- 1Department of Immunology, Rady Faculty of Health Sciences, University of Manitoba, Winnipeg, MB, Canada
- 2Department of Medical Microbiology and Infectious Diseases, Rady Faculty of Health Sciences, University of Manitoba, Winnipeg, MB, Canada
- 3Centre for the AIDS Programme of Research in South Africa (CAPRISA), Durban, South Africa
The risk of HIV acquisition is low on a per-contact basis but increased by transmission co-factors such as other sexually transmitted infections (STIs). Human papillomavirus (HPV) is a prevalent STI that most individuals will acquire HPV in their lifetime. Current HPV vaccines can prevent newly acquired infections, but are largely ineffective against established HPV, complicating worldwide eradication efforts. In addition to being the causative agent of cervical cancer, accumulating evidence suggests that HPV infection and/or accompanying cervical inflammation increase the risk of HIV infection in men and women. The fact that immunological features observed during HPV infection overlap with cellular and molecular pathways known to enhance HIV susceptibility underscore the potential interplay between these two viral infections that fuel their mutual spread. Here we review current insights into how HPV infection and the generation of anti-HPV immunity contribute to higher HIV transmission rates, and the impact of HPV on mucosal inflammation, immune cell trafficking, and epithelial barrier function.
Introduction
HPV is the most common viral infection of reproductive tracts and the vast majority of sexually active individuals will acquire this virus at some point in their lives (Chesson et al., 2014). At 4 years following the first sexual intercourse, more than 50% of young women will become infected with a cervical HPV virus (Brianti et al., 2017). A majority of HPV infections are cleared without treatment within a few months of acquisition, and 90% of the infections are cleared within 2 years. Approximately 60% of infections will induce seroconversion and cervical samples will display mild (if any) abnormalities (Schiffman et al., 2007). However, in some cases of apparent HPV clearance it is believed that a state of latency is established, where immune control lowers viral replication to undetectable levels without achieving complete eradication (Liu et al., 2014; Shew et al., 2015; Fu et al., 2016). The difficulty in defining HPV infections as newly acquired, re-activation of latent virus, temporary deposition by an HPV-positive partner, or auto-inoculation from other sites, remains an epidemiological and clinical challenge (Strickler et al., 2005; Baay et al., 2011; Fu et al., 2015). While cervical lesions can be treated through electrocoagulation, cryotherapy, laser ablation or local surgery, when undiagnosed infection persists, there is an increased chance of developing pre-cancerous lesions (McCredie et al., 2008; Asiaf et al., 2014).
Despite availability of several effective, prophylactic HPV vaccines, the burden of HPV-associated pathology and cancer remains high (Kombe Kombe et al., 2020). The two most widely used prophylactic HPV vaccines are “Gardasil”, which provides protection against HPV 6, 11, 16, 18, 31, 33, 45, 52, and 58, and “Cervarix”, which provides protection against HPV 16 and 18 (Asiaf et al., 2014). These vaccines are prepared from purified virus-like particles (VLPs) of the highly immunogenic major capsid (L1) protein and delivered intramuscularly, inducing strong antibody responses in nearly all immunized individuals (Yang et al., 2004; Yan et al., 2005). While anti-HPV antibody responses during natural infection are poor, HPV vaccines are able to elicit high neutralizing antibody titres against the L1 capsid that are 10-10,000 times higher than during natural infection (Harro et al., 2001; Garland et al., 2007; Paavonen et al., 2009). Barriers to vaccine uptake, including misinformation, vaccine mistrust, social stigma, and vaccine availability, have limited many countries from reaching the recommended coverage of >80% (Bigaard and Franceschi, 2021). Additionally, the ability of HPV to induce latency complicates eradication efforts worldwide, as current vaccines are not effective against type-matched infections acquired prior to vaccination. Despite induction of strong cell-mediated immunity during HPV infection, it remains controversial whether sterilizing immunity is achieved or whether virus enters a latent state with the possibility of reactivating disease at a later time (Gravitt, 2012; Maglennon et al., 2014; Wang et al., 2015; Hinten et al., 2017). In this mini-review, we will discuss how HPV pathology and the underlying immune responses against infection alter the mucosal landscape that can favor acquisition of other STIs such as HIV. The ability of HPV to establish a chronic infection within the genital mucosal epithelium has implications on HIV acquisition risk and prevention strategies, and remains an area of active research, in particular with novel therapeutic vaccines reaching trial stages in 2021 (Ayesha et al., 2021).
The Natural History of HPV Infection
Human papillomaviruses belong to the Papillomaviridae family and there are over 200 genotypes of HPV which are divided into low-risk HPV, causing anogenital and cutaneous warts or no obvious disease, and high-risk HPV, which in some cases lead to oropharyngeal and anogenital cancers (Forman et al., 2012; de Martel et al., 2012; Asiaf et al., 2014; Buchanan et al., 2016; de Martel et al., 2017; de Martel et al., 2020). Phylogenetically, HPV is classified into five genera (Bernard et al., 2010; Schiffman et al., 2016), with alpha HPV infecting mucocutaneous surfaces. While some alpha HPV present without pathology, others cause either highly productive warts or anogenital (cervical, vulvar, vaginal, penile, and anal) cancers (Brianti et al., 2017). HPV16 and HPV18 account for approximately 70% of all cervical cancers (Braaten and Laufer, 2008). HPV DNA has also been detected in oropharyngeal cancers, and some HPV have been discovered to contribute to cancers at cutaneous sites (Zhou et al., 2019). Many aspects of the natural history of HPV infection in women remain unclear due to the limitations of interpreting HPV infection status and practical limitations to conduct longitudinal studies spanning longer than 5-10 years. Typically, HPV is detected by identifying viral DNA in patient cervical samples, where DNA-negative women, tested by routine PCR, have been classified as “HPV uninfected”. Once HPV becomes undetectable, however, it is uncertain whether HPV is truly cleared, enters a latent state, or simply persists at low, undetectable levels. As the cervical epithelium constantly turns over, HPV latency may rapidly re-establish HPV infection. There is epidemiologic evidence that 3.3-19.4% of women who were observed to have cleared a specific HPV genotype subsequently experienced recurrent infection of the same HPV genotype (Strickler et al., 2005; Insinga et al., 2010; Trottier et al., 2010; Winer et al., 2011; Gravitt, 2012; Rodriguez et al., 2012). Periodical shedding of HPV (Liu et al., 2014), an increase in HPV prevalence during initiation of immune suppression (Hinten et al., 2017), and re-appearance of HPV following a negative test have all been observed (Polman et al., 2017). Additionally, whole cervix analysis utilizing the SPF10-PCR-DEIA-LiPA25 system, a broad-spectrum PCR assay which allows for amplification of 65-bp fragment in the L1 region from a wide range of HPV types, detected HPV in two patients who underwent hysterectomy unrelated to cervical abnormality and had tested HPV-negative. In patient 1, HPV31 was detected, whereas in patient 2, HPV18 and HPV53 were detected. The infection was very focal in both patients, and there was no progression to productive infection (Hammer et al., 2019). These data indicate that both patients had latent HPV infection without prior knowledge, underscoring the complexity of HPV infection in women.
The HPV Life Cycle
Human papilloma viral particles are 50-60 nm in diameter and lack a lipid envelope (Figure 1). Double stranded viral DNA is approximately 8 kbp, circular, packed with host histones and encapsulated by viral-encoded late proteins (Conway and Meyers, 2009). The HPV genome consists of 8 open reading frames, which can be divided into late (L) proteins, early (E) proteins, and an upstream regulatory region (URR) (Conway and Meyers, 2009). L1 and L2 encode for the major and minor capsid proteins, respectively. L1 consists of variable and constant regions, with the constant regions carrying the surface-specific antigenic epitopes that interact with the host membrane upon entry and are conserved among different HPV genotypes (Lowe et al., 2008; Dasgupta et al., 2011). E1 and E2 regulate the viral life cycle and viral genome replication, E4 regulates early viral gene products, and E6-7 cause cell cycle dysregulation and serve as oncogenes (Pinidis et al., 2016).
