- 1Department of Microbiology, Harbin Medical University, Harbin, China
- 2Department of Pathophysiology, Harbin Medical University, Harbin, China
- 3Wu Lien-Teh Institute, Harbin Medical University, Harbin, China
- 4Heilongjiang Provincial Key Laboratory of Infection and Immunity, Harbin, China
- 5Key Laboratory of Pathogen Biology, Harbin, China
The effects of hydrogen sulfide (H2S) on diabetic metabolic disorders are still controversial, and the mechanisms underlying these effects remain largely unknown. This study was conducted to investigate the potential relationship between the gut microbiota and the improvement of diabetic metabolic disorders by exogenous H2S in obese db/db mice. The db/db mice were treated with sodium hydrosulfide (NaHS) (80 μmol/kg), or vehicle for 16 weeks, respectively. We measured the serum H2S, obesity parameters, glucose homeostasis, and triglyceride. The sequencing of bacterial 16S rRNA gene and fungal internal transcribed spacer (ITS) in the cecal contents of NaHS-treated mice was performed to evaluate the gut microbial communities. We found that supplying exogenous H2S for 16 weeks significantly inhibited the increase of serum triglyceride, blood glucose, and insulin levels and altered specifically the gut bacterial microbiota structure in db/db mice. The relative abundance of some bacterial genera was correlated with the H2S or blood glucose level. Indeed, exogenous H2S increased Firmicutes and decreased Bacteroidetes at the phylum level along with changes of abundance of multifarious genera. Among them, Unclassified_Enterobacteriaceae, Prevotella, and Lactobacillus decreased and Unclassified_Ruminococcaceae, Oscillospira, Ruminococcus, Sutterella, and Desulfovibrio increased. For fungi, exogenous H2S decreased the abundance of Candida and Aspergillus. Here we demonstrated that, in diabetes, microbial dysbiosis may not be just limited to bacteria due to the inter-linked metabolic interactions among bacteria and fungi in the gut. The beneficial effects of exogenous H2S on diabetic metabolic disorders are likely associated with the alterations of specific microbiota.
Introduction
Recently, increasing evidence strongly supports that the abnormal composition of gut microbiota is closely associated with obesity or diabetes (Scheithauer et al., 2020; Arora et al., 2021; Fang et al., 2021). Meanwhile, probiotics (Kashiwagi et al., 2021), prebiotics (Zheng et al., 2018), and dietary supplements (Chen et al., 2019; Li et al., 2020) have been used in animal studies to assess the effects of altered gut microbiota on obesity and type 2 diabetes.
Leptin receptor-deficient mice (Db/db) mice have been reported with microbiota disorders showing that Firmicutes decreased significantly in abundance, which is the most abundant phylum in db/m mice. Several bacterial taxa, including Lactobacillus and some Bacteroides, were less abundant, and Akkermansia muciniphila was more abundant in db/db mice (Singh et al., 2020). In addition, sulfur-containing metabolites are significantly altered and play a key role in type 2 diabetes metabolism in db/db mice (Walker et al., 2014).
Hydrogen sulfide (H2S), as a new gasotransmitter in the body, plays an important role in diverse physiological functions, such as antioxidative stress, anti-inflammation, and anti-hypertension (Sokolov et al., 2021). Some studies have shown that H2S levels in the blood circulation decreased significantly in the diabetic animal models (Jain et al., 2010; Suzuki et al., 2011), obese people (Whiteman et al., 2010), and patients with type 2 diabetes (Jain et al., 2010; Whiteman et al., 2010).
To date, accumulative evidence from animal models demonstrated that supplying exogenous H2S donors is beneficial for the recovery of diabetes-related disorders (Wallace and Wang, 2015; Li et al., 2021; Zhang et al., 2021). Nevertheless, some studies have shown various effects of exogenous H2S in different models associated with metabolic disorder. In Goto-Kakizaki (GK) diabetic rats, chronic NaHS treatment (30 μmol·kg-1·day-1) decreased fasting blood glucose, increased insulin sensitivity, and increased glucose tolerance (Xue et al., 2013). Similar insulin-sensitizing effects of NaHS treatment were also observed in Wistar rats (Xue et al., 2013). Type 2 diabetes is often accompanied by obesity. Wu et al. employed a diet-induced obesity (DIO) mouse model to find that the administration of H2S donor NaHS could significantly recover the hepatic structure and decrease the accumulation of lipids including triglyceride and total cholesterol in high-fat diet (HFD)-induced obese mice (Wu et al., 2015). Recent studies have shown that db/db mice treated with NaHS for 10 weeks displayed improvements in glucose tolerance and serum insulin levels but did not alter the increase in body weight or serum triglyceride levels (Sun et al., 2018). However, different results were reported showing that there was no effect on blood glucose, serum lipids, and glucose tolerance in db/db mice after NaHS injection (Wu et al., 2017). Chronic administration of NaHS in particular at high doses impaired carbohydrate metabolism in type 2 diabetic rats (Gheibi et al., 2019). Consistent with this, the dose of NaHS at 120 μmol·kg-1·day-1 did not exert an insulin-sensitizing effect in GK rats (Xue et al., 2013). Taken together, the differences in diabetic model, NaHS dosage, or treatment course could be considered in influence factors of H2S effect on diabetes.
In recent years, it has been found that exogenous H2S donors appear to be able to influence the gut microbiota (Blackler et al., 2015; Motta et al., 2015; Walsh et al., 2020). For instance, exogenous H2S donors protect against NSAID-induced enteropathy through modulation of the microbiota which caused a significant decrease in multiple Clostridiales families, such as Ruminococcaceae and Eubacteriaceae, and an increase in abundance of Mucispirillum (Blackler et al., 2015). Low levels of endogenous or exogenous H2S directly stabilize mucus layers, prevent fragmentation and adherence of the microbiota biofilm to the epithelium, inhibit the release of invasive pathobionts, and help resolve inflammation and tissue injury (Motta et al., 2015). The cross talk between H2S, the gut microbiota, and health has been the topics of recent reviews (Buret et al., 2022). Other studies have reported that dietary H2S may also modulate the abundance and function of microbiota (Burrichter et al., 2018; Frommeyer et al., 2020). However, there are still limited data which are available to verify the effects of H2S on the gut microbiota. Although we may propose that exogenous H2S may help resolve inflammation and tissue injury in gut by stabilizing mucus layers and prevent fragmentation of the biofilm, extensive studies are needed to characterize changes in microbiome in the context of exposure to exogenous H2S.
Therefore, an exploration of whether and how H2S beneficially modifies the gut microbial profile and its effects on metabolism will provide supports for the therapeutic application of exogenous H2S and probiotics on type 2 diabetes metabolism. To this end, we aimed to investigate the potential relationship between the regulation of obesity as well as glucose homeostasis by exogenous H2S and the alterations of gut microbiota in obese db/db mice.
Materials and Methods
Experimental Animals and Treatment
Wild-type male C57BL/6 mice and leptin receptor-deficient (db/db) mice with the same background (10 weeks old) were purchased from the Animal Laboratory Centre of Nanjing University (Nanjing, China). The animals were housed in a climate- and temperature-controlled room, on a 12-h light/dark cycle. The mice were maintained on a standard diet and water ad libitum.
In vivo, H2S exists in two forms: 1/3 in the form of gas H2S and 2/3 in the form of sodium hydrosulfide (NaHS), with a dynamic equilibrium between H2S and NaHS (Hosoki et al., 1997). Therefore, NaHS is often used as a donor for exogenous H2S in experiments (Sun et al., 2018). The db/db mice treated with NaHS (80 μmol/kg; Sigma, St. Louis, MO, USA) by intraperitoneal injection every 2 days for 16 weeks (n = 6) or with equal amounts of saline (n = 6) were allocated to DB-H2S or DB group, respectively. Wild-type mice treated with saline were used as WT group (n = 6) (Sun et al., 2018).
All mice were visually inspected every day, and the body weight was recorded once a week. At the end of the treatment, all mice were euthanized via diethyl ether-induced anesthesia. The mouse serum and cecal contents were collected for the analyses of biochemical parameters and gut microbiota, respectively. Usually, in mice, the major fermentation capacity in cecum may impact on the diversity and composition of gut microbial communities that are responsible not only for the fermentation of indigestible food components but also for the production of essential complements to the host, such as vitamin K and B and short-chain fatty acids (Nguyen et al., 2015). Therefore, cecal contents are usually used in mouse gut microbiome studies (with the exception of longitudinal studies, where pellets are sampled) (Everard et al., 2014; Lambert et al., 2015; Lin et al., 2018).
The animal experiments were performed according to the Guide for the Care and Use of Laboratory Animals published by the China National Institutes of Health and approved by the Animal Care Committees of Harbin Medical University, China.
Random Blood Glucose and Oral Glucose Tolerance Test
We randomly measured the blood glucose levels of blood samples obtained from the tail every week to verify the development of diabetes. The mice were fasted for 12 h and were administered with 50% glucose solution (2 g/kg) by gavage for the oral glucose tolerance test (OGTT). Tail vein blood was collected at 0, 15, 30, 60, 90, and 120 min after the administration. All blood samples were tested using a glucometer (Bayer, Leverkusen, Germany) (Ma et al., 2017).
Biochemical Analyses
The measurement of H2S production in serum followed the established protocol (Kang et al., 2009). Briefly, serum was mixed with 10% trichloroacetic acid. The reaction was stopped by 1% zinc acetate, followed by incubation with N,N-dimethyl-p-phenylenediamine sulfate (DPD) for 15 min. The absorbance at 670 nm was measured with a spectrophotometer (Thermo Fisher Scientific, Waltham, MA, USA).
Then serum triglyceride was determined by using the mouse triglyceride (TG) ELISA Kit (Mlbio, Shanghai, China) and serum insulin was measured with the mouse insulin (INS) ELISA kit (Mlbio, Shanghai, China) according to the manufacturers’ instructions.
DNA Isolation and Sequencing of Bacterial 16S rRNA and Fungal Internal Transcribed Spacer (ITS) Gene
Total DNA of cecal contents was extracted by using the FastDNA SPIN Extraction Kit (MP Biomedicals, Santa Ana, CA, USA). The quantity and quality of extracted DNA were measured using a spectrophotometer (Thermo Fisher Scientific, Waltham, MA, USA) and agarose gel electrophoresis, respectively.
