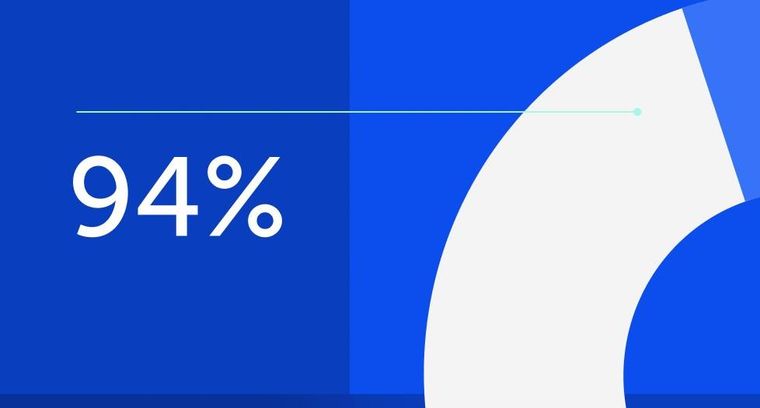
94% of researchers rate our articles as excellent or good
Learn more about the work of our research integrity team to safeguard the quality of each article we publish.
Find out more
REVIEW article
Front. Cell. Infect. Microbiol., 18 March 2022
Sec. Bacteria and Host
Volume 12 - 2022 | https://doi.org/10.3389/fcimb.2022.849915
This article is part of the Research TopicListeria: Environmental Adaption, Infection and Host InteractionsView all 7 articles
Many bacterial species, including several pathogens, can enter a so-called “viable but non-culturable” (VBNC) state when subjected to stress. Bacteria in the VBNC state are metabolically active but have lost their ability to grow on standard culture media, which compromises their detection by conventional techniques based on bacterial division. Under certain conditions, VBNC bacteria can regain their growth capacity and, for pathogens, their virulence potential, through a process called resuscitation. Here, we review the current state of knowledge of the VBNC state of Listeria monocytogenes (Lm), a Gram-positive pathogenic bacterium responsible for listeriosis, one of the most dangerous foodborne zoonosis. After a brief summary of characteristics of VBNC bacteria, we highlight work on VBNC Lm in the environment and in agricultural and food industry settings, with particular emphasis on the impact of antimicrobial treatments. We subsequently discuss recent data suggesting that Lm can enter the VBNC state in the host, raising the possibility that VBNC forms contribute to the asymptomatic carriage of this pathogen in wildlife, livestock and even humans. We also consider the resuscitation and virulence potential of VBNC Lm and the danger posed by these bacteria to at-risk individuals, particularly pregnant women. Overall, we put forth the hypothesis that VBNC forms contribute to adaptation, persistence, and transmission of Lm between different ecological niches in the One-Health continuum, and suggest that screening for healthy carriers, using alternative techniques to culture-based enrichment methods, should better prevent listeriosis risks.
Listeria monocytogenes (Lm) is a ubiquitous Gram-positive bacterium that contaminates food production lines and is pathogenic for humans and various animal species (Bagatella et al., 2021; Quereda et al., 2021). This facultative intracellular pathogen has evolved sophisticated mechanisms to enter, grow and survive in many eukaryotic cell types and to infect several organs, including the intestine, liver, spleen, brain and placenta (Radoshevich and Cossart, 2017). Lm is the etiological agent of listeriosis, a disease characterized by bacteremia, central nervous system infections, spontaneous abortions and perinatal infections (Schlech, 2019; Charlier et al., 2020; Bagatella et al., 2021). Listeriosis leads to the highest number of hospitalizations and case fatality rate of any foodborne zoonosis in Europe (European Food Safety et al., 2021), as well as in the United States (Scallan et al., 2011). These rates have not yet been estimated in other regions of the world, but it is noteworthy that countries in Asia and Africa, as well as Australia, have experienced several listeriosis outbreaks in recent decades, with the largest ever in South Africa (2017-2018; 1060 cases, 216 deaths) (Kaptchouang Tchatchouang et al., 2020). The foods responsible of this massive outbreak were processed meat products, named polony. Foods associated with listeriosis include raw meat and fish, seafood, unpasteurized dairy products and foods contaminated after processing, including soft cheeses and deli meats. In recent years, increased surveillance for Lm has shown that this dangerous germ can contaminate a variety of ready-to-eat (RTE) foods, including uncooked vegetables and fresh-cut fruits (Buchanan et al., 2017). For example, in Europe, a multi-country outbreak was attributed to consumption of frozen sweet corn (2015-2018; 53 cases, 10 deaths) (McLauchlin et al., 2021), while recent outbreaks in the United States have been associated with consumption of enoki mushrooms (2020; 36 cases, 4 deaths) and packaged salads (2021; 27 cases, 3 deaths, in two separate outbreaks) (https://www.cdc.gov/listeria/outbreaks/index.html).
The remarkable adaptation of Lm to different ecosystems, in the environment (e.g., river, lake and sea water, sewage, soil, plants, decaying plants, fodder, silage, agricultural effluents), a variety of foods, and hosts of diverse species, makes it an important archetype of the “One-Health” approach to infectious diseases, which recognizes the interconnection between human health, animal health and environmental ecosystems. However, there is much to discover about the ecology of Lm, in terms of natural reservoirs and mechanisms of transmission. In particular, the potential for asymptomatic carriage of this pathogen has been less explored than its mechanisms of pathogenicity. However, healthy carriers play an important role as reservoirs and dispersion factors of Lm in the environment. As early as the 1960s, Gray and Killinger (1966) cited 37 mammalian species from which this pathogen was isolated in the feces (Gray and Killinger, 1966). Lm is also found in many species of wild mammals, such as rats, hares, hedgehogs, badgers, deer, moose, otters, raccoons, foxes, bears, boars, monkeys (Giovannini et al., 1988; Inoue et al., 1991; Low and Donachie, 1997; Yoshida et al., 2000; Lyautey et al., 2007; Rothenburger et al., 2015; Weindl et al., 2016; Hydeskov et al., 2019; Parsons et al., 2019; Fredriksson-Ahomaa et al., 2020; Palacios-Gorba et al., 2021) and even aquatic mammals, such as dolphins (Sevellec et al., 2020). Lm is also a pathogen of farmed mammals, such as sheep, goats, cattle, pigs, horses and rabbits, and pet animals, such as dogs and cats (Weber et al., 1995; Lyautey et al., 2007; Oevermann et al., 2010; Leclercq et al., 2014; Rothenburger et al., 2015). Oevermann and colleagues recently discussed the current knowledge of listeriosis pathology in naturally susceptible species, focusing on ruminants and the neuro-invasive form of the disease (Bagatella et al., 2021). While most of these studies have looked for Lm in sick animals, healthy animals can constitute an important reservoir, releasing the pathogen in the environment through fecal excretion or abortion products. In this regard, it is worth mentioning that Lm prevalence can sometimes be as high as 30-40% in the feces of healthy cattle (Weber et al., 1995; Hurtado et al., 2017). Healthy carriage of Lm also exists in humans, as described in several studies (Lamont and Postlethwaite, 1986; Muller, 1990; MacGowan et al., 1991; Mascola et al., 1992; Iida et al., 1998; Grif et al., 2001; Grif et al., 2003). However, the techniques used to determine the prevalence of asymptomatic carriage have lacked sensitivity and have generally relied on testing for culturable bacteria in feces. A very recent study has significantly improved the knowledge of the frequency of this carriage, by studying the prevalence of Lm DNA in the stool of a French cohort of 900 healthy individuals. The use of PCR amplification of the Lm virulence gene hly revealed a significant proportion of 10% of asymptomatic Lm carriers in this population (Hafner et al., 2021). This study also indicated that metagenomics based on 16S rRNA gene sequencing (i.e., metataxonomics) was less efficient in detecting healthy Lm carriers (an order of 5%), whereas full metagenomic studies using shotgun sequencing failed in detecting Lm DNA signal in the gut microbiota. This highlights the importance of using sensitive molecular techniques to detect healthy carriers. However, it should be remembered that these molecular biology-based approaches cannot determine whether the detected DNA is from live or dead bacteria.
Lm is known to be tolerant to many physical and physicochemical stresses, allowing it to survive in a wide range of environmental conditions (Tasara and Stephan, 2006; Gahan and Hill, 2014; Dorey et al., 2019; Matereke and Okoh, 2020; Quereda et al., 2021; Wiktorczyk-Kapischke et al., 2021). The ability of Lm to enter a so-called Viable But Non-Culturable state (VBNC) is also a key factor in the survival of this non-spore-forming bacterium following stress. This property was first described 20 years ago for Lm present in water (Besnard et al., 2000) and more recently within human cells (Kortebi et al., 2017), findings which raise the issue of detecting “silent” forms of this pathogen in agriculture, food and asymptomatic hosts (Bierne et al., 2018). Here, we review the state of knowledge on the VBNC status of Lm. The various techniques associated with detection of VBNC forms will not be extensively discussed in this review, but are summarized in Table 1, and we refer readers to excellent recent reviews on this topic (Kumar and Ghosh, 2019; Dong et al., 2020; Wideman et al., 2021). We apologize to the authors whose work was not cited, due to space constraints.
Microorganisms colonize an impressive variety of ecological niches and survive a wide range of adverse environmental conditions. The survival strategies employed by bacteria encompass two main mechanisms. The first one is sporulation, which consists of the formation of a specialized cell (or endospore), metabolically inactive and resistant to many environmental perturbations (e.g., heat treatment, high pressure, dehydration, lack of nutrients, antimicrobial agents, UV and γ radiation). When conditions become favorable for regrowth, the spore is able to germinate to regenerate a vegetative bacterium (Setlow and Johnson, 2019). The second mechanism consists of entry into a state of dormancy, allowing non-spore-forming bacteria to survive environmental stress (e.g., nutrient starvation, light exposure, temperature change, osmotic pressure, oxygen concentration, pH), antimicrobial compounds (e.g., disinfectants, antibiotics) or host factors (Kaprelyants et al., 1993). This dormant state is a reversible state of slowed activity, in which a subpopulation of bacterial cells can survive for prolonged periods without cell division (Table 2). In bacterial species that transiently lose the ability to grow on standard culture media on which they are known to grow, this is referred to as a VBNC state. Bacteria in the VBNC state (here termed “VBNC bacteria”) fail to multiply and form colonies on plates, while maintaining a slowed metabolic activity. First described in 1982 for Gram-negative bacteria [Escherichia coli and Vibrio cholerae, (Xu et al., 1982)], the VBNC state is, like sporulation, a reversible state. VBNC bacteria can regain their lost culturability under certain conditions called “resuscitation” (Oliver, 2010; Li et al., 2014; Zhao et al., 2017). Illustrating this property, Vibrio vulnificus, an environmental bacterium living in estuarine waters, enters a VBNC state during winter months and regrows during warmer months (Whitesides and Oliver, 1997).
Table 2 Definitions of terms used to describe different survival strategies of bacteria to cope with environmental, antimicrobial, or host-derived stress.
It should be noted that, in addition to the VBNC state, other physiological states associated with the survival of bacteria in stressful conditions have been described, such as the sublethal state or the persistent state. The terminology used can be confusing, as the terms sometimes cover overlapping phenotypes, or phenotypes associated with clinical pictures (i.e., persistent infection and latent infection). For clarity, we provide a table defining these terms (Table 2). In particular, so-called “persisters” appear following antibiotic treatment, to which they adapt and acquire tolerance to the treatment (Gollan et al., 2019). Some authors propose that persisters and VBNC bacteria are actually part of the same continuum in the natural life cycle of microorganisms, between actively growing, dormant and dead cells. VBNC cells would thus be in a deeper dormant state than persister cells (Ayrapetyan et al., 2018). A recent study suggests that sublethal injury is also an initial stage of dormancy that is followed by the VBNC state (Arvaniti et al., 2021). It can be pointed out that VBNC cell subpopulations can also exist naturally in unstressed environments, as the result of a stochastic physiological phenomenon, which leads to a bacterial pool predisposed to resist potential future stresses (Ayrapetyan et al., 2015; Goncalves and De Carvalho, 2016).