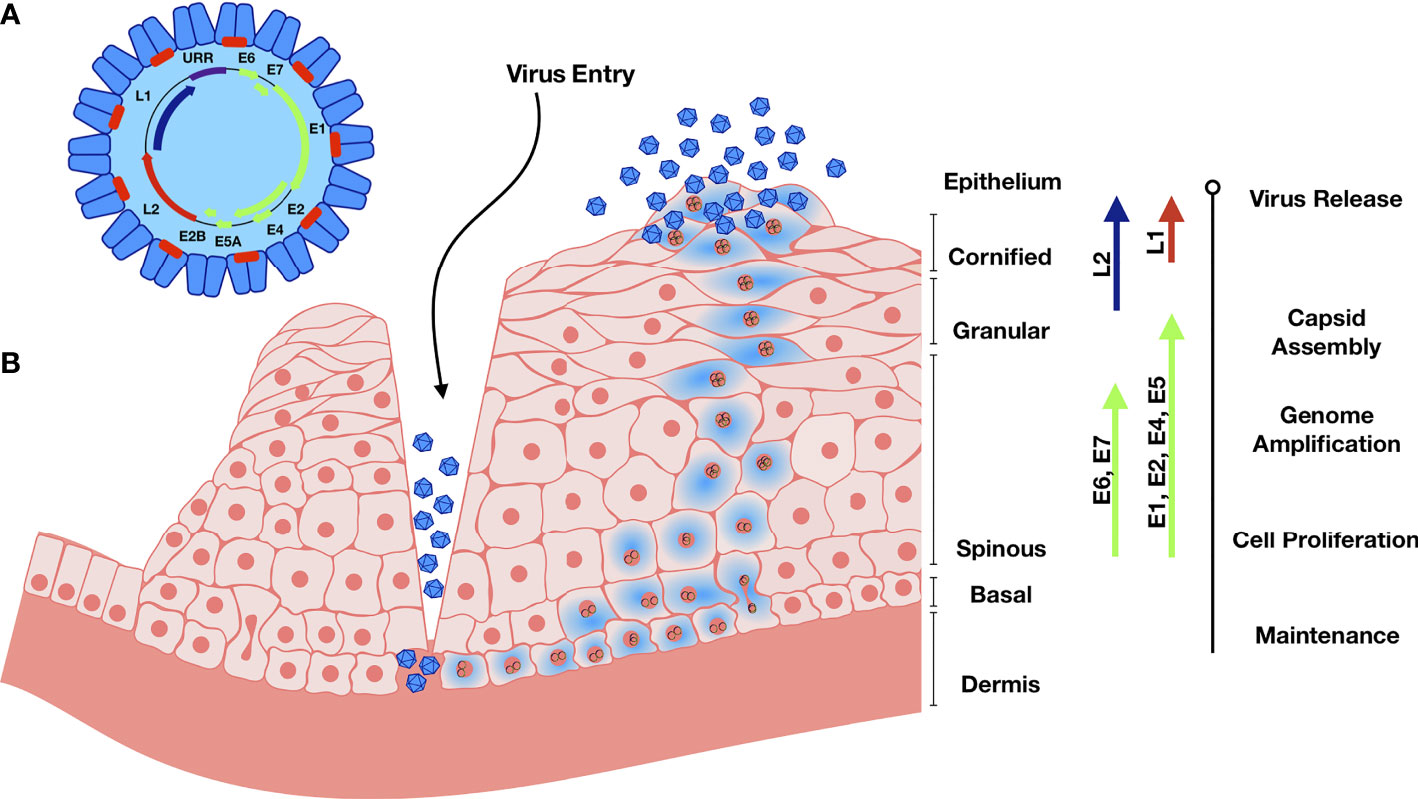
Figure 1 Genome organization and Life cycle of HPV. (A) The circular dsDNA genome is represented in the black circle. Position of early genome is represented by green, and L1 and L2 in blue and red respectively. URR – Upstream Regulatory Region. (B) Abrasion provides HPV access to basal keratinocytes. Viral DNA replication relies on the host DNA replication machinery and is supported by the early viral proteins E1 and E2. The viral genome replicates in the infected basal and stem cells and establishes HPV episome copies, which split between the daughter progeny as cells divide. The early viral proteins E6 and E7 stimulate the continued proliferation. Upon terminal cell differentiation in the upper epithelium, L1 and L2 become activated to package the high number of viral DNA copies. E4 disintegrates the cytokeratin filaments and the virus is released as keratinocyte remnants are sloughed off at the epithelial surface.
HPV has a unique mode of infection compared to other viruses. HPV reaches its target tissue, the basal cells of stratified squamous epithelium, only though micro-abrasions or epithelial trauma. Once the virions reach these cells, they cannot bind the cell surface receptor involved in internalization directly. After the basement membrane (BM) is exposed through epithelial trauma, HPV L1 major capsid protein binds the heparin sulfate proteoglycans (HSPGs) on BM (Schiller et al., 2010). This binding induces a cyclophilin B-dependent conformational change in the L1 3D structure, exposing the N-terminus of L2 minor capsid protein for cleavage by furin, a cellular proteinase (Richards et al., 2006; Bienkowska-Haba et al., 2009; Schiller et al., 2010). Proteolysis of L2 is followed by two subsequent interactions of L1 with HSPGs, resulting in further 3D conformational rearrangements of L1 and L2. These structural modifications result in the loss of bonds involved in the initial L1 interaction, resulting in viral movement to the keratinocyte zone and exposure of viral residues to the endocytic compartment to promote viral internalization by endocytosis (Schiller et al., 2010). While the infection occurs in non-differentiated cells, new viral progeny are produced primarily in terminally differentiated keratinocytes, while dividing basal cells serve as viral reservoirs (Meyers et al., 1992; McLaughlin-Drubin and Meyers, 2005; Conway and Meyers, 2009). Upon viral entry, the viral genome is transported into the nucleus and maintained as episomal DNA (McBride and Oliveira, 2006). Infection proceeds slowly, where viral transcription is initiated at 12-24 hours post-entry. Interestingly, when infected basal cells divide, two equally infected daughter cells are produced but contribute to disease progression differently: one remains in the basal layer while the other cell begins to differentiate and migrate through the upper layers of the epithelium (Lehman and Botchan, 1998; Bastien and McBride, 2000). During cell division, protein E2 tethers viral genomes to the cellular mitotic chromosomes, ensuring that the genome becomes enclosed within the nuclear envelope and segregated equally between the daughter cells (McBride and Oliveira, 2006). As the basal cell enters the differentiation process, proteins E1 and E2 form a complex with the viral DNA to recruit host DNA polymerase. E6 can bind the tumor suppressor p53 which promotes its ubiquitination and degradation (Rolfe et al., 1995). Protein E7 binds several host proteins, such as the retinoblastoma tumor suppressor family and cell-cycle regulatory proteins (Conway and Meyers, 2009). This modulation affects the cell cycle checkpoints, delays differentiation, and allows for HPV replication. E7 functions to maintain a cell mitotically active phenotype that drives expression of replication factors required for viral amplification that can reach thousands of copies per cell (Morris et al., 1993; Barksdale and Baker, 1993). Proteins L1 and L2 are then synthesized and are responsible for the viral DNA packaging and are only expressed in the terminally differentiated keratinocytes (Barksdale and Baker, 1993).
Subversion of Host Immunity by HPV: Lessons Learned From Cervical Cancer
The HPV lifecycle is exclusively intraepithelial and new viral progeny are shed from the surface of the squamous epithelium. During the late phase of infection, infected cells increase expression of capsid proteins, which are highly immunogenic (Cason et al., 1989), but are shed quickly from the outer layers of the epithelium where there are low numbers of antigen presenting cells. Viral assembly and virion release from differentiated cells occur with minimal cytopathic effects, resulting in poor recognition by the host mucosal immunity. Protective anti-HPV antibodies only develop at 6-12 months after HPV infection, if at all, suggesting that T cells are responsible for controlling early viral replication (Carter et al., 1996). CD4+ T cells are of particular importance, exemplified by patients with regressed HPV+ lesions containing significantly higher numbers of HPV16 E6 and E7-specific CD4+ T cells (Kadish et al., 1997; Dillon et al., 2007; Kim et al., 2012). HIV+ individuals that have decreased CD4+ T cell numbers are susceptible to multiple HPV infections, prolonged viral persistence, and increased risk of cervical neoplasia (Ahdieh et al., 2001; Levi et al., 2004; Palefsky et al., 2006). Patients with high-grade cervical intraepithelial neoplasia have reduced Th1-type responses, and are often impaired (Welters et al., 2006), also supporting the notion that Th1 responses are crucial during HPV infection. Th1 responses are characterized by IFNγ and IL-2 production, and the lack of these cytokines is associated with persistent HPV infection and development of high-grade disease (Clerici et al., 1997; Dupuy et al., 1997; Scott et al., 1999). Interestingly, patients with high grade HPV+ lesions have a skewed Th2 response, a shift away from Th1 that may represent a mechanism employed by HPV to blunt host responses (Clerici et al., 1997; al-Saleh et al., 1998). Similarly, Th17 cells are primarily tumorigenic in the context of HPV infection and increased Th17 cell infiltration is associated with progression to invasive cervical cancer (Walch-Ruckheim et al., 2015; Xue et al., 2018). CD8+ T cells expressing α4β7 integrin, important for homing to mucosal sites, are present in healing lesions but reduced or absent in lesions which progress to cancer (McKenzie et al., 1991).
Viral nucleic acid sensing by TLR9 is thought to be important in the initial response to intracellular HPV by keratinocytes. Interestingly, TLR9 expression is highly upregulated by HPV-infected cells during low-risk HPV infection, but marginally during high-risk HPV (Cannella et al., 2015), suggesting blockade of this innate sensing pathway by virus. Additionally, TLR gene polymorphisms may contribute to cancer susceptibility: TLR2 del allele is significantly associated with cervical cancer susceptibility and TLR2 ins/del genotype is strongly associated with tobacco usage in women with cervical cancer, while TLR4 Thr/Ile genotype was significantly associated with the early stage of cervical cancer in North Indian women (Pandey et al., 2009). No association between TLR3 and TLR9 gene polymorphisms and risk of developing cervical cancer or tobacco usage was observed (Pandey et al., 2011).
Activated keratinocytes produce antiviral and inflammatory mediators including type I interferons, IL-1, IL-6, IL-8, IL-10, IL-18, and TNF that function to recruit and stimulate a predominantly Th1-mediated immune response. Regional production of pro-inflammatory cytokines leads to the subsequent activation of Langerhans cells (LCs) and tissue resident macrophages and function to recruit CD4+, CD8+, and CD56+ cells to the site of lesion (Monnier-Benoit et al., 2006; Woo et al., 2008; Karim et al., 2013). As part of their immune evasion strategy, HPV-infected keratinocytes express low amounts of viral proteins to limit antigen presentation during the early phases of infection (Stoler et al., 1992). HPV oncoprotein E7 recruits various histone modification enzymes to suppress the transcription of TLR9 over time (Doorbar, 2005; Miller, 2008). E6 and E7 also block the pathogen recognition receptors (PRR) signal transduction, interfere with IFN-α/β receptor signalling pathway, and downregulate the NF-κB signaling pathway (Zhou et al., 2019). Lastly, HPV E7 can interact with and repress the MHC I promoter, while HPV E5 can reduce MHC I and CD1d expression by blocking the transport of these proteins to the cell surface (Zhou et al., 2019), limiting recognition by cytotoxic CD8 T cells. Together, these are several strategies employed by HPV to dampen host immunity and promote viral persistence and cancer progression. Interestingly, there are reports demonstrating that HPV can regulate the inflammatory response by manipulating the NF-κB signaling pathway and regulating the inflammatory cytokine cascade in order to induce chronic inflammation (Mangino et al., 2016). TLR9 levels have been recently demonstrated to be elevated in chronic HPV infections (Cannella et al., 2015), suggesting that TLR9 inhibition by virus may not be complete in some cases. In this scenario, sustained TLR9 expression without proper antiviral response can also drive chronic inflammation, leaving the epithelial barrier vulnerable to other infections and promote cancer progression (Figure 2) (Cannella et al., 2015).
