- 1Laboratory of Research in Biofilms Rosário Oliveira (LIBRO), Centre of Biological Engineering (CEB), University of Minho, Braga, Portugal
- 2LABBELS –Associate Laboratory , Braga/Guimarães, Portugal
- 3Faculty of Pharmacy of the University of Coimbra, University of Coimbra, Coimbra, Portugal
- 4The Chemical Process Engineering and Forest Products Research Centre (CIEPQPF), Department of Chemical Engineering, Faculty of Sciences and Technology, University of Coimbra, Coimbra, Portugal
Bacterial vaginosis (BV) is associated with serious gynaecologic and obstetric complications. The hallmark of BV is the presence of a polymicrobial biofilm on the vaginal epithelium, but BV aetiology is still a matter of debate. We have previously developed an in vitro biofilm model that included three BV-associated species, but, up to now, no studies are available whereby more bacterial species are grown together to better mimic the in vivo situation. Herein, we characterized the first polymicrobial BV biofilm consisting of six cultivable BV-associated species by using both in vitro and ex vivo vaginal tissue models. Both models revealed that the six species were able to incorporate the polymicrobial biofilm, at different bacterial concentrations. As it has been thought that this polymicrobial biofilm may increase the survival of BV-associated species when exposed to antibiotics, we also assessed if the Thymbra capitata essential oil (EO), which has recently been shown to be highly bactericidal against several Gardnerella species, could maintain its anti-biofilm activity against this polymicrobial biofilm. Under our experimental conditions, T. capitata EO exhibited a high antibacterial effect against polymicrobial biofilms, in both tested models, with a significant reduction in the biofilm biomass and the number of culturable cells. Overall, this study shows that six BV-associated species can grow together and form a biofilm both in vitro and when using an ex vivo model. Moreover, the data obtained herein should be considered in further applications of T. capitata EO as an antimicrobial agent fighting BV.
Introduction
Bacterial vaginosis (BV) is the worldwide leading vaginal bacterial infection identified in women of childbearing age, with a high prevalence in the general population, ranging from 23% to 29% across regions (Peebles et al., 2019). If left untreated, BV may cause serious obstetric and gynaecologic complications, including preterm delivery (Romero et al., 2004; Shimaoka et al., 2019), spontaneous abortion (Leitich and Kiss, 2007; Işik et al., 2016), low birth weight (Goldenberg and Culhane, 2003; Dingens et al., 2016), pelvic inflammatory disease (Ness et al., 2005; Gondwe et al., 2020), infertility (Salah et al., 2013), and may also lead to an increased risk of acquisition and transmission of several sexually transmitted infectious agents (Gillet et al., 2011; Haddad et al., 2018). Although the understanding of BV etiology is still limited, it is known that BV is characterized by a decrease of beneficial vaginal bacteria and by an increase of strict and facultative anaerobic bacteria (Chen et al., 2021) leading to the development of a polymicrobial biofilm (Swidsinski et al., 2005). This biofilm is thought to protect BV-associated species when exposed to antibiotics, and this leads to recurrent episodes of BV (Bradshaw et al., 2006a; Bradshaw et al., 2012). In addition, the frequent ineffectiveness of standard antibiotics against BV has been also related to high rates of bacterial resistance (Swidsinski et al., 2008; Swidsinski et al., 2011). Consequently, in an attempt to overcome BV treatment failure, natural compounds have been proposed as a promising and effective approach to treat BV (Falconi-McCahill, 2019). Plant-derived products, such as essential oils (EO), represent a therapy on the rise (Stan et al., 2021). EO are complex natural mixtures of volatile compounds produced by aromatic plants, which have been used since ancient times due to their medicinal properties (Bakkali et al., 2008). Furthermore, it has been proposed that the use of EO is associated with a low risk of development of antimicrobial resistance (Becerril et al., 2012; Hammer et al., 2012), which represents a great advantage compared to antibiotics.
Thymbra capitata (L.) Cav. [Coridothymus capitatus (L.) Rchb. F.] is a circum-Mediterranean plant belonging to the Lamiaceae family, widespread in the Mediterranean bay (Verdeguer et al., 2020). This plant is traditionally considered to have strong antiseptic properties, being used for the treatment of cutaneous infections such as acne (Proença-da-Cunha et al., 2014), and in mouthwashes against gum infections (Figueiredo et al., 2008). Several in vitro studies have found that T. capitata EO exhibited high antimicrobial activity against Candida spp. (Palmeira-De-Oliveira et al., 2012), Listeria monocytogenes (Faleiro et al., 2005), and Aspergillus species (Salgueiro et al., 2004). More important, this EO also showed potent antibacterial activity against Gardnerella spp. (Machado et al., 2017). Despite Gardnerella spp. prominence, BV is also associated with other bacterial species and, thus, it is of utmost importance to also evaluate their susceptibility to T. capitata EO. Therefore, in this study, we characterized, for the first time, a polymicrobial biofilm formed by G. vaginalis, Fannyhessea vaginae (Swidsinski et al., 2005), Lactobacillus iners (Fredricks et al., 2005), Mobiluncus curtisii (Fredricks et al., 2007), Peptostreptococcus anaerobius (Hillier et al., 1993), and Prevotella bivia (Onderdonk et al., 2016), both using an in vitro and an ex vivo model. We also assessed the susceptibility of these polymicrobial biofilms to T. capitata EO, further demonstrating the potential of this natural product as an anti-BV agent.
Material and Methods
Plant Material, EO Extraction and Analysis
Aerial parts of T. capitata plant were collected at the flowering stage in Carvoeiro, Algarve, Portugal (37°05’33.9”N 8°27’55.5”W). The oil was isolated by hydrodistillation for 3 h from air-dried material, using a Clevenger-type apparatus according to the European Pharmacopoeia (Council of Europe, 2010). EO composition was established by the combination of gas chromatography with FID detectors (GC-FID) and gas chromatography-mass spectroscopy (GC/MS) analysis, as previously reported (Sousa et al., 2022). The EO components were identified by considering, concurrently: (i) the acquired retention indices on two columns with different phases, SPB-1 (polydimethylsiloxane) and SupelcoWax-10 (polyethyleneglycol), determined by linear interpolation relative to the retention times of C8–C23 of n-alkanes and compared with reference data from authentic products (available in the laboratory database of the Faculty of Pharmacy, University of Coimbra) and literature data (Wallace, 2021); (ii) the acquired mass spectra compared with reference data from the laboratory database, the Wiley/NIST library (McLafferty, 2009) and literature (Adams, 2007). The relative amount of each component was estimated from GC peaks areas without any correction regarding FID responses.
Bacterial Species and Growth Conditions
Gardnerella vaginalis ATCC 14018 and other five cultivable bacterial species associated with BV were used in the current study, namely Fannyhessea vaginae ATCC BAA-55, Lactobacillus iners CCUG 28746, Mobiluncus curtisii ATCC 35241, Peptostreptococcus anaerobius ATCC 27337, and Prevotella bivia ATCC 29303. All species were grown as described before (Rosca et al., 2021), on plates containing Columbia Blood Agar Base medium (CBA) (Oxoid, Basingstoke, UK) supplemented with 5% (v/v) defibrinated horse blood (Oxoid) and incubated, for 48 h, at 37°C in the presence of 10% carbon dioxide (G. vaginalis) or in anaerobic conditions (the other five BV-associated species) generated by using AnaeroGen sachets (Thermo Fisher Scientific, Gloucester, UK) in sealed jars (Oxoid).
