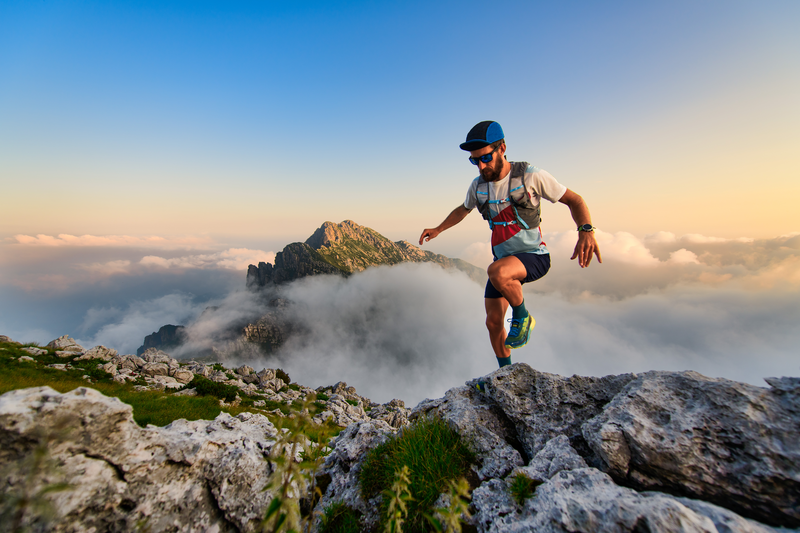
94% of researchers rate our articles as excellent or good
Learn more about the work of our research integrity team to safeguard the quality of each article we publish.
Find out more
ORIGINAL RESEARCH article
Front. Cell. Infect. Microbiol. , 27 May 2022
Sec. Clinical Microbiology
Volume 12 - 2022 | https://doi.org/10.3389/fcimb.2022.819802
This article is part of the Research Topic Women in Clinical Microbiology 2021 View all 10 articles
The distribution of the microbiome in women with advanced maternal age (AMA) is poorly understood. To gain insight into this, the vaginal and gut microbiota of 62 women were sampled and sequenced using the 16S rRNA technique. These women were divided into three groups, namely, the AMA (age ≥ 35 years, n = 13) group, the non-advanced maternal age (NMA) (age < 35 years, n = 38) group, and the control group (non-pregnant healthy women, age >35 years, n = 11). We found that the alpha diversity of vaginal microbiota in the AMA group significantly increased. However, the beta diversity significantly decreased in the AMA group compared with the control group. There was no significant difference in the diversity of gut microbiota among the three groups. The distributions of microbiota were significantly different among AMA, NMA, and control groups. In vaginal microbiota, the abundance of Lactobacillus was higher in the pregnant groups. Bifidobacterium was significantly enriched in the AMA group. In gut microbiota, Prevotella bivia was significantly enriched in the AMA group. Vaginal and gut microbiota in women with AMA were noticeably different from the NMA and non-pregnant women, and this phenomenon is probably related to the increased risk of complications in women with AMA.
The International Federation of Gynecology and Obstetrics refers to maternal age ≥35 years at the time of expected delivery as advanced maternal age (AMA). The proportion of births to women of AMA has increased over time throughout the developed world and in developing countries because of improved awareness and availability of effective contraception and improvement in assisted reproductive technology (Hsieh et al., 2010; Matthews and Hamilton, 2014; Arya et al., 2018). According to a survey in 2011, the proportion of AMA was 10.1%, including 8.3% aged 35–39 and 1.8% aged 40 or older in China (Liu et al., 2015). Women with AMA are also at increased risk of adverse pregnancy outcomes, such as gestational diabetes mellitus, hypertensive disorders of pregnancy, preterm delivery, fetal dysplasia, and fetal growth restriction (Cakmak et al., 2017; Fuchs et al., 2018; Marozio et al., 2019; Teng et al., 2020).
Pregnancy is a special physiological process in women. The microecology of different body sites will change during pregnancy as the hormones, immunity, metabolism, and other changes occur in women. Several differences in vaginal microecology occur during pregnancy in women of different ages (Li and Wang, 2020). Age is also a factor affecting the composition of oral microbiota in pregnant women (Wang T. et al., 2021). The diversity of gut microbiota decreases significantly in women with AMA and gestational diabetes (Huang, 2019). In addition, the colonization and diversity of gut microbiota are influenced by internal and external environmental factors during the co-evolution of the gut microbiome with their hosts, such as gender, age, race, dietary characteristics of the hosts, genotype, immune system, and region (Gohir et al., 2015b; Winer et al., 2016; Kvit and Kharchenko, 2017). It is necessary to understand the vaginal and gut microbiome in women with AMA. Therefore, we propose a hypothesis that the microbiota in women with AMA may be dysregulated. In order to provide direction for the prevention of adverse pregnancy outcome of women with AMA, we need to explore the specific bacteria of women with AMA. To confirm our hypothesis and explore the correlation between the microbiome and advanced pregnancy, we tested the distribution of vaginal and gut microbiota in women with AMA during the third trimester using 16S rRNA gene sequencing in this research.
A total of 51 healthy pregnant women who had antenatal care at Zhujiang Hospital, Southern Medical University were recruited from May 2020 to August 2020. They were divided into the AMA (age ≥ 35 years, n = 13) and non-advanced maternal age (NMA) (age < 35 years, n = 38) groups based on the age at the expected date of delivery. All of them were singleton pregnancies. A number of 11 non-pregnant healthy women over 35 years old were recruited as the control group. Women experiencing or undergoing the following were excluded: hematochezia, vaginal bleeding, sexual intercourse, rigorous cleaning with or without the use of any cleaning or disinfecting agents in the vaginal or perianal area within 7 days of sampling, treatment with antibiotics within 30 days of sampling, prenatal bleeding, premature rupture of membranes, placenta previa, placental abruption, or previa blood vessels.
We collected vaginal and rectal swabs from non-pregnant healthy women who were not menstruating and women between the 36th and 37th week of gestation in AMA and NMA groups. Vaginal secretions were sampled from the lower third of the vagina and feces were sampled from the rectum using a sterile swab. Meanwhile, vaginal and gut secretions were collected in the same swab for every pregnant women to culture whether they were infected with group B streptococcus. Moreover, the blank control group included three swabs, namely, a blank sample swab, a swab with the laboratory air sample, and a swab with the laboratory distilled water sample. All swabs were placed on ice immediately before being snap-frozen and stored at −80°C within 5 min of collection until DNA extraction.