Since its discovery in 1982, the VBNC state has been described for more than 100 species, two-thirds of which are pathogenic species (Oliver, 2010; Li et al., 2014; Ramamurthy et al., 2014; Zhao et al., 2017; Dong et al., 2020). This list is constantly growing. In general, bacteria capable of entering the VBNC state have a broad phylogenetic distribution, suggesting that the VBNC state is a general strategy adopted by different genera to survive adverse conditions (Li et al., 2014; Pinto et al., 2015). Currently, the majority of species described as entering the VBNC state are Gram-negative bacteria. Among Gram-positive bacteria, in addition to Lm, there are other pathogens of the firmicutes phylum (e.g., Staphylococcus aureus, Enterococcus faecalis), and actinobacteria (e.g., Mycobacterium tuberculosis). Because of their non-culturable nature and resuscitation capacity, VBNC bacteria are a public health problem. For species responsible for food poisoning, VBNC forms escape quality controls in food industries, as detection of contamination is most often based on methods dependent on bacterial growth in conventional enrichment media.
VBNC bacteria are also suspected of causing asymptomatic infections. However, while the ability to enter a VBNC state has been described for many pathogens, the demonstration that this state exists in the host is virtually undocumented, except in the case of Mycobacterium tuberculosis (Mtb). Human infection with Mtb produces an active form of the disease, tuberculosis (TB), one of the top ten causes of death worldwide, as well as an asymptomatic form called latent tuberculosis infection (LTBI) (Gengenbacher and Kaufmann, 2012; Dutta and Karakousis, 2014; Peddireddy et al., 2017). Mathematical models based on immunoassays suggest that a quarter of the world’s population is affected by LTBI, with a risk of reactivation to TB in about 5-10% of cases. In LTBI, Mtb bacilli enter a dormant state characterized by reduced metabolic activity in response to stressful intracellular conditions and activation of the host immune response. The bacteria enter a non-culturable state in the lungs, but also potentially in other organs (Dutta and Karakousis, 2014), without causing any symptoms, a VBNC state termed “latency”. Studies suggest that Mtb bacilli coexist in mixed populations with culturable stages, in a physiological continuum from persistence to dormancy (Gengenbacher and Kaufmann, 2012; Dutta and Karakousis, 2014; Gample et al., 2019). Reactivation of TB shows that latent VBNC cells are able to retain or, at least, regain their virulence potential upon resuscitation. Resuscitation factors (Rpfs), initially identified in the bacterium Micrococcus luteus (Kaprelyants et al., 1994; Mukamolova et al., 1998) and later in mycobacteria (Mukamolova et al., 2002; Rosser et al., 2017) are involved in this process. Some fatty acid species, adenylate cyclase, transcription factors regulating respiration pathways, and carbohydrate metabolism, also appear to be involved in Mtb resuscitation (Shleeva et al., 2013; Salina et al., 2019).
To our knowledge, the entry of Lm into the VBNC state in a natural environment was first suggested by Colburn et al. (1990), following the observation of lower frequency of culturable bacteria detected in marine water samples than in fresh water samples of the same estuary in California (Colburn et al., 1990). Bremer et al. (1998) also hypothesized this after examining the survival of Lm in sterilized seawater in the laboratory (Bremer et al., 1998). After 26 days, a fraction of the cell population seemed to be VBNC, as the number of viable cells quantified by measurement of respiratory activity (i.e., CTC-DAPI, Table 1) was greater than that determined by traditional colony forming units (CFU) counts on tryptic soy yeast extract agar plates. Besnard et al. (2000) then formally established this capacity of Lm to enter the VBNC state (Besnard et al., 2000), by culturing bacteria in a nutrient-free water or “microcosm water” (i.e., sterile filtered water of pH = 6), a medium used to study the VBNC state of Campylobacter jejuni (Rollins and Colwell, 1986). Under these conditions, and using Direct Viable Counts (DVC) and CTC-DAPI methods (Table 1), these authors showed that two strains of Lm, CNL 895807 and ScottA, formed VBNC bacteria after four to six weeks of nutrient deprivation. Furthermore, these forms retained metabolic activity for up to ten weeks. The same team later revealed the influence of inoculum concentration, light exposure, temperature, strain used, pH and NaCl concentration on the efficiency of the phenomenon (Besnard et al., 2002). Thus, a high inoculum concentration decreased the induction of the VBNC state, probably due to a higher nutrient flux within the population. Conversely, higher salt concentration or incubation temperatures stimulated entry into the VBNC state. A strain effect was also found: for the Scott A and CNL 895807 strains, the VBNC state was maintained throughout the experiment, whereas the VBNC state was transient for the LO28 and ATCC 19115 strains. VBNC bacteria induced in microcosm water conserve a metabolic activity, as demonstrated by de novo production of ATP and mRNA (Lindback et al., 2010). In the latter study, the quantification of viable bacteria was estimated by the LIVE/DEAD BacLight assay, a dual-staining microscopy method that assesses membrane integrity (Table 1).
More recently, a study showed that tap water was also a highly deprived medium in which Lm can enter the VBNC state, particularly within biofilms formed on stainless steel surfaces (Giao and Keevil, 2014). Thus, although Lm is not known to be a waterborne pathogen, the potential for Lm cells to remain on surfaces with only water as a nutrient medium can have a significant impact on public health. If decontamination is insufficient after surface cleaning, Lm could remain on food industry surfaces (e.g., floors, walls, workbenches, sinks, refrigerators) and be transferred into food products.
The studies mentioned in this section are listed in Table 3.
In addition to water, all ecosystems in the agricultural and food industries can be potentially impacted by VBNC Lm contamination. Because of the health risks of such contamination, many studies have addressed this problem by modeling the contamination by VBNC Lm in reconstituted systems (also called bacterial microcosms). The main technical difficulty is to correctly differentiate dead from VBNC cells in complex matrices. Works have examined the persistence of Lm in urban or agricultural effluents, after inoculation of bacteria into sewage sludge (Wery et al., 2006), cattle manure (Klein et al., 2011), or digestates from agricultural waste methanizers (Maynaud et al., 2016). In these studies, comparison of viable bacterial counts estimated by amplification of a bacterial gene by quantitative real-time PCR (qPCR) (Table 1), to that of culturable bacteria by CFU, showed a slower decline of Lm cells counted by PCR than by CFU, suggesting the presence of non-culturable cells in these microcosms. However, qPCR does not distinguish DNA of non-culturable bacteria from dead bacteria, generating a risk of overestimating the number of VBNC bacteria. Accurate quantification of only viable bacteria has been improved by a so-called “viability-PCR” (v-PCR), a method combining the use of an amplification inhibitor, such as propidium monoazide (PMA) or ethidium monoazide (EMA), and qPCR (PMA-qPCR; EMA-qPCR, Table 1). In particular, PMA is a DNA intercalant which enters into cells with compromised membrane integrity and blocks amplification of their DNA. Treating bacterial cells with PMA, prior to DNA extraction, therefore allows for more selective detection of viable bacteria (although there remains a bias for DNA amplification from dead cells that would have retained an intact membrane) (Dong et al., 2020; Fleischmann et al., 2021; Wideman et al., 2021). This technique provided strong evidence for the presence of VBNC Lm in pig manure (Desneux et al., 2016), as well as in food environments (see below and Table 1).
Other studies reported induction of the VBNC state of Lm on the surface of plants, including parsley (Dreux et al., 2007) and spinach leaves (Highmore et al., 2018). Indeed, Lm has evolved mechanisms to colonize plants (Truong et al., 2021). The possibility that conventional culture-based counts underestimate the number of viable Lm on plant products must be considered in the context of cross-contamination risks, when a contaminated product is washed and pathogens are transferred from the contaminated product to water and then from water to clean product. Two studies looked at this problem, investigating whether process wash water (PWW) could be a vector for VBNC contamination. Lm contamination of a PWW was modeled in the laboratory, either by inoculating a PWW of shredded lettuce with a cocktail of six Lm strains (Truchado et al., 2020) or spinach and lettuce rinse waters with a cocktail of three Lm strains (Gu et al., 2020). Potential VBNC were detected in these wastewaters by the PMA-qPCR method or viability microscopy with the BacLight assay (Table 1). Importantly, in both cases, exposure to sanitizers increased the formation of unculturable viable cells (see next section).
With respect to foods, there is ample evidence for the presence of VBNC in food matrices or food processing environments. Olszewska et al. (2015) studied the fate of Lm after bacterial inoculation of long-ripened hard cheese samples from retail market, left in different packaging conditions for up to 90 days at 6°C (Olszewska et al., 2015). As an approach to assess bacterial viability in complex matrices, these authors used direct epifluorescence filtration, based on fluorescence staining with carboxyfluorescein diacetate (CFDA), which allows tracing bacteria that exhibit metabolic activity (Table 1). Their results showed significant discrepancies between CFDA and CFU numbers at each sampling point during cheese storage, regardless of packaging conditions, indicating the existence of VBNC cells. Overney et al. (2016) studied the behavior of Lm grown in two food contaminants, smoked salmon juice or meat exudate, by quantifying total cells by qPCR, viable cells by PMA-qPCR, and culturable cells by CFU counting (Overney et al., 2016). Meat exudate appeared to be a much more stressful medium than smoked salmon juice, and generated VBNC cells. These authors proposed that these differences between meat exudate and smoked salmon juice could be explained by the difference in pH (5.4 for meat exudate, 6.2 for smoked salmon juice), which is an environmental stress. Importantly, there is evidence for the existence of VBNC forms, no longer in laboratory models, but directly in food samples. With a DVC method coupled with FISH using a 16S rRNA probe (Table 1), Moreno et al. (2012) suggested the existence of VBNC Lm in RTE vegetable samples (Moreno et al., 2012). Recently, a study using PMA-qPCR provided strong arguments for the existence of VBNC Lm in RTE salads (Bernardo et al., 2021). Sources of product contamination can come directly from surfaces in the production environment. By directly sampling critical areas of four seafood plants, over eight months, Brauge et al. (2020) detected VBNC Lm, primarily after cleaning and disinfection operations (Brauge et al., 2020), highlighting the fact that all industrial disinfectants can fail to inactivate Lm cells on inert surfaces, as discussed below.
The studies mentioned in this section are listed in Table 4.
The eradication of microorganisms in food processing plants, hospitals and domestic environments is most often done through disinfectants, while preservatives are used to maintain the microbiological quality of food, and antibiotics remain the first-line treatment for bacterial infections. However, many of these antimicrobial agents are not very effective on VBNC bacteria, which not only have a slower metabolism, but a different morphology and composition, than vegetative bacteria, making them more resistant to physico-chemical stress. (Li et al., 2014; Dong et al., 2020). Additionally, and importantly, as entering a VBNC state is a physiological adaptation to stress, these antimicrobial treatments may stimulate formation of VBNC bacteria. In the case of Lm, an initial study suggested that exposure of bacteria to potassium sorbate could promote the formation of VBNC Lm at 37°C (Cunningham et al., 2009). Later, chlorinated treatments were associated with the appearance of VBNC forms, both in fresh produce (Highmore et al., 2018) and in wastewater (Truchado et al., 2020). This is of particular concern because chlorine is the most commonly used sanitizer in industry and its efficacy is typically assessed using culturable bacterial counts (López-Gálvez et al., 2019). It is worth noting that bacterial adaptation to chlorine-containing disinfectants induces physicochemical and morphological changes in Lm described for other species entering the VBNC state, in particular a transition from the typical rod shape of Lm bacilli to a coccoid shape (Gao and Liu, 2014). Treatments with lower concentrations of chlorine or benzalkonium chloride also show induction of VBNC Lm (Afari and Hung, 2018; Noll et al., 2020; Truchado et al., 2020). VBNC Lm also emerged in presence of other antimicrobial agents, such as surfactants (Robben et al., 2018), preservatives (Zolfaghari et al., 2020), essential oils (De Medeiros Barbosa et al., 2020) or peracetic acid (Gu et al., 2020; Arvaniti et al., 2021). Chiara et al. (2021) interestingly showed that the origin of the bacterial cells has a significant impact on the viability and culturability of Lm against antimicrobial treatments. Thus, mild heat treatment combined or not with terpenoids mainly affected cell culturability (assessed by CFU count) rather than viability (assessed by flow cytometry, Table 1) (Chiara et al., 2021).
