- 1Department of Endodontics, Affiliated Stomatology Hospital of Guangzhou Medical University, Guangdong Engineering Research Center of Oral Restoration and Reconstruction, Guangzhou Key Laboratory of Basic and Applied Research of Oral Regenerative Medicine, Guangzhou, China
- 2Guangzhou Medical University School and Hospital of Stomatology, Guangzhou, China
- 3Novel Bacteria and Drug Discovery Research Group, Microbiome and Bioresource Research Strength, Jeffrey Cheah School of Medicine and Health Sciences, Monash University, Bandar Sunway, Malaysia
- 4Department of Biological Sciences, School of Medical and Life Sciences, Sunway University, Petaling Jaya, Malaysia
Children with cerebral palsy (CP) present a higher prevalence and severity of caries. Although researchers have studied multiple risk factors for caries in CP, the role of microorganisms in caries remains one of the critical factors worth exploring. In order to explore the differences in the supragingival plaque microbiota (SPM), supragingival plaque samples were collected from 55 CP children and 23 non-CP children for 16S rRNA sequencing. Distinct SPM composition was found between CP children with severe caries (CPCS) and non-CP children with severe caries (NCPCS). Further subanalysis was also done to identify if there were any differences in SPM among CP children with different degrees of caries, namely, caries-free (CPCF), mild to moderate caries (CPCM), and severe caries (CPCS). After selecting the top 15 most abundant species in all groups, we found that CPCS was significantly enriched for Fusobacterium nucleatum, Prevotella intermedia, Campylobacter rectus, Porphyromonas endodontalis, Catonella morbi, Alloprevotella tannerae, Parvimonas micra, Streptobacillus moniliformis, and Porphyromonas canoris compared to NCPCS. By comparing CPCF, CPCM, and CPCS, we found that the core caries-associated microbiota in CP children included Prevotella, Alloprevotella, Actinomyces, Catonella, and Streptobacillus, while Capnocytophaga and Campylobacter were dental health-associated microbiota in CP children. Alpha diversity analysis showed no significant difference between NCPCS and CPCS, but the latter had a much simpler core correlation network than that of NCPCS. Among CP children, CPCM and CPCF displayed lower bacterial diversity and simpler correlation networks than those of CPCS. In summary, the study showed the specific SPM characteristics of CPCS compared to NCPCS and revealed the core SPM in CP children with different severities of caries (CPCF, CPCM, and CPCS) and their correlation network. Hopefully, the study would shed light on better caries prevention and therapies for CP children. Findings from the current study offer exciting insights that warrant larger cohort studies inclusive of saliva and feces samples to investigate the potential pathogenic role of oral microbiota through the oral–gut–brain axis in CP children with caries.
Introduction
As a multifactorial and dynamic disease, dental caries is often linked with bacterial overgrowth in the oral cavity and excessive sugar intake (Selwitz et al., 2007). Frequent intake of fermentable carbohydrates (primarily sugars) promotes the growth of acid-producing bacteria in the supragingival plaque, resulting in the decline of pH value. As this undesirable condition progresses and dysbiosis occurs, the teeth will gradually demineralize and eventually lead to caries development (Selwitz et al., 2007). By the same token, emerging evidence showed that maintaining “harmony” in oral microbiota is essential, as bacterial overgrowth or dysbiosis in the oral microbiota can trigger both oral and systemic diseases (Latti et al., 2018; Deo and Deshmukh, 2019). Keeping in mind that bacteria in the oral cavity can be ingested and translocate to other parts of the gastrointestinal tract, the overgrowth of the unwanted bacterial population in the oral cavity poses great risk to human health, potentially triggering a “chain reaction” via disruption of the gut microbiota either via displacing natural inhabitants in the gastrointestinal tract or even killing them via production of harmful toxins. Consequently, the anatomical communication (Sansores-España et al., 2021) between the oral cavity and gut offers a new perspective in the well-established gut–brain axis that is associated with neuroinflammation, neurodegenerative diseases (e.g., impaired motor function or dementia), and neuropsychiatric disorders (e.g., depression, anxiety, bipolar disorder) (Kelly et al., 2015; Lee et al., 2018; Mohajeri et al., 2018; Lee et al., 2019; Parker et al., 2020), specifically on how microbes in the oral cavity take part in the development of neurological/neurodegenerative disease via the oral–gut–brain axis. For the case of dental caries, a recently published scoping review (Latti et al., 2018) indicated the lack of evidence from human studies to clearly explain the association of dental caries (Ferreira et al., 2021a) and systemic diseases, which constitutes a research gap (Wu et al., 2016; Maitre et al., 2020; Orr et al., 2020). Understanding changes in the oral microbiota signatures, specifically dental caries as one of the most prevalent oral diseases, holds promise for better insight into the relationship of the oral–gut–brain axis.
Cerebral palsy (CP) is defined as a neurological disorder of postural and other motor dysfunctions caused by non-progressive brain injury in the immature stage of brain development within 1 month after birth (Gajewska et al., 2014). The prevalence of CP is approximately 3 per 1,000 births (Michael-Asalu et al., 2019), impacting 17 million worldwide (Graham et al., 2016). Despite the rapid progress in prenatal diagnosis and perinatal and neonatal medicine, the incidence rate of CP did not reflect any decreasing trend (Graham et al., 2016). Intriguingly, CP patients often exhibit a higher prevalence of caries with greater severity (Selwitz et al., 2007). In a study from Saudi Arabia, all the enrolled CP patients had caries, and the average DMFT/dmft (Permanent teeth: Decayed, Missing, Filled Teeth; Primary teeth: decayed, missing, filled teeth) index of these CP patients is 9.98, which is much higher than the average level of DMFT/dmft index in the country (DMFT: 3.5, dmft: 5.0) (Al Agili, 2013; Wyne et al., 2017). There are multiple factors that contribute to the correlation between CP and dental caries, including dietary characteristics, low self-care ability, pathological neural activity, salivary characteristics, and pharmacological factors. For example, CP patients suffer from neurodysplasia and the inability to chew food independently, which necessitates a unique dietary plan to consume almost exclusively non-solid diets (including high-sugar diets) (Akhter et al., 2017). Coupled with pathological neural activities, such as continuous bite reflex, these conditions constitute challenges for the nursing staff to maintain daily oral hygiene for CP patients that directly (or indirectly) contributes to plaque accumulation (Dos Santos and Nogueira, 2005). The saliva of CP patients tends to be low in amount, buffer capacity, and pH value but high in osmotic pressure (Dos Santos et al., 2002; Malta et al., 2020), which negatively affects buffering bacteria-produced acids and tooth remineralization. Also, therapeutic drugs of CP, such as oxcarbazepine, have a high total soluble solid (TSS) value and low pH value, which are cariogenic and corrosive to the tooth surface (Alves et al., 2016). Although the microbiology (biofilm)-related aspect is a key element in the pathogenesis of caries, the supragingival plaque microbiota (SPM) of CP patients remains to be studied.
The current study aims to study the differences in the SPM composition in CP children with different caries severity to reveal the core cariogenic bacteria before comparing to non-CP children with severe caries. Findings from this study expand current knowledge on the role of dental caries and oral microbiota in CP children while serving as a cornerstone to decipher communications in the oral–gut–brain axis, which could be exploited in designing effective prevention and treatment plans in the near future.
Materials and Methods
Ethics Statement
The study was approved by the Ethics Committee of the Maternity and Child Healthcare Hospital (Longgang district, Shenzhen, China) with the registration number LGFYYXLLS-2021-001. All of the written informed consents were acquired from the CP children’s guardians and non-CP children’s parents.
Participant Recruitment
The CP children were recruited from the Longgang district and diagnosed by the Department of Neurology, Maternity and Child Healthcare Hospital (Longgang, Shenzhen, China), with the following inclusion criteria: 1) Aged 6–14 years; 2) Subjects were diagnosed with clear clinical manifestations of CP according to the diagnosis guidelines (National Guideline, 2017); 3) The diagnostic criteria of dental caries refer to the standards of WHO Basic Methods of Oral Health Survey (4th edition) (World Health, 1997); 4) dmft and DMFT (dmft + DMFT for mixed dentition) are used to record the severity of caries: very low (0.0–1.1), low (1.2–2.6), moderate (2.7–4.4), high (4.5–6.5), and very high (6.6 and above). The non-CP children were selected from Fu’an Middle School (Longgang, China) with the following standards: 1) Aged 6–14 years; 2) No hereditary diseases (e.g., phenylketonuria); 3) No metabolic disease (e.g., diabetes); 4) No gastrointestinal disease; 5) The severity criteria of caries matched with recruited CP children and diagnosed based on the standards to the WHO Basic Methods of Oral Health Survey (4th edition). Moreover, the subjects were excluded from the study if they were exposed to antibiotics, probiotics, or proton pump inhibitors 2 weeks before sample collection. Children treated with long-term fluorine and pit and fissure sealing were also excluded. In total, 55 CP children and 23 non-CP children were enrolled between November 2020 and December 2020. The information for enrolled participants is shown in Table 1.
Grouping and Supragingival Plaque Collection
The study was only blind to the evaluators of clinical parameters. The subjects were grouped as follows: CPCS: CP children with high or very high severity of caries, DMFT/dmft >4.4; NCPCS: Non-CP children with high or very high severity of caries, DMFT/dmft >4.4; CPCM: CP children with mild-to-moderate caries, 0< DMFT/dmft ≤4.4; CPCF: CP children without caries, DMFT/dmft = 0.
The supragingival plaque was sampled as previously described (Sato et al., 2015; Coker et al., 2021; Yang et al., 2021) with some modifications. All subjects were sampled in the morning (between 9:00 and 11:00 a.m.) and refrained from food 3 h before sample collection. Subjects were requested to gargle with sterile distilled water, and the tooth surface to be sampled was thoroughly dried with sterile cotton balls prior to sample collection. During the oral examination, supragingival plaque on the buccal surface of the right maxillary first molar was collected from CP and non-CP children using sterile Gracey curettes. The samples were then refrigerated at -80°C until subsequent experiments.
DNA Extraction, Library Construction, and Sequencing
DNA extraction was performed by PowerSoil DNA Isolation Kit (Mo Bio Laboratories, Carlsbad CA, USA). DNA concentration and purity were estimated by 1% agarose gels on Agilent5400 (Agilent Technologies, Inc., Santa Clara CA, USA). The DNA library of 16S rRNA V3–V4 region was constructed by the PCR amplification and sequenced on the Illumina MiSeq Sequencing platform (Illumina, San Diego CA, USA).