Similar to Wang’s study (Wang Y. et al., 2021), a self-designed questionnaire by us was also used to evaluate the past year frequency and structure of maternal diet prior to delivery. As recommended by the Chinese Society of Nutrition (Zeng, 2018), six common foods for Chinese women during pregnancy were collected, namely, rice, flour, meat, vegetables, fruit, and yogurt. We assessed the intake of these foods every day.
The information of women was obtained from medical records. Neonatal information and histology of the placentas were collected after delivery. The histology of the placentas included normal placenta and mild, moderate, or severe fetal membrane inflammation. This study was carried out with the approval of the Ethics Service Committee of Zhujiang Hospital, Southern Medical University (China).
We extracted the total bacterial DNA from vaginal secretions and feces by a hexadecyltrimethylammonium bromide (CTAB) and magnetic soil and stool DNA kit. The purity and concentration of the DNA were monitored on 1% agarose gel electrophoresis. We used genome DNA that had been diluted with sterile water to 1 ng/μl as a template to perform polymerase chain reaction (PCR). To identify the bacterial diversity, we used the barcoded 515F 5′-CCTAYGGGRBGCASCAG-3′ and 806R 5′-GGACTACNNGGGTATCTAAT-3′ primers to amplify bacterial 16S rRNA V3–V4 fragments. Thermal cycling consisted of initial denaturation at 98°C for 1 min, followed by 30 cycles of denaturation at 98°C for 10 s, annealing at 50°C for 30 s, elongation at 72°C for 30 s, and finally 72°C for 5 min. The same volume of 1X loading buffer (contained SYB green) was mixed with PCR products and electrophoresis was carried out on 2% agarose gel for detection. PCR products were mixed in equidensity ratios. Then, the mixture of PCR products was purified with a Qiagen Gel Extraction Kit (Qiagen, Germany). Sequencing libraries were generated using the TruSeq® DNA PCR-Free Sample Preparation Kit (Illumina, USA) following the manufacturer’s recommendations and index codes were added. The library quality was assessed on the Qubit@ 2.0 Fluorometer (Thermo Scientific) and the Agilent Bioanalyzer 2100 system. Lastly, the library was sequenced on an Illumina NovaSeq platform and 250-bp paired-end reads were generated.
Paired-end reads were assigned to samples based on their unique barcode and truncated by cutting off the barcode and primer sequence. Paired-end reads were merged using FLASH (Magoc and Salzberg, 2011), a very fast and accurate analysis tool, which was designed to merge paired-end reads when at least some of the reads overlap the read generated from the opposite end of the same DNA fragment, and the splicing sequences were called raw tags. Quality filtering on the raw tags was performed under specific filtering conditions to obtain high-quality clean tags (Bokulich et al., 2013) according to the QIIME (Caporaso et al., 2010) quality-controlled process. The tags were compared with the Silva database using the UCHIME algorithm (Edgar et al., 2011) to detect chimera sequences, and then the chimera sequences were removed (Haas et al., 2011). Then, the effective tags were finally obtained.
Alpha diversity is applied in analyzing the complexity of species diversity of a sample through three indices, namely, the Shannon index, phylogenetic diversity (PD), and abundance-based coverage estimator (ACE). The Shannon index considers the total number of species and their relative abundances. PD is based on the proportion of branch length in a phylogenetic tree that leads to different organisms, whereas ACE is used to estimate the total number of species contained in a community sample. All these indices in our samples were calculated with QIIME (Version 1.9.1) and displayed with R software (Version 2.15.3).
Beta diversity analysis was used to evaluate differences of samples in species complexity. Beta diversity on both weighted and unweighted UniFrac distances were calculated by QIIME (Version 1.9.1). Principal coordinate analysis (PCoA) was performed to obtain principal coordinates and visualize complex, multidimensional data. A distance matrix of weighted or unweighted UniFrac distances among samples obtained before was transformed into a new set of orthogonal axes, by which the maximum variation factor is demonstrated by the first principal coordinate, the second maximum variation factor by the second principal coordinate, and so on. PCoA was displayed by the WGCNA package, stat packages, and the ggplot2 package in R software (Version 2.15.3). UniFrac evaluates microbiota similarity based on the shared evolutionary history of bacterial taxa (Lozupone and Knight, 2005) but does not control subject covariate information.
Linear discriminant analysis effect size (LEfSe) (Segata et al., 2011) is a tool for discovering and interpreting biomarkers and emphasizes statistical significance and biological correlation. It can search for statistically different biomarkers between groups.
The social characteristics of participants and diet were analyzed based on t-tests, performed in IBM SPSS (Statistical Package for the Social Sciences, version 21.0) at a 5% level of significance. The measurement data were tested for normality using the Kolmogorov–Smirnov test first. If the data conformed to normal distribution, they were expressed as mean ± standard deviation (x ± s) and analyzed with t-test. If the data were not normally distributed, the median was used for expression and the Mann–Whitney test was used for comparison. t-test and Wilcoxon tests were used for the analyses of alpha and beta diversity indexes between groups. Two-tailed p-values <0.05 were considered statistically significant unless otherwise stated.
The diets of women consisted mainly of grains, vegetables, and meat in the AMA and NMA groups (Table 1). No significant differences were observed with regard to pre-pregnancy body mass index (BMI), mode of delivery, gestational age of sample collection and delivery, gender of newborns, or weight and height of newborns between the AMA and NMA groups (p > 0.05). Gravidity and parity in the AMA group were higher than those in the NMA group (p < 0.05) (Table 1). No women were infected with group B streptococcus in the two groups. No difference was observed in the histology of placentas between the two groups (Z = −1.08, p = 0.28).
DNA extraction, PCR amplification, and electrophoretic detection in all the samples were deemed successful except for the samples in the blank control group.
In terms of the three alpha diversity measures in vaginal microbiota, we observed that the index of PD and ACE in AMA was obviously higher than that in NMA (Figures 1A–C). The AMA group had a significantly higher alpha diversity than the NMA group (ACE p < 0.05). More species were detected in the vaginal microbiota of the AMA group, and the community diversity was higher. On the contrary, the Shannon index was lower, and PD and ACE were higher in the AMA group than in the control group. The Shannon index indicates the total number of taxa in the sample and their percentage. The higher the community diversity and the more even the species distribution, the higher the Shannon index will be. Therefore, the distribution of species in the AMA group was more uneven than that in the control group. As for gut microbiota, the alpha diversity of the AMA group was slightly lower than that of the NMA group, but significantly higher than that of the control group (ACE p < 0.05) (Figures 1D–F). The community diversity was lower, and distribution was uneven in the gut microbiota of the AMA group, whereas all differences in the gut microbiota between the AMA and NMA groups were not statistically significant (p > 0.05).