Minimum Inhibitory Concentration (MIC) and Minimum Lethal Concentration (MLC) Determination
MIC and MLC values of T. capitata EO were determined by broth macrodilution method, in glass flasks (McCartney type), as previously performed (Machado et al., 2017), with some minor modifications. Furthermore, MIC values of metronidazole were also determined according to the broth microdilution method, in 96-well tissue culture plates (Orange Scientific, Braine-l’Alleud, Belgium), as done before (Jorgensen and Ferraro, 2009), with a few minor changes. Then, after performing serial dilutions for the EO or metronidazole, using New York City III broth supplemented with 10% (v/v) inactivated horse serum (Biowest, Nuaillé, France) (NYC III), bacterial suspensions of the tested species with an optical density (OD) of ~0.1 (Biochrom EZ Read 800 Plus, Cambridge, UK) at 620 nm were added to the prepared EO or metronidazole dilutions. Subsequently, the glass flasks or the 96-well tissue culture plates were incubated for 48 h at 37°C in anaerobic conditions, as described above. NYC III broth and bacterial suspensions without EO or metronidazole were used as negative and positive controls, respectively. Furthermore, an additional negative control was included for the EO experiment, where dimethyl-sulfoxide (DMSO) (Scharlau, Spain) was added to the bacterial suspension, without EO, and no negative effect was observed on bacterial growth. After 48 h of incubation, the MIC value was determined by reading the OD at 620 nm. MLC was determined by plating 10 µL of each dilution on CBA plates and defined as the lowest concentration of EO that prevented the growth of treated bacteria on agar plates. The MIC and MLC assays were repeated at least three independent times, with technical duplicates for each determination.
In Vitro Biofilm Formation and Quantification
A previously described in vitro polymicrobial biofilm formation model (Rosca et al., 2021) was followed in this study, but using six, instead of three BV-associated species. Briefly, the inoculum of each species was grown in NYC III broth and incubated for 24 h at 37°C under anaerobic conditions, as above mentioned. Subsequently, the 24 h cultures were adjusted to a concentration of ~ 1.0 × 107 cells mL-1, and the six species were further co-incubated anaerobically in 24-well tissue culture plates (Orange Scientific) for 24 h at 37°C. We used ~1.0 × 107 cells mL-1 as starting inoculum for each species, since we have previously demonstrated it to be a good starting concentration, to allow bacterial integration in the polymicrobial biofilms (Castro et al., 2021; Rosca et al., 2021). The final volume in each well was 1 mL. Single-species biofilms were grown as controls. After incubation, the biofilms were washed once with 1 mL 1× phosphate-buffered saline (PBS) and then used for quantification of their biomass and cells culturability. To quantify the biomass of single-species and polymicrobial biofilms, we used the crystal violet (CV) method, as described before (Castro et al., 2021). To further determine the culturability of cells from biofilms, we used the colony-forming units (CFU) method. Therefore, after being washed with 1 mL of 1× PBS, the biofilm in each well of the 24-well plates was scrapped off and resuspended in 1 mL of NYC III broth. Ten-fold serial dilutions were performed in 0.9% (w/v) NaCl (Liofilchem, Roseto degli Abruzzi, Italy) and plated on CBA plates to allow CFU counting. The CBA plates were further incubated anaerobically at 37°C for up to 72 h. At least three independent assays, with technical duplicates, were performed.
Formation and Characterization of Polymicrobial Biofilms on the Reconstructed Human Vaginal Epithelium
To mimic the vaginal epithelial environment, a reconstructed human vaginal epithelium (SkinEthic™, Episkin, Lyon, France) was used. A 24 h inoculum of each tested species was resuspended in a medium simulating genital tract secretions (mGTS) (Stingley et al., 2014), at a concentration of ~ 1.0 × 107 cells mL-1. A mixture containing a 1:1 ratio of each species was dispensed on the SkinEthic™ tissues for colonization. The SkinEthic™ tissues were then incubated anaerobically for 9 h. The SkinEthic™ tissues were then processed as follows. For qPCR quantification, the tissues were washed once with 0.9% (w/v) NaCl and then processed as described below. To perform the culturability assays, the SkinEthic™ tissues were washed once with 0.9% (w/v) NaCl and 500 µL of mGTS was added before performing serial dilutions and plating them on CBA plates. A cycle of sonication for 10 sec with an amplitude of 33% was performed to displace the cells from the SkinEthic™ tissues. For microscopic analysis, the SkinEthic™ tissues were placed in 4% paraformaldehyde (Thermo Fisher Scientific) and then embedded in paraffin (Leica TP1020, Nussloch, Germany). Paraffin tissue blocks were prepared (Leica EG 1140 H, Nussloch, Germany) and 3-μm-thick sections were obtained using a microtome (Microm HM 325, Walldorf, Germany). For the deparaffinization step, sections were placed in xylene (Thermo Fisher Scientific) twice, followed by a hydration step with 100% and 50% of ethanol (Thermo Fisher Scientific) and a final step in distilled water. All steps were performed for 5 min. Samples were then stained with Periodic Acid-Schiff (PAS), as described before (Chawla et al., 2017). The samples were analysed using an Olympus BX51 microscope (Olympus, Lisbon, Portugal). The experiment was performed in duplicate and repeated three independent times.
Characterization of In Vitro and Ex Vivo Polymicrobial Biofilms by Quantitative PCR (qPCR) Quantification
Genomic DNA (gDNA) from the in vitro and ex vivo polymicrobial biofilms as well as from pure cultures of the six species (for the qPCR calibration curves), were performed as described before (Cerca et al., 2022). To assess the efficiency and variability of gDNA extraction between samples, 10 µL of luciferase complementary DNA (cDNA), obtained as described before (Magalhães et al., 2019), was added to each sample before proceeding with gDNA isolation. Specific primers were designed with CLC genomics workbench version 21 (QIAGEN), and primer specificity was first confirmed using Primer-BLAST and then experimentally determined by qPCR. Primers are described in Table 1. The qPCR runs were performed in a CFX96™ thermal cycler (Bio-Rad, CA, USA) with the following cycle parameters: 95°C for 3 min, followed by 40 cycles of 95°C for 5 s, and 60°C for 20 s. Non-template controls were used to define the negative threshold to be considered for each primer set. Melt analysis was performed to ensure the absence of unspecific products and primer-dimers. PCR amplification efficiency was determined from the slope of a standard curve. Bacterial load in each sample was interpolated from the averaged standard curves. All assays were repeated at least three independent times with three technical replicates.
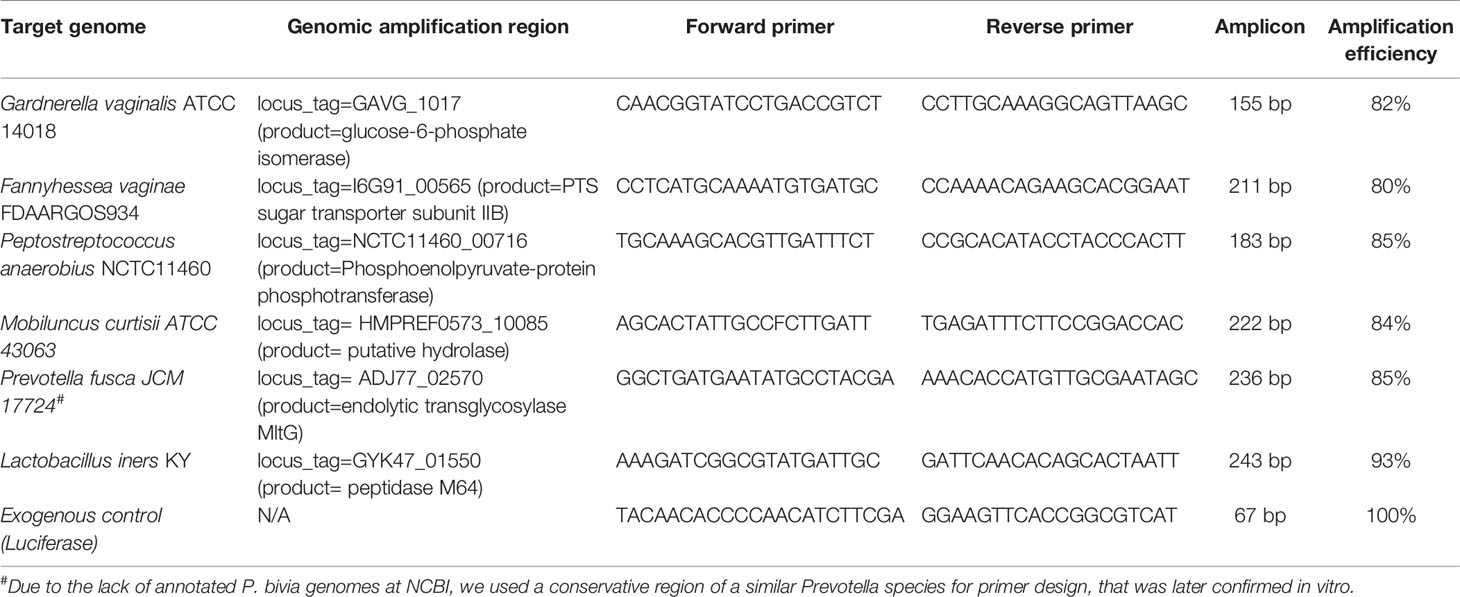
Table 1 Specific primers for the quantification of the six bacterial species present in the polymicrobial biofilm.