The V3–V4 regions of the bacterial 16S rRNA genes were amplified using primers 338F (5′-ACTCCTACGGGAGGCAGCA-3′) and 806R (5′-GGACTACHVGGGTWTCTAAT-3′). The internal transcribed spacer (ITS) regions of fungi were amplified with primers ITS5F (5′-GGAAGTAAAAGTCGTAACAAGG-3′) and ITS1R (5′-GCTGCGTTCTTCATCGATGC-3′). Sample-specific 7-bp barcodes were incorporated into the primers for multiplex sequencing. The detailed PCR components and procedures were conducted according to a previous method (Zhou et al., 2019). PCR amplicons were purified using Agencourt AMPure Beads (Beckman Coulter, Indianapolis, IN, USA) and quantified with the PicoGreen dsDNA Assay Kit (Invitrogen, Carlsbad, CA, USA). After the individual quantification step, amplicons were pooled in equal amounts and subjected to paired-end 2 × 300-bp sequencing using the Illumina MiSeq platform and the MiSeq Reagent Kit v3 from Shanghai Personal Biotechnology Co., Ltd. (Shanghai, China).
Sequencing Data Processing and Analyses
Sequencing data were processed using a quantitative analysis of microbial ecology (QIIME, v1.8.0). Briefly, raw sequencing reads that exactly matched the barcode were assigned to the corresponding samples and identified as valid sequences. The low-quality sequences (length < 150 bp, average Phred scores < 20, containing ambiguous bases, and single-nucleotide repeats > 8 bp) were filtered (Gill et al., 2006; Chen and Jiang, 2014). Paired-end reads were assembled using FLASH (Magoc and Salzberg, 2011). After chimera detection, the remaining high-quality sequences were clustered into operational taxonomic units (OTUs) at 97% sequence identity by UCLUST (Edgar, 2010). The default parameters were used to select the representative sequence from each OTU. OTU taxonomy classification was performed by BLAST searching the representative set of sequences against the Greengenes database (DeSantis et al., 2006). An OTU table was further generated to record the abundance of each OTU in each sample and the taxonomy of these OTUs. OTUs with a total content of less than 0.001% in all samples were discarded (Bokulich et al., 2013).
Sequencing data analyses were mainly performed using QIIME (version 1.8.0) and R packages (version 3.2.0). OTU-level alpha-diversity indices including Chao1 and Shannon were calculated using the OTU table in QIIME. Rarefaction plots were generated with iterations of 20 at each sampling depth of 10 and increments of 500. The unweighted and weighted UniFrac distance matrices were calculated and used for principal coordinate analysis (PCoA), and analysis of similarities (ANOSIM) was processed in QIIME. Bubble charts containing bacterial genus taxa with a relative abundance ≥ 0.1% and fungal genus taxa with a relative abundance ≥ 0.5% in at least one group were created by R software.
The sequences generated in this study are available in the National Center for Biotechnology Information (NCBI) Sequence Read Archive (accession number PRJNA780813).
Statistical Analyses
Statistical analyses and graphing were performed using GraphPad Prism software (version 6.0). The area under the curve (AUC) for each OGTT was calculated through trapezoidal approximation. All data are presented as mean ± standard error of mean (SEM). Differences among groups for statistical significance were determined using one-way analysis of variance (ANOVA) followed by Tukey’s multiple-comparison test or Kruskal–Wallis test followed by Dunn’s multiple-comparison test. Correlations between bacterial or fungal abundance and metabolic parameters were assessed by Spearman’s correlation analysis. A p-value < 0.05 was considered statistically significant.
Results
Effects of Exogenous H2S on Serum H2S Level, Obesity Parameters and Glucose Homeostasis
Previous studies have reported the increase of body weight, glucose intolerance, and the levels of serum glucose, serum insulin, serum triglyceride, and free fatty acid in the db/db mice, recapitulating hallmark features of type 2 diabetes (Ma et al., 2017; Sun et al., 2018; Sun et al., 2019). By treating with NaHS for 10 or 12 weeks, db/db mice displayed improvements in glucose tolerance and serum insulin levels, while treatment for 12 weeks also decreased the plasma free fatty acid levels in db/db mice (Sun et al., 2018; Sun et al., 2019).
Here, we measured the body weight, serum H2S, triglyceride, and glucose levels to explore whether a longer time of NaHS treatment could prevent the obesity in db/db mice (Figure 1). We found that the db/db mice had a significant decrease in serum H2S level compared with wild-type mice. By treatment with NaHS for 16 weeks, the mice already showed obvious improvement in serum H2S level compared to db/db mice (Figure 1A). NaHS treatment significantly improved the obesity condition by slowing the body weight gain (Figure 1B and Supplementary Figure 1), decreasing the level of serum triglyceride in db/db mice (Figure 1C).
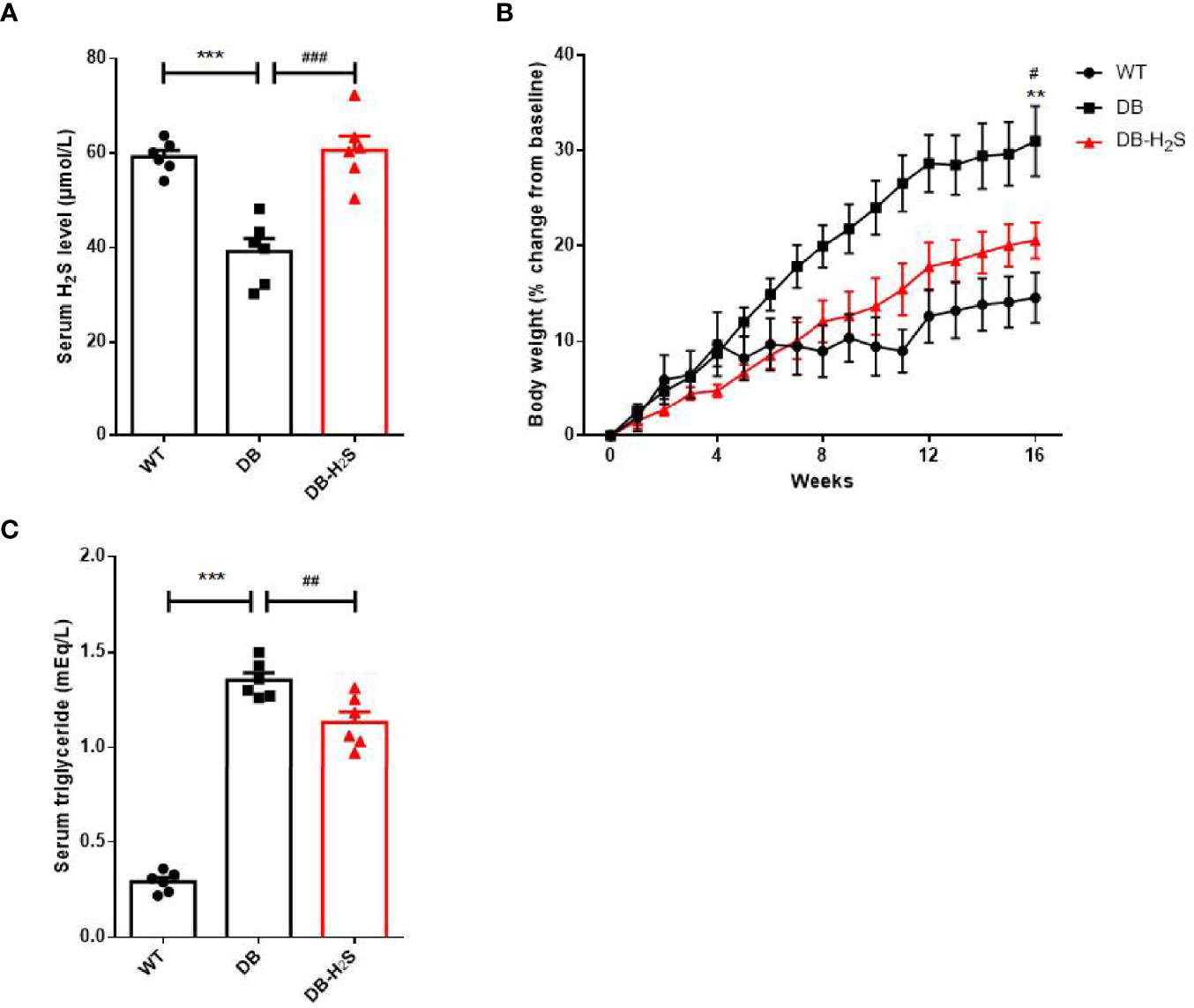
Figure 1 Effects of exogenous H2S on serum H2S level and obesity parameters. (A) Serum H2S level at week 16. (B) Percent change in body weight from baseline over 16 weeks. (C) Serum triglyceride level at week 16. WT, wild-type mouse group (n = 6); DB, db/db mouse group (n = 6); and DB-H2S, db/db mouse treated with NaHS group (n = 6). Data are shown as mean ± SEM. Differences were analyzed by one-way ANOVA with Tukey’s multiple-comparison test and denoted as follows: **p < 0.01, ***p < 0.001, WT vs. DB; #p < 0.05, ##p < 0.01, ###p < 0.001, DB-H2S vs. DB.
Furthermore, administration of NaHS for 16 weeks induced a modest but significant decrease in the blood glucose level of db/db mice (Figure 2A). Similarly, a significantly lower level of serum insulin (Figure 2B) occurred in the treated db/db mice at the end of study. The results of OGTT at week 16 are presented in Figures 2C, D. The blood glucose baselines for OGTT started at relatively high levels in DB and DB-H2S groups, and glucose tolerance in the DB-H2S group was not obviously improved compared to the DB group after glucose administration. Likewise, the DB-H2S group did not have a significantly lower AUC value for OGTT compared to the DB group. These results suggested that NaHS administration was able to alleviate hyperglycemia but difficult to ameliorate impaired glucose tolerance.