Figure 1 (A–C) Alpha diversity index differences in vaginal microbiota between groups (Wilcoxon test). (D–F) Alpha diversity index differences in gut microbiota between groups (Wilcoxon test). NS, not statistically significant; *p < 0.05; **p < 0.01.
PCoA was performed using weighted UniFrac distance analysis to cluster the three groups at the OTU levels. We found that PCoA clearly separated patients in the control group and pregnant groups in terms of vaginal and gut microbiota. However, the distance between the AMA and NMA groups was close, which implies that the compositions of the microbiomes were similar (Figures 2A, B). The median and mean of the AMA and NMA groups were approximate. Thus, no difference in vaginal and gut microbiota was observed between the two groups (Figure 2C). The beta diversity of the vaginal microbiota in the AMA group significantly decreased compared with the control group (p < 0.001), whereas no difference in gut microbiota was noticed between the two groups (Figure 2D).
Figure 2 (A, B) PCoA (principal coordinates analysis) based on weighted UniFrac distance. (C, D) Beta diversity index differences between groups (Wilcoxon test). The abscissa represents a principal component, the ordinate represents another. The percentage represents the contribution value of the principal component to the sample difference. Each dot represents a sample, and samples in the same group are indicated in the same color.
The vaginal microbiota of the three groups mainly included Firmicutes, Actinobacteriota, Proteobacteria, and Bacteroidota. Firmicutes were abundant in pregnant women, with a proportion of 83.4% in the AMA group and 84.1% in the NMA group, whereas a value of 52.6% was observed in the control group. The proportion of Actinobacteriota and Proteobacteria was higher in the control group at values of 28.4% and 10.9%, respectively, whereas 12.6% and 0.8% were noticed in the AMA group (Figure 3A). At the genus level, the vaginal microbiota of the three groups mainly included Lactobacillus, Bifidobacterium, Gardnerella, Streptococcus, Escherichia-Shigella, and Prevotella, among others. Lactobacillus predominated in vaginal microbiota, and a lower proportion was observed in the control group (38.6%) than the AMA (81.2%) and NMA (81.8%) groups. The proportion of Gardnerella was higher in the control group (19%) than in the AMA group (5.1%) (Figure 3B). From the above two figures, we found that the composition of the vaginal microbiome in AMA and NMA groups was similar. However, the distribution in the control group was distinctly different.
Figure 3 (A) Abundance of species at the phylum level in vaginal microbiota. (B) Abundance of species at the genus level in vaginal microbiota. (C) Abundance of species at the phylum level in gut microbiota. (D) Abundance of species at the genus level in gut microbiota.
The gut microbiota of the three groups was mostly made up of Firmicutes, Bacteroidota, Fusobacteriota, Campilobacterota, Proteobacteria, and Actinobacteriota. Firmicutes were still abundant in pregnant women, with a proportion of 55% in the AMA group and 51.9% in the NMA group, whereas a 35.3% proportion was recorded in the control group. The proportion of Bacteroidota was higher in the control group (47.9%), whereas 35.4% was observed in the AMA group (Figure 3C). At the genus level, the gut microbiota of the three groups mainly included Bacteroides, Prevotella, Porphyromonas, Lactobacillus, and Fenollaria, among others. Prevotella predominated in the vaginal microbiota, featuring a lower proportion in the control group (25.9%) than the AMA (19.7%) and NMA (16.8%%) groups. The proportion of Bacteroides was higher in the control group (16.1%) than in the AMA group (5.8%) (Figure 3D).
We performed LEfSe analysis and linear discriminant analysis (LDA) to distinguish the three groups by identifying microbiota biomarkers at different taxonomic levels and estimating the effect size of each differentially abundant microbiota. This LDA score was set to 4. The larger the LDA score, the greater the effect of the microbiota had on the difference of the abundance between the comparative groups. Compared with the NMA group, the vaginal microbiota in the AMA group was mostly enriched with Bifidobacterium. However, Proteobacteria, Bifidobacterium bifidum, Gammaproteobacteria, and Lactobacillus johnsonii were significantly abundant in the NMA group (Figure 4A). Compared with the control group, the vaginal microbiota in the AMA group was mostly enriched with Firmicutes, Lactobacillales, Lactobacillaceae, Lactobacillus, Lactobacillus iners, and Bifidobacterium dentium. However, Prevotella timonensis, Bacteroidales, Ureaplasma parvum, Mycoplasmataceae, Aquabacterium, Fusabacteriales, Comamonadaceae, Escherichia coli, Enterobacteriaceae, Streptococcus agalactiae, Bifidobacterium, Actinobacteria, Gardnerella, and Lactobacillus johnsonii were significantly abundant in the control group (Figure 4B).
Figure 4 (A) LEfSe (LDA effect size) between AMA and NMA groups in vaginal microbiota. (B) LEfSe between AMA and control groups in vaginal microbiota. (C) LEfSe between AMA and NMA groups in gut microbiota. (D) LEfSe between AMA and control groups in gut microbiota.
As for the gut microbiota in the three groups, the abundance of Prevotella bivia was significantly higher in the AMA group than in the NMA group (Figure 4C). The abundances of Peptonophilus, Dialister, Veillonellaceae, Negativicutes, Clostridia, Firmicutes, and Veillonellales (Selenomonadales) were significantly higher in the AMA group compared with the control group. However, the abundance of Bacteroidota, Bacteroides vulgatus, and Gampylobacter hominis was significantly higher in the control group than in the AMA group (Figure 4D).
Recent studies have shown possible links between microbiota and pregnancy complication (Guzzardi et al., 2022; Molina-Vega et al., 2022; Yeo et al., 2022). However, the relationship between microbiota and maternal age has been rarely reported. Therefore, we investigated the changes of vaginal and gut microbiota in women with AMA by using 16S rRNA sequencing.
