- Department of Gastroenterology, The First Affiliated Hospital of Nanchang University, Nanchang, China
Helicobacter pylori (H. pylori) infection is considered a class I carcinogen in the pathogenesis of gastric cancer. In recent years, the interaction relationship between H. pylori infection and autophagy has attracted increasing attention. Most investigators believe that the pathogenesis of gastric cancer is closely related to the formation of an autophagosome-mediated downstream signaling pathway by H. pylori infection-induced cells. Autophagy is involved in H. pylori infection and affects the occurrence and development of gastric cancer. In this paper, the possible mechanism by which H. pylori infection affects autophagy and the progression of related gastric cancer signaling pathways are reviewed.
Introduction
Nowadays, the prevalence of gastric cancer has been increasing, which ranks the fifth among malignant tumors and the third most common cause of cancer death in the world (Tsai et al., 2017; Smyth et al., 2020). Although therapy for malignant tumors has significantly improved, the five-year survival rate of gastric cancer remains less than 30%. The relationship between Helicobacter pylori (H. pylori) infection and the prevalence of gastric cancer has been thoroughly identified. The World Health Organization (WHO) has listed H. pylori as a class 1 carcinogen, almost all gastric cancer cases are related to H. pylori (Ahn and Lee, 2015; Plummer et al., 2015; Schulz et al., 2016).
H. pylori was first recognized in 1983, which is a helical, highly mobile gram-negative pathogenic bacterium of 0.6 x 3.5micron size. For all that H. pylori is an extracellular pathogen, it can still cause persistent infection through residing and colonizing in the luminal surface of the gastric epithelium (Wroblewski et al., 2010). Numerous upper gastrointestinal disorders are associated with H. pylori infection, which is thought to infect over 50% of the worldwide population, which can cause gastritis, peptic ulcers, gastric cancer and so on (Blaser, 1987). H. pylori has such high pathogenicity, mainly depends on its various virulence factors, such as urease, vacuolar cytotoxin A (VacA), cytotoxin-associated-gene A (CagA), gamma-glutamyltranspeptidase (HpGGT), HP0175, high temperature requirement A (HtrA), and neutrophil-activating protein (NAP). In addition, some outer membrane proteins (OMPs), including outer inflammatory protein A (OipA), can also produce varying degrees of virulence (Pormohammad et al., 2018). In terms of the risk of gastric cancer, VacA and CagA are the most characteristic virulence factors, they both greatly promote the development of gastric cancer (Matos et al., 2013).
The specific mechanism of gastric cancer induced by H. pylori infection is not as clear as a bell. Previous studies have suggested that H. pylori can induce defective autophagy or inhibit autophagy, which enabling H. pylori to proliferate (Xie et al., 2020). It has been considered that VacA can disrupt lysosome activity, as well as CagA can enter gastric epithelial cells and induce interleukin-8 secretion (He et al., 2020). In addition, H. pylori can initiate abnormal activation of cellular signaling pathways, such as Nod1-NF-KB/MAPK-ERK/FOXO4 pathway, allowing cells to escape autophagy and leading to the occurrence of gastric cancer (Brandt et al., 2015; Eslami et al., 2019; Takahashi-Kanemitsu et al., 2020). Nowadays, autophagy have become the focus regarding the pathogenesis and targeted therapy in gastric cancer, This article reviews the effect of H. pylori infection on autophagy function and the role of autophagy in gastric cancer, so that can provide new targeted autophagy therapies and delay the progression of gastric cancer.
Autophagy
Mechanism of Autophagy
Autophagy is a common physiological process, it means to eat oneself, which is mainly used to provide energy and degrade some components in cells (Cuervo and Wong, 2014; Parzych and Klionsky, 2014). When cells are under stress conditions, such as starvation and hypoxia, the body phagocytoses diseased organelles or damaged cytoplasmic proteins to form autophagosome. Then it combining with lysosomes to form autolysosome, thereby maintaining the cellular homeostasis. This process can degrade and reuse degenerated cells, recycling their contents for subsequent use. On the one hand, autophagy can eliminate damaged cells when stimulated by adverse external factors, on the other hand, autophagy can promote tumor cell survival by preventing the accumulation of toxins or metabolites (Maes et al., 2013). Therefore, autophagy is a double-edged sword, and it has a two-way effect that can either promote or inhibit cell survival.
The mechanism of autophagy is simply the recognition of selective substrates by specific receptors, which are mainly divided into non-selective and selective isolation (Ryter et al., 2013; Lai et al., 2018). According to previous descriptions and summaries, the pathways of autophagy are mainly divided into the following three types, including chaperone‐mediated autophagy (CMA), micro-autophagy, and macro-autophagy (Mizushima and Levine, 2010; Galluzzi et al., 2017). CMA is a selective lysosomal pathway, the chaperone complex delivers the CMA substrate to the receptor protein on the lysosome membrane and assemble into a poly translocation complex. Besides, it can translocate cytoplasmic proteins into lysosomes, relying directly on recognition of targeted sequences by cytoplasmic HSC70, and then delivers cargo proteins to lysosomal-associated membrane protein2 (LAMP2). HSC70 is an essential prerequisite for CMA which can promote the classification of certain proteins in LAMP2 (Kaushik and Cuervo, 2012). Micro-autophagy mainly transfers cytoplasmic components to the lysosome, then the cholesterol-dependent vacuolar membrane directly invades into the lumen and compresses the newly formed vesicle finally. Simply speaking, micro-autophagy is a process that lysosome directly phagocytosis by intruding into lysosome membrane (Kaushik and Cuervo, 2012). Macro-autophagy is a process in which lysosomal fusion is involved in the breakdown of damaged organelles as well as the aggregation of proteins and pathogens. Importantly, macro-autophagy is the most reported autophagy subtype in H. pylori infection. Among up-regulating some survival promoting autophagy factors and down-regulating some death promoting apoptotic factors, macro-autophagy can alleviate the inflammatory response of H. pylori infection (Terebiznik et al., 2009; Yang and Chien, 2009).
Autophagy-Related Genes
Shortly after the initial discovery of APG1-1, the autophagy research community identified nearly 70 autophagy-associated genes in different eukaryotes and named them autophagy-associated genes (ATGs) (Boneca et al., 2008). ATGs is involved in inducing membrane separation, cytoplasm phagocytosis, autophagy vesicles formation and fusion with lysosomes. More than 40 ATGs are related to the regulation of autophagy, the typical autophagy mechanism consists of more than 30 ATGs and was originally identified in yeast (Mizushima et al., 2011). ATGs constitute the core molecular mechanisms and functions of autophagy in several successive steps of the autophagy cascade to coordinate this process. ATG5 is the core components of autophagy related mechanisms, it is involved in elongation of the autophagosome (Yang and Klionsky, 2010; Reggiori et al., 2012). ATG7 is also critical to autophagy, the autophagy defect model is often be prepared by knocking out ATG7 in some experiments. Proteins in the ATG8 family are the first building blocks of the core autophagy mechanism and need to be characterized in molecular detail. ATG9 is the only complete membrane protein and plays a central role in mediating autophagosome formation. ATG11 is a scaffold protein required for selective autophagy and is also required for mitosis. The 62 amino acid regions of ATG11 are sufficient to support the autophagy function of ATG11 and ATG1 kinase activity. ATG16L1, a mammalian ATG16 homolog, is one of the gene clusters involved in autophagy, and mice lacking ATG16L showed impaired LC3 lipids. ATG19 bridges cargo proteins through the double membrane of ATG11 and ATG8 modified Cvt vesicles. In addition, ATG36 binds to ATG8 and the adapter ATG11, which associates selective autophagy type receptors with core autophagy mechanisms (Fujita et al., 2008).
Several studies have found that the levels of relevant ATGs expression are largely regulated in H. pylori infection, and inhibit autophagy function can promote the colonization of H. pylori in gastric epithelial cells (Odenbreit et al., 2000). The latest report found that a total of 28 ATGs were significantly down-regulated in H. pylori-infected AGS cells, and ATG16L and ATG5 were significantly lower in H. pylori-positive patients than in H. pylori-negative patients (Castaño-Rodríguez et al., 2015). To some certain extent, down-regulating some core autophagy genes can inhibit intracellular autophagy, which can further provide a good survival environment for H. pylori colonization.
H. pylori Virulence Factors That Can Induce Autophagy in Gastritis Epithelium
H. pylori has many virulence factors, among which VacA, CagA, HpGGT and HP0175 can mainly induce autophagy in gastric mucosal epithelial cells. These virulence factors influence the autophagy flow by mediating relevant autophagy pathways, which in turn produce a series of different effects in cells (Table 1).
VacA
VacA is a well-known virulence factor in H. pylori, which causes various cellular impaired functions in infected cells (Yamaoka, 2010). VacA toxin can also cause a series of changes in cells, such as vacuolization, disruption of lysosome function, and promoting immune regulation (Willhite et al., 2003). The pathogenicity of VacA is mainly determined by mosaic recombination between two main alleles (s1, s2, i1, i2, m1, m2) (Yamaoka, 2010). Strains with s1, m1 and i1 alleles produced more active toxins and were highly correlated with the occurrence of gastric cancer (Rhead et al., 2007).
Recent studies suggested that, the autophagy response process can be triggered by VacA, which can promote the formation of tyrosine phosphatase-related vacuoles as soon as activating VacA channels (Yahiro et al., 2012). Furthermore, VacA induces autophagy through endoplasmic reticulum stress, and inhibition of autophagy alleviates VacA-induced AGS cell death (Kroemer et al., 2010; Isomoto et al., 2010). VacA can induce autophagy when H. pylori temporarily infected gastric epithelial cells, which can degrade toxins, mitigate cell damage and further maintain cell homeostasis (Terebiznik et al., 2006; Terebiznik et al., 2009). But after long-term infection, it can destroy the autophagy pathway and further led to intracellular autophagy dysfunction (Ricci, 2016). When the cells were co-cultured with VacA for a long time, it can induce the collection of the autophagic substrate p62 and inhibit the expression of cathepsin D in autophagosome. P62 can connect with Rad51 subsequently, and then can increase the ubiquitination and degradation of Rad51, which suppress the function of repair damaged DNA (Xie et al., 2020). Thus, VacA mainly inhibits the autophagy of gastric mucosa epithelial cells in long-lasting H. pylori infection.
CagA
CagA protein has structural diversity and strong immunogenicity, and is most related to H. pylori and gastric pathology (Odenbreit et al., 2000). The motifs of EPIYA in CagA strain can be divided into EPIYA-a, EPIYA-b, EPIYA-c and EPIYA-d. Western CagA strains usually include EPIYA-a, EPIYA-b and EPIYA-c sequences, while East Asian CagA strains only have EPIYA-a, EPIYA-b and a specific EPIYA-d, which can be partly explained by the highest incidence of gastric cancer in East Asian countries (Vianna et al., 2015; Kumar and Dhiman, 2018). Similar to VacA, CagA can induce a variety of cellular changes, including cytoplasmic vacuolation, endoplasmic reticulum stress, and mitochondrial dysfunction, and further lead to inflammation (Terebiznik et al., 2009). More and more evidence suggested that there may be a functional link between VacA and CagA virulence factors. Some articles have pointed out that there is a correlation between weak vacuolation and strong CagA phosphorylation, activing VacA is a key factor in CagA phosphorylation (Tsugawa et al., 2012).
