- 1Department of Emergency and Critical Care Medicine, Min-Sheng General Hospital, Taoyuan, Taiwan
- 2Department of Pharmacy, College of Pharmacy and Health care, Tajen University, Pingtung, Taiwan
- 3Division of Critical Care Medicine, Department of Emergency and Critical Care Medicine, Shuang Ho Hospital, Taipei Medical University, New Taipei City, Taiwan
- 4Department of Emergency, School of Medicine, College of Medicine, Taipei Medical University, Taipei, Taiwan
- 5Departments of Laboratory Medicine and Internal Medicine, China Medical University Hospital, School of Medicine, China Medical University, Taichung, Taiwan
- 6School of Medicine, China Medical University, Taichung, Taiwan
- 7Ph.D Program for Aging, School of Medicine, China Medical University, Taichung, Taiwan
- 8Departments of Laboratory Medicine and Internal Medicine, National Taiwan University Hospital, National Taiwan University College of Medicine, Taipei, Taiwan
Infections caused by multidrug-resistant (MDR) and extensively drug-resistant (XDR) Gram-negative bacteria (GNB), including carbapenem-resistant (CR) Enterobacterales (CRE; harboring mainly blaKPC, blaNDM, and blaOXA-48-like genes), CR- or MDR/XDR-Pseudomonas aeruginosa (production of VIM, IMP, or NDM carbapenemases combined with porin alteration), and Acinetobacter baumannii complex (producing mainly OXA-23, OXA-58-like carbapenemases), have gradually worsened and become a major challenge to public health because of limited antibiotic choice and high case-fatality rates. Diverse MDR/XDR-GNB isolates have been predominantly cultured from inpatients and hospital equipment/settings, but CRE has also been identified in community settings and long-term care facilities. Several CRE outbreaks cost hospitals and healthcare institutions huge economic burdens for disinfection and containment of their disseminations. Parenteral polymyxin B/E has been observed to have a poor pharmacokinetic profile for the treatment of CR- and XDR-GNB. It has been determined that tigecycline is suitable for the treatment of bloodstream infections owing to GNB, with a minimum inhibitory concentration of ≤ 0.5 mg/L. Ceftazidime-avibactam is a last-resort antibiotic against GNB of Ambler class A/C/D enzyme-producers and a majority of CR-P. aeruginosa isolates. Furthermore, ceftolozane-tazobactam is shown to exhibit excellent in vitro activity against CR- and XDR-P. aeruginosa isolates. Several pharmaceuticals have devoted to exploring novel antibiotics to combat these troublesome XDR-GNBs. Nevertheless, only few antibiotics are shown to be effective in vitro against CR/XDR-A. baumannii complex isolates. In this era of antibiotic pipelines, strict implementation of antibiotic stewardship is as important as in-time isolation cohorts in limiting the spread of CR/XDR-GNB and alleviating the worsening trends of resistance.
Introduction
Infections caused by multidrug-resistant (MDR) and extensively drug-resistant (XDR) Gram-negative bacteria (GNB) have become major challenges for global health institutions because of the limited antibiotic options and high mortality rates (Li et al., 2020; Chen H.Y. et al., 2021; Wang et al., 2021; Zhang et al., 2021b). Among the clinically important XDR-GNB species that harbor plasmidic genes encoding a wide variety of carbapenemases, isolates of sequence type (ST) 258, ST11, and ST147 Enterobacterales (mainly producing Klebsiella pneumoniae carbapenemase [KPC], metallo-β-lactamase [MBL, especially New Delhi MBL {NDM}], and oxacillinase [OXA], etc.), ST111 and ST235 Pseudomonas aeruginosa (mainly producing NDM, imipenemase [IMP], Verona integron-encoded MBL [VIM], and OXA, etc.), and ST2, ST32, ST92, and ST368 Acinetobacter baumannii complex (mainly producing MBL, NDM, and OXA, etc.), they usually co-harbor other resistant β-lactamases (various extended-spectrum β-lactamases [ESBLs] and/or AmpC β-lactamases), thus constitute a worrisome global threat because of their high potential for transmission (Tsakris et al., 2006; Vourli et al., 2006; Miriagou et al., 2007; Zappas et al., 2008; Giakkoupi et al., 2009; Da Silva et al., 2010; Kumarasamy et al., 2010; Papagiannitsis et al., 2010; Wang et al., 2010; Gupta et al., 2011; Chang et al., 2015; Huang et al., 2016; Roy Chowdhury et al., 2017; Yang et al., 2018; Nordmann and Poirel, 2019; Tavoschi et al., 2020; Yoon and Jeong, 2021). Similar to other surveys (Papadimitriou-Olivgeris et al., 2013; Lübbert et al., 2014), the study conducted by McConville et al. revealed that colonization with carbapenem-resistant (CR) Enterobacterales (CRE), which is a prerequisite for CRE infection (Kelly et al., 2017), was an independent predictor of 90-day mortality (adjusted odds ratio [OR]: 2.3; 95% confidence interval [CI]: 1.0–5.3; P = 0.056) (McConville et al., 2017). Furthermore, infections caused by CR- or carbapenemase-producing (CP)-GNB were shown to result in high mortality rates (Morata et al., 2012; Poulikakos et al., 2014; Cheng et al., 2015; Micek et al., 2015). This review focuses on global trends, resistance mechanisms, economic burdens, infection control policy and treatment options (including β-lactam combination agents with avibactam, zidebactam, enmetazobactam, relebactam, vaborbactam, nacubactam, durlobactam, and taniborbactam) of CR- and CP-GNB.
Global Trends of Carbapenem Resistance Among Important GNB Species
The increase in carbapenem resistance in clinically important GNB gradually worsened after 2005, particularly in GNB isolates cultured from patients hospitalized in the intensive care unit (ICU) (Davoudi-Monfared and Khalili, 2018). For example, from 2007 to 2008, Iran reported high imipenem resistance and MDR rates (41.8% and 56.3%, respectively) among P. aeruginosa isolates, especially those carrying class 1 integrons, or those cultured from patients undergoing surgery or hospitalized at the burn unit (Yousefi et al., 2010). Additionally, high MDR/CR rates (> 30%) among HAP-related P. aeruginosa isolates were observed in many member states of the European Union (EU) in the last decade (Micek et al., 2015). In contrast, the MDR rate of Taiwanese P. aeruginosa isolates was less than 18% before 2015. However, the 2016–2018 susceptibility survey of 1,127 P. aeruginosa isolates in Taiwan revealed a trend toward prominent escalation in the annual non-susceptible (NS) rates to anti-pseudomonal carbapenems (from 19.7% in 2016 to 27.5% in 2018 [Figure 1]; 95% CI: 0.545–0.936; P = 0.016) (Jean et al., 2021). When assessed using linear regression analysis, these results corresponded with the annual proportions of pneumonia-causing P. aeruginosa isolates (r = 0.980, P = 0.127) (Jean et al., 2021). An outbreak of XDR-P. aeruginosa infections was reported in a tertiary care pediatric hospital in Italy between 2011 and 2012 (Ciofi Degli Atti et al., 2014). The risk of colonization with CP-P. aeruginosa isolates harboring blaVIM was also reported to apparently increase with the length of hospital stay (especially > 30-day durations) (Neidhöfer et al., 2021).
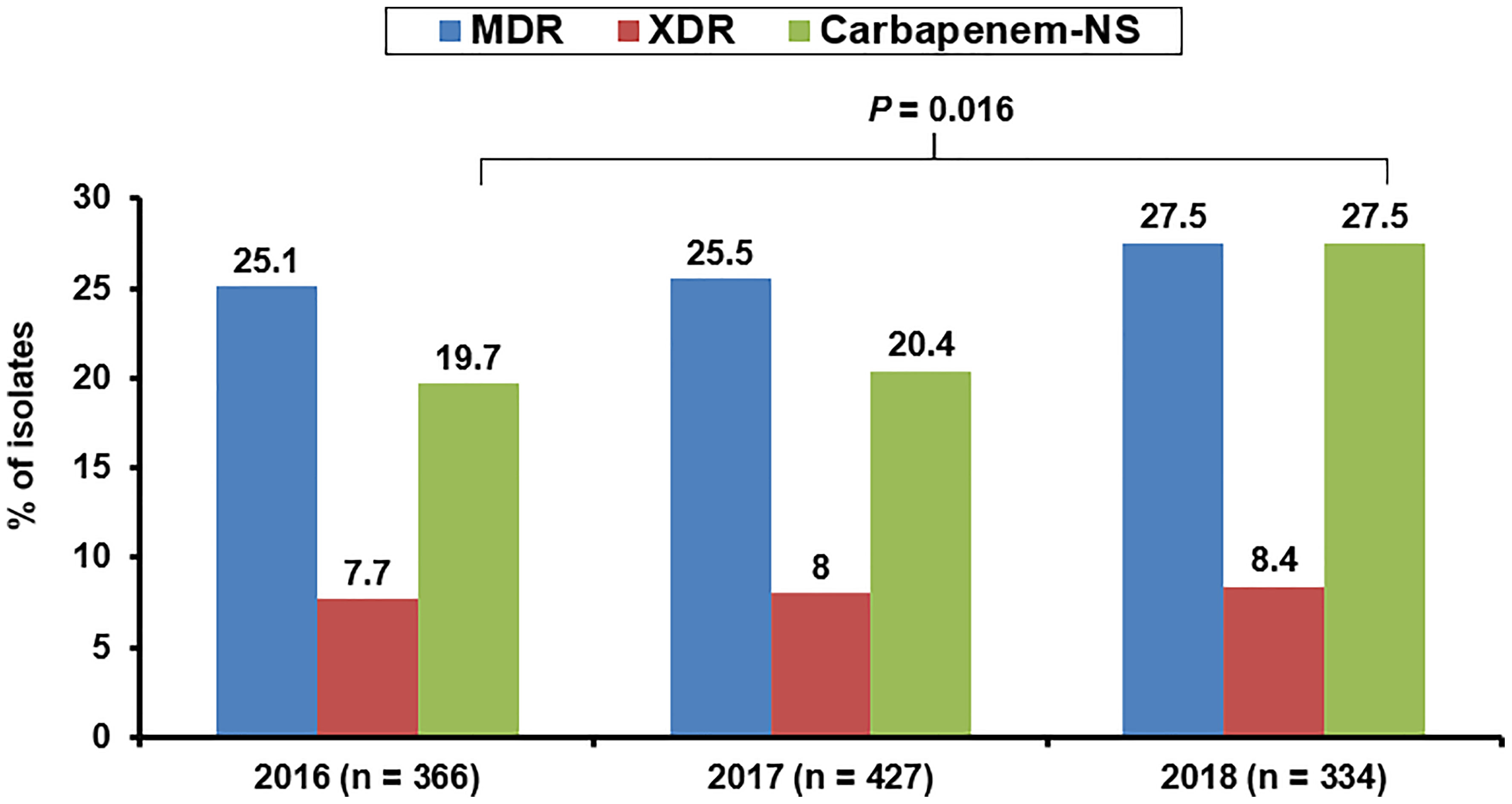
Figure 1 Annual rates of non-susceptibility to any anti-pseudomonal carbapenem agent among Pseudomonas aeruginosa cultured from three infection sources (respiratory tract, abdomen, and urinary tract) of patients hospitalized in Taiwan between 2016 and 2018.
After 2005, the isolates of MDR-A. baumannii complex harboring blaOXA-51 on the ISAba1 element and/or blaVIM/blaIMP on the class 1 integron displayed 45%–80% carbapenem resistance rates and were frequently associated with several clusters in the southern states of the EU after 2005 (Kempf and Rolain, 2012). Moreover, the plasmids on Acinetobacter species and some clinical A. baumannii isolates that harbor genetic determinants encoding various carbapenem-hydrolyzing class D β-lactamases (blaOXA-23, blaOXA-58, blaOXA-58-like, blaOXA-72, etc.) have also been shown to confer high-level resistance to all carbapenem agents in China and Taiwan (Jean et al., 2015). A study conducted at a German hospital by Neidhöfer et al. observed that the blaOXA-23-encoding A. baumannii complex was more frequently introduced into the hospital by patients residing in the Arabian Peninsula than those of German ethnicity, raising the concern of ethnic factors affecting the infection due to CP-A. baumannii (Neidhöfer et al., 2021). Furthermore, nosocomial infections due to MDR-A. baumannii complex in pediatrics hospitalized at ICU were reported in Turkey (Ozdemir et al., 2011). In contrast to a few Asian countries where an escalating antimicrobial resistance rate was observed among isolates of A. baumannii complex after 2011 (Jean et al., 2013), isolates of A. baumannii complex accounted for solely 2.8% of the implicated organisms among episodes of hospital-acquired pneumonia (HAP) acquired in ICUs in US hospitals between 2015 and 2017 (Sader et al., 2018).
In 2012, a 4.6% colonization rate was reported in a rectal swab CRE survey (utilizing MacConkey agar plate for culture and Xpert MDRO cartridge testing) for patients residing in the nursing homes of Rhode Island, USA (Cunha et al., 2016). In stark contrast, Asia and Africa are two leading continents with the highest global CR prevalence rates among Enterobacterales species (Tilahun et al., 2021). Notably, numerous outbreaks related to CRE involving a wide range of microbial species harboring diverse carbapenemase-encoding genes (blaKPC, blaNDM, blaVIM, blaOXA, etc.) have been reported in the literature (Chitnis et al., 2012; Kanamori et al., 2017; Mehta and Muscarella, 2020; Tavoschi et al., 2020; De Man et al., 2021). From March 2017 to September 2018, an epidemic spread of CRE, which was rapidly transmitted between hospitalized patients and associated with high mortality rates at 12 Vietnamese hospitals was reported by Tran et al. (Tran et al., 2019). Hospital-acquired infections and carbapenem therapy were determined to be two independent risk factors contributing to CRE colonization (ORs: 1.74 and 1.79, respectively) (Tran et al., 2019). It is noteworthy that CRE infections were also reported among pediatrics with co-morbidities during the last decade in Los Angeles, USA (Pannaraj et al., 2015). Additionally, endoscopy has surprisingly been found to be an under-recognized source of CRE transmission (Mehta and Muscarella, 2020). Consequently, there is a strong need for the utilization of high-level disinfection for cleaning the endoscopic equipment.
An outbreak owing to genetically related KPC-producing K. pneumoniae that persistently existed between March 2009 and February 2011 was reported at one acute care hospital in the USA (Chitnis et al., 2012). In addition, between November 2018 and October 2019, a delayed identification of a cluster involving 1,645 patients who were infected or colonized with NDM-producing CP-Enterobacterales (CPE) was reported in Tuscany, Italy. The majority (accounting for 90.9%) of the implicated CPE isolates that were mostly cultured from the intestinal tract of a total of 1,270 (77.2%) cases were ST147 and blaNDM-1-harboring CP-K. pneumoniae (Tavoschi et al., 2020).
Stenotrophomonas maltophilia, intrinsically resistant to all carbapenems, is an environmental MDR organism. Although no dominant S. maltophilia clone was identified globally (Kaiser et al., 2009; Duan et al., 2020), it has emerged as an important hospital-acquired pathogen globally (mainly causing primary bacteremia, pneumonia, catheter-associated infection, etc.) among hospitalized patients who receive broad-spectrum antibiotics (especially β-lactams) and/or immunosuppressive agents (Falagas et al., 2009; Brooke, 2012).
Resistance Mechanisms And Case-Fatality Rates Due To Important CR-GNB Infections
Research has revealed that isolates of A. baumannii possess several virulence factors, including pili, outer membrane protein A, lipopolysaccharide capsule, and phospholipase (Richards et al., 2015). Resistance mechanisms of MDR or XDR-A. baumannii complex, listed as one of the critical priority pathogens by experts of the World Health Organization (Tacconelli et al., 2018), usually include paucity of porins, constitutional expression of efflux pumps (AbeABC, AbeFGH, and AbeIJK), and expression of genes encoding various resistance β-lactamases (AmpC cephalosporinase, class B [NDM and VIM] and/or class D [OXA-23, OXA-58-like, etc.] β-lactamases) (Piperaki et al., 2019). High carriage rates of diverse types of OXA enzymes (blaOXA-51 and blaOXA-23, followed by blaOXA-58, blaOXA-24/40-like, etc.)-encoding genes (77%–100%) have been reported in clinical CR-A. baumannii isolates globally (Chang et al., 2015; Cortivo et al., 2015; Elabd et al., 2015; Lowings et al., 2015; Kateete et al., 2016; Wang T. H. et al., 2018; Ezadi et al., 2020). Furthermore, several acquired insertion sequences or transposons have been determined to promote the overexpression and spread of plasmid-associated blaOXA-58 genes in Acinetobacter species (Chen et al., 2010; Jean et al., 2015). In contrast to other regions, NDM-encoding genes have been frequently detected in CR-A. baumannii isolates in India (Vijayakumar et al., 2016). They frequently caused ventilator-associated pneumonia (VAP), catheter-associated, and bloodstream infections (BSIs) among debilitated patients hospitalized in the ICU, resulting in > 50% case-fatality rates (Poulikakos et al., 2014; Cheng et al., 2015).
Infections caused by CR/MDR-P aeruginosa are also usually observed in immunocompromised patients (recipients of chemotherapy and/or high-dose corticosteroids), who suffer from pneumonia (Morata et al., 2012) and require mechanical ventilation (Richards et al., 2015). Micek et al. observed that the prevalence rates of MDR phenotypes among P. aeruginosa isolates implicated in HAP in Europe between 2013 and 2014 ranged from 22.2% to 44.2% (Micek et al., 2015). Furthermore, compared to pneumonia due to non-CR/MDR P. aeruginosa isolates, patients with pneumonia caused by CR/MDR-P. aeruginosa had higher lengths of mechanical ventilation (13.1 days vs 17.0 days; P = 0.006) (Micek et al., 2015). The case-fatality rates among patients with pneumonia caused by CR/MDR-P. aeruginosa have been reported to range from 44.7% to 64% (Morata et al., 2012; Micek et al., 2015).