Some new technologies to ensure the preservation and safety of RTE without the use of disinfectant molecules, or heating treatment, may also induce a loss of cultivability of Lm while maintaining a viable subpopulation. This is suggested by experiments conducted not directly on Lm, but on the closely related non-pathogenic species Listeria innocua, to evaluate a pulsed light treatment. A significant discrepancy was observed between the number of conventional CFUs and the various viability staining parameters, suggesting that a significant proportion of bacteria shifted to the VBNC state after pulsed light treatment. The loss of cultivability was associated with oxidative stress and DNA damages, rather than cell membrane disruption or inactivation of intracellular enzymes (Kramer and Muranyi, 2014). It is important to mention that not only may VBNC Lm be induced by industrial treatment, but also tolerate higher concentrations of antimicrobials after adaptation to a first treatment (Noll et al., 2020) or upon a combination of treatments (Robben et al., 2018). In addition, VBNC forms induced by industrial cleaning products can become tolerant to antibiotics, particularly those used to treat listeriosis, such as ampicillin and gentamicin (Robben et al., 2019; Noll et al., 2020).
Lm like many other bacterial species can form biofilms, which also counteract general cleaning and disinfection procedures and make it difficult to remove this pathogen from food processing surfaces (Bridier et al., 2015). It is of note that Lm can enter a VBNC state within a biofilm (Chae and Schraft, 2001; Giao and Keevil, 2014; Brauge et al., 2018). Highmore et al. (2018) showed that VBNC Lm adhering to the surface of spinach leaves are present at junctions and stomata, a location favoring biofilm formation on the leaf surface. Despite the protective effect of the biofilm, chlorine exposure manages to induce the VBNC state within the biofilm (Highmore et al., 2018). The molecular characteristics of the bacterial surface appear to play a role in the efficiency to enter the VBNC state. For example, Brauge et al. (2018) showed that Lm mutants lacking N-acetylglucosamine in wall teichoic acids generate a higher population of VBNC in a biofilm (Brauge et al., 2018). The same team thereafter revealed that exposure of Lm biofilms on stainless steel or polyvinyl chloride to quaternary ammonium and hydrogen peroxide disinfectants reduced cultivable populations and induced VBNC populations (Brauge et al., 2020). Similarly, Overney et al. (2017) showed induction of the VBNC state in Lm biofilms formed on stainless steel after treatment with a chlorinated alkaline cleaner or quaternary ammonium disinfectant (Overney et al., 2017). Of note, in the presence of disinfectants, the difference between initial and final cell number was not significantly different when bacteria were grown in the presence of smoked salmon juice or meat exudate (Overney et al., 2017), which contrasts with the effect of the food soil itself (Overney et al., 2016) and further highlights the impact of stress induced by cleaning procedures on VBNC formation.
The studies mentioned in this section are listed in Table 5.
The hypothesis that Lm can enter a VBNC state in the host, particularly in an intracellular phase, has emerged from work in human epithelial cell infection models. The study of prolonged Lm infection in hepatocytes or trophoblast cells revealed a change in the pathogen’s intracellular lifestyle, between the well-known active phases of cytosolic replication and intercellular dissemination (Radoshevich and Cossart, 2017) and a novel so-called “persistence” phase, in which the bacteria enter into dormancy within vacuoles, named LisCVs (Listeria-containing-vacuoles) (Kortebi et al., 2017). Thus, after a few days of infection, cytosolic bacteria cease to produce the actin polymerization factor ActA and are captured by intracellular membranes in an a xenophagy-like process, forming acidic vacuoles marked by the lysosomal protein LAMP1 (Figure 1A). In these single-membrane perinuclear compartments (Figure 1B), a bacterial subpopulation is degraded, while a majority population resists stress and enters a state of slowed growth. LisCVs remain intact in dividing cells during mitosis (Figure 1C), in the same way that lysosomes do not disintegrate during mitosis, but are partitioned as separate vesicles. This ability of LisCVs to segregate in daughter cells could be a way for dormant intracellular Lm to spread during epithelial tissue renewal.
Figure 1 Evidence for an intracellular VBNC state of L. monocytogenes (adapted from (Kortebi et al., 2017). (A) Simplified diagram of the different phases of the intracellular life of Lm in epithelial cells. Bacteria are internalized into the host cell and are contained in an entry vacuole. After escape into the cytosol, bacteria multiply and produce ActA, which allows them to polymerize actin (F-actin), move into the infected cell, and spread to adjacent cells (not shown). After a few days of infection, cytosolic bacteria cease to produce ActA and are captured in membrane compartments, forming Listeria-containing vacuoles (LisCVs) marked with LAMP1 (represented by red stars). In these acidic vacuoles, a subpopulation of bacteria can resist degradation and multiply slowly, up to entry into dormancy. (B, C) Observation of LisCVs by epifluorescence microscopy in human placental JEG3 cells infected for 3 days with Lm. LisCVs labelled with LAMP1 are in red, Listeria in green, DNA (stained with DAPI) in blue, and F-actin in white. (B) A cell contains several perinuclear LisCVs. The arrow indicates a LisCV shown at higher magnification in the image on the right. Bars: 2 μm. (C) LisCVs are present in mitotic cells infected with either the wild-type Lm strain (WT, left) or a ΔactA mutant (right). Bars: 2 μm. White arrows indicate representative LisCVs. (D–F) Subculturing of JEG3 cells infected with ΔactA bacteria leads to VBNC bacteria. (D) Cells infected for 3 days were subcultured and propagated until day 13 (d13) and stained as in (C). Intracellular bacteria in LAMP1-positive compartments are indicated by arrows. At the same time, plating of infected cell lysates onto agar plates produces no colony (not shown). (E) High magnification images of non-culturable LAMP1-positive bacteria at d13. A bacterium with a division septum (s) is shown on the right. Bar: 5 μm. (F) JEG3 cells infected with non-culturable bacteria were permeabilized with 0.1% Triton X-100 and stained with SYTO9 and PI (see BacLight assay, Table 1). Intact VBNC bacteria are stained green (arrow), while damaged bacteria (*) and nuclei are stained red. “Ph.C.”: phase contrast. Bar: 1 μm. The top right squared images show higher magnifications, with one bacterium with an intact membrane (green) and three bacteria with a compromised membrane (red).
Several evidences strongly suggest that a subpopulation of bacteria enters the VBNC state in LisCVs. First, after three days of infection, the number of intracellular bacteria quantified by microscopy is much higher than that determined by the classical CFU method (Kortebi et al., 2017). Second, the Baclight Live/Dead technique (Table 1) indicates that two-thirds of the vacuolar bacteria retain their membrane integrity. Additionally, when the actA gene is kept inactive, vacuolar Listeria all cease to be culturable on agar media and can propagate during host cell mitosis (Figure 1C), without being degraded by intracellular host defense mechanisms, such as autophagy (Kortebi et al., 2017). After several passages, parasitized host cells still harbor non-culturable bacteria in LAMP1-positive compartments (Figure 1D). A few bacteria exhibit a division septum (Figure 1E) and several retain membrane integrity, as indicated by the Baclight assay (Figure 1F), which is an indication of viability. Inhibition of ActA production is necessary to maintain the VBNC state during long-term infection, as this phenotype is reversible upon re-expression of the actA gene in an ΔactA mutant. Of note, reactivation of a subpopulation of vacuolar dormant bacteria was observed randomly during host cell passages. This re-growth process occurs by a stimulus not identified to date (Kortebi et al., 2017).
Overall, these results invite to consider the possibility that Lm may generate intracellular VBNC forms that might not be detected in tissue samples by bacterial growth-based diagnostic tests. The capacity to persist in intracellular vacuoles could also be involved in Lm escape from antibiotic therapy. We found that a high-dose of gentamicin cleared cytosolic Lm but not vacuolar Lm (Kortebi et al., 2017). LisCV could thus be a niche where Lm is protected from gentamicin and other antibiotics, similar to the aminoglycoside-tolerant small colony variants of Staphylococcus aureus (Mitchell et al., 2010), or the extracellular persisters of Lm, which emerge during bacterial growth in antibiotic-supplemented broth (Knudsen et al., 2013). In addition to antibiotic tolerance, it is possible that VBNC Lm-bearing host cells are not recognized and destroyed efficiently by immune cells. Related to this idea, we recently demonstrated that the vacuolar persistence phase of Lm in hepatocytes coincided with transcriptional inhibition of genes encoding acute phase proteins, which are important effectors of innate immunity (Descoeudres et al., 2021).
The existence of VBNC Lm in vivo remains to be formally demonstrated, but some studies support the hypothesis. As early as 1948, while trying to isolate the germs responsible for bovine listeriosis, Gray et al. (1948) reported the non-culturability of Lm (Gray et al., 1948). Thus, the plating on tryptose agar of crushed brains of animals presenting clinical signs of listeriosis revealed no, or very few, bacterial colonies after 24 hours at 37°C. Surprisingly, re-spreading the same samples several months later, after storage in a refrigerator at 4°C, generated numerous colonies. As Gray and Killinger, (1966) reported in a well-documented review, this phenomenon has been described in several other studies showing that after natural or laboratory infections, culture from tissue suspension or swabs did not always reveal the presence of Lm (Gray and Killinger, 1966). It was necessary to refrigerate infected tissue for several weeks or months at low temperatures to detect the bacteria. Since that time, culture media for isolating Lm have been greatly improved, allowing more efficient culturability of the pathogen. However, these early observations are still intriguing and highlight the possibility that a listerial infection may be overlooked simply because the bacteria were not successfully isolated on the first culture attempt. Furthermore, the mechanism of the culturability enhancement effect at 4°C described by Gray et al. (1948) is not understood, and it is surprising that this phenomenon has not been further investigated. Recently, however, the existence of VBNC Lm has been evoked in a model of maternal-neonatal listeriosis in macaque monkeys, based on inconsistent results between CFU counts, which were sometimes negative on blood agar plates, while microscopy data showed Gram-positive rods in tissues (Wolfe et al., 2019). This model is of particular interest because the placentation, physiology, and immunology of pregnancy in the macaque is similar to that of the human species. A notable finding of this study is that some female macaques infected with a low inoculum of Lm were able to carry their pregnancies to term with no apparent signs of disease in either the mother or the neonate, whereas histological and cytokine examination of tissues after surgical removal revealed intrauterine signs of inflammation. This suggests the low-level impact of asymptomatic listeriosis, the subsequent consequences of which on the child are not known (Wolfe et al., 2019).
Molecular biology techniques based on PCR or DNA sequencing are currently the most effective in suggesting the presence of VBNC forms in host. From this perspective, we can cite two recent studies highlighting incidents of unusual detection of Lm DNA by metataxonomics or metagenomics approaches (Table 1). The first detected Lm DNA among all bacterial species identified in milk directly collected from the udder of healthy cows in a sterile manner (Pang et al., 2018). The second studied the placenta microbiome of women with gestational or delivery problems compared to women with normal pregnancies. The results revealed the presence of Lm DNA in one placenta, that of a mother whose pregnancy resulted in a premature birth but was not diagnosed for listeriosis (De Goffau et al., 2019). While these data do not demonstrate the existence of VBNC Lm in vivo, they do raise the importance of developing sensitive techniques to screen for Lm in at-risk humans, as well as in potentially healthy animals carrying the pathogen (see next section). As mentioned above, a PCR-based approach was much more effective in detecting Lm in the feces of healthy humans than metataxonomics or metagenomics (Hafner et al., 2021). Therefore, PCR-based approaches should be preferred to detect VBNC bacteria. However, development of sensitive viability estimation methods is required to determine the physiological status of the bacteria present in the tested samples.