Bioinformatics Analysis
The high-throughput sequencing results were sorted according to the barcode identifier, raw reads corresponding to each sample were obtained, and low-quality sequences and chimeras were excluded. Sequence Mosaic was performed according to the overlap of paired sequences using FLASH (v1.2.11, http://ccb.jhu.edu/software/FLASH/index.shtml). Subsequently, operational taxonomic unit (OTU) cluster analysis was performed for the assembled tag sequences with 97% sequence similarity using Usearch software (v10.0) (Edgar, 2013). According to the rarefaction curve estimation, we set the normalized assembled tag sequences as 19,489. In addition, the representative OTU sequences were annotated with microbial species classification by comparing to the Greengene database (v13.5) (DeSantis et al., 2006). Finally, the species annotation information on the corresponding phyla, classes, orders, families, genus, and species was obtained. The abundance and diversity of microbial composition of each sample were further calculated using Mothur software (v1.43.0) (Schloss et al., 2009), and the composition trend of samples was obtained by Nonmetric Multidimensional Scaling (NMDS) analysis. The correlation networks were visualized using the Cytoscape software (v3.4.0) (Shannon et al., 2003). The Shannon index was calculated using the “vegan” package in R (v3.5.1) (Oksanen et al., 2015).
Statistical Analysis
All the statistical analyses were conducted in R (version 3.6.0). To detect the differences in the microbiota (genus and species level) and functional categories between CPCS and NCPCS (P < 0.05), Wilcoxon rank-sum test was applied (“stats” package). Kruskal–Wallis rank sum test (“stats” package) was utilized to evaluate microbe (genus and species level) and functional categories among CPCS, CPCM, and CPCF (P < 0.05). Nonparametric multivariate analysis (PERMANOVA) (“vegan” package) of variance was used to determine the influence of each factor on the microbiota composition (α = 0.05).
Results
Sample Characteristics and Data Output
All study participants were in mixed dentition. There were no significant differences in gender and age composition among the four groups (P > 0.05, Table 1). CP exhibited a considerable influence on the SPM difference between NCPCS and CPCS, while the severity of caries was the main driving factor among CPCS, CPCM, and CPCF. After 16S rRNA sequencing and taxonomy annotation, tags, OTUs, and the number of detected genera and species were demonstrated in Table 2. A total of 3,464,930 tags were high-quality reads from 16S rRNA sequencing, and the average tag number on each sample was 44,422 ± 11,788, ranging from 19,489 to 78,717. The OTU number showed a statistically significant distinction between NCPCS and CPCS (P < 0.05) and also among CPCS, CPCM, and CPCF (P < 0.05). In all samples, rarefaction curves reached the plateau, suggesting a sufficient sequencing depth for the following analysis (Figure 1).
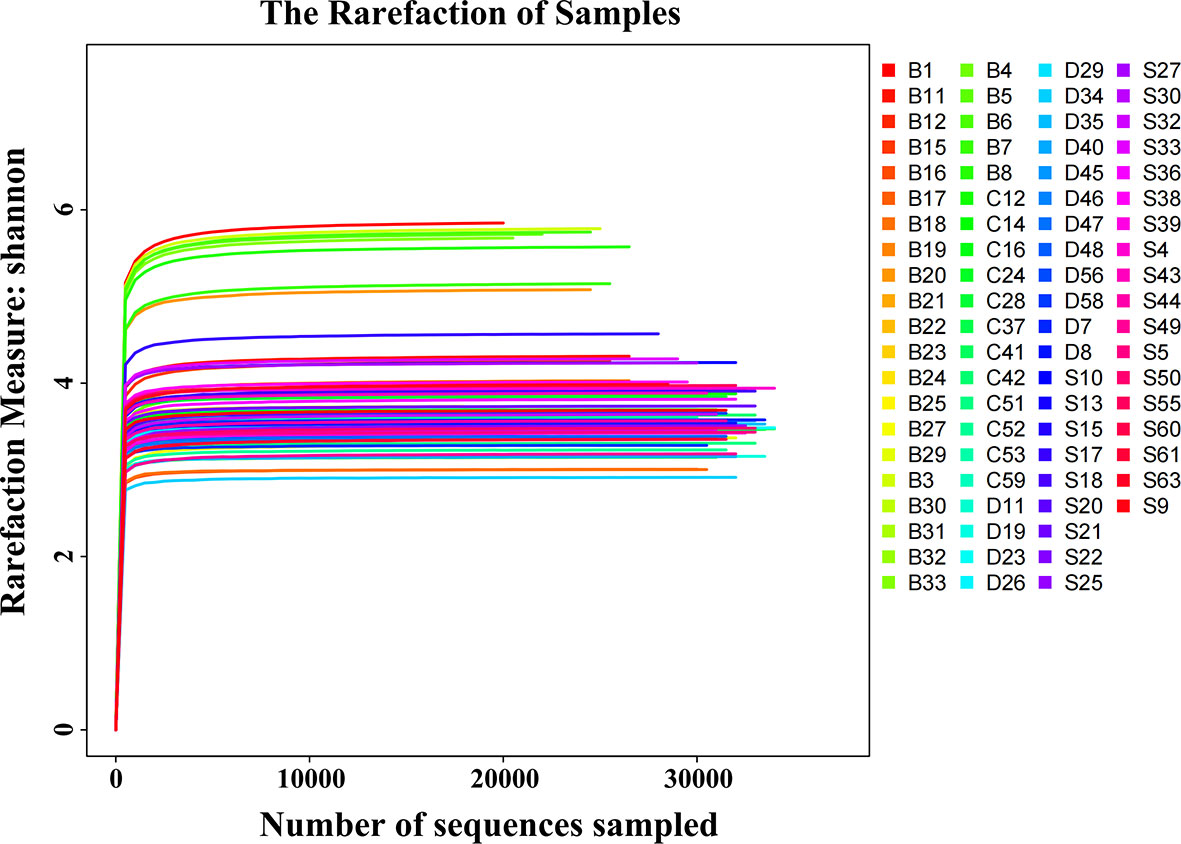
Figure 1 Shannon rarefaction curves of all SPM samples. The horizontal coordinate is the number of sequences sampled. The vertical coordinate is the corresponding Shannon index of the sample. In the case of very few sampled sequences, the Shannon index has reached a plateau. S, CP children with severe or extremely severe caries; B, non-CP children with severe or extremely severe caries; C, CP children with mild-to-moderate caries; D, CP children without caries.
The Supragingival Plaque Microbiota of CPCS Is Different From That of NCPCS
As shown in Figure 2A, no statistical difference of microbial diversity was identified between NCPCS (4.34 ± 0.99) and CPCS (3.75 ± 0.35) by Shannon indexes (P > 0.05). NMDS analysis illustrated that the SPM samples from NCPCS were mostly apart from CPCS (Figure 2C). Qualitatively, CPCS separated from NCPCS mainly resulted from the enrichment in Prevotella and deficiency in Capnocytophaga and Leptotrichia.
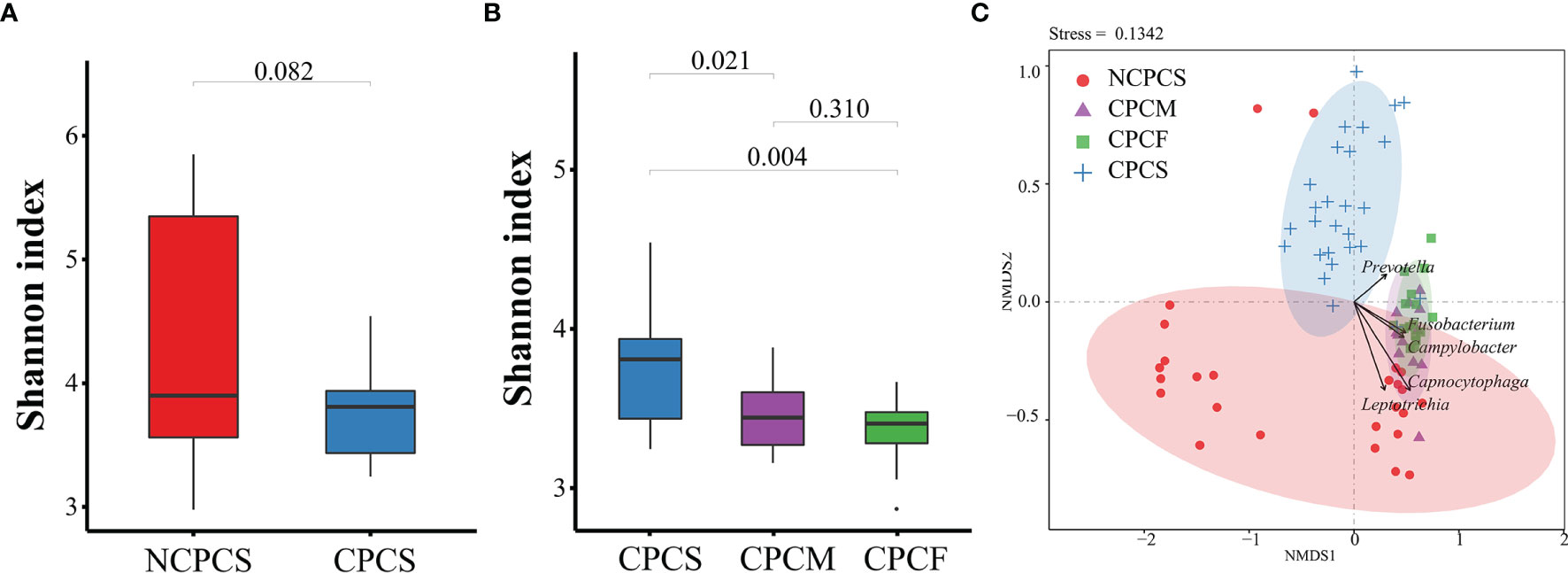
Figure 2 Bacterial diversity of subgingival plaque microbiota and NMDS distribution analysis. (A) The Shannon index detection between NCPCS and CPCS. (B) The Shannon index among CPCS, CPCM, and CPCF. (C) NMDS analysis of the SPM samples from NCPCS, CPCM and CPCF, and CPCS. NCPCS, non-CP children with severe or extremely severe caries; CPCS, CP children with severe or extremely severe caries; CPCM, CP children with mild-to-moderate caries; CPCF, CP children without caries.
At the genus level, the top 23 genera from the NCPCS and CPCS were identified, and 16 of them were differentially enriched (Figure 3B and Supplementary Table S1). In comparison with NCPCS, CPCS was more abundant in the following 10 genera: Prevotella, Fusobacterium, Porphyromonas, Saccharibacteria, Catonella, Alloprevotella, Streptobacillus, Parvimonas, Peptostreptococcaceae, and SR1 [P < 0.05, false discovery rate (FDR) < 0.05]. In contrast, 6 genera were found to be significantly enriched in NCPCS (P < 0.05, FDR < 0.05): Leptotrichia, Capnocytophaga, Gp1, Rothia, Aquamicrobium, and Corynebacterium.