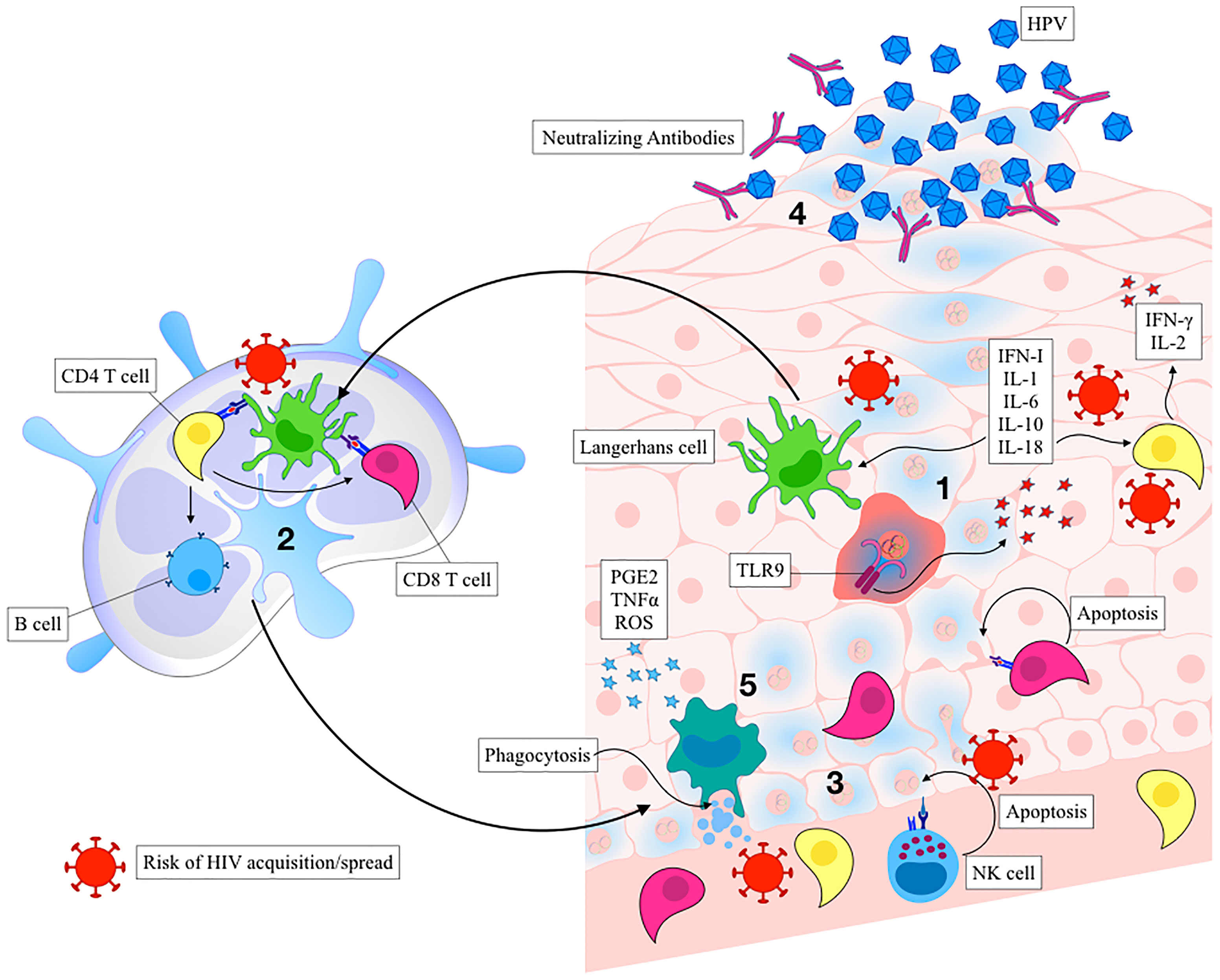
Figure 2 Immune control of HPV infection and the risk of HIV acquisition. Activated keratinocytes trigger the release of pro-inflammatory cytokines and chemokines leading to recruitment of Langerhans cells and other antigen-presenting cells (APCs) (Step 1). Inflammation is a known risk for HIV acquisition. Activated APCs process the antigen and migrate to the draining lymph node, where they help activate antigen specific CD8+, CD4+, and B cells (Step 2). HIV can utilize immune cell trafficking to spread from APCs to T Cells. The inflammatory state also activates innate immune responses, such as natural killer cells (Step 3), also associated with the increased risk of HIV acquisition. Inflammation drives infiltration of CD8+ T cells that kill viral-infected cells, and CD4+ T that contribute to viral clearance while also serving as HIV target cells. Plasma cells secrete neutralizing antibodies that function to neutralize cell-free HPV, preventing further infectious spread (Step 4). Macrophages and dendritic cells induce apoptosis and phagocytose apoptotic bodies, producing anti-inflammatory mediators and initiating the healing process (Step 5).
Macrophages activated by regional pro-inflammatory cytokines can kill HPV-infected cells via tumor necrosis factor (TNF) (Routes et al., 2005) or antibody-dependent cytotoxicity mediated mechanisms. The oncogene E6 prevents the release of monocyte chemotactic protein-1 (MCP-1) by normal keratinocytes in response to TNF, impairing local macrophage recruitment during HPV infection (Hacke et al., 2010). High grade HPV+ lesions are characterized by high infiltration of predominantly M2-polarized tumor-associated macrophages (TAMs) (Hammes et al., 2007; Lepique et al., 2009) and are associated with poor prognosis (Lepique et al., 2009; Davis et al., 2016). M2 TAMs may differentiate from recruited monocytes within the immunosuppressive tumor microenvironment consisting of TGF-β, IL-10, IL-6, and PGE2 (Mantovani and Sica, 2010). It is speculated that M2 polarization of TAMs leads to an insufficient production of IL-12 that is necessary for NK, NKT, and Th1 cell function (Gabrilovich et al., 2012). Additionally, TAMs play an important role in recruitment of Tregs via CCL22, which are further maintained and stimulated via high levels of IL-10 (Gabrilovich et al., 2012).
Interference of innate immunity delays or impairs the induction of a robust adaptive immune response to HPV infection. Upon viral insult, Langerhans cells (LCs) that reside in the epidermis normally migrate to secondary lymphoid organs and prime the adaptive immune response. HPV has been shown to impair trafficking of APCs to the lymph node, delaying the onset of the immune response. Low infiltration of LCs into HPV-associated cancerous tumors is associated with disease severity (Caberg et al., 2009; Jiang and Xue, 2015). This is possibly due to downregulation of CCL20 through the inhibition of NF-κB signaling pathway (Guess and McCance, 2005; Le Borgne et al., 2006). Additionally, E-cadherin, which allows the LCs to remain in the epidermis to capture viral particles, is downregulated in HPV-associated tumors (Guess and McCance, 2005; Hubert et al., 2005) likely through E7-mediated promoter methylation. CCR7 expression on DCs was also observed to be downregulated, resulting in their reduced homing ability to lymph nodes (Laurson et al., 2010; Pahne-Zeppenfeld et al., 2014). Altogether, impairment of the LC trafficking to the lymph node can contribute to poor priming of effector T cells through reduced presentation capacity of viral antigens. Consequently, while CD8+ T cells infiltrate HPV-associated tumors, they do not seem to impair tumor growth, indicative of sub-optimal priming (Cromme et al., 1995).
Maturation of APCs is also impacted in HPV-infected tissues, as expression of MHC and co-stimulatory molecules CD80 and CD86 are downregulated (Bashaw et al., 2017). Many types of cancers establish an immunosuppressive environment that include expression of IL-10, TGF, IL-6, prostaglandin E2 (PGE2), and granulocyte-macrophage colony-stimulation factor (GM-CSF) that can reduce DC maturation potential (Herfs et al., 2009; Bayne et al., 2012; Torres-Poveda et al., 2014; Brencicova et al., 2017; Remes Lenicov et al., 2018). When HPV 16 E7-transgenic mouse grafts are grafted onto control mice, they do not get rejected due to recruitment of DCs expressing high levels of indoleamine 2,3-dioxygenase 1 (IDO1) (Mittal et al., 2013). The mechanism of IDO1-driven immunosuppression includes generation of regulatory T cells and suppressing effector responses by depleting tryptophan, which is important for T cell function (Kobayashi et al., 2008; Mittal et al., 2013). These studies are consistent with clinical observations that HPV+ cervical lesions express high levels of IDO1 (Kobayashi et al., 2008), suggesting that IDO1+ DCs may drive cancer progression by generating Tregs and/or inducing T cell anergy.