A study conducted by Kao et al., who analyzed the resistance mechanisms in 87 BSI-causing imipenem-resistant P. aeruginosa isolates collected in southern Taiwan between 2000 and 2010, revealed that carbapenemases (mainly VIM and OXA), active efflux pumps, and AmpC β-lactamase overproduction were found in 10.3%, 74.4%, and 51.3% of the P. aeruginosa isolates, respectively (Kao et al., 2016). The prevalence rate of metallo-β-lactamase (VIM, 6.4%) among Taiwanese imipenem-resistant P. aeruginosa isolates was similar to that in another Chinese study (8.5%) (Wang et al., 2010). However, another molecular study that examined CR and XDR-P. aeruginosa isolates (n = 466) collected in Canada between 2007 and 2016 revealed that solely 4.3% (n = 20) harbored a carbapenemase-encoding gene, with Guiana extended-spectrum-5 producers (n = 7 [35%]) surpassing other β-lactamase producers (McCracken et al., 2019). In stark contrast, the 2015–2017 CR-P. A study by Schäfer et al. at three medical centers in Germany revealed that 30.6% (19/62) of the samples harbored either blaVIM-1 (n = 2) or blaVIM-2 (n = 17) genes (Schäfer et al., 2019). The blaVIM carriage rate among CR-P. aeruginosa isolates was similar to that in the 2007–2009 survey conducted in Uganda (32%) (Kateete et al., 2016), and that in the 2015–2016 survey of Dubai hospitals in the United Arab Emirates (32%) (Moubareck et al., 2019). Among worldwide MDR-P. aeruginosa isolates collected in the last decade, clones ST111, ST175, and ST235 have been identified to carry genomic islands (Roy Chowdhury et al., 2017; Yoon and Jeong, 2021). ST235 (producing KPC, and mainly identified in Europe and Asia) and ST111 (producing VIM-2, and mainly identified in all six continents, except Oceania) clones are the most worrisome class A/B carbapenemase-producers of P. aeruginosa, because they are virulent and associated with poor outcomes (Yoon and Jeong, 2021). IMP followed by NDM and VIM have become the two most prevalent class B carbapenemases in worldwide P. aeruginosa isolates (Yoon and Jeong, 2021).
In the BSI-CRE (n=83) study conducted by Tamma et al. at the Johns Hopkins Hospital, USA, between March 2013 and April 2016, 37 (45%) isolates were CP-CRE, of which 92% harbored the blaKPC-2 gene (Tamma et al., 2017). The CP prevalence rate was highly similar to that reported in a Taiwanese 2017 study (Jean et al., 2018a). In contrast, approximately 90% (121/135) of CRE isolates collected from cancer patients between October 2016 and September 2017 in Egypt, where NDM and OXA-48-like enzymes are prevalent, harbored one or more of the carbapenemase-encoding genes, as revealed by polymerase chain reaction (PCR) (Tawfick et al., 2020). Moreover, PCR analysis of rectal swabs from 590 patients hospitalized in Kuwait between April 2017 and March 2018 revealed that 38 (65.5%) out of the 58 patients (9.8%) with rectal CRE colonization harbored the blaOXA-181-like gene (combined with blaKPC-2 [n = 5], blaVIM-1 [n = 4], and blaNDM-5 [n = 3]) (Fadhli et al., 2020). Previous studies have revealed that high treatment failure rates are associated with KPC-producing Enterobacterales infections, especially among immunocompromised hosts (Jean et al., 2015; Tawfick et al., 2020). In similarity to invasive infections (bacteremia and pneumonia) that are caused by MDR-P. aeruginosa or MDR-A. baumannii complex resulting in poorer outcomes than those caused by susceptible isolates (Lee et al., 2011; Micek et al., 2015), the all-cause mortality rates related to diverse CRE infections have been notably reported to range from 22% to 72% (Borer et al., 2009; Hirsch and Tam, 2010a; Kalpoe et al., 2012; Tumbarello et al., 2012; Chen H. Y. et al., 2021). Furthermore, a study conducted by Tamma et al. revealed that 32% (12/37) of patients infected with CP-CRE BSIs died within 14 days of admission, with an adjusted OR of 4.92 as compared to non-CP-CRE BSI (Tamma et al., 2017).
The major mechanisms of resistance to carbapenems in S. maltophilia isolates mainly include plasmid-encoding L1/L2 β-lactamases (Avison et al., 2001), and chromosomally encoded MDR efflux pumps (Poole, 2004). High rates of case-fatality (42.6%) and attributable mortality (37.5%) were notably reported in patients with S. maltophilia-causing pneumonia and septicemia, respectively (Falagas et al., 2009; Tseng et al., 2009). Additionally, a Taiwanese study regarding S. maltophilia bacteremia indicated that pediatric patients who had malignancy or failed to remove central venous catheters were at high risk of in-hospital mortality (Wu et al., 2006).
Table 1 presents the prevalence rates of genes encoding carbapenemases among isolates of CRE, CR-Pseudomonas aeruginosa, and CR-A. baumannii in different surveys conducted in different countries. Additionally, Table 2 illustrates the enzymatic and non-enzymatic mechanisms conferring carbapenem resistance among isolates of CRE, CR-P. aeruginosa, and CR-A. baumannii complex.
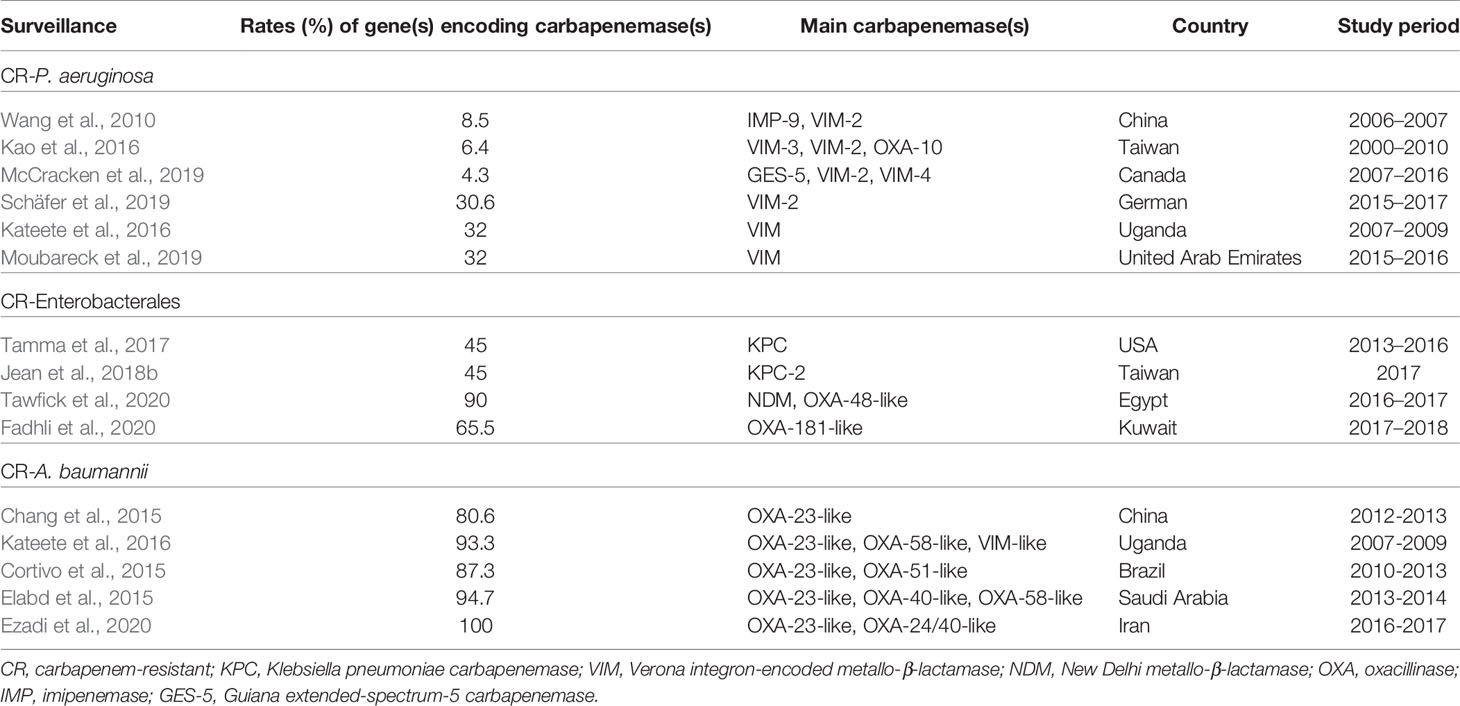
Table 1 Rates of gene(s) encoding carbapenemases among isolates of carbapenem-resistant (CR) Enterobacterales, CR- Pseudomonas aeruginosa and CR-Acinetobacter baumannii complex in different surveillances.
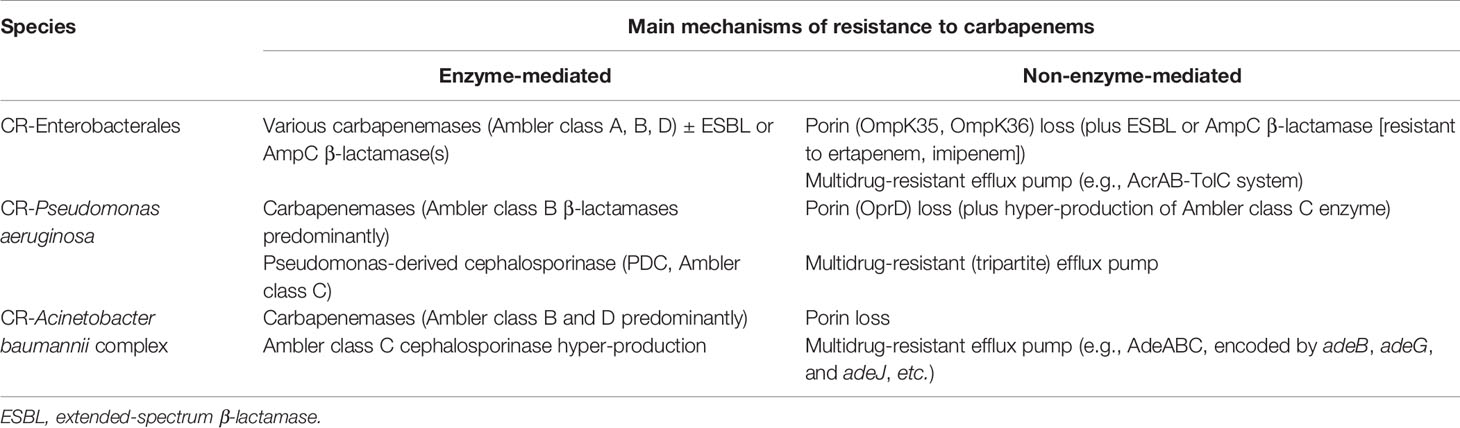
Table 2 The mechanisms of carbapenem resistance in carbapenem-resistant (CR) Enterobacterales species, CR-Pseudomonas aeruginosa, and CR-Acinetobacter baumannii complex.
Economic Burden Caused by CRE Outbreaks And Screening Strategies
The huge economic burden caused by MDR-GNB infections, most of which were limited to CRE, has been intensively analyzed. Bartsch et al. investigated the median cost of CRE infections with an incidence of 2.93 per 100,000 persons in the USA in 2015. They estimated that it cost hospitals 275 million US dollars, third-party payers 147 million US dollars, and society 553 million US dollars. In addition, costs increased proportionally with the incidence of CRE, with increases of 2.0-fold, 3.4-fold, and 5.1-fold for incidence rates of 6, 10, and 15 per 100,000 persons, respectively (Bartsch et al., 2017). In-hospital outbreaks of CR- or CP-GNB further exacerbate the already high-cost burden. An outbreak of CPE (caused by mainly NDM-producing K. pneumoniae), which occurred at a London hospital from July 2014 to October 2015, cost €1.1 million, with €54,000 spent on antibiotics for 18 patients who needed treatment, €94,000 on laboratory screening, €153,000 on Estates renovations, and €822,000 as opportunity cost (staff time, bed closure, and elective surgical missed revenue) (Otter et al., 2017). Furthermore, an investigation conducted by Yang et al. at a tertiary teaching hospital in China from 2011 to 2016 revealed that the direct economic burden and disability-adjusted life-year loss caused by BSI due to CR-P. aeruginosa was ≥ 3-fold higher than that of carbapenem-susceptible P. aeruginosa BSI (Yang et al., 2021). According to Semin-Pelletier et al., frequent readmissions of patients who had previous infections due to OXA-48-producing K. pneumoniae isolates, the large number of transfers between wards, and a delay in the implementation of successive cohort units greatly contributed to the incomplete success of containing the spread of CPE (Semin-Pelletier et al., 2015). A few physicians have adopted aggressive surveys of samples from patients to interrupt the transmission of CRE/CPE. For example, a survey at a tertiary teaching hospital in Malaysia revealed that 5.74% of Enterobacterales isolates cultured from various clinical samples (of which rectal swab screening accounted for 49.3%) were CRE, most of which were K. pneumoniae isolates harboring blaNDM-1 (Zaidah et al., 2017). In the multicenter survey conducted by Jimenez et al. in Miami, Florida from 2012 to 2016, active surveillance testing revealed that the overall CRE prevalence was 0.077 cases per 100 patient-admissions, while the incidence density was 1.46 cases per 10,000 patient-days. It is also noteworthy that rates of CRE (dominated by K. pneumoniae, Enterobacter cloacae, and Escherichia coli) steadily increased during the first 3 years of the study period, and declined after implementation of infection control strategies (contact precautions, environmental disinfection, etc.) (Jimenez et al., 2020).
Owing to the high burden of medical costs and high case-fatality rates seen for inpatients with CPE infections, attempts have been made to improve the cost-effectiveness of screening for CPE among all hospital inpatients (Kang et al., 2020). Among the different culture methods, the ChromID CARBA method (bioMérieux, Marcy l’Etoile, France) has been to perform rapidly and best in the detection of CPE (> 100 colony forming units/spot) from rectal swabs, in terms of sensitivity (92.4%) and specificity (96.9%) (Vrioni et al., 2012; Wilkinson et al., 2012). Despite a lower reagent cost, culture-based methods are, in fact, less sensitive than molecular methods for the detection of infection or colonization of CPE. Moreover, they are labor-intensive and slower to yield results (Richter and Marchaim, 2017). Lapointe-Shaw et al. observed that screening for CPE might be cost-effective as compared to not screening, if the prevalence of CPE was above 0.3% among the isolates of Enterobacterales under survey (Lapointe-Shaw et al., 2017).
Community-Acquired/Community-Onset CRE, and CRE, CR-P. Aeruginosa as Well as CR-A. Baumannii Complex Recovered from The Long-Term Care Facility (LTCF)
Apart from hospital acquired CRE, the presence of CRE in the community setting is also a potentially worrisome threat to the public health of ambulatory patients (Van Duin and Paterson, 2016; Jean et al., 2018b). A meta-analysis conducted by Kelly et al. revealed that 5.6%–10.8% of rates of colonized CRE isolates belonged to the community-associated/community-onset category (CA/CO; defined as identification of asymptomatic CRE colonization at the time of admission) in the USA-based studies, while percentages ranging from 0.04% to 29.5% of colonized CRE categorized as CA/CO in origin were reported globally (Kelly et al., 2017). Compared to healthcare-acquired CRE, a Taiwanese survey conducted by Tang et al. revealed that CA-CRE was more likely to occur in elderly female patients and result in urinary tract infection (UTI) (Tang et al., 2016). In a study conducted in China, Hu et al. also found that CO-CRE isolates (n = 28, accounting for 43.8% of all infection-causing CRE isolates enrolled) were mainly cultured from urine samples (75%). Of the CO-CRE isolates, 8 (28.6%) were clonally unrelated E. coli isolates, all of which were NDM producers that were less resistant to aztreonam, ciprofloxacin, levofloxacin, and chloramphenicol. pneumoniae (Hu et al., 2020).
The emergence of CRE at an LTCF (an institution that provides long-term rehabilitation and skilled nursing care) is also a major healthcare issue (Vrioni et al., 2012). The prevalence and incidence rate of colonized CRE in LTCFs in the USA ranged from 3% to 30.4% (Lin et al., 2013; McKinnell et al., 2019) and 1.07 to 6.83 cases per 10,000 patient-days (Brennan et al., 2014; Chopra et al., 2018), respectively. In contrast, the CRE prevalence rates differed widely among the states of the EU (Chen C.C. et al., 2021). A significant association (ranging from 12% to 15.5%) between CRE colonization in nursing home residents with hospital admissions was observed in Spain (predominantly K. pneumoniae harboring blaOXA-48-like gene) (Palacios-Baena et al., 2016) and Israel (mainly KPC-producing K. pneumoniae) (Ben-David et al., 2011). Accordingly, nursing home residents have become CRE reservoirs that should not be ignored. Multiple risk factors, including fecal incontinence (OR: 5.78) (Mills et al., 2016), an immunocompromised condition (OR: 3.92), comorbidities (Charlson score > 3; OR: 4.85) (Bhargava et al., 2014), use of gastrointestinal devices (OR: 19.7) (McKinnell et al., 2019), intravascular indwelling devices or urinary catheters (OR: 5.21) (Lin et al., 2013), and mechanical ventilation (OR: 3.56) (Mills et al., 2016), sharing a room with a known CRE carrier (Chitnis et al., 2012), and prior antibiotic exposure (OR: 3.89) (Chitnis et al., 2012; Bhargava et al., 2014; Brennan et al., 2014), etc., has significantly associated with increased vulnerability to CRE colonization and/or infections in LTCF residents. It is noteworthy that patients from LTCFs who were colonized or infected with CRE had notably poor clinical outcomes, with a mortality rate of up to 75% among patients with CRE infection (Borer et al., 2012). In contrast, the prevalence rates of CRE colonization among residents of LTCFs ranged from 13% to 22.7% in Asia (Lee et al., 2017; Hagiya et al., 2018; Jean et al., 2018b; Le et al., 2020). As stated in a survey on the distribution of carbapenemase-encoding genes among LTCF residents (Jean et al., 2015), ST258 CR-K. pneumoniae harboring blaKPC-2 or blaKPC-3 were the predominant clones present in residents of LTCFs in the USA, whereas other carbapenemases (including NDM, VIM, IMP, and OXA-48-like) were uncommon among CRE isolates for them (Prasad et al., 2016; Reuben et al., 2017; Dubendris et al., 2020; Chen C.C. et al., 2021). In Japan, various types of IMP (predominantly IMP-42, IMP-11, followed by IMP-6) carbapenemases have been detected among overall CRE isolates in LTCF residents in Japan (Hagiya et al., 2018; Hayakawa et al., 2020). Data from the CRE survey of LTCF residents in Spain revealed that OXA-48-like enzymes, followed by VIM-1, IMP, and KPC are the main carbapenemases that can be found there (Palacios-Baena et al., 2016).
Although P. aeruginosa is not a common colonized GNB cultured from LTCF residents, as reported by the survey conducted by O’Fallon et al. in the USA (O’Fallon et al., 2009), and Italian surveys of Giufrè et al. (Giufrè et al., 2017) and March et al. (March et al., 2017), it has been shown to be easily transmitted through numerous routes, including patient-to-patient contact and environmental contamination (Jefferies et al., 2012). Thus, it is plausible that the CP-P. aeruginosa (harboring blaVIM) isolates were cultured from LTCF residents who have history of frequent hospitalizations (Nucleo et al., 2018). A study conducted by Raman et al. revealed that MDR/CR-P. aeruginosa can be identified from patients who were transferred from chronic care facilities and exposed to piperacillin-tazobactam (adjusted OR: 2.64) or carbapenem (adjusted OR: 4.36) (Raman et al., 2018).
Table 3 illustrates the summary of CRE, CR-P. aeruginosa, and CR-A. baumannii cultured from the community setting and LTCF.