CagA can be phosphorylated by tyrosine when it enters gastric epithelial cells, and tyrosine can be activated by a series of signaling factors, such as the PI3K/AKT/mTOR pathway signaling factors (Selbach et al., 2015; Tohidpour, 2016; Li et al., 2017). These can change the cytoskeleton of polyactin, causing an inflammatory response and suppressing autophagy (Mimuro et al., 2002; Li et al., 2017; Eslami et al., 2019). A new study suggests that CagA negatively regulates autophagy through the c-Met-PI3K/Akt-MTOR signaling pathway (Fan et al., 1996). In this study, compared with patients infected with CagA-negative H. pylori strain, SQSTM1 was significantly accumulated in the gastric mucosa of patients infected with the CagA-positive H. pylori strain. Besides, CagA-positive H. pylori significantly increased Akt phosphorylation levels at Ser473 as well as p-mTOR and P-S6 in AGS cells. In addition, CagA has been considered to increase the expression of miR-543, which can inhibit autophagy by combining with SIRT1, then further increase EMT and promote migration in gastric cancer (Semino-Mora et al., 2003; Suzuki et al., 2009). The expression of CagA is significantly higher in CD44 positive gastric cancer cells, mainly because CD44 positive gastric cancer stem cells can inhibit autophagy and further increase the expression level of CagA (Li et al., 2017). Even more important, CagA-positive strains can colonize in gastric mucosal epithelial tissues for a long time. It can upregulate inflammatory cytokines in H. pylori infected patients, and also can down-regulate related autophagy function. Therefore, the inhibition of autophagy by CagA promoted gastritis and thus initiated the occurrence of gastric cancer. Given the pluripotency of CagA, the interaction between CagA and autophagy regulation mechanism needs to be further studied.
HpGGT
H. pylori gamma-glutamyl transpeptidase (HpGGT) is commonly found in all H. pylori strains, which plays a role in gastric mucosal epithelial cells (Chevalier et al., 1999). HpGGT, as an inhibitor of autophagy, can promote the internalization of bacteria in gastric cells, which can lead to chronic gastric inflammation and even gastric tumors. There has been suggested that HpGGT damages the autophagy flux by disrupting lysosomal membrane integrity in AGS cells, resulting in reduced lysosomal cathepsin B activity (Bravo et al., 2019). They considered a functional connection between HpGGT and VacA exists. In the early stage of H. pylori infection in AGS cells, VacA can induce autophagy, which can be seen through the transformation of the protein LC3 (microtubule-associated protein 1 light chain 3, MAP1LC3/LC3). In the late stage, HpGGT reduces cathepsin B activity and prevents lysosome degradation, which appears to inhibit autophagy. It should be noted that HpGGT inhibited autophagy after H. pylori infection in GES-1 cells, but which was not associated with cathepsin B. When H. pylori infects gastric epithelial cells, HpGGT inhibits autophagy and leads to genomic instability, which creates an environment conducive to carcinogenesis in turn. Thus, the exact mechanism by HpGGT regulates autophagy in various gastric epithelial cells remains to be determined, and how it promotes epigenetic changes in the progression of gastric cancer needs further study.
HP0175
H. pylori contains a large number of secretory proteins, including HP0175, a peptide-based proline cis-trans isomerase (PPIase), that is highly recognized in serum and AGS cells of H. pylori-infected patients (Kim et al., 2002). Previous studies have shown that HP0175 induces apoptosis in gastric epithelial cells (Basak et al., 2005). Recent years, new studies have found that HP0175 can also mediate autophagy in gastric epithelial cells (Halder et al., 2015). HP0175 upregulates Beclin-1, ATG5, and UKL1 in AGS cells through transcription factors ATF4 and CHOP, in addition, it can induce LC3I to LC3II conversion in a PERK-dependent manner which depends on the unfolded protein response (UPR). This is the first time that HP0175 has been demonstrated to be associated with UPR and autophagy in H. pylori-infected gastric epithelial cells, and the in-depth mechanism of autophagy regulation still needs to further study.
Autophagy is a dynamic process in gastric epithelial cells infected with H. pylori, it can respond to a range of disturbances in intracellular or extracellular homeostasis (Mizushima and Levine, 2010). In summary, autophagy protects gastric mucosal epithelial cells during the acute phase of H. pylori infection. VacA secreted by H. pylori induces autophagy, then autophagy degrades CagA and VacA, which reduce the damage in gastric mucosal epithelium. However, during chronic H. pylori infection, VacA destroys the autophagy pathway in gastric mucosal epithelial cells, limits the degradation of VacA, and results in the accumulation of autophagy substrates, causing severe DNA damage and accelerating the process of H. pylori infection-mediated gastric cancer pathogenesis (Zhang et al., 2019). Moreover, VacA damages autophagy function and then leads to the reduced degradation of CagA, which further enhances damage to host cells (Deen et al., 2015) (Figure 1).
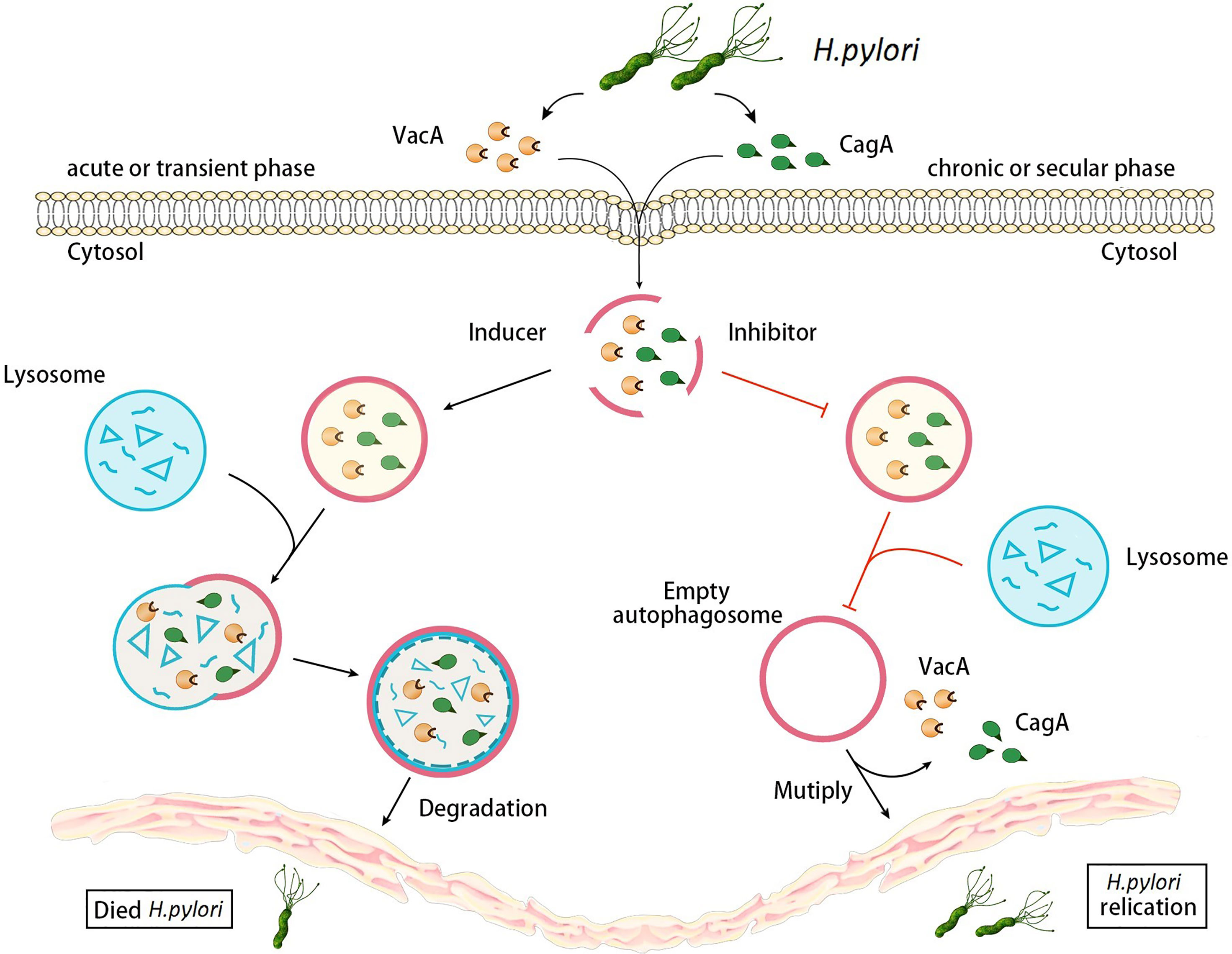
Figure 1 Changes of autophagy in gastric epithelial cells during different stages of H. pylori infection. During the acute stage of H. pylori infection, VacA and CagA secreted by H. pylori infection induce autophagy, and then autophagy degrades VacA and CagA. However, during chronic H. pylori infections, VacA and CagA inhibit autophagy, limiting the degradation of VacA and causing the accumulation of autophagy substrates. Moreover, autophagy destruction of VacA leads to the reducing degradation of CagA, which further enhances damage to host cells.
The Correlation Between H. pylori Infection and Core Autophagy Protein Markers
H. pylori can cause an increase in autophagosomes, it surrounded by a bilayer membrane is the characteristic of the formation in autophagosome (Allen et al., 2000; Allen, 2007). Intracellular H. pylori can induce autophagy by regulating autophagy-related genes, which further change a series of core autophagy protein markers.
SQSTM1/p62
SQSTM1/p62, a ubiquitin-binding protein, which are both selective autophagy substrates and autophagy receptor proteins involved in autophagy (Puissant et al., 2012). It can deliver specific organelles and protein aggregates to autophagosomes for degradation. The accumulation of SQSTM1/p62 and ubiquitinated proteins can be used as indicators of autophagy inhibition, which serve as a receptor protein that can connect to the ubiquitinated structures of LC3B and misfolded proteins. The degradation of SQSTM1/p62 reflects the transformation of autophagy mechanism, and defective autophagy can result in the accumulation of SQSTM1/p62. Among them, E3 ubiquitin ligase TRIM32 can be used as a substrate for the autophagy receptor SQSTM1/p62, and targeted TRIM32 can degraded by the selective autophagy.
It has been previously reported that the protein levels of SQSTM1/p62 were significantly increased in H. pylori-infected gastric cells (Raju et al., 2012). Further animal models shown that SQSTM1/p62 levels are negatively correlated with Rad51 levels in H. pylori-infected gastric mucosa cells (Suarez et al., 2019). SQSTM1/p62 induces the ubiquitination of Rad51 mediated by H. pylori infection. The main mechanism is that H. pylori infection leading the nuclear translocation of SQSTM1/p62 through co-locating with Rad51, H. pylori infection can promote nuclear entry of SQSTM1/P62 and interaction with Rad51. Co-culturing AGS cells and GES-1 cells with H. pylori, when knockdown SQSTM1/p62 with the SQSTM1/p62 plasmid, Rad51 can be increased (Li et al., 2015).
In addition, clinical literature further found an increase of SQSTM1/p62 in gastric biopsies from patients infected with the virulent VacAs1m1 strain, compared with patients infected with the non-toxic strain VacAs2m2 (Tang et al., 2012; Lim et al., 2017). The accumulation of SQSTM1/p62 in vivo and in vitro biopsy samples from patients infected with the VacA-positive strain was mainly associated with impaired autophagy function. The expression of SQSTM1/p62 expression in gastric biopsies was also higher in patients infected with the CagA-positive strain than in patients infected with the CagA-negtive strain.
MAP1LC3/LC3
Like SQSTM1/p62, MAP1LC3/LC3 is a core protein marker in autophagy, and MAP1LC3/LC3-associated phagocytosis (LAP) was found to play a key role in removing bacteria from some certain cells (Raju et al., 2012). LC3 can be divided into two subtypes, which are type I LC3 (LC3-I) and type II LC3 (LC3-II). LC3-I is the soluble form of LC3, and LC3-II is the membrane-bound form of LC3. In the formation of autophagosomes, LC3 undergoes multiple forms of transformation. Pro-LC3 is an untreated form of LC3, which is converted to LC3-I by hydrolyzed via ATG4. Conjugation of LC3 to lipids (LC3-II) requires the processing of LC3 precursors and LC3-I, which is mediated by ATG422, after that, ATG3 further converts LC3-I to LC3-II (Florey and Overholtzer, 2012; Deen et al., 2015; Li et al., 2017). In short, LC3-I conversion LC3-II (LC3-II/LC3-I) is a marker of autophagy induction. LC3 can bind directly to SQSTM1/p62, and LC3 binds to the LC3 interaction region (LIR) of p62 through the interaction between the n-terminal base residue of SQSTM1/p62 and the acid cluster on SQSTM1/p62. SQSTM1/p62 can interact directly with LC3 and even be degraded in autophagosomes (Kim et al., 2013; Mehta et al., 2014). The interaction between LC3 and SQSTM1/p62 is believed to be the mechanism that links the protein aggregates containing SQSTM1/p62 to the autophagy mechanism (Deen et al., 2013; Greenfield and Jones, 2013).