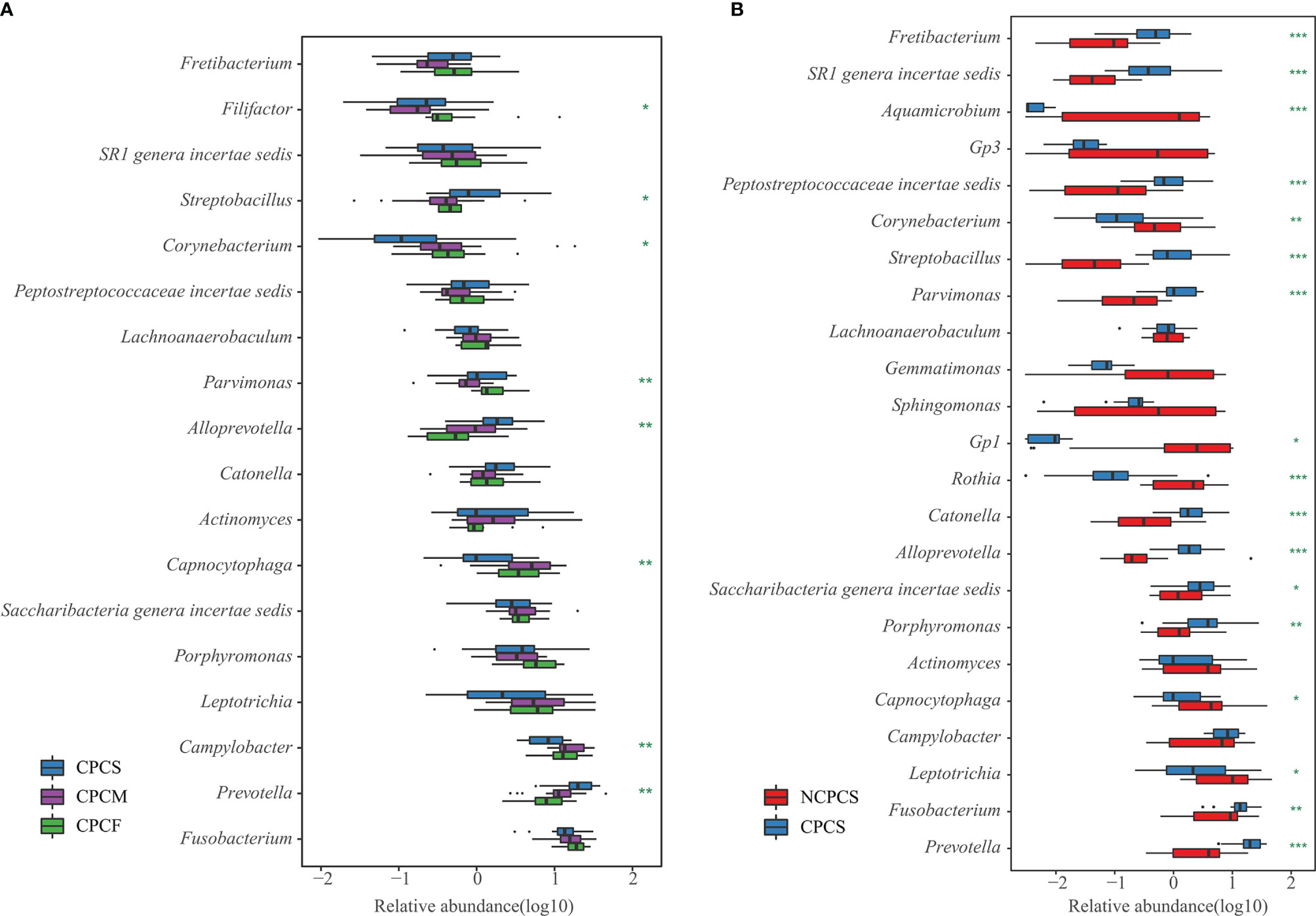
Figure 3 Genus differential box plot of supragingival plaque microbiota. (A) Genera with dominant abundance in CPCS, CPCM, and CPCF were demonstrated. The blue, pruple, and green boxes represent group CPCS, CPCM, and CPCF, respectively. (B) Dominant genera were selected from NCPCS and CPCS. NCPCS and CPCS were respectively represented with red and blue boxes. *P < 0.05, **P < 0.01 and ***P < 0.001. NCPCS, non-CP children with severe or extremely severe caries; CPCS, CP children with severe or extremely severe caries; CPCM, CP children with mild-to-moderate caries; CPCF, CP children without caries.
At the species level, the top 15 species from the NCPCS and CPCS are shown in Figure 4. The abundance differences were attributed to the enrichment of Fusobacterium nucleatum, Prevotella intermedia, Campylobacter rectus, Catonella morbi, Porphyromonas endodontalis, Alloprevotella tannerae, Parvimonas micra, Streptobacillus moniliformis, Porphyromonas canoris, Eubacterium yurii, and uncultured bacterium X112 in CPCS and of Leptotrichia buccalis, Capnocytophaga granulosa, Actinomyces oris, and Leptotrichia shahii in NCPCS (Supplementary Table S1).
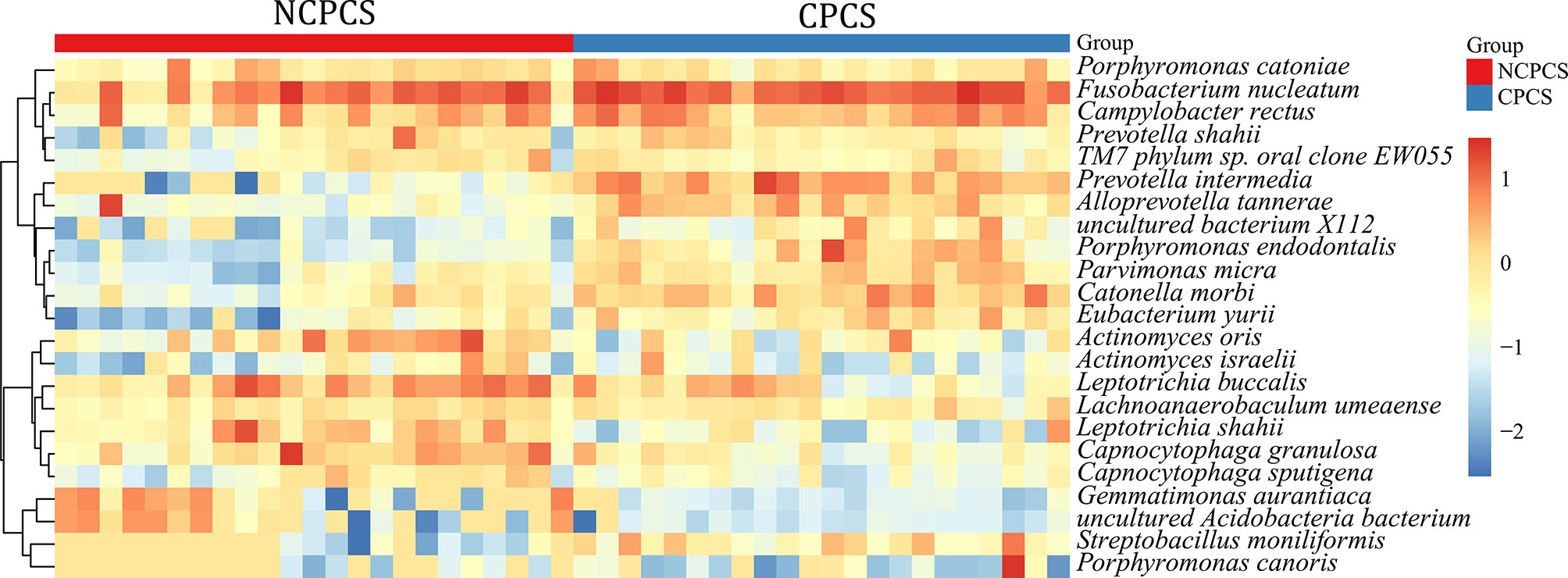
Figure 4 Relative abundance of species in NCPCS and CPCS supragingival plaque microbiota. Different color reflects relative abundance from low (blue) to high (red). NCPCS, non-CP children with severe or extremely severe caries; CPCS, CP children with severe or extremely severe caries.
The Core Supragingival Plaque Microbiota of CP Children Was Revealed by Comparing CPCF, CPCM, and CPCS
Based on the OTU distribution, the Shannon indexes of CPCS (3.75 ± 0.35), CPCM (3.47 ± 0.24), and CPCF (3.36 ± 0.2) showed a decreasing trend. The Shannon index of CPCS differed greatly from that of CPCM and CPCF. According to NMDS analysis, there was a distinct separation of CPCS from CPCF and CPCM, whereas CPCF mostly overlapped with CPCM (Figure 2B). Abundance in Fusobacterium and Campylobacter of CPCM and CPCF might explain their separation from CPCS. However, no statistical difference was noticed between CPCM and CPCF.
Our results revealed that the dominant genera of CPCS, CPCM, and CPCF showed high similarity, suggesting “the core microbiota” in CP children. With dominant proportions in all three groups, Prevotella, Fusobacterium, Campylobacter, Leptotrichia, Porphyromonas, Saccharibacteria, Actinomyces, Catonella, Alloprevotella, Capnocytophaga, Parvimonas, Streptobacillus, Peptostreptococcaceae, SR1, and Lachnoanaerobaculum were “the core microbiota” of the supragingival plaque in CP children with caries, with total relative abundance accounting for 74.44% in CPCS, 81.40% in CPCM, and 77.85% in CPCF. Prevotella, Alloprevotella, and Streptobacillus were enriched with greater severity of caries, whereas the relative abundances of Campylobacter and Capnocytophaga were decreased (Figure 3A and Supplementary Table S2).
Fourteen species were observed to have different relative abundances among CPCS, CPCM, and CPCF (P < 0.05, FDR < 0.05) (Figure 5 and Supplementary Table S2). C. rectus, Porphyromonas catoniae, L. buccalis, C. granulosa, TM7 phylum sp. Oral clone EW055, and Filifactor villosus were found to be enriched gradually from CPCS to CPCF, while the relative abundances of P. endodontalis, A. tannerae, P. intermedia, and S. moniliformis were enriched among CPCS.
