait: none of the animals presented positive results for fresh blood and hemoculture exams. In total, 86/195 (44.1%) of the dogs presented serological titers for T. cruzi (Table 3). Analyzing the results per year and locations (Table 3), 2005 and 2007 presented higher T. cruzi infection rates in dogs.
Table 3 Trypanosoma cruzi infection of dogs demonstrated by serological exams from Jaguaruana municipality, Ceará state, Brazil.
In this study, three findings deserve attention: 1) the richness of Trypanosoma spp. found in D. albiventris but also in R. rattus in a rural area, therefore not representative of the original Caatinga landscape; 2) the first finding of C. mellificae infection in R. rattus; and 3) the richness of uncharacterized genotypes of T. cruzi. The aim of this study was to characterize trypanosomatid richness in D. albiventris and R. rattus and T. cruzi infection in dogs from a locality of the Caatinga biome that years ago was endemic for Chagas disease. Currently, no cases of children under 10 years of age have been reported (Lima et al., 2012 and unpublished results 2007), showing the results of the successful South cone initiative along with popular awareness of the disease and a certain improvement in residences.
We used the mini-exon target as a first attempt to characterize the isolates from D. albiventris and R. rattus. This technique showed that most isolates were infected by T. cruzi DTU TcI in a single infection except for one isolate that presented mixed infection with T. rangeli. Three isolates of R. rattus were demonstrated to be TcII by RFLP-PCR. The mini-exon target is a good molecular target to characterize T. cruzi DTU TcI. However, as we deal with sylvatic environment samples, which often present mixed infections, the diagnosis of the infection is unclear. Therefore, we tried to characterize the samples using SSU rDNA through Sanger sequencing, which also failed because the samples presented a double peak in the electropherogram, confirming the probably mixed infections. This is a limitation of the methodology given that it is only possible to identify them by combining Sanger sequencing with the molecular cloning methodology for the diagnosis of mixed infection, which makes the procedure considerably more laborious (Zepeda Mendoza et al., 2015; Huggins et al., 2019).
Hence, we performed NGS, which allowed us to detect 15 trypanosomatid species/genotypes infecting R. rattus, D. albiventris, and triatomines in single and mixed infections. NGS allowed the identification of a broad range of samples simultaneously, in addition to identifying the possible parasite species circulating in a given sample, even using culture isolate samples, which have gone through parasite species selection process, which could affect the results observed. Some studies have already used this methodology to identify trypanosomatids in samples from different mammal species, and it was possible to identify even more than three species in a single sample and even species of the Kinetoplastea class infecting mammals (Barbosa et al., 2017; Dario et al., 2017a; Dario et al., 2017b; Cooper et al., 2018; Huggins et al., 2019; Patiño et al., 2021). This is a promising methodology in parasitological studies that is able to unravel a world that we did not have access to before due to diagnostic limitations. This method will offer new knowledge, such as new species and genotypes and their phylogenetical relations. Here, we observed a trypanosomatid sequence that phylogenetically grouped together with sequences from the T. cruzi clade as well as a previously undescribed host-parasite interaction, namely, R. rattus infected by C. mellificae. In addition, according to Austen and Barbosa (2021), mixed infection detection in a host and the understanding of multihost parasite dynamics are priorities from now on, and detection of this type of infection is only possible through NGS.
Rattus rattus is a synanthropic species that frequents human living areas. They are considered to be of economic and sanitary importance, as they cause damage to food stocks and are associated with the transmission of several zoonoses that are very general in their diet, and they present a great climbing ability. Rodents are usually suggested to play a secondary role as reservoirs of T. cruzi in the sylvatic environment (Rademaker et al., 2009; Jansen et al., 2018). However, this notion was not observed in this Caatinga region. Animals from this order can be involved in the T. cruzi transmission cycle in different ways: a) rodents can share some of their microhabitats with triatomines of the genera Triatoma and Panstrongylus (Carcavallo et al., 1998); b) as they are one of the main predation targets, rodents are important in the study of the transmission cycle of T. cruzi; and c) some rodent species are used in the peridomestic environment and thus may act as links between the transmission cycles (Roque et al., 2008). This work demonstrates a richness of trypanosomatids that was completely unknown in R. rattus and raises the question - how are these parasites that are in such low parasitemias transmitted?
Didelphis albiventris, similar to the other species of the genus, is considered a sylvatic taxon characterized by its high competence in adapting to artificial habitats. In fact, opossums are extremely adaptable to both the environment and diet. In the forest, opossums are also skilled tree climbers, although they are somewhat clumsy on the ground. They prey on eggs and small vertebrates as well as insects. The interaction of opossums with humans is quite old. These animals use human buildings as shelters, whereas humans often use opossums as a source of protein. Marsupials from the Didelphis genus are the main reservoir of T. cruzi in different biomes, as demonstrated by high rates of positive hemocultures (Jansen et al., 2018). These animals present generalist habits, use the different forest strata and easily adapt to modified environments, especially the peridomestic environment (Jansen et al., 2018). This set of events explains why these animals always have high rates of infection by T. cruzi, and this would not be different in the Caatinga.
According to our results, the T. cruzi transmission cycle has different profiles in nature, and the animals involved in transmission in this region of the Caatinga biome include D. albiventris and R. rattus species. Both studied species, but mainly Didelphis spp., are considered important reservoirs of T. cruzi (Jansen et al., 2015; Jansen et al., 2018). Moreover, in the studied localities, we observed these mammal species display subpatent parasitemias by an impressive richness by T. cruzi genotypes in the absence of new human cases, showing how powerful the adoption of easy prophylactic measures, such as improving the residences, may be effective against Chagas disease.