H. pylori infection triggered autophagy in gastric epithelial cells, which indicated by the presence of many GFP-LC3-positive structures clearly visible in the cytoplasm. Autophagy labeled LC3 dot structures co-located with H. pylori in H. pylori-infected AGS cells. Consistent with this finding, the expression of LC3-II and LC3 site formation were increased in AGS cells due to H. pylori infection. Furthermore, studies have found that VacA can induce vacuoles in gastric epithelial cells to act as the intracellular niche of H. pylori, then with the presence of LC3, it can further promote the fusion of lysosomal and the degradation of luminal content in vacuoles (Sanjuan et al., 2007; Barth et al., 2010; Glick et al., 2010; Florey et al., 2011; Martinez et al., 2011).
Cathepsin D/CTSD
Cathepsin D(CTSD) is an aspartic acid protease in lysosomes, which is one of the main lysosomal proteases necessary for maintaining protein balance in cells. CTSD directly participates in autophagy and degradation of cell structures through reverse endocytosis and phagocytosis. Meanwhile, only CTSD can directly degrade the AGE transferred to the lysosome by endocytosis (Henault et al., 2012; Ma et al., 2012). It has been suggested that long-term exposure to VacA can lead to the formation of defective autophagosomes lacking CTSD and reduce their catalytic activity. It may be due to the fact that VacA neutralizes the acidic environment in the lysosomal lumen, which inhibits further maturation of proCTSD (Rijnboutt et al., 1992). Currently, it is believed that VacA can inhibit the fusion of autophagosomes with lysosomes in AGS, mainly because VacA changes the acidic environment of lysosomes, which can further cause the deficiency of CTSD, thus making the degradation function of autophagosomes unable to complete (Delbrück et al., 1994). Therefore, in line with the effect of VacA on the accumulation of SQSTM1/P62, the VacA-induced deficiency of CTSD can also promote the survival and replication of H. pylori in gastric epithelial cells58.
The Autophagy Mediated Signaling Pathways in H. pylori-Associated Gastric Cancer
The Nod1-NF-kB/MAPK-ERK/FOXO4 Pathway
NOD1, a member of nod-like receptor (NLR) family, is an intracellular immune receptor that senses peptidoglycan of Gram-negative bacteria (Allen, 2007). NOD1-specific single nucleotide polymorphisms (SNPs) have been associated with H. pylori infection and gastric cancer (Puissant et al., 2012). It was found that co-culture with H. pylori can up-regulate the mRNA and protein levels of NOD1-RIP2-NF -κB in gastric cancer cells, and also found that NOD1-RIP2-NF -κB pathway can activate autophagy. NOD1 receptor can detect intracellular H. pylori and recruit ATG16L1 to the entry site of bacteria, then it can regulate lysosomal and activate the NF -κB signaling pathway at the same time, which can cause the occurrence of inflammation (Blaser, 1987). In addition, long-term stimulation of H. pylori lysate promoted the phosphorylation of ERK, then it inhibited the levels of FOXO4 and its downstream genes, such as BNIP3, BCL-6, and ATG12, which indicated that the FOXO pathway can regulate cell autophagy. Therefore, H. pylori can inhibit autophagy and apoptosis through the Nod1-NF-kB/MAPK-ERK/FOXO4 signaling pathway.
The Wnt/β-catenin Pathway
The Wnt/β-catenin signaling pathway is involved in multiple physiological processes, such as growth and development, cell regeneration, and organ formation. The abnormal proliferation of gastric cancer cells is related to their abnormal activation (Wang et al., 2019). When Wnt signal ligand binds to the cell membrane surface receptor protein frizzled, it activates proteins scattered throughout the cell, thereby activating Wnt signaling. These scattered proteins inhibit the activity of β-catenin complex, thereby stabilizing the free β-catenin in the cell. When β-catenin turns into the nucleus, it attaches to the T cell factor (TCF)/lymphoid enhancing factor (LEF) transcription factor family to promote downstream target gene (e.g., c-myc, cyclin D1) transcription, and abnormal activation of target genes promotes the proliferation and growth of tumor cells, inducing gastric cancer pathogenesis.
Recent research has revealed that, in the autophagy regulation process, the Wnt/β-catenin signaling pathway plays a crucial role in tumor cells (Yong et al., 2016). After H. pylori infection, autophagy is reduced by VacA, the CagA secretion system is activated, and β-catenin is accumulated in cells. This accumulation activates the Wnt signaling pathway and accelerates the activation of downstream genes, such as c-myc and cyclin D1. Therefore, this transcriptional activity leads to the onset of gastric cancer.
The PI3K/Akt/mTOR Pathway
The PI3K/Akt/mTOR signaling pathway is an important signal transduction system that can affect the growth, proliferation, survival, metabolism, and angiogenesis of tumor cells. Meanwhile, it especially plays a key role in the invasion, migration and infiltration phases of H. pylori-associated gastric cancer (Yang et al., 2017; Ke et al., 2018). PI3K is a lipid kinase family composed of a regulatory subunit (p85) and a catalytic subunit (p110). PI3K promotes phosphorylation of the 3-hydroxyl group of phosphoinositide, and PI3P synthesized by type III kinase acts as the initiation signal to form autophagosome. Akt mediates Beclin-1 phosphorylation and promotes the interaction among the Beclin-1, vimentin and 14-3-3. Increase the expression of Vimentin can induce autophagy, and then promote the Akt-induced carcinogenesis (Wang et al., 2019). When infected with H. pylori, Akt mediates beclin-1 phosphorylation, which activates the autophagy system, increases Vimentin and 14-3-3 protein simultaneously. When Beclin-1 gene is mutated, autophagy can still be activated via PI3K-Akt-mTOR if Akt-mediated phosphorylation fails. mTOR is an effector molecule downstream of the PI3K-Akt pathway that is an important substance negatively regulating autophagy. By inhibiting autophagy, mTOR regulates cell growth, proliferation and protein synthesis (Kidd et al., 2014). It has been found that the expression of mTOR increased in human and mice gastric epithelial cells when infected by H. pylori, and the cause of its increase was largely determined by CagA (Lee et al., 2018). In addition, gastritis was significantly relieved in mTOR knockout (KO) mice which infected with H. pylori. These findings support that H. pylori promotes gastritis and gastric cancer through the PI3K/Akt/mTOR signaling pathway (Figure 2).
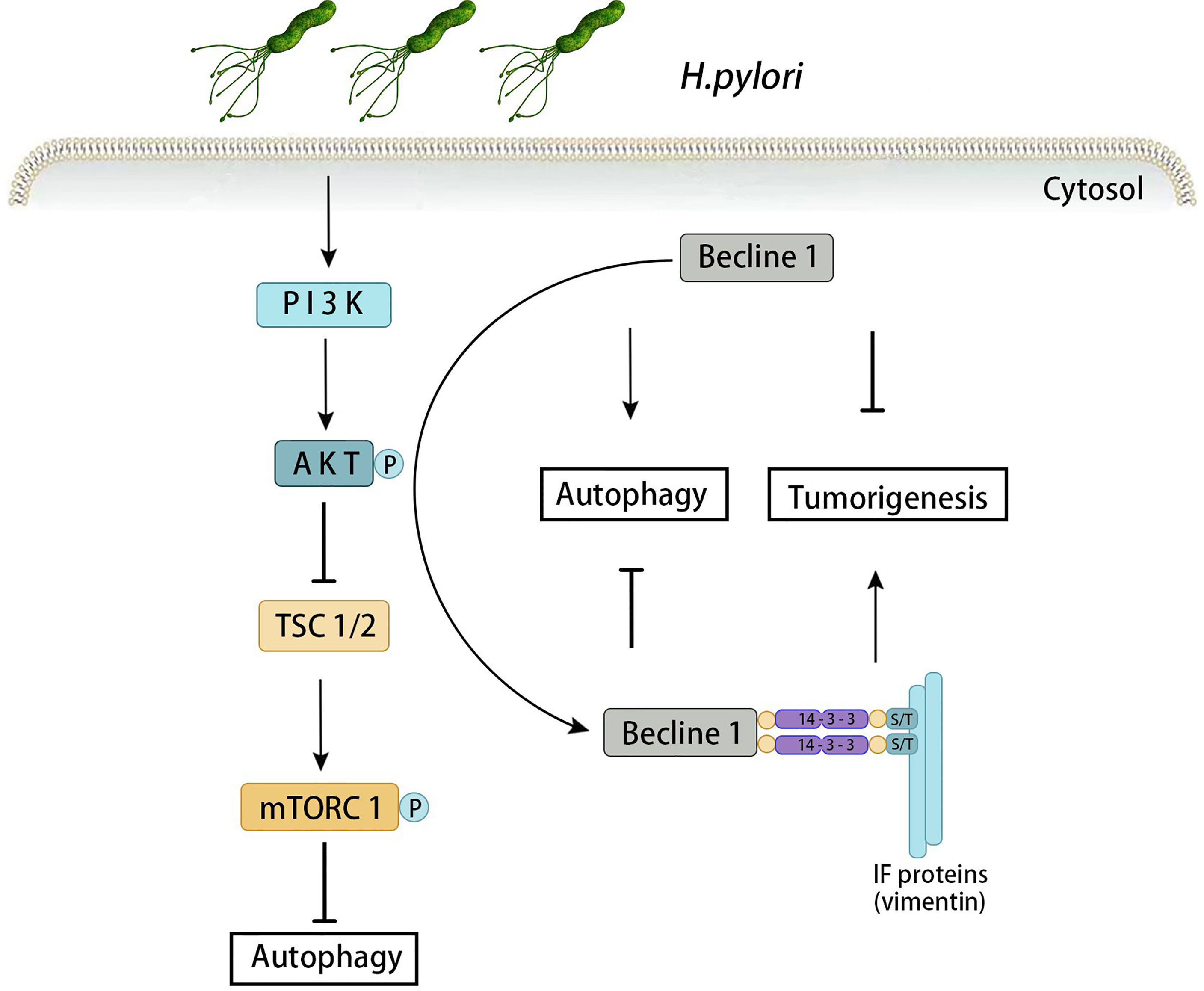
Figure 2 Autophagy induced by PI3K-Akt-mTOR signaling pathway in H. pylori-associated gastric cancer. When infected with H. pylori, Akt mediates beclin-1 phosphorylation, which activates the autophagy system, increases Vimentin and 14-3-3 protein simultaneously. Increase the expression of Vimentin can induce autophagy, and then promote the Akt-induced carcinogenesis. When Beclin-1 gene is mutated, autophagy can still be activated via PI3K-Akt-mTOR if Akt-mediated phosphorylation fails.
The VEGF Pathway
Vascular endothelial growth factor (VEGF) is a vascular permeability factor that participates in a series of reactions, such as the degradation of the vascular basement membrane, the proliferation of vascular endothelial cells, and the deposition of a new basement membrane. During development, these processes result in embryonic blood vessels being formed, and after birth, they allow existing blood vessels to continue to grow (Feng et al., 2020). Studies have shown that single nucleotide polymorphisms of VEGF are the predictive and prognostic indicators for solid tumors, such as gastric cancer, colorectal cancer, and breast cancer (Gao et al., 2019). The high expression of VEGFR-2 may be related to tumor staging, recurrence and metastasis in gastric cancer. High expression of the VEGFR-2 and the VEGFR-2 rs1870377 A>T gene polymorphisms may a prognostic factor in gastric cancer (Zeng et al., 2019). Beclin-1 overexpression was found to markedly attenuate the ability of gastric cancer cells to migrate and invade possibly due to VEGF hypoexpression (Zhu et al., 2019). It also indicates that VEGF can regulate autophagy related genes and thus affect the occurrence and development of gastric cancer.