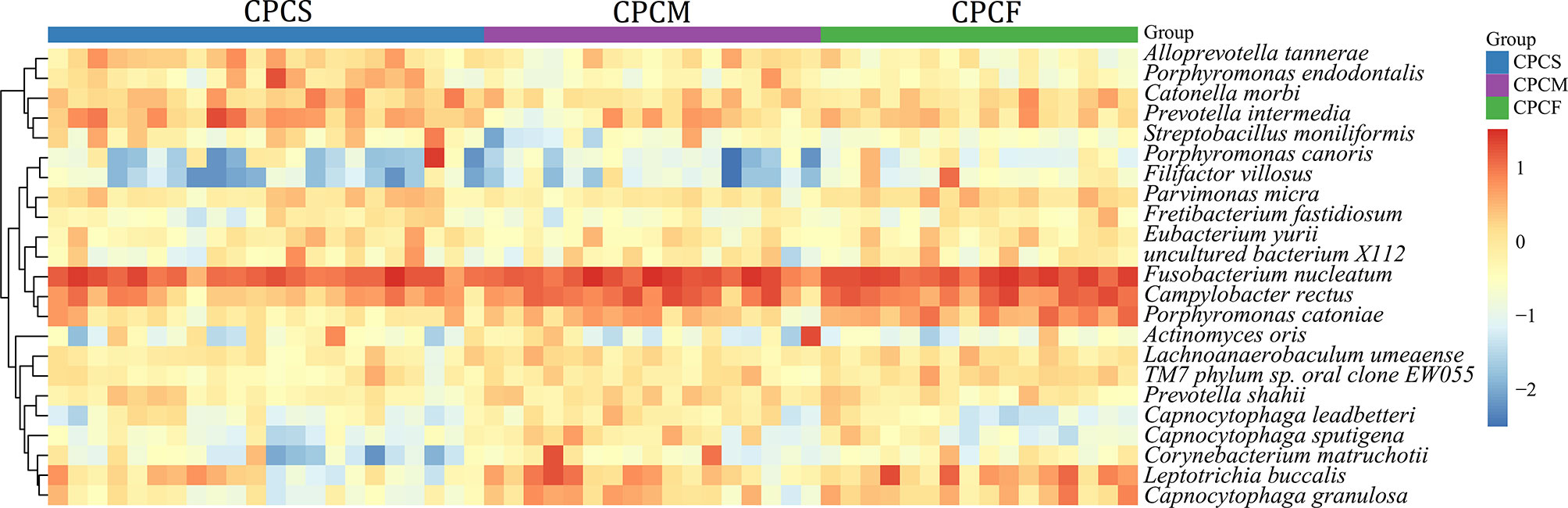
Figure 5 Relative abundance of species in supragingival plaque microbiota of CPCS, CPCM, and CPCF. Different colors reflect relative abundance from low (blue) to high (red). CPCS, CP children with severe or extremely severe caries; CPCM, CP children with mild-to-moderate caries; CPCF, CP children without caries.
Establishing the Complex Networks of Core Supragingival Plaque Microbiota Correlation Among CP Children With Different Caries Severities
The complex correlation networks of core SPM were constructed for all groups respectively at the species level (Figure 6). Under the same situation of severe caries, a more complex network was established for NCPCS compared to CPCS. Furthermore, the complexity of the network was reduced when the severity of caries was decreased from CPCS to CPCF. As the most abundant species both in NCPCS and CPCS, F. nucleatum exhibited a much more complicated interaction in NCPCS. C. morbi and C. rectus were included in species positively correlated with F. nucleatum in NCPCS, while C. rectus and F. nucleatum also presented positive interaction in CPCS. With a much higher abundance in CPCS, P. endodontalis and P. micra showed a positive interaction with each other. Besides, it demonstrated a positive correlation between L. buccalis and L. shahii in NCPCS.
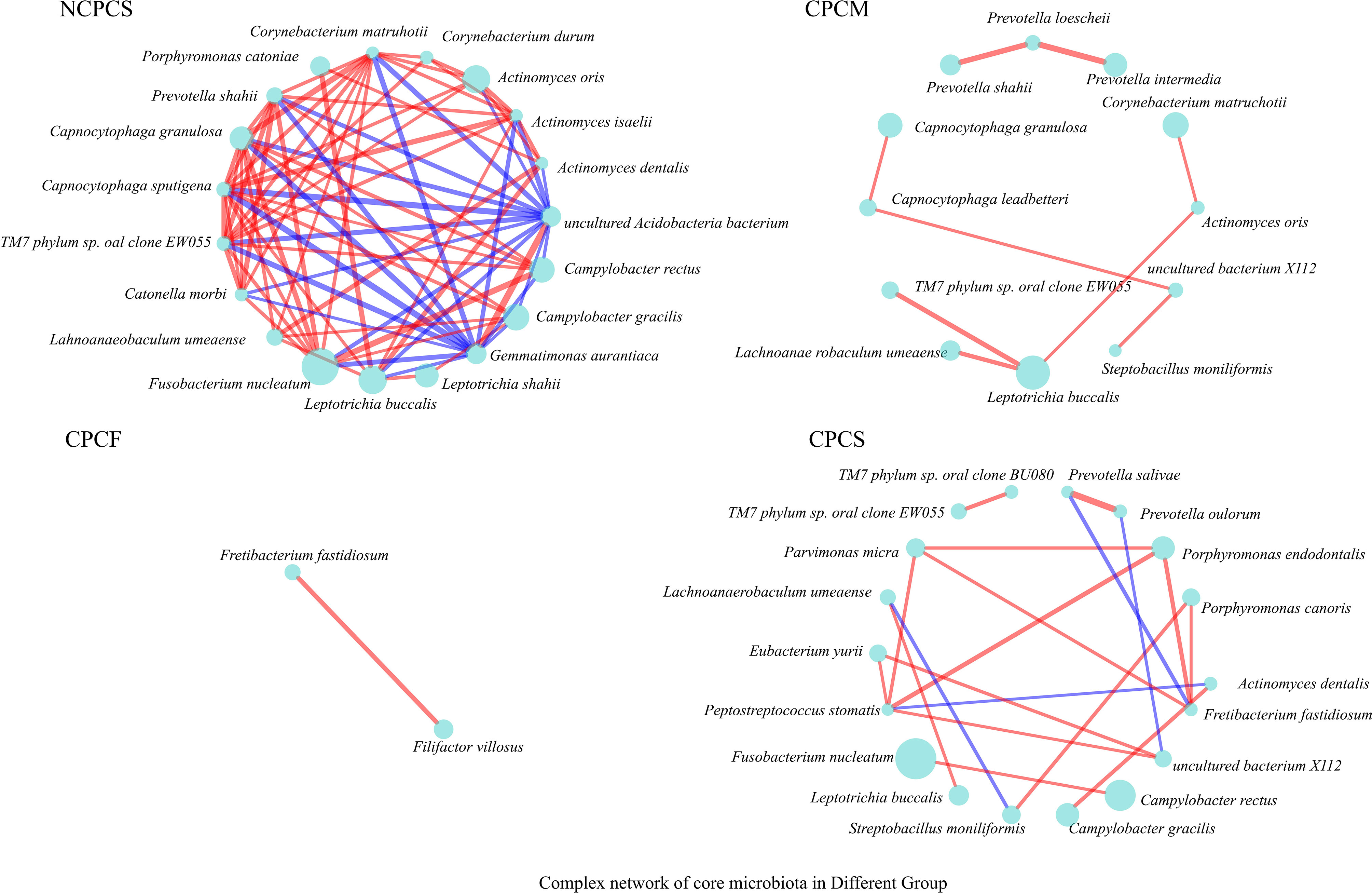
Figure 6 The complex network of core SPM correlation in all groups. Genera with >0.5% relative abundance were chosen to establish correlation networks (P value ≤0.05, R-value ≥0.6). The circle size represents relative abundance. The red and blue lines suggest positive and negative correlations (proportional to line thickness), respectively.
Discussion
Dental caries is regarded as the most common chronic childhood disease (Selwitz et al., 2007). In general, CP patients exhibit a higher prevalence of caries with greater severity. At the time of writing, most studies (Thorburn et al., 2009; Huang et al., 2021) report the role of gut microbiota in CP via conducting metagenomic studies on fecal samples. A recent study by Huang et al. (2019) reported higher gut microbial diversity in the CP children compared to the healthy group (P < 0.001). Subsequent functional prediction and analysis of the gut microbiota in CP children discussed that the gut dysbiosis observed may potentially promote neuroinflammation and subsequently lead to the development of neurodegenerative disease (Ferreira et al., 2021a; Ferreira et al., 2021b), mainly attributed to Streptococcus sp. Having said that, the role of oral microbiota in CP has garnered much attention from the scientific community with the discovery of overlapping microbial signatures between oral and fecal specimens that emphasizes the importance of the oral–gut–brain axis in human diseases (Olsen and Hicks, 2020). Here, the current study reported the characteristics of SPM in CPCS compared to NCPCS and further concluded the specific microbiota in CP children with different severities of caries (CPCF, CPCM, and CPCS).
To the best of our knowledge, multiple studies have suggested that a high prevalence and severity of caries exist in CP, but no reports describing SPM in CP children are available (Akhter et al., 2017; Bensi et al., 2020). Among “the core microbiota” detected in CP children, Prevotella, Alloprevotella, Catonella, and Actinomyces were highly associated with caries according to previous reports (Chhour et al., 2005; Jiang et al., 2014; Espinoza et al., 2018; He et al., 2018; Al-Hebshi et al., 2019). The abundances of Prevotella, Alloprevotella, and Streptobacillus were not only increased from CPCF to CPCS but also in CPCS compared to NCPCS. These observations suggest their roles in caries induction and importance as predictive markers in CP children. Notably, Prevotella may be considered as the main microbial predictor for caries instead of Streptococcus mutans due to its high activity in producing carbohydrate-derived acid (Teng et al., 2015). Streptobacillus is not commonly detected in oral diseases, but it has been found to overgrow in the supragingival plaque of caries-active patients (Li et al., 2016), implying its potential cariogenic role. In addition, the results showed that both Capnocytophaga and Campylobacter exhibited the lowest abundance among subjects with high severity of caries. Capnocytophaga is dominant in caries-free subjects and offers strong co-aggregation ability with other dental health-associated bacteria (Jiang et al., 2014), while Campylobacter utilizes organic acid to maintain pH homeostasis (Gross et al., 2010). Thus, Prevotella, Alloprevotella, Actinomyces, Catonella, and Streptobacillus may be utilized as the core caries-associated genera in CP children, while the maintenance of Capnocytophaga and Campylobacter in oral microbiota might be essential to promote the caries-free condition in CP children.
According to the ecological plaque hypothesis, the most convincing etiologic theory of dental caries emphasizes that dental caries results from an unfavorable shift in the balance of the resident microbiota driven by changes in the oral environment (Selwitz et al., 2007). Similarly, several teams (Blumberg and Powrie, 2012; Zackular et al., 2016; Meijers et al., 2019) have advocated the idea that disease may not arise from a single pathogenic bacterium, but it may be more helpful to investigate the ratio of pathogenic to more protective or beneficial species. Despite the absence of S. mutans in CP children, the unique oral environment in CP may be attributed to movement and development problems, causing the SPM to shape differently compared to non-CP children. Oral motor dysfunction results in less efficient food intake and residue clearance in CP patients (Benfer et al., 2012). Additionally, the daily diet of CP patients mainly consists of semisolid or liquid foods and often contain a high proportion of sugar; collectively, these can lead to the increased adhesion of diets and plaque formation (Selwitz et al., 2007; De Camargo and Antunes, 2008; Arvedson, 2013; Akhter et al., 2017). Furthermore, CP patients show low pH, low buffering capacity, low salivary flow rate, and high osmotic pressure in saliva (Anjugam et al., 2021). These risk factors establish a particular oral environment for CP patients, providing an evolutionary drive for microorganisms to adapt to this variation. For instance, the low pH oral environment tends to induce a biofilm composition toward a higher abundance of acidogenic and aciduric taxa (Senneby et al., 2017). Focusing on dental caries, the shift to reduced bacterial diversity is caused by increased carbohydrate consumption and fermentation, resulting in acid production and secretion (Ribeiro et al., 2017; Olsen and Hicks, 2020). None of the three CP groups (CPCS, CPCM, CPCF) had a higher Shannon index than the non-CP group (NCPCS), which conversely indicates a lower diversity in CP patients that can be possibly attributed to selective pressure from environmental factors (e.g., low pH). This observation is in line with the core microbiota of CP, as most of them display aciduric characteristics.