In our study, we found that the vaginal microbiota in the three groups is mostly made up of Firmicutes, Actinobacteriota, Proteobacteria, and Bacteroidota, as shown in Figure 3. Similar to previous reports (Li et al., 2021), we observed that Lactobacillus was the dominant bacterium in the vagina of women in the three groups. The composition of the female genital tract microbiota is influenced by numerous factors, including age, pH in vagina, hormonal secretions, the menstrual cycle, contraceptives, antibiotic use, and sexual activity (Prince et al., 2015; Nasioudis et al., 2017). Lactobacillus, the dominant bacterium in the vagina of women during pregnancy (Dominguez-Bello et al., 2010; Ma et al., 2012), can bind to the surface of vaginal epithelial cells to prevent the attachment of other microorganisms in the vagina. It can not only produce lactic acid by decomposing glycogen in the vagina to maintain a stable pH, but also kill intracellular microorganisms by inducing the autophagy of vaginal epithelial cells (Witkin and Linhares, 2017). The stability and abundance of Lactobacillus species in the vaginal microbiota depend on hormone levels (Hay, 2005). In the present study, the abundance of Lactobacillus was the highest in the vagina of women in the three groups, as the yellow part shows in Figures 3A, B, especially in the AMA and NMA groups. This was consistent with Pacha-Herrera’s report earlier, in which a higher amount of Lactobacillus was found in pregnant women when compared to non-pregnant women (Pacha-Herrera et al., 2020). The abundance of Lactobacillus was markedly lower in the control group, and this finding was related to the increase in estrogen in women during pregnancy (Taddei et al., 2018). A high proportion of Gardnerella (19%), which is an anaerobic bacterium considered one of the etiological agents of bacterial vaginosis (Morrill et al., 2020), was observed in the control group. Gardnerella was the dominant bacterium in the vagina of women over 35 years old. This result of our research was consistent with that of Chen et al., (2021).
In our research, the alpha diversity (PD and ACE) of vaginal microbiota was significantly higher in the AMA group than in the NMA group (p < 0.05), which means that the number of operational taxonomic units was higher in the AMA group. Thus, we speculated that more miscellaneous bacteria were present in the vaginal microbiota of women with AMA. However, due to the limited sample size of the AMA group, our conjecture needs to be further verified. In our research, the abundance of Bifidobacterium was higher in the vaginal microbiota of the AMA group. Freitas and Hill (2017) observed that Bifidobacterium has a protective effect on the vagina of older women. This bacterium can also reduce the risk of preterm birth and improve the microecology of the vaginal microbiome (Tabatabaei et al., 2019). L. johnsonii, which is one of the most commonly detected strains in the vagina of reproductive women (Dobrut et al., 2018; Mehta et al., 2020), was significantly abundant in the NMA group in our study. This bacterium can not only inhibit the growth of pathogens (Escherichia coli, Gardnerella vaginalis, Proteus mirabilis, and Candida albicans) (Ahire et al., 2021) but also reduce the incidence of preterm birth (Tabatabaei et al., 2019). A significant drop of Lactobacillus was also observed in the women with aerobic vaginitis of different ages (Pacha-Herrera et al., 2020). Therefore, the abundance of L. johnsonii decreased significantly in the AMA group, and this result may be associated with the increased risk of complications during pregnancy in women with AMA. The beta diversity of vaginal microbiota in the AMA group significantly decreased compared with the control group (p < 0.001). This finding demonstrated the significant difference between women with AMA and non-pregnant women in terms of vaginal microbiota. Freitas et al. (2017) also reported that the vaginal microbiome of healthy pregnant women had reduced richness and diversity and less Mycoplasma and Ureaplasma sp. compared with non-pregnant women. The abundance of Prevotella, Bacteroidales, Ureaplasma, Mycoplasmataceae, Streptococcus, Comamonadaceae, Escherichia, and Gardnerella was significantly higher in the control group than in the AMA group. Most of these bacteria were anaerobic, the increased abundance of which was related to bacterial vaginosis, premature rupture of membranes, preterm birth, gestational diabetes mellitus, and preeclampsia (Fettweis et al., 2019; Kong et al., 2019; Liu et al., 2019; Lin et al., 2020; Mohamed et al., 2020; Sprong et al., 2020). Gardnerella and Prevotella, in particular, increase the risk of bacterial vaginosis from previous studies (Pacha-Herrera et al., 2020). It is well known that bacterial vaginosis in pregnancy is associated with an increased risk of preterm birth (Foessleitner et al., 2021). Therefore, the increased abundance of beneficial bacterium and the decrease in pathogenic bacterium and miscellaneous species may be a self-protective mechanism for pregnant women to reduce the incidence of infection and miscarriage.
In recent years, studies related to gut microbiota and pregnancy have been extensively focused. The gut microbiota, composed of trillions of symbiotic microorganisms, is essential for host’s health and survival (Fung et al., 2017). The gut microbiota changes along with modifications in hormone levels and immune metabolism during gestation (Gohir et al, 2015a). The microbial diversity in the gut at the start of pregnancy appears to be similar to that in nonpregnant women, and the abundance of gut bacteria associated with inflammatory states increases as pregnancy advances (Edwards et al., 2017). Zhang et al. (2021) used 16S rRNA sequencing to explore the longitudinal changes of gut microbiota in diabetic mice. They observed a trend toward increased diversity and richness from 6 to 26 weeks in mice. In the present study, we observed a trend toward decreased diversity and richness in the AMA group as indicated by the PD, ACE, and Shannon indices, also suggesting that aging is an important factor affecting microbial composition. Similar to Lee and Sanlibaba’s studies (Lee et al., 2022; Sanlibaba et al., 2022), the gut microbiota changes are generally characterized by the decrease in biodiversity and enrichment of opportunistic pathogens with aging. The gestational gut microbiota is characterized by a low alpha diversity index (intraindividual bacterial diversity) and a high beta diversity index (interindividual bacterial diversity) in the third trimester (Wallace et al., 2019; Mesa et al., 2020). We also found that the alpha diversity of the AMA group was slightly lower than that of the NMA group. The difference between the two groups was not statistically significant; it might be related to the small sample size of this study. Firmicutes and Bacteroidota are the most abundant gut microbiota in human beings (Ley, 2016). Liu et al. (2017) indicated that the gut microbiota of pregnant women was mostly made up of Firmicutes, Bacteroidota, Fusobacteriota, and Proteobacteria in South China. In addition, they observed that the abundance of Bacteroidota was higher than that of Firmicutes in the gut microbiota in the third trimester. However, the results of our study showed the contrary; the proportion of Bacteroidota was the highest in non-pregnant women. The microbiota undergoes changes according to age, represented by a human study showing that the core microbiota of elderly subjects was different from that of younger adults, with a higher proportion of Bacteroides species (Claesson et al., 2011). The results are consistent with ours, in which the proportion of Bacteroides was higher in the control group. Prevotella bivia, a kind of Gram-negative anaerobic bacillus, was enriched in the AMA group and a part of the human oral microbiome (Accetto and Avgustin, 2021). Prevotella species are more common in non-Westerners who consume a plant-rich diet (Wu et al., 2011). The association with a plant-rich diet has suggested that Prevotella is a beneficial microbe. They are dominant in the rumen and hindgut of cattle and sheep, where they facilitate the fermentation of protein and carbohydrate from foods. Consumption of dietary fiber improves glucose metabolism, which is associated with increased abundance of Prevotella (Kovatcheva-Datchary et al., 2015). However, Prevotella in the gut has also been linked to inflammatory conditions (Dillon et al., 2016). The reasons for the increased abundance of Prevotella of gut microbiota in women with AMA still need further research. Peptonophilus, Dialister, Veillonellaceae, Negativicutes, and Clostridia were concentrated in the AMA group. Dialister is enriched in the gut microbiota of patients with osteoporosis and may be a microbial marker of spondylarthritis disease (Xu et al., 2020). A decrease in blood pressure in rats was associated with the lowering of Veillonellaceae, which includes succinate-producing bacteria (Galla et al., 2020). The abundance of Veillonellaceae increased in the gut microbiota of mice with abnormal glucose metabolism (Cheng et al., 2018). A research in Pakistan showed that Negativicutes are enriched in the gut microbiota of obese type 2 diabetes mellitus patients (Ahmad et al., 2019). Therefore, the accumulation of these bacterium may be associated with the increased risk of gestational diabetes and gestational hypertension in AMA.