The TGF-β Pathway
TGF-β is an important representative of the TGF-β family, which includes 3 subtypes (β1, β2 and β3). TGF-β1 was first discovered in lymphocytes and monocytes, which is the most common form of TGF-β (Zheng et al., 2020). The TGF-β signaling pathway is connected with the angiogenesis, invasion, metastasis in gastric cancer (Yue and Mulder, 2001).
In terms of autophagy-related research, studies have shown that TGF-β participates in the regulation of autophagy activity in H. pylori-associated gastric cancer, which is a powerful activator of autophagy (Liu et al., 2016). In addition, it has been found that the level of TGF-β1 is significantly increased in the H. pylori-positive gastric cancer patients (Guo et al., 2012). Meanwhile, the expression of autophagy related genes, BNIP3, Beclin-1, the transformation of LC3 I to II indicated that TGF-β1 enhanced autophagy. To a certain extent, the primary role of TGF-β1 is to inhibit the formation of tumor cells during the early stage of H. pylori-associated gastric cancer. However, with the further development of gastric cancer, TGF-β1 cooperates with the VEGF signaling pathway to promote angiogenesis around the tumor, enhancing the ability of tumor cells to invade and metastasize and accelerating the progression of gastric cancer (Wu et al., 2016).
The miRNA-Related Signaling Pathway
Studies have shown that miRNAs play quite important roles in growth, autophagy and invasion during the pathogenesis of gastric cancer (Tsugawa et al., 2012; Yang et al., 2018). Some microRNA (miRNA)s act as tumor suppressors in gastric cancer, while others act as oncogenic genes by regulating the expression levels of various genes. H. pylori is an important factor that can further influence autophagy by regulating the function of miRNAs (Muhammad et al., 2017). The expression of miRNA (miR)-30b are increased most among upregulating miRNAs. Previous literatures have pointed out that H. pylori infection can induce changes in miR-30b expression in gastric epithelial cells (Yin et al., 2019). Notably, they found that miR-30b regulates the autophagy process during persistent H. pylori infection, thereby promoting the persistence of H. pylori infection. Beclin-1 and ATG12, as important proteins involved in autophagy, are new targets of miR-30b (Pang et al., 2020). Besides, miR-30d inhibits the activity of Beclin-1, which prevents further nucleation of autophagy vesicles (Ashrafizadeh et al., 2020). Down-regulating miR-28 can activate the PTEN/PI3K/Akt signaling pathway, which can induce the infiltration and metastasis of gastric cancer (Li et al., 2018). Targeting miR-93-5p can negatively regulate the tumor suppressor AHNAK via autophagy and promote the epithelial-mesenchymal transition, and then promote the occurrence and development of gastric cancer (Shen et al., 2020). In addition, methylation silencing caused by H. pylori may damage autophagy and promote the occurrence of gastric cancer.
Drugs Therapy Targeting Autophagy in H. pylori-Associated Gastric Cancer
Studies have shown that loss of autophagy may contribute to the development of cancer, but autophagy may also contribute to the development of tumors in turn. On the one hand, autophagy can prevent oxidative stress and DNA damage, which play a key role in inhibiting gastric cancer. On the other hand, autophagy can provide tumor cells with essential metabolites for growth, which can promote the development of gastric cancer (Parzych and Klionsky, 2014; Wang et al., 2021). Changes in the level of autophagy may to a large extent increase the autophagy inducing or inhibiting autophagy activity of tumor cells, and the role of autophagy may be different at different stages of gastric cancer development. In the early stage of tumor growth, tumor vascularization is insufficient and the nutrient supply of cancer cells is limited, thus need enhance autophagy function to provide nutrition for tumors. As soon as the tumor entered the development stage, gene variation accumulated, which inactivated tumor suppressor genes and then reduced autophagy activity (Yang and Klionsky, 2009).Therefore, different drugs play different anti-cancer roles by activating or inhibiting autophagy in different stages of H. pylori-associated gastric cancer.
Chloroquine and Chloroquine Derivatives
Blocking autophagy may be a therapeutic approach to sensitize gastric cancer cells to chemotherapy. Up till now, chloroquine (CQ) and chloroquine derivatives are the lysosomal inhibitors which were most widely drugs used in targeting autophagy therapy. CQ and CQ derivatives have been used in many recent clinical trials and is a most important autophagy modulator in clinical practice. Chloroquine (CQ) blocks autophagy by disrupting lysosome function, and further induces cancer cell death by inhibiting autophagy-mediated ROS20 (Fukuda et al., 2015). Existing literature suggests that, in human cancer cell lines and mouse models, CQ may exhibit significant antitumor activity by inhibiting autophagy induction after cancer treatment (Zhang et al., 2018). In addition, the combination of CQ and cisplatin can improve the sensitivity of cisplatin resistant cells, which indicate that regulation of autophagy can alter the drug resistance of gastric cancer in chemotherapy (Sun et al., 2017).
Proteasome Inhibitors
Proteasome inhibitors are a novel treatment for cancer, which prevent cancer progression by inhibiting the degradation of ubiquitin-proteasome dependent proteins. Bortezomib is a typical representative of 26S proteasome inhibitors, it has previously been reported primarily for the therapy of multiple myeloma (Richardson and Anderson, 2003). Besides that, research has also found that bortezomib also has anti-tumor effects in other malignancies, such as breast, prostate and colon cancers (Dolcet et al., 2006; Periyasamy-Thandavan et al., 2010; Hong et al., 2012). The increase of autophagy capacity after bortezomib treatment mainly depended on the up-regulation on LC3B by ATF4 (Chen et al., 2011; Befani et al., 2012). In addition, bortezomib was found to inhibit the proliferation in gastric cancer cells, and was more effective in gastric cancer with decreasing the NF-κB activity via autophagy (Kao et al., 2014). Bortezomib is a potential novel molecular-targeted autophagy agent with far-reaching promise in the therapy of the unresectable gastric cancer.
Histone Deacetylase Inhibitors
Histone deacetylase inhibitors have become a class of potential antitumor drugs, which can reactivate genes silenced by histone deacetylation. Histone deacetylase inhibitors can induce the autophagy function through FOXO1 signaling pathway, and can also induce the autophagy function by inhibiting mTOR. In addition, it can induce autophagy through the AMPK-FOXO1 signaling pathway in gastric cancer (Chen et al., 2021). There have been reported that histone deacetylase 2 was increased in human gastric cancer, and histone deacetylase 3 can inhibit the expression of DTWD1, which is a cancer suppressor gene in gastric cancer. Cancer suppressor genes Per1 and Per2 can be promoted by the histone deacetylase inhibitors especially in the human gastric cancer cells (Eckschlager et al., 2017). Sgc-7901 xenograft tumor model showed that Valproic acid (2-propylpentanoic acid, VPA) could inhibit histone deacetylase 1/2(HDAC1/2) activity and induce autophagy in gastric cancer cells, which can further inhibit the HDAC1/PTEN/Akt signaling pathway as well as regulate Bclin-1 and Beclin-2 (Sun et al., 2020).
IFN-γ
The natural killer cell (NK) cells are cytotoxic innate lymphocytes that are critical to anti-infection response and play an important role in resisting viral infection, they also have the unique ability to kill cancer cells, limit tumor growth and metastasis (Zingoni et al., 2017). Recent research has confirmed the significance of NK cells in promoting the immune response especially in tumors which intrinsic autophagy loss. In fact, inhibiting autophagy genes lead the massive NK cells proliferation, which can suppress the progression in solid tumors. NK cell activity is decreased in gastric cancer, and gastric cancer mesenchymal stem cells promote tumor growth and inhibit NK cell function through the mTOR signaling pathway. Interferon -γ (IFN-γ) is an important mediator of innate and adaptive immunity, which also an important tumor suppressor (Billiau et al., 1998; Yang et al., 2022). IFN-γ induces a variety of immunomodulatory molecules by regulating the expression levels of multiple genes, such as promote NK cell activity119.IFN-γ induces gastric epithelial autophagy by increasing Beclin-1, which inhibits carcinogenesis in the gastric mucosa via further reducing epithelial cell apoptosis (Tu et al., 2011). In conclusion, as a means of surviving in limited nutritional conditions, restore and replace dysfunctional NK cells may be an attractive therapeutic strategy. Targeting autophagy to activate NK cells activity has great potential in the treatment of gastric cancer patients (Zhou et al., 2018).
Metformin
Metformin is an oral drug, commonly used to treat type 2 diabetes. With more and more understanding of metformin, the function of metformin can improve the prognosis of cancer patients has been identified, thus the randomized controlled trials were implemented to verify the capacity of metformin to prevent cancer or improve cancer outcomes (Kasznicki et al., 2014). Furthermore, some reports suggest that the potential of metformin anticancer properties are related to the regulation of many miRNAs. For instance, metformin targets miRNA-222 to inhibit lung cancer multiplication, and metformin targets miRNA-21 and miRNA-145 to treat relapsed colon cancer (Quinn et al., 2013). Recent studies have shown that metformin acts as an autophagy agonist that induces autophagy by activating AMPK, and this survival promoting process can inhibit gastric cancer growth, reduce invasion and migration of gastric cancer cells, and inhibit the carcinogenic properties of gastric cancer stem cells (Ben Sahra et al., 2011; Meng et al., 2015; Lee et al., 2020). In addition, studies have further suggested that metformin promotes Beclin1-dependent autophagy to inhibit the progression of gastric cancer, which associated with changing the mRNA expression levels of LC3B and Beclin-1 (Takahashi et al., 2014).
Calcium Ion Channel Inhibitors
The involvement of Calcium ion (Ca2+) channels in the regulation of autophagy as an autophagy trigger was discovered in the early 1990s. Studies suggest that intracellular Ca2+ signaling is related to autophagy, the increase of Ca2+ in free cells can activate AMPK and trigger autophagy. In addition, intracellular Ca2+ signaling is required for mTOR dependent autophagy and the lysosomal Ca2+ signaling pathway activates autophagy through the calcineurin. Ca2+ signaling is enhanced when cells are exposed to stress, that is, the induction of autophagy requires an increase in Ca2+ levels (Kondratskyi et al., 2013). Nifedipine and other calcium ion channel inhibitors, may be the potential candidates for targeted autophagy in the therapy of gastric cancer (Filippi-Chiela et al., 2016).
Statins
Recent reports suggest that statins modulate autophagy in tumor cells, and statin contribute to decrease cholesterol levels in the membrane of the phagosome, which in turn activates the host-induced autophagy (Zhu et al., 2021). The nuclear factor-κB is related to the autophagy induced by statins, which can also induce autophagy activated by AMPK, leading to the endoplasmic reticular cell response (Li et al., 2021). In addition, statins can reduce the risk of heterochronous gastric cancer in patients without H. pylori infection (Liao et al., 2016). These results suggest that statins can not only reduce infection caused by H. pylori, but can play a significant role in the therapy of gastric cancer by targeting the activation of autophagy pathway.