The Effect of EO on In Vitro and Ex Vivo Biofilms Biomass and Biofilm Cells Culturability
For the in vitro model, the 24 h biofilms were exposed to 1 mL of T. capitata EO at a concentration of 0.63 µL mL-1, diluted in NYC III broth containing DMSO, and further incubated anaerobically at 37°C for an additional 24 h. DMSO had the role to improve the solubility of EO in the culture medium. The biomass and cell culturability of the in vitro biofilms treated with EO were quantified as described above. For each experiment, initial and final controls, respectively, 24 h and 48 h biofilms were included. The 48 h controls consisted of biofilms to which 1 mL of fresh culture medium without EO was added. For the ex vivo SkinEthic™ model, EO challenge at a concentration of 0.32 µL mL-1 in mGTS was performed after 9 h of biofilm incubation and allowed to act for 14 h. Total incubation and EO exposure time was limited to a maximum of 24 h in order to follow the manufacturer’s instructions for guaranteeing SkinEthic™ tissue viability. Controls included SkinEthic™ tissues not exposed to the EO. Biofilms biomass was qualitatively determined by optical microscopy, and cell culturability was determined by CFU plating, as mentioned above. The CFU detection limit was log 3 CFU mL-1.
Statistical Analysis
All data were analysed with GraphPad Prism version 7 (La Jolla, CA) using two-way ANOVA with Dunnett’s or Tukey’s multiple comparisons tests. Values with a p < 0.05 were considered statistically significant.
Results
Ability of the Six BV-Associated Species to Form an In Vitro and Ex Vivo Polymicrobial Biofilm
Although BV is associated with the presence of a polymicrobial biofilm on the vaginal epithelium (Swidsinski et al., 2005), up to date, no functional in vitro studies have assessed BV-associated biofilms composed of more than three different species (Rosca et al., 2021). Since in vivo evidence supports the role of more species to be present during BV, in this study, we aimed to assess if six relevant BV-associated species could, in fact, establish a polymicrobial biofilm. As shown in Figure 1A, total biofilm biomass of the polymicrobial biofilms was significantly higher than the biomass of any tested single-species biofilms (p < 0.05). Taking into consideration that each species can contribute differently to biomass accumulation, we also determined the total number of culturable cells, which was also higher in the polymicrobial biofilms, as shown in Figure 1B. This suggests that synergistic interactions were present during the formation of this biofilm. In order to see if this phenomenon also occurs in conditions more similar to the in vivo situation, we further used an ex vivo model. As can be observed in Figure 2A, the BV-associated species used in this study were also able to form a prominent biofilm structure on the SkinEthic™ tissues.
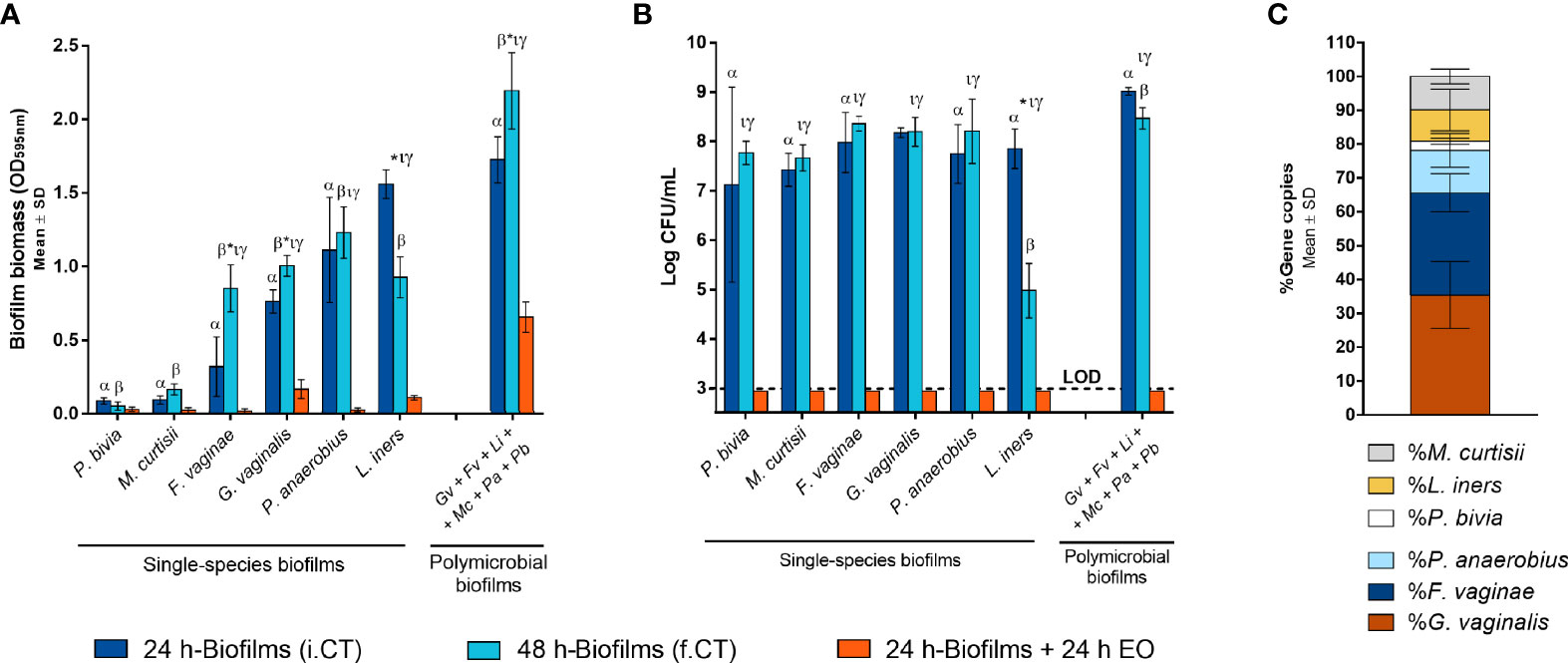
Figure 1 Single-species and polymicrobial BV biofilms. (A) Biomass quantification of the single-species and polymicrobial biofilms prior to and after EO exposure using crystal violet method. (B) Culturable cells quantification from the single-species and polymicrobial biofilms prior to and after EO treatment, by CFU method. (C) Determination of the percentage distribution of each bacterial species in 24 h polymicrobial BV biofilm, by qPCR. i.CT stands for initial control, before the medium replacement; f.CT stands for final control, after incubation with fresh medium. Statistically significant differences between polymicrobial vs single-species biofilms are represented with α for 24 h incubation time and with β for 48 h incubation time (two-way ANOVA and Dunnett’s multiple comparisons test, p < 0.05). Values are significantly different for * i.CT vs f.CT, τ i.CT vs EO, and γ f.CT vs EO (two-way ANOVA and Tukey’s multiple comparisons test, p < 0.05). Gardnerella vaginalis (Gv); Fannyhessea vaginae (Fv); Lactobacillus iners (Li); Mobiluncus curtisii (Mc); Peptostreptococcus anaerobius (Pa); Prevotella bivia (Pb); Essential oil (EO); Limit of detection (LOD).