The DTUs TcI, TcII, and TcIV were previously described as circulating in small wild mammals with the latter noted in triatomines in the Caatinga biome. DTU TcI is the most common genotype found in T. cruzi (Zingales et al., 2012; Jansen et al., 2015; Brenière et al., 2016). DTU TcII, which was formerly associated with human disease, was the second most frequent in wild mammals, and similar findings were noted in the studied locality (Lisboa et al., 2015; Dario et al., 2017b). This is the first report of the DTU TcIV infecting triatomine in the Caatinga biome. This finding, which was previously observed only in triatomines of the Atlantic Forest (Dario et al., 2016; Dario et al., 2017b; Dario et al., 2018), demonstrates that this DTU can infect a variety of triatomine species and landscapes. This finding shows that no triatomine species is correlated with a certain DTU.
The DTU TcI is a genotype that presents an intraspecific genetic diversity diagnosed by several molecular markers (Salazar et al., 2006; Herrera et al., 2007a; O’Connor et al., 2007; Spotorno et al., 2008; Falla et al., 2009; Ramirez et al., 2011; Roman et al., 2018). Several subdivisions of TcI have been proposed. In Colombia, this genotype is subdivided into two groups through nuclear targets and multilocus sequence typing: one associated with wild transmission (TcISILV) and the other with domestic transmission cycles (TcIDOM) (Ramirez et al., 2011). Here, through NGS, we were able to detect this intraspecific diversity reported in TcI, as we have observed seven different haplotypes circulating in small wild mammals and triatomines, including five new haplotypes that seem to be observed exclusively in the Caatinga biome. Roman et al. (2018) reported a set of TcI isolates from the Caatinga biome that grouped together with no other sequence from a different biome, which could indicate a geographical association. To this end, we should use the same methodology to affirm this association.
We observed different trypanosomatid distributions in Jaguaruana municipality. The Caatinguinha location was the area with the greatest diversity of TcI haplotypes and can be considered a hotspot area for this genotype. The Saquinho, Figueiredo do Bruno, and Córrego das Melancias locations presented the greatest trypanosomatid richness, and some T. cruzi DTUs were reported only in specific locations. Even though we are dealing with a single biome, we can affirm that microhabitats may influence parasite occurrence and diversity as observed in this region.
This is the second study employing NGS involving mammals and trypanosomatid infection in Brazil. The first was performed in the Atlantic Forest and investigated trypanosomatid infection in bats (Dario et al., 2017a). Here, we observed trypanosomatid species richness circulating in R. rattus and D. albiventris, i.e., nonflying mammals. Both studies revealed an unsusceptible richness of trypanosomatids in these distinct taxa, demonstrating that this diversity is common. Our findings reinforce the marsupial as a bioaccumulator of DTU TcI and demonstrate R. rattus as an important host of this genotype in this region. An interesting point to highlight is how this DTU diversity was able to maintain itself among these animals despite the low parasitemias. One explanation is that the triatomines presented the same haplotypes observed in the mammals, so they can be responsible for the transmission by being predated by the animals.
Trypanosoma rangeli lineage A and B infections were observed in D. albiventris and R. rattus. This is the first report of T. rangeli lineage B in the Caatinga, demonstrating that this lineage, together with lineage A (Dario et al., 2021b) presents a broad distribution in Brazil. Didelphis albiventris infection by T. rangeli lineage A was previously described (Dario et al., 2021b). We are adding one more rodent species infected by T. rangeli in Brazil, R. rattus. This new finding demonstrates that rodents with different ecological habits are capable of being infected with this species. Trypanosoma dionisii gains another mammalian host (R. rattus), proving to be a generalist species. Trypanosoma lainsoni, a species described in rodents (Naiff and Barrett, 2013), was observed in D. albiventris for the first time in the Caatinga biome; the first report on this species was in the Cerrado (Nantes et al., 2021), expanding its distribution spectrum. Here, we also report for the first time R. rattus infected by C. mellificae, a so-called monoxenous trypanosomatid. This species is increasingly dispersed among mammals in nature (Rangel et al., 2019; Alves et al., 2021; Dario et al., 2021a). This finding supports the likelihood that C. mellificae has a very eclectic vector or different vectors that make this species so widespread in Brazilian biomes, even in environments with a certain degree of degradation, as the infection was reported in a synanthropic animal.
Dogs presented high serological rates for T. cruzi infection in 2005 (Lima et al., 2012) and 2007 in the present study, but no parasitological exam yielded positive results. It is interesting that in Caatinguinha and Córrego das Melancias, locations where T. cruzi infection was detected in R. rattus and D. albiventris, the infection by T. cruzi was also reflected in domestic dogs, as most were infected. This observation confirmed the role of dogs’ sentinel host in the T. cruzi transmission cycle (Xavier et al., 2012), indicating the presence of the wild transmission cycle in the surroundings of the domestic environment.
In addition, the finding of so many ZOTUs/ASVs demonstrated that the Trypanosoma genus and within it, the T. cruzi clade, still offer unanswered questions. We highlight the following important questions here: What will be the impact of these mixed infections on the host in terms of their biological condition and infective potential? Are these ZOTUs/ASVs able to maintain themselves in the memory host or just in the vectors or the opposite? The same questions apply to C. mellificae, a monoxenous trypanosomatid that is increasingly found in subpatent infections in a broad spectrum of mammals. In conclusion, we can affirm that the Caatinga biome demonstrates remarkable trypanosomatid species/genotypes and haplotype richness. Two species are involved in T. cruzi enzootic transmission, D. albiventris and R. rattus, demonstrating that each animal has a different role in the transmission cycle (varies in different places and times) and therefore the importance of its investigation. The role of the dog in signaling the presence of T. cruzi was reinforced. Once again, there is much more to be unraveled than knowledge about trypanosomatids in nature given that new interactions and dispersions are observed. Thus, we are discovering a new world based on these findings.
The datasets presented in this study can be found in online repositories. The name of the repository and accession numbers can be found below: SRA, NCBI; PRJNA382386: SSR17675332 to SSR17675366.