Underlying HPV Infection Increase HIV Acquisition Risk
The per-exposure risk of HIV acquisition ranges from 0.08% for receptive vaginal sex (Boily et al., 2009) to 1.4% for receptive anal sex (Baggaley et al., 2010), but HIV transmission rates can be increased due to co-factors such as high viral load (VL), which increases the risk 26-fold (Wawer et al., 2005; Hollingsworth et al., 2008; Baeten et al., 2011). It is now well-recognized that high vaginal inflammation, defined by elevated concentrations of cervicovaginal cytokines, is strongly associated with elevated HIV acquisition risk, the underlying causes ranging from bacteria/viral sexually transmitted infections and a non-Lactobacillus, bacterial infections causing bacterial vaginosis (BV) (Ward and Ronn, 2010; Masson et al., 2015; Zevin et al., 2016; Liebenberg et al., 2017; Liebenberg et al., 2019). For example, cohort analyses of HIV-1-seronegative female sex workers in Nairobi, Kenya showed that genital ulcers and gonococcal infections are associated with an increased risk of HIV-seroconversion (Fowke et al., 1996; McKinnon et al., 2015). Similarly, HSV-2 infection is associated with a 3-fold increase in HIV acquisition risk in both men and women (Freeman et al., 2006). While high STI and HIV acquisition may be linked to increased exposures, underlying genital infections can independently increase HIV acquisition risk by disrupting mucosal barrier integrity, increased expression of neutrophil proteases and inducing an influx of CD4+ T cell populations in the genital epithelium and sub-mucosa, the prime targets of HIV during mucosal exposure (Arnold et al., 2016; McKinnon et al., 2018). Meta-analyses showed that a high proportion of HIV infections can be attributed to HPV infection of any genotype, associated with a near doubling of HIV acquisition risk in both men and women (Houlihan et al., 2012; Lissouba et al., 2013). A 2.4-fold increase in HIV infection was observed in individuals infected with either oncogenic or non-oncogenic HPV in sub-Saharan Africa, particularly in those with non-persistent infections (Averbach et al., 2010; Low et al., 2011). While biological explanations for how underlying HPV infections might increase HIV acquisition risk are incomplete, high CD4+ T cell numbers found within the stroma and epithelium are a marker for HPV lesion regression (Monnier-Benoit et al., 2006) and thereby increasing the number of susceptible target T cells for HIV (Table 1) (Mehandru et al., 2004; Veazey et al., 2008; McKinnon et al., 2011). Overlap in inflammatory mediators required for HPV clearance are also associated with high HIV acquisition risk, such as MCP-1, IL-8 and IP-10 (Masson et al., 2015; Arnold et al., 2016; Gosmann et al., 2017; McKinnon et al., 2018; Liebenberg et al., 2019). Increase in pro-inflammatory cytokine and neutrophil protease levels are associated with disruption in epithelial cell differentiation, cell-cell contacts, and epithelial barrier function and integrity (Kaul et al., 2008; Anahtar et al., 2015; Arnold et al., 2016). Increased Langerhans cells have also been shown to associate with HPV clearance (Shannon et al., 2017), and individuals with reduced LC numbers of develop HPV-positive cervical intraepithelial lesions (Mota et al., 1999). However, increased LC numbers may play a role in enhancing HIV susceptibility by binding HIV gp120 and facilitating transmission to CD4+ T cells (Arrighi et al., 2004; Koh et al., 2020). Finally, NK cells correlate with HPV clearance (Prata et al., 2015; Bjorkstrom et al., 2021), but NK cells were also shown to be biomarkers of increased HIV risk (Naranbhai et al., 2012).
Concluding Remarks
While HPV infection can cause cervical cancer in women, it is also a strong risk factor for HIV acquisition. This may have a biological basis; HPV infection induces genital inflammation, vaginal barrier disruption, and influx of activated target T cells, which have all been implicated in HIV risk. However, a clear mechanistic understanding of the factors that directly facilitate HIV acquisition is lacking. The fact that HPV can establish a latent state and re-establish infection complicates HIV prevention strategies in high-risk individuals, prompting the need for a better understanding of the cellular and molecular basis of anti-HPV immune control. Recent characterization of a mouse papillomavirus to study mucosal disease progression, host immune control and cervical cancer development in vivo would be an invaluable tool to address some of these outstanding questions (Cladel et al., 2017). Murine PV infection models can be integrated with other technologies, such as spatial transcriptomics analysis, to reveal how tissue heterogeneity and viral gene expression impacts host mucosal antiviral responses. These and other complementary approaches may identify common biological pathways activated by both HPV and HIV that can be therapeutically targeted to reduce HIV acquisition risk.
Author Contributions
Conceptualization, writing and editing, RZ, TM, and LM. Figures, RZ. Supervision, TM and LM. Funding acquisition, TM and LM. All authors contributed to the article and approved the submitted version.
Funding
This work was supported by the CIHR grant MRT-CDAA-159236 to TM and a studentship from Research Manitoba and University of Manitoba Student Fellowship to RZ. LM is supported by CIHR New Investigator Award.
Conflict of Interest
The authors declare that the research was conducted in the absence of any commercial or financial relationships that could be construed as a potential conflict of interest.
Publisher’s Note
All claims expressed in this article are solely those of the authors and do not necessarily represent those of their affiliated organizations, or those of the publisher, the editors and the reviewers. Any product that may be evaluated in this article, or claim that may be made by its manufacturer, is not guaranteed or endorsed by the publisher.
Acknowledgments
All figures were created using Keynote.
References
Ahdieh, L., Klein, R. S., Burk, R., Cu-Uvin, S., Schuman, P., Duerr, A., et al. (2001). Prevalence, Incidence, and Type-Specific Persistence of Human Papillomavirus in Human Immunodeficiency Virus (HIV)-Positive and HIV-Negative Women. J. Infect. Dis. 184 (6), 682–690. doi: 10.1086/323081
al-Saleh, W., Giannini, S. L., Jacobs, N., Moutschen, M., Doyen, J., Boniver, J., et al. (1998). Correlation of T-Helper Secretory Differentiation and Types of Antigen-Presenting Cells in Squamous Intraepithelial Lesions of the Uterine Cervix. J. Pathol. 184 (3), 283–290. doi: 10.1002/(SICI)1096-9896(199803)184:3<283::AID-PATH25>3.0.CO;2-K
Anahtar, M. N., Byrne, E. H., Doherty, K. E., Bowman, B. A., Yamamoto, H. S., Soumillon, M., et al. (2015). Cervicovaginal Bacteria Are a Major Modulator of Host Inflammatory Responses in the Female Genital Tract. Immunity 42 (5), 965–976. doi: 10.1016/j.immuni.2015.04.019
Arnold, K. B., Burgener, A., Birse, K., Romas, L., Dunphy, L. J., Shahabi, K., et al. (2016). Increased Levels of Inflammatory Cytokines in the Female Reproductive Tract Are Associated With Altered Expression of Proteases, Mucosal Barrier Proteins, and an Influx of HIV-Susceptible Target Cells. Mucosal Immunol. 9 (1), 194–205. doi: 10.1038/mi.2015.51
Arrighi, J. F., Pion, M., Wiznerowicz, M., Geijtenbeek, T. B., Garcia, E., Abraham, S., et al. (2004). Lentivirus-Mediated RNA Interference of DC-SIGN Expression Inhibits Human Immunodeficiency Virus Transmission From Dendritic Cells to T Cells. J. Virol. 78 (20), 10848–10855. doi: 10.1128/JVI.78.20.10848-10855.2004
Asiaf, A., Ahmad, S. T., Mohammad, S. O., Zargar, M. A. (2014). Review of the Current Knowledge on the Epidemiology, Pathogenesis, and Prevention of Human Papillomavirus Infection. Eur. J. Cancer Prev. 23 (3), 206–224. doi: 10.1097/CEJ.0b013e328364f273
Averbach, S. H., Gravitt, P. E., Nowak, R. G., Celentano, D. D., Dunbar, M. S., Morrison, C. S., et al. (2010). The Association Between Cervical Human Papillomavirus Infection and HIV Acquisition Among Women in Zimbabwe. AIDS 24 (7), 1035–1042. doi: 10.1097/qad.0b013e3283377973
Ayesha, N., Aboulaghras, S., Jahangeer, M., Riasat, A., Ramzan, R., Fatima, R., et al. (2021). Physiopathology and Effectiveness of Therapeutic Vaccines Against Human Papillomavirus. Environ. Sci. Pollut. Res. Int. 28 (35), 47752–47772. doi: 10.1007/s11356-021-15441-w
Baay, M. F., Francois, K., Lardon, F., Van Royen, P., Pauwels, P., Vermorken, J. B., et al. (2011). The Presence of Y Chromosomal Deoxyribonucleic Acid in the Female Vaginal Swab: Possible Implications for Human Papillomavirus Testing. Cancer Epidemiol. 35 (1), 101–103. doi: 10.1016/j.canep.2010.10.005
Baeten, J. M., Kahle, E., Lingappa, J. R., Coombs, R. W., Delany-Moretlwe, S., Nakku-Joloba, E., et al. (2011). Genital HIV-1 RNA Predicts Risk of Heterosexual HIV-1 Transmission. Sci. Transl. Med. 3 (77), 77ra29. doi: 10.1126/scitranslmed.3001888
Baggaley, R. F., White, R. G., Boily, M. C. (2010). HIV Transmission Risk Through Anal Intercourse: Systematic Review, Meta-Analysis and Implications for HIV Prevention. Int. J. Epidemiol. 39 (4), 1048–1063. doi: 10.1093/ije/dyq057
Barksdale, S. K., Baker, C. C. (1993). Differentiation-Specific Expression From the Bovine Papillomavirus Type 1 P2443 and Late Promoters. J. Virol. 67 (9), 5605–5616. doi: 10.1128/JVI.67.9.5605-5616.1993
Bashaw, A. A., Leggatt, G. R., Chandra, J., Tuong, Z. K., Frazer, I. H. (2017). Modulation of Antigen Presenting Cell Functions During Chronic HPV Infection. Papillomavirus Res. 4, 58–65. doi: 10.1016/j.pvr.2017.08.002
Bastien, N., McBride, A. A. (2000). Interaction of the Papillomavirus E2 Protein With Mitotic Chromosomes. Virology 270 (1), 124–134. doi: 10.1006/viro.2000.0265
Bayne, L. J., Beatty, G. L., Jhala, N., Clark, C. E., Rhim, A. D., Stanger, B. Z., et al. (2012). Tumor-Derived Granulocyte-Macrophage Colony-Stimulating Factor Regulates Myeloid Inflammation and T Cell Immunity in Pancreatic Cancer. Cancer Cell 21 (6), 822–835. doi: 10.1016/j.ccr.2012.04.025
Bernard, H. U., Burk, R. D., Chen, Z., van Doorslaer, K., Zur Hausen, H., De Villiers, E. M. (2010). Classification of Papillomaviruses (Pvs) Based on 189 PV Types and Proposal of Taxonomic Amendments. Virology 401 (1), 70–79. doi: 10.1016/j.virol.2010.02.002
Bienkowska-Haba, M., Patel, H. D., Sapp, M. (2009). Target Cell Cyclophilins Facilitate Human Papillomavirus Type 16 Infection. PloS Pathog. 5 (7), e1000524. doi: 10.1371/journal.ppat.1000524
Bigaard, J., Franceschi, S. (2021). Vaccination Against HPV: Boosting Coverage and Tackling Misinformation. Mol. Oncol. 15 (3), 770–778. doi: 10.1002/1878-0261.12808
Bjorkstrom, N. K., Strunz, B., Ljunggren, H. G. (2021). Natural Killer Cells in Antiviral Immunity. Nat. Rev. Immunol. 11:1–12. doi: 10.1038/s41577-021-00558-3
Boily, M. C., Baggaley, R. F., Wang, L., Masse, B., White, R. G., Hayes, R. J., et al. (2009). Heterosexual Risk of HIV-1 Infection Per Sexual Act: Systematic Review and Meta-Analysis of Observational Studies. Lancet Infect. Dis. 9 (2), 118–129. doi: 10.1016/S1473-3099(09)70021-0
Braaten, K. P., Laufer, M. R. (2008). Human Papillomavirus (HPV), HPV-Related Disease, and the HPV Vaccine. Rev. Obstet. Gynecol. 1 (1), 2–10.