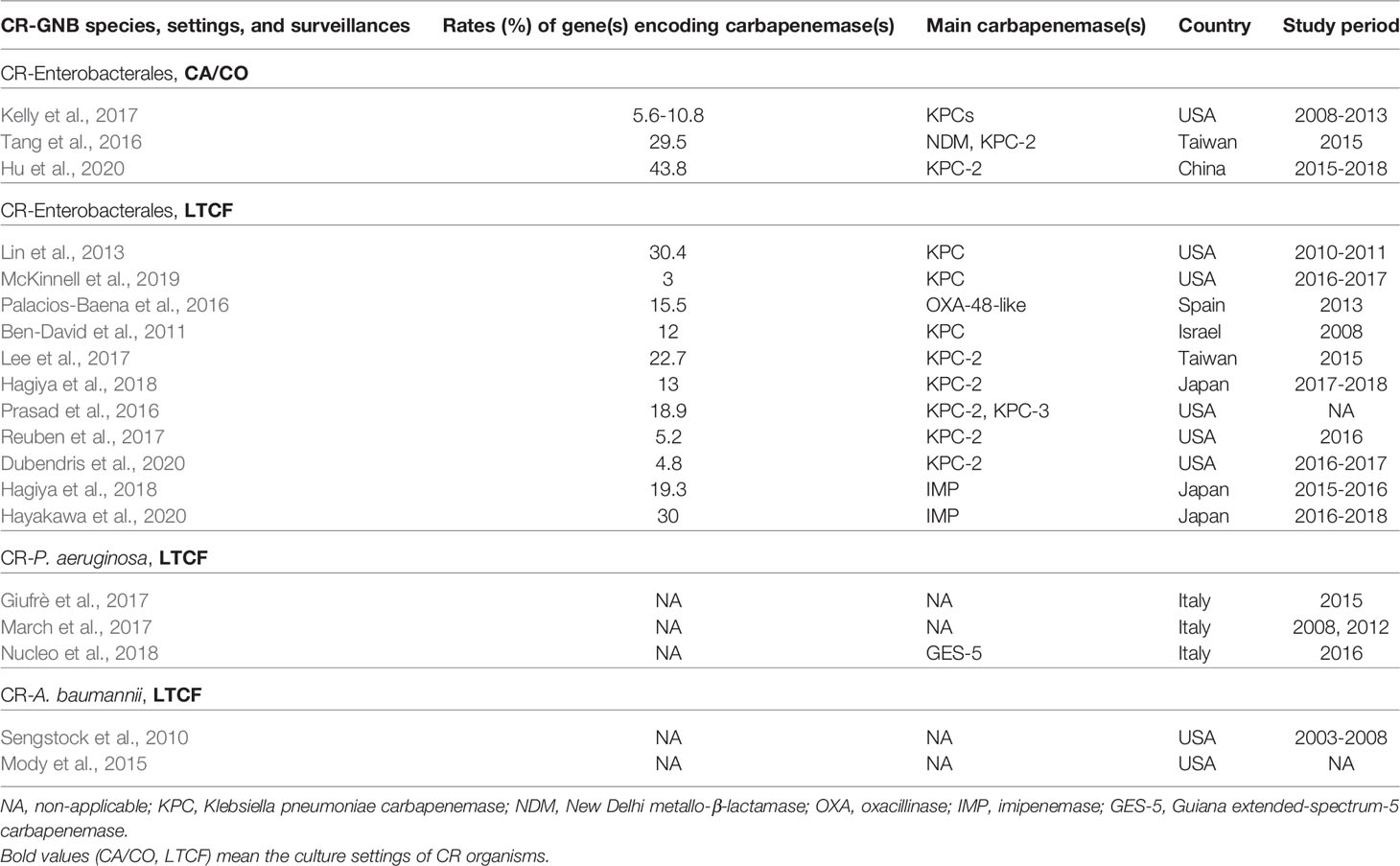
Table 3 Summary of reports on carbapenem-resistant (CR) Enterobacterales (CRE), and CRE, CR-P. aeruginosa, and CR-A. baumannii complex at the community setting, community-acquired/community-onset (CA/CO), and the long-term care facility (LTCF).
Infection Control Policy Regarding CR- or XDR-GNB
Early identification of in-hospital patients with risk factors for CRE acquisition (72.7% of which were K. pneumoniae), such as co-colonization with MDR-A. baumannii complex (adjusted OR: 15.6) or ESBL-producing GNB (adjusted OR: 4.7; might be linked to recent carbapenem use), exposure to glycopeptide antibiotics (adjusted OR: 3.6), and admissions within one year (adjusted OR: 3.9), is beneficial for avoiding potential spread (Kang et al., 2019). In addition, excessive consumption of carbapenems (especially group 2 agents) is considered to be an important predisposing factor that contributes to the worsening rates of infections caused by CR-P. aeruginosa (estimated OR: 2.87 - 40.96) (Voor In ’t Holt et al., 2014), CRE (McLaughlin et al., 2013), and CR-A. baumannii complex (Sheng et al., 2010; Yoon et al., 2014). Consequently, appropriate antibiotic control policies (especially strict implementation of antibiotic stewardship) in hospitals, education of primary care staff to prevent the dissemination of high-risk hospital and LTCF microorganisms, and contacting isolation cohorts (Barlam et al., 2016; Hellyer et al., 2016) are of paramount importance in containing the spread of CR- or MDR/XDR-GNB strains and decreasing case-fatality rates. Similarly, there is a need to implement periodical surveillance testing to determine in-time targeted interventions (e.g., isolating or cohorting CRE carriers and nursing staff) are needed to effectively lessen the CRE outbreaks at LTCFs (Ben-David et al., 2011; Chitnis et al., 2012; Chen H. Y. et al., 2021).
Different Phenotypic, Biochemial and Immunological Techniques for Diagnosis of CR-GNB
The distinguishment of CR-GNB that are due to the non-carbapenemase-mediated mechanisms from CP-GNB is important, because CP-GNB are prone to disseminate between patients more readily than non-CP-GNB (Goodman et al., 2016). Among the phenotypic CRE diagnostic assays commonly used in clinical microbiology laboratories, the modified Hodge test was applied earliest to detect potential KPC producers of CRE. However, it showed poor sensitivity in detection of the NDM and OXA-48-like CPE, and false-positive results are seen in CRE owing to the porin alteration combined with hyperproduction of ESBL and/or AmpC β-lactamase (Tamma and Simner, 2018; Clinical and Laboratory Standards Institute (CLSI), 2021). The Carba NP test and its variants are suitable for detection of various carbapenemases in CRE and CR-P. aeruginosa (Tamma and Simner, 2018). In spite of requiring the acquisition of dedicated reagents and being interpreted subjectively, the Carba NP test is a convenient biochemical test that provides the results within 15-30 minutes and thus has been applied in the clinical microbiological laboratories worldwide (Clinical and Laboratory Standards Institute (CLSI), 2021). Additionally, the CarbAcineto NP test was shown to perform well in detecting carbapenemases produced by CR-A. baumannii (Dortet et al., 2014).
Utilization of the modified carbapenem inactivation method (mCIM) in combination with the EDTA-modified CIM (eCIM) test could reliably differentiate MBL-producing CRE strains (those displaying a negative result on only the eCIM test) from serine-class carbapenemase producers (showing positive results for both tests) (Tamma and Simner, 2018; Jean et al., 2019). Nevertheless, it takes approximately 6-12 h to obtain the results and is interpreted subjectively as well. It is also noteworthy that the mCIM/eCIM test performed less well in detection of VIM-producing P. aeruginosa and OXA-producing A. baumannii isolates than CPE (Tamma and Simner, 2018).
The other carbapenemase detection tests include lateral flow immunoassays (antibody-based rapid diagnostic, easy-to-use methods, such as NG-Test Carba 5 [Hardy Diagnostics, CA, USA], and Resist-3 O.K.N. that detects OXA-48-like, KPC and NDM but not VIM and IMP-like MBLs [Coris BioConcept, Gembloux, Belgium]), targeted carbapenemase assays (inhibitor [phenylboronic acid for KPC and EDTA for MBL]-based, easy-to-use methods) for diverse carbapenemases, and matrix-assisted laser desorption–ionization time of flight mass spectrometry (hydrolysis approach) (Wareham and Abdul Momin, 2017; Tamma and Simner, 2018).
Antibiotic Treatment Against CR- or XDR-GNB
Conventional Antibiotic Regimens Against CRE
In a study by Garonzik et al., optimum dosages of polymyxin B and colistin (polymyxin E), two old antibiotics of revival in this century, have been proposed to maximize their efficacy against infections related to MDR-GNB, with a minimum inhibitory concentration (MIC) of >1 mg/L for colistin (Garonzik et al., 2011). Clinical, pharmacokinetic (PK) and pharmacodynamic (PD) data of polymyxin B/E for several GNB species have been shown to have limited clinical efficacy, even if an intermediate result (i.e., MIC ≤ 2 mg/L) was achieved (Clinical and Laboratory Standards Institute (CLSI), 2021). Moreover, monotherapy with intravenous colistin against CR-K. pneumoniae or E. coli bacteremia was also not suggested, because of its association with a high (57.1%) mortality rate (hazard ratio: 5.57; 95% CI, 2.13 - 14.61; P < 0.001) as compared to other comparative antibiotics (Lin et al., 2018). Despite controversies, during the interval of an aerosolized colistimethate sodium (CMS) dosing (2 million units [MU]) using jet or ultrasonic nebulizer, a high pulmonary area under the concentration-time curve of colistin (ranging 18.9 – 73.1 μg•h/mL), as well as a high maximum pulmonary colistin concentration (6.00 ± 3.45 μg/mL) were achieved in humans, with no increase in nephrotoxicity (Yapa et al., 2014). Thus, a high-dose regimen of aerosolized CMS monotherapy (4 MU administered every 8 h) was applied for the treatment of VAP caused by a few notable MDR-GNB (P. aeruginosa and A. baumannii complex predominantly) (Abdellatif et al., 2016). In addition, impaired uptake of fosfomycin (related to glpT, uhpT, and uhpA) (Takahata et al., 2010) and the existence of fosfomycin-modified genes (e.g., fosA3, which encodes fosfomycin-modifying enzymes) in transposon elements and conjugative plasmids confer Enterobacterales species, especially E. coli, exhibiting resistance to fosfomycin (Yang et al., 2019). High prevalence rates of fosfomycin resistance genes in CRE have been determined in several parts of East Asia (e.g., China and Japan) (Zhang et al., 2017; Wang Q. et al., 2018), and resistance to fosfomycin has also emerged in the USA and the states of the EU (Alrowais et al., 2015; Benzerara et al., 2017). Therefore, the use of systemic colistin and fosfomycin is recommended as an adjunctive treatment for CRE or CR-P. aeruginosa infections (Amladi et al., 2019; Loose et al., 2019).
Before the launch of ceftazidime-avibactam for the clinical treatment of CPE infection in 2015, Gutiérrez-Gutiérrez et al. concluded that therapy with a combination regimen of antibiotics (including at least one in vitro active drug against the implicated BSI isolate and started in the first five days after infection) was associated with decreased 30-day all-cause mortality rates among patients infected with BSI owing to CPE (mainly KPC-producing K. pneumoniae) and high INCREMENT-CPE mortality scores (high Pitt bacteremic scores in patients who had ≥ 2 points of Charlson comorbidity score and BSI not originating from UTI or biliary tract infection) (Gutiérrez-Gutiérrez et al., 2016; Gutiérrez-Gutiérrez et al., 2017). Despite its bacteriostatic nature and relatively low serum concentration under standard-dose administration (100 mg loading followed by 50 mg every 12 h) (Jean and Hsueh, 2014), the study by Lin et al. on CRE bacteremia revealed that tigecycline was a good choice if the antibiotic had an MIC ≤ 0.5 mg/L against the isolates, based on the 90% probability of target attainment and 82% probability of cumulative fraction of response (Lin et al., 2018). Nevertheless, heterogeneity in adequate regimens of antibiotics against CPE has been suggested for both in vitro efficacy and clinical treatment effects (Bulik and Nicolau, 2011; Jernigan et al., 2012; Tzouvelekis et al., 2014; Lowman and Schleicher, 2015; De Pascale et al., 2017; Tang et al., 2019). For example, Tang et al. determined that in vitro treatment with 1× MIC using combinations of amikacin or gentamicin, and tigecycline or doxycycline for 24 h resulted in bactericidal activity of 84%–100% in 13 KPC-K. pneumoniae isolates; in addition, the combination of doxycycline plus gentamicin or amikacin was synergistic for all the tested KPC-K. pneumoniae isolates (Tang et al., 2019). A review of CPE therapy by Tzouvelekis et al. and an investigation conducted by Daikos et al. concluded that the lowest mortality rate (18.8%) was observed in patients treated with combinations of various antibiotics (Daikos et al., 2014; Tzouvelekis et al., 2014). Compared to other antibiotic regimens, Tumbarello et al. further demonstrated that post-antibiogram therapy with a combination of tigecycline, colistin, and meropenem (at a dosage of 2 g every 8 h intravenously) was associated with lower mortality rates (OR, 0.11; 95% CI: 0.02 - 0.69; and P = 0.01) for patients with BSI due to KPC-producing K. pneumoniae, especially those with an MIC ≤ 16 mg/L for meropenem (Tumbarello et al., 2012). In addition, Lowman et al. observed that carbapenem-based therapy improved survival in 20 critically ill patients infected with CPE harboring blaOXA-48-like gene, although the severity of the underlying illness significantly impacted their outcomes as well (Lowman and Schleicher, 2015). The combination of doripenem (8 mg/L) and colistin (1 mg/L) was observed to have in vitro bactericidal efficacy against 75% (9/12) of KPC-producing K. pneumoniae isolates (Jernigan et al., 2012). As compared to the clinical treatment using a single antibiotic (colistin, tigecycline, or gentamicin), De Pascale et al. determined that a double carbapenem regimen (comprising meropenem at a dosage of 2 g every 8 h intravenously, and ertapenem at a dosage of either 2 g once daily or 1 g every 12 h intravenously) resulted in a significantly lower rate of 28-day mortality (47.9% vs 29.2%; P = 0.04), higher rates of clinical cure (31.3% vs 65%; P = 0.03) and microbiological eradication (25.9% vs 57.9%; P = 0.04) for patients with severe infections due to CR-K. pneumoniae (> 90% of which harbor blaKPC) (De Pascale et al., 2017). A similar in vivo effect was also reported by Builk et al. in a murine thigh model with KPC-producing K. pneumoniae infection, using simulated mega-dose doripenem (2 g every 8 h) combined with ertapenem (1 g once daily) (Bulik and Nicolau, 2011).
Novel Antibiotics Against CR/CP-Enterobacterales, and CR-P. Aeruginosa
Ceftazidime combined with avibactam, a diazabicyclooctane (DBO) β-lactamase inhibitor, is effective against most Ambler class A/C/D enzymes, providing a new option for the treatment of CRE/CPE infections (Liu et al., 2021); however, mutations in Asp179Tyr, Val240Gly, Ala240Val, Ala177Glu, Thr243Met substitutions, and 165-166Glu-Leu insertion, have been shown to compromise the in vitro efficacy of ceftazidime-avibactam while sparing several other novel antibiotics against CRE isolates (Wang Y. et al., 2020). In addition, recent investigations have revealed that a significant proportion of CR-E. cloacae complex exhibited significantly higher NS rates to ceftazidime-avibactam (Yin et al., 2019; Kawai et al., 2020). In the small case series covering diverse CRE infections (n = 37, with five-sixths being CR-K. pneumoniae), Shields et al. observed that ceftazidime-avibactam therapy achieved a success rate of 70% and 50%, respectively, for bacteremia and pneumonia, and 100% for acute pyelonephritis. Furthermore, resistance to ceftazidime-avibactam among CRE developed notably following its therapy for 10–19 days (median, 15 days) (Shields et al., 2016). The overall 30-day survival rate of the series by Shields et al. was 76% (Shields et al., 2016), similar to that of another survey on CR-K. pneumoniae therapy using antibiotic combination regimens (72.5%) (Capone et al., 2013). In a small cohort (n = 38) survey conducted by Alraddadi et al. to explore the outcomes of patients with various infections caused by CRE (largely OXA-48-producing K. pneumoniae isolates), ceftazidime-avibactam therapy provided a better clinical remission rate than comparative antibiotics, including colistin, tigecycline, and meropenem (80% vs 53.6%; P = 0.14) (Alraddadi et al., 2019). Nevertheless, no difference was observed in the 30-day all-cause mortality rates between the two groups (50% vs 57.1%; P = 0.71) (Alraddadi et al., 2019). Furthermore, in a head-to-head study conducted by Ackley et al. who compared the clinical efficacy and development of resistance between ceftazidime-avibactam and meropenem-vaborbactam (a β-lactamase inhibitor that comprises structurally boronic acid [BA]) against CRE, a 2.9% rate of resistance developed after ceftazidime-avibactam monotherapy, while similar rates of clinical success and 90-day mortality were observed between the two groups (Ackley et al., 2020).
Among other potential novel antibiotics, ceftolozane-tazobactam was inactive against isolates of CPE isolates, several AmpC-producing Enterobacterales, and most ESBL-producing K. pneumoniae (Jean et al., 2021). Cefepime in combination with enmetazobactam (formerly AAI101, a β-lactamase inhibitor that structurally comprises penicillanic acid sulfone), exhibited lower MICs against isolates of ESBL-producing Enterobacterales and KPC-producing E. coli than CP-K. pneumoniae (Morrissey et al., 2019; Papp-Wallace et al., 2019; Jean et al., 2022). In contrast, cefepime combined with zidebactam (formerly WCK 5222, a structural DBO β-lactamase inhibitor) displayed superior in vitro activity against the Ambler class B enzyme producers of Enterobacterales than ceftazidime-avibactam and imipenem in combination with relebactam (also a structural DBO β-lactamase inhibitor), meropenem in combination with vaborbactam, cefepime-enmetazobactam, durlobactam (formerly ETX 2514, a structural DBO β-lactamase inhibitor, penicillin-binding protein [PBP] 2 inhibitor, and in vitro activity against P. aeruginosa AmpC β-lactamase), and plazomycin (Landman et al., 2010; Karlowsky et al., 2020; Novelli et al., 2020; Hagihara et al., 2021; Kuo et al., 2021; Shapiro et al., 2021; Jean et al., 2022). Furthermore, the anti-CPE spectra of aztreonam-avibactam and cefepime combined with taniborbactam [also a structural BA β-lactamase inhibitor against all metallo-β-lactamases (MBL), except for the IMP types], are considerably similar to those of cefepime-zidebactam (Karlowsky et al., 2017; Wang X. et al., 2020; Sader et al., 2021) and cefiderocol (Hsueh et al., 2019). Nacubactam (formerly OP0595 or RG6080, a structural DBO β-lactamase and PBP2 inhibitor) was demonstrated to be active in vitro against diverse β-lactamase producers, including a few NDM or VIM-producing Enterobacterales species (mainly E. coli and Enterobacter spp.) (Mushtaq et al., 2019). When nacubactam was combined with meropenem, it was shown to be active in vitro against the CRE isolates of any β-lactamase producer. Phase 3 clinical investigation is currently being conducted to test the efficacy of nacubactam in combination with meropenem against CRE isolates harboring the blaKPC gene (Barnes et al., 2019).