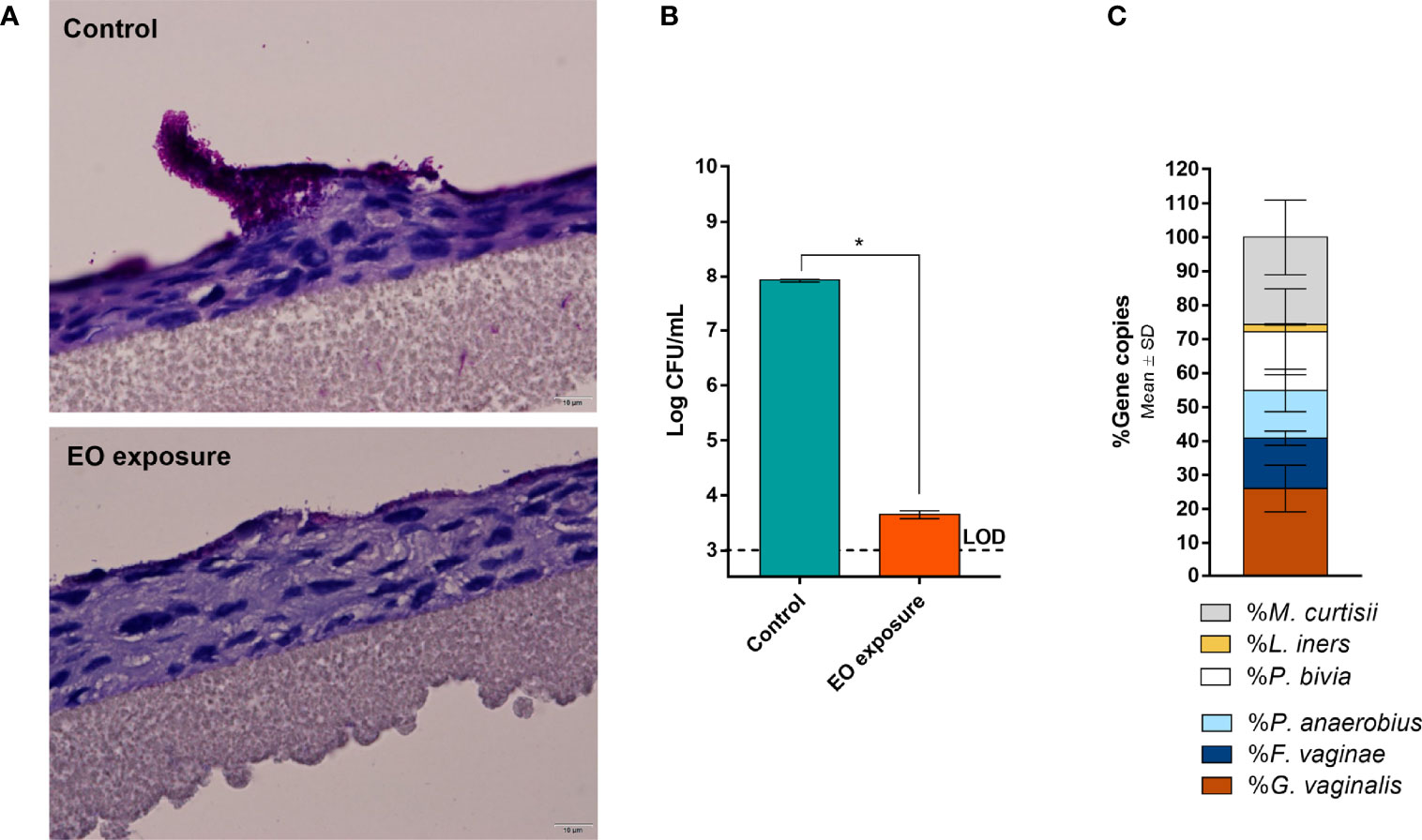
Figure 2 Polymicrobial BV biofilm developed on a reconstructed human vaginal epithelium. (A) Periodic Acid-Schiff staining images with the BV biofilm before and after EO treatment at 600× magnification. (B) Cells culturability from the control and EO treated biofilms as determined by CFU method. (C) Bacterial distribution in the polymicrobial BV biofilms as determined by qPCR after extraction of genomic DNA of each bacterial species. *Values are significantly different for control vs EO-exposed biofilms (paired t-test, p < 0.05). Essential oil (EO); Limit of detection (LOD). More examples are shown in Supplementary Material.
Importantly, to support our claims that all six species were present after the biofilm incubation period, we determined the biofilm composition after removal of the bulk liquid, by performing gDNA extraction followed by qPCR quantification. In both models, all six species were found inside the biofilm structure, although with slightly different cell percentages between the models (Figures 1C and 2C). It is worth mentioning that the ex vivo model experimental conditions were distinct from the in vitro, and these two models were used with the goal to further support our conclusions. Due to the different experimental conditions, these two models are not meant to be directly compared.
Susceptibility of BV-Associated Species Planktonic Cells to EO and Metronidazole
The EO used herein was obtained with a yield of 2.7% (v/w). It is characterized by a high percentage of oxygen-containing monoterpenes, being mainly composed of carvacrol (73.9%) followed by its biogenetic precursors, γ-terpinene (7.4%), and p-cymene (4.9%). The full composition of this EO has been described before (Sousa et al., 2022). In vitro antibacterial activity of this EO against each species tested in the current study was evaluated by determining both MIC and MLC values. For comparative purposes, the MIC of metronidazole, the first-line treatment for BV (Workowski and Bolan, 2015), was also determined. As shown in Table 2, four out of six species were not susceptible to metronidazole, including G. vaginalis and F. vaginae, that have been shown to constitute the majority of the biofilm biomass (Figure 1C). Furthermore, the EO exhibited a variable antimicrobial effect against BV-associated bacteria, with a concentration of 0.63 µL mL-1 inhibiting the growth of P. anaerobius, while a concentration of 0.16 – 0.31 µL mL-1 being already sufficient to inhibit the growth of F. vaginae and M. curtisii.

Table 2 Determination of the minimum inhibitory (MIC) and minimum lethal (MLC) concentrations of Thymbra capitata essential oil against BV-associated species and comparison with standard antibiotics.
Impact of EO on Biofilms Biomass and Biofilm Cells Culturability
Despite BV has been associated with a polymicrobial biofilm, we first tested the EO antimicrobial activity against single-species biofilms, for comparative purposes. As shown before (Rosca et al., 2021), the ability to form single-species biofilms varied significantly between species, with P. bivia and M. curtisii forming negligible biofilms after 24 h of incubation. Interestingly, when using the highest MLC concentration determined for the selected species (0.63 µL mL-1), most biofilms biomass was eradicated, except for G. vaginalis and L. iners (Figure 1A). On the polymicrobial biofilm, higher biomass remained after the EO challenge, but when analysing the total culturable cells of the remaining biofilms, no bacteria were quantified in any of the single-species or polymicrobial biofilms (Figure 1B). To better assess the EO antimicrobial potential against a BV polymicrobial biofilm, we also used an ex vivo model, wherein a polymicrobial biofilm was established on a reconstructed human vaginal epithelium. When applying a lower (0.32 µL mL-1), but non-cytotoxic concentration of the EO (Sousa et al., 2022), a significant reduction of the biofilm biomass was also observed (Figure 2A), similar to what occurred in the in vitro experiment (Figure 1A). However, contrary to the in vitro experiment, EO exposure did not completely eradicate culturable cells, with only a 4-log reduction observed (Figure 2B). However, both the EO exposure periods (24 vs 14 h) and the EO concentrations (0.63 vs 0.32 µL mL-1) were different, a consequence of the limitation of the ex vivo model, in order to maintain the reconstructed human vaginal epithelium viable.