Compared with NCPCS, CPCS exhibits higher abundance in F. nucleatum, P. intermedia, C. rectus, C. morbi, P. endodontalis, A. tannerae, P. micra, and S. moniliformis. Apart from C. rectus, S. moniliformis, and P. endodontalis, others are enriched in caries-active children (Hahn et al., 2004; Bizhang et al., 2011; Lima et al., 2011; Schulze-Schweifing et al., 2014; Espinoza et al., 2018). Among them, P. intermedia, P. endodontalis, and A. tannerae are enriched in the CPCS group compared to those in CPCM and CPCF groups. These three species are proposed to have a core caries-inducing and predictive role in CP children. Under the circumstance that acidogenic bacteria in the SPM metabolize carbohydrates (especially sugars), their abundance in the oral microbiota can exacerbate the formation of dental caries. On top of that, previous studies showed that almost all of the species enriched in CPCS are aciduric, indicating a lower pH oral environment for CP children (Aamdal-Scheie et al., 1996; Takahashi et al., 1997; Bizhang et al., 2011; Kianoush et al., 2014; Antezack et al., 2021). F. nucleatum and P. intermedia could produce acidic products by metabolizing the carbohydrate components adhered to the tooth surface (Takahashi et al., 1997). Additionally, F. nucleatum is positively correlated with C. rectus both in NCPCS and CPCS. C. rectus is considered one of the dental health-associated species. In addition to the impact of the oral environment, its enrichment in CPCS might attribute to the abundance of F. nucleatum due to its co-aggregation mechanism (Horiuchi et al., 2020). On the other hand, C. morbi is capable of metabolizing sugars such as lactose and maltose into lactate, acetate, and formate (Sizova et al., 2013). It is noteworthy that P. endodontalis and P. micra often indicate endodontic lesions and are mainly detected in deep dentin caries (Rôças et al., 2015). They are enriched in CPCS, while they can hardly be detected in NCPCS. Although the same severity of caries based on DMFT/dmft was shown in NCPCS and CPCS, the enrichment of P. endodontalis and P. micra indicates deeper caries progression in CP children. The SPM of CP children and their toxic metabolite [e.g., short-chain fatty acid (SCFA)] may invade the pulp through dentinal tubules, causing irreversible pulpitis and even invading the bloodstream via the pulp capillaries (Figure 7) (Narengaowa et al., 2021).
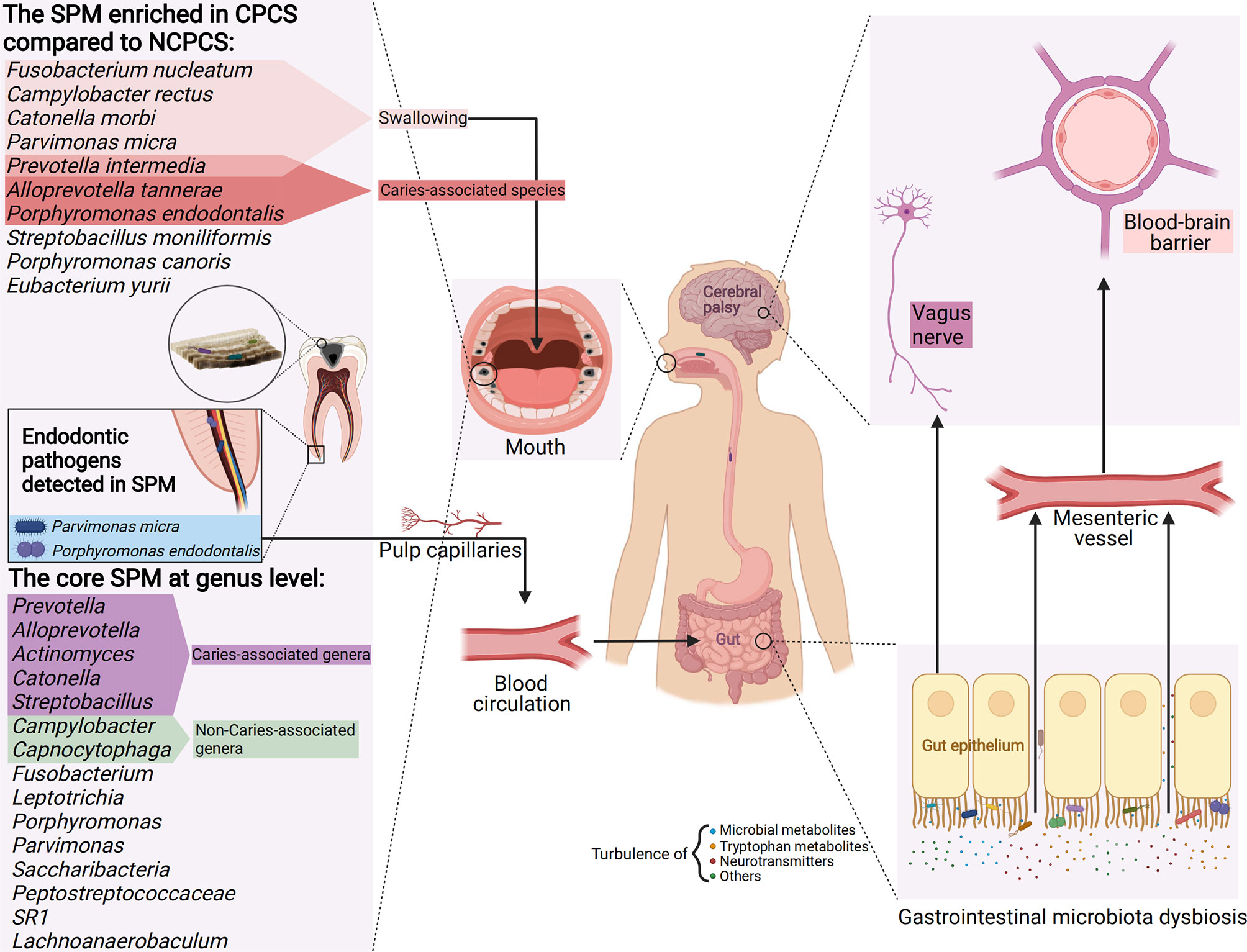
Figure 7 A model of CP’s SPM and its potential impact on gastrointestinal microbiota dysbiosis and the gut–brain axis. The enriched genera in CPCS compared with NCPCS and the core genera (dominant in CPCS, CPCM, and CPCF) detected in the SPM of CP children are presented. SPM reaches the stomach and intestines by enteral (e.g., eating and swallowing) and hematogenous route (e.g., invasion of pulp capillaries through the root canal). Enriched in the SPM of CP, aciduric bacteria may survive the barrage of gastric acid and colonize in the gastrointestinal tract, thus establishing microbial dysbiosis and chronic inflammation. Through the vagus nerve pathway (neurotransmitters turbulence, e.g., GABA and acetylcholine), tryptophan metabolism (e.g., quinolinic acid and kynurenic acid) and microbial metabolite disorder (e.g., SCFA, peptidoglycans), developmental brain defects, and neuroinflammation and neurodegenerative diseases might be induced, thus aggravating neurological symptoms in CP children (Created with Biorender.com).
The enriched bacteria identified in NCPCS include the genus of Leptotrichia, Capnocytophaga, Rothia, and Corynebacterium. Among them, Leptotrichia (which is a bacterium widely reported to have caries potential) showed the highest abundance in NCPCS (He et al., 2018). It is noteworthy that Rothia, not detected in CPCS, could buffer extracellular pH by utilizing lactate and generating ammonia from dietary nitrate (Havsed et al., 2021). More importantly, Corynebacterium is a dental health-associated base of plaque structure and community interactions (Schoilew et al., 2019). In this case, the lower abundance in the CPCS group led to a simpler correlation network in CPCS. Our data also demonstrated that Capnocytophaga correlated positively with Rothia in NCPCS. Therefore, several genera with the function of buffering pH, stabilizing plaque flora structure, and congregating with health-associated genera were found in the NCPCS group, which were quite different from those of the CPCS group. CPCS shaped a much simpler correlation network than NCPCS, which demonstrated that the SPM of caries in CP is more vulnerable. Its instability offers possibilities for a pH-driven shift of SPM in CPCS.
Although gastric acidity defends against the enteral transmission of oral bacteria, its influence is limited to acid-resistant oral bacteria (Kitamoto et al., 2020). Hezel and Weitzberg (2015) found that dental plaque interacts highly with gastrointestinal bacteria. Here, the aciduric bacteria abundant in CPCS such as P. intermedia, C. morbi, P. micra, F. nucleatum, and C. rectus have been extensively reported to colonize and proliferate in the gastrointestinal tract through hematogenous or enteral route (Aamdal-Scheie et al., 1996; Coker et al., 2018; Hu et al., 2018; Walker et al., 2018; Komiya et al., 2019; Kitamoto et al., 2020; Ortigão et al., 2020; Contaldo et al., 2021). P. endodontalis has been reported to be associated with gastric carcinogenesis, and its genus Porphyromonas has a key role in competing for colonization with the native genus in the small intestine (Hu et al., 2018; Li et al., 2019). P. endodontalis lacks noticeable aciduric ability, but as a specific pathogen of the dental pulp, it may access the gastrointestinal tract through the blood channel of pulp capillaries (Figure 7). In this context, SPM acts as a reservoir of these potential intestinal pathogens, causing microbial dysbiosis and chronic inflammation in gastrointestinal flora (Olsen and Hicks, 2020). The gut dysbiosis affects the permeability of gastrointestinal mucosa, perpetuates chronic inflammatory states, and reduces the production of beneficial SCFA (e.g., anticarcinogenic, anti-inflammatory properties) (Ding and Schloss, 2014; Coker et al., 2018; Kitamoto et al., 2020; Ortigão et al., 2020; Contaldo et al., 2021). Certain oral anaerobic genera such as Porphyromonas, Prevotella, and Fusobacterium are closely related to chronic inflammation of the gastrointestinal tract (Contaldo et al., 2021). Prevotella was found to be a member of “the core microbiota” of SPM in CP children, while it is also enriched in CP children’s gut microbiota according to a previous study (Huang et al., 2019). Thus, it is possible that the translocation of Prevotella occurs that promotes its persistence in the gastrointestinal tract of CP children or even induces gut dysbiosis. Given that microbes are capable of producing tryptophan metabolites (Rastelli et al., 2018) and SCFAs that can function as neurotransmitters, the imbalance in gut microbiota is highly likely to contribute to the etiology of neurodegenerative disorders and negatively influence neurodevelopment (Engevik and Versalovic, 2017; Mohajeri et al., 2018; Parker et al., 2020; Swann et al., 2020; Narengaowa et al., 2021; Roth et al., 2021). While the postural and other motor dysfunctions in CP patients are permanent but variable (Blair et al., 2007), these bacterial metabolites might exacerbate CP patients’ symptoms, especially at the age of brain development. Even though F. nucleatum had a positive correlation with C. morbi and C. rectus in NCPCS, the deficiency of F. nucleatum may restrain the overgrowth of C. morbi and C. rectus, thus decreasing their probability of translocating to the gastrointestinal tract. This warrants further study on F. nucleatum as a dental caries treatment target in CP patients by altering the oral–gut–brain communications, which in turn could reduce gastrointestinal diseases and neurological symptoms observed in CP children (Figure 7).