Brencicova, E., Jagger, A. L., Evans, H. G., Georgouli, M., Laios, A., Attard Montalto, S., et al. (2017). Interleukin-10 and Prostaglandin E2 Have Complementary But Distinct Suppressive Effects on Toll-Like Receptor-Mediated Dendritic Cell Activation in Ovarian Carcinoma. PloS One 12 (4), e0175712. doi: 10.1371/journal.pone.0175712
Brianti, P., De Flammineis, E., Mercuri, S. R. (2017). Review of HPV-Related Diseases and Cancers. New Microbiol. 40 (2), 80–85.
Buchanan, T. R., Graybill, W. S., Pierce, J. Y. (2016). Morbidity and Mortality of Vulvar and Vaginal Cancers: Impact of 2-, 4-, and 9-Valent HPV Vaccines. Hum. Vaccin. Immunother. 12 (6), 1352–1356. doi: 10.1080/21645515.2016.1147634
Caberg, J. H., Hubert, P., Herman, L., Herfs, M., Roncarati, P., Boniver, J., et al. (2009). Increased Migration of Langerhans Cells in Response to HPV16 E6 and E7 Oncogene Silencing: Role of CCL20. Cancer Immunol. Immunother. 58 (1), 39–47. doi: 10.1007/s00262-008-0522-5
Cannella, F., Pierangeli, A., Scagnolari, C., Cacciotti, G., Tranquilli, G., Stentella, P., et al. (2015). TLR9 Is Expressed in Human Papillomavirus-Positive Cervical Cells and Is Overexpressed in Persistent Infections. Immunobiology 220 (3), 363–368. doi: 10.1016/j.imbio.2014.10.012
Carter, J. J., Koutsky, L. A., Wipf, G. C., Christensen, N. D., Lee, S. K., Kuypers, J., et al. (1996). The Natural History of Human Papillomavirus Type 16 Capsid Antibodies Among a Cohort of University Women. J. Infect. Dis. 174 (5), 927–936. doi: 10.1093/infdis/174.5.927
Cason, J., Patel, D., Naylor, J., Lunney, D., Shepherd, P. S., Best, J. M., et al. (1989). Identification of Immunogenic Regions of the Major Coat Protein of Human Papillomavirus Type 16 That Contain Type-Restricted Epitopes. J. Gen. Virol. 70 (Pt 11), 2973–2987. doi: 10.1099/0022-1317-70-11-2973
Chesson, H. W., Dunne, E. F., Hariri, S., Markowitz, L. E. (2014). The Estimated Lifetime Probability of Acquiring Human Papillomavirus in the United States. Sex Transm. Dis. 41 (11), 660–664. doi: 10.1097/OLQ.0000000000000193
Cladel, N. M., Budgeon, L. R., Balogh, K. K., Cooper, T. K., Brendle, S. A., Christensen, N. D., et al. (2017). Mouse Papillomavirus Infection Persists in Mucosal Tissues of an Immunocompetent Mouse Strain and Progresses to Cancer. Sci. Rep. 7 (1), 16932. doi: 10.1038/s41598-017-17089-4
Clerici, M., Merola, M., Ferrario, E., Trabattoni, D., Villa, M. L., Stefanon, B., et al. (1997). Cytokine Production Patterns in Cervical Intraepithelial Neoplasia: Association With Human Papillomavirus Infection. J. Natl. Cancer Inst. 89 (3), 245–250. doi: 10.1093/jnci/89.3.245
Conway, M. J., Meyers, C. (2009). Replication and Assembly of Human Papillomaviruses. J. Dent. Res. 88 (4), 307–317. doi: 10.1177/0022034509333446
Cromme, F. V., Walboomers, J. M., Van Oostveen, J. W., Stukart, M. J., De Gruijl, T. D., Kummer, J. A., et al. (1995). Lack of Granzyme Expression in T Lymphocytes Indicates Poor Cytotoxic T Lymphocyte Activation in Human Papillomavirus-Associated Cervical Carcinomas. Int. J. Gynecol. Cancer 5 (5), 366–373. doi: 10.1046/j.1525-1438.1995.05050366.x
Dasgupta, J., Bienkowska-Haba, M., Ortega, M. E., Patel, H. D., Bodevin, S., Spillmann, D., et al. (2011). Structural Basis of Oligosaccharide Receptor Recognition by Human Papillomavirus. J. Biol. Chem. 286 (4), 2617–2624. doi: 10.1074/jbc.M110.160184
Davis, R. J., Van Waes, C., Allen, C. T. (2016). Overcoming Barriers to Effective Immunotherapy: Mdscs, Tams, and Tregs as Mediators of the Immunosuppressive Microenvironment in Head and Neck Cancer. Oral. Oncol. 58, 59–70. doi: 10.1016/j.oraloncology.2016.05.002
de Martel, C., Ferlay, J., Franceschi, S., Vignat, J., Bray, F., Forman, D., et al. (2012). Global Burden of Cancers Attributable to Infections in 2008: A Review and Synthetic Analysis. Lancet Oncol. 13 (6), 607–615. doi: 10.1016/S1470-2045(12)70137-7
de Martel, C., Georges, D., Bray, F., Ferlay, J., Clifford, G. M. (2020). Global Burden of Cancer Attributable to Infections in 2018: A Worldwide Incidence Analysis. Lancet Glob. Health 8 (2), e180–ee90. doi: 10.1016/S2214-109X(19)30488-7
de Martel, C., Plummer, M., Vignat, J., Franceschi, S. (2017). Worldwide Burden of Cancer Attributable to HPV by Site, Country and HPV Type. Int. J. Cancer 141 (4), 664–670. doi: 10.1002/ijc.30716
Dillon, S., Sasagawa, T., Crawford, A., Prestidge, J., Inder, M. K., Jerram, J., et al. (2007). Resolution of Cervical Dysplasia Is Associated With T-Cell Proliferative Responses to Human Papillomavirus Type 16 E2. J. Gen. Virol. 88 (Pt 3), 803–813. doi: 10.1099/vir.0.82678-0
Doorbar, J. (2005). The Papillomavirus Life Cycle. J. Clin. Virol. 32 (Suppl 1), S7–15. doi: 10.1016/j.jcv.2004.12.006
Dupuy, C., Buzoni-Gatel, D., Touze, A., Le Cann, P., Bout, D., Coursaget, P. (1997). Cell Mediated Immunity Induced in Mice by HPV 16 L1 Virus-Like Particles. Microb. Pathog. 22 (4), 219–225. doi: 10.1006/mpat.1996.0113
Forman, D., de Martel, C., Lacey, C. J., Soerjomataram, I., Lortet-Tieulent, J., Bruni, L., et al. (2012). Global Burden of Human Papillomavirus and Related Diseases. Vaccine 30 (Suppl 5), F12–F23. doi: 10.1016/j.vaccine.2012.07.055
Fowke, K. R., Nagelkerke, N. J., Kimani, J., Simonsen, J. N., Anzala, A. O., Bwayo, J. J., et al. (1996). Resistance to HIV-1 Infection Among Persistently Seronegative Prostitutes in Nairobi, Kenya. Lancet 348 (9038), 1347–1351. doi: 10.1016/S0140-6736(95)12269-2
Freeman, E. E., Weiss, H. A., Glynn, J. R., Cross, P. L., Whitworth, J. A., Hayes, R. J. (2006). Herpes Simplex Virus 2 Infection Increases HIV Acquisition in Men and Women: Systematic Review and Meta-Analysis of Longitudinal Studies. AIDS 20 (1), 73–83. doi: 10.1097/01.aids.0000198081.09337.a7
Fu, T. C., Carter, J. J., Hughes, J. P., Feng, Q., Hawes, S. E., Schwartz, S. M., et al. (2016). Re-Detection vs. New Acquisition of High-Risk Human Papillomavirus in Mid-Adult Women. Int. J. Cancer 139 (10), 2201–2212. doi: 10.1002/ijc.30283
Fu, T. C., Hughes, J. P., Feng, Q., Hulbert, A., Hawes, S. E., Xi, L. F., et al. (2015). Epidemiology of Human Papillomavirus Detected in the Oral Cavity and Fingernails of Mid-Adult Women. Sex Transm. Dis. 42 (12), 677–685. doi: 10.1097/OLQ.0000000000000362
Gabrilovich, D. I., Ostrand-Rosenberg, S., Bronte, V. (2012). Coordinated Regulation of Myeloid Cells by Tumours. Nat. Rev. Immunol. 12 (4), 253–268. doi: 10.1038/nri3175
Garland, S. M., Hernandez-Avila, M., Wheeler, C. M., Perez, G., Harper, D. M., Leodolter, S., et al. (2007). Quadrivalent Vaccine Against Human Papillomavirus to Prevent Anogenital Diseases. N. Engl. J. Med. 356 (19), 1928–1943. doi: 10.1056/NEJMoa061760
Gosmann, C., Anahtar, M. N., Handley, S. A., Farcasanu, M., Abu-Ali, G., Bowman, B. A., et al. (2017). Lactobacillus-Deficient Cervicovaginal Bacterial Communities Are Associated With Increased HIV Acquisition in Young South African Women. Immunity 46 (1), 29–37. doi: 10.1016/j.immuni.2016.12.013