Discussion
It is already known that BV is associated with the presence of a polymicrobial biofilm on the vaginal epithelium (Swidsinski et al., 2005). Despite this knowledge, little is known about how this biofilm is formed and what kind of interactions might occur between bacterial species forming it. While direct evidence of Gardnerella spp. and F. vaginae co-existing in the same biofilm have already been described in vivo (Swidsinski et al., 2005), the presence of the other species has mostly been derived from microbiome studies (Fredricks et al., 2005; Fredricks et al., 2007). As such, there is interest in trying to determine if these species can, in fact, interact and grow together in a biofilm. Aiming to better determine the role/impact of bacterial biofilms in BV, biofilm studies have been reported, wherein single- (Janulaitiene et al., 2018; Khan et al., 2019; Li et al., 2020), dual- (Castro et al., 2019; Castro et al., 2020) or triple-species (Rosca et al., 2021) in vitro BV-associated biofilms have been characterized. By providing direct evidence that the same biofilm contains the six tested BV-associated species, we are the first to show that close cooperation between these species can occur, at least in vitro and in an ex vivo vaginal model, as discussed next.
The six species in this study were not randomly selected. They have been commonly used in BV-related microbiome studies (Rosca et al., 2020) and have been previously reported as potential pathogens involved in BV development (Ravel et al., 2011; Onderdonk et al., 2016; Diop et al., 2019). Of note, while all six species were inoculated at a 1:1 ratio, we could not claim that after the incubation period, all six species incorporated the biofilm. Indeed, cumulating evidence from experimental and metabolic model-based studies demonstrated that often microorganisms compete for limited resources, such as space and nutrients (Freilich et al., 2011; Foster and Bell, 2012), at times leading to exclusion. As no specific fluorescence in situ hybridization (FISH) probes exist for all six species, we have characterized the ecological niche of this polymicrobial biofilm using qPCR (Bordagaray et al., 2021). As demonstrated by our results, all six species were able to incorporate the biofilm, either using the in vitro or ex vivo models. Still, some interesting differences were observed between these models, with P. bivia and M. curtisii having, respectively, a 6.4- and 2.6-fold higher prevalence in the ex vivo model, while L. iners and F. vaginae had a 4.3- and 2.0-fold higher prevalence in the in vitro model, respectively. G. vaginalis and P. anaerobius were the two less affected species. These differences are not surprising, since both the adhesion surface, the growth media, and the incubation time (24 h vs 9 h) used were very different, and these are known to affect biofilm formation ability (Tolker-Nielsen, 2015). The choice of mGTS for the ex vivo model aimed to better mimic in vivo conditions. While the ex vivo model still lacks some relevant components, such as the presence of host immune components (Castro et al., 2018; Delgado-Diaz et al., 2020) and human hormones (Moncla et al., 2016), the observed biofilm biomass was similar to what has been described in vivo (Hardy et al., 2016). As functional studies are not possible to perform in vivo, these in vitro and ex vivo models are fundamental to better understand the aetiology of BV, despite the limitations.
Once formed, the presence of this polymicrobial BV biofilm leads to negative consequences for women's health as it is considered one of the major factors responsible for the treatment failure. This may be due to the fact that this biofilm could provide protection and allow BV-associated bacteria to display increased tolerance to antibiotics, and this may be further contributing to high rates of BV recurrence (Vodstrcil et al., 2021). To overcome this problem, alternative approaches to existing antibiotics are being currently pursued (Sherrard et al., 2018; Marrazzo et al., 2019). Herein, we further explore the potential of T. capitata EO against BV. We have previously shown T. capitata EO to be highly effective against Gardnerella spp. single-species biofilms and non-toxic for several lactobacilli (Machado et al., 2017) while also not being toxic for the reconstructed human vaginal epithelium (at the used concentrations) (Sousa et al., 2022). In the present study, the EO showed high antimicrobial activity at MLC concentration against the single-species biofilms. This was specially relevant because most of the tested species were resistant to metronidazole. These results are very encouraging as several previous studies have also shown that BV-associated species are often resistant to antibiotics (Ferris et al., 2004; Bahar et al., 2005; Bradshaw et al., 2006b; Alves et al., 2014; Castro et al., 2015). Furthermore, our data also showed that EO at MLC concentration had a significant reduction effect on the total biomass of polymicrobial biofilms and this should be considered in further applications of this EO as an antimicrobial agent.
Taken together, this brief report brings novelty to the BV research field as (i) this is the first time six BV-associated species have been reported to establish an in vitro biofilm; (ii) this is the first time such biofilm is shown to be formed on a reconstructed human vaginal epithelium using an ex vivo model; and (iii) this is the first study to demonstrate the antimicrobial activity of T. capitata EO against some prominent bacterial species associated with BV, in either pure cultures or in a six-species biofilm consortium. Nonetheless, this study has some limitations and further work should be performed in order to address (i) the possible presence of viable but non-culturable cells and (ii) the composition of the biofilm after EO exposure, by developing a method that allows discrimination between live and dead cells, such as viability PCR (Latka et al., 2022) or FISH (Castro et al., 2022). As we are already heading toward a post-antibiotic era in which many bacterial infections will be impossible to treat (Hauser et al., 2016), it is of utmost importance to focus attention on new antimicrobial treatment options, and T. capitata EO could be an ideal candidate in this regard.
Data Availability Statement
The original contributions presented in the study are included in the article/Supplementary Material. Further inquiries can be directed to the corresponding author.
Author Contributions
LS collected the plants; LS and CC extracted and characterized the EO; ASR performed the planktonic and biofilm experiments. JC and LGVS performed the experiments with the reconstructed human vaginal epithelium. AF and NC performed the qPCR experiments. NC designed the study. ASR and NC drafted the manuscript. All authors critically reviewed and approved the final manuscript.
Funding
This work was supported by the Portuguese Foundation for Science and Technology (FCT) by the research project [PTDC/BIA-MIC/28271/2017] under the scope of COMPETE 2020 [POCI-01-0145-FEDER-028271] and by the strategic funding of unit [UID/BIO/04469/2020]. ASR acknowledges the support of FCT individual fellowship [PD/BD/128037/2016]. The founder had no role in the study design and collection, analysis, and interpretation of the results.
Conflict of Interest
This work has been submitted as part of a patent request.
Publisher’s Note
All claims expressed in this article are solely those of the authors and do not necessarily represent those of their affiliated organizations, or those of the publisher, the editors and the reviewers. Any product that may be evaluated in this article, or claim that may be made by its manufacturer, is not guaranteed or endorsed by the publisher.
Acknowledgments
We thank Paula Gonçalves (ICVS-UM) for her help with the histological cuts.
Supplementary Material
The Supplementary Material for this article can be found online at: https://www.frontiersin.org/articles/10.3389/fcimb.2022.824860/full#supplementary-material
References
Adams, R. P. (2007). Identification of Essential Oil Components by Gas Chromatography/ Mass Spectrometry. 4th Edition (Carol Stream, IL: Allured Publ.).