The animal study was reviewed and approved by Ethical Committee for Animal Use of the Oswaldo Cruz Foundation (P0179-03).
AJ, MD, SX, and CF contributed to the conception and design of the study. CL and SX organized the database. MD, CF, FS, CL, FO, and SX performed the research and analyses. MD and AJ wrote the first draft of the manuscript. All authors contributed to manuscript revision and read and approved the submitted version.
This study was funded by the Fundação Oswaldo Cruz, Instituto Nacional de Câncer, Conselho Nacional de Desenvolvimento Científico e Tecnológico (CNPq), Coordenação de Aperfeiçoamento de Pessoal de Nível Superior (CAPES) and Fundaçao de Amparo a Pesquisa do Estado do Rio de Janeiro (Faperj). MD receives a postdoctoral fellow from Faperj (E-26/202.414/2019). FO receives a master’s grant from CAPES. FMS receives a postdoctoral fellow from CAPES (88887.162877/2018-00). AR is financially supported by CNPq/Universal (425293/2018-1) and Jovem Cientistas do Nosso Estado/Faperj (E-26/202.794/2019). SX received financial support from CNPq/Universal (422489/2018-2). AJ is financially supported by CNPq (Bolsista de Produtividade, nível 1A).
The authors declare that the research was conducted in the absence of any commercial or financial relationships that could be construed as a potential conflict of interest.
All claims expressed in this article are solely those of the authors and do not necessarily represent those of their affiliated organizations, or those of the publisher, the editors and the reviewers. Any product that may be evaluated in this article, or claim that may be made by its manufacturer, is not guaranteed or endorsed by the publisher.
We would like to thank Dr. Daniel Mattos and Thalita Basso Scandolara from the Laboratório de Genética (Instituto Nacional de Câncer – INCA) for the technical support on the NGS methodology; the Laboratório de Genética from INCA that sequenced our DNA libraries; the PDTIS/Fiocruz sequencing platform for sequence our samples through Sanger methodology; and Carlos Ardé and Marcos Antônio dos Santos Lima (Laboratório de Biologia de Tripanosomatídeos IOC/Fiocruz) for technical support in the hemocultures. A special thanks to Alexandre Werneck, who helped with the NGS analysis.
The Supplementary Material for this article can be found online at: https://www.frontiersin.org/articles/10.3389/fcimb.2022.851903/full#supplementary-material
Supplementary Table 1 | Kinetoplastid 18S rDNA sequence database.
Alves, F. M., Rangel, D. A., Vilar, E. M., Pavan, M. G., Moratelli, R., Roque, A. L. R., et al. (2021) Trypanosoma spp. Neobats: Insights About Those Poorly Known Trypanosomatids. Parasites Wildlife. 16, 145–152. doi: 10.1016/j.ijppaw.2021.09.003
Araújo Filho, J. A. (1996). Desenvolvimento Sustentável Da Caatinga (Sobral (CE: Ministério da Agricultura/EMBRAPA/CNPC), 45 p.
Austen, J. M., Barbosa, A. D. (2021). Diversity and Epidemiology of Bat Trypanosomes: A One Health Perspective. Pathog. 10, 1148. doi: 10.3390/pathogens10091148
Bandelt, H. J., Forster, P., Röhl, A. (1999). Median-Joining Networks for Inferring Intraspecific Phylogenies. Mol. Biol. Evol. 16, 37–48. doi: 10.1093/oxfordjournals.molbev.a026036
Barbosa, A. D., Gofton, A. W., Paparini, A., Codello, A., Greay, T., Gillett, A., et al. (2017). Increased Genetic Diversity and Prevalence of Co-Infection With Trypanosoma Spp. In Koalas (Phascolarctos Cinereus) and Their Ticks Identified Using Next-Generation Sequencing (NGS). PloS One 12 (7), e0181279. doi: 10.1371/journal.pone.0181279
Bouckaert, R., Vaughan, T. G., Barido-Sottani, J., Duchêne, S., Fourment, M., Gavryushkina, A., et al. (2019). BEAST 2.5: An Advanced Software Platform for Bayesian Evolutionary Analysis. PloS Comput. Biol. 15, e1006650. doi: 10.1371/journal.pcbi.1006650
Brenière, S. F., Waleckx, E., Barnabé, C. (2016). Over Six Thousand Trypanosoma cruzi Strains Classified Into Discrete Typing Units (DTUs): Attempt at an Inventory. PloS Negl. Trop. Dis. 10 (8), e0004792. doi: 10.1371/journal.pntd.0004792
Callahan, B. J., McMurdie, P. J., Rosen, M. J., Han, A. W., Johnson, A. J. A., Holmes, S. P. (2016). DADA2: High-Resolution Sample Inference From Illumina Amplicondata. Nat. Methods 13, 581–583. doi: 10.1038/nmeth.3869
Camargo, M. E. (1966). Fluorescent Antibody Test for the Serodiagnoses of American Trypanosomiasis: Technical Modification Employing Preserved Culture Forms of Trypanosoma cruzi in a Slide Test. Rev. Inst. Med. Trop. Sao Paulo 8, 227–234.
Carcavallo, R. U., Franca-Rodríguez, M. E., Salvatella, R., Curto de Casas, S. I., Sherlock, I., Galvão, C., et al. (1998). Habitats E Fauna Relacionada. Eds. Carcavallo, R. U., Galíndez Girón, I., Jurberg, J., Lent, H. (Rio de Janeiro, RJ: Fiocruz), 561–600.