Astaxanthin
Astaxanthin, a carotene pigment, is a beneficial compound with excellent antioxidant effects (Hussein et al., 2006). It can inhibit lipid peroxidation, protects cells and reduce DNA damage, enabling proteins inside cells to function better. Clinically, astaxanthin reduces the incidence of atherosclerosis by prolonging the oxidation time of low-density lipoprotein (LDL) and inhibits apoptosis by reducing reactive oxygen species (ROS) (Ikeda et al., 2008; Kim et al., 2018). A recent report suggests that astaxanthin protects AGS cells from H. pylori infection by upregulating cellular protective autophagy and modulating AMPK pathways (Lee et al., 2020). In this study, astaxanthin pretreatment of corresponding cells resulted in increased protein expression levels of AMPK phosphorylation, as well as increased fluorescence of LC3B and acidic vesicle organelles in immunofluorescence, which suggest that astaxanthin can induce autophagy. Further experiments showed that astaxanthin induced autophagy by increasing AMPK phosphorylation and inhibiting its downstream target mTOR. Because mTOR inhibits ULK1 phosphorylation, astaxanthin activates ULK1 and then induces autophagosome formation by influencing the interaction between P62 and LC3B-II in cells. Therefore, astaxanthin has important prospects in targeting autophagy therapy in gastric cancer.
The Combination of Catechins and Sialic Acid
Catechin is a substance with multi-hydroxyl structure, which can play a variety of pharmacological effects, such as antioxidant, antibacterial and anti-tumor (Mabe et al., 1999). Sialic acid, a tetrapeptide containing arginine, is found naturally in saliva and has a powerful antioxidant function (Chun et al., 2007). The potential of catechins and sialic acid for the prevention and therapy of H. pylori infected gastric epithelial cells has been explored in vitro and in vivo (Yang et al., 2008; Yang and Chien, 2009; Yang et al., 2013). The combination of catechins and sialic acid can significantly enhanced autophagy mediated by Beclin-1. Catechins and sialic acid reduce H. pylori density on the surface of the gastric lumen through their antibacterial and anti-adhesion properties firstly, then they inhibit the production of ROS derived from CD68 and NADPH oxidase, which can induce autophagy mediated by Beclin-1, reduce caspase-1 activation and IL-1β secretion subsequently. In addition, the combination of catechins and sialic acid can eradicate H. pylori in a dose-dependent manner, which can be the novel anti-H. pylori drugs and have important significance in the therapy of H. pylori-associated gastric cancer.
Discussion
The mechanisms of autophagy regulated by H. pylori infection in gastric epithelial cell are quite complicated. The signaling pathways of gastric cancer that are related to H. pylori infection mediated by autophagy involve a multichannel and multifactor protein network regulation system. Various signaling pathways play their respective roles and can interact with each other. Once a signaling pathway is activated or abnormally induced, it may cause a series of cellular immune damage, eventually leading to the occurrence of gastric cancer. Therefore, targeted autophagy therapy of H. pylori-associated gastric cancer has become a new hot spot, representing a fresh orientation for the therapy of gastric cancer that combines different signal transduction pathways. Through targeted autophagy therapy, seeking multiple regulatory points of autophagy pathways and blocking signal transduction, thus finally achieve inhibition of gastric cancer development.
Author Contributions
YY wrote the manuscript; XS and CX revised the review. All authors contributed to the article and approved the submitted version.
Funding
The National Natural Science Foundation of China (No. 81060038 and 81270479), grants from the Jiangxi Province Talent 555 Project, and the National Science and Technology Major Projects for “Major New Drugs Innovation and Development” of China (No. 2011ZX09302-007-03).
Conflict of Interest
The authors declare that the research was conducted in the absence of any commercial or financial relationships that could be construed as a potential conflict of interest.
Publisher’s Note
All claims expressed in this article are solely those of the authors and do not necessarily represent those of their affiliated organizations, or those of the publisher, the editors and the reviewers. Any product that may be evaluated in this article, or claim that may be made by its manufacturer, is not guaranteed or endorsed by the publisher.
References
Ahn, H. J., Lee, D. S. (2015). Helicobacter Pylori in Gastric Carcinogenesis. World J. Gastrointest. Oncol. 7 (12), 455–465. doi: 10.4251/wjgo.v7.i12.455
Allen, L. A. (2007). Phagocytosis and Persistence of Helicobacter Pylori. Cell Microbiol. 9 (4), 817–828. doi: 10.1111/j.1462-5822.2007.00906.x
Allen, L. A., Schlesinger, L. S., Kang, B. (2000). Virulent Strains of Helicobacter Pylori Demonstrate Delayed Phagocytosis and Stimulate Homotypic Phagosome Fusion in Macrophages. J. Exp. Med. 191 (1), 115–128. doi: 10.1084/jem.191.1.115
Ashrafizadeh, M., Najafi, M., Ang, H. L., Moghadam, E. R., Mahabady, M. K., Zabolian, A., et al. (2020). PTEN, a Barrier for Proliferation and Metastasis of Gastric Cancer Cells: From Molecular Pathways to Targeting and Regulation. Biomedicines 8 (8), 264. doi: 10.3390/biomedicines8080264
Barth, S., Glick, D., Macleod, K. F. (2010). Autophagy: Assays and Artifacts. J. Pathol. 221 (2), 117–124. doi: 10.1002/path.2694
Basak, C., Pathak, S. K., Bhattacharyya, A., Pathak, S., Basu, J., Kundu, M. (2005). The Secreted Peptidyl Prolyl Cis,Trans-Isomerase HP0175 of Helicobacter Pylori Induces Apoptosis of Gastric Epithelial Cells in a TLR4- and Apoptosis Signal-Regulating Kinase 1-Dependent Manner. J. Immunol. 174 (9), 5672–5680. doi: 10.4049/jimmunol.174.9.5672
Befani, C. D., Vlachostergios, P. J., Hatzidaki, E., Patrikidou, A., Bonanou, S., Simos, G., et al. (2012). Bortezomib Represses HIF-1α Protein Expression and Nuclear Accumulation by Inhibiting Both PI3K/Akt/TOR and MAPK Pathways in Prostate Cancer Cells. J. Mol. Med. (Berl.) 90 (1), 45–54. doi: 10.1007/s00109-011-0805-8
Ben Sahra, I., Regazzetti, C., Robert, G., Laurent, K., Le Marchand-Brustel, Y., Auberger, P., et al. (2011). Metformin, Independent of AMPK, Induces mTOR Inhibition and Cell-Cycle Arrest Through REDD1. Cancer Res. 71 (13), 4366–4372. doi: 10.1158/0008-5472.CAN-10-1769
Billiau, A., Heremans, H., Vermeire, K., Matthys, P. (1998). Immunomodulatory Properties of Interferon-Gamma. An Update. Ann. N. Y. Acad. Sci. 856, 22–32. doi: 10.1111/j.1749-6632.1998.tb08309.x
Blaser, M. J. (1987). Gastric Campylobacter-Like Organisms, Gastritis, and Peptic Ulcer Disease. Gastroenterology 93 (2), 371–383. doi: 10.1016/0016-5085(87)91028-6
Boneca, I. G., Ecobichon, C., Chaput, C., Mathieu, A., Guadagnini, S., Prévost, M. C., et al. (2008). Development of Inducible Systems to Engineer Conditional Mutants of Essential Genes of Helicobacter Pylori. Appl. Environ. Microbiol. 74 (7), 2095–2102. doi: 10.1128/AEM.01348-07
Brandt, S., Kwok, T., Hartig, R., König, W., Backert, S. (2005). NF-kappaB Activation and Potentiation of Proinflammatory Responses by the Helicobacter Pylori CagA Protein. Proc. Natl. Acad. Sci. U. S. A. 102 (26), 9300–9305. doi: 10.1073/pnas.0409873102
Bravo, J., Díaz, P., Corvalán, A. H., Quest, A. F. G. (2019). A Novel Role for Helicobacter Pylori Gamma-Glutamyltranspeptidase in Regulating Autophagy and Bacterial Internalization in Human Gastric Cells. Cancers (Basel) 11 (6). doi: 10.3390/cancers11060801
Castaño-Rodríguez, N., Kaakoush, N. O., Goh, K. L., Fock, K. M., Mitchell, H. M. (2015). Autophagy in Helicobacter Pylori Infection and Related Gastric Cancer. Helicobacter 20 (5), 353–369. doi: 10.1111/hel.12211
Chen, D., Frezza, M., Schmitt, S., Kanwar, J., Dou, Q. P. (2011). Bortezomib as the First Proteasome Inhibitor Anticancer Drug: Current Status and Future Perspectives. Curr. Cancer Drug Targets 11 (3), 239–253. doi: 10.2174/156800911794519752
Chen, Y. H., Li, C. L., Chen, W. J., Liu, J., Wu, H. T. (2021). Diverse Roles of FOXO Family Members in Gastric Cancer. World J. Gastrointest. Oncol. 13 (10), 1367–1382. doi: 10.4251/wjgo.v13.i10.1367
Chevalier, C., Thiberge, J. M., Ferrero, R. L., Labigne, A. (1999). Essential Role of Helicobacter Pylori Gamma-Glutamyltranspeptidase for the Colonization of the Gastric Mucosa of Mice. Mol. Microbiol. 31 (5), 1359–1372. doi: 10.1046/j.1365-2958.1999.01271.x
Chun, O. K., Chung, S. J., Song, W. O. (2007). Estimated Dietary Flavonoid Intake and Major Food Sources of U.S. Adults. J. Nutr. 137 (5), 1244–1252. doi: 10.1093/jn/137.5.1244
Cuervo, A. M., Wong, E. (2014). Chaperone-Mediated Autophagy: Roles in Disease and Aging. Cell Res. 24 (1), 92–104. doi: 10.1038/cr.2013.153
Deen, N. S., Gong, L., Naderer, T., Devenish, R. J., Kwok, T. (2015). Analysis of the Relative Contribution of Phagocytosis, LC3-Associated Phagocytosis, and Canonical Autophagy During Helicobacter Pylori Infection of Macrophages. Helicobacter 20 (6), 449–459. doi: 10.1111/hel.12223
Deen, N. S., Huang, S. J., Gong, L., Kwok, T., Devenish, R. J. (2013). The Impact of Autophagic Processes on the Intracellular Fate of Helicobacter Pylori: More Tricks From an Enigmatic Pathogen? Autophagy 9 (5), 639–652. doi: 10.4161/auto.23782
Delbrück, R., Desel, C., von Figura, K., Hille-Rehfeld, A. (1994). Proteolytic Processing of Cathepsin D in Prelysosomal Organelles. Eur. J. Cell Biol. 64 (1), 7–14.