Alves, P., Castro, J., Sousa, C., Cereija, T. B., Cerca, N. (2014). Gardnerella vaginalis Outcompetes 29 Other Bacterial Species Isolated From Patients With Bacterial Vaginosis, Using in an In Vitro Biofilm Formation Model. J. Infect. Dis. 210, 593–596. doi: 10.1093/infdis/jiu131
Bahar, H., Torun, M. M., Öçer, F., Kocazeybek, B. (2005). Mobiluncus Species in Gynaecological and Obstetric Infections: Antimicrobial Resistance and Prevalence in a Turkish Population. Int. J. Antimicrob. Agents 25, 268–271. doi: 10.1016/j.ijantimicag.2004.09.019
Bakkali, F., Averbeck, S., Averbeck, D., Idaomar, M. (2008). Biological Effects of Essential Oils - A Review. Food Chem. Toxicol. 46, 446–475. doi: 10.1016/j.fct.2007.09.106
Becerril, R., Nerín, C., Gómez-Lus, R. (2012). Evaluation of Bacterial Resistance to Essential Oils and Antibiotics After Exposure to Oregano and Cinnamon Essential Oils. Foodborne Pathog. Dis. 9, 699–705. doi: 10.1089/fpd.2011.1097
Bordagaray, M. J., Fernández, A., Garrido, M., Astorga, J., Hoare, A., Hernández, M. (2021). Systemic and Extraradicular Bacterial Translocation in Apical Periodontitis. Front. Cell. Infect. Microbiol. 11. doi: 10.3389/fcimb.2021.649925
Bradshaw, C. S., Morton, A. N., Hocking, J., Garland, S. M., Morris, M. B., Moss, L. M., et al. (2006a). High Recurrence Rates of Bacterial Vaginosis Over the Course of 12 Months After Oral Metronidazole Therapy and Factors Associated With Recurrence. J. Infect. Dis. 193, 1478–1486. doi: 10.1086/503780
Bradshaw, C. S., Pirotta, M., de Guingand, D., Hocking, J. S., Morton, A. N., Garland, S. M., et al. (2012). Efficacy of Oral Metronidazole With Vaginal Clindamycin or Vaginal Probiotic for Bacterial Vaginosis: Randomised Placebo-Controlled Double-Blind Trial. PloS One 7, 1–10. doi: 10.1371/journal.pone.0034540
Bradshaw, C. S., Tabrizi, S. N., Fairley, C. K., Morton, A. N., Rudland, E., Garland, S. M. (2006b). The Association of Atopobium Vaginae and Gardnerella vaginalis With Bacterial Vaginosis and Recurrence After Oral Metronidazole Therapy. J. Infect. Dis. 194, 828–836. doi: 10.1086/506621
Castro, J., Alves, P., Sousa, C., Cereija, T., França, Â., Jefferson, K. K., et al. (2015). Using an in-Vitro Biofilm Model to Assess the Virulence Potential of Bacterial Vaginosis or non-Bacterial Vaginosis Gardnerella vaginalis Isolates. Sci. Rep. 5, 11640. doi: 10.1038/srep11640
Castro, J., Jefferson, K., Cerca, N. (2018). Innate Immune Components Affect Growth and Virulence Traits of Bacterial Vaginosis-Associated and non-BV Associated Gardnerella vaginalis Strains Similarly. Pathog. Dis. 76, fty089. doi: 10.1093/femspd/fty089
Castro, J., Machado, D., Cerca, N. (2019). Unveiling the Role of Gardnerella vaginalis in Polymicrobial Bacterial Vaginosis Biofilms: The Impact of Other Vaginal Pathogens Living as Neighbors. ISME J. 13, 1306–1317. doi: 10.1038/s41396-018-0337-0
Castro, J., Rosca, A. S., Cools, P., Vaneechoutte, M., Cerca, N. (2020). Gardnerella vaginalis Enhances Atopobium Vaginae Viability in an In Vitro Model. Front. Cell. Infect. Microbiol. 10. doi: 10.3389/fcimb.2020.00083
Castro, J., Rosca, A. S., Muzny, C. A., Cerca, N. (2021). Atopobium Vaginae and Prevotella Bivia are Able to Incorporate and Influence Gene Expression in a Pre-Formed Gardnerella vaginalis Biofilm. Pathogens 10, 247. doi: 10.3390/pathogens10020247
Castro, J., Sousa, L. G. V., França, A., Tisakova, L., Corsini, L., Cerca, N. (2022). Exploiting the Anti-Biofilm Effect of the Engineered Phage Endolysin PM-477 to Disrupt In Vitro Single- and Dual-Species Biofilms of Vaginal Pathogens Associated With Bacterial Baginosis. Antibiotics. 11, 558. doi: 10.3390/antibiotics11050558
Cerca, N., Lima, Â., França, A. (2022). Accurate qPCR Quantification in Polymicrobial Communities Requires Assessment of gDNA Extraction Efficiency. J. Microbiol. Methods 194, 106421. doi: 10.1016/j.mimet.2022.106421
Chawla, M., Verma, V., Kapoor, M., Kapoor, S. (2017). A Novel Application of Periodic Acid–Schiff (PAS) Staining and Fluorescence Imaging for Analysing Tapetum and Microspore Development. Histochem. Cell Biol. 147, 103–110. doi: 10.1007/s00418-016-1481-0
Chen, X., Lu, Y., Chen, T., Li, R. (2021). The Female Vaginal Microbiome in Health and Bacterial Vaginosis. Front. Cell. Infect. Microbiol. 11. doi: 10.3389/FCIMB.2021.631972
Council of Europe. (2020). European Pharmacopoeia. (European Directorate for the Quality of Medicines and Healthcare of the Council of Europe, 2010).
Delgado-Diaz, D. J., Tyssen, D., Hayward, J. A., Gugasyan, R., Hearps, A. C., Tachedjian, G. (2020). Distinct Immune Responses Elicited From Cervicovaginal Epithelial Cells by Lactic Acid and Short Chain Fatty Acids Associated With Optimal and Non-Optimal Vaginal Microbiota. Front. Cell. Infect. Microbiol. 9. doi: 10.3389/fcimb.2019.00446
Dingens, A. S., Fairfortune, T. S., Reed, S., Mitchell, C. (2016). Bacterial Vaginosis and Adverse Outcomes Among Full-Term Infants: A Cohort Study. BMC Pregnancy Childbirth 16, 278. doi: 10.1186/s12884-016-1073-y
Diop, K., Dufour, J.-C., Levasseur, A., Fenollar, F. (2019). Exhaustive Repertoire of Human Vaginal Microbiota. Hum. Microbiome J. 11, 100051. doi: 10.1016/j.humic.2018.11.002
Falconi-McCahill, A. (2019). Bacterial Vaginosis: A Clinical Update With a Focus on Complementary and Alternative Therapies. J. Midwifery Womens Health 64, 578–591. doi: 10.1111/jmwh.13013
Faleiro, L., Miguel, G., Gomes, S., Costa, L., Venâncio, F., Teixeira, A., et al. (2005). Antibacterial and Antioxidant Activities of Essential Oils Isolated From Thymbra Capitata L. (Cav.) and Origanum Vulgare L. J. Agric. Food Chem. 53, 8162–8168. doi: 10.1021/jf0510079
Ferris, M. J., Masztal, A., Aldridge, K. E., Fortenberry, J. D., Fidel, P. L., Martin, D. H. (2004). Association of Atopobium Vaginae, a Recently Described Metronidazole Resistant Anaerobe, With Bacterial Vaginosis. BMC Infect. Dis. 4, 5. doi: 10.1186/1471-2334-4-5
Figueiredo, A., Barroso, J., Pedro, L., Salgueiro, L., Miguel, M., Faleiro, M. (2008). Portuguese Thymbra and Thymus Species Volatiles: Chemical Composition and Biological Activities. Curr. Pharm. Des. 14, 3120–3140. doi: 10.2174/138161208786404218
Foster, K. R., Bell, T. (2012). Competition, Not Cooperation, Dominates Interactions Among Culturable Microbial Species. Curr. Biol. 22, 1845–1850. doi: 10.1016/j.cub.2012.08.005
Fredricks, D. N., Fiedler, T. L., Marrazzo, J. M. (2005). Molecular Identification of Bacteria Associated With Bacterial Vaginosis. N. Engl. J. Med. 353, 1899–1911. doi: 10.1056/NEJMoa043802