Unintentional movement of the limbs and head is one of the most common symptoms of CP patients, manifested as uncontrollable mastication and tongue stiffness, which brings difficulties to X-ray filming and intraoral surgery. During the intraoral operation, CP patients may bite on a handpiece, causing severe consequences such as pulp exposure, bur deformation, and even injuries to the operator (Al-Allaq et al., 2015). As a result, the caries treatment plan for CP patients tends to be tooth extraction (Camoin et al., 2020). The therapy of caries through microbiome-based intervention (by targeting the core “pathogenic” microbiota) might be an attractive alternative measure for CP patients. Nevertheless, if the oral environment caused by CP is not improved, its selective pressure on SPM will still lead to the development of dental caries based on the ecological plaque hypothesis. Since the SPM characteristics of CPCS are pH-driven, controlling factors that induce an acidic oral environment is recommended to ensure long-term remission of dental caries. Daily measures include enforcing strict limits on sugar content in the semisolid or liquid diet for CP patients and reasonably adding fluoride salt to their diet. In addition, it is advisable to plan diet intervals, carefully use drugs that can acidify saliva, and clean teeth with weak alkaline mouthwash after meals.
Several limitations and future work of this study ought to be taken into consideration. As it can be challenging to collect samples in CP children, DMFT/dmft was used in this experiment to grade the severity of caries, but it failed to assess the depth of caries and see whether there is a dental pulp lesion. Besides, the metabolites of oral flora in CP children and their potential pathogenic characteristics are yet to be investigated. Therefore, further investigations involving a larger sample size with saliva and fecal sample collection would enhance our understanding of the oral–gut–brain connections. Collecting saliva and feces samples simultaneously could also allow further investigation into the correlation between oral and gastrointestinal bacteria in CP children. Moreover, more clinical indicators of CP such as Gross Motor Function Classification System, Barthel index, Wee-FIM, and oral condition such as Oral Health index and Plaque index as well as their related risk factors (e.g., dietary structure, socioeconomic status of parents or guardians, family care) should be carefully considered to explore associations between CP, caries, and microbiota.
Conclusion
The study revealed a unique and vulnerable SPM in CPCS by comparing it with that of NCPCS and illustrating their core microbiota correlation networks. The subanalysis of CP children with different severities of caries displays the core SPM, as well as the caries-associated and non-caries-associated bacteria in CP children. Moreover, a potential pathogenic role of SPM in CP children via the oral–gut–brain axis is proposed. The characteristics of SPM also provide potential prevention and treatment measures for CP children with caries.
Data Availability Statement
The datasets presented in this study can be found in online repositories. The names of the repository/repositories and accession number(s) can be found below: https://db.cngb.org/, CNP0002325.
Ethics Statement
Written informed consent was obtained from the minors’ legal guardian/next of kin for the publication of any potentially identifiable images or data included in this article.
Author Contributions
ML, QJ, HS, and LW contributed to the project idea and research design. ML recruited the children and performed information collection, oral examination, and supragingival plaque sampling. ML, YS, and KW performed bioinformatics analysis. ML, YS, KW, and WX participated in analyzing the data, interpreting the results, and drafting the paper. ML and YS optimized the data output. LW, QJ, and HS scheduled and wrote and improved the article. All authors have read and approved the final article.
Funding
The work was supported by the 2020 College Students’ Innovative Entrepreneurial Training Plan Program in Guangdong province, China (No. S202010570033).
Conflict of Interest
The authors declare that the research was conducted in the absence of any commercial or financial relationships that could be construed as a potential conflict of interest.
Publisher’s Note
All claims expressed in this article are solely those of the authors and do not necessarily represent those of their affiliated organizations, or those of the publisher, the editors and the reviewers. Any product that may be evaluated in this article, or claim that may be made by its manufacturer, is not guaranteed or endorsed by the publisher.
Acknowledgments
We are grateful to all the participants in this study. We feel so appreciative that the doctors and nurses at the Maternity and Child Healthcare Hospital (Longgang, Shenzhen, China) assisted our team with cerebral palsy diagnosis, oral examinations, and the sample collection of supragingival plaque. In particular, Dr. Zhichao Zheng put forward many valuable suggestions for improvement and proposed different overall conceptual frameworks to help us produce high-quality research articles.
Supplementary Material
The Supplementary Material for this article can be found online at: https://www.frontiersin.org/articles/10.3389/fcimb.2022.814473/full#supplementary-material
Supplementary Table 1 | Dominant bacteria with significant difference in abundance between CPCS and NCPCS (%).
Supplementary Table 2 | Dominant bacteria with significant difference in abundance among CPCS, CPCM, and CPCF (%).
References
Aamdal-Scheie, A., Luan, W. M., Dahlén, G., Fejerskov, O. (1996). Plaque pH and Microflora of Dental Plaque on Sound and Carious Root Surfaces. J. Dent. Res. 75 (11), 1901–1908. doi: 10.1177/00220345960750111301
Akhter, R., Hassan, N. M., Martin, E. F., Muhit, M., Haque, M. R., Smithers-Sheedy, H., et al. (2017). Risk Factors for Dental Caries Among Children With Cerebral Palsy in a Low-Resource Setting. Dev. Med. Child Neurol. 59 (5), 538–543. doi: 10.1111/dmcn.13359
Al Agili, D. E. (2013). A Systematic Review of Population-Based Dental Caries Studies Among Children in Saudi Arabia. Saudi Dental J. 25 (1), 3–11. doi: 10.1016/j.sdentj.2012.10.002
Al-Allaq, T., DeBord, T. K., Liu, H., Wang, Y., Messadi, D. V. (2015). Oral Health Status of Individuals With Cerebral Palsy at a Nationally Recognized Rehabilitation Center. Spec. Care Dentist 35 (1), 15–21. doi: 10.1111/scd.12071
Al-Hebshi, N. N., Baraniya, D., Chen, T., Hill, J., Puri, S., Tellez, M., et al. (2019). Metagenome Sequencing-Based Strain-Level and Functional Characterization of Supragingival Microbiome Associated With Dental Caries in Children. J. Oral. Microbiol. 11 (1), 1557986. doi: 10.1080/20002297.2018.1557986
Alves, V., Cardoso, A., Cavalcanti, Y., Padilha, W. (2016). Efeito Sobre a Morfologia do Esmalte Dental E Análise Físico-Química De Medicamentos Utilizados Por Pacientes Pediátricos Com Paralisia Cerebral. Rev. Odontologia da UNESP 45 (4), 201–205. doi: 10.1590/1807-2577.27415
Anjugam, P., Mahesh Mathian, V., Gawthaman, M., Vinod, S., Yamuna Devi, E. (2021). Salivary Biomarker Levels and Oral Health Status of Children With Cerebral Palsy and Their Healthy Siblings: A Comparative Study. Rambam Maimonides Med. J. 12 (2), e0015. doi: 10.5041/rmmj.10437
Antezack, A., Boxberger, M., La Scola, B., Monnet-Corti, V. (2021). Isolation and Description of Sp. Nov., a Novel Species, Isolated From a Stable Periodontitis Subject. Pathog. (Basel Switzerland) 10 (3). doi: 10.3390/pathogens10030367
Arvedson, J. C. (2013). Feeding Children With Cerebral Palsy and Swallowing Difficulties. Eur. J. Clin. Nutr. 67 Suppl 2, S9–12. doi: 10.1038/ejcn.2013.224
Benfer, K. A., Weir, K. A., Bell, K. L., Ware, R. S., Davies, P. S. W., Boyd, R. N. (2012). Longitudinal Cohort Protocol Study of Oropharyngeal Dysphagia: Relationships to Gross Motor Attainment, Growth and Nutritional Status in Preschool Children With Cerebral Palsy. BMJ Open 2 (4), e001460. doi: 10.1136/bmjopen-2012-001460
Bensi, C., Costacurta, M., Docimo, R. (2020). Oral Health in Children With Cerebral Palsy: A Systematic Review and Meta-Analysis. Spec. Care Dentist 40 (5), 401–411. doi: 10.1111/scd.12506
Bizhang, M., Ellerbrock, B., Preza, D., Raab, W., Singh, P., Beikler, T., et al. (2011). Detection of Nine Microorganisms From the Initial Carious Root Lesions Using a TaqMan-Based Real-Time PCR. Oral. Dis. 17 (7), 642–652. doi: 10.1111/j.1601-0825.2011.01815.x
Blair, E., Badawi, N., Watson, L. (2007). Definition and Classification of the Cerebral Palsies: The Australian View. Dev. Med. Child Neurol. Suppl. 109, 33–34. doi: 10.1111/j.1469-8749.2007.tb12625.x
Blumberg, R., Powrie, F. (2012). Microbiota, Disease, and Back to Health: A Metastable Journey. Sci. Transl. Med. 4 (137), 137rv137. doi: 10.1126/scitranslmed.3004184
Camoin, A., Tardieu, C., Dany, L., Saliba-Serre, B., Faulks, D., Coz, P. L. (2020). Inequalities in Treatment Planning for Children With Intellectual Disabilities: A Questionnaire Study of Dentists in Europe. Spec. Care Dentist 40 (4), 356–363. doi: 10.1111/scd.12482
Chhour, K. L., Nadkarni, M. A., Byun, R., Martin, F. E., Jacques, N. A., Hunter, N. (2005). Molecular Analysis of Microbial Diversity in Advanced Caries. J. Clin. Microbiol. 43 (2), 843–849. doi: 10.1128/jcm.43.2.843-849.2005