VBNC forms participate in the dispersal and silent transmission of pathogens, but their ability to cause disease requires returning to an active state of virulence. This involves a conversion of dormant, non-culturable cells to an active, culturable state, a process termed “resuscitation” (Roszak et al., 1984; Baffone et al., 2006; Oliver, 2010; Ayrapetyan et al., 2018). Resuscitation is not synonymous with regrowth: a bacterium can be resurrected without dividing, and it is only after having completed the molecular program of exit from dormancy that it can divide on a nutrient-rich medium. To achieve the resuscitation of VBNC cells various physicochemical methods have been tested on different bacterial species, such as temperature shifts or supplementation with various nutrients or metabolites (Pinto et al., 2011; Li et al., 2014; Dong et al., 2020). However, in the case of Lm, most of these methods have failed to promote the resuscitation of VBNC forms (Lindback et al., 2010; Afari and Hung, 2018; Robben et al., 2018; Gu et al., 2020). The relative humidity of the environment could play a role for Lm exit from the VBNC state on leaf surfaces: resuscitation of VBNC Lm was observed when the relative humidity was close to 100%, but not in a dry environment (Dreux et al., 2007). Another study showed Lm cells heated in presence or absence of terpenoids recovered a partial culturability when inoculated on Gorgonzola rind, suggesting that VBNC cells can resuscitate under favorable conditions on food, such as higher availability of nutrients and other factors intrinsic to this food matrix (pH, water activity, relative humidity) (Chiara et al., 2021). However, these data did not exclude that the phenomenon resulted from a subpopulation of bacteria that remained culturable.
The possibility of VBNC Lm resuscitation in the host was first questioned by the experiments of Cappelier et al. (2005) showing that Lm VBNC remained avirulent in vitro, in tests of infection of human colon cells (HT-29), and in vivo, in a murine listeriosis model (Cappelier et al., 2005). Nevertheless, the same authors later observed that Listeria VBNC could resuscitate after incubation for two to six days in an embryonated chicken egg and regain their pathogenicity (Cappelier et al., 2007). Importantly, embryonated eggs allowed better recovery than non-embryonated eggs, suggesting that the substance involved in recovery is located in the chick embryo. Experiments in the worm Caenorhabditis elegans also support the hypothesis of VBNC Lm resuscitation in the host, through the observation of bacterial colonization of the intestinal tract after inoculation of VBNC forms (Highmore et al., 2018). Furthermore, in models of VBNC Lm formation in human epithelial cells, data suggest that subpopulations of unculturable Lm have the ability to return to an active growth stage, upon passages of infected host cells (Kortebi et al., 2017).
As previously mentioned, a family of proteins named Rpfs are involved in the resuscitation mechanism of Micrococcus luteus (Kaprelyants et al., 1994; Mukamolova et al., 1998) and Mycobacteria (Mukamolova et al., 2002; Rosser et al., 2017). Lm encodes two Rpf orthologs: Lmo0186 and Lmo2522 (Ravagnani et al., 2005; Pinto et al., 2013). Like the Rpf prototypes (Mukamolova et al., 2006; Telkov et al., 2006), Lm Rpf-like proteins have a lytic transglycosylase domain predicted to cleave glycosidic bonds in the peptidoglycan (PG). In agreement with this, recombinant Lmo0186 and Lmo2522 proteins have a muralytic activity on crude preparations of Lm cell walls, as well as on (NAG)3 MUF, an artificial polymer commonly used as a substrate for lysozyme (Pinto et al., 2013). However, while these enzymes demonstrate bacterial growth stimulating properties in minimal media, data indicating that they resuscitate VBNC Lm are still missing. More generally, if the muralytic activities of Rpfs are known to play a role in bacterial division, the functional mechanism of resuscitation remains to be clarified. Several hypotheses are proposed. An Rpf could act as a cytokine that, after being secreted by culturable cells, activates a receptor on the surface of VBNC cells and triggers the bacterial resuscitation process (Panutdaporn et al., 2006). Alternatively, it is not Rpf directly, but second messengers derived from PG lysis, that activate the regulatory cascade necessary for the resumption of growth, both of the local cell and, through diffusion, of adjacent bacteria (Nikitushkin et al., 2013). Another hypothesis proposes that an Rpf may be required to cleave a modified PG and release physical constraints, allowing cell growth to resume. This hypothesis is based on the observation that, in some bacterial species, the PG of VBNC cells undergoes increased total cross-linking and modifications, such as O-acetylation, which create a physical barrier for cell growth (Signoretto et al., 2000; Signoretto et al., 2002; Pfeffer et al., 2006). These hypotheses are not mutually exclusive. In any case, the conditions that trigger the expression of genes encoding Rpfs remain to be elucidated.
The asymptomatic phase of invasive listeriosis (i.e., the incubation period) can be long, particularly when associated with pregnancy [up to 3 months, (Goulet et al., 2013) (Angelo et al., 2016)], but the mechanisms associated with this asymptomatic phase are poorly understood. Furthermore, in maternal-neonatal (MN) listeriosis, women usually present with mild febrile symptoms or may even be asymptomatic (Charlier et al., 2020) and blood culture remains negative in about half of the cases tested (Charlier et al., 2017). This raises the question whether detection methods based on the culturability of the pathogen in pregnant women could miss VBNC Lm. This problem of screening could be particularly critical, should Lm cause early miscarriages. Currently, the dogma is that MN listeriosis is associated with late stages of pregnancy (i.e., second or third trimester) (Charlier et al., 2020) but miscarriages occurring before 14 weeks of amenorrhea are generally not tested for Lm. Early spontaneous abortion associated with listeriosis may therefore be underdiagnosed. In support of this hypothesis, in a recent study examining 43 patients with spontaneous abortions, two vaginal swabs were positive for Lm by PCR test, of which one was negative for Lm by culture on agar plates (Fall et al., 2020). In addition to under-diagnosis of non-culturable Lm, reactivation of these dormant bacteria after a silent phase should also be considered. Data from the 1960s suggested that Lm present in the reproductive tract could reactivate during pregnancy and cause early fetal loss (Rappaport et al., 1960; Dungal, 1961; Gray et al., 1963; Gray and Killinger, 1966). Experimental work in a laboratory model of feto-placental listeriosis in rabbits supported this hypothesis. After inoculation with Lm, some rabbits showed spontaneous abortions with isolation of the bacterium, and after a novel gestation without further inoculation of the pathogen, could show reproductive failure during the following months (Gray et al., 1955). These observations opened the hypothesis that Lm could persist in the reproductive tract for a relatively long period of time without signs of disease, and trigger infection in subsequent pregnancies. This has however not been investigated further. As mentioned previously, recent data suggest that VBNC Lm might be present in the fetoplacental unit in a listeriosis model in macaques (Wolfe et al., 2019). It seems important to reconsider the notion of persistence of unculturable Lm in the female genital tract and subsequently in recurrent pregnancy losses (RPL). RPL is defined as three or more consecutive pregnancy losses before 20 weeks’ gestation. Its etiology is often unclear and may be multifactorial (Pandey et al., 2005). A role for latent listeriosis in this condition has been suggested (Rappaport et al., 1960; Dungal, 1961; Romana et al., 1989), but more research is needed to validate or refute this hypothesis.
Outside of pregnancy cases, Lm is so far not described as a pathogen causing subclinical silent infections, but we cannot exclude that this bacterium hides in the organism for long periods of time, especially in a VBNC state within an intracellular vacuole (Bierne et al., 2018), as has been described for Mtb. High-risk factors for sporadic non-perinatal listeriosis are primarily immunosuppressive diseases (Friesema et al., 2015; Charlier et al., 2017). The awakening of dormant intracellular Lm, long after ingestion of contaminated food, should be investigated as a potential cause of sporadic listeriosis cases. The existence of a dormant subpopulation of Lm could also be implicated in cases of recurrent listeriosis (McLauchlin et al., 1991; Sauders et al., 2001). For example, in a cancer immunotherapy approach using an attenuated Lm strain as a vaccine vector, the inoculated strain led to bacteremia 31 months after the initial injection, despite the administration of antibiotic therapy in the patient (Fares et al., 2018).This strain was never detected in the patient’s medical follow-up, as the blood culture remained negative. This phenomenon remains unexplained, but the investigators point to the fact that the patient had prostheses that may favor the formation of a Lm biofilm. While Lm is rarely implicated in prosthetic joint infections, there are now increasing reports of cases, particularly in immunocompromised individuals (Charlier et al., 2012) and chronic infections with Lm linked to these prostheses have been reported (Kleemann et al., 2009; Muchaamba et al., 2020; Hutchins et al., 2021). It is possible that the molecular mechanisms of fracture repair cause a momentary suppression of local immunity, while the development of a biofilm on the joint offers Lm protection from antibiotic treatment (Fares et al., 2018). In addition, the settlement of Lm in the VBNC state, either in an extracellular biofilm or within intracellular vacuoles, could contribute to this asymptomatic persistence and evasion of antibiotic therapy. These hypotheses, as well as the mechanism leading to the re-emergence of the persistent strain after such a long period of asymptomatic carriage, remain to be explored.
The spread of human infectious diseases emerging from animal reservoirs, such as avian influenza caused by the H5N1 virus and Covid-19 caused by the SARS-CoV-2 virus, have brought to the forefront the fact that zoonotic diseases represent a major public health risk. It has also become clear that the asymptomatic carriage plays a key role in the transmission of these dangerous pathogens. Because of its ubiquity in the environment, animals, and humans, which are the three pillars of the One Health continuum, as well as in the agri-food industry that connects them (Figure 2), Lm represents an exceptional bacterial model to address these issues, including the mechanism of circulation of this pathogen in a chain of contamination involving different ecosystems. In addition to vegetative culturable Lm cells, dormant VBNC Lm need further investigation, as entry and exit from the VBNC state is likely one of the key mechanisms for Lm adaptation to stress in these different ecological niches, as are spores for spore-forming bacterial species. Since VBNC Lm forms are ubiquitous in the One Health continuum (Figure 2), their non-detection by conventional analytical methods poses a threat to public health and food safety. In the future, the implementation of a One Health approach to assess the impact of VBNC Lm in infection should be based on: (i) a more systematic sampling process in interconnected systems, which are animal and plant production systems (farms and their immediate environment), food production systems (food processing/packaging sites and food stores), and medical and hospital systems (samples from symptomatic listeriosis patients and at-risk asymptomatic individuals), (ii) the use of diagnostic methods without growth-based enrichment steps, to detect the presence of unculturable Lm, and (iii) whole-genome sequencing of isolated strains and comparative genomics, in order to determine which strains are most likely to enter the VBNC state, their ability to circulate, and in which ecosystem.
Figure 2 The VBNC state of L. monocytogenes in the One Health continuum. (1) Lm is present in a wide range of environmental ecosystems (e.g., sea, rivers, lakes, soil, plants, fodder, and possibly within unicellular eukaryotes), either in a vegetative culturable state (VC) or in a viable but non-culturable (VBNC) dormant state that allows long-term survival under hostile conditions. (2) VC and VBNC bacteria are ingested by animals, in particular farmed and wild mammals, via food or water. (3) VBNC forms could be reactivated into VC forms in the gut by a resuscitation process. Active VC forms can lead to invasive infection or enter a steady state with the host, resulting in long-term asymptomatic carriage. Entry of bacteria into the VBNC state could contribute to this asymptomatic carriage, by promoting silent colonization of the gastrointestinal tract (gut-liver axis) or the female reproductive system. Resuscitation of dormant VBNC Lm in an asymptomatic host would reactivate a latent infection, leading to listeriosis or, if the infection is controlled by the host, to shedding of Lm into the environment. (4) Excretion of Lm from animals, in feces, abortive products or cadavers, releases the pathogen into the environment, initiating a new cycle. (5) During processing of animal or plant products, Lm can contaminate food industry production lines. Antimicrobial treatments and/or biofilm formation favor the appearance of VBNC bacteria and their persistence on surfaces, process wash waters, or in food matrices. VBNC Lm can thus contaminate raw or ready-to-eat foods. (6) Humans become infected by eating foods contaminated with VC or VBNC Lm. (7) As in animals, resuscitation of VBNC Lm in the gut might cause invasive listeriosis, mainly in at-risk populations (pregnant women, the elderly or immunocompromised persons). The VC Lm forms might also switch to the VBNC state in tissues, resulting in asymptomatic colonization of healthy carriers or a latent infection phase in at-risk individuals. (8) Human shedding of Lm releases the pathogen into the environment.