Charles, R. A., Kjos, S., Ellis, A. E., Barnes, J. C., Yabsley, M. J. (2013). Southern Plains Woodrats (Neotoma micropus) From Southern Texas are Importante Reservoirs of Two Genotypes of Trypanosoma cruzi and Host of a Putative Novel Trypanosoma Species. Vector Borne Zoonotic Dis. 13 (1), 22–30. doi: 10.1089/vbz.2011.0817
Cooper, C., Keatley, S., Northover, A., Gofton, A. W., Brigg, F., Lymbery, A. J., et al. (2018). Next Generation Sequencing Reveals Widespread Trypanosome Diversity and Polyparasitism in Marsupials From Western Australia. Int. J. Parasitol. Parasites Wildl. 7 (1), 58–67. doi: 10.1016/j.ijppaw.2018.01.005
Dario, M. A., Andrade, T. E. S., dos Santos, C. B., Fux, B., Brandão, A. A., Falqueto, A. (2018). Molecular Characterization of Trypanosoma cruzi Samples Derived From Triatoma vitticeps and Panstrongylus geniculatus of the Atlantic Rainforest, Southeast Brazil. Parasite. 25, 59. doi: 10.1051/parasite/2018060
Dario, M. A., Lisboa, C. V., Costa, L. M., Moratelli, R., Nascimento, M. P., Costa, L. P., et al. (2017b). High Trypanosoma Spp. Diversity Is Maintained by Bats and Triatomines in Espírito Santo State, Brazil. PloS One 12 (11), e0188412. doi: 10.1371/journal.pone.0188412
Dario, M. A., Lisboa, C. V., Silva, M. V., Herrera, H. M., Rocha, F. L., Furtado, M. C., et al. (2021a). Crithidia mellificae Infection in Different Mammalian Species in Brazil. Int. J. Parasitol. Parasites Wildl. 15, 58–69. doi: 10.1016/j.ijppaw.2021.04.003
Dario, M. A., Moratelli, R., Schwabl, P., Jansen, A. M., Llewellyn, M. S. (2017a). Small Subunit Ribosomal Metabarcoding Reveals Extraordinary Trypanosomatid Diversity in Brazilian Bats. PloS Negl. Trop. Dis. 11, e0005790. doi: 10.1371/journal.pntd.0005790
Dario, M. A., Pavan, M. G., Rodrigues, M. S., Lisboa, C. V., Kluyber, D., Desbiez, A. L. J., et al. (2021b). Trypanosoma rangeli Genetic, Mammalian Hosts, and Geographical Diversity From Five Brazilian Biomes. Pathog. 10, 736. doi: 10.3390/pathogens10060736
Dario, M. A., Rodrigues, M. S., Barros, J. H., Xavier, S. C., D’Andrea, P. S., Roque, A. L., et al. (2016) Ecological Scenario and Trypanosoma cruzi DTU Characterization of a Fatal Acute Chagas Disease CaseTransmitted Orally (Espı´rito Santo State, Brazil. Parasit. Vectors (2010) 9:477. doi: 10.1186/s13071-016-1754-4
d’Avila-Levy, C. M., Yurchenko, V., Votýpka, J., Grellier, P. (2016). Protist Collections: Essential for Future Research. Trends Parasitol. 32, 840–842. doi: 10.1016/j.pt.2016.08.001
Edgar, R. C., Flyvbjerg, H. (2015). Error Filtering, Pair Assembly and Error Correction For Next-Generation Sequencing Reads Nat. Bioinformatics. 31(21), 3476–3482. doi: 10.1093/bioinformatics/btv401
Edgar, R. C. (2016). UNOISE2: Improved Error-Correction For Illumina 16S and ITS Amplicon Sequencing. bioRxiv doi: 10.1101/081257
Falla, A., Herrera, C., Fajardo, A., Montilla, M., Vallejo, G., Guhl, F. (2009). Haplotype Identification Within Trypanosoma cruzi I in Colombian Isolates From Several Reservoirs, Vectors and Humans. Acta Trop. 110 (1), 15–21. doi: 10.1016/j.actatropica.2008.12.003
Fernandes, O., Santos, S. S., Cupolillo, E., Mendonça, B., Derre, R., Junqueira, A. C. V., et al. (2001). A Mini-Exon Multiplex Polymerase Chain Reaction to Distinguish the Major Groups of Trypanosoma cruzi and T. rangeli in the Brazilian Amazon. Trans. R. Soc. Trop. Med. Hyg. 95, 97–99. doi: 10.1016/S0035-9203(01)90350-5
Gurgel-Gonçalves, R., Galvão, C., Costa, J., Peterson, A. T. (2012). Geographic Distribution of Chagas Disease Vectors in Brazil Based on Ecological Niche Modeling. J. Trop. Med. 2012, 705326. doi: 10.1155/2012/705326
Gürtler, R. E., Cardinal, M. V. (2015). Reservoir Host Competence and the Role of Domestic and Commensal Hosts in the Transmission of Trypanosoma cruzi. Acta Trop. 151, 32–50. doi: 10.1016/j.actatropica.2015.05.029
Gürtler, R. E., Cecere, M. C., Lauricella, M. A., Cardinal, M. V., Kitron, U., Cohen, J. E. (2007). Domestic Dogs and Cats as Sources of Trypanosoma cruzi Infection in Rural Northwestern Argentina. Parasitol 134, 69–82. doi: 10.1017/S0031182006001259
Herrera, C., Bargues, M. D., Fajardo, A., Montilla, M., Triana, O., Vallejo, G. A., et al. (2007). Identifying Four Trypanosoma cruzi I Isolate Haplotypes From Different Geographic Regions in Colombia. Infect. Genet. Evol. 7 (4), 535–539. doi: 10.1016/j.meegid.2006.12.003