Dolcet, X., Llobet, D., Encinas, M., Pallares, J., Cabero, A., Schoenenberger, J. A., et al. (2006). Proteasome Inhibitors Induce Death But Activate NF-kappaB on Endometrial Carcinoma Cell Lines and Primary Culture Explants. J. Biol. Chem. 281 (31), 22118–22130. doi: 10.1074/jbc.M601350200
Eckschlager, T., Plch, J., Stiborova, M., Hrabeta, J. (2017). Histone Deacetylase Inhibitors as Anticancer Drugs. Int. J. Mol. Sci. 18 (7). doi: 10.3390/ijms18071414
Eslami, M., Yousefi, B., Kokhaei, P., Arabkari, V., Ghasemian, A. (2019). Current Information on the Association of Helicobacter Pylori With Autophagy and Gastric Cancer. J. Cell Physiol. doi: 10.1002/jcp.28279
Fan, X., Long, A., Goggins, M., Fan, X., Keeling, P. W., Kelleher, D. (1996). Expression of CD44 and its Variants on Gastric Epithelial Cells of Patients With Helicobacter Pylori Colonisation. Gut 38 (4), 507–512. doi: 10.1136/gut.38.4.507
Feng, G. J., Chen, Y., Li, K. (2020). Helicobacter Pylori Promote Inflammation and Host Defense Through the cagA-Dependent Activation of Mtorc1. J. Cell. Physiol. 235 (12), 10094–10108. doi: 10.1002/jcp.29826
Filippi-Chiela, E. C., Viegas, M. S., Thomé, M. P., Buffon, A., Wink, M. R., Lenz, G. (2016). Modulation of Autophagy by Calcium Signalosome in Human Disease. Mol. Pharmacol. 90 (3), 371–384. doi: 10.1124/mol.116.105171
Florey, O., Kim, S. E., Sandoval, C. P., Haynes, C. M., Overholtzer, M. (2011). Autophagy Machinery Mediates Macroendocytic Processing and Entotic Cell Death by Targeting Single Membranes. Nat. Cell Biol. 13 (11), 1335–1343. doi: 10.1038/ncb2363
Florey, O., Overholtzer, M. (2012). Autophagy Proteins in Macroendocytic Engulfment. Trends Cell Biol. 22 (7), 374–380. doi: 10.1016/j.tcb.2012.04.005
Fujita, N., Itoh, T., Omori, H., Fukuda, M., Noda, T., Yoshimori, T. (2008). The Atg16L Complex Specifies the Site of LC3 Lipidation for Membrane Biogenesis in Autophagy. Mol. Biol. Cell 19 (5), 2092–2100. doi: 10.1091/mbc.e07-12-1257
Fukuda, T., Oda, K., Wada-Hiraike, O., Sone, K., Inaba, K., Ikeda, Y., et al. (2015). The Anti-Malarial Chloroquine Suppresses Proliferation and Overcomes Cisplatin Resistance of Endometrial Cancer Cells via Autophagy Inhibition. Gynecol. Oncol. 137 (3), 538–545. doi: 10.1016/j.ygyno.2015.03.053
Galluzzi, L., Bravo-San Pedro, J. M., Levine, B., Green, D. R., Kroemer, G. (2017). Pharmacological Modulation of Autophagy: Therapeutic Potential and Persisting Obstacles. Nat. Rev. Drug Discov. 16 (7), 487–511. doi: 10.1038/nrd.2017.22
Gao, J., Hu, H., Wang, X. (2019). Clinically Relevant Concentrations of Lidocaine Inhibit Tumor Angiogenesis Through Suppressing VEGF/VEGFR2 Signaling. Cancer Chemother. Pharmacol. 83 (6), 1007–1015. doi: 10.1007/s00280-019-03815-4
Glick, D., Barth, S., Macleod, K. F. (2010). Autophagy: Cellular and Molecular Mechanisms. J. Pathol. 221 (1), 3–12. doi: 10.1002/path.2697
Greenfield, L. K., Jones, N. L. (2013). Modulation of Autophagy by Helicobacter Pylori and its Role in Gastric Carcinogenesis. Trends Microbiol. 21 (11), 602–612. doi: 10.1016/j.tim.2013.09.004
Guo, W., Dong, Z., Guo, Y., Kuang, G., Yang, Z., Shan, B. (2012). Concordant Repression and Aberrant Methylation of Transforming Growth Factor-Beta Signaling Pathway Genes Occurs Early in Gastric Cardia Adenocarcinoma. Mol. Biol. Rep. 39 (10), 9453–9462. doi: 10.1007/s11033-012-1810-x
Halder, P., Datta, C., Kumar, R., Sharma, A. K., Basu, J., Kundu, M. (2015). The Secreted Antigen, HP0175, of Helicobacter Pylori Links the Unfolded Protein Response (UPR) to Autophagy in Gastric Epithelial Cells. Cell. Microbiol. 17 (5), 714–729. doi: 10.1111/cmi.12396
Henault, J., Martinez, J., Riggs, J. M., Tian, J., Mehta, P., Clarke, L., et al. (2012). Noncanonical Autophagy is Required for Type I Interferon Secretion in Response to DNA-Immune Complexes. Immunity 37 (6), 986–997. doi: 10.1016/j.immuni.2012.09.014
He, Y., Wang, C., Zhang, X., Lu, X., Xing, J., Lv, J., et al. (2020). Sustained Exposure to Lysate Inhibits Apoptosis and Autophagy of Gastric Epithelial Cells. Front. Oncol. 10, 581364. doi: 10.3389/fonc.2020.581364
Hong, Y. S., Hong, S.-W., Kim, S.-M., Jin, D.-H., Shin, J.-S., Yoon, D. H., et al. (2012). Bortezomib Induces G2-M Arrest in Human Colon Cancer Cells Through ROS-Inducible Phosphorylation of ATM-CHK1. Int. J. Oncol. 41 (1), 76–82. doi: 10.3892/ijo.2012.1448
Hussein, G., Sankawa, U., Goto, H., Matsumoto, K., Watanabe, H. (2006). Astaxanthin, a Carotenoid With Potential in Human Health and Nutrition. J. Nat. Prod. 69, 443–449. doi: 10.1021/np050354+
Ikeda, Y., Tsuji, S., Satoh, A., Ishikura, M., Shirasawa, T., Shimizu, T. (2008). Protective Effects of Astaxanthin on 6-Hydroxydopamine-Induced Apoptosis in Human Neuroblastoma SH-SY5Y Cells. J. Neurochem. 107, 1730–1740. doi: 10.1111/j.1471-4159.2008.05743.x
Isomoto, H., Moss, J., Hirayama, T. (2010). Pleiotropic Actions of Helicobacter Pylori Vacuolating Cytotoxin, VacA. Tohoku J. Exp. Med. 220 (1). doi: 10.1620/tjem.220.3
Kao, C., Chao, A., Tsai, C. L., Chuang, W. C., Huang, W. P., Chen, G. C., et al. (2014). Bortezomib Enhances Cancer Cell Death by Blocking the Autophagic Flux Through Stimulating ERK Phosphorylation. Cell Death Dis. 5 (11), e1510. doi: 10.1038/cddis.2014.468
Kasznicki, J., Sliwinska, A., Drzewoski, J. (2014). Metformin in Cancer Prevention and Therapy. Ann. Transl. Med. 2 (6), 57. doi: 10.3978/j.issn.2305-5839.2014.06.01
Kaushik, S., Cuervo, A. M. (2012). Chaperone-Mediated Autophagy: A Unique Way to Enter the Lysosome World. Trends Cell Biol. 22 (8), 407–417. doi: 10.1016/j.tcb.2012.05.006
Ke, J., Ma, P., Chen, J., Qin, J., Qian, H. (2018). LGR6 Promotes the Progression of Gastric Cancer Through PI3K/AKT/mTOR Pathway. Onco. Targets Ther. 11, 3025–3033. doi: 10.2147/OTT.S149303
Kidd, M. E., Shumaker, D. K., Ridge, K. M. (2014). The Role of Vimentin Intermediate Filaments in the Progression of Lung Cancer. Am. J. Respir. Cell Mol. Biol. 50 (1), 1–6. doi: 10.1165/rcmb.2013-0314TR
Kim, S. H., Lim, J. W., Kim, H. (2018). Astaxanthin Inhibits Mitochondrial Dysfunction and Interleukin-8 Expression in Helicobacter Pylor-Infected Gastric Epithelial Cells. Nutrients 10, 1320. doi: 10.3390/nu10091320
Kim, N., Weeks, D. L., Shin, J. M., Scott, D. R., Young, M. K., Sachs, G. (2002). Proteins Released by Helicobacter Pylori In Vitro. J. Bacteriol. 184 (22), 6155–6162. doi: 10.1128/JB.184.22.6155-6162.2002
Kim, J. Y., Zhao, H., Martinez, J., Doggett, T. A., Kolesnikov, A. V., Tang, P. H., et al. (2013). Noncanonical Autophagy Promotes the Visual Cycle. Cell 154 (2), 365–376. doi: 10.1016/j.cell.2013.06.012
Kondratskyi, A., Yassine, M., Kondratska, K., Skryma, R., Slomianny, C., Prevarskaya, N. (2013). Calcium-Permeable Ion Channels in Control of Autophagy and Cancer. Front. Physiol. 4, 272. doi: 10.3389/fphys.2013.00272
Kroemer, G., Mariño, G., Levine, B. (2010). Autophagy and the Integrated Stress Response. Mol. Cell 40 (2), 280–293. doi: 10.1016/j.molcel.2010.09.023
Kumar, S., Dhiman, M. (2018). Inflammasome Activation and Regulation During Helicobacter Pylori Pathogenesis. Microb. Pathog. 125, 468–474. doi: 10.1016/j.micpath.2018.10.012
Lai, C. H., Huang, J. C., Cheng, H. H., Wu, M. C., Huang, M. Z., Hsu, H. Y., et al. (2018). Helicobacter Pylori Cholesterol Glucosylation Modulates Autophagy for Increasing Intracellular Survival in Macrophages. Cell Microbiol. 20 (12), e12947. doi: 10.1111/cmi.12947
Lee, H., Lim, J. W., Kim, H. (2020). Effect of Astaxanthin on Activation of Autophagy and Inhibition of Apoptosis in Helicobacter Pylori-Infected Gastric Epithelial Cell Line AGS. Nutrients 12 (6). doi: 10.3390/nu12061750
Lee, H. J., Venkatarame Gowda Saralamma, V., Kim, S. M., Ha, S. E., Raha, S., Lee, W. S., et al. (2018). Autophagy, and Apoptosis in Gastric Cancer Cell via PI3K/AKT/mTOR Signaling Pathway. Nutrients 10 (8). doi: 10.3390/nu10081043
Liao, W. C., Huang, M. Z., Wang, M. L., Lin, C. J., Lu, T. L., Lo, H. R., et al. (2016). Statin Decreases Helicobacter Pylori Burden in Macrophages by Promoting Autophagy. Front. Cell Infect. Microbiol. 6, 203. doi: 10.3389/fcimb.2016.00203
Li, Z., Li, J., Miao, X., Cui, W., Miao, L., Cai, L. (2021). A Minireview: Role of AMP-Activated Protein Kinase (AMPK) Signaling in Obesity-Related Renal Injury. Life Sci. 265, 118828. doi: 10.1016/j.lfs.2020.118828
Lim, M. C. C., Maubach, G., Sokolova, O., Feige, M. H., Diezko, R., Buchbinder, J., et al. (2017). Pathogen-Induced Ubiquitin-Editing Enzyme A20 Bifunctionally Shuts Off NF-κb and Caspase-8-Dependent Apoptotic Cell Death. Cell Death Differ. 24 (9), 1621–1631. doi: 10.1038/cdd.2017.89
Li, N., Tang, B., Jia, Y. P., Zhu, P., Zhuang, Y., Fang, Y., et al. (2017). Helicobacter Pylori CagA Protein Negatively Regulates Autophagy and Promotes Inflammatory Response via C-Met-PI3K/Akt-mTOR Signaling Pathway. Front. Cell. Iinfect. Microbiol. 7, 417. doi: 10.3389/fcimb.2017.00417
Liu, F.-L., Mo, E.-P., Yang, L., Du, J., Wang, H.-S., Zhang, H., et al. (2016). Autophagy Is Involved in TGF-β1-Induced Protective Mechanisms and Formation of Cancer-Associated Fibroblasts Phenotype in Tumor Microenvironment. Oncotarget 7 (4), 4122–4141. doi: 10.18632/oncotarget.6702
Li, Z. X., Wang, Y. M., Tang, F. B., Zhang, L., Zhang, Y., Ma, J. L., et al. (2015). NOD1 and NOD2 Genetic Variants in Association With Risk of Gastric Cancer and Its Precursors in a Chinese Population. PloS One 10 (5). doi: 10.1371/journal.pone.0124949