In the context of the food industry, safety standards concerning Lm contamination are all based on the growth capacity of this pathogen via the determination of CFU, both in the United States, with a zero-tolerance policy, and in Europe, with slightly less strict criteria for certain categories of RTE foods considered low risk (EC Regulation 2073/2005). These standards do not take into account the potential risk related to the presence of VBNC forms. As eradication of VBNC bacteria is difficult, their presence should be taken into account in risk assessment modeling and health regulation issues. In the context of VBNC bacteria in the host, many questions arise. For example, what are the physiological similarities and differences between bacteria in the VBNC state in an environmental niche and in a host? Are there strain-specific variations, with hypervirulent clones also more prone to silently persist as VBNC forms in the host, compared to hypovirulent clones? Do VBNC Lm survive asymptomatically in a host while being silently excreted through fecal or uterine discharges until the host dies, or can they reactivate in the same individual when the immune system is weakened, which would be consistent with latent listeriosis (Figure 2)? What would be the mechanisms of immune tolerance by the host of these VBNC bacterial parasites? What are the reservoir tissues (e.g., intestine, liver, gallbladder, endometrium)? What are the reservoir hosts (including protozoa)? Answering these questions will require clinically relevant animal models and overcoming the problem of obtaining tissue samples from asymptomatic hosts. Additionally, in medicine, as in the agri-food industry, several challenges remain to be addressed, first of all, the development of sensitive, easy-to-use and inexpensive techniques to facilitate large-scale detection and tracing of VBNC Lm. Detection techniques based on PCR, DNA sequencing, or mass spectrometry, are improving and their development is crucial, as is the development of techniques demonstrating the viability of these non-culturable microorganisms. Second, it is necessary to identify the factors affecting the recovery and regrowth of VBNC cells. Finally, the pathogenic potential of VBNC forms must be properly assessed.
AL, HB: Writing–original draft preparation. AL, EM, HB: Writing–review and editing. AL, EM: Table preparation. HB: Figure preparation. EM, HB: Funding acquisition. HB: Supervision and Conceptualization. All authors contributed to the article and approved the submitted version.
This work was funded by grants from ANR PERMALI (N°ANR-20-CE35-0001-01) and iXcore Foundation (2015) to HB, and INRAE-MICA division (AAP 2019-2020) to EM.
The authors declare that the research was conducted in the absence of any commercial or financial relationships that could be construed as a potential conflict of interest.
All claims expressed in this article are solely those of the authors and do not necessarily represent those of their affiliated organizations, or those of the publisher, the editors and the reviewers. Any product that may be evaluated in this article, or claim that may be made by its manufacturer, is not guaranteed or endorsed by the publisher.
We are very grateful to Lilliana Radoshevich (University of Iowa) for critical reading and corrections of this manuscript. We would also like to thank Lise Chaumont, Filipe Carvalho, Alessandro Pagliuso, and Mounia Kortebi, for helpful discussions. We would like to thank the INRAE-MICA division, the iXcore Research Foundation, ANR and Université Paris Saclay for financial support.
Afari, G. K., Hung, Y. C. (2018). Detection and Verification of the Viable But Nonculturable (VBNC) State of Escherichia Coli O157:H7 and Listeria Monocytogenes Using Flow Cytometry and Standard Plating. J. Food Sci. 83, 1913–1920. doi: 10.1111/1750-3841.14203
Angelo, K. M., Jackson, K. A., Wong, K. K., Hoekstra, R. M., Jackson, B. R. (2016). Assessment of the Incubation Period for Invasive Listeriosis. Clin. Infect. Dis. 63, 1487–1489. doi: 10.1093/cid/ciw569
Arvaniti, M., Tsakanikas, P., Papadopoulou, V., Giannakopoulou, A., Skandamis, P. (2021). Listeria Monocytogenes Sublethal Injury and Viable-But-Nonculturable State Induced by Acidic Conditions and Disinfectants. Microbiol. Spectr. 9, e0137721. doi: 10.1128/Spectrum.01377-21
Ayrapetyan, M., Williams, T. C., Baxter, R., Oliver, J. D. (2015). Viable But Nonculturable and Persister Cells Coexist Stochastically and Are Induced by Human Serum. Infect. Immun. 83, 4194–4203. doi: 10.1128/IAI.00404-15
Ayrapetyan, M., Williams, T., Oliver, J. D. (2018). Relationship Between the Viable But Nonculturable State and Antibiotic Persister Cells. J. Bacteriol. 200, 1–15. doi: 10.1128/JB.00249-18
Baffone, W., Casaroli, A., Citterio, B., Pierfelici, L., Campana, R., Vittoria, E., et al. (2006). Campylobacter Jejuni Loss of Culturability in Aqueous Microcosms and Ability to Resuscitate in a Mouse Model. Int. J. Food Microbiol. 107, 83–91. doi: 10.1016/j.ijfoodmicro.2005.08.015
Bagatella, S., Tavares-Gomes, L., Oevermann, A. (2021). Listeria Monocytogenes at the Interface Between Ruminants and Humans: A Comparative Pathology and Pathogenesis Review. Vet. Pathol., 3009858211052659. doi: 10.1177/03009858211052659
Bernardo, R., Duarte, A., Tavares, L., Barreto, A. S., Henriques, A. R. (2021). Listeria Monocytogenes Assessment in a Ready-To-Eat Salad Shelf-Life Study Using Conventional Culture-Based Methods, Genetic Profiling, and Propidium Monoazide Quantitative PCR. Foods 10 (235), 1–14. doi: 10.3390/foods10020235
Besnard, V., Federighi, M., Cappelier, J. M. (2000). Development of a Direct Viable Count Procedure for the Investigation of VBNC State in Listeria Monocytogenes. Lett. Appl. Microbiol. 31, 77–81. doi: 10.1046/j.1472-765x.2000.00771.x
Besnard, V., Federighi, M., Declerq, E., Jugiau, F., Cappelier, J. M. (2002). Environmental and Physico-Chemical Factors Induce VBNC State in Listeria Monocytogenes. Vet. Res. 33, 359–370. doi: 10.1051/vetres:2002022
Bierne, H., Milohanic, E., Kortebi, M. (2018). To Be Cytosolic or Vacuolar: The Double Life of Listeria Monocytogenes. Front. Cell. Infect. Microbiol. 8, 136. doi: 10.3389/fcimb.2018.00136
Brauge, T., Faille, C., Leleu, G., Denis, C., Hanin, A., Midelet, G. (2020). Treatment With Disinfectants may Induce an Increase in Viable But Non Culturable Populations of Listeria Monocytogenes in Biofilms Formed in Smoked Salmon Processing Environments. Food Microbiol. 92, 103548. doi: 10.1016/j.fm.2020.103548
Brauge, T., Faille, C., Sadovskaya, I., Charbit, A., Benezech, T., Shen, Y., et al. (2018). The Absence of N-Acetylglucosamine in Wall Teichoic Acids of Listeria Monocytogenes Modifies Biofilm Architecture and Tolerance to Rinsing and Cleaning Procedures. PloS One 13, e0190879. doi: 10.1371/journal.pone.0190879
Bremer, P. J., Osborne, C. M., Kemp, R. A., Smith, J. J. (1998). Survival of Listeria Monocytogenes in Sea Water and Effect of Exposure on Thermal Resistance. J. Appl. Microbiol. 85, 545–553. doi: 10.1046/j.1365-2672.1998.853533.x
Bridier, A., Sanchez-Vizuete, P., Guilbaud, M., Piard, J. C., Naitali, M., Briandet, R. (2015). Biofilm-Associated Persistence of Food-Borne Pathogens. Food Microbiol. 45, 167–178. doi: 10.1016/j.fm.2014.04.015
Buchanan, R. L., Gorris, L. G. M., Hayman, M. M., Jackson, T. C., Whiting, R. C. (2017). A Review of Listeria Monocytogenes: An Update on Outbreaks, Virulence, Dose-Response, Ecology, and Risk Assessments. Food Control 75, 1–13. doi: 10.1016/j.foodcont.2016.12.016
Cappelier, J. M., Besnard, V., Roche, S., Garrec, N., Zundel, E., Velge, P., et al. (2005). Avirulence of Viable But Non-Culturable Listeria Monocytogenes Cells Demonstrated by In Vitro and In Vivo Models. Vet. Res. 36, 589–599. doi: 10.1051/vetres:2005018
Cappelier, J. M., Besnard, V., Roche, S. M., Velge, P., Federighi, M. (2007). Avirulent Viable But Non Culturable Cells of Listeria Monocytogenes Need the Presence of an Embryo to Be Recovered in Egg Yolk and Regain Virulence After Recovery. Vet. Res. 38, 573–583. doi: 10.1051/vetres:2007017
Chae, M., Schraft, H. (2001). Cell Viability of Listeria Monocytogenes Biofilms. Food Microbiol. 18, 103–112. doi: 10.1006/fmic.2000.0374
Charlier, C., Disson, O., Lecuit, M. (2020). Maternal-Neonatal Listeriosis. Virulence 11, 391–397. doi: 10.1080/21505594.2020.1759287
Charlier, C., Leclercq, A., Cazenave, B., Desplaces, N., Travier, L., Cantinelli, T., et al. (2012). Listeria Monocytogenes-Associated Joint and Bone Infections: A Study of 43 Consecutive Cases. Clin. Infect. Dis. 54, 240–248. doi: 10.1093/cid/cir803
Charlier, C., Perrodeau, E., Leclercq, A., Cazenave, B., Pilmis, B., Henry, B., et al. (2017). Clinical Features and Prognostic Factors of Listeriosis: The MONALISA National Prospective Cohort Study. Lancet Infect. Dis. 17, 510–519. doi: 10.1016/S1473-3099(16)30521-7
Chiara, M., Giulia, T., Federica, B., Diego, M., Robin, D., Fausto, G., et al. (2021). Listeria Monocytogenes Sensitivity to Antimicrobial Treatments Depends on Cell Origin. Sci. Rep. 11, 21263. doi: 10.1038/s41598-021-00767-9
Colburn, K. G., Kaysner, C. A., Abeyta, C., Jr., Wekell, M. M. (1990). Listeria Species in a California Coast Estuarine Environment. Appl. Environ. Microbiol. 56, 2007–2011. doi: 10.1128/aem.56.7.2007-2011.1990
Cunningham, E., O’byrne, C., Oliver, J. D. (2009). Effect of Weak Acids on Listeria Monocytogenes Survival: Evidence for a Viable But Nonculturable State in Response to Low pH. Food Control 20, 1141–1144. doi: 10.1016/j.foodcont.2009.03.005
De Goffau, M. C., Lager, S., Sovio, U., Gaccioli, F., Cook, E., Peacock, S. J., et al. (2019). Human Placenta Has No Microbiome But Can Contain Potential Pathogens. Nature 572, 329–334. doi: 10.1038/s41586-019-1451-5
De Medeiros Barbosa, I., Tayse Da Cruz Almeida, E., Alves Gomes, A. C., Leite De Souza, E. (2020). Evidence on the Induction of Viable But Non-Culturable State in Listeria Monocytogenes by Origanum Vulgare L. And Rosmarinus Officinalis L. Essential Oils in a Meat-Based Broth. Innov. Food Sci. Emerg. Technol. 62, 1–10. doi: 10.1016/j.ifset.2020.102351
Descoeudres, N., Jouneau, L., Henry, C., Gorrichon, K., Derré-Bobillot, A., Serror, P., et al. (2021). An Immunomodulatory Transcriptional Signature Associated With Persistent Listeria Infection in Hepatocytes. Front. Cell. Infect. Microbiol. 11, 761945. doi: 10.3389/fcimb.2021.761945