Herrera, L., D’Andrea, P. S., Xavier, S. C. C., Mangia, R. H., Fernandes, O., Jansen, A. M. (2005). Trypanosoma cruzi Infection in Wild Mammals of the National Park ‘‘Serra Da Capivara’’, and its Surroundings (Piauí, Brazil), Endemic for Chagas Disease. Trans. R. Soc Trop. Med. Hyg. 99, 379–388. doi: 10.1016/j.trstmh.2004.07.006
Herrera, C. P., Licon, M. H., Nation, C. S., Jameson, S. B., Wesson, D. M. (2015). Genotype Diversity of Trypanosoma cruzi in Small Rodents and Triatoma sanguisuga From a Rural Area in New Orleans, Louisiana. Parasitol. Vectors. 8, 123. doi: 10.1186/s13071-015-0730-8
Herrera, H. M., Rademaker, V., Abreu, U. G., D’Andrea, P. S., Jansen, A. M. (2007). Variables That Modulate the Spatial Distribution of Trypanosoma cruzi and Trypanosoma evansi in the Brazilian Pantanal. Acta Trop. 102, 55–62. doi: 10.1016/j.actatropica.2007.03.001
Hoang, D. T., Chernomor, O., von Haeseler, A., Minh, B. Q., Vinh, L. S. (2018). UFBoot2: Improving the Ultrafast Bootstrap Approximation. Mol. Biol. Evol. 35, 518–522. doi: 10.1093/molbev/msx281
Hoare, C. A. (1966). The Classification of Mammalian Trypanosomes. Ergeb. Mikrobiol. Immunitatsforsch. Exp. Ther. 39, 43–57. doi: 10.1007/978-3-662-38353-7_3
Huggins, L. G., Koehler, A. V., Ng-Nguyen, D., Wilcox, S., Schunack, B., Inpankaew, T., et al. (2019). A Novel Metabarcoding Diagnostic Tool to Explore Protozoan Haemoparasite Diversity in Mammals: A Proof-of-Concept Study Using Canines From the Tropics. Sci. Rep. 9 (1), 1–10. doi: 10.1038/s41598-019-49118-9
IBGE-Instituto Brasileiro de Geografia e Estatística (2010). Available at: www.ibge.gov.br.
Instituto Chico Mendes de Conservação da Biodiversidade (2018) Livro Vermelho Da Fauna Brasileira Ameaçada De Extinção. Available at: www.icmbio.gov.br/portal/component/content/article/10187 (Accessed December 1, 2021).
Ishemgulova, A., Butenko, A., Kortišová, L., Boucinha, C., Grybchuk-Ieremenko, A., Morelli, K. A., et al. (2017). Molecular Mechanisms of Thermal Resistance of the Insect Trypanosomatid Crithidia thermophila. PloS One 12 (3), e0174165. doi: 10.1371/journal.pone.0174165
Jansen, A. M., das Chagas Xavier, S. C., Roque, A. L. R. (2018). Trypanosoma cruzi Transmission in the Wild and Its Most Important Reservoir Hosts in Brazil. Parasitol. Vectors. 11, 502. doi: 10.1186/s13071-018-3067-2
Jansen, A. M., das Chagas Xavier, S. C., Roque, A. L. R. (2020). Landmarks of the Knowledge and Trypanosoma cruzi Biology in the Wild Environment. Front. Cell. Infect. Microbiol. 10, 10. doi: 10.3389/fcimb.2020.00010
Jansen, A. M., Xavier, S. C., Roque, A. L. R. (2015). The Multiple and Complex and Changeable Scenarios of the Trypanosoma cruzi Transmission Cycle in the Sylvatic Environment. Acta Trop. 151, 1–15. doi: 10.1016/j.actatropica.2015.07.018
Joshi, N., Fass, J. (2011) Sickle: A Sliding-Window, Adaptive, Quality-Based Trimming Tool for FastQ file (1.29) [Software]. Available at: https://github.com/najoshi/sickle
Kalyaanamoorthy, S., Minh, B. Q., Wong, T. K. F., von Haesele, A., Jermiin, L. S. (2017). Model Finder: Fast Model Selection for Accurate PhylogeneticEstimates Nat. Methods. 14, 587–589. doi: 10.1038/nmeth.4285
Katoh, S. (2013). MAFFT Multiple Sequence Alignment Software Version 7: Improvements in Performance and Usability. Mol. Biol. Evol. 30, 772–780. doi: 10.1093/molbev/mst010
Kumar, S., Stecher, G., Li, M., Knyaz, C., Tamura, K. (2018). MEGA X: Molecular Evolutionary Genetics Analysis Across Computing Platforms. Mol. Biol. Evol. 35, 1547–1549. doi: 10.1093/molbev/msy096
Langmead, B., Salzberg, S. L.. (2012)Fast Gapped-Read Alignment With Bowtie 2 Nat. Methods 9, 357–359. doi: 10.1038/nmeth.1923
Langride, D. F., McGhee, R. B. (1967). Crithidia mellificae N. Sp. An Acidophilic Trypanosomatid of the Honeybee Apis mellifera. J. Protozool. 14, 485–487. doi: 10.1111/j.1550-7408.1967.tb02033.x
Léger, L. (1902). Sur Un Flagelle Parasite De L’Anopheles maculipennis. Compt. Rend. Soc Biol. 54, 354–356.
Lima, M. M., Sarquis, O., de Oliveira, T. G., Gomes, T. F., Coutinho, C., Daflon-Teixeira, N. F., et al. (2012). Investigation of Chagas Disease in Four Periurban Areas in Northeastern Brazil: Epidemiologic Survey in Man, Vectors, non-Human Hosts and Reservoirs. Trans. R. Soc. Trop. Med. Hyg. 106, 143–149. doi: 10.1016/j.trstmh.2011.10.013
Lipa, J. J., Triggiani, O. (1988). Crithidia bombi Sp.N. A Flagellated Parasite of a Bumblebee Bombus terrestris L. (Hymenoptera, Apidae). Acta Protozool. 27, 287–290.