As stated in several studies, septicemia or pneumonia caused by MDR-P. aeruginosa isolates resulted in poor patient outcomes (Hirsch and Tam, 2010b; Morata et al., 2012; Micek et al., 2015; Matos et al., 2018; Urzedo et al., 2020). Recommendations for the treatment of MDR/XDR-GNB infections have been published based on the susceptibility and PK/PD profiles of conventional and novel antibiotics (Bassetti et al., 2019; Jean et al., 2019; Jean et al., 2020). Antibiotic combination therapy is likely to select mutants displaying a broader resistance phenotype (e.g., mutational inactivation of the repressor gene mexR that regulates the multidrug efflux operon mexAB–oprM for P. aeruginosa) than before (Vestergaard et al., 2016). Nevertheless, a few combination regimens of dual antibiotics exhibited synergistic or additive effects in vitro (determined using fractional inhibitory concentration index) against CR- or MDR/XDR-P. aeruginosa isolates (Erdem et al., 2002; Siriyong et al., 2019; Olsson et al., 2020). In addition, the combination of gentamicin and ciprofloxacin has good potential for inhibiting biofilm formation synthesized from P. aeruginosa (71.4%) (Wang et al., 2019). De-escalation of antibiotics into a single agent is strongly recommended when susceptibility of the implicated GNB (including MDR/XDR-P. aeruginosa) isolate is known, and there is a significant improvement in the patient’s condition (Boyd and Nailor, 2011). Among the novel antibiotics that have been launched to combat MDR-GNB isolates, ceftolozane-tazobactam has excellent in vitro activity against global CR- and XDR-P. aeruginosa strains, including those with overexpression of efflux pumps but no carbapenemase production (Hong et al., 2013; Jean et al., 2021). Despite not being validated by several randomized clinical studies, ceftazidime-avibactam (Sader et al., 2017b; Kuo et al., 2021), cefepime-zidebactam (Sader et al., 2017a; Kuo et al., 2021; Jean et al., 2022), cefiderocol (Hsueh et al., 2019; Yamano, 2019; Bassetti et al., 2021; Liu et al., 2021), imipenem-relebactam (showing excellent in vitro activity relative to imipenem solely against OprD-losing P. aeruginosa isolates with Pseudomonas-derived cephalosporinase hyper-production) (Tselepis et al., 2020; Kuo et al., 2021), meropenem-vaborbactam (Novelli et al., 2020; Kuo et al., 2021), meropenem-nacubactam (Asempa et al., 2020), and cefepime-taniborbactam (Wang X. et al., 2020) are considered as promising alternatives against infections caused by CR- or MDR-P. aeruginosa isolates. Although aztreonam-avibactam has excellent in vitro potential to inhibit CPE (including producers of MBL; MIC90 ≤ 8 mg/L) (Karlowsky et al., 2017; Wang Y. et al., 2020), relatively high MIC90 levels of this novel antibiotic were observed against the global overall and MBL-positive P. aeruginosa isolates tested (32 mg/L and 32 mg/L, respectively) (Karlowsky et al., 2017).
Conventional and Novel Antibiotics Against CR-A. Baumannii
A 3-h intravenous infusion of 2 g meropenem every 8 h produced a high percentage (72.89 ± 22.40%) of time above the serum concentration of 8 mg/L after the third dose (Jaruratanasirikul et al., 2005). This regimen provides therapeutic benefits for the treatment of VAP caused by CR-A. baumannii isolates. Unfortunately, a high in vitro resistance to meropenem (MIC > 64 mg/L) was exhibited by most XDR/CR-A. baumannii and CRE isolates, whose susceptibility was not restored by sulbactam addition (Hsueh et al., 2002). Because of the extremely high likelihood of resistance to the majority of antibiotics, various regimens of antibiotic combinations have been proposed for the treatment of XDR-A. baumannii in the PubMed database. For instance, Jean et al. demonstrated that a prolonged intravenous infusion (> 3 h) of imipenem combined with tigecycline provided a significantly better survival rate for patients with XDR-A. baumannii bacteremic VAP than imipenem plus sulbactam (64.3% vs 14.3%) (Jean et al., 2016). Time-kill kinetic analysis proved that the former regimen inhibited the in vitro growth of XDR-A. baumannii (Poulikakos et al., 2014). In addition, treatment with a colistin-carbapenem (doripenem) regimen also significantly improved the 28-day survival rates among solid-organ transplant recipients with various XDR-A. baumannii infections (OR: 7.88; 95% CI: 1.60 – 38.76; P = 0.01) (Shields et al., 2012). In partial similarity to the meta-analysis conducted by Kengkla et al., who substituted tigecycline for carbapenem (Kengkla et al., 2018), Pongpech et al. suggested that the triple combination of meropenem, colistin, and sulbactam was a good regimen in vitro against carbapenem-NS A. baumannii complex that did not harbor genes encoding class B carbapenemase (Pongpech et al., 2010). Furthermore, to effectively treat severe VAP or BSI caused by MDR or XDR-A. baumannii complex, Piperaki et al. suggested a combination regimen comprising two active in vitro agents. Antibiotic options include high-dose ampicillin-sulbactam, high-dose tigecycline (or minocycline), and polymyxin or aminoglycoside. If two in vitro active agents are not available, in vitro synergy studies are valuable in choosing the most appropriate targeted combination scheme (Piperaki et al., 2019). Novel antibiotics, including ceftolozane-tazobactam, imipenem-relebactam, meropenem-vaborbactam, ceftazidime-avibactam, aztreonam-avibactam, cefepime-enmetazobactam, and cefepime-zidebactam, have significantly poor in vitro activity against XDR-A. baumannii complex, while exhibiting excellent in vitro efficacy against CRE or CR-P. aeruginosa (Biedenbach et al., 2015; Livermore et al., 2017; Sader et al., 2017a; Groft et al., 2021; Jean et al., 2021; Liu et al., 2021; Zhang et al., 2021a). In contrast, cefiderocol, a novel siderophore modified from ceftazidime, exhibited excellent in vitro activity against several carbapenem-NS GNB species, including A. baumannii complex (Hsueh et al., 2019); however, compared to the best available therapy, higher all-cause mortality rates were observed during the early hospitalization course when cefiderocol was administered for the treatment of patients with nosocomial pneumonia and BSI caused by CR-Acinetobacter species (Bassetti et al., 2021). The combination of sulbactam with durlobactam has been shown to effectively inhibit CR-Acinetobacter species (Shapiro et al., 2021). In addition to cefiderocol (Sader et al., 2017b) and eravacycline (a novel fluorocycline agent of the tetracycline family) (Jean et al., 2019; Jean et al., 2022), several novel drugs and new combination regimens, including TP-6076 (a fully synthetic fluorocycline antibiotic under development), WCK 4234 (a structural DBO β-lactamase inhibitor) with meropenem, LN-1-255 (a β-lactamase inhibitor modified from the penicillanic acid sulfone), taniborbactam, SPR741 (a cationic peptide derived from polymyxin B as an antibiotic adjuvant), and phage therapy (mostly applied in animal models for the treatment of infections caused by A. baumannii complex and P. aeruginosa) (Pires et al., 2015; Yin et al., 2017), etc., have been investigated to evaluate their feasibility for clinical use and potential efficacy against troublesome CR/XDR-A. baumannii isolates (Isler et al., 2018).
Table 4 compares the spectra of novel antibiotics against carbapenemase-producing GNB. Additionally, Table 5 presents the spectra of important carbapenemase inhibitors against various plasmid-mediated carbapenemases in GNB.
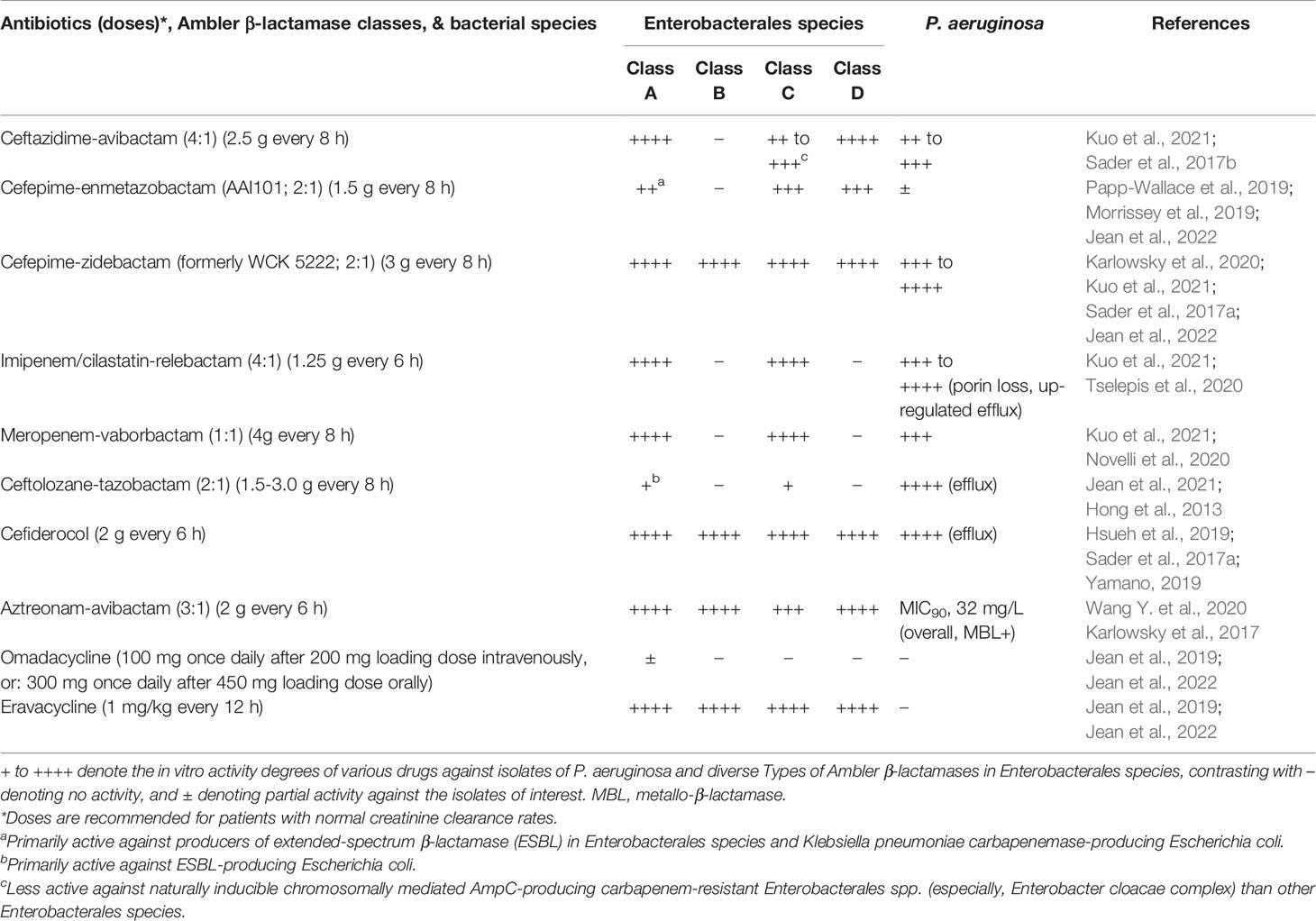
Table 4 Comparison of spectra among novel antibiotics against carbapenem-resistant Gram-negative bacteria (Enterobacterales species, and Pseudomonas aeruginosa).
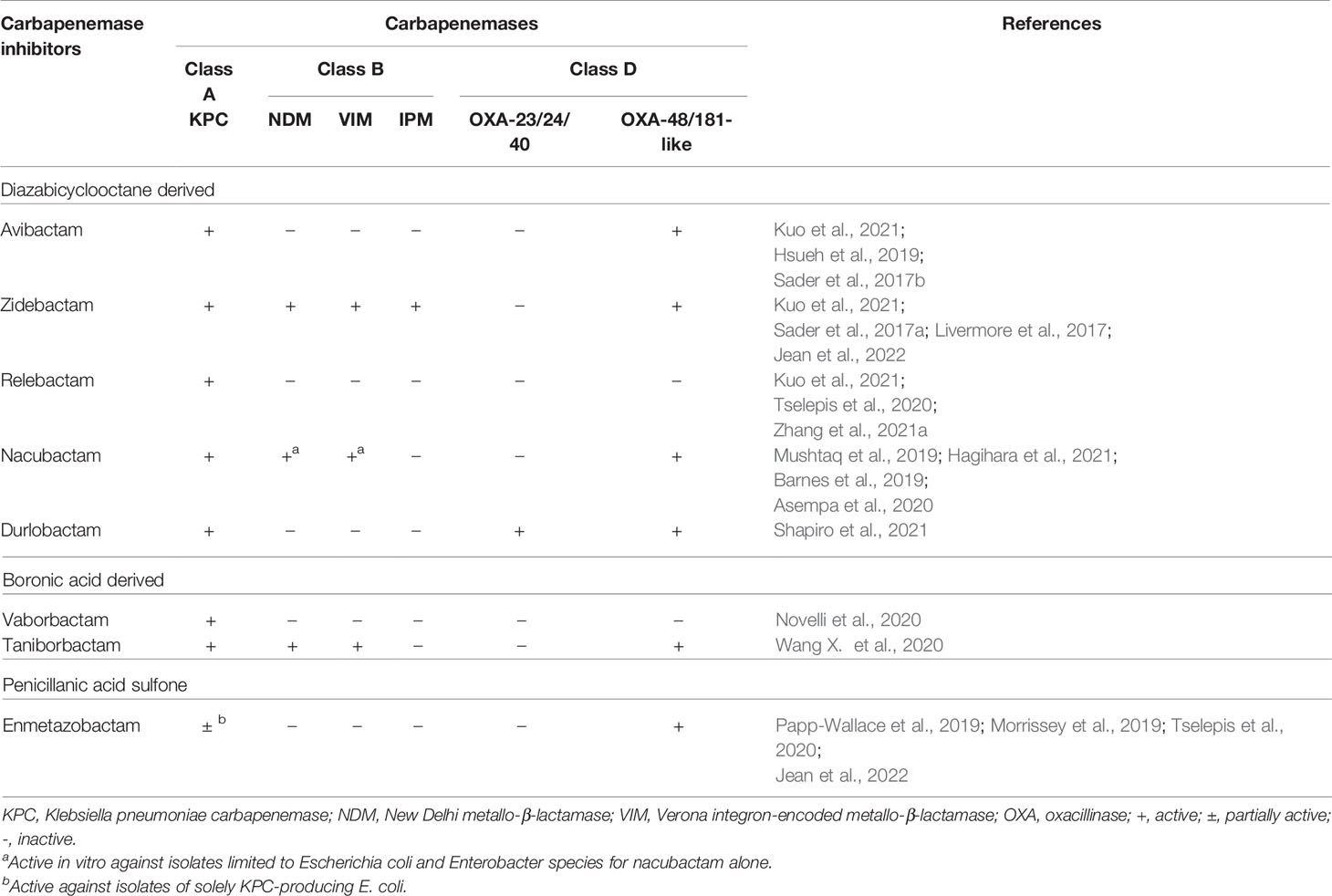
Table 5 Spectra of important carbapenemase inhibitors against various carbapenemases on Gram-negative bacteria.
Summary
In the present MDR/XDR-GNB era, we have encountered an antibiotic pipeline scenario. Although a few novel antibiotics have been effective in vitro against several CR-GNB, their clinical efficacy requires further validation. The judicious prescription of these valuable antibiotics, strict implementation of antibiotic stewardship policy, adequate disinfection of equipment and environment of hospital and LTCF settings, in combination with in-time screening to initiate necessary cohort isolation, are a few measures that must be vigorously undertaken to lessen the rapidly worsening trends of resistance in clinically important GNBs.
Author Contributions
S-SJ and P-RH collected and analyzed the data. S-SJ and DH participated in writing the manuscript. S-SJ, DH, and P-RH read and approved the final version of the manuscript. All authors contributed to the manuscript and approved the submitted version.
Conflict of Interest
The authors declare that the research was conducted in the absence of any commercial or financial relationships that could be construed as a potential conflict of interest.
Publisher’s Note
All claims expressed in this article are solely those of the authors and do not necessarily represent those of their affiliated organizations, or those of the publisher, the editors and the reviewers. Any product that may be evaluated in this article, or claim that may be made by its manufacturer, is not guaranteed or endorsed by the publisher.