Gravitt, P. E. (2012). Evidence and Impact of Human Papillomavirus Latency. Open Virol. J. 6, 198–203. doi: 10.2174/1874357901206010198
Guess, J. C., McCance, D. J. (2005). Decreased Migration of Langerhans Precursor-Like Cells in Response to Human Keratinocytes Expressing Human Papillomavirus Type 16 E6/E7 Is Related to Reduced Macrophage Inflammatory Protein-3alpha Production. J. Virol. 79 (23), 14852–14862. doi: 10.1128/JVI.79.23.14852-14862.2005
Hacke, K., Rincon-Orozco, B., Buchwalter, G., Siehler, S. Y., Wasylyk, B., Wiesmuller, L., et al. (2010). Regulation of MCP-1 Chemokine Transcription by P53. Mol. Cancer 9, 82. doi: 10.1186/1476-4598-9-82
Hammer, A., de Koning, M. N., Blaakaer, J., Steiniche, T., Doorbar, J., Griffin, H., et al. (2019). Whole Tissue Cervical Mapping of HPV Infection: Molecular Evidence for Focal Latent HPV Infection in Humans. Papillomavirus Res. 7, 82–87. doi: 10.1016/j.pvr.2019.02.004
Hammes, L. S., Tekmal, R. R., Naud, P., Edelweiss, M. I., Kirma, N., Valente, P. T., et al. (2007). Macrophages, Inflammation and Risk of Cervical Intraepithelial Neoplasia (CIN) Progression–Clinicopathological Correlation. Gynecol. Oncol. 105 (1), 157–165. doi: 10.1016/j.ygyno.2006.11.023
Harro, C. D., Pang, Y. Y., Roden, R. B., Hildesheim, A., Wang, Z., Reynolds, M. J., et al. (2001). Safety and Immunogenicity Trial in Adult Volunteers of a Human Papillomavirus 16 L1 Virus-Like Particle Vaccine. J. Natl. Cancer Inst. 93 (4), 284–292. doi: 10.1093/jnci/93.4.284
Herfs, M., Herman, L., Hubert, P., Minner, F., Arafa, M., Roncarati, P., et al. (2009). High Expression of PGE2 Enzymatic Pathways in Cervical (Pre)Neoplastic Lesions and Functional Consequences for Antigen-Presenting Cells. Cancer Immunol. Immunother. 58 (4), 603–614. doi: 10.1007/s00262-008-0584-4
Hinten, F., Hilbrands, L. B., Meeuwis, K. A. P., IntHout, J., Quint, W. G. V., Hoitsma, A. J., et al. (2017). Reactivation of Latent HPV Infections After Renal Transplantation. Am. J. Transplant. 17 (6), 1563–1573. doi: 10.1111/ajt.14181
Hollingsworth, T. D., Anderson, R. M., Fraser, C. (2008). HIV-1 Transmission, by Stage of Infection. J. Infect. Dis. 198 (5), 687–693. doi: 10.1086/590501
Houlihan, C. F., Larke, N. L., Watson-Jones, D., Smith-McCune, K. K., Shiboski, S., Gravitt, P. E., et al. (2012). Human Papillomavirus Infection and Increased Risk of HIV Acquisition. A Systematic Review and Meta-Analysis. AIDS 26 (17), 2211–2222. doi: 10.1097/QAD.0b013e328358d908
Hubert, P., Caberg, J. H., Gilles, C., Bousarghin, L., Franzen-Detrooz, E., Boniver, J., et al. (2005). E-Cadherin-Dependent Adhesion of Dendritic and Langerhans Cells to Keratinocytes Is Defective in Cervical Human Papillomavirus-Associated (Pre)Neoplastic Lesions. J. Pathol. 206 (3), 346–355. doi: 10.1002/path.1771
Insinga, R. P., Perez, G., Wheeler, C. M., Koutsky, L. A., Garland, S. M., Leodolter, S., et al. (2010). Incidence, Duration, and Reappearance of Type-Specific Cervical Human Papillomavirus Infections in Young Women. Cancer Epidemiol. Biomarkers Prev. 19 (6), 1585–1594. doi: 10.1158/1055-9965.EPI-09-1235
Jiang, B., Xue, M. (2015). Correlation of E6 and E7 Levels in High-Risk HPV16 Type Cervical Lesions With CCL20 and Langerhans Cells. Genet. Mol. Res. 14 (3), 10473–10481. doi: 10.4238/2015.September.8.8
Kadish, A. S., Ho, G. Y., Burk, R. D., Wang, Y., Romney, S. L., Ledwidge, R., et al. (1997). Lymphoproliferative Responses to Human Papillomavirus (HPV) Type 16 Proteins E6 and E7: Outcome of HPV Infection and Associated Neoplasia. J. Natl. Cancer Inst. 89 (17), 1285–1293. doi: 10.1093/jnci/89.17.1285
Karim, R., Tummers, B., Meyers, C., Biryukov, J. L., Alam, S., Backendorf, C., et al. (2013). Human Papillomavirus (HPV) Upregulates the Cellular Deubiquitinase UCHL1 to Suppress the Keratinocyte’s Innate Immune Response. PloS Pathog. 9 (5), e1003384. doi: 10.1371/journal.ppat.1003384
Kaul, R., Rebbapragada, A., Hirbod, T., Wachihi, C., Ball, T. B., Plummer, F. A., et al. (2008). Genital Levels of Soluble Immune Factors With Anti-HIV Activity may Correlate With Increased HIV Susceptibility. AIDS 22 (15), 2049–2051. doi: 10.1097/QAD.0b013e328311ac65
Kim, K. H., Greenfield, W. W., Cannon, M. J., Coleman, H. N., Spencer, H. J., Nakagawa, M. (2012). CD4+ T-Cell Response Against Human Papillomavirus Type 16 E6 Protein Is Associated With a Favorable Clinical Trend. Cancer Immunol. Immunother. 61 (1), 63–70. doi: 10.1007/s00262-011-1092-5
Kobayashi, A., Weinberg, V., Darragh, T., Smith-McCune, K. (2008). Evolving Immunosuppressive Microenvironment During Human Cervical Carcinogenesis. Mucosal Immunol. 1 (5), 412–420. doi: 10.1038/mi.2008.33
Koh, W. H., Lopez, P., Ajibola, O., Parvarchian, R., Mohammad, U., Hnatiuk, R., et al. (2020). HIV-Captured Dcs Regulate T Cell Migration and Cell-Cell Contact Dynamics to Enhance Viral Spread. iScience 23 (8), 101427. doi: 10.1016/j.isci.2020.101427
Kombe Kombe, A. J., Li, B., Zahid, A., Mengist, H. M., Bounda, G. A., Zhou, Y., et al. (2020). Epidemiology and Burden of Human Papillomavirus and Related Diseases, Molecular Pathogenesis, and Vaccine Evaluation. Front. Public Health 8, 552028. doi: 10.3389/fpubh.2020.552028
Laurson, J., Khan, S., Chung, R., Cross, K., Raj, K. (2010). Epigenetic Repression of E-Cadherin by Human Papillomavirus 16 E7 Protein. Carcinogenesis 31 (5), 918–926. doi: 10.1093/carcin/bgq027
Le Borgne, M., Etchart, N., Goubier, A., Lira, S. A., Sirard, J. C., van Rooijen, N., et al. (2006). Dendritic Cells Rapidly Recruited Into Epithelial Tissues via CCR6/CCL20 Are Responsible for CD8+ T Cell Crosspriming In Vivo. Immunity 24 (2), 191–201. doi: 10.1016/j.immuni.2006.01.005
Lehman, C. W., Botchan, M. R. (1998). Segregation of Viral Plasmids Depends on Tethering to Chromosomes and Is Regulated by Phosphorylation. Proc. Natl. Acad. Sci. U. S. A. 95 (8), 4338–4343. doi: 10.1073/pnas.95.8.4338
Lepique, A. P., Daghastanli, K. R., Cuccovia, I. M., Villa, L. L. (2009). HPV16 Tumor Associated Macrophages Suppress Antitumor T Cell Responses. Clin. Cancer Res. 15 (13), 4391–4400. doi: 10.1158/1078-0432.CCR-09-0489
Levi, J. E., Fernandes, S., Tateno, A. F., Motta, E., Lima, L. P., Eluf-Neto, J., et al. (2004). Presence of Multiple Human Papillomavirus Types in Cervical Samples From HIV-Infected Women. Gynecol. Oncol. 92 (1), 225–231. doi: 10.1016/j.ygyno.2003.10.004
Liebenberg, L. J., Masson, L., Arnold, K. B., McKinnon, L. R., Werner, L., Proctor, E., et al. (2017). Genital-Systemic Chemokine Gradients and the Risk of HIV Acquisition in Women. J. Acquir. Immune Defic. Syndr. 74 (3), 318–325. doi: 10.1097/QAI.0000000000001218
Liebenberg, L. J. P., McKinnon, L. R., Yende-Zuma, N., Garrett, N., Baxter, C., Kharsany, A. B. M., et al. (2019). HPV Infection and the Genital Cytokine Milieu in Women at High Risk of HIV Acquisition. Nat. Commun. 10 (1), 5227. doi: 10.1038/s41467-019-13089-2
Lissouba, P., Van de Perre, P., Auvert, B. (2013). Association of Genital Human Papillomavirus Infection With HIV Acquisition: A Systematic Review and Meta-Analysis. Sex Transm. Infect. 89 (5), 350–356. doi: 10.1136/sextrans-2011-050346