Desneux, J., Biscuit, A., Picard, S., Pourcher, A. M. (2016). Fate of Viable But Non-Culturable Listeria Monocytogenes in Pig Manure Microcosms. Front. Microbiol. 7, 245. doi: 10.3389/fmicb.2016.00245
Dong, K., Pan, H., Yang, D., Rao, L., Zhao, L., Wang, Y., et al. (2020). Induction, Detection, Formation, and Resuscitation of Viable But Non-Culturable State Microorganisms. Compr. Rev. Food Sci. Food Saf. 19, 149–183. doi: 10.1111/1541-4337.12513
Dorey, A., Marinho, C., Piveteau, P., O'byrne, C. (2019). Role and Regulation of the Stress Activated Sigma Factor Sigma B (Sigma(B)) in the Saprophytic and Host-Associated Life Stages of Listeria Monocytogenes. Adv. Appl. Microbiol. 106, 1–48. doi: 10.1016/bs.aambs.2018.11.001
Dreux, N., Albagnac, C., Federighi, M., Carlin, F., Morris, C. E., Nguyen-The, C. (2007). Viable But Non-Culturable Listeria Monocytogenes on Parsley Leaves and Absence of Recovery to a Culturable State. J. Appl. Microbiol. 103, 1272–1281. doi: 10.1111/j.1365-2672.2007.03351.x
Dungal, N. (1961). Listeriosis in Four Siblings. Lancet 2, 513–516. doi: 10.1016/S0140-6736(61)92955-5
Dutta, N. K., Karakousis, P. C. (2014). Latent Tuberculosis Infection: Myths, Models, and Molecular Mechanisms. Microbiol. Mol. Biol. Rev. 78, 343–371. doi: 10.1128/MMBR.00010-14
European Food Safety Authority, European Centre for Disease Prevention and Control (2021). The European Union One Health 2019 Zoonoses Report. EFSA J. 19, e06406. doi: 10.2903/j.efsa.2021.6406
Fall, N. S., Sarr, M., Diagne, N., Bassene, H., Sokhna, C., Lagier, J. C., et al. (2020). Listeria Monocytogenes Detected in Vaginal Self-Samples of 2 Women After Spontaneous Miscarriage, Senegal, West Africa. Eur. J. Clin. Microbiol. Infect. Dis. 39, 393–394. doi: 10.1007/s10096-019-03739-0
Fares, E., Mccloskey, C. B., Gutierrez, A., Princiotta, M., Salinas, L. J., Drevets, D. A. (2018). Vaccine Strain Listeria Monocytogenes Bacteremia Occurring 31 Months After Immunization. Infection. 47, 489–492. doi: 10.1007/s15010-018-1249-7
Fleischmann, S., Robben, C., Alter, T., Rossmanith, P., Mester, P. (2021). How to Evaluate Non-Growing Cells-Current Strategies for Determining Antimicrobial Resistance of VBNC Bacteria. Antibiotics (Basel) 10 (115), 1–24. doi: 10.3390/antibiotics10020115
Fredriksson-Ahomaa, M., London, L., Skrzypczak, T., Kantala, T., Laamanen, I., Bistrom, M., et al. (2020). Foodborne Zoonoses Common in Hunted Wild Boars. Ecohealth 17, 512–522. doi: 10.1007/s10393-020-01509-5
Friesema, I. H., Kuiling, S., van der Ende, A., Heck, M. E., Spanjaard, L., Van Pelt, W. (2015). Risk Factors for Sporadic Listeriosis in the Netherlands 2008 to 2013. Eurosurveillance 20, 15–19. doi: 10.2807/1560-7917.ES2015.20.31.21199
Gahan, C. G., Hill, C. (2014). Listeria Monocytogenes: Survival and Adaptation in the Gastrointestinal Tract. Front. Cell. Infect. Microbiol. 4, 9. doi: 10.3389/fcimb.2014.00009
Gample, S. P., Agrawal, S., Sarkar, D. (2019). Evidence of Nitrite Acting as a Stable and Robust Inducer of Non-Cultivability in Mycobacterium Tuberculosis With Physiological Relevance. Sci. Rep. 9, 9261. doi: 10.1038/s41598-019-45652-8
Gao, H., Liu, C. (2014). Biochemical and Morphological Alteration of Listeria Monocytogenes Under Environmental Stress Caused by Chloramine-T and Sodium Hypochlorite. Food Control 46, 455–461. doi: 10.1016/j.foodcont.2014.05.016
Gengenbacher, M., Kaufmann, S. H. (2012). Mycobacterium Tuberculosis: Success Through Dormancy. FEMS Microbiol. Rev. 36, 514–532. doi: 10.1111/j.1574-6976.2012.00331.x
Giao, M. S., Keevil, C. W. (2014). Listeria Monocytogenes Can Form Biofilms in Tap Water and Enter Into the Viable But Non-Cultivable State. Microb. Ecol. 67, 603–611. doi: 10.1007/s00248-013-0364-3
Giovannini, A., Cancellotti, F. M., Turilli, C., Randi, E. (1988). Serological Investigations for Some Bacterial and Viral Pathogens in Fallow Deer (Cervus Dama) and Wild Boar (Sus Scrofa) of the San Rossore Preserve, Tuscany, Italy. J. Wildl. Dis. 24, 127–132. doi: 10.7589/0090-3558-24.1.127
Gollan, B., Grabe, G., Michaux, C., Helaine, S. (2019). Bacterial Persisters and Infection: Past, Present, and Progressing. Annu. Rev. Microbiol. 73, 359–385. doi: 10.1146/annurev-micro-020518-115650
Goncalves, F. D., De Carvalho, C. C. (2016). Phenotypic Modifications in Staphylococcus Aureus Cells Exposed to High Concentrations of Vancomycin and Teicoplanin. Front. Microbiol. 7, 13. doi: 10.3389/fmicb.2016.00013
Goulet, V., King, L. A., Vaillant, V., De Valk, H. (2013). What Is the Incubation Period for Listeriosis? BMC Infect. Dis. 13, 11. doi: 10.1186/1471-2334-13-11
Gray, M. L., Killinger, A. H. (1966). Listeria Monocytogenes and Listeric Infections. Bacteriol. Rev. 30, 309–382. doi: 10.1128/br.30.2.309-382.1966
Gray, M. L., Seeliger, H. P. R., Potel, J. (1963). Perinatal Infections Due to Listeria Monocytogenes. Do These Affect Subsequent Pregancies ? Clin. Pediatr. 2, 614–623.
Gray, M. L., Singh, C., Thorp, F., Jr. (1955). Abortion, Stillbirth, Early Death of Young in Rabbits by Listeria Monocytogenes. I. Ocular Instillation. Proc. Soc. Exp. Biol. Med. 89, 163–169. doi: 10.3181/00379727-89-21746
Gray, M. L., Stafseth, H. J., Thorp, F., Sholl, L. B., Riley, W. F. (1948). A New Technique for Isolating Listerellae From the Bovine Brain. J. Bacteriol. 55, 471–476. doi: 10.1128/jb.55.4.471-476.1948
Grif, K., Hein, I., Wagner, M., Brandl, E., Mpamugo, O., Mclauchlin, J., et al. (2001). Prevalence and Characterization of Listeria Monocytogenes in the Feces of Healthy Austrians. Wien. Klin. Wochenschr. 113, 737–742.
Grif, K., Patscheider, G., Dierich, M. P., Allerberger, F. (2003). Incidence of Fecal Carriage of Listeria Monocytogenes in Three Healthy Volunteers: A One-Year Prospective Stool Survey. Eur. J. Clin. Microbiol. Infect. Dis. 22, 16–20. doi: 10.1007/s10096-002-0835-9
Gu, G., Bolten, S., Mowery, J., Luo, Y., Gulbronson, C., Nou, X. (2020). Susceptibility of Foodborne Pathogens to Sanitizers in Produce Rinse Water and Potential Induction of Viable But Non-Culturable State. Food Control 112, 107138. doi: 10.1016/j.foodcont.2020.107138
Hafner, L., Pichon, M., Burucoa, C., Nusser, S. H. A., Moura, A., Garcia-Garcera, M., et al. (2021). Listeria Monocytogenes Faecal Carriage Is Common and Depends on the Gut Microbiota. Nat. Commun. 12, 6826. doi: 10.1038/s41467-021-27069-y
Highmore, C. J., Warner, J. C., Rothwell, S. D., Wilks, S. A., Keevil, C. W. (2018). Viable-But-Nonculturable Listeria Monocytogenes and Salmonella Enterica Serovar Thompson Induced by Chlorine Stress Remain Infectious. mBio 9, 1–12. doi: 10.1128/mBio.00540-18
Hurtado, A., Ocejo, M., Oporto, B. (2017). Salmonella Spp. And Listeria Monocytogenes Shedding in Domestic Ruminants and Characterization of Potentially Pathogenic Strains. Vet. Microbiol. 210, 71–76. doi: 10.1016/j.vetmic.2017.09.003
Hutchins, C., Sayavedra, L., Diaz, M., Gupta, P., Tissingh, E., Elumogo, C., et al. (2021). Genomic Analysis of a Rare Recurrent Listeria Monocytogenes Prosthetic Joint Infection Indicates a Protected Niche Within Biofilm on Prosthetic Materials. Sci. Rep. 11, 21864. doi: 10.1038/s41598-021-01376-2
Hydeskov, H. B., Amar, C. F. L., Fernandez, J. R., John, S. K., Macgregor, S. K., Cunningham, A. A., et al. (2019). Listeria Monocytogenes Infection of Free-Living Western European Hedgehogs (Erinaceus Europaeus). J. Zoo Wildl. Med. 50, 183–189. doi: 10.1638/2018-0093
Iida, T., Kanzaki, M., Nakama, A., Kokubo, Y., Maruyama, T., Kaneuchi, C. (1998). Detection of Listeria Monocytogenes in Humans, Animals and Foods. J. Vet. Med. Sci. 60, 1341–1343. doi: 10.1292/jvms.60.1341
Inoue, S., Iida, T., Tanikawa, T., Maruyama, T., Morita, C. (1991). Isolation of Listeria Monocytogenes From Roof Rats (Rattus Rattus) in Buildings in Tokyo. J. Vet. Med. Sci. 53, 521–522. doi: 10.1292/jvms.53.521
Kaprelyants, A. S., Gottschal, J. C., Kell, D. B. (1993). Dormancy in Non-Sporulating Bacteria. FEMS Microbiol. Rev. 10, 271–285. doi: 10.1111/j.1574-6968.1993.tb05871.x
Kaprelyants, A. S., Mukamolova, G. V., Kell, D. B. (1994). Estimation of Dormant Micrococcus Luteus Cells by Penicillin Lysis and by Resuscitation in Cell-Free Spent Culture Medium at High Dilution. FEMS Microbiol. Lett. 115, 347–352. doi: 10.1111/j.1574-6968.1994.tb06662.x
Kaptchouang Tchatchouang, C. D., Fri, J., De Santi, M., Brandi, G., Schiavano, G. F., Amagliani, G., et al. (2020). Listeriosis Outbreak in South Africa: A Comparative Analysis With Previously Reported Cases Worldwide. Microorganisms 8 (135), 1–18. doi: 10.3390/microorganisms8010135
Kleemann, P., Domann, E., Chakraborty, T., Bernstein, I., Lohoff, M. (2009). Chronic Prosthetic Joint Infection Caused by Listeria Monocytogenes. J. Med. Microbiol. 58, 138–141. doi: 10.1099/jmm.0.004234-0
Klein, M., Brown, L., Ashbolt, N. J., Stuetz, R. M., Roser, D. J. (2011). Inactivation of Indicators and Pathogens in Cattle Feedlot Manures and Compost as Determined by Molecular and Culture Assays. FEMS Microbiol. Ecol. 77, 200–210. doi: 10.1111/j.1574-6941.2011.01098.x
Knudsen, G. M., Ng, Y., Gram, L. (2013). Survival of Bactericidal Antibiotic Treatment by a Persister Subpopulation of Listeria Monocytogenes. Appl. Environ. Microbiol. 79, 7390–7397. doi: 10.1128/AEM.02184-13
Kortebi, M., Milohanic, E., Mitchell, G., Pechoux, C., Prevost, M. C., Cossart, P., et al. (2017). Listeria Monocytogenes Switches From Dissemination to Persistence by Adopting a Vacuolar Lifestyle in Epithelial Cells. PloS Pathog. 13, e1006734. doi: 10.1371/journal.ppat.1006734
Kramer, B., Muranyi, P. (2014). Effect of Pulsed Light on Structural and Physiological Properties of Listeria Innocua and Escherichia Coli. J. Appl. Microbiol. 116, 596–611. doi: 10.1111/jam.12394
Kumar, S. S., Ghosh, A. R. (2019). Assessment of Bacterial Viability: A Comprehensive Review on Recent Advances and Challenges. Microbiology. 13, 706–721. doi: 10.1099/mic.0.000786
Lamont, R. J., Postlethwaite, R. (1986). Carriage of Listeria Monocytogenes and Related Species in Pregnant and Non-Pregnant Women in Aberdeen, Scotland. J. Infect. 13, 187–193. doi: 10.1016/S0163-4453(86)93121-X
Leclercq, A., Oevermann, A., Danguy-Des-DeíSerts, R., Granier, S., Hammack, T., Jinneman, K., et al. (2014). “Listeria Monocytogenes,” in OIE Terrestrial Manual (Paris: World Organization for Animal Health (Oie)).