References
Abdellatif, S., Trifi, A., Daly, F., Mahjoub, K., Nasri, R., Lakhal, S. B. (2016). Efficacy and Toxicity of Aerosolised Colistin in Ventilator-Associated Pneumonia: A Prospective, Randomised Trial. Ann. Intensive Care 6, 26. doi: 10.1186/s13613-016-0127-7
Ackley, R., Roshdy, D., Meredith, J., Minor, S., Anderson, W. E., Capraro, G. A., et al. (2020). Meropenem-Vaborbactam Versus Ceftazidime-Avibactam for Treatment of Carbapenem-Resistant Enterobacteriaceae Infections. Antimicrob. Agents Chemother. 64, e02313-19. doi: 10.1128/AAC.02313-19
Alraddadi, B. M., Saeedi, M., Qutub, M., Alshukairi, A., Hassanien, A., Wali, G. (2019). Efficacy of Ceftazidime-Avibactam in the Treatment of Infections Due to Carbapenem-Resistant Enterobacteriaceae. BMC. Infect. Dis. 19, 772. doi: 10.1186/s12879-019-4409-1
Alrowais, H., McElheny, C. L., Spychala, C. N., Sastry, S., Guo, Q., Butt, A. A., et al. (2015). Fosfomycin Resistance in Escherichia Coli, Pennsylvania, USA. Emerg. Infect. Dis. 21, 2045–2047. doi: 10.3201/eid2111.150750
Amladi, A. U., Abirami, B., Devi, S. M., Sudarsanam, T. D., Kandasamy, S., Kekre, N., et al. (2019). Susceptibility Profile, Resistance Mechanisms & Efficacy Ratios of Fosfomycin, Nitrofurantoin & Colistin for Carbapenem-Resistant Enterobacteriaceae Causing Urinary Tract Infections. Indian J. Med. Res. 149, 185–191. doi: 10.4103/ijmr.IJMR_2086_17
Asempa, T. E., Motos, A., Abdelraouf, K., Bissantz, C., Zampaloni, C., Nicolau, D. P. (2020). Meropenem-Nacubactam Activity Against AmpC-Overproducing and KPC-Expressing Pseudomonas Aeruginosa in a Neutropenic Murine Lung Infection Model. Int. J. Antimicrob. Agents 55, 105838. doi: 10.1016/j.ijantimicag.2019.10.019
Avison, M. B., Higgins, C. S., von Heldreich, C. J., Bennett, P. M., Walsh, T. R. (2001). Plasmid Location and Molecular Heterogeneity of the L1 and L2 Beta-Lactamase Genes of Stenotrophomonas Maltophilia. Antimicrob. Agents Chemother. 45, 413–419. doi: 10.1128/AAC.45.2.413-419.2001
Barlam, T. F., Cosgrove, S. E., Abbo, L. M., MacDougall, C., Schuetz, A. N., Septimus, E. J., et al. (2016). Implementing an Antibiotic Stewardship Program: Guidelines by the Infectious Diseases Society of America and the Society for Healthcare Epidemiology of America. Clin. Infect. Dis. 62, e51–e77. doi: 10.1093/cid/ciw118
Barnes, M. D., Taracila, M. A., Good, C. E., Bajaksouzian, S., Rojas, L. J., van Duin, D., et al. (2019). Nacubactam Enhances Meropenem Activity Against Carbapenem-Resistant Klebsiella Pneumoniae Producing KPC. Antimicrob. Agents Chemother. 63, e00432-19. doi: 10.1128/AAC.00432-19
Bartsch, S. M., McKinnell, J. A., Mueller, L. E., Miller, L. G., Gohil, S. K., Huang, S. S., et al. (2017). Potential Economic Burden of Carbapenem-Resistant Enterobacteriaceae (CRE) in the United States. Clin. Microbiol. Infect. 23, 48.e9–48.e16. doi: 10.1016/j.cmi.2016.09.003
Bassetti, M., Echols, R., Matsunaga, Y., Ariyasu, M., Doi, Y., Ferrer, R., et al. (2021). Efficacy and Safety of Cefiderocol or Best Available Therapy for the Treatment of Serious Infections Caused by Carbapenem-Resistant Gram-Negative Bacteria (CREDIBLE-CR): A Randomised, Open-Label, Multicentre, Pathogen-Focused, Descriptive, Phase 3 Trial. Lancet Infect. Dis. 21, 226–240. doi: 10.1016/S1473-3099(20)30796-9
Bassetti, M., Peghin, M., Vena, A., Giacobbe, D. R. (2019). Treatment of Infections Due to MDR Gram-Negative Bacteria. Front. Med. (Lausanne) 6, 74. doi: 10.3389/fmed.2019.00074
Ben-David, D., Masarwa, S., Navon-Venezia, S., Mishali, H., Fridental, I., Rubinovitch, B., et al. (2011). Carbapenem-Resistant Klebsiella Pneumoniae in Post-Acute-Care Facilities in Israel. Infect. Control Hosp. Epidemiol. 32, 845–853. doi: 10.1086/661279
Benzerara, Y., Gallah, S., Hommeril, B., Genel, N., Decré, D., Rottman, M., et al. (2017). Emergence of Plasmid-Mediated Fosfomycin-Resistance Genes Among Escherichia Coli Isolates, France. Emerg. Infect. Dis. 23, 1564–1567. doi: 10.3201/eid2309.170560
Bhargava, A., Hayakawa, K., Silverman, E., Haider, S., Alluri, K. C., Datla, S., et al. (2014). Risk Factors for Colonization Due to Carbapenem-Resistant Enterobacteriaceae Among Patients Exposed to Long-Term Acute Care and Acute Care Facilities. Infect. Control Hosp. Epidemiol. 35, 398–405. doi: 10.1086/675614
Biedenbach, D. J., Kazmierczak, K., Bouchillon, S. K., Sahm, D. F., Bradford, P. A. (2015). In Vitro Activity of Aztreonam-Avibactam Against a Global Collection of Gram-Negative Pathogens From 2012 and 2013. Antimicrob. Agents Chemother. 59, 4239–4248. doi: 10.1128/AAC.00206-15
Borer, A., Saidel-Odes, L., Eskira, S., Nativ, R., Riesenberg, K., Livshiz-Riven, I., et al. (2012). Risk Factors for Developing Clinical Infection With Carbapenem-Resistant Klebsiella Pneumoniae in Hospital Patients Initially Only Colonized With Carbapenem-Resistant K. Pneumoniae. Am. J. Infect. Control 40, 421–425. doi: 10.1016/j.ajic.2011.05.022
Borer, A., Saidel-Odes, L., Riesenberg, K., Eskira, S., Peled, N., Nativ, R., et al. (2009). Attributable Mortality Rate for Carbapenem-Resistant Klebsiella Pneumoniae Bacteremia. Infect. Control Hosp. Epidemiol. 30, 972–976. doi: 10.1086/605922
Boyd, N., Nailor, M. D. (2011). Combination Antibiotic Therapy for Empiric and Definitive Treatment of Gram-Negative Infections: Insights From the Society of Infectious Diseases Pharmacists. Pharmacotherapy 31, 1073–1084. doi: 10.1592/phco.31.11.1073
Brennan, B. M., Coyle, J. R., Marchaim, D., Pogue, J. M., Boehme, M., Finks, J., et al. (2014). Statewide Surveillance of Carbapenem-Resistant Enterobacteriaceae in Michigan. Infect. Control Hosp. Epidemiol. 35, 342–349. doi: 10.1086/675611
Brooke, J. S. (2012). Stenotrophomonas Maltophilia: An Emerging Global Opportunistic Pathogen. Clin. Microbiol. Rev. 25, 2–41. doi: 10.1128/CMR.00019-11
Bulik, C. C., Nicolau, D. P. (2011). Double-Carbapenem Therapy for Carbapenemase-Producing Klebsiella Pneumoniae. Antimicrob. Agents Chemother. 55, 3002–3004. doi: 10.1128/AAC.01420-10
Capone, A., Giannella, M., Fortini, D., Giordano, A., Meledandri, M., Ballardini, M., et al. (2013). High Rate of Colistin Resistance Among Patients With Carbapenem-Resistant Klebsiella Pneumoniae Infection Accounts for an Excess of Mortality. Clin. Microbiol. Infect. 19, E23–E30. doi: 10.1111/1469-0691.12070
Chang, Y., Luan, G., Xu, Y., Wang, Y., Shen, M., Zhang, C., et al. (2015). Characterization of Carbapenem-Resistant Acinetobacter Baumannii Isolates in a Chinese Teaching Hospital. Front. Microbiol. 6, 910. doi: 10.3389/fmicb.2015.00910
Chen, T. L., Chang, W. C., Kuo, S. C., Lee, Y. T., Chen, C. P., Siu, L. K., et al. (2010). Contribution of a Plasmid-Borne blaOXA-58 Gene With its Hybrid Promoter Provided by IS1006 and an ISAba3-Like Element to β-Lactam Resistance in Acinetobacter Genomic Species 13TU. Antimicrob. Agents Chemother. 54, 3107–3112. doi: 10.1128/AAC.00128-10
Cheng, A., Chuang, Y. C., Sun, H. Y., Sheng, W. H., Yang, C. J., Liao, C. H., et al. (2015). Excess Mortality Associated With Colistin-Tigecycline Compared With Colistin-Carbapenem Combination Therapy for Extensively Drug-Resistant Acinetobacter Baumannii Bacteremia: A Multicenter Prospective Observational Study. Crit. Care Med. 43, 1194–1204. doi: 10.1097/CCM.0000000000000933
Chen, H. Y., Jean, S. S., Lee, Y. L., Lu, M. C., Ko, W. C., Liu, P. Y., et al. (2021a). Carbapenem-Resistant Enterobacterales in Long-Term Care Facilities: A Global and Narrative Review. Front. Cell. Infect. Microbiol. 11, 601968. doi: 10.3389/fcimb.2021.601968
Chen, C. C., Lai, C. C., Huang, H. L., Su, Y. T., Chiu, Y. H., Toh, H. S., et al. (2021b). Antimicrobial Ability and Mechanism Analysis of Lactobacillus Species Against Carbapenemase-Producing Enterobacteriaceae. J. Microbiol. Immunol. Infect. 54, 447–456. doi: 10.1016/j.jmii.2020.01.005
Chitnis, A. S., Caruthers, P. S., Rao, A. K., Lamb, J., Lurvey, R., Beau De Rochars, V., et al. (2012). Outbreak of Carbapenem-Resistant Enterobacteriaceae at a Long-Term Acute Care Hospital: Sustained Reductions in Transmission Through Active Surveillance and Targeted Interventions. Infect. Control Hosp. Epidemiol. 33, 984–992. doi: 10.1086/667738
Chopra, T. C., Rivard, C., Awali, R. A., Krishna, A., Bonomo, R. A., Perez, F., et al. (2018). Epidemiology of Carbapenem-Resistant Enterobacteriaceae at a Long-Term Acute Care Hospital. Open Forum. Infect. Dis. 5, ofy224. doi: 10.1093/ofid/ofy224
Ciofi Degli Atti, M., Bernaschi, P., Carletti, M., Luzzi, I., García-Fernández, A., Bertaina, A., et al. (2014). An Outbreak of Extremely Drug-Resistant Pseudomonas Aeruginosa in a Tertiary Care Pediatric Hospital in Italy. BMC. Infect. Dis. 14, 494. doi: 10.1186/1471-2334-14-494
Clinical and Laboratory Standards Institute (CLSI) (2021). “Performance Standards for Antimicrobial Susceptibility Testing: 31st Informational Supplement,” in CLSI Document M100-S31 (Wayne, PA, USA: CLSI).
Cortivo, G. D., Gutberlet, A., Ferreira, J. A., Ferreira, L. E., Deglmann, R. C., Westphal, G. A., et al. (2015). Antimicrobial Resistance Profiles and Oxacillinase Genes in Carbapenem-Resistant Acinetobacter Baumannii Isolated From Hospitalized Patients in Santa Catarina. Brazil Rev. Soc Bras. Med. Trop. 48, 699–705. doi: 10.1590/0037-8682-0233-2015
Cunha, C. B., Kassakian, S. Z., Chan, R., Tenover, F. C., Ziakas, P., Chapin, K. C., et al. (2016). Screening of Nursing Home Residents for Colonization With Carbapenem-Resistant Enterobacteriaceae Admitted to Acute Care Hospitals: Incidence and Risk Factors. Am. J. Infect. Control 44, 126–130. doi: 10.1016/j.ajic.2015.09.019
Daikos, G. L., Tsaousi, S., Tzouvelekis, L. S., Anyfantis, I., Psichogiou, M., Argyropoulou, A., et al. (2014). Carbapenemase-Producing Klebsiella Pneumoniae Bloodstream Infections: Lowering Mortality by Antibiotic Combination Schemes and the Role of Carbapenems. Antimicrob. Agents Chemother. 58, 2322–2328. doi: 10.1128/AAC.02166-13
Da Silva, G. J., Mendonça, N., Batista, G., Duarte, A. (2010). Sequence Types of Portuguese Carbapenem-Resistant Acinetobacter Baumannii Isolates Collected Over 10 Years. J. Antimicrob. Chemother. 65, 2254–2256. doi: 10.1093/jac/dkq274
Davoudi-Monfared, E., Khalili, H. (2018). The Threat of Carbapenem-Resistant Gram-Negative Bacteria in a Middle East Region. Infect. Drug Resist. 11, 1831–1880. doi: 10.2147/IDR.S176049
De Man, T. J. B., Yaffee, A. Q., Zhu, W., Batra, D., Alyanak, E., Rowe, L. A., et al. (2021). Multispecies Outbreak of Verona Integron-Encoded Metallo-β-Lactamase-Producing Multidrug Resistant Bacteria Driven by a Promiscuous Incompatibility Group A/C2 Plasmid. Clin. Infect. Dis. 72, 414–420. doi: 10.1093/cid/ciaa049
De Pascale, G., Martucci, G., Montini, L., Panarello, G., Cutuli, S. L., Di Carlo, D., et al. (2017). Double Carbapenem as a Rescue Strategy for the Treatment of Severe Carbapenemase-Producing Klebsiella Pneumoniae Infections: A Two-Center, Matched Case-Control Study. Crit. Care 21, 173. doi: 10.1186/s13054-017-1769-z
Dortet, L., Poirel, L., Errera, C., Nordmann, P. (2014). CarbAcineto NP Test for Rapid Detection of Carbapenemase-Producing Acinetobacter Spp. J. Clin. Microbiol. 52, 2359–2364. doi: 10.1128/JCM.00594-14
Duan, Z., Qin, J., Liu, Y., Li, C., Ying, C. (2020). Molecular Epidemiology and Risk Factors of Stenotrophomonas Maltophilia Infections in a Chinese Teaching Hospital. BMC. Microbiol. 20, 294. doi: 10.1186/s12866-020-01985-3
Dubendris, H., MacFarquhar, J., Kornegay, R., Gable, P., Boyd, S., Walters, M., et al. (2020). Imipenemase-Producing Carbapenem-Resistant Enterobacteriaceae Transmission in a Long-Term-Care Facility During a Community-Wide Multidrug Resistant Organism Outbreak-North Carolina 2017. Am. J. Infect. Control 48, 320–323. doi: 10.1016/j.ajic.2019.05.022
Elabd, F. M., Al-Ayed, M. S. Z., Asaad, A. M., Alsareii, S. A., Qureshi, M. A., Musa, H. A. (2015). Molecular Characterization of Oxacillinases Among Carbapenem-Resistant Acinetobacter Baumannii Nosocomial Isolates in a Saudi Hospital. J. Infect. Public Health 8, 242–247. doi: 10.1016/j.jiph.2014.10.002
Erdem, I., Kaynar-Tascioglu, J., Kaya, B., Goktas, P. (2002). The Comparison of in the Vitro Effect of Imipenem or Meropenem Combined With Ciprofloxacin or Levofloxacin Against Multidrug-Resistant Pseudomonas Aeruginosa Strains. Int. J. Antimicrob. Agents 20, 384–386. doi: 10.1016/s0924-8579(02)00192-9
Ezadi, F., Jamali, A., Heidari, A., Javid, N., Ardebili, A. (2020). Heteroresistance to Colistin in Oxacillinase-Producing Carbapenem-Resistant Acinetobacter Baumannii Clinical Isolates From Gorgan, Northern Iran. J. Glob. Antimicrob. Resist. 21, 380–385. doi: 10.1016/j.jgar.2019.11.010
Fadhli, A. H. A., Jamal, W. Y., Rotimi, V. O. (2020). Prevalence of Carbapenem-Resistant Enterobacteriaceae and Emergence of High Rectal Colonization Rates of blaOXA-181-Positive Isolates in Patients Admitted to Two Major Hospital Intensive Care Units in Kuwait. PLoS One 15, e0241971. doi: 10.1371/journal.pone.0241971
Falagas, M. E., Kastoris, A. C., Vouloumanou, E. K., Rafailidis, P. I., Kapaskelis, A. M., Dimopoulos, G. (2009). Attributable Mortality of Stenotrophomonas Maltophilia Infections: A Systematic Review of the Literature. Future Microbiol. 4, 1103–1109. doi: 10.2217/fmb.09.84
Garonzik, S. M., Li, J., Thamlikitkul, V., Paterson, D. L., Shoham, S., Jacob, J., et al. (2011). Population Pharmacokinetics of Colistin Methanesulfonate and Formed Colistin in Critically Ill Patients From a Multicenter Study Provide Dosing Suggestions for Various Categories of Patients. Antimicrob. Agents Chemother. 55, 3284–3294. doi: 10.1128/AAC.01733-10
Giakkoupi, P., Pappa, O., Polemis, M., Vatopoulos, A. C., Miriagou, V., Zioga, A., et al. (2009). Emerging Klebsiella Pneumoniae Isolates Coproducing KPC-2 and VIM-1 Carbapenemases. Antimicrob. Agents Chemother. 53, 4048–4050. doi: 10.1128/AAC.00690-09
Giufrè, M., Ricchizzi, E., Accogli, M., Barbanti, F., Monaco, M., de Araujo, F. P., et al. (2017). Colonization by Multidrug-Resistant Organisms in Long-Term Care Facilities in Italy: A Point-Prevalence Study. Clin. Microbiol. Infect. 23, 961–967. doi: 10.1016/j.cmi.2017.04.006
Goodman, K. E., Simner, P. J., Tamma, P. D., Milstone, A. M. (2016). Infection Control Implications of Heterogeneous Resistance Mechanisms in Carbapenem-Resistant Enterobacteriaceae (CRE). Expert. Rev. Anti Infect. Ther. 14, 95–108. doi: 10.1586/14787210.2016.1106940
Groft, L. M., Claeys, K. C., Heil, E. L. (2021). An Evaluation of Meropenem/Vaborbactam for the Treatment of Nosocomial Pneumonia. Expert. Opin. Pharmacother. 22, 265–271. doi: 10.1080/14656566.2020.1840552
Gupta, N., Limbago, B. M., Patel, J. B., Kallen, A. J. (2011). Carbapenem-Resistant Enterobacteriaceae: Epidemiology and Prevention. Clin. Infect. Dis. 53, 60–67. doi: 10.1093/cid/cir202
Gutiérrez-Gutiérrez, B., Salamanca, E., de Cueto, M., Hsueh, P. R., Viale, P., Paño-Pardo, J. R., et al. (2016). A Predictive Model of Mortality in Patients With Bloodstream Infections Due to Carbapenemase-Producing Enterobacteriaceae. Mayo. Clin. Proc. 91, 1362–1371. doi: 10.1016/j.mayocp.2016.06.024
Gutiérrez-Gutiérrez, B., Salamanca, E., de Cueto, M., Hsueh, P. R., Viale, P., Paño-Pardo, J. R., et al. (2017). Effect of Appropriate Combination Therapy on Mortality of Patients With Bloodstream Infections Due to Carbapenemase-Producing Enterobacteriaceae (INCREMENT): A Retrospective Cohort Study. Lancet Infect. Dis. 17, 726–734. doi: 10.1016/S1473-3099(17)30228-1
Hagihara, M., Kato, H., Sugano, T., Okade, H., Sato, N., Shibata, Y., et al. (2021). Pharmacodynamic Evaluation of Meropenem, Cefepime, or Aztreonam Combined With a Novel β-Lactamase Inhibitor, Nacubactam, Against Carbapenem-Resistant and/or Carbapenemase-Producing Klebsiella Pneumoniae and Escherichia Coli Using a Murine Thigh-Infection Model. Int. J. Antimicrob. Agents 57, 106330. doi: 10.1016/j.ijantimicag.2021.106330
Hagiya, H., Yamamoto, N., Kawahara, R., Akeda, Y., Shanmugakani, R. K., Ueda, A., et al. (2018). Risk Factors for Fecal Carriage of IMP-6-Producing Enterobacteriaceae at a Long-Term Care Hospital in Japan: A Follow-Up Report From the Northern Osaka Multicentre Study Group. J. Infect. Chemother. 24, 769–772. doi: 10.1016/j.jiac.2018.03.009