Lisboa, C. V., Monteiro, R. V., Martins, A. F., Xavier, S. C., Lima, V .D. S., Jansen, A. M., et al. (2015)Infection with Trypanosoma cruzi TcII and TcI in Free-Ranging Population of Lion Tamarins (Leontopithecus spp): An 11-Year Follow-Up. Mem. Inst. Oswaldo Cruz 110 (3), 394–402. doi: 10.1590/0074-02760140400
Magoč, T., Salzberg, S. L. (2011). FLASH: Fast Length Adjustment of Short Reads to Improve Genome Assemblies. Bioinformatics 27 (21), 2957–2963. doi: 10.1093/bioinformatics/btr507
Maslov, D. A., Lukes, J., Jirku, M., Simpson, L. (1996). Phylogeny of Trypanosomes as Inferred From the Small and Large Subunit rRNAs: Implications for the Evolution of Parasitism in the Trypanosomatid Protozoa. Mol. Biochem. Parasitol. 75 (2), 197–205. doi: 10.1016/0166-6851(95)02526-X
Maslov, D. A., Opperdoes, F. R., Kostyogv, A. Y., Hasimi, H., Lukeš, J., Yurchenko, V. (2019). Recent Advances in Trypanosomatid Research: Genome Organization, Expression, Metabolismo, Taxonomy and Evolution. Parasitol 146, 1–27. doi: 10.1017/S0031182018000951
McMurdie, P. J., Holmes, S. (2013). Phyloseq: An R Package for Reproducible Inter-Active Analysis and Graphics of Microbiome Census Data. PloS One 8, e61217. doi: 10.1371/journal.pone.0061217
Morales, E. A., Mayor, P., Bowler, M., Aysanoa, E., Pérez-Velez, E. S., Pérez, J., et al. (2017). Prevalence of Trypanosoma cruzi and Other Trypanosomatids in Frequently-Hunted Wild Mammals From the Peruvian Amazon. Am. J. Trop. Med. Hyg. 5, 1482–1485. doi: 10.4269/ajtmh.17-0028
Myers, N., Mittermeier, R. A., Mittermeier, C. G., Fonseca, G. A. B., Kent, J. (2000). Biodiversity Hotspots for Conservation Priorities. Nature 403, 853–859. doi: 10.1038/35002501
Naiff, R. D., Barrett, T. V. (2013). Trypanosoma (Megatrypanum) lainsoni N. Sp. From Mesomys hispidus (Rodentia: Echimyidae) in Brazil: Trypomastigotes Described From Experimentally Infected Laboratory Mice. Parasite 20, 51. doi: 10.1051/parasite/2013049
Nantes, W. A. G., Santos, F. M., de Macedo, G. C., Barreto, W. T. G., Gonçalves, L. R., Rodrigues, M. S., et al. (2021). Trypanosomatid Species in Didelphis albiventris From Urban Forest Fragments. Parasitol. Res. 120 (1), 223–231. doi: 10.1007/s00436-020-06921-y
Nguyen, L. T., Schmidt, H. A., von Haeseler, A., Minh, B. Q. (2015). IQ-TREE: A Fast and Effective Stochastic Algorithm for Estimating Maximum Likelihood Phylogenies. Mol. Biol. Evol. 32, 268–274. doi: 10.1093/molbev/msu300
Noireau, F., Diosque, P., Jansen, A. M. (2009). Trypanosoma cruzi: Adaptation to its Vectors and its Hosts. Vet. Res. 40 (2), 26. doi: 10.1051/vetres/2009009
Noyes, H. A., Stevens, J. R., Teixeira, M., Phelan, J., Holz, P. (1999). A Nested PCR for the ssrRNA Gene Detects Trypanosoma binneyi in the Platypus and Trypanosoma Sp. In Wombats and Kangaroos in Australia. Int. J. Parasitol. 29, 331–339. doi: 10.1016/S0020-7519(98)00167-2
O’Connor, O., Bosseno, M. F., Barnabé, C., Douzery, E. J., Brenière, S. F. (2007). Genetic Clustering of Trypanosoma cruzi I Lineage Evidenced by Intergenic Miniexon Gene Sequencing. Infect. Genet. Evol. 7 (5), 587–593. doi: 10.1016/j.meegid.2007.05.003
Olifiers, N., Jansen, A. M., Herrera, H. M., de Cassia Bianchi, R., D’Andrea, P. S., de Miranda Mourão, G., et al. (2015). Co-Infection and Wild Animal Health: Effects of Trypanosomatids and Gastrointestinal Parasites on Coatis of the Brazilian Pantanal. PloS One 10 (12), e0143997. doi: 10.1371/journal.pone.0143997
Orozco, M. M., Piccinali, R. V., Mora, M. S., Enriquez, G. F., Cardinal, M. V., Gürtler, R. E. (2014). The Role of Sigmodontine Rodents as Sylvatic Hosts of Trypanosoma cruzi in the Argentinean Chaco. Infect. Genet. Evol. 22, 12–22. doi: 10.1016/j.meegid.2013.12.020
Patiño, L. H., Castillo-Castañeda, A. C., Muñoz, M., Jaimes, J. E., Luna-Niño, N., Hernández, C., et al. (2021). Development of an Amplicon-Based Next-Generation Sequencing Protocol to Identify Leishmania Species and Other Trypanosomatids in Leishmaniasis Endemic Areas. Microbiol. Spectr. 9 (2), e0065221. doi: 10.1128/Spectrum.00652-21
Pineda, V., Saldana, A., Monfante, I., Santamaria, A., Gottdenker, N. L., Yabsley, M. J., et al. (2011). Prevalence of Trypanosome Infections in Dogs From Chagas Disease Endemic Regions in Panama, Central America. Vet. Parasitol. 178, 360–363. doi: 10.1016/j.vetpar.2010.12.043