Fredricks, D. N., Fiedler, T. L., Thomas, K. K., Oakley, B. B., Marrazzo, J. M. (2007). Targeted PCR for Detection of Vaginal Bacteria Associated With Bacterial Vaginosis. J. Clin. Microbiol. 45, 3270–3276. doi: 10.1128/JCM.01272-07
Freilich, S., Zarecki, R., Eilam, O., Segal, E. S., Henry, C. S., Kupiec, M., et al. (2011). Competitive and Cooperative Metabolic Interactions in Bacterial Communities. Nat. Commun. 2, 589–595. doi: 10.1038/ncomms1597
Gillet, E., Meys, J. F., Verstraelen, H., Bosire, C., De Sutter, P., Temmerman, M., et al. (2011). Bacterial Vaginosis is Associated With Uterine Cervical Human Papillomavirus Infection: A Meta-Analysis. BMC Infect. Dis. 11, 10. doi: 10.1186/1471-2334-11-10
Goldenberg, R. L., Culhane, J. F. (2003). Infection as a Cause of Preterm Birth. Clin. Perinatol. 30, 677–700. doi: 10.1016/S0095-5108(03)00110-6
Gondwe, T., Ness, R., Totten, P. A., Astete, S., Tang, G., Gold, M. A., et al. (2020). Novel Bacterial Vaginosis-Associated Organisms Mediate the Relationship Between Vaginal Douching and Pelvic Inflammatory Disease. Sex Transm. Infect. 96, 439–444. doi: 10.1136/sextrans-2019-054191
Haddad, L. B., Wall, K. M., Kilembe, W., Vwalika, B., Khu, N. H., Brill, I., et al. (2018). Bacterial Vaginosis Modifies the Association Between Hormonal Contraception and HIV Acquisition. AIDS 32, 595–604. doi: 10.1097/QAD.0000000000001741
Hammer, K. A., Carson, C. F., Rileya, T. V. (2012). Effects of Melaleuca Alternifolia (Tea Tree) Essential Oil and the Major Monoterpene Component Terpinen-4-Ol on the Development of Single- and Multistep Antibiotic Resistance and Antimicrobial Susceptibility. Antimicrob. Agents Chemother. 56, 909–915. doi: 10.1128/AAC.05741-11
Hardy, L., Jespers, V., Abdellati, S., De Baetselier, I., Mwambarangwe, L., Musengamana, V., et al. (2016). A Fruitful Alliance: The Synergy Between Atopobium Vaginae and Gardnerella vaginalis in Bacterial Vaginosis-Associated Biofilm. Sex Transm. Infect. 92, 487–491. doi: 10.1136/sextrans-2015-052475
Hauser, A. R., Mecsas, J., Moir, D. T. (2016). Beyond Antibiotics: New Therapeutic Approaches for Bacterial Infections. Clin. Infect. Dis. 63, 89–95. doi: 10.1093/cid/ciw200
Hillier, S. L., Krohn, M. A., Rabe, L. K., Klebanoff, S. J., Eschenbach, D. A. (1993). The Normal Vaginal Flora, H2O2-Producing Lactobacilli, and Bacterial Vaginosis in Pregnant Women. Clin. Infect. Dis. 16, S273–S281. doi: 10.1093/clinids/16.supplement_4.s273
Işik, G., Demirezen, Ş., Dönmez, H. G., Beksaç, M. S. (2016). Bacterial Vaginosis in Association With Spontaneous Abortion and Recurrent Pregnancy Losses. J. Cytol 33, 135–140. doi: 10.4103/0970-9371.188050
Janulaitiene, M., Gegzna, V., Baranauskiene, L., Bulavaite, A., Simanavicius, M., Pleckaityte, M. (2018). Phenotypic Characterization of Gardnerella vaginalis Subgroups Suggests Differences in Their Virulence Potential. PloS One 13, e0200625. doi: 10.1186/s12879-017-2501-y
Jorgensen, J. H., Ferraro, M. J. (2009). Antimicrobial Susceptibility Testing: A Review of General Principles and Contemporary Practices. Clin. Infect. Dis. 49, 1749–1755. doi: 10.1086/647952
Khan, S., Voordouw, M. J., Hill, J. E. (2019). Competition Among Gardnerella Subgroups From the Human Vaginal Microbiome. Front. Cell. Infect. Microbiol. 9. doi: 10.3389/fcimb.2019.00374
Latka, A., Simaey, L. V., Reynders, M., Cools, P., Rogier, T., Lebbe, B., et al. (2022). Optimization of Propidium Monoazide qPCR (viability-qPCR) to Quantify the Killing by the Gardnerella-Specific Endolysin PM-477, Directly in Vaginal Samples From Women With Bacterial Vaginosis. Antibiotics 11, 111. doi: 10.3390/antibiotics11010111
Leitich, H., Kiss, H. (2007). Asymptomatic Bacterial Vaginosis and Intermediate Flora as Risk Factors for Adverse Pregnancy Outcome. Best Pract. Res. Clin. Obstet. Gynaecol. 21, 375–390. doi: 10.1016/j.bpobgyn.2006.12.005
Li, T., Zhang, Z., Wang, F., He, Y., Zong, X., Bai, H., et al. (2020). Antimicrobial Susceptibility Testing of Metronidazole and Clindamycin Against Gardnerella vaginalis in Planktonic and Biofilm Formation. Can. J. Infect. Dis. Med. Microbiol. 2020, 1–7. doi: 10.1155/2020/1361825
Machado, D., Gaspar, C., Palmeira-de-Oliveira, A., Cavaleiro, C., Salgueiro, L., Martinez-de-Oliveira, J., et al. (2017). Thymbra Capitata Essential Oil as Potential Therapeutic Agent Against Gardnerella vaginalis Biofilm-Related Infections. Future Microbiol. 12, 407–416. doi: 10.2217/fmb-2016-0184
Magalhães, A. P., França, Â., Pereira, M. O., Cerca, N. (2019). RNA-Based qPCR as a Tool to Quantify and to Characterize Dual-Species Biofilms. Sci. Rep. 9, 13639–13650. doi: 10.1038/s41598-019-50094-3
Marrazzo, J. M., Dombrowski, J. C., Wierzbicki, M. R., Perlowski, C., Pontius, A., Dithmer, D., et al. (2019). Safety and Efficacy of a Novel Vaginal Anti-Infective, TOL-463, in the Treatment of Bacterial Vaginosis and Vulvovaginal Candidiasis: A Randomized, Single-Blind, Phase 2, Controlled Trial. Clin. Infect. Dis. 68, 803–809. doi: 10.1093/cid/ciy554
McLafferty, F. W. (2009). Wiley Registry of Mass Spectral Data 9th/NIST 08’ (Mass spectral library) 9th ed. Wiley.
Moncla, B. J., Chappell, C. A., Debo, B. M., Meyn, L. A. (2016). The Effects of Hormones and Vaginal Microflora on the Glycome of the Female Genital Tract: Cervical-Vaginal Fluid. PloS One 11, e0158687. doi: 10.1371/journal.pone.0158687
Ness, R. B., Kip, K. E., Hillier, S. L., Soper, D. E., Stamm, C. A., Sweet, R. L., et al. (2005). A Cluster Analysis of Bacterial Vaginosis-Associated Microflora and Pelvic Inflammatory Disease. Am. J. Epidemiol. 162, 585–590. doi: 10.1093/aje/kwi243
Onderdonk, A. B., Delaney, M. L., Fichorova, N. (2016). The Human Microbiome During Bacterial Vaginosis. Clin. Microbiol. Rev. 29, 223–238. doi: 10.1128/CMR.00075-15
Palmeira-De-Oliveira, A., Gaspar, C., Palmeira-De-Oliveira, R., Silva-Dias, A., Salgueiro, L., Cavaleiro, C., et al. (2012). The Anti-Candida Activity of Thymbra Capitata Essential Oil: Effect Upon Pre-Formed Biofilm. J. Ethnopharmacol. 140, 379–383. doi: 10.1016/j.jep.2012.01.029
Peebles, K., Velloza, J., Balkus, J. E., McClelland, R. S., Barnabas, R. V. (2019). High Global Burden and Costs of Bacterial Vaginosis: A Systematic Review and Meta-Analysis. Sex Transm. Dis. 46, 304–311. doi: 10.1097/OLQ.0000000000000972
Proença-da-Cunha, A., Ribeiro, J. A., Roque, O. R. (2014). Plantas Aromáticas Em Portugal – Caracterização E Utilizações (Lisboa, Portugal: Fundação Calouste Gulbenkian).