Hayakawa, K., Nakano, R., Hase, R., Shimatani, M., Kato, H., Hasumi, J., et al. (2020). Comparison Between IMP Carbapenemase-Producing Enterobacteriaceae and non-Carbapenemase-Producing Enterobacteriaceae: A Multicentre Prospective Study of the Clinical and Molecular Epidemiology of Carbapenem-Resistant Enterobacteriaceae. J. Antimicrob. Chemother. 75, 697–708. doi: 10.1093/jac/dkz501
Hellyer, T. P., Ewan, V., Wilson, P., Simpson, A. J. (2016). The Intensive Care Society Recommended Bundle of Interventions for the Prevention of Ventilator-Associated Pneumonia. J. Intensive Care Soc 17, 238–243. doi: 10.1177/1751143716644461
Hirsch, E. B., Tam, V. H. (2010a). Detection and Treatment Options for Klebsiella Pneumoniae Carbapenemases (KPCs): An Emerging Cause of Multidrug-Resistant Infection. J. Antimicrob. Chemother. 65, 1119–1125. doi: 10.1093/jac/dkq108
Hirsch, E. B., Tam, V. H. (2010b). Impact of Multidrug-Resistant Pseudomonas Aeruginosa Infection on Patient Outcomes. Expert. Rev. Pharmacoecon. Outcomes Res. 10, 441–451. doi: 10.1586/erp.10.49
Hong, M. C., Hsu, D. I., Bounthavong, M. (2013). Ceftolozane/tazobactam: A Novel Antipseudomonal Cephalosporin and β-Lactamase-Inhibitor Combination. Infect. Drug Resist. 6, 215–223. doi: 10.2147/IDR.S36140
Hsueh, S. C., Lee, Y. J., Huang, Y. T., Liao, C. H., Tsuji, M., Hsueh, P. R. (2019). In Vitro Activities of Cefiderocol, Ceftolozane/Tazobactam, Ceftazidime/Avibactam and Other Comparative Drugs Against Imipenem-Resistant Pseudomonas Aeruginosa and Acinetobacter Baumannii, and Stenotrophomonas Maltophilia, All Associated With Bloodstream Infections in Taiwan. J. Antimicrob. Chemother. 74, 380–386. doi: 10.1093/jac/dky425
Hsueh, P. R., Teng, L. J., Chen, C. Y., Chen, W. H., Yu, C. J., Ho, S. W., et al. (2002). Pandrug-Resistant Acinetobacter Baumannii Causing Nosocomial Infections in a University Hospital, Taiwan. Emerg. Infect. Dis. 8, 827–832. doi: 10.3201/eid0805.020014
Huang, G., Yin, S., Gong, Y., Zhao, X., Zou, L., Bei Jiang, B., et al. (2016). Multilocus Sequence Typing Analysis of Carbapenem-Resistant Acinetobacter Baumannii in a Chinese Burns Institute. Front. Microbiol. 7, 1717. doi: 10.3389/fmicb.2016.01717
Hu, H., Mao, J., Chen, Y., Wang, J., Zhang, P., Jiang, Y., et al. (2020). Clinical and Microbiological Characteristics of Community-Onset Carbapenem-Resistant Enterobacteriaceae Isolates. Infect. Drug Resist. 13, 3131–3143. doi: 10.2147/IDR.S260804
Isler, B., Doi, Y., Bonomo, R. A., Paterson, D. L. (2018). New Treatment Options Against Carbapenem-Resistant Acinetobacter Baumannii Infections. Antimicrob. Agents Chemother. 63, e01110-18. doi: 10.1128/AAC.01110-18
Jaruratanasirikul, S., Sriwiriyajan, S., Punyo, J. (2005). Comparison of the Pharmacodynamics of Meropenem in Patients With Ventilator-Associated Pneumonia Following Administration by 3-Hour Infusion or Bolus Injection. Antimicrob. Agents Chemother. 49, 1337–1339. doi: 10.1128/AAC.49.4.1337-1339.2005
Jean, S. S., Chang, Y. C., Lin, W. C., Lee, W. S., Hsueh, P. R., Hsu, C. W. (2020). Epidemiology, Treatment, and Prevention of Nosocomial Bacterial Pneumonia. J. Clin. Med. 9, 275. doi: 10.3390/jcm9010275
Jean, S. S., Gould, I. M., Lee, W. S., Hsueh, P. R. (2019). New Drugs for Multidrug-Resistant Gram-Negative Organisms: Time for Stewardship. Drugs 79, 705–714. doi: 10.1007/s40265-019-01112-1
Jean, S. S., Hsieh, T. C., Hsu, C. W., Lee, W. S., Bai, K. J., Lam, C. (2016). Comparison of the Clinical Efficacy Between Tigecycline Plus Extended-Infusion Imipenem and Sulbactam Plus Imipenem Against Ventilator-Associated Pneumonia With Pneumonic Extensively Drug-Resistant Acinetobacter Baumannii Bacteremia, and Correlation of Clinical Efficacy With In Vitro Synergy Tests. J. Microbiol. Immunol. Infect. 49, 924–933. doi: 10.1016/j.jmii.2015.06.009
Jean, S. S., Hsueh, P. R. (2014). Spread of Klebsiella Pneumoniae Carbapenemase-2-Producing Klebsiella Pneumoniae Clones in Asia. Future Microbiol. 9, 273–275. doi: 10.2217/fmb.13.157
Jean, S. S., Hsueh, P. R., Lee, W. S., Yu, K. W., Liao, C. H., Chang, F. Y., et al. (2013). Carbapenem Susceptibilities and non-Susceptibility Concordance to Different Carbapenems Amongst Clinically Important Gram-Negative Bacteria Isolated From Intensive Care Units in Taiwan: Results From the Surveillance of Multicentre Antimicrobial Resistance in Taiwan (SMART) in 2009. Int. J. Antimicrob. Agents 41, 457–462. doi: 10.1016/j.ijantimicag.2013.02.001
Jean, S. S., Ko, W. C., Lu, M. C., Lee, W. S., Hsueh, P. R. (2022). Multicenter Surveillance of In Vitro Activities of Cefepime-Zidebactam, Cefepime-Enmetazobactam, Omadacycline, Eravacycline, and Comparator Antibiotics Against Enterobacterales, Pseudomonas Aeruginosa, and Acinetobacter Baumannii Complex Causing Bloodstream Infection in Taiwan 2020. Expert. Rev. Anti Infect. Ther. 1–13. doi: 10.1080/14787210.2022.2021876
Jean, S. S., Lee, W. S., Lam, C., Hsu, C. W., Chen, R. J., Hsueh, P. R. (2015). Carbapenemase-Producing Gram-Negative Bacteria: Current Epidemics, Antimicrobial Susceptibility and Treatment Options. Future Microbiol. 10, 407–425. doi: 10.2217/fmb.14.135
Jean, S. S., Lee, Y. L., Liu, P. Y., Lu, M. C., Ko, W. C., Hsueh, P. R. (2021). Multicenter Surveillance of Antimicrobial Susceptibilities and Resistance Mechanisms Among Enterobacterales Species and non-Fermenting Gram-Negative Bacteria From Different Infection Sources in Taiwan From 2016 to 2018. J. Microbiol. Immunol. Infect. S1684–1182(21)00182-1. doi: 10.1016/j.jmii.2021.07.015
Jean, S. S., Lee, N. Y., Tang, H. J., Lu, M. C., Ko, W. C., Hsueh, P. R. (2018b). Carbapenem-Resistant Enterobacteriaceae Infections: Taiwan Aspects. Front. Microbiol. 9, 2888. doi: 10.3389/fmicb.2018.02888
Jean, S. S., Lu, M. C., Shi, Z. Y., Tseng, S. H., Wu, T. S., Lu, P. L., et al. (2018a). In Vitro Activity of Ceftazidime-Avibactam, Ceftolozane-Tazobactam, and Other Comparable Agents Against Clinically Important Gram-Negative Bacilli: Results From the 2017 Surveillance of Multicenter Antimicrobial Resistance in Taiwan (SMART). Infect. Drug Resist. 11, 1983–1992. doi: 10.2147/IDR.S175679
Jefferies, J. M. C., Cooper, T., Yam, T., Clarke, S. C. (2012). Pseudomonas Aeruginosa Outbreaks in the Neonatal Intensive Care Unit–a Systematic Review of Risk Factors and Environmental Sources. J. Med. Microbiol. 61, 1052–1061. doi: 10.1099/jmm.0.044818-0
Jernigan, M. G., Press, E. G., Nguyen, M. H., Clancy, C. J., Shields, R. K. (2012). The Combination of Doripenem and Colistin is Bactericidal and Synergistic Against Colistin-Resistant, Carbapenemase-Producing Klebsiella Pneumoniae. Antimicrob. Agents Chemother. 56, 3395–3398. doi: 10.1128/AAC.06364-11
Jimenez, A., Trepka, M. J., Munoz-Price, L. S., Pekovic, V., Ibrahimou, B., Abbo, L. M., et al. (2020). Epidemiology of Carbapenem-Resistant Enterobacteriaceae in Hospitals of a Large Healthcare System in Miami, Florida From 2012 to 2016: Five Years of Experience With an Internal Registry. Am. J. Infect. Control 48, 1341–1347. doi: 10.1016/j.ajic.2020.04.013
Kaiser, S., Biehler, K., Jonas, D. (2009). A Stenotrophomonas Maltophilia Multilocus Sequence Typing Scheme for Inferring Population Structure. J. Bacteriol. 191, 2934–2943. doi: 10.1128/JB.00892-08
Kalpoe, J. S., Sonnenberg, E., Factor, S. H., del Rio Martin, J., Schiano, T., Patel, G., et al. (2012). Mortality Associated With Carbapenem-Resistant Klebsiella Pneumoniae Infections in Liver Transplant Recipients. Liver Transpl. 18, 468–474. doi: 10.1002/lt.23374
Kanamori, H., Parobek, C. M., Juliano, J. J., van Duin, D., Cairns, B. A., Weber, D. J., et al. (2017). A Prolonged Outbreak of KPC-3-Producing Enterobacter Cloacae and Klebsiella Pneumoniae Driven by Multiple Mechanisms of Resistance Transmission at a Large Academic Burn Center. Antimicrob. Agents Chemother. 61, e01516-16. doi: 10.1128/AAC.01516-16
Kang, C. M., Chen, X. J., Chih, C. C., Hsu, C. C., Chen, P. H., Lee, T. F., et al. (2020). Rapid Identification of Bloodstream Bacterial and Fungal Pathogens and Their Antibiotic Resistance Determinants From Positively Flagged Blood Cultures Using the BioFire FilmArray Blood Culture Identification Panel. J. Microbiol. Immunol. Infect. 53, 882–891. doi: 10.1016/j.jmii.2020.03.018
Kang, J. S., Yi, J., Ko, M. K., Lee, S. O., Lee, J. E., Kim, K. H. (2019). Prevalence and Risk Factors of Carbapenem-Resistant Enterobacteriaceae Acquisition in an Emergency Intensive Care Unit in a Tertiary Hospital in Korea: A Case-Control Study. J. Korean Med. Sci. 34, e140. doi: 10.3346/jkms.2019.34.e140
Kao, C. Y., Chen, S. S., Hung, K. H., Wu, H. M., Hsueh, P. R., Yan, J. J., et al. (2016). Overproduction of Active Efflux Pump and Variations of OprD Dominate in Imipenem-Resistant Pseudomonas Aeruginosa Isolated From Patients With Bloodstream Infections in Taiwan. BMC. Microbiol. 16, 107. doi: 10.1186/s12866-016-0719-2
Karlowsky, J. A., Hackel, M. A., Bouchillon, S. K., Sahm, D. F. (2020). In Vitro Activity of WCK 5222 (Cefepime-Zidebactam) Against Worldwide Collected Gram-Negative Bacilli Not Susceptible to Carbapenems. Antimicrob. Agents Chemother. 64, e01432-20. doi: 10.1128/AAC.01432-20
Karlowsky, J. A., Kazmierczak, K. M., de Jonge, B. L. M., Hackel, M. A., Sahm, D. F., Bradford, P. A. (2017). In Vitro Activity of Aztreonam-Avibactam Against Enterobacteriaceae and Pseudomonas Aeruginosa Isolated by Clinical Laboratories in 40 Countries From 2012 to 2015. Antimicrob. Agents Chemother. 61, e00472-17. doi: 10.1128/AAC.00472-17
Kateete, D. P., Nakanjako, R., Namugenyi, J., Erume, J., Joloba, M. L., Najjuka, C. F. (2016). Carbapenem Resistant Pseudomonas Aeruginosa and Acinetobacter Baumannii at Mulago Hospital in Kampala, Uganda, (2007-2009). Springerplus 5, 1308. doi: 10.1186/s40064-016-2986-7
Kawai, A., McElheny, C. L., Iovleva, A., Kline, E. G., Sluis-Cremer, N., Shields, R. N., et al. (2020). Structural Basis of Reduced Susceptibility to Ceftazidime-Avibactam and Cefiderocol in Enterobacter Cloacae Due to AmpC R2 Loop Deletion. Antimicrob. Agents Chemother. 64, e00198-20. doi: 10.1128/AAC.00198-20
Kelly, A. M., Mathema, B., Larson, E. L. (2017). Carbapenem-Resistant Enterobacteriaceae in the Community: A Scoping Review. Int. J. Antimicrob. Agents 50, 127–134. doi: 10.1016/j.ijantimicag.2017.03.012
Kempf, M., Rolain, J. M. (2012). Emergence of Resistance to Carbapenems in Acinetobacter Baumannii in Europe: Clinical Impact and Therapeutic Options. Int. J. Antimicrob. Agents 39, 105–114. doi: 10.1016/j.ijantimicag.2011.10.004
Kengkla, K., Kongpakwattana, K., Saokaew, S., Apisarnthanarak, A., Chaiyakunapruk, N. (2018). Comparative Efficacy and Safety of Treatment Options for MDR and XDR Acinetobacter Baumannii Infections: A Systematic Review and Network Meta-Analysis. J. Antimicrob. Chemother. 73, 22–32. doi: 10.1093/jac/dkx368
Kumarasamy, K. K., Toleman, M. A., Walsh, T. R., Bagaria, J., Butt, F., Balakrishnan, R., et al. (2010). Emergence of a New Antibiotic Resistance Mechanism in India, Pakistan, and the UK: A Molecular, Biological, and Epidemiological Study. Lancet Infect. Dis. 10, 597–602. doi: 10.1016/S1473-3099(10)70143-2
Kuo, S. C., Wang, Y. C., Tan, M. C., Huang, W. C., Shiau, Y. R., Wang, H. Y., et al. (2021). In Vitro Activity of Imipenem/Relebactam, Meropenem/Vaborbactam, Ceftazidime/Avibactam, Cefepime/Zidebactam and Other Novel Antibiotics Against Imipenem-non-Susceptible Gram-Negative Bacilli From Taiwan. J. Antimicrob. Chemother. 76, 2071–2078. doi: 10.1093/jac/dkab141
Landman, D., Babu, E., Shah, N., Kelly, P., Bäcker, M., Bratu, S., et al. (2010). Activity of a Novel Aminoglycoside, ACHN-490, Against Clinical Isolates of Escherichia Coli and Klebsiella Pneumoniae From New York City. J. Antimicrob. Chemother. 65, 2123–2127. doi: 10.1093/jac/dkq278
Lapointe-Shaw, L., Voruganti, T., Kohler, P., Thein, H.-H., Sander, B., McGeer, A. (2017). Cost-Effectiveness Analysis of Universal Screening for Carbapenemase-Producing Enterobacteriaceae in Hospital Inpatients. Eur. J. Clin. Microbiol. Infect. Dis. 36, 1047–1055. doi: 10.1007/s10096-016-2890-7
Lee, Y. C., Huang, Y. T., Tan, C. K., Kuo, Y. W., Liao, C. H., Lee, P. I., et al. (2011). Acinetobacter Baumannii and Acinetobacter Genospecies 13TU and 3 Bacteraemia: Comparison of Clinical Features, Prognostic Factors and Outcomes. J. Antimicrob. Chemother. 66, 1839–1846. doi: 10.1093/jac/dkr200
Lee, C. M., Lai, C. C., Chiang, H. T., Lu, M. C., Wang, L. F., Tsai, T. L., et al. (2017). Presence of Multidrug-Resistant Organisms in the Residents and Environments of Long-Term Care Facilities in Taiwan. J. Microbiol. Immunol. Infect. 50, 133–144. doi: 10.1016/j.jmii.2016.12.001
Le, M. N. T., Kayama, S., Yoshikawa, M., Hara, T., Kashiyama, S., Hisatsune, J., et al. (2020). Oral Colonisation by Antimicrobial-Resistant Gram-Negative Bacteria Among Long-Term Care Facility Residents: Prevalence, Risk Factors, and Molecular Epidemiology. Antimicrob. Resist. Infect. Control 9, 45. doi: 10.1186/s13756-020-0705-1
Li, J., Bi, W., Dong, G., Zhang, Y., Wu, Q., Dong, T., et al. (2020). The New Perspective of Old Antibiotic: In Vitro Antibacterial Activity of TMP-SMZ Against Klebsiella Pneumoniae. J. Microbiol. Immunol. Infect. 53, 757–765. doi: 10.1016/j.jmii.2018.12.013
Lin, M. Y., Lyles-Banks, R. D., Lolans, K., Hines, D. W., Spear, J. B., Petrak, R., et al. (2013). The Importance of Long-Term Acute Care Hospitals in the Regional Epidemiology of Klebsiella Pneumoniae Carbapenemase-Producing Enterobacteriaceae. Clin. Infect. Dis. 57, 1246–1252. doi: 10.1093/cid/cit500
Lin, Y. T., Su, C. F., Chuang, C., Lin, J. C., Lu, P. L., Huang, C. T., et al. (2018). Appropriate Treatment for Bloodstream Infections Due to Carbapenem-Resistant Klebsiella Pneumoniae and Escherichia Coli: A Nationwide Multicenter Study in Taiwan. Open Forum. Infect. Dis. 6, ofy336. doi: 10.1093/ofid/ofy336
Liu, P. Y., Ko, W. C., Lee, W. S., Lu, P. L., Chen, Y. H., Cheng, S. H., et al. (2021). In Vitro Activity of Cefiderocol, Cefepime/Enmetazobactam, Cefepime/Zidebactam, Eravacycline, Omadacycline, and Other Comparative Agents Against Carbapenem-non-Susceptible Pseudomonas Aeruginosa and Acinetobacter Baumannii Isolates Associated From Bloodstream Infection in Taiwan Between 2018-2020. J. Microbiol. Immunol. Infect. S1684–1182(21)00186-9. doi: 10.1016/j.jmii.2021.08.012
Livermore, D. M., Mushtaq, S., Warner, M., Vickers, A., Woodford, N. (2017). In Vitro Activity of Cefepime/Zidebactam (WCK 5222) Against Gram-Negative Bacteria. J. Antimicrob. Chemother. 72, 1373–1385. doi: 10.1093/jac/dkw593
Loose, M., Link, I., Naber, K. G., Wagenlehner, F. M. E. (2019). Carbapenem-Containing Combination Antibiotic Therapy Against Carbapenem-Resistant Uropathogenic Enterobacteriaceae. Antimicrob. Agents Chemother. 64, e01839-19. doi: 10.1128/AAC.01839-19
Lowings, M., Ehlers, M. M., Dreyer, A. W., Kock, M. M. (2015). High Prevalence of Oxacillinases in Clinical Multidrug-Resistant Acinetobacter Baumannii Isolates From the Tshwane Region, South Africa - an Update. BMC. Infect. Dis. 15, 521. doi: 10.1186/s12879-015-1246-8
Lowman, W., Schleicher, G. (2015). Antimicrobial Treatment and Outcomes of Critically Ill Patients With OXA-48-Like Carbapenemase-Producing Enterobacteriaceae Infections. Diagn. Microbiol. Infect. Dis. 81, 138–140. doi: 10.1016/j.diagmicrobio.2014.09.023
Lübbert, C., Becker-Rux, D., Rodloff, A. C., Laudi, S., Busch, T., Bartels, M., et al. (2014). Colonization of Liver Transplant Recipients With KPC-Producing Klebsiella Pneumoniae is Associated With High Infection Rates and Excess Mortality: A Case-Control Analysis. Infection 42, 309–316. doi: 10.1007/s15010-013-0547-3
March, A., Aschbacher, R., Sleghel, F., Soelva, G., Kaczor, M., Migliavacca, R., et al. (2017). Colonization of Residents and Staff of an Italian Long-Term Care Facility and an Adjacent Acute Care Hospital Geriatric Unit by Multidrug-Resistant Bacteria. New. Microbiol. 40, 258–263.