Li, L., Zhu, X., Shou, T., Yang, L., Cheng, X., Wang, J., et al. (2018). MicroRNA-28 Promotes Cell Proliferation and Invasion in Gastric Cancer via the PTEN/PI3K/AKT Signalling Pathway. Mol. Med. Rep. 17 (3), 4003–4010. doi: 10.3892/mmr.2017.8299
Ma, J., Becker, C., Lowell, C. A., Underhill, D. M. (2012). Dectin-1-Triggered Recruitment of Light Chain 3 Protein to Phagosomes Facilitates Major Histocompatibility Complex Class II Presentation of Fungal-Derived Antigens. J. Biol. Chem. 287 (41), 34149–34156. doi: 10.1074/jbc.M112.382812
Mabe, K., Yamada, M., Oguni, I., Takahashi, T. (1999). In Vitro and In Vivo Activities of Tea Catechins Against Helicobacter Pylori. Antimicrob. Agents Chemother. 43 (7), 1788–1791. doi: 10.1128/AAC.43.7.1788
Maes, H., Rubio, N., Garg, A. D., Agostinis, P. (2013). Autophagy: Shaping the Tumor Microenvironment and Therapeutic Response. Trends Mol. Med. 19 (7), 428–446. doi: 10.1016/j.molmed.2013.04.005
Martinez, J., Almendinger, J., Oberst, A., Ness, R., Dillon, C. P., Fitzgerald, P., et al. (2011). Microtubule-Associated Protein 1 Light Chain 3 Alpha (LC3)-Associated Phagocytosis is Required for the Efficient Clearance of Dead Cells. Proc. Natl. Acad. Sci. U. S. A. 108 (42), 17396–17401. doi: 10.1073/pnas.1113421108
Matos, J. I., de Sousa, H. A. C., Marcos-Pinto, R., Dinis-Ribeiro, M. (2013). Helicobacter Pylori CagA and VacA Genotypes and Gastric Phenotype: A Meta-Analysis. Eur. J. Gastroenterol. Hepatol. 25 (12), 1431–1441. doi: 10.1097/MEG.0b013e328364b53e
Mehta, P., Henault, J., Kolbeck, R., Sanjuan, M. A. (2014). Noncanonical Autophagy: One Small Step for LC3, One Giant Leap for Immunity. Curr. Opin. Immunol. 26, 69–75. doi: 10.1016/j.coi.2013.10.012
Meng, S., Cao, J., He, Q., Xiong, L., Chang, E., Radovick, S., et al. (2015). Metformin Activates AMP-Activated Protein Kinase by Promoting Formation of the αβγ Heterotrimeric Complex. J. Biol. Chem. 290 (6), 3793–3802. doi: 10.1074/jbc.M114.604421
Mimuro, H., Suzuki, T., Tanaka, J., Asahi, M., Haas, R., Sasakawa, C. (2002). Grb2 is a Key Mediator of Helicobacter Pylori CagA Protein Activities. Mol. Cell 10 (4), 745–755. doi: 10.1016/S1097-2765(02)00681-0
Mizushima, N., Levine, B. (2010). Autophagy in Mammalian Development and Differentiation. Nat. Cell Biol. 12 (9), 823–830. doi: 10.1038/ncb0910-823
Mizushima, N., Yoshimori, T., Ohsumi, Y. (2011). The Role of Atg Proteins in Autophagosome Formation. Annu. Rev. Cell Dev. Biol. 27, 107–132. doi: 10.1146/annurev-cellbio-092910-154005
Muhammad, J. S., Nanjo, S., Ando, T., Yamashita, S., Maekita, T., Ushijima, T., et al. (2017). Autophagy Impairment by Helicobacter Pylori-Induced Methylation Silencing of MAP1LC3Av1 Promotes Gastric Carcinogenesis. Int. J. Cancer 140 (10), 2272–2283. doi: 10.1002/ijc.30657
Odenbreit, S., Püls, J., Sedlmaier, B., Gerland, E., Fischer, W., Haas, R. (2000). Translocation of Helicobacter Pylori CagA Into Gastric Epithelial Cells by Type IV Secretion. Science 287 (5457), 1497–1500. doi: 10.1126/science.287.5457.1497
Pang, K., Song, J., Bai, Z., Zhang, Z. (2020). miR-15a-5p Targets PHLPP2 in Gastric Cancer Cells to Modulate Platinum Resistance and is a Suitable Serum Biomarker for Oxaliplatin Resistance. Neoplasma 67 (5), 1114–1121. doi: 10.4149/neo_2020_190904N861
Parzych, K. R., Klionsky, D. J. (2014). An Overview of Autophagy: Morphology, Mechanism, and Regulation. Antioxid. Redox Signal. 20 (3), 460–473. doi: 10.1089/ars.2013.5371
Periyasamy-Thandavan, S., Jackson, W. H., Samaddar, J. S., Erickson, B., Barrett, J. R., Raney, L., et al. (2010). Bortezomib Blocks the Catabolic Process of Autophagy via a Cathepsin-Dependent Mechanism, Affects Endoplasmic Reticulum Stress and Induces Caspase-Dependent Cell Death in Antiestrogen-Sensitive and Resistant ER+ Breast Cancer Cells. Autophagy 6 (1), 19–35. doi: 10.4161/auto.6.1.10323
Plummer, M., Franceschi, S., Vignat, J., Forman, D., de Martel, C. (2015). Global Burden of Gastric Cancer Attributable to Helicobacter Pylori. Int. J. Cancer 136 (2), 487–490. doi: 10.1002/ijc.28999
Pormohammad, A., Ghotaslou, R., Leylabadlo, H. E., Nasiri, M. J., Dabiri, H., Hashemi, A. (2018). Risk of Gastric Cancer in Association With Helicobacter Pylori Different Virulence Factors: A Systematic Review and Meta-Analysis. Microb. Pathog. 118, 214–219. doi: 10.1016/j.micpath.2018.03.004
Puissant, A., Fenouille, N., Auberger, P. (2012). When Autophagy Meets Cancer Through P62/SQSTM1. Am. J. Cancer Res. 2 (4), 397–413.
Quinn, B. J., Kitagawa, H., Memmott, R. M., Gills, J. J., Dennis, P. A. (2013). Repositioning Metformin for Cancer Prevention and Treatment. Trends Endocrinol. Metab. 24 (9), 469–480. doi: 10.1016/j.tem.2013.05.004
Raju, D., Hussey, S., Ang, M., Terebiznik, M. R., Sibony, M., Galindo-Mata, E., et al. (2012). Vacuolating Cytotoxin and Variants in Atg16L1 That Disrupt Autophagy Promote Helicobacter Pylori Infection in Humans. Gastroenterology 142 (5), 1160–1171. doi: 10.1053/j.gastro.2012.01.043
Reggiori, F., Komatsu, M., Finley, K., Simonsen, A. (2012). Autophagy: More Than a Nonselective Pathway. Int. J. Cell Biol. 2012, 219625. doi: 10.1155/2012/219625
Rhead, J. L., Letley, D. P., Mohammadi, M., Hussein, N., Mohagheghi, M. A., Eshagh Hosseini, M., et al. (2007). A New Helicobacter Pylori Vacuolating Cytotoxin Determinant, the Intermediate Region, Is Associated With Gastric Cancer. Gastroenterology 133 (3), 926–936. doi: 10.1053/j.gastro.2007.06.056
Ricci, V. (2016). Relationship Between VacA Toxin and Host Cell Autophagy in Helicobacter Pylori Infection of the Human Stomach: A Few Answers, Many Questions. Toxins (Basel) 8 (7). doi: 10.3390/toxins8070203
Richardson, P. G., Anderson, K. C. (2003). Bortezomib: A Novel Therapy Approved for Multiple Myeloma. Clin. Adv. Hematol. Oncol. 1 (10), 596–600.
Rijnboutt, S., Stoorvogel, W., Geuze, H. J., Strous, G. J. (1992). Identification of Subcellular Compartments Involved in Biosynthetic Processing of Cathepsin D. J. Biol. Chem. 267 (22), 15665–15672. doi: 10.1016/S0021-9258(19)49587-2
Ryter, S. W., Cloonan, S. M., Choi, A. M. K. (2013). Autophagy: A Critical Regulator of Cellular Metabolism and Homeostasis. Mol. Cells 36 (1). doi: 10.1007/s10059-013-0140-8
Sanjuan, M. A., Dillon, C. P., Tait, S. W., Moshiach, S., Dorsey, F., Connell, S., et al. (2007). Toll-Like Receptor Signalling in Macrophages Links the Autophagy Pathway to Phagocytosis. Nature 450 (7173), 1253–1257. doi: 10.1038/nature06421
Schulz, C., Schütte, K., Malfertheiner, P. (2016). Helicobacter Pylori and Other Gastric Microbiota in Gastroduodenal Pathologies. Dig. Dis. 34 (3), 210–216. doi: 10.1159/000443353
Selbach, M., Moese, S., Hurwitz, R., Hauck, C. R., Meyer, T. F., Backert, S. (2015). The Helicobacter Pylori CagA Protein Induces Cortactin Dephosphorylation and Actin Rearrangement by C-Src Inactivation. EMBO J. 22 (3), 515–528. doi: 10.1093/emboj/cdg050
Semino-Mora, C., Doi, S. Q., Marty, A., Simko, V., Carlstedt, I., Dubois, A. (2003). Intracellular and Interstitial Expression of Helicobacter Pylori Virulence Genes in Gastric Precancerous Intestinal Metaplasia and Adenocarcinoma. J. Infect. Dis. 187 (8), 1165–1177. doi: 10.1086/368133
Shen, E., Wang, X., Liu, X., Lv, M., Zhang, L., Zhu, G., et al. (2020). MicroRNA-93-5p Promotes Epithelial-Mesenchymal Transition in Gastric Cancer by Repressing Tumor Suppressor AHNAK Expression. Cancer Cell Int. 20, 76. doi: 10.1186/s12935-019-1092-7
Smyth, E. C., Nilsson, M., Grabsch, H. I., Van Grieken, N. C., Lordick, F. (2020). Gastric Cancer. Lancet 396 (10251), 635–648. doi: 10.1016/S0140-6736(20)31288-5
Suarez, G., Romero-Gallo, J., Piazuelo, M. B., Sierra, J. C., Delgado, A. G., Washington, M. K., et al. (2019). Nod1 Imprints Inflammatory and Carcinogenic Responses Toward the Gastric Pathogen Helicobacter Pylori. Cancer Res. 79 (7), 1600–1611. doi: 10.1158/0008-5472.CAN-18-2651
Sun, J., Piao, J., Li, N., Yang, Y., Kim, K. Y., Lin, Z. (2020). Valproic Acid Targets HDAC1/2 and HDAC1/PTEN/Akt Signalling to Inhibit Cell Proliferation via the Induction of Autophagy in Gastric Cancer. FEBS J. 287 (10), 2118–2133. doi: 10.1111/febs.15122
Sun, M.-Y., Zhu, J.-Y., Zhang, C.-Y., Zhang, M., Song, Y.-N., Rahman, K., et al. (2017). Autophagy Regulated by lncRNA HOTAIR Contributes to the Cisplatin-Induced Resistance in Endometrial Cancer Cells. Biotechnol. Lett. 39 (10), 1477–1484. doi: 10.1007/s10529-017-2392-4
Suzuki, M., Mimuro, H., Kiga, K., Fukumatsu, M., Ishijima, N., Morikawa, H., et al. (2009). Helicobacter Pylori CagA Phosphorylation-Independent Function in Epithelial Proliferation and Inflammation. Cell Host Microbe 5 (1), 23–34. doi: 10.1016/j.chom.2008.11.010
Takahashi-Kanemitsu, A., Knight, C. T., Hatakeyama, M. (2020). Molecular Anatomy and Pathogenic Actions of Helicobacter Pylori CagA That Underpin Gastric Carcinogenesis. Cell Mol. Immunol. 17 (1), 50–63. doi: 10.1038/s41423-019-0339-5
Takahashi, A., Kimura, F., Yamanaka, A., Takebayashi, A., Kita, N., Takahashi, K., et al. (2014). Metformin Impairs Growth of Endometrial Cancer Cells via Cell Cycle Arrest and Concomitant Autophagy and Apoptosis. Cancer Cell Int. 14, 53. doi: 10.1186/1475-2867-14-53