Liu, S. H., Cummings, D. A., Zenilman, J. M., Gravitt, P. E., Brotman, R. M. (2014). Characterizing the Temporal Dynamics of Human Papillomavirus DNA Detectability Using Short-Interval Sampling. Cancer Epidemiol. Biomarkers Prev. 23 (1), 200–208. doi: 10.1158/1055-9965.EPI-13-0666
Low, A. J., Clayton, T., Konate, I., Nagot, N., Ouedraogo, A., Huet, C., et al. (2011). Genital Warts and Infection With Human Immunodeficiency Virus in High-Risk Women in Burkina Faso: A Longitudinal Study. BMC Infect. Dis. 11, 20. doi: 10.1186/1471-2334-11-20
Lowe, J., Panda, D., Rose, S., Jensen, T., Hughes, W. A., Tso, F. Y., et al. (2008). Evolutionary and Structural Analyses of Alpha-Papillomavirus Capsid Proteins Yields Novel Insights Into L2 Structure and Interaction With L1. Virol. J. 5, 150. doi: 10.1186/1743-422X-5-150
Maglennon, G. A., McIntosh, P. B., Doorbar, J. (2014). Immunosuppression Facilitates the Reactivation of Latent Papillomavirus Infections. J. Virol. 88 (1), 710–716. doi: 10.1128/JVI.02589-13
Mangino, G., Chiantore, M. V., Iuliano, M., Fiorucci, G., Romeo, G. (2016). Inflammatory Microenvironment and Human Papillomavirus-Induced Carcinogenesis. Cytokine Growth Factor Rev. 30, 103–111. doi: 10.1016/j.cytogfr.2016.03.007
Mantovani, A., Sica, A. (2010). Macrophages, Innate Immunity and Cancer: Balance, Tolerance, and Diversity. Curr. Opin. Immunol. 22 (2), 231–237. doi: 10.1016/j.coi.2010.01.009
Masson, L., Passmore, J. A., Liebenberg, L. J., Werner, L., Baxter, C., Arnold, K. B., et al. (2015). Genital Inflammation and the Risk of HIV Acquisition in Women. Clin. Infect. Dis. 61 (2), 260–269. doi: 10.1093/cid/civ298
McBride, A. A., Oliveira, J. G. (2006). Mcphillips MG. Partitioning Viral Genomes in Mitosis: Same Idea, Different Targets. Cell Cycle 5 (14), 1499–1502. doi: 10.4161/cc.5.14.3094
McCredie, M. R., Sharples, K. J., Paul, C., Baranyai, J., Medley, G., Jones, R. W., et al. (2008). Natural History of Cervical Neoplasia and Risk of Invasive Cancer in Women With Cervical Intraepithelial Neoplasia 3: A Retrospective Cohort Study. Lancet Oncol. 9 (5), 425–434. doi: 10.1016/S1470-2045(08)70103-7
McKenzie, J., King, A., Hare, J., Fulford, T., Wilson, B., Stanley, M. (1991). Immunocytochemical Characterization of Large Granular Lymphocytes in Normal Cervix and HPV Associated Disease. J. Pathol. 165 (1), 75–80. doi: 10.1002/path.1711650112
McKinnon, L. R., Izulla, P., Nagelkerke, N., Munyao, J., Wanjiru, T., Shaw, S. Y., et al. (2015). Risk Factors for HIV Acquisition in a Prospective Nairobi-Based Female Sex Worker Cohort. AIDS Behav. 19 (12), 2204–2213. doi: 10.1007/s10461-015-1118-7
McKinnon, L. R., Liebenberg, L. J., Yende-Zuma, N., Archary, D., Ngcapu, S., Sivro, A., et al. (2018). Genital Inflammation Undermines the Effectiveness of Tenofovir Gel in Preventing HIV Acquisition in Women. Nat. Med. 24 (4), 491–496. doi: 10.1038/nm.4506
McKinnon, L. R., Nyanga, B., Chege, D., Izulla, P., Kimani, M., Huibner, S., et al. (2011). Characterization of a Human Cervical CD4+ T Cell Subset Coexpressing Multiple Markers of HIV Susceptibility. J. Immunol. 187 (11), 6032–6042. doi: 10.4049/jimmunol.1101836
McLaughlin-Drubin, M. E., Meyers, C. (2005). Propagation of Infectious, High-Risk HPV in Organotypic “Raft” Culture. Methods Mol. Med. 119, 171–186. doi: 10.1385/1-59259-982-6:171
Mehandru, S., Poles, M. A., Tenner-Racz, K., Horowitz, A., Hurley, A., Hogan, C., et al. (2004). Primary HIV-1 Infection Is Associated With Preferential Depletion of CD4+ T Lymphocytes From Effector Sites in the Gastrointestinal Tract. J. Exp. Med. 200 (6), 761–770. doi: 10.1084/jem.20041196
Meyers, C., Frattini, M. G., Hudson, J. B., Laimins, L. A. (1992). Biosynthesis of Human Papillomavirus From a Continuous Cell Line Upon Epithelial Differentiation. Science 257 (5072), 971–973. doi: 10.1126/science.1323879
Miller, L. S. (2008). Toll-Like Receptors in Skin. Adv. Dermatol. 24, 71–87. doi: 10.1016/j.yadr.2008.09.004
Mittal, D., Kassianos, A. J., Tran, L. S., Bergot, A. S., Gosmann, C., Hofmann, J., et al. (2013). Indoleamine 2,3-Dioxygenase Activity Contributes to Local Immune Suppression in the Skin Expressing Human Papillomavirus Oncoprotein E7. J. Invest. Dermatol. 133 (12), 2686–2694. doi: 10.1038/jid.2013.222
Monnier-Benoit, S., Mauny, F., Riethmuller, D., Guerrini, J. S., Capilna, M., Felix, S., et al. (2006). Immunohistochemical Analysis of CD4+ and CD8+ T-Cell Subsets in High Risk Human Papillomavirus-Associated Pre-Malignant and Malignant Lesions of the Uterine Cervix. Gynecol. Oncol. 102 (1), 22–31. doi: 10.1016/j.ygyno.2005.11.039
Morris, J. D., Crook, T., Bandara, L. R., Davies, R., LaThangue, N. B., Vousden, K. H. (1993). Human Papillomavirus Type 16 E7 Regulates E2F and Contributes to Mitogenic Signalling. Oncogene 8 (4), 893–898.
Mota, F., Rayment, N., Chong, S., Singer, A., Chain, B. (1999). The Antigen-Presenting Environment in Normal and Human Papillomavirus (HPV)-Related Premalignant Cervical Epithelium. Clin. Exp. Immunol. 116 (1), 33–40. doi: 10.1046/j.1365-2249.1999.00826.x
Naranbhai, V., Abdool Karim, S. S., Altfeld, M., Samsunder, N., Durgiah, R., Sibeko, S., et al. (2012). Innate Immune Activation Enhances Hiv Acquisition in Women, Diminishing the Effectiveness of Tenofovir Microbicide Gel. J. Infect. Dis. 206 (7), 993–1001. doi: 10.1093/infdis/jis465
Paavonen, J., Naud, P., Salmeron, J., Wheeler, C. M., Chow, S. N., Apter, D., et al. (2009). Efficacy of Human Papillomavirus (HPV)-16/18 AS04-Adjuvanted Vaccine Against Cervical Infection and Precancer Caused by Oncogenic HPV Types (PATRICIA): Final Analysis of a Double-Blind, Randomised Study in Young Women. Lancet 374 (9686), 301–314. doi: 10.1016/S0140-6736(09)61248-4
Pahne-Zeppenfeld, J., Schroer, N., Walch-Ruckheim, B., Oldak, M., Gorter, A., Hegde, S., et al. (2014). Cervical Cancer Cell-Derived Interleukin-6 Impairs CCR7-Dependent Migration of MMP-9-Expressing Dendritic Cells. Int. J. Cancer 134 (9), 2061–2073. doi: 10.1002/ijc.28549
Palefsky, J. M., Gillison, M. L., Strickler, H. D. (2006). Chapter 16: HPV Vaccines in Immunocompromised Women and Men. Vaccine 24 (Suppl 3), S3/140–S3/146. doi: 10.1016/j.vaccine.2006.05.120
Pandey, S., Mittal, B., Srivastava, M., Singh, S., Srivastava, K., Lal, P., et al. (2011). Evaluation of Toll-Like Receptors 3 (C.1377C/T) and 9 (G2848A) Gene Polymorphisms in Cervical Cancer Susceptibility. Mol. Biol. Rep. 38 (7), 4715–4721. doi: 10.1007/s11033-010-0607-z
Pandey, S., Mittal, R. D., Srivastava, M., Srivastava, K., Singh, S., Srivastava, S., et al. (2009). Impact of Toll-Like Receptors [TLR] 2 (-196 to -174 Del) and TLR 4 (Asp299Gly, Thr399Ile) in Cervical Cancer Susceptibility in North Indian Women. Gynecol. Oncol. 114 (3), 501–505. doi: 10.1016/j.ygyno.2009.05.032
Pinidis, P., Tsikouras, P., Iatrakis, G., Zervoudis, S., Koukouli, Z., Bothou, A., et al. (2016). Human Papilloma Virus’ Life Cycle and Carcinogenesis. Maedica (Bucur) 11 (1), 48–54.