Podlipaev, S. (2001). The More Insect Trypanosomatids Under Study-the More Diverse Trypanosomatidae Appears. Int. J. Parasitol. 31, 648–652. doi: 10.1016/S0020-7519(01)00139-4
Polzin, T., Daneschmand, S. V. (2003). On Steiner Trees and Minimum Spanning Trees in Hypergraphs. Oper. Res. Lett. 31, 12–20. doi: 10.1016/S0167-6377(02)00185-2
Quast, C., Pruesse, E., Yilmaz, P., Gerken, J., Schweer, T., Yarza, P., et al. (2013)The SILVA Ribosomal RNA GeneDatabase Project: Improved Data Processing and Web-Based Tools. Nucl. Acids Res 41 (D1), 590–596. doi: 10.1093/nar/gks1219
Rademaker, V., Herrera, H. M., Raffel, T. R., D’Andrea, P. S., Freitas, T. P., Abreu, U. G., et al. (2009). What is the Role of Small Rodents in the Transmission Cycle of Trypanosoma cruzi and Trypanosoma evansi (Kinetoplastida Trypanosomatidae)? A Study Case in the Brazilian Pantanal. Acta Trop. 111, 102–107. doi: 10.1016/j.actatropica.2009.02.006
Rambaut, A., Drummond, A. J., Xie, D., Baele, G., Suchard, M. A. (2018). Posterior Summarisation in Bayesian Phylogenetics Using Tracer 1.7. Systemat. Biol. 67 (5), 901–904. doi: 10.1093/sysbio/syy032
Ramirez, J. D., Duque, M. C., Guhl, F. (2011). Phylogenetic Reconstruction Based on Cytochrome B (Cytb) Gene Sequences Reveals Distinct Genotypes Within Colombian Trypanosoma cruzi I Populations. Acta Trop. 119 (1), 61–65. doi: 10.1016/j.actatropica.2011.04.009
Ramsey, J. M., Gutiérrez-Cabrera, A. E., Salgado-Ramírez, L., Peterson, A. T., Sánchez-Cordero, V., Ibarra-Cerdeña, C. N. (2012). Ecological Connectivity of Trypanosoma cruzi Reservoirs and Triatoma pallidipennis Hosts in Na Anthropogenic Landscape With Endemic Chagas Disease. PloS One 7 (9), e46013. doi: 10.1371/journal.pone.0046013
Rangel, D. A., Lisboa, C. V., Novaes, R. L. M., Silva, B. A., de Franca Souza, R., Jansen, A. M., et al. (2019). Isolation and Characterization of Trypanosomatids, Including Crithidia mellificae, in Bats From the Atlantic Forest of Rio De Janeiro, Brazil. PloS Negl. Trop. Dis. 13 (7), e0007527. doi: 10.1371/journal.pntd.0007527
R Development Core. (2021). A Language and Environment for Statistical Computing. R Foundation for Statistical Computing 2. Available at: https://www.R-project.org.
Rodrigues, M. S., Lima, L., das Chagas Xavier, S. C., Herrera, H. M., Rocha, F. L., Roque, A. L. R., et al. (2019). Uncovering Trypanosoma Spp. Diversity of Wild Mammals by the Use of DNA From Blood Clots. Int. J. Parasitol. Parasites Wildl 14, 171–181. doi: 10.1016/j.ijppaw.2019.02.004
Rognes, T., Flouri, T., Nichols, B., Quince, C., Mahé, F. (2016). VSEARCH: A Versatile Open Source Tool for Metagenomics. PeerJ. 4, e2584. doi: 10.7717/peerj.2584
Roman, F., Iñiguez, A. M., Yeo, M., Jansen, A. M. (2018). Multilocus Sequence Typing: Genetic Diversity in Trypanosoma cruzi I (TcI) Isolates From Brazilian Didelphids. Parasitol. Vectors. 11, 107. doi: 10.1186/s13071-018-2696-9
Roque, A. L., D’Andrea, P. S., de Andrade, G. B., Jansen, A. M. (2005). Trypanosoma cruzi: Distinct Patterns of Infection in the Sibling Caviomorph Rodent Species Thrichomys apereoides laurentius and Thrichomys pachyurus (Rodentia, Echimyidae). Exp. Parasitol. 111, 37–46. doi: 10.1016/j.exppara.2005.05.003
Roque, A. L. R., Jansen, A. M. (2008). Importância Dos Animais Domésticos Sentinelas Na Identificação De Áreas De Risco De Emergência De Doença De Chagas. Rev. Soc Bras. Med. Trop. 41 (III), 191–193.
Roque, A. L. R., Xavier, S. C. C., da Rocha, M. G., Duarte, A. C. M., D’Andrea, P. S., Jansen, A. M. (2008). Trypanosoma cruzi Transmission Cycle Among Wild and Domestic Mammals in Three Areas of Orally Transmitted Chagas Disease Outbreaks. Am. J. Trop. Med. Hyg. 79 (5), 742–749. doi: 10.4269/ajtmh.2008.79.742
Rozas, M., de Doncker, S., Adaui, V., Coronado, X., Barnabé, C., Tibyarenc, M., et al. (2007). Multilocus Polymerase Chain Reaction Restriction Fragment-Length Polymorphism Genotyping of Trypanosoma cruzi (Chagas Disease): Taxonomic and Clinical Applications. J. Infect. Dis. 195 (9), 1381–1388. doi: 10.1086/513440
Salazar, A., Schijman, A. G., Triana-Chávez, O. (2006). High Variability of Colombian Trypanosoma cruzi Lineage I Stock as Revealed by Low-Stringency Single Primer-PCR Minicircle Signatures. Acta Trop. 100 (1-2), 110–118. doi: 10.1016/j.actatropica.2006.10.003
Sambrook, J., Russel, D. W. (2001). Molecular Cloning - A Laboratory Manual (New York: Cold Spring Harbor Laboratory Press).