As far as we know, this research is the first study that analyzed the vaginal and gut microbiota of women with AMA. We observed that the alpha diversity of vaginal microbiota in women with AMA significantly increased. The beta diversity was significantly compared with that of non-pregnant older women. The presence of various statistical biomarkers in the AMA group suggests significant differences in the distribution of microbiota in women with AMA and NMA. However, this was only a preliminary research because the total quantity of the samples was limited. Although we have not found the kind of specific bacterium of women with AMA, the dysregulation in vaginal and gut microbiota of women with AMA was confirmed in our study. In the future, we would like to increase the sample size and detect the metabolic characteristics of the microbiome in women with AMA by metagenomic sequencing and to further find the type of specific bacteria from women with AMA and deeply explore the relationship between specific bacteria and adverse pregnancy outcomes in women with AMA.
The original contributions presented in the study are publicly available. These data can be found here: NCBI, PRJNA812337.
The studies involving human participants were reviewed and approved by the Medical Ethical Committee of Zhujiang Hospital, Southern Medical University. The patients/participants provided their written informed consent to participate in this study.
YH: Writing—review and editing, methodology, and resources. DL: Writing—original draft, data curation, and formal analysis. WC: Formal analysis and writing—review and editing. HZ: Data curation and formal analysis. MIS: Resources and software. CL: Supervision and project administration. SP: Conceptualization, visualization, funding acquisition, and project administration. All authors contributed to the article and approved the submitted version.
This work was funded by grants from the National Key R&D Program of China (2019YFC0121904).
The authors declare that the research was conducted in the absence of any commercial or financial relationships that could be construed as a potential conflict of interest.
All claims expressed in this article are solely those of the authors and do not necessarily represent those of their affiliated organizations, or those of the publisher, the editors and the reviewers. Any product that may be evaluated in this article, or claim that may be made by its manufacturer, is not guaranteed or endorsed by the publisher.
Accetto, T., Avgustin, G. (2021). Non-Oral Prevotella Stepping Into the Spotlight. Anaerobe 68, 102321. doi: 10.1016/j.anaerobe.2021.102321
Ahire, J. J., Sahoo, S., Kashikar, M. S., Heerekar, A., Lakshmi, S. G., Madempudi, R. S. (2021). In Vitro Assessment of Lactobacillus Crispatus UBLCp01, Lactobacillus Gasseri UBLG36, and Lactobacillus Johnsonii UBLJ01 as a Potential Vaginal Probiotic Candidate. Probiot. Antimicrob. Proteins. doi: 10.1007/s12602-021-09838-9
Ahmad, A., Yang, W., Chen, G., Shafiq, M., Javed, S., Ali, Z. S., et al. (2019). Analysis of Gut Microbiota of Obese Individuals With Type 2 Diabetes and Healthy Individuals. PLoS One 14 (12), e226372. doi: 10.1371/journal.pone.0226372
Arya, S., Mulla, Z. D., Plavsic, S. K. (2018). Outcomes of Women Delivering at Very Advanced Maternal Age. J. Womens. Health (Larchmt). 27 (11), 1378–1384. doi: 10.1089/jwh.2018.7027
Bokulich, N. A., Subramanian, S., Faith, J. J., Gevers, D., Gordon, J. I., Knight, R., et al. (2013). Quality-Filtering Vastly Improves Diversity Estimates From Illumina Amplicon Sequencing. Nat. Methods 10 (1), 57–59. doi: 10.1038/nmeth.2276
Cakmak, C. F., Aygun, C., Kucukoduk, S., Bek, Y. (2017). Maternal and Neonatal Outcomes in Advanced Maternal Age: A Retrospective Cohort Study. J. Matern. Fetal. Neonatal. Med. 30 (20), 2452–2456. doi: 10.1080/14767058.2016.1253058
Caporaso, J. G., Kuczynski, J., Stombaugh, J., Bittinger, K., Bushman, F. D., Costello, E. K., et al. (2010). QIIME Allows Analysis of High-Throughput Community Sequencing Data. Nat. Methods 7 (5), 335–336. doi: 10.1038/nmeth.f.303
Chen, N., Chen, C., Wang, X., Lu, R., Gao, H., Li, S., et al. (2021). Analysis of the Lower Genital Tract Microbiome of 373 Women Using Metagenomic Shotgun Sequencing. Chin. J. Family Plann. Gynecotokol. 13 (07), 88–90+93+100. doi: 10.3969/j.issn.1674-4020.2021.07.25
Cheng, J., Xue, F., Zhang, M., Cheng, C., Qiao, L., Ma, et al. (2018). TRIM31 Deficiency Is Associated With Impaired Glucose Metabolism and Disrupted Gut Microbiota in Mice. Front. Physiol. 9. doi: 10.3389/fphys.2018.00024
Claesson, M. J., Cusack, S., O'Sullivan, O., Greene-Diniz, R., de Weerd, H., Flannery, E., et al. (2011). Composition, Variability, and Temporal Stability of the Intestinal Microbiota of the Elderly. Proc. Natl. Acad. Sci. U. S. A. 108 Suppl 1, 4586–4591. doi: 10.1073/pnas.1000097107