Coker, M. O., Akhigbe, P., Osagie, E., Idemudia, N. L., Igedegbe, O., Chukwumah, N., et al. (2021). Dental Caries and its Association With the Oral Microbiomes and HIV in Young Children-Nigeria (DOMHaIN): A Cohort Study. BMC Oral. Health 21 (1), 620. doi: 10.1186/s12903-021-01944-y
Coker, O. O., Dai, Z., Nie, Y., Zhao, G., Cao, L., Nakatsu, G., et al. (2018). Mucosal Microbiome Dysbiosis in Gastric Carcinogenesis. Gut 67 (6), 1024–1032. doi: 10.1136/gutjnl-2017-314281
Contaldo, M., Fusco, A., Stiuso, P., Lama, S., Gravina, A. G., Itro, A., et al. (2021). Oral Microbiota and Salivary Levels of Oral Pathogens in Gastro-Intestinal Diseases: Current Knowledge and Exploratory Study. Microorganisms 9 (5), 1064. doi: 10.3390/microorganisms9051064
De Camargo, M. A., Antunes, J. L. (2008). Untreated Dental Caries in Children With Cerebral Palsy in the Brazilian Context. Int. J. Paediatr. Dent. 18 (2), 131–138. doi: 10.1111/j.1365-263X.2007.00829.x
Deo, P. N., Deshmukh, R. (2019). Oral Microbiome: Unveiling the Fundamentals. J. Oral. Maxillofac Pathol. 23 (1), 122–128. doi: 10.4103/jomfp.JOMFP_304_18
DeSantis, T. Z., Hugenholtz, P., Larsen, N., Rojas, M., Brodie, E. L., Keller, K., et al. (2006). Greengenes, a Chimera-Checked 16S rRNA Gene Database and Workbench Compatible With ARB. Appl. Environ. Microbiol. 72 (7), 5069–5072. doi: 10.1128/aem.03006-05
Ding, T., Schloss, P. D. (2014). Dynamics and Associations of Microbial Community Types Across the Human Body. Nature 509 (7500), 357–360. doi: 10.1038/nature13178
Dos Santos, M. T., Masiero, D., Simionato, M. R. (2002). Risk Factors for Dental Caries in Children With Cerebral Palsy. Spec. Care Dentist 22 (3), 103–107. doi: 10.1111/j.1754-4505.2002.tb01171.x
Dos Santos, M. T., Nogueira, M. L. (2005). Infantile Reflexes and Their Effects on Dental Caries and Oral Hygiene in Cerebral Palsy Individuals. J. Oral. Rehabil. 32 (12), 880–885. doi: 10.1111/j.1365-2842.2005.01518.x
Edgar, R. C. (2013). UPARSE: Highly Accurate OTU Sequences From Microbial Amplicon Reads. Nat. Methods 10 (10), 996–998. doi: 10.1038/nmeth.2604
Engevik, M. A., Versalovic, J. (2017). Biochemical Features of Beneficial Microbes: Foundations for Therapeutic Microbiology. Microbiol. Spectr. 5 (5), BAD-0012-2016. doi: 10.1128/microbiolspec.BAD-0012-2016
Espinoza, J. L., Harkins, D. M., Torralba, M., Gomez, A., Highlander, S. K., Jones, M. B., et al. (2018). Supragingival Plaque Microbiome Ecology and Functional Potential in the Context of Health and Disease. mBio 9 (6), 1–21. doi: 10.1128/mBio.01631-18
Ferreira, A., Eveloff, R. J., Freire, M., Santos, M. (2021a). The Impact of Oral-Gut Inflammation in Cerebral Palsy. Front. Immunol. 12. doi: 10.3389/fimmu.2021.619262
Ferreira, A., Freire, M., Siqueira, V., Ferreira, C., Santos, M. (2021b). Brain Injury and Neuroinflammation of the Gut-Brain Axis in Subjects With Cerebral Palsy. doi: 10.5772/intechopen.95763
Gajewska, E., Sobieska, M., Samborski, W. (2014). Associations Between Manual Abilities, Gross Motor Function, Epilepsy, and Mental Capacity in Children With Cerebral Palsy. Iran J. Child Neurol. 8 (2), 45–52. doi: 10.22037/ijcn.v8i2.4735
Graham, H. K., Rosenbaum, P., Paneth, N., Dan, B., Lin, J. P., Damiano, D. L., et al. (2016). Cerebral Palsy. Nat. Rev. Dis. Primers 2, 15082. doi: 10.1038/nrdp.2015.82
Gross, E. L., Leys, E. J., Gasparovich, S. R., Firestone, N. D., Schwartzbaum, J. A., Janies, D. A., et al. (2010). Bacterial 16S Sequence Analysis of Severe Caries in Young Permanent Teeth. J. Clin. Microbiol. 48 (11), 4121–4128. doi: 10.1128/jcm.01232-10
Hahn, C. L., Best, A. M., Tew, J. G. (2004). Comparison of Type 1 and Type 2 Cytokine Production by Mononuclear Cells Cultured With Streptococcus Mutans and Selected Other Caries Bacteria. J. Endod. 30 (5), 333–338. doi: 10.1097/00004770-200405000-00007
Havsed, K., Stensson, M., Jansson, H., Carda-Diéguez, M., Pedersen, A., Neilands, J., et al. (2021). Bacterial Composition and Metabolomics of Dental Plaque From Adolescents. Front. Cell. Infect. Microbiol. 11. doi: 10.3389/fcimb.2021.716493
He, J., Tu, Q., Ge, Y., Qin, Y., Cui, B., Hu, X., et al. (2018). Taxonomic and Functional Analyses of the Supragingival Microbiome From Caries-Affected and Caries-Free Hosts. Microb. Ecol. 75 (2), 543–554. doi: 10.1007/s00248-017-1056-1
Hezel, M. P., Weitzberg, E. (2015). The Oral Microbiome and Nitric Oxide Homoeostasis. Oral. Dis. 21 (1), 7–16. doi: 10.1111/odi.12157
Horiuchi, A., Kokubu, E., Warita, T., Ishihara, K. (2020). Synergistic Biofilm Formation by Parvimonas Micra and Fusobacterium Nucleatum. Anaerobe 62, 102100. doi: 10.1016/j.anaerobe.2019.102100
Huang, C., Li, Y., Feng, X., Li, D., Li, X., Ouyang, Q., et al. (2019). Distinct Gut Microbiota Composition and Functional Category in Children With Cerebral Palsy and Epilepsy. Front. Pediatr. 7. doi: 10.3389/fped.2019.00394
Huang, C., Li, X., Wu, L., Wu, G., Wang, P., Peng, Y., et al. (2021). The Effect of Different Dietary Structure on Gastrointestinal Dysfunction in Children With Cerebral Palsy and Epilepsy Based on Gut Microbiota. Brain Dev. 43 (2), 192–199. doi: 10.1016/j.braindev.2020.09.013
Hu, Y. L., Pang, W., Huang, Y., Zhang, Y., Zhang, C. J. (2018). The Gastric Microbiome Is Perturbed in Advanced Gastric Adenocarcinoma Identified Through Shotgun Metagenomics. Front. Cell Infect. Microbiol. 8. doi: 10.3389/fcimb.2018.00433
Jiang, W., Ling, Z., Lin, X., Chen, Y., Zhang, J., Yu, J., et al. (2014). Pyrosequencing Analysis of Oral Microbiota Shifting in Various Caries States in Childhood. Microb. Ecol. 67 (4), 962–969. doi: 10.1007/s00248-014-0372-y
Kelly, J. R., Kennedy, P. J., Cryan, J. F., Dinan, T. G., Clarke, G., Hyland, N. P. (2015). Breaking Down the Barriers: The Gut Microbiome, Intestinal Permeability and Stress-Related Psychiatric Disorders. Front. Cell. Neurosci. 9. doi: 10.3389/fncel.2015.00392
Kianoush, N., Adler, C. J., Nguyen, K.-A. T., Browne, G. V., Simonian, M., Hunter, N. (2014). Bacterial Profile of Dentine Caries and the Impact of pH on Bacterial Population Diversity. PloS One 9 (3), e92940. doi: 10.1371/journal.pone.0092940
Kitamoto, S., Nagao-Kitamoto, H., Hein, R., Schmidt, T. M., Kamada, N. (2020). The Bacterial Connection Between the Oral Cavity and the Gut Diseases. J. Dent. Res. 99 (9), 1021–1029. doi: 10.1177/0022034520924633
Komiya, Y., Shimomura, Y., Higurashi, T., Sugi, Y., Arimoto, J., Umezawa, S., et al. (2019). Patients With Colorectal Cancer Have Identical Strains of Fusobacterium Nucleatum in Their Colorectal Cancer and Oral Cavity. Gut 68 (7), 1335–1337. doi: 10.1136/gutjnl-2018-316661
Latti, B. R., Kalburge, J. V., Birajdar, S. B., Latti, R. G. (2018). Evaluation of Relationship Between Dental Caries, Diabetes Mellitus and Oral Microbiota in Diabetics. J. Oral. Maxillofac Pathol. 22 (2), 282. doi: 10.4103/jomfp.JOMFP_163_16
Lee, L. H., Ser, H. L., Khan, T., Gan, K.-G., Goh, B. H., Ab Mutalib, N.-S. (2019). IDDF2019-ABS-0321 Relationship Between Autism and Gut Microbiome: Current Status and Update Gut 68, A40.42–A40A41. doi: 10.1136/gutjnl-2019-IDDFAbstracts.76
Lee, L.-H., Ser, H.-L., Khan, T. M., Long, M., Chan, K.-G., Goh, B.-H., et al. (2018). IDDF2018-ABS-0239 Dissecting the Gut and Brain: Potential Links Between Gut Microbiota in Development of Alzheimer’s Disease? Gut 67, A18–A18. doi: 10.1136/gutjnl-2018-IDDFabstracts.37
Li, B., Ge, Y., Cheng, L., Zeng, B., Yu, J., Peng, X., et al. (2019). Oral Bacteria Colonize and Compete With Gut Microbiota in Gnotobiotic Mice. Int. J. Oral. Sci. 11 (1), 10. doi: 10.1038/s41368-018-0043-9
Lima, K. C., Coelho, L. T., Pinheiro, I. V., Rôças, I. N., Siqueira, J. F., Jr. (2011). Microbiota of Dentinal Caries as Assessed by Reverse-Capture Checkerboard Analysis. Caries Res. 45 (1), 21–30. doi: 10.1159/000322299
Li, Y., Zou, C. G., Fu, Y., Li, Y., Zhou, Q., Liu, B., et al. (2016). Oral Microbial Community Typing of Caries and Pigment in Primary Dentition. BMC Genomics 17, 558. doi: 10.1186/s12864-016-2891-z
Maitre, Y., Micheneau, P., Delpierre, A., Mahalli, R., Guerin, M., Amador, G., et al. (2020). Did the Brain and Oral Microbiota Talk to Each Other? A Review of the Literature. J. Clin. Med. 9 (12), 3879. doi: 10.3390/jcm9123876
Malta, C. P., Barcelos, R. C. S., Rosa, H. Z., Burger, M. E., Bento, L. W. (2020). Effect of Cerebral Palsy and Dental Caries on Dental Plaque Index, Salivary Parameters and Oxidative Stress in Children and Adolescents. Eur. Arch. Paediatr. Dent. doi: 10.1007/s40368-020-00509-x
Meijers, B., Evenepoel, P., Anders, H. J. (2019). Intestinal Microbiome and Fitness in Kidney Disease. Nat. Rev. Nephrol. 15 (9), 531–545. doi: 10.1038/s41581-019-0172-1
Michael-Asalu, A., Taylor, G., Campbell, H., Lelea, L. L., Kirby, R. S. (2019). Cerebral Palsy: Diagnosis, Epidemiology, Genetics, and Clinical Update. Adv. Pediatr. 66, 189–208. doi: 10.1016/j.yapd.2019.04.002
Mohajeri, M. H., La Fata, G., Steinert, R. E., Weber, P. (2018). Relationship Between the Gut Microbiome and Brain Function. Nutr. Rev. 76 (7), 481–496. doi: 10.1093/nutrit/nuy009
Narengaowa, Kong, W., Lan, F., Awan, U. F., Qing, H., Ni, J. (2021). The Oral-Gut-Brain AXIS: The Influence of Microbes in Alzheimer's Disease. Front. Cell Neurosci. 15. doi: 10.3389/fncel.2021.633735
National Guideline, A. (2017). “"National Institute for Health and Care Excellence: Guidelines,",” in Cerebral Palsy in Under 25s: Assessment and Management (London: National Institute for Health and Care Excellence (NICE)Copyright National Institute for Health and Care Excellence 2017).