Ravel, J., Gajer, P., Abdo, Z., Schneider, G. M., Koenig, S. S. K., McCulle, S. L., et al. (2011). Vaginal Microbiome of Reproductive-Age Women. Proc. Natl. Acad. Sci. 108, 4680–4687. doi: 10.1073/pnas.1002611107
Romero, R., Chaiworapongsa, T., Kuivaniemi, H., Tromp, G. (2004). Bacterial Vaginosis, the Inflammatory Response and the Risk of Preterm Birth: A Role for Genetic Epidemiology in the Prevention of Preterm Birth. Am. J. Obstet. Gynecol. 190, 1509–1519. doi: 10.1016/j.ajog.2004.01.002
Rosca, A. S., Castro, J., Cerca, N. (2020). Evaluation of Different Culture Media to Support In Vitro Growth and Biofilm Formation of Bacterial Vaginosis-Associated Anaerobes. PeerJ 8, e9917. doi: 10.7717/peerj.9917
Rosca, A. S., Castro, J., França, Â., Vaneechoutte, M., Cerca, N. (2021). Gardnerella vaginalis Dominates Multi-Species Biofilms in Both Pre-Conditioned and Competitive In Vitro Biofilm Formation Models. Microb. Ecol. doi: 10.1038/s41598-019-50094-3
Salah, R. M., Allam, A. M., Magdy, A. M., Mohamed, A. S. (2013). Bacterial Vaginosis and Infertility: Cause or Association? Eur. J. Obstet. Gynecol. Reprod. Biol. 167, 59–63. doi: 10.1016/j.ejogrb.2012.10.031
Salgueiro, L. R., Pinto, E., Gonçalves, M. J., Pina-Vaz, C., Cavaleiro, C., Rodrigues, A. G., et al. (2004). Chemical Composition and Antifungal Activity of the Essential Oil of Thymbra Capitata. Planta Med. 70, 572–575. doi: 10.1055/s-2004-827162
Sherrard, J., Wilson, J., Donders, G., Mendling, W., Jensen, J. S. (2018). 2018 European (IUSTI/WHO) International Union Against Sexually Transmitted Infections (IUSTI) World Health Organisation (WHO) Guideline on the Management of Vaginal Discharge. Int. J. STD AIDS 29, 1258–1272. doi: 10.1177/0956462418785451
Shimaoka, M., Yo, Y., Doh, K., Kotani, Y., Suzuki, A., Tsuji, I., et al. (2019). Association Between Preterm Delivery and Bacterial Vaginosis With or Without Treatment. Sci. Rep. 9, 509. doi: 10.1038/s41598-018-36964-2
Sousa, L. G. V., Castro, J., Cavaleiro, C., Salgueiro, L., Tomás, M., Palmeira-Oliveira, R., et al. (2022). Synergistic Effects of Carvacrol, α-Terpinene, γ-Terpinene, ρ-Cymene and Linalool Against Gardnerella Species. Sci. Rep. 12, 1–16. doi: 10.1038/s41598-022-08217-w
Stan, D., Enciu, A. M., Mateescu, A. L., Ion, A. C., Brezeanu, A. C., Stan, D., et al. (2021). Natural Compounds With Antimicrobial and Antiviral Effect and Nanocarriers Used for Their Transportation. Front. Pharmacol. 12. doi: 10.3389/fphar.2021.723233
Stingley, R. L., Liu, H., Mullis, L. B., Elkins, C. A., Hart, M. E. (2014). Staphylococcus Aureus Toxic Shock Syndrome Toxin-1 (TSST-1) Production and Lactobacillus Species Growth in a Defined Medium Simulating Vaginal Secretions. J. Microbiol. Methods 106, 57–66. doi: 10.1016/j.mimet.2014.08.002
Swidsinski, A., Dörffel, Y., Loening-Baucke, V., Schilling, J., Mendling, W. (2011). Response of Gardnerella vaginalis Biofilm to 5 Days of Moxifloxacin Treatment. FEMS Immunol. Med. Microbiol. 61, 41–46. doi: 10.1111/j.1574-695X.2010.00743.x
Swidsinski, A., Mendling, W., Loening-Baucke, V., Ladhoff, A., Swidsinski, S., Hale, L. P., et al. (2005). Adherent Biofilms in Bacterial Vaginosis. Obstet. Gynecol. 106, 1013–1023. doi: 10.1097/01.AOG.0000183594.45524.d2
Swidsinski, A., Mendling, W., Loening-Baucke, V., Swidsinski, S., Dörffel, Y., Scholze, J., et al. (2008). An Adherent Gardnerella vaginalis Biofilm Persists on the Vaginal Epithelium After Standard Therapy With Oral Metronidazole. Am. J. Obstet. Gynecol. 198, 1–6. doi: 10.1016/j.ajog.2007.06.039
Tolker-Nielsen, T. (2015). Biofilm Development. Microbiol. Spectr. 3, MB–0001-2014. doi: 10.1128/microbiolspec.MB-0001-2014
Verdeguer, M., Torres-Pagan, N., Muñoz, M., Jouini, A., García-Plasencia, S., Chinchilla, P., et al. (2020). Herbicidal Activity of Thymbra Capitata (L.) Cav. Essential Oil. Molecules 25, 2832. doi: 10.3390/molecules25122832
Vodstrcil, L. A., Muzny, C. A., Plummer, E. L., Sobel, J. D., Bradshaw, C. S. (2021). Bacterial Vaginosis: Drivers of Recurrence and Challenges and Opportunities in Partner Treatment. BMC Med. 19, 1–12. doi: 10.1186/S12916-021-02077-3
Wallace, W. E. (2021). “Retention Indices”, in NIST Chemistry WebBook, NIST Standard Reference Database Number 69. Eds. Linstrom, P. J., Mallard, W. G. (Gaithersburg MD: National Institute of Standards and Technology), 20899. doi: 10.18434/T4D303
Keywords: bacterial vaginosis (BV), polymicrobial biofilms, alternative therapy, essential oils, microbial interactions
Citation: Rosca AS, Castro J, Sousa LGV, França A, Cavaleiro C, Salgueiro L and Cerca N (2022) Six Bacterial Vaginosis-Associated Species Can Form an In Vitro and Ex Vivo Polymicrobial Biofilm That Is Susceptible to Thymbra capitata Essential Oil. Front. Cell. Infect. Microbiol. 12:824860. doi: 10.3389/fcimb.2022.824860
Received: 29 November 2021; Accepted: 12 April 2022;
Published: 04 May 2022.
Edited by:
Diane McDougald, University of Technology Sydney, AustraliaReviewed by:
Christophe Beloin, Institut Pasteur, FranceAntónio Machado, Universidad San Francisco de Quito, Ecuador
Copyright © 2022 Rosca, Castro, Sousa, França, Cavaleiro, Salgueiro and Cerca. This is an open-access article distributed under the terms of the Creative Commons Attribution License (CC BY). The use, distribution or reproduction in other forums is permitted, provided the original author(s) and the copyright owner(s) are credited and that the original publication in this journal is cited, in accordance with accepted academic practice. No use, distribution or reproduction is permitted which does not comply with these terms.
*Correspondence: Nuno Cerca, bnVub2NlcmNhQGNlYi51bWluaG8ucHQ=