Dillon, S. M., Lee, E. J., Kotter, C. V., Austin, G. L., Gianella, S., Siewe, B., et al. (2016). Gut Dendritic Cell Activation Links an Altered Colonic Microbiome to Mucosal and Systemic T-Cell Activation in Untreated HIV-1 Infection. Mucosal Immunol. 9 (1), 24–37. doi: 10.1038/mi.2015.33
Dobrut, A., Gosiewski, T., Pabian, W., Bodaszewska-Lubas, M., Ochonska, D., Bulanda, M., et al. (2018). The Dynamics of Vaginal and Rectal Lactobacillus Spp. Flora in Subsequent Trimesters of Pregnancy in Healthy Polish Women, Assessed Using the Sanger Sequencing Method. BMC Pregnancy Childbirth. 18 (1), 350. doi: 10.1186/s12884-018-1987-7
Dominguez-Bello, M. G., Costello, E. K., Contreras, M., Magris, M., Hidalgo, G., Fierer, N., et al. (2010). Delivery Mode Shapes the Acquisition and Structure of the Initial Microbiota Across Multiple Body Habitats in Newborns. Proc. Natl. Acad. Sci. U. S. A. 107 (26), 11971–11975. doi: 10.1073/pnas.1002601107
Edgar, R. C., Haas, B. J., Clemente, J. C., Quince, C., Knight, R. (2011). UCHIME Improves Sensitivity and Speed of Chimera Detection. Bioinformatics 27 (16), 2194–2200. doi: 10.1093/bioinformatics/btr381
Edwards, S. M., Cunningham, S. A., Dunlop, A. L., Corwin, E. J. (2017). The Maternal Gut Microbiome During Pregnancy. MCN. Am. J. Matern. Child Nurs. 42 (6), 310–317. doi: 10.1097/NMC.0000000000000372
Fettweis, J. M., Serrano, M. G., Brooks, J. P., Edwards, D. J., Girerd, P. H., Parikh, H. I., et al. (2019). The Vaginal Microbiome and Preterm Birth. Nat. Med. 25 (6), 1012–1021. doi: 10.1038/s41591-019-0450-2
Foessleitner, P., Kiss, H., Deinsberger, J., Ott, J., Zierhut, L., Rosta, K., et al. (2021). Screening Pregnant Women for Bacterial Vaginosis Using a Point-of-Care Test: A Prospective Validation Study. J. Clin. Med. 10 (11), 2275. doi: 10.3390/jcm10112275
Freitas, A. C., Chaban, B., Bocking, A., Rocco, M., Yang, S., Hill, J. E., et al. (2017). The Vaginal Microbiome of Pregnant Women Is Less Rich and Diverse, With Lower Prevalence of Mollicutes, Compared to Non-Pregnant Women. Sci. Rep. 7 (1), 9212. doi: 10.1038/s41598-017-07790-9
Freitas, A. C., Hill, J. E. (2017). Quantification, Isolation and Characterization of Bifidobacterium From the Vaginal Microbiomes of Reproductive Aged Women. Anaerobe 47, 145–156. doi: 10.1016/j.anaerobe.2017.05.012
Fuchs, F., Monet, B., Ducruet, T., Chaillet, N., Audibert, F. (2018). Effect of Maternal Age on the Risk of Preterm Birth: A Large Cohort Study. PLoS One 13 (1), e191002. doi: 10.1371/journal.pone.0191002
Fung, T. C., Olson, C. A., Hsiao, E. Y. (2017). Interactions Between the Microbiota, Immune and Nervous Systems in Health and Disease. Nat. Neurosci. 20 (2), 145–155. doi: 10.1038/nn.4476
Galla, S., Chakraborty, S., Cheng, X., Yeo, J. Y., Mell, B., Chiu, N., et al. (2020). Exposure to Amoxicillin in Early Life Is Associated With Changes in Gut Microbiota and Reduction in Blood Pressure: Findings From a Study on Rat Dams and Offspring. J. Am. Heart Assoc. 9 (2), e14373. doi: 10.1161/JAHA.119.014373
Gohir, W., Ratcliffe, E. M., Sloboda, D. M. (2015a). Of the Bugs That Shape Us: Maternal Obesity, the Gut Microbiome, and Long-Term Disease Risk. Pediatr. Res. 77 (1-2), 196–204. doi: 10.1038/pr.2014.169
Gohir, W., Whelan, F. J., Surette, M. G., Moore, C., Schertzer, J. D., Sloboda, D. M. (2015b). Pregnancy-Related Changes in the Maternal Gut Microbiota Are Dependent Upon the Mother's Periconceptional Diet. Gut. Microbes 6 (5), 310–320. doi: 10.1080/19490976.2015.1086056
Guzzardi, M. A., Ederveen, T., Rizzo, F., Weisz, A., Collado, M. C., Muratori, F., et al. (2022). Maternal Pre-Pregnancy Overweight and Neonatal Gut Bacterial Colonization Are Associated With Cognitive Development and Gut Microbiota Composition in Pre-School-Age Offspring. Brain Behav. Immun. 100, 311–320. doi: 10.1016/j.bbi.2021.12.009
Haas, B. J., Gevers, D., Earl, A. M., Feldgarden, M., Ward, D. V., Giannoukos, G., et al. (2011). Chimeric 16s rRNA Sequence Formation and Detection in Sanger and 454-Pyrosequenced PCR Amplicons. Genome Res. 21 (3), 494–504. doi: 10.1101/gr.112730.110
Hay, P. (2005). Life in the Littoral Zone: Lactobacilli Losing the Plot. Sex. Transm. Infect. 81 (2), 100–102. doi: 10.1136/sti.2003.007161
Hsieh, T. T., Liou, J. D., Hsu, J. J., Lo, L. M., Chen, S. F., Hung, T. H. (2010). Advanced Maternal Age and Adverse Perinatal Outcomes in an Asian Population. Eur. J. Obstet. Gynecol. Reprod. Biol. 148 (1), 21–26. doi: 10.1016/j.ejogrb.2009.08.022
Huang, Y. (2019). Distribution Characterristics of Gut Microbiota in Pregnant Women With Gestational Diabetes Mellitus (Guangzhou, China: Southern Medical University).