Oksanen, J., Blanchet, F. G., Kindt, R., Legendre, P., Minchin, P., O'Hara, B., et al. (2015). Vegan: Community Ecology Package. R Package Version 2. 2–1 2, 1-2.
Olsen, I., Hicks, S. D. (2020). Oral Microbiota and Autism Spectrum Disorder (ASD). J. Oral. Microbiol. 12 (1), 1702806. doi: 10.1080/20002297.2019.1702806
Orr, M. E., Reveles, K. R., Yeh, C. K., Young, E. H., Han, X. (2020). Can Oral Health and Oral-Derived Biospecimens Predict Progression of Dementia? Oral. Dis. 26 (2), 249–258. doi: 10.1111/odi.13201
Ortigão, R., Pimentel-Nunes, P., Dinis-Ribeiro, M., Libânio, D. (2020). Gastrointestinal Microbiome - What We Need to Know in Clinical Practice. GE Port J. Gastroenterol. 27 (5), 336–351. doi: 10.1159/000505036
Parker, A., Fonseca, S., Carding, S. R. (2020). Gut Microbes and Metabolites as Modulators of Blood-Brain Barrier Integrity and Brain Health. Gut Microbes 11 (2), 135–157. doi: 10.1080/19490976.2019.1638722
Rastelli, M., Knauf, C., Cani, P. D. (2018). Gut Microbes and Health: A Focus on the Mechanisms Linking Microbes, Obesity, and Related Disorders. Obes. (Silver Spring) 26 (5), 792–800. doi: 10.1002/oby.22175
Ribeiro, A. A., Azcarate-Peril, M. A., Cadenas, M. B., Butz, N., Paster, B. J., Chen, T., et al. (2017). The Oral Bacterial Microbiome of Occlusal Surfaces in Children and its Association With Diet and Caries. PloS One 12 (7), e0180621. doi: 10.1371/journal.pone.0180621
Rôças, I. N., Lima, K. C., Assunção, I. V., Gomes, P. N., Bracks, I. V., Siqueira, J. F., Jr. (2015). Advanced Caries Microbiota in Teeth With Irreversible Pulpitis. J. Endod. 41 (9), 1450–1455. doi: 10.1016/j.joen.2015.05.013
Roth, W., Zadeh, K., Vekariya, R., Ge, Y., Mohamadzadeh, M. (2021). Tryptophan Metabolism and Gut-Brain Homeostasis. Int. J. Mol. Sci. 22 (6), 15. doi: 10.3390/ijms22062973
Sansores-España, L., Melgar-Rodríguez, S., Olivares-Sagredo, K., Cafferata, E., Martínez-Aguilar, V., Vernal, R., et al. (2021). Oral-Gut-Brain Axis in Experimental Models of Periodontitis: Associating Gut Dysbiosis With Neurodegenerative Diseases. Front. Aging 2. doi: 10.3389/fragi.2021.781582
Sato, Y., Yamagishi, J., Yamashita, R., Shinozaki, N., Ye, B., Yamada, T., et al. (2015). Inter-Individual Differences in the Oral Bacteriome Are Greater Than Intra-Day Fluctuations in Individuals. PloS One 10 (6), e0131607. doi: 10.1371/journal.pone.0131607
Schloss, P. D., Westcott, S. L., Ryabin, T., Hall, J. R., Hartmann, M., Hollister, E. B., et al. (2009). Introducing Mothur: Open-Source, Platform-Independent, Community-Supported Software for Describing and Comparing Microbial Communities. Appl. Environ. Microbiol. 75 (23), 7537–7541. doi: 10.1128/aem.01541-09
Schoilew, K., Ueffing, H., Dalpke, A., Wolff, B., Frese, C., Wolff, D., et al. (2019). Bacterial Biofilm Composition in Healthy Subjects With and Without Caries Experience. J. Oral. Microbiol. 11 (1), 1633194. doi: 10.1080/20002297.2019.1633194
Schulze-Schweifing, K., Banerjee, A., Wade, W. G. (2014). Comparison of Bacterial Culture and 16S rRNA Community Profiling by Clonal Analysis and Pyrosequencing for the Characterization of the Dentine Caries-Associated Microbiome. Front. Cell Infect. Microbiol. 4. doi: 10.3389/fcimb.2014.00164
Selwitz, R. H., Ismail, A. I., Pitts, N. B. (2007). Dental Caries. Lancet 369 (9555), 51–59. doi: 10.1016/s0140-6736(07)60031-2
Senneby, A., Davies, J. R., Svensäter, G., Neilands, J. (2017). Acid Tolerance Properties of Dental Biofilms In Vivo. BMC Microbiol. 17 (1), 165. doi: 10.1186/s12866-017-1074-7
Shannon, P., Markiel, A., Ozier, O., Baliga, N. S., Wang, J. T., Ramage, D., et al. (2003). Cytoscape: A Software Environment for Integrated Models of Biomolecular Interaction Networks. Genome Res. 13 (11), 2498–2504. doi: 10.1101/gr.1239303
Sizova, M. V., Muller, P., Panikov, N., Mandalakis, M., Hohmann, T., Hazen, A., et al. (2013). Stomatobaculum Longum Gen. Nov., Sp. Nov., an Obligately Anaerobic Bacterium From the Human Oral Cavity. Int. J. System. Evol. Microbiol. 63 (Pt 4), 1450–1456. doi: 10.1099/ijs.0.042812-0
Swann, J. R., Spitzer, S. O., Diaz Heijtz, R. (2020). Developmental Signatures of Microbiota-Derived Metabolites in the Mouse Brain. Metabolites 10 (5), 172. doi: 10.3390/metabo10050172
Takahashi, N., Saito, K., Schachtele, C. F., Yamada, T. (1997). Acid Tolerance and Acid-Neutralizing Activity of Porphyromonas Gingivalis, Prevotella Intermedia and Fusobacterium Nucleatum. Oral. Microbiol. Immunol. 12 (6), 323–328. doi: 10.1111/j.1399-302x.1997.tb00733.x
Teng, F., Yang, F., Huang, S., Bo, C., Xu, Z. Z., Amir, A., et al. (2015). Prediction of Early Childhood Caries via Spatial-Temporal Variations of Oral Microbiota. Cell Host Microbe 18 (3), 296–306. doi: 10.1016/j.chom.2015.08.005
Thorburn, K., Jardine, M., Taylor, N., Reilly, N., Sarginson, R. E., van Saene, H. K. (2009). Antibiotic-Resistant Bacteria and Infection in Children With Cerebral Palsy Requiring Mechanical Ventilation. Pediatr. Crit. Care Med. 10 (2), 222–226. doi: 10.1097/PCC.0b013e31819368ac
Walker, M. Y., Pratap, S., Southerland, J. H., Farmer-Dixon, C. M., Lakshmyya, K., Gangula, P. R. (2018). Role of Oral and Gut Microbiome in Nitric Oxide-Mediated Colon Motility. Nitric. Oxide 73, 81–88. doi: 10.1016/j.niox.2017.06.003
World Health, O. (1997). "Oral Health Surveys : Basic Methods". 4th ed (Geneva: World Health Organization).
Wu, B., Fillenbaum, G. G., Plassman, B. L., Guo, L. (2016). Association Between Oral Health and Cognitive Status: A Systematic Review. J. Am. Geriatr. Soc. 64 (4), 739–751. doi: 10.1111/jgs.14036
Wyne, A. H., Al-Hammad, N. S., Splieth, C. H. (2017). Dental Caries and Related Risk Factors in Saudi Cerebral Palsy Children. Neurosci. (Riyadh) 22 (4), 282–286. doi: 10.17712/nsj.2017.4.20170191
Yang, X., He, L., Yan, S., Chen, X., Que, G. (2021). The Impact of Caries Status on Supragingival Plaque and Salivary Microbiome in Children With Mixed Dentition: A Cross-Sectional Survey. BMC Oral. Health 21 (1), 319. doi: 10.1186/s12903-021-01683-0
Keywords: cerebral palsy, caries, supragingival plaque microbiota, correlation network, oral–brain connection, 16S rRNA sequencing
Citation: Liu M, Shi Y, Wu K, Xie W, Ser H-L, Jiang Q and Wu L (2022) From Mouth to Brain: Distinct Supragingival Plaque Microbiota Composition in Cerebral Palsy Children With Caries. Front. Cell. Infect. Microbiol. 12:814473. doi: 10.3389/fcimb.2022.814473
Received: 21 November 2021; Accepted: 14 March 2022;
Published: 11 April 2022.
Edited by:
Huajun Zheng, Shanghai Institute for Biomedical and Pharmaceutical Technologies, ChinaReviewed by:
Nar Singh Chauhan, Maharshi Dayanand University, IndiaArmelia Sari Widyarman, Trisakti University, Indonesia
Copyright © 2022 Liu, Shi, Wu, Xie, Ser, Jiang and Wu. This is an open-access article distributed under the terms of the Creative Commons Attribution License (CC BY). The use, distribution or reproduction in other forums is permitted, provided the original author(s) and the copyright owner(s) are credited and that the original publication in this journal is cited, in accordance with accepted academic practice. No use, distribution or reproduction is permitted which does not comply with these terms.
*Correspondence: Lihong Wu, wcanhong@163.com; Qianzhou Jiang, 958276432@qq.com; Hooi-Leng Ser, hooilengs@sunway.edu.my