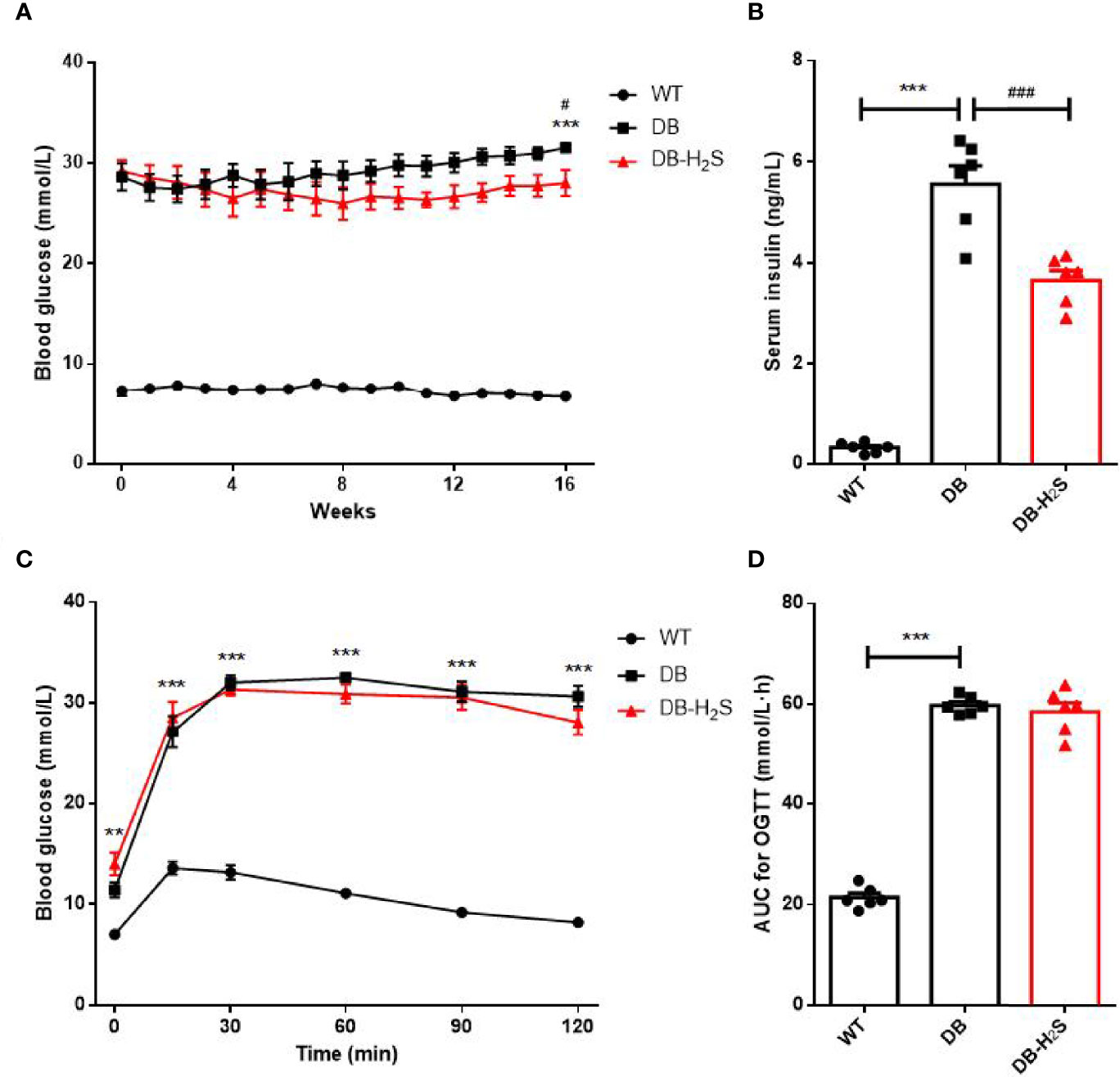
Figure 2 Effects of exogenous H2S on glucose homeostasis. (A) Random blood glucose. (B) Serum insulin level at week 16. (C) Oral glucose tolerance test (OGTT) at week 16. (D) Area under the curve (AUC) for the OGTT. WT, wild-type mouse group (n = 6); DB, db/db mouse group (n = 6); DB-H2S, db/db mouse treated with NaHS group (n = 6). Data are shown as mean ± SEM. Differences were analyzed by one-way ANOVA with Tukey’s multiple-comparison test and denoted as follows: **p < 0.01, ***p < 0.001, WT vs. DB; #p < 0.05, ###p <0.001, DB-H2S vs. DB.
Effect of Exogenous H2S on the Gut Bacterial Microbiota Community Structure
It has been reported that compared to wild-type mice, db/db mice show a significantly different gut microbiota composition, especially the increased level of the phylum Firmicutes and decreased level of the phylum Bacteroidetes (Zheng et al., 2018). To further determine the relationship between exogenous H2S, obesity condition, glucose metabolism, and the gut microbiota, we analyzed the gut microbiota of db/db mice after treatment with NaHS for 16 weeks.
Firstly, the gut bacterial microbiota was analyzed by sequencing the V3–V4 regions of the bacterial 16S rRNA gene. After removing the low-quality sequences, an average 30,772 (20,126–44,585) clean reads were generated from each sample. The high-quality sequences were then delineated into 3,779 OTUs (243–1,605 OTUs per sample) on the basis of 97% similarity. The sample tags and OTUs are shown in Supplementary Table 1. The observed species and Shannon diversity rarefaction curves reached the saturation phase. This indicated that the sequence depth obtained was adequate for all samples (Supplementary Figure 2).
We analyzed the alpha-diversity, which consisted of community richness and diversity (richness and evenness), among the three groups (Figures 3A, B). The bacterial richness (represented by the Chao1 index) and diversity (represented by the Shannon index) in the WT group were significantly higher than those in DB and DB-H2S groups (Figures 3A, B). However, exogenous H2S failed to alter the alpha-diversity indicating that this treatment was not beneficial for the recovery of the richness and diversity of the microbial community in db/db mice.
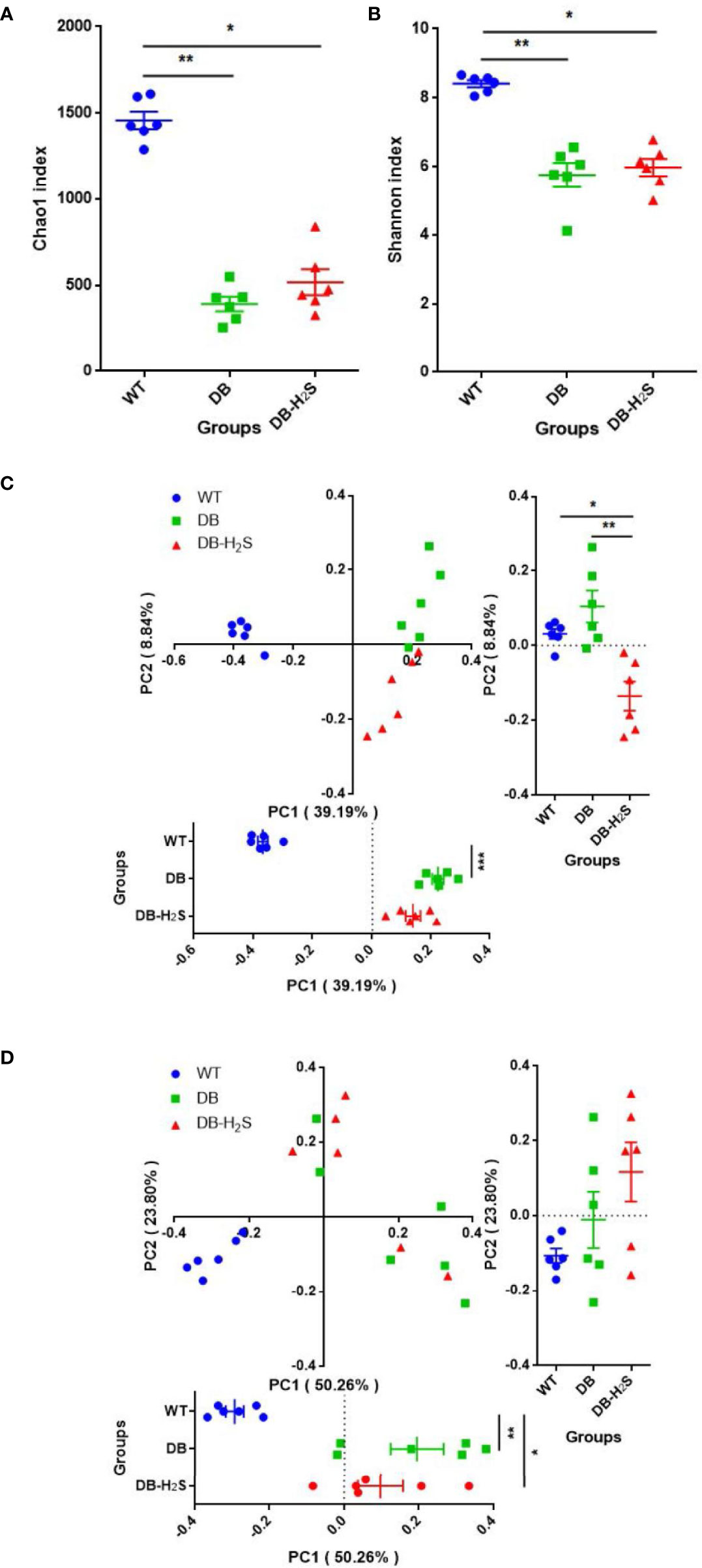
Figure 3 Effect of exogenous H2S on the gut microbiota structure. (A) Chao1 index. (B) Shannon index. (C) Unweighted UniFrac distance-based principal coordinate analysis (PCoA). (D) Weighted UniFrac distance-based PCoA. WT, wild-type mouse group (n = 6); DB, db/db mouse group (n = 6); DB-H2S, db/db mouse treated with NaHS group (n = 6). Data are shown as mean ± SEM. Differences were analyzed by the Kruskal–Wallis test with Dunn’s multiple-comparison test and denoted as follows: *p < 0.05, **p < 0.01, ***p < 0.001.
Beta-diversity analysis was performed based on the unweighted and weighted UniFrac distance-based PCoA (Figures 3C, D). Unweighted UniFrac metrics clearly showed different microbial structures in the three groups (ANOSIM, R = 0.7486, p = 0.001) (Figure 3C). Compared to the DB group, the DB-H2S group had a more similar gut bacterial microbiota structure with that in the WT group at the first principal coordinate (PC1) (Figure 3C). The PCoA, based on weighted UniFrac metrics, shows distinct clustering (ANOSIM, R = 0.5819, p = 0.001) of groups by diabetes rather than treatment (Figure 3D). Notably, the bacterial community structure among the mice in the WT group was very similar, while that in the mice of the DB group as well as DB-H2S had apparent heterogeneity (Figures 3C, D). These results indicated that the exogenous H2S intervention may have benefits on gut bacterial microbiota structure shift to that in the mice of the WT group.
Effect of Exogenous H2S on the Gut Bacterial Microbiota Composition
To further understand the microbial composition among the mice of the three groups, taxonomy-based analysis at the phylum and genus levels was performed (Figure 4 and Supplementary Figure 3). We found that more than 99% of the sequences were within the top three phyla, Firmicutes, Bacteroidetes, and Proteobacteria (Figure 4). Compared with the WT group, the decreased level of Firmicutes (57.43% versus 19.07%) and increased level of Bacteroidetes (28.58% versus 69.45%) were observed in the DB group. NaHS treatment increased the relative abundance of Firmicutes (29.64% versus 19.07%) and suppressed Bacteroidetes (58.15% versus 69.45%) in db/db mice (Figure 4).