Li, L., Mendis, N., Trigui, H., Oliver, J. D., Faucher, S. P. (2014). The Importance of the Viable But Non-Culturable State in Human Bacterial Pathogens. Front. Microbiol. 5, 258. doi: 10.3389/fmicb.2014.00258
Lindback, T., Rottenberg, M. E., Roche, S. M., Rorvik, L. M. (2010). The Ability to Enter Into an Avirulent Viable But Non-Culturable (VBNC) Form Is Widespread Among Listeria Monocytogenes Isolates From Salmon, Patients and Environment. Vet. Res. 41, 8. doi: 10.1051/vetres/2009056
López-Gálvez, F., Tudela, J. A., Allende, A., Gil, M. I. (2019). Microbial and Chemical Characterization of Commercial Washing Lines of Fresh Produce Highlights the Need for Process Water Control. Innov. Food Sci. Emerg. Technol. 51, 211–219. doi: 10.1016/j.ifset.2018.05.002
Low, J. C., Donachie, W. (1997). A Review of Listeria Monocytogenes and Listeriosis. Vet. J. 153, 9–29. doi: 10.1016/S1090-0233(97)80005-6
Lyautey, E., Hartmann, A., Pagotto, F., Tyler, K., Lapen, D. R., Wilkes, G., et al. (2007). Characteristics and Frequency of Detection of Fecal Listeria Monocytogenes Shed by Livestock, Wildlife, and Humans. Can. J. Microbiol. 53, 1158–1167. doi: 10.1139/W07-084
Macgowan, A. P., Marshall, R. J., Mackay, I. M., Reeves, D. S. (1991). Listeria Faecal Carriage by Renal Transplant Recipients, Haemodialysis Patients and Patients in General Practice: Its Relation to Season, Drug Therapy, Foreign Travel, Animal Exposure and Diet. Epidemiol. Infect. 106, 157–166. doi: 10.1017/S0950268800056521
Mascola, L., Sorvillo, F., Goulet, V., Hall, B., Weaver, R., Linnan, M. (1992). Fecal Carriage of Listeria Monocytogenes–Observations During a Community-Wide, Common-Source Outbreak. Clin. Infect. Dis. 15, 557–558. doi: 10.1093/clind/15.3.557
Matereke, L. T., Okoh, A. I. (2020). Listeria Monocytogenes Virulence, Antimicrobial Resistance and Environmental Persistence: A Review. Pathogens 9 (528), 1–12. doi: 10.3390/pathogens9070528
Maynaud, G., Pourcher, A. M., Ziebal, C., Cuny, A., Druilhe, C., Steyer, J. P., et al. (2016). Persistence and Potential Viable But Non-Culturable State of Pathogenic Bacteria During Storage of Digestates From Agricultural Biogas Plants. Front. Microbiol. 7, 1469. doi: 10.3389/fmicb.2016.01469
Mclauchlin, J., Aird, H., Amar, C., Barker, C., Dallman, T., Lai, S., et al. (2021). An Outbreak of Human Listeriosis Associated With Frozen Sweet Corn Consumption: Investigations in the UK. Int. J. Food Microbiol. 338, 108994. doi: 10.1016/j.ijfoodmicro.2020.108994
Mclauchlin, J., Audurier, A., Taylor, A. G. (1991). Treatment Failure and Recurrent Human Listeriosis. J. Antimicrob. Chemother. 27, 851–857. doi: 10.1093/jac/27.6.851
Mitchell, G., Brouillette, E., Seguin, D. L., Asselin, A. E., Jacob, C. L., Malouin, F. (2010). A Role for Sigma Factor B in the Emergence of Staphylococcus Aureus Small-Colony Variants and Elevated Biofilm Production Resulting From an Exposure to Aminoglycosides. Microb. Pathog. 48, 18–27. doi: 10.1016/j.micpath.2009.10.003
Moreno, Y., Sánchez-Contreras, J., Montes, R. M., García-Hernández, J., Ballesteros, L., Ferrús, M. A. (2012). Detection and Enumeration of Viable Listeria Monocytogenes Cells From Ready-to-Eat and Processed Vegetable Foods by Culture and DVC-FISH. Food Control 27, 374–379. doi: 10.1016/j.foodcont.2012.04.017
Muchaamba, F., Eshwar, A. K., Von Ah, U., Stevens, M. J. A., Tasara, T. (2020). Evolution of Listeria Monocytogenes During a Persistent Human Prosthetic Hip Joint Infection. Front. Microbiol. 11, 1726. doi: 10.3389/fmicb.2020.01726
Mukamolova, G. V., Kaprelyants, A. S., Young, D. I., Young, M., Kell, D. B. (1998). A Bacterial Cytokine. Proc. Natl. Acad. Sci. U. S. A. 95, 8916–8921. doi: 10.1073/pnas.95.15.8916
Mukamolova, G. V., Murzin, A. G., Salina, E. G., Demina, G. R., Kell, D. B., Kaprelyants, A. S., et al. (2006). Muralytic Activity of Micrococcus Luteus Rpf and Its Relationship to Physiological Activity in Promoting Bacterial Growth and Resuscitation. Mol. Microbiol. 59, 84–98. doi: 10.1111/j.1365-2958.2005.04930.x
Mukamolova, G. V., Turapov, O. A., Young, D. I., Kaprelyants, A. S., Kell, D. B., Young, M. (2002). A Family of Autocrine Growth Factors in Mycobacterium Tuberculosis. Mol. Microbiol. 46, 623–635. doi: 10.1046/j.1365-2958.2002.03184.x
Muller, H. E. (1990). Listeria Isolations From Feces of Patients With Diarrhea and From Healthy Food Handlers. Infection 18, 97–99. doi: 10.1007/BF01641423
Nikitushkin, V. D., Demina, G. R., Shleeva, M. O., Kaprelyants, A. S. (2013). Peptidoglycan Fragments Stimulate Resuscitation of "Non-Culturable" Mycobacteria. Antonie Van Leeuwenhoek 103, 37–46. doi: 10.1007/s10482-012-9784-1
Noll, M., Trunzer, K., Vondran, A., Vincze, S., Dieckmann, R., Al Dahouk, S., et al. (2020). Benzalkonium Chloride Induces a VBNC State in Listeria Monocytogenes. Microorganisms 8 (184), 1–10. doi: 10.3390/microorganisms8020184
Oevermann, A., Di Palma, S., Doherr, M. G., Abril, C., Zurbriggen, A., Vandevelde, M. (2010). Neuropathogenesis of Naturally Occurring Encephalitis Caused by Listeria Monocytogenes in Ruminants. Brain Pathol. 20, 378–390. doi: 10.1111/j.1750-3639.2009.00292.x
Oliver, J. D. (2010). Recent Findings on the Viable But Nonculturable State in Pathogenic Bacteria. FEMS Microbiol. Rev. 34, 415–425. doi: 10.1111/j.1574-6976.2009.00200.x
Olszewska, M. A., Panfil-Kuncewicz, H., Łaniewska-Trokenheim, L. (2015). Detection of Viable But Nonculturable Cells of Listeria Monocytogenes With the Use of Direct Epifluorescent Filter Technique. J. Food Saf. 35, 86–90. doi: 10.1111/jfs.12130
Overney, A., Chassaing, D., Carpentier, B., Guillier, L., Firmesse, O. (2016). Development of Synthetic Media Mimicking Food Soils to Study the Behaviour of Listeria Monocytogenes on Stainless Steel Surfaces. Int. J. Food Microbiol. 238, 7–14. doi: 10.1016/j.ijfoodmicro.2016.08.034
Overney, A., Jacques-Andre-Coquin, J., Ng, P., Carpentier, B., Guillier, L., Firmesse, O. (2017). Impact of Environmental Factors on the Culturability and Viability of Listeria Monocytogenes Under Conditions Encountered in Food Processing Plants. Int. J. Food Microbiol. 244, 74–81. doi: 10.1016/j.ijfoodmicro.2016.12.012
Palacios-Gorba, C., Moura, A., Leclercq, A., Gomez-Martin, A., Gomis, J., Jimenez-Trigos, E., et al. (2021). Listeria Spp. Isolated From Tonsils of Wild Deer and Boars: Genomic Characterization. Appl. Environ. Microbiol. 87 (6), 1–9. doi: 10.1128/AEM.02651-20.