Matos, E. C. O., Andriolo, R. B., Rodrigues, Y. C., Lima, P. D. L., Carneiro, I. C. D. R. S., Lima, K. V. B. (2018). Mortality in Patients With Multidrug-Resistant Pseudomonas Aeruginosa Infections: A Meta-Analysis. Rev. Soc Bras. Med. Trop. 51, 415–420. doi: 10.1590/0037-8682-0506-2017
McConville, T. H., Sullivan, S. B., Gomez-Simmonds, A., Whittier, S., Uhlemann, A. C. (2017). Carbapenem-Resistant Enterobacteriaceae Colonization (CRE) and Subsequent Risk of Infection and 90-Day Mortality in Critically Ill Patients, an Observational Study. PLoS One 12, e0186195. doi: 10.1371/journal.pone.0186195
McCracken, M. G., Adam, H. J., Blondeau, J. M., Walkty, A. J., Karlowsky, J. A., Hoban, D. J., et al. (2019). Characterization of Carbapenem-Resistant and XDR Pseudomonas Aeruginosa in Canada: Results of the CANWARD 2007-16 Study. J. Antimicrob. Chemother. 74, 32–38. doi: 10.1093/jac/dkz285
McKinnell, J. A., Singh, R. D., Miller, L. G., Kleinman, K., Gussin, G., He, J., et al. (2019). The SHIELD Orange County Project: Multidrug-Resistant Organism Prevalence in 21 Nursing Homes and Long-Term Acute Care Facilities in Southern California. Clin. Infect. Dis. 69, 1566–1573. doi: 10.1093/cid/ciz119
McLaughlin, M., Advincula, M. R., Malczynski, M., Qi, C., Bolon, M., Scheetz, M. H. (2013). Correlations of Antibiotic Use and Carbapenem Resistance in Enterobacteriaceae. Antimicrob. Agents Chemother. 57, 5131–5133. doi: 10.1128/AAC.00607-13
Mehta, A. C., Muscarella, L. F. (2020). Bronchoscope-Related "Superbug" Infections. Chest 157, 454–469. doi: 10.1016/j.chest.2019.08.003
Micek, S. T., Wunderink, R. G., Kollef, M. H., Chen, C., Rello, J., Chastre, J., et al. (2015). An International Multicenter Retrospective Study of Pseudomonas Aeruginosa Nosocomial Pneumonia: Impact of Multidrug Resistance. Crit. Care 19, 219. doi: 10.1186/s13054-015-0926-5
Mills, J. P., Talati, N. J., Alby, K., Han, J. H. (2016). The Epidemiology of Carbapenem-Resistant Klebsiella Pneumoniae Colonization and Infection Among Long-Term Acute Care Hospital Residents. Infect. Control Hosp. Epidemiol. 37, 55–60. doi: 10.1017/ice.2015.254
Miriagou, V., Tzouvelekis, L. S., Flevari, K., Tsakiri, M., Douzinas, E. E. (2007). Providencia Stuartii With VIM-1 Metallo-Beta-Lactamase. J. Antimicrob. Chemother. 60, 183–184. doi: 10.1093/jac/dkm139
Mody, L., Gibson, K. E., Horcher, A., Prenovost, K., McNamara, S. E., Foxman, B., et al. (2015). Prevalence of and Risk Factors for Multidrug-Resistant Acinetobacter Baumannii Colonization Among High-Risk Nursing Home Residents. Infect. Control Hosp. Epidemiol. 36, 1155–1162. doi: 10.1017/ice.2015.143
Morata, L., Cobos-Trigueros, N., Martínez, J. A., Soriano, A., Almela, M., Marco, F., et al. (2012). Influence of Multidrug Resistance and Appropriate Empirical Therapy on the 30-Day Mortality Rate of Pseudomonas Aeruginosa Bacteremia. Antimicrob. Agents Chemother. 56, 4833–4837. doi: 10.1128/AAC.00750-12
Morrissey, I., Magnet, S., Hawser, S., Shapiro, S., Knechtle, P. (2019). In Vitro Activity of Cefepime-Enmetazobactam Against Gram-Negative Isolates Collected From U.S. And European Hospitals During 2014-2015. Antimicrob. Agents Chemother. 63, e00514-19. doi: 10.1128/AAC.00514-19
Moubareck, C. A., Halat, D. H., Akkawi, C., Nabi, A., AlSharhan, M. A., AlDeesi, Z. O., et al. (2019). Role of Outer Membrane Permeability, Efflux Mechanism, and Carbapenemases in Carbapenem-Nonsusceptible Pseudomonas Aeruginosa From Dubai Hospitals: Results of the First Cross-Sectional Survey. Int. J. Infect. Dis. 84, 143–150. doi: 10.1016/j.ijid.2019.04.027
Mushtaq, S., Vickers, A., Woodford, N., Haldimann, A., Livermore, D. M. (2019). Activity of Nacubactam (RG6080/OP0595) Combinations Against MBL-Producing Enterobacteriaceae. J. Antimicrob. Chemother. 74, 953–960. doi: 10.1093/jac/dky522
Neidhöfer, C., Buechler, C., Neidhöfer, G., Bierbaum, G., Hannet, I., Hoerauf, A., et al. (2021). Global Distribution Patterns of Carbapenemase-Encoding Bacteria in a New Light: Clues on a Role for Ethnicity. Front. Cell. Infect. Microbiol. 11, 659753. doi: 10.3389/fcimb.2021.659753
Nordmann, P., Poirel, L. (2019). Epidemiology and Diagnostics of Carbapenem Resistance in Gram-Negative Bacteria. Clin. Infect. Dis. 69, S521–S528. doi: 10.1093/cid/ciz824
Novelli, A., Del Giacomo, P., Rossolini, G. M., Tumbarello, M. (2020). Meropenem/vaborbactam: A Next Generation β-Lactam β-Lactamase Inhibitor Combination. Expert. Rev. Anti Infect. Ther. 18, 643–655. doi: 10.1080/14787210.2020.1756775
Nucleo, E., Caltagirone, M., Marchetti, V. M., D’Angelo, R., Fogato, E., Confalonieri, M., et al. (2018). Colonization of Long-Term Care Facility Residents in Three Italian Provinces by Multidrug-Resistant Bacteria. Antimicrob. Resist. Infect. Control 7, 33. doi: 10.1186/s13756-018-0326-0
O’Fallon, E., Pop-Vicas, A., D’Agata, E. (2009). The Emerging Threat of Multidrug-Resistant Gram-Negative Organisms in Long-Term Care Facilities. J. Gerontol. A. Biol. Sci. Med. Sci. 64, 138–141. doi: 10.1093/gerona/gln020
Olsson, A., Wistrand-Yuen, P., Nielsen, E. I., Friberg, L. E., Sandegren, L., Lagerbäck, P., et al. (2020). Efficacy of Antibiotic Combinations Against Multidrug-Resistant Pseudomonas Aeruginosa in Automated Time-Lapse Microscopy and Static Time-Kill Experiments. Antimicrob. Agents Chemother. 64, e02111-19. doi: 10.1128/AAC.02111-19
Otter, J. A., Burgess, P., Davies, F., Mookerjee, S., Singleton, J., Gilchrist, M., et al. (2017). Counting the Cost of an Outbreak of Carbapenemase-Producing Enterobacteriaceae: An Economic Evaluation From a Hospital Perspective. Clin. Microbiol. Infect. 23, 188–196. doi: 10.1016/j.cmi.2016.10.005
Ozdemir, H., Kendirli, T., Ergün, H., Ciftçi, E., Tapisiz, A., Güriz, H., et al. (2011). Nosocomial Infections Due to Acinetobacter Baumannii in a Pediatric Intensive Care Unit in Turkey. Turk. J. Pediatr. 53, 255–260.
Palacios-Baena, Z. R., Oteo, J., Conejo, C., Larrosa, M. N., Bou, G., Fernández-Martínez, M., et al. (2016). Comprehensive Clinical and Epidemiological Assessment of Colonisation and Infection Due to Carbapenemase-Producing Enterobacteriaceae in Spain. J. Infect. 72, 152–160. doi: 10.1016/j.jinf.2015.10.008
Pannaraj, P. S., Bard, J. D., Cerini, C., Weissman, S. J. (2015). Pediatric Carbapenem-Resistant Enterobacteriaceae in Los Angeles, California, a High-Prevalence Region in the United States. Pediatr. Infect. Dis. J. 34, 11–16. doi: 10.1097/INF.0000000000000471
Papadimitriou-Olivgeris, M., Marangos, M., Fligou, F., Christofidou, M., Sklavou, C., Vamvakopoulou, S., et al. (2013). KPC-Producing Klebsiella Pneumoniae Enteric Colonization Acquired During Intensive Care Unit Stay: The Significance of Risk Factors for its Development and its Impact on Mortality. Diagn. Microbiol. Infect. Dis. 77, 169–173. doi: 10.1016/j.diagmicrobio.2013.06.007
Papagiannitsis, C. C., Giakkoupi, P., Vatopoulos, A. C., Tryfinopoulou, K., Miriagou, V., Tzouvelekis, L. S. (2010). Emergence of Klebsiella Pneumoniae of a Novel Sequence Type (ST383) Producing VIM-4, KPC-2 and CMY-4 β-Lactamases. Int. J. Antimicrob. Agents 36, 573–574. doi: 10.1016/j.ijantimicag.2010.07.018
Papp-Wallace, K. M., Bethel, C. R., Caillon, J., Barnes, M. D., Potel, G., Bajaksouzian, S., et al. (2019). Beyond Piperacillin-Tazobactam: Cefepime and AAI101 as a Potent β-Lactam-β-Lactamase Inhibitor Combination. Antimicrob. Agents Chemother. 63, e00105-19. doi: 10.1128/AAC.00105-19
Piperaki, E. T., Tzouvelekis, L. S., Miriagou, V., Daikos, G. L. (2019). Carbapenem-Resistant Acinetobacter Baumannii: In Pursuit of an Effective Treatment. Clin. Microbiol. Infect. 25, 951–957. doi: 10.1016/j.cmi.2019.03.014
Pires, D. P., Boas, D. V., Sillankorva, S., Azeredo, J. (2015). Phage Therapy: A Step Forward in the Treatment of Pseudomonas Aeruginosa Infections. J. Virol. 89, 7449–7456. doi: 10.1128/JVI.00385-15
Pongpech, P., Amornnopparattanakul, S., Panapakdee, S., Fungwithaya, S., Nannha, P., Dhiraputra, C., et al. (2010). Antibacterial Activity of Carbapenem-Based Combinations Againts Multidrug-Resistant Acinetobacter Baumannii. J. Med. Assoc. Thai. 93, 161–171.
Poole, K. (2004). Efflux-Mediated Multiresistance in Gram-Negative Bacteria. Clin. Microbiol. Infect. 10, 12–26. doi: 10.1111/j.1469-0691.2004.00763.x
Poulikakos, P., Tansarli, G. S., Falagas, M. E. (2014). Combination Antibiotic Treatment Versus Monotherapy for Multidrug-Resistant, Extensively Drug-Resistant, and Pandrug-Resistant Acinetobacter Infections: A Systematic Review. Eur. J. Clin. Microbiol. Infect. Dis. 33, 1675–1685. doi: 10.1007/s10096-014-2124-9
Prasad, N., Labaze, G., Kopacz, J., Chwa, S., Platis, D., Pan, C. X., et al. (2016). Asymptomatic Rectal Colonization With Carbapenem-Resistant Enterobacteriaceae and Clostridium Difficile Among Residents of a Long-Term Care Facility in New York City. Am. J. Infect. Control 44, 525–532. doi: 10.1016/j.ajic.2015.11.021
Raman, G., Avendano, E. E., Chan, J., Merchant, S., Puzniak, L. (2018). Risk Factors for Hospitalized Patients With Resistant or Multidrug-Resistant Pseudomonas Aeruginosa Infections: A Systematic Review and Meta-Analysis. Antimicrob. Resist. Infect. Control 7, 79. doi: 10.1186/s13756-018-0370-9
Reuben, J., Donegan, N., Wortmann, G., DeBiasi, R., Song, X., Kumar, P., et al. (2017). Healthcare Antibiotic Resistance Prevalence - DC (HARP-DC): A Regional Prevalence Assessment of Carbapenem-Resistant Enterobacteriaceae (CRE) in Healthcare Facilities in Washington, District of Columbia. Infect. Control Hosp. Epidemiol. 38, 921–929. doi: 10.1017/ice.2017.110
Richards, A. M., Kwaik, Y. A., Lamont, R. J. (2015). Code Blue: Acinetobacter Baumannii, a Nosocomial Pathogen With a Role in the Oral Cavity. Mol. Oral. Microbiol. 30, 2–15. doi: 10.1111/omi.12072
Richter, S. S., Marchaim, D. (2017). Screening for Carbapenem-Resistant Enterobacteriaceae: Who, When, and How? Virulence 8, 417–426. doi: 10.1080/21505594.2016.1255381
Roy Chowdhury, P., Scott, M. J., Djordjevic, S. P. (2017). Genomic Islands 1 and 2 Carry Multiple Antibiotic Resistance Genes in Pseudomonas Aeruginosa ST235, ST253, ST111 and ST175 and are Globally Dispersed. J. Antimicrob. Chemother. 72, 620–622. doi: 10.1093/jac/dkw471
Sader, H. S., Carvalhaes, C. G., Arends, S. J. R., Castanheira, M., Mendes, R. E. (2021). Aztreonam/avibactam Activity Against Clinical Isolates of Enterobacterales Collected in Europe, Asia and Latin America in 2019. J. Antimicrob. Chemother. 76, 659–666. doi: 10.1093/jac/dkaa504
Sader, H. S., Castanheira, M., Huband, M., Jones, R. N., Flamm, R. K. (2017a). WCK 5222 (Cefepime-Zidebactam) Antimicrobial Activity Against Clinical Isolates of Gram-Negative Bacteria Collected Worldwide in 2015. Antimicrob. Agents Chemother. 61, e00072-17. doi: 10.1128/AAC.00072-17
Sader, H. S., Castanheira, M., Mendes, R. E., Flamm, R. K. (2018). Frequency and Antimicrobial Susceptibility of Gram-Negative Bacteria Isolated From Patients With Pneumonia Hospitalized in ICUs of US Medical Centres, (2015-17). J. Antimicrob. Chemother. 73, 3053–3059. doi: 10.1093/jac/dky279
Sader, H. S., Huband, M. D., Castanheira, M., Flamm, R. K. (2017b). Pseudomonas Aeruginosa Antimicrobial Susceptibility Results From Four Years, (2012 to 2015) of the International Network for Optimal Resistance Monitoring Program in the United States. Antimicrob. Agents Chemother. 61, e02252-16. doi: 10.1128/AAC.02252-16
Schäfer, E., Malecki, M., Tellez-Castillo, C. J., Pfennigwerth, N., Marlinghaus, L., Higgins, P. G., et al. (2019). Molecular Surveillance of Carbapenemase-Producing Pseudomonas Aeruginosa at Three Medical Centres in Cologne, Germany. Antimicrob. Resist. Infect. Control 8, 208. doi: 10.1186/s13756-019-0665-5
Semin-Pelletier, B., Cazet, L., Bourigault, C., Juvin, M. E., Boutoille, D., Raffi, F., et al. (2015). Challenges of Controlling a Large Outbreak of OXA-48 Carbapenemase-Producing Klebsiella Pneumoniae in a French University Hospital. J. Hosp. Infect. 89, 248–253. doi: 10.1016/j.jhin.2014.11.018
Sengstock, D. M., Thyagarajan, R., Apalara, J., Mira, A., Chopra, T., Kaye, K. S. (2010). Multidrug-Resistant Acinetobacter Baumannii: An Emerging Pathogen Among Older Adults in Community Hospitals and Nursing Homes. Clin. Infect. Dis. 50, 1611–1616. doi: 10.1086/652759
Shapiro, A. B., Moussa, S. H., McLeod, S. M., Durand-Réville, T., Miller, A. A. (2021). Durlobactam, a New Diazabicyclooctane β-Lactamase Inhibitor for the Treatment of Acinetobacter Infections in Combination With Sulbactam. Front. Microbiol. 12, 709974. doi: 10.3389/fmicb.2021.709974
Sheng, W. H., Liao, C. H., Lauderdale, T. L., Ko, W. C., Chen, Y. S., Liu, J. W., et al. (2010). A Multicenter Study of Risk Factors and Outcome of Hospitalized Patients With Infections Due to Carbapenem-Resistant Acinetobacter Baumannii. Int. J. Infect. Dis. 14, e764–e769. doi: 10.1016/j.ijid.2010.02.2254
Shields, R. K., Clancy, C. J., Gillis, L. M., Kwak, E. J., Silveira, F. P., Massih, R. C. A., et al. (2012). Epidemiology, Clinical Characteristics and Outcomes of Extensively Drug-Resistant Acinetobacter Baumannii Infections Among Solid Organ Transplant Recipients. PLoS One 7, e52349. doi: 10.1371/journal.pone.0052349
Shields, R. N., Potoski, B. A., Haidar, G., Hao, B., Doi, Y., Chen, L., et al. (2016). Clinical Outcomes, Drug Toxicity, and Emergence of Ceftazidime-Avibactam Resistance Among Patients Treated for Carbapenem-Resistant Enterobacteriaceae Infections. Clin. Infect. Dis. 63, 1615–1618. doi: 10.1093/cid/ciw636
Siriyong, T., Murray, R. M., Bidgood, L. E., Young, S. A., Wright, F., Parcell, B. J., et al. (2019). Dual β-Lactam Combination Therapy for Multi-Drug Resistant Pseudomonas Aeruginosa Infection: Enhanced Efficacy In Vivo and Comparison With Monotherapies of Penicillin-Binding Protein Inhibition. Sci. Rep. 9, 9098. doi: 10.1038/s41598-019-45550-z