Silva, J. M. C., Tabarelli, M., Fonseca, M. T., Lins, L. V. (2004). Biodiversidade Da Caatinga: Áreas E Ações Prioritárias Para a Conservação (Brasília, DF: MMA/UFPE/Conservation International – Biodiversitas – Embrapa Semi-árido).
Smith, A., Clark, P., Averis, S., Lymbery, A. J., Wayne, A. F., Morris, K. D., et al. (2008). Trypanosomes in a Declining Species of Threatened Australian Marsupial, the Brush-Tailed Bettong Bettongia Penicillate (Marsupialia: Potoroidae). Parasitol 35, 1329–1335. doi: 10.1017/S0031182008004824
Souza, B. I. F., Artigas, R. C., Lima, E. R. V. (2015). Caatinga E Desertificação. Mercator. 14 (1), 131–150. doi: 10.4215/RM2015.1401.0009
Farias, MS, Cestari, VRF, Garces, TS, Maranhão, TA, Moreira, TMM (2020). Spatiotemporal Trends of Chagas disease-Related Mortality in the Northeast of Brazil, 2007–2017. Parasitology 147, 1552–58. doi: 10.1017/S0031182020001419
Spotorno, O. A. E., Córdova, L., Solari, A. I. (2008). Differentiation of Trypanosoma cruzi I Subgroup Through Characterization of Cytochrome B Gene Sequences. Infect. Genet. Evol. 8, 898–900. doi: 10.1016/j.meegid.2008.08.006
Vaz, V. C., D’Andrea, P. S., Jansen, A. M. (2007). Effects of Habitat Fragmentation on Wild Mammal Infection by Trypanosoma cruzi. Parasitology 134, 1785–1193. doi: 10.1017/S003118200700323X
Vickerman, K. (1976). Comparative Cell Biology of the Kinetoplastid Flagellates (London: Academic Press).
Wilson, D. E., Reeder, D. M. (2005). Mammal Species of the World: A Taxonomic and Geographic Reference (Baltimore: The John Hopkins University Press).
Xavier, S. C., Roque, A. L., Lima, V. S., Monteiro, K. J., Otaviano, J. C., Ferreira da Silva, L. F., et al. (2012). Lower Richness of Small Wild Mammal Species and Chagas Disease Risk. PLoS Negl Trop Dis 6 (5), e1647. doi: 10.1371/journal.pntd.0001647
Yeo, M., Acosta, N., Llewellyn, M., Sánchez, H., Adamson, S., Miles, G. A. J., et al. (2005). Origins of Chagas Disease: Didelphis Species are Natural Hosts of Trypanosoma cruzi I and Armadillos Hosts of Trypanosoma cruzi II, Including Hybrids. Int. J. Parasitol. 35, 225–233. doi: 10.1016/j.ijpara.2004.10.024
Yurchenko, V., Votýpka, J., Tesarová, M., Klepetková, H., Kraeva, N., Jirků, M., et al. (2014). Ultrastructure and Molecular Phylogeny of Four New Species of Monoxenous Trypanosomatids From Flies (Diptera: Brachycera) With Redefinition of the Genus Wallaceina. Folia Parasitol. 61, 97–112. doi: 10.14411/fp.2014.023
Zepeda Mendoza, M. L., Sicheritz-Pontén, T., Gilbert, M. T. P. (2015). Environmental Genes and Genomes: Understanding the Diferences and Challenges in the Approaches and Software for Their Analyses. Brief. Bioinform. 16, 745–758. doi: 10.1093/bib/bbv001
Zhang, D., Gao, F., Jakovlić, I., Zou, H., Zhang, J., Li, W. X., et al. (2020), PhyloSuite: An Integrated and Scalable Desktop Platform for Streamlined Molecular Sequence Data Management and Evolutionary Phylogenetics Studies. Mol. Ecol. Res. 20 (1), 348–355. doi: 10.1111/1755-0998.13096
Keywords: Trypanosomatidae richness, Trypanosoma cruzi infection, T. cruzi DTU TcI haplotype, synantropic mammals, Canis familiaris, Caatinga biome
Citation: Dario MA, Furtado C, Lisboa CV, de Oliveira F, Santos FM, D’Andrea PS, Roque ALR, Xavier SCdC and Jansen AM (2022) Trypanosomatid Richness Among Rats, Opossums, and Dogs in the Caatinga Biome, Northeast Brazil, a Former Endemic Area of Chagas Disease. Front. Cell. Infect. Microbiol. 12:851903. doi: 10.3389/fcimb.2022.851903
Received: 10 January 2022; Accepted: 11 April 2022;
Published: 20 June 2022.
Edited by:
Javier Moreno, Instituto de Salud Carlos III (ISCIII), SpainReviewed by:
Juan David Ramírez, Icahn School of Medicine at Mount Sinai, United StatesCopyright © 2022 Dario, Furtado, Lisboa, de Oliveira, Santos, D’Andrea, Roque, Xavier and Jansen. This is an open-access article distributed under the terms of the Creative Commons Attribution License (CC BY). The use, distribution or reproduction in other forums is permitted, provided the original author(s) and the copyright owner(s) are credited and that the original publication in this journal is cited, in accordance with accepted academic practice. No use, distribution or reproduction is permitted which does not comply with these terms.
*Correspondence: Maria Augusta Dario, bWFyaWEuZGFyaW9AaW9jLmZpb2NydXouYnI=
Disclaimer: All claims expressed in this article are solely those of the authors and do not necessarily represent those of their affiliated organizations, or those of the publisher, the editors and the reviewers. Any product that may be evaluated in this article or claim that may be made by its manufacturer is not guaranteed or endorsed by the publisher.
Research integrity at Frontiers
Learn more about the work of our research integrity team to safeguard the quality of each article we publish.