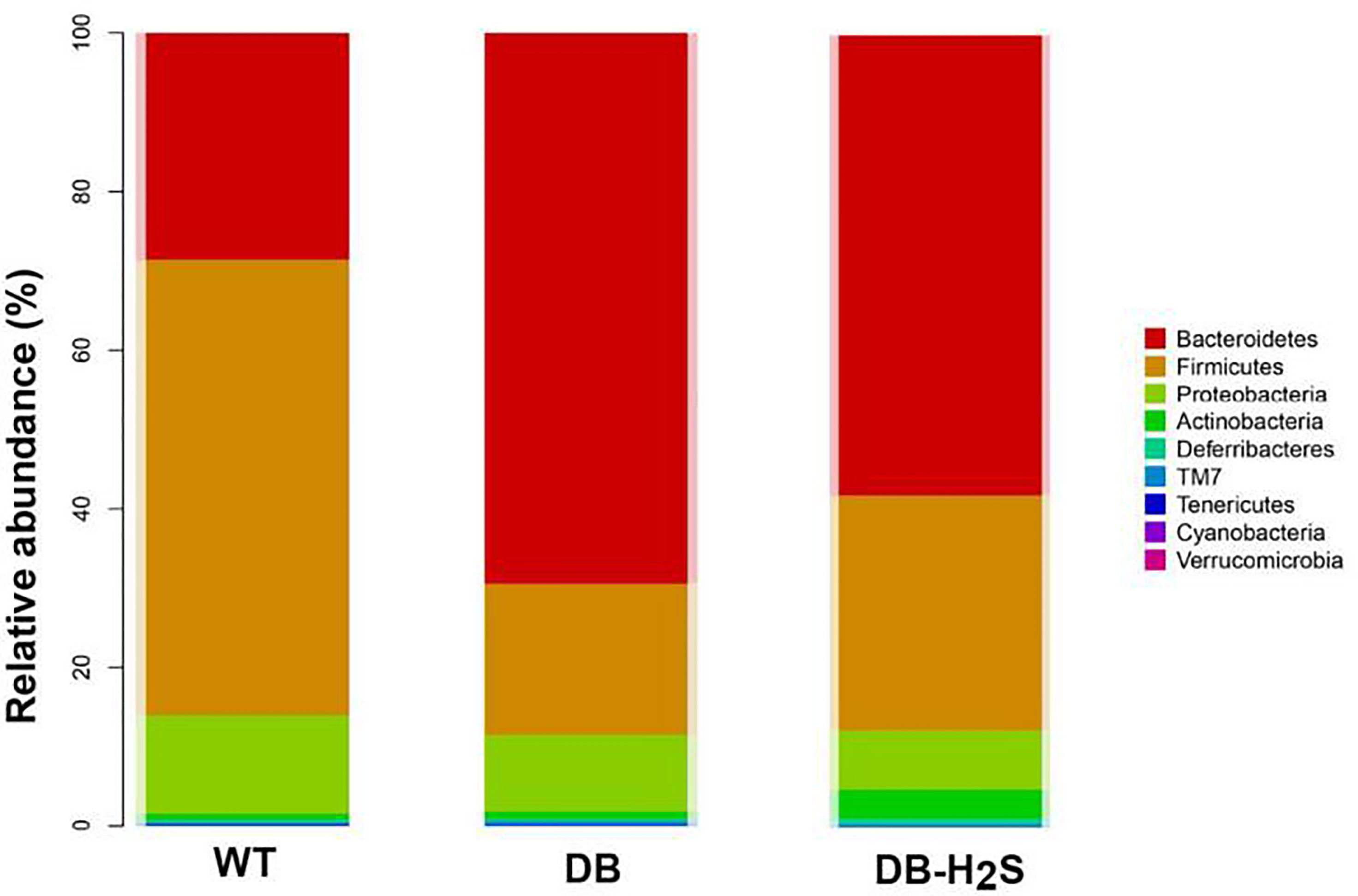
Figure 4 Effect of exogenous H2S on the gut microbiota composition. Changes in bacterial taxonomic composition of the gut microbiota at the phylum level. WT, wild-type mouse group (n = 6); DB, db/db mouse group (n = 6); DB-H2S, db/db mouse treated with NaHS group (n = 6).
At the genus level, the 20 most abundant genera displayed all changes among the different groups (Supplementary Table 2). As shown in Table 1 and Supplementary Figure 3, the bacterial composition at the genus level tended to be dispersed. The relative abundance of genera like Unclassified_S24-7, Bacteroides, Lactobacillus, Unclassified_Enterobacteriaceae, [Prevotella], and Prevotella in the WT group decreased compared with the DB group. Meanwhile, Unclassified_Ruminococcaceae, Helicobacter, Oscillospira, Desulfovibrio, [Ruminococcus], Ruminococcus, and Sutterella increased compared with the DB group. By treatment with exogenous H2S, the db/db mice already showed an improvement in the above high-abundance bacterial genera, such as lessened Unclassified_S24-7 and enhancive Unclassified_Ruminococcaceae.
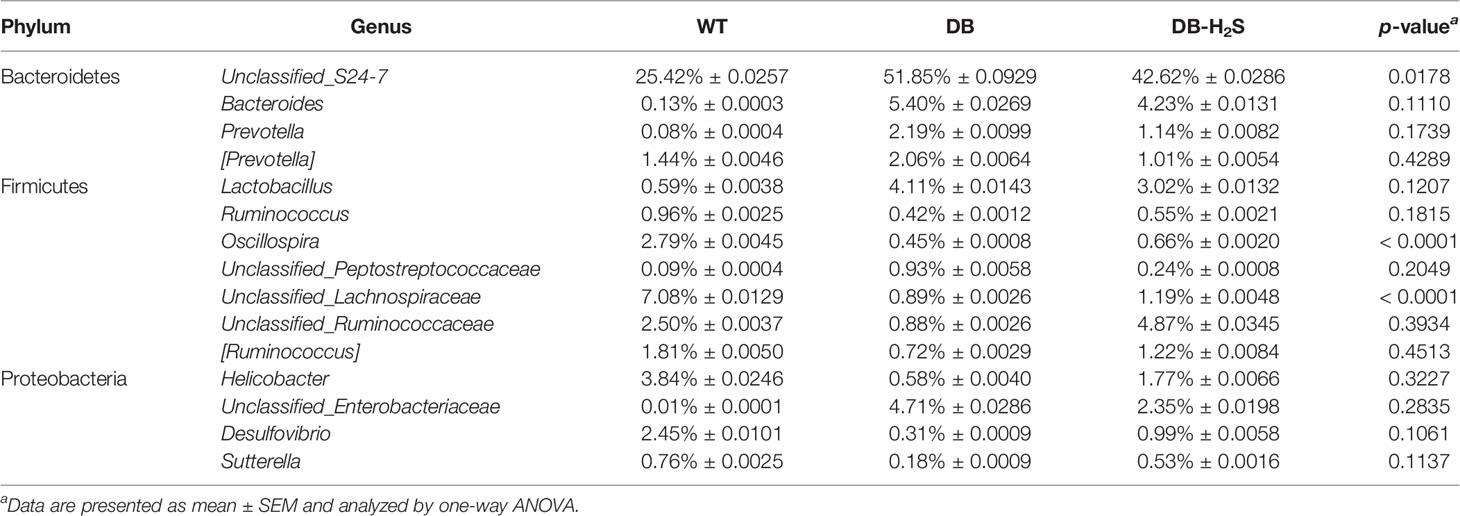
Table 1 The relative abundance of bacterial genera that showed an improvement by treatment with exogenous NaHS.
Effect of Exogenous H2S on the Gut Mycobiome
Next, the gut mycobiome was analyzed by sequencing the ITS region of the fungal gene to further explore whether exogenous H2S may alter the gut mycobiome. In fact, the mycobiome, referring principally to the fungal component of microbiota, comprises approximately 0.03%–2% of total gut microorganisms (Mar Rodriguez et al., 2015). Therefore, analyzing mycobiome may provide additional information of gut microbiota of db/db mice and exogenous H2S treatment effects.
Sequencing effort yielded 778,583 sequence reads (range 27,870−62,386), which were binned into 1,415 OTUs. The fungal observed species and Shannon diversity rarefaction curves also reached the saturation phase (Supplementary Figure 4). There were no significant differences in the richness and diversity of fungal species in the mice of all groups (Figures 5A, B). Fungal structures by PCoA were markedly different among the three groups (unweighted, ANOSIM, R = 0.7885, p = 0.001; weighted, ANOSIM, R = 0.4683, p = 0.001), while the DB-H2S group revealed a more dispersed cluster (Figures 5C, D).
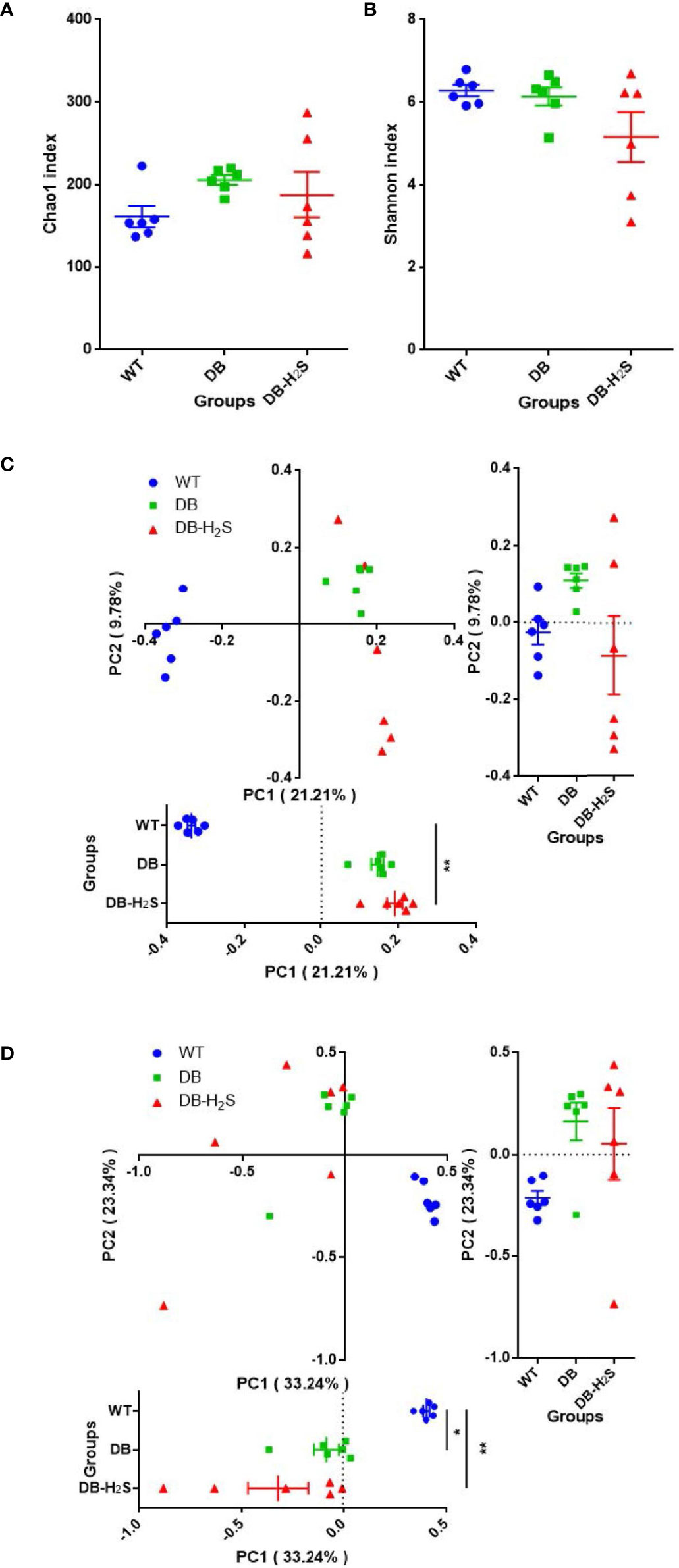
Figure 5 Effect of exogenous H2S on the gut mycobiome structure. (A) Chao1 index. (B) Shannon index. (C) Unweighted UniFrac distance-based principal coordinate analysis (PCoA). (D) Weighted UniFrac distance-based PCoA. WT, wild-type mouse group (n = 6); DB, db/db mouse group (n = 6); DB-H2S, db/db mouse treated with NaHS group (n = 6). Data are shown as mean ± SEM. Differences were analyzed by the Kruskal–Wallis test with Dunn’s multiple-comparison test and denoted as follows: *p < 0.05, **p < 0.01.