Pandey, M. K., Rani, R., Agrawal, S. (2005). An Update in Recurrent Spontaneous Abortion. Arch. Gynecol. Obstet. 272, 95–108. doi: 10.1007/s00404-004-0706-y
Pang, M., Xie, X., Bao, H., Sun, L., He, T., Zhao, H., et al. (2018). Insights Into the Bovine Milk Microbiota in Dairy Farms With Different Incidence Rates of Subclinical Mastitis. Front. Microbiol. 9, 2379. doi: 10.3389/fmicb.2018.02379
Panutdaporn, N., Kawamoto, K., Asakura, H., Makino, S. I. (2006). Resuscitation of the Viable But Non-Culturable State of Salmonella Enterica Serovar Oranienburg by Recombinant Resuscitation-Promoting Factor Derived From Salmonella Typhimurium Strain LT2. Int. J. Food Microbiol. 106, 241–247. doi: 10.1016/j.ijfoodmicro.2005.06.022
Parsons, C., Niedermeyer, J., Gould, N., Brown, P., Strules, J., Parsons, A. W., et al. (2019). Listeria Monocytogenes at the Human-Wildlife Interface: Black Bears (Ursus Americanus) as Potential Vehicles for Listeria. Microb. Biotechnol. 13 (3), 706–721. doi: 10.1111/1751-7915.13509
Peddireddy, V., Doddam, S. N., Ahmed, N. (2017). Mycobacterial Dormancy Systems and Host Responses in Tuberculosis. Front. Immunol. 8, 84. doi: 10.3389/fimmu.2017.00084
Pfeffer, J. M., Strating, H., Weadge, J. T., Clarke, A. J. (2006). Peptidoglycan O Acetylation and Autolysin Profile of Enterococcus Faecalis in the Viable But Nonculturable State. J. Bacteriol. 188, 902–908. doi: 10.1128/JB.188.3.902-908.2006
Pinto, D., Almeida, V., Almeida Santos, M., Chambel, L. (2011). Resuscitation of Escherichia Coli VBNC Cells Depends on a Variety of Environmental or Chemical Stimuli. J. Appl. Microbiol. 110, 1601–1611. doi: 10.1111/j.1365-2672.2011.05016.x
Pinto, D., Santos, M. A., Chambel, L. (2015). Thirty Years of Viable But Nonculturable State Research: Unsolved Molecular Mechanisms. Crit. Rev. Microbiol. 41, 61–76. doi: 10.3109/1040841X.2013.794127
Pinto, D., Sao-Jose, C., Santos, M. A., Chambel, L. (2013). Characterization of Two Resuscitation Promoting Factors of Listeria Monocytogenes. Microbiology 159, 1390–1401. doi: 10.1099/mic.0.067850-0
Quereda, J. J., Moron-Garcia, A., Palacios-Gorba, C., Dessaux, C., Garcia-Del Portillo, F., Pucciarelli, M. G., et al. (2021). Pathogenicity and Virulence of Listeria Monocytogenes: A Trip From Environmental to Medical Microbiology. Virulence 12, 2509–2545. doi: 10.1080/21505594.2021.1975526
Radoshevich, L., Cossart, P. (2017). Listeria Monocytogenes: Towards a Complete Picture of Its Physiology and Pathogenesis. Nat. Rev. Microbiol. 16, 32–46. doi: 10.1038/nrmicro.2017.126
Ramamurthy, T., Ghosh, A., Pazhani, G. P., Shinoda, S. (2014). Current Perspectives on Viable But Non-Culturable (VBNC) Pathogenic Bacteria. Front. Public Health 2, 103. doi: 10.3389/fpubh.2014.00103
Rappaport, F., Rabinovitz, M., Toaff, R., Krochik, N. (1960). Genital Listeriosis as a Cause of Repeated Abortion. Lancet 1, 1273–1275. doi: 10.1016/S0140-6736(60)92253-4
Ravagnani, A., Finan, C. L., Young, M. (2005). A Novel Firmicute Protein Family Related to the Actinobacterial Resuscitation-Promoting Factors by Non-Orthologous Domain Displacement. BMC Genomics 6, 39. doi: 10.1186/1471-2164-6-39
Robben, C., Fister, S., Witte, A. K., Schoder, D., Rossmanith, P., Mester, P. (2018). Induction of the Viable But Non-Culturable State in Bacterial Pathogens by Household Cleaners and Inorganic Salts. Sci. Rep. 8, 15132. doi: 10.1038/s41598-018-33595-5
Robben, C., Witte, A. K., Schoder, D., Stessl, B., Rossmanith, P., Mester, P. (2019). A Fast and Easy ATP-Based Approach Enables MIC Testing for Non-Resuscitating VBNC Pathogens. Front. Microbiol. 10, 1365. doi: 10.3389/fmicb.2019.01365
Rollins, D. M., Colwell, R. R. (1986). Viable but Nonculturable Stage of Campylobacter jejuni and Its Role in Survival in the Natural Aquatic Environment. Appl. Environ. Microbiol. 52, 531–538. doi: 10.1128/aem.52.3.531-538.1986
Romana, C., Salleras, L., Sage, M. (1989). Latent Listeriosis may Cause Habitual Abortion Intrauterine Deaths, Fetal Malformations. When Diagnosed and Treated Adequately Normal Children Will Be Born. Acta Microbiol. Hung. 36, 171–172.
Rosser, A., Stover, C., Pareek, M., Mukamolova, G. V. (2017). Resuscitation-Promoting Factors Are Important Determinants of the Pathophysiology in Mycobacterium Tuberculosis Infection. Crit. Rev. Microbiol. 43, 621–630. doi: 10.1080/1040841X.2017.1283485
Roszak, D. B., Grimes, D. J., Colwell, R. R. (1984). Viable But Nonrecoverable Stage of Salmonella Enteritidis in Aquatic Systems. Can. J. Microbiol. 30, 334–338. doi: 10.1139/m84-049
Rothenburger, J. L., Bennett, K. R., Bryan, L., Bollinger, T. K. (2015). Septicemic Listeriosis in Wild Hares From Saskatchewan, Canada. J. Wildl. Dis. 51, 503–508. doi: 10.7589/2014-06-159
Salina, E. G., Grigorov, A. S., Bychenko, O. S., Skvortsova, Y. V., Mamedov, I. Z., Azhikina, T. L., et al. (2019). Resuscitation of Dormant "Non-Culturable" Mycobacterium Tuberculosis Is Characterized by Immediate Transcriptional Burst. Front. Cell. Infect. Microbiol. 9, 272. doi: 10.3389/fcimb.2019.00272
Sauders, B. D., Wiedmann, M., Desjardins, M., Fenlon, C., Davenport, N., Hibbs, J. R., et al. (2001). Recurrent Listeria Monocytogenes Infection: Relapse or Reinfection With a Unique Strain Confirmed by Molecular Subtyping. Clin. Infect. Dis. 33, 257–259. doi: 10.1086/321821
Scallan, E., Hoekstra, R. M., Angulo, F. J., Tauxe, R. V., Widdowson, M. A., Roy, S. L., et al. (2011). Foodborne Illness Acquired in the United States–Major Pathogens. Emerg. Infect. Dis. 17, 7–15. doi: 10.3201/eid1701.P11101
Schlech, W. F. (2019). Epidemiology and Clinical Manifestations of Listeria Monocytogenes Infection. Microbiol. Spectr. 7, 1–12. doi: 10.1128/9781683670131.ch50
Setlow, P., Johnson, E. A. (2019). “Spores and Their Significance,” in Food Microbiology, Fundamentals and Frontiers, 5th Edn. Eds. Doyle, M. P., Diez-Gonzalez, F., Hill, C. (Washington, DC: ASM Press), 23–64.
Sevellec, Y., Torresi, M., Felix, B., Palma, F., Centorotola, G., Bilei, S., et al. (2020). First Report on the Finding of Listeria Mnocytogenes ST121 Strain in a Dolphin Brain. Pathogens 9 (802), 1–13. doi: 10.3390/pathogens9100802
Shleeva, M., Goncharenko, A., Kudykina, Y., Young, D., Young, M., Kaprelyants, A. (2013). Cyclic AMP-Dependent Resuscitation of Dormant Mycobacteria by Exogenous Free Fatty Acids. PloS One 8, e82914. doi: 10.1371/journal.pone.0082914
Signoretto, C., Lleo, M., Canepari, P. (2002). Modification of the Peptidoglycan of Escherichia Coli in the Viable But Nonculturable State. Curr. Microbiol. 44, 125–131. doi: 10.1007/s00284-001-0062-0
Signoretto, C., Lleo, M. M., Tafi, M. C., Canepari, P. (2000). Cell Wall Chemical Composition of Enterococcus Faecalis in the Viable But Nonculturable State. Appl. Environ. Microbiol. 66, 1953–1959. doi: 10.1128/AEM.66.5.1953-1959.2000
Tasara, T., Stephan, R. (2006). Cold Stress Tolerance of Listeria Monocytogenes: A Review of Molecular Adaptive Mechanisms and Food Safety Implications. J. Food Prot. 69, 1473–1484. doi: 10.4315/0362-028X-69.6.1473
Telkov, M. V., Demina, G. R., Voloshin, S. A., Salina, E. G., Dudik, T. V., Stekhanova, T. N., et al. (2006). Proteins of the Rpf (Resuscitation Promoting Factor) Family Are Peptidoglycan Hydrolases. Biochemistry (Mosc) 71, 414–422. doi: 10.1134/S0006297906040092
Truchado, P., Gil, M. I., Larrosa, M., Allende, A. (2020). Detection and Quantification Methods for Viable But Non-Culturable (VBNC) Cells in Process Wash Water of Fresh-Cut Produce: Industrial Validation. Front. Microbiol. 11, 673. doi: 10.3389/fmicb.2020.00673
Truong, H. N., Garmyn, D., Gal, L., Fournier, C., Sevellec, Y., Jeandroz, S., et al. (2021). Plants as a Realized Niche for Listeria Monocytogenes. Microbiologyopen 10, e1255. doi: 10.1002/mbo3.1255
Weber, A., Potel, J., Schafer-Schmidt, R., Prell, A., Datzmann, C. (1995). [Studies on the Occurrence of Listeria Monocytogenes in Fecal Samples of Domestic and Companion Animals]. Zentralbl. Hyg. Umweltmed. 198, 117–123.
Weindl, L., Frank, E., Ullrich, U., Heurich, M., Kleta, S., Ellerbroek, L., et al. (2016). Listeria Monocytogenes in Different Specimens From Healthy Red Deer and Wild Boars. Foodborne Pathog. Dis. 13, 391–397. doi: 10.1089/fpd.2015.2061
Wery, N., Pourcher, A. M., Stan, V., Delgenes, J. P., Picard-Bonnaud, F., Godon, J. J. (2006). Survival of Listeria Monocytogenes and Enterococcus Faecium in Sludge Evaluated by Real-Time PCR and Culture Methods. Lett. Appl. Microbiol. 43, 131–136. doi: 10.1111/j.1472-765X.2006.01946.x
Whitesides, M. D., Oliver, J. D. (1997). Resuscitation of Vibrio Vulnificus From the Viable But Nonculturable State. Appl. Environ. Microbiol. 63, 1002–1005. doi: 10.1128/aem.63.3.1002-1005.1997
Wideman, N. E., Oliver, J. D., Crandall, P. G., Jarvis, N. A. (2021). Detection and Potential Virulence of Viable But Non-Culturable (VBNC) Listeria Monocytogenes: A Review. Microorganisms 9 (194), 1–11. doi: 10.3390/microorganisms9010194
Wiktorczyk-Kapischke, N., Skowron, K., Grudlewska-Buda, K., Walecka-Zacharska, E., Korkus, J., Gospodarek-Komkowska, E. (2021). Adaptive Response of Listeria Monocytogenes to the Stress Factors in the Food Processing Environment. Front. Microbiol. 12, 710085. doi: 10.3389/fmicb.2021.710085
Wolfe, B., Kerr, A. R., Mejia, A., Simmons, H. A., Czuprynski, C. J., Golos, T. G. (2019). Sequelae of Fetal Infection in a Non-Human Primate Model of Listeriosis. Front. Microbiol. 10, 2021. doi: 10.3389/fmicb.2019.02021
Xu, H. S., Roberts, N., Singleton, F. L., Attwell, R. W., Grimes, D. J., Colwell, R. R. (1982). Survival and Viability of Nonculturableescherichia Coli Andvibrio Cholerae in the Estuarine and Marine Environment. Microb. Ecol. 8, 313–323. doi: 10.1007/BF02010671
Yoshida, T., Sugimoto, T., Sato, M., Hirai, K. (2000). Incidence of Listeria Monocytogenes in Wild Animals in Japan. J. Vet. Med. Sci. 62, 673–675. doi: 10.1292/jvms.62.673
Zhao, X., Zhong, J., Wei, C., Lin, C. W., Ding, T. (2017). Current Perspectives on Viable But Non-Culturable State in Foodborne Pathogens. Front. Microbiol. 8, 580. doi: 10.3389/fmicb.2017.00580
Keywords: zoonosis, VBNC, dormancy, asymptomatic infections, foodborne pathogen, risk assessment, pregnancy, infectious diseases
Citation: Lotoux A, Milohanic E and Bierne H (2022) The Viable But Non-Culturable State of Listeria monocytogenes in the One-Health Continuum. Front. Cell. Infect. Microbiol. 12:849915. doi: 10.3389/fcimb.2022.849915
Received: 06 January 2022; Accepted: 07 February 2022;
Published: 18 March 2022.
Edited by:
Changyong Cheng, Zhejiang A & F University, ChinaReviewed by:
Yanhong Liu, United States Department of Agriculture (USDA), United StatesCopyright © 2022 Lotoux, Milohanic and Bierne. This is an open-access article distributed under the terms of the Creative Commons Attribution License (CC BY). The use, distribution or reproduction in other forums is permitted, provided the original author(s) and the copyright owner(s) are credited and that the original publication in this journal is cited, in accordance with accepted academic practice. No use, distribution or reproduction is permitted which does not comply with these terms.
*Correspondence: Hélène Bierne , aGVsZW5lLmJpZXJuZUBpbnJhZS5mcg==
Disclaimer: All claims expressed in this article are solely those of the authors and do not necessarily represent those of their affiliated organizations, or those of the publisher, the editors and the reviewers. Any product that may be evaluated in this article or claim that may be made by its manufacturer is not guaranteed or endorsed by the publisher.
Research integrity at Frontiers
Learn more about the work of our research integrity team to safeguard the quality of each article we publish.