Kong, Y., Yang, T., Yang, T., Ruan, Z., Song, T., Ding, H., et al. (2019). Correlation Between Ureaplasma Spp. Sub-Group 1 and Preterm Pre-Labour Rupture of Membranes Revealed by an eMLST Scheme. Infect. Genet. Evol. 68, 172–176. doi: 10.1016/j.meegid.2018.12.025
Kovatcheva-Datchary, P., Nilsson, A., Akrami, R., Lee, Y. S., De Vadder, F., Arora, T., et al. (2015). Dietary Fiber-Induced Improvement in Glucose Metabolism Is Associated With Increased Abundance of Prevotella. Cell Metab. 22 (6), 971–982. doi: 10.1016/j.cmet.2015.10.001
Kvit, K. B., Kharchenko, N. V. (2017). Gut Microbiota Changes as a Risk Factor for Obesity. Wiad. Lek. 70 (2), 231–235. PMID: 28511167.
Lee, H. Y., Lee, S. H., Min, K. J. (2022). The Increased Abundance of Commensal Microbes Decreases Drosophila Melanogaster Lifespan Through an Age-Related Intestinal Barrier Dysfunction. Insects 13 (2), 219. doi: 10.3390/insects13020219
Ley, R. E. (2016). Gut Microbiota in 2015: Prevotella in the Gut: Choose Carefully. Nat. Rev. Gastroenterol. Hepatol. 13 (2), 69–70. doi: 10.1038/nrgastro.2016.4
Li, D., Huang, Y., Sadykova, A., Zheng, W., Lin, L., Jin, C., et al. (2021). Composition of the Microbial Communities at Different Body Sites in Women With Preterm Birth and Their Newborns. Med. Microecol. 9, 100046. doi: 10.1016/j.medmic.2021.100046
Lin, C. Y., Lin, C. Y., Yeh, Y. M., Yang, L. Y., Lee, Y. S., Chao, A., et al. (2020). Severe Preeclampsia Is Associated With a Higher Relative Abundance of Prevotella Bivia in the Vaginal Microbiota. Sci. Rep. 10 (1), 18249. doi: 10.1038/s41598-020-75534-3
Liu, H., Pan, L. L., Lv, S., Yang, Q., Zhang, H., Chen, W., et al. (2019). Alterations of Gut Microbiota and Blood Lipidome in Gestational Diabetes Mellitus With Hyperlipidemia. Front. Physiol. 10. doi: 10.3389/fphys.2019.01015
Liu, J., Yang, H., Yin, Z., Jiang, X., Zhong, H., Qiu, D., et al. (2017). Remodeling of the Gut Microbiota and Structural Shifts in Preeclampsia Patients in South China. Eur. J. Clin. Microbiol. Infect. Dis. 36 (4), 713–719. doi: 10.1007/s10096-016-2853-z
Liu, X., Yuan, Y., Liu, Y., Zhang, W. (2015). Relationship Between Maternal Age and Hypertensive Disorders in Pregnancy. Natl. Med. J. China 95 (01), 19–22. doi: CNKI:SUN:ZGGZ.0.2015-08-041
Li, D., Wang, S. (2020). Analysis of Vaginal Microecology in Early Pregnancy in Women of Different Ages. Chin. J. Med. 55 (09), 1005–1007. doi: 10.3969/j.issn.1008-1070.2020.09.025
Lozupone, C., Knight, R. (2005). UniFrac: A New Phylogenetic Method for Comparing Microbial Communities. Appl. Environ. Microbiol. 71 (12), 8228–8235. doi: 10.1128/AEM.71.12.8228-8235.2005
Ma, B., Forney, L. J., Ravel, J. (2012). Vaginal Microbiome: Rethinking Health and Disease. Annu. Rev. Microbiol. 66, 371–389. doi: 10.1146/annurev-micro-092611-150157
Magoc, T., Salzberg, S. L. (2011). FLASH: Fast Length Adjustment of Short Reads to Improve Genome Assemblies. Bioinformatics 27 (21), 2957–2963. doi: 10.1093/bioinformatics/btr507
Marozio, L., Picardo, E., Filippini, C., Mainolfi, E., Berchialla, P., Cavallo, F., et al. (2019). Maternal Age Over 40 Years and Pregnancy Outcome: A Hospital-Based Survey. J. Matern. Fetal. Neonatal. Med. 32 (10), 1602–1608. doi: 10.1080/14767058.2017.1410793
Matthews, T. J., Hamilton, B. E. (2014). First Births to Older Women Continue to Rise. NCHS. Data Brief 152, 1–8. PMID: 24813228.
Mehta, O., Ghosh, T. S., Kothidar, A., Gowtham, M. R., Mitra, R., Kshetrapal, P., et al. (2020). Vaginal Microbiome of Pregnant Indian Women: Insights Into the Genome of Dominant Lactobacillus Species. Microb. Ecol. 80 (2), 487–499. doi: 10.1007/s00248-020-01501-0
Mesa, M. D., Loureiro, B., Iglesia, I., Fernandez, G. S., Llurba, O. E., Garcia, A. O., et al. (2020). The Evolving Microbiome From Pregnancy to Early Infancy: A Comprehensive Review. Nutrients 12 (1), 133. doi: 10.3390/nu12010133
Mohamed, I., Zakeer, S., Azab, M., Hanora, A. (2020). Changes in Vaginal Microbiome in Pregnant and Nonpregnant Women With Bacterial Vaginosis: Toward Microbiome Diagnostics? OMICS 24 (10), 602–614. doi: 10.1089/omi.2020.0096
Molina-Vega, M., Picon-Cesar, M. J., Gutierrez-Repiso, C., Fernandez-Valero, A., Lima-Rubio, F., Gonzalez-Romero, S., et al. (2022). Metformin Action Over Gut Microbiota Is Related to Weight and Glycemic Control in Gestational Diabetes Mellitus: A Randomized Trial. BioMed. Pharmacother. 145, 112465. doi: 10.1016/j.biopha.2021.112465
Morrill, S., Gilbert, N. M., Lewis, A. L. (2020). Gardnerella Vaginalis as a Cause of Bacterial Vaginosis: Appraisal of the Evidence From In Vivo Models. Front. Cell Infect. Microbiol. 10. doi: 10.3389/fcimb.2020.00168
Nasioudis, D., Forney, L. J., Schneider, G. M., Gliniewicz, K., France, M., Boester, A., et al. (2017). Influence of Pregnancy History on the Vaginal Microbiome of Pregnant Women in Their First Trimester. Sci. Rep. 7 (1), 10201. doi: 10.1038/s41598-017-09857-z
Pacha-Herrera, D., Vasco, G., Cruz-Betancourt, C., Galarza, J. M., Barragan, V., Machado, A. (2020). Vaginal Microbiota Evaluation and Lactobacilli Quantification by qPCR in Pregnant and Non-Pregnant Women: A Pilot Study. Front. Cell Infect. Microbiol. 10. doi: 10.3389/fcimb.2020.00303