At the phylum level, 80%–90% of the gut fungal community (in the cecum content) was dominated by Ascomycota and Basidiomycota (Figure 6). Bubble charts exhibited that in the WT group, Candida, Aspergillus, Trichosporon, and Mortierella reduced in the genus level compared with the DB group. Compared to the DB group, the relative abundance of genera like Candida, Aspergillus, Trichosporon, and Mortierella also has a decline in the DB-H2S group (Table 2 and Supplementary Figure 5). Furthermore, we found that the Simplicillium and Parmelina genera were enriched in the DB-H2S group (Supplementary Figure 5).
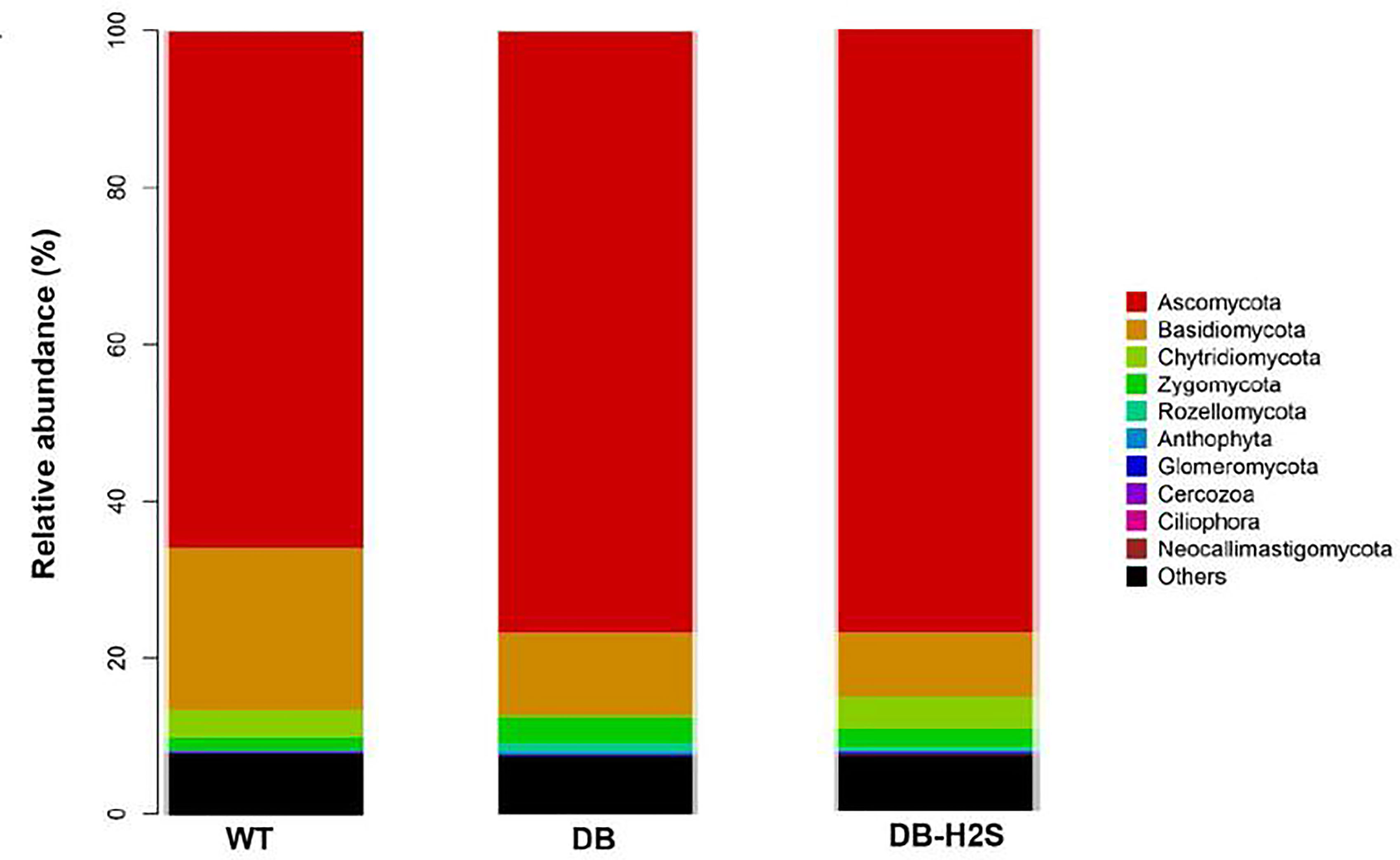
Figure 6 Effect of exogenous H2S on the gut mycobiome composition. Changes in the fungal taxonomic composition of the gut microbiota at the phylum level. WT, wild-type mouse group (n = 6); DB, db/db mouse group (n = 6); DB-H2S, db/db mouse treated with NaHS group (n = 6).
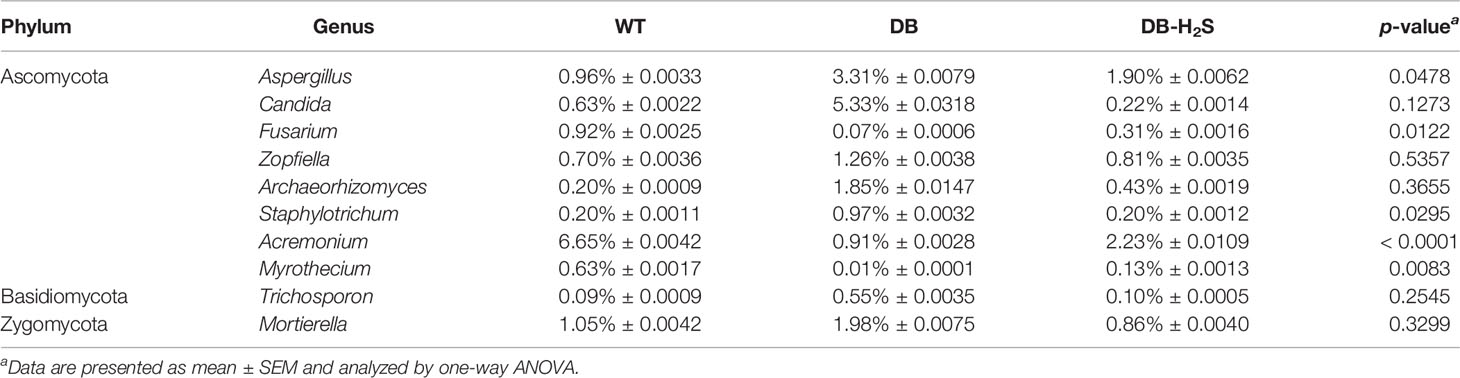
Table 2 The relative abundance of fungal genera that showed an improvement by treatment with exogenous NaHS.
Correlations Between Metabolic Parameters and Exogenous H2S-Induced Alterations of the Gut Microbiota
Spearman correlation analysis was performed to determine the correlations between the metabolic parameters and the microbial abundance at the genus level. The analysis revealed significant negative correlations between the serum H2S level and the abundances of Unclassified_Enterobacteriaceae (r = −0.4870, p = 0.0404) and Prevotella (r = −0.5108, p = 0.0303), and positive correlations between the serum H2S level and the abundances of Unclassified_Ruminococcaceae (r = 0.5645, p = 0.0147) and Sutterella (r = 0.5624, p = 0.0151) (Figures 7A–D). Meanwhile, we found a significant negative correlation between the blood glucose level and the abundance of Desulfovibrio (r = −0.6846, p = 0.0017) (Figure 7E). In addition, a significant negative correlation of the serum H2S level with the abundance of fungal genus Candida was discovered (r = −0.4974, p = 0.0357) (Figure 7F).