Polman, N. J., Veldhuijzen, N. J., Heideman, D. A. M., Snijders, P. J. F., Meijer, C., Berkhof, J. (2017). HPV-Positive Women With Normal Cytology Remain at Increased Risk of CIN3 After a Negative Repeat HPV Test. Br. J. Cancer 117 (10), 1557–1561. doi: 10.1038/bjc.2017.309
Prata, T. T., Bonin, C. M., Ferreira, A. M., Padovani, C. T., Fernandes, C. E., Machado, A. P., et al. (2015). Local Immunosuppression Induced by High Viral Load of Human Papillomavirus: Characterization of Cellular Phenotypes Producing Interleukin-10 in Cervical Neoplastic Lesions. Immunology 146 (1), 113–121. doi: 10.1111/imm.12487
Remes Lenicov, F., Paletta, A. L., Gonzalez Prinz, M., Varese, A., Pavillet, C. E., Lopez Malizia, A., et al. (2018). Prostaglandin E2 Antagonizes TGF-Beta Actions During the Differentiation of Monocytes Into Dendritic Cells. Front. Immunol. 9, 1441. doi: 10.3389/fimmu.2018.01441
Richards, R. M., Lowy, D. R., Schiller, J. T., Day, P. M. (2006). Cleavage of the Papillomavirus Minor Capsid Protein, L2, at a Furin Consensus Site Is Necessary for Infection. Proc. Natl. Acad. Sci. U. S. A. 103 (5), 1522–1527. doi: 10.1073/pnas.0508815103
Rodriguez, A. C., Schiffman, M., Herrero, R., Hildesheim, A., Bratti, C., Sherman, M. E., et al. (2012). Low Risk of Type-Specific Carcinogenic HPV Re-Appearance With Subsequent Cervical Intraepithelial Neoplasia Grade 2/3. Int. J. Cancer 131 (8), 1874–1881. doi: 10.1002/ijc.27418
Rolfe, M., Beer-Romero, P., Glass, S., Eckstein, J., Berdo, I., Theodoras, A., et al. (1995). Reconstitution of P53-Ubiquitinylation Reactions From Purified Components: The Role of Human Ubiquitin-Conjugating Enzyme UBC4 and E6-Associated Protein (E6AP). Proc. Natl. Acad. Sci. U. S. A. 92 (8), 3264–3268. doi: 10.1073/pnas.92.8.3264
Routes, J. M., Morris, K., Ellison, M. C., Ryan, S. (2005). Macrophages Kill Human Papillomavirus Type 16 E6-Expressing Tumor Cells by Tumor Necrosis Factor Alpha- and Nitric Oxide-Dependent Mechanisms. J. Virol. 79 (1), 116–123. doi: 10.1128/JVI.79.1.116-123.2005
Schiffman, M., Castle, P. E., Jeronimo, J., Rodriguez, A. C., Wacholder, S. (2007). Human Papillomavirus and Cervical Cancer. Lancet 370 (9590), 890–907. doi: 10.1016/S0140-6736(07)61416-0
Schiffman, M., Doorbar, J., Wentzensen, N., de Sanjose, S., Fakhry, C., Monk, B. J., et al. (2016). Carcinogenic Human Papillomavirus Infection. Nat. Rev. Dis. Primers 2, 16086. doi: 10.1038/nrdp.2016.86
Schiller, J. T., Day, P. M., Kines, R. C. (2010). Current Understanding of the Mechanism of HPV Infection. Gynecol. Oncol. 118 (1 Suppl), S12–S17. doi: 10.1016/j.ygyno.2010.04.004
Scott, M., Stites, D. P., Moscicki, A. B. (1999). Th1 Cytokine Patterns in Cervical Human Papillomavirus Infection. Clin. Diagn. Lab. Immunol. 6 (5), 751–755. doi: 10.1128/CDLI.6.5.751-755.1999
Shannon, B., Yi, T. J., Perusini, S., Gajer, P., Ma, B., Humphrys, M. S., et al. (2017). Association of HPV Infection and Clearance With Cervicovaginal Immunology and the Vaginal Microbiota. Mucosal Immunol. 10 (5), 1310–1319. doi: 10.1038/mi.2016.129
Shew, M. L., Ermel, A. C., Tong, Y., Tu, W., Qadadri, B., Brown, D. R. (2015). Episodic Detection of Human Papillomavirus Within a Longitudinal Cohort of Young Women. J. Med. Virol. 87 (12), 2122–2129. doi: 10.1002/jmv.24284
Stoler, M. H., Rhodes, C. R., Whitbeck, A., Wolinsky, S. M., Chow, L. T., Broker, T. R. (1992). Human Papillomavirus Type 16 and 18 Gene Expression in Cervical Neoplasias. Hum. Pathol. 23 (2), 117–128. doi: 10.1016/0046-8177(92)90232-r
Strickler, H. D., Burk, R. D., Fazzari, M., Anastos, K., Minkoff, H., Massad, L. S., et al. (2005). Natural History and Possible Reactivation of Human Papillomavirus in Human Immunodeficiency Virus-Positive Women. J. Natl. Cancer Inst. 97 (8), 577–586. doi: 10.1093/jnci/dji073
Torres-Poveda, K., Bahena-Roman, M., Madrid-Gonzalez, C., Burguete-Garcia, A. I., Bermudez-Morales, V. H., Peralta-Zaragoza, O., et al. (2014). Role of IL-10 and TGF-Beta1 in Local Immunosuppression in HPV-Associated Cervical Neoplasia. World J. Clin. Oncol. 5 (4), 753–763. doi: 10.5306/wjco.v5.i4.753
Trottier, H., Ferreira, S., Thomann, P., Costa, M. C., Sobrinho, J. S., Prado, J. C., et al. (2010). Human Papillomavirus Infection and Reinfection in Adult Women: The Role of Sexual Activity and Natural Immunity. Cancer Res. 70 (21), 8569–8577. doi: 10.1158/0008-5472.CAN-10-0621
Veazey, R. S., Acierno, P. M., McEvers, K. J., Baumeister, S. H., Foster, G. J., Rett, M. D., et al. (2008). Increased Loss of CCR5+ CD45RA- CD4+ T Cells in CD8+ Lymphocyte-Depleted Simian Immunodeficiency Virus-Infected Rhesus Monkeys. J. Virol. 82 (11), 5618–5630. doi: 10.1128/JVI.02748-07
Walch-Ruckheim, B., Mavrova, R., Henning, M., Vicinus, B., Kim, Y. J., Bohle, R. M., et al. (2015). Stromal Fibroblasts Induce CCL20 Through IL6/C/Ebpbeta to Support the Recruitment of Th17 Cells During Cervical Cancer Progression. Cancer Res. 75 (24), 5248–5259. doi: 10.1158/0008-5472.CAN-15-0732
Wang, J. W., Jiang, R., Peng, S., Chang, Y. N., Hung, C. F., Roden, R. B. (2015). Immunologic Control of Mus Musculus Papillomavirus Type 1. PloS Pathog. 11 (10), e1005243. doi: 10.1371/journal.ppat.1005243
Ward, H., Ronn, M. (2010). Contribution of Sexually Transmitted Infections to the Sexual Transmission of HIV. Curr. Opin. HIV AIDS 5 (4), 305–310. doi: 10.1097/COH.0b013e32833a8844
Wawer, M. J., Gray, R. H., Sewankambo, N. K., Serwadda, D., Li, X., Laeyendecker, O., et al. (2005). Rates of HIV-1 Transmission Per Coital Act, by Stage of HIV-1 Infection, in Rakai, Uganda. J. Infect. Dis. 191 (9), 1403–1409. doi: 10.1086/429411
Welters, M. J., van der Logt, P., van den Eeden, S. J., Kwappenberg, K. M., Drijfhout, J. W., Fleuren, G. J., et al. (2006). Detection of Human Papillomavirus Type 18 E6 and E7-Specific CD4+ T-Helper 1 Immunity in Relation to Health Versus Disease. Int. J. Cancer 118 (4), 950–956. doi: 10.1002/ijc.21459
Winer, R. L., Hughes, J. P., Feng, Q., Xi, L. F., Cherne, S., O’Reilly, S., et al. (2011). Early Natural History of Incident, Type-Specific Human Papillomavirus Infections in Newly Sexually Active Young Women. Cancer Epidemiol. Biomarkers Prev. 20 (4), 699–707. doi: 10.1158/1055-9965.EPI-10-1108
Woo, Y. L., Sterling, J., Damay, I., Coleman, N., Crawford, R., van der Burg, S. H., et al. (2008). Characterising the Local Immune Responses in Cervical Intraepithelial Neoplasia: A Cross-Sectional and Longitudinal Analysis. BJOG 115 (13), 1616–1621. doi: 10.1111/j.1471-0528.2008.01936.x
Xue, J., Wang, Y., Chen, C., Zhu, X., Zhu, H., Hu, Y. (2018). Effects of Th17 Cells and IL-17 in the Progression of Cervical Carcinogenesis With High-Risk Human Papillomavirus Infection. Cancer Med. 7 (2), 297–306. doi: 10.1002/cam4.1279
Yang, R., Murillo, F. M., Lin, K. Y., WHt, Y., Uematsu, S., Takeda, K., et al. (2004). Human Papillomavirus Type-16 Virus-Like Particles Activate Complementary Defense Responses in Key Dendritic Cell Subpopulations. J. Immunol. 173 (4), 2624–2631. doi: 10.4049/jimmunol.173.4.2624
Yan, M., Peng, J., Jabbar, I. A., Liu, X., Filgueira, L., Frazer, I. H., et al. (2005). Activation of Dendritic Cells by Human Papillomavirus-Like Particles Through TLR4 and NF-Kappab-Mediated Signalling, Moderated by TGF-Beta. Immunol. Cell Biol. 83 (1), 83–91. doi: 10.1111/j.1440-1711.2004.01291.x
Zevin, A. S., McKinnon, L., Burgener, A., Klatt, N. R. (2016). Microbial Translocation and Microbiome Dysbiosis in HIV-Associated Immune Activation. Curr. Opin. HIV AIDS 11 (2), 182–190. doi: 10.1097/COH.0000000000000234
Keywords: HPV, HIV, mucosal immunology, sexually transmitted infection, transmission
Citation: Zayats R, Murooka TT and McKinnon LR (2022) HPV and the Risk of HIV Acquisition in Women. Front. Cell. Infect. Microbiol. 12:814948. doi: 10.3389/fcimb.2022.814948
Received: 14 November 2021; Accepted: 03 January 2022;
Published: 10 February 2022.
Edited by:
Sonia Navas-Martin, Drexel University, United StatesReviewed by:
Saumya Pandey, IndiraIVF Hospital, IndiaCopyright © 2022 Zayats, Murooka and McKinnon. This is an open-access article distributed under the terms of the Creative Commons Attribution License (CC BY). The use, distribution or reproduction in other forums is permitted, provided the original author(s) and the copyright owner(s) are credited and that the original publication in this journal is cited, in accordance with accepted academic practice. No use, distribution or reproduction is permitted which does not comply with these terms.
*Correspondence: Thomas T. Murooka, dGhvbWFzLm11cm9va2FAdW1hbml0b2JhLmNh; Lyle R. McKinnon, THlsZS5NY0tpbm5vbkB1bWFuaXRvYmEuY2E=
†These authors share senior authorship