Prince, A. L., Chu, D. M., Seferovic, M. D., Antony, K. M., Ma, J., Aagaard, K. M. (2015). The Perinatal Microbiome and Pregnancy: Moving Beyond the Vaginal Microbiome. Cold Spring Harb. Perspect. Med. 5 (6), a023051. doi: 10.1101/cshperspect.a023051
Sanlibaba, P., Toprak, Z., Tezel, B. U. (2022). The Role of Probiotics on Slowing Down the Aging Process. Acta Sci. Pol. Technol. Aliment. 21 (1), 53–66. doi: 10.17306/J.AFS.1013
Segata, N., Izard, J., Waldron, L., Gevers, D., Miropolsky, L., Garrett, W. S., et al. (2011). Metagenomic Biomarker Discovery and Explanation. Genome Biol. 12 (6), R60. doi: 10.1186/gb-2011-12-6-r60
Sprong, K. E., Mabenge, M., Wright, C. A., Govender, S. (2020). Ureaplasma Species and Preterm Birth: Current Perspectives. Crit. Rev. Microbiol. 46 (2), 169–181. doi: 10.1080/1040841X.2020.1736986
Tabatabaei, N., Eren, A. M., Barreiro, L. B., Yotova, V., Dumaine, A., Allard, C., et al. (2019). Vaginal Microbiome in Early Pregnancy and Subsequent Risk of Spontaneous Preterm Birth: A Case-Control Study. BJOG 126 (3), 349–358. doi: 10.1111/1471-0528.15299
Taddei, C. R., Cortez, R. V., Mattar, R., Torloni, M. R., Daher, S. (2018). Microbiome in Normal and Pathological Pregnancies: A Literature Overview. Am. J. Reprod. Immunol. 80 (2), e12993. doi: 10.1111/aji.12993
Teng, X., Shane, M. I., Pan, S. (2020). The Changing Situation About Maternal Age, Risk Factors and Pregnancy Outcomes After the Two-Child Policy: A Retrospective Cohort Study. Ann. Palliat. Med. 9 (3), 824–834. doi: 10.21037/apm.2020.04.27
Wallace, J. G., Bellissimo, C. J., Yeo, E., Fei, X. Y., Petrik, J. J., Surette, M. G., et al. (2019). Obesity During Pregnancy Results in Maternal Intestinal Inflammation, Placental Hypoxia, and Alters Fetal Glucose Metabolism at Mid-Gestation. Sci. Rep. 9 (1), 17621. doi: 10.1038/s41598-019-54098-x
Wang, T., Li, Q., Liu, H., Li, F., Hu, A., Zheng, Y. (2021). Characteristics of Oral Microbiota in Pregnant Women. Chin. J. Health Lab. Tec. 31 (02), 129–133. doi: CNKI:SUN:ZWJZ.0.2021-02-001
Wang, Y., Xie, T., Wu, Y., Liu, Y., Zou, Z., Bai, J. (2021). Impacts of Maternal Diet and Alcohol Consumption During Pregnancy on Maternal and Infant Gut Microbiota. Biomolecules 11 (3), 369. doi: 10.3390/biom11030369
Winer, D. A., Luck, H., Tsai, S., Winer, S. (2016). The Intestinal Immune System in Obesity and Insulin Resistance. Cell Metab. 23 (3), 413–426. doi: 10.1016/j.cmet.2016.01.003
Witkin, S. S., Linhares, I. M. (2017). Why do Lactobacilli Dominate the Human Vaginal Microbiota? BJOG 124 (4), 606–611. doi: 10.1111/1471-0528.14390
Wu, G. D., Chen, J., Hoffmann, C., Bittinger, K., Chen, Y. Y., Keilbaugh, S. A., et al. (2011). Linking Long-Term Dietary Patterns With Gut Microbial Enterotypes. Science 334 (6052), 105–108. doi: 10.1126/science.1208344
Xu, Z., Xie, Z., Sun, J., Huang, S., Chen, Y., Li, C., et al. (2020). Gut Microbiome Reveals Specific Dysbiosis in Primary Osteoporosis. Front. Cell Infect. Microbiol. 10. doi: 10.3389/fcimb.2020.00160
Yeo, E., Brubaker, P. L., Sloboda, D. M. (2022). The Intestine and the Microbiota in Maternal Glucose Homeostasis During Pregnancy. J. Endocrinol. doi: 10.1530/JOE-21-0354
Zeng, G. (2018). Interpretation of “Dietary Guidelines for Pregnant Women in 2016” by The Chinese Nutrition Society. J. Pract. Obstetr. Gynecol. 34 (04), 265–267. doi: CNKI:SUN:SFCZ.0.2018-04-012
Keywords: advanced maternal age, vaginal microbiota, gut microbiota, diversity, abundance
Citation: Huang Y, Li D, Cai W, Zhu H, Shane MI, Liao C and Pan S (2022) Distribution of Vaginal and Gut Microbiome in Advanced Maternal Age. Front. Cell. Infect. Microbiol. 12:819802. doi: 10.3389/fcimb.2022.819802
Received: 22 November 2021; Accepted: 20 April 2022;
Published: 27 May 2022.
Edited by:
Sophia Vourli, University General Hospital Attikon, GreeceReviewed by:
Almagul Kushugulova, Nazarbayev University, KazakhstanCopyright © 2022 Huang, Li, Cai, Zhu, Shane, Liao and Pan. This is an open-access article distributed under the terms of the Creative Commons Attribution License (CC BY). The use, distribution or reproduction in other forums is permitted, provided the original author(s) and the copyright owner(s) are credited and that the original publication in this journal is cited, in accordance with accepted academic practice. No use, distribution or reproduction is permitted which does not comply with these terms.
*Correspondence: Can Liao, Y2FubGlhbzYwMDhAMTYzLmNvbQ==; Shilei Pan, MTM2MDI4ODI5MThAMTYzLmNvbQ==
†These authors have contributed equally to this work
Disclaimer: All claims expressed in this article are solely those of the authors and do not necessarily represent those of their affiliated organizations, or those of the publisher, the editors and the reviewers. Any product that may be evaluated in this article or claim that may be made by its manufacturer is not guaranteed or endorsed by the publisher.
Research integrity at Frontiers
Learn more about the work of our research integrity team to safeguard the quality of each article we publish.