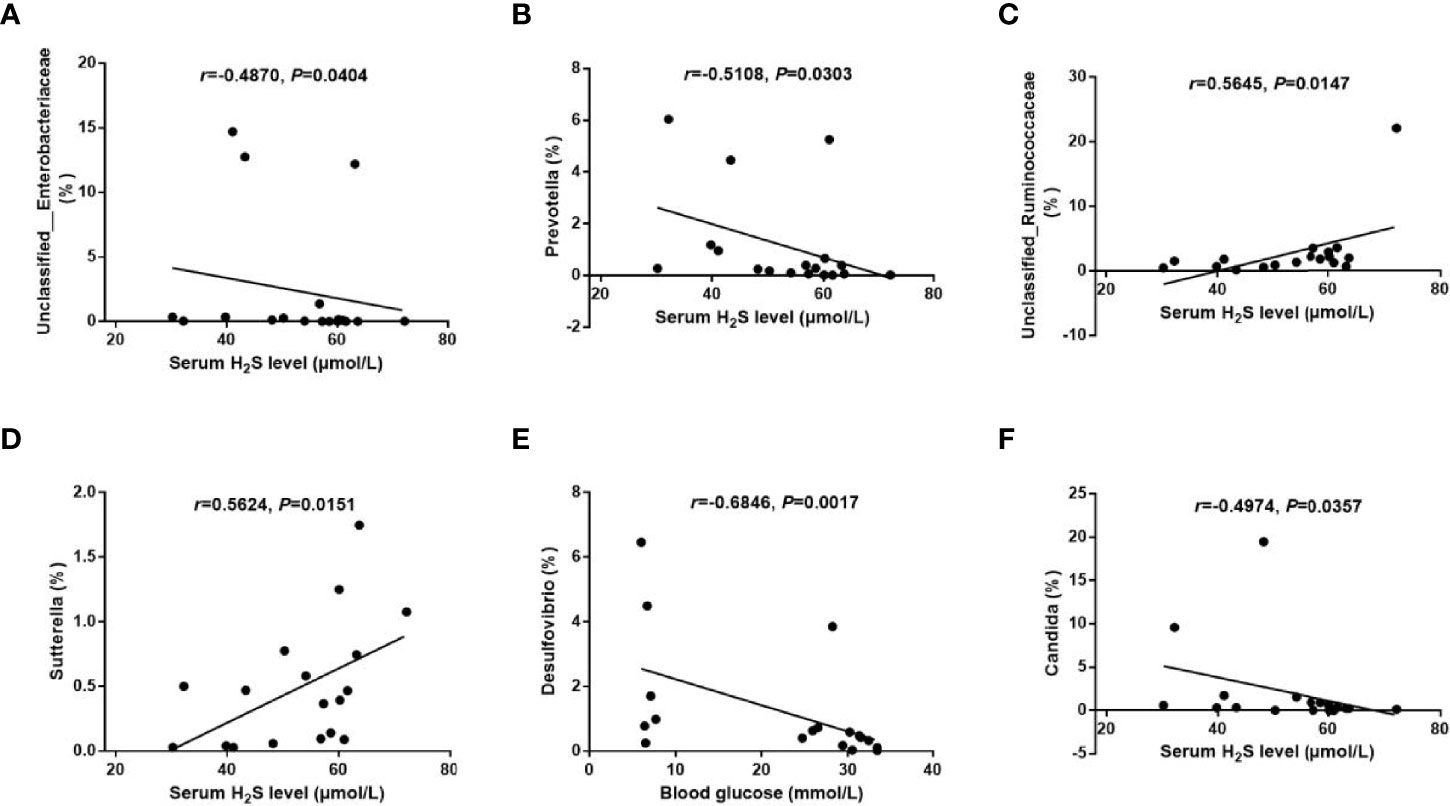
Figure 7 Correlations between the metabolic parameters and the gut microbiota abundance. (A–D): Correlations of the serum H2S level with the abundance of bacterial genera Unclassified_Enterobacteriaceae (A), Prevotella (B), Unclassified_Ruminococcaceae (C), and Sutterella (D). (E) Correlation of the blood glucose level with the abundance of bacterial genus Desulfovibrio. (F) Correlation of the serum H2S level with the abundance of fungal genus Candida. The data were analyzed by Spearman correlation analysis.
Discussion
This study demonstrated that NaHS administration in db/db mice modifies host metabolism and is associated with changes in the gut microbial composition. NaHS-treated mice exhibited a reduced body weight gain ratio, serum triglyceride, blood glucose, and serum insulin, thereby suggesting that the NaHS treatment manner in this study may also act as a beneficial function in the context of obesity and type 2 diabetes. To our knowledge, this is a new high-throughput study analyzing the effects of exogenous H2S on the gut microbiota and the first study showing the pattern of gut fungal mycobiome in db/db mice.
Except that H2S formation in the pancreas and liver was increased in diabetic rats (Yusuf et al., 2005), decreased H2S level in blood in patients or various animal models of diabetes has been mostly reported (Brancaleone et al., 2008; Jain et al., 2010; Peake et al., 2013; Gheibi et al., 2019). In this study, NaHS administration increased serum H2S in db/db mice and reached the serum H2S level in wild-type mice. Recently, Gheibi et al. have explained the reasons for the lower blood H2S levels: hyperglycemia results in an increase in H2S consumption and the activities of H2S-generating enzymes are lower in blood of diabetic patients (Gheibi et al., 2019). These are consistent with our results reported in this study. The activity and expression of H2S-synthesizing enzymes were increased in the pancreas and liver which also result in a higher generation amount of H2S (Yusuf et al., 2005). In terms of glucose homeostasis, exogenous H2S significantly decreased blood glucose but did not improve glucose tolerance in our present study. A thorough and longitudinal treatment protocol should be designed to demonstrate the controversy about the role of H2S in diabetes and find effective therapeutic strategies by regulating the H2S level.
Here, we found that gut-dominating bacterial phyla identified in this study were in line with the findings of other studies (Song et al., 2017; Zheng et al., 2018). The changes in the relative abundance of the two dominant bacterial divisions, Firmicutes and Bacteroidetes, have been widely reported to associate with obesity (Ley et al., 2005; Turnbaugh et al., 2006) or type 2 diabetes (Everard et al., 2011; Geurts et al., 2011). In this regard, the increased ratio of Firmicutes to Bacteroidetes was generally considered as a marker of gut dysbiosis in obesity and type 2 diabetes. However, these in some literature reports were not uniformly observed (Duncan et al., 2008; Larsen et al., 2010). Also, dietary capsaicin has been reported to improve glucose homeostasis with an increase in the phylum Firmicutes and a corresponding decrease in the Bacteroidetes in obese diabetic ob/ob mice (Song et al., 2017) which is similar to exogenous H2S reported here. Therefore, the ratio of Firmicutes to Bacteroidetes might only be an indicator, but a detailed view at the genus level is even more important in regard to distinction of their functions.
In this study, we observed that Unclassified_Enterobacteriaceae and Prevotella decreased after NaHS administration and negatively correlated with the serum H2S level. The population of Enterobacteriaceae, a family containing several opportunistic pathogens, has been reported to induce obesity and insulin resistance (Fei and Zhao, 2013). The species of Prevotella was also demonstrated to induce insulin resistance (Pedersen et al., 2016). Moreover, the Lachnospiraceae (including [Ruminococcus] genus here) and Ruminococcaceae families (including Unclassified_Ruminococcaceae, Oscillospira, and Ruminococcus genera here) were two main butyrate-producing taxonomic groups and showed to be associated with healthier phenotypes (Garcia-Mazcorro et al., 2016). Moreover, our results demonstrated that these bacteria were increased in NaHS-treated db/db mice. Interestingly, Lactobacillus with probiotic characteristics was found to have higher abundance in db/db mice in our study. Increased abundance of Lactobacillus was also observed in long-standing diabetic subjects (Bhute et al., 2017). This may be related to different species of the genus Lactobacillus. Some earlier studies have suggested that germ-free mice have between 50% and 80% less H2S in their tissues and circulation (Shen et al., 2013). The gut microbiota break down protein and complex carbohydrates into short-chain fatty acids and gases (e.g., hydrogen) that are utilized by sulfate-reducing bacteria to produce H2S. In a study of healthy individuals in the United States, approximately 50% of those had their gut colonized by sulfate-reducing bacteria, with a member of the genus of Desulfovibrio being the primary H2S producer (Rey et al., 2013). In this study, we observed that Desulfovibrio increased after NaHS administration, although the difference was not statistically significant. Previous studies also have shown that there was no significant difference in abundance of Desulfovibrio between the wild-type mice and treated mice (Hsu et al., 2021). Since Desulfovibrio was proposed as an inflammation activator, the effects of exogenous and endogenous H2S on gut inflammation should be investigated extensively. Currently, the role of H2S in intestinal inflammation is complex and sometimes contradictory. The therapeutic delivery of exogenous H2S into the gut restored the microbiota biofilm and mucus production and reduced gut inflammation (Motta et al., 2015). Thus, we believe that the beneficial effects of exogenous H2S on type 2 diabetes may be induced by metabolites associated with the specific bacterial changes at the genus level. The association needs to be further validated by metabolomics.
There are a few studies about the mycobiome of the type 2 diabetes population in comparison to healthy controls. For instance, a pilot study has suggested that candida appears to be more prevalent in the feces of patients with type 2 diabetes (Gosiewski et al., 2014). A research about Indian type 2 diabetic subjects has shown that opportunistic fungal pathogens such as Candida and Aspergillus were found to be enriched in newly diagnosed diabetic subjects (Bhute et al., 2017). To our knowledge, no study exists about the gut mycobiome of diabetic animal models. Next-generation sequencing will be valuable for characterizing the gut mycobiome associated with metabolism disorder. Here, that the fungal structure in db/db mice has changed substantially reflects the close association of gut mycobiome with diabetes. Consequently, it is speculated that gut dysbiosis in diabetes creates the environment for fungal overgrowth. We also observed that Candida and Aspergillus decreased after NaHS administration and Candida negatively correlated with the serum H2S level. Although for decades fungi are considered harmful to their host, the modifications of fungal communities in the gut should be paid more attention.
In conclusion, we found that exogenous H2S led to significant improvement of diabetic metabolic disorders in db/db mice. The beneficial effects of exogenous H2S on diabetic metabolic disorders are likely related to the alterations of both bacterial and fungal microbiota. Some remarkable genera were proved to possess significant correlations with the serum H2S and blood glucose levels. This relationship can still be found in the gut mycobiome. These results offer a novel insight that alterations in the gut microbiota composition may be the potential mechanism underlying the effects of exogenous H2S on diabetic metabolic disorders. The main strength of this research is that a role of exogenous H2S intervention in type 2 diabetes was associated with gut microbiota (bacterial and fungal aspects). This study has some limitations, in that our results were based on a relatively small sample size and the cross-sectional design. Lastly, we have not demonstrated a causal relationship between gut microbiota and exogenous H2S-mediated metabolic improvement in db/db mice.
Data Availability Statement
The original contributions presented in the study are publicly available. These data can be found as follows: NCBI, PRJNA780813.
Ethics Statement
The animal study was reviewed and approved by the Animal Care Committees of Harbin Medical University, China.
Author Contributions
HL and W-HZ conducted the study and designed the experiment. LL and WZ performed the experiments. JL and Z-WG performed the data analysis. JL and Z-WG wrote the draft of the manuscript and revised the manuscript. All authors contributed to the article and approved the submitted version.
Funding
This work was supported by the Graduate Innovation Foundation of Harbin Medical University (YJSCX2016-41HYD) and National Science Foundation of China (81970317).
Conflict of Interest
The authors declare that the research was conducted in the absence of any commercial or financial relationships that could be construed as a potential conflict of interest.
Publisher’s Note
All claims expressed in this article are solely those of the authors and do not necessarily represent those of their affiliated organizations, or those of the publisher, the editors and the reviewers. Any product that may be evaluated in this article, or claim that may be made by its manufacturer, is not guaranteed or endorsed by the publisher.
Acknowledgments
We are grateful to the Harbin Medical University for the Graduate Innovation Support Program.