Tacconelli, E., Carrara, E., Savoldi, A., Harbarth, S., Mendelson, M., Monnet, D. L., et al. (2018). Discovery, Research, and Development of New Antibiotics: The WHO Priority List of Antibiotic-Resistant Bacteria and Tuberculosis. Lancet Infect. Dis. 18, 318–327. doi: 10.1016/S1473-3099(17)30753-3
Takahata, S., Ida, T., Hiraishi, T., Sakakibara, S., Maebashi, K., Terada, S., et al. (2010). Molecular Mechanisms of Fosfomycin Resistance in Clinical Isolates of Escherichia Coli. Int. J. Antimicrob. Agents 35, 333–337. doi: 10.1016/j.ijantimicag.2009.11.011
Tamma, P. D., Goodman, K. E., Harris, A. D., Tekle, T., Roberts, A., Taiwo, A., et al. (2017). Comparing the Outcomes of Patients With Carbapenemase-Producing and Non-Carbapenemase-Producing Carbapenem-Resistant Enterobacteriaceae Bacteremia. Clin. Infect. Dis. 64, 257–264. doi: 10.1093/cid/ciw741
Tamma, P. D., Simner, P. J. (2018). Phenotypic Detection of Carbapenemase-Producing Organisms From Clinical Isolates. J. Clin. Microbiol. 56, e01140-18. doi: 10.1128/JCM.01140-18
Tang, H. J., Hsieh, C. F., Chang, P. C., Chen, J. J., Lin, Y. H., Lai, C. C., et al. (2016). Clinical Significance of Community- and Healthcare-Acquired Carbapenem-Resistant Enterobacteriaceae Isolates. PLoS One 11, e0151897. doi: 10.1371/journal.pone.0151897
Tang, H. J., Lai, C. C., Chen, C. C., Zhang, C. C., Weng, T. C., Chiu, Y. H., et al. (2019). Colistin-Sparing Regimens Against Klebsiella Pneumoniae Carbapenemase-Producing K. Pneumoniae Isolates: Combination of Tigecycline or Doxycycline and Gentamicin or Amikacin. J. Microbiol. Immunol. Infect. 52, 273–281. doi: 10.1016/j.jmii.2016.03.003
Tavoschi, L., Forni, S., Porretta, A., Righi, L., Pieralli, F., Menichetti, F., et al. (2020). Prolonged Outbreak of New Delhi Metallo-Beta-Lactamase-Producing Carbapenem-Resistant Enterobacterales (NDM-CRE), Tuscany, Italy 2018 to 2019. Euro. Surveill. 25, 2000085. doi: 10.2807/1560-7917.ES.2020.25.6.2000085
Tawfick, M. M., Alshareef, W. A., Bendary, H. A., Elmahalawy, H., Abdulall, A. K. (2020). The Emergence of Carbapenemase blaNDM Genotype Among Carbapenem-Resistant Enterobacteriaceae Isolates From Egyptian Cancer Patients. Eur. J. Clin. Microbiol. Infect. Dis. 39, 1251–1259. doi: 10.1007/s10096-020-03839-2
Tilahun, M., Kassa, Y., Gedefie, A., Ashagire, M. (2021). Emerging Carbapenem-Resistant Enterobacteriaceae Infection, its Epidemiology and Novel Treatment Options: A Review. Infect. Drug Resist. 14, 4363–4374. doi: 10.2147/IDR.S337611
Tran, D. M., Larsson, M., Olson, L., Hoang, N. T. B., Le, N. K., Khu, D. T. K., et al. (2019). High Prevalence of Colonisation With Carbapenem-Resistant Enterobacteriaceae Among Patients Admitted to Vietnamese Hospitals: Risk Factors and Burden of Disease. J. Infect. 79, 115–122. doi: 10.1016/j.jinf.2019.05.013
Tsakris, A., Ikonomidis, A., Pournaras, S., Tzouvelekis, L. S., Sofianou, D., Legakis, N. J., et al. (2006). VIM-1 Metallo-Beta-Lactamase in Acinetobacter Baumannii. Emerg. Infect. Dis. 12, 981–983. doi: 10.3201/eid1206.051097
Tselepis, L., Langley, G. W., Aboklaish, A. F., Widlake, E., Jackson, D. E., Walsh, T. R., et al. (2020). In Vitro Efficacy of Imipenem-Relebactam and Cefepime-AAI101 Against a Global Collection of ESBL-Positive and Carbapenemase-Producing Enterobacteriaceae. Int. J. Antimicrob. Agents 56, 105925. doi: 10.1016/j.ijantimicag.2020.105925
Tseng, C. C., Fang, W. F., Huang, K. T., Chang, P. W., Tu, M. L., Shiang, Y. P., et al. (2009). Risk Factors for Mortality in Patients With Nosocomial Stenotrophomonas Maltophilia Pneumonia. Infect. Control Hosp. Epidemiol. 30, 1193–1202. doi: 10.1086/648455
Tumbarello, M., Viale, P., Viscoli, C., Trecarichi, E. M., Tumietto, F., Marchese, A., et al. (2012). Predictors of Mortality in Bloodstream Infections Caused by Klebsiella Pneumoniae Carbapenemase-Producing K. Pneumoniae: Importance of Combination Therapy. Clin. Infect. Dis. 55, 943–950. doi: 10.1093/cid/cis588
Tzouvelekis, L. S., Markogiannakis, A., Piperaki, E., Souli, M., Daikos, G. L. (2014). Treating Infections Caused by Carbapenemase-Producing Enterobacteriaceae. Clin. Microbiol. Infect. 20, 862–872. doi: 10.1111/1469-0691.12697
Urzedo, J. E., de Paula Menezes, R., Porto, J. P., Ferreira, M. L., Gonçalves, I. R., de Brito, C. S., et al. (2020). High Mortality by Nosocomial Infections Caused by Carbapenem-Resistant P. Aeruginosa in a Referral Hospital in Brazil: Facing the Perfect Storm. J. Med. Microbiol. 69, 1388–1397. doi: 10.1099/jmm.0.001273
Van Duin, D., Paterson, D. L. (2016). Multidrug-Resistant Bacteria in the Community: Trends and Lessons Learned. Infect. Dis. Clin. North Am. 30, 377–390. doi: 10.1016/j.idc.2016.02.004
Vestergaard, M., Paulander, W., Marvig, R. L., Clasen, J., Jochumsen, N., Molin, S., et al. (2016). Antibiotic Combination Therapy can Select for Broad-Spectrum Multidrug Resistance in Pseudomonas Aeruginosa. Int. J. Antimicrob. Agents 47, 48–55. doi: 10.1016/j.ijantimicag.2015.09.014
Vijayakumar, S., Gopi, R., Gunasekaran, P., Bharathy, M., Walia, K., Anandan, S., et al. (2016). Molecular Characterization of Invasive Carbapenem-Resistant Acinetobacter Baumannii From a Tertiary Care Hospital in South India. Infect. Dis. Ther. 5, 379–387. doi: 10.1007/s40121-016-0125-y
Voor In ’t Holt, A. F., Severin, J. A., Lesaffre, E. M. E. H., Vos, M. C. (2014). A Systematic Review and Meta-Analyses Show That Carbapenem Use and Medical Devices are the Leading Risk Factors for Carbapenem-Resistant Pseudomonas Aeruginosa. Antimicrob. Agents Chemother. 58, 2626–2637. doi: 10.1128/AAC.01758-13
Vourli, S., Tsorlini, H., Katsifa, H., Polemis, M., Tzouvelekis, L. S., Kontodimou, A., et al. (2006). Emergence of Proteus Mirabilis Carrying the Bla Metallo-Beta-Lactamase Gene. Clin. Microbiol. Infect. 12, 691–694. doi: 10.1111/j.1469-0691.2006.01489
Vrioni, G., Daniil, I., Voulgari, E., Ranellou, K., Koumaki, V., Ghirardi, S., et al. (2012). Comparative Evaluation of a Prototype Chromogenic Medium (ChromID CARBA) for Detecting Carbapenemase-Producing Enterobacteriaceae in Surveillance Rectal Swabs. J. Clin. Microbiol. 50, 1841–1846. doi: 10.1128/JCM.06848-11
Wang, T. H., Leu, Y. S., Wang, N. Y., Liu, C. P., Yan, T. R. (2018). Prevalence of Different Carbapenemase Genes Among Carbapenem-Resistant Acinetobacter Baumannii Blood Isolates in Taiwan. Antimicrob. Resist. Infect. Control 7, 123. doi: 10.1186/s13756-018-0410-5
Wang, L., Luca, M. D., Tkhilaishvili, T., Trampuz, A., Moreno, M. G. (2019). Synergistic Activity of Fosfomycin, Ciprofloxacin, and Gentamicin Against Escherichia Coli and Pseudomonas Aeruginosa Biofilms. Front. Microbiol. 10, 2522. doi: 10.3389/fmicb.2019.02522
Wang, C. H., Ma, L., Huang, L. Y., Yeh, K. M., Lin, J. C., Siu, L. K., et al. (2021). Molecular Epidemiology and Resistance Patterns of blaOXA-48Klebsiella Pneumoniae and Escherichia Coli: A Nationwide Multicenter Study in Taiwan. J. Microbiol. Immunol. Infect. 54, 665–672. doi: 10.1016/j.jmii.2020.04.006
Wang, Y., Wang, J., Wang, R., Cai, Y. (2020). Resistance to Ceftazidime-Avibactam and Underlying Mechanisms. J. Glob. Antimicrob. Resist. 22, 18–27. doi: 10.1016/j.jgar.2019.12.009
Wang, Q., Wang, X., Wang, J., Ouyang, P., Jin, C., Wang, R., et al. (2018). Phenotypic and Genotypic Characterization of Carbapenem-Resistant Enterobacteriaceae: Data From a Longitudinal Large-Scale CRE Study in China, (2012-2016). Clin. Infect. Dis. 67, S196–S205. doi: 10.1093/cid/ciy660
Wang, X., Zhao, C., Wang, Q., Wang, Z., Liang, X., Zhang, F., et al. (2020). In Vitro Activity of the Novel β-Lactamase Inhibitor Taniborbactam (VNRX-5133), in Combination With Cefepime or Meropenem, Against MDR Gram-Negative Bacterial Isolates From China. J. Antimicrob. Chemother. 75, 1850–1858. doi: 10.1093/jac/dkaa053
Wang, J., Zhou, J. Y., Qu, T. T., Shen, P., Wei, Z. Q., Yu, Y. S., et al. (2010). Molecular Epidemiology and Mechanisms of Carbapenem Resistance in Pseudomonas Aeruginosa Isolates From Chinese Hospitals. Int. J. Antimicrob. Agents 35, 486–491. doi: 10.1016/j.ijantimicag.2009.12.014
Wareham, D. W., Abdul Momin, M. H. F. (2017). Rapid Detection of Carbapenemases in Enterobacteriaceae: Evaluation of the Resist-3 O.K.N. (OXA-48, KPC, NDM) Lateral Flow Multiplexed Assay. J. Clin. Microbiol. 55, 1223–1225. doi: 10.1128/JCM.02471-16
Wilkinson, K. M., Winstanley, T. G., Lanyon, C., Cummings, S. P., Raza, M. W., Perry, J. D. (2012). Comparison of Four Chromogenic Culture Media for Carbapenemase-Producing Enterobacteriaceae. J. Clin. Microbiol. 50, 3102–3104. doi: 10.1128/JCM.01613-12
Wu, P. S., Lu, C. Y., Chang, L. Y., Hsueh, P. R., Lee, P. I., Chen, J. M., et al. (2006). Stenotrophomonas Maltophilia Bacteremia in Pediatric Patients – a 10-Year Analysis. J. Microbiol. Immunol. Infect. 39, 144–149.
Yamano, Y. (2019). In Vitro Activity of Cefiderocol Against a Broad Range of Clinically Important Gram-Negative Bacteria. Clin. Infect. Dis. 69, S544–S551. doi: 10.1093/cid/ciz827
Yang, Y., Chen, J., Lin, D., Xu, X., Cheng, J., Sun, C. (2018). Prevalence and Drug Resistance Characteristics of Carbapenem-Resistant Enterobacteriaceae in Hangzhou, China. Front. Med. 12, 182–188. doi: 10.1007/s11684-017-0529-4
Yang, T. Y., Lu, P. L., Tseng, S. P. (2019). Update on Fosfomycin-Modified Genes in Enterobacteriaceae. J. Microbiol. Immunol. Infect. 52, 9–21. doi: 10.1016/j.jmii.2017.10.006
Yang, K., Xiao, T., Shi, Q., Zhu, Y., Ye, J., Zhou, Y., et al. (2021). Socioeconomic Burden of Bloodstream Infections Caused by Carbapenem-Resistant and Carbapenem-Susceptible Pseudomonas Aeruginosa in China. J. Glob. Antimicrob. Resist. 26, 101–107. doi: 10.1016/j.jgar.2021.03.032
Yapa, S., Li, J., Patel, K., Wilson, J. W., Dooley, M. J., George, J., et al. (2014). Pulmonary and Systemic Pharmacokinetics of Inhaled and Intravenous Colistin Methanesulfonate in Cystic Fibrosis Patients: Targeting Advantage of Inhalational Administration. Antimicrob. Agents Chemother. 58, 2570–2579. doi: 10.1128/AAC.01705-13
Yin, S., Huang, G., Zhang, Y., Jiang, B., Yang, Z., Dong, Z., et al. (2017). Phage Abp1 Rescues Human Cells and Mice From Infection by Pan-Drug Resistant Acinetobacter Baumannii. Cell. Physiol. Biochem. 44, 2337–2345. doi: 10.1159/000486117
Yin, D., Wu, S., Yang, Y., Shi, Q., Dong, D., Zhu, D., et al. (2019). Results From the China Antimicrobial Surveillance Network (CHINET) in 2017 of the In Vitro Activities of Ceftazidime-Avibactam and Ceftolozane-Tazobactam Against Clinical Isolates of Enterobacteriaceae and Pseudomonas Aeruginosa. Antimicrob. Agents Chemother. 63, e02431-18. doi: 10.1128/AAC.02431-18
Yoon, E. J., Jeong, S. H. (2021). Mobile Carbapenemase Genes in Pseudomonas Aeruginosa. Front. Microbiol. 12, 614058. doi: 10.3389/fmicb.2021.614058
Yoon, Y. K., Yang, K. S., Lee, S. E., Kim, H. J., Sohn, J. W., Kim, M. J. (2014). Effects of Group 1 Versus Group 2 Carbapenems on the Susceptibility of Acinetobacter Baumannii to Carbapenems: A Before and After Intervention Study of Carbapenem-Use Stewardship. PLoS One 9, e99101. doi: 10.1371/journal.pone.0099101
Yousefi, S., Nahaei, M. R., Farajnia, S., Ghojazadeh, M., Akhi, M. T., Sharifi, Y., et al. (2010). Class 1 Integron and Imipenem Resistance in Clinical Isolates of Pseudomonas Aeruginosa: Prevalence and Antibiotic Susceptibility. Iran J. Microbiol. 2, 115–121.
Zaidah, A. R., Mohammad, N. I., Suraiya, S., Harun, A. (2017). High Burden of Carbapenem-Resistant Enterobacteriaceae (CRE) Fecal Carriage at a Teaching Hospital: Cost-Effectiveness of Screening in Low-Resource Setting. Antimicrob. Resist. Infect. Control 6, 42. doi: 10.1186/s13756-017-0200-5
Zappas, S., Giakkoupi, P., Vourli, S., Hadjichristodoulou, C., Polemis, M., Tzouvelekis, L. S., et al. (2008). Epidemiology of Metalloenzyme-Producing Pseudomonas Aeruginosa in a Tertiary Hospital in Greece. J. Chemother. 20, 307–311. doi: 10.1179/joc.2008.20.3.307
Zhang, H., Jia, P., Zhu, Y., Zhang, G., Zhang, J., Kang, W., et al. (2021a). Susceptibility to Imipenem/Relebactam of Pseudomonas Aeruginosa and Acinetobacter Baumannii Isolates From Chinese Intra-Abdominal, Respiratory and Urinary Tract Infections: SMART 2015 to 2018. Infect. Drug Resist. 14, 3509–3518. doi: 10.2147/IDR.S325520
Zhang, R., Liu, L., Zhou, H., Chan, E. W., Li, J., Fang, Y., et al. (2017). Nationwide Surveillance of Clinical Carbapenem-Resistant Enterobacteriaceae (CRE) Strains in China. EBioMedicine 19, 98–106. doi: 10.1016/j.ebiom.2017.04.032
Zhang, H., Zhang, G., Yang, Y., Zhang, J., Li, D., Duan, S., et al. (2021b). Antimicrobial Resistance Comparison of Klebsiella Pneumoniae Pathogens Isolated From Intra-Abdominal and Urinary Tract Infections in Different Organs, Hospital Departments and Regions of China Between 2014 and 2017. J. Microbiol. Immunol. Infect. 54, 639–648. doi: 10.1016/j.jmii.2020.03.009
Keywords: carbapenem-resistant, extensively-drug resistant, gram-negative bacteria, ceftazidime-avibactam, enterobacterales, Pseudomonas aeruginosa, Acinetobacter baumannii complex
Citation: Jean S-S, Harnod D and Hsueh P-R (2022) Global Threat of Carbapenem-Resistant Gram-Negative Bacteria. Front. Cell. Infect. Microbiol. 12:823684. doi: 10.3389/fcimb.2022.823684
Received: 28 November 2021; Accepted: 15 February 2022;
Published: 15 March 2022.
Edited by:
Milena Dropa, University of São Paulo, BrazilReviewed by:
Subhasree Roy, National Institute of Cholera and Enteric Diseases (ICMR), IndiaPatrice Nordmann, Université de Fribourg, Switzerland
Copyright © 2022 Jean, Harnod and Hsueh. This is an open-access article distributed under the terms of the Creative Commons Attribution License (CC BY). The use, distribution or reproduction in other forums is permitted, provided the original author(s) and the copyright owner(s) are credited and that the original publication in this journal is cited, in accordance with accepted academic practice. No use, distribution or reproduction is permitted which does not comply with these terms.
*Correspondence: Po-Ren Hsueh, hsporen@gmail.com