Tang, B., Li, N., Gu, J., Zhuang, Y., Li, Q., Wang, H. G., et al. (2012). Compromised Autophagy by MIR30B Benefits the Intracellular Survival of Helicobacter Pylori. Autophagy 8 (7), 1045–1057. doi: 10.4161/auto.20159
Terebiznik, M. R., Raju, D., Vázquez, C. L., Torbricki, K., Kulkarni, R., Blanke, S. R., et al. (2009). Effect of Helicobacter Pylori’s Vacuolating Cytotoxin on the Autophagy Pathway in Gastric Epithelial Cells. Autophagy 5 (3), 370–379. doi: 10.4161/auto.5.3.7663
Terebiznik, M. R., Vazquez, C. L., Torbicki, K., Banks, D., Wang, T., Hong, W., et al. (2006). Helicobacter Pylori VacA Toxin Promotes Bacterial Intracellular Survival in Gastric Epithelial Cells. Infect. Immun. 74 (12), 6599–6614. doi: 10.1128/IAI.01085-06
Tohidpour, A. (2016). CagA-Mediated Pathogenesis of Helicobacter Pylori. Microb. Pathog 93, 44–55. doi: 10.1016/j.micpath.2016.01.005
Tsai, M. C., Wang, C. C., Lee, H. L., Peng, C. M., Yang, T. W., Chen, H. Y., et al. (2017). Health Disparities are Associated With Gastric Cancer Mortality-to-Incidence Ratios in 57 Countries. World J. Gastroenterol. 23 (44), 7881–7887. doi: 10.3748/wjg.v23.i44.7881
Tsugawa, H., Suzuki, H., Saya, H., Hatakeyama, M., Hirayama, T., Hirata, K., et al. (2012). Reactive Oxygen Species-Induced Autophagic Degradation of Helicobacter Pylori CagA is Specifically Suppressed in Cancer Stem-Like Cells. Cell Host Microbe 12 (6), 764–777. doi: 10.1016/j.chom.2012.10.014
Tu, S. P., Quante, M., Bhagat, G., Takaishi, S., Cui, G., Yang, X. D., et al. (2011). IFN-γ Inhibits Gastric Carcinogenesis by Inducing Epithelial Cell Autophagy and T-Cell Apoptosis. Cancer Res. 71 (12), 4247–4259. doi: 10.1158/0008-5472.CAN-10-4009
vianna, J. S., Ramis, I. B., Halicki, P. C., Gastal, O. L., Silva, R. A., Junior, J. S., et al. (2015). Detection of Helicobacter Pylori CagA EPIYA in Gastric Biopsy Specimens and its Relation to Gastric Diseases. Diagn. Microbiol. Infect. Dis. 83 (2), 89–92. doi: 10.1016/j.diagmicrobio.2015.05.017
Wang, Y., Du, J., Wu, X., Abdelrehem, A., Ren, Y., Liu, C., et al. (2021). Crosstalk Between Autophagy and Microbiota in Cancer Progression. Mol. Cancer 20 (1), 163. doi: 10.1186/s12943-021-01461-0
Wang, C., Tan, C., Wen, Y., Zhang, D., Li, G., Chang, L., et al. (2019). FOXP1-Induced lncRNA CLRN1-AS1 Acts as a Tumor Suppressor in Pituitary Prolactinoma by Repressing the Autophagy via Inactivating Wnt/β-Catenin Signaling Pathway. Cell Death Dis. 10 (7), 499. doi: 10.1038/s41419-019-1694-y
Willhite, D. C., Cover, T. L., Blanke, S. R. (2003). Cellular Vacuolation and Mitochondrial Cytochrome C Release are Independent Outcomes of Helicobacter Pylori Vacuolating Cytotoxin Activity That Are Each Dependent on Membrane Channel Formation. J. Biol. Chem. 278 (48), 48204–48209. doi: 10.1074/jbc.M304131200
Wroblewski, L. E., Peek, R. M., Jr., Wilson, K. T. (2010). Helicobacter Pylori and Gastric Cancer: Factors That Modulate Disease Risk. Clin. Microbiol. Rev. 23 (4), 713–739. doi: 10.1128/CMR.00011-10
Wu, Y. C., Tang, S. J., Sun, G. H., Sun, K. H. (2016). CXCR7 Mediates Tgfβ1-Promoted EMT and Tumor-Initiating Features in Lung Cancer. Oncogene 35 (16), 2123–2132. doi: 10.1038/onc.2015.274
Xie, C., Li, N., Wang, H., He, C., Hu, Y., Peng, C., et al. (2020). Inhibition of Autophagy Aggravates DNA Damage Response and Gastric Tumorigenesis via Rad51 Ubiquitination in Response to Infection. Gut Microbes 11 (6), 1567–1589. doi: 10.1080/19490976.2020.1774311
Yahiro, K., Satoh, M., Nakano, M., Hisatsune, J., Isomoto, H., Sap, J., et al. (2012). Low-Density Lipoprotein Receptor-Related Protein-1 (LRP1) Mediates Autophagy and Apoptosis Caused by Helicobacter Pylori VacA. J. Biol. Chem. 287 (37), 31104–31115. doi: 10.1074/jbc.M112.387498
Yamaoka, Y. (2010). Mechanisms of Disease: Helicobacter Pylori Virulence Factors. Nat. Rev. Gastroenterol. Hepatol. 7 (11), 629–641. doi: 10.1038/nrgastro.2010.154
Yang, J. C., Chien, C. T. (2009). A New Approach for the Prevention and Treatment of Helicobacter Pylori Infection via Upregulation of Autophagy and Downregulation of Apoptosis. Autophagy 5 (3), 413–414. doi: 10.4161/auto.5.3.7826
Yang, L., Huang, F., Mei, J., Wang, X., Zhang, Q., Wang, H., et al. (2017). Posttranscriptional Control of PD-L1 Expression by 17β-Estradiol via PI3K/Akt Signaling Pathway in Erα-Positive Cancer Cell Lines. Int. J. Gynecol. Cancer 27 (2), 196–205. doi: 10.1097/IGC.0000000000000875
Yang, Z., Klionsky, D. J. (2009). An Overview of the Molecular Mechanism of Autophagy. Curr. Topics Microbiol. Immunol. 335, 1–32. doi: 10.1007/978-3-642-00302-8_1
Yang, Z., Klionsky, D. J. (2010). Eaten Alive: A History of Macroautophagy. Nat. Cell Biol. 12 (9), 814–822. doi: 10.1038/ncb0910-814
Yang, L., Li, C., Jia, Y. (2018). MicroRNA-99b Promotes -Induced Autophagyand Suppresses Carcinogenesis by Targeting mTOR. Oncol. Lett. 16 (4), 5355–5360. doi: 10.3892/ol.2018.9269
Yang, J. C., Shun, C. T., Chien, C. T., Wang, T. H. (2008). Effective Prevention and Treatment of Helicobacter Pylori Infection Using a Combination of Catechins and Sialic Acid in AGS Cells and BALB/c Mice. J. Nutr. 138 (11), 2084–2090. doi: 10.3945/jn.108.090985
Yang, J. C., Yang, H. C., Shun, C. T., Wang, T. H., Chien, C. T., Kao, J. Y. (2013). atechins and Sialic Acid Attenuate Helicobacter Pylori-Triggered Epithelial Caspase-1 Activity and Eradicate Helicobacter Pylori Infection. Evidence-Based Complementary and Alternative Medicine. C eCAM, 248585. doi: 10.1155/2013/248585
Yang, Q., Zhang, S., Wu, S., Yao, B., Wang, L., Li, Y., et al. (2022). Identification of Nafamostat Mesylate as a Selective Stimulator of NK Cell IFN-γ Production via Metabolism-Related Compound Library Screening. Immunologic. Res. 1–11. doi: 10.1007/s12026-022-09266-z
Yin, C., Zheng, X., Xiang, H., Li, H., Gao, M., Meng, X., et al. (2019). Differential Expression Profile Analysis of Cisplatin−Regulated miRNAs in a Human Gastric Cancer Cell Line. Mol. Med. Rep. 20 (2), 1966–1976. doi: 10.3892/mmr.2019.10430
Yong, X., Tang, B., Xiao, Y.-F., Xie, R., Qin, Y., Luo, G., et al. (2016). Helicobacter Pylori Upregulates Nanog and Oct4 via Wnt/β-Catenin Signaling Pathway to Promote Cancer Stem Cell-Like Properties in Human Gastric Cancer. Cancer Lett. 374 (2), 292–303. doi: 10.1016/j.canlet.2016.02.032
Yue, J., Mulder, K. M. (2001). Transforming Growth Factor-Beta Signal Transduction in Epithelial Cells. Pharmacol. Ther. 91 (1). doi: 10.1016/s0163-7258(01)00143-7
Zeng, Y., Yao, X., Liu, X., He, X., Li, L., Liu, X., et al. (2019). Anti-Angiogenesis Triggers Exosomes Release From Endothelial Cells to Promote Tumor Vasculogenesis. J. Extracell. Vesicles 8 (1), 1629865. doi: 10.1080/20013078.2019.1629865
Zhang, F., Chen, C., Hu, J., Su, R., Zhang, J., Han, Z., et al. (2019). Molecular Mechanism of Helicobacter Pylori-Induced Autophagy in Gastric Cancer. Oncol. Lett. 18 (6), 6221–6227. doi: 10.3892/ol.2019.10976
Zhang, L., Hu, W., Cho, C. H., Chan, F. K., Yu, J., Fitzgerald, J. R., et al. (2018). Reduced Lysosomal Clearance of Autophagosomes Promotes Survival and Colonization of Helicobacter Pylori. J. Pathol. 244 (4), 432–444. doi: 10.1002/path.5033
Zheng, H. C., Zhao, S., Xue, H., Zhao, E. H., Jiang, H. M., Hao, C. L. (2020). The Roles of Beclin 1 Expression in Gastric Cancer: A Marker for Carcinogenesis, Aggressive Behaviors and Favorable Prognosis, and a Target of Gene Therapy. Front. Oncol. 10, 613679. doi: 10.3389/fonc.2020.613679
Zhou, W. J., Chang, K. K., Wu, K., Yang, H. L., Mei, J., Xie, F., et al. (2018). Rapamycin Synergizes With Cisplatin in Antiendometrial Cancer Activation by Improving IL-27-Stimulated Cytotoxicity of NK Cells. Neoplasia 20 (1), 69–79. doi: 10.1016/j.neo.2017.11.003
Zhu, P. F., Wang, M. X., Chen, Z. L., Yang, L. (2021). Targeting the Tumor Microenvironment: A Literature Review of the Novel Anti-Tumor Mechanism of Statins. Front. Oncol. 11, 761107. doi: 10.3389/fonc.2021.761107
Zhu, X., Wang, Y., Xue, W., Wang, R., Wang, L., Zhu, M.-L., et al. (2019). The VEGFR-2 Protein and the VEGFR-2 Rs1870377 a>T Genetic Polymorphism are Prognostic Factors for Gastric Cancer. Cancer Biol. Ther. 20 (4), 497–504. doi: 10.1080/15384047.2018.1537575
Keywords: autophagy, Helicobacter pylori, gastric cancer, signaling pathways, therapy
Citation: Yang Y, Shu X and Xie C (2022) An Overview of Autophagy in Helicobacter pylori Infection and Related Gastric Cancer. Front. Cell. Infect. Microbiol. 12:847716. doi: 10.3389/fcimb.2022.847716
Received: 03 January 2022; Accepted: 16 March 2022;
Published: 08 April 2022.
Edited by:
Benoit Chassaing, Institut National de la Santé et de la Recherche Médicale (INSERM), FranceReviewed by:
Wei Hu, Southern Medical University, ChinaHuarong Chen, The Chinese University of Hong Kong, China
Yingbo Lin, Karolinska Institutet (KI), Sweden
Copyright © 2022 Yang, Shu and Xie. This is an open-access article distributed under the terms of the Creative Commons Attribution License (CC BY). The use, distribution or reproduction in other forums is permitted, provided the original author(s) and the copyright owner(s) are credited and that the original publication in this journal is cited, in accordance with accepted academic practice. No use, distribution or reproduction is permitted which does not comply with these terms.
*Correspondence: Xu Shu, jxmushx@126.com; Chuan Xie, xcsghhz@ncu.edu.cn