Supplementary Material
The Supplementary Material for this article can be found online at: https://www.frontiersin.org/articles/10.3389/fcimb.2022.801331/full#supplementary-material
References
Arora, A., Behl, T., Sehgal, A., Singh, S., Sharma, N., Bhatia, S., et al. (2021). Unravelling the Involvement of Gut Microbiota in Type 2 Diabetes Mellitus. Life Sci. 273, 119311. doi: 10.1016/j.lfs.2021.119311
Bhute, S. S., Suryavanshi, M. V., Joshi, S. M., Yajnik, C. S., Shouche, Y. S., Ghaskadbi, S. S. (2017). Gut Microbial Diversity Assessment of Indian Type-2-Diabetics Reveals Alterations in Eubacteria, Archaea, and Eukaryotes. Front. Microbiol. 8. doi: 10.3389/fmicb.2017.00214
Blackler, R. W., Motta, J. P., Manko, A., Workentine, M., Bercik, P., Surette, M. G., et al. (2015). Hydrogen Sulphide Protects Against NSAID-Enteropathy Through Modulation of Bile and the Microbiota. Br. J. Pharmacol. 172 (4), 992–1004. doi: 10.1111/bph.12961
Bokulich, N. A., Subramanian, S., Faith, J. J., Gevers, D., Gordon, J. I., Knight, R., et al. (2013). Quality-Filtering Vastly Improves Diversity Estimates From Illumina Amplicon Sequencing. Nat. Methods 10 (1), 57–59. doi: 10.1038/nmeth.2276
Brancaleone, V., Roviezzo, F., Vellecco, V., De Gruttola, L., Bucci, M., Cirino, G. (2008). Biosynthesis of H2S Is Impaired in non-Obese Diabetic (NOD) Mice. Br. J. Pharmacol. 155 (5), 673–680. doi: 10.1038/bjp.2008.296
Buret, A. G., Allain, T., Motta, J. P., Wallace, J. L. (2022). Effects of Hydrogen Sulfide on the Microbiome: From Toxicity to Therapy. Antioxid Redox Signal 36 (4-6), 211–219. doi: 10.1089/ars.2021.0004
Burrichter, A., Denger, K., Franchini, P., Huhn, T., Müller, N., Spiteller, D., et al. (2018). Anaerobic Degradation of the Plant Sugar Sulfoquinovose Concomitant With H(2)S Production: Escherichia Coli K-12 and Desulfovibrio Sp. Strain DF1 as Co-Culture Model. Front. Microbiol. 9. doi: 10.3389/fmicb.2018.02792
Chen, H., Jiang, W. (2014). Application of High-Throughput Sequencing in Understanding Human Oral Microbiome Related With Health and Disease. Front. Microbiol. 5. doi: 10.3389/fmicb.2014.00508
Chen, T., Liu, A. B., Sun, S., Ajami, N. J., Ross, M. C., Wang, H., et al. (2019). Green Tea Polyphenols Modify the Gut Microbiome in Db/Db Mice as Co-Abundance Groups Correlating With the Blood Glucose Lowering Effect. Mol. Nutr. Food Res. 63 (8), e1801064. doi: 10.1002/mnfr.201801064
DeSantis, T. Z., Hugenholtz, P., Larsen, N., Rojas, M., Brodie, E. L., Keller, K., et al. (2006). Greengenes, a Chimera-Checked 16S rRNA Gene Database and Workbench Compatible With ARB. Appl. Environ. Microbiol. 72 (7), 5069–5072. doi: 10.1128/aem.03006-05
Duncan, S. H., Lobley, G. E., Holtrop, G., Ince, J., Johnstone, A. M., Louis, P., et al. (2008). Human Colonic Microbiota Associated With Diet, Obesity and Weight Loss. Int. J. Obes. (Lond) 32 (11), 1720–1724. doi: 10.1038/ijo.2008.155
Edgar, R. C. (2010). Search and Clustering Orders of Magnitude Faster Than BLAST. Bioinformatics 26 (19), 2460–2461. doi: 10.1093/bioinformatics/btq461
Everard, A., Lazarevic, V., Derrien, M., Girard, M., Muccioli, G. G., Neyrinck, A. M., et al. (2011). Responses of Gut Microbiota and Glucose and Lipid Metabolism to Prebiotics in Genetic Obese and Diet-Induced Leptin-Resistant Mice. Diabetes 60 (11), 2775–2786. doi: 10.2337/db11-0227
Everard, A., Matamoros, S., Geurts, L., Delzenne, N. M., Cani, P. D. (2014). Saccharomyces Boulardii Administration Changes Gut Microbiota and Reduces Hepatic Steatosis, Low-Grade Inflammation, and Fat Mass in Obese and Type 2 Diabetic Db/Db Mice. mBio 5 (3), e01011–e01014. doi: 10.1128/mBio.01011-14
Fang, Y., Zhang, C., Shi, H., Wei, W., Shang, J., Zheng, R., et al. (2021). Characteristics of the Gut Microbiota and Metabolism in Patients With Latent Autoimmune Diabetes in Adults: A Case-Control Study. Diabetes Care. 44 (12), 2738–2746. doi: 10.2337/dc20-2975
Fei, N., Zhao, L. (2013). An Opportunistic Pathogen Isolated From the Gut of an Obese Human Causes Obesity in Germfree Mice. Isme J. 7 (4), 880–884. doi: 10.1038/ismej.2012.153
Frommeyer, B., Fiedler, A. W., Oehler, S. R., Hanson, B. T., Loy, A., Franchini, P., et al. (2020). Environmental and Intestinal Phylum Firmicutes Bacteria Metabolize the Plant Sugar Sulfoquinovose via a 6-Deoxy-6-Sulfofructose Transaldolase Pathway. iScience 23 (9), 101510. doi: 10.1016/j.isci.2020.101510
Garcia-Mazcorro, J. F., Ivanov, I., Mills, D. A., Noratto, G. (2016). Influence of Whole-Wheat Consumption on Fecal Microbial Community Structure of Obese Diabetic Mice. PeerJ 4, e1702. doi: 10.7717/peerj.1702
Geurts, L., Lazarevic, V., Derrien, M., Everard, A., Van Roye, M., Knauf, C., et al. (2011). Altered Gut Microbiota and Endocannabinoid System Tone in Obese and Diabetic Leptin-Resistant Mice: Impact on Apelin Regulation in Adipose Tissue. Front. Microbiol. 2. doi: 10.3389/fmicb.2011.00149
Gheibi, S., Jeddi, S., Kashfi, K., Ghasemi, A. (2019). Effects of Hydrogen Sulfide on Carbohydrate Metabolism in Obese Type 2 Diabetic Rats. Molecules 24 (1), 190. doi: 10.3390/molecules24010190
Gill, S. R., Pop, M., Deboy, R. T., Eckburg, P. B., Turnbaugh, P. J., Samuel, B. S., et al. (2006). Metagenomic Analysis of the Human Distal Gut Microbiome. Science 312 (5778), 1355–1359. doi: 10.1126/science.1124234
Gosiewski, T., Salamon, D., Szopa, M., Sroka, A., Malecki, M. T., Bulanda, M. (2014). Quantitative Evaluation of Fungi of the Genus Candida in the Feces of Adult Patients With Type 1 and 2 Diabetes - A Pilot Study. Gut Pathog. 6 (1), 43. doi: 10.1186/s13099-014-0043-z
Hosoki, R., Matsuki, N., Kimura, H. (1997). The Possible Role of Hydrogen Sulfide as an Endogenous Smooth Muscle Relaxant in Synergy With Nitric Oxide. Biochem. Biophys. Res. Commun. 237 (3), 527–531. doi: 10.1006/bbrc.1997.6878
Hsu, C. N., Hou, C. Y., Chang-Chien, G. P., Lin, S., Tain, Y. L. (2021). Maternal Garlic Oil Supplementation Prevents High-Fat Diet-Induced Hypertension in Adult Rat Offspring: Implications of H2S-Generating Pathway in the Gut and Kidneys. Mol. Nutr. Food Res. 65 (11), e2001116. doi: 10.1002/mnfr.202001116
Jain, S. K., Bull, R., Rains, J. L., Bass, P. F., Levine, S. N., Reddy, S., et al. (2010). Low Levels of Hydrogen Sulfide in the Blood of Diabetes Patients and Streptozotocin-Treated Rats Causes Vascular Inflammation? Antioxid Redox Signal 12 (11), 1333–1337. doi: 10.1089/ars.2009.2956
Kang, K., Zhao, M., Jiang, H., Tan, G., Pan, S., Sun, X. (2009). Role of Hydrogen Sulfide in Hepatic Ischemia-Reperfusion-Induced Injury in Rats. Liver Transpl 15 (10), 1306–1314. doi: 10.1002/lt.21810
Kashiwagi, Y., Aburaya, S., Sugiyama, N., Narukawa, Y., Sakamoto, Y., Takahashi, M., et al. (2021). Porphyromonas Gingivalis Induces Entero-Hepatic Metabolic Derangements With Alteration of Gut Microbiota in a Type 2 Diabetes Mouse Model. Sci. Rep. 11 (1), 18398. doi: 10.1038/s41598-021-97868-2
Lambert, J. E., Myslicki, J. P., Bomhof, M. R., Belke, D. D., Shearer, J., Reimer, R. A. (2015). Exercise Training Modifies Gut Microbiota in Normal and Diabetic Mice. Appl. Physiol. Nutr. Metab. 40 (7), 749–752. doi: 10.1139/apnm-2014-0452
Larsen, N., Vogensen, F. K., van den Berg, F. W., Nielsen, D. S., Andreasen, A. S., Pedersen, B. K., et al. (2010). Gut Microbiota in Human Adults With Type 2 Diabetes Differs From Non-Diabetic Adults. PloS One 5 (2), e9085. doi: 10.1371/journal.pone.0009085
Ley, R. E., Backhed, F., Turnbaugh, P., Lozupone, C. A., Knight, R. D., Gordon, J. I. (2005). Obesity Alters Gut Microbial Ecology. Proc. Natl. Acad. Sci. U.S.A. 102 (31), 11070–11075. doi: 10.1073/pnas.0504978102
Lin, R., He, X., Chen, H., He, Q., Yao, Z., Li, Y., et al. (2018). Oil Tea Improves Glucose and Lipid Levels and Alters Gut Microbiota in Type 2 Diabetic Mice. Nutr. Res. 57, 67–77. doi: 10.1016/j.nutres.2018.05.004
Li, C., Wang, X., Sun, S., Liu, S., Huan, Y., Li, R., et al. (2020). Effects of a Ready-to-Eat Cereal Formula Powder on Glucose Metabolism, Inflammation, and Gut Microbiota in Diabetic Db/Db Mice. Food Sci. Nutr. 8 (8), 4523–4533. doi: 10.1002/fsn3.1761
Li, Y., Xie, K. F., Chang, Y. H., Wang, C., Chen, Y., Wang, M. J., et al. (2021). S-Propargyl-Cysteine Attenuates Diabetic Cardiomyopathy in Db/Db Mice Through Activation of Cardiac Insulin Receptor Signaling. Front. Cardiovasc. Med. 8. doi: 10.3389/fcvm.2021.737191
Magoc, T., Salzberg, S. L. (2011). FLASH: Fast Length Adjustment of Short Reads to Improve Genome Assemblies. Bioinformatics 27 (21), 2957–2963. doi: 10.1093/bioinformatics/btr507
Mar Rodriguez, M., Perez, D., Javier Chaves, F., Esteve, E., Marin-Garcia, P., Xifra, G., et al. (2015). Obesity Changes the Human Gut Mycobiome. Sci. Rep. 5, 14600. doi: 10.1038/srep14600
Ma, S., Zhong, D., Ma, P., Li, G., Hua, W., Sun, Y., et al. (2017). Exogenous Hydrogen Sulfide Ameliorates Diabetes-Associated Cognitive Decline by Regulating the Mitochondria-Mediated Apoptotic Pathway and IL-23/IL-17 Expression in Db/Db Mice. Cell. Physiol. Biochem. 41 (5), 1838–1850. doi: 10.1159/000471932
Motta, J. P., Flannigan, K. L., Agbor, T. A., Beatty, J. K., Blackler, R. W., Workentine, M. L., et al. (2015). Hydrogen Sulfide Protects From Colitis and Restores Intestinal Microbiota Biofilm and Mucus Production. Inflammation Bowel Dis. 21 (5), 1006–1017. doi: 10.1097/mib.0000000000000345
Nguyen, T. L., Vieira-Silva, S., Liston, A., Raes, J. (2015). How Informative Is the Mouse for Human Gut Microbiota Research? Dis. Model Mech. 8 (1), 1–16. doi: 10.1242/dmm.017400
Peake, B. F., Nicholson, C. K., Lambert, J. P., Hood, R. L., Amin, H., Amin, S., et al. (2013). Hydrogen Sulfide Preconditions the Db/Db Diabetic Mouse Heart Against Ischemia-Reperfusion Injury by Activating Nrf2 Signaling in an Erk-Dependent Manner. Am. J. Physiol. Heart Circ. Physiol. 304 (9), H1215–H1224. doi: 10.1152/ajpheart.00796.2012
Pedersen, H. K., Gudmundsdottir, V., Nielsen, H. B., Hyotylainen, T., Nielsen, T., Jensen, B. A., et al. (2016). Human Gut Microbes Impact Host Serum Metabolome and Insulin Sensitivity. Nature 535 (7612), 376–381. doi: 10.1038/nature18646
Rey, F. E., Gonzalez, M. D., Cheng, J., Wu, M., Ahern, P. P., Gordon, J. I. (2013). Metabolic Niche of a Prominent Sulfate-Reducing Human Gut Bacterium. Proc. Natl. Acad. Sci. U.S.A. 110 (33), 13582–13587. doi: 10.1073/pnas.1312524110
Scheithauer, T. P. M., Rampanelli, E., Nieuwdorp, M., Vallance, B. A., Verchere, C. B., van Raalte, D. H., et al. (2020). Gut Microbiota as a Trigger for Metabolic Inflammation in Obesity and Type 2 Diabetes. Front. Immunol. 11. doi: 10.3389/fimmu.2020.571731
Shen, X., Carlstrom, M., Borniquel, S., Jadert, C., Kevil, C. G., Lundberg, J. O. (2013). Microbial Regulation of Host Hydrogen Sulfide Bioavailability and Metabolism. Free Radic. Biol. Med. 60, 195–200. doi: 10.1016/j.freeradbiomed.2013.02.024
Singh, H., Miyamoto, S., Darshi, M., Torralba, M. G., Kwon, K., Sharma, K., et al. (2020). Gut Microbial Changes in Diabetic Db/Db Mice and Recovery of Microbial Diversity Upon Pirfenidone Treatment. Microorganisms 8 (9), 1347. doi: 10.3390/microorganisms8091347
Sokolov, A. S., Nekrasov, P. V., Shaposhnikov, M. V., Moskalev, A. A. (2021). Hydrogen Sulfide in Longevity and Pathologies: Inconsistency Is Malodorous. Ageing Res. Rev. 67, 101262. doi: 10.1016/j.arr.2021.101262
Song, J. X., Ren, H., Gao, Y. F., Lee, C. Y., Li, S. F., Zhang, F., et al. (2017). Dietary Capsaicin Improves Glucose Homeostasis and Alters the Gut Microbiota in Obese Diabetic Ob/Ob Mice. Front. Physiol. 8. doi: 10.3389/fphys.2017.00602
Sun, Y., Teng, Z., Sun, X., Zhang, L., Chen, J., Wang, B., et al. (2019). Exogenous H(2)S Reduces the Acetylation Levels of Mitochondrial Respiratory Enzymes via Regulating the NAD(+)-SIRT3 Pathway in Cardiac Tissues of Db/Db Mice. Am. J. Physiol. Endocrinol. Metab. 317 (2), E284–e297. doi: 10.1152/ajpendo.00326.2018
Sun, Y., Tian, Z., Liu, N., Zhang, L., Gao, Z., Sun, X., et al. (2018). Exogenous H2S Switches Cardiac Energy Substrate Metabolism by Regulating SIRT3 Expression in Db/Db Mice. J. Mol. Med. (Berl) 96 (3-4), 281–299. doi: 10.1007/s00109-017-1616-3
Suzuki, K., Olah, G., Modis, K., Coletta, C., Kulp, G., Gero, D., et al. (2011). Hydrogen Sulfide Replacement Therapy Protects the Vascular Endothelium in Hyperglycemia by Preserving Mitochondrial Function. Proc. Natl. Acad. Sci. U.S.A. 108 (33), 13829–13834. doi: 10.1073/pnas.1105121108
Turnbaugh, P. J., Ley, R. E., Mahowald, M. A., Magrini, V., Mardis, E. R., Gordon, J. I. (2006). An Obesity-Associated Gut Microbiome With Increased Capacity for Energy Harvest. Nature 444 (7122), 1027–1031. doi: 10.1038/nature05414
Walker, A., Lucio, M., Pfitzner, B., Scheerer, M. F., Neschen, S., de Angelis, M. H., et al. (2014). Importance of Sulfur-Containing Metabolites in Discriminating Fecal Extracts Between Normal and Type-2 Diabetic Mice. J. Proteome Res. 13 (10), 4220–4231. doi: 10.1021/pr500046b
Wallace, J. L., Wang, R. (2015). Hydrogen Sulfide-Based Therapeutics: Exploiting a Unique But Ubiquitous Gasotransmitter. Nat. Rev. Drug Discov. 14 (5), 329–345. doi: 10.1038/nrd4433
Walsh, B. J. C., Wang, J., Edmonds, K. A., Palmer, L. D., Zhang, Y., Trinidad, J. C., et al. (2020). The Response of Acinetobacter Baumannii to Hydrogen Sulfide Reveals Two Independent Persulfide-Sensing Systems and a Connection to Biofilm Regulation. mBio 11 (3), e01254-20. doi: 10.1128/mBio.01254-20
Whiteman, M., Gooding, K. M., Whatmore, J. L., Ball, C. I., Mawson, D., Skinner, K., et al. (2010). Adiposity Is a Major Determinant of Plasma Levels of the Novel Vasodilator Hydrogen Sulphide. Diabetologia 53 (8), 1722–1726. doi: 10.1007/s00125-010-1761-5
Wu, J., Tian, Z., Sun, Y., Lu, C., Liu, N., Gao, Z., et al. (2017). Exogenous H2S Facilitating Ubiquitin Aggregates Clearance via Autophagy Attenuates Type 2 Diabetes-Induced Cardiomyopathy. Cell Death Dis. 8 (8), e2992. doi: 10.1038/cddis.2017.380. 5.638.
Wu, D., Zheng, N., Qi, K., Cheng, H., Sun, Z., Gao, B., et al. (2015). Exogenous Hydrogen Sulfide Mitigates the Fatty Liver in Obese Mice Through Improving Lipid Metabolism and Antioxidant Potential. Med. Gas Res. 5 (1), 1. doi: 10.1186/s13618-014-0022-y
Xue, R., Hao, D. D., Sun, J. P., Li, W. W., Zhao, M. M., Li, X. H., et al. (2013). Hydrogen Sulfide Treatment Promotes Glucose Uptake by Increasing Insulin Receptor Sensitivity and Ameliorates Kidney Lesions in Type 2 Diabetes. Antioxid Redox Signal 19 (1), 5–23. doi: 10.1089/ars.2012.5024
Yusuf, M., Kwong Huat, B. T., Hsu, A., Whiteman, M., Bhatia, M., Moore, P. K. (2005). Streptozotocin-Induced Diabetes in the Rat Is Associated With Enhanced Tissue Hydrogen Sulfide Biosynthesis. Biochem. Biophys. Res. Commun. 333 (4), 1146–1152. doi: 10.1016/j.bbrc.2005.06.021
Zhang, L., Jiang, X., Liu, N., Li, M., Kang, J., Chen, L., et al. (2021). Exogenous H(2) S Prevents the Nuclear Translocation of PDC-E1 and Inhibits Vascular Smooth Muscle Cell Proliferation in the Diabetic State. J. Cell Mol. Med. 25 (17), 8201–8214. doi: 10.1111/jcmm.16688
Zheng, J., Yuan, X., Cheng, G., Jiao, S., Feng, C., Zhao, X., et al. (2018). Chitosan Oligosaccharides Improve the Disturbance in Glucose Metabolism and Reverse the Dysbiosis of Gut Microbiota in Diabetic Mice. Carbohydr Polym 190, 77–86. doi: 10.1016/j.carbpol.2018.02.058
Keywords: exogenous H2S, type 2 diabetes, db/db mice, metabolic disorders, gut microbiota
Citation: Liu J, Zhao W, Gao Z-W, Liu N, Zhang W-H and Ling H (2022) Effects of Exogenous Hydrogen Sulfide on Diabetic Metabolic Disorders in db/db Mice Are Associated With Gut Bacterial and Fungal Microbiota. Front. Cell. Infect. Microbiol. 12:801331. doi: 10.3389/fcimb.2022.801331
Received: 25 October 2021; Accepted: 02 March 2022;
Published: 29 March 2022.
Edited by:
Yuguang Du, Chinese Academy of Sciences (CAS), ChinaReviewed by:
Kit Leong Cheong, Shantou University, ChinaDuolong Zhu, Baylor College of Medicine, United States
Copyright © 2022 Liu, Zhao, Gao, Liu, Zhang and Ling. This is an open-access article distributed under the terms of the Creative Commons Attribution License (CC BY). The use, distribution or reproduction in other forums is permitted, provided the original author(s) and the copyright owner(s) are credited and that the original publication in this journal is cited, in accordance with accepted academic practice. No use, distribution or reproduction is permitted which does not comply with these terms.
*Correspondence: Hong Ling, bGluZ2hAZW1zLmhyYm11LmVkdS5jbg==; Wei-Hua Zhang, emhhbmd3aDExNkAxMjYuY29t
†These authors have